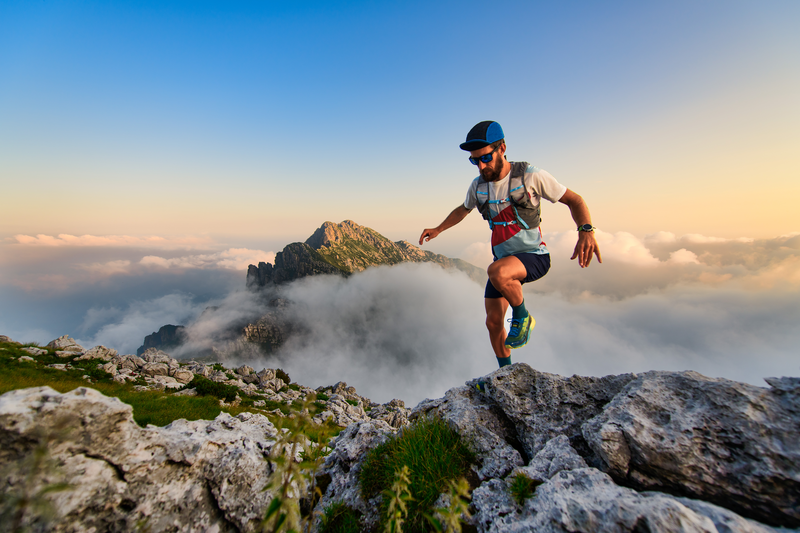
95% of researchers rate our articles as excellent or good
Learn more about the work of our research integrity team to safeguard the quality of each article we publish.
Find out more
HYPOTHESIS AND THEORY article
Front. Oncol. , 06 October 2023
Sec. Gynecological Oncology
Volume 13 - 2023 | https://doi.org/10.3389/fonc.2023.1240309
Estrous cycles are recurring changes in therian mammals induced by estrogen, progesterone, and other hormones culminating in endometrial proliferation, ovulation, and implantation if fertilization occurred. In women, the estrous cycle is the menstrual cycle; but, unlike most mammals, the end of an infertile cycle is marked by endometrial sloughing and the start of another without an anestrous phase. Women stop cycling at menopause, while in most mammals, cycles continue until death. Epidemiologic studies identified menarche, menopause, births, lactation, and oral contraceptive (OC) use as key risk factors for ovarian, breast, and endometrial cancers. A composite variable was created to estimate the number of cycles not interrupted by events that stop ovulation. Captured by the phrase “incessant ovulation”, repetitive cycles were first postulated to affect ovarian cancer risk and later extended to breast and endometrial cancers. These associations could be explained by cumulative effects of repetitive tissue changes within reproductive organs, immune consequences of repetitive ovulation through the glycoprotein mucin 1, and residual effects of past ovulations that enhance ovarian production of testosterone. The latter two pathways could affect the risk for cancers in other organs not considered “reproductive”.
Estrous cycles are recurring events in placenta-forming mammals induced by estrogen, progesterone, and other hormones that culminate in changes to the uterine lining, ovulation, and support for an implanted zygote if fertilization occurred. Nutritional, environmental, and genetic factors determine the onset of sexual maturity, which occurs relatively later in animals with greater longevity and requires a certain body size to be achieved on an allometric scale. Estrous cycles vary in duration, frequency, and seasonality and, in most mammals, repeat until death (1). In women, the estrous cycle is the menstrual cycle; but unlike in most mammals, the end of an infertile cycle is marked by the sloughing of the endometrial lining and the immediate start of another without an anestrous phase (2). Women are polyestrous with an average cycle length of 28 days repeating until menopause. Ages at the first and last periods are important landmarks for the beginning of sexual maturity and the end of reproductive potential. With average ages at menopause and menarche approximately 51 and 12, the reproductive span is approximately 39 years.
The variable, ovulatory (or menstrual) years, is estimated by subtracting the age at menarche from the age at menopause (or current age if the woman is premenopausal) from which time pregnant, nursing, or using oral contraceptives (or other hormones stopping ovulation) are subtracted. Lifetime ovulatory (or menstrual) cycles are estimated by multiplying ovulatory years by cycles per year, approximately 13, for a cycle length of 28 days. More ovulatory cycles and greater cancer risk were first linked to ovarian cancer and later extended to breast and endometrial cancers. Here, we review the strength of the epidemiologic evidence for ovulatory years as a risk factor for these cancers, discuss mechanisms by which they might increase risk, and briefly examine how these mechanisms could link ovulatory cycles to cancers in organs not considered “reproductive”.
Globally, in 2020, it was estimated that breast, endometrial, and ovarian cancers totaled approximately 3 million new cases and a million deaths (3). Worldwide, incidence and mortality rates for breast, endometrial, and ovarian cancers are highly correlated (4); there is a greater-than-expected number of endometrial and ovarian cancers that occur after a diagnosis of breast cancer and a greater-than-expected number of breast cancer(s) after a diagnosis of ovarian or endometrial cancer (5). This suggests, and is the case, that ovarian, breast, and endometrial cancers share many common risk factors, especially related to reproductive events (Table 1).
Later age at menarche reduces the risk for all three cancers (6–11), but no single pattern of cycle regularity or length appears to affect risks in the same way. For ovarian cancer, long (>35 days) or irregular cycles reduce the risk for most histologic types (12). However, the disorder polycystic ovarian syndrome (PCOS), characterized by irregular, anovulatory cycles, does not affect the risk for ovarian cancer overall (13), while PCOS markedly increases endometrial cancer risk (14). The evidence is inconsistent about the effect of irregular cycles and PCOS on breast cancer risk (14–16). There is unequivocal evidence that the use of oral contraceptives (OCs) reduces the risk for ovarian and endometrial cancers (17–20). Some studies (21, 22), but not all (23), suggest that OCs may increase the risk for breast cancer in premenopausal women. For all three cancers, childbirth lowers risk (24–33); an earlier age at first birth reduces the risk for both ovarian and breast cancers (24, 26, 29–34). However, for ovarian cancer, there is evidence that a later age at and shorter interval since the last birth may lower risk more than early age at first birth (35), which also appears to be the case for endometrial cancer (36). Breastfeeding decreases the risk for all three cancers proportional to the length of breastfeeding (37–41). Finally, late age at menopause increases the risk for all three cancers (8, 42–44).
Not included in Table 1 are reproductive events that do not or would not obviously stop ovulation such as tubal ligation, use of intrauterine devices (IUDs), and menopausal hormone use. Tubal ligation is clearly associated with reduced risks for ovarian cancer (45) and, possibly, endometrial cancer (46), but not breast cancer (47). IUD use, even if inert (not progestogen-secreting), clearly reduces the risk for endometrial cancer (48), but limited data suggest no clear effects on breast and ovarian cancers including the progestogen-secreting types (49–51). The literature on menopausal hormones and risks for breast, endometrial, and ovarian cancers is extensive. At the risk of over-simplification, estrogen-only preparation increase the risk for endometrial cancer and certain types of ovarian cancer, while breast cancer risk principally involves combination therapy. We provide a brief comment on the types of oral and non-oral hormonal contraception in relation to ovarian cancer. More contemporary, lower-dose, combination OCs are associated with a decrease in ovarian risk but less so for progestogen-only types (52). Alternatively, based on the equivalent duration of use, Depo-Provera injections may confer a greater reduction in risk than combined OCs, possibly related to residual anovulation that may follow its cessation (53).
While epidemiologic studies of reproductive cancers have mostly focused on the effects of individual reproductive events, interest in a composite variable that combines these events has emerged. Before discussing the evidence linking ovulatory years and cancer risk, we consider issues related to constructing the algorithm to estimate them. Yang et al. evaluated 14 algorithms in relation to ovarian and endometrial cancers (54). They identified five similar algorithms and, among controls, found a high correlation of estimates. All five algorithms used ages at menarche and menopause (or current age) and number of births, while later models added durations of OC use and breastfeeding, number of miscarriages and abortions, and cycle length. Rules for linking anovulatory time with pregnancies varied with some using actual time pregnant or assigning times depending on the outcome—commonly 0.75 or 1 year for term pregnancies and 3 months for a miscarriage or abortion. Women who had a hysterectomy (without removal of ovaries) prior to menopause were either excluded or assigned an average age (approximately 50) for the last period. Women who “always” had irregular periods were either excluded or placed in a separate category.
Another issue relates to co-variates that should be adjusted for in estimating the risk for cancers that might be associated with the length of ovulatory years (LOY). We think it is unnecessary to adjust for the component variables used in calculating ovulatory years since these are already part of the variable. Adjusting for variables that are components of LOY weakens estimates of LOY’s effect on disease risk (and vice versa) (54). In addition to demographic factors, the two important variables that should be considered are body mass index (BMI) and smoking history. Women with greater BMI report earlier menarche (55); greater BMI increases the risk for certain histologic types of ovarian cancer (56), postmenopausal breast cancer (57), and endometrial cancer, markedly so (58). Current and former smoking is associated with earlier menopause (59), increased risk for mucinous ovarian cancer (60), no clear effect on breast cancer risk (61), and decreased risk for endometrial cancer (62).
Citing a study in which ovarian adenocarcinomas developed in hens housed to maximize egg production, Fathalla (63) first linked ovulation to ovarian cancer, coining the phrase “incessant ovulation”. First, to address the hypothesis, Casagrande et al. calculated ovulatory years from ages at menarche and last period minus “protected time” (i.e., births, incomplete pregnancies, and duration of OC use) (64). More ovulatory years increased the risk for ovarian cancer and its inverse, protected time, decreased risk. Additional studies followed confirming the association—some larger ones are noted here (54, 65–68). Associations are stronger with invasive serous, endometrioid, and clear cell cancers and weaker with low-grade or borderline serous tumors and mucinous cancers (68).
Since ovulatory years are not fully “set” until menopause, this might suggest that the association with ovarian cancer will be more apparent after menopause. In fact, the association is stronger in premenopausal women (67). This is also seen with its component variables where the protective effects of pregnancies, OC use, and breastfeeding are stronger in premenopausal than postmenopausal women (67). Purdie et al. reported that more ovulatory years accumulated when women were 20–29 carried a greater risk for ovarian cancer than the number of ovulatory years (in equivalent categories) accumulated at ages 30–39 or 40–49 (66). This suggests that more ovulations earlier in reproductive life carry a greater risk for ovarian cancer occurring before menopause. If so, the reproductive experiences of these women will have been subtracted from those of women who develop ovarian cancer after menopause, accounting for their apparently lower risk with ovulatory years. This would be an example of the depletion of susceptible individuals.
There are numerous data that breast cancer risk is increased by an earlier age at menarche and a later age at menopause (8), and subtracting the two also predicts risk. Longer total menstrual years (69) or longer menstrual years prior to a first livebirth (70) was linked to increased breast cancer risk. A 1984 paper argued that “sterile” menstrual cycles should be distinguished from total menstrual cycles by subtracting time pregnant, lactating, or using OCs to better estimate breast cancer risk (71). Two cohort studies provide evidence that more “sterile” menstrual cycles do increase breast cancer risk. One study found that either total cycles or total cycles prior to a first livebirth significantly increased breast cancer risk (72). Notably, this study examined the effect of lifetime menstrual cycles in models that either included or excluded OC users. The former model was slightly more significant than the model excluding OC users, suggesting that counting OC use adds to the protective effect of other factors that prevent ovulation. A second study confirmed increased breast cancer risk with lifetime (sterile) menstrual cycles but not with the number of cycles prior to a first livebirth (73). Both studies adjusted for BMI but not smoking. The effect of lifetime cycles on types of breast cancer has not been studied, although the large meta-analysis of the effects of earlier menarche and later menopause was most apparent for lobular and estrogen receptor-positive breast cancer (8).
Although PCOS clearly increases endometrial cancer risk (14), there is good evidence that repeated (but regular) ovulatory cycles also increase risk. A case–control study by Pettersson et al. found that a menstrual span of more than 40 years (subtracting births and OC use) led to a fourfold increase in risk compared to women with <25 years (74). Additional case–control and cohort studies found that a longer menstrual span, subtracting at least pregnancies, was associated with a significantly elevated risk for endometrial cancer (54, 75, 76). Notably, the last study showed the association pertained to women with high and low BMI and, also, adjusted for smoking (76). Although this study tabulated data to illustrate that a shorter menstrual span is protective relative to a longer one, their data translate to a nearly identical finding as Pettersson’s study (74); i.e., 40 or more menstrual years equates with about a fivefold increase in risk relative to women with <25 menstrual years.
Thus, LOY is a risk factor common to ovarian, breast, and endometrial cancers. Mechanisms that could explain these associations could either be organ-specific or based on common pathways.
A menstrual cycle is divided into a follicular phase, where follicle-stimulating hormone (FSH) promotes a cohort of developing follicles to secrete estrogen and the endometrium proliferates; ovulation, where the dominant follicle releases its oocyte associated with a spike in FSH and luteinizing hormone (LH); a luteal phase, where the granulosa-thecal layer from the dominant follicle is converted to a progesterone-secreting corpus luteum and the endometrium is converted to a secretory type; and menstrual phase, where the endometrium is shed and a new cohort of follicles selected, if fertilization did not occur (77). The superficial two-thirds of the endometrium (decidua functionalis) is the zone that proliferates, undergoes secretory transition, and sheds if no implantation occurred. The deepest region (decidua basalis) does not undergo significant proliferation but is the source of endometrial regeneration after each menses (78). Tissues undergoing large numbers of cell divisions have a greater likelihood of DNA replication errors compared to tissues with less-active divisions. Estimates of the rate of sporadic mutations in human cells, on the order of 10−7 mutations per gene per cell division (79), suggest that the number of cells with “first hits” on a carcinogenic pathway may number in the hundreds for every gram (approximately 109 cells/g) of proliferating tissue (80). Sporadic mutations of the PTEN tumor suppressor gene, associated with type 1 endometrial cancer, are observed in almost half of histologically normal endometria of naturally cycling women (81). While most OC users do have periods, the endometrium is exposed to progestins for a longer duration, which may decrease the likelihood of survival of PTEN mutant clones in the endometrium (82, 83).
The breast also undergoes cyclic changes, especially involving the terminal ductal lobular units thought to be the source of both lobular and ductal breast cancer (84). Similar to using morphologic changes to date the endometrium during a cycle, changes in breast lobules were considered to correlate with the cycle phase. A study of surgical specimens from premenopausal women with benign breast disease not currently using OCs analyzed the degree of epithelial–myoepithelial distinction, vacuolation, stromal edema, apoptosis, and mitosis as key features (85). Scores were the highest in the late luteal phase, indicating a greater degree of proliferation. Immunohistochemical studies using the Mib-1 antibody to detect the proliferative marker, Ki67+, confirmed this (86). Ki67+ cells were also counted in normal breast tissue in Nurses’ Health Study (NHS) participants with prior biopsies (for benign disease) and demonstrated that women with higher counts had an increased risk for future breast cancer (87). These data suggested to Brisken the direct effects of progesterone (in combination with high estradiol) on breast epithelium (88). However, progesterone and estradiol could be acting indirectly through the action of other hormones elevated in the luteal phase including growth hormone (89). In any case, exposure to a greater number of luteal phases could explain why more cycles increase breast cancer risk.
Proliferative changes in the ovary during a cycle primarily involve stromal, granulosa, and thecal cells that surround immature oocytes and promote them to develop into mature oocytes. However, cancers arising from granulosa, thecal, or germ cells are relatively rare in adult women in whom the predominant types are epithelial tumors. The surface epithelium of the ovary was thought to be the origin of these tumors, and repeated disruption and repair of this layer with each ovulation might lead to p53 mutations, predisposing to epithelial ovarian cancer where defective p53 is a hallmark feature (65). However, a subsequent study did not confirm that those ovarian cancers most clearly linked to a greater number of ovulatory cycles were more likely to be p53 positive (90). Nevertheless, surface epithelial disruption and implantation of endometrial cells shed through the fallopian tubes during menses might be involved in the pathogenesis of other types of ovarian cancer such as clear cell and endometrioid (91).
Around the turn of the century, secretory cells in the distal (fimbriated) end of the fallopian tubes (FTSEC) began to receive attention as the source of high-grade serous ovarian cancers, the most common and lethal type (92). A case was made for the progression of FTSEC with accumulating defective P53 advancing to lesions called serous tubal intraepithelial carcinoma (STIC) (93). STIC could rapidly progress to stage III ovarian cancer by shedding malignant cells into the pelvic cavity to implant. However, rather than the cyclic proliferation of FTSEC causing precursor lesions, repeated exposure of FTSEC to follicular fluid (FF) with ovulation might be the instigating factor. FF contains growth factors, cytokines, and reactive oxygen species (ROS) capable of inducing DNA damage (94). In vitro studies demonstrated that exposure of tubal cells in culture to FF led to the proliferation of FTSEC and P53 accumulation (95), suggesting inflammation as the basis for this progression. Inflammation as a common pathway for cancer, in general, was proposed by Cousens and Werb (96) and for ovarian cancer, in particular, by Sanchez-Pietro et al. (97). This paper was entitled “Etiopathogenesis of ovarian cancer. An inflamm-aging entity?” and reviews the broader role of inflammation in ovarian cancer. However, we point out that the association between ovulation and ovarian cancer is not a simple reflection of more ovulations equals greater age, since age was adjusted for in examining this association.
In addition to the cumulative effects of ovulatory cycles on tissues specific to the breast, endometrium, and ovaries, there are mechanisms that offer more general explanations for LOY as a risk factor for reproductive cancers and, perhaps, other cancers. These include an immune-based model and a hormonal model.
An immune-based model involves human mucin 1 (MUC1), a transmembrane-glycoprotein expressed at the apical cell surfaces of normal glandular epithelium of many organs including breast, salivary glands, gastrointestinal tract, pancreas, bladder, prostate, fallopian tubes, endometrium, and some hematopoietic cells (98). In cancers arising from ductal epithelium, MUC1 becomes overexpressed on the entire cell surface, and a less-glycosylated form of MUC1 is produced. This overexpression favors metastases through several mechanisms: dampening immune response; interacting with other transmembrane proteins, like EGFR; and engaging cytoplasmic-signaling proteins such as Src and beta-catenin (99). Elevated levels of MUC1 are associated with poor prognosis for breast, prostate, ovarian, gastric, bile duct, colon, and non-small cell lung cancers (99). MUC1 is detected by assays based on antibodies against various MUC1 epitopes leading to a variety of names in the literature including CA15.3, DF3 antigen, human milk fat globule (HMFG) antigen, epithelial membrane antigen (EMA), polymorphic epithelium mucin (PEM), and episalin (98).
Assays to measure anti-MUC1 antibodies have also been developed using various MUC1 epitopes associated with tumor-like MUC1 (100). IgM and IgG anti-MUC1 antibodies have been identified in patients with various adenocarcinomas, and their presence has been associated with better prognosis in breast cancer (101), colorectal cancer (102), non-small cell lung cancer (103), and pancreatic cancer (104). These observations prompted interest in immune-based treatments involving MUC1 including vaccines based on MUC1 epitopes coupled with various adjuvants, passive immunization with anti-MUC1 antibodies, transfer of MUC1-specific cytotoxic T cells, and others (105). Most trials involve individuals with advanced cancer, but at least one trial involved patients with colon adenomas at risk for colorectal cancer, demonstrating that high levels of anti-MUC1 IgG could be achieved with a MUC1-based vaccine except in individuals with evidence of pre-existing circulating myeloid-derived suppressor cells (106).
Natural antibodies against MUC1 have also been found in healthy individuals, raising important questions. What events are associated with the appearance of anti-MUC1 antibodies, and do these antibodies contribute to successful immunosurveillance of MUC1+ cancers? Anti-MUC1 antibodies generated in the absence of cancer appear to be promoted by inflammatory events involving tissues that normally produce MUC1. These events may include childhood mumps, puerperal mastitis, and tubal ligation—each shown to raise anti-MUC1 antibodies and each shown to lower the risk for ovarian cancer in case–control studies (107–109). Importantly, there are prospective data from two studies suggesting that natural antibodies against MUC1 can lower the risk for ovarian cancer. Data from the NHS showed that women with higher anti-MUC1 antibodies subsequently had a lower risk for ovarian cancer developing before age 65 (110), and data from the EPIC cohort showed that elevated levels of anti-MUC1 antibodies may lower the risk for serous ovarian cancers arising within 3 years of the blood draw (111). More data on antibody levels at various times after the event that provokes antibodies are necessary to support this premise.
Pointing out that the presence of anti-MUC1 antibodies leads to reduced risk for ovarian cancer, the corollary follows that their absence may increase the risk for cancer. We have shown that a greater number of ovulatory cycles are associated with lower anti-MUC1 antibody levels in three different populations using two different assays to measure anti-MUC1 antibodies (68, 110, 111). We previously interpreted this as indicating that repeated exposure to MUC1 from the endometrium during the luteal phase of cycles may lead to a downregulation of MUC1 immunity and lower antibody levels (68), but it is also possible that lower anti-MUC1 antibody levels with repeated cycles result simply from absence of those events known to raise antibody levels such as pregnancies and breastfeeding. In either case, this brings us to the relevance of MUC1 immunity to the topic of this paper—incessant ovulation. As a common explanation, immunity related to human mucin 1 could explain the risk for not only ovarian, breast, and endometrial cancers but also other MUC1-expressing cancers that have been targets of MUC1 vaccine trials (104), such as colorectal cancer and lung cancer, and point to the possible relevance of ovulatory cycles to their epidemiology.
Prospective studies found that the combination of elevated serum testosterone and estradiol in postmenopausal women predicts future risk for endometrial, breast, and ovarian cancers (112–115). Relating this pattern to the number of past ovulations requires answers to the following questions. What is known about the hormone-producing potential of the postmenopausal ovary? What biological events occurring within the ovary during an ovulatory cycle might enhance later hormone production? Finally, is there any direct evidence that LOY does, in fact, affect hormone levels in postmenopausal women?
The answer to the first question comes from studies in which ovarian veins were cannulated in postmenopausal women at the time of hysterectomy and/or oophorectomy and levels of sex hormones coming directly from the ovaries compared with levels in the peripheral blood (116–118). These studies demonstrated substantially higher levels of testosterone in the blood from the ovaries compared to peripheral levels with gradients up to 20-fold higher and persisting in women even 20 years past menopause. Cannulation studies were also performed in women with ovarian and endometrial cancers and women with breast cancer later undergoing oophorectomy and showed even higher gradients of hormone levels, especially testosterone, compared to women without cancer (119–121). Because levels of testosterone and androstenedione dropped postoperatively (118), the cannulation studies counter the argument that the adrenals are the primary source of androgens in postmenopausal women (122). Cross-sectional studies show that women who had bilateral oophorectomy prior to menopause have low testosterone levels when compared to those with intact ovaries (123).
Biological events during an ovulatory cycle that might change the ovary’s potential for androgen production likely involve the ovary’s germ cell reserve, the process of follicle maturation, and the fate of follicles recruited during a cycle but not selected for ovulation (124, 125). Primordial follicles are oocytes surrounded by a single layer of pre-granulosa cells. The maximum quota of primordial follicles, likely more than a million, is established at birth of which approximately 300,000 remain when puberty begins. During each menstrual cycle, a cohort of follicles is selected to undergo maturation involving the growth of the granulosa layer, formation of a thecal-cell layer, secretion of follicular fluid to form antral follicles, and selection of, usually, just one to become a Graafian follicle from which the oocyte will be released. Remnants of the dominant follicle then form the corpus luteum, which produces progesterone to maintain early pregnancy but will regress without a fertilized oocyte. During a cycle, some follicles that reach the early-antral stage will become quiescent and remain at that stage as a more dynamic reserve than primordial follicles. However, the remainder of those follicles that developed further during a cycle will undergo apoptosis to become atretic follicles. Follicular atresia is likely most relevant to the consequences of repetitive ovulation (126). Evidence that an antral follicle is on the path to atresia is apoptosis within the granulosa cell layer. Estradiol drops from both a decrease in granulosa cell number and reduced aromatization of androgens secreted by thecal cells into estradiol. Thecal cells remain viable after the disappearance of granulosa cells and retain their ability to secrete androgens, but their longer-term fate is unclear.
Possibly relevant to the fate of thecal remnants are two related conditions called ovarian stromal hyperplasia (OSH) and hyperthecosis (OHT). OSH refers to the nodular or diffuse proliferation of the stromal cells of the ovary, while OHT is distinguished by the presence of round to polyhedral theca-lutein-like cells occurring singly or within nests within a stroma that is itself proliferative (127). For decades, these conditions have been of interest in the context of describing ovaries removed from women with endometrial cancer (128). A link between OSH and breast cancer has also been reported (129); case reports describe OHT at the edge of epithelial ovarian malignancies (130). A study that linked elevated testosterone to OSH involved (premenopausal) women presenting with hirsutism who had oophorectomy or wedge resection. Cannulation studies like those described earlier were performed and revealed elevated testosterone and dihydrotestosterone levels in the blood from ovaries, especially from ovaries with more extensive OHT and larger gradients compared to peripheral levels compared to women without OHT (131).
Given this background, is there evidence that LOY influences postmenopausal hormone levels? Two studies in the current literature address this with conflicting results (132, 133). The most recent paper used data from the NHS on hormone levels measured in the blood collected from 1,976 healthy postmenopausal women in 1990 (133). This study excluded women who had bilateral oophorectomy but included hysterectomized women who retained at least one ovary. Age at menopause was imputed in the latter group. In this study, greater LOY was associated with higher testosterone as well as greater estradiol in those women with above-average BMI. This could reflect the accumulation of functioning stromal and thecal cells from repeated ovulation that secrete testosterone, which is converted to estradiol peripherally. An earlier study of 860 postmenopausal women also looked at hormonal levels in relation to lifetime ovulatory cycles (132). This study did not find a relationship between the number of cycles and testosterone or estradiol levels. Adjustment variables, similar to NHS, were used in this study except that OC use was adjusted for, although it was already part of the algorithm to calculate cycles. As mentioned earlier, adjusting for variables that are already components of LOY weakens estimates of its effect on disease risk. In addition, compared to NHS, this study was smaller and had a higher percentage of nulliparous women with lower parity, greater use of birth control pills, and more current smokers. Finally, there was a greater prevalence of hysterectomized women who were excluded in this study. There are many cross-sectional studies of hormone levels in postmenopausal women with sufficient data on reproductive variables to calculate LOY and confirm whether, in fact, more LOY leads to higher testosterone.
We pointed out that the model involving a link between LOY and mucin immunity may pertain to cancers in “non-reproductive” organs. The same may apply to the model involving LOY and sex hormones. Melanoma may be such a cancer. A large prospective study of melanoma in French women looked at ovulatory age and found that postmenopausal women with LOI <33 years had half the risk for melanoma compared to women with LOI >39 years, RR (and 95% CI = 0.51 (0.28, 0.91), equating with greater risk for more ovulatory years (134). Details for constructing the algorithm were not discussed but, apparently, did not include OC use. An earlier case–control study also looked at ovulatory years using a more detailed algorithm that included ages at menarche and menopause (or current age) reduced by 1 year for each pregnancy and total years of OC use. This study also found a greater risk for melanoma associated with longer ovulatory years when adjusted for subjects’ estimated sunlight exposure (135), suggesting additive effects involving sun exposure and LOY. Given our contention that the key effect of past ovulations is on testosterone, this adds relevance to an experimental study reporting that S91 murine melanoma cells grow in response to testosterone and that this effect is modulated by light (136). Additionally, although case reports carry little scientific weight, one that reported the onset of dysplastic nevi in a postmenopausal woman who began using a transdermal patch with testosterone and estradiol that stopped after the patch was discontinued appears relevant (137).
A somewhat unique feature of estrous cycles in women compared to other mammals includes the sloughing of the endometrial lining at the end of a cycle in which an oocyte was not fertilized. This allows estimates of the onset of sexual maturity, end of reproductive potential, and, in between, the frequency of presumed ovulatory cycles that were not interrupted by pregnancies, breastfeeding, or OC use. There are substantial epidemiologic data that support the premise that more estimated ovulatory cycles (captured by the phrase “incessant ovulation”) increase risks for ovarian, breast, and endometrial cancers. One pathway through which these effects may occur includes cumulative effects of repetitive tissue changes within reproductive organs with each ovulatory cycle. In the terminal ductal lobular units of the breast, proliferative activity is increased during the luteal phase of the cycle, which could be a key factor in both lobular and ductal breast cancer. In the endometrium, repetitive shedding and regrowth of the endometrium may lead to the accumulation of mutations in the tumor suppressor, PTEN, a marker for endometrial cancer. In ovaries, the secretory epithelium of the tubal fimbria, with each ovulation, is exposed to follicular fluid whose inflammatory properties may induce the proliferation of cells with defective p53 leading to high-grade serous cancers. Although the vagina and cervix also undergo cyclic changes, cancer involving these organs appears to be dominated by infectious agents. However, it is possible that tissue changes in other organs could be affected by repetitive cycles; ovulatory years should be examined for any cancer in which there are male–female differences in occurrence.
A second pathway might involve immune events related to human mucin 1, a transmembrane glycoprotein, expressed at the apical cell surfaces of normal glandular epithelium of many organs including the breast, fallopian tubes, and endometrium. In cancers arising from these tissues, MUC1 becomes overexpressed on the entire cell surface favoring metastases through several mechanisms and associated with poor prognosis. Conversely, some patients with MUC1-positive cancers have anti-MUC1 antibodies; their presence is associated with better prognosis. Anti-MUC1 antibodies have also been identified in healthy individuals without cancer, and there is some evidence to suggest these antibodies may lower the risk of ovarian cancer. Repetitive ovulation enters the story based on the observation that more ovulatory cycles are associated with lower anti-MUC1 antibodies, which are associated with increased risk for ovarian cancer. It is not clear, however, whether lower anti-MUC1 antibodies seen with more ovulatory cycles reflect immune downregulation from the cyclic elevation of MUC1 released during ovulation or menstruation or simply reflect fewer events like pregnancy and breastfeeding known to produce anti-MUC1 antibodies. This pathway could apply to cancer in which immune-based treatments involving MUC1 have shown some success including colon and small cell lung cancers. The role of ovulatory years in the epidemiology of these cancers should be investigated; the possibility that immune reactions are related to other mucins such as MUC16 (CA125) should be considered.
A third pathway involves residual effects of past ovulations that enhance the postmenopausal ovary’s production of testosterone, which can then be converted to estradiol in peripheral tissue, especially in women with greater BMI. This effect may result from the accumulation of residual stromal and thecal cells recruited during ovulatory cycles that display greater resistance to apoptosis than granulosa cells. Two observations support the relevance of this pathway to explain why more ovulations increase the risk for breast, endometrial, and ovarian cancers. First is the observation that a pattern of elevated testosterone and estradiol in healthy postmenopausal women is associated with a greater risk for these cancers. Second, the ovaries of women with breast and endometrial cancers display patterns described as OSH and OHT. Like the possible pathway involving the immune effect related to mucins, this pathway could support a credible link between more ovulations and cancer in non-reproductive organs including melanoma in which testosterone may play a role.
In conclusion, the estimation of ovulatory cycles deserves much greater attention as a factor in the epidemiology of cancer in women.
The author confirms being the sole contributor of this work and has approved it for publication.
The project was supported by the National Institutes of Health R35CA197605. The content is solely the responsibility of the author and does not necessarily represent the official views of the National Institutes of Health.
DWC reports being paid for expert testimony in litigation related to talc and ovarian cancer.
All claims expressed in this article are solely those of the authors and do not necessarily represent those of their affiliated organizations, or those of the publisher, the editors and the reviewers. Any product that may be evaluated in this article, or claim that may be made by its manufacturer, is not guaranteed or endorsed by the publisher.
1. Harvey PH, Zammuto RM. Patterns of mortality and age at first reproduction in natural populations of mammals. Nature (1985) 315:319–20. doi: 10.1038/315319a0
2. Martin RD. The evolution of human reproduction: a primatological perspective. Am J Phys Anthropol (2007) Suppl 45:59–84. doi: 10.1002/ajpa.20734
3. Sung H, Ferlay J, Siegel RL, Laversanne M, Soerjomataram I, Jemal A, et al. Global cancer statistics 2020: GLOBOCAN estimates of incidence and mortality worldwide for 36 cancers in 185 countries. CA: A Cancer J Clin (2021) 71:209–49. doi: 10.3322/caac.21660
4. Ferlay J, Soerjomataram I, Dikshit R, Eser S, Mathers C, Rebelo M, et al. Cancer incidence and mortality worldwide: sources, methods and major patterns in GLOBOCAN 2012. Int J Cancer (2015) 136:E359–86. doi: 10.1002/ijc.29210
5. Curtis RE, Freedman DM, Ron E, Ries LAG, Hacker DG, Edwards BK, et al (eds). New Malignancies Among Cancer Survivors: SEER Cancer Registries, 1973-2000. Bethesda, MD: National Cancer Institute (2006), pp. 1973–2000.
6. Franceschi S, La Vecchia C, Booth M, Tzonou A, Negri E, Parazzini F, et al. Pooled analysis of 3 European case-control studies of ovarian cancer: II. Age at menarche and at menopause. Int J Cancer (1991) 49:57–60. doi: 10.1002/ijc.2910490111
7. Yang H, Dai H, Li L, Wang X, Wang P, Song F, et al. Age at menarche and epithelial ovarian cancer risk: A meta-analysis and Mendelian randomization study. Cancer Med (2019) 8:4012–22. doi: 10.1002/cam4.2315
8. Collaborative Group on Hormonal Factors in Breast Cancer. Menarche, menopause, and breast cancer risk: individual participant meta-analysis, including 118 964 women with breast cancer from 117 epidemiological studies. Lancet Oncol (2012) 13:1141–51. doi: 10.1016/s1470-2045(12)70425-4
9. Brinton LA, Berman ML, Mortel R, Twiggs LB, Barrett RJ, Wilbanks GD, et al. Reproductive, menstrual, and medical risk factors for endometrial cancer: results from a case-control study. Am J Obstet Gynecol (1992) 167:1317–25. doi: 10.1016/s0002-9378(11)91709-8
10. Brinton LA, Schairer C, Hoover RN, Fraumeni JF Jr. Menstrual factors and risk of breast cancer. Cancer Invest (1988) 6:245–54. doi: 10.3109/07357908809080645
11. Gong TT, Wang YL, Ma XX. Age at menarche and endometrial cancer risk: a dose-response meta-analysis of prospective studies. Sci Rep (2015) 5:14051. doi: 10.1038/srep14051
12. Harris HR, Babic A, Webb PM, Nagle CM, Jordan SJ, Risch HA, et al. Ovarian cancer association, C, and Australian ovarian cancer study, G. Polycystic ovary syndrome, oligomenorrhea, and risk of ovarian cancer histotypes: evidence from the ovarian cancer association consortium. Cancer Epidemiol Biomarkers Prev (2018) 27:174–82. doi: 10.1158/1055-9965.EPI-17-0655
13. Harris HR, Cushing-Haugen KL, Webb PM, Nagle CM, Jordan SJ, Australian Ovarian Cancer Study, G, et al. Association between genetically predicted polycystic ovary syndrome and ovarian cancer: a Mendelian randomization study. Int J Epidemiol (2019) 48:822–30. doi: 10.1093/ije/dyz113
14. Harris HR, Terry KL. Polycystic ovary syndrome and risk of endometrial, ovarian, and breast cancer: a systematic review. Fertil Res Pract (2016) 2:14. doi: 10.1186/s40738-016-0029-2
15. Parazzini F, La Vecchia C, Negri E, Franceschi S, Tozzi L. Lifelong menstrual pattern and risk of breast cancer. Oncology (1993) 50:222–5. doi: 10.1159/000227183
16. Kvale G, Heuch I. Menstrual factors and breast cancer risk. Cancer (1988) 62:1625–31. doi: 10.1002/1097-0142(19881015)62:8<1625::aid-cncr2820620828>3.0.co;2-k
17. Vessey MP, Painter R. Endometrial and ovarian cancer and oral contraceptives–findings in a large cohort study. Br J Cancer (1995) 71:1340–2. doi: 10.1038/bjc.1995.260
18. Collaborative Group on Epidemiological Studies of Ovarian, C, Beral V, Doll R, Hermon C, Peto R, Reeves G. Ovarian cancer and oral contraceptives: collaborative reanalysis of data from 45 epidemiological studies including 23,257 women with ovarian cancer and 87,303 controls. Lancet (2008) 371:303–14. doi: 10.1016/S0140-6736(08)60167-1
19. Havrilesky LJ, Moorman PG, Lowery WJ, Gierisch JM, Coeytaux RR, Urrutia RP, et al. Oral contraceptive pills as primary prevention for ovarian cancer: a systematic review and meta-analysis. Obstet Gynecol (2013) 122:139–47. doi: 10.1097/AOG.0b013e318291c235
20. Weiderpass E, Adami HO, Baron JA, Magnusson C, Lindgren A, Persson I. Use of oral contraceptives and endometrial cancer risk (Sweden). Cancer Causes Control (1999) 10:277–84. doi: 10.1023/a:1008945721786
21. Uk National Case-Control Study, Group. Oral contraceptive use and breast cancer risk in young women. UK National Case-Control Study Group. Lancet (1989) 1:973–82. doi: 10.1016/S0140-6736(89)92627
22. Meirik O, Lund E, Adami HO, Bergström R, Christoffersen T, Bergsjö P. Oral contraceptive use and breast cancer in young women. A joint national case-control study in Sweden and Norway. Lancet (1986) 2:650–4. doi: 10.1016/s0140-6736(86)90166-2
23. Marchbanks PA, McDonald JA, Wilson HG, Folger SG, Mandel MG, Daling JR, et al. Oral contraceptives and the risk of breast cancer. N Engl J Med (2002) 346:2025–32. doi: 10.1056/NEJMoa013202
24. Negri E, Franceschi S, Tzonou A, Booth M, La Vecchia C, Parazzini F, et al. Pooled analysis of 3 European case-control studies: I. Reproductive factors and risk of epithelial ovarian cancer. Int J Cancer (1991) 49:50–6. doi: 10.1002/ijc.2910490110
25. Whittemore AS, Harris R, Itnyre J. Characteristics relating to ovarian cancer risk: collaborative analysis of 12 US case-control studies. II. Invasive epithelial ovarian cancers in white women. Collaborative Ovarian Cancer Group. Am J Epidemiol (1992) 136:1184–203. doi: 10.1093/oxfordjournals.aje.a116427
26. Adami HO, Hsieh CC, Lambe M, Trichopoulos D, Leon D, Persson I, et al. Parity, age at first childbirth, and risk of ovarian cancer. Lancet (1994) 344:1250–4. doi: 10.1016/s0140-6736(94)90749-8
27. Titus-Ernstoff L, Perez K, Cramer DW, Harlow BL, Baron JA, Greenberg ER. Menstrual and reproductive factors in relation to ovarian cancer risk. Br J Cancer (2001) 84:714–21. doi: 10.1054/bjoc.2000.1596
28. Albrektsen G, Heuch I, Kvåle G. Reproductive factors and incidence of epithelial ovarian cancer: a Norwegian prospective study. Cancer Causes Control (1996) 7:421–7. doi: 10.1007/bf00052668
29. Ewertz M, Duffy SW, Adami HO, Kvåle G, Lund E, Meirik O, et al. Age at first birth, parity and risk of breast cancer: a meta-analysis of 8 studies from the Nordic countries. Int J Cancer (1990) 46:597–603. doi: 10.1002/ijc.2910460408
30. Lambe M, Hsieh CC, Chan HW, Ekbom A, Trichopoulos D, Adami HO. Parity, age at first and last birth, and risk of breast cancer: a population-based study in Sweden. Breast Cancer Res Treat (1996) 38:305–11. doi: 10.1007/bf01806150
31. Parazzini F, La Vecchia C, Negri E, Fedele L, Balotta F. Reproductive factors and risk of endometrial cancer. Am J Obstet Gynecol (1991) 164:522–7. doi: 10.1016/s0002-9378(11)80012-8
32. Parslov M, Lidegaard O, Klintorp S, Pedersen B, Jonsson L, Eriksen PS, et al. Risk factors among young women with endometrial cancer: a Danish case-control study. Am J Obstet Gynecol (2000) 182:23–9. doi: 10.1016/s0002-9378(00)70486-8
33. Lambe M, Wuu J, Weiderpass E, Hsieh CC. Childbearing at older age and endometrial cancer risk (Sweden). Cancer Causes Control (1999) 10:43–9. doi: 10.1023/a:1008860615584
34. MacMahon B, Cole P, Lin TM, Lowe CR, Mirra AP, Ravnihar B, et al. Age at first birth and breast cancer risk. Bull World Health Organ (1970) 43:209–21.
35. Wu Y, Sun W, Xin X, Wang W, Zhang D. Age at last birth and risk of developing epithelial ovarian cancer: a meta-analysis. Biosci Rep (2019) 39:1–11. doi: 10.1042/bsr20182035
36. Setiawan VW, Pike MC, Karageorgi S, Deming SL, Anderson K, Bernstein L, et al. Age at last birth in relation to risk of endometrial cancer: pooled analysis in the epidemiology of endometrial cancer consortium. Am J Epidemiol (2012) 176:269–78. doi: 10.1093/aje/kws129
37. Luan NN, Wu QJ, Gong TT, Vogtmann E, Wang YL, Lin B. Breastfeeding and ovarian cancer risk: a meta-analysis of epidemiologic studies. Am J Clin Nutr (2013) 98:1020–31. doi: 10.3945/ajcn.113.062794
38. Li DP, Du C, Zhang ZM, Li GX, Yu ZF, Wang X, et al. Breastfeeding and ovarian cancer risk: a systematic review and meta-analysis of 40 epidemiological studies. Asian Pac J Cancer Prev (2014) 15:4829–37. doi: 10.7314/apjcp.2014.15.12.4829
39. Layde PM, Webster LA, Baughman AL, Wingo PA, Rubin GL, Ory HW. The independent associations of parity, age at first full term pregnancy, and duration of breastfeeding with the risk of breast cancer. Cancer and Steroid Hormone Study Group. J Clin Epidemiol (1989) 42:963–73. doi: 10.1016/0895-4356(89)90161-3
40. Rosenblatt KA, Thomas DB. Prolonged lactation and endometrial cancer. WHO Collaborative Study of Neoplasia and Steroid Contraceptives. Int J Epidemiol (1995) 24:499–503. doi: 10.1093/ije/24.3.499
41. Newcomb PA, Trentham-Dietz A. Breast feeding practices in relation to endometrial cancer risk, USA. Cancer Causes Control (2000) 11:663–7. doi: 10.1023/a:1008978624266
42. Wentzensen N, Poole EM, Trabert B, White E, Arslan AA, Patel AV, et al. Ovarian cancer risk factors by histologic subtype: an analysis from the ovarian cancer cohort consortium. J Clin Oncol (2016) 34:2888–98. doi: 10.1200/JCO.2016.66.8178
43. Trichopoulos D, MacMahon B, Cole P. Menopause and breast cancer risk. J Natl Cancer Inst (1972) 48:605–13. https://doi-org.ezp-prod1.hul.harvard.edu/10.1093/jnci/48.3.605.
44. Kvåle G, Heuch I, Ursin G. Reproductive factors and risk of cancer of the uterine corpus: a prospective study. Cancer Res (1988) 48:6217–21.
45. Rice MS, Murphy MA, Tworoger SS. Tubal ligation, hysterectomy and ovarian cancer: A meta-analysis. J Ovarian Res (2012) 5:13. doi: 10.1186/1757-2215-5-13
46. Loghmani L, Saedi N, Omani-Samani R, Safiri S, Sepidarkish M, Maroufizadeh S, et al. Tubal ligation and endometrial Cancer risk: a global systematic review and meta-analysis. BMC Cancer (2019) 19:942. doi: 10.1186/s12885-019-6174-3
47. Brinton LA, Gammon MD, Coates RJ, Hoover RN. Tubal ligation and risk of breast cancer. Br J Cancer (2000) 82:1600–4. doi: 10.1054/bjoc.1999.1182
48. Felix AS, Gaudet MM, La Vecchia C, Nagle CM, Shu XO, Weiderpass E, et al. Intrauterine devices and endometrial cancer risk: a pooled analysis of the Epidemiology of Endometrial Cancer Consortium. Int J Cancer (2015) 136:E410–22. doi: 10.1002/ijc.29229
49. D'Alessandro G, Frigerio M, Barra F, Costantini S, Gustavino C, Ferrero S. Systematic review and meta-analysis on the impact of the levonorgestrel-releasing intrauterine system in reducing risk of ovarian cancer. Int J Gynaecol Obstet (2022) 156:418–24. doi: 10.1002/ijgo.13737
50. Dinger J, Bardenheuer K, Minh TD. Levonorgestrel-releasing and copper intrauterine devices and the risk of breast cancer. Contraception (2011) 83:211–7. doi: 10.1016/j.contraception.2010.11.009
51. Yang J, Sasamoto N, Babic A, Vitonis AF, Townsend MK, Titus L, et al. Intrauterine device use and risk of ovarian cancer: Results from the New England Case-Control study and Nurses' Health Studies. Int J Cancer (2021) 149:75–83. doi: 10.1002/ijc.33531
52. Iversen L, Fielding S, Lidegaard O, Morch LS, Skovlund CW, Hannaford PC. Association between contemporary hormonal contraception and ovarian cancer in women of reproductive age in Denmark: prospective, nationwide cohort study. BMJ (2018) 362:k3609. doi: 10.1136/bmj.k3609
53. Phung MT, Lee AW, Wu AH, Berchuck A, Cho KR, Cramer DW, et al. Depot-medroxyprogesterone acetate use is associated with decreased risk of ovarian cancer: the mounting evidence of a protective role of progestins. Cancer Epidemiol Biomarkers Prev (2021) 30:927–35. doi: 10.1158/1055-9965.EPI-20-1355
54. Yang HP, Murphy KR, Pfeiffer RM, George N, Garcia-Closas M, Lissowska J, et al. Lifetime number of ovulatory cycles and risks of ovarian and endometrial cancer among postmenopausal women. Am J Epidemiol (2016) 183:800–14. doi: 10.1093/aje/kwv308
55. Karapanou O, Papadimitriou A. Determinants of menarche. Reprod Biol Endocrinol (2010) 8:115. doi: 10.1186/1477-7827-8-115
56. Olsen CM, Nagle CM, Whiteman DC, Ness R, Pearce CL, Pike MC, et al. Obesity and risk of ovarian cancer subtypes: evidence from the Ovarian Cancer Association Consortium. Endocr Relat Cancer (2013) 20:251–62. doi: 10.1530/ERC-12-0395
57. Xue F, Diabetes KB. metabolic syndrome, and breast cancer: a review of the current evidence. Am J Clin Nutr (2007) 86:s823–35. doi: 10.1093/ajcn/86.3.823S
58. Zhang Y, Liu H, Yang S, Zhang J, Qian L, Chen X. Overweight, obesity and endometrial cancer risk: results from a systematic review and meta-analysis. Int J Biol Markers (2014) 29:e21–9. doi: 10.5301/jbm.5000047
59. Midgette AS, Baron JA. Cigarette smoking and the risk of natural menopause. Epidemiology (1990) 1:474–80. doi: 10.1097/00001648-199011000-00010
60. Faber MT, Kjaer SK, Dehlendorff C, Chang-Claude J, Andersen KK, Hogdall E, et al. Cigarette smoking and risk of ovarian cancer: a pooled analysis of 21 case-control studies. Cancer Causes Control (2013) 24:989–1004. doi: 10.1007/s10552-013-0174-4
61. Terry PD, Rohan TE. Cigarette smoking and the risk of breast cancer in women: a review of the literature. Cancer Epidemiol Biomarkers Prev (2002) 11:953–71.
62. Zhou B, Yang L, Sun Q, Cong R, Gu H, Tang N, et al. Cigarette smoking and the risk of endometrial cancer: a meta-analysis. Am J Med (2008) 121:501–8 e3. doi: 10.1016/j.amjmed.2008.01.044
63. Fathalla MF. Incessant ovulation–a factor in ovarian neoplasia? Lancet (1971) 2:163. doi: 10.1016/s0140-6736(71)92335-x
64. Casagrande JT, Louie EW, Pike MC, Roy S, Ross RK, Henderson BE. "Incessant ovulation" and ovarian cancer. Lancet (1979) 2:170–3. doi: 10.1016/s0140-6736(79)91435-1
65. Schildkraut JM, Bastos E, Berchuck A. Relationship between lifetime ovulatory cycles and overexpression of mutant p53 in epithelial ovarian cancer. J Natl Cancer Inst (1997) 89:932–8. doi: 10.1093/jnci/89.13.932
66. Purdie DM, Bain CJ, Siskind V, Webb PM, Green AC. Ovulation and risk of epithelial ovarian cancer. Int J Cancer (2003) 104:228–32. doi: 10.1002/ijc.10927
67. Tung KH, Wilkens LR, Wu AH, McDuffie K, Nomura AM, Kolonel LN, et al. Effect of anovulation factors on pre- and postmenopausal ovarian cancer risk: revisiting the incessant ovulation hypothesis. Am J Epidemiol (2005) 161:321–9. doi: 10.1093/aje/kwi046
68. Terry KL, Titus-Ernstoff L, McKolanis JR, Welch WR, Finn OJ, Cramer DW. Incessant ovulation, mucin 1 immunity, and risk for ovarian cancer. Cancer Epidemiol Biomarkers Prev (2007) 16:30–5. doi: 10.1158/1055-9965.Epi-06-0688
69. Whitehead J, Carlile T, Kopecky KJ, Thompson DJ, Gilbert FI Jr., Present AJ, et al. The relationship between Wolfe's classification of mammograms, accepted breast cancer risk factors, and the incidence of breast cancer. Am J Epidemiol (1985) 122:994–1006. doi: 10.1093/oxfordjournals.aje.a114203
70. Brignone G, Cusimano R, Dardanoni G, Gugliuzza M, Lanzarone F, Scibilia V, et al. A case-control study on breast cancer risk factors in a Southern European population. Int J Epidemiol (1987) 16:356–61. doi: 10.1093/ije/16.3.356
71. Johansson ED. The sterile menstrual cycle. The driving force behind pathology in the reproductive organs. Acta Obstet Gynecol Scand Suppl (1984) 123:147–50. doi: 10.3109/00016348409157003
72. Clavel-Chapelon F, Group EN. Cumulative number of menstrual cycles and breast cancer risk: results from the E3N cohort study of French women. Cancer Causes Control (2002) 13:831–8. doi: 10.1023/a:1020684821837
73. Chavez-MacGregor M, Elias SG, Onland-Moret NC, van der Schouw YT, Van Gils CH, Monninkhof E, et al. Postmenopausal breast cancer risk and cumulative number of menstrual cycles. Cancer Epidemiol Biomarkers Prev (2005) 14:799–804. doi: 10.1158/1055-9965.EPI-04-0465
74. Pettersson B, Adami HO, Bergstrom R, Johansson ED. Menstruation span–a time-limited risk factor for endometrial carcinoma. Acta Obstet Gynecol Scand (1986) 65:247–55. doi: 10.3109/00016348609155179
75. McPherson CP, Sellers TA, Potter JD, Bostick RM, Folsom AR. Reproductive factors and risk of endometrial cancer. The Iowa Women's Health Study. Am J Epidemiol (1996) 143:1195–202. doi: 10.1093/oxfordjournals.aje.a008707
76. Gavrilyuk O, Braaten T, Weiderpass E, Licaj I, Lund E. Lifetime number of years of menstruation as a risk index for postmenopausal endometrial cancer in the Norwegian Women and Cancer Study. Acta Obstet Gynecol Scand (2018) 97:1168–77. doi: 10.1111/aogs.13381
77. Noyes RW, Hertig AT, Rock J. Dating the endometrial biopsy. Am J Obstet Gynecol (1975) 122:262–3. doi: 10.1016/s0002-9378(16)33500-1
78. Wilborn WH, Flowers CE. Cellular mechanisms for endometrial conservation during menstrual bleeding. Semin Reprod Med (1984) 2:307–41. doi: 10.1055/s-2007-1023848
79. Cairns J. Mutation and cancer: the antecedents to our studies of adaptive mutation. Genetics (1998) 148:1433–40. doi: 10.1093/genetics/148.4.1433
80. Moolgavkar SH, Knudson AG Jr. Mutation and cancer: a model for human carcinogenesis. J Natl Cancer Inst (1981) 66:1037–52. doi: 10.1093/jnci/66.6.1037
81. Mutter GL, Ince TA, Baak JP, Kust GA, Zhou XP, Eng C. Molecular identification of latent precancers in histologically normal endometrium. Cancer Res (2001) 61:4311–4.
82. Orbo A, Rise CE, Mutter GL. Regression of latent endometrial precancers by progestin infiltrated intrauterine device. Cancer Res (2006) 66:5613–7. doi: 10.1158/0008-5472.CAN-05-4321
83. Zheng W, Baker HE, Mutter GL. Involution of PTEN-null endometrial glands with progestin therapy. Gynecol Oncol (2004) 92:1008–13. doi: 10.1016/j.ygyno.2003.11.026
84. Wellings SR, Jensen HM, Marcum RG. An atlas of subgross pathology of the human breast with special reference to possible precancerous lesions. J Natl Cancer Inst (1975) 55:231–73. doi: 10.1093/jnci/55.2.231
85. Ramakrishnan R, Khan SA, Badve S. Morphological changes in breast tissue with menstrual cycle. Mod Pathol (2002) 15:1348–56. doi: 10.1097/01.MP.0000039566.20817.46
86. Soderqvist G, Isaksson E, von Schoultz B, Carlstrom K, Tani E, Skoog L. Proliferation of breast epithelial cells in healthy women during the menstrual cycle. Am J Obstet Gynecol (1997) 176:123–8. doi: 10.1016/s0002-9378(97)80024-5
87. Huh SJ, Oh H, Peterson MA, Almendro V, Hu R, Bowden M, et al. The proliferative activity of mammary epithelial cells in normal tissue predicts breast cancer risk in premenopausal women. Cancer Res (2016) 76:1926–34. doi: 10.1158/0008-5472.Can-15-1927
88. Brisken C. Progesterone signalling in breast cancer: a neglected hormone coming into the limelight. Nat Rev Cancer (2013) 13:385–96. doi: 10.1038/nrc3518
89. Lombardi S, Honeth G, Ginestier C, Shinomiya I, Marlow R, Buchupalli B, et al. Growth hormone is secreted by normal breast epithelium upon progesterone stimulation and increases proliferation of stem/progenitor cells. Stem Cell Rep (2014) 2:780–93. doi: 10.1016/j.stemcr.2014.05.005
90. Webb PM, Green A, Cummings MC, Purdie DM, Walsh MD, Chenevix-Trench G. Relationship between number of ovulatory cycles and accumulation of mutant p53 in epithelial ovarian cancer. J Natl Cancer Inst (1998) 90:1729–34. doi: 10.1093/jnci/90.22.1729
91. Kurman RJ, Shih Ie M. The origin and pathogenesis of epithelial ovarian cancer: a proposed unifying theory. Am J Surg Pathol (2010) 34:433–43. doi: 10.1097/PAS.0b013e3181cf3d79
92. Medeiros F, Muto MG, Lee Y, Elvin JA, Callahan MJ, Feltmate C, et al. The tubal fimbria is a preferred site for early adenocarcinoma in women with familial ovarian cancer syndrome. Am J Surg Pathol (2006) 30:230–6. doi: 10.1097/01.pas.0000180854.28831.77
93. Kindelberger DW, Lee Y, Miron A, Hirsch MS, Feltmate C, Medeiros F, et al. Intraepithelial carcinoma of the fimbria and pelvic serous carcinoma: Evidence for a causal relationship. Am J Surg Pathol (2007) 31:161–9. doi: 10.1097/01.pas.0000213335.40358.47
94. Baskind NE, Orsi NM, Sharma V. Follicular-phase ovarian follicular fluid and plasma cytokine profiling of natural cycle in vitro fertilization patients. Fertil Steril (2014) 102:410–8. doi: 10.1016/j.fertnstert.2014.04.032
95. Bahar-Shany K, Brand H, Sapoznik S, Jacob-Hirsch J, Yung Y, Korach J, et al. Exposure of fallopian tube epithelium to follicular fluid mimics carcinogenic changes in precursor lesions of serous papillary carcinoma. Gynecol Oncol (2014) 132:322–7. doi: 10.1016/j.ygyno.2013.12.015
97. Sanchez-Prieto M, Sanchez-Borrego R, Lubian-Lopez DM, Perez-Lopez FR. Etiopathogenesis of ovarian cancer. An inflamm-aging entity? Gynecol Oncol Rep (2022) 42:101018. doi: 10.1016/j.gore.2022.101018
98. von Mensdorff-Pouilly S, Snijdewint FG, Verstraeten AA, Verheijen RH, Kenemans P. Human MUC1 mucin: a multifaceted glycoprotein. Int J Biol Markers (2000) 15:343–56. doi: 10.1177/172460080001500413
99. Horm TM, Schroeder JA. MUC1 and metastatic cancer: expression, function and therapeutic targeting. Cell Adh Migr (2013) 7:187–98. doi: 10.4161/cam.23131
100. Von Mensdorff-Pouilly S, Moreno M, Verheijen RH. Natural and induced humoral responses to MUC1. Cancers (Basel) (2011) 3:3073–103. doi: 10.3390/cancers3033073
101. Blixt O, Bueti D, Burford B, Allen D, Julien S, Hollingsworth M, et al. Autoantibodies to aberrantly glycosylated MUC1 in early stage breast cancer are associated with a better prognosis. Breast Cancer Res (2011) 13:R25. doi: 10.1186/bcr2841
102. Nakamura H, Hinoda Y, Nakagawa N, Makiguchi Y, Itoh F, Endo T, et al. Detection of circulating anti-MUC1 mucin core protein antibodies in patients with colorectal cancer. J Gastroenterol (1998) 33:354–61. doi: 10.1007/s005350050096
103. Hirasawa Y, Kohno N, Yokoyama A, Kondo K, Hiwada K, Miyake M. Natural autoantibody to MUC1 is a prognostic indicator for non-small cell lung cancer. Am J Respir Crit Care Med (2000) 161:589–94. doi: 10.1164/ajrccm.161.2.9905028
104. Hamanaka Y, Suehiro Y, Fukui M, Shikichi K, Imai K, Hinoda Y. Circulating anti-MUC1 IgG antibodies as a favorable prognostic factor for pancreatic cancer. Int J Cancer (2003) 103:97–100. doi: 10.1002/ijc.10801
105. Gao T, Cen Q, Lei H. A review on development of MUC1-based cancer vaccine. BioMed Pharmacother (2020) 132:110888. doi: 10.1016/j.biopha.2020.110888
106. Kimura T, McKolanis JR, Dzubinski LA, Islam K, Potter DM, Salazar AM, et al. MUC1 vaccine for individuals with advanced adenoma of the colon: a cancer immunoprevention feasibility study. Cancer Prev Res (Phila) (2013) 6:18–26. doi: 10.1158/1940-6207.CAPR-12-0275
107. Cramer DW, Titus-Ernstoff L, McKolanis JR, Welch WR, Vitonis AF, Berkowitz RS, et al. Conditions associated with antibodies against the tumor-associated antigen MUC1 and their relationship to risk for ovarian cancer. Cancer Epidemiol Biomarkers Prev (2005) 14:1125–31. doi: 10.1158/1055-9965.EPI-05-0035
108. Cramer DW, Vitonis AF, Pinheiro SP, McKolanis JR, Fichorova RN, Brown KE, et al. Mumps and ovarian cancer: modern interpretation of an historic association. Cancer Causes Control (2010) 21:1193–201. doi: 10.1007/s10552-010-9546-1
109. Cramer DW, Williams K, Vitonis AF, Yamamoto HS, Stuebe A, Welch WR, et al. Puerperal mastitis: a reproductive event of importance affecting anti-mucin antibody levels and ovarian cancer risk. Cancer Causes Control (2013) 24:1911–23. doi: 10.1007/s10552-013-0266-1
110. Pinheiro SP, Hankinson SE, Tworoger SS, Rosner BA, McKolanis JR, Finn OJ, et al. Anti-MUC1 antibodies and ovarian cancer risk: prospective data from the Nurses' Health Studies. Cancer Epidemiol Biomarkers Prev (2010) 19:1595–601. doi: 10.1158/1055-9965.EPI-10-0068
111. Cramer DW, Fichorova RN, Terry KL, Yamamoto H, Vitonis AF, Ardanaz E, et al. Anti-CA15.3 and anti-CA125 antibodies and ovarian cancer risk: results from the EPIC cohort. Cancer Epidemiol Biomarkers Prev (2018) 27:790–804. doi: 10.1158/1055-9965.EPI-17-0744
112. Cauley JA, Lucas FL, Kuller LH, Stone K, Browner W, Cummings SR. Elevated serum estradiol and testosterone concentrations are associated with a high risk for breast cancer. Study of Osteoporotic Fractures Research Group. Ann Intern Med (1999) 130:270–7. doi: 10.7326/0003-4819-130-4_part_1-199902160-00004
113. Allen NE, Key TJ, Dossus L, Rinaldi S, Cust A, Lukanova A, et al. Endogenous sex hormones and endometrial cancer risk in women in the European Prospective Investigation into Cancer and Nutrition (EPIC). Endocr Relat Cancer (2008) 15:485–97. doi: 10.1677/ERC-07-0064
114. Ose J, Poole EM, Schock H, Lehtinen M, Arslan AA, Zeleniuch-Jacquotte A, et al. Androgens are differentially associated with ovarian cancer subtypes in the ovarian cancer cohort consortium. Cancer Res (2017) 77:3951–60. doi: 10.1158/0008-5472.CAN-16-3322
115. Johansson A, Schmitz D, Hoglund J, Hadizadeh F, Karlsson T, Ek WE. Investigating the effect of estradiol levels on the risk of breast, endometrial, and ovarian cancer. J Endocr Soc (2022) 6:bvac100. doi: 10.1210/jendso/bvac100
116. Judd HL, Judd GE, Lucas WE, Yen SS. Endocrine function of the postmenopausal ovary: concentration of androgens and estrogens in ovarian and peripheral vein blood. J Clin Endocrinol Metab (1974) 39:1020–4. doi: 10.1210/jcem-39-6-1020
117. Sluijmer AV, Heineman MJ, De Jong FH, Evers JL. Endocrine activity of the postmenopausal ovary: the effects of pituitary down-regulation and oophorectomy. J Clin Endocrinol Metab (1995) 80:2163–7. doi: 10.1210/jcem.80.7.7608272
118. Fogle RH, Stanczyk FZ, Zhang X, Paulson RJ. Ovarian androgen production in postmenopausal women. J Clin Endocrinol Metab (2007) 92:3040–3. doi: 10.1210/jc.2007-0581
119. Aiman J, Forney JP, Parker CR Jr. Secretion of androgens and estrogens by normal and neoplastic ovaries in postmenopausal women. Obstet Gynecol (1986) 68:1–5. doi: 10.1097/00006250-198609000-00007
120. Botella-Llusiá J, Oriol-Bosch A, Sánchez-Garrido F, Tresguerres JAF. Testosterone and 17β-oestradiol secretion of the human ovary. II. Normal postmenopausal women, postmenopausal women with endometrial hyperplasia and postmenopausal women with adenocarcinoma of the endometrium. Maturitas (1980) 2:7–12. doi: 10.1016/0378-5122(80)90054-7
121. Horton R, ROmanoff E, Walker J. Androstenedione and testosterone in ovarian venous and peripheral plasma during ovariectomy for breast cancer. J Clin Endocrinol Metab (1966) 26:1267–9. doi: 10.1210/jcem-26-11-1267
122. Couzinet B, Meduri G, Lecce MG, Young J, Brailly S, Loosfelt H, et al. The postmenopausal ovary is not a major androgen-producing gland. J Clin Endocrinol Metab (2001) 86:5060–6. doi: 10.1210/jcem.86.10.7900
123. Key TJ, Appleby PN, Reeves GK, Roddam AW, Helzlsouer KJ, Alberg AJ, et al. Circulating sex hormones and breast cancer risk factors in postmenopausal women: reanalysis of 13 studies. Br J Cancer (2011) 105:709–22. doi: 10.1038/bjc.2011.254
124. Findlay JK, Hutt KJ, Hickey M, Anderson RA. How is the number of primordial follicles in the ovarian reserve established? Biol Reprod (2015) 93:111. doi: 10.1095/biolreprod.115.133652
125. Monniaux D, Clement F, Dalbies-Tran R, Estienne A, Fabre S, Mansanet C, et al. The ovarian reserve of primordial follicles and the dynamic reserve of antral growing follicles: what is the link? Biol Reprod (2014) 90:85. doi: 10.1095/biolreprod.113.117077
126. Maxson WS, Haney AF, Schomberg DW. Steroidogenesis in porcine atretic follicles: loss of aromatase activity in isolated granulosa and theca. Biol Reprod (1985) 33:495–501. doi: 10.1095/biolreprod33.2.495
127. Boss JH, Scully RE, Wegner KH, Cohen RB. Structural variations in the adult ovary. Clinical significance. Obstet Gynecol (1965) 25:747–64.
128. Woll E, Hertig AT, Smith GVS, Johnson LC. The ovary in endometrial carcinoma with notes on the morphological history of the aging ovary. Am J Obstet Gynecol (1948) 56:617–33. doi: 10.1016/0002-9378(48)90400-1
129. Sommers SC, Teloh HA. Ovarian stromal hyperplasia in breast cancer. AMA Arch Pathol (1952) 53:160–6. doi: 10.1097/00006254-195208000-00045
130. Blanco LZ Jr., Kuhn E, Morrison JC, Bahadirli-Talbott A, Smith-Sehdev A, Kurman RJ. Steroid hormone synthesis by the ovarian stroma surrounding epithelial ovarian tumors: a potential mechanism in ovarian tumorigenesis. Mod Pathol (2017) 30:563–76. doi: 10.1038/modpathol.2016.219
131. Nagamani M, Lingold JC, Gomez LG, Garza JR. Clinical and hormonal studies in hyperthecosis of the ovaries. Fertil Steril (1981) 36:326–32. doi: 10.1016/s0015-0282(16)45733-x
132. Chavez-MacGregor M, van Gils CH, van der Schouw YT, Monninkhof E, van Noord PA, Peeters PH. Lifetime cumulative number of menstrual cycles and serum sex hormone levels in postmenopausal women. Breast Cancer Res Treat (2008) 108:101–12. doi: 10.1007/s10549-007-9574-z
133. Cramer DW, Vitonis AF, Huang T, Shafrir AL, Eliassen AH, Barbieri RL, et al. Estimated ovulatory years prior to menopause and postmenopausal endogenous hormone levels. Cancer Epidemiol Biomarkers Prev (2023) 32(7):976–85. doi: 10.1158/1055-9965.EPI-23-0102
134. Kvaskoff M, Bijon A, Mesrine S, Boutron-Ruault MC, Clavel-Chapelon F. Cutaneous melanoma and endogenous hormonal factors: a large French prospective study. Am J Epidemiol (2011) 173:1192–202. doi: 10.1093/aje/kwq503
135. Green A, Bain C. Hormonal factors and melanoma in women. Med J Aust (1985) 142:446–8. doi: 10.5694/j.1326-5377.1985.tb113446.x
136. Allil PA, Visconti MA, Castrucci AM, Isoldi MC. Photoperiod and testosterone modulate growth and melanogenesis of s91 murine melanoma. Med Chem (2008) 4:100–5. doi: 10.2174/157340608783789185
Keywords: ovulatory years, gynecologic cancer, breast cancer, testosterone, estradiol
Citation: Cramer DW (2023) Incessant ovulation: a review of its importance in predicting cancer risk. Front. Oncol. 13:1240309. doi: 10.3389/fonc.2023.1240309
Received: 14 June 2023; Accepted: 16 August 2023;
Published: 06 October 2023.
Edited by:
Louis Dubeau, University of Southern California, United StatesReviewed by:
Rafael Sanchez-Borrego, Hospital Quirón Teknon, SpainCopyright © 2023 Cramer. This is an open-access article distributed under the terms of the Creative Commons Attribution License (CC BY). The use, distribution or reproduction in other forums is permitted, provided the original author(s) and the copyright owner(s) are credited and that the original publication in this journal is cited, in accordance with accepted academic practice. No use, distribution or reproduction is permitted which does not comply with these terms.
*Correspondence: Daniel W. Cramer, ZGNyYW1lckBid2guaGFydmFyZC5lZHU=
Disclaimer: All claims expressed in this article are solely those of the authors and do not necessarily represent those of their affiliated organizations, or those of the publisher, the editors and the reviewers. Any product that may be evaluated in this article or claim that may be made by its manufacturer is not guaranteed or endorsed by the publisher.
Research integrity at Frontiers
Learn more about the work of our research integrity team to safeguard the quality of each article we publish.