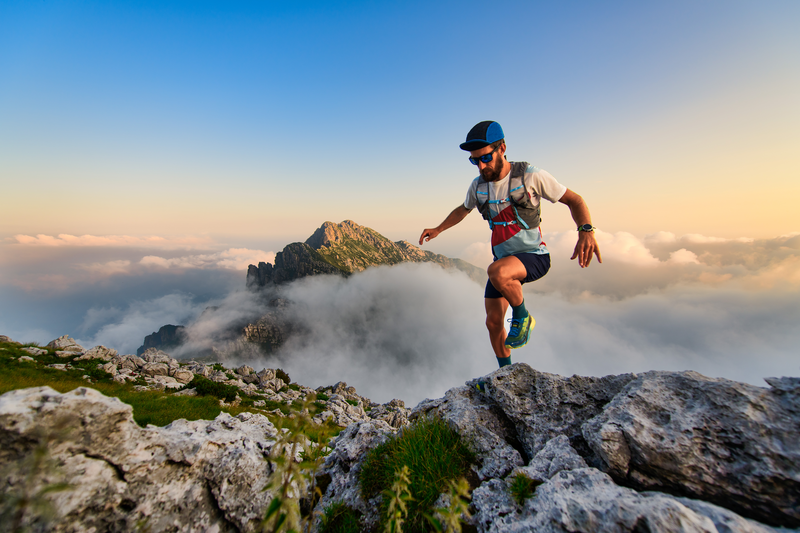
94% of researchers rate our articles as excellent or good
Learn more about the work of our research integrity team to safeguard the quality of each article we publish.
Find out more
ORIGINAL RESEARCH article
Front. Oncol. , 21 August 2023
Sec. Cancer Immunity and Immunotherapy
Volume 13 - 2023 | https://doi.org/10.3389/fonc.2023.1229905
This article is part of the Research Topic Insights and Implications of Mechanisms Shaping Glioma Immune Landscape, Immunotherapy Resistance, and Relevant Translational Research View all 6 articles
Background: Glioma is the most common primary brain tumor. Traditional treatments for glioma include surgical resection, radiotherapy, chemotherapy, and bevacizumab therapy, but their efficacies are limited. Immunotherapy provides a new direction for glioma treatment. This study aimed to summarize the knowledge structure and research hotspots of glioma immunotherapy through a bibliometric analysis.
Method: Publications pertaining to glioma immunotherapy published during the period from 1st January 1990 to 27th March 2023 were downloaded from the Web of Science Core Collection (WoSCC). Bibliometric analysis and visualization were performed using the CiteSpace, VOSviewer, Online Analysis Platform of Literature Metrology, and R software. The hotspots and prospects of glioma immunotherapy research were illustrated via analyzing the countries, institutions, journals, authors, citations and keywords of eligible publications.
Results: A total of 1,929 publications pertaining to glioma immunotherapy in 502 journals were identified as of 27th March 2023, involving 9,505 authors from 1,988 institutions in 62 countries. Among them were 1,285 articles and 644 reviews. Most of publications were produced by the United States. JOURNAL OF NEURO-ONCOLOGY published the majority of publications pertaining to glioma immunotherapy. Among the authors, Lim M contributed the largest number of publications. Through analyzing keyword bursts and co-cited references, immune-checkpoint inhibitors (ICIs) were identified as the research focus and hotspot.
Conclusion: Using a bibliometric analysis, this study provided the knowledge structure and research hotspots in glioma immunotherapy research during the past 33 years, with ICIs staying in the current and future hotspot. Our findings may direct the research of glioma immunotherapy in the future.
Glioma, the most common primary brain tumor, usually originates from glial cells or precursor cells and appears in many forms, such as astrocytoma, oligodendroglioma, and ventricular meningioma (1, 2). According to the revised 2016 WHO classification of CNS tumors, glioma is classified into four grades. Adult glioma is classified into three main categories based on the absence or presence of mutations in IDH, or 1p and 19q (1p/19q) chromosomal co-deletion: IDH-mutant, 1p/19q-co-deleted tumors which are predominantly oligodendrocytic; IDH-mutant, non-1p/19q-co-deleted tumors which usually have an astrocytic form; and IDH-wild-type tumors which are dominated by glioblastoma (GBM) (WHO grade IV) (3). GBM is the most malignant glioma. In the US, the total annual incidence of glioma is approximately 6 cases per 100,000 people, with half made up by GBM cases and the majority by males (4). Traditional treatments for glioma include surgical resection, radiotherapy, chemotherapy, and bevacizumab therapy, but their efficacies are limited. Owing to the diffuse growth of glioma, surgery cannot remove the entire tumor, thus resulting in a high recurrence rate (5). In 2005, Stupp R’s team found that temozolomide combined with radiotherapy shows significant benefit in the treatment of patients with GBM (6). Friedman HS’s team have confirmed the antitumor activity of bevacizumab (a synthetic drug targeting the monoclonal antibody vascular endothelial growth factor [VEGF]) and bevacizumab in combination with irinotecan (7). However, no evidence is available to support that conventional therapeutic interventions can significantly prolong the survival of patients with tumor relapse (2, 8, 9). In addition, the blood-brain barrier restricts the distribution of drugs, the aggressive growth of tumors fails the local treatment, and the immunosuppression and high toxicity limit the use of traditional drugs, all bringing a poor prognosis to patients with glioma. Therefore, there is an urgent need to develop new therapies.
With the discovery of functional lymphatic vessels within the dural sinuses (10), more evidence suggests the existence of active immune surveillance in the central nervous system (CNS) (9). In contrast, microglia/macrophages (GIMs) function as major immune cells, but they play a negative role in the glioma microenvironment (11). Glioma cells release some immunosuppressive factors, such as transforming growth factor-β (TGF-β), thus allowing for immune escape (12). New data indicate that the immunosuppressive environment of glioma is multifactorial. However, immunotherapy has shown to modify this environment by inhibiting some cytokines or suppressing tumor cells, thus exerting favorable anti-tumor effects. Therefore, immunotherapy may open a new avenue for the treatment of glioma.
Research on glioma immunotherapy is extensive, involving basic and clinical studies. Immunotherapy can be accomplished in many approaches, including vaccine therapy, viral therapy, CAR-T therapy, and immune checkpoint inhibitors (ICIs). Related studies have been well reviewed from various perspectives. However, there have been no analyses encompassing the publication volume, influential countries/regions, institutions, authors, global collaborations, knowledge structure and research hotspots.
Bibliometric analysis is a common method to qualitatively and quantitatively analyze the characteristics of publications, such as countries, institutions, and research collaboration (13–15). Through this tool, the knowledge structure and research hotspots of a certain field can be sorted out, thereafter providing references for future research (16, 17). To our knowledge, bibliometric analysis has never been employed to evaluate the studies about glioma immunotherapy. Based on publications screened out of the Web of Science, this study for the first time summarized the knowledge structure and research hotspots of glioma immunotherapy, using bibliometric analysis combined with other statistical tools of CiteSpace, VOSviewer, R software, and Online Analysis Platform of Literature Metrology.
The Web of Science Core Collection (WoSCC) is one big and comprehensive database for scientific research (18), with more than 12 000 high-quality journals. Also, previous studies have indicated that WoSCC is the most appropriate database for bibliometric analysis (19). According to the literature related to glioma, we determined the common keywords about glioma, and then we preliminarily formulated the search terms related to “glioma”. At the same time, by reading the original articles and reviews of glioma immunotherapy, we have a preliminary understanding of the mainstream immunotherapy methods for glioma, which have been included in the relevant key words of “immunotherapy”. Taking the intersection of the two, we get our search formula. Publications pertaining to glioma immunotherapy were searched from WoSCC with the following queries: #1: TI=(“glioma”) OR AK=(“glioma”) OR TI=(“malignant glioma”) OR AK=(“malignant glioma”) OR TI=(“high grade glioma”) OR AK=(“high grade glioma”) OR TI=(“glioblastoma”) OR AK=(“glioblastoma”); #2: TI=(“immunotherapy*”) OR AK=(“immunotherapy*”) OR TI=(“immune NEAR/2 therap*”) OR AK=(“immune NEAR/2 therap*”) OR TI=(checkpoint inhibitor) OR AK=(checkpoint inhibitor) OR TI=(PD-1) OR AK=(PD-1) OR TI=(PD-L1) OR AK=(PD-L1) OR TI=(nivolumab) OR AK=(nivolumab) OR TI=(pembrolizumab) OR AK=(pembrolizumab) OR TI=(CAR-T) OR AK=(CAR-T) OR TI=(chimeric antigen receptor T-cell therapy) OR AK=(chimeric antigen receptor T-cell therapy) OR TI=(dendritic cell vaccines) OR AK=(dendritic cell vaccines) OR TI=(viral therapy) OR AK=(viral therapy); the ultimate dataset: #1 AND #2. Only English-written articles or reviews published during the period from 1st January 1990 to 27th March 2023 were chosen. A truncation symbol “*” was used and the use of truncation searches improved recall and prevented missing inspection. The articles with research content related to the theme of “glioma immunotherapy” were included by reading the titles, abstracts and keywords of the detected articles. Articles with incomplete research information, book chapters, proceedings papers, early accesses, retrieved publications and duplicate articles were excluded. Eventually, after excluding 22 book chapters, 20 proceedings papers, 4 early accesses, 2 retrieved publications, a total of 1,929 eligible publications were included (Figure 1). All data used in this work were downloaded from a public database; therefore, no ethics approval or informed consent was required. The above processes were carried out under the guidance of glioma experts and bibliometrics experts.
The publications were downloaded and their data were put into Microsoft Office Excel 2019, analyzed and visualized using CiteSpace 6.2.R2, VOSviewer 1.6.18, Online Analysis Platform of Literature Metrology (https://bibliometric.com/) and R 4.2.2 (20–22).
Tables were depicted using Microsoft Office Excel. The Online Analysis Platform of Literature Metrology was used to visualize networks of collaborations between countries/regions and trends in the volume of publications over time. R software was used to visualize the collaborative relationships between countries, authors, and institutions, and to analyze references.
VOSviewer is a widely-used software in bibliometric analysis developed by van Eck and Waltman. It is powerful in building and visualizing bibliometric networks, such as the co-authorship, co-occurrence and co-citation networks of counties, organizations, journals, authors and keywords (23, 24). It was used in the present study to visualize co-authorship between authors, institutions and countries, and the co-occurrence of keywords. Co-authorship analysis is a measure to establish similar relationships among items through the number of coauthored documents, and co-occurrence analysis is to build a network that represents the relationships between items according to the quantity of publications occurring together. Co-citation refers to that two documents were cited simultaneously in the bibliography of the third document (19, 23).
CiteSpace is another information visualization software for bibliometric analysis founded by Professor Chaomei Chen (25). This software can be used to find the key points, especially intellectual pivotal points, or turning points in the research history of a certain field, and predict the research trend through data mining. Additionally, this software is unique in that it can be used to build a dual map of journals, which is generated in the context of 10 000 journals indexed in WoSCC (24). In this study, it was used to (1) analyze the countries, institutions and authors involved in the study; (2) construct dual-map of journals involved; (3) demonstrate reference clustering and references/keywords burst. In CiteSpace, the results are visualized as nodes and lines, and for these nodes, the centrality score is calculated to measure the relative importance of a node in a network. When a node has a centrality score greater than or equal to 0.1, it is usually considered a relatively important hub node (19, 25). In co-cited reference clustering, CiteSpace adopts several metrics to measure the outcome clusters, which include modularity and silhouette. The modularity measures the significance of the divided modules. While the modularity value is greater than 0.3, the clustering structure is usually considered as significant. The silhouette is an indicator used to measure the consistency of a cluster. A cluster is considered reasonable or convincing when its values are greater than 0.5 or 0.7, respectively (19, 26).
From 1st January 1990 to 27th March 2023, a total of 1,929 publications pertaining to glioma immunotherapy were identified, including 1,285 articles and 644 reviews (Figure 1). All of them had been cited for 61,875 times, and 47,878 times after removing self-citations. The mean citation and H-index of a publication were 32.08 times and 109, respectively. Until 2005, just less than 20 articles were published in this field each year (Figure 2). However, in the following decade (except for a few short periods showing a decrease in publication volume), articles related to glioma immunotherapy flourished gradually. Starting from 2006, the annual number of articles published kept increasing, with over 30 articles in each of the 8 years. Starting from 2016, the number of articles in this field grew rapidly. Except for the number of publications in 2019 remained the same as that in the previous year, the number of articles published in all other years had continuously increased, so did the number of citations. The number of articles published in 2023 (as of March) had reached 30, and is predicted to explode in the future.
Figure 2 A trend in the annual publications on glioma immunotherapy. The purple column represents annual publication number, and the blue line represents the development trend of annual citation number.
A total of 62 countries participated in the research of glioma immunotherapy. Table 1 presents the top 10 countries/regions with the largest numbers of articles published, and Figure 3A presents a map of the contributions from different countries. The top country was the United States (n=918, 47.589%), followed by China (n=429, 22.240%), Germany (n=165, 8.554%), Japan (n=104, 5.391%), and Italy (n=101, 5.236%), while the rest countries/regions had released less than 60 publications. Among them, the number of citations of published articles from the United States was 39,991, far exceeding the 7,460 from China, in the second place, and the 7,296 from Germany, in the third place. H-index indicated that each of at least H publications was cited for at least H times (27). A high H-index is parallel to a high academic influence of a country. The United States ranked first with a score of 97, far above other countries. Centrality score of a single country indicates the position of a country in this scientific field. The United States ranked first with a score of 0.64, followed by Germany (centrality=0.23), China (centrality=0.11), France (centrality=0.11); the other countries in the top ten achieved a centrality less than 0.1. As shown in Figure 3B, the US had a relatively stable contribution, which was manifested by its stable number of articles published in this field every year; China, the second contributor, published a large number of articles in the last few years, especially in 2021 and 2022, which could be confirmed by the trend chart of China’s publications over the years (Supplementary Figure 1). It is also worth mentioning that China ranked second in the numbers of publications (429). China published articles in a number almost one-half of that in the US (Table 1), but its total number of citations was just one-fifth of that in the US (7,460), and its average number of citations per article ranked the bottom in the top ten (17.43). Figures 3C, D show the cooperation patterns between different countries/regions. International cooperation was still relatively frequent, mostly occurring between European countries, East Asian countries, and American countries. Among them, cooperation with the US or cooperation between the US and China was the most frequent. In Figure 3D, the colors corresponding to the nodes and timeline represent the time of cooperation between the two. Around 2015, the US was the most active country in this research field, cooperating with many countries. However, around 2020, China became the most active country, and in recent years, most countries have enhanced their cooperation with China.
Figure 3 (A) A geographic distribution map displaying the global contributions to the research of glioma immunotherapy. The darker the color (blue), the more publications published in this country, and gray represents no publication. (B) The annual numbers of publications from the top 10 countries/regions between 1990 and 2023. Each color represents a country. (C) A network map displaying the collaboration between countries/regions. Each country corresponds to an arc area of different colors, and the size of the area represents the number of publications. The line between arcs represents the cooperative relationship between countries. The thickness of the line represents the intensity of cooperation. (D) A co-authorship network between countries/regions based on time by VOSviewer. Each node represents a country/region, and the connection lines between nodes represent a cooperative relationship between the two. The thickness of the line represents the intensity of cooperation. The color of each node corresponds to the color on the timeline and represents the time when cooperation occurs with other nodes.
Table 2 shows the top 10 institutions with the highest total numbers of publications, with Harvard University (n=118) leading in the top five, followed by Duke University (n=82), Harvard Medical School (n=75), Johns Hopkins University (n=69) and University of California San Francisco (n=65). In terms of citations, however, the top five changed to Harvard University (7261), Duke University (4985), UTMD Anderson Cancer Center (4414), University of California San Francisco (4327) and Johns Hopkins University (4001). Harvard University, Duke University, Johns Hopkins University and the University of California San Francisco ranked the top five in terms of either total publications or total citations. Notably, UTMD Anderson Cancer Center, which ranked only 7th in total publications with 55, rose to the third place in the total citations with 4414. In addition, among the top ten institutions, all but University of California Los Angeles (centrality 0.1) had centrality values less than 0.1. The different colors in Figure 4 represent different clusters, and the major institutions are divided into four clusters. The research content in each cluster was closer and more collaborative, which could also be confirmed by the graph drawn by R Software (Supplementary Figure 2). Cooperation among major institutions was relatively regional. For example, US institutions were more likely to cooperate within the US, while major Chinese institutions tended to work together. But this did not mean the lack of international cooperation (Figure 4; Supplementary Figure 2).
Figure 4 The network of collaboration between institutions contributing to the research on glioma immunotherapy by VOSviewer. Each node represents an institution. The size of the node represents the number of publications issued by the institution. Nodes with the same color represent that they belong to the same cluster, indicating that their research are similar.
Of the 1929 articles published in 502 journals, the largest proportion (100, 5.184% of the total) was accepted by JOURNAL OF NEURO-ONCOLOGY (4.506, Q2) (Table 3), followed by FRONTIERS IN IMMUNOLOGY (8.787, Q1), FRONTIERS IN ONCOLOGY (5.738, Q2), NEURO ONCOLOGY (13.029, Q1), and CANCERS (6.575, Q1). Among the top 10 articles, nine journals had an impact factor (IF) of more than 5.0, and eight were in the Q1 JCR division, indicating that most of the articles had been published in journals of high quality and influence.
Table 3 Top 10 journals publishing the most articles about glioma immunotherapy and top 10 journals with the most co-cited publications pertaining to glioma immunotherapy.
While in the analysis of co-cited journals (Table 3), CLINICAL CANCER RESEARCH (13.801, Q1) had the highest number of co-citations, followed by CANCER RESEARCH (13.312, Q1), NEURO ONCOLOGY (13.029, Q1), NEW ENGLAND JOURNAL OF MEDICINE (176.082, Q1) and JOURNAL OF NEURO-ONCOLOGY (4.506, Q2). Among the top 10 journals cited, nine had an IF exceeding 10 and were in the Q1 JCR division. Among them were also famous journals, such as NEW ENGLAND JOURNAL OF MEDICINE (176.082, Q1), NATURE (69.504, Q1), JOURNAL OF CLIMATIC ONCOLOGY (50.739, Q1), NATURE MEDICINE (87.244, Q1), and SCIENCE (63.832, Q1).
A dual-map overlay displays the distribution of journals in different disciplines, and the trajectories between the cited and citing publications (28, 29). The distributions of citing journals (left) and cited journals (right) in each field are shown in Figure 5, in which the connection lines represent the trajectories through which the citing publications cited the cited publications. The horizontal and vertical axes of the oval, respectively, represent the number of authors and publications, the length of which is positively correlated with the number (28). Dual map contained three main pathways, showing that the articles in glioma immunotherapy mainly cited the research outcomes in Molecular Medicine, Biology, and Genetics, and were published in two different fields: Molecular Medicine, Biology, Immunology; and Medicine, Medical, Surgery.
Figure 5 Dual-map overlay displaying trajectories between citing and cited journals. On the left is the citing literature; on the right is the cited literature, and the connection line between the two shows the citation pathway. The horizontal and vertical axes of the oval, respectively, represent the number of authors and publications, the length of which is positively correlated with the number.
The top 10 productive authors in the research on glioma immunotherapy are listed in Figure 6A. Lim M was the most productive, with 54 publications and 3449 citations. From the perspective of H-index, the gaps between the top 10 authors were not significant; but in terms of citation, Weller M left a significant gap in front of others. His articles had been cited for the largest times (3756 times), with each of his articles cited by 110.47 times averagely. In addition, as shown in Figure 6B, the collaborative relationships between authors indicated a lack of collaboration at the global level. The authors were more likely to collaborate in their own cluster, and the collaboration between different clusters remained to be expanded. This phenomenon is also visualized in Supplementary Figure 3 drawn by VOSviewer. The nodes represent the clusters of authors, and there are fewer connections between clusters.
Figure 6 (A) Top 10 authors having published the most articles about glioma immunotherapy. The gray, orange and blue columns represent the H-index of each author, the average number of citations of each author and the number of papers published by each author, respectively. (B) A network analysis of collaborations between authors contributing to the research on glioma immunotherapy. Each node represents an author. The thickness of the line between two nodes represents the intensity of cooperation. Nodes with the same color represent that they belong to the same cluster, indicating that their research are similar.
Table 4 shows the details of the top 10 most cited articles. The most cited article was produced by BROWN CE et al., published in NEW ENGLAND JOURNAL OF MEDICINE in 2016, and titled “Regression of Glioblastoma after Chimeric Antigen Receptor T-Cell Therapy” (30). In a clinical study conducted by their team, they administered CAR-T therapy targeting IL-13Ra2 to a patient with recurrent GBM, and observed a reduction in the size of all lesions and an increase in cytokine and immune cell levels in the cerebrospinal fluid, as well as a significant improvement in the patient’s quality of life.
After normalizing the total number of citations by R software, the highest score (22.42) was achieved by a review from TAN AC et al. published in 2020 in CA-A CANCER JOURNAL FOR CLINICIANS (31). This article reviewed the current mainstream treatment of glioblastoma. For newly diagnosed tumors, standard treatments included concurrent radiotherapy with temozolomide and further adjuvant temozolomide after surgery. For recurrent tumors, surgery, radiotherapy, chemotherapy or systemic therapy with bevacizumab were all alternative treatments. Finally, the author suggested that in order to improve the prognosis of patients with glioblastoma, more biomarker-based treatment methods should be carried out in the future.
The cluster analysis of co-cited references can reveal the knowledge structure in a research field. Clusters were generated by log-likelihood ratio (LLR) algorithm based on the keywords extracted from the co-cited references (32). The top 11 clusters are depicted in Supplementary Figure 4. The number of references (size), silhouette value, mean publication year of cited references (i.e., the main year of this cluster), and label of each cluster obtained by LLR algorithm are listed in Table 5. The overall modularity and weighed mean silhouette of all the clusters were 0.7676 and 0.9185, respectively, suggesting that the clustering results were convincing. In particular, the silhouette value of each cluster was higher than 0.8, indicating that the co-cited references in one cluster were well matched, with a high heterogeneity. Cluster#0 (tumor microenvironment) was the largest one consisting of 187 co-cited references, followed by Cluster#1(pd-1), Cluster#2 (microglia), Cluster#3 (oncolytic), Cluster#4 (dendritic cells), Cluster#5 (chimeric antigen receptor), Cluster#6 (peptide vaccination), Cluster#7 (epidermal growth factor receptor), Cluster#8 (interleukin-2) and Cluster#9 (bevacizumab). A timeline was used to visualize the references in each cluster and the association between clusters (Figure 7A). Cluster#1 had the largest number of periods, while Cluster#8 contained studies that had started earlier. From the perspective of time, Cluster#0, #4, #6-8 all contained early studies with closer connections. From the perspective of label, most of them were early treatment strategies for glioma. However, Cluster#1-3, 5, #9, which demonstrated more and newer references, contained four relatively new therapeutic strategies, namely PD-1, oncolytic therapy (virus therapy), CAR-T therapy and bevacizumab.
Figure 7 (A) Timeline visualization of co-citation clusters contributing to glioma immunotherapy. Each node on the timeline of each cluster represents a reference, and the color of each cluster represents its main time of existence. The size of each node represents the cited times of this reference. (B) Top 25 references with the strongest citation bursts. The red segment represents the begin and end year of the burst duration.
The analysis of co-cited references can outline the trends in a certain research field, and predict the research hotspots in the future (14). Its results become more pronounced if further processed with co-cited reference bursts. Selection criteria of co-cited references using CiteSpace were as follows: the number of states=2; γ [0,1] =1.0; minimum duration=2. The top 25 co-citation bursts are shown in Figure 7B. Among them, the first co-citation burst emerged in 2001, when an article was published by Cancer Research, titled “Vacation of malignant glioma patients with peptide-pulsed dendritic cells elicits systemic cytotoxicity and internal T-cell infiltration” Yu JS (33). It reported the feasibility, safety, and biological activity of a DCs-based vaccine in the treatment of malignant glioma. It can be seen that when immunotherapy for glioma had just slipped into the research hotspot in the early 21st century, DCs were then exploited to design a breakthrough treatment.
It is worth noting that as of 2023, two articles are still in a prominent state, including “Neoadjuvant anti-PD-1 immunotherapy promotes a survival benefit with intratumoral and systemic immune responses in recurrent glioblastoma” authored by Cloughey TFADDIN and published in Nature Medicine in 2019 (34) and “Effect of Nivolumab vs Bevacizumab in Patients With Recurrent Glioblastoma: The CheckMate 143 Phase 3 Randomized Clinical Trial” authored by Reardon DA and published in JAMA Oncology in 2020 (35). Cloughey TF et al. found that neoadjuvant therapy of PD-1 blockers was stronger anti-tumor effects on GBM (34), while Reardon DA et al. confirmed through clinical studies that the safety of PD-1 inhibitor Nivolumab in the treatment of GBM was consistent with those in other tumor types (35). With two reports on PD-1 inhibitors in glioma therapy highlighted during this period, it can be tentatively assumed that PD-1 inhibitors remain in the mainstream of glioma immunotherapy research.
Keywords can also be analyzed to display research hotspots and directions. Keywords that had been cited for more than 30 times were visualized and presented in Figure 8A through VOSviewer. The center node was immunotherapy (total link strength 7724), followed by glioblastoma (total link strength 5596). Figure 8B shows the distribution of keywords over time, and the most recent included “tumor microenvironment”, “prognosis”, “blockade”, “classification”, “pd-l1”, “pembrolizumab” and so on. Figure 8C shows the density distribution of keywords. The three most densely cited keywords were immunology (1273 times), glioblastoma (905 times) and glioma (697 times).
Figure 8 (A) Network visualization of keywords based on VOSviewer. All keywords are divided into 4 clusters of 4 colors: green, red, yellow, and blue. (B) Overlay visualization of keywords based on VOSviewer. The color of each keyword corresponds to the color of the timeline in the bottom right corner, with purple or blue representing relatively early keywords and yellow representing current hot keywords. (C) Density visualization of keywords based on VOSviewer. Keywords with higher weights are brighter. (D) Top 25 keywords in the strongest citation bursts. The red segment represents the begin and end year of the burst duration.
CiteSpace was used to detect keyword bursts (Figure 8D), aiming to discover changes in hotspots over time. Three keywords “brain tumor”, “adoptive immunotherapy” and “activated killer cells” made the earliest burst in 1991. From 1990 to 2023, the keywords with the highest burst intensity were “malignant glioma” (28.86) and “dendritic cells” (28.73). The keywords that had burst out after 2016 included “safety”, “nivolumab”, “mismatch repair”, “double blind” and “tumor microenvironment”, with the latter two remaining in the current hotspot.
To our knowledge, this is the first bibliometric analysis of glioma immunotherapy-related studies to date, which details the knowledge structure and research hotspots in glioma immunotherapy research during the past 33 years.
As of March 27, 2023, a total of 9505 authors from 1988 institutions in 62 countries/regions had published 1929 articles related to glioma immunotherapy in 502 journals. The number of publications per year and the trends in the numbers of publications over years can be analyzed to offer a comprehensive understanding of research in the field. During the 24-year period from 1990 to 2013, the number of studies and reports related to glioma immunotherapy was not large, while after 2015, the publication volume in this field has grown rapidly, which may be due to the discovery of immune amnesty in the CNS. Prior to 2015, it had long been believed that the CNS lacks lymphatic circuits, and Peter Medawar had reported that implantation of foreign grafts into the brain of rodents does not elicit an immune response, while their implantation into the periphery causes immune rejection (36–38). Their experimental data assumed that the CNS is an immune amnesty zone, which may relate to the limits immune-related studies of glioma. However, in 2015, Louveau et al. verified the presence of functional lymphatic vessels within the dural sinus (10). A powerful inflammation can arouse a strong immune response in the brain (2), suggesting that an active immune surveillance may exist in the CNS (9). The knowledge about immune mechanisms in the CNS has been continuously refreshed since 2015, thus igniting the hope of using immunotherapy to treat glioma. Therefore, related research has abounded as never before.
In the analysis of countries/regions, we found that the top countries publishing the most articles were the US, China, Germany, Japan and Italy, led by the US and China. This may hint at a significant connection between research achievement and the economy of a country, which is consistent with opinions previously reported (39). We also know that all these top five countries have constructed a powerful health care system, which may ensure their academic achievements (40). All the data in the present study suggest that the US is a leader in this research field. The publication volume from China was one-half of that from the US, but only one-fifth in terms of total citations, which indicates that the research in China has a big yield, but its quality needs to be improved. As shown by the timeline, more countries chose to cooperate with the U.S. in 2010, while after that, China became the magnet in this field, which indicates the rising influence of Chinese researchers.
Harvard University, Duke University, Johns Hopkins University and University of California San Francisco ranked high in terms of both total publications, total citations, as well as H-index values, proving that their strength in this research field. All these universities are located in the US, which indirectly confirms the absolute centrality of American research in this field. In addition, UTMD Anderson Cancer Center launched fewer publications, but received the highest number of citations, indicating the high quality and recognition of its research. From the centrality value, University of California Los Angeles demonstrated an increasing profile in the field; meanwhile, the centrality values of the top ten institutions were low, which may be related to the fact that currently no institutions can take a permanent core position in this field. Moreover, domestic cooperation was more popular than international cooperation, suggesting that the latter should also be encouraged.
We further found that the publications involved a wide range of disciplines, as shown by the results presented in the dual-map. IF and JCR division are two important indicators for the quality of a journal. In this field of research, most of the top 10 journals with the largest volume had an IF between 5-15 and a JCR Q1 division. And from the co-cited journals, the majority of journals had an IF over 10 and a JCR Q1 division, and some were famous worldwide. It may suggest that glioma immunotherapy still stands in the research frontier, and is being transformed with theoretical and technological advances. Moreover, despite their fruitful results, there is still room for improvement in related research.
Analysis of journals can guide the study design, journal selection, and article submission of researchers in a given field (17, 41). In terms of publication volume, the top three journals were JOURNAL OF NEURO-ONCOLOGY (4.506, Q2), FRONTIERS IN IMMUNOLOGY (8.787, Q1) and FRONTIERS IN ONCOLOGY (5.738, Q2). Among them, JOURNAL OF NEURO-ONCOLOGY is a journal specialized in neuro-oncology, while FRONTIERS IN IMMUNOLOGY and FRONTIERS IN ONCOLOGY are comprehensive journals in the fields of immunology and oncology, respectively. From the results of the dual-map analysis, publications in the fields of Molecular Medicine, Biology, Genetics were frequently cited by publications in the fields of Molecular Medicine, Biology, Immunology, as well as in the fields of Medicine, Medical, Surgery neighborhoods, indicating the focus paid to either basic or clinical research.
A total of 9505 authors worldwide had been involved in research on glioma immunotherapy. Collaboration mainly occurred between authors in a cluster, and seldom between clusters, which has a correlation with the insufficient international cooperation and communications, suggesting that researchers should strengthen international collaboration. In the number of publications and H-index, the gaps between the top ten authors were not wide, but in total citations and average citations, we were surprised to find that the others lagged far behind Weller M, which may indicate that he has a highest reputation among the top ten researchers. Weller M et al. proposed a reason for the failure of adoptive cellular immunotherapy in malignant glioma: immunosuppressive factors such as transforming growth factor-β (TGF-β) released from glioma cells inhibit T-cell proliferation and thus suppress immune responses. However, they found that in vitro killing mediated by Fas/APO-1 was not inhibited by TGF- β. The resistance of human glioma cells to Fas/APO-1 antibody-mediated apoptosis was mainly associated with a low level of Fas/APO-1 expression, but cytokines IFN-γ and TNF-α enhanced the sensitivity to Fas/APO-1-mediated killing. Therefore, they concluded that a multimodal immunotherapy targeting cytokines and Fas/APO-1 may be designed to fight against glioma (42, 43). In addition, they believed that glioma can be treated by antagonizing TGF-β (44).
The combination of reference clustering, timeline, and bursts better pictured the evolution of glioma immunotherapy research.
In the early period, supported by basic research, vaccine therapies were spawned, including that based on dendritic cells (DCs). DCs are specialized antigen presenting cells that activate T cells. Both Akasaki Y and Kikuchi T’s teams found that fusion cells (FCs) from DCs with glioma cells can effectively activate anti-tumor immune responses, thus imparting lethality against glioma cells in the lateral abdomen or brain of mice (45, 46). Yamanaka R et al. confirmed that in patients treated with DCs vaccine, no serious adverse effects were observed (47). Also, their patients who had received DCs-based vaccine therapy showed a longer survival than those receiving conventional treatment (48). However, one limitation of this vaccine therapy is that it is favorable for patients with resectable tumors, which restricts its wider application (49).
Another form of vaccine is a peptide prepared by targeting tumor antigens or tumor-related antigens to induce immune response in tumor tissues. Widely used is epidermal growth factor receptor variant III (EGFRvIII), a tumor-specific mutant that mediates tumor proliferation and migration. The high expression of EGFRvIII in tumors, such as glioma, and its silencing in normal tissues makes it an ideal target for immunotherapy (50). However, since EGFRvIII is only expressed in 25-30% of GBMs, this vaccine therapy cannot be taken to counter the resting tumors (49, 51). Furthermore, the expression of EGFRvIII in tumor progression is instable (2). Van den Bent MJ found that EGFRvIII loses its specific expression during amplification in half of the GBMs originally expressing EGFRvIII (52). This instability has also been reported in a study by Felsberg J et al (53).
Viral therapy works by infesting tumor cells to produce cellular damage. In recent years, viruses have been found able to trigger an anti-tumor immune response. Viral therapy can also shift the tumor microenvironment from immunosuppressive to pro-inflammatory (54). Therefore, it is also considered as a type of immunotherapy. Many replication-competent viruses are now used in viral therapy, such as poliovirus, retrovirus, adenovirus, and herpes simplex virus (HSV) (2). Polioviruses, such as polio-rhinovirus chimera (PVSRIPO), act by binding to receptor CD155, which is widely expressed in solid tumors and their microenvironments. Clinical studies demonstrated higher survival rates at 24 and 36 months in patients receiving PVSRIPO immunotherapy than in historical controls, with no neurotoxicity-related adverse events (55). A typical tumor lysis therapy using retroviruses is Toca-511 (a non-cleavage retrovirus expressing cytosine deaminase), which is specific for cells with division potential, like tumor cells that cannot respond to innate immunity and interferons (56). A clinical study by the Cloughesy TF team showed that Toca-511 triggers a durable complete response, and suggested a positive correlation between a sustained response and an overall survival (57).
In the last decade, Chimeric antigen receptor T cell therapy (CAR-T cell therapy) and Immune-checkpoint inhibitors (ICIs) have been prioritized in designing immunotherapies. CAR-T cells activate anti-tumor immunity by expressing chimeric antigen receptor (CAR) on the surface of autologous or allogeneic T cells, targeting tumor-associated antigens and binding, activating T cells and releasing relevant cytokines without relying on MHC molecules for antigen presentation and co-stimulatory molecules (58). Currently, the intensely studied target antigens in CAR-T cell therapy for GBM include IL-13Ra2, EGFRvIII and Her2 (49). In a clinical study conducted by Brown CE’s team, they administered CAR-T therapy targeting IL-13Ra2 to a patient with recurrent GBM, and observed a size reduction in all lesions and an increase in cytokine and immune cell levels in the cerebrospinal fluid, as well as a significant improvement in the patient’s quality of life (30). O’Rourke DM et al., on the other hand, confirmed the feasibility and safety of CAR-T cell therapy targeting EGFRvIII, without non-tumor toxic reactions or cytokine release syndrome in patients (59). However, after treatment, they found an upregulation of immunosuppressive molecules in the tumor environment and an increase in regulatory T cells (Treg), suggesting that overlooking the effects of CAR-T therapy, tumor tissues can still restore the immunosuppressive environment through adaptive regulation on realize immune escape (2, 59), which limits the application of CAR-T cell therapy. Its efficacy may be impaired by the stress metabolic environment of the tumor, such as hypoxia and nutrient deprivation (58). In conclusion, single CAR-T cell therapies cannot eradicate tumors, and should be combined with anti-TGF-β molecules, anti-IL-6 antibodies and immune checkpoint blocking antibodies (such as PD-1/PD-L1 inhibitors), or IDO inhibitors, or macrophage colony-stimulating factor 1 receptor (CSF1R) inhibitors, or prior conditioning chemotherapy (fludarabine and cyclophosphamide) (2, 58, 60).
Through the combination of reference clustering, timeline, and bursts, it is not difficult to find that the frequency of PD-1 is high. According to the reference clustering, PD-1 represents one of the largest and most important clusters. PD-1 is densely studied in latest studies, and the references in these studies are frequently cited. Additionally, the latest reference bursts and most of the top 10 co-cited references are related to PD-1 research. In conclusion, immune-checkpoint inhibitors (ICIs) represented by PD-1, are in current research hotspot and the main component in the immunotherapy for glioma.
In recent years, ICIs, especially those targeting CTLA-4 and PD-1/PD-1, have become the cornerstones in major cancer therapies (61, 62). CTLA-4 inhibits the pathway of T cells by binding to ligand CD80 or CD86 expressed in antigen presenting cells (APCs) (63). The combination of PD-1 with its ligand PD-L1 inhibits the activation, migration, and cell-killing ability of T cells (63, 64). ICIs counteract the inhibitory effect of tumor cells on T-cell activation by blocking the binding of co-inhibitory receptors to their ligands, thus enhancing anti-tumor effects (9). Currently, ICIs, such as ipilimumab, nivolumab and pembrolizumab, have been used in the treatment of solid tumors, including melanoma, non-small cell lung cancer, breast cancer, lymphoma, head and neck cancer, bladder cancer and other malignant tumors (65–67). For glioma, ICIs either alone or in combination with multiple drugs or other therapies have shown encouraging results in preclinical trials. In a study by Fecci PE et al, CTLA-4 blockers can achieve a long-term survival in 80% of treated mice, without causing experimental metaplastic encephalomyelitis (68). Reardon DA et al. found that the combination of anti-CTLA-4 and anti-PD-1 can cure 75% of mice (69). Wainwright DA et al. reported that triple blockade of IDO, CTLA-4 and PD-L1 prolongated the survival of all mice in the experiment (70). And PD-1 blockers combined with local radiotherapy can realize a long-term survival in mice with brain tumors in situ (71). In clinical studies, ICIs have shown evident therapeutic efficacy. A CheckMate 143 Phase III randomized clinical trial compared the efficacy of nivolumab and bevacizumab in the treatment of recurrent GBM. Nivolumab enabled a median survival of 9.8 months, and bevacizumab 10.0 months. The safety of nivolumab in treating GBM patients is consistent with that in other tumor types (35). However, the efficacy of ICIs is not ideal. Reiss SN et al. found in a retrospective study that in treating rGBM with pembrolizumab, PFS was only prolonged in a small fraction of patients, with an OS of 4 months (72). Multiple reasons may explain this unsatisfactory clinical efficacy. For example, compared with other tumors, GBM has a lower mutation rate and a low level of T cell infiltration, which reduces the efficacy of ICIs (73). Also, the blood-brain barrier can discount the efficacy of ICIs (2, 9).
Efforts have been released to improve the efficacy of single ICIs, including combination of ICIs with radiotherapy, chemotherapy, as well as neoadjuvant therapy. Schalper KA et al. reported that neoadjuvant nivolumab increases the expression of chemokine transcripts, immune cell infiltration, and TCR clonal diversity in tumor-infiltrating T lymphocytes (74). Cloughesy TF, on the other hand, reported that if treated with neoadjuvant pembrolizumab, the patients who received continuous adjuvant chemotherapy after surgery had a longer overall survival, compared to those who received adjuvant PD-1 blockade therapy after surgery (34). It is foreseeable that as ICI research progresses, more targets will be raked out for ICIs and more types of ICIs will be synthesized in the future. Then how to combine these ICIs, how to combine ICIs with conventional treatments (radiotherapy, chemotherapy, etc.), or how to standardize ICI treatment protocols will be the key to improving the efficacy of ICIs. In the future, ICI research will focus on the search for targets, as well the optimization of therapeutic strategies.
The analysis of keywords can tease out the hotspots in the research field, while keyword bursts can demonstrate how research hotspots have changed over time. In the early 1990s, “malignant glioma” and “dendritic cells” rushed into the hotspot, and dendritic cells were then used to design vaccines for glioma treatment, because of their antigen-presenting cell characteristics (46). According to VOSviewer’s analysis, popular keywords that are closer to the present time include “tumor microenvironment”, “prognosis”, “blockade”, “classification”, “pd-l1” and “pembrolizumab”. CiteSpace showed that keywords that had burst after 2016 included “safety”, “nivolumab”, “mismatch repair”, “double blind”, and “tumor microenvironment”, with “double blind” and “tumor microenvironment” still in the swim. Based on the outcome of the two software, it is not difficult to find many keywords that are related to the treatment of PD-1, including “PD-L1”, “pembrolizumab” and “nivolumab”, which again suggests that ICIs such as PD-1 is the mainstream immunotherapy for glioma. “Safety” can suggest that, with multiple immunotherapies showing clinical responses for glioma, the survival and ensure the safety of patients should be well concerned, which is also in line with the fundamental principles of drug development. Meanwhile, “tumor microenvironment” was mentioned by both software, reflecting the importance of tumor microenvironment for glioma treatment. Therefore, current immunotherapy research still aims to suppress the immunosuppressive cells by various methods, and to “blockade” the immune escape of the tumor by various small molecules, such as ICI or antibodies. In short, ICIs such as PD-1 are in the current hotspot of glioma immunotherapy. Through ICIs, the immune escape of glioma may be hindered by modulating the tumor microenvironment, thus allowing various therapies to exert their anti-tumor effects. Therefore, the hotspot of future research on glioma immunotherapy will still be ICIs represented by PD-1. Based on the limited number of ICI targets currently available, it is necessary to explore new and more ICI targets and apply them for clinical treatments. In addition, due to the poor efficacy of a single ICI treatment, it is urgent to conduct research on the combination therapy of multiple ICIs and the combination therapy of ICIs and other therapies to increase the anti-tumor effect. In the future, the research of glioma immunotherapy will no longer be limited to the exploration of certain therapeutic methods. It is advisable for researchers to focus on the comprehensive therapy based on ICIs, with the aim of achieving anti-tumor effect by comprehensively inhibiting the tumor microenvironment, so as to improve the prognosis of patients.
There are still several limitations to this study. First of all, all publications pertaining to glioma immunotherapy were searched out of the WoSCC. Although it is one of the most reliable online databases (75), some publications may have been missed. Secondly, only publications in English were collected, which means several potential studies in other languages could be missed. Thirdly, since our study is based on published literature, which might introduce publication bias. Accordingly, we expanded the search scope as much as possible to prevent missing literature in order to reduce publication bias. Fourthly, there may have differences in keywords bursts, clustering analysis of co-citations and institutions contributing to glioma immunotherapy due to the limitations of CiteSpace, VOSviewer and other software.
In summary, this article reviewed the global trends in the research field of glioma immunotherapy. The results showed that research related to glioma immunotherapy had been widely conducted worldwide and reaped heavy fruits, with the US being the biggest contributor and a powerful leader, and China becoming increasingly active and influential in this area. Emerging research trends have been identified by analyzing keyword bursts and clustering of co-citations. Several immunotherapies are available for glioma, including vaccine therapy, viral lysis therapy, CAR-T therapy, and ICIs. Search for ICI targets and comprehensive ICI treatments has entered and will stay in the research hotspot. This study offers a new insight into the knowledge structure and research prospects of glioma immunotherapy via a qualitative, quantitative and visual method.
The raw data supporting the conclusions of this article will be made available by the authors, without undue reservation.
TZ, YQ, and TC: writing-review and editing. YY, YS, and YW: writing-original draft. YX, YZZ, YYZ and MZ: data curation. All authors contributed to the article and approved the submitted version.
This work was supported by the National Natural Science Foundation of China (no. 81971153, 81200902).
The authors declare that the research was conducted in the absence of any commercial or financial relationships that could be construed as a potential conflict of interest.
All claims expressed in this article are solely those of the authors and do not necessarily represent those of their affiliated organizations, or those of the publisher, the editors and the reviewers. Any product that may be evaluated in this article, or claim that may be made by its manufacturer, is not guaranteed or endorsed by the publisher.
The Supplementary Material for this article can be found online at: https://www.frontiersin.org/articles/10.3389/fonc.2023.1229905/full#supplementary-material
1. Xu S, Tang L, Li X, Fan F, Liu Z. Immunotherapy for glioma: Current management and future application. Cancer r (2020) 476:1–2. doi: 10.1016/j.canlet.2020.02.002
2. Lim M, Xia Y, Bettegowda C, Weller M. Current state of immunotherapy for glioblastoma. Nat Rev Clin Oncol (2018) 15(7):422–42. doi: 10.1038/s41571-018-0003-5
3. Louis DN, Perry A, Reifenberger G, von Deimling A, Figarella-Branger D, Cavenee WK, et al. The 2016 world health organization classification of tumors of the central nervous system: a summary. Acta Neuropathol (2016) 131(6):803–20. doi: 10.1007/s00401-016-1545-1
4. Ostrom QT, Gittleman H, Truitt G, Boscia A, Kruchko C, Barnholtz-Sloan JS. CBTRUS statistical report: primary brain and other central nervous system tumors diagnosed in the United States in 2011-2015. Neuro Oncol (2018) 20(suppl_4):iv1–iv86. doi: 10.1093/neuonc/noy131
5. McGirt MJ, Chaichana KL, Attenello FJ, Weingart JD, Than K, Burger PC, et al. Extent of surgical resection is independently associated with survival in patients with hemispheric infiltrating low-grade gliomas. Neurosurgery (2008) 63(4):700–7707–8. doi: 10.1227/01.NEU.0000325729.41085.73
6. Stupp R, Mason WP, van den Bent MJ, Weller M, Fisher B, Taphoorn MJ, et al. Radiotherapy plus concomitant and adjuvant temozolomide for glioblastoma. N Engl J Med (2005) 352(10):987–96. doi: 10.1056/NEJMoa043330
7. Friedman HS, Prados MD, Wen PY, Mikkelsen T, Schiff D, Abrey LE, et al. Bevacizumab alone and in combination with irinotecan in recurrent glioblastoma. J Clin Oncol (2009) 27(28):4733–40. doi: 10.1200/JCO.2008.19.8721
8. Gallego O. Nonsurgical treatment of recurrent glioblastoma. Curr Oncol (2015) 22(4):e273–281. doi: 10.3747/co.22.2436
9. Yang M, Oh IY, Mahanty A, Jin WL, Yoo JS. Immunotherapy for glioblastoma: current state, challenges, and future perspectives. Cancers (Basel) (2020) 12(9). doi: 10.3390/cancers12092334
10. Louveau A, Smirnov I, Keyes TJ, Eccles JD, Rouhani SJ, Peske JD, et al. Structural and functional features of central nervous system lymphatic vessels. Nature (2015) 523(7560):337–41. doi: 10.1038/nature14432
11. Hussain SF, Yang D, Suki D, Aldape K, Grimm E, Heimberger AB. The role of human glioma-infiltrating microglia/macrophages in mediating antitumor immune responses. Neuro Oncol (2006) 8(3):261–79. doi: 10.1215/15228517-2006-008
12. Weller M, Fontana A. The failure of current immunotherapy for Malignant glioma. Tumor-derived TGF-beta, T-cell apoptosis, and the immune privilege of the brain. Brain Res Brain Res Rev (1995) 21(2):128–51. doi: 10.1016/0165-0173(95)00010-0
13. Moed HF. New developments in the use of citation analysis in research evaluation. Arch Immunol Ther Exp (Warsz) (2009) 57(1):13–8. doi: 10.1007/s00005-009-0001-5
14. Qin YF, Ren SH, Shao B, Qin H, Wang HD, Li GM, et al. The intellectual base and research fronts of IL-37: A bibliometric review of the literature from WoSCC. Front Immunol (2022) 13:931783. doi: 10.3389/fimmu.2022.931783
15. Yeung AWK, Atanasov AG, Sheridan H, Klager E, Eibensteiner F, Volkl-Kernsock S, et al. Open innovation in medical and pharmaceutical research: A literature landscape analysis. Front Pharmacol (2020) 11:587526. doi: 10.3389/fphar.2020.587526
16. Gao Y, Wang Y, Zhai X, He Y, Chen R, Zhou J, et al. Publication trends of research on diabetes mellitus and T cells (1997-2016): A 20-year bibliometric study. PloS One (2017) 12(9):e0184869. doi: 10.1371/journal.pone.0184869
17. Su Y, Ruan Z, Wang R, Hao S, Tang Y, Huang X, et al. Knowledge mapping of targeted immunotherapy for myasthenia gravis from 1998 to 2022: A bibliometric analysis. Front Immunol (2022) 13:998217. doi: 10.3389/fimmu.2022.998217
18. Ma C, Su H, Li H. Global research trends on prostate diseases and erectile dysfunction: A bibliometric and visualized study. Front Oncol (2020) 10:627891. doi: 10.3389/fonc.2020.627891
19. Wu H, Cheng K, Tong L, Wang Y, Yang W, Sun Z. Knowledge structure and emerging trends on osteonecrosis of the femoral head: a bibliometric and visualized study. J Orthop Surg Res (2022) 17(1):194. doi: 10.1186/s13018-022-03068-7
20. Liang YD, Li Y, Zhao J, Wang XY, Zhu HZ, Chen XH. Study of acupuncture for low back pain in recent 20 years: a bibliometric analysis via CiteSpace. J Pain Res (2017) 10:951–64. doi: 10.2147/JPR.S132808
21. Zhao J, Yu G, Cai M, Lei X, Yang Y, Wang Q, et al. Bibliometric analysis of global scientific activity on umbilical cord mesenchymal stem cells: a swiftly expanding and shifting focus. Stem Cell Res Ther (2018) 9(1):32. doi: 10.1186/s13287-018-0785-5
22. Miao L, Zhang J, Zhang Z, Wang S, Tang F, Teng M, et al. A bibliometric and knowledge-map analysis of CAR-T cells from 2009 to 2021. Front Immunol (2022) 13:840956. doi: 10.3389/fimmu.2022.840956
23. van Eck NJ, Waltman L. Software survey: VOSviewer, a computer program for bibliometric mapping. Scientometrics (2010) 84(2):523–38. doi: 10.1007/s11192-009-0146-3
24. Cheng K, Zhang H, Guo Q, Zhai P, Zhou Y, Yang W, et al. Emerging trends and research foci of oncolytic virotherapy for central nervous system tumors: A bibliometric study. Front Immunol (2022) 13:975695. doi: 10.3389/fimmu.2022.975695
25. Wu Z, Cheng K, Shen Z, Lu Y, Wang H, Wang G, et al. Mapping knowledge landscapes and emerging trends of sonodynamic therapy: A bibliometric and visualized study. Front Pharmacol (2022) 13:1048211. doi: 10.3389/fphar.2022.1048211
26. Sabe M, Pillinger T, Kaiser S, Chen C, Taipale H, Tanskanen A, et al. Half a century of research on antipsychotics and schizophrenia: A scientometric study of hotspots, nodes, bursts, and trends. Neurosci Biobehav Rev (2022) 136:104608. doi: 10.1016/j.neubiorev.2022.104608
27. Bertran K, Cortey M, Diaz I. The use of H-index to assess research priorities in poultry diseases. Poult Sci (2020) 99(12):6503–12. doi: 10.1016/j.psj.2020.09.017
28. Chen CM, Leydesdorff L. Patterns of connections and movements in dual-map overlays: A new method of publication portfolio analysis. J Assoc Inf Sci Tech (2014) 65(2):334–51. doi: 10.1002/asi.22968
29. Zhang J, Song L, Xu L, Fan Y, Wang T, Tian W, et al. Knowledge domain and emerging trends in ferroptosis research: A bibliometric and knowledge-map analysis. Front Oncol (2021) 11:686726. doi: 10.3389/fonc.2021.686726
30. Brown CE, Alizadeh D, Starr R, Weng L, Wagner JR, Naranjo A, et al. Regression of glioblastoma after chimeric antigen receptor T-cell therapy. N Engl J Med (2016) 375(26):2561–9. doi: 10.1056/NEJMoa1610497
31. Tan AC, Ashley DM, Lopez GY, Malinzak M, Friedman HS, Khasraw M. Management of glioblastoma: State of the art and future directions. CA Cancer J Clin (2020) 70(4):299–312. doi: 10.3322/caac.21613
32. Zhu X, Hu J, Deng S, Tan Y, Qiu C, Zhang M, et al. Comprehensive bibliometric analysis of the kynurenine pathway in mood disorders: focus on gut microbiota research. Front Pharmacol (2021) 12:687757. doi: 10.3389/fphar.2021.687757
33. Yu JS, Wheeler CJ, Zeltzer PM, Ying H, Finger DN, Lee PK, et al. Vaccination of Malignant glioma patients with peptide-pulsed dendritic cells elicits systemic cytotoxicity and intracranial T-cell infiltration. Cancer Res (2001) 61(3):842–7.
34. Cloughesy TF, Mochizuki AY, Orpilla JR, Hugo W, Lee AH, Davidson TB, et al. Neoadjuvant anti-PD-1 immunotherapy promotes a survival benefit with intratumoral and systemic immune responses in recurrent glioblastoma. Nat Med (2019) 25(3):477–86. doi: 10.1038/s41591-018-0337-7
35. Reardon DA, Brandes AA, Omuro A, Mulholland P, Lim M, Wick A, et al. Effect of nivolumab vs bevacizumab in patients with recurrent glioblastoma: the checkMate 143 phase 3 randomized clinical trial. JAMA Oncol (2020) 6(7):1003–10. doi: 10.1001/jamaoncol.2020.1024
36. Billingham RE, Brent L, Medawar PB. Actively acquired tolerance of foreign cells. Nature (1953) 172(4379):603–6. doi: 10.1038/172603a0
37. Billingham RE, Brent L, Medawar PB, Sparrow EM. Quantitative studies on tissue transplantation immunity. I. The survival times of skin homografts exchanged between members of different inbred strains of mice. Proc R Soc Lond B Biol Sci (1954) 143(910):43–58. doi: 10.1098/rspb.1954.0053
38. Medawar PB. Immunity to homologous grafted skin; the fate of skin homografts transplanted to the brain, to subcutaneous tissue, and to the anterior chamber of the eye. Br J Exp Pathol (1948) 29(1):58–69.
39. Sun HL, Bai W, Li XH, Huang H, Cui XL, Cheung T, et al. Schizophrenia and inflammation research: A bibliometric analysis. Front Immunol (2022) 13:907851. doi: 10.3389/fimmu.2022.907851
40. Wu H, Cheng K, Guo Q, Yang W, Tong L, Wang Y, et al. Mapping knowledge structure and themes trends of osteoporosis in rheumatoid arthritis: A bibliometric analysis. Front Med (Lausanne) (2021) 8:787228. doi: 10.3389/fmed.2021.787228
41. Wu H, Li Y, Tong L, Wang Y, Sun Z. Worldwide research tendency and hotspots on hip fracture: a 20-year bibliometric analysis. Arch Osteoporos (2021) 16(1):73. doi: 10.1007/s11657-021-00929-2
42. Weller M, Frei K, Groscurth P, Krammer PH, Yonekawa Y, Fontana A. Anti-Fas/APO-1 antibody-mediated apoptosis of cultured human glioma cells. Induction and modulation of sensitivity by cytokines. J Clin Invest (1994) 94(3):954–64. doi: 10.1172/JCI117462
43. Weller M, Malipiero U, Aguzzi A, Reed JC, Fontana A. Protooncogene bcl-2 gene transfer abrogates Fas/APO-1 antibody-mediated apoptosis of human Malignant glioma cells and confers resistance to chemotherapeutic drugs and therapeutic irradiation. J Clin Invest (1995) 95(6):2633–43. doi: 10.1172/JCI117965
44. Wick W, Naumann U, Weller M. Transforming growth factor-beta: a molecular target for the future therapy of glioblastoma. Curr Pharm Des (2006) 12(3):341–9. doi: 10.2174/138161206775201901
45. Akasaki Y, Kikuchi T, Homma S, Abe T, Kofe D, Ohno T. Antitumor effect of immunizations with fusions of dendritic and glioma cells in a mouse brain tumor model. J Immunother (2001) 24(2):106–13. doi: 10.1097/00002371-200103000-00004
46. Kikuchi T, Akasaki Y, Irie M, Homma S, Abe T, Ohno T. Results of a phase I clinical trial of vaccination of glioma patients with fusions of dendritic and glioma cells. Cancer Immunol Immunother (2001) 50(7):337–44. doi: 10.1007/s002620100205
47. Yamanaka R, Abe T, Yajima N, Tsuchiya N, Homma J, Kobayashi T, et al. Vaccination of recurrent glioma patients with tumour lysate-pulsed dendritic cells elicits immune responses: results of a clinical phase I/II trial. Br J Cancer (2003) 89(7):1172–9. doi: 10.1038/sj.bjc.6601268
48. Yu JS, Liu G, Ying H, Yong WH, Black KL, Wheeler CJ. Vaccination with tumor lysate-pulsed dendritic cells elicits antigen-specific, cytotoxic T-cells in patients with Malignant glioma. Cancer Res (2004) 64(14):4973–9. doi: 10.1158/0008-5472.CAN-03-3505
49. McGranahan T, Therkelsen KE, Ahmad S, Nagpal S. Current state of immunotherapy for treatment of glioblastoma. Curr Treat Options Oncol (2019) 20(3):24. doi: 10.1007/s11864-019-0619-4
50. Sampson JH, Archer GE, Mitchell DA, Heimberger AB, Bigner DD. Tumor-specific immunotherapy targeting the EGFRvIII mutation in patients with Malignant glioma. Semin Immunol (2008) 20(5):267–75. doi: 10.1016/j.smim.2008.04.001
51. Weller M, Kaulich K, Hentschel B, Felsberg J, Gramatzki D, Pietsch T, et al. Assessment and prognostic significance of the epidermal growth factor receptor vIII mutation in glioblastoma patients treated with concurrent and adjuvant temozolomide radiochemotherapy. Int J Cancer (2014) 134(10):2437–47. doi: 10.1002/ijc.28576
52. van den Bent MJ, Gao Y, Kerkhof M, Kros JM, Gorlia T, van Zwieten K, et al. Changes in the EGFR amplification and EGFRvIII expression between paired primary and recurrent glioblastomas. Neuro Oncol (2015) 17(7):935–41. doi: 10.1093/neuonc/nov013
53. Felsberg J, Hentschel B, Kaulich K, Gramatzki D, Zacher A, Malzkorn B, et al. Epidermal growth factor receptor variant III (EGFRvIII) positivity in EGFR-amplified glioblastomas: prognostic role and comparison between primary and recurrent tumors. Clin Cancer Res (2017) 23(22):6846–55. doi: 10.1158/1078-0432.CCR-17-0890
54. Peruzzi P, Chiocca EA. Viruses in cancer therapy - from benchwarmers to quarterbacks. Nat Rev Clin Oncol (2018) 15(11):657–8. doi: 10.1038/s41571-018-0077-0
55. Desjardins A, Gromeier M, Herndon JE 2nd, Diago O, Burnett R, Inagaki A, et al. Recurrent glioblastoma treated with recombinant poliovirus. N Engl J Med (2018) 379(2):150–61. doi: 10.1056/NEJMoa1716435
56. Perez OD, Logg CR, Hiraoka K, Beaubier N, Bolognesi DP, Friedman AH, et al. Design and selection of Toca 511 for clinical use: modified retroviral replicating vector with improved stability and gene expression. Mol Ther (2012) 20(9):1689–98. doi: 10.1038/mt.2012.83
57. Cloughesy TF, Landolfi J, Vogelbaum MA, Ostertag D, Elder JB, Bloomfield S, et al. Durable complete responses in some recurrent high-grade glioma patients treated with Toca 511 + Toca FC. Neuro Oncol (2018) 20(10):1383–92. doi: 10.1093/neuonc/noy075
58. Bagley SJ, Desai AS, Linette GP, June CH, O’Rourke DM. CAR T-cell therapy for glioblastoma: recent clinical advances and future challenges. Neuro Oncol (2018) 20(11):1429–38. doi: 10.1093/neuonc/noy032
59. O’Rourke DM, Nasrallah MP, Desai A, Melenhorst JJ, Mansfield K, Morrissette JJD, et al. A single dose of peripherally infused EGFRvIII-directed CAR T cells mediates antigen loss and induces adaptive resistance in patients with recurrent glioblastoma. Sci Transl Med (2017) 9(399). doi: 10.1126/scitranslmed.aaa0984
60. Dai H, Wang Y, Lu X, Han W. Chimeric antigen receptors modified T-cells for cancer therapy. J Natl Cancer Inst (2016) 108(7). doi: 10.1093/jnci/djv439
61. Tarhini AA, Iqbal F. CTLA-4 blockade: therapeutic potential in cancer treatments. Onco Targets Ther (2010) 3:15–25. doi: 10.2147/OTT.S4833
62. Luke JJ, Ott PA. PD-1 pathway inhibitors: the next generation of immunotherapy for advanced melanoma. Oncotarget (2015) 6(6):3479–92. doi: 10.18632/oncotarget.2980
63. Wei SC, Duffy CR, Allison JP. Fundamental mechanisms of immune checkpoint blockade therapy. Cancer Discovery (2018) 8(9):1069–86. doi: 10.1158/2159-8290.CD-18-0367
64. Kleffel S, Posch C, Barthel SR, Mueller H, Schlapbach C, Guenova E, et al. Melanoma cell-intrinsic PD-1 receptor functions promote tumor growth. Cell (2015) 162(6):1242–56. doi: 10.1016/j.cell.2015.08.052
65. Darvin P, Toor SM, Sasidharan Nair V, Elkord E. Immune checkpoint inhibitors: recent progress and potential biomarkers. Exp Mol Med (2018) 50(12):1–11. doi: 10.1038/s12276-018-0191-1
66. Necchi A, Anichini A, Raggi D, Briganti A, Massa S, Luciano R, et al. Pembrolizumab as neoadjuvant therapy before radical cystectomy in patients with muscle-invasive urothelial bladder carcinoma (PURE-01): an open-label, single-arm, phase II study. J Clin Oncol (2018) 36(34):3353–60. doi: 10.1200/JCO.18.01148
67. Forde PM, Chaft JE, Smith KN, Anagnostou V, Cottrell TR, Hellmann MD, et al. Neoadjuvant PD-1 blockade in resectable lung cancer. N Engl J Med (2018) 378(21):1976–86. doi: 10.1056/NEJMoa1716078
68. Fecci PE, Ochiai H, Mitchell DA, Grossi PM, Sweeney AE, Archer GE, et al. Systemic CTLA-4 blockade ameliorates glioma-induced changes to the CD4+ T cell compartment without affecting regulatory T-cell function. Clin Cancer Res (2007) 13(7):2158–67. doi: 10.1158/1078-0432.CCR-06-2070
69. Reardon DA, Gokhale PC, Klein SR, Ligon KL, Rodig SJ, Ramkissoon SH, et al. Glioblastoma eradication following immune checkpoint blockade in an orthotopic, immunocompetent model. Cancer Immunol Res (2016) 4(2):124–35. doi: 10.1158/2326-6066.CIR-15-0151
70. Wainwright DA, Chang AL, Dey M, Balyasnikova IV, Kim CK, Tobias A, et al. Durable therapeutic efficacy utilizing combinatorial blockade against IDO, CTLA-4, and PD-L1 in mice with brain tumors. Clin Cancer Res (2014) 20(20):5290–301. doi: 10.1158/1078-0432.CCR-14-0514
71. Zeng J, See AP, Phallen J, Jackson CM, Belcaid Z, Ruzevick J, et al. Anti-PD-1 blockade and stereotactic radiation produce long-term survival in mice with intracranial gliomas. Int J Radiat Oncol Biol Phys (2013) 86(2):343–9. doi: 10.1016/j.ijrobp.2012.12.025
72. Reiss SN, Yerram P, Modelevsky L, Grommes C. Retrospective review of safety and efficacy of programmed cell death-1 inhibitors in refractory high grade gliomas. J Immunother Cancer (2017) 5(1):99. doi: 10.1186/s40425-017-0302-x
73. Hodges TR, Ott M, Xiu J, Gatalica Z, Swensen J, Zhou S, et al. Mutational burden, immune checkpoint expression, and mismatch repair in glioma: implications for immune checkpoint immunotherapy. Neuro Oncol (2017) 19(8):1047–57. doi: 10.1093/neuonc/nox026
74. Schalper KA, Rodriguez-Ruiz ME, Diez-Valle R, Lopez-Janeiro A, Porciuncula A, Idoate MA, et al. Neoadjuvant nivolumab modifies the tumor immune microenvironment in resectable glioblastoma. Nat Med (2019) 25(3):470–6. doi: 10.1038/s41591-018-0339-5
Keywords: glioma, immunotherapy, bibliometric, Citespace, VOSviewer, ICIs
Citation: Yuan Y, Su Y, Wu Y, Xue Y, Zhang Y, Zhang Y, Zheng M, Chang T, Qu Y and Zhao T (2023) Knowledge structure and hotspots research of glioma immunotherapy: a bibliometric analysis. Front. Oncol. 13:1229905. doi: 10.3389/fonc.2023.1229905
Received: 27 May 2023; Accepted: 03 August 2023;
Published: 21 August 2023.
Edited by:
Wenxue Ma, University of California, San Diego, United StatesReviewed by:
Fanen Yuan, University of Pittsburgh, United StatesCopyright © 2023 Yuan, Su, Wu, Xue, Zhang, Zhang, Zheng, Chang, Qu and Zhao. This is an open-access article distributed under the terms of the Creative Commons Attribution License (CC BY). The use, distribution or reproduction in other forums is permitted, provided the original author(s) and the copyright owner(s) are credited and that the original publication in this journal is cited, in accordance with accepted academic practice. No use, distribution or reproduction is permitted which does not comply with these terms.
*Correspondence: Tianzhi Zhao, emhhb3RpYW56aGkxOTgxQDE2My5jb20=; Yan Qu, eWFucXUwMTIzQGZtbXUuZWR1LmNu; Ting Chang, Y2hhbmd0aW5nMTk4MUAxNjMuY29t
†These authors have contributed equally to this work and share first authorship
Disclaimer: All claims expressed in this article are solely those of the authors and do not necessarily represent those of their affiliated organizations, or those of the publisher, the editors and the reviewers. Any product that may be evaluated in this article or claim that may be made by its manufacturer is not guaranteed or endorsed by the publisher.
Research integrity at Frontiers
Learn more about the work of our research integrity team to safeguard the quality of each article we publish.