- National Cancer Center/National Clinical Research Center for Cancer/Cancer Hospital, Chinese Academy of Medical Sciences and Peking Union Medical College, Beijing, China
Breast cancer is a significant global health concern, and the discovery of endocrine therapy has played a crucial role in the treatment of estrogen-positive breast cancer. However, these therapies are often associated with osteoporosis-related adverse events, which increase the risk of fractures in breast cancer patients and can result in limited mobility and reduced quality of life. Previous studies have shown that osteoporosis is essential side effects of the breast cancer therapy, although the exact mechanisms remain mostly unclear. Current clinical treatments, such as bisphosphonates, cause side effects and may impact the therapeutic response to endocrine drugs. In this review, we explore the likelihood of endocrine therapy-induced osteoporosis in estrogen-positive breast cancer therapy and discuss the involved mechanisms as well as the therapeutic potential of drugs and drug combination strategies.
1 Introduction
Breast cancer is the most common cancer in women worldwide, accounting for 11.7% of total cases and 24.5% of cases in females according to the “Global Cancer Statistics 2020” (1) released by the International Agency for Research on Cancer (IARC) of the World Health Organization. It is also the leading cause of cancer deaths among women, accounting for 6.9% of total cancer deaths and 15.5% of female deaths. As of January 1, 2022, approximately 4.1 million women in the United States have a history of breast cancer (2). Current treatment options for breast cancer include surgery, radiotherapy, chemotherapy, endocrine therapy, targeted therapy, and traditional Chinese medicine (3). Surgery and radiotherapy are local treatments, and adjuvant systemic treatment is often required before or after surgery for non-metastatic breast cancer patients to reduce the recurrence rate. However, chemotherapy drugs can produce serious clinical side effects due to their high toxicity. Targeted drugs are only suitable for patients with positive human epidermal growth factor 2 (HER2). About 70% of patients with breast cancer are estrogen receptor (ER) positive and/or progesterone receptor (PR) positive. Endocrine-assisted therapy has demonstrated good efficacy in this subtype of patients and is an important treatment option for them. However, long-term use of these medications may lead to osteoporosis, joint and muscle pain (4), endometrial thickening (5), hot flusher, sweating, irritability, fatigue, insomnia and other adverse reactions. Osteoporosis increases the risk of fractures in breast cancer patients, resulting in limited mobility and reduced quality of life. The probability of osteoporosis associated with endocrine therapy is related to the type of medication used by the patient. Although lifestyle interventions and bone density assessments are the primary preventive measures, they may not always solve this problem. Medication such as bisphosphonates may be necessary if severe osteoporosis occurs, but these drugs can lead to side effects that may impact the therapeutic response to endocrine drugs. It is unclear whether osteoporosis drugs interact with endocrine drugs or otherwise impact breast cancer treatment.
Osteoporosis caused by endocrine therapy is mainly related to a decrease of estrogen in patients. Estrogen plays an essential role in the development and structure of bones, and estrogen deficiency promotes bone resorption and loss (6). Specifically, estrogen inhibits bone renewal by reducing osteoclast (OC) -mediated bone resorption and enhancing osteoblast (OB) -mediated bone formation (7). Estrogen deficiency promotes OC differentiation and bone resorption leading to bone loss, but the exact mechanism is not yet clear. This review aims to provide an overview of endocrine therapy for breast cancer, explore the occurrence of osteoporosis caused by endocrine therapy and its possible mechanism, as well as examine the prevention and treatment of this type of osteoporosis. Our objective is to promote rational drug use and improve the quality of life of breast cancer patients.
2 Overview of osteoporosis associated with endocrine therapy in breast cancer
Breast cancer is a malignant tumor caused by uncontrolled proliferation of ductal epithelial cells of the breast, however its specific mechanisms are not fully understood. Research has revealed that the development of breast cancer is associated with various risk factors including genetic factors and unhealthy lifestyle choices (8, 9). Breast cancer can be categorized as luminal A (ER and PR positive, HER2 negative), luminal B (ER and PR positive, HER2 positive), HER2 positive, or triple negative based on molecular pathology. Both luminal A and B breast cancers can be treated with endocrine drugs or adjuvant therapy.
Osteoporosis is a disease characterized by a decrease in bone density and an increased risk of fracture. Osteoporosis is common in menopausal women, where it is associated with bone loss due to decreased estrogen levels, and the elderly population, in which it causes unbalanced bone resorption and formation. One meta-analysis showed that the global prevalence of osteoporosis in the elderly was as high as 21.7%, with the highest prevalence in the Asian population (24.3%) (10). The analysis estimated that in 2019, the prevalence of osteoporosis in Chinese men and women aged 50+ was 6.46% and 29.13%, respectively (11). This data suggests that elderly women have a higher incidence of osteoporosis.
The strategy of endocrine therapy is to reduce the production of estrogen or the binding level of estrogen to ERs in tumor cells, thereby inhibiting the growth and proliferation of tumor. Estrogen stimulates OBs and inhibits OCs, therefore the main cause of endocrine treatment-related osteoporosis in breast cancer is bone loss induced by estrogen depletion. Patients receiving this treatment often experience adverse effects such as estrogen deficiency-related osteoporosis-induced brittle fractures. The risk of osteoporosis in patients varies based on the targets of various endocrine drugs. The most accurate clinical indicator of osteoporosis is the measurement of bone mineral density (BMD) or bone mineral content (BMC) using dual-energy X-ray absorptiometry (12). BMD can predict bone fracture risk and is typically measured at the femoral neck, total hip, or lumbar spine. T-score is generally used to evaluate osteoporosis clinically. Patients with T-score lower than 2.5 can be preliminarily diagnosed as suffering from osteoporosis.
The main anti-estrogenic drugs commonly used in clinical practice are tamoxifen (TAM), toremifene, raloxifene, and fulvestrant. These drugs compete with estrogen in the body by binding ERs. TAM, despite being an ER agonist in the bone and uterus, acts as an antagonist in breast tissue and is considered a selective estrogen modulator (SERM) due to its tissue-specific effects (7, 13). Toremifene is a chlorinated derivative of TAM and is also used in breast cancer treatment. TAM is one of the most commonly used endocrine drugs in the treatment of breast cancer and has been used for around 50 years. A 2020 nationwide retrospective cohort study conducted by Lee Jihyound and other Korean researchers using data from the Health Insurance Review and Assessment Service (HIRA) concluded that TAM does not increase the risk of osteoporosis and osteoporotic fractures in breast cancer patients under 40 years of age and has a protective effect on bones in breast cancer patients aged 40 to 49 years (14). However, another research suggested that the 5-year rate of osteoporotic fracture for patients treated with tamoxifen was 6.9% (15). There was no significant difference (HR = 1.09; 95% CI = 0.96–1.23, p-value = 0.18) from the data of aromatase inhibitors (7.5%) mentioned in the paper. So more extensive real-world studies of TAM are needed to provide sufficient evidence of its association with osteoporosis in breast cancer patients who use the drug. Fluvestrant is a selective ER down-regulator (SERD) and induces the degradation of ERs by competitively binding with them. Although no studies have been conducted to correlate the use of fulvestrant with osteoporosis, there is a risk that the drug may cause osteoporosis based on its therapeutic mechanism. Thus, BMD is typically measured during the clinical use of fulvestrant to monitor the development of osteoporosis.
Aromatase inhibitors are often the first-choice treatment in postmenopausal women with breast cancer. When combined with aromatase, these drugs work by blocking the conversion of androgens into estrogen in the body, which reduces the estrogen levels and ultimately inhibits the growth of tumor cells. However, the use of aromatase inhibitors exacerbates the age-related reduction in BMD (16) due to the link between estrogen levels and bone health (Table 1) (20). Anastrozole, letrozole, and exemestane are frequently used aromatase inhibitors, with the latter being a steroid. Exemestane is structurally similar to androstendione, the natural substrate of aromatase, and binds irreversibly to the aromatase to inactivate it. A randomized phase III trial showed no significant differences in efficacy or side effects among these three aromatase inhibitors (17). On the other hand, a meta-analysis revealed that all aromatase inhibitors had a higher incidence of osteoporosis compared to tamoxifen, but that the incidence of osteoporosis was lower in patients using exemestane than in those using anastrozole (OR: 0.8594, 95% CI: 0.5766-1.168) and letrozole (OR: 0.7358, 95% CI: 0.4301-1.307) (21). There may be differences between the two classes of aromatase inhibitors in terms of bone-related metabolism. Anastrozole use accelerates bone loss in patients with breast cancer, but the associated bone loss appears to be manageable and partially reversible with discontinuation of treatment, particularly in the lumbar spine (22–24). Irene E.G. van Hellemond et al. (18) concluded from a phase III DATA study that adjuvant use of anastrozole after 2-3 years of tamoxifen decreased BMD in postmenopausal breast cancer patients. In contrast, prolonged anastrozole treatment did not increase the incidence of osteoporosis. Similarly, patients treated with exemestane had a 2.6% decrease in spinal BMD from baseline at 6 months and only a 0.2% decrease from 6 months to 12 months (25).
LHRH analogues, such as goserelin, leuprorelin and triptorelin, are commonly used ovarian castrating drugs. This class of drug inhibits the secretion of luteinizing hormone and follicle-stimulating hormone in the anterior pituitary gland, resulting in significantly reduced estrogen in patients. Thus, these drugs are most suitable for premenopausal women. However, the use of these drugs can also results in decreased BMD, leading to osteopenia or osteoporosis (26). While these drugs have achieved some therapeutic success, their use is limited and there is a need for development of new breast cancer treatments.
Recently, CDK4/6 inhibitors have entered the clinic and have shown promise in treating ER-positive and HER2-negative advanced breast cancers. CDK4/6 inhibitors are cyclin-dependent kinase inhibitors that prevent tumor cells from entering the S phase from the G1 phase by acting on the cyclin-CDK4/6 complex, thereby inhibiting tumor growth. Palbociclib, ribociclib, abemaciclib, and trilaciclib are currently available CDK4/6 inhibitors. To date, there have been no reported cases of osteopenia or osteoporosis in breast cancer patients caused by CDK4/6 inhibitor use, although more research is required.
3 Mechanisms of endocrine drugs in breast cancer and drug-related osteoporosis
Several drugs have been studied that target ERs, which are activated by the female sex hormone estrogen. ERs are divided into two receptor subtypes, ERα and ERβ, which differ primarily in location (27). ERα is mainly found in breast cells and bone (28), making it a central target in the pathogenesis of breast cancer (29). There are two main estrogen synthesis pathways in humans: direct synthesis and secretion by the ovaries and synthesis from aromatase within the adrenal gland, fat, and other tissues. The former pathway is most prevalent in premenopausal women, while the latter is most common in postmenopausal women. Furthermore, there are three main types of estrogen in women: estrone (E1), estradiol (E2 or 17β-estradiol), and estradiol (E3). E2 is the most potent estrogen and is the main product of female premenopausal biosynthetic reactions (27).
PRs are typically co-expressed with ERs, but their role in the development of breast cancer is not fully understood. PR antagonists have not been clinically used due to serious side effects (30). The combination of estrogen and progesterone significantly affects metabolism, with estrogen tending to target tumor-promoting genes that alter glucose metabolism and progesterone targeting fat storage (31). Postmenopausal women who use estrogen and progesterone together have an increased risk of breast cancer (26%) (32) and increased breast cancer mortality (33). Additionally, a case cohort study of postmenopausal women demonstrated an increased risk of breast cancer with elevated circulating progesterone levels (16%) (34). Thus, PR plays an important role in the occurrence and progression of breast cancer. PR has two subtypes, PRA and PRB, and studies have found that a PR can bind to an ER after it is combined with its agonist, thus affecting ER-related behaviors (35). Both natural and synthetic progesterone antagonize the mitotic effect of estrogen (36). PRB is activated by a ligand, and it then activates the transcription factor EB which induces autophagy in breast cancer cells (37). Moreover, PRs can interact with STAT1 to inhibit IFN-induced STAT1 phosphorylation, thereby inhibiting carcinoma development (38). Some evidence suggests that the role of progesterone in breast tumor promotion and growth may be mediated by a receptor activator of NF-κB ligand (RANKL)-dependent effect. RANKL inhibition does not directly interfere with progesterone and PR interaction (39).
Endocrine therapies for breast cancer can be divided into several categories based on the production pathway and role of the hormone receptors in the occurrence and progression of breast cancer (Table 2), including antiestrogen drugs (selective ER regulator, selective ER downregulation), aromatase inhibitors, luteinizing hormone releasing hormone analogues (LHRH analogues), progesterone drugs, and CDK4/6 inhibitors. The action and targets of these drugs are shown in Figures 1, 2 (produced by BioRender, and the website is https://www.biorender.com/).
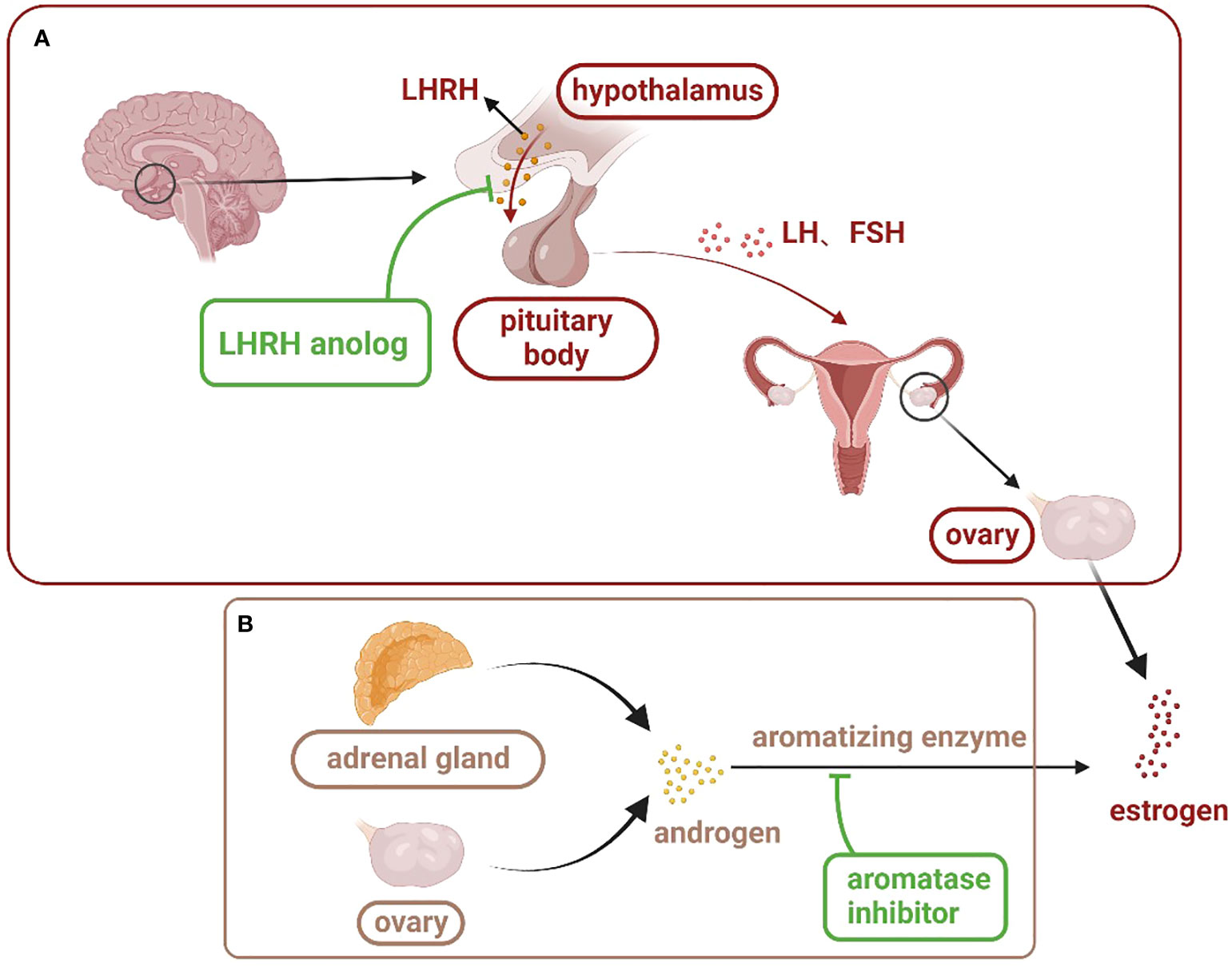
Figure 1 Estrogen production pathway in women: (A) is the main estrogen production pathway in premenopausal women. LHRH analogues inhibit the action of LHRH on the pituitary gland through competitive binding, thus inhibiting the secretion of estrogen in the ovary. (B) is the main estrogen production pathway in postmenopausal women. Aromatase inhibitors bind to androgens and prevent their conversion to estrogen. (LHRH, luteinizing hormone releasing hormone; LH, luteinizing hormone; FSH, follicle-stimulating hormone).
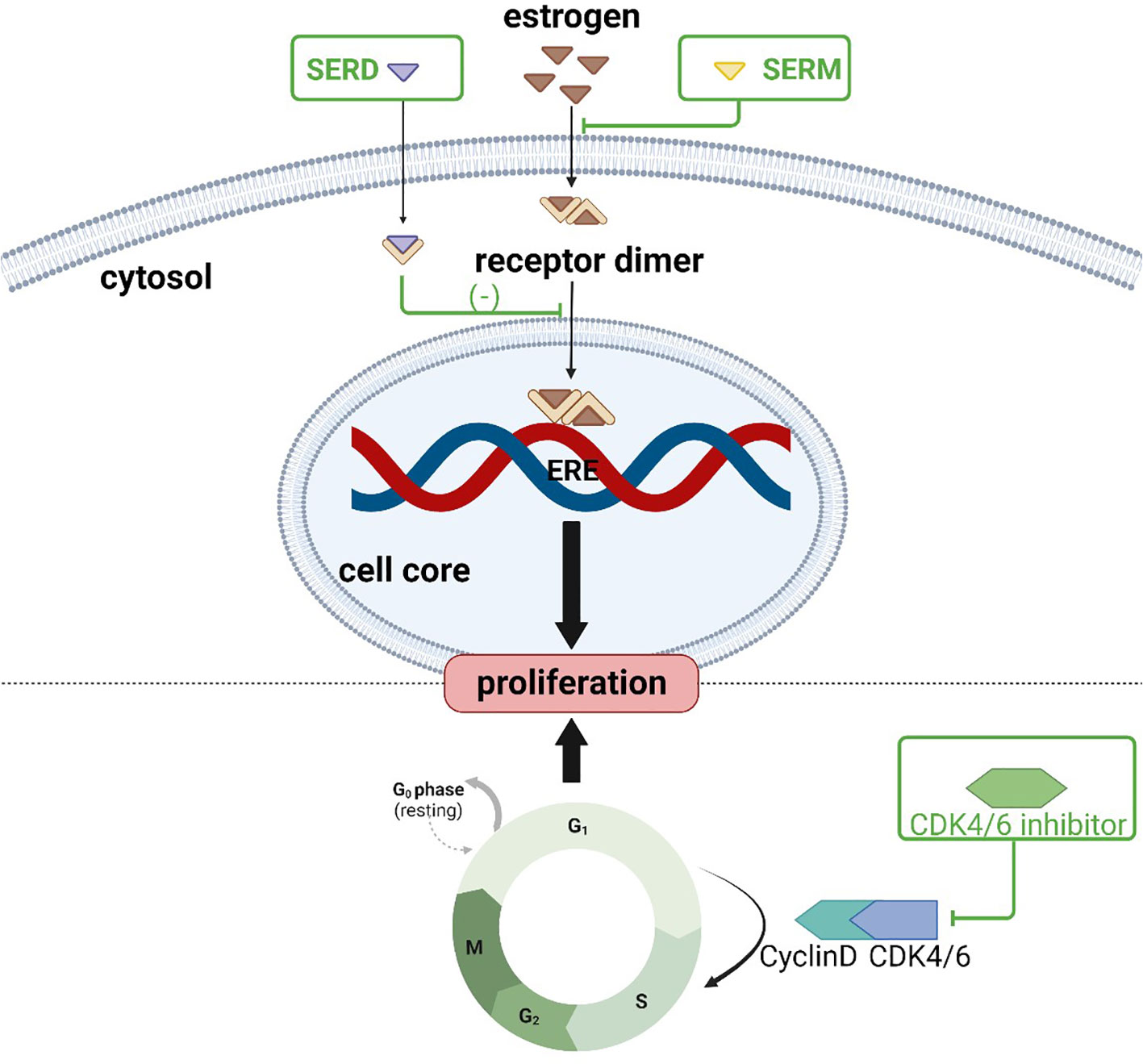
Figure 2 Action sites of anti-estrogen and CDK4/6 inhibitors: SERM and SERD bind to estrogen receptors, inhibiting downstream pathways and preventing tumor cell proliferation; CDK4/6 inhibitors prevent dividing tumor cells from the G1 phase into the S phase.
Estrogen is an important hormone that helps regulate the metabolic process of bone by inhibiting OCs thereby preventing osteoporosis. Bone loss caused by endocrine therapy in patients with hormone receptor positive breast cancer is mainly due to estrogen deprivation (40). At the osteocyte level, estrogen inhibits OC differentiation which reduces the number of active remodeling units. The mechanism of osteoporosis associated with endocrine therapy in breast cancer is related to changes in cytokines, the RANK pathway, Wnt pathway, and MicroRNA after estrogen level reduction (Figure 3). However, the mechanism of its action in the skeleton caused by crosstalk between various pathways in skeletal cells has not been fully elucidated.
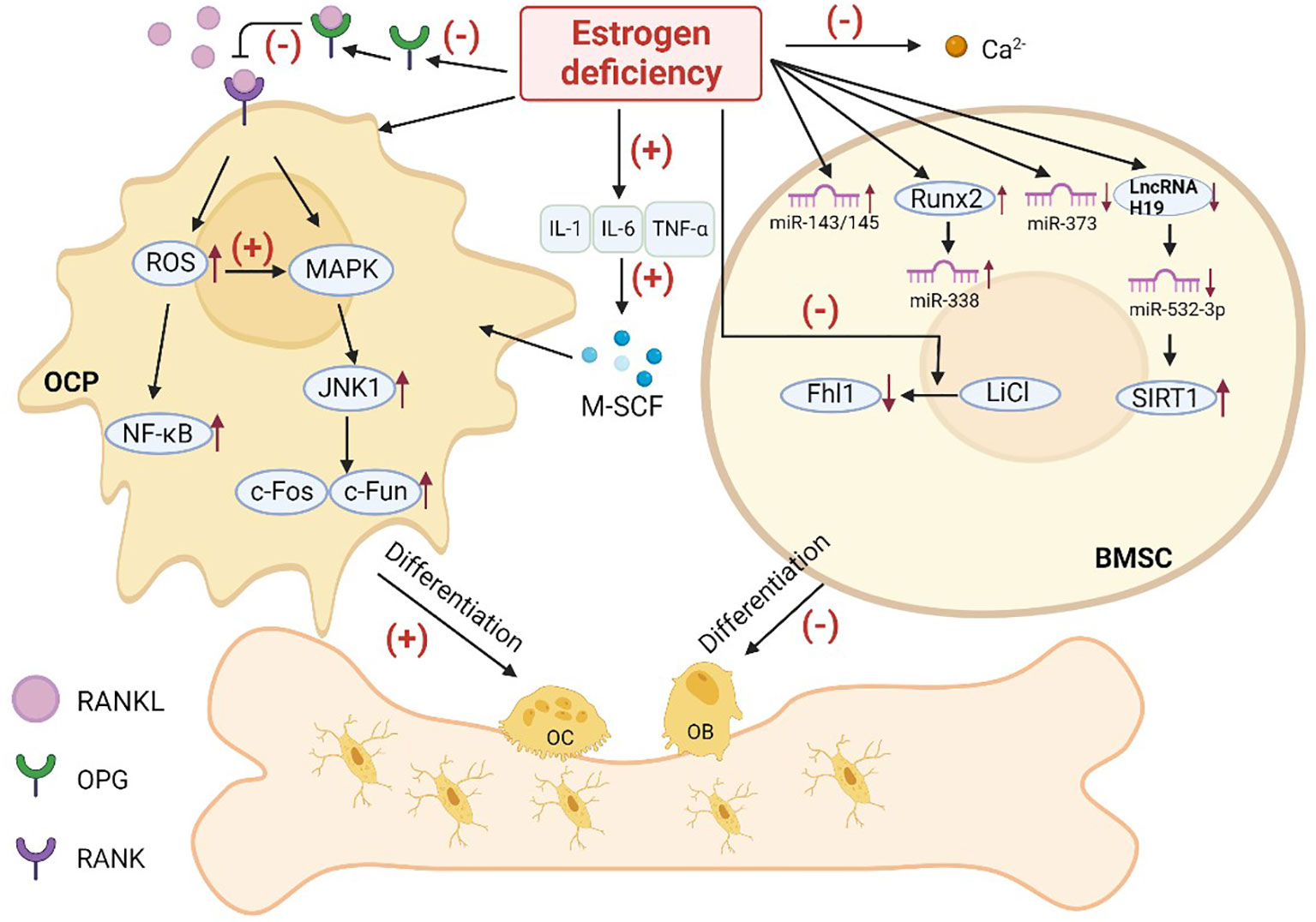
Figure 3 Possible pathways of osteoporosis caused by estrogen deficiency. (OC, osteoblast; OB, osteoblast; OCP, osteoclast precursor; BMSC, bone marrow mesenchymal stem cell; OPG, osteoprotegerin; RANKL, receptor activator of NF-κB ligand; RANK, receptor activator of NF-κB; IL-1, interleukin-1; IL-6, interleukin-6; TNF-α, tumor necrosis factor-α; M-SCF:; ROS, reactive oxygen species).
3.1 Cytokine
Previous studies have suggested that estrogen may exert bone-protective effects by inhibiting inflammatory cytokines, such as interleukin-1 (IL-1), IL-6, and tumor necrosis factor-α (TNF-α), that promote OC formation and bone resorption. Estrogen selectively regulates IL-1R isoforms in OC or OC-like cells thereby decreasing their IL-1 responsiveness and survival (41). Conversely, this IL-1 inhibition may be lost as estrogen levels decline, resulting in OC-mediated bone loss. Estrogen deficiency leads to increased secretion of IL-1 and TNF, increased macrophage colony-stimulating factor (M-CSF) (42), and increased osteoclasts. Animal studies have shown that IL-6-deficient ovariectomized (OVX) female mice do not experience significant changes in bone mass and are protected from bone loss caused by estrogen depletion (43).
3.2 RANK/RANKL/OPG pathway
Prior research has shown that the effect of estrogen on bone is related to the RANKL pathway, which promotes the transformation of osteoclast precursors (OCPs) into mature OCs. Activators of RANKL signaling include reactive oxygen species (ROS), the NF-κB pathway, and mitogen activated protein kinase (MAPK) (44).
The protein osteoprotegerin (OPG) can bind to RANKL within the bone remodeling environment to prevent excessive OC formation. Estrogen activates p38α (a p38MAPKs) to maintain OPG gene expression and production in bone marrow mesenchymal stem cells (BMSCs) to protect the bone (45, 46).
Reactive oxygen species (ROS), including superoxide ion (O2−) and hydrogen peroxide (H2O2), are important components that regulate OC differentiation. The downstream target of ROS remains unclear, but increased oxidative stress may promote the cellular activity of OCs by triggering the NF-κB and MAPK signaling pathways (47). Under normal physiological conditions, ROS produced by OCs stimulate and promote bone tissue absorption (48). However, deficiency of the potent antioxidant estrogen leads to the accumulation of ROS in the body which in turn affects osteogenic differentiation of stem cells and the formation of osteoporosis (49).
Estrogen can inhibit RANKL expression in bone lining cells (50), and the reduced rate of RANKL formation subsequently reduces the differentiation of OCPs into OCs. Another mechanism by which E2 downregulates OC formation is by decreasing OCP reactivity to RANKL (51). E2 down-regulates the activation of Jun N-terminal kinase1 (JNK1). This decreased JNK1 activity leads to decreased nuclear levels and DNA binding of the key OC transcription factors c-Fos and c-Jun, which weakens the differentiation ability of OCPs. It was shown that when estrogen levels were reduced in vivo, RANKL expression was increased, the response of OCPs to free RANKL was enhanced, and bone resorption was increased.
E2 inhibits OC differentiation and stimulates mature apoptosis (52) by increasing the expression of transient receptor potential vanilloid 5 (TRPV5) channels (53). The specific mechanism is as follows: E2 enhances the expression of TRPV5 through the interaction of ERα with NF-κB, and NF-κB can directly bind to the TRPV5 promoter region from −286 nt ~−277 nt fragments. When estrogen is deficient, the expression of the TRPV5 channel weakens, OC differentiation enhances, and the risk of osteoporosis increases.
3.3 Wnt pathway
The Wnt pathway is highly conserved and present in both invertebrates and vertebrates. It plays a critical role in various physiological processes during early animal embryo development, including organ formation and tissue regeneration. The Wnt signaling pathway also regulates proliferation and differentiation of OBs by influencing gene transcription. Estrogen deficiency reduces GSK3β phosphorylation in OBs, leading to inhibition of the Wnt/β-catenin pathway and decreased OB proliferation (54). The Wnt agonist LiCl has been shown to induce the expression of the Fhl1 gene, which in turn promotes the expression of osteogenic markers such as Runt-associated transcription factor 2 (Runx2), osteocalcin (OCN), and osteopontin (OPN). This ultimately enhances OB differentiation significantly. Estrogen cannot directly act on OBs, but it can coordinate with LiCl to stimulate Fhl1 expression and promote OB differentiation (55).
3.4 MicroRNA
MicroRNA (miRNA) is a type of non-coding single-stranded RNA that plays a crucial role in the regulation of post-transcriptional gene expression and is essential for mammal bone development (56). Overexpression of miR-373 can promote the differentiation of BMSCs into OBs and reverse the decreased osteogenic differentiation ability of BMSCs caused by osteoporosis. Estrogen deficiency causes decreased miR-373 expression (57), significant enrichment of the miR-338 cluster (58), and elevated expression of miR-143/145 (59), leading to a decreased differentiation of BMSCs into OBs. Furthermore, estrogen can decrease the expression of miR-532-3p through up-regulation of long noncoding RNA H19 (lncRNA H19). LncRNA H19-mediated disruption of the miR-532-3p/SIRT1 axis has been shown to induce osteogenic differentiation of BMSCs which alleviated osteoporosis in OVX rats (60). However, osteogenic differentiation induced by this pathway is reduced if estrogen is deficient in vivo. Estrogen up-regulates miR-27a, which inhibits peroxisome proliferator-activated receptor gamma (PPARγ) in OCs and adenomatous polyposis coli (APC) expression, resulting in decreased OC production and reduced bone resorption (61). The estrogen-activated ERα pathway inhibits the production of miR-21 (62), which enhances Fasl protein to stimulate caspase-3 activity and induces the Fas/FasL system to regulate the lifespan of mature OCs (63), thereby inducing OC apoptosis. However, studies have also shown that estrogen deficiency-related decreased expression of miR-128 can lead to decreased OC production (64), thereby delaying or preventing the occurrence of osteoporosis. Overall, estrogen can inhibit the activity of OCs, reduce bone resorption, promote OB formation, and enhance bone formation by interacting with various miRNAs in OBs and OCs. Further research is required to uncover the regulatory pathways of estrogen and miRNAs on bone remodeling in various bone cell types.
In addition, estrogen directly affects calcium absorption in the gut, and calcium plays an important role in bones (65). Therefore, low estrogen levels can lead to insufficient calcium intake in the body, which is a primary cause of osteoporosis.
These targets and pathways associated with osteoporosis are also present in other organs and tissues. Therefore, the design of drugs for the treatment of osteoporosis for these targets should also consider the side effects on organs and tissues. Specific targets of bone metabolism are necessary to explore for better treatment of bone-related diseases.
4 Strategies to prevent or treat osteoporosis caused by endocrine therapy for breast cancer
Although osteoporosis cannot be cured, it can be prevented, slowed down, or stopped through various means, including increasing bone formation and inhibiting bone resorption. Biochemical markers of bone turnover can indicate the events that occur during the bone remodeling cycle and are divided into bone formation and bone resorption markers (66). Clinical practices currently use serum osteocalcin, serum bone alkaline phosphatase (BAP), urinary N-terminal peptide of type 1 collagen (NTx), and urinary C-terminal peptide of type 1 collagen (CTx) to monitor bone turnover. Various guidelines (67–69) have recommended reducing the risk of fragility fractures through screening, life interventions, and pharmacological treatment as a primary goal of osteoporosis prevention and treatment. For breast cancer patients on endocrine medications, especially aromatase inhibitors, regular monitoring of BMD is paramount. Appropriate medications should be used to prevent the development of osteoporosis, and the patients who have been diagnosed with osteoporosis require medications to stop continued bone loss and prevent fractures. Anti-resorptive agents and anabolic agents are common medications used to prevent and treat osteoporosis (Table 3). Importantly, osteoporosis associated with breast cancer endocrine therapy differs from conventional osteoporosis management due to the need to balance the outcome and course of the cancer treatment. However, due to limited information on osteoporosis management in cancer patients, current clinical strategies for endocrine treatment-related osteoporosis in breast cancer reference normal osteoporosis (75).
4.1 Lifestyle intervention
In order to prevent or treat osteoporosis, breast cancer patients should quit using tobacco products and alcohol (76), take adequate calcium and vitamin D supplements (77), and exercise moderately. It may also be beneficial for patients to consume appropriate antioxidant functional foods. For example, one study reported that green tea consumption and exercise were negatively associated with osteoporosis (78). Additional studies have found that tea consumption reduces the risk of osteoporosis (79), and that weight-bearing and resistance exercise maintains BMD and improves the quality of life in postmenopausal women with low bone mass (80). However, there was not a strong BMD improvement in breast cancer patients.
4.2 Antiresorptive agent
Antiresorptive agents, including bisphosphonates, RANKL inhibitors, estrogens and SERMs, are the preferred drugs for the treatment of osteoporosis (81). Table 4 provides a list of clinical trials that have evaluated the use of antiresorptive agents. A recently published real-world study showed that early postmenopausal women with ER-positive breast cancer who were treated with aromatase inhibitors in combination with anti-bone resorptive therapy experienced a significant increase in both femur and lumbar BMD after 24 months (6.28%, 7.79%, respectively) (85). Anti-bone resorption therapy can significantly improve BMD in postmenopausal women with early breast cancer who are using aromatase inhibitors, thereby preventing bone loss. One study found that the combination of letrozole and zoledronic acid inhibited the growth of and induced apoptosis in MCF-7 and T-47D human breast cancer cell lines (86). As a bisphosphate, zoledronic acid can be used in the treatment and prevention of osteoporosis, though the clinical effect of the combined use of letrozole and zoledronic acid requires additional studies. A 24-month randomized controlled trial showed increased BMD and decreased bone resorption in total hip joint (+1.81%) and spine segments 1 to 4 (+2.85%) in subjects taking risedronate, calcium, and vitamin D without exercise (87). This indicates that bisphosphonates, calcium, and vitamin D are effective treatments for bone loss in postmenopausal breast cancer patients with hip and spine bone loss. However, oral bisphosphonates can have side effects that may cause patients to discontinue the drug midway through its use. For example, bisphosphates can cause irritation to the mucosa of the gastrointestinal tract, leading to esophagitis, dysphagia, and gastric ulcers (75). Additionally, some patients have experienced hypocalcemia (88). One study reported that approximately 20% of patients who received intravenous zoledronic acid experienced side effects including flu-like syndrome (69%), arthralgia (7.7%), and even renal failure (89).
Denosumab specifically targets RANKL, inhibits OC activation and progression caused by related pathways, reduces bone resorption, and increases BMD in the treatment of postmenopausal women with osteoporosis. The use of denosumab has been reported to increase BMD in the lumbar spine, hip, and femoral neck in breast cancer patients treated with aromatase inhibitors (90). Results from a randomized controlled trial exhibited that subcutaneous administration of denosumab every 6 months significantly reduced clinical fracture rates and significantly delayed time to first fracture in postmenopausal patients with hormone receptor-positive early-stage breast cancer treated with adjuvant aromatase inhibitors (91). However, denosumab does not specifically target bone (92), therefore it inhibits the RANKL pathway in other tissues. Its common side effects include hypocalcemia (93), nasopharyngitis, upper respiratory tract infections, urinary tract infections, arthralgia, headache, constipation, and skin rash (75).
Raloxifene, a selective hormone receptor modulator (SERM), is widely used for the prevention and treatment of postmenopausal osteoporosis (94). Results of a randomized clinical trial showed that 3 years of raloxifene treatment maintained BMD and reduced bone turnover. Moreover, raloxifene increased BMD in the spine and femoral neck and reduced the risk of vertebral fractures in postmenopausal women with osteoporosis (95). An animal trial also demonstrated that the osteoporosis drug lasoxifene has the potential to treat ER-positive patients who are resistant to aromatase inhibitors (96).
Hormone therapy is not a feasible treatment option for osteoporosis associated with endocrine therapy in breast cancer patients, as estrogen can promote the progression of breast tumors and affect the treatment of breast cancer.
4.3 Anabolic agent
Antiresorptive agents do not restore the bone mass and structure that is lost due to increased bone remodeling. On the other hand, anabolic agents increase the likelihood of new bone formation (97). In a randomized controlled trial, the most commonly used parathyroid hormone analog, Teriparatide, was found to be associated with a lower risk of new vertebral fractures (5.4%) at 24 months of use compared to risedronate (12.0%) in postmenopausal women with severe osteoporosis (98). However, some scientists argue that comparison between these two drugs is not clinically significant as they are administered in different ways (99). A recent meta-analysis revealed that teriparatide was linked to a reduced risk of vertebral fracture compared to bisphosphonate (RR= 0.57, 95% CI: 0.35-0.93, P = 0.024), and that the drug increased the mean percentage change in femoral neck BMD at 18 months (P < 0.05) (89). In conclusion, teriparatide is an effective drug for reducing the risk of vertebral fractures in postmenopausal women with osteoporosis, and it can increase the BMD of lumbar spine and femoral neck in the long term.
4.4 Potential drug development
Research on the mechanisms of osteoporosis caused by estrogen deficiency in vivo may lead to new breakthroughs in the development of osteoporosis drugs. Studies have shown that Connexin 43 half-channels (Cx43 HCs) demonstrate protective effects on bone cells and can inhibit bone loss in estrogen-deficient mice following ovariectomy (100). Another promising avenue of research is the mitochondrial protein deacetylase, Sirtuin-3 (Sirt3), which has a high mitochondrial content in OCs. Inhibition of this enzyme can damage mitochondria-related functions in OCs and reverse the increased bone resorption and bone mass loss caused by estrogen deficiency (70). Sirt3 inhibitors are promising for osteopenia caused by endocrine therapy in breast cancer.
Scientists are also investigating natural compounds and active ingredients in traditional Chinese medicine as potential drugs to treat osteoporosis. For instance, isosinensetin, a flavonoid present in citrus fruits with antioxidant properties, has been shown to reduce bone loss in OVX mice and alleviate estrogen deficiency-induced osteoporosis in mice (71). Obacunone, a small molecule with a wide range of biological activities, can inhibit the formation and absorption function of OCs in vitro by targeting inhibitory factor of macrophage migration inhibitory factor (MIF) (44). Thus, it is expected to be an effective drug for relieving osteoporosis caused by estrogen deficiency. Traditional Chinese medicine also shows promise in treating osteoporosis by invigorating the kidneys and spleen. One animal study showed that Bushen Jianpi Decoction improved bone loss without affecting estrogen levels in mice and may increase the sensitivity of breast tumor cells to endocrine drugs, thereby improving efficacy (101). Boldine, an alkaloid isolated from the Bordeaux tree, has a protective effect against bone loss in mice caused by estrogen deficiency (72) by inhibiting OC formation induced by activator of nuclear factor κB ligand receptor by impacting the AKT signaling pathway. Icariside I (GH01), a novel isopentenyl flavonoid isolated from epimedii, was shown to effectively ameliorate estrogen deficiency-induced osteoporosis and strengthen the trabecular and cortical bone in OVX mouse models by simultaneously regulating OB and OC differentiation (73). Eucommia ulmoides and psoralea were commonly used drugs (102). Corylifol A, an isoflavone isolated from psoralea fruit, inhibits OCs and absorption by inhibiting intracellular ROS levels to prevent bone loss caused by estrogen deficiency (74). Psoralen extracted from psoralen seed has been studied for its in anti-tumor effects, but it has also been shown to impact certain pathways that may result in anti-osteoporosis effects (103). Acid polysaccharide EuOCP3 extracted from Eucommia ulmoides skin can restore cortical bone thickness, increase mineralized bone area, increase the number of OBs, and reduce the number of OCs on the cortical bone surface in osteoporotic mice (104). The total flavones of Radix osteoblastum are widely used in the treatment of postmenopausal osteoporosis (105) are expected to emerge as a new treatment for breast cancer-related osteoporosis. There are also many effective Chinese medicine ingredients for osteoporosis, such as resveratrol, puerarin, astragaloside IV, and Danshensu (106).
5 Summery and prospects
Endocrine therapy for breast cancer can lead to osteoporosis, particularly in postmenopausal women who use aromatase inhibitors, which impacts patient quality of life. There are limited data on the management and prevention of cancer-related osteoporosis, with no drugs specifically targeted for these patients. More clinical studies are needed to provide more effective and safe treatment options. Traditional Chinese medicine has great potential in the prevention and treatment of osteoporosis caused by endocrine therapy in breast cancer. As the acquired and intrinsic resistance mechanisms of endocrine therapy drug and CDK4/6 inhibitors is gradually revealed, there is a need to develop novel endocrine drugs with higher efficacy and fewer side effects for breast cancer patients (107). Blood vessels and lymphatic vessels are important components of bone. Some studies have shown that the vascular system plays an important role in the process of bone metastasis (108). It is necessary to explore their relationship with osteoporosis to provide new treatment strategies. An animal study showed that Substance P(SP), an endogenous neuropeptide, blocked H-type vascular loss and sustained angiogenic factor enrichment in pretreated OVX mice. SP can mediate early vascular protection and inhibit bone density loss (109). The relationship between bone lymphatics and osteoporosis remains to be further explored. Additionally, the pathogenesis of breast cancer is not yet fully understood, and there is a need for more effective therapeutic drugs to reduce the occurrence of adverse reactions related to endocrine therapy.
Author contributions
JX collected the related paper, drafted, and revised the manuscript. BC participated in the design of the review, and helped to draft and revise the manuscript. CL and GL designed the review. All authors contributed to the article and approved the submitted version.
Funding
This work has been financially supported by CAMS Innovation Fund for Medical Sciences (CIFMS) (Grant no. 2021-I2M-1-014).
Conflict of interest
The authors declare that the research was conducted in the absence of any commercial or financial relationships that could be construed as a potential conflict of interest.
Publisher’s note
All claims expressed in this article are solely those of the authors and do not necessarily represent those of their affiliated organizations, or those of the publisher, the editors and the reviewers. Any product that may be evaluated in this article, or claim that may be made by its manufacturer, is not guaranteed or endorsed by the publisher.
References
1. Sung H, Ferlay J, Siegel RL, Laversanne M, Soerjomataram I, Jemal A, et al. Global cancer statistics 2020: GLOBOCAN estimates of incidence and mortality worldwide for 36 cancers in 185 countries. CA: A Cancer J Clin (2021) 71(3):209–49. doi: 10.3322/caac.21660
2. Giaquinto AN, Sung H, Miller KD, Kramer JL, Newman LA, Minihan A, et al. Breast cancer statistics, 2022. CA: A Cancer J Clin (2022) 72(6):524–41. doi: 10.3322/caac.21754
3. National Health Commission of the People's Republic of China.Guidelines for the diagnosis and treatment of breast cancer (2022 edition). Chinese Journal of rational drug use (2022) 19(10):1–26.
4. Hadji P, Kieback DG, Tams J, Hasenburg A, Ziller M. Correlation of treatment-emergent adverse events and clinical response to endocrine therapy in early breast cancer: a retrospective analysis of the German cohort of TEAM. Ann Oncol (2012) 23(10):2566–72. doi: 10.1093/annonc/mds055
5. Lahti E, Blanco G, Kauppila A, Apaja-Sarkkinen M, Taskinen PJ, Laatikainen T. Endometrial changes in postmenopausal breast cancer patients receiving tamoxifen. Obstet Gynecol (1993) 81(5(5 ( Pt 1):660–4.
6. Levin VA, Jiang X, Kagan R. Estrogen therapy for osteoporosis in the modern era. Osteoporosis Int (2018) 29(5):1049–55. doi: 10.1007/s00198-018-4414-z
7. Deroo BJ, Korach KS. Estrogen receptors and human disease. J Clin Invest (2006) 116(3):561–70. doi: 10.1172/JCI27987
8. Collaborative Group on Hormonal Factors in Breast C. Alcohol, tobacco and breast cancer – collaborative reanalysis of individual data from 53 epidemiological studies, including 58 515 women with breast cancer and 95 067 women without the disease. Br J Cancer (2002) 87(11):1234–45. doi: 10.1038/sj.bjc.6600596
9. Jung S, Wang M, Anderson K, Baglietto L, Bergkvist L, Bernstein L, et al. Alcohol consumption and breast cancer risk by estrogen receptor status: in a pooled analysis of 20 studies. Int J Epidemiol (2015) 45(3):916–28. doi: 10.1093/ije/dyv156
10. Salari N, Darvishi N, Bartina Y, Larti M, Kiaei A, Hemmati M, et al. Global prevalence of osteoporosis among the world older adults: a comprehensive systematic review and meta-analysis. J Orthopaedic Surg Res (2021) 16(1):669. doi: 10.1186/s13018-021-02821-8
11. Zeng Q, Li N, Wang Q, Feng J, Sun D, Zhang Q, et al. The prevalence of osteoporosis in China, a nationwide, multicenter DXA survey. J Bone Mineral Res (2019) 34(10):1789–97. doi: 10.1002/jbmr.3757
12. Marshall D, Johnell O, Wedel H. Meta-analysis of how well measures of bone mineral density predict occurrence of osteoporotic fractures. BMJ (1996) 312(7041):1254–9. doi: 10.1136/bmj.312.7041.1254
13. Hayes DF, Rae JM. Pharmacogenomics and endocrine therapy in breast cancer. J Clin Oncol (2020) 38(6):525–8. doi: 10.1200/JCO.19.03119
14. Lee J, Alqudaihi HM, Kang MS, Kim J, Lee JW, Ko BS, et al. Effect of tamoxifen on the risk of osteoporosis and osteoporotic fracture in younger breast cancer survivors: a nationwide study. Front Oncol (2020) 10. doi: 10.3389/fonc.2020.00366
15. Blanchette PS, Lam M, Le B, Richard L, Shariff SZ, Ouédraogo AM, et al. The association between endocrine therapy use and osteoporotic fracture among post-menopausal women treated for early-stage breast cancer in Ontario, Canada. Breast (2021) 60:295–301. doi: 10.1016/j.breast.2021.09.010
16. Hamood R, Hamood H, Merhasin I, Keinan-Boker L. Hormone therapy and osteoporosis in breast cancer survivors: assessment of risk and adherence to screening recommendations. Osteoporosis Int (2019) 30(1):187–200. doi: 10.1007/s00198-018-4758-4
17. De Placido S, Gallo C, De Laurentiis M, Bisagni G, Arpino G, Sarobba MG, et al. Adjuvant anastrozole versus exemestane versus letrozole, upfront or after 2 years of tamoxifen, in endocrine-sensitive breast cancer (FATA-GIM3): a randomised, phase 3 trial. Lancet Oncol (2018) 19(4):474–85. doi: 10.1016/S1470-2045(18)30116-5
18. van Hellemond IEG, Smorenburg CH, Peer PGM, Swinkels ACP, Seynaeve CM, van der Sangen MJC, et al. Assessment and management of bone health in women with early breast cancer receiving endocrine treatment in the DATA study. Int J Cancer (2019) 145(5):1325–33. doi: 10.1002/ijc.32205
19. Goss PE, Ingle JN, Pritchard KI, Ellis MJ, Sledge GW, Budd GT, et al. Exemestane versus anastrozole in postmenopausal women with early breast cancer: NCIC CTG MA.27–a randomized controlled phase III trial. J Clin Oncol (2013) 31(11):1398–404. doi: 10.1200/jco.2012.44.7805
20. Lee Y-K, Lee E-G, Kim HY, Lee Y, Lee S-M, Suh D-C, et al. Osteoporotic fractures of the spine, hip, and other locations after adjuvant endocrine therapy with aromatase inhibitors in breast cancer patients: a meta-analysis. J Korean Med Sci (2020) 35(46):e403. doi: 10.3346/jkms.2020.35.e403
21. Chen S, Lan B, Lv D, Ma F. Bone safety profile of steroidal aromatase inhibitor in comparison to non-steroidal aromatase inhibitors in postmenopausal women with breast cancer: a network meta-analysis. J Clin Oncol (2021) 39(15_suppl):527–. doi: 10.1200/JCO.2021.39.15_suppl.527
22. Eastell R, Hannon RA, Cuzick J, Dowsett M, Clack G, Adams JE. Effect of an aromatase inhibitor on BMD and bone turnover markers: 2-year results of the anastrozole, tamoxifen, alone or in combination (ATAC) trial (18233230). J Bone Mineral Res (2006) 21(8):1215–23. doi: 10.1359/jbmr.060508
23. Confavreux CB, Fontana A, Guastalla JP, Munoz F, Brun J, Delmas PD. Estrogen-dependent increase in bone turnover and bone loss in postmenopausal women with breast cancer treated with anastrozole. Prev bisphosphonates Bone (2007) 41(3):346–52. doi: 10.1016/j.bone.2007.06.004
24. Sestak I, Blake G, Patel R, Cuzick J, Howell A, Coleman R, et al. Off-treatment bone mineral density changes in postmenopausal women receiving anastrozole for 5 years: 7-year results from the IBIS-II prevention trial. Br J Cancer (2021) 124(8):1373–8. doi: 10.1038/s41416-020-01228-2
25. Hadji P, Ziller M, Kieback DG, Dornoff W, Tessen HW, Menschik T, et al. Effects of exemestane and tamoxifen on bone health within the tamoxifen exemestane adjuvant multicentre (TEAM) trial: results of a German, 12-month, prospective, randomised substudy. Ann Oncol (2009) 20(7):1203–9. doi: 10.1093/annonc/mdn762
26. Jonat W. Role of LHRH agonists in premenopausal women with oestrogen receptor-positive breast cancer: the ZEBRA experience. Eur J Cancer (2002) 38:39–40. doi: 10.1016/S0959-8049(02)00280-0
27. Saha T, Makar S, Swetha R, Gutti G, Singh SK. Estrogen signaling: an emanating therapeutic target for breast cancer treatment. Eur J Medicinal Chem (2019) 177:116–43. doi: 10.1016/j.ejmech.2019.05.023
28. Hewitt SC, Couse JF, Korach KS. Estrogen receptor transcription and transactivation estrogen receptor knockout mice: what their phenotypes reveal about mechanisms of estrogen action. Breast Cancer Res (2000) 2(5):345. doi: 10.1186/bcr79
29. Waks AG, Winer EP. Breast cancer treatment. JAMA (2019) 321(3):316–. doi: 10.1001/jama.2018.20751
30. Brisken C. Progesterone signalling in breast cancer: a neglected hormone coming into the limelight. Nat Rev Cancer (2013) 13(6):385–96. doi: 10.1038/nrc3518
31. Ward AV, Matthews SB, Fettig LM, Riley D, Finlay-Schultz J, Paul KV, et al. Estrogens and progestins cooperatively shift breast cancer cell metabolism. Cancers (2022) 14(7):1776. doi: 10.3390/cancers14071776
32. Investigators WGftWsHI. Risks and benefits of estrogen plus progestin in healthy postmenopausal WomenPrincipal results from the women's health initiative randomized controlled trial. JAMA (2002) 288(3):321–33. doi: 10.1001/jama.288.3.321
33. Chlebowski RT, Anderson GL, Gass M, Lane DS, Aragaki AK, Kuller LH, et al. Estrogen plus progestin and breast cancer incidence and mortality in postmenopausal women. JAMA (2010) 304(15):1684–92. doi: 10.1001/jama.2010.1500
34. Trabert B, Bauer DC, Buist DSM, Cauley JA, Falk RT, Geczik AM, et al. Association of circulating progesterone with breast cancer risk among postmenopausal women. JAMA Network Open (2020) 3(4):e203645–e. doi: 10.1001/jamanetworkopen.2020.3645
35. Mohammed H, Russell IA, Stark R, Rueda OM, Hickey TE, Tarulli GA, et al. Progesterone receptor modulates ERα action in breast cancer. Nature (2015) 523(7560):313–7. doi: 10.1038/nature14583
36. Finlay-Schultz J, Gillen AE, Brechbuhl HM, Ivie JJ, Matthews SB, Jacobsen BM, et al. Breast cancer suppression by progesterone receptors is mediated by their modulation of estrogen receptors and RNA polymerase III. Cancer Res (2017) 77(18):4934–46. doi: 10.1158/0008-5472.CAN-16-3541
37. Tan S, Bajalovic N, Wong ESP, Lin VCL. Ligand-activated progesterone receptor b activates transcription factor EB to promote autophagy in human breast cancer cells. Exp Cell Res (2019) 382(1):111433. doi: 10.1016/j.yexcr.2019.05.014
38. Goodman ML, Trinca GM, Walter KR, Papachristou EK, D’Santos CS, Li T, et al. Progesterone receptor attenuates STAT1-mediated IFN signaling in breast cancer. J Immunol (2019) 202(10):3076–86. doi: 10.4049/jimmunol.1801152
39. Gonzalez-Suarez E, Jacob AP, Jones J, Miller R, Roudier-Meyer MP, Erwert R, et al. RANK ligand mediates progestin-induced mammary epithelial proliferation and carcinogenesis. Nature (2010) 468(7320):103–7. doi: 10.1038/nature09495
40. Castañeda S, Casas A, González-del-Alba A, Martínez-Díaz-Guerra G, Nogués X, Ojeda Thies C, et al. Bone loss induced by cancer treatments in breast and prostate cancer patients. Clin Trans Oncol (2022) 24(11):2090–106. doi: 10.1007/s12094-022-02872-1
41. Sunyer T, Lewis J, Collin-Osdoby P, Osdoby P. Estrogen’s bone-protective effects may involve differential IL-1 receptor regulation in human osteoclast-like cells. J Clin Invest (1999) 103(10):1409–18. doi: 10.1172/JCI4682
42. Kimble RB, Srivastava S, Ross FP, Matayoshi A, Pacifici R. Estrogen deficiency increases the ability of stromal cells to support murine osteoclastogenesis via an interleukin-1and tumor necrosis factor-mediated stimulation of macrophage colony-stimulating factor production*. J Biol Chem (1996) 271(46):28890–7. doi: 10.1074/jbc.271.46.28890
43. Poli V, Balena R, Fattori E, Markatos A, Yamamoto M, Tanaka H, et al. Interleukin-6 deficient mice are protected from bone loss caused by estrogen depletion. EMBO J (1994) 13(5):1189–96. doi: 10.1002/j.1460-2075.1994.tb06368.x
44. He J, Zheng L, Li X, Huang F, Hu S, Chen L, et al. Obacunone targets macrophage migration inhibitory factor (MIF) to impede osteoclastogenesis and alleviate ovariectomy-induced bone loss. J Advanced Res (2023) 1–14. doi: 10.1016/j.jare.2023.01.003
45. Cong Q, Jia H, Biswas S, Li P, Qiu S, Deng Q, et al. p38α MAPK regulates lineage commitment and OPG synthesis of bone marrow stromal cells to prevent bone loss under physiological and pathological conditions. Stem Cell Rep (2016) 6(4):566–78. doi: 10.1016/j.stemcr.2016.02.001
46. Hofbauer LC, Khosla S, Dunstan CR, Lacey DL, Spelsberg TC, Riggs BL. Estrogen stimulates gene expression and protein production of osteoprotegerin in human osteoblastic cells*. Endocrinology (1999) 140(9):4367–70. doi: 10.1210/endo.140.9.7131
47. Hong G, Chen Z, Han X, Zhou L, Pang F, Wu R, et al. A novel RANKL-targeted flavonoid glycoside prevents osteoporosis through inhibiting NFATc1 and reactive oxygen species. Clin Trans Med (2021) 11(5):e392. doi: 10.1002/ctm2.392
48. Agidigbi TS, Kim C. Reactive oxygen species in osteoclast differentiation and possible pharmaceutical targets of ROS-mediated osteoclast diseases. Int J Mol Sci (2019) 20(14):3576. doi: 10.3390/ijms20143576
49. Ding W, Zhou Q, Lu Y, Wei Q, Tang H, Zhang D, et al. ROS-scavenging hydrogel as protective carrier to regulate stem cells activity and promote osteointegration of 3D printed porous titanium prosthesis in osteoporosis. Front Bioeng Biotechnol (2023) 11:1103611. doi: 10.3389/fbioe.2023.1103611
50. Streicher C, Heyny A, Andrukhova O, Haigl B, Slavic S, Schüler C, et al. Estrogen regulates bone turnover by targeting RANKL expression in bone lining cells. Sci Rep (2017) 7(1):6460. doi: 10.1038/s41598-017-06614-0
51. Srivastava S, Toraldo G, Weitzmann MN, Cenci S, Ross FP, Pacifici R. Estrogen decreases osteoclast formation by down-regulating receptor activator of NF-κB ligand (RANKL)-induced JNK activation*. J Biol Chem (2001) 276(12):8836–40. doi: 10.1074/jbc.M010764200
52. Song T, Lin T, Ma J, Guo L, Zhang L, Zhou X, et al. Regulation of TRPV5 transcription and expression by E2/ERα signalling contributes to inhibition of osteoclastogenesis. J Cell Mol Med (2018) 22(10):4738–50. doi: 10.1111/jcmm.13718
53. Chen F, OuYang Y, Ye T, Ni B, Chen A. Estrogen inhibits RANKL-induced osteoclastic differentiation by increasing the expression of TRPV5 channel. J Cell Biochem (2014) 115(4):651–8. doi: 10.1002/jcb.24700
54. Song S, Guo Y, Yang Y, Fu D. Advances in pathogenesis and therapeutic strategies for osteoporosis. Pharmacol Ther (2022) 237:108168. doi: 10.1016/j.pharmthera.2022.108168
55. Wu S-M, Shih L-H, Lee J-Y, Shen Y-J, Lee H-H. Estrogen enhances activity of wnt signaling during osteogenesis by inducing Fhl1 expression. J Cell Biochem (2015) 116(7):1419–30. doi: 10.1002/jcb.25102
56. Ji X, Chen X, Yu X. MicroRNAs in osteoclastogenesis and function: potential therapeutic targets for osteoporosis. Int J Mol Sci (2016) 17(3):349. doi: 10.3390/ijms17030349
57. Li X-LW L-Y, Wang G-S, Zhao H-Y. MiR-373 promotes the osteogenic differentiation of BMSCs from the estrogen deficiency induced osteoporosis. Eur Rev Med Pharmacol Sci (2019) 23 - N. 17:7247–55. doi: 10.26355/eurrev_201909_18827
58. Lin C, Yu S, Jin R, Xiao Y, Pan M, Pei F, et al. Circulating miR-338 cluster activities on osteoblast differentiation: potential diagnostic and therapeutic targets for postmenopausal osteoporosis. Theranostics (2019) 9(13):3780–97. doi: 10.7150/thno.34493
59. Xu R, Shen X, Xie H, Zhang H, Liu D, Chen X, et al. Identification of the canonical and noncanonical role of miR-143/145 in estrogen-deficient bone loss. Theranostics (2021) 11(11):5491–510. doi: 10.7150/thno.55041
60. Li T, Jiang H, Li Y, Zhao X, Ding H. Estrogen promotes lncRNA H19 expression to regulate osteogenic differentiation of BMSCs and reduce osteoporosis via miR-532-3p/SIRT1 axis. Mol Cell Endocrinol (2021) 527:111171. doi: 10.1016/j.mce.2021.111171
61. Guo L, Chen K, Yuan J, Huang P, Xu X, Li C, et al. Estrogen inhibits osteoclasts formation and bone resorption via microRNA-27a targeting PPARγ and APC. J Cell Physiol (2019) 234(1):581–94. doi: 10.1002/jcp.26788
62. Sugatani T, Hruska KA. Down-regulation of miR-21 biogenesis by estrogen action contributes to osteoclastic apoptosis. J Cell Biochem (2013) 114(6):1217–22. doi: 10.1002/jcb.24471
63. Nakamura T, Imai Y, Matsumoto T, Sato S, Takeuchi K, Igarashi K, et al. Estrogen prevents bone loss via estrogen receptor α and induction of fas ligand in osteoclasts. Cell (2007) 130(5):811–23. doi: 10.1016/j.cell.2007.07.025
64. Shen G, Ren H, Shang Q, Zhang Z, Zhao W, Yu X, et al. miR-128 plays a critical role in murine osteoclastogenesis and estrogen deficiency-induced bone loss. Theranostics (2020) 10(10):4334–48. doi: 10.7150/thno.42982
65. Nie X, Jin H, Wen G, Xu J, An J, Liu X, et al. Estrogen regulates duodenal calcium absorption through differential role of estrogen receptor on calcium transport proteins. Digestive Dis Sci (2020) 65(12):3502–13. doi: 10.1007/s10620-020-06076-x
66. Hart SM, Eastell R. Biochemical markers of bone turnover. Curr Opin Nephrol Hypertension (1999) 8(4):421–7. doi: 10.1097/00041552-199907000-00005
67. Gregson CL, Armstrong DJ, Bowden J, Cooper C, Edwards J, Gittoes NJL, et al. UK Clinical guideline for the prevention and treatment of osteoporosis. Arch Osteoporosis (2022) 17(1):58. doi: 10.1007/s11657-022-01061-5
68. LeBoff MS, Greenspan SL, Insogna KL, Lewiecki EM, Saag KG, Singer AJ, et al. The clinician’s guide to prevention and treatment of osteoporosis. Osteoporosis Int (2022) 33(10):2049–102. doi: 10.1007/s00198-021-05900-y
69. Belaya Z, Rozhinskaya L, Dedov I, Drapkina O, Fadeev V, Golounina O, et al. A summary of the Russian clinical guidelines on the diagnosis and treatment of osteoporosis. Osteoporosis Int (2023). 34, 429–447. doi: 10.1007/s00198-022-06667-6
70. Ling W, Krager K, Richardson KK, Warren AD, Ponte F, Aykin-Burns N, et al. Mitochondrial Sirt3 contributes to the bone loss caused by aging or estrogen deficiency. JCI Insight (2021) 6(10):e146728. doi: 10.1172/jci.insight.146728
71. Qin Y, Song D, Liao S, Chen J, Xu M, Su Y, et al. Isosinensetin alleviates estrogen deficiency-induced osteoporosis via suppressing ROS-mediated NF-κB/MAPK signaling pathways. Biomedicine Pharmacother (2023) 160:114347. doi: 10.1016/j.biopha.2023.114347
72. Chen K, Z-t Lv, Cheng P, Zhu W-t, Liang S, Yang Q, et al. Boldine ameliorates estrogen deficiency-induced bone loss via inhibiting bone resorption. Front Pharmacol (2018) 9. doi: 10.3389/fphar.2018.01046
73. Chen C, Wu M, Lei H, Cao Z, Wu F, Song Y, et al. A novel prenylflavonoid icariside I ameliorates estrogen deficiency-induced osteoporosis via simultaneous regulation of osteoblast and osteoclast differentiation. ACS Pharmacol Trans Sci (2023) 6(2):270–80. doi: 10.1021/acsptsci.2c00192
74. Xu Y, Song D, Lin X, Peng H, Su Y, Liang J, et al. Corylifol a protects against ovariectomized-induced bone loss and attenuates RANKL-induced osteoclastogenesis via ROS reduction, ERK inhibition, and NFATc1 activation. Free Radical Biol Med (2023) 196:121–32. doi: 10.1016/j.freeradbiomed.2023.01.017
75. Khan MI. Management of bone loss due to endocrine therapy during cancer treatment. Osteoporosis Int (2023) 34, 671–680, doi: 10.1007/s00198-023-06672-3
76. Shapiro CL, Poznak CV, Lacchetti C, Kirshner J, Eastell R, Gagel R, et al. Management of osteoporosis in survivors of adult cancers with nonmetastatic disease: ASCO clinical practice guideline. J Clin Oncol (2019) 37(31):2916–46. doi: 10.1200/JCO.19.01696
77. Antonini M, Salerno GR, Vieira ND, Cardoso MS, Solino M, Vasconcelos RNB, et al. Abstract P1-13-04: evaluation of the impact vitamin d and calcium supplementation on bone mineral density in breast cancer patients using or not taking aromatase inhibitors: 5-year follow-up. Cancer Res (2022) 82(4_Supplement):P1–13-04-P1-13-04. doi: 10.1158/1538-7445.SABCS21-P1-13-04
78. Wang Y, Ding H, Wang X, Wei Z, Feng S. Associated factors for osteoporosis and fracture in Chinese elderly. Med Sci Monit (2019) 25:5580–8. doi: 10.12659/MSM.914182
79. Sun K, Wang L, Ma Q, Cui Q, Lv Q, Zhang W, et al. Association between tea consumption and osteoporosis: a meta-analysis. Medicine (2017) 96(49):e9034. doi: 10.1097/MD.0000000000009034
80. Pasqualini L, Ministrini S, Lombardini R, Bagaglia F, Paltriccia R, Pippi R, et al. Effects of a 3-month weight-bearing and resistance exercise training on circulating osteogenic cells and bone formation markers in postmenopausal women with low bone mass. Osteoporosis Int (2019) 30(4):797–806. doi: 10.1007/s00198-019-04908-9
81. Chen L-R, Ko N-Y, Chen K-H. Medical treatment for osteoporosis: from molecular to clinical opinions. Int J Mol Sci (2019) 20(9):2213. doi: 10.3390/ijms20092213
82. Livi L, Scotti V, Desideri I, Saieva C, Cecchini S, Francolini G, et al. Phase 2 placebo-controlled, single-blind trial to evaluate the impact of oral ibandronate on bone mineral density in osteopenic breast cancer patients receiving adjuvant aromatase inhibitors: 5-year results of the single-centre BONADIUV trial. Eur J Cancer (2019) 108:100–10. doi: 10.1016/j.ejca.2018.12.005
83. Monda V, Lupoli GA, Messina G, Peluso R, Panico A, Villano I, et al. Improvement of bone physiology and life quality due to association of risedronate and anastrozole. Front Pharmacol (2017) 8. doi: 10.3389/fphar.2017.00632
84. Greenspan SL, Vujevich KT, Brufsky A, Lembersky BC, van Londen GJ, Jankowitz RC, et al. Prevention of bone loss with risedronate in breast cancer survivors: a randomized, controlled clinical trial. Osteoporosis Int (2015) 26(6):1857–64. doi: 10.1007/s00198-015-3100-7
85. Mugnier B, Goncalves A, Daumas A, Couderc A-L, Mezni E, Viret F, et al. Prevention of aromatase inhibitor–induced bone loss with anti-resorptive therapy in post-menopausal women with early-stage breast cancer. Osteoporosis Int (2023) 34, 703–711. doi: 10.1007/s00198-023-06683-0
86. Sheweita SA, Ammar RG, Sabra SA, Sultan AS. Letrozole and zoledronic acid changed signalling pathways involved in the apoptosis of breast cancer cells. J Taibah Univ Med Sci (2021) 16(1):112–20. doi: 10.1016/j.jtumed.2020.10.017
87. Waltman NL, Twiss JJ, Ott CD, Gross GJ, Lindsey AM, Moore TE, et al. The effect of weight training on bone mineral density and bone turnover in postmenopausal breast cancer survivors with bone loss: a 24-month randomized controlled trial. Osteoporosis Int (2010) 21(8):1361–9. doi: 10.1007/s00198-009-1083-y
88. Reid IR. Efficacy, effectiveness and side effects of medications used to prevent fractures. J Internal Med (2015) 277(6):690–706. doi: 10.1111/joim.12339
89. Yuan F, Peng W, Yang C, Zheng J. Teriparatide versus bisphosphonates for treatment of postmenopausal osteoporosis: a meta-analysis. Int J Surg (2019) 66:1–11. doi: 10.1016/j.ijsu.2019.03.004
90. Ellis GK, Bone HG, Chlebowski R, Paul D, Spadafora S, Smith J, et al. Randomized trial of denosumab in patients receiving adjuvant aromatase inhibitors for nonmetastatic breast cancer. J Clin Oncol (2008) 26(30):4875–82. doi: 10.1200/JCO.2008.16.3832
91. Gnant M, Pfeiler G, Dubsky PC, Hubalek M, Greil R, Jakesz R, et al. Adjuvant denosumab in breast cancer (ABCSG-18): a multicentre, randomised, double-blind, placebo-controlled trial. Lancet (2015) 386(9992):433–43. doi: 10.1016/S0140-6736(15)60995-3
92. Bundred N. Antiresorptive therapies in oncology and their effects on cancer progression. Cancer Treat Rev (2012) 38(6):776–86. doi: 10.1016/j.ctrv.2012.02.002
93. Dell’Aquila E, Armento G, Iuliani M, Simonetti S, D’Onofrio L, Zeppola T, et al. Denosumab for cancer-related bone loss. Expert Opin Biol Ther (2020) 20(11):1261–74. doi: 10.1080/14712598.2020.1814731
94. Kanis JA, Cooper C, Rizzoli R, Reginster JY, on behalf of the Scientific Advisory Board of the European Society for C, Economic Aspects of O, et al. European Guidance for the diagnosis and management of osteoporosis in postmenopausal women. Osteoporosis Int (2019) 30(1):3–44. doi: 10.1007/s00198-018-4704-5
95. Ettinger B, Black DM, Mitlak BH, Knickerbocker RK, Nickelsen T, Genant HK, et al. Reduction of vertebral fracture risk in postmenopausal women with osteoporosis treated with RaloxifeneResults from a 3-year randomized clinical trial. JAMA (1999) 282(7):637–45. doi: 10.1001/jama.282.7.637
96. Lainé M, Fanning SW, Chang Y-F, Green B, Greene ME, Komm B, et al. Lasofoxifene as a potential treatment for therapy-resistant ER-positive metastatic breast cancer. Breast Cancer Res (2021) 23(1):54. doi: 10.1186/s13058-021-01431-w
97. Kraenzlin ME, Meier C. Parathyroid hormone analogues in the treatment of osteoporosis. Nat Rev Endocrinol (2011) 7(11):647–56. doi: 10.1038/nrendo.2011.108
98. Kendler DL, Marin F, Zerbini CAF, Russo LA, Greenspan SL, Zikan V, et al. Effects of teriparatide and risedronate on new fractures in post-menopausal women with severe osteoporosis (VERO): a multicentre, double-blind, double-dummy, randomised controlled trial. Lancet (2018) 391(10117):230–40. doi: 10.1016/S0140-6736(17)32137-2
99. Liel Y. Teriparatide vs risedronate for osteoporosis. Lancet (2018) 391(10133):1895. doi: 10.1016/S0140-6736(18)30754-2
100. Ma L, Hua R, Tian Y, Cheng H, Fajardo RJ, Pearson JJ, et al. Connexin 43 hemichannels protect bone loss during estrogen deficiency. Bone Res (2019) 7(1):11. doi: 10.1038/s41413-019-0050-2
101. Huang X-H, Liang R-H, Su L, Guo W, Wang C-J. Mechanism of bushen jianpi decoction in preventing and treating osteoporosis caused by aromatase inhibitors in breast cancer treatment. Cancer Biomarkers (2017) 18:183–90. doi: 10.3233/CBM-160281
102. Jiayi L, Yuzhen P, Lijiang Z. Based on association rules and factor analysis to explore the rule of traditional Chinese medicine prescription for bone loss after endocrine therapy for breast cancer. Clin J Traditional Chin Med (2022) 34(07):1274–80. doi: 10.16448/j.cjtcm.2022.0720
103. Xin Z, Wu X, Yu Z, Shang J, Xu B, Jiang S, et al. Mechanisms explaining the efficacy of psoralidin in cancer and osteoporosis, a review. Pharmacol Res (2019) 147:104334. doi: 10.1016/j.phrs.2019.104334
104. Song J, Zhang Y, Zhu Y, Jin X, Li L, Wang C, et al. Structural characterization and anti-osteoporosis effects of polysaccharide purified from eucommia ulmoides Oliver cortex based on its modulation on bone metabolism. Carbohydr Polymers (2023) 306:120601. doi: 10.1016/j.carbpol.2023.120601
105. Zhang F, Li Q, Wu J, Ruan H, Sun C, Zhu J, et al. Total flavonoids of drynariae rhizoma improve glucocorticoid-induced osteoporosis of rats: UHPLC-MS-Based qualitative analysis, network pharmacology strategy and pharmacodynamic validation. Front Endocrinol (2022) 13. doi: 10.3389/fendo.2022.920931
106. Jin-long Z, Ling-feng Z, Gui-hong L, He-tao H, Wei-yi Y, Ming-hui L, et al. Research progress on mechanism of active components of Chinese materiamedica in treatment of osteoporosis based on signaling pathway. Chinese Traditional and Herbal Drugs (2020) 51(23):6084–94.
107. Scheidemann ER, Shajahan-Haq AN. Resistance to CDK4/6 inhibitors in estrogen receptor-positive breast cancer. Int J Mol Sci (2021) 22(22):12292. doi: 10.3390/ijms222212292
108. Singh A, Veeriah V, Xi P, Labella R, Chen J, Romeo SG, et al. Angiocrine signals regulate quiescence and therapy resistance in bone metastasis. JCI Insight (2019) 4(13):e125679. doi: 10.1172/jci.insight.125679
Keywords: breast cancer, endocrine therapy, estrogen, osteoporosis, aromatase inhibitors
Citation: Xu J, Cao B, Li C and Li G (2023) The recent progress of endocrine therapy-induced osteoporosis in estrogen-positive breast cancer therapy. Front. Oncol. 13:1218206. doi: 10.3389/fonc.2023.1218206
Received: 06 May 2023; Accepted: 23 June 2023;
Published: 07 July 2023.
Edited by:
Deniz Can Guven, Hacettepe University, TürkiyeReviewed by:
Sachin Chaugule, University of Massachusetts Medical School, United StatesAnjali P. Kusumbe, University of Oxford, United Kingdom
Copyright © 2023 Xu, Cao, Li and Li. This is an open-access article distributed under the terms of the Creative Commons Attribution License (CC BY). The use, distribution or reproduction in other forums is permitted, provided the original author(s) and the copyright owner(s) are credited and that the original publication in this journal is cited, in accordance with accepted academic practice. No use, distribution or reproduction is permitted which does not comply with these terms.
*Correspondence: Chunyu Li, chunyu_li@126.com; Guohui Li, lgh0603@cicams.ac.cn
†These authors have contributed equally to this work