- 1Faculty Technische Natuurwetenschappen (TNW), Group Nanobiophysics, Twente University, Enschede, Netherlands
- 2Department of Surgery, Netherlands Cancer Institute, Amsterdam, Netherlands
- 3Department of Pathology, Netherlands Cancer Institute, Amsterdam, Netherlands
- 4Faculty of Medicine, University of Iceland, Reykjavik, Iceland
- 5GROW School for Oncology and Developmental Biology, University of Maastricht, Maastricht, Netherlands
- 6Department of Biomedical Engineering and Physics, Amsterdam Cardiovascular Sciences, Cancer Center Amsterdam, Amsterdam Universitair Medisch Centrum (UMC), University of Amsterdam, Amsterdam, Netherlands
With the shift towards organ preserving treatment strategies in rectal cancer it has become increasingly important to accurately discriminate between a complete and good clinical response after neoadjuvant chemoradiotherapy (CRT). Standard of care imaging techniques such as CT and MRI are well equipped for initial staging of rectal tumors, but discrimination between a good clinical and complete response remains difficult due to their limited ability to detect small residual vital tumor fragments. To identify new promising imaging techniques that could fill this gap, it is crucial to know the size and invasion depth of residual vital tumor tissue since this determines the requirements with regard to the resolution and imaging depth of potential new optical imaging techniques. We analyzed 198 pathology slides from 30 rectal cancer patients with a Mandard tumor regression grade 2 or 3 after CRT that underwent surgery. For each patient we determined response pattern, size of the largest vital tumor fragment or bulk and the shortest distance from the vital tumor to the luminal surface. The response pattern was shrinkage in 14 patients and fragmentation in 16 patients. For both groups combined, the largest vital tumor fragment per patient was smaller than 1mm for 38% of patients, below 0.2mm for 12% of patients and for one patient as small as 0.06mm. For 29% of patients the vital tumor remnant was present within the first 0.01mm from the luminal surface and for 87% within 0.5mm. Our results explain why it is difficult to differentiate between a good clinical and complete response in rectal cancer patients using endoscopy and MRI, since in many patients submillimeter tumor fragments remain below the luminal surface. To detect residual vital tumor tissue in all patients included in this study a technique with a spatial resolution of 0.06mm and an imaging depth of 8.9mm would have been required. Optical imaging techniques offer the possibility of detecting majority of these cases due to the potential of both high-resolution imaging and enhanced contrast between tissue types. These techniques could thus serve as a complimentary tool to conventional methods for rectal cancer response assessment.
1 Introduction
Over the last decade, rectal cancer treatment has shifted towards organ preserving treatment, having the foremost advantage of improving the patient’s quality of life (1, 2). The standard-of-care for intermediate risk and locally advanced rectal cancer is neoadjuvant chemoradiotherapy (CRT) to reduce the size or extent of the tumor, followed by a total mesorectal excision (TME) where the rectum is surgically removed together with surrounding tissue and draining lymph nodes. Moreover, novel advances in rectal cancer treatment indicate the promising role of CRT in patients with bulky and distal tumors of the rectum, providing insights for organ preserving treatment in these complex tumors (3, 4). Since a TME often results in loss of organ function and considerable side effects, there is an increasing interest in organ-sparing treatment to improve the quality of life of patients. Patients with a good clinical response (defined as a near-complete or major response after CRT, with the possibility of residual tumor (5)) could receive additional local tumor treatment (e.g. a local tumor excision or internal boost radiation). In addition to CRT, novel advances in rectal cancer treatment have shown promising results with immunotherapy, especially in patients with microsatellite instable (MSI)-high tumors (6, 7).
In patients with a complete response (without any residual tumor) on the other hand non-operative management can be considered and these patients can be monitored according to watch-and-wait (W&W). After CRT, 20% of patients have a pathological complete response and 42-60% of patients have a good clinical response (8, 9). For organ-sparing treatment to become even more successful, it is important that clinicians can accurately identify the optimal treatment for each patient based on the degree of tumor response to CRT. However, the current workflow for response assessment has difficulty discriminating between patients with a good clinical response and a clinical complete response (10).
Response assessment is currently performed based on a combination of endoscopy, magnetic resonance imaging (MRI) and digital rectal examination. Endoscopic biopsies are only rarely used for initial response assessment because of frequent false positive results. Based on this clinical response assessment patients undergo further treatment or are enrolled in W&W. After surgery the resected tissue is analyzed by a pathologist, resulting in a pathological response assessment, which remains the gold standard. In approximately 15% of patients that are considered clinical incomplete responders, no residual tumor tissue is present upon histopathological evaluation of the resected specimen (11). These patients undergo major surgery where organ-sparing treatment could have been possible. Additionally, approximately 25% of patients thought to have responded completely based on a clinical evaluation still harbor unrecognized residual tumor (12). Based on the clinical evaluation these patients can be enrolled in W&W, but they developed a local regrowth requiring additional surgery (13). Improving the accuracy of response assessment thus holds the promise of improving treatment decisions and outcome for rectal cancer patients.
The difficulty of MRI to accurately assess tumor response can be explained by the fact that CRT can result in small tumor fragments scattered throughout fibrotic tissue (14). Not only does MRI have difficulty in discriminating between fibrosis and tumor tissue (15, 16) but a major concern in response assessment is also missing small fragments of residual vital tumor tissue, leading to the cautious strategy to perform major surgery whenever residual tumor tissue is suspected (17). CRT treatment can result in response patterns of either shrinkage or fragmentation of rectal tumors. Tumor shrinkage is characterized by a decrease in concentric tumor size, while fragmentation is defined by destruction of the main tumor mass after treatment and formation of small groups of tumor cells embedded in fibrosis. Fragmentation is reported in 40-80% of patients with rectal cancer (18, 19) and increases the chance of radiological understaging because of the difficulty of detecting small tumor fragments (20). While, to the best of our knowledge, the size distribution of tumor fragments in rectal cancer have not been published, a recent study showed that tumor fragment size in esophageal adenocarcinoma can be as small as several micrometers (18). With these dimensions, MR imaging lack resolution and accuracy in visualizing residual tumor fragments. Thus, to improve response assessment and treatment after CRT, an imaging technique is required that can detect small vital tumor fragments within fibrotic tissue.
To identify promising new techniques to improve response assessment in rectal cancer, one requirement placed on such a technique is that it can distinguish between vital tumor tissue and fibrosis. A second requirement is that the resolution needs to be high enough so it can detect small residual tumor fragments. While techniques like MRI can image the full body, for most other imaging techniques there is a trade-off between the resolution and imaging depth. Therefore, a third requirement is that a new technique needs to be able to image deep enough below the luminal surface so that it can detect residual vital tumor tissue in deeper tissue layers. While general response patterns in rectal cancer have been described in literature, no quantitative description of the size and depth distributions of residual tumor fragments after CRT has been given. Nonetheless, these quantitative measures potentially play a pivotal role in explaining why so many tumors are misclassified by current imaging methods and consequently could provide the theoretical framework more optimal imaging methods in the future. The aim of this study is to provide a quantitative histopathological description of the size and depth distributions of residual tumor tissue after CRT treatment which can be used to select promising new imaging techniques for response assessment based on their resolution and imaging depth.
2 Patients, materials and methods
2.1 Test cohort
Histological slides of rectal resection specimens from 30 patients with rectal tumors that had been treated with CRT and underwent rectal surgery were retrieved from the pathology archive at the Netherlands Cancer Institute. The original pathology report contained information on tumor regression grade (TRG), scored according to Mandard (21). Since we aimed to improve discrimination between a good clinical and a complete response, only cases with a substantial pathological response after CRT treatment were included - scored as Mandard TRG 2 (rare residual tumor cells and clusters scattered through fibrosis) or TRG 3 (increase in the number of residual tumor cells when compared to Mandard TRG 2, while fibrosis still predominates when compared to Mandard TRG 4).
This retrospective medical data/biospecimen study was carried out pursuant to Dutch legislation and international standards. Clinical information such as demographics and tumor characteristics were collected from the medical records (Table 1). Archival H&E slides were scanned using a PANNORAMIC® 1000 scanner from 3DHISTECH at a 40x magnification.
2.2 Assessment of tumor response
Indica Labs’ HALO software (v3.4.2986.185) (23) was used to classify tissue, normal mucosa, and tumor areas on the scanned histopathology slides, and to subsequently measure size and volume of tumor cell clusters and distances between tumor cell clusters. The DenseNet AI v2 plugin classifier was trained with 3 complete annotated slides, where a certified pathologist (JGvdB) annotated the full regions of background, normal mucosa, tumor and all other tissues. The classifier was trained for a total of 26345 iterations with a Cross-Entropy of 0.1. After training, performance of the classifier was verified by JGvdB in random slides included in this study. In total, 198 slides were examined, consisting of all H&E tumor slides per case.
First, we determined for each patient whether the response pattern was of fragmentation or shrinkage type. Tumor fragmentation was defined as clusters of cells which do not form a bulk and have at least 3 mm distance between fragments (18). If the response pattern was fragmentation, we measured the width (the short axis) of the widest tumor fragment per patient to be able to determine the ability of different imaging techniques to detect small fragments based on their resolution. We chose the width since that defines the required resolution – a long and narrow fragment of e.g. 3 by 0.05 mm would not be detected by a technique with a resolution of 1 mm. We chose the widest fragment per patient since the detection of only that fragment could already be enough to determine an incomplete response. In patients where the response pattern was of shrinkage type we measured the largest width of vital tumor tissue. For all patients we determined the shortest distance from the luminal surface to the vital tumor, in order to determine the ability of different imaging techniques to detect this residual tumor tissue based on the imaging depth. Finally, per patient we measured the tumor volume (based on all slides of a single patient) and the tumor area (based on the single slide with largest tumor area of each patient).
2.3 Statistical analysis
Statistical analysis was performed using IBM SPSS statistics v27 (SPSS Inc., United States). Normal distribution was assessed with the Shapiro-Wilk test. Statistical analysis for normally distributed data was performed with an unpaired t-test, and for non-normally distributed data using a Mann-Whitney test. A p-value ≤0.05 was considered statistically significant.
3 Results
3.1 Histopathological evaluation of tumor response
The HALO tissue classification algorithm identified tumor (red), normal mucosa (blue), other tissue types (green) and background (grey) in each histological slide (Figure 1), which was used for a quantitative analysis of the tumor response pattern (Table 2). Examples of the two main tumor response patterns, tumor fragmentation and shrinkage are shown in Figure 2.
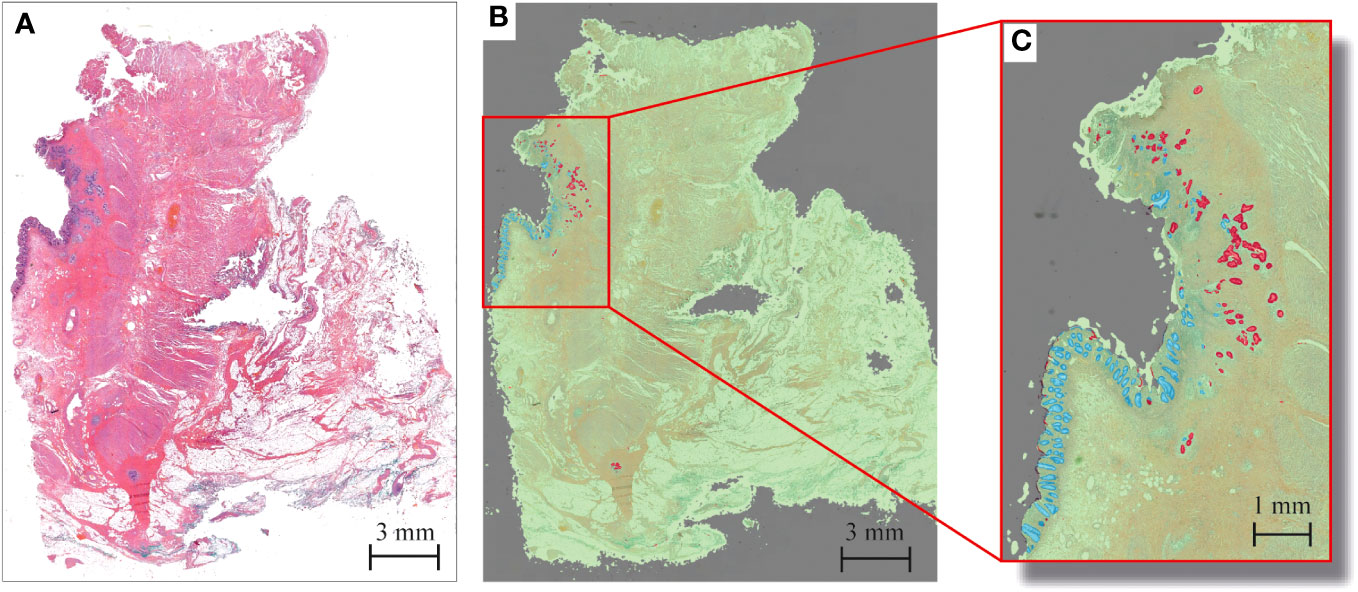
Figure 1 Example of tumor response segmentation by artificial intelligence HALO software in rectal cancer after CRT in a patient displaying Mandard TRG 2. (A) Haematoxylin and eosin (H&E) snapshot of tissue slide. (B) Corresponding tissue labels created by HALO tissue segmentation to enable easy tumor visualization. Red = tumor; blue = mucosa; green = other tissue; gray = background. (C) Zoom-in overview of tumor response pattern, with tumor fragments as small as several micrometers.
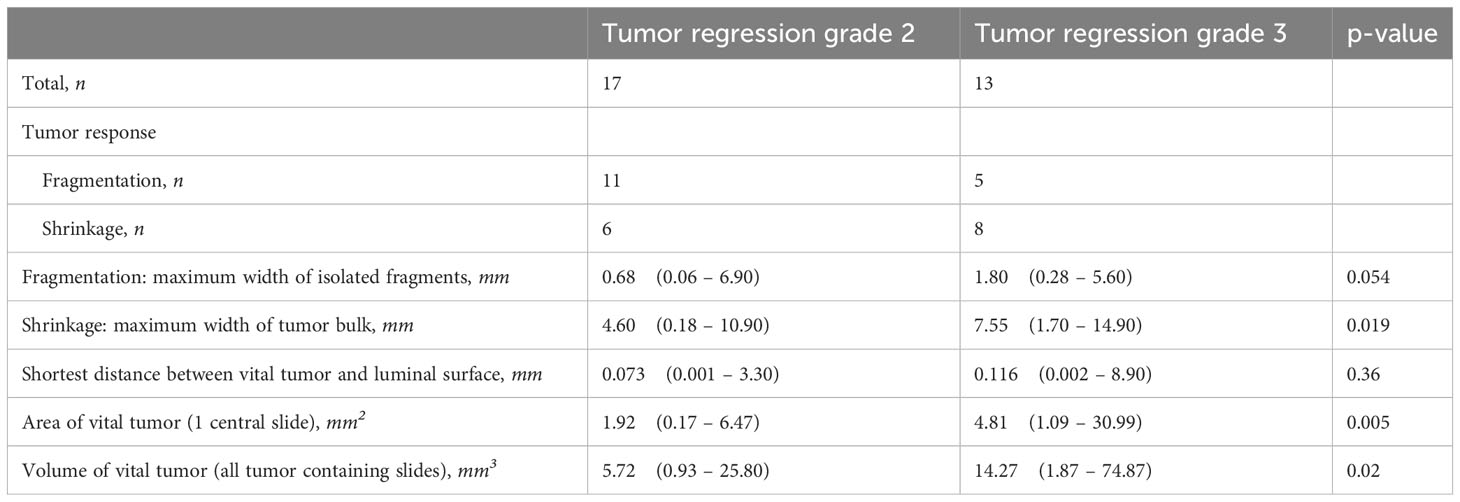
Table 2 Tumor response patterns based on quantitative analysis of histopathology slides. Data is presented as median with range (smallest to largest).
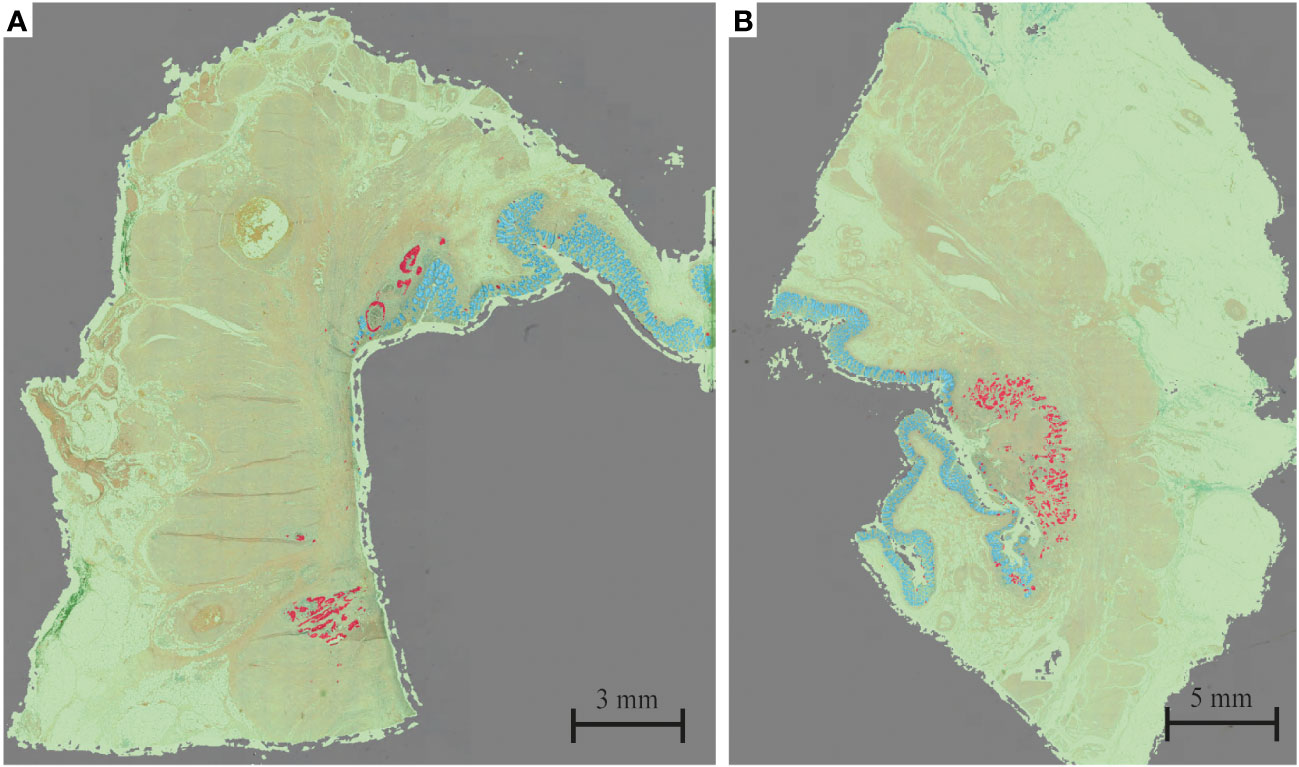
Figure 2 Example of (A) tumor fragmentation and (B) tumor shrinkage. Red = tumor; blue = mucosa; green = other tissue; gray = background.
Overall, the response pattern was of shrinkage type in 14 patients and of fragmentation type in 16 patients. For both groups combined, the largest vital tumor fragment per patient was smaller than 1 mm for 38% of patients, below 0.2 mm for 12% of patients and for one patient was as small as 0.06 mm. For 29% of patients residual vital tumor was present within the first 0.01 mm from the luminal surface and for 87% within the first 0.5 mm. In one patient there was 8.9 mm of healthy tissue between the residual vital tumor tissue and luminal surface. Moreover, invasion depth for both Mandard TRG 2 and TRG 3 were similarly distributed.
3.2 Tumor fragmentation
The response pattern was of fragmentation type in 65% and 38% in TRG 2 and TRG 3 cases, respectively. The median size of the widest isolated tumor fragments per patient was 0.68 mm for TRG 2 cases, and 1.80 mm in TRG 3 cases. In 63% of patients the widest fragments measured below 1.0 mm and 78% below 2.0 mm in size (Figure 3A). Residual tumor fragments were widely spread throughout the original tumor bed, encapsulated by fibrotic tissue. Individual tumor fragments could be as small as 0.06 mm.
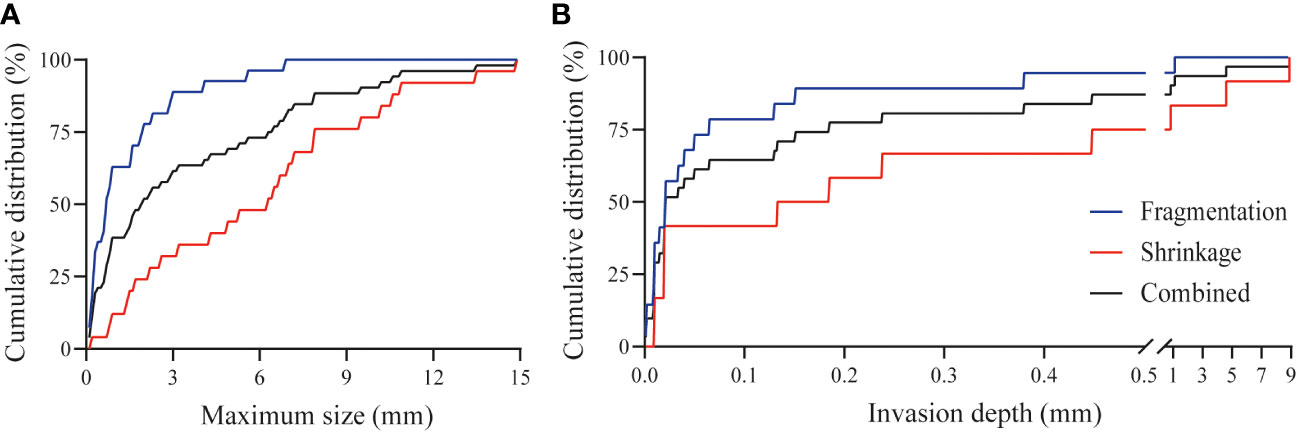
Figure 3 Tumor characteristics after CRT. (A) Cumulative distribution of the maximum width of tumor fragments in case of tumor fragmentation (n=16, blue line), tumor shrinkage (n=14, red line) and combined cohort (n=30, black line) after CRT. For both groups combined, the largest vital tumor fragment per patient was smaller than 1 mm for 38% of patients, below 0.2 mm for 12% of patients and for one patient was as small as 0.06 mm. (B) Cumulative distribution of the minimum invasion depth from the luminal surface in case of tumor fragmentation (n=16, blue line), tumor shrinkage (n=14, red line), and the combined cohort (n=30, black line) after CRT. For 29% of patients residual vital tumor was present within the first 0.01 mm from the luminal surface and for 87% within the first 0.5 mm. In one patient there was 8.9 mm of healthy tissue between the residual tumor tissue and the luminal surface.
3.3 Tumor shrinkage
The response pattern was of shrinkage type in 35% and 62% in TRG 2 and TRG 3 cases, respectively. The median value of the tumor width per patient was 4.60 mm and 7.55 mm in TRG 2 and TRG 3 cases, respectively. The spread of the width was very large, varying from several hundred micrometers to 1.5 centimeters. Detailed analysis showed that the width of the residual vital tumor bulk was smaller than 1.0 mm in 12% of cases, whereas 50% was smaller than 6.3 mm (Figure 3A).
3.4 Tumor invasion
Residual vital tumor was observed in all layers of the intestine. The most common location was the submucosa or muscle layers, within 1 mm of the mucosal lining of the rectum (ypT1-2), however, vital tumor fragments were also observed in the mesorectal tissue, and extending into other organs (ypT3-4). The tumor invasion depth varied between several micrometers and up to 8.9 mm from the mucosa. In case of fragmentation, residual tumor fragments were present within 0.5 mm from the luminal surface in 95% of cases, whereas for shrinkage this was less, i.e. 75% (Figure 3B).
4 Discussion
This study provides an overview of the quantitative histopathological characteristics of the size and depth distributions of residual vital tumor tissue after CRT treatment in rectal cancer. In our study population, 63% of patients with a TRG 2 response after CRT harbor residual vital tumor fragments of less than 1 mm. Importantly, vital tumor fragments were mostly present within 0.5 mm of the luminal surface, yet could also be located in the mesorectal tissue and extending to other organs. However, in case of a TRG 2 response of large primary tumors, vital tumor fragments were observed extending up to 8.9 mm from the luminal surface.
For years, assessing treatment response in rectal cancer has been investigated intensively, focusing on clinicopathological characteristics or biomarkers as predictors, with mixed results (24, 25). For that reason, alternative approaches for response assessment, such as (novel) optical imaging techniques, should be explored. Optical imaging techniques use light to obtain highly detailed images and signals of organs, tissues, cells or molecules in a minimally invasive or non-invasive way. Optical imaging techniques harbor many advantages, such as the capability of high resolutions, high specificity for set targets and feasibility for real-time imaging. Moreover, optical imaging has the additional benefit that they lack harmful radiation and can therefore be used repeatedly for monitoring of disease progression or treatment effect.
As illustrated in Figure 4, the demand for high resolutions (<1 mm) required to detect small tumor fragments can be achieved with optical imaging. Figure 4 presents only a small sample of available optical imaging techniques, each with their own specific biochemical or structural targets. For example, optical coherence tomography (OCT) uses the refractive properties of light waves in tissue to provide visualization of cross-sectional and 3D images of tissues (26), fluorescence lifetime imaging (FLIm) provides information about the biochemical composition of tissues by measuring the decay of fluorescent molecules (27) and photoacoustic imaging (PAI) utilizes laser-generated ultrasound waves to display tissue morphology and vasculature (28).
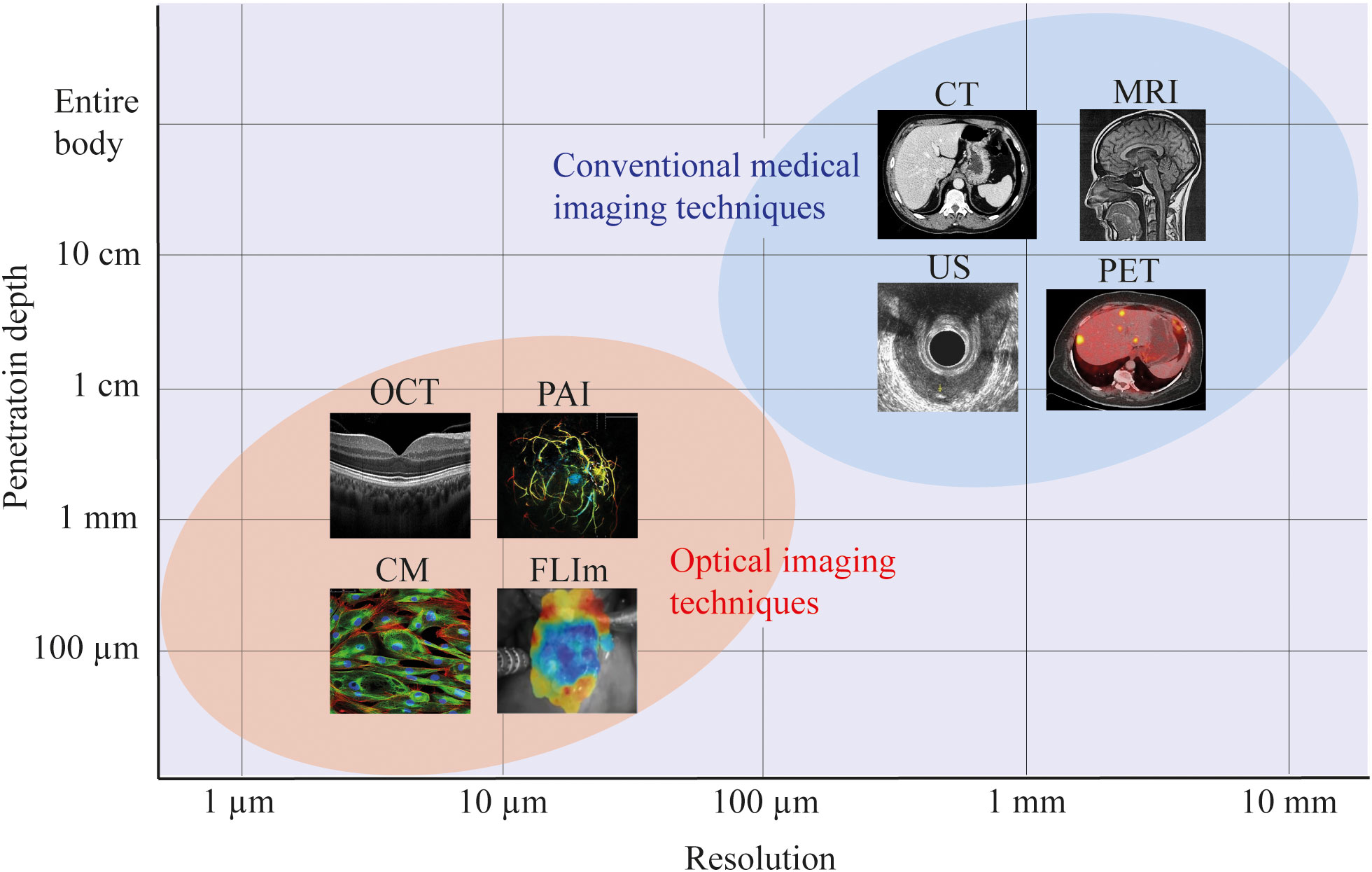
Figure 4 Resolution and penetration depths of several imaging modalities. For most optical imaging modalities there is a trade-off between the resolution and imaging depth, achieving more accurate resolutions, at the cost of penetration depth within the tissue of interest. In this figure, a schematic representation is used to indicate the approximate resolution and penetration depth of optical imaging versus conventional medical imaging modalities. For all imaging modalities, the exact resolution and penetration depth will depend on the specific setup. CM, confocal microscopy; FLIm, fluorescence lifetime imaging; OCT, optical coherence tomography; PAI, photoacoustic imaging; US, ultrasound; CT, computed tomography; MRI, magnetic resonance imaging; PET, positron emission tomography.
It is important to realize that there is an inherent trade-off between resolution and imaging depth. By selecting a technique with a higher resolution, the imaging depth will decrease. However, most optical imaging setups allow for interchanging these parameters, thereby selecting the desired resolution and imaging depths for a specific application. The results from our analysis offers the theoretical framework to evaluate the prospects of different optical imaging techniques. Moreover, challenges such as limitation in field of view, resolution (29) or feasibility for in vivo use (30, 31) have been addressed by multiple studies, providing solutions and opportunities for further research. For example, techniques as hyperspectral laparoscopes (32), confocal laser endomicroscopy (33) and tethered capsules (34) have indicated the potential of optical imaging in endoscopic use. Despite these advances, a commercially available optical imaging device is not yet available for tumor response assessment in rectal cancer. Hence, exploration of optical imaging techniques could be the way forward towards accurately defining treatment response assessment in rectal cancer patients. However, it is important to realize that implementation of any technique in the rectal cavity needs an optimized design for intended use.
While the results presented in this study show the potential for optical imaging in treatment response assessment, they also explain why it is difficult to discriminate between a good clinical and a complete response using conventional clinical examination. The foremost reason is that CRT can result in submillimeter residual tumor fragments below the resolution of MRI, and can be scattered throughout the intestinal tissue layers, rendering them invisible for endoscopy.
Currently, MRI is the golden standard for treatment response assessment in rectal cancer. While conventional MRI can achieve a resolution of approximately 1 mm (Figure 4), this is insufficient to identify the submillimeter tumor fragments demonstrated in the present study. Moreover, CRT-induced fibrosis in the tumor bed, replacing vital tumor, decreases the accuracy of MRI to detect residual viable tumor due to the lack of contrast between fibrosis and tumor fragments (35–37). As such, the dimension and distribution of tumor fragments, together with the surrounding fibrosis provides a big challenge for radiologists to accurately assess treatment response with MRI. Currently it is possible to improve this resolution using Ultra High Field (UHF) 7-9 Tesla machines, achieving a resolution below 0.5 mm (38). Even so, in 21% of our patients the largest tumor fragments was less than 0.5 mm. Furthermore, the problem of distinguishing fibrosis from tumor in these UHF scans remains. Another possibility for improving the diagnostic capability of MRI for response imaging of rectal cancer is the use of diffusion-weighted imaging (DWI). DWI offers the possibility to visualize a functional parameter utilizing the diffusion of water molecules within tissues. The advantage of DWI would be the possibility to increase the contrast between tumor and fibrosis. In assessment of breast and renal cancer response to CRT, DWI improved the evaluation of treatment response (39–41). However, the limiting factor of the resolution with respect to the small size of the fragments remains.
In addition to shrinkage and fragmentation of the tumor, in approximately 20% of patients undergoing CRT, microscopic intramural spread (MIS) is present (42), i.e. residual tumor extension beneath normal appearing mucosa. In clinical practice, MIS is commonly used for planning additional radiotherapy, or take into account when selecting the resection plane around the visible tumor. Whilst multiple studies have focused on retrospective assessing the MIS after CRT (42–44), intraoperative assessment remains challenging due to the limited size of the residual tumor fragments in most cases. Moreover, a tumor-positive circumferential resection margin (CRM) after CRT remains an important prognostic factor for local recurrence and overall survival, and can be as high as 31.8% (45). Hence, intraoperative assessment of the MIS potentially allows for more accurate selection of the resection plane, and (novel) optical imaging techniques could provide the tools to decrease tumor-positive CRM rates.
In this study, an AI algorithm was trained for labelling of residual tumor in pathology slides of rectal cancer. Such an algorithm enables the analysis of a large number of slides in detail. Moreover, the same analysis by a pathologist would have taken up a considerable amount of time. In future studies with (novel) imaging techniques, such an AI algorithm could provide key insights into the performance of these techniques by providing detailed histopathological information of the imaged tissue. Furthermore, our detailed analysis revealed that invasion depth of residual tumor fragments/bulk was not related to the Mandard TRG. However, most patients with a TRG 3 response displayed larger tumor volumes and larger tumor diameter than TRG 2 tumors. Thus, Mandard TRG is not only a measure of response, but can also be a measure of residual tumor burden.
There are some limitations to this study. First, 30 patients were included, resulting in 198 pathology slides. The novelty of the segmentation tool required us to manually check the performance of the segmentation in every segmented slice, resulting in a lower patient population. Secondly, an uncertainty remains about what portion of the residual tumor in the histological assessment is still vital and can result in a regrowth. However, in this study we assumed that our assessment of the vital portion of the tumor is right. Moreover, it is important to keep in mind that the AI algorithm was only trained on 3 completely annotated slides, while many more are needed optimizing the algorithm for general application. This could be seen as a limitation, since the trained AI for this study might lack robustness for independent analysis of histology slides. However, the AI was used as a tool for manual annotation, and not as a replacement for an experienced pathologist, and therefore the minimal training size was adequate for the application as used here. Furthermore, the quantitative measurements reported in this paper were verified manually to be accurate, and thus do not rely heavily on the AI for segmentation borders. Moreover, the AI performed well and no major adjustment had to be done to the segmentation once trained.
For successful organ-sparing treatment, it is crucial that clinicians can accurately identify the optimal treatment for each patient based on the response of the tumor to CRT. Resolutions of several micrometers are required for visualizing residual tumor fragments, and an imaging depth of several millimeters is essential for detecting fragments in all layers of the rectal wall. Moreover, it is important to realize that the histopathological characteristics of the tissue are paramount in the selection of an imaging technique. For in vivo application, it is important to realize that the imaging technique has to be implemented in an endorectal probe for optimal access to the tumor (46). Many optical imaging techniques have been transformed from table-top setups to endoscopic imaging probes, showing the potential of optical imaging techniques for colorectal response assessment (33, 34, 47–49). results presented in this study show that conventional imaging methods (mainly CT and MRI) lack the resolution for detecting residual vital tumor after CRT in rectal cancer, and hence have limited value in the therapeutic decision-making process around W&W in clinical practice. Our results, however, provide a theoretical basis for novel research in imaging techniques that can achieve the needed resolutions. Depending on the exact application, optical techniques have their own benefits over conventional CT and MR imaging.
To summarize, optical imaging techniques have the prospect of becoming a complimentary tool next to conventional methods for rectal cancer response assessment, since these techniques offer high-resolution imaging with enhanced contrast between tissue types.
Data availability statement
The raw data supporting the conclusions of this article will be made available by the authors, without undue reservation.
Ethics statement
The studies involving humans were approved by Institutional Review Board of the Netherlands Cancer Institute. The studies were conducted in accordance with the local legislation and institutional requirements. The ethics committee/institutional review board waived the requirement of written informed consent for participation from the participants or the participants’ legal guardians/next of kin because written informed consent was not required according IRB guidelines.
Author contributions
Study design: AP and TR. Data collection: SS, JB, PS, IS, BG, GB, and AP. Data interpretation: SS, JB, PS and AP. Writing manuscript: SS, AP, and MW. Reviewing manuscript: JB, PS, IS, BG, GB and TR. All authors read and approved the final manuscript.
Acknowledgments
We would like to acknowledge the NKI-AVL Core Facility Molecular Pathology & Biobanking (CFMPB) for supplying NKI-AVL Biobank material and/or lab support.
Conflict of interest
The authors declare that the research was conducted in the absence of any commercial or financial relationships that could be construed as a potential conflict of interest.
Publisher’s note
All claims expressed in this article are solely those of the authors and do not necessarily represent those of their affiliated organizations, or those of the publisher, the editors and the reviewers. Any product that may be evaluated in this article, or claim that may be made by its manufacturer, is not guaranteed or endorsed by the publisher.
References
1. Tekkis P, Tait D, Cunningham D, Brown G. Is organ preservation in rectal cancer ready for prime time? Lancet (2018) 391(10139):2480–2. doi: 10.1016/S0140-6736(18)31324-2
2. Grotenhuis BA, Beets GL. Watch-and-wait is an option in rectal cancer patients: from controversy to common clinical practice. Clin Oncol (2022) 35(2):124–9. doi: 10.1016/j.clon.2022.11.011
3. Bahadoer RR, Dijkstra EA, van Etten B, Marijnen CAM, Putter H, Kranenbarg EMK, et al. Short-course radiotherapy followed by chemotherapy before total mesorectal excision (TME) versus preoperative chemoradiotherapy, TME, and optional adjuvant chemotherapy in locally advanced rectal cancer (RAPIDO): a randomised, open-label, phase 3 trial. Lancet Oncol (2021) 22(1):29–42. doi: 10.1016/S1470-2045(20)30555-6
4. Fokas E, Schlenska-Lange A, Polat B, Klautke G, Grabenbauer GG, Fietkau R, et al. Chemoradiotherapy plus induction or consolidation chemotherapy as total neoadjuvant therapy for patients with locally advanced rectal cancer: long-term results of the CAO/ARO/AIO-12 randomized clinical trial. JAMA Oncol (2022) 8(1):1–10. doi: 10.1001/jamaoncol.2021.5445
5. Custers PA, Geubels BM, Beets GL, Triest Van B, Maas M. Defining near-complete response following (chemo) radiotherapy for rectal cancer: systematic review. Br J Surg (2022) 110(1):43–9. doi: 10.1093/bjs/znac372
6. Chen G, Jin Y, Guan W, Zhang R, Xiao W, Cai P, et al. Neoadjuvant PD-1 blockade with sintilimab in mismatch-repair deficient, locally advanced rectal cancer: an open-label, single-centre phase 2 study. Lancet Gastroenterol Hepatol (2023) 8(5):422–31. doi: 10.1016/S2468-1253(22)00439-3
7. Cercek A, Lumish M, Sinopoli J, Weiss J, Shia J, Lamendola-Essel M, et al. PD-1 blockade in mismatch repair–deficient, locally advanced rectal cancer. N Engl J Med (2022) 386(25):2363–76. doi: 10.1056/NEJMoa2201445
8. Maas M, Nelemans PJ, Valentini V, Das P, Rödel C, Kuo LJ, et al. Long-term outcome in patients with a pathological complete response after chemoradiation for rectal cancer: A pooled analysis of individual patient data. Lancet Oncol (2010) 11(9):835–44. doi: 10.1016/S1470-2045(10)70172-8
9. Ryan JE, Warrier SK, Lynch AC, Ramsay RG, Phillips WA, Heriot AG. Predicting pathological complete response to neoadjuvant chemoradiotherapy in locally advanced rectal cancer: A systematic review. Color Dis (2016) 18(3):234–46. doi: 10.1111/codi.13207
10. van der Sande ME, Beets GL, Hupkens BJ, Breukink SO, Melenhorst J, Bakers FC, et al. Response assessment after (chemo)radiotherapy for rectal cancer: Why are we missing complete responses with MRI and endoscopy? Eur J Surg Oncol (2019) 45(6):1011–7. doi: 10.1016/j.ejso.2018.11.019
11. Haak HE, Maas M, Trebeschi S, Beets-Tan RGH. Modern MR imaging technology in rectal cancer; there is more than meets the eye. Front Oncol (2020) 10:1–7. doi: 10.3389/fonc.2020.537532
12. van der Valk MJM, Hilling DE, Bastiaannet E, Meershoek-Klein Kranenbarg E, Beets GL, Figueiredo NL, et al. Long-term outcomes of clinical complete responders after neoadjuvant treatment for rectal cancer in the International Watch & Wait Database (IWWD): an international multicentre registry study. Lancet (2018) 391(10139):2537–45. doi: 10.1016/S0140-6736(18)31078-X
13. Deidda S, Elmore U, Rosati R, De Nardi P, Vignali A, Puccetti F, et al. Association of delayed surgery with oncologic long-term outcomes in patients with locally advanced rectal cancer not responding to preoperative chemoradiation. JAMA Surg (2021) 156(12):1141–9. doi: 10.1001/jamasurg.2021.4566
14. Nagtegaal ID, Glynne-Jones R. How to measure tumour response in rectal cancer? An explanation of discrepancies and suggestions for improvement. Cancer Treat Rev (2020) 84:101964. doi: 10.1016/j.ctrv.2020.101964
15. Beets-Tan RGH, Lambregts DMJ, Maas M, Bipat S, Barbaro B, Curvo-Semedo L, et al. Magnetic resonance imaging for clinical management of rectal cancer: Updated recommendations from the 2016 European Society of Gastrointestinal and Abdominal Radiology (ESGAR) consensus meeting. Eur Radiol (2018) 28(4):1465–75. doi: 10.1007/s00330-017-5026-2
16. Barbaro B, Fiorucci C, Tebala C, Valentini V, Gambacorta MA, Vecchio FM, et al. Locally advanced rectal cancer: MR imaging in prediction of response after preoperative chemotherapy and radiation therapy. Radiology (2009) 250(3):730–9. doi: 10.1148/radiol.2503080310
17. Maas M, Lambregts DMJ, Nelemans PJ, Heijnen LA, Martens MH, Leijtens JWA, et al. Assessment of clinical complete response after chemoradiation for rectal cancer with digital rectal examination, endoscopy, and MRI: selection for organ-saving treatment. Ann Surg Oncol (2015) 22(12):3873–80. doi: 10.1245/s10434-015-4687-9
18. Graham Martinez C, Kus Öztürk S, Al-Kaabi A, Valkema MJ, Bokhorst JM, Rosman C, et al. Shrinkage versus fragmentation response in neoadjuvantly treated oesophageal adenocarcinoma: significant prognostic relevance. Histopathology (2022) 80, 982–94. doi: 10.1111/his.14644
19. Gosens MJEM, Klaassen RA, Tan-Go I, Rutten HJT, Martijn H, Van Den Brule AJC, et al. Circumferential margin involvement is the crucial prognostic factor after multimodality treatment in patients with locally advanced rectal carcinoma. Clin Cancer Res (2007) 13(22):6617–23. doi: 10.1158/1078-0432.CCR-07-1197
20. Fernández-Aceñero MJ, Muñoz LE, Varela JS, Sánchez JAC, del Arco CD, Paredes BG, et al. Prognostic influence of histopathological regression patterns in rectal adenocarcinoma receiving neoadjuvant therapy. J Gastrointest Oncol (2017) 8(1):49–54. doi: 10.21037/jgo.2017.01.02
21. Mandard A -M, Dalibard F, Mandard J -C, Marnay J, Henry-Amar M, Petiot J -F, et al. Pathologic assessment of tumor regression after preoperative chemoradiotherapy of esophageal carcinoma. Clinicopathologic correlations. Cancer. (1994) 73(11):2680–6.
22. ClinicalTrials.gov. Neoadjuvant Treatment in Rectal Cancer With Radiotherapy Followed by Atezolizumab and Bevacizumab. United States: TARZAN (2019).
23. Sobottka B, Nowak M, Frei AL, Haberecker M, Merki S, Aebersold R, et al. Establishing standardized immune phenotyping of metastatic melanoma by digital pathology. Lab Investig (2021) 101(12):1561–70. doi: 10.1038/s41374-021-00653-y
24. Fischer J, Eglinton TW, Richards SJG, Frizelle FA. Predicting pathological response to chemoradiotherapy for rectal cancer: a systematic review. Expert Rev Anticancer Ther [Internet]. (2021) 21(5):489–500. doi: 10.1080/14737140.2021.1868992
25. Huh JW, Kim HR, Kim YJ. Clinical prediction of pathological complete response after preoperative chemoradiotherapy for rectal cancer. Dis Colon Rectum. (2013) 56(6):698–703. doi: 10.1097/DCR.0b013e3182837e5b
26. Aumann S, Donner S, Fischer J, Müller F. Optical coherence tomography (OCT): principle and technical realization. In: High Resolution Imaging in Microscopy and Ophthalmology, vol. p. United States: Springer, Cham (2019). p. 59–85.
27. Datta R, Heaster TM, Sharick JT, Gillette AA, Skala MC. Fluorescence lifetime imaging microscopy: fundamentals and advances in instrumentation, analysis, and applications. J BioMed Opt. (2020) 25(07):1. doi: 10.1117/1.JBO.25.7.071203
28. Chapman WC, Mutch M. Co-registered photoacoustic and ultrasound imaging of human colorectal cancer. J BioMed Opt. (2019) 24(12):1. doi: 10.1117/1.JBO.24.12.121913
29. Zhang B, Hou W, Jin G, Zhu J. Simultaneous improvement of field-of-view and resolution in an imaging optical system. Opt Express. (2021) 29(6):9346. doi: 10.1364/OE.420222
30. Yao L, Zhou Y, Liu K, Yin X, Deng X, Ding Z, et al. Endoscopic OCT angiography using clinical proximal-end scanning catheters. Photonics (2022) 9(5):329. doi: 10.3390/photonics9050329
31. Luo H, Li S, Zeng Y, Cheema H, Otegbeye E, Ahmed S, et al. Human colorectal cancer tissue assessment using optical coherence tomography catheter and deep learning. J Biophotonics (2022) 15(6):1–8. doi: 10.1002/jbio.202100349
32. Pfahl A, Köhler H, Thomaßen MT, Maktabi M, Bloße AM, Mehdorn M, et al. Clinical evaluation of a laparoscopic hyperspectral imaging system. Surg Endosc (2022) 36(10):7794–9. doi: 10.1007/s00464-022-09282-y
33. Pilonis ND, Januszewicz W, di Pietro M. Confocal laser endomicroscopy in gastro-intestinal endoscopy: Technical aspects and clinical applications. Transl Gastroenterol Hepatol (2022) 7(September 2019):1–20. doi: 10.21037/tgh.2020.04.02
34. Liang K, Ahsen OO, Murphy A, Zhang J, Nguyen TH, Potsaid B, et al. Tethered capsule en face optical coherence tomography for imaging Barrett’s oesophagus in unsedated patients. BMJ Open Gastroenterol (2020) 7(1):1–8. doi: 10.1136/bmjgast-2020-000444
35. Van Der Paardt MP, Zagers MB, Beets-Tan RGH, Stoker J, Bipat S. Patients who undergo preoperative chemoradiotherapy for locally advanced rectal cancer restaged by using diagnostic MR imaging: A systematic review and meta-analysis. Radiology (2013) 269(1):101–12. doi: 10.1148/radiol.13122833
36. Tan CH. Use of computed tomography in the management of colorectal cancer. World J Radiol (2010) 2(5):151. doi: 10.4329/wjr.v2.i5.151
37. Cote A, Florin GF, Mois E, Elisei R, Badea R, Mare C, et al. The accuracy of endorectal ultrasonography and high-resolution magnetic resonance imaging for restaging rectal cancer after neoadjuvant chemoradiotherapy. Ann Ital Chir. (2018) 89:168–76.
38. Stucht D, Danishad KA, Schulze P, Godenschweger F, Zaitsev M, Speck O. Highest resolution in vivo human brain MRI using prospective motion correction. PloS One (2015) 10(7):1–17. doi: 10.1371/journal.pone.0133921
39. Belli P, Costantini M, Ierardi C, Bufi E, Amato D, Mule’ A, et al. Diffusion-weighted imaging in evaluating the response to neoadjuvant breast cancer treatment. Breast J (2011) 17(6):610–9. doi: 10.1111/j.1524-4741.2011.01160.x
40. Ahmed SA, Taher MGA, Ali WA, Ebrahem MAES. Diagnostic performance of contrast-enhanced dynamic and diffusion-weighted MR imaging in the assessment of tumor response to neoadjuvant therapy in muscle-invasive bladder cancer. Abdom Radiol (2021) 46(6):2712–21. doi: 10.1007/s00261-021-02963-7
41. Akkavak Palazalı G, Yılmaz R, Palazalı O, Dursun M. Magnetic resonance imaging evaluation of pathological response in breast cancer after neoadjuvant chemotherapy. Indian J Surg (2022) 85:39–44. doi: 10.1007/s12262-022-03337-z
42. Verrijssen AS, Guillem J, Perez R, Bujko K, Guedj N, Habr-Gama A, et al. Microscopic intramural extension of rectal cancer after neoadjuvant chemoradiation: A meta-analysis based on individual patient data. Radiother Oncol (2020) 144:37–45. doi: 10.1016/j.radonc.2019.10.003
43. Guedj N, Maggiori L, Poté N, Norkowski E, Cros J, Bedossa P, et al. Distal intramural and tumor spread in the mesorectum after neoadjuvant radiochemotherapy in rectal cancer: About 124 consecutive patients. Hum Pathol (2016) 52:164–72. doi: 10.1016/j.humpath.2016.01.017
44. Sun G, Ye X, Zheng K, Zhang H, Broens P, Trzpis M, et al. Measurement of distal intramural spread and the optimal distal resection by naked eyes after neoadjuvant radiation for rectal cancers. World J Surg Oncol (2022) 20(1):1–10. doi: 10.1186/s12957-022-02756-2
45. Detering R, Rutgers MLW, Bemelman WA, Hompes R, Tanis PJ. Prognostic importance of circumferential resection margin in the era of evolving surgical and multidisciplinary treatment of rectal cancer: A systematic review and meta-analysis. Surg (United States) (2021) 170(2):412–31. doi: 10.1016/j.surg.2021.02.029
46. Plekhanov AA, Sirotkina MA, Gubarkova EV, Kiseleva EB, Sovetsky AA, Karabut MM, et al. Towards targeted colorectal cancer biopsy based on tissue morphology assessment by compression optical coherence elastography. Front Oncol (2023) 13(March):1–15. doi: 10.3389/fonc.2023.1121838
47. Li Y, Zhu Z, Chen JJ, Jing JC, Sun C-H, Kim S, et al. Multimodal endoscopy for colorectal cancer detection by optical coherence tomography and near-infrared fluorescence imaging. BioMed Opt Express. (2019) 10(5):2419. doi: 10.1364/BOE.10.002419
48. Elena ET, Gheonea DI, Săftoiu A. Advances in endoscopic ultrasound imaging of colorectal diseases. World J Gastroenterol (2016) 22(5):1756–66. doi: 10.3748/wjg.v22.i5.1756
Keywords: tumor response, regression, rectal cancer, fragmentation, neoadjuvant, optical imaging, watch-and-wait
Citation: van der Stel SD, van den Berg JG, Snaebjornsson P, Seignette IM, Witteveen M, Grotenhuis BA, Beets GL, Post AL and Ruers TJM (2023) Size and depth of residual tumor after neoadjuvant chemoradiotherapy in rectal cancer – implications for the development of new imaging modalities for response assessment. Front. Oncol. 13:1209732. doi: 10.3389/fonc.2023.1209732
Received: 21 April 2023; Accepted: 21 August 2023;
Published: 05 September 2023.
Edited by:
Marco Rengo, Sapienza University of Rome, ItalyReviewed by:
Davide Ciardiello, University of Campania Luigi Vanvitelli, ItalyAnton A. Plekhanov, Privolzhsky Research Medical University (PIMU), Russia
Copyright © 2023 van der Stel, van den Berg, Snaebjornsson, Seignette, Witteveen, Grotenhuis, Beets, Post and Ruers. This is an open-access article distributed under the terms of the Creative Commons Attribution License (CC BY). The use, distribution or reproduction in other forums is permitted, provided the original author(s) and the copyright owner(s) are credited and that the original publication in this journal is cited, in accordance with accepted academic practice. No use, distribution or reproduction is permitted which does not comply with these terms.
*Correspondence: Stefan D. van der Stel, s.vd.stel@nki.nl