- Department of Clinical Laboratory Medicine, Xijing Hospital, Fourth Military Medical University, Xi’an, Shaanxi, China
Liquid biopsy as a non-invasive method has a bright future in cancer diagnosis. Tumor-related extracellular vesicles (EVs) and their components (nucleic acids, proteins, and lipids) in biofluids may exert multiple functions in tumor growth, metastasis, immune escape, and angiogenesis. Among all the components, nucleic acids have attracted the most interest due to their simplicity of extraction and detection. In this review, the biological functions of EVs in prostate cancer (PCa) genesis and progression were summarized. Moreover, the diagnostic value of EV RNA markers found in clinical body fluid samples was reviewed, including their trends, challenging isolation methods, and diagnostic efficacy. Lastly, because relatively much progress has been made in PCa, studies on EV DNA markers are also discussed.
1 Introduction
As a new and minimally invasive method, liquid biopsy is aimed at isolating genetic material from biological fluids for tumor diagnosis. It is promising to overcome the invasive sampling and heterogeneity problems of tissue biopsy to achieve better screening and monitoring of tumor recurrence risk. As one of the three targets of liquid biopsy, these lipid-bilayered vesicles with cellular origins are released into the extracellular region by a variety of cells, including cancer cells. Extracellular vesicles (EVs) can be categorized into exosomes, microvesicles, and apoptotic bodies according to their size, origin, morphology, and mode of release. Tumor-derived EVs are thought to mediate intracellular communication as “horizontal” transfer cargo and reflect their cell-type origin, suggesting they could offer a good source of novel tumor diagnosis biomarkers.
EVs are exciting in a vast range of biofluids (blood, urine, bronchoalveolar lavage fluid, ascites and pleural effusion, breast milk, saliva, etc.) and contain a myriad of functional biomolecules (proteins, lipids, RNA, DNA). Considering EVs in biological fluids generally reflect their parent cells’ information, their unique composition could assist in cancer detection. Compared to lipids and proteins, the easy-to-detect nature of nucleic acids makes them an attractive source for clinical application (1). RNA, in particular, has been the most exploited because it is protected from nucleases by lipid bilayers and is easier to detect using PCR and next-generation sequencing (NGS). Another component of nucleic acids, DNA, has been less studied but has gained more attention in recent years.
Prostate cancer (PCa) has the highest incidence of male genitourinary malignancy and is the most common cancer in Western men. It is well known that the routine screening method prostate-specific antigen (PSA) has many limitations due to its poor specificity. For example, in addition to the elevation in PCa, PSA also increases during anal digital examination, catheter insertion, prostate inflammation, and hypertrophy (2). Therefore, novel, noninvasive, and highly specific diagnostic biomarkers are highly needed. Although it is controversial whether the number of EVs in tumor patients is increased relative to healthy controls, the reported PCa studies are relatively consistent in suggesting that the secretion of EVs in PCa patients is five to 10 times higher than that in healthy subjects or patients with benign prostatic hyperplasia (BPH) (3–5). As an androgen-dependent tumor, androgen deprivation therapy (ADT) is the main standard treatment for PCa and the basis for the combination of various treatment options. Studies have shown that after ADT, EVs isolated from the urine of PCa patients or cell culture supernatant were approximately two- to threefold decreased (6). In terms of quantity, all this evidence suggests the potential of EVs for diagnosis and treatment efficacy prediction for PCa. Therefore, this study took PCa as the object to review the diagnostic value of EV RNA markers found based on clinical body fluid samples, as well as the relevant progress of PCa EV DNA markers in recent years.
2 The biological function of EVs in PCa
The potential of EVs for clinical application may be determined by their biological function. The education function of tumor-related EVs to target cells is through the horizontal transfer of carried biomolecules, which is involved in the modulation of cancer metabolism and the microenvironment. Several studies have confirmed that intercellular transfer of EV cargo changes the phenotype of recipient cells and contributes to PCa occurrence and development. A model of the action mechanism is shown in Figure 1, and the literature related to EV function is summarized in Table 1.
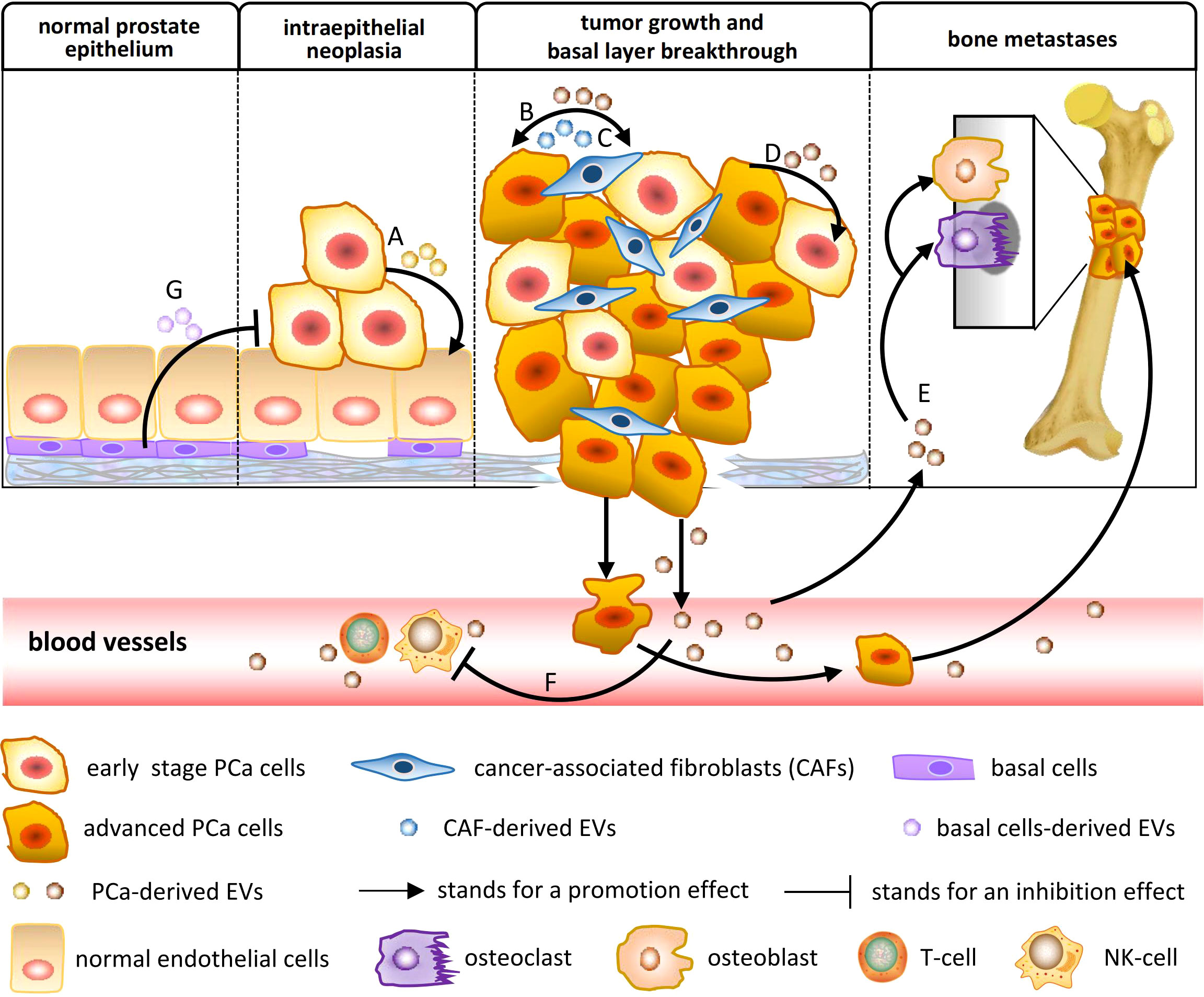
Figure 1 The biological function of EVs in PCa occurrence and development. (A) PCa-derived EVs mediate epithelial–mesenchymal transition (EMT), increasing the migration and invasion of noncancerous prostate epithelial cells. (B) PCa-derived EVs triggered fibroblast differentiation to a distinctive myofibroblast phenotype, resembling stromal cells isolated from PCa tissue, which support angiogenesis in vitro and accelerate tumor growth in vivo. (C) Cancer-associated fibroblast (CAF)-derived EVs can reprogram the metabolic machinery of PCa, supplying metabolomics for PCa cells and promoting tumor growth. (D) PCa-derived EVs confer prosurvival signals to alter the phenotype of other PCa cells in their surrounding environment, including reducing apoptosis, increasing proliferation, inducing migration, and transferring resistance. (E) PCa-derived EVs regulate the bone microenvironment by affecting osteoblast and osteoclast differentiation, which facilitates PCa cell proliferation and invasion. (F) PCa-derived EVs promote immune suppression and tumor escape through the downregulation of the cytotoxic response of cellular immunity. (G) Adipose-derived stromal cell (APS) is known as one of the mesenchymal stem cells (MSCs). APS-derived EVs could inhibit metastatic PCa progression.
At present, a few studies have suggested that the nucleic acid component of EVs could be useful for developing novel biomarkers. In one such study, Hessvik et al. evaluated EVs derived from the cell culture medium of PCa epithelial cell lines PC-3 and the human prostate (noncancer) epithelial cell line RWPE-1. Microarray identified 26 unique miRNAs in the EVs of RWPE-1, 20 unique miRNAs in the EVs of PC-3, and 362 overlapping miRNAs between the two (20). These unique EV miRNAs from noncancer and PCa cell lines could lead to the discovery of novel biomarkers. Furthermore, lots of comparison studies were conducted between different PCa clinical conditions such as aggressiveness [Gleason Score (GS)], metastasis, androgen resistance, and response to antitumor drugs (3, 21). These results demonstrated that EV RNA expression patterns can be related to specific PCa clinical situations, fueling the hope to find more valuable biomarkers to distinguish tumor subtypes and predict treatment response. The other EV nucleic acid component, DNA, was first observed in 2014; it was found later and got less attention than EV RNA (22). In addition, using PCa as a model, it has been proven that in different subtypes of EVs, DNA components are mainly encapsulated in large EVs (1~10 μm diameter) (23). In this review, we systematically summarize studies investigating EV nucleic acids (RNA and DNA) in biofluids of prostate cancer patients and discuss the utility of the identified biomarkers in various clinical scenarios. Furthermore, we outline the biological questions and technical challenges that have arisen from these studies.
3 EV RNA biomarker in PCa
At present, due to the simplicity of isolation and detection, nucleic acids have attracted the most interest in EV content. Under such circumstances, research on tumor miRNA marker screening has gradually turned from free-circulating miRNAs to EV-encapsulated miRNAs in body fluids. Compared to free circulating RNA in body fluids, EV RNA has advantages such as: (i) EV double-layer lipid membrane could protect RNA against nuclease degradation (24), which allows for higher level and easier detection; (ii) EVs selectively encapsulate RNA. Matthew et al. have demonstrated the selective sorting of miRNAs into EVs (25). In PCa, Hessvik et al. identified 31 miRNAs only detected in EVs through a comparison of the miRNA profile of EVs vs. parent cells using a PC-3 cell line (20). (iii) For urological tumor diagnosis, urine supplies a noninvasive approach to detect tumor-derived biomarkers. Among the known forms of miRNA packaging, EV-enclosed miRNA is thought to overcome urinary impurity interference and reflect the vital activity of cancer cells. A urine sample is easier to get than blood, has a larger volume and less damage, and may truly be “noninvasive”. In addition, prior prostate massage has been demonstrated to enhance the efficiency of urinary EV detection (26–28).
Based on deep sequencing analysis of the EV RNA subtypes, pioneering work has identified mature miRNA as having the highest proportion, ranging from 29% to 44% of the total EV RNA, with long noncoding RNA (lncRNA) and mRNA accounting for most of the remaining EV RNA (29–31). For RNA marker screening, comparisons have most commonly been made between different cell lines (for example, androgen-sensitive vs. androgen-independent; less aggressive vs. aggressive, etc.) or between different clinical conditions (health control or BPH vs. PCa patients, localized PCa vs. metastatic PCa, castration-sensitive vs. castration-resistant PCa, nonrecurrent PCa vs. recurrent PCa, etc.). Quite all studies demonstrate that such specific cultures or clinical situations can be related to specific expression patterns, and EV RNA biomarker specificity increases with the development of tumors. Therefore, the diagnostic potential of EV RNA markers has received extensive attention. Many studies used a comparison between clinical sample groups to screen EV RNA markers with significant expression differences. However, the high heterogeneity among single papers was relevant to sample type, EV separation method, and specimen size, which concluded that only a few RNAs, such as miR-141, miR-375, or PCA3, had a common trend and most were different. In order to gain the whole picture of the reported PCa RNA biomarkers, we summarized the published screening studies to show the biomarker types, expression trends, experimental design, and conclusions, as shown in Table 2. It should be noted that the samples we used here for comparison were all derived from patients’ blood or urine and did not contain cell culture supernatant, although there was also a study comparing EV miRNA profiles between PCa cell lines (PC-3) and noncancerous lines (RWPE-1) cultured in vitro (20).
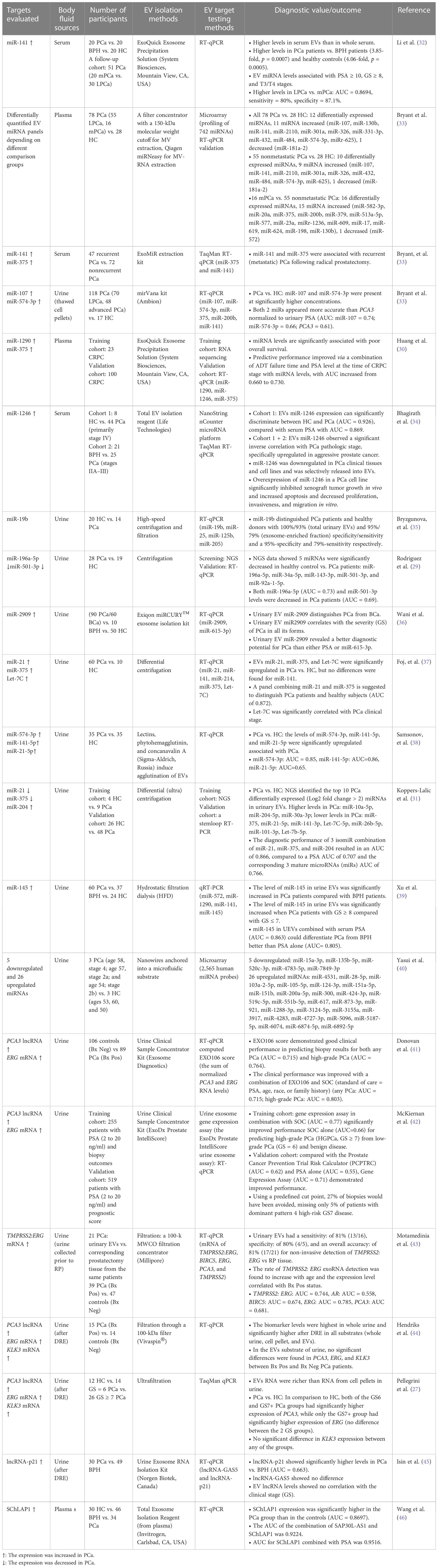
Table 2 Summary of clinical studies investigating EV nucleic acid targets as potential PCa biomarkers.
From a clinical perspective, 90% of the studies (19/21) have focused on the early diagnosis of PCa, which aims to distinguish PCa patients from BPH or health control. Only two studies have been designed to predict hormone resistance (30, 33). In fact, this is inconsistent with the actual needs of PCa diagnosis and treatment, such as distinguishing PCa with high metastasis risk and predicting the progression to CRPC. The main reason for taking the easy way for screening is the difficulty of collecting accurately grouped specimens in advanced PCa. One recent study focused on the differences between different PCa stages. They compared one case each in the organ-confined PC (OC) group, the extracapsular-extending PC (EC) group, and the seminal vesicle-invading PC (SI) group with healthy controls. The selected mRNAs and miRNAs with the highest change folds were different, indicating the discrimination potential related to PCa progression (47). Considering that the sample size was too small, the study was not included in Table 2.
From a methodological point of view, after the potential EV RNA markers were screened out through NGS or microarrays, RT-qPCR was usually adopted for further validation. The final diagnostic performance was shown in the receiver operating characteristic curve (ROC) and area under the curve (AUC). In general, the promising PCa early detection EV miRNA biomarkers include miR-141, miR-375, and miR-1246 from blood (serum or plasma) and miR-141, miR-375, Let-7C, miR-2909, miR-145, miR-21, miR-574-3p, miR-19b, miR-196a-5p, and miR-501-3p from urine. With better sampling potential than blood, urinary EVs have a broader RNA biomarker spectrum with mRNA of ERG, TMPRSS2:ERG genes (27, 41–44), and lncRNA PCA3 and p21 (27, 41, 42, 44, 45). However, up to now, no higher-level evidence has been found in studies on PCa EV RNA markers in either blood or urine samples, such as meta-analysis, which is also the premise for further clinical transformation. After all, the ultimate goal of tumor biomarker screening is not just for research, but for clinical detection.
Notably, based on data from a single study, PCa is the cancer type that is at the forefront of clinical transformation. In 2016, Exosome Diagnostics company passed FDA approval and launched the first PCa EV diagnostic product ExoDx® Prostate (IntelIScore), which was certificated to have a good clinical performance (AUC = 0.77). It is also one of the only two available commercial EV kits for tumor detection until now. The other is the EV blood test kit ExoDx Lung (ALK) for detecting EML-4-ALK mutations in patients with nonsmall cell lung cancer (NSCLC). ExoDx® Prostate is based on urinary EVs and provides a score by detecting the transcripts (mRNA) of PCA3 and TMPRSS2:ERG genes binding with standard of care (SOC = PSA, age, race, and family history of PCa). This method could help determine whether a prostate biopsy is needed by assessing the risk for high-grade (GS ≥ 7) and more aggressive PCa and has proven that 27% of biopsies would have been avoided (42). This study finally achieved the first completely noninvasive, nondigital rectal examination (DRE), urine-based liquid biopsy test of Exosome Diagnostics Company, which is a landmark for EV clinical diagnosis transformation.
4 EV DNA biomarker in PCa
At present, the research on EV nucleic acid biomarkers is mainly focused on EV RNA, while the research on EV DNA is just starting. Since 2014, some studies have gradually characterized the properties of EV DNA. First, how the DNA is extracted determines which part of the DNA on the EVs is studied. EV DNA consists of two parts, including those that adhere to the outside of the EV (external DNA) and those that are wrapped inside the EV (internal DNA). In order to better characterize the internal DNA, which is relatively impervious to external interference, EVs were pretreated with DNase and RNase to digest the external EV DNA before lysis with Triton X (48). Second, the focus is on how to characterize DNA traits. Thakur et al. demonstrated the double-stranded structure of EV DNA visually for the first time through enzyme digestion and atomic force microscopy. Gel electrophoresis further confirmed the molecular weight difference between EV DNA and genomic DNA (gDNA) in melanoma, which were mainly concentrated at 250 bp~2.5 kb and 2.5~10 kb, respectively. Despite being highly fragmented, EV DNA has been shown, without specific fragments, to be highly enriched or consumed compared with gDNA by high-throughput whole-genome sequencing (22, 49). Quantitative analysis showed that EV DNA could be detected in all tumor types, including PCa (22RV cell line), at a concentration ranging from 0 to 10 ng/µg of EV protein (22). Thirdly, the mechanism by which EVs transmit dsDNA is still controversial. It is currently considered that cells can maintain homeostasis by excreting harmful DNA through EVs (48). In addition to being a method of excretion of waste, other studies confirmed that EVs horizontally transfer genes between cells and can educate downstream cells. It has been reported that tumor EVs carry oncogene sequences such as c-Myc to transmit the genetic information of “mutation and amplification” to normal cells (50).
As PCa biomarkers, EV DNA is gradually showing some diagnostic potential, which is deployed relatively earlier and more mature than the other tumors. However, relevant studies are still relatively few, and there are still many gaps. PCa EV subpopulations carry different gDNA fragments (51) with large PCa EVs (1–10 µm) carrying most DNA (23), which indicates that the DNA molecules are selectively and cell-dependently packed into specific EV subtypes. EV DNA derived from LNCaP and PC-3 cells was sequenced to detect PCa-related mutations of MLH1, PTEN, and TP53 genes. A frameshift mutation (delAA) in codon 6 of the PTEN gene and a point mutation (C>G) in codon 215 of the TP53 gene was detected, the same as in their parental tumor cells (51). The above results showed that EV DNA has the same potential as circulating tumor DNA (ctDNA) to detect tumor-specific genetic mutations, which prompts its potential as an alternative target for gDNA from tumor tissue or cells.
5 Conclusion and perspectives
Based on body fluid specimens like blood, liquid biopsy can overcome tissue heterogeneity and the difficulty of sampling, which have great potential for use as noninvasive diagnostic biomarkers compared with traditional tissue biopsy. As one of the three targets of liquid biopsy, EVs have some obvious advantages. On the one hand, the collection of blood and urine to obtain EVs is easily available and minimally invasive, which helps facilitate repeated sampling and achieve dynamic and real-time monitoring of molecular changes in tumors. On the other hand, the accuracy of tumor detection could be improved by analyzing tumor-derived EVs or even selecting specific subtypes of tumor-derived EVs to increase analytical sensitivity and specificity. More recent efforts have demonstrated that tumor-derived EVs have the potential to be used as a reliable source of cancer-related diagnostic biomarkers. In particular, the nucleic acid component is stable and easy to detect and seems to be the most promising biomarker for EVs. Since EV miRNA was first reported in 2007, publications about EV RNA biomarkers have grown exponentially. Apart from miRNA, other EV nucleic acid molecules, such as oncogenic mRNA (including fusion genes and splice variant transcripts), lncRNA, and dsDNA (including cancer-driven mutation genes), also attract lots of attention. The richness of the contents, the stability of the double-layer membrane structure, and the diversity of detection methods of EVs make them have unique advantages in tumor diagnosis.
Researchers have found that EVs in biofluids contain a group of proteomic and genetic signatures of cancer, thus presenting an enormous opportunity in terms of cancer diagnosis. However, this is technically challenging due to the lack of effective and standard methods for enriching tumor-derived EVs from total EVs distinguish from EVs secreted by normal cells or specific-size EVs from total tumor-derived EVs (isolate large vesicles to extract DNA). Therefore, obtaining high purity and selected EVs is necessary before further exploration of their molecular mechanism and function. So far, many EV isolation and purification approaches have been reported, including ultracentrifugation (UC), filtration, chemical precipitation, affinity-binding beads, and microfluidics techniques. The choice of EV isolation method depends on the sample source, molecule to be detected, and downstream detection methods. UC and density gradient ultracentrifugation (DG-UC) have always been regarded as the most common approaches used to isolate total EVs. However, sequential centrifugation is time-consuming (90 min times two rounds) and instrument-demanding, and it has the problem of co-purification of non-EV-related proteins (protein aggregates and lipoproteins). In recent years, immunocapture using antibodies to target tumor-specific proteins on EV surface (e.g., PSMA or EpCAM for PCa (46)) has been optimized and has emerged as a viable choice for EV purification. In addition, the separation method of EVs should be simplified for clinical application practice.
In addition to the concerns over isolation approaches, there are further uncertainties over protocol standardization and how to define the preanalytical and analytical variables that impact outcome measures. It remains unclear how to translate omics techniques and omics information into early PCa diagnosis and prognosis forecasting. Large-scale clinical translation has not yet been carried out, and the majority of EV biomarker papers now only discuss the sensitivity, specificity, or AUC of the diagnostic model. However, it should be noted that if EVs are to be introduced into clinical laboratories as tumor markers, comprehensive verification work must be conducted to generate enough data that can define the test’s performance. The information for a mature kit should be applied, such as sample type, sampling method, anticoagulant, transportation and storage conditions, and analysis parameters including accuracy, sensitivity, specificity, linearity, lower detection limit, measurement uncertainty, etc. However, due to the need for large-scale and rigorous performance verification before clinical application, this process is time-consuming and costly. Therefore, there is still a long but promising journey between these novel EV biomarkers and their clinical translation for routine diagnostic use.
Author contributions
YD, XH, and JYL contributed to the conception and design of the study. BZ organized the database. TD performed the statistical analysis. YD wrote the first draft of the manuscript. RL, JJL, LY, and LZ wrote sections of the manuscript. All authors contributed to the article and approved the submitted version.
Conflict of interest
The authors declare that the research was conducted in the absence of any commercial or financial relationships that could be construed as a potential conflict of interest.
Publisher’s note
All claims expressed in this article are solely those of the authors and do not necessarily represent those of their affiliated organizations, or those of the publisher, the editors and the reviewers. Any product that may be evaluated in this article, or claim that may be made by its manufacturer, is not guaranteed or endorsed by the publisher.
References
1. Kalluri R. The biology and function of exosomes in cancer. J Clin Invest (2016) 126(4):1208–15. doi: 10.1172/JCI81135
2. Saini S. PSA and beyond: alternative prostate cancer biomarkers. Cell Oncol (Dordr) (2016) 39(2):97–106. doi: 10.1007/s13402-016-0268-6
3. Mizutani K, Terazawa R, Kameyama K, Kato T, Horie K, Tsuchiya T, et al. Isolation of prostate cancer-related exosomes. Anticancer Res (2014) 34(7):3419–23.
4. Turay D, Khan S, Diaz Osterman CJ, Curtis MP, Khaira B, Neidigh JW, et al. Proteomic profiling of serum-derived exosomes from ethnically diverse prostate cancer patients. Cancer Invest (2016) 34(1):1–11. doi: 10.3109/07357907.2015.1081921
5. Logozzi M, Angelini DF, Iessi E, Mizzoni D, Di Raimo R, Federici C, et al. Increased PSA expression on prostate cancer exosomes in in vitro condition and in cancer patients. Cancer Lett (2017) 403:318–29. doi: 10.1016/j.canlet.2017.06.036
6. Ozgur E. Gezer U investigation of lncRNA H19 in prostate cancer cells and secreted exosomes upon androgen stimulation or androgen receptor blockage. Bratisl Lek Listy (2020) 121(5):362–5. doi: 10.4149/BLL_2020_058
7. Bijnsdorp IV, Geldof AA, Lavaei M, Piersma SR, van Moorselaar RJ, Jimenez CR. Exosomal ITGA3 interferes with non-cancerous prostate cell functions and is increased in urine exosomes of metastatic prostate cancer patients. J Extracell Vesicles (2013) 2:22097. doi: 10.3402/jev.v2i0.22097
8. Webber JP, Spary LK, Sanders AJ, Chowdhury R, Jiang WG, Steadman R, et al. Differentiation of tumour-promoting stromal myofibroblasts by cancer exosomes. Oncogene (2015) 34(3):290–302. doi: 10.1038/onc.2013.560
9. Zhao H, Yang L, Baddour J, Achreja A, Bernard V, Moss T, et al. Tumor microenvironment derived exosomes pleiotropically modulate cancer cell metabolism. (2016) 5:e10250. doi: 10.7554/eLife.10250.022
10. Sadovska L, Zayakin P, Bajo-Santos C, Endzelins E, Auders J, Keisa L, et al. Effects of urinary extracellular vesicles from prostate cancer patients on the transcriptomes of cancer-associated and normal fibroblasts. BMC Cancer (2022) 22(1):1055. doi: 10.1186/s12885-022-10107-3
11. Hosseini-Beheshti E, Choi W, Weiswald LB, Kharmate G, Ghaffari M, Roshan-Moniri M, et al. Exosomes confer pro-survival signals to alter the phenotype of prostate cells in their surrounding environment. ONCOTARGET (2016) 7(12):14639–58. doi: 10.18632/oncotarget.7052
12. Corcoran C, Rani S Fau - O'Brien K, O'Brien K Fau - O'Neill A, O'Neill A Fau - Prencipe M, Prencipe M Fau - Sheikh R, Sheikh R Fau - Webb G, et al. Docetaxel-resistance in prostate cancer: evaluating associated phenotypic changes and potential for resistance transfer via exosomes. PLOSONE (2012) 7(12):e50999. doi: 10.1371/journal.pone.0050999
13. Mo C, Huang B, Zhuang J, Jiang S, Guo S, Mao X. LncRNA nuclear-enriched abundant transcript 1 shuttled by prostate cancer cells-secreted exosomes initiates osteoblastic phenotypes in the bone metastatic microenvironment via miR-205-5p/runt-related transcription factor 2/splicing factor proline- and glutamine-rich/polypyrimidine tract-binding protein 2 axis. Clin Transl Med (2021) 11(8):e493. doi: 10.1002/ctm2.493
14. Hu CY, Chen J, Qin XH, You P, Ma J, Zhang J, et al. Long non-coding RNA NORAD promotes the prostate cancer cell extracellular vesicle release via microRNA-541-3p-regulated PKM2 to induce bone metastasis of prostate cancer. J Exp Clin Cancer Res (2021) 40(1):98. doi: 10.1186/s13046-021-01891-0
15. Li Q, Hu J, Shi Y, Xiao M, Bi T, Wang C, et al. Exosomal lncAY927529 enhances prostate cancer cell proliferation and invasion through regulating bone microenvironment. Cell Cycle (2021) 20(23):2531–46. doi: 10.1080/15384101.2021.1992853
16. Itoh T, Ito Y, Ohtsuki Y, Ando M, Tsukamasa Y, Yamada N, et al. Microvesicles released from hormone-refractory prostate cancer cells facilitate mouse pre-osteoblast differentiation. J Mol Histol (2012) 43(5):509–15. doi: 10.1007/s10735-012-9415-1
17. Yu L, Sui B, Fan W, Lei L, Zhou L, Yang L, et al. Exosomes derived from osteogenic tumor activate osteoclast differentiation and concurrently inhibit osteogenesis by transferring COL1A1-targeting miRNA-92a-1-5p. J Extracellular Vesicles (2021) 10(3):e12056. doi: 10.1002/jev2.12056
18. Lundholm M, Schroder M, Nagaeva O, Baranov V, Widmark A, Mincheva-Nilsson L, et al. Prostate tumor-derived exosomes down-regulate NKG2D expression on natural killer cells and CD8+ T cells: mechanism of immune evasion. PloS One (2014) 9(9):e108925. doi: 10.1371/journal.pone.0108925
19. Takahara K, Ii M, Inamoto T, Nakagawa T, Ibuki N, Yoshikawa Y, et al. microRNA-145 mediates the inhibitory effect of adipose tissue-derived stromal cells on prostate cancer. Stem Cells Dev (2016) 25(17):1290–8. doi: 10.1089/scd.2016.0093
20. Hessvik NP, Phuyal S, Brech A, Sandvig K, Llorente A. Profiling of microRNAs in exosomes released from PC-3 prostate cancer cells. Biochim Biophys Acta (2012) 1819(11-12):1154–63. doi: 10.1016/j.bbagrm.2012.08.016
21. Park YH, Shin HW, Jung AR, Kwon OS, Choi YJ, Park J, et al. Prostate-specific extracellular vesicles as a novel biomarker in human prostate cancer. Sci Rep (2016) 6:30386. doi: 10.1038/srep30386
22. Thakur BK, Zhang H, Becker A, Matei I, Huang Y, Costa-Silva B, et al. Double-stranded DNA in exosomes: a novel biomarker in cancer detection. Cell Res (2014) 24(6):766–9. doi: 10.1038/cr.2014.44
23. Vagner T, Spinelli C, Minciacchi VR, Balaj L, Zandian M, Conley A, et al. Large Extracellular vesicles carry most of the tumour DNA circulating in prostate cancer patient plasma. J Extracellular Vesicles (2018) 7(1):1505403. doi: 10.1080/20013078.2018.1505403
24. Takahashi RU, Prieto-Vila M, Hironaka A, Ochiya T. The role of extracellular vesicle microRNAs in cancer biology. Clin Chem Lab Med (2017) 55(5):648–56. doi: 10.1515/cclm-2016-0708
25. Tomasetti M, Lee W, Santarelli L, Neuzil J. Exosome-derived microRNAs in cancer metabolism: possible implications in cancer diagnostics and therapy. Exp Mol Med (2017) 49(1):e285. doi: 10.1038/emm.2016.153
26. Dijkstra S, Birker IL, Smit FP, Leyten GH, de Reijke TM, van Oort IM, et al. Prostate cancer biomarker profiles in urinary sediments and exosomes. J Urol (2014) 191(4):1132–8. doi: 10.1016/j.juro.2013.11.001
27. Pellegrini KL, Patil D, Douglas KJS, Lee G, Wehrmeyer K, Torlak M, et al. Detection of prostate cancer-specific transcripts in extracellular vesicles isolated from post-DRE urine. Prostate (2017) 77(9):990–9. doi: 10.1002/pros.23355
28. Duijvesz D, Versluis CYL, van der Fels CAM, Vredenbregt-van den Berg MS, Leivo J, Peltola MT, et al. Immuno-based detection of extracellular vesicles in urine as diagnostic marker for prostate cancer. Int J Cancer (2015) 137(12):2869–78. doi: 10.1002/ijc.29664
29. Rodriguez M, Bajo-Santos C, Hessvik NP, Lorenz S, Fromm B, Berge V, et al. Identification of non-invasive miRNAs biomarkers for prostate cancer by deep sequencing analysis of urinary exosomes. Mol Cancer (2017) 16(1):156. doi: 10.1186/s12943-017-0726-4
30. Huang X, Yuan T, Liang M, Du M, Xia S, Dittmar R, et al. Exosomal miR-1290 and miR-375 as prognostic markers in castration-resistant prostate cancer. Eur Urol (2015) 67(1):33–41. doi: 10.1016/j.eururo.2014.07.035
31. Koppers-Lalic D, Hackenberg M, de Menezes R, Misovic B, Wachalska M, Geldof A, et al. Non-invasive prostate cancer detection by measuring miRNA variants (isomiRs) in urine extracellular vesicles. Oncotarget (2016) 7(16):22566–78. doi: 10.18632/oncotarget.8124
32. Li Z, Ma YY, Wang J, Zeng XF, Li R, Kang W, et al. Exosomal microRNA-141 is upregulated in the serum of prostate cancer patients. Onco Targets Ther (2016) 9:139–48. doi: 10.2147/OTT.S95565
33. Bryant RJ, Pawlowski T, Catto JWF, Marsden G, Vessella RL, Rhees B, et al. Changes in circulating microRNA levels associated with prostate cancer. Br J Cancer (2012) 106(4):768–74. doi: 10.1038/bjc.2011.595
34. Bhagirath D, Yang TL, Bucay N, Sekhon K, Majid S, Shahryari V, et al. microRNA-1246 is an exosomal biomarker for aggressive prostate cancer. Cancer Res (2018) 78(7):1833–44. doi: 10.1158/0008-5472.CAN-17-2069
35. Bryzgunova OE, Zaripov MM, Skvortsova TE, Lekchnov EA, Grigor'eva AE, Zaporozhchenko IA, et al. Comparative study of extracellular vesicles from the urine of healthy individuals and prostate cancer patients. PloS One (2016) 11(6):e0157566. doi: 10.1371/journal.pone.0157566
36. Wani S, Kaul D, Mavuduru RS, Kakkar N, Bhatia A. Urinary-exosomal miR-2909: a novel pathognomonic trait of prostate cancer severity. J Biotechnol (2017) 259:135–9. doi: 10.1016/j.jbiotec.2017.07.029
37. Foj L, Ferrer F, Serra M, Arevalo A, Gavagnach M, Gimenez N, et al. Exosomal and non-exosomal urinary miRNAs in prostate cancer detection and prognosis. Prostate (2017) 77(6):573–83. doi: 10.1002/pros.23295
38. Samsonov R, Shtam T, Burdakov V, Glotov A, Tsyrlina E, Berstein L, et al. Lectin-induced agglutination method of urinary exosomes isolation followed by mi-RNA analysis: application for prostate cancer diagnostic. Prostate (2016) 76(1):68–79. doi: 10.1002/pros.23101
39. Xu Y, Qin S, An T, Tang Y, Huang Y, Zheng L. MiR-145 detection in urinary extracellular vesicles increase diagnostic efficiency of prostate cancer based on hydrostatic filtration dialysis method. Prostate (2017) 77(10):1167–75. doi: 10.1002/pros.23376
40. Yasui T, Yanagida T, Ito S, Konakade Y, Takeshita D, Naganawa T, et al. Unveiling massive numbers of cancer-related urinary-microRNA candidates via nanowires. Sci Adv (2017) 3(12):e1701133. doi: 10.1126/sciadv.1701133
41. Donovan MJ, Noerholm M, Bentink S, Belzer S, Skog J, O'Neill V, et al. A molecular signature of PCA3 and ERG exosomal RNA from non-DRE urine is predictive of initial prostate biopsy result. Prostate Cancer Prostatic Dis (2015) 18(4):370–5. doi: 10.1038/pcan.2015.40
42. McKiernan J, Donovan MJ, O'Neill V, Bentink S, Noerholm M, Belzer S, et al. A novel urine exosome gene expression assay to predict high-grade prostate cancer at initial biopsy. JAMA Oncol (2016) 2(7):882–9. doi: 10.1001/jamaoncol.2016.0097
43. Motamedinia P, Scott AN, Bate KL, Sadeghi N, Salazar G, Shapiro E, et al. Urine exosomes for non-invasive assessment of gene expression and mutations of prostate cancer. PloS One (2016) 11(5):e0154507. doi: 10.1371/journal.pone.0154507
44. Hendriks RJ, Dijkstra S, Jannink SA, Steffens MG, van Oort IM, Mulders PF, et al. Comparative analysis of prostate cancer specific biomarkers PCA3 and ERG in whole urine, urinary sediments and exosomes. Clin Chem Lab Med (2016) 54(3):483–92. doi: 10.1515/cclm-2015-0599
45. Isin M, Uysaler E, Ozgur E, Koseoglu H, Sanli O, Yucel OB, et al. Exosomal lncRNA-p21 levels may help to distinguish prostate cancer from benign disease. Front Genet (2015) 6:168. doi: 10.3389/fgene.2015.00168
46. Wang Y-H, Ji J, Wang B-C, Chen H, Yang Z-H, Wang K, et al. Tumor-derived exosomal long noncoding RNAs as promising diagnostic biomarkers for prostate cancer. Cell Physiol Biochem (2018) 46(2):532–45. doi: 10.1159/000488620
47. Chisholm J, Haas-Neill S, Margetts P. Al-Nedawi K characterization of proteins, mRNAs, and miRNAs of circulating extracellular vesicles from prostate cancer patients compared to healthy subjects. Front Oncol (2022) 12:895555. doi: 10.3389/fonc.2022.895555
48. Takahashi A, Okada R, Nagao K, Kawamata Y, Hanyu A, Yoshimoto S, et al. Exosomes maintain cellular homeostasis by excreting harmful DNA from cells. Nat Commun (2017) 8:15287. doi: 10.1038/ncomms15287
49. Kahlert C, Melo SA, Protopopov A, Tang J, Seth S, Koch M, et al. Identification of double-stranded genomic DNA spanning all chromosomes with mutated KRAS and p53 DNA in the serum exosomes of patients with pancreatic cancer. J Biol Chem (2014) 289(7):3869–75. doi: 10.1074/jbc.C113.532267
50. Balaj L, Lessard R, Dai L, Cho YJ, Pomeroy SL, Breakefield XO, et al. Tumour microvesicles contain retrotransposon elements and amplified oncogene sequences. Nat Commun (2011) 2:180. doi: 10.1038/ncomms1180
Keywords: prostate cancer, extracellular vesicles, nucleic acids, diagnosis, biomarker
Citation: Diao Y, Zhu B, Ding T, Li R, Li J, Yang L, Zhou L, Hao X and Liu J (2023) Tumor-derived extracellular vesicle nucleic acids as promising diagnostic biomarkers for prostate cancer. Front. Oncol. 13:1201554. doi: 10.3389/fonc.2023.1201554
Received: 06 April 2023; Accepted: 14 June 2023;
Published: 29 June 2023.
Edited by:
Michela Pozzobon, University of Padua, ItalyReviewed by:
Ilaria Giusti, University of L’Aquila, ItalyNatasa Zarovni, Independent researcher, Milan, Italy
Copyright © 2023 Diao, Zhu, Ding, Li, Li, Yang, Zhou, Hao and Liu. This is an open-access article distributed under the terms of the Creative Commons Attribution License (CC BY). The use, distribution or reproduction in other forums is permitted, provided the original author(s) and the copyright owner(s) are credited and that the original publication in this journal is cited, in accordance with accepted academic practice. No use, distribution or reproduction is permitted which does not comply with these terms.
*Correspondence: Jiayun Liu, jiayun@fmmu.edu.cn
‡ORCID: Jinjie Li, orcid.org/0000-0002-4439-6276
Xiaoke Hao, orcid.org/0000-0001-9948-0048