- 1Department of Medicine, St. Elizabeth’s Medical Center, Boston University, Boston, MA, United States
- 2Hematology and Bone Marrow Transplant Unit, IRCCS San Raffaele Scientific Institute, Milano, Italy
- 3Clinical Research Division, Fred Hutchinson Cancer Center, Seattle, WA, United States
- 4Department of Pediatrics, University of Washington, Seattle, WA, United States
Introduction: Acute kidney injury (AKI) is a frequent early complication post hematopoietic stem cell transplant (HSCT), associated with high morbidity and mortality. Cord blood transplant (CBT) recipients are potentially exposed to more nephrotoxic insults, compared to patients undergoing HSCT from other donor sources. We aimed to identify risk factors for AKI in patients undergoing CBT. We also aimed to identify the impact of AKI on chronic kidney disease (CKD) and survival outcomes by one-year post-CBT.
Methods: Adults and children who underwent a first CBT at our Institution were retrospectively evaluated. AKI was staged according to Kidney Disease Improving Global Outcomes (KDIGO) definitions. Cox regression models were used to estimate the association of demographic factors and post-CBT parameters with the cause-specific hazard of AKI.
Results: We identified 276 patients. Median age was 32 years, 28% (77/276) were children (<18 years) and 129 (47%) were white. A myeloablative conditioning regimen was administered to 243 patients (88%) and 248 (90%) received cyclosporine for GVHD prophylaxis. One-hundred and eighty-six patients (67%) developed AKI by day 60 post-transplant, with 72 (26%) developing severe AKI (stage 2 and 3). In a multivariable analysis, each increase in bilirubin level of 1 mg/dL was associated with a 23% increase in the risk of severe AKI (adjusted HR 1.23, 95% CI 1.13 – 1.34, p<.0001). Conversely, systemic steroid administration appeared to be protective of severe AKI (unadjusted HR 0.36, 95% CI 0.18 – 0.72, p=.004) in a univariate model . Two-hundred-forty-seven patients were evaluable at the one-year time point. Among those, 100 patients (40%) developed CKD one-year post-CBT. Severe AKI was associated with a higher hazard of non-relapse mortality (adjusted HR=3.26, 95% CI 1.65-6.45, p=.001) and overall mortality (adjusted HR=2.28, 95% CI 1.22-4.27, p=.01).
Discussion: AKI is a frequent complication after CBT and is associated with worse outcomes. Questions remain as to the mechanism of the protective role of steroids on kidney function in the setting of CBT.
Introduction
Cord blood transplantation (CBT) is an established alternative to traditional hematopoietic stem cell transplantation (HSCT) in patients who lack a suitable Human Leukocyte Antigen (HLA)-matched donor. Traditional advantages of CBT include higher degree of HLA mismatches allowance, ease of collection and low rate of chronic graft versus host disease (cGVHD) (1, 2). However, CBT recipients are at higher risk of non-relapse mortality (NRM) when compared to those undergoing HSCT from related or unrelated HLA-matched donors (3, 4). This increased NRM mostly derives from a delayed engraftment and the lack of immunological memory of CB T-cells, resulting in a higher incidence of opportunistic infection (2, 5).
Despite significant improvements in HSCT, acute kidney injury (AKI) and chronic kidney disease (CKD) remain common complications post-HSCT (6, 7) and are associated with an increased mortality risk (8–14). Rates of developing AKI range from 10% in autologous HSCT recipients up to 73% in allogeneic HSCT recipients (6, 7). Causes of AKI in this population vary, and can be graft-related, infection-related, GVHD-related, or iatrogenic. Transplant-associated thrombotic microangiopathy (TA-TMA) is also an increasingly recognized cause of AKI following allogenic HSCT, whose hallmarks are endothelial cell injury and complement cascade activation (15). While overall survival is comparable to that observed after HSCTs from matched-unrelated donor and possibly matched-sibling donor (16), CBT recipients are exposed to more nephrotoxic insults, including infectious complications (e.g., gram-negative rods, cytomegalovirus, BK virus), and consequently antibiotics and anti-viral agents (6, 17–20). A recent study by Gutgarts et al. found a very high rate of AKI (83%) in the first 100 days post-transplant in 153 adults who received CBT +/- haploidentical cell infusion after reduced intensity conditioning regimen (21). Researchers in this study identified African ancestry, concomitant haploidentical CD34+ cell infusion, nephrotoxic medication, ICU admission, and lower albumin level at baseline as risk factors for developing AKI (21).
Hence, we decided to retrospectively evaluate the rate of AKI in a large cohort of patients receiving CBT at the Fred Hutchinson Cancer Center (FHCC) and compare to the available studies. Furthermore, we decided to explore risk factors associated with the development of AKI, as well as the impact of AKI on CKD development. Unlike previous work, our cohort was represented by both adult and pediatric CBT recipients, receiving both myeloablative (MAC) and non-myeloablative conditioning (NAC).
Subjects and methods
Study design and patient selection
Adult and pediatric patients undergoing first single unit or double units CBT at the FHCC between August 2006 and June 2018 on research protocols approved by the Center’s Institutional Review Board were eligible for this retrospective analysis. Patients diagnosed with multiple myeloma and non-malignant diseases were excluded. CBT was considered when HLA-compatible related or unrelated donors were unavailable. Selected CB units were required to be matched to the recipient at ≥ 4 of the 6 HLA loci based on intermediate resolution typing at HLA-A and –B and allele-level for HLA-DRB1 typing; for double units CBT recipients, units must be a minimum match of 3 of the 6 HLA loci. No patients received concomitant haploidentical cell infusion. Hematopoietic cell transplantation-specific comorbidity index was calculated for all patients ≥ 45 years-old (22).
Patients received either NAC or MAC regimen (Table 1). NAC regimens included fludarabine (Flu) 150 mg/m2, cyclophosphamide (Cy) 50 mg/kg, and total body irradiation (TBI) 200 – 300 cGy. MAC regimens included one of the following regimens: Flu, Cy and high dose TBI (1230-1300 cGy) or Treosulfan (14 or 10 g/m2), Flu and TBI 200 – 300 cGy. In all patients included in the analysis, prophylaxis for GVHD consisted of cyclosporine beginning on day -3 (therapeutic range, 275-350 ng/mL) and continuing for a minimum of 180 days, and mycophenolate mofetil (MMF) beginning on day 0 (starting dose 15 mg/kg i.v. every 8 h; maximum 1500 mg every 8 h) and administered until at least day 30-45 or potentially longer in the presence of active GVHD. Pre-engraftment syndrome (PES) data were not collected. Acute GVHD (aGVHD) was graded using Mount Sinai Acute GVHD International Consortium criteria based on stages of organ involvement and categorized as grades 0–4 (23). Time to neutrophil engraftment was defined as the first of two consecutive days with an absolute neutrophil count (ANC) of 0.5 x 109 per liter or higher, and time to platelet engraftment as the first of 7 consecutive days with an unsupported platelet count of 20 x 109 per liter or higher. Post-transplant granulocyte-colony stimulating factor (G-CSF) was given until the absolute neutrophil (ANC) recovery to > 2.5 x 109/L was stable for three days, and then reduced to as needed while maintaining an ANC > 1.0 x 109/L. Sinusoidal obstruction syndrome was defined using the modified Seattle criteria (24). TA-TMA was defined using the criteria proposed by Cho et al. in 2010 (25).
Data collection
We retrospectively reviewed medical records and the clinical database of CBT recipients. Standard biochemistry panel, including kidney and liver function, is collected daily until hospital discharge and then at each follow-up appointment.
We estimated the glomerular filtration rate (eGFR) using the serum creatinine based Schwartz formula for children (age ≤18) and the CKD Epidemiology Collaboration (CKD-EPI) creatinine-only equation for adults (26, 27). Cytomegalovirus (CMV) reactivation was defined as the presence of any viral load and CMV viral loads were monitored routinely per standard practice, whereas testing for serum adenovirus (AdV) and serum BK virus was performed as clinically indicated at the discretion of the attending physician. We collected data regarding the use of potential nephrotoxic antimicrobial drugs, namely vancomycin, amphotericin, and gentamicin within 60 days from transplantation. Any positive CMV level triggered the initiation of treatment with either foscarnet or ganciclovir. Calcineurin inhibitor (CNI) level monitoring was performed according to institutional guidelines.
Definition
We defined AKI according to the Kidney Disease Improving Global Outcomes (KDIGO) guidelines from 2012; AKI is defined as a 0.3 mg/dL increase in baseline serum creatinine within 48 hours or an increase of 1.5 times baseline within 7 days. Stage 1 AKI: an increase in serum creatinine of 1.5-1.9 times baseline; stage 2: an increase of 2.0-2.9 times baseline; and stage 3: an increase in serum creatinine of ≥ 3.0 times baseline or a serum creatinine absolute value of ≥ 4.0 mg/dL (28). We defined severe AKI as stage ≥2 within the first 60 days post-transplant (29).
CKD was defined as a >20% decrease in eGFR from baseline to one-year post-CBT (day 365 +/- 90 days) (30). Bilirubin level was monitored in the first 28 days from transplant.
Statistical analysis
Baseline demographics of interest included age at transplant, sex, ethnicity, underlying diagnosis, donor sex, donor CMV status, number of CB units, and conditioning regimen.
Primary endpoints of interest were AKI of any stage by 60 days post CBT, severe AKI, overall mortality (OM) and non-relapse mortality (NRM).
Cox regression models were used to estimate the association of demographic factors and post-CBT parameters with the cause-specific hazard of AKI. Univariate models were used to estimate the impact of factors on risk of developing AKI, and a multivariable model was constructed from factors with a p-value such that p<.05. At least 10 events per degree of freedom were required in the multivariable model. Variables [e.g., occurrence of aGVHD, receipt of medications, laboratory values (such as bilirubin level)] that occurred or were assessed following CBT were treated as time-dependent covariates. Continuous variables were modeled as continuous linear covariates (in some cases after a log-transformation). The impact of AKI on OM and NRM were assessed using Cox regression with AKI modeled as a time-dependent covariate, and models were adjusted for age, sex, CMV serostatus, disease severity (intermediate vs high).
CKD incidence at one-year post-CBT was examined as a secondary endpoint. Patients who did not have an available creatinine value within 90 days of day 365 were excluded from the analysis. We compared proportions of patients who developed CKD among those who previously had AKI to the proportion who developed CKD among those who did not have AKI using a chi-square test.
All listed p-values are two-sided, and no adjustments were made for multiple comparisons. Statistical analyses were performed using SAS Statistical Software Version 9.4.
Results
Patient characteristics
A total of 276 adults and pediatric patients underwent a first CBT between August 2006 and June 2018 at FHCC. Baseline patient demographics and transplant characteristics are shown in Table 1. The median age at transplant was 32 (range, 0-73). Acute leukemia was the most frequent diagnosis (227 patients, 82%). Of the 127 patients eligible for screening, HCT-CI was available for 111 patients (87%, median 2, range 0-8). Two-hundred and forty-three patients (88%) received a MAC regimen and 218 (79%) received a double unit CBT.
The median time to ANC engraftment was 19 days (range 6 – 89) and the median time of platelet engraftment was 34 days (range 9 – 99). Seventy-eight percent (215/276) of patients developed aGVHD, 177 of whom (64%) had aGVHD prior to day 60. Twenty percent (52/276) experienced grade 3-4 aGVHD. In 260 (94%) patients we were able to evaluate the occurrence of SOS and TA-TMA. Only 3 and 4 patients were diagnosed with SOS and TA-TMA, respectively, and because of their rarity they were not included in the analysis. Patients were followed for a median of 25.4 months (range 0.2 – 135.4 months); death from any cause occurred in 102 patients (37%). Vancomycin, gentamycin, and amphotericin were administered in 145 (53%), 33 (12%) and 14 (5%) patients, respectively. A positive PCR on blood for CMV and BK virus was documented in 126 (46%) and 41 (15%) of patients, respectively. AdV detection in blood occurred in 5 (2%) patients and because of its rarity was not included in the analysis.
Incidence of AKI post-CBT
Among the 276 patients 67% (186/276) had AKI of any stage. Stage 1 AKI occurred in 41% (114/276), stage 2 in 16% (43/276), stage 3 in 11% (29/276) of patients; 72 of the 276 (26%) patients had severe AKI (defined as ≥ stage 2). The median time to AKI, defined as the time that the probability of AKI reached or crossed 50%, was 39 days. The timing of AKI among those who developed AKI ranged from 0 to 60 days, with the median time of AKI occurrence being 22 days.
Risk factors for AKI in CBT recipients
The associations of demographic data at baseline and post-CBT parameters with the risk of AKI are summarized in Table 2 (AKI of any stage) and Table 3 (severe AKI), including both univariate and multivariable models.
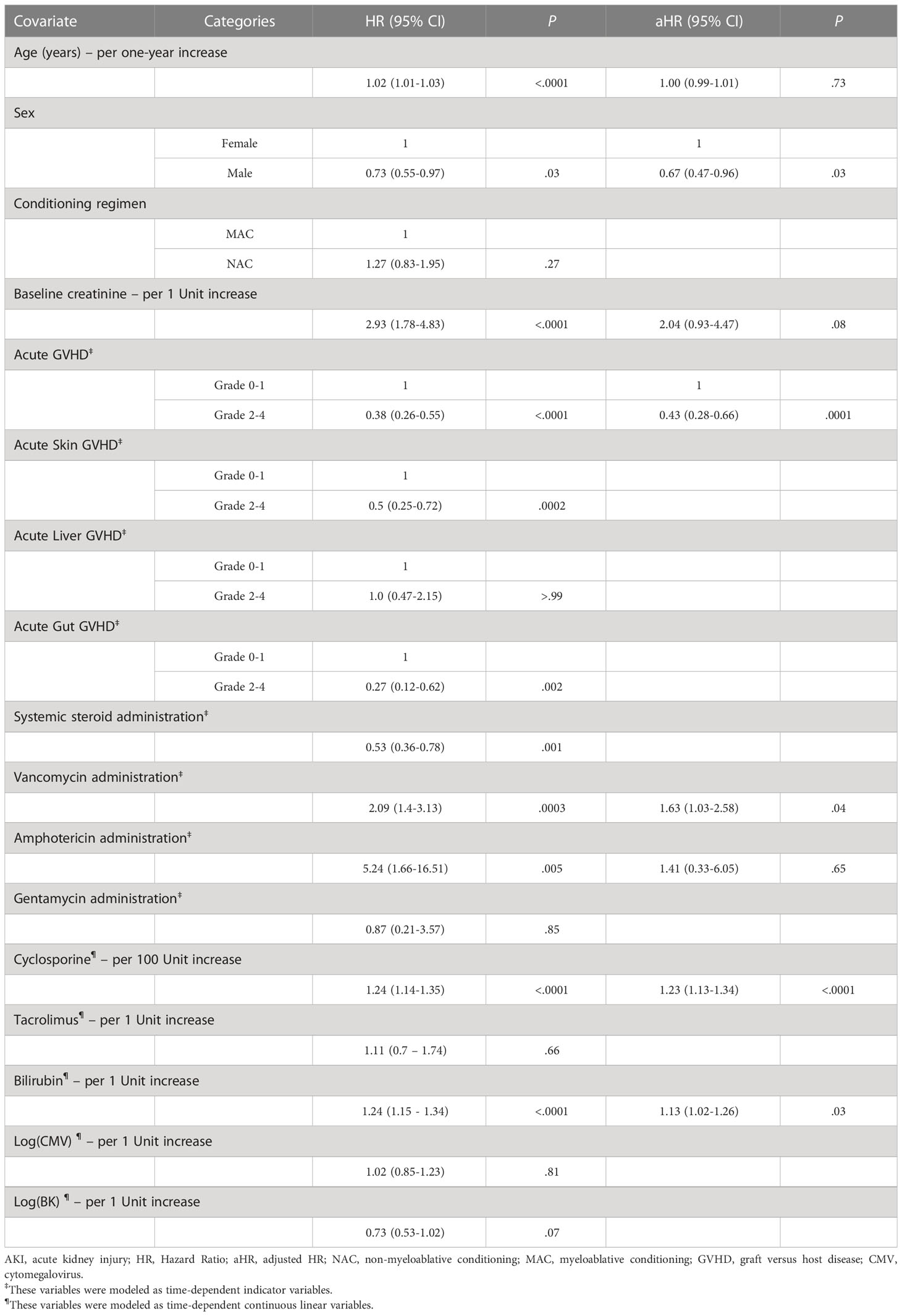
Table 2 Univariate and Multivariable Cox Regression Models for risk factors for Day 60 AKI of any stage.
Among several factors of note in Table 2, the multivariable model showed that each 1mg/dL increase in the post-CBT bilirubin level led to an increased risk of AKI of any stage (HR 1.13, 95% CI 1.02 – 1.26), as did the use of vancomycin (adjusted HR (aHR) 1.63, 95% CI 1.03 – 2.58) and each 100 ng/mL increase in the level of cyclosporine (aHR 1.23, 95% CI 1.13 – 1.34). Presence of grades II-IV acute GVHD and male sex showed reduced risk of AKI of any stage, compared to grades 0-I and female sex, respectively (aHR 0.43, 95% CI 0.28 – 0.66: aHR 0.67, 95% CI 0.47 – 0.96).
For severe AKI, increasing bilirubin following CBT led to a higher risk of severe AKI in the multivariable model (aHR 1.23, 95% CI 1.13 – 1.34), while systemic steroid use led to a lower risk of severe AKI (HR 0.36, 95% CI 0.18 – 0.72) in a univariate model.
Overall survival and non-relapse mortality
Day-100 and day-365 estimates of overall survival were 90% (95% CI: 86% - 93%) and 71% (95% CI: 65% - 76%), respectively. We evaluated the impact of AKI on the risk of OM and NRM after adjusting for age, sex, CMV serostatus, and disease severity (Table 4).
The adjusted HRs for OM and NRM among patients with severe AKI relative to those without AKI or stage-1 AKI were 2.28 (95% CI: 1.22-4.27) and 3.26 (95% CI: 1.65-6.45), respectively. While these regression models treat AKI as a time-dependent covariate, for graphical purposes we performed a landmark analysis at day 60. Figure 1 shows OS after day 60 among those who survived to day 60 (254 of 276 patients), grouping patients into those who developed AKI of any stage in the first 60 days post-CBT and those who did not.
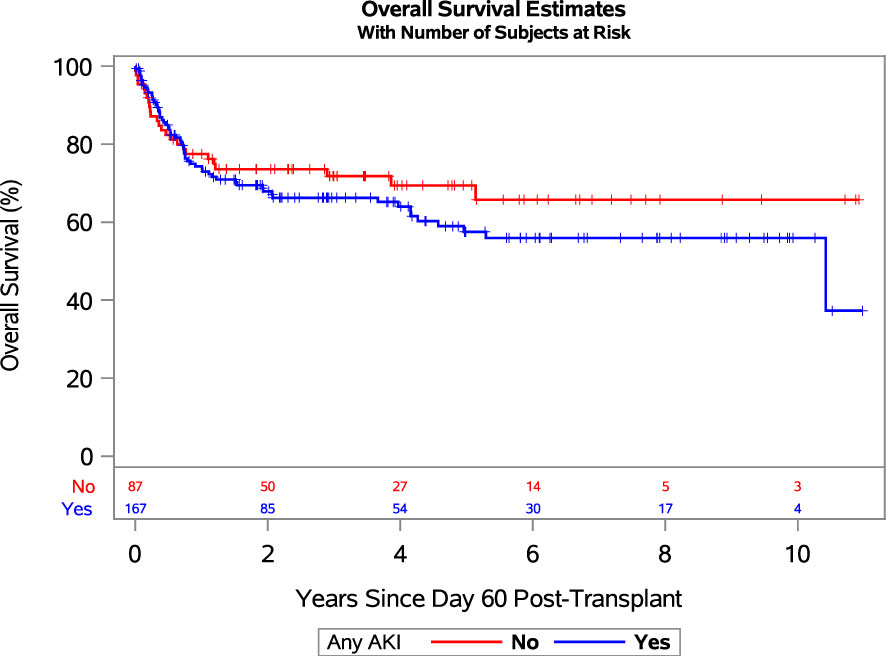
Figure 1 Landmark analysis showing overall survival since day 60 post-transplant for patients who developed any grade AKI (blue line) and no AKI (red line). Abbreviations: AKI, acute kidney injury.
Incidence of CKD and association with AKI
Of the 276 patients who underwent CBT, 70 (25%) died within 1 year from transplant, while 29 (11%) patients did not have available data to calculate eGFR at one year (or within the 90-day window surrounding one year). Among 247 patients whose CKD status at one year was known (including those who died before one year), 100 (40%) had CKD. Among 164 patients with AKI of any stage, 66 (40%) developed CKD at one year, and among 83 patients who did not have AKI, 34 (41%) developed CKD. These results were qualitatively similar when the proportion of CKD was compared between those with severe AKI to those with no AKI or stage-1 AKI. Namely, among 69 patients with severe AKI, 26 (38%) developed CKD at one year compared to 74 of 178 (42%) patients who had no AKI or stage-1 AKI.
If one instead restricts the above analysis to the 178 patients who survived to the one-year time point and whose CKD status at one year was known, 116 patients had AKI of any stage. Among these 116 patients with AKI, 66 (57%) went on to develop CKD at one year compared to 34 of 62 (55%) patients without AKI. Similarly, 26 of 44 (59%) patients with severe AKI had CKD at one year compared to 74 of 134 (55%) patients without AKI or with stage-1 AKI.
Discussion
To our knowledge, this is the largest study evaluating factors associated with AKI and its impact on patient outcomes after CBT in both adult and pediatric recipients. We found that both any AKI (67%) and severe AKI (26%) occur frequently in our population. We also found that severe AKI increases the risk of NRM and OM relative to patients who did not develop AKI or had only stage-1 AKI. Increasing bilirubin level following CBT led to an increased risk of both any grade and grade ≥2 AKI. In contrast, steroid use reduced the risk of severe AKI in a univariate model. The proportion of patients with AKI who developed CKD at one year was similar regardless of AKI status, and this held true even after excluding from analysis patients who died before one year.
The relatively high rate of AKI observed in our cohort is consistent with the literature on HSCT. In a previous study from our group, the incidence of AKI ranged between 50% in NAC and 73% in MAC allogenic transplants (6). Similarly, in a more recent retrospective analysis of HSCT recipients (other than CBT) ranging from 19 to 79 years old (14), AKI of any grade and severe AKI occurred in 64% and 30% of patients, respectively. However, when focusing solely on the CBT recipients, a higher frequency of any stage AKI (83%) and, of severe AKI (54%) was detected by Gutgarts et al. (21). The reasons for this discrepancy could be several. In that study, vancomycin was administered prophylactically to all patients and further nephrotoxic medications (other than GVHD prophylaxis), were given to an additional 24% of patients (21). Conversely, in our cohort, vancomycin was administered to 53% of patients and its use was associated with a higher risk of AKI of any stage. Additionally, only a minority of patients received gentamicin and amphotericin (12% and 5%, respectively), which might partially explain the apparent lack of association between exposure to those two agents and kidney injury. Furthermore, none of our patients received concomitant haploidentical CD34+ cell infusion, which was identified as a risk factor in a multivariable analysis by Gutgarts et al (21).
In our analysis, BK reactivation did not seem to increase the risk of AKI, although such an association has been described (31); this might be partially explained by the small numbers of infections by day 60 (15%), and by the limits of data capture, since BK viremia was not routinely monitored. AdV reactivation occurred in 2% of patients and was thus excluded from the statistical analysis. As expected, we observed a high rate of CMV reactivation, which was associated with a numerical increase in the risk of severe AKI, although it did not reach statistical significance at the multivariable analysis. The direction of this observation is consistent with previous studies (30, 32) where CMV viremia was associated with AKI or with a decline of GFR over time. The lack of statistical significance of our finding might be explained by the difference in the study populations and by fact that treating CMV reactivation at any level of viral load has resulted in a lower incidence of multiple reactivations and disease (33–35). The recent introduction of letermovir prophylaxis might further decrease the impact of early CMV reactivation on post-HSCT outcomes (33).
The kidney toxicity of cyclosporine has been very well described; however, the results in this HSCT patient population are mixed. The effects of cyclosporine use on the kidney include vasoconstriction of the afferent glomerular arteriole, activation of the renin-angiotensin system, tubulo-interstitial scarring, leading ultimately to a decrease in eGFR (36). Although early studies have pointed at CNIs as a risk factor for AKI after HSCT, more recent studies have failed to find a strong association (11, 12, 30). We observed a demonstrable increase in the risk of AKI of any stage with increased levels of cyclosporine. The risk of severe AKI was also increased with higher levels of cyclosporine, but after adjusting for other factors, the association, while still positive, was closer to the null of no association. Gutgarts and colleagues observed an association in the same direction and suggested a reduction of cyclosporine target level in CBT recipients (21).
In our analysis the intensity of the conditioning regimen did not show a demonstrable association with AKI, and the direction of association was different for AKI of any stage compared to severe AKI. Although historically MAC was reported to be associated with a higher incidence of AKI (6, 37, 38), these studies included stem cell sources other than CB. In Gurgarts’ study, no patients received NAC conditioning and therefore no conclusion could be derived for this subset of patients (21). Notably, a more recent study from the same group (14) identified a higher incidence of AKI among patients receiving NAC conditioning regimens with donor sources other than CB. However, more studies are needed to clarify the association between the type of conditioning regimen and the development of AKI in patients undergoing CBT.
Another risk factor for AKI identified in the current study was increased bilirubin level. Several insults can lead to bilirubin elevation, including iatrogenic toxicity, sepsis with multi-organ failure, and SOS. Previous studies evaluated the association between AKI and elevated bilirubin, either as independent variable or in the context of SOS (39–42). In most of these studies (41, 42), although the direction of the association between bilirubin elevation and AKI was consistent with the one we detected, only SOS was significantly associated at the multivariable analysis. The pathophysiology underlying AKI in patients with SOS appears to be a variant of the hepatorenal syndrome (12, 43, 44). In our study, the diagnosis of SOS was extremely rare. This finding is likely related to not using busulfan in the conditioning regimen for CBT. This study confirms the association between bilirubin elevation and AKI in the CBT setting, where it was not previously described.
The seemingly protective effect aGVHD had on AKI in regression models was unexpected. Given its magnitude, we hypothesized that not aGVHD itself, but rather its treatment with systemic steroids could be playing a role in protecting kidney function. That said, since presence of aGVHD and receipt of steroids are obviously strongly correlated, we only allowed presence of aGVHD in the multivariable regression models. Although no consensus has been reached regarding the kidney involvement in the GVHD process, kidney lymphocyte infiltration and local upregulation of inflammatory genes have been described in animal models of aGVHD (45–47). Additionally, documentation of glomerulitis, renal tubulitis, and peritubular capillaritis have been observed in HSCT-recipients with cGVHD, suggesting a possible direct inflammation of kidney tissues (48). Previous reports have also described responsiveness to steroid and/or other immunosuppressants used to treat nephrotic syndrome (including minimal change disease and membranous glomerulonephritis) occurring as late post-HSCT complications, likely as part of cGVHD syndrome (49–51). However, reports on kidney aGVHD in humans are more limited, mainly due to challenges in obtaining kidney biopsies and lack of established histopathologic criteria. In a recent prospective case series on 8 HSCT patients, a kidney biopsy was performed to aid in the management of persistent kidney dysfunction, revealing a high frequency of thrombotic microangiopathy and GVHD as a cause of AKI/CKD in this setting (52). Therefore, we suggest that among different causes of AKI, providers should keep in mind a possible GVHD etiology and discuss biopsy when deemed to affect patient management.
The similar frequency of CKD development at one year between those with and without AKI by day 60 was also unexpected. Previous studies identified AKI as a risk factor for subsequent development of CKD, with an incidence of CKD ranging from 16% to 34% among long-term HSCT survivors (10, 14, 21, 53–55). In their study, Gutgarts et al. reported an incidence of 34% of CKD at two years post HSCT and this was more frequent in patients with a previous AKI with eGFR <60 ml/min per 1.73 m (2) and lasting ≥28 days. In our study, the incidence of CKD appeared higher (40%) compared to previous analyses and with similar rates among patients with or without prior AKI. The reasons for such discrepancy and for the lack of association between AKI and CKD are not entirely clear, but could be related to the different definitions of CKD used in previous studies resulting in either over or underestimation of CKD.
There are several limitations to this study, which can be primarily attributed to its retrospective nature. As the FHCC serves a large geographical area, not all CBT recipients return to our center for the one-year visit, leading to a lack of information at this timepoint and thus an incomplete secondary analysis of the association of AKI and CKD by one-year post-CBT. In addition, due to challenges in retrospective data capture, some variables were not collected, such as volume status, duration of nephrotoxic medications, vancomycin levels, and urine studies (e.g., proteinuria). One of the limitations of our study was also the inability of reporting the incidence and timing of PES. The latter was related to the clinical difficulty of discriminating between PES and hyperacute GVHD based on time of onset and type of treatment of these two overlapping entities. Lastly, we used serum creatinine to determine eGFR in the diagnosis of CKD, which may result in an inaccurate estimation in the setting of decreased muscle mass, particularly in children (56).
Overall, we describe a high rate of AKI in a large CBT cohort of pediatric and adult patients. Elevated bilirubin levels significantly increase the risk of AKI, indicating the need to clarify the significance and pathophysiology of this association through further studies. Lastly, we reported for the first time a negative correlation between aGVHD, and the subsequent use of steroids, and the development of AKI. However, prospective analyses are needed to confirm their possibly protective role, while histopathological data might shed light on the underlying pathophysiological mechanism.
Data availability statement
The raw data supporting the conclusions of this article will be made available by the authors, without undue reservation.
Ethics statement
The studies involving human participants were reviewed and approved by Fred Hutchinson Cancer Research Center Institutional Review Board. Written informed consent to participate in this study was provided by the participants’ legal guardian/next of kin.
Author contributions
FM and SH contributed equally to the manuscript and share senior authorship. FM, SH, TG, and KB conceived the study. KB and TG analyzed the results. PL and EX wrote the initial draft of the manuscript. All authors contributed to the article and approved the submitted version.
Conflict of interest
The authors declare that the research was conducted in the absence of any commercial or financial relationships that could be construed as a potential conflict of interest.
Publisher’s note
All claims expressed in this article are solely those of the authors and do not necessarily represent those of their affiliated organizations, or those of the publisher, the editors and the reviewers. Any product that may be evaluated in this article, or claim that may be made by its manufacturer, is not guaranteed or endorsed by the publisher.
References
1. Ponce DM, Gonzales A, Lubin M, Castro-Malaspina H, Giralt S, Goldberg JD, et al. Graft-versus-host disease after double-unit cord blood transplantation has unique features and an association with engrafting unit-to-recipient HLA match. Biol Blood Marrow Transplant (2013) 19(6):904–11. doi: 10.1016/j.bbmt.2013.02.008
2. Laughlin MJ, Eapen M, Rubinstein P, Wagner JE, Zhang MJ, Champlin RE, et al. Outcomes after transplantation of cord blood or bone marrow from unrelated donors in adults with leukemia. N Engl J Med (2004) 351(22):2265–75. doi: 10.1056/NEJMoa041276
3. Ballen KK, Gluckman E, Broxmeyer HE. Umbilical cord blood transplantation: the first 25 years and beyond. Blood. (2013) 122(4):491–8. doi: 10.1182/blood-2013-02-453175
4. Rubinstein P, Carrier C, Scaradavou A, Kurtzberg J, Adamson J, Migliaccio AR, et al. Outcomes among 562 recipients of placental-blood transplants from unrelated donors. N Engl J Med (1998) 339(22):1565–77. doi: 10.1056/NEJM199811263392201
5. Ruggeri A, Labopin M, Sormani MP, Sanz G, Sanz J, Volt F, et al. Engraftment kinetics and graft failure after single umbilical cord blood transplantation using a myeloablative conditioning regimen. Haematologica. (2014) 99(9):1509–15. doi: 10.3324/haematol.2014.109280
6. Hingorani S. Renal complications of hematopoietic-cell transplantation. New Engl J Med (2016) 374(23):2256–67. doi: 10.1056/NEJMra1404711
7. Flores FX, Brophy PD, Symons JM, Fortenberry JD, Chua AN, Alexander SR, et al. Continuous renal replacement therapy (CRRT) after stem cell transplantation. a report from the prospective pediatric CRRT registry group. Pediatr Nephrol (2008) 23(4):625–30. doi: 10.1007/s00467-007-0672-2
8. Kogon A, Hingorani S. Acute kidney injury in hematopoietic cell transplantation. Semin Nephrol (2010) 30(6):615–26. doi: 10.1016/j.semnephrol.2010.09.009
9. Liu H, Li YF, Liu BC, Ding JH, Chen BA, Xu WL, et al. A multicenter, retrospective study of acute kidney injury in adult patients with nonmyeloablative hematopoietic SCT. Bone Marrow Transplant (2010) 45(1):153–8. doi: 10.1038/bmt.2009.99
10. Ando M, Ohashi K, Akiyama H, Sakamaki H, Morito T, Tsuchiya K, et al. Chronic kidney disease in long-term survivors of myeloablative allogeneic haematopoietic cell transplantation: prevalence and risk factors. Nephrol Dial Transplant (2010) 25(1):278–82. doi: 10.1093/ndt/gfp485
11. Kagoya Y, Kataoka K, Nannya Y, Kurokawa M. Pretransplant predictors and posttransplant sequels of acute kidney injury after allogeneic stem cell transplantation. Biol Blood Marrow Transplant (2011) 17(3):394–400. doi: 10.1016/j.bbmt.2010.07.010
12. Bao YS, Xie RJ, Wang M, Feng SZ, Han MZ. An evaluation of the RIFLE criteria for acute kidney injury after myeloablative allogeneic haematopoietic stem cell transplantation. Swiss Med Wkly (2011) 141:w13225. doi: 10.4414/smw.2011.13225
13. Ando M. An overview of kidney disease following hematopoietic cell transplantation. Intern Med (2018) 57(11):1503–8. doi: 10.2169/internalmedicine.9838-17
14. Abramson MH, Gutgarts V, Zheng J, Maloy MA, Ruiz JD, Scordo M, et al. Acute kidney injury in the modern era of allogeneic hematopoietic stem cell transplantation. Clin J Am Soc Nephrol (2021) 16(9):1318–27. doi: 10.2215/CJN.19801220
15. Young JA, Pallas CR, Knovich MA. Transplant-associated thrombotic microangiopathy: theoretical considerations and a practical approach to an unrefined diagnosis. Bone Marrow Transplant (2021) 56(8):1805–17. doi: 10.1038/s41409-021-01283-0
16. Milano F, Gooley T, Wood B, Woolfrey A, Flowers ME, Doney K, et al. Cord-blood transplantation in patients with minimal residual disease. New Engl J Med (2016) 375(10):944–53. doi: 10.1056/NEJMoa1602074
17. Ruggeri A, Peffault de Latour R, Carmagnat M, Clave E, Douay C, Larghero J, et al. Outcomes, infections, and immune reconstitution after double cord blood transplantation in patients with high-risk hematological diseases. Transpl Infect Dis (2011) 13(5):456–65. doi: 10.1111/j.1399-3062.2011.00632.x
18. Sauter C, Abboud M, Jia X, Heller G, Gonzales AM, Lubin M, et al. Serious infection risk and immune recovery after double-unit cord blood transplantation without antithymocyte globulin. Biol Blood Marrow Transplant (2011) 17(10):1460–71. doi: 10.1016/j.bbmt.2011.02.001
19. Linder KA, McDonald PJ, Kauffman CA, Revankar SG, Chandrasekar PH, Miceli MH. Infectious complications after umbilical cord blood transplantation for hematological malignancy. Open Forum Infect Dis (2019) 6(2). doi: 10.1093/ofid/ofz037
20. Karantanos T, Kim HT, Tijaro-Ovalle NM, Li L, Cutler C, Antin JH, et al. Reactivation of BK virus after double umbilical cord blood transplantation in adults correlates with impaired reconstitution of CD4+ and CD8+ T effector memory cells and increase of T regulatory cells. Clin Immunol (2019) 207:18–23. doi: 10.1016/j.clim.2019.06.010
21. Gutgarts V, Sathick IJ, Zheng J, Politikos I, Devlin SM, Maloy MA, et al. Incidence and risk factors for acute and chronic kidney injury after adult cord blood transplantation. Biol Blood Marrow Transplant (2020) 26(4):758–63. doi: 10.1016/j.bbmt.2019.12.768
22. Sorror ML, Logan BR, Zhu X, Rizzo JD, Cooke KR, McCarthy PL, et al. Prospective validation of the predictive power of the hematopoietic cell transplantation comorbidity index: a center for international blood and marrow transplant research study. Biol Blood Marrow Transplant (2015) 21(8):1479–87. doi: 10.1016/j.bbmt.2015.04.004
23. Harris AC, Young R, Devine S, Hogan WJ, Ayuk F, Bunworasate U, et al. International, multi-center standardization of acute graft-versus-host disease clinical data collection: a report from the MAGIC consortium. Biol Blood Marrow Transplant (2016) 22(1):4–10. doi: 10.1016/j.bbmt.2015.09.001
24. McDonald GB, Sharma P, Matthews DE, Shulman HM, Thomas ED. Venocclusive disease of the liver after bone marrow transplantation: diagnosis, incidence, and predisposing factors. Hepatology. (1984) 4(1):116–22. doi: 10.1002/hep.1840040121
25. Cho BS, Yahng SA, Lee SE, Eom KS, Kim YJ, Kim HJ, et al. Validation of recently proposed consensus criteria for thrombotic microangiopathy after allogeneic hematopoietic stem-cell transplantation. Transplantation. (2010) 90(8):918–26. doi: 10.1097/TP.0b013e3181f24e8d
26. Inker LA, Schmid CH, Tighiouart H, Eckfeldt JH, Feldman HI, Greene T, et al. Estimating glomerular filtration rate from serum creatinine and cystatin c. N Engl J Med (2012) 367(1):20–9. doi: 10.1056/NEJMoa1114248
27. Schwartz GJ, Muñoz A, Schneider MF, Mak RH, Kaskel F, Warady BA, et al. New equations to estimate GFR in children with CKD. J Am Soc Nephrol (2009) 20(3):629–37. doi: 10.1681/ASN.2008030287
28. Khwaja A. KDIGO clinical practice guidelines for acute kidney injury. Nephron Clin Pract (2012) 120(4):c179–184. doi: 10.1159/000339789
29. Stevens PE, Levin A. Kidney disease: improving global outcomes chronic kidney disease guideline development work group members. evaluation and management of chronic kidney disease: synopsis of the kidney disease: improving global outcomes 2012 clinical practice guideline. Ann Intern Med (2013) 158(11):825–30. doi: 10.7326/0003-4819-158-11-201306040-00007
30. Hingorani S, Pao E, Stevenson P, Schoch G, Laskin BL, Gooley T, et al. Changes in glomerular filtration rate and impact on long-term survival among adults after hematopoietic cell transplantation. Clin J Am Soc Nephrol (2018) 13(6):866–73. doi: 10.2215/CJN.10630917
31. Haines HL, Laskin BL, Goebel J, Davies SM, Yin HJ, Lawrence J, et al. Blood, and not urine, BK viral load predicts renal outcome in children with hemorrhagic cystitis following hematopoietic stem cell transplantation. Biol Blood Marrow Transplantation (2011) 17(10):1512–9. doi: 10.1016/j.bbmt.2011.02.012
32. Madsen K, Cote G, Pelletier K, Kitchlu A, Chen S, Mattsson J, et al. Incidence, outcomes and predictors of acute kidney injury post allogeneic stem cell transplant. Blood. (2020) 136:16–7. doi: 10.1182/blood-2020-136746
33. Hill JA, Zamora D, Xie H, Thur LA, Delaney C, Dahlberg A, et al. Delayed-onset cytomegalovirus infection is frequent after discontinuing letermovir in cord blood transplant recipients. Blood Advances (2021) 5(16):3113–9. doi: 10.1182/bloodadvances.2021004362
34. Hill JA, Pergam SA, Cox E, Xie H, Leisenring WM, Boeckh M, et al. A modified intensive strategy to prevent cytomegalovirus disease in seropositive umbilical cord blood transplantation recipients. Biol Blood Marrow Transplantation (2018) 24(10):2094–100. doi: 10.1016/j.bbmt.2018.05.008
35. Hill JA, Mayer BT, Xie H, Leisenring WM, Huang ML, Stevens-Ayers T, et al. Kinetics of double-stranded DNA viremia after allogeneic hematopoietic cell transplantation. Clin Infect Diseases (2018) 66(3):368–75. doi: 10.1093/cid/cix804
36. Busauschina A, Schnuelle P, van der Woude FJ. Cyclosporine nephrotoxicity. Transplant Proc (2004) 36(2 Suppl):229S–33S. doi: 10.1016/j.transproceed.2004.01.021
37. Lopes JA, Jorge S, Silva S, de Almeida E, Abreu F, Martins C, et al. Acute renal failure following myeloablative autologous and allogeneic hematopoietic cell transplantation. Bone Marrow Transplant (2006) 38(10):707. doi: 10.1038/sj.bmt.1705513
38. Ando M, Mori J, Ohashi K, Akiyama H, Morito T, Tsuchiya K, et al. A comparative assessment of the RIFLE, AKIN and conventional criteria for acute kidney injury after hematopoietic SCT. Bone Marrow Transplant (2010) 45(9):1427–34. doi: 10.1038/bmt.2009.377
39. Zager RA, O’Quigley J, Zager BK, Alpers CE, Shulman HM, Gamelin LM, et al. Acute renal failure following bone marrow transplantation: a retrospective study of 272 patients. Am J Kidney Dis (1989) 13(3):210–6. doi: 10.1016/s0272-6386(89)80054-x
40. Gruss E, Bernis C, Tomas JF, Garcia-Canton C, Figuera A, Motellón JL, et al. Acute renal failure in patients following bone marrow transplantation: prevalence, risk factors and outcome. Am J Nephrol (1995) 15(6):473–9. doi: 10.1159/000168889
41. Parikh CR, McSweeney PA, Korular D, Ecder T, Merouani A, Taylor J, et al. Renal dysfunction in allogeneic hematopoietic cell transplantation. Kidney Int (2002) 62(2):566–73. doi: 10.1046/j.1523-1755.2002.00455.x
42. Hingorani SR, Guthrie K, Batchelder A, Schoch G, Aboulhosn N, Manchion J, et al. Acute renal failure after myeloablative hematopoietic cell transplant: incidence and risk factors. Kidney Int (2005) 67(1):272–7. doi: 10.1111/j.1523-1755.2005.00078.x
43. Krishnappa V, Gupta M, Manu G, Kwatra S, Owusu OT, Raina R. Acute kidney injury in hematopoietic stem cell transplantation: a review. Int J Nephrol (2016) 2016:5163789. doi: 10.1155/2016/5163789
44. Humphreys BD, Soiffer RJ, Magee CC. Renal failure associated with cancer and its treatment: an update. JASN. (2005) 16(1):151–61. doi: 10.1681/ASN.2004100843
45. Higo S, Shimizu A, Masuda Y, Nagasaka S, Kajimoto Y, Kanzaki G, et al. Acute graft-Versus-Host disease of the kidney in allogeneic rat bone marrow transplantation. PloS One (2014) 9(12):e115399. doi: 10.1371/journal.pone.0115399
46. Schmid PM, Bouazzaoui A, Schmid K, Birner C, Schach C, Maier LS, et al. Acute renal graft-Versus-Host disease in a murine model of allogeneic bone marrow transplantation. Cell Transplant (2017) 26(8):1428–40. doi: 10.1177/0963689717720295
47. Amin R, He R, Gupta D, Zheng W, Burmakin M, Mohammad DK, et al. The kidney injury caused by the onset of acute graft-versus-host disease is associated with down-regulation of αKlotho. Int Immunopharmacol (2020) 78:106042. doi: 10.1016/j.intimp.2019.106042
48. Mii A, Shimizu A, Kaneko T, Fujita E, Fukui M, Fujino T, et al. Renal thrombotic microangiopathy associated with chronic graft-versus-host disease after allogeneic hematopoietic stem cell transplantation. Pathol Int (2011) 61(9):518–27. doi: 10.1111/j.1440-1827.2011.02704.x
49. Petropoulou AD, Robin M, Rocha V, Ribaud P, Xhaard A, Abboud I, et al. Nephrotic syndrome associated with graft rejection after unrelated double cord blood transplantation. Transplantation. (2010) 90(7):801–2. doi: 10.1097/TP.0b013e3181ee4431
50. Beyar-Katz O, Davila EK, Zuckerman T, Fineman R, Haddad N, Okasha D, et al. Adult nephrotic syndrome after hematopoietic stem cell transplantation: renal pathology is the best predictor of response to therapy. Biol Blood Marrow Transplant (2016) 22(6):975–81. doi: 10.1016/j.bbmt.2015.12.014
51. Terrier B, Delmas Y, Hummel A, Presne C, Glowacki F, Knebelmann B, et al. Post-allogeneic haematopoietic stem cell transplantation membranous nephropathy: clinical presentation, outcome and pathogenic aspects. Nephrol Dialysis Transplantation (2007) 22(5):1369–76. doi: 10.1093/ndt/gfl795
52. Choe HK, Srivatana V, Gergis U, Shore TB, Mayer SA, Hsu J, et al. Kidney dysfunction post-allogeneic transplant: high incidence of TMA and kidney GvHD. Blood. (2017) 130(Supplement 1):5500. doi: 10.1182/blood.V130.Suppl_1.5500.5500
53. Hingorani S, Guthrie KA, Schoch G, Weiss NS, McDonald GB. Chronic kidney disease in long-term survivors of hematopoietic cell transplant. Bone Marrow Transplant (2007) 39(4):223–9. doi: 10.1038/sj.bmt.1705573
54. Jo T, Arai Y, Kondo T, Kitano T, Hishizawa M, Yamashita K, et al. Chronic kidney disease in long-term survivors after allogeneic hematopoietic stem cell transplantation: retrospective analysis at a single institute. Biol Blood Marrow Transplantation (2017) 23(12):2159–65. doi: 10.1016/j.bbmt.2017.08.016
55. Pelletier K, Côté G, Madsen K, Chen S, Kim SJ, Chan CT, et al. Chronic kidney disease, survival and graft-versus-host-disease-free/relapse-free survival in recipients of allogeneic hematopoietic stem cell transplant. Clin Kidney J (2022) 15(8):1583–92. doi: 10.1093/ckj/sfac091
Keywords: cord blood transplantation (CBT), allogenic stem cell transplantation, acute kidney injury (AKI), chronic kidney disease (CKD), bilirubin, steroid, graft versus host disease (GVHD)
Citation: Lopedote P, Xue E, Chotivatanapong J, Pao EC, Wychera C, Dahlberg AE, Thur L, Roberts L, Baker K, Gooley TA, Hingorani S and Milano F (2023) Acute kidney injury and chronic kidney disease in umbilical cord blood transplant recipients. Front. Oncol. 13:1186503. doi: 10.3389/fonc.2023.1186503
Received: 14 March 2023; Accepted: 26 April 2023;
Published: 16 May 2023.
Edited by:
Raffaele Palmieri, University of Rome Tor Vergata, ItalyReviewed by:
Yuyan Shen, Chinese Academy of Medical Sciences and Peking Union Medical College, ChinaShinsuke Takagi, Toranomon Hospital, Japan
Copyright © 2023 Lopedote, Xue, Chotivatanapong, Pao, Wychera, Dahlberg, Thur, Roberts, Baker, Gooley, Hingorani and Milano. This is an open-access article distributed under the terms of the Creative Commons Attribution License (CC BY). The use, distribution or reproduction in other forums is permitted, provided the original author(s) and the copyright owner(s) are credited and that the original publication in this journal is cited, in accordance with accepted academic practice. No use, distribution or reproduction is permitted which does not comply with these terms.
*Correspondence: Filippo Milano, fmilano@fredhutch.org
†These authors have contributed equally and share last authorship