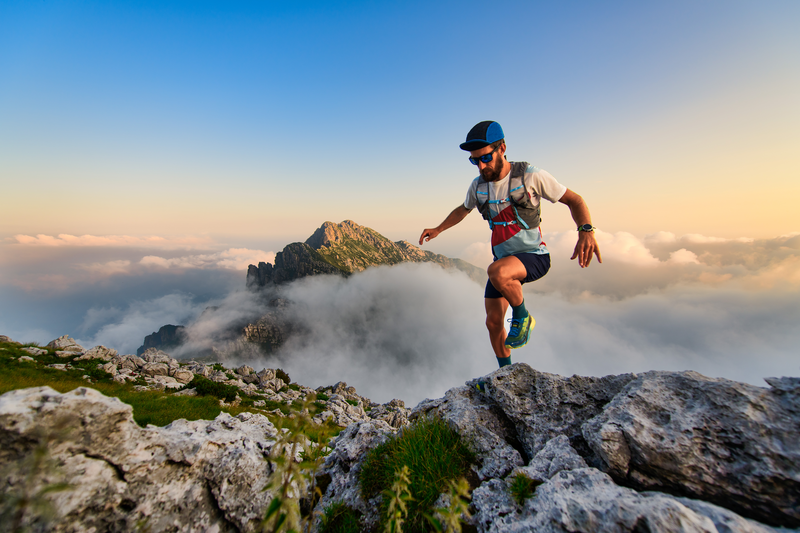
95% of researchers rate our articles as excellent or good
Learn more about the work of our research integrity team to safeguard the quality of each article we publish.
Find out more
EDITORIAL article
Front. Oncol. , 20 March 2023
Sec. Molecular and Cellular Oncology
Volume 13 - 2023 | https://doi.org/10.3389/fonc.2023.1179214
This article is part of the Research Topic Epigenetics and Cellular Plasticity in Glioblastoma View all 6 articles
Editorial on the Research Topic
Epigenetics and cellular plasticity in glioblastoma
Glioblastoma (GBM) is the most lethal and prevalent primary brain tumor in adults with a five-year survival rate of only around 5 percent, afflicting about 3.19 people in every 100,000 (1). While chemotherapy, radiotherapy, and surgical removal are offered as current standard treatments, these tumors have a high probability of recurrence, demanding improved treatment options (2). It is well established that GBM’s aggressiveness, treatment resistance, and recurrence is mostly driven by a subset of cells within the tumor bulk that exhibit stem cell properties (3–6). GBM stem cells (GSCs), originate mainly from subventricular zone (SVZ) neural stem cells that have acquired low-level oncogenic driver mutations (7). Although SVZ neural stem cells can undergo fate specific lineage restriction following exposure to differentiation cues (8, 9), GSCs fail to assume complete lineage specification and instead maintain some level of stemness potential (10–12).
Epigenetics and epitranscriptomics—molecular changes of cellular DNA, chromatin, and RNA—can alter the levels of gene transcripts and subsequently change the amount of proteins produced, including those responsible for tumor suppression, progression, or maintenance (13). Until now, there is limited understanding regarding the epigenetic factors that define GSCs’ failure to attenuate their stemness potential in the face of differentiation cues. Origin and maintenance of GSC plasticity are regulated by intrinsic cell processes affecting DNA, chromatin, and RNA of GSCs, as well as by extrinsic factors of the tumor microenvironment that propagate cancer stem cell phenotypes. Both intrinsic and extrinsic epigenetic pressure contribute to the ability of GSCs to remain plastic, maintain a poised state, and constantly interconvert between more and less differentiated states which, allows GSCs and differentiated progenies to adopt a population equilibrium that facilitates tumor persistence (10, 12, 14).
In the early 2000’s, Helen Blau et.al., introduced an evolving concept for adult stem cells suggesting that stem cells is rather a biological function instead of an entity (15). According to this view, at least some stem cells in adult tissues are highly plastic and amenable to changes when given the appropriate microenvironment. GBM is a perfect example of cancer evolution where cells retain an inherent level of plasticity through activation or maintenance of progenitor developmental programs. Thus, the concept of GSC as a function of GBM cancer cells rather than a separate entity could help explain the heterogeneity and cellular adaptation of these tumors. In such model, GSCs are interconverting between stem cell and poised stem cell stages through the constant influence of intrinsic and extrinsic epigenetic mechanisms (Figure 1). Studies of the GSC epigenome, epitranscriptome, non-coding RNome and 3D genome architecture can reshape our view of GBM tumor evolution and inform the design of new epigenetic targeting therapies.
Figure 1 Intrinsic and extrinsic epigenetic pressure maintains GBM cells at an adaptive or "poised" stem cell stage.
This Research Topic includes 5 original research papers. Yu et al., combined analysis of transcriptome and DNA methylation profiles of TCGA to derive a 6-gene model-based risk score. This approach allowed the authors to predict survival and the role of personalized treatment plans for GBM. Wang et al., analyzed tumor associated fibroblasts infiltration by Estimating the Proportion of Immune and Cancer cells (EPIC) based on multiple glioma databases. They describe a novel transcript signature that predicts glioma prognosis. Shi et al., showed that individual glioblastoma cell lines displayed increased expression of the short splice variant of YKL-40 after low serum treatment. In addition, unlike the full-length (FL) version, which was localized to all cell compartments, the short isoform could not be secreted and was localized only to the cytoplasm. Functionally, FL YKL-40 promoted cell proliferation and migration, whereas SV YKL-40 suppressed them. The authors described pathways that regulate the expression of the SV YKL-40 and discuss the significance for development of new therapies. Majc et al., investigated the use of bioactive peptides from venoms as novel therapies targeting cancer specific pathways in glioblastoma. Finally, Basilico et al., revealed that changing the rigidity of the mechanical environment tuned the response of glioblastoma cell lines through change in morphological features, epithelial-mesenchymal markers (E-, N-Cadherin), EGFR and ROS expressions in an interrelated manner. Their work highlights the importance of microenvironment stiffness in the regulation of glioblastoma invasive properties.
NT wrote the initial draft of the editorial. H-WL edited and both agreed on the final version.
The authors declare that the research was conducted in the absence of any commercial or financial relationships that could be construed as a potential conflict of interest.
All claims expressed in this article are solely those of the authors and do not necessarily represent those of their affiliated organizations, or those of the publisher, the editors and the reviewers. Any product that may be evaluated in this article, or claim that may be made by its manufacturer, is not guaranteed or endorsed by the publisher.
1. Tamimi AF, Juweid M. Glioblastoma. Vleeschouwer S, editor. Brisbane (AU): Exon Publications (2017).
2. Campos B, Olsen LR, Urup T, Poulsen HS. A comprehensive profile of recurrent glioblastoma. Oncogene (2016) 35:5819–25. doi: 10.1038/onc.2016.85
3. Bao S, Wu Q, McLendon RE, Hao Y, Shi Q, Hjelmeland AB, et al. Glioma stem cells promote radioresistance by preferential activation of the DNA damage response. Nature (2006) 444:756–60. doi: 10.1038/nature05236
4. Chen J, Li Y, Yu TS, McKay RM, Burns DK, Kernie SG, et al. A restricted cell population propagates glioblastoma growth after chemotherapy. Nature (2012) 488:522–6. doi: 10.1038/nature11287
5. Zheng H, Ying H, Yan H, Kimmelman AC, Hiller DJ, Chen AJ, et al. p53 and pten control neural and glioma stem/progenitor cell renewal and differentiation. Nature (2008) 455:1129–33. doi: 10.1038/nature07443
6. Zhu Z, Khan MA, Weiler M, Blaes J, Jestaedt L, Geibert M, et al. Targeting self-renewal in high-grade brain tumors leads to loss of brain tumor stem cells and prolonged survival. Cell Stem Cell (2014) 15:185–98. doi: 10.1016/j.stem.2014.04.007
7. Lee JH, Lee JE, Kahng JY, Kim SH, Park JS, Yoon SJ, et al. Human glioblastoma arises from subventricular zone cells with low-level driver mutations. Nature (2018) 560:243–7. doi: 10.1038/s41586-018-0389-3
8. Clarke DL, Johansson CB, Wilbertz J, Veress B, Nilsson E, Karlstrom H, et al. Generalized potential of adult neural stem cells. Science (2000) 288:1660–3. doi: 10.1126/science.288.5471.1660
9. Bonaguidi MA, McGuire T, Hu M, Kan L, Samanta J, Kessler JA. LIF and BMP signaling generate separate and discrete types of GFAP-expressing cells. Development (2005) 132:5503–14. doi: 10.1242/dev.02166
10. Caren H, Stricker SH, Bulstrode H, Gagrica S, Johnstone E, Bartlett TE, et al. Glioblastoma stem cells respond to differentiation cues but fail to undergo commitment and terminal cell-cycle arrest. Stem Cell Rep (2015) 5:829–42. doi: 10.1016/j.stemcr.2015.09.014
11. Lan X, Jorg DJ, Cavalli FMG, Richards LM, Nguyen LV, Vanner RJ, et al. Fate mapping of human glioblastoma reveals an invariant stem cell hierarchy. Nature (2017) 549:227–32. doi: 10.1038/nature23666
12. Dirkse A, Golebiewska A, Buder T, Nazarov PV, Muller A, Poovathingal S, et al. Stem cell-associated heterogeneity in glioblastoma results from intrinsic tumor plasticity shaped by the microenvironment. Nat Commun (2019) 10:1787. doi: 10.1038/s41467-019-09853-z
13. Montella L, Cuomo M, Del Gaudio N, Buonaiuto M, Costabile D, Visconti R, et al. Epigenetic alterations in glioblastomas: Diagnostic, prognostic and therapeutic relevance. Int J Cancer (2022). doi: 10.1002/ijc.34381
14. Auffinger B, Tobias AL, Han Y, Lee G, Guo D, Dey M, et al. Conversion of differentiated cancer cells into cancer stem-like cells in a glioblastoma model after primary chemotherapy. Cell Death Differ (2014) 21:1119–31. doi: 10.1038/cdd.2014.31
Keywords: epigenetics, cancer stem cell, plasticity, poised, glioblastoma
Citation: Lo H-W and Tapinos N (2023) Editorial: Epigenetics and cellular plasticity in glioblastoma. Front. Oncol. 13:1179214. doi: 10.3389/fonc.2023.1179214
Received: 03 March 2023; Accepted: 09 March 2023;
Published: 20 March 2023.
Edited and Reviewed by:
Tao Liu, University of New South Wales, AustraliaCopyright © 2023 Lo and Tapinos. This is an open-access article distributed under the terms of the Creative Commons Attribution License (CC BY). The use, distribution or reproduction in other forums is permitted, provided the original author(s) and the copyright owner(s) are credited and that the original publication in this journal is cited, in accordance with accepted academic practice. No use, distribution or reproduction is permitted which does not comply with these terms.
*Correspondence: Nikos Tapinos, bmlrb3NfdGFwaW5vc0Bicm93bi5lZHU=
Disclaimer: All claims expressed in this article are solely those of the authors and do not necessarily represent those of their affiliated organizations, or those of the publisher, the editors and the reviewers. Any product that may be evaluated in this article or claim that may be made by its manufacturer is not guaranteed or endorsed by the publisher.
Research integrity at Frontiers
Learn more about the work of our research integrity team to safeguard the quality of each article we publish.