- 1Oncology Unit (UOC) Oncologia A, Department of Radiological, Oncological and Anathomo-patological Science, Policlinico Umberto I, Sapienza University of Rome, Rome, Italy
- 2Oncology Unit, Department of Clinical and Molecular Medicine, Sant’ Andrea University Hospital, Sapienza University of Rome, Rome, Italy
- 3Department of Clinical and Molecular Medicine, Anatomia Patologica Unit, Sant’ Andrea University Hospital, Sapienza University of Rome, Rome, Italy
- 4Scientific Direction, Istituto Dermopatico dell’Immacolata (IDI-IRCCS), Rome, Italy
Introduction: Chemokines are small, secreted peptides involved in the mediation of the immune cell recruitment. Chemokines have been implicated in several diseases including autoimmune diseases, viral infections and also played a critical role in the genesis and development of several malignant tumors. CXCL12 is a homeostatic CXC chemokine involved in the process of proliferation, and tumor spread. Pancreatic ductal adenocarcinoma (PDAC) is one of the most aggressive tumors, that is still lacking effective therapies and with a dramatically poor prognosis.
Method: We conducted a scientific literature search on Pubmed and Google Scholar including retrospective, prospective studies and reviews focused on the current research elucidating the emerging role of CXCL12 and its receptors CXCR4 – CXCR7 in the pathogenesis of pancreatic cancer.
Results: Considering the mechanism of immunomodulation of the CXCL12-CXCR4-CXCR7 axis, as well as the potential interaction with the microenvironment in the PDAC, several combined therapeutic approaches have been studied and developed, to overcome the “cold” immunological setting of PDAC, like combining CXCL12 axis inhibitors with anti PD-1/PDL1 drugs.
Conclusion: Understanding the role of this chemokine’s axis in disease initiation and progression may provide the basis for developing new potential biomarkers as well as therapeutic targets for related pancreatic cancers.
1 Introduction
Pancreatic ductal adenocarcinoma (PDAC) is one of the most aggressive tumors and the seventh leading cause of cancer-related death worldwide (1). The incidence of PDAC is increasing by 0.5% to 1.0% per year (2) and the 5-years survival rate is 9% (3). Approximately 80% of patients present with advanced disease or distant metastasis at diagnosis, with a very poor prognosis (4). The lack of effective therapeutic opportunities, in part due to the inter- and intratumor heterogeneity, contributes to making PDAC still a deadly malignancy. Multiple signaling pathways involved in pancreatic cancer tumorigenesis - Ras-ERK pathway (5), multiple genes/proteins, such as NOP14 (6), DCLK1 (7), interleukin-22/interleukin-22 receptor (8), FoxQ1 (9), CHIP (10) and microRNAs (11) - were recently found to play an important role in migration and invasion of pancreatic cancer cells. Besides this complex set of pathways, it has recently been highlighted the effect of chemokines in malignant behaviors of cancer cells (12). Chemokines are chemoattracting proteins that binds to and activate their corresponding receptors. There are four families of chemokines, CXC, CC, CX3C, and C. Among them, CXC ligand 12 (CXCL12) of the CXC chemokines family, was previously shown to have important impact on the proliferation and invasion of many types of cancer cells, through its specific receptors CXCR4 (12) CXCR7 (13). In addition, CXCR7 is involved in a broad range of cancer progression processes, such as growth, migration, chemotaxis, adhesion and spreading (14). Specifically, in pancreatic cancer CXCL12-CXCR7 axis promotes migration and invasion of pancreatic cancer cells, through mTOR and Rho/ROCK pathway (15). Tough, it has been hypothesized a prognostic role and a potential therapeutic target for this pathway. In this review, we summarize the current evidences about CXCL12 - CXCR4 - CXCR7 mechanisms of action, especially in PDAC, with the aim to identify new potential biomarkers and therapeutic targets for this aggressive malignancy.
2 Methods
A comprehensive literature search in the electronic database of PubMed, and Google Scholar was conducted in January 2023. The literature search was performed using keywords. Retrospective and prospective studies published from January 2020 to December 2022 including the following key words: “CXCL12”, “CXCR4”, “CXCR7”, “axis”, “pancreatic cancer”, “CXCL12 axis in pancreatic cancer”, “CXCL12 and carcinogenesis”, “CXCL12 inhibition”, “CXCL12 and tumor microenvironment” were included in the analysis.
3 Critical analysis
3.1 Mechanism of action of chemokines: The role in the generation and development of malignant tumors, including PDAC
Chemokines are small, secreted peptides involved in the mediation of immune cell recruitment. They are considered key regulators of development, differentiation and anatomic location of leukocytes and can control cell movement during inflammation and homeostasis (16, 17). Based on the great amount of pathway in which they are involved, chemokines play a critical role in several diseases which comprise autoimmune diseases, viral infections as well as many cancer types (18–24).
Chemokines are divided in four subtypes depending on the position and number of cysteine residues in the N-terminus: C, CC, CXC and CX3C chemokines (25). There are two families of chemokines receptors: conventional (cCKR) and atypical chemokine receptors (ACKR). They exert their functions binding to seven- transmembrane- spanning G protein-coupled cell-surface receptors (26). Ligand-receptor binding induces a conformational change in the receptor which led to intracellular signal transduction and activation of downstream signaling pathways (26–28) (29). In pancreatic cancer, CXCL12 chemokine, released by activated cancer‐associated fibroblasts (CAFs) binds to its two receptors CXCR4 and ACKR3 on the cells surface, activating phospholipase C, MAPK, and PI3K-Akt-mTOR, as well as JAK/STAT pathways, promoting tumor growth and invasion (see Figure 1) (31–34).
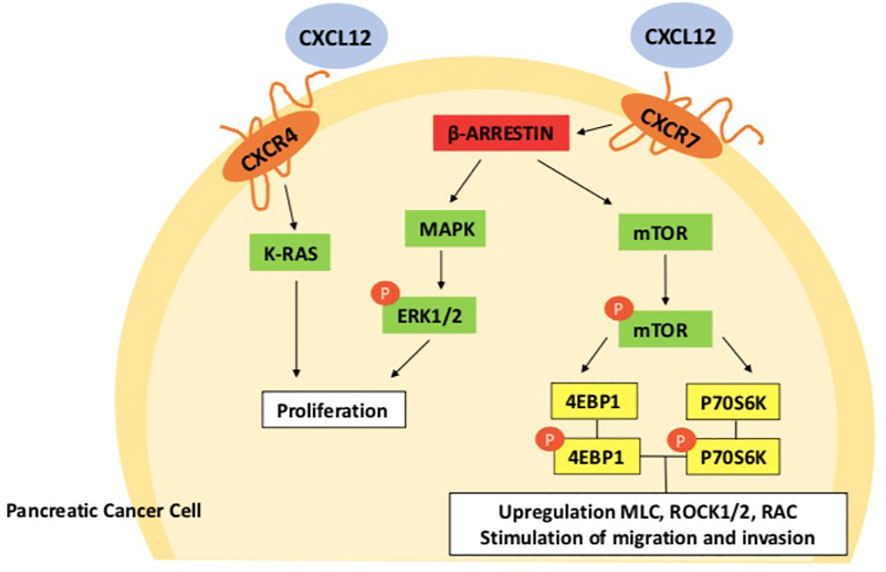
Figure 1 CXCL12-CXCR4-CXCR7 axis in pancreatic cancer cell. After CXCL12 and chemokine (C-X-C motif) receptor 7 (CXCR7) are activated, β-arrestin 2 causes extracellular regulatory protein kinases 1/2 (ERK ½) to become phosphorylated, which promotes the growth of pancreatic cancer cells. By activating the mammalian target of rapamycin (mTOR) signaling pathways, CXCR 7 interacts to stromal cell-derived factor-1 to increase the migration and invasion of pancreatic cancer cells (30). CXCR7, Chemokine (C-X-C motif) receptor 7; SDF-1, Stromal cell-derived factor-1; ERK1/2, Extracellular regulated protein kinases 1/2; mTOR, Mammalian target of rapamycin; Rho/ROCK, Rho/Rho associated coiled-coil forming protein kinase.
Some chemokines, specifically the CXC chemokines group, are involved in cancer growth promoting angiogenesis. The presence or lack of the ELR(Glu-Leu-Arg) motif allows CXC chemokines to be classified as angiogenic or angiostatic. More specifically, angiogenesis is stimulated by ELR-positive chemokines (CXCL1, CXCL6, and CXCL8) while it is inhibited by ELR-negative chemokines (CXCL4, CXCL10 an endogenous tumor angiogenesis inhibitor (35) and CXCL14) (36). CXCL12 (SDF-1) is an ELR-negative chemokine but, despite being ELR negative it represents one of the most powerful angiogenesis-promoting chemokines (37).
Focusing on the role of the CXCL12 axis, in vivo and in vitro studies revealed a relationship between microvessel density and the degree of CXCL12/CXCR4 expression in cancer cells. There are several ways through which CXCL12/CXCR4 axis could control tumor angiogenesis: increasing vascular endothelial growth factor (VEGF) expression in tumor tissue via the PI3K/Akt signaling pathway (38); increasing several angiogenesis-related genes in cancer cells (39) and directing endothelial progenitor cells toward the site of neovascularization (40, 41). Indeed, a study showed that human intestinal microvasculature co-expresses CXCR4 and CXCL12 and that CXCL12 promotes chemotaxis, proliferation of endothelial cells and angiogenesis (42). Moreover, CXCL12 positively affects VEGF expression by endothelial cells; consequently, increased VEGF levels up-regulate CXCR4 levels (43).
CXCL12 axis can influence tumor cell biology by direct effects, promoting cancer cell growth, metastasis, and angiogenesis, but also indirectly, recruiting CXCR4/CXCR7-positive cancer cells to CXCL12- expressing organs (44). An experimental study showed that CXCL12 was only weakly expressed in quiescent tissues, but when VEGF expression was experimentally increased, using the conditional genetic switch, the expression of CXCL12 in perivascular fibroblasts and smooth muscle cells grown dramatically. VEGF-dependent mechanisms also led to the induction of CXCL12 in ischemic, inflammatory, and neoplastic tissues (45).
Besides angiogenesis, CXCL12 and its receptor CXCR4 are involved in promoting tumor invasion and metastases in several neoplasm (46–48) including PDAC (15). Some evidence suggested a possible role of CXCL12/CXCR7 on the epithelial-to-mesenchymal transition, a critical step that initiates metastasis in colorectal (49), esophageal (50) and ovarian cancers (51). The relation between metastasizing and angiogenesis was demonstrated by the inhibition of phosphoglycerate kinase (PGK) expression, an angiostatic kinase, by the great expression of CXCL12/CXCR4 on metastasis (52). Moreover, some studies showed that in animal models of breast, renal cell, and non-small cell lung cancer, immunoneutralization of CXCL12 or CXCR4 attenuated tumor metastases, but had no effect on the extent of angiogenesis or tumor size of the primary tumor (53, 54), suggesting that the CXCL12–CXCR4 biological axis promotes metastases independently from angiogenesis (55).
In addition, chemokines are key components of immune system response against cancer cells (56, 57). On the one hand, oncogenic driver mutations and continuous immunological pressure frequently control the expression of chemokines and their receptors, dynamically modulating the tumor immune environment, on the other, the complex and dynamic network of cytokines, chemokines, and growth factors in the tumor microenvironment (TME) promotes intracellular and intercellular communication that modulates tumor/stroma interactions, including immune responses. The TME consists of resident non-cancerous cells like stromal fibroblasts, endothelial cells, and immune cells, proteolytic enzymes, growth factors, inflammatory cytokines, and the extracellular matrix (ECM) (58). In TME paracrine and autocrine signaling by chemokines, generate complex communications between tumor cells and the TME cells (59). Chemokines facilitate interaction between cancer cells and the TME’s surrounding non-neoplastic cells, such as fibroblasts and endothelial cells. Additionally, chemokines boost neutrophil infiltration and activate tumor-associated macrophages (TAM) (60). CXCL12 in the TME could be secreted by cancer-associated fibroblasts (CAF), stimulating tumor growth directly, acting through CXCR4 and promoting invasiveness (61).
Differences in quantity, composition, functional status, location, and type of immune cells of TME, have an impact on the prognosis and therapeutic response, assuming pro-tumor or anti-tumor characteristics. The TME and the immune profile of cancers have a different behavior, not only across tumor types but also among patients with the same tumor type and in different tumor sites within the same patient (62–64). In colon cancer, chemokines in TME have direct pro-tumor effects: CCL2 targeting vascular endothelial cells via the Janus kinase 2 (JAK2)–STAT5 and p38 mitogen-activated protein kinase pathways, CCL3 and CCL5 can promote tumor invasion and metastasis (64). In non-small cell lung cancer, CXCR4+ tumor cells may have stem-like properties, showing a high metastatic potential and radiation resistance (65). In pancreatic cancer the CC-chemokine ligand 20 (CCL20) and its receptor CC-chemokine receptor 6 (CCR6) mediate the cancer migration and metastasizing. CCL20 is the strongest target gene in TNF-related apoptosis-inducing ligand (TRAIL) resistance through the NF-kB subunit RelA. Both autocrine CCL20 and paracrine immune cell recruitment help pancreatic cancer cells develop TRAIL resistance (66). Immune cells known as TAM that exhibit M2-type macrophage markers have been found to accelerate tumor growth and inhibit cytotoxic T-cell defenses. Additionally, IL4-stimulated M2-type macrophages highly express CCL20 and support pancreatic cancer cell invasion and the epithelial-mesenchymal transition (EMT). Another study found that the CCL20-CCR6 axis enhanced liver metastasis and pancreatic cancer growth in a mouse model (67).
Finally, The CXCL12-CXCR4-CXCR7 axis promotes tumor-related inflammation and metastasis, by regulating the trafficking of immune and tumor cells (68–70), while recent research has highlighted an immunosuppressive role of the CXCL12/CXCR4 axis through the recruitment of specific populations of immunosuppressive cells within the TME (71)
In pancreatic cancer, a high expression of CXCL12 and CXCR4 is correlated with a worse prognosis (72, 73).
3.2 CXCL12 role in carcinogenesis and progression: Preclinical studies and observational evidence in solid tumors, including PDAC
As described above, CXCL12 and its receptors activate key survival pathways, playing a central role in several processes involved in cancer development and growth, including PDAC (74). In solid and hematologic malignancies, the CXCL12-CXCR4-CXCR7 axis was related to resistance to therapies. Moreover, high CXCL12 expression correlates with worst survival in patients with esophagogastric, pancreatic or lung cancer (72). Indeed, CXCL12 can promote local invasion of cancer cells, while loss of CXCL12 promotes tumor cell migration to organs expressing high levels of CXCL12 such as the liver, bone marrow and lung (72).
In mycosis fungoides (MF) the CXCL12/CXCR4 axis is one of the mechanisms by which CAFs promote tumor cell migration and drug resistance. A study demonstrated that CXCL12 was significantly upregulated in fibroblasts of MF patients when compared to control, enhancing MF resistance to chemotherapy (75). Similarly, CXCL12 and its receptors CXCR4 and CXCR7 are also involved in prognosis and progression of different solid tumors (76). In breast cancer CXCR7-deficient mouse, higher local recurrences were reported after resection, suggesting that CXCR7 may have tumor-suppressor functions involved into the metastatic cascade (77).
With regards to PDAC carcinogenesis, increased CXCL12 levels were directly associated with increasing PanIN grade (78). CXCL12- CXCR4 axis plays an important role in the progression and organ specific metastatic spread (79), enhances cancer growth and restrict immune surveillance across the tumor through local autocrine and paracrine mechanisms (80).
Furthermore, in PDAC, CXCL12 induces shape changes in pancreatic cancer cells which make them resemble to migrating cells and increase cell migration in vitro (81) [e.g. increased expression of MMP-2, MMP-9 and uPA (82)]. To support tumor growth and expansion, CXCL12 axis can attract CXCR4-positive inflammatory, vascular, and stromal cells into the tumor mass, favoring a cross talking process with TME (83). The crosstalk between CXCL12/CXCR4 signaling and EMT and loss of cell adhesion which promotes cell cycle progression (82) was highlighted also in other type of cancers, like human sacral chondrosarcoma (84).
In PDAC tissues and pancreatic cancer cell lines SATB-1 - a nuclear matrix attachment region-binding protein which controls the transcription and expression of all gene - is overexpressed, and CXCL12, which is released by CAFs, increases SATB-1 expression (85, 86).
CXCR4 and CXCR7 are often co-expressed in PDAC specimens and cell lines, with discordant results. In early pancreatic cancer cell lines, CXCR7 knockdown, but not CXCR4 knockdown, does arrest CXCL12- mediated proliferative changes. Opposite results were obtained for the metastatic pancreatic cancer cell line. These results indicate that both CXCR4 and CXCR7 can mediate CXCL12-driven proliferation. CXCR4 and CXCR7 signaling is b-arrestin-2-dependent and controls CXCL12 signals to the MAPK pathway (87).
In advanced colorectal cancer, CXCR7 expression is associated with lymph node metastasis and progression, being employed as prediction marker for lymph node involvement (88). Generally, tumors that exploit CXCL12/CXCR4 axis tend to grow and metastasize in tissues like the liver, lung, adrenal glands, and bone marrow, whereas tumors that exploit CXCL12/CXCR7 axis are more often responsible for lymphatic metastases due to CXCR7 expression in endothelial cells, T and B lymphocytes, and dendritic cells (13, 89). The interplay among CXCL12/CXCR4/CXCR7 axis and metastases is reinforced by a preclinical study that evidenced how the systemic treatment with CXCR7 antagonists reduces tumor expansion in lungs of experimental mice inoculated with HT-29 or C26 cancer cells (90).
In small cell lung cancer (SCLC), CXCL12 induces the overexpression of CXCR4 through some integrins (2, 4, 5, and ß1 integrins), which results in a significant affinity of cancer cells for extracellular matrix and confers chemoresistance to tumors. CXCR4 inhibitors may lessen this chemoresistance effect (91).
The CXCL12-CXCR4/CXCR7 axis is a highly conserved mechanism for organogenesis and tissue healing in gastrointestinal malignancies (gastric, pancreatic, and colorectal cancer). A complete knockout is incompatible with life. Cancer cells may be able to take advantage of this chemokine pathway due to genetic abnormalities. Increased CXCR4 expression on tumor cells not only enable them to spread to CXCL12 gradients that are phisiologically present in the body, but CXCR4 binding on tumor cells also promotes resistance to therapies by reducing pro-apoptotic signaling. Additionally, by interfering with proper immune cell migration, changes in the ability of TME stromal cells to produce CXCL12 may hinder effective cell-mediated tumor killing, resulting in enhanced tumor growth, showing how CXCL12-CXCR4/CXCR7 axis could be considered as a mechanism of immune resistance in gastrointestinal malignancies (92).
Considering CXCL12–CXCR4/CXCR7 axis involvement in survival, tumor growth, angiogenesis, metastasis, TME, and drug resistance, it has been investigated as promising target for therapeutic interventions (93, 94).
3.3 -The axis CXCL12-CXCR4-CXCR7 in PDAC: Could it be considered as new potential biomarkers and therapeutic targets?
CXCL12-CXCR4-CXCR7 axis has been associated to cancer progression in several tumor types (53, 95, 96). Thus, it seems interesting to investigate their possible role in PDAC environment. The TME induces changes in cellular components (97) by secreting exosomes and soluble factors such as VEGF, CXCL1, and CXCL8- (98, 99) to promote colonization (100, 101). As previously described, in PDAC, TME may have a tumor-promoting or tumor-suppressive action based on the expression of specific factors such as CAFs, stellate cells, different immune cells and cytokines (102). Hence, the TME, with its complexity of signal pathways (103), acts as a key determinant by which PDAC acquires therapeutic resistance to currently available treatment and it could represent a target for new therapeutic strategies based on context-dependent stromal alterations (104, 105). CXCL12/CXCR4 axis is one of the most prominent chemokine moderators of the TME (68). The examination of formalin-fixed paraffin embedded (FFPE) specimens obtained from patients who had undergone resection for pancreatic adenocarcinoma, showed both high frequency of CXCR4/CXCR7 co-expression in human pancreatic cancer tissues compared to normal tissues and the increase in staining intensity over tumor stage (87), confirming a decisive role in the pathogenesis of PDAC.
In literature has been reported the effect of upregulation of SATB-1 – a nuclear matrix attachment region-binding protein called AT-rich sequence-binding protein 1- by CXCL12, CAFs of pancreatic cancer stroma via the CXCL12/CXCR4 axis. CXCL12/CXCR4/SATB-1 axis accounts for the malignant progression, but also gemcitabine resistance of pancreatic cancer cells, suggesting a potential target of chemosensitivity in therapeutic management of PDAC patients (86). In addition, CXCL12 overexpression correlated with the lymph node metastasis, whereas SATB-1 overexpression correlated with differentiation, T stage, TNM stage, and lymph node metastasis. Furthermore, higher CXCL12 or SATB-1 staining intensity correlated with poorer prognosis in PDAC patients suggesting also a possible prognostic role in these tumors (86).
The implication of chemokines in PDAC tumorigenesis, prognosis, and resistance to therapy and the increasingly urgent need to understand the signaling mechanisms, supported the development of molecularly targeted pancreatic cancer therapies.
Recently, several preclinical and ongoing clinical studies have been analyzing the therapeutic option of CXCL12-CXCR4/CXCR7 axis. Moreover, different molecules have been developed in several areas of medicine as antagonists of the CXCR4 receptor (106, 107). In vitro studies showed that, resistance to gemcitabine mediated by CXCR4 chemokine receptor (108) and polo-like kinase 1 (PLK1) (109, 110) can be overcome combining a polymeric cholesterol-modified CXCR4 (PAMD-CHOL) antagonist to a PLK1 knockdown by siRNA. The biodistribution of the nanoparticles in orthotopic pancreatic cancer models revealed strong accumulation in primary and metastatic tumors. In a therapeutic study in vivo, the triple combination of gemcitabine with PAMD-CHOL/siPLK1 showed superior anticancer activity when compared with single and dual combination controls (111). An interesting retrospective analysis of the INT-11 trial demonstrated that tipifarnib, a farnesyl transferase inhibitor that inhibits CXCL12 expression in pancreatic fibroblasts, could sensitize PDAC to gemcitabine in a subset of PDAC patients (112).
In orthotopic pancreatic tumor–bearing mice treated with gemcitabine combined to a CXCR4 antagonist (AMD3100) or hedgehog inhibitor (GDC-0449) showed significant suppression of tumor growth. Those target agents act on a pathway signaling which promote pancreatic tumor desmoplasia by inducing proliferation and differentiation of pancreatic stellate cells into myofibroblasts (113).
The involvement of CXCR4 in radiation resistance was previously demonstrated in several tumors (114–116). Kato and colleagues confirmed the correlation between the high intensity immunostaining of CXCR4 in pancreatic cancer cells and survival or stage of cancer. Moreover, they found that CXCR4 expression and invasion were enhanced in radiation-resistant pancreatic cancer cell lines compared to normal cancer cell lines. The CXCR4 antagonist AMD070 suppressed the cancer cell invasion enhanced by CXCL12 treatment, and when used in combination with irradiation, AMD070 suppressed the colonization of radiation-resistant pancreatic cancer cells (117). Alternatively, CXCR4-CXCL12 axis can be inhibited by directly preventing CXCL12 binding to CXCR4 or CXCR7. Olaptesed pegol (NOX-A12) is a novel non-immunogenic synthetic oligonucleotide that inhibits the chemoattractant stromal cell-derived factor-1 (SDF-1 or CXCL12) (118, 119). Currently, there is a Phase 1/2 clinical trial looking at Olaptesed in combination therapy with Pembrolizumab in metastatic pancreatic and colorectal cancers (NCT03168139). The preclinical studies focused on the therapeutic role of CXCL12-CXCR4/CXCR7 axis are summarized in Table 1.
3.4 Immunoresistance phenotype of PDAC: The role of immunotherapy
The TME of PDAC is highly immunosuppressive and is characterized by an extensive and dense fibrous stroma that can account for up to 80% of the total tumor volume (120) and is responsible for an abundant desmoplastic reaction, creating a physical barrier that makes the drug perfusion difficult (121). The crosstalk between pancreatic cancer-associated stroma, cancer cells and soluble proteins such as cytokines and growth factors (122) could represent a target of therapeutic strategies modulating tumor-promoting and tumor-suppressive signals (104, 105). The immune resistance of PDAC cannot be explained only by the presence of desmoplastic peritumoral reaction, but also by further mechanisms such as the minimal antitumor T cells infiltration (123) and the synergism with other regulatory immune cells to evade immune surveillance (124), tumor cell-intrinsic pathways involving the KRAS oncogene (125) and its downstream effector PIK3CA (126), the tumor suppressor gene p53 (127) and NF-κB that contributes to tumor growth by increasing the expression of CXCL12, which prevents cytotoxic T cells from infiltrating the tumor and killing cancer cells (128, 129).
The production of immunosuppressive chemokines and cytokines represents one important mechanism of immune evasion in PDAC and the CXCL12-CXCR4/CXCR7 chemokine axis, as described above, has a prominent role in modulating the trafficking of immune cell populations in the microenvironment (92).
Although all these evidences highlights how the TME may represent an area of interest in pancreatic cancer immuno-therapeutic exploration, the efficacy of immunotherapy in PDAC appeared poorly encouraging survival benefit (130–132) despite the observation of PD1 expression in such patients (130, 133, 134). Indeed, the PDAC was historically defined as a non-immunogenic tumor that displays a paucity of antigens to be recognized as foreign by the host immune system, the presence of peripheral T cells specific for an abundant PDAC antigen, mesothelin, and the lack of these T cells in the tumor microenvironment are consistent with the existence of local immunosuppression (135). On the one hand the limited T-cell infiltration has been hypothesized to be one crucial reason for the failure of checkpoint inhibitor therapy (136–138), on the other, the inhibition of the CXCL12/CXCR4 axis appeared as a promising TME modulatory strategy to improve ICIs outcomes (136, 139).
Considering the mechanism of immunomodulation of the CXCL12-CXCR4-CXCR7 axis, and of the potential interaction with the microenvironment in the PDAC, several combined therapeutic approaches have been studied and developed, to overcome the “cold” immunological setting of PDAC.
Recently, a dose escalation trial was conducted with the objective to assess the impact on the tumor microenvironment of selective CXCR4 antagonist named AMD3100 - also known as plerixafor - in advanced PDAC, ovarian and colorectal cancer, to identify the proof of mechanism, by demonstrating alterations in T-cell tumor distribution, ideally associated with loss of tumor cells, measured by immunostaining, and changes in FDG uptake (NCT03277209).
The depletion of tumor stroma or targeting CXCL12 from CAF exhibited a synergistic effect with immune checkpoint inhibitors in PDAC generating genetically engineered mouse models, suggesting that the conditioning of the PDAC stroma could be a prerequisite for attaining therapeutic responses to immunotherapies (136, 140). An interesting preclinical study showed how in a murine pancreatic cancer model, despite the presence of antitumor T cells, immunotherapeutic antibodies (anti-PDL1) are ineffective, but removing the CAF expressing fibroblast activation protein (FAP) from tumors or inhibiting the interaction of its chemokine, CXCL12, with CXCR4, permitted immune control of tumor growth and uncovered the efficacy of these immunotherapeutic antibodies. FAP+ CAFs are the only tumoral source of CXCL12 and administering AMD3100 also revealed the antitumor effects of an immunotherapeutic antibody and greatly diminished cancer cells (136).
Inhibiting CXCR4 by continuous infusion of AMD3100 in an experimental microsatellite stable (MSS) PDAC and colorectal cancer human cells, induced an integrated immune response with intratumoral T and NK cells accumulation and activation and B cell response, that was detected by transcriptional analysis of paired biopsies of metastases from patients (141). Combination therapy showed efficacy through intravenous injection of traps - plasmid DNA encoding for CXCL12 and PD-L1 - in mice bearing orthotopic pancreatic cancer. Expression of traps was mainly seen in the tumor. Moreover, combination trap therapy shrunk both tumor and metastases, and significantly prolonged the host survival. The authors also found a modification of the immunosuppressive TME as CXCL12 trap allowed T-cell penetration into the tumor, and PD-L1 trap allowed the infiltrated T-cells to kill the tumor cells (139).
In clinical practice most studies addressing the CXCL12-CXCR4 axis have tackled hematological malignancies (142, 143), while in solid tumors the trials are less numerous (Table 2).
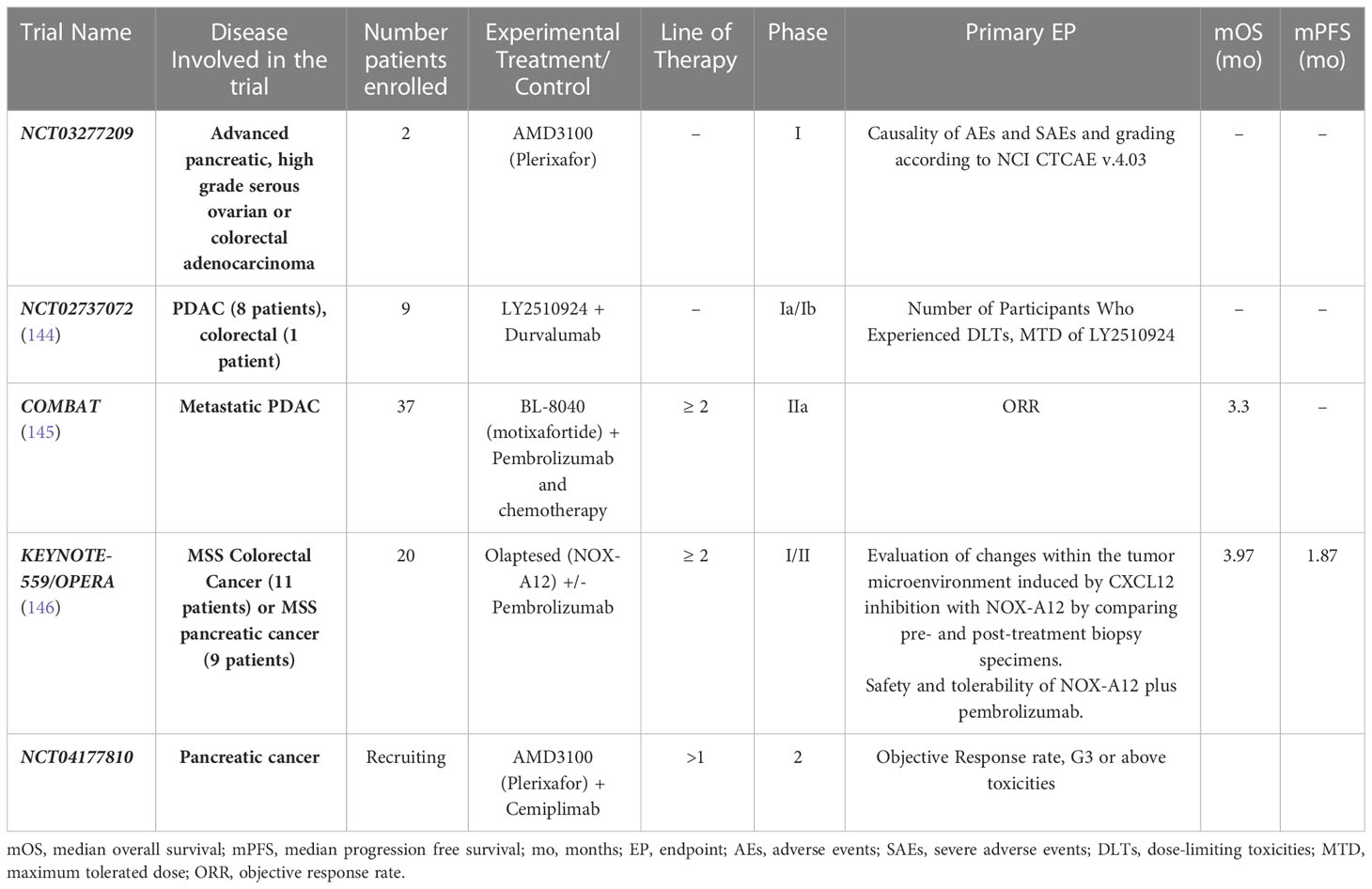
Table 2 Recruiting and completed trials of CXCL12-CXCR4-CXCR77 inhibitors plus immunotherapy in PDAC.
The attractive immunomodulating role of CXCR4 was investigated in phase I in which the primary objective was to assess the maximum dose tolerated and safety of an antagonist of CXCR4 LY2510924 administered subcutaneous daily in combination with durvalumab in patients with advanced solid tumors (8 PDAC, 1 colorectal). No dose limiting toxicities were reported and about activity four patients reported stable disease and one patient partial response (144).
In pretreated PDAC patients, monotherapy with BL-8040 - an inhibitor of CXCR4 activity - followed by repeated cycles of pembrolizumab every 3 weeks and BL-8040 three times weekly, showed a DCR of 34.5%, although only one patient reported a partial response. After this priming phase and based on the results of this initial cohort, the expansion cohort of triple combination therapy of BL-8040, pembrolizumab and irinotecan-based chemotherapy, showed surprisingly, an ORR, DCR and median duration of response of 32%, 77% and 7.8 months respectively, suggesting a possible expansion of the chemotherapy’s benefit by combined CXCR4 and PD-1 blockade (145).
In the phase I/II Keynote-559 patients affected by microsatellite stable (MSS) metastatic colorectal and pancreatic cancers were treated with a fixed dose of olaptesed pegol (also known as NOX-A12) as a monotherapy for 2 weeks, followed by a combined therapy with 200 mg pembrolizumab one time every 3 weeks until disease progression or limiting toxicity. Olaptesed pegol is an oligonucleotide that binds to SDF-1 thereby preventing the binding of SDF-1 to its receptors CXCR4 and CXCR7 and blocking the subsequent receptor activation, with consequent prevention of angiogenesis, proliferation, invasion and metastasis. Furthermore NOX-A12 facilitating the influx of immune effector cells into solid tumors, may allow an effective response to immune checkpoint inhibitors. The treatment with NOX-A12 and pembrolizumab was well tolerated and demonstrated to be able to stabilize the disease in heavily pretreated MSS patients for prolonged periods, for almost a quarter of the patient’s overall survival close to 12 months could be reached (146).
4 Future perspective and conclusions
Considering the critical and non-systematic nature of this review, and the heterogeneity of the included studies, which not allow conclusive evidence, our work highlights the crucial role that plays CXCL12-CXCR4-CXCR7 axis in carcinogenesis, disease progression and drug resistance of pancreatic cancer. Moreover, compared to previous published reviews (80, 147), ours work focused on the immunomodulatory activity of this chemokine’s axis, speculating on the possibility of exploiting them for therapeutic purposes.
This axis represents, as reported in a recent meta-analysis a potential predictive factor of worse prognosis of PDAC, esophagogastric and lung cancers (72). Consequently, a deeper understanding of the pancreatic cancer biology, including new insight into the tumor metabolism and TME, could lead to the development of promising and innovative therapeutic strategies.
According to this evidence, several preclinical and clinical studies are ongoing to analyze therapeutic opportunities targeting the CXCL12-CXCR4/CXCR7 axis in the complex field of pancreatic TME. Currently it has been widely supposed that targeting a single molecule or single pathway was unlikely to yield more pancreatic cancer therapies, therefore future approaches about combination and/or multi-modal strategies that target multiple features of the TME simultaneously might be more successful than the single agent therapy. Furthermore, while the possibility to overcome resistance to radiotherapy and chemotherapy treatment has been already investigated - although mainly in preclinical studies - the immunomodulating role of CXCL12-CXCR4/CXCR7 antagonists could represent a future challenge in the therapeutic landscape of PDAC.
In conclusion, the present review suggests how modulating TME with a combination of chemotherapy, PD-1/PD-L1 and CXCL12-CXCR4-CXCR7 axis antagonists could represent one of the future therapeutic options to improve the immune responses in immune refractory cancers, including PDAC.
Author contributions
Conception/Design: MR. Data analysis and interpretation: MR, GA. Manuscript writing: GA, MC, MR. Final approval of manuscript: MR, GA. Supervision: GB, PM, FM, EP, DS. All authors contributed to the article and approved the submitted version.
Conflict of interest
The authors declare that the research was conducted in the absence of any commercial or financial relationships that could be construed as a potential conflict of interest.
Publisher’s note
All claims expressed in this article are solely those of the authors and do not necessarily represent those of their affiliated organizations, or those of the publisher, the editors and the reviewers. Any product that may be evaluated in this article, or claim that may be made by its manufacturer, is not guaranteed or endorsed by the publisher.
References
1. Sung H, Ferlay J, RL S, Laversanne M, Soerjomataram I, Jemal A, et al. Global cancer statistics 2020: GLOBOCAN estimates of incidence and mortality worldwide for 36 cancers in 185 countries. CA Cancer J Clin (2021) 71(3):209–49. doi: 10.3322/caac.21660
2. Rawla P, Sunkara T, Gaduputi V. Epidemiology of pancreatic cancer: Global trends, etiology and risk factors. World J Oncol (2019) 10:10–27. doi: 10.14740/wjon1166
3. Miller KD, Fidler-Benaoudia M, Keegan TH, Hipp HS, Jemal A, Siegel RL. Cancer statistics for adolescents and young adults, 2020. CA Cancer J Clin (2020) 70(6):443–59. doi: 10.3322/caac.21637
4. Kuehn BM. Looking to long-term survivors for improved pancreatic cancer treatment. JAMA (2020) 324(22):2242–4. doi: 10.1001/jama.2020.21717
5. Neuzillet C, Hammel P, Tijeras-Raballand A, Couvelard A, Raymond E. Targeting the ras-ERK pathway in pancreatic adenocarcinoma. Cancer Metastasis Rev (2013) 32:147–62. doi: 10.1007/s10555-012-9396-2
6. Zhou B, Wu Q, Chen G, Zhang TP, Zhao YP. NOP14 promotes proliferation and metastasis of pancreatic cancer cells. Cancer Lett (2012) 322:195–203. doi: 10.1016/j.canlet.2012.03.010
7. Zhou B, Irwanto A, Guo YM, Bei JX, Wu Q, Chen G, et al. Exome sequencing and digital PCR analyses reveal novel mutated genes related to the metastasis of pancreatic ductal adenocarcinoma. Cancer Biol Ther (2012) 13:871–9. doi: 10.4161/cbt.20839
8. Wen Z, Liao Q, Zhao J, Hu Y, You L, Lu Z, et al. High expression of interleukin-22 and its receptor predicts poor prognosis in pancreatic ductal adenocarcinoma. Ann Surg Oncol (2014) 21:125–32. doi: 10.1245/s10434-013-3322-x
9. Bao B, Azmi AS, Aboukameel A, Ahmad A, Bolling-Fischer A, Sethi S, et al. Pancreatic cancer stem-like cells display aggressive behavior mediated via activation of FoxQ1. Biol Chem (2014) 289:14520–33. doi: 10.1074/jbc.M113.532887
10. Wang T, Yang J, Xu J, Li J, Cao Z, Zhou L, et al. CHIP is a novel tumor suppressor in pancreatic cancer through targeting EGFR. Oncotarget (2014) 5:1969–86. doi: 10.18632/oncotarget.1890
11. Zhang L, Jamaluddin MS, Weakley SM, Yao Q, Chen C. Roles and mechanisms of microRNAs in pancreatic cancer. World J Surg (2011) 35:1725–31. doi: 10.1007/s00268-010-0952-z
12. Mukaida N, Sasaki S, Baba T. Chemokines in cancer development and progression and their potential as targeting molecules for cancer treatment. Mediators Inflammation (2014) 2014:170381. doi: 10.1155/2014/170381
13. Balabanian K, Lagane B, Infantino S, Chow KY, Harriague J, Moepps B, et al. The chemokine SDF-1/CXCL12 binds to and signals through the orphan receptor RDC1 in T lymphocytes. J Biol Chem (2005) 280:35760–6. doi: 10.1074/jbc.M508234200
14. Sun X, Cheng G, Hao M, Zheng J, Zhou X, Zhang J, et al. CXCL12/CXCR4/CXCR7 chemokine axis and cancer progression. Cancer Metastasis Rev (2010) 29:709–22. doi: 10.1007/s10555-010-9256-x
15. Guo JC, Li J, Zhou L, Yang JY, Zhang ZG, Liang ZY, et al. CXCL12-CXCR7 axis contributes to the invasive phenotype of pancreatic cancer. Oncotarget (2016) 7(38):62006–18. doi: 10.18632/oncotarget.11330
16. Rot A, von Andrian UH. Chemokines in innate and adaptive host defense: Basic chemokinese grammar for immune cells. Annu Rev Immunol (2004) 22:891–928. doi: 10.1146/annurev.immunol.22.012703.104543
17. Luther SA, Cyster JG. Chemokines as regulators of T cell differentiation. Nat Immunol (2001) 2:102–7. doi: 10.1038/84205
18. Kulbe H, Levinson NR, Balkwill F, Wilson JL. The chemokine network in cancer - much more than directing cell movement. Int J Dev Biol (2004) 48:489–96. doi: 10.1387/ijdb.041814hk
19. Vicari AP, Caux C. Chemokines in cancer. Cytokine Growth Factor Rev (2002) 13:143–54. doi: 10.1016/S1359-6101(01)00033-8
20. Janssens R, Struyf S, Proost P. Pathological roles of the homeostatic chemokine CXCL12. Cytokine Growth Factor Rev (2018) 44:51–68. doi: 10.1016/j.cytogfr.2018.10.004
22. Mollica Poeta V, Massara M, Capucetti A, Bonecchi R. Chemokines and chemokine receptors: New targets for cancer. Immunother Front Immunol (2019) 10:379. doi: 10.3389/fimmu.2019.00379
23. Balkwill F. Cancer and the chemokine network. Nat Rev Cancer (2004) 4:540–50. doi: 10.1038/nrc1388
24. Ratajczak M, Zuba-Surma E, Kucia M, Reca R, Wojakowski W, Ratajczak J. The pleiotropic effects of the SDF-1-CXCR4 axis in organogenesis, regeneration and tumorigenesis. Leukemia (2006) 20:1915–24. doi: 10.1038/sj.leu.2404357
25. Nomiyama H, Osada N, Yoshie O. Systematic classification of vertebrate chemokines based on conserved synteny and evolutionary history. Gene Cell (2013) 18:1–16. doi: 10.1111/gtc.12013
26. Kufareva I, Salanga CL, Handel TM. Chemokine and chemokine receptor structure and interactions: Implications for therapeutic strategies. Immunol Cell Biol (2015) 93:372–83. doi: 10.1038/icb.2015.15
27. Gao H, Priebe W, Glod J, Banerjee D. Activation of signal transducers and activators of transcription 3 and focal adhesion kinase by stromal cell-derived factor 1 is required for migration of human mesenchymal stem cells in response to tumor cell-conditioned medium. Stem Cells (2009) 27:857–65. doi: 10.1002/stem.23
28. Huang C-Y, Lee C-Y, Chen M-Y, Yang W-H, Chen Y-H, Chang C-H, et al. Stromal cell-derived factor-1/CXCR4 enhanced motility of human osteosarcoma cells involves MEK1/2, ERK and NF-kappaB-dependent pathways. J Cell Physiol (2009) 221:204–12. doi: 10.1002/jcp.21846
29. Song ZY, Wang F, Cui SX, Qu XJ. Knockdown of CXCR4 inhibits CXCL12-induced angiogenesis in HUVECs through downregulation of the MAPK/ERK and PI3K/AKT and the wnt/beta-catenin pathways. Cancer Investig (2018) 36:10–8. doi: 10.1080/07357907.2017.1422512
30. Xin Q, Sun Q, Zhang CS, Zhang Q, Li CJ. Functions and mechanisms of chemokine receptor 7 in tumors of the digestive system. World J Clin cases (2020) 8(12):2448–63. doi: 10.12998/wjcc.v8.i12.2448
31. Pan B, Liao Q, Niu Z, Zhou L, Zhao Y. Cancer-associated fibroblasts in pancreatic adenocarcinoma. Future Oncol (2015) 11(18):2603–10. doi: 10.2217/FON.15.176
32. Qian D, Lu Z, Xu Q, Wu P, Tian L, Zhao L, et al. Galectin-1-driven upregulation of SDF-1 in pancreatic stellate cells promotes pancreatic cancer metastasis. Cancer Lett (2017) 397:43–51. doi: 10.1016/j.canlet.2017.03.024
33. Matsuo Y, Ochi N, Sawai H, Yasuda A, Takahashi H, Funahashi H, et al. CXCL8/IL-8 and CXCL12/SDF-1alpha co-operatively promote invasiveness and angiogenesis in pancreatic cancer. Int J Cancer (2009) 124(4):853–61. doi: 10.1002/ijc.24040
34. Teicher BA, Fricker SP. CXCL12 (SDF-1)/CXCR4 pathway in cancer. Clin Cancer Res (2010) 16(11):2927–31. doi: 10.1158/1078-0432.CCR-09-2329
35. Carmeliet P, Jain RK. Angiogenesis in cancer and other diseases. Nature (2000) 407:249–57. doi: 10.1038/35025220
36. Strieter RM, Polverini PJ, Kunkel SL, Arenberg DA, Burdick MD, Kasper J, et al. The functional role of the ELR motif in CXC chemokine-mediated angiogenesis. J Biol Chem (1995) 270:27348–57. doi: 10.1074/jbc.270.45.27348
37. Liang Z, Brooks J, Willard M, Liang K, Yoon Y, Kang S, et al. CXCR4/CXCL12 axis promotes VEGF-mediated tumor angiogenesis through akt signaling pathway. Biochem Biophys Res Commun (2007) 359:716–22. doi: 10.1016/j.bbrc.2007.05.182
38. Lafleur MA, Handsley MM, Edwards DR. Metalloproteinases and their inhibitors in angiogenesis. Expet Rev Mol Med (2003) 5:1–39. doi: 10.1017/S1462399403006628
39. Chu C-Y, Cha S-T, Lin W-C, Lu P-H, Tan C-T, Chang C-C, et al. Stromal cell- derived factor-1α (SDF-1α/CXCL12)-enhanced angiogenesis of human basal cell carcinoma cells involves ERK1/2–NF-κB/interleukin-6 pathway. Carcinogenesis (2009) 30:205–13. doi: 10.1093/carcin/bgn228
40. Yu P, Zhang Z, Li S, Wen X, Quan W, Tian Q, et al. Progesterone modulates endothelial progenitor cell (EPC) viability through the CXCL12/CXCR4/PI3K/Akt signalling pathway. Cell Prolif (2016) 49:48–57. doi: 10.1111/cpr.12231
41. Shi Y, Riese DJ 2nd, Shen J. The role of the CXCL12/CXCR4/CXCR7 chemokine axis in cancer. Front Pharmacol (2020) 11:574667. doi: 10.3389/fphar.2020.574667
42. Heidemann J, Ogawa H, Rafiee P, Lugering N, Maaser C, Domschke W, et al. Mucosal angiogenesis regulation by CXCR4 and its ligand CXCL12 expressed by human intestinal microvascular endothelial cells. Am J Physiol Gastrointest Liver Physiol (2004) 286:G1059–68. doi: 10.1152/ajpgi.00417.2003
43. Salcedo R, Wasserman K, Young HA, Grimm MC, Howard OM, Anver MR, et al. Vascular endothelial growth factor and basic fibroblast growth factor induce expression of CXCR4 on human endothelial cells: In vivo neovascularization induced by stromal-derived factor- 1alpha. Am J Pathol (1999) 154:1125–35. doi: 10.1016/S0002-9440(10)65365-5
44. Duda DG, Kozin SV, Kirkpatrick ND, Xu L, Fukumura D, Jain R. K CXCL12 (SDF1alpha)-CXCR4/CXCR7 pathway inhibition: An emerging sensitizer for anticancer therapies? Clin Canc Res (1999) 17:2074–80. doi: 10.1158/1078-0432.CCR-10-2636
45. Ruiz de Almodovar C, Luttun A, Carmeliet P. An SDF-1 trap for myeloid cells stimulates angiogenesis. Cell (2006) 124:18–21. doi: 10.1016/j.cell.2005.12.023
46. Pan J, Burdick MD, Belperio JA, Xue YY, Gerard C, Sharma S, et al. Strieter RM.CXCR3/CXCR3 ligand biological axis impairs RENCA tumor growth by a mechanism of immunoangiostasis. J Immunol (2006) 176(3):1456–64. doi: 10.4049/jimmunol.176.3.1456
47. Miao Z, Luker KE, Summers BC, Berahovich R, Bhojani MS, Rehemtulla A, et al. CXCR7 (RDC1) promotes breast and lung tumor growth in vivo and is expressed on tumor-associated vasculature. Proc Natl Acad Sci U.S.A. (2007) 104(40):15735–40. doi: 10.1073/pnas.0610444104
48. Li XX, Zheng HT, Huang LY, Shi DB, Peng JJ, Liang L, et al. Silencing of CXCR7 gene represses growth and invasion and induces apoptosis in colorectal cancer through ERK and β-arrestin pathways. Int J Oncol (2014) 45(4):1649–57. doi: 10.3892/ijo.2014.2547
49. Si M, Song Y, Wang X, Wang D, Liu X, Qu X, et al. CXCL12/CXCR7/β-arrestin1 biased signal promotes epithelial-to-mesenchymal transition of colorectal cancer by repressing miRNAs through YAP1 nuclear translocation. Cell Biosci (2022) 12(1):171. doi: 10.1186/s13578-022-00908-1
50. Guo J, Tong CY, Shi JG, Li XJ. C-X-C motif chemokine ligand 12 (CXCL12)/C-X-C motif chemokine receptor 7(CXCR7) regulates epithelial-mesenchymal transition process and promotes the metastasis of esophageal cancer by activating signal transducer and activator of transcription 3 (STAT3). Bioengineered (2022) 13(3):7425–38. doi: 10.1080/21655979.2022.2048984
51. Xu L, Li C, Hua F, Liu X. The CXCL12/CXCR7 signalling axis promotes proliferation and metastasis in cervical cancer. Med Oncol (2021) 38(5):58. doi: 10.1007/s12032-021-01481-2
52. Wang J, Wang J, Dai J, Jung Y, Wei CL, Wang Y, et al. A glycolytic mechanism regulating an angiogenic switch in prostate cancer. Canc Res (2007) 67:149–59. doi: 10.1158/0008-5472.CAN-06-2971
53. Müller A, Homey B, Soto H, Ge N, Catron D, Buchanan M, et al. Involvement of chemokine receptors in breast cancer metastasis. Nature (2001) 410(6824):50–6. doi: 10.1038/35065016
54. Phillips RJ, Burdick MD, Lutz M, Belperio JA, Keane MP, Strieter RM. The stromal derived factor-1/CXCL12-CXC chemokine receptor 4 biological axis in non-small cell lung cancer metastases. Am J Respir Crit Care Med (2003) 167(12):1676–86. doi: 10.1164/rccm.200301-071OC
55. Keeley EC, Mehrad B, Strieter RM. CXC chemokines in cancer angiogenesis and metastases. Adv Cancer Res (2010) 106:91–111. doi: 10.1016/S0065-230X(10)06003-3
56. Ozga AJ, Chow MT. Luster AD.Chemokines and the immune response to cancer. Immunity (2021) 54(5):859–74. doi: 10.1016/j.immuni.2021.01.012
57. Nagarsheth N, Wicha MS, Zou W. Chemokines in the cancer microenvironment and their relevance in cancer immunotherapy. Rev Immunol (2017) 17(9):559–72. doi: 10.1038/nri.2017.49
58. Spill F, Reynolds DS, Kamm RD, Zaman MH. Impact of the physical microenvironment on tumor progression and metastasis. Curr Opin Biotechnol (2016) 40:41–8:2016. doi: 10.1016/j.copbio.2016.02.007
59. Raman D, Baugher PJ, Thu YM. Role of chemokines in tumor growth. Cancer Lett (2007) 256:137–65. doi: 10.1016/j.canlet.2007.05.013
60. Hartmann TN, Burger M, Burger JA. The role of adhesion molecules and chemokine receptor CXCR4 (CD184) in small cell lung cancer. J Biol Regul Homeost Agents (2004) 18:126–30. doi: 10.1038/sj.onc.1207097
61. Orimo A, Gupta PB, Sgroi DC, Arenzana-Seisdedos F, Delaunay T, Naeem R, et al. Stromal fibroblasts present in invasive human breast carcinomas promote tumor growth and angiogenesis through elevated SDF1/CXCL12 secretion. Cell (2005) 121:335–48. doi: 10.1016/j.cell.2005.02.034
62. Zhang Q, He Y, Luo N, Patel SJ, Han Y, Gao R, et al. Landscape and dynamics of single immune cells in hepatocellular carcinoma. Cell (2019) 179:829–845.e20. doi: 10.1016/j.cell.2019.10.003
63. Galon J, Bruni D. Tumor immunology and tumor evolution: Intertwined histories. Immunity (2020) 52:55–81. doi: 10.1016/j.immuni.2019.12.018
64. Wolf MJ, Hoos A, Bauer J, Boettcher S, Knust M, Weber A, et al. Endothelial CCR2 signaling induced by colon carcinoma cells enables extravasation via the JAK2–Stat5 and p38MAPK pathway. Cancer Cell (2012) 22:91–105. doi: 10.1016/j.ccr.2012.05.023
65. Jung MJ, Rho JK, Kim YM, Jung JE, Jin YB, Ko YG, et al. Upregulation of CXCR4 is functionally crucial for maintenance of stemness in drug-resistant non-small cell lung cancer cells. Oncogene (2013) 32:209–21. doi: 10.1038/onc.2012.37
66. Griesmann H, Drexel C, Milosevic N, Sipos B, Rosendahl J, Gress TM, et al. Role of CCL20 mediated immune cell recruitment in NF-kappaB mediated TRAIL resistance of pancreatic cancer. Biochim Et Biophys Acta Mol Cell Res (2017) 1864:782–96. doi: 10.1016/j.bbamcr.2017.02.005
67. Liu B, Jia Y, Ma J, Wu S, Jiang H, Cao Y, et al. Tumor-associated macrophage-derived CCL20 enhances the growth and metastasis of pancreatic cancer. Acta Biochim Et Biophys Sin (2016) 48:1067–74. doi: 10.1093/abbs/gmw101
68. Chatterjee S, Behnam Azad B, Nimmagadda S. The intricate role of CXCR4 in cancer. Adv Cancer Res (2014) 124:31–82. doi: 10.1016/B978-0-12-411638-2.00002-1
69. Bai R, Jie X, Sun J, Liang Z, Yoon Y, Feng A, et al. Development of CXCR4 modulators based on the lead compound RB-108. Eur J Med Chem (2019) 173:32–43. doi: 10.1016/j.ejmech.2019.03.065
70. Lecavalier-Barsoum M, Chaudary N, Han K, Pintilie M, Hill RP, Milosevic M. Targeting CXCL12/CXCR4 and myeloid cells to improve the therapeutic ratio in patient-derived cervical cancer models treated with radio-chemotherapy. Br J Cancer (2019) 121(3):249–56. doi: 10.1038/s41416-019-0497-3
71. Portella L, Bello AM, Scala S. CXCL12 signaling in the tumor microenvironment. s.l: Adv Exp Med Biol (2021) 1302:51–70. doi: 10.1007/978-3-030-62658-7_5
72. Samarendra H, Jones K, Petrinic T, Silva MA, Reddy S, Soonawalla Z, et al. A meta-analysis of CXCL12 expression for cancer prognosis. Br J Cancer (2017) 117(1):124–35. doi: 10.1038/bjc.2017.134
73. Ding Y, Du Y. Clinicopathological significance and prognostic role of chemokine receptor CXCR4 expression in pancreatic ductal adenocarcinoma, a meta-analysis and literature review. Int J Surg (2019) 65:32–8. doi: 10.1016/j.ijsu.2019.03.009
74. Marchesi F, Monti P, Leone BE, Zerbi A, Vecchi A, Piemonti L, et al. Increased survival, proliferation, and migration in metastatic human pancreatic tumor cells expressing functional CXCR4. Cancer Res (2004) 64:8420–7. doi: 10.1158/0008-5472.CAN-04-1343
75. Aronovich A, Moyal L, Gorovitz B, Amitay-Laish I, Naveh HP, Forer Y, et al. Cancer-associated fibroblasts in mycosis fungoides promote tumor cell migration and drug resistance through CXCL12/CXCR4. J Invest Dermatol (2021) 141(3):619–627.e2. doi: 10.1016/j.jid.2020.06.034
76. Kure S, Matsuda Y, Hagio M, Ueda J, Naito Z, Ishiwata T. Expression of cancer stem cell markers in pancreatic intraepithelial neoplasias and pancreatic ductal adenocarcinomas. Int J Oncol (2012) 41(2012):1314–24. doi: 10.3892/ijo.2012.1565
77. Stacer AC, Fenner J, Cavnar SP, Xiao A, Zhao S, Chang SL, et al. Endothelial CXCR7 regulates breast cancer metastasis. Oncogene (2016) 35:1716–24. doi: 10.1038/onc.2015.236
78. Thomas RM, Kim J, Revelo-Penafiel MP, Angel R, Dawson DW, Lowy AM. The chemokine receptor CXCR4 is expressed in pancreatic intraepithelial neoplasia. Gut (2008) 57:1555–60. doi: 10.1136/gut.2007.143941
79. Zhong W, Chen W, Zhang D, Sun J, Li Y, Zhang J, et al. CXCL12/CXCR4 axis plays pivotal roles in the organ-specific metastasis of pancreatic adenocarcinoma: A clinical study. Exp Ther Med (2012) 4(3):363–9. doi: 10.3892/etm.2012.631
80. Sleightholm RL, Neilsen BK, Li J, Steele MM, Singh RK, Hollingsworth MA, et al. Emerging roles of the CXCL12/CXCR4 axis in pancreatic cancer progression and therapy. Pharmacol Ther (2017) 179:158–70. doi: 10.1016/j.pharmthera.2017.05.012
81. Billadeau D, Chatterjee S, Bramati P, Sreekumar R, Shah V, Hedin K, et al. Characterization of the CXCR4 signaling in pancreatic cancer cells. Int J Gastrointestinal Cancer (2006) 37:2006. doi: 10.1016/j.canlet.2012.02.035
82. Li X, Ma Q, Xu Q, Liu H, Lei J, Duan W, et al. SDF-1/CXCR4 signaling induces pancreatic cancer cell invasion and epithelial-mesenchymal transition in vitro through non-canonical activation of hedgehog pathway. Canc Lett (2012) 322(2):169–76. doi: 10.1016/j.canlet.2012.02.035
83. Burger JA, Kipps TJ. CXCR4: A key receptor in the crosstalk between tumor cells and their microenvironment. Blood (2006) 107(5):1761–7. doi: 10.1182/blood-2005-08-3182
84. Yang P, Wang G, Huo H, Li Q, Zhao Y, Liu Y, et al. SDF-1/CXCR4 signaling up-regulates survivin to regulate human sacral chondrosarcoma cell cycle and epithelial-mesenchymal transition via ERK and PI3K/AKT pathway. Med Oncol (2015) 32:377. doi: 10.1007/s12032-014-0377-x
85. Dickinson LA, Joh T, Kohwi Y, Kohwi-Shigematsu T. A tissue-specific MAR/SAR DNA-binding protein with unusual binding site recognition. Cell (1992) 70:631–45. doi: 10.1016/0092-8674(92)90432-C
86. Wei L, Ye H, Li G, Lu Y, Zhou Q, Zheng S, et al. Cancer-associated fibroblasts promote progression and gemcitabine resistance via the SDF-1/SATB-1 pathway in pancreatic cancer. Cell Death Dis (2018) 9(11):1065. doi: 10.1038/s41419-018-1104-x
87. Heinrich EL, Lee W, Lu J, Lowy AM, Kim J. Chemokine CXCL12 activates dual CXCR4 and CXCR7-mediated signaling pathways in pancreatic cancer cells. J Trans Med (2012) 10:68. doi: 10.1186/1479-5876-10-68
88. Wang HX, Tao LY, Qi KE, Zhang HY, Feng D, Wei WJ, et al. Role of CXC chemokine receptor type 7 in carcinogenesis and lymph node metastasis of colon cancer. Mol Clin Oncol (2015) 3:1229–32. doi: 10.3892/mco.2015.643
89. Luker KE, Luker GD. Functions of CXCL12 and CXCR4 in breast cancer. s.l: Cancer Lett (2006) 238:30–41. doi: 10.1016/j.canlet.2005.06.021
90. Guillemot E, Karimdjee-Soilihi B, Pradelli E, Benchetrit M, Goguet-Surmenian E, Millet MA, et al. CXCR7 receptors facilitate the progression of colon carcinoma within lung not within liver. Br J Cancer (2012) 107:1944–9. doi: 10.1038/bjc.2012.503
91. Hartmann TN, Burger JA, Glodek A, Fujii N, Burger M. CXCR4 chemokine receptor and integrin signalling co-operate in mediating adhesion and chemoresistance in small cell lung cancer (SCLC) cells. Oncogene (2005) 24:4462–71. doi: 10.1038/sj.onc.1208621
92. Daniel SK, Seo YD, Pillarisetty VG. The CXCL12-CXCR4/CXCR7 axis as a mechanism of immune resistance in gastrointestinal malignancies. Semin Cancer Biol (2020) 65:176–88. doi: 10.1016/j.semcancer.2019.12.007
93. Meads MB, Gatenby RA, Dalton WS. Environment-mediated drug resistance: A major contributor to minimal residual disease. Nat Rev Cancer (2009) 9:665–74. doi: 10.1038/nrc2714
94. Khare T, Bissonnette M, Khare S. CXCL12-CXCR4/CXCR7 axis in colorectal cancer: Therapeutic target in preclinical and clinical studies. Int J Mol Sci (2021) 22(14):7371. doi: 10.3390/ijms22147371
95. Rubin J, Kung A, Klein R, Chan J, Sun Y, Schmidt K, et al. A small-molecule antagonist of CXCR4 inhibits intracranial growth of primary brain tumors. Proc Natl Acad Sci USA (2003) 100(23):13513–8. doi: 10.1073/pnas.2235846100
96. Zeelenberg I, Ruuls-Van Stalle L, Roos E. The chemokine receptor CXCR4 is required for outgrowth of colon carcinoma micrometastases. Cancer Res (2003) 63(13):3833–9. doi: 10.1158/1078-0432.CCR-05-0537
97. Fink DM, Steele MM, Hollingsworth MA. The lymphatic system and pancreatic cancer. Cancer Lett (2016) 381:217–36. doi: 10.1016/j.canlet.2015.11.048
98. Griesmann H, Drexel C, Milosevic N, Sipos B, Rosendahl J, Gress TM, et al. Pharmacological macrophage inhibition decreases metastasis formation in a genetic model of pancreatic cancer. Gut (2017) 66:1278–85. doi: 10.1136/gutjnl-2015-310049
99. Huang C, Li Z, Li N, Li Y, Chang A, Zhao T, et al. Interleukin 35 expression correlates with microvessel density in pancreatic ductal adenocarcinoma, recruits monocytes, and promotes growth and angiogenesis of xenograft tumors in mice. s.l: Gastroenterol (2018) 154:675–88. doi: 10.1053/j.gastro.2017.09.039
100. Ray K. Pancreatic cancer: Pancreatic cancer exosomes prime the liver for metastasis. Nat Rev Gastroenterol Hepatol (2015) 12:371. doi: 10.1038/nrgastro.2015.93
101. Giovannetti E, van der Borden CL, Frampton AE, Ali A, Firuzi O, Peters GJ. Never let it go: stopping key mechanisms underlying metastasis to fight pancreatic cancer. Semin Cancer Biol (2017) 44:43–59. doi: 10.1016/j.semcancer.2017.04.006
102. Chang JH, Jiang Y, Pillarisetty VG. Role of immune cells in pancreatic cancer from bench to clinical application: An updated review. Medicine (2016) 95:e5541. doi: 10.1097/MD.0000000000005541
103. Melstrom LG, Salazar MD, Diamond DJ. The pancreatic cancer microenvironment: A true double agent. J Surg Oncol (2017) 116(1):7–15. doi: 10.1002/jso.24643
104. Ansari D, Carvajo M, Bauden M, Andersson R. Pancreatic cancer stroma: Controversies and current insights. Scand J Gastroenterol (2017) 52:641–6. doi: 10.1080/00365521.2017.1293726
105. Rhim AD, Oberstein PE, Thomas DH, Mirek ET, Palermo CF, Sastra SA, et al. Stromal elements act to restrain, rather than support, pancreatic ductal adenocarcinoma. Cancer Cell (2014) 25:735–47. doi: 10.1016/j.ccr.2014.04.021
106. De Clercq E. AMD3100/CXCR4 inhibitor. Front Immunol (2015) 6:276. doi: 10.3389/fimmu.2015.00276
107. Abraham M, Pereg Y, Bulvik B, Klein S, Mishalian I, Wald H, et al. Single dose of the CXCR4 antagonist BL-8040 induces rapid mobilization for the collection of human CD34+ cells in healthy volunteers. Clin Cancer Res (2017) 23(22):6790–801. doi: 10.1158/1078-0432.CCR-16-2919
108. Morimoto M, Matsuo Y, Koide S, Tsuboi K, Shamoto T, Sato T, et al. Enhancement of the CXCL12/CXCR4 axis due to acquisition of gemcitabine resistance in pancreatic cancer: effect of CXCR4 antagonists. BMC Cancer (2016) 16:305. doi: 10.1186/s12885-016-2340-z
109. Jimeno A, Rubio-Viqueira B, Rajeshkumar NV, Chan A, Solomon A, Hidalgo M. A fine-needle aspirate-based vulnerability assay identifies polo-like kinase 1 as a mediator of gemcitabine resistance in pancreatic cancer. Mol Cancer Ther (2010) 9(2):311–8. doi: 10.1158/1535-7163.MCT-09-0693
110. Li J, Wang R, Schweickert PG, Karki A, Yang Y, Kong Y, et al. Plk1 inhibition enhances the efficacy of gemcitabine in human pancreatic cancer. Cell Cycle (2016) 15(5):711–9. doi: 10.1080/15384101.2016.1148838
111. Tang S, Hang Y, Ding L, Tang W, Yu A, Zhang C, et al. Intraperitoneal siRNA nanoparticles for augmentation of gemcitabine efficacy in the treatment of pancreatic cancer. Mol Pharm (2021) 18(12):4448–58. doi: 10.1021/acs.molpharmaceut.1c00653
112. Malik S, Westcott JM, Brekken RA, Burrows FJ. CXCL12 in pancreatic cancer: Its function and potential as a therapeutic drug target. Cancers (Basel) (2021) 14(1):86. doi: 10.3390/cancers14010086
113. Khan AM. Co-targeting of CXCR4 and hedgehog pathways disrupts tumor-stromal crosstalk and improves chemotherapeutic efficacy in pancreatic cancer. J Biol Chem (2020) 295(25):8413–24. doi: 10.1074/jbc.RA119.011748
114. Wang D, Jiao C, Zhu Y, Liang D, Zao M, Meng X, et al. Activation of CXCL12/CXCR4 renders colorectal cancer cells less sensitive to radiotherapy via up-regulating the expression of survivin. Exp Biol Med (Maywood) (2017) 242(4):429–35. doi: 10.1177/1535370216675068
115. Oweida A, Phan A, Vancourt B, Robin T, Hararah MK, Bhatia S, et al. Hypofractionated radiotherapy is superior to conventional fractionation in an orthotopic model of anaplastic thyroid cancer. Thyroid (2018) 28:739–747, 2018. doi: 10.1089/thy.2017.0706
116. Kim JY, Kim HJ, Jung CW, Lee TS, Kim EH, Park MJ. CXCR4 uses STAT3-mediated slug expression to maintain radioresistance of non-small cell lung cancer cells: Emerges as a potential prognostic biomarker for lung cancer. Cell Death Dis (2021) 12:48. doi: 10.1038/s41419-020-03280-5
117. Kato T, Matsuo Y, Ueda G, Murase H, Aoyama Y, Omi K, et al. Enhanced CXCL12/CXCR4 signaling increases tumor progression in radiation-resistant pancreatic cancer. Oncol Rep (2022) 47(4):68. doi: 10.3892/or.2022.8279
118. Kovacevic KD, Gilbert JC, Jilma B. Pharmacokinetics, pharmacodynamics and safety of aptamers. s.l: Adv Drug Delivery Rev (2018) 134:36–50. doi: 10.1016/j.addr.2018.10.008
119. Vater A, Klussmann S. Turning mirror-image oligonucleotides into drugs: The evolution of spiegelmer(®) therapeutics. Drug Discovery Today (2015) 20(1):147–55. doi: 10.1016/j.drudis.2014.09.004
120. Erkan M, Michalski CW, Rieder S, Reiser-Erkan C, Abiatari I, Kolb A, et al. The activated stroma index is a novel and independent prognostic marker in pancreatic ductal adenocarcinoma. Clin Gastroenterol Hepatol (2008) 6(10):1155–61. doi: 10.1016/j.cgh.2008.05.006
121. Olive KP, Jacobetz MA, Davidson CJ, Gopinathan A, McIntyre D, Honess D, et al. Inhibition of hedgehog signaling enhances delivery of chemotherapy in a mouse model of pancreatic cancer. Science (2009) 324(2009):1457–61. doi: 10.1126/science.1171362
122. Ren B, Cui M, Yang G, Wang H, Feng M, You L, et al. Tumor microenvironment participates in metastasis of pancreatic cancer. Mol Cancer (2018) 17:108. doi: 10.1186/s12943-018-0858-1
123. Vonderheide RH, Bayne LJ. Inflammatory networks and immune surveillance of pancreatic carcinoma. Curr Opin Immunol (2013) 25:200–5. doi: 10.1016/j.coi.2013.01.006
124. Meyer MA, Baer JM, Knolhoff BL, Nywening TM, Panni RZ, Su X, et al. Breast and pancreatic cancer interrupt IRF8-dependent dendritic cell development to overcome immune surveillance. Nat Commun (2018) 9:1250. doi: 10.1038/s41467-018-03600-6
125. Pylayeva-Gupta Y, Lee KE, Hajdu CH, Miller G, Bar-Sagi D. Oncogenic kras-induced GM-CSF production promotes the development of pancreatic neoplasia. s.l: Cancer Cell (2012) 21:836–47. doi: 10.1016/j.ccr.2012.04.024
126. Sivaram N, McLaughlin PA, Han HV, Petrenko O, Jiang YP, Ballou LM, et al. Tumor-intrinsic PIK3CA represses tumor immunogenicity in a model of pancreatic cancer. J Clin Invest (2019) 129:3264–76. doi: 10.1172/JCI123540
127. Hashimoto S, Furukawa S, Hashimoto A, Tsutaho A, Fukao A, Sakamura Y, et al. ARF6 and AMAP1 are major targets of KRAS and TP53 mutations to promote invasion, PD-L1 dynamics, and immune evasion of pancreatic cancer. Proc Natl Acad Sci USA (2019) 116:17450–9. doi: 10.1073/pnas.1901765116
128. Wang S, Zheng Y, Yang F, Zhu L, Zhu XQ, Wang ZF, et al. The molecular biology of pancreatic adenocarcinoma: Translational challenges and clinical perspectives. Sig Transduct Target Ther (2021) 6:249. doi: 10.1038/s41392-021-00659-4
129. Garg B, Giri B, Modi S, Sethi V, Castro I, Umland O, et al. NFκB in pancreatic stellate cells reduces infiltration of tumors by cytotoxic T cells and killing of cancer cells, via up-regulation of CXCL12. Gastroenterology (2018) 155:880–891.e888. doi: 10.1053/j.gastro.2018.05.051
130. Royal RE, Levy C, Turner K, Mathur A, Hughes M, Kammula US, et al. Phase 2 trial of single agent ipilimumab (anti-CTLA-4) for locally advanced or metastatic pancreatic adenocarcinoma. J Immunother (2010) 33:828–33. doi: 10.1097/CJI.0b013e3181eec14c
131. Brahmer JR, Tykodi SS, Chow LQ, Hwu WJ, Topalian SL, Hwu P, et al. Safety and activity of anti-PD-L1 antibody in patients with advanced cancer. N Engl J Med (2012) 366:2455–65. doi: 10.1056/NEJMoa1200694
132. Marabelle A, Le DT, Ascierto PA, Di Giacomo AM, De Jesus-Acosta A, Delord JP, et al. Efficacy of pembrolizumab in patients with noncolorectal high microsatellite instability/mismatch repair-deficient cancer: Results from the phase II KEYNOTE-158. Study J Clin Oncol (2020) 38:1–10. doi: 10.1200/JCO.19.02105
133. Nomi T, Sho M, Akahori T, Hamada K, Kubo A, Kanehiro H, et al. Clinical significance and therapeutic potential of the programmed death-1 ligand/programmed death-1 pathway in human pancreatic cancer. Clin Cancer Res (2017) 13:2151–7. doi: 10.1158/1078-0432.CCR-06-2746
134. Brahmer JR, Drake CG, Wollner I, Powderly JD, Picus J, Sharfman WH, et al. Phase I study of single-agent anti-programmed death-1 (MDX-1106) in refractory solid tumors: Safety, clinical activity, pharmacodynamics, and immunologic correlates. J Clin Oncol (2010) 28:3167–75. doi: 10.1200/JCO.2009.26.7609
135. Ying H, Dey P, Yao W, Kimmelman AC, Draetta GF, Maitra A, et al. Genetics and biology of pancreatic ductal adenocarcinoma. Genes Dev (2016) 30(4):355–85. doi: 10.1101/gad.275776.115
136. Feig C, Jones JO, Kraman M, Wells RJ, Deonarine A, Chan DS, et al. Targeting Cxcl12 from fap-expressing carcinoma- associated fibroblasts synergizes with anti-Pd-L1 immunotherapy in pancreatic cancer. Proc Natl Acad Sci U.S.A. (2013) 110:20212–7. doi: 10.1073/pnas.1320318110
137. Zhang Y, Velez-Delgado A, Mathew E, Li D, Mendez FM, Flannagan K, et al. Myeloid cells are required for pd-1/Pd-L1 checkpoint activation and the establishment of an immunosuppressive environment in pancreatic cancer. s.l: Gut (2017) 66:124–36. doi: 10.1136/gutjnl-2016-312078
138. Niccolai E, Prisco D, D’Elios MM, Amedei A. What is recent in pancreatic cancer immunotherapy? BioMed Res Int (2013) 492372:2013. doi: 10.1155/2013/492372
139. Miao L, Li J, Liu Q, Feng R, Das M, Lin CM, et al. Transient and local expression of chemokine and immune checkpoint traps to treat pancreatic cancer. ACS Nano (2017) 11(9):8690–706. doi: 10.1021/acsnano.7b01786
140. Ozdemir BC, Pentcheva-Hoang T, Carstens JL, Zheng X, Wu CC, Simpson TR, et al. Depletion of carcinoma-associated fibroblasts and fibrosis induces immunosuppression and accelerates pancreas cancer with reduced survival. Cancer Cell (2014) 25:719–34. doi: 10.1016/j.ccr.2014.04.005
141. Biasci D, Smoragiewicz M, Connell CM, Wang Z, Gao Y, Thaventhiran JED, et al. CXCR4 inhibition in human pancreatic and colorectal cancers induces an integrated immune response. Proc Natl Acad Sci U.S.A. (2020) 117(46):28960–70. doi: 10.1073/pnas.2013644117
142. Ghobrial IM, Liu CJ, Redd RA, Perez RP, Baz R, Zavidij O, et al. A phase Ib/II trial of the first-in-class anti-CXCR4 antibody ulocuplumab in combination with lenalidomide or bortezomib plus dexamethasone in relapsed multiple myeloma. s.l: Clin Cancer Res (2020) 26:344–53, 2020. doi: 10.1158/1078-0432.CCR-19-0647
143. Steurer M, Montillo M, Scarfò L, Mauro FR, Andel J, Wildner S, et al. Olaptesed pegol (NOX-A12) with bendamustine and rituximab: A phase IIA study in patients with relapsed/refractory chronic lymphocytic leukemia. s.l: Haematol (2019) 104:2053–60, 2019. doi: 10.3324/haematol.2018.205930
144. O’Hara MH, Messersmith W, Kindler H, Zhang W, Pitou C, Szpurka AM, et al. Safety and pharmacokinetics of CXCR4 peptide antagonist, LY2510924, in combination with durvalumab in advanced refractory solid tumors. J Pancreat Cancer (2020) 6(1):21–31. doi: 10.1089/pancan.2019.0018
145. Bockorny B, Semenisty V, Macarulla T, Borazanci E, Wolpin BM, Stemmer SM, et al. BL-8040, a CXCR4 antagonist, in combination with pembrolizumab and chemotherapy for pancreatic cancer: the COMBAT trial. Nat Med (2020) 26(6):878–85. doi: 10.1038/s41591-020-0880-x
146. Suarez-Carmona M, Williams A, Schreiber J, Hohmann N, Pruefer U, Krauss J, et al. Combined inhibition of CXCL12 and PD-1 in MSS colorectal and pancreatic cancer: Modulation of the microenvironment and clinical effects. J Immunother Cancer (2021) 9(10):e002505. doi: 10.1136/jitc-2021-002505
147. Shakir M, Tang D, Zeh HJ, Tang SW, Anderson CJ, Bahary N, et al. The chemokine receptors CXCR4/CXCR7 and their primary heterodimeric ligands CXCL12 and CXCL12/high mobility group box 1 in pancreatic cancer growth and development: Finding flow. Pancreas (2015) 44(4):528–34. doi: 10.1097/MPA.0000000000000298
Keywords: pancreatic ductal adenocarcinoma (PDAC), CXCL12, CXCR4, CXCR7, chemokines, biomarkers, tumor micreoenvironment (TME)
Citation: Roberto M, Arrivi G, Di Civita MA, Barchiesi G, Pilozzi E, Marchetti P, Santini D, Mazzuca F and Tomao S (2023) The role of CXCL12 axis in pancreatic cancer: New biomarkers and potential targets. Front. Oncol. 13:1154581. doi: 10.3389/fonc.2023.1154581
Received: 30 January 2023; Accepted: 09 March 2023;
Published: 23 March 2023.
Edited by:
Tao Zhang, Memorial Sloan Kettering Cancer Center, United StatesReviewed by:
Hongyan Xie, Massachusetts General Hospital, Harvard Medical School, United StatesJie Zhang, Cleveland Clinic, United States
Copyright © 2023 Roberto, Arrivi, Di Civita, Barchiesi, Pilozzi, Marchetti, Santini, Mazzuca and Tomao. This is an open-access article distributed under the terms of the Creative Commons Attribution License (CC BY). The use, distribution or reproduction in other forums is permitted, provided the original author(s) and the copyright owner(s) are credited and that the original publication in this journal is cited, in accordance with accepted academic practice. No use, distribution or reproduction is permitted which does not comply with these terms.
*Correspondence: Mattia Alberto Di Civita, bWF0dGlhYWxiZXJ0by5kaWNpdml0YUB1bmlyb21hMS5pdA==
†These authors have contributed equally to this work and share first authorship
‡These authors have contributed equally to this work and share last authorship