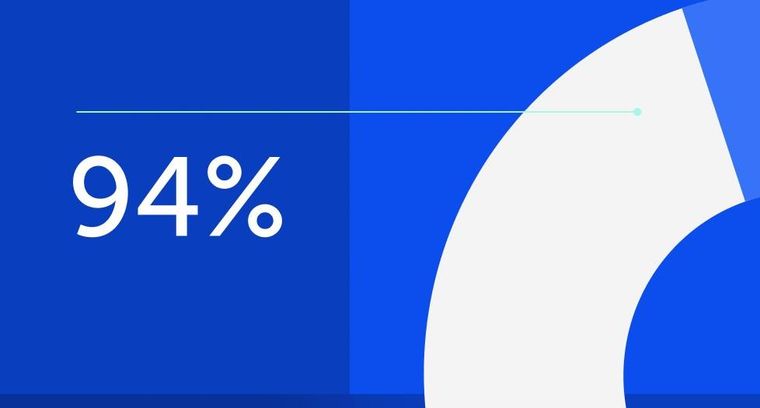
94% of researchers rate our articles as excellent or good
Learn more about the work of our research integrity team to safeguard the quality of each article we publish.
Find out more
ORIGINAL RESEARCH article
Front. Oncol., 17 August 2023
Sec. Skin Cancer
Volume 13 - 2023 | https://doi.org/10.3389/fonc.2023.1154107
This article is part of the Research TopicAdvances in Molecular and Pharmacological Mechanisms of Novel Targeted Therapies for MelanomaView all 12 articles
Background: Despite numerous observational studies on the association between serum 25-Hydroxyvitamin D levels and cutaneous melanoma, causal inferences remain ambiguous due to confounding and reverse causality. This study aimed to elucidate the causal relationship between serum 25-Hydroxyvitamin D levels and melanoma incidence using Mendelian randomization (MR).
Methods: A two-sample MR was conducted using genetic variants associated with serum 25-Hydroxyvitamin D levels as instrumental variables. Summary statistics for these variants were derived from genome-wide association studies, and those for melanoma risk were obtained from a comprehensive melanoma case-control study. Robustness of the results was assessed through sensitivity analyses, including the “leave-one-out” approach and tests for potential pleiotropy.
Results: The MR analysis provided substantial evidence of a positive causal relationship between serum 25-Hydroxyvitamin D levels and the incidence of cutaneous melanoma, suggesting that each unit increase in serum 25-Hydroxyvitamin D levels corresponds with an increased risk of melanoma. Tests for pleiotropy showed minimal effects, and the sensitivity analysis confirmed no disproportionate influence by any individual single nucleotide polymorphism (SNP).
Conclusion: The findings indicated a potentially causal positive association between serum 25-Hydroxyvitamin D levels and melanoma risk, challenging traditional beliefs about vitamin D’s role in melanoma. This emphasizes the need for a balanced and personalized approach to vitamin D supplementation and sun exposure, particularly in high-risk populations. These results should be interpreted with caution due to potential unrecognized pleiotropy and confounding factors. Future research should focus on validating these findings in diverse populations and exploring underlying biological mechanisms.
Cutaneous melanoma, a malignant neoplasm stemming from skin melanocytes (1), is a major worldwide health concern due to escalating incidence and high mortality rates (2). Over the past few decades, this aggressive skin cancer with a pronounced metastatic propensity has seen a marked increase in prevalence, underscoring the urgency of a thorough understanding of its etiology (3). The etiology of melanoma is multifactorial, involving a complex combination of environmental and genetic determinants (4). Exposure to ultraviolet (UV) radiation is a well-established risk factor, playing a pivotal role in the disease’s onset and progression (5). However, our understanding of other potential modifiable risk factors, such as Vitamin D—which is predominantly generated through UV exposure—is less clear and warrants further investigation (6).
Despite significant advancements in early detection, prevention measures, and therapeutic strategies, melanoma presents considerable challenges (7). These challenges are largely due to its resistance to conventional treatments, advanced stages at diagnosis, and high metastatic potential (8, 9). Comprehensive insight into the disease’s etiology, risk factors, and the specific determinants of pathogenesis is crucial for devising more effective prevention strategies, targeted therapies, and improving overall prognosis for patients. This further emphasizes the importance of examining genetic and environmental interactions, especially concerning potential modifiable factors such as Vitamin D (10).
Vitamin D, synthesized primarily through sunlight exposure and dietary intake, is crucial for multiple physiological functions including bone health, immune regulation, and cell differentiation and proliferation (11, 12). The regulation of vitamin D metabolism involves the significant action of several enzymes, particularly CYP27A1, CYP27B1, and CYP24A1, which are genes critical for the synthesis and degradation of this vitamin (13, 14). Primarily expressed in the liver, CYP27A1 initiates the conversion of vitamin D into its active form, calcitriol, through a process known as hydroxylation (15). This conversion is further catalyzed by CYP27B1, which is predominantly expressed in the kidneys (16, 17). Meanwhile, CYP24A1, largely found in the kidneys and various other tissues, oversees the breakdown of active vitamin D metabolites into inactive forms (18). This degradation process is integral for maintaining vitamin D homeostasis, emphasizing the crucial role of CYP24A1 in this biological regulatory system (19). The primary circulating form, 25-Hydroxyvitamin D (25(OH)D), serves as a reliable biomarker of Vitamin D status (20). The potential protective role of Vitamin D, specifically serum 25(OH)D, against various cancers, including cutaneous melanoma - a highly aggressive skin cancer - has been a subject of significant research interest (10, 21). This interest is further amplified by the dual role of sunlight as a source of Vitamin D synthesis and a known risk factor for melanoma (22, 23).
However, the epidemiological evidence linking serum 25(OH)D levels and melanoma incidence has been inconsistent (24). Some studies indicate a protective role of higher serum 25(OH)D levels against melanoma (25–28), while others suggest no significant association or produce contradictory results (29–34). These discrepancies are thought to arise from confounding variables such as lifestyle, genetics, sunlight exposure, skin type, and the potential for reverse causation, thus complicating the inference of a causal relationship (35). Given these limitations inherent in observational studies, there is a pressing need for more robust research methodologies that can provide more valid causal inferences.
This research utilizes a two-sample Mendelian Randomization (MR) approach to investigate the potential causal link between serum 25-Hydroxyvitamin D levels and the incidence of cutaneous melanoma (36, 37). The MR methodology, which employs Single Nucleotide Polymorphisms (SNPs) as instrumental variables (IVs), offers an effective strategy to estimate causal relationships, mitigating bias from confounding factors and reverse causation that often confound traditional observational studies (38, 39). The study relies on three fundamental MR assumptions: relevance, independence, and exclusion restriction, to ensure that selected SNPs have a robust association with serum 25-Hydroxyvitamin D levels, are not associated with confounding variables, and affect melanoma risk exclusively through their impact on serum 25-Hydroxyvitamin D levels (40). The selection of SNPs and the outcome data were sourced from large-scale, publicly available genome-wide association study (GWAS) datasets (41).
Three key MR analysis methods were applied: Inverse Variance Weighted (IVW), weighted median, and MR-Egger regression (42). These techniques provide a comprehensive examination of the potential causal relationship while addressing varying conditions of instrument validity and pleiotropy. To verify the robustness and validity of the findings, a series of sensitivity analyses were conducted, including Cochran’s Q Test, a Pleiotropy Test, and a “leave-one-out” analysis (43). The Radial MR method, an innovative technique for outlier identification and exclusion, was also employed, thereby enhancing the reliability of the findings (44). The analysis of this study indicate a statistically significant causal association between serum 25-Hydroxyvitamin D levels and melanoma incidence.
Our findings not only shed light on the potential role of Vitamin D in melanoma pathogenesis but also underscore the possible implications for prevention and therapeutic strategies, particularly in regard to vitamin D optimization strategies. This study bridges a gap in the existing literature and sets the foundation for future research, although clinical decision-making should carefully consider the inherent limitations of MR studies, individual health considerations, and the multifaceted nature of melanoma etiology. Our findings point to new avenues for melanoma prevention, but further investigation is warranted to fully elucidate the precise biological implications and clinical applicability of serum 25-Hydroxyvitamin D levels in melanoma risk.
Our study was undertaken following the framework of a two-sample MR model utilizing preselected instrumental variables (37, 45). The schematic framework of the MR design is delineated in Figure 1. The validity of our research hinged on three pivotal assumptions (40): (1) Relevance Assumption: the Single Nucleotide Polymorphisms demonstrated a robust association with serum 25-Hydroxyvitamin D concentrations which were measured using a validated assay method; (2) Independence Assumption: SNPs were not linked to confounding variables which were identified based on established biological and epidemiological knowledge about potential confounders of the association between 25-Hydroxyvitamin D and melanoma; (3) Exclusion Restriction Assumption: SNPs influence melanoma outcomes solely through their potential impact on serum 25-Hydroxyvitamin D levels which required a comprehensive review of the existing literature to exclude other potential causal pathways (39). In adherence to MR analysis standards, we carefully selected SNPs that were previously reported to be strongly associated with serum 25-Hydroxyvitamin D concentrations. These were chosen as they were not linked to known confounding variables and their influence on melanoma was only due to their potential impact on serum 25-Hydroxyvitamin D levels, thereby satisfying the three key MR assumptions.
Figure 1 Flow diagram delineating the design process of a two-sample Mendelian randomization study. This figure includes a description of the selection of instrumental variables, identification of exposure and outcome datasets, and the methods used for the MR analysis and sensitivity analysis.
In order to ensure the robustness of our study, a comprehensive verification of these assumptions was performed through a thorough statistical analysis of SNP–exposure and SNP–outcome associations (46). This was crucial in order to meet the rigorous statistical requirements for a valid MR study and strengthen the credibility of our conclusions. To further substantiate our findings and strengthen the validity of our study, our analyses also accounted for potential bidirectional relationships, secondary pleiotropic effects, and population stratification, which may pose plausible threats to the validity of these assumptions.
The MR analyses were executed using R software (version 4.0.3), supplemented with the “TwoSampleMR” (version 0.5.6) and “RadialMR” (version 1.0) packages. The R environment was preferred due to its extensive statistical functionalities and capacity to handle large-scale genomic datasets, crucial for executing an MR study of this magnitude (47). The TwoSampleMR package enables the implementation of two-sample MR analysis by providing functions for data extraction, harmonization, and performing several statistical methods, while the RadialMR package, based on modified second-order weights, allows for the detection and subsequent exclusion of outliers (37).
Genetic associations for the serum 25-Hydroxyvitamin D levels (ebi-a-GCST90000618) were sought in publicly available genome-wide association study datasets, which contained data for 496,946 samples and 6,896,093 SNPs (48). These large, heterogeneous datasets provide a valuable and diverse genetic background for assessing the association of SNPs with vitamin D levels (49). These large datasets were chosen to ensure adequate power to detect even small effect sizes and to allow for the inclusion of a large number of IVs (50). These datasets fulfilled the minimum criteria requisite for importation from the European Bioinformatics Institute (EBI) database of complete GWAS summary data (51). We pinpointed SNPs exhibiting robust associations with serum 25-Hydroxyvitamin D, establishing a stringent threshold for statistical significance (P < 5*10^-9), linkage disequilibrium (LD) r^2 < 0.001, and LD distance > 10,000 kb. The F statistic was employed to rule out weak instrument bias that might contravene the first MR assumption, thereby evaluating the strength of the association between SNPs and serum 25-Hydroxyvitamin D levels (52, 53). This rigorous selection process ensures the minimization of false-positive results, enhancing the reliability of our IVs. The use of such large and comprehensive GWAS datasets ensures the robustness and external validity of our findings (54). The stringent criteria set for SNP selection help ensure the quality of IVs and the accuracy of subsequent analyses.
Regarding the outcome data, we obtained melanoma skin cancer GWAS data ieu-b-4969 from the ieu-b datasets, a summary data compilation generated by several consortia that were manually curated, initially created for MR-Base (55). This dataset was selected for its extensive coverage and high-quality data, ensuring that the subsequent analyses would be adequately powered and encompass a comprehensive range of genetic variations associated with melanoma (56). This melanoma data consisted of 375,767 samples, and included 11,396,019 SNPs which were all carefully checked for quality control measures including genotyping accuracy and Hardy-Weinberg equilibrium. We emphasized harmonization to minimize inconsistencies and discrepancies between the different datasets, which is a critical aspect when working with such large-scale genetic data. The data was harmonized for subsequent MR analysis including the alignment of the effect allele and standardization of the units of measurement for both the exposure and outcome variables. Moreover, in order to mitigate any potential bias, we strictly observed a minor allele frequency (MAF) cut-off of 0.01, thus ensuring that all included SNPs had sufficient population frequency to warrant their inclusion (57).
A two-sample MR analysis was performed employing three primary methods: inverse variance weighted median, weighted median, and MR-Egger, aiming to assess the potential causal relationship between serum 25-Hydroxyvitamin D levels and melanoma (37). These three methods each address different potential sources of bias in MR analyses, and thus together provide a robust and comprehensive evaluation of the causal relationship. IVW approach combines the strengths of different SNPs and their individual effects in an efficient manner to yield an overall estimate. Weighted median allows for more heterogeneity, enabling up to 50% of the genetic variants to be invalid instruments. Meanwhile, MR-Egger provides a measure of directional pleiotropy and is less prone to bias when the assumptions of the other two methods are violated. Each analysis was conducted using the corresponding two-sample MR packages in R, per developers’ guidelines. The use of multiple methods provides a comprehensive and robust assessment of potential causal relationships, while also providing an opportunity for comparison and cross-validation of the results.
The IVW approach combined meta-analysis with Wald estimates for each SNP to yield an aggregate effect estimate for melanoma. IVW results remain unbiased provided no horizontal pleiotropy is observed (58). The Wald ratio for each SNP was calculated as the ratio of the SNP-outcome association to the SNP-exposure association (59). Horizontal pleiotropy, where genetic variants affect the outcome through pathways other than the exposure, can introduce bias into the MR estimates. The assumption of no horizontal pleiotropy is critical as it ensures that the SNP’s effect on melanoma is channeled solely through its influence on serum 25-Hydroxyvitamin D levels, thereby ensuring valid estimates (60).
While estimates from the random and fixed effects IVW models are identical, the variance in the random effects model is inflated to account for SNP heterogeneity. Consequently, the fixed-effect model was implemented in scenarios devoid of observed heterogeneity (p > 0.05) which assumes that the true effect size is the same for all SNPs and any variation is due to sampling error, thus providing a more conservative estimate. Adoption of the appropriate model as per the observed heterogeneity helps prevent inaccuracies that could arise due to the misapplication of a fixed or random effects model.
MR-Egger regression, grounded on the assumption of instrument strength independence from direct effect, enables the evaluation of pleiotropy presence via the intercept term (61). This intercept can be interpreted as an estimate of the average direct effect of the genetic variants on the outcome, not through the exposure. In other words, it provides a measure of the overall directional pleiotropy. An intercept value equal to zero suggests nonexistence of horizontal pleiotropy and MR-Egger regression outcome consistency with IVW. This method also allows for the assessment of any potential directional pleiotropy - a significant deviation from zero indicates that the IVs may be affecting the outcome through pathways other than 25-Hydroxyvitamin D levels. Pleiotropy, if undetected, can introduce bias and misdirect our interpretations of the results.
The correlation LD between selected SNPs and potential confounding factors required careful assessment to ensure methodological robustness and conformity with the second MR assumption, as any correlation is unacceptable. In the context of an MR analysis, SNPs in LD could violate the Independence Assumption and confound the results. Therefore, a clumping procedure was undertaken to ensure the SNPs were in minimal LD with each other, thereby enhancing the validity of our study. This step is crucial as it reduces the possibility of SNP-SNP interaction, which can confound the results.
We utilized Cochran’s Q Test and a Pleiotropy Test to assess the robustness of our findings (62). Cochran’s Q statistics were employed to quantify the heterogeneity among the IVs (63). Heterogeneity among the IVs could reflect an invalid assumption of no horizontal pleiotropy or a violation of the Exclusion Restriction Assumption. This allowed us to understand if the individual SNP effects were more varied than what would be expected by chance alone. In addition, to pinpoint potentially heterogeneous SNPs, a “leave-one-out” analysis was carried out (64). This analysis evaluated the reliability of the relationship between SNPs and exposure, and assessed whether any particular SNP was contributing disproportionately to significant results. Evidence of heterogeneity suggests that certain genetic instruments may be invalid (p < 0.05). The leave-one-out analysis is a robust way to identify any single genetic instrument that may unduly influence the study results, ensuring the stability of our MR estimates. Such an analysis is invaluable in identifying and excluding potential outlier SNPs that may unduly influence the MR estimates.
Pleiotropy tests were carried out to investigate the influence of serum 25-Hydroxyvitamin D levels on melanoma risk within the context of MR analysis (65). A p-value less than 0.05 indicates an absence of horizontal pleiotropy among selected genetic instruments and suggests the need for a more comprehensive modelling framework to identify outliers. Detecting pleiotropy early is vital for maintaining the integrity of the study, as unidentified pleiotropy can potentially bias the MR results. Any indication of pleiotropy prompted an in-depth exploration of the data and warranted a comprehensive examination of the outliers in our modelling framework. Figure 2 is the schematic representation of the comprehensive design of the analysis process for the Mendelian randomization study (66).
Figure 2 Schematic representation of the comprehensive design of the Mendelian randomization study. This figure provides a visual summary of our study design, illustrating the key steps in the data acquisition, analysis, and interpretation process.
Our study utilized an innovative methodology, employing modified second-order weights, to investigate potential outliers within MR analysis. This was facilitated through the use of the “RadialMR” package (version 1.0) in the R programming environment, permitting the identification of outliers that could distort the causal estimates and enabling subsequent reanalysis after their exclusion (44). The modified second-order weights calculated using RadialMR account for both the first and second moments of the error term. This is in contrast to the traditional MR-Egger regression that only considers the first moment. By considering both moments, RadialMR can detect influential outliers that might bias the MR estimates and remove them, thus providing a more robust and reliable estimate of the causal effect. The entire process was automated within the package, ensuring the standardization of the method across all the data.
Radial MR has been increasingly recognized for its capability to detect and adjust for potential outlier SNPs. By reweighting the SNP estimates and corresponding standard errors based on their deviance from the overall MR estimate, the Radial MR methodology can help limit undue influence from outlier SNPs. It offers another layer of robustness to our study and can contribute significantly to the precision of the estimates. In the course of the Radial MR analysis, SNPs identified as outliers were removed in a stepwise manner and the MR estimates were recalculated at each step. The iterative nature of the Radial MR analysis allowed us to examine the impact of each SNP and assess the stability of our results. If a particular SNP caused a significant deviation in the MR estimate, this might indicate a violation of the assumptions underlying MR analysis, such as pleiotropy or linkage with confounding factors.
A statistically significant causal association was inferred between serum 25-Hydroxyvitamin D levels and the incidence of melanoma, as determined by the Inverse Variance Weighted (IVW) Mendelian Randomization (MR) method (β = 0.0022159, p = 0.0494391) (Figure 3). This approach uses genetic variants as instrumental variables (IVs) to dissect causal associations in observational studies. With the avoidance of environmental confounding and bias due to reverse causation, which commonly plague conventional observational studies, the MR approach gives robust evidence of causality.
Figure 3 Scatter plot illustrating the associations between melanoma skin cancer (y-axis) and Serum 25-Hydroxyvitamin D levels (x-axis). The slope of the regression line serves as an estimate of the causal effect between these variables.
In the realm of MR, the β coefficient indicates the magnitude of the effect size or the estimated change in the outcome (melanoma incidence in this case) associated with a one unit increase in the exposure (serum 25-Hydroxyvitamin D levels). The p-value is indicative of the statistical significance of this association, with our p = 0.0494391 suggesting that this association is significant at the 5% level. The result indicates that for each unit increase in serum 25-Hydroxyvitamin D levels, the incidence of melanoma increases by 0.0022159 units. It’s worth noting that this β coefficient, despite being relatively small, points towards a positive relationship, suggesting an increase in melanoma incidence with elevated serum 25-Hydroxyvitamin D levels. It’s important to acknowledge the fact that even a seemingly minuscule rise in melanoma risk can be of significant public health relevance, given the severity and escalating incidence of this form of skin cancer worldwide (67).
The scatter plot shown in Figure 3 visually portrays the individual genetic variants that contribute to the aggregate data point, representing the causal estimate. Each data point on the scatter plot signifies a genetic variant, with its position being determined by its association with both melanoma risk and vitamin D levels. The slope of the regression line captures the average causal effect of these genetic variants, providing a visual representation of the aggregate causal estimate determined by the IVW method.
In the context of MR studies, heterogeneity refers to the variability in the estimates of the causal effect derived from each individual genetic variant. The Cochran Q-test of heterogeneity, applied to the IVW method, indicated the absence of significant heterogeneity in the study (Q = 95.46249, P = 0.1299563) (Table 1). This Cochran Q-test result further bolsters the study’s validity by demonstrating the homogeneity of the genetic instruments used (68). A non-significant Q statistic indicates that the variation across the different causal estimates is within what might be expected due to sampling variability, suggesting the lack of bias in the causal estimation. This Q statistic and associated p-value highlight that the individual effects sizes derived from the different SNPs included in the MR analysis do not significantly differ from each other, thereby confirming homogeneity among the studies and supporting the overall validity of the pooled causal estimate. However, the p-value above 0.05 does not entirely exclude the possibility of minor heterogeneity among the included SNPs.
Table 1 Detailed MR findings of the causal association between serum 25-Hydroxyvitamin D levels and melanoma incidence.
Table 1 presents the results from various Mendelian randomization analyses, employing different methodologies such as Inverse Variance Weighted, MR Egger, Weighted Median, Simple Mode, and Weighted Mode. Each row provides key metrics including the number of SNPs (nSNP), effect size (β), standard error (Se), p-value, Q p-value (a measure of heterogeneity), and a Pleiotropy test result, thus offering a comprehensive view of the genetic association and potential bias in each analysis method.
In the robustness assessment through a “leave-one-out” sensitivity analysis, the IVW estimates obtained after successively excluding each SNP approximated the IVW estimates from the complete set of SNPs. This consistency suggested that no individual SNP exerted a substantial influence on the estimated causal relationship (Figure 4). This analysis is pivotal in mitigating the potential of a single SNP disproportionately skewing the estimated effect size. The consistent estimates from this analysis highlight the robustness of the IVW method, reducing the likelihood of overestimated or spurious causal inferences. If the revised estimates do not deviate significantly from the original, it implies that no single SNP disproportionally affects the results. It indicates that the identified correlation does not rely on any particular SNP, lending credibility to the inference that the effect is a genuine result of the overall genetic variation.
Figure 4 Leave-one-out sensitivity analysis of the impact of serum 25-Hydroxyvitamin D levels on melanoma skin cancer incidence. Each circle represents an estimate of the causal effect susceptibility between Serum 25-Hydroxyvitamin D levels and melanoma skin cancer, with the bars indicating the confidence interval (CI).
This figure visualizes the dispersion of the causal effect estimates upon successive omission of each SNP. The position of the circles along the y-axis represents the effect estimate, while the horizontal bars reflect the degree of uncertainty around these estimates. The tight clustering of these points along the y-axis reflects the consistency of the estimates, reinforcing the stability of the causal inference.
A detailed annotation of the SNPs illustrated on the ordinate of Figure 4 is provided in Supplementary Table 1 by searching in the PhenoScanner V2 database (69, 70). This table not only lists the gene symbols corresponding to each SNP, but it also highlights the traits associated with these genetic variations, thereby emphasizing potential phenotypic implications of these genomic discrepancies. This feature underscores the potential phenotypic ramifications inherent in these genetic variations, highlighting the complex interplay between genotype and phenotype. In interpreting these genetic associations, it is imperative to consider the broader genomic context in which these SNPs exist. Notably, while the SNPs’ impact on 25-Hydroxyvitamin D levels might influence melanoma risk, they could also confer pleiotropic effects that potentially influence other phenotypes. This data provides an exhaustive map of the genomic landscape surrounding the examined association, enabling a deeper understanding of the genetic underpinnings potentially influencing both Vitamin D levels and melanoma risk
To further evaluate the robustness of the MR study, a pleiotropic test was conducted. Pleiotropy is a phenomenon where a single gene or genetic variant influences multiple traits. It can be a potential source of bias in MR studies, making its assessment vital for ensuring the accuracy of the results. In order for MR to maintain validity, it is essential that there is no violation of the exclusion restriction assumption - that is, genetic variants should not be directly associated with the outcome beyond their influence on the exposure. Pleiotropy, particularly horizontal pleiotropy, where the effect of a genetic variant on the outcome surpasses its impact on the exposure, can potentially bias the MR results. Our pleiotropic test indicated no substantial pleiotropic effect, reinforcing the validity of our findings (Figure 5). The genetic variants used as IVs in our MR analysis did not show signs of significantly influencing melanoma incidence through pathways other than their impact on serum 25-Hydroxyvitamin D levels. This is a critical consideration, as ignoring potential pleiotropy could lead to erroneous interpretations of the causal relationship. The absence of horizontal pleiotropy is crucial in our MR framework as it upholds the direction and magnitude of the estimated causal relationship between the variables (60).
Figure 5 Funnel plot of the estimated causal effect of serum 25-Hydroxyvitamin D levels on melanoma skin cancer incidence. Each point symbolizes the estimated causal effect of each instrumental variable (IV). The dark blue vertical line represents the MR-Egger method-derived causal effect estimate, while the light blue line signifies the equivalent estimate derived via the IVW method.
This funnel plot serves as a visual assessment of potential pleiotropic effects. The spread of the points provides an indication of the degree of heterogeneity across the causal estimates obtained from individual IVs. The symmetry of the plot around the causal effect line further reinforces the absence of substantial horizontal pleiotropy. The clustering of IVs around the vertical line, indicating the MR-Egger method-derived causal effect estimate, supports the notion of symmetric distribution, a key assumption in MR-Egger regression, thereby demonstrating the absence of substantial horizontal pleiotropy (71). The close alignment of the MR-Egger and IVW estimates further supports the argument that pleiotropy is unlikely to have significantly distorted our results.
In the final stages of our study, the Radial MR method was harnessed to assess the outliers identified earlier. The outcome of this rigorous analysis revealed a positive correlation in MR results, even upon outlier exclusion. This persistent correlation underscores the robustness of our findings against statistical anomalies (Figure 6). The Radial MR method, an advanced outlier-detection technique, provides another level of reliability by ensuring the robustness of the study’s conclusions despite the presence of potential outliers. The persistent positive correlation indicates that the key findings of the study are not overly reliant on a small number of influential data points. Outliers in genetic association studies can often be a consequence of various factors such as genetic heterogeneity, population stratification, or genotyping errors (72). By conducting a Radial MR analysis, which excludes potential outliers, we have ensured that the observed correlation is not an artifact of a few extreme observations, further strengthening the robustness of our conclusions. Despite the presence of outliers, as indicated by the yellow portions of the plot, the overall shape and pattern of the plot underscores the consistent association between Vitamin D levels and melanoma risk.
Figure 6 A Radial MR plot detailing the IVW radial of SNPs. The blue portions of the plot denote variant SNPs, while the yellow portions signify the identified outliers. This plot gives a visual representation of the impact of each SNP on the overall result, distinguishing between regular genetic variants and the outliers. The IVW radial plot indicates that the influence of the outliers on the overall outcome is minimal, supporting the conclusion that our results are robust.
In summation, our Mendelian randomization study provides evidence for a causal relationship between serum 25-Hydroxyvitamin D levels and the risk of melanoma. This relationship has been reinforced through a series of rigorous validations including sensitivity, pleiotropic, and outlier analyses. Our results propose a positive causal effect of serum 25-Hydroxyvitamin D levels on melanoma incidence.
However, it is pertinent to emphasize that MR results should not be interpreted in isolation, but need to be considered within the context of the broader body of evidence. While MR analyses provide evidence for causal relationships in an observational setting, validation through experimental or interventional studies is necessary to gain insight into the actual biological mechanisms that underpin this relationship. The ideal way to pursue this would be through prospective cohort studies or randomized controlled trials. Moreover, any conclusions should be interpreted keeping in view the overall health benefits of vitamin D, and the complex interplay between vitamin D physiology, skin cancer biology, and genetics.
Our two-sample MR study, using the IVW method and leveraging genetic variants as IVs, provided substantial evidence for a causal association between serum 25-Hydroxyvitamin D levels and the incidence of cutaneous melanoma. This approach circumvented the environmental confounding and reverse causation bias, commonly encountered in traditional observational studies. Our study yielded a β coefficient of 0.0022159, indicating a significant positive correlation between increased serum 25-Hydroxyvitamin D levels and melanoma incidence. This relationship was consistently shown to be statistically significant, albeit with a small β coefficient, indicating that each unit increase in serum 25-Hydroxyvitamin D levels corresponds with an increased risk of melanoma. This is notable considering the increasing global incidence of this skin cancer.
We assessed the robustness of our results through multiple measures. The lack of significant heterogeneity among the genetic variants used reinforced the validity of our pooled causal estimate. Further, the “leave-one-out” sensitivity analysis affirmed that no individual SNP exerted a disproportionate influence on the estimated causal relationship. Tests for potential pleiotropy found minimal effects, which further validated the causality inferred. The Radial MR analysis, even after outlier exclusion, consistently demonstrated a persistent positive correlation between serum 25-Hydroxyvitamin D levels and melanoma incidence.
The study’s results have profound public health implications, suggesting that, contrary to traditional beliefs, elevated serum 25-Hydroxyvitamin D levels may play a contributory role in the development of melanoma. This provides a nuanced perspective on melanoma etiology, which can guide future investigations and potentially inform prevention strategies. Despite the generally beneficial health effects attributed to serum 25-Hydroxyvitamin D (73), its potential influence on melanoma development should not be overlooked.
This study presents a unique perspective, in contrast to some existing literature, by revealing a positive causal relationship between increased serum 25-Hydroxyvitamin D levels and melanoma risk (27, 34). This finding diverges from previous observational studies that have often suggested an inverse or null association, potentially due to limitations inherent in such studies such as confounding and reverse causality (27, 74–76). The divergence from observational studies reflects the intricate nature of Vitamin D metabolism, immune modulation, skin carcinogenesis, and the multifaceted role of UV radiation (77, 78). It further emphasizes the importance of nuanced, context-specific investigations and robust methodologies to decipher this complex relationship (28, 34, 79).
These divergent findings underscore the complex interplay between Vitamin D physiology, sun exposure necessary for Vitamin D synthesis, and the risk of skin cancer (80, 81). On one hand, Vitamin D is acknowledged for its beneficial roles in health (82), while on the other hand, sun exposure, being a significant source of Vitamin D, is also a primary risk factor for melanoma due to potential DNA damage from ultraviolet radiation (83). This dynamic highlights the intricate balance between the potential benefits and hazards of sun exposure. UV radiation is a shared risk factor for melanoma and a primary source of Vitamin D synthesis, creating a complex interplay between the potential benefits and hazards of sun exposure and Vitamin D’s generally protective effects (32).
The research findings necessitate a reassessment of current recommendations concerning sun exposure and vitamin D supplementation, especially for high-risk populations (84). The study questions the perception of vitamin D as an exclusively beneficial agent, highlighting the potential risks associated with its excessive intake. This calls for a nuanced understanding of vitamin D’s role in melanoma pathogenesis and advocates a careful risk-benefit assessment regarding vitamin D supplementation and sun exposure. A key insight from the study is the critical need for personalized medicine strategies that take into account individuals’ genetic susceptibility when deciding about Vitamin D supplementation.
The study underscores the utility of Mendelian randomization as a robust tool in biomedical research, capable of identifying causal relationships that might be overlooked in traditional observational studies. This strengthens the opportunity to delve deeper into the complex interplay between vitamin D metabolism, genetics, and skin cancer biology (85). However, despite the potential association between elevated vitamin D levels and melanoma, the study reaffirms the established health benefits of maintaining adequate vitamin D levels. It emphasizes the importance of a balanced approach to vitamin D supplementation and sun exposure, alongside careful monitoring of serum vitamin D levels, especially in populations at high risk of melanoma.
From a policy perspective, these findings prompt a thorough reevaluation of vitamin D supplementation guidelines, particularly for high-risk populations (86). Regarding biomedical research, the discovery of this relationship presents opportunities to probe the underlying biological mechanisms that may explain how elevated serum 25-Hydroxyvitamin D levels contribute to increased melanoma risk (87). Nonetheless, given the intricate nature of vitamin D metabolism and its various health benefits, the study’s findings should be interpreted with caution.
While the study provides valuable insights, it does possess a few inherent limitations. Firstly, the study assumes that the instrumental variables, in this case, genetic variants, impact the outcome solely via their effect on the exposure, a requirement known as the exclusion restriction criterion. However, despite our rigorous tests, we cannot definitively exclude the possibility of unrecognized pleiotropy, where a gene could affect multiple traits, or unknown confounding factors influencing our findings. Secondly, our analysis does not provide detailed insights into the biological mechanisms connecting serum 25-Hydroxyvitamin D levels and melanoma risk. The identified causal relationship does not fully unravel the complex biology of Vitamin D and its role in melanoma incidence. Further experimental validation or prospective cohort studies are necessary to comprehend these mechanisms.
Our study also acknowledges limitations related to the generalizability of the findings. The genetic instruments used were primarily identified in populations of European ancestry, potentially limiting the application of our results to other ethnic groups. Additionally, our study did not account for individual-level confounders, nor did it explore potential non-linear relationships between serum 25-Hydroxyvitamin D levels and melanoma risk. The study also overlooked the modulatory roles of factors like age, sex, and environmental UV exposure, given its reliance on summarized population-level data. Moreover, our analysis assumes a linear relationship between exposure and outcome, which may oversimplify the biological reality.
Future research should adopt an integrative approach that validates initial findings, investigates underlying biological mechanisms, expands the scope of genetic studies, replicates results in diverse populations, and explores potential confounding factors. Studies should focus on establishing the optimal range of vitamin D levels that balance the potential risks and benefits, the health implications of vitamin D in skin cancer prevention, and the association of vitamin D with other forms of skin cancer.
The comprehensive MR study evidenced a positive causal relationship between serum 25-Hydroxyvitamin D levels and melanoma incidence, holding significant implications for public health policies, clinical guidelines, and cancer prevention strategies. However, given the intricacy of vitamin D metabolism, skin cancer biology, and the broader health benefits of vitamin D, these findings necessitate cautious interpretation and further exploration. Continued research is needed to consolidate these findings, unravel the complex interplay between genetics, environment, and biology, and fully understand the biological mechanisms underlying this association. Despite the inherent limitations, Mendelian randomization proves a valuable tool in biomedical research, resolving causal ambiguities and enhancing context-specific, evidence-based health interventions. Future research should focus on corroborating these findings, dissecting all contributing factors to melanoma risk, and illuminating novel therapeutic targets. Ultimately, any health policy or strategy modulating vitamin D levels must balance these findings with the broader health benefits of vitamin D.
The original contributions presented in the study are included in the article/Supplementary Material. Further inquiries can be directed to the corresponding authors.
BC, QL and BW made substantial contributions to the conception and design of the study. BC, QL, RK and XS performed data acquisition and analysis. BC, JY and XN made substantial contributions to drafting the article and graphs. XL and BW reviewed and marked the complete manuscript. All the authors read and approved the final manuscript.
This work was supported by grants from Special Scientific Research Project of Fujian Provincial Finance Project (No. 2019B031); Young and Middle-aged Key Personnel Training Project of Fujian Provincial Health Commission(No. 2020GGB029); Youth Scientific Research Project of Fujian Provincial Health Commission(No. 2020QNA048); Startup Fund for Scientific Research of Fujian Medical University (No. 2019QH2030).
Genetic association estimates for the study were obtained from the UK Biobank study and FinnGen consortium. The authors thank all investigators for sharing these data.
The authors declare that the research was conducted in the absence of any commercial or financial relationships that could be construed as a potential conflict of interest.
All claims expressed in this article are solely those of the authors and do not necessarily represent those of their affiliated organizations, or those of the publisher, the editors and the reviewers. Any product that may be evaluated in this article, or claim that may be made by its manufacturer, is not guaranteed or endorsed by the publisher.
The Supplementary Material for this article can be found online at: https://www.frontiersin.org/articles/10.3389/fonc.2023.1154107/full#supplementary-material
Supplementary Table 1 | Gene symbols and traits corresponding to the SNPs listed in the ordinate of .
1. Centeno PP, Pavet V, Marais R. The journey from melanocytes to melanoma. Nat Rev Cancer (2023) 23(6):372–90. doi: 10.1038/s41568-023-00565-7
2. Guo W, Wang H, Li C. Signal pathways of melanoma and targeted therapy. Signal Transduct Target Ther (2021) 6(1):424. doi: 10.1038/s41392-021-00827-6
3. Yang K, Oak ASW, Slominski RM, Brożyna AA, Slominski AT. Current molecular markers of melanoma and treatment targets. Int J Mol Sci (2020) 21(10):3535. doi: 10.3390/ijms21103535
4. Gandini S, Sera F, Cattaruzza MS, Pasquini P, Picconi O, Boyle P, et al. Meta-analysis of risk factors for cutaneous melanoma: II. Sun exposure. Eur J Cancer (Oxford England: 1990) (2005) 41(1):45–60. doi: 10.1016/j.ejca.2004.10.016
5. Berwick M, Armstrong BK, Ben-Porat L, Fine J, Kricker A, Eberle C, et al. Sun exposure and mortality from melanoma. J Natl Cancer Institute (2005) 97(3):195–9. doi: 10.1093/jnci/dji019
6. Brożyna AA, Hoffman RM, Slominski AT. Relevance of vitamin D in melanoma development, progression and therapy. Anticancer Res (2020) 40(1):473–89. doi: 10.21873/anticanres.13976
7. Curti BD, Faries MB. Recent advances in the treatment of melanoma. New Engl J Med (2021) 384(23):2229–40. doi: 10.1056/NEJMra2034861
8. Huang AC, Zappasodi R. A decade of checkpoint blockade immunotherapy in melanoma: understanding the molecular basis for immune sensitivity and resistance. Nat Immunol (2022) 23(5):660–70. doi: 10.1038/s41590-022-01141-1
9. Aroldi F, Middleton MR. Long-term outcomes of immune checkpoint inhibition in metastatic melanoma. Am J Clin Dermatol (2022) 23(3):331–8. doi: 10.1007/s40257-022-00681-4
10. Slominski AT, Brożyna AA, Zmijewski MA, Jóźwicki W, Jetten AM, Mason RS, et al. Vitamin D signaling and melanoma: role of vitamin D and its receptors in melanoma progression and management. J Tech Methods Pathol (2017) 97(6):706–24. doi: 10.1038/labinvest.2017.3
11. Gallagher JC, Rosen CJ. Vitamin D: 100 years of discoveries, yet controversy continues. Lancet Diabetes Endocrinol (2023) 11(5):362–74. doi: 10.1016/S2213-8587(23)00060-8
12. Rebelos E, Tentolouris N, Jude E. The role of vitamin D in health and disease: A narrative review on the mechanisms linking vitamin D with disease and the effects of supplementation. Drugs (2023) 83(8):665–85. doi: 10.1007/s40265-023-01875-8
13. Jolliffe DA, Walton RT, Griffiths CJ, Martineau AR. Single nucleotide polymorphisms in the vitamin D pathway associating with circulating concentrations of vitamin D metabolites and non-skeletal health outcomes: Review of genetic association studies. J Steroid Biochem Mol Biol (2016) 164:18–29. doi: 10.1016/j.jsbmb.2015.12.007
14. Tuckey RC, Cheng CYS, Slominski AT. The serum vitamin D metabolome: What we know and what is still to discover. J Steroid Biochem Mol Biol (2019) 186:4–21. doi: 10.1016/j.jsbmb.2018.09.003
15. Norlin M, Wikvall K. Enzymatic activation in vitamin D signaling - Past, present and future. Arch Biochem Biophysics (2023) 742:109639. doi: 10.1016/j.abb.2023.109639
16. Latacz M, Snarska J, Kostyra E, Fiedorowicz E, Savelkoul HF, Grzybowski R, et al. Single nucleotide polymorphisms in 25-hydroxyvitamin D3 1-alpha-hydroxylase (CYP27B1) gene: the risk of Malignant tumors and other chronic diseases. Nutrients (2020) 12(3):801. doi: 10.3390/nu12030801
17. Meyer MB, Pike JW. Mechanistic homeostasis of vitamin D metabolism in the kidney through reciprocal modulation of Cyp27b1 and Cyp24a1 expression. J Steroid Biochem Mol Biol (2020) 196:105500. doi: 10.1016/j.jsbmb.2019.105500
18. Zhou Y, Zhao LJ, Xu X, Ye A, Travers-Gustafson D, Zhou B, et al. DNA methylation levels of CYP2R1 and CYP24A1 predict vitamin D response variation. J Steroid Biochem Mol Biol (2014) 144 Pt A:207–14. doi: 10.1016/j.jsbmb.2013.10.004
19. Jones G, Prosser DE, Kaufmann M. 25-Hydroxyvitamin D-24-hydroxylase (CYP24A1): its important role in the degradation of vitamin D. Arch Biochem Biophysics (2012) 523(1):9–18. doi: 10.1016/j.abb.2011.11.003
20. Slominski AT, Kim TK, Shehabi HZ, Semak I, Tang EK, Nguyen MN, et al. In vivo evidence for a novel pathway of vitamin D3 metabolism initiated by P450scc and modified by CYP27B1. Off Publ Fed Am Societies Exp Biol (2012) 26(9):3901–15. doi: 10.1096/fj.12-208975
21. Martin-Gorgojo A, Gilaberte Y, Nagore E. Vitamin D and skin cancer: an epidemiological, patient-centered update and review. Nutrients (2021) 13(12):4292. doi: 10.3390/nu13124292
22. Osborne JE, Hutchinson PE. Vitamin D and systemic cancer: is this relevant to Malignant melanoma? Br J Dermatol (2002) 147(2):197–213. doi: 10.1046/j.1365-2133.2002.04960.x
23. Berwick M, Garcia A. Solar UV exposure and mortality from skin tumors: an update. Adv Exp Med Biol (2020) 1268:143–54. doi: 10.1007/978-3-030-46227-7_7
24. Slominski AT, Brożyna AA, Skobowiat C, Zmijewski MA, Kim TK, Janjetovic Z, et al. On the role of classical and novel forms of vitamin D in melanoma progression and management. J Steroid Biochem Mol Biol (2018) 177:159–70. doi: 10.1016/j.jsbmb.2017.06.013
25. Newton-Bishop JA, Beswick S, Randerson-Moor J, Chang YM, Affleck P, Elliott F, et al. Serum 25-hydroxyvitamin D3 levels are associated with breslow thickness at presentation and survival from melanoma. J Clin Oncol (2009) 27(32):5439–44. doi: 10.1200/JCO.2009.22.1135
26. Randerson-Moor JA, Taylor JC, Elliott F, Chang YM, Beswick S, Kukalizch K, et al. Vitamin D receptor gene polymorphisms, serum 25-hydroxyvitamin D levels, and melanoma: UK case-control comparisons and a meta-analysis of published VDR data. Eur J Cancer (Oxford England: 1990) (2009) 45(18):3271–81. doi: 10.1016/j.ejca.2009.06.011
27. Tsai TY, Kuo CY, Huang YC. The association between serum vitamin D level and risk and prognosis of melanoma: a systematic review and meta-analysis. J Eur Acad Dermatol Venereol (2020) 34(8):1722–9. doi: 10.1111/jdv.16189
28. Cattaruzza MS, Pisani D, Fidanza L, Gandini S, Marmo G, Narcisi A, et al. 25-Hydroxyvitamin D serum levels and melanoma risk: a case-control study and evidence synthesis of clinical epidemiological studies. Eur J Cancer Prev (2019) 28(3):203–11. doi: 10.1097/CEJ.0000000000000437
29. Asgari MM, Maruti SS, Kushi LH, White E. A cohort study of vitamin D intake and melanoma risk. J Invest Dermatol (2009) 129(7):1675–80. doi: 10.1038/jid.2008.451
30. Tang JY, Fu T, Leblanc E, Manson JE, Feldman D, Linos E, et al. Calcium plus vitamin D supplementation and the risk of nonmelanoma and melanoma skin cancer: post hoc analyses of the women’s health initiative randomized controlled trial. J Clin Oncol (2011) 29(22):3078–84. doi: 10.1200/JCO.2011.34.5967
31. Befon A, Katoulis AC, Georgala S, Katsampas A, Chardalia V, Melpidou A, et al. Serum total 25-hydroxyvitamin D levels in patients with cutaneous Malignant melanoma: A case-control study in a low-risk Southern European population. Dermatol Pract Conceptual (2020) 10(1):e2020010. doi: 10.5826/dpc.1001a10
32. Mahamat-Saleh Y, Aune D, Schlesinger S. 25-Hydroxyvitamin D status, vitamin D intake, and skin cancer risk: a systematic review and dose-response meta-analysis of prospective studies. Sci Rep (2020) 10(1):13151. doi: 10.1038/s41598-020-70078-y
33. Stenehjem JS, Støer NC, Ghiasvand R, Grimsrud TK, Babigumira R, Rees JR, et al. Prediagnostic serum 25-hydroxyvitamin D and melanoma risk. Sci Rep (2020) 10(1):20129. doi: 10.1038/s41598-020-77155-2
34. Liyanage UE, Law MH, Barrett JH, Iles MM, MacGregor S. Is there a causal relationship between vitamin D and melanoma risk? A Mendelian randomization study. Br J Dermatol (2020) 182(1):97–103. doi: 10.1111/bjd.18238
35. Hutchinson PE, Pringle JH. Consideration of possible effects of vitamin D on established cancer, with reference to Malignant melanoma. Pigment Cell Melanoma Res (2022) 35(4):408–24. doi: 10.1111/pcmr.13040
36. Lawlor DA, Harbord RM, Sterne JA, Timpson N, Davey Smith G. Mendelian randomization: using genes as instruments for making causal inferences in epidemiology. Stat Med (2008) 27(8):1133–63. doi: 10.1002/sim.3034
37. Bowden J, Del Greco MF, Minelli C, Davey Smith G, Sheehan N, Thompson J. A framework for the investigation of pleiotropy in two-sample summary data Mendelian randomization. Stat Med (2017) 36(11):1783–802. doi: 10.1002/sim.7221
38. Burgess S, Butterworth A, Thompson SG. Mendelian randomization analysis with multiple genetic variants using summarized data. Genet Epidemiol (2013) 37(7):658–65. doi: 10.1002/gepi.21758
39. Burgess S, Small DS, Thompson SG. A review of instrumental variable estimators for Mendelian randomization. Stat Methods Med Res (2017) 26(5):2333–55. doi: 10.1177/0962280215597579
40. Davies NM, Holmes MV, Davey Smith G. Reading Mendelian randomisation studies: a guide, glossary, and checklist for clinicians. BMJ (Clin Res ed) (2018) 362:k601. doi: 10.1136/bmj.k601
41. Hemani G, Tilling K, Davey Smith G. Orienting the causal relationship between imprecisely measured traits using GWAS summary data. PloS Genet (2017) 13(11):e1007081. doi: 10.1371/journal.pgen.1007081
42. jBowden J, Davey Smith G, Haycock PC, Burgess S. Consistent estimation in mendelian randomization with some invalid instruments using a weighted median estimator. Genet Epidemiol (2016) 40(4):304–14. doi: 10.1002/gepi.21965
43. Poldrack RA, Huckins G, Varoquaux G. Establishment of best practices for evidence for prediction: A review. JAMA Psychiatry (2020) 77(5):534–40. doi: 10.1001/jamapsychiatry.2019.3671
44. Bowden J, Spiller W, Del Greco MF, Sheehan N, Thompson J, Minelli C, et al. Improving the visualization, interpretation and analysis of two-sample summary data Mendelian randomization via the Radial plot and Radial regression. Int J Epidemiol (2018) 47(4):1264–78. doi: 10.1093/ije/dyy101
45. Davey Smith G, Hemani G. Mendelian randomization: genetic anchors for causal inference in epidemiological studies. Hum Mol Genet (2014) 23(R1):R89–98. doi: 10.1093/hmg/ddu328
46. Burgess S, Scott RA, Timpson NJ, Davey Smith G, Thompson SG. Using published data in Mendelian randomization: a blueprint for efficient identification of causal risk factors. Eur J Epidemiol (2015) 30(7):543–52. doi: 10.1007/s10654-015-0011-z
47. Yavorska OO, Burgess S. MendelianRandomization: an R package for performing Mendelian randomization analyses using summarized data. Int J Epidemiol (2017) 46(6):1734–9. doi: 10.1093/ije/dyx034
48. Harold D, Abraham R, Hollingworth P, Sims R, Gerrish A, Hamshere ML, et al. Genome-wide association study identifies variants at CLU and PICALM associated with Alzheimer’s disease. Nat Genet (2009) 41(10):1088–93. doi: 10.1038/ng.440
49. Loh PR, Kichaev G, Gazal S, Schoech AP, Price AL. Mixed-model association for biobank-scale datasets. Nat Genet (2018) 50(7):906–8. doi: 10.1038/s41588-018-0144-6
50. Pierce BL, Ahsan H, Vanderweele TJ. Power and instrument strength requirements for Mendelian randomization studies using multiple genetic variants. Int J Epidemiol (2011) 40(3):740–52. doi: 10.1093/ije/dyq151
51. Hemani G, Zheng J, Elsworth B, Wade KH, Haberland V, Baird D, et al. The MR-Base platform supports systematic causal inference across the human phenome. eLife (2018) 7:e34408. doi: 10.7554/eLife.34408
52. Burgess S, Thompson SG. Bias in causal estimates from Mendelian randomization studies with weak instruments. Stat Med (2011) 30(11):1312–23. doi: 10.1002/sim.4197
53. Burgess S, Thompson SG. Avoiding bias from weak instruments in Mendelian randomization studies. Int J Epidemiol (2011) 40(3):755–64. doi: 10.1093/ije/dyr036
54. Xin J, Gu D, Chen S, Ben S, Li H, Zhang Z, et al. SUMMER: a Mendelian randomization interactive server to systematically evaluate the causal effects of risk factors and circulating biomarkers on pan-cancer survival. Nucleic Acids Res (2023) 51(D1):D1160–d7. doi: 10.1093/nar/gkac677
55. Bell KJL. Causal inference in melanoma epidemiology using Mendelian randomization. Br J Dermatol (2020) 182(1):13–4. doi: 10.1111/bjd.18646
56. Dusingize JC, Olsen CM, An J, Pandeya N, Law MH, Thompson BS, et al. Body mass index and height and risk of cutaneous melanoma: Mendelian randomization analyses. Int J Epidemiol (2020) 49(4):1236–45. doi: 10.1093/ije/dyaa009
57. Rüeger S, McDaid A, Kutalik Z. Evaluation and application of summary statistic imputation to discover new height-associated loci. PloS Genet (2018) 14(5):e1007371. doi: 10.1371/journal.pgen.1007371
58. Verbanck M, Chen CY, Neale B, Do R. Publisher Correction: Detection of widespread horizontal pleiotropy in causal relationships inferred from Mendelian randomization between complex traits and diseases. Nat Genet (2018) 50(8):1196. doi: 10.1038/s41588-018-0164-2
59. Brion MJ, Shakhbazov K, Visscher PM. Calculating statistical power in Mendelian randomization studies. Int J Epidemiol (2013) 42(5):1497–501. doi: 10.1093/ije/dyt179
60. Verbanck M, Chen CY, Neale B, Do R. Detection of widespread horizontal pleiotropy in causal relationships inferred from Mendelian randomization between complex traits and diseases. Nat Genet (2018) 50(5):693–8. doi: 10.1038/s41588-018-0099-7
61. Bowden J, Davey Smith G, Burgess S. Mendelian randomization with invalid instruments: effect estimation and bias detection through Egger regression. Int J Epidemiol (2015) 44(2):512–25. doi: 10.1093/ije/dyv080
62. Burgess S, Bowden J, Fall T, Ingelsson E, Thompson SG. Sensitivity analyses for robust causal inference from mendelian randomization analyses with multiple genetic variants. Epidemiol (Cambridge Mass) (2017) 28(1):30–42. doi: 10.1097/EDE.0000000000000559
63. Sanderson E, Davey Smith G, Windmeijer F, Bowden J. An examination of multivariable Mendelian randomization in the single-sample and two-sample summary data settings. Int J Epidemiol (2019) 48(3):713–27. doi: 10.1093/ije/dyy262
64. Cheng H, Garrick DJ, Fernando RL. Efficient strategies for leave-one-out cross validation for genomic best linear unbiased prediction. J Anim Sci Biotechnol (2017) 8:38. doi: 10.1186/s40104-017-0164-6
65. Hemani G, Bowden J, Davey Smith G. Evaluating the potential role of pleiotropy in Mendelian randomization studies. Hum Mol Genet (2018) 27(R2):R195–r208. doi: 10.1093/hmg/ddy163
66. Pierce BL, Burgess S. Efficient design for Mendelian randomization studies: subsample and 2-sample instrumental variable estimators. Am J Epidemiol (2013) 178(7):1177–84. doi: 10.1093/aje/kwt084
67. Burgess S, Davey Smith G, Davies NM, Dudbridge F, Gill D, Glymour MM, et al. Guidelines for performing Mendelian randomization investigations. Wellcome Open Res (2019) 4:186. doi: 10.12688/wellcomeopenres.15555.1
68. Greco MF, Minelli C, Sheehan NA, Thompson JR. Detecting pleiotropy in Mendelian randomisation studies with summary data and a continuous outcome. Stat Med (2015) 34(21):2926–40. doi: 10.1002/sim.6522
69. Kamat MA, Blackshaw JA, Young R, Surendran P, Burgess S, Danesh J, et al. PhenoScanner V2: an expanded tool for searching human genotype-phenotype associations. Bioinf (Oxford England) (2019) 35(22):4851–3. doi: 10.1093/bioinformatics/btz469
70. Staley JR, Blackshaw J, Kamat MA, Ellis S, Surendran P, Sun BB, et al. PhenoScanner: a database of human genotype-phenotype associations. Bioinf (Oxford England) (2016) 32(20):3207–9. doi: 10.1093/bioinformatics/btw373
71. Burgess S, Thompson SG. Interpreting findings from Mendelian randomization using the MR-Egger method. Eur J Epidemiol (2017) 32(5):377–89. doi: 10.1007/s10654-017-0255-x
72. Birke M, Schöpe J, Wagenpfeil S, Vogt T, Reichrath J. Association of vitamin D receptor gene polymorphisms with melanoma risk: A meta-analysis and systematic review. Anticancer Res (2020) 40(2):583–95. doi: 10.21873/anticanres.13988
73. Piotrowska A, Wierzbicka J, Żmijewski MA. Vitamin D in the skin physiology and pathology. Acta Biochim Polonica (2016) 63(1):17–29. doi: 10.18388/abp.2015_1104
74. Evans SR, Houghton AM, Schumaker L, Brenner RV, Buras RR, Davoodi F, et al. Vitamin D receptor and growth inhibition by 1,25-dihydroxyvitamin D3 in human Malignant melanoma cell lines. J Surg Res (1996) 61(1):127–33. doi: 10.1006/jsre.1996.0092
75. Garland CF, Garland FC, Gorham ED, Lipkin M, Newmark H, Mohr SB, et al. The role of vitamin D in cancer prevention. Am J Public Health (2006) 96(2):252–61. doi: 10.2105/AJPH.2004.045260
76. Caini S, Boniol M, Tosti G, Magi S, Medri M, Stanganelli I, et al. Vitamin D and melanoma and non-melanoma skin cancer risk and prognosis: a comprehensive review and meta-analysis. Eur J Cancer (Oxford England: 1990) (2014) 50(15):2649–58. doi: 10.1016/j.ejca.2014.06.024
77. Becker AL, Carpenter EL, Slominski AT, Indra AK. The role of the vitamin D receptor in the pathogenesis, prognosis, and treatment of cutaneous melanoma. Front Oncol (2021) 11:743667. doi: 10.3389/fonc.2021.743667
78. Bolerazska B, Rabajdova M, Spakova I, Marekova M. Current knowledge on the active form of Vitamin D synthesized in the skin and its effects on Malignant melanoma. Neoplasma (2017) 64(1):1–12. doi: 10.4149/neo_2017_101
79. Shellenberger RA, Gowda S, Kurn H, Albright J, Mayo MH. Vitamin D insufficiency and serum levels related to the incidence and stage of cutaneous melanoma: a systematic review and meta-analysis. Melanoma Res (2023) 33(4):265–74. doi: 10.1097/CMR.0000000000000897
80. Orlow I, Shi Y, Kanetsky PA, Thomas NE, Luo L, Corrales-Guerrero S, et al. The interaction between vitamin D receptor polymorphisms and sun exposure around time of diagnosis influences melanoma survival. Pigment Cell Melanoma Res (2018) 31(2):287–96. doi: 10.1111/pcmr.12653
81. Uitterlinden AG, Fang Y, Van Meurs JB, Pols HA, Van Leeuwen JP. Genetics and biology of vitamin D receptor polymorphisms. Gene (2004) 338(2):143–56. doi: 10.1016/j.gene.2004.05.014
82. Deeb KK, Trump DL, Johnson CS. Vitamin D signalling pathways in cancer: potential for anticancer therapeutics. Nat Rev Cancer (2007) 7(9):684–700. doi: 10.1038/nrc2196
83. Armstrong BK, Kricker A. The epidemiology of UV induced skin cancer. J Photochem Photobiol B Biol (2001) 63(1-3):8–18. doi: 10.1016/S1011-1344(01)00198-1
84. Paolino G, Moliterni E, Corsetti P, Didona D, Bottoni U, Calvieri S, et al. Vitamin D and melanoma: state of the art and possible therapeutic uses. Giornale Italiano di Dermatol e Venereol: Organo Ufficiale Societa Italiana di Dermatol e Sifilografia (2019) 154(1):64–71. doi: 10.23736/S0392-0488.17.05801-1
85. Moliterni E, Paolino G, Veronese N, Bottoni U, Corsetti P, Cardone M, et al. Prognostic correlation between vitamin D serological levels, Body Mass Index and clinical-pathological features in melanoma patients. Giornale Italiano di Dermatol e Venereol: Organo Ufficiale Societa Italiana di Dermatol e Sifilografia (2018) 153(5):732–3. doi: 10.23736/S0392-0488.17.05652-8
86. Pellegrini M, D’Eusebio C, Ponzo V, Tonella L, Finocchiaro C, Fierro MT, et al. Nutritional interventions for patients with melanoma: from prevention to therapy-an update. Nutrients (2021) 13(11):4018. doi: 10.3390/nu13114018
Keywords: serum 25-hydroxyvitamin D, cutaneous melanoma, Mendelian randomization, genetic variants, causal inference, sun exposure
Citation: Cai B, Lin Q, Ke R, Shan X, Yu J, Ni X, Lin X and Wang B (2023) Causal association between serum 25-Hydroxyvitamin D levels and cutaneous melanoma: a two-sample Mendelian randomization study. Front. Oncol. 13:1154107. doi: 10.3389/fonc.2023.1154107
Received: 30 January 2023; Accepted: 03 August 2023;
Published: 17 August 2023.
Edited by:
Rosario Caltabiano, University of Catania, ItalyReviewed by:
Lukasz Laczmanski, Polish Academy of Sciences, PolandCopyright © 2023 Cai, Lin, Ke, Shan, Yu, Ni, Lin and Wang. This is an open-access article distributed under the terms of the Creative Commons Attribution License (CC BY). The use, distribution or reproduction in other forums is permitted, provided the original author(s) and the copyright owner(s) are credited and that the original publication in this journal is cited, in accordance with accepted academic practice. No use, distribution or reproduction is permitted which does not comply with these terms.
*Correspondence: Xinjian Lin, eGxpbkBmam11LmVkdS5jbg==; Biao Wang, Ymlhb3dhbmdAZmptdS5lZHUuY24=
†These authors have contributed equally to this work
Disclaimer: All claims expressed in this article are solely those of the authors and do not necessarily represent those of their affiliated organizations, or those of the publisher, the editors and the reviewers. Any product that may be evaluated in this article or claim that may be made by its manufacturer is not guaranteed or endorsed by the publisher.
Research integrity at Frontiers
Learn more about the work of our research integrity team to safeguard the quality of each article we publish.