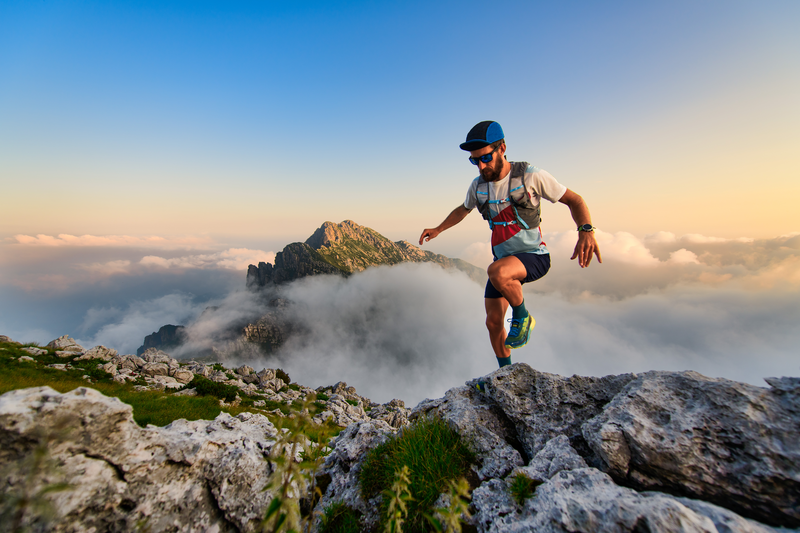
94% of researchers rate our articles as excellent or good
Learn more about the work of our research integrity team to safeguard the quality of each article we publish.
Find out more
REVIEW article
Front. Oncol. , 29 March 2023
Sec. Radiation Oncology
Volume 13 - 2023 | https://doi.org/10.3389/fonc.2023.1133909
While proton radiation therapy offers substantially better dose distribution characteristics than photon radiation therapy in certain clinical applications, data demonstrating a quantifiable clinical advantage is still needed for many treatment sites. Unfortunately, the number of patients treated with proton radiation therapy is still comparatively small, in some part due to the lack of evidence of clear benefits over lower-cost photon-based treatments. This review is designed to present the comparative clinical outcomes between proton and photon therapies, and to provide an overview of the current state of knowledge regarding the effectiveness of proton radiation therapy.
Radiation therapy is a primary method of cancer treatment, is used to treat approximately 50% of all cancer patients (1), and is a component of the treatment of 29% of cancer survivors in the United States (2). Advancements in photon radiation therapy techniques have steadily improved dose conformity around tumors, however, the high dose in adjacent normal tissues still limits dose escalation or even the delivery of necessary curative doses for certain types of cancers. Proton radiation therapy offers the potential for substantial improvements in the dose distribution in tumor and normal tissues, and may provide a clinical benefit for certain types of tumors, especially pediatric tumors and tumors located in anatomically challenging areas (3, 4).
As of October 2022, worldwide there were 118 proton radiation therapy centers in operation (42 in the United States) (5); 34 under construction (6); and 32 in the planning stage (7). However, as of December 2021 only an estimated 279,455 patients have been treated with proton radiation therapy worldwide (8). For comparison, IMV Medical Information Division estimates there were a total of 1.06 million radiation therapy patients in 2020 in the United States alone (9). A major hurdle for the use of proton radiation therapy is high treatment cost and lack of evidence of increased efficacy of proton radiation therapy over lower-cost photon-based treatments (10, 11). Therefore, randomized controlled comparative effectiveness trials are needed to qualify and quantify the potential superiority of proton radiation therapy in a given clinical scenario.
The aim of this review is to detail the comparative clinical outcomes between proton and photon therapies from existing and ongoing comparative clinical research literature and clinical trial protocols, and to provide an overview of the effectiveness of proton radiation therapy.
The resources used in the compilation of research articles for this review included PubMed, ScienceDirect, Scopus, and Google Scholar databases. The Keywords or MeSH terms included “proton therapy,” “proton radiation therapy,” “proton beam therapy,” and “charged particle radiation therapy.” Filter criteria were as follows:
Inclusion criteria:
1. Comparative articles between external beam photon and proton radiation therapy (additionally, electron beam as part of a conventional photon-electron regimen)
2. Clinical research focused on radiation treatment-related clinical outcomes, which contained results for toxic effects, quality of life, local control, local recurrence, local failure, secondary malignancies, and survival rates
3. Published or accepted by 2021
4. In English
Exclusion criteria:
a. Abstract only
b. Treatment planning or dosimetric comparison articles
c. Estimated or calculated clinical outcomes
d. Cancer-related clinical outcomes
e. Patient cohort less than five
In total, 63 interventional comparative articles meeting these criteria returned and were incorporated into this review, including 6 randomized studies (1 prostate, 3 lung, 1 esophagus, 1 adult central nervous system cancers) and 57 non-randomized reports (8 prostate, 3 breast, 9 lung, 8 esophagus, 7 head and neck, as well as 4 adult and 18 pediatric central nervous system cancers).
The clinical trial data for this review was collected from the U.S. National Library of Medicine ClinicalTrials.gov. Using headings including the same terms previously listed and selecting “Interventional Studies (Clinical Trials)” for study type, 196 clinical trials were identified. Among these, 36 comparative clinical trials aimed at evaluating clinical outcomes between external beam photon and proton radiation therapy were identified: 28 protocols recruiting, 2 completed, 3 not yet recruiting, 1 withdrawn, 1 active but not recruiting, 1 terminated.
In the following sections, comparative clinical outcomes between proton and photon therapies will be summarized. Additional information for selected non-randomized studies is shown in Tables 1–7, randomized studies in Table 8, and clinical trials in Table 9.
Table 1 Non-randomized clinical studies of proton versus photon radiation therapy – prostate cancer.
Prostate cancer is the most common cancer diagnosed in men, with about 248,530 new cases and 34,130 deaths in the United States in 2021 (75). Since prostate cancer patients have a high long-term survival rate, minimizing treatment-related toxicities [gastrointestinal (GI) and/or genitourinary (GU)] and preserving quality of life (QoL) is a major goal of treatment.
A single institutional Medicare database propensity-matched study showed intensity-modulated radiation therapy (IMRT)-treated nonmetastatic prostate cancer patients had a lower incidence of GI toxicity than proton radiation therapy (12). The incidence of GU toxicity did not differ significantly between cohorts. However, Yu et al. (13) performed a multi-institutional study based on a national Medicare database and indicated there was a statistically significant reduction of GU morbidity rate at 6 months post-treatment in proton radiation therapy compared to IMRT and no difference at 12 months for early-stage prostate cancer patients after adjusting for potential confounders. Also, no statistically significant difference in GI morbidity was observed between groups. A claim-based propensity-matched study also indicated that proton radiation therapy was associated with lower incidences of urinary morbidity and erectile dysfunction, but a higher incidence of bowel morbidity at 2 years post-treatment, as compared to IMRT, among younger prostate cancer patients (< 65 years old) with private insurance (14). Since the Medicare database and medical claims could cause misclassification bias due to lack of detailed clinical information, Hoppe et al. (15) evaluated the patient-reported QoL between passive scattering proton therapy (PSPT) and IMRT for localized prostate cancer patients from nine University of Florida affiliated hospitals and found no differences in expanded prostate cancer index composite (EPIC)-26 summary scores for bowel, urinary, and sexual function domains between groups at 6 months to 2 years follow up after adjusting for potential confounders. A case-matched provider-reported-outcome study also showed no statistically significant differences between PSPT and IMRT for localized prostate cancer patients in acute or late grade ≥ 2 GI or GU toxicity rate within 5 years follow up, although planned doses to the bladder and rectum were significantly reduced in the PSPT group (16) (Table 1).
On the other hand, Bai et al. (17) assessed the patient-reported bowel and urinary toxicities between intensity-modulated proton therapy (IMPT) and IMRT at an early stage of post-treatment (immediately following and at 3 months post-treatment) for stage T1-2N0M0 prostate cancer patients in a single institution. Without adjusting for potential confounders, the IMPT group had a statistically smaller decline in EPIC-26 score for the bowel function domain than the IMRT group at both follow-up points and no difference for the urinary function domain. Without adjusting for confounders, Khmelevsky et al. (18) also presented that photon radiation therapy with a proton boost was associated with a statistically significantly lower incidence of acute and late grade 2 GI toxicity compared to photons only for patients with stage T1-3N0-1M0 prostate cancer. There were no statistically significant differences for acute and late grade 3-4 GI toxicity, acute and late GU toxicity, 5- and 10-year recurrence-free survival and OS between cohorts. Nevertheless, a propensity-matched study from the National Cancer Database reported proton-based treatment achieved higher 10-year OS than photon-based treatment (3D-conformal radiation therapy (3D-CRT)/IMRT) for stage T1-3N0M0 prostate cancer patients (19) (Table 1).
To date, there is only one randomized phase III study. This study, published in 1995, indicated that stage T3-4Nx,0-2M0 prostate cancer patients treated with high dose proton boost therapy experienced a significantly higher late treatment-induced rectal bleeding rate, but a lower local tumor persistence/palpable and/or symptomatic regrowth rate, as compared to the patients treated with conventional dose photon boost therapy (Table 8) (69). There were no statistically significant differences in acute grade 3-5 toxicity, late urinary toxicities, late sexual function, 8-year disease-specific survival, total recurrence-free survival and OS between groups. However, the patients with poorly differentiated tumors in the proton boost group experienced a significantly increased local control rate. Currently, three randomized clinical trials (NCT04190446: phase II, NCT01617161: phase III, NCT04083937: phase III) comparing treatment-related toxicities and QoL between IMRT and proton radiation therapy in prostate cancer are recruiting (Table 9).
Breast cancer is the most commonly diagnosed cancer in women with an estimated 281,550 new cases and 43,600 deaths in the United States in 2021 (75). Radiation therapy complications include short-term (mainly skin toxicity) and long-term (such as ischemic heart disease, chronic radiation pneumonitis, nerve damage, etc.), and can negatively affect patient QoL.
Without adjusting for potential confounders, a multi-institutional prospective study indicated that PSPT resulted in a higher incidence of long-term (7-year) skin toxicities (telangiectasia, pigmentation change and other late skin toxicities) compared to 3D-CRT for stage I breast cancer patients (20). The 7-year local failure rate did not differ significantly between cohorts. A multivariable analysis based on the National Cancer Database revealed no statistically significant difference in 5-year OS between proton and photon therapies for stage 0-III breast cancer patients (21). On the other hand, a single institutional propensity-matched retrospective study showed pencil beam scanning proton therapy (PBSPT) treated patients had a higher incidence of acute grade ≥ 2 radiation dermatitis than photon radiation therapy treated patients with primary or recurrent stage IA-IIIC breast cancer, even though no statistically significant difference in skin dose was observed between groups (22). However, there were no statistically significant differences in acute grade ≥ 3 radiation dermatitis and acute grade ≥ 2 skin hyperpigmentation (Table 2).
As discussed above, the published comparative clinical studies between proton and photon therapies for breast cancer are very limited and no comparative randomized clinical study has been published. Except skin toxicities, there were no published comparative studies investigating complications in other organs, such as lung and heart, where superior dose sparing in proton radiation therapy has been shown in numerous other studies. Three recruiting randomized clinical trials (NCT04443413: phase II, NCT04291378: phase III, NCT02603341) will primarily compare treatment-related heart disease and complication rates between proton and photon therapies in breast cancer (Table 9).
Lung cancer, most commonly non-small cell lung cancer (NSCLC), continues to be the leading cause of cancer death for both men and women in the United States in 2021 (75). Thoracic radiation therapy can have unwanted side effects affecting nearby functional lung, heart, and esophagus, which can adversely affect QoL and survival. Therefore, the incidence of treatment-related pulmonary, cardiac, and esophageal complications must be considered when choosing the optimal treatment plan.
Two studies retrospectively analyzed the radiation-induced toxicities among patients enrolled in a randomized clinical trial (23, 24). Comparing PSPT and IMRT cohorts with stage II-IV NSCLC, these studies indicated the incidence of radiation-induced pericardial effusion and esophageal toxicity (based on either esophagitis grade distribution or esophageal expansion imaging biomarker values) did not differ significantly between cohorts. Remick et al. (25) also reported that no statistically significant differences in acute esophagitis incidence, 2-year OS, or local recurrence-free survival were achieved between double scattering proton therapy (DSPT)/IMPT and IMRT in stage I-IV NSCLC patients. However, without adjusting for potential confounders, Sejpal et al. (26) showed proton radiation therapy achieved lower incidence of acute grade ≥ 3 esophagitis and pneumonitis than 3D-CRT and IMRT for stage IB-IV and recurrent NSCLC patients, but no statistically significant difference for OS was found between cohorts. A recently published study also indicated PSPT/IMPT treated patients experienced a lower risk of acute grade ≥ 2 esophagitis and a trend of reducing the risk of acute grade ≥ 2 cardiac toxicity and acute grade ≥ 2 pneumonitis compared to IMRT treated patients with stage II-IV NSCLC using multivariate analysis (27). There were no statistically significant differences in 1-, 2-, and 5- year OS, progression-free survival, disease-specific survival, or local control between groups (Table 3).
Comparing uniform scanning proton therapy (USPT)/PBSPT with IMRT, a single institutional study reported no statistically significant differences in grade ≥ 2 pneumonitis and esophagitis rates, acute dermatitis, OS, progression-free survival, or locoregional control for stage III lung cancer patients without adjusting for potential confounders (28). Yu et al. (29) also showed there were no statistically significant differences in the subacute (3 months post-treatment) grade 3 pneumonitis, esophagitis and dyspnea rates, 1-year OS, freedom from distant metastasis rate, or freedom from locoregional recurrence rate between IMPT and IMRT treated stage I-IV NSCLC patients using multivariable analysis. For early stage (I-II) NSCLC patients with underlying idiopathic pulmonary fibrosis (IPF), however, a multivariate analysis indicated there was a trend toward increased OS in the proton radiation therapy cohort (stereotactic body proton therapy (SBPT)/IMPT, 8 patients) compared to the photon cohort (stereotactic body radiation therapy (SBRT)/3D-CRT/IMRT, 22 patients) for 6-month and 1-year OS (30). Severe treatment-related pulmonary toxicity rates did not differ significantly between groups, possibly due to the small sample size of the study. However, 18.2% of patients in the photon radiation therapy group died of treatment-related pulmonary complications, but there were no treatment-related fatalities in the proton radiation therapy group. Another propensity-matched study from this institution reported that PBSPT treated patients had a lower risk of grade 4 radiation-induced lymphopenia than IMRT treated patients with locally advanced NSCLC (31) (Table 3).
A completed randomized phase II clinical trial (NCT00915005) confirmed that there was no statistically significant difference in radiation-induced pneumonitis between PSPT and IMRT for stage II-IV NSCLC patients (70, 71). In addition, another randomized phase II clinical trial (NCT01511081) showed that the SBPT group (10 patients) achieved better 3-year OS, progression-free survival and local control rate as compared to the SBRT group (9 patients) with early-stage (stage I or recurrent) NSCLC (72). However, this trial was terminated due to poor accrual numbers (Table 8). Three other randomized clinical trials (NCT02731001, NCT01993810: phase III, NCT01629498: phase I/II) comparing the toxicities and survival rates between photon and proton therapies in NSCLC are currently recruiting (Table 9).
A single institutional retrospective study of stage I-IVA esophageal cancer treated using PSPT, 3D-CRT and IMRT showed a reduction in the incidence of postoperative pulmonary and GI complications between PSPT and 3D-CRT, but no statistically significant difference was found between PSPT and IMRT (32). A multi-institutional study also reported that PSPT treated patients had a lower rate of pulmonary and cardiac complications than 3D-CRT treated patients with stage I-IV esophageal cancer, whereas no statistically significant difference was found between PSPT and IMRT treated patients (33). Xi et al. (34) also showed that no significant grade ≥ 3 toxicity (mainly pulmonary, GI and cardiac complications) differences existed between PSPT/IMPT and IMRT for stage I-III esophageal cancer patients. However, the distant recurrence risk was significantly reduced, and the 5-year OS, progression-free survival and distant metastasis free survival were significantly improved in the proton group, especially in stage III esophageal cancer patients, using multivariate analysis. In contrast, without adjusting for potential confounders, Suh et al. (35) showed that no statistically significant differences in the 5-year progression-free survival, 5-year OS and the incidence of esophagitis, pneumonitis and pleural and pericardial effusion between PSPT/USPT/PBSPT and 3D-CRT/IMRT groups were seen in T1-3N0M0 thoracic esophageal cancer patients (Table 4).
Table 4 Non-randomized clinical studies of proton versus photon radiation therapy - esophageal cancer.
Comparing IMPT with IMRT, a single institutional study revealed that there were no statistically significant differences in acute grade 3 toxicity (mainly GI complication) and 1-year OS between groups for locally advanced esophageal cancer patients using multivariate analysis (36). Without adjusting for potential confounders, DeCesaris et al. (37) also reported that 18-month OS, locoregional control and distant metastatic control were similar between PBSPT and photon treated patients with stage IIB-IVA distal esophageal cancer. However, there were two propensity-matched studies showed that proton radiation therapy (PBSPT) was associated with a significantly lower incidence of grade 4 lymphopenia compared to 3D-CRT/IMRT for stage I-IV esophageal cancer patients (38, 39) (Table 4).
The only published randomized phase IIB clinical study (NCT01512589) reported that the significant dose sparing of lung, heart, liver and lymphocytes in a PSPT/IMPT group resulted in reduced total toxicity burden and postoperative complications scores compared to an IMRT group for stage I-III esophageal cancer patient, but the QoL, 3-year progression-free survival and OS were similar between groups (Table 8) (73). Two additional ongoing randomized phase III clinical trials (NCT05055648, NCT03801876) will attempt to clarify the superior safety and efficacy of proton radiation therapy for esophageal cancer in the next several years (Table 9).
For stage T1-4N0-3 nasopharynx cancer, a retrospective case-matched study showed that IMPT treated patients had a lower requirement for gastrostomy tube placement than IMRT treated patients, which is likely driven by the lower dose to the oral cavity from IMPT (40). Similarly, a multivariate analysis from McDonald et al. (41) reported that proton radiation therapy for stage T1-4N0-2 nasopharynx and paranasal sinus cancer patients resulted in a lower requirement for gastrostomy tube insertion and opioid pain medication at the end of radiation therapy and one month post-treatment, which may also be due to the significant mean dose reduction to oral cavity, esophagus, larynx, and parotid glands, as compared to IMRT. To compare the radiation-related toxicities and survival rates between IMRT only and IMRT with PBSPT boost, Alterio et al. (42) analyzed outcomes of stage T3-4N0-2 nasopharyngeal carcinoma patients and showed that patients treated with a PBSPT boost experienced significantly lower risk of acute grade 3 mucositis and acute grade 2 xerostomia. However, no statistical differences were found for late toxicities, local progression-free survival, progression-free survival and local control rate between groups (Table 5).
Table 5 Non-randomized clinical studies of proton versus photon radiation therapy – head and neck cancer.
For stage T1-4N0-3 oropharyngeal cancer, a retrospective patient-reported outcome study from a symptom inventory-head and neck (MDASI-HN) module survey at MD Anderson indicated that there was a statistical reduction of top 5 symptom scores (food taste, dry mouth, swallowing/chewing, fatigue and appetite) in the IMPT group compared to the IMRT group during the subacute phase (first 3 months post- treatment) without adjusting for potential confounders (43). However, the top 11 symptom scores (food taste, dry mouth, swallowing/chewing, fatigue, appetite, mucus, sleep, mouth sores, drowsiness and distress) did not differ significantly between groups during the acute phase (6- to 7-week period during treatment) and chronic phase (after 3 months post-treatment). Meanwhile, a case-matched study reported that IMPT was associated with a lower incidence of patient-reported grade ≥ 2 xerostomia at 3 months post-treatment and a lower risk of grade 3 weight loss or gastrostomy tube presence at one year post-treatment compared to IMRT for stage T1-4N0-3 oropharyngeal cancer patients (44). However, there were no significant differences in patient-reported grade ≥ 2 dermatitis or mucositis and fatigue, 3-year OS, progression-free survival, locoregional control rate and distant control rate between groups. Another patient-reported outcome study also indicated that PBSPT treated patients had a statistically significant lower xerostomia score and less head and neck pain than IMRT/volumetric modulated arc therapy (VMAT) treated patients with stage I-IVA oropharynx cancer at one year post-treatment, which is likely due to significant dose sparing of the oral cavity structure (45) (Table 5).
To compare radiation-induced toxicities between uniform scanning proton therapy (USPT) and IMRT for major salivary gland cancer or cutaneous squamous cell carcinoma, Romesser et al. (46) showed USPT was associated with a lower risk of acute grade ≥ 2 treatment-related toxicities (mucositis, dysgeusia, and nausea) compared to IMRT for patients who received ipsilateral head and neck radiation, which may be a result of the significant dose sparing of oral cavity and brainstem. However, 1-year actuarial locoregional control rate, actuarial distant metastasis-free survival, and actuarial OS did not differ significantly between cohorts (Table 5). To date, no comparative randomized clinical study for head and neck cancers has been published, but many randomized clinical trials (3 phase II, 1 phase II/III, 2 not specify) comparing treatment-induced toxicities between photon and proton therapies are currently recruiting (Table 9).
For craniospinal irradiation (CSI), Gunther et al. (47) reported that PSPT CSI treated patients experienced a lower risk of acute grade 1-3 mucositis than 3D-CRT-CSI treated patients with leukemia/lymphoma/myeloma with CNS involvement/elapse. No statistically significant differences in acute GI symptoms, CNS toxicity/relapse, infection, or 6-month survival rate were observed between cohorts. However, Brown et al. (48) demonstrated proton CSI was associated with a lower risk of acute GI and hematologic morbidities compared to photon CSI for stage M0-4 medulloblastoma patients. The reduced risk of acute GI morbidities in proton CSI patients, including weight, grade 2 nausea/vomiting, and esophagitis-related medical management, was most likely due to the significant dose sparing of the esophagus, stomach, and bowel. The reduced risk of acute hematologic morbidities (bone marrow suppression) in proton CSI patients, including less decline of peripheral white blood cells (WBC), hemoglobin, and platelets, was mainly driven by the significantly lower mean vertebral dose. Proton CSI patients also had a significantly lower incidence of grade ≥ 1 anemia than photon CSI patients, whereas the incidence of grade ≥ 1 leukopenia and thrombocytopenia did not differ significantly (Table 6).
Table 6 Non-randomized clinical studies of proton versus photon radiation therapy – adult CNS cancer.
For cranial irradiation, Song et al. (49) showed there were no statistically significant differences in grade ≥ 2 symptomatic brain injury, 2-year progression-free survival and OS between USPT/PBSPT and VMAT/tomotherapy for grade I-III meningioma. However, a retrospective study based on the National Cancer Database indicated that grade I-IV glioma patients treated with proton radiation therapy achieved superior 5-year OS compared to photon radiation therapy after propensity score weighting (50) (Table 6). Recently, a completed randomized phase II clinical trial (NCT01854554) for glioblastoma reported that patients treated with PSPT/IMPT had a statistically reduced rate of acute grade ≥ 3 lymphopenia compared to IMRT/VMAT, which is likely due to the reduced brain volume irradiated by low and intermediate doses (Table 8) (74).
As listed above, the comparative clinical studies between proton and photon therapies for adult CNS cancer are limited. Ongoing randomized clinical trials (NCT04752280, NCT03180502: phase II, NCT02179086: phase II, NCT04536649: phase III) may provide additional clinical evidence to clarify the effectiveness of proton radiation therapy (Table 9).
Due to the high radiosensitivity of developing tissues and the long life-expectancy of childhood cancer survivors, severe long-term side effects and radiation-induced secondary malignancies are major concerns when treating pediatric cancer patients with radiation. Therefore, sufficient avoidance of non-target tissues to mitigate treatment-related toxicities is crucial for treatment planning.
For cognitive development following CSI, Kahalley et al. (51) compared intelligence quotient (IQ) change over time between PSPT/IMPT and 3D-CRT/IMRT following treatment of pediatric brain cancer patients in a single institutional study. The study reported that there was no significant IQ decline over time from proton radiation therapy, while photon radiation therapy patients exhibited a significantly lower and steadily decreasing IQ score for both craniospinal and focal irradiation. Subgroup evaluation also indicated that photon CSI is associated with a reduced IQ of 12.5 points compared to proton CSI. The authors did not mention the IQ comparison between focal photon and proton therapies. The authors further evaluated different domains of intellectual outcomes from a multi-institution database and determined that patients treated with proton CSI had better intellectual outcomes in global IQ, perceptual reasoning, and working memory as compared to photon CSI (52). The verbal reasoning score did not differ between cohorts and patients in both cohorts experienced a significant reduction in processing speed score. However, Eaton et al. (53) recently indicated that PSPT CSI-treated patients had higher verbal reasoning score, mean full-scale IQ, and perceptual reasoning score as compared to 3D-CRT/IMRT CSI-treated patients with standard-risk pediatric medulloblastoma in a multi-institutional case-matched study. No statistically significant differences in processing speed and working memory were detected between groups. Gross et al. (54) also compared different intellectual parameters and reported pediatric brain cancer patients treated with PSPT/IMPT showed higher full-scale IQ and processing speed index than those treated with 3D-CRT/IMRT for both craniospinal and focal irradiation. The subgroup investigation indicated that pediatric patients treated with proton CSI achieved higher full-scale IQ and verbal IQ than those treated with photon CSI, and proton focal irradiation resulted in higher processing speed index than photon focal irradiation. However, a recently published long-term (average 7.2 years post-treatment) study compared cognitive and academic outcomes between craniospinal and focal proton and photon therapies for pediatric primary brain cancer patients and showed there were no statistically significant differences in full-scale IQ, verbal comprehension, perceptual reasoning, working memory and processing speed index between cohorts (55) (Table 7).
Table 7 Non-randomized clinical studies of proton versus photon radiation therapy – pediatric CNS cancer.
Table 9 Non-randomized and randomized clinical trials of proton versus photon radiation therapy (see www.clinicaltrials.gov).
For endocrine metabolism following CSI, Bielamowicz et al. (56) showed that there was no statistically significant difference in primary and central hypothyroidism incidence between PSPT CSI and 3D-CRT CSI with IMRT boost in standard and high risk pediatric medulloblastoma patients in a single institutional study. However, an extension of this study with longer follow up time (median 5.6 years post-treatment) indicated that PSPT CSI treated patients had a lower risk of primary hypothyroidism than the patients in 3D-CRT CSI with IMRT boost group (57). No statistically significant differences in the risk of central hypothyroidism, growth hormone deficiency and adrenal insufficiency were found between groups. A propensity-matched multi-institutional study also reported that PSPT CSI resulted in a lower hypothyroidism rate, sex hormone deficiency incidence, and endocrine replacement therapy requirement than 3D-CRT/IMRT CSI for standard risk pediatric medulloblastoma patients after median 5.8 years post-treatment (58). There were no statistically significant differences in the risk of growth hormone deficiency, adrenal insufficiency, and precocious puberty between cohorts (Table 7).
For radiation-induced hematologic toxicity following CSI, a recently published multi-institutional retrospective study showed that DSPT CSI was associated with reduced acute hematologic toxicity, including leukopenia, lymphopenia, and anemia, as compared to photon CSI for a multivariable analysis in pediatric medulloblastoma patients, whereas the 5-year OS did not differ between the cohorts (59). Without adjusting for potential confounders, Song et al. (60) found there were lower incidences of grade ≥ 3 thrombocytopenia, platelet transfusion, and diarrhea in the proton CSI group than the photon CSI group for pediatric brain tumors (mainly medulloblastoma) patients at the National Cancer Center. Proton CSI was also associated with less reduction of white blood cells and platelets than photon CSI at one month post-treatment. In a single institutional study, Yoo et al. (61) also found PBSPT CSI patients had a lower decline of lymphocyte and platelet counts and lower risk of acute grade 3 anemia compared to 3D-CRT/helical tomotherapy CSI patients with pediatric brain cancer. However, there were no statistically significant differences in hemoglobin level, grade 3 thrombocytopenia, grade 4 lymphopenia, platelet transfusion, diarrhea and 3-year OS between groups without adjusting for potential confounders. Paulino et al. (62) also reported no statistically significant differences in 5- and 10-year OS and secondary malignant neoplasms risk between PSPT CSI and 3D-CRT CSI with IMRT boost for pediatric medulloblastoma patients. A multi-institutional case-matched study also showed that there were no significant differences in 6-year OS, 6-year recurrence-free survival and the patterns of failure between proton CSI and 3D-CRT/IMRT CSI in pediatric standard risk medulloblastoma patients (63) (Table 7).
In addition, for pediatric medulloblastoma patients, studies indicated that there were no statistically significant differences in grade 3 and 4 ototoxicity between PSPT CSI and 3D-CRT CSI with IMRT boost based on multiple evaluation scales (64), and cavernous malformations (CM) or CM-like lesions between proton and photon radiotherapy (65) (Table 7).
For cranial irradiation, a multi-institutional study of pediatric craniopharyngioma reported no statistically significant differences in 3-year OS, 3-year nodular failure-free survival and 3-year cystic failure-free survival between IMRT and proton radiation therapy (86% PSPT) using multivariable analysis (66). Based on the same database, Sato et al. (67) confirmed there was no statistically significant difference in 3-year OS between proton radiation therapy and IMRT for grade II-III pediatric intracranial ependymomas. However, proton radiation therapy was associated with higher 3-year progression-free survival compared to IMRT without adjusting for potential confounders. Nevertheless, another multi-institutional parent proxy-reported quality of life study showed that proton treated patients received better health related QoL than photon treated patients with pediatric brain tumor without adjusting for potential confounders (68) (Table 7).
To date, no randomized comparative clinical trial has been performed due to ethical barriers, since it would be difficult to suggest that there is clinical equipoise given the relative superiority of the proton dose distributions in such cases.
According to the limited published comparative clinical studies mentioned above, the clinical benefit of proton radiation therapy is likely to vary between different radiation therapy techniques for different cancer sites, which makes it more difficult to demonstrate definitive advantages for proton radiation therapy.
Several esophageal cancer studies presented in this review have shown reduced incidence of radiation-induced toxicities (pulmonary, GI or cardiac toxicities) between PSPT and 3D-CRT, but no significant difference between PSPT and IMRT. This is likely because the highly conformal dose delivery capabilities in advanced photon therapy techniques (76, 77) may result in better clinical outcomes than 3D-CRT. Furthermore, there are no significant differences for radiation-induced GU/urinary toxicities in prostate cancer patients and for radiation-induced esophagitis and pneumonitis among NSCLC patients between proton therapy (mainly PSPT/DSPT) and IMRT. Therefore, proton therapy might not result in better clinical outcomes than intensity modulated photon treatment for certain cancer sites, for several potential reasons. First, the dose to organs at risk (OARs) can be maintained within tolerance doses using intensity modulated photon therapy for many of these sites. Therefore, the significant dose sparing capabilities of proton therapy may not translate into a remarkable clinical benefit. Second, a lack of significant clinical benefit in proton therapy may be due to the anatomic non-coincidence of the dose spared-regions and the regions that experienced radiation-induced toxicities. Palma et al. (71) presented that the significantly spared regions by PSPT as compared with IMRT are largely within the lower part of the lungs and the heart, whereas the radiation-induced pneumonitis affected regions are within the medial-anterior and upper parts of the thorax. Therefore, there was no statistically significant difference in radiation-induced pneumonitis between PSPT and IMRT for NSCLC patients. Cella et al. (23) also reported a similar occurrence in cardiac toxicity for NSCLC patients. The lack of superior effectiveness observed in the PSPT group may also be due to the lack of anatomic overlap between spared areas by PSPT as compared with IMRT and the areas that experienced radiation-induced pericardial effusion. Third, since proton beam delivery is very sensitive to tissue density changes, any anatomical variations or changes (e.g. patient weight, tumor changes, patient setup variation), and motions (e.g. respiration, cardiac activity, bladder filling) that occur in the beam path can have a much greater impact on the spatial dose distribution, resulting in substantially increased doses to OARs or reduced target dose coverage. To mitigate these anatomic changes and motions, development and application of robust optimization methods (78), tracking/gating techniques or breath hold methods (79, 80), in-room/real time image guidance (e.g. 4D-CT, cone-beam CT, MRI, optical surface monitoring system (OSMS)) (81) and in vivo range verification (e.g. positron emission tomography (PET), prompt gammas (PG) imaging) (82, 83) are currently being investigated. Fourth, uncertainty in the range of proton beams, due to uncertainties in CT data and the subsequent conversion to proton stopping power, results in substantial uncertainties in the delivered dose distribution. Dual-energy CT (DECT)-based SPR prediction (84) and proton CT (pCT) (85) may help overcome the limitation of CT Hounsfield unit-based SPR prediction (86) and thus potentially reduce the range uncertainty from 3.5% to 1.7% - 2.2% in DECT and 0.5% in pCT (87, 88). However, many aspects of these technologies are still in research and development. Last, the proton beam delivery techniques used in these studies are mainly PSPT or DSPT, which is a scattering process and generates a conformal dose at the distal side of the target volume, while the proximal side exhibits a much less conformal dose distribution. Modern proton techniques, such as PBSPT or IMPT, utilize a scanning rather than a scattering system to deliver uniform dose to a target volume in layers of proton “spots”, essentially “painting” the target volume (89). More comparative clinical studies between photon radiation therapy and these advanced proton techniques are needed to truly evaluate the effectiveness of proton radiation therapy in these cancer sites. Unfortunately, the clinical benefits of reducing the radiation-induced toxicities for breast cancer and adult CNS cancer are uncertain due to the limited amount of comparative clinical data.
Studies of head and neck cancers mainly focus on the comparison between PBSPT/IMPT and IMRT, and indicate that PBSPT/IMPT resulted in reduced gastrostomy tube usage and late radiation-induced xerostomia compared to IMRT. This benefit of proton therapy is likely due to 1) use of advanced proton spot scanning techniques and 2) the differences in beam delivery patterns and exit dose between proton and photon therapy, specifically that fewer proton beam entry paths and the lack of exit dose can potentially avoid many critical structures. These advantages of proton therapy also translate into a superior clinical benefit among pediatric CNS cancer patients. The studies showed that craniospinal proton radiation therapy (PSPT/IMPT) is most likely associated with reduced short-term (< 5 years post-treatment) effects on pediatric patient cognitive development, long-term (median 5.7 years post-treatment) hypothyroidism incidence and hematologic toxicities as compared to craniospinal photon radiation therapy (3D-CRT/IMRT). Interestingly, these comparative studies also indicated that proton radiation therapy (mainly PBSPT/IMPT) is most likely associated with a lower severe radiation-induced lymphopenia rate compared to IMRT in thoracic and craniospinal radiation therapy. T-lymphocytes play a central role in anticancer immune response and severe treatment-related lymphopenia is associated with poor survival rates in chemotherapy and/or radiotherapy (90, 91). Cho et al. (92) demonstrated that radiation treatment-related lymphopenia is also correlated with inferior survival rates for NSCLC patients treated with immunotherapy. The lymphocyte sparing achieved from advanced radiation techniques is certainly beneficial, however, whether it could enhance anticancer immune response and improve survival rates remains to be verified. While the studies in this review showed that the survival rates may not be significantly improved with proton radiation therapy for either adult or pediatric cancers, this is to be expected for studies in which the target dose is similar regardless of the delivery technique. Better dose shaping and reduction of uncertainties in dose calculation and delivery can reduce treatment margins, which can reduce normal tissue doses and potentially allow for dose escalation in the target. An ongoing clinical trial (NCT02179086) may provide valuable evidence of improved survival by comparing dose-escalated proton therapy vs standard-dose photon therapy.
Such studies, however, have been met with challenges. Due to issues surrounding equipoise, treatment costs, insurance coverage, and the relatively small number of charged-particle radiation therapy centers in operation, it can be difficult to activate and complete a randomized controlled clinical study in a timely manner. Therefore, most of the currently available data are non-randomized retrospective studies, which contain inevitable misclassification and selection biases despite adjustment for potential confounders by multivariable regression analysis and propensity/case-matched analysis. Moreover, the studies based on multi-institutional or medical databases potentially involve heterogeneity in treatment protocols and techniques. Some of the studies even use both older and modern delivery techniques, such as PSPT/DSPT and PBSPT/IMPT techniques for proton radiation therapy, and 3D-CRT and IMRT techniques for photon radiation therapy, without presenting the number of patients treated with each technique. Some studies did not indicate the type of proton or photon techniques they used. This could cause an imprecise comparison between proton and photon therapies since PBSPT/IMPT can offer better healthy tissue sparing and may result in lower toxicities than PSPT/DSPT (93). Finally, small sample size, limited follow-up duration and preconceived bias from patient-reported outcomes could also increase the uncertainty of study conclusions.
This review has presented currently available comparative clinical outcomes between proton and photon therapies for several cancer types. Overall, passive scattering proton therapy shows similar clinical outcomes to intensity modulated photon therapy for prostate, lung and esophageal cancers, while active scanning proton therapy appears to result in a decrease in certain radiation-induced side effects as compared to intensity modulated photon therapy for head and neck, thoracic, craniospinal, and pediatric CNS cancers. However, the evidence is not definitive and further demonstration of the clinical benefit of proton radiation therapy will depend on the findings of ongoing and future comparative randomized clinical trials. In the meantime, further development of beam delivery and imaging techniques is necessary to fully take advantage of the dose shaping capabilities of proton radiation therapy and achieve its full clinical potential.
ZC and JB contributed to the scope and design of the review. ZC performed the resources search and original draft. ZC, JB, MD, MJ revised and edited the draft. All authors contributed to the manuscript and approved the submitted version.
The authors would like to acknowledge the review and comments provided by Dr. Jiajian Shen, Department of Radiation Oncology, Mayo Clinic, Phoenix, AZ, United States.
The authors declare that the research was conducted in the absence of any commercial or financial relationships that could be construed as a potential conflict of interest.
All claims expressed in this article are solely those of the authors and do not necessarily represent those of their affiliated organizations, or those of the publisher, the editors and the reviewers. Any product that may be evaluated in this article, or claim that may be made by its manufacturer, is not guaranteed or endorsed by the publisher.
1. Delaney G, Jacob S, Featherstone C, Barton M. The role of radiotherapy in cancer treatment: Estimating optimal utilization from a review of evidence-based clinical guidelines. Cancer (2005) 104(6):1129–37. doi: 10.1002/cncr.21324
2. Bryant AK, Banegas MP, Martinez ME, Mell LK, Murphy JD. Trends in radiation therapy among cancer survivors in the united states, 2000-2030. Cancer Epidemiol Biomarkers Prev (2017) 26(6):963–70. doi: 10.1158/1055-9965
3. Kandula S, Zhu X, Garden AS, Gillin M, Rosenthal DI, Ang KK, et al. Spot-scanning beam proton therapy vs intensity-modulated radiation therapy for ipsilateral head and neck malignancies: A treatment planning comparison. Med Dosim (2013) 38(4):390–4. doi: 10.1016/j.meddos.2013.05.001
4. Sakthivel V, Ganesh KM, McKenzie C, Boopathy R, Selvaraj J. Second malignant neoplasm risk after craniospinal irradiation in X-ray-based techniques compared to proton therapy. Australas Phys Eng Sci Med (2019) 42(1):201–9. doi: 10.1007/s13246-019-00731-y
5. Particle Therapy Co-Operative Group (PTCOG). Particle therapy facilities in operation (2022). Available at: https://www.ptcog.ch/index.php/facilities-in-operation (Accessed December 12, 2022).
6. Particle Therapy Co-Operative Group (PTCOG). Particle therapy facilities under construction (2022). Available at: https://www.ptcog.ch/index.php/facilities-under-construction (Accessed December 12, 2022).
7. Particle Therapy Co-Operative Group (PTCOG). Particle therapy facilities in planning stage (2022). Available at: https://www.ptcog.ch/index.php/facilities-in-planning-stage (Accessed December 12, 2022).
8. Particle Therapy Co-Operative Group (PTCOG). Patient statistics (2022). Available at: https://www.ptcog.ch/images/patientstatistics/Patientstatistics-updateDec2021_Sep62022_provisional.pdf (Accessed December 12, 2022).
9. Young L. (2020). Available at: https://imvinfo.com/although-2020-capital-budgets-for-radiation-therapy-have-been-impacted-investments-are-being-shifted-to-2021-2022/.
10. Verma V, Mishra MV, Mehta MP. A systematic review of the cost and cost-effectiveness studies of proton radiotherapy. Cancer (2016) 122(10):1483–501. doi: 10.1002/cncr.29882
11. Li G, Qiu B, Huang YX, Doyen J, Bondiau PY, Benezery K, et al. Cost-effectiveness analysis of proton beam therapy for treatment decision making in paranasal sinus and nasal cavity cancers in China. BMC Cancer (2020) 20(1):599. doi: 10.1186/s12885-020-07083-x
12. Sheets NC, Goldin GH, Meyer AM, Wu Y, Chang Y, Stürmer T, et al. Intensity-modulated radiation therapy, proton therapy, or conformal radiation therapy and morbidity and disease control in localized prostate cancer. JAMA (2012) 307(15):1611–20. doi: 10.1001/jama.2012.460
13. Yu JB, Soulos PR, Herrin J, Cramer LD, Potosky AL, Roberts KB, et al. Proton versus intensity-modulated radiotherapy for prostate cancer: patterns of care and early toxicity. J Natl Cancer Inst (2013) 105(1):25–32. doi: 10.1093/jnci/djs463
14. Pan HY, Jiang J, Hoffman KE, Tang C, Choi SL, Nguyen QN, et al. Comparative toxicities and cost of intensity-modulated radiotherapy, proton radiation, and stereotactic body radiotherapy among younger men with prostate cancer. J Clin Oncol (2018) 36(18):1823–30. doi: 10.1200/JCO.2017.75.5371
15. Hoppe BS, Michalski JM, Mendenhall NP, Morris CG, Henderson RH, Nichols RC, et al. Comparative effectiveness study of patient-reported outcomes after proton therapy or intensity-modulated radiotherapy for prostate cancer. Cancer (2014) 120(7):1076–82. doi: 10.1002/cncr.28536
16. Fang P, Mick R, Deville C, Both S, Bekelman JE, Christodouleas JP, et al. A case-matched study of toxicity outcomes after proton therapy and intensity-modulated radiation therapy for prostate cancer. Cancer (2015) 121(7):1118–27. doi: 10.1002/cncr.29148
17. Bai M, Gergelis KR, Sir M, Whitaker TJ, Routman DM, Stish BJ, et al. Comparing bowel and urinary domains of patient-reported quality of life at the end of and 3 months post radiotherapy between intensity-modulated radiotherapy and proton beam therapy for clinically localized prostate cancer. Cancer Med (2020) 9(21):7925–34. doi: 10.1002/cam4.3414
18. Khmelevsky EV, Kancheli IN, Khoroshkov VS, Kaprin AD. Morbidity dynamics in proton-photon or photon radiation therapy for locally advanced prostate cancer. Rep Pract Oncol Radiother (2018) 23(1):21–7. doi: 10.1016/j.rpor.2017.11.001
19. Liu Y, Patel SA, Jani AB, Gillespie TW, Patel PR, Godette KD, et al. Overall survival after treatment of localized prostate cancer with proton beam therapy, external-beam photon therapy, or brachytherapy. Clin Genitourin Cancer (2021) 19(3):255–66.e7. doi: 10.1016/j.clgc.2020.08.009
20. Galland-Girodet S, Pashtan I, MacDonald SM, Ancukiewicz M, Hirsch AE, Kachnic LA, et al. Long-term cosmetic outcomes and toxicities of proton beam therapy compared with photon-based 3-dimensional conformal accelerated partial-breast irradiation: A phase 1 trial. Int J Radiat Oncol Biol Phys (2014) 90(3):493–500. doi: 10.1016/j.ijrobp.2014.04.008
21. Chowdhary M, Lee A, Gao S, Wang D, Barry PN, Diaz R, et al. Is proton therapy a "Pro" for breast cancer? a comparison of proton vs. non-proton radiotherapy using the national cancer database. Front Oncol (2019) 8:678. doi: 10.3389/fonc.2018.00678
22. DeCesaris CM, Rice SR, Bentzen SM, Jatczak J, Mishra MV, Nichols EM. Quantification of acute skin toxicities in patients with breast cancer undergoing adjuvant proton versus photon radiation therapy: A single institutional experience. Int J Radiat Oncol Biol Phys (2019) 104(5):1084–90. doi: 10.1016/j.ijrobp.2019.04.015
23. Cella L, Monti S, Xu T, Liuzzi R, Stanzione A, Durante M, et al. Probing thoracic dose patterns associated to pericardial effusion and mortality in patients treated with photons and protons for locally advanced non-small-cell lung cancer. Radiother Oncol (2021) 160:148–58. doi: 10.1016/j.radonc.2021.04.025
24. Niedzielski JS, Yang J, Mohan R, Titt U, Mirkovic D, Stingo F, et al. Differences in normal tissue response in the esophagus between proton and photon radiation therapy for non-small cell lung cancer using in vivo imaging biomarkers. Int J Radiat Oncol Biol Phys (2017) 99(4):1013–20. doi: 10.1016/j.ijrobp.2017.07.005
25. Remick JS, Schonewolf C, Gabriel P, Doucette A, Levin WP, Kucharczuk JC, et al. First clinical report of proton beam therapy for postoperative radiotherapy for non-Small-Cell lung cancer. Clin Lung Cancer (2017) 18(4):364–71. doi: 10.1016/j.cllc.2016.12.009
26. Sejpal S, Komaki R, Tsao A, Chang JY, Liao Z, Wei X, et al. Early findings on toxicity of proton beam therapy with concurrent chemotherapy for nonsmall cell lung cancer. Cancer (2011) 117(13):3004–13. doi: 10.1002/cncr.25848
27. Boyce-Fappiano D, Nguyen QN, Chapman BV, Allen PK, Gjyshi O, Pezzi TA, et al. Single institution experience of proton and photon-based postoperative radiation therapy for non-small-cell lung cancer. Clin Lung Cancer (2021) 22(5):e745–55. doi: 10.1016/j.cllc.2021.02.002
28. Zou Z, Bowen SR, Thomas HMT, Sasidharan BK, Rengan R, Zeng J. Scanning beam proton therapy versus photon IMRT for stage III lung cancer: Comparison of dosimetry, toxicity, and outcomes. Adv Radiat Oncol (2020) 5(3):434–43. doi: 10.1016/j.adro.2020.03.001
29. Yu NY, DeWees TA, Liu C, Daniels TB, Ashman JB, Beamer SE, et al. Early outcomes of patients with locally advanced non-small cell lung cancer treated with intensity-modulated proton therapy versus intensity-modulated radiation therapy: The Mayo clinic experience. Adv Radiat Oncol (2020) 5(3):450–8. doi: 10.1016/j.adro.2019.08.001
30. Kim H, Pyo H, Noh JM, Lee W, Park B, Park HY, et al. Preliminary result of definitive radiotherapy in patients with non-small cell lung cancer who have underlying idiopathic pulmonary fibrosis: Comparison between X-ray and proton therapy. Radiat Oncol (2019) 14(1):19. doi: 10.1186/s13014-019-1221-4
31. Kim N, Myoung Noh J, Lee W, Park B, Park H, Young Park J, et al. Proton beam therapy reduces the risk of severe radiation-induced lymphopenia during chemoradiotherapy for locally advanced non-small cell lung cancer: A comparative analysis of proton versus photon therapy. Radiother Oncol (2021) 156:166–73. doi: 10.1016/j.radonc.2020.12.019
32. Wang J, Wei C, Tucker SL, Myles B, Palmer M, Hofstetter WL, et al. Predictors of postoperative complications after trimodality therapy for esophageal cancer. Int J Radiat Oncol Biol Phys (2013) 86(5):885–91. doi: 10.1016/j.ijrobp.2013.04.006
33. Lin SH, Merrell KW, Shen J, Verma V, Correa AM, Wang L, et al. Multi-institutional analysis of radiation modality use and postoperative outcomes of neoadjuvant chemoradiation for esophageal cancer. Radiother Oncol (2017) 123(3):376–81. doi: 10.1016/j.radonc.2017.04.013
34. Xi M, Xu C, Liao Z, Chang JY, Gomez DR, Jeter M, et al. Comparative outcomes after definitive chemoradiotherapy using proton beam therapy versus intensity modulated radiation therapy for esophageal cancer: A retrospective, single-institutional analysis. Int J Radiat Oncol Biol Phys (2017) 99(3):667–76. doi: 10.1016/j.ijrobp.2017.06.2450
35. Suh YG, Bayasgalan U, Kim HT, Lee JM, Kim MS, Lee Y, et al. Photon Versus proton beam therapy for T1-3 squamous cell carcinoma of the thoracic esophagus without lymph node metastasis. Front Oncol (2021) 11:699172. doi: 10.3389/fonc.2021.699172
36. Bhangoo RS, DeWees TA, Yu NY, Ding JX, Liu C, Golafshar MA, et al. Acute toxicities and short-term patient outcomes after intensity-modulated proton beam radiation therapy or intensity-modulated photon radiation therapy for esophageal carcinoma: A Mayo clinic experience. Adv Radiat Oncol (2020) 5(5):871–9. doi: 10.1016/j.adro.2020.04.026
37. DeCesaris CM, Berger M, Choi JI, Carr SR, Burrows WM, Regine WF, et al. Pathologic complete response (pCR) rates and outcomes after neoadjuvant chemoradiotherapy with proton or photon radiation for adenocarcinomas of the esophagus and gastroesophageal junction. J Gastrointest Oncol (2020) 11(4):663–73. doi: 10.21037/jgo-20-205
38. Shiraishi Y, Fang P, Xu C, Song J, Krishnan S, Koay EJ, et al. Severe lymphopenia during neoadjuvant chemoradiation for esophageal cancer: A propensity matched analysis of the relative risk of proton versus photon-based radiation therapy. Radiother Oncol (2018) 128(1):154–60. doi: 10.1016/j.radonc.2017.11.028
39. Routman DM, Garant A, Lester SC, Day CN, Harmsen WS, Sanheuza CT, et al. A comparison of grade 4 lymphopenia with proton versus photon radiation therapy for esophageal cancer. Adv Radiat Oncol (2019) 4(1):63–9. doi: 10.1016/j.adro.2018.09.004
40. Holliday EB, Garden AS, Rosenthal DI, Fuller D, Morrison WH, Gunn GB. Proton therapy reduces treatment-related toxicities for patients with nasopharyngeal cancer: A case-match control study of intensity-modulated proton therapy and intensity-modulated photon therapy. I (2015) 2(1):19–28. doi: 10.14338/IJPT-15-00011.1
41. McDonald MW, Liu Y, Moore MG, Johnstone PA. Acute toxicity in comprehensive head and neck radiation for nasopharynx and paranasal sinus cancers: Cohort comparison of 3D conformal proton therapy and intensity modulated radiation therapy. Radiat Oncol (2016) 11:32. doi: 10.1186/s13014-016-0600-3
42. Alterio D, D'Ippolito E, Vischioni B, Fossati P, Gandini S, Bonora M, et al. Mixed-beam approach in locally advanced nasopharyngeal carcinoma: IMRT followed by proton therapy boost versus IMRT-only. evaluation of toxicity and efficacy. Acta Oncol (2020) 59(5):541–8. doi: 10.1080/0284186X.2020.1730001
43. Sio TT, Lin HK, Shi Q, Gunn GB, Cleeland CS, Lee JJ, et al. Intensity modulated proton therapy versus intensity modulated photon radiation therapy for oropharyngeal cancer: First comparative results of patient-reported outcomes. Int J Radiat Oncol Biol Phys (2016) 95(4):1107–14. doi: 10.1016/j.ijrobp.2016.02.044
44. Blanchard P, Garden AS, Gunn GB, Rosenthal DI, Morrison WH, Hernandez M, et al. Intensity-modulated proton beam therapy (IMPT) versus intensity-modulated photon therapy (IMRT) for patients with oropharynx cancer - a case matched analysis. Radiother Oncol (2016) 120(1):48–55. doi: 10.1016/j.radonc.2016.05.022
45. Sharma S, Zhou O, Thompson R, Gabriel P, Chalian A, Rassekh C, et al. Quality of life of postoperative photon versus proton radiation therapy for oropharynx cancer. Int J Part Ther (2018) 5(2):11–7. doi: 10.14338/IJPT-18-00032.1
46. Romesser PB, Cahlon O, Scher E, Zhou Y, Berry SL, Rybkin A, et al. Proton beam radiation therapy results in significantly reduced toxicity compared with intensity-modulated radiation therapy for head and neck tumors that require ipsilateral radiation. Radiother Oncol (2016) 118(2):286–92. doi: 10.1016/j.radonc.2015.12.008
47. Gunther JR, Rahman AR, Dong W, Yehia ZA, Kebriaei P, Rondon G, et al. Craniospinal irradiation prior to stem cell transplant for hematologic malignancies with CNS involvement: Effectiveness and toxicity after photon or proton treatment. Pract Radiat Oncol (2017) 7(6):e401–8. doi: 10.1016/j.prro.2017.05.002
48. Brown AP, Barney CL, Grosshans DR, McAleer MF, de Groot JF, Puduvalli VK, et al. Proton beam craniospinal irradiation reduces acute toxicity for adults with medulloblastoma. Int J Radiat Oncol Biol Phys (2013) 86(2):277–84. doi: 10.1016/j.ijrobp.2013.01.014
49. Song J, Aljabab S, Abduljabbar L, Tseng YD, Rockhill JK, Fink JR, et al. Radiation-induced brain injury in patients with meningioma treated with proton or photon therapy. J Neurooncol (2021) 153(1):169–80. doi: 10.1007/s11060-021-03758-y
50. Jhaveri J, Cheng E, Tian S, Buchwald Z, Chowdhary M, Liu Y, et al. Proton vs. photon radiation therapy for primary gliomas: An analysis of the national cancer data base. Front Oncol (2018) 8:440. doi: 10.3389/fonc.2018.00440
51. Kahalley LS, Ris MD, Grosshans DR, Okcu MF, Paulino AC, Chintagumpala M, et al. Comparing intelligence quotient change after treatment with proton versus photon radiation therapy for pediatric brain tumors. J Clin Oncol (2016) 34(10):1043–9. doi: 10.1200/JCO.2015.62.1383
52. Kahalley LS, Peterson R, Ris MD, Janzen L, Okcu MF, Grosshans DR, et al. Superior intellectual outcomes after proton radiotherapy compared with photon radiotherapy for pediatric medulloblastoma. J Clin Oncol (2020) 38(5):454–61. doi: 10.1200/JCO.19.01706
53. Eaton BR, Fong GW, Ingerski LM, Pulsifer MB, Goyal S, Zhang C, et al. Intellectual functioning among case-matched cohorts of children treated with proton or photon radiation for standard-risk medulloblastoma. Cancer (2021) 127(20):3840–6. doi: 10.1002/cncr.33774
54. Gross JP, Powell S, Zelko F, Hartsell W, Goldman S, Fangusaro J, et al. Improved neuropsychological outcomes following proton therapy relative to X-ray therapy for pediatric brain tumor patients. Neuro Oncol (2019) 21(7):934–43. doi: 10.1093/neuonc/noz070
55. Child AE, Warren EA, Grosshans DR, Paulino AC, Okcu MF, Ris MD, et al. Long-term cognitive and academic outcomes among pediatric brain tumor survivors treated with proton versus photon radiotherapy. Pediatr Blood Cancer (2021) 68(9):e29125. doi: 10.1002/pbc.29125
56. Bielamowicz K, Okcu MF, Sonabend R, Paulino AC, Hilsenbeck SG, Dreyer Z, et al. Hypothyroidism after craniospinal irradiation with proton or photon therapy in patients with medulloblastoma. Pediatr Hematol Oncol (2018) 35(4):257–67. doi: 10.1080/08880018.2018.1471111
57. Aldrich KD, Horne VE, Bielamowicz K, Sonabend RY, Scheurer ME, Paulino AC, et al. Comparison of hypothyroidism, growth hormone deficiency, and adrenal insufficiency following proton and photon radiotherapy in children with medulloblastoma. J Neurooncol (2021) 155(1):93–100. doi: 10.1007/s11060-021-03847-y
58. Eaton BR, Esiashvili N, Kim S, Patterson B, Weyman EA, Thornton LT, et al. Endocrine outcomes with proton and photon radiotherapy for standard risk medulloblastoma. Neuro Oncol (2016) 18(6):881–7. doi: 10.1093/neuonc/nov302
59. Liu KX, Ioakeim-Ioannidou M, Susko MS, Rao AD, Yeap BY, Snijders AM, et al. A multi-institutional comparative analysis of proton and photon therapy-induced hematologic toxicity in patients with medulloblastoma. Int J Radiat Oncol Biol Phys (2021) 109(3):726–35. doi: 10.1016/j.ijrobp.2020.09.049
60. Song S, Park HJ, Yoon JH, Kim DW, Park J, Shin D, et al. Proton beam therapy reduces the incidence of acute haematological and gastrointestinal toxicities associated with craniospinal irradiation in pediatric brain tumors. Acta Oncol (2014) 53(9):1158–64. doi: 10.3109/0284186X.2014.887225
61. Yoo GS, Yu JI, Cho S, Han Y, Oh Y, Lim DH, et al. Chronological analysis of acute hematological outcomes after proton and photon beam craniospinal irradiation in pediatric brain tumors. Cancer Res Treat (2022) 54(3):907–16. doi: 10.4143/crt.2021.332
62. Paulino AC, Ludmir EB, Grosshans DR, Su JM, McGovern SL, Okcu MF, et al. Overall survival and secondary malignant neoplasms in children receiving passively scattered proton or photon craniospinal irradiation for medulloblastoma. Cancer (2021) 127(20):3865–71. doi: 10.1002/cncr.33783
63. Eaton BR, Esiashvili N, Kim S, Weyman EA, Thornton LT, Mazewski C, et al. Clinical outcomes among children with standard-risk medulloblastoma treated with proton and photon radiation therapy: A comparison of disease control and overall survival. Int J Radiat Oncol Biol Phys (2016) 94(1):133–8. doi: 10.1016/j.ijrobp.2015.09.014
64. Paulino AC, Mahajan A, Ye R, Grosshans DR, Fatih Okcu M, Su J, et al. Ototoxicity and cochlear sparing in children with medulloblastoma: Proton vs. photon radiotherapy. Radiother Oncol (2018) 128(1):128–32. doi: 10.1016/j.radonc.2018.01.002
65. Trybula SJ, Youngblood MW, Kemeny HR, Clark JR, Karras CL, Hartsell WF, et al. Radiation induced cavernomas in the treatment of pediatric medulloblastoma: Comparative study between proton and photon radiation therapy. Front Oncol (2021) 11:760691. doi: 10.3389/fonc.2021.760691
66. Bishop AJ, Greenfield B, Mahajan A, Paulino AC, Okcu MF, Allen PK, et al. Proton beam therapy versus conformal photon radiation therapy for childhood craniopharyngioma: Multi-institutional analysis of outcomes, cyst dynamics, and toxicity. Int J Radiat Oncol Biol Phys (2014) 90(2):354–61. doi: 10.1016/j.ijrobp.2014.05.051
67. Sato M, Gunther JR, Mahajan A, Jo E, Paulino AC, Adesina AM, et al. Progression-free survival of children with localized ependymoma treated with intensity-modulated radiation therapy or proton-beam radiation therapy. Cancer (2017) 123(13):2570–8. doi: 10.1002/cncr.30623
68. Yock TI, Bhat S, Szymonifka J, Yeap BY, Delahaye J, Donaldson SS, et al. Quality of life outcomes in proton and photon treated pediatric brain tumor survivors. Radiother Oncol (2014) 113(1):89–94. doi: 10.1016/j.radonc.2014.08.017
69. Shipley WU, Verhey LJ, Munzenrider JE, Suit HD, Urie MM, McManus PL, et al. Advanced prostate cancer: The results of a randomized comparative trial of high dose irradiation boosting with conformal protons compared with conventional dose irradiation using photons alone. Int J Radiat Oncol Biol Phys (1995) 32(1):3–12. doi: 10.1016/0360-3016(95)00063-5
70. Liao Z, Lee JJ, Komaki R, Gomez DR, O'Reilly MS, Fossella FV, et al. Bayesian Adaptive randomization trial of passive scattering proton therapy and intensity-modulated photon radiotherapy for locally advanced non-Small-Cell lung cancer. J Clin Oncol (2018) 36(18):1813–22. doi: 10.1200/JCO.2017.74.0720
71. Palma G, Monti S, Xu T, Scifoni E, Yang P, Hahn SM, et al. Spatial dose patterns associated with radiation pneumonitis in a randomized trial comparing intensity-modulated photon therapy with passive scattering proton therapy for locally advanced non-small cell lung cancer. Int J Radiat Oncol Biol Phys (2019) 104(5):1124–32. doi: 10.1016/j.ijrobp.2019.02.039
72. Nantavithya C, Gomez DR, Wei X, Komaki R, Liao Z, Lin SH, et al. Phase 2 study of stereotactic body radiation therapy and stereotactic body proton therapy for high-risk, medically inoperable, early-stage non-small cell lung cancer. Int J Radiat Oncol Biol Phys (2018) 101(3):558–63. doi: 10.1016/j.ijrobp.2018.02.022
73. Lin SH, Hobbs BP, Verma V, Tidwell RS, Smith GL, Lei X, et al. Randomized phase IIB trial of proton beam therapy versus intensity-modulated radiation therapy for locally advanced esophageal cancer. J Clin Oncol (2020) 38(14):1569–79. doi: 10.1200/JCO.19.02503
74. Mohan R, Liu AY, Brown PD, Mahajan A, Dinh J, Chung C, et al. Proton therapy reduces the likelihood of high-grade radiation-induced lymphopenia in glioblastoma patients: Phase II randomized study of protons vs photons. Neuro Oncol (2021) 23(2):284–94. doi: 10.1093/neuonc/noaa182
75. Siegel RL, Miller KD, Fuchs HE, Jemal A. Cancer statistics, 2021. CA Cancer J Clin (2021) 71(1):7–33. doi: 10.3322/caac.21654
76. Galvin JM, Ezzell G, Eisbrauch A, Yu C, Butler B, Xiao Y, et al. Implementing IMRT in clinical practice: A joint document of the American society for therapeutic radiology and oncology and the American association of physicists in medicine. Int J Radiat Oncol Biol Phys (2004) 58(5):1616–34. doi: 10.1016/j.ijrobp.2003.12.008
77. Otto K. Volumetric modulated arc therapy: IMRT in a single gantry arc. Med Phys (2008) 35(1):310–7. doi: 10.1118/1.2818738
78. Badiu V, Souris K, Buti G, Villarroel EB, Lambrecht M, Sterpin E. Improved healthy tissue sparing in proton therapy of lung tumors using statistically sound robust optimization and evaluation. Phys Med (2022) 96:62–9. doi: 10.1016/j.ejmp.2022.02.018
79. Keall PJ, Mageras GS, Balter JM, Emery RS, Forster KM, Jiang SB, et al. The management of respiratory motion in radiation oncology report of AAPM task group 76. Med Phys (2006) 33(10):3874–900. doi: 10.1118/1.2349696
80. Emert F, Missimer J, Eichenberger PA, Walser M, Gmür C, Lomax AJ, et al. Enhanced deep-inspiration breath hold superior to high-frequency percussive ventilation for respiratory motion mitigation: A physiology-driven, MRI-guided assessment toward optimized lung cancer treatment with proton therapy. Front Oncol (2021) 11:621350. doi: 10.3389/fonc.2021.621350
81. Zhang M, Zou W, Teo BKK. Image guidance in proton therapy for lung cancer. Transl Lung Cancer Res (2018) 7(2):160–70. doi: 10.21037/tlcr.2018.03.26
82. Zhang J, Lu Y, Sheng Y, Wang W, Hong Z, Sun Y, et al. A comparative study of two In vivo PET verification methods in clinical cases. Front Oncol (2021) 11:617787. doi: 10.3389/fonc.2021.617787
83. Richter C, Pausch G, Barczyk S, Priegnitz M, Keitz I, Thiele J, et al. First clinical application of a prompt gamma based in vivo proton range verification system. Radiother Oncol (2016) 118(2):232–7. doi: 10.1016/j.radonc.2016.01.004
84. Yang M, Virshup G, Clayton J, Zhu XR, Mohan R, Dong L. Theoretical variance analysis of single- and dual-energy computed tomography methods for calculating proton stopping power ratios of biological tissues. Phys Med Biol (2010) 55(5):1343–62. doi: 10.1088/0031-9155/55/5/006
85. Dedes G, Dickmann J, Niepel K, Wesp P, Johnson RP, Pankuch M, et al. Experimental comparison of proton CT and dual energy x-ray CT for relative stopping power estimation in proton therapy. Phys Med Biol (2019) 64(16):165002. doi: 10.1088/1361-6560/ab2b72
86. Schneider U, Pedroni E, Lomax A. The calibration of CT hounsfield units for radiotherapy treatment planning. Phys Med Biol (1996) 41(1):111–24. doi: 10.1088/0031-9155/41/1/009
87. Li B, Lee HC, Duan X, Shen C, Zhou L, Jia X, et al. Comprehensive analysis of proton range uncertainties related to stopping-power-ratio estimation using dual-energy CT imaging. Phys Med Biol (2017) 62(17):7056–74. doi: 10.1088/1361-6560/aa7dc9
88. Hansen DC, Seco J, Sørensen TS, Petersen JB, Wildberger JE, Verhaegen F, et al. A simulation study on proton computed tomography (CT) stopping power accuracy using dual energy CT scans as benchmark. Acta Oncol (2015) 54(9):1638–42. doi: 10.3109/0284186X.2015.1061212
89. LaRiviere MJ, Santos PMG, Hill-Kayser CE, Metz JM. Proton therapy. Hematol Oncol Clin North Am (2019) 33(6):989–1009. doi: 10.1016/j.hoc.2019.08.006
90. Ahn S, Park JS, Jang J, Ahn KJ, Hong YK, Yang SH, et al. The association between total lymphocyte count after concomitant chemoradiation and overall survival in patients with newly diagnosed glioblastoma. J Clin Neurosci (2020) 71:21–5. doi: 10.1016/j.jocn.2019.11.017
91. Abravan A, Faivre-Finn C, Kennedy J, McWilliam A, van Herk M. Radiotherapy-related lymphopenia affects overall survival in patients with lung cancer. J Thorac Oncol (2020) 15(10):1624–35. doi: 10.1016/j.jtho.2020.06.008
92. Cho Y, Park S, Byun HK, Lee CG, Cho J, Hong MH, et al. Impact of treatment-related lymphopenia on immunotherapy for advanced non-small cell lung cancer. Int J Radiat Oncol Biol Phys (2019) 105(5):1065–73. doi: 10.1016/j.ijrobp.2019.08.047
Keywords: proton radiation therapy, proton radiotherapy, proton therapy clinical trials, proton therapy non-randomized study, proton therapy randomized study
Citation: Chen Z, Dominello MM, Joiner MC and Burmeister JW (2023) Proton versus photon radiation therapy: A clinical review. Front. Oncol. 13:1133909. doi: 10.3389/fonc.2023.1133909
Received: 29 December 2022; Accepted: 13 March 2023;
Published: 29 March 2023.
Edited by:
Guang Li, Memorial Sloan Kettering Cancer Center, United StatesReviewed by:
Uwe Oelfke, Institute of Cancer Research (ICR), United KingdomCopyright © 2023 Chen, Dominello, Joiner and Burmeister. This is an open-access article distributed under the terms of the Creative Commons Attribution License (CC BY). The use, distribution or reproduction in other forums is permitted, provided the original author(s) and the copyright owner(s) are credited and that the original publication in this journal is cited, in accordance with accepted academic practice. No use, distribution or reproduction is permitted which does not comply with these terms.
*Correspondence: Zhe Chen, Z2s0ODA1QHdheW5lLmVkdQ==
Disclaimer: All claims expressed in this article are solely those of the authors and do not necessarily represent those of their affiliated organizations, or those of the publisher, the editors and the reviewers. Any product that may be evaluated in this article or claim that may be made by its manufacturer is not guaranteed or endorsed by the publisher.
Research integrity at Frontiers
Learn more about the work of our research integrity team to safeguard the quality of each article we publish.