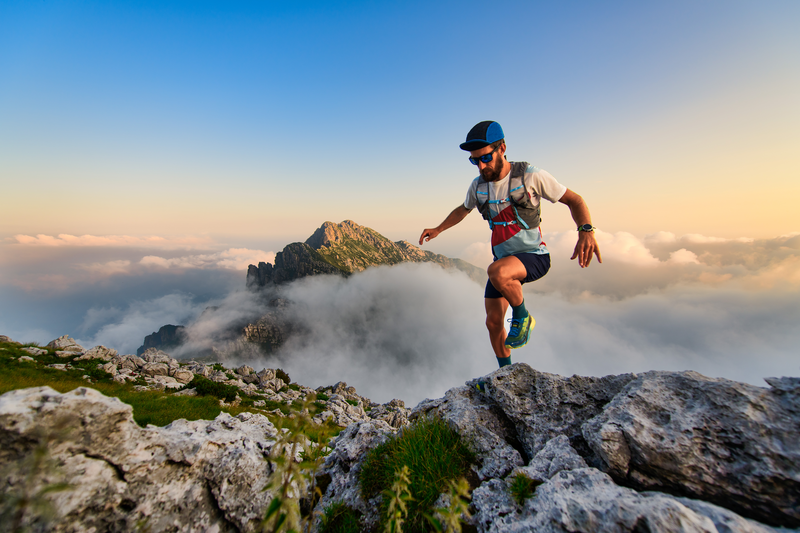
94% of researchers rate our articles as excellent or good
Learn more about the work of our research integrity team to safeguard the quality of each article we publish.
Find out more
REVIEW article
Front. Oncol. , 13 March 2023
Sec. Gastrointestinal Cancers: Colorectal Cancer
Volume 13 - 2023 | https://doi.org/10.3389/fonc.2023.1132141
This article is part of the Research Topic Strategies in Overcoming the Chemoresistance in Colorectal Cancer View all 11 articles
In recent years, the incidences and mortalities from colorectal cancer (CRC) have been increasing; therefore, there is an urgent need to discover newer drugs that enhance drug sensitivity and reverse drug tolerance in CRC treatment. With this view, the current study focuses on understanding the mechanism of CRC chemoresistance to the drug as well as exploring the potential of different traditional Chinese medicine (TCM) in restoring the sensitivity of CRC to chemotherapeutic drugs. Moreover, the mechanism involved in restoring sensitivity, such as by acting on the target of traditional chemical drugs, assisting drug activation, increasing intracellular accumulation of anticancer drugs, improving tumor microenvironment, relieving immunosuppression, and erasing reversible modification like methylation, have been thoroughly discussed. Furthermore, the effect of TCM along with anticancer drugs in reducing toxicity, increasing efficiency, mediating new ways of cell death, and effectively blocking the drug resistance mechanism has been studied. We aimed to explore the potential of TCM as a sensitizer of anti-CRC drugs for the development of a new natural, less-toxic, and highly effective sensitizer to CRC chemoresistance.
As reported by Global Cancer 2020, colorectal cancer (CRC) ranks second and third in global cancer incidence and mortality (1, 2). It has become a great threat to human health. This high incidence rate can be attributed to the asymptomatic manifestations of early CRC and the lack of experience in endoscopic detection so that about 50% of all patients with CRC are at or above the local progressive stage at the time of their first diagnosis (3, 4). Reducing the incidence and mortality of digestive tract tumors and optimizing the treatment scheme of digestive tract tumors are some of the major public health issues that need to be addressed.
Presently, the treatment of CRC involves surgery, radiotherapy, immunotherapy, targeted therapy, and other comprehensive treatments (5). For patients with advanced CRC, chemotherapy is one of the most important treatments. Efforts have been made to use 5-fluorouracil (5-FU) combined with calcium folinate or oxaliplatin (OXA) as the first-line treatment for metastatic CRC, with a drug resistance rate was >40% (6). Some studies have even pointed out that 90% of patients with metastatic CRC died because of chemoresistance (7). This finding depicts that clinical drug resistance and chemoresistance are the major obstacles to the successful cure of cancer. Reducing chemoresistance, minimizing side effects, improving the patient’s prognosis, and quality of life are important factors to be taken into consideration during the development of new treatment options for advanced CRC.
Chemoresistance in CRC hampers the use of standard chemotherapy as a treatment option. Efforts have been made to develop treatment options relying on reversing chemoresistance by targeting newer cytotoxic drugs, regulating drug resistance to conventional drugs, and studying the changes of in tumor gene spectrum before and after chemotherapy. However, most of these strategies result in serious side effects, involve higher treatment costs, and face a crunch in technical expertise (8, 9). The benefits of natural products render them a promising alternative for treating cancer chemoresistance. It has been reported that 50% of the anti-cancer drugs approved by the Food Drug Administration (FDA) originate directly or indirectly from natural products or their derivatives (10). Traditional Chinese medicine (TCM) is multi-targeted and less toxic. TCM treatments are unique, safe, and effective for cancer therapy in China. It is also known to improve the quality of life, prevent metastasis and recurrence, resist chemoresistance, increase efficiency, reduce toxicity, and enhance immunity. Owing to these properties, TCM has gained tremendous research attention worldwide (11). Moreover, the synergistic effects of the TCM extracts and compounds (such as curcumin, berberine, Sophora japonica, and Andrographis paniculate) when combined with traditional chemotherapy could resist chemotherapy tolerance, which is noteworthy.
The present paper focuses on understanding the mechanism of chemoresistance of CRC and explores the potential of TCM and its active ingredients as chemosensitizers in reversing chemotherapy tolerance, reducing toxicity, and increasing the efficiency of CRC. Furthermore, the related mechanisms in vivo and in vitro have been summarized. Finally, based on the current research on the function mechanism of TCM, we believe that TCM as an anticancer treatment option seems promising in solving the difficulties of clinical anticancer treatment.
The chemical resistance of tumor cells is dependent on the outward transporter of the ATP-binding Cassette Family (ABC). Its increased expression often causes drug efflux, thereby leading to multiple drug resistance (MDR) (Figure 1). ABC transporter is a transmembrane protein that assists in transporting various substances across cell membranes. Upon substrate binding, ATP hydrolyzes and actively pumps drugs out of the cell, thereby preventing the accumulation of toxic substances within the cell. Similarly, in cancer cells, the efflux mechanism reduces the concentration of antitumor drugs within cancer cells, thereby protecting the cancer cells (12). It has been reported that the expression of ABC transporter is low in CRC cases, but significantly increased when drug resistance is induced in a variety of CRC cells (13–15).
Anti-MDR strategies include P- glycoprotein (P-gp) inhibitors, RNA interference, nano-drugs and combination drugs, but these transporter inhibitors have not yet shown definite clinical benefits (16–19). The importance of developing new inhibitors with high efficiency and low side effects is self-evident. Several studies have reported that a variety of TCMs and their active ingredients can effectively inhibit the expression and efflux of transporters, reverse the chemoresistance of CRC, and act as chemical sensitizers while exerting cytotoxic effects. Our previous research found that Gegen Qinlian Decoction can inhibit the expression of ABC transporters in vivo and in vitro, and synergize with Oxaliplatin to treat OXA-resistant CRC, and restore the sensitivity to OXA (20).
Glycyrrhiza uralensis Fisch. inhibited the efflux function of transporter P-gp in a CRC cell model and played a therapeutic role with Aconitum carmichaelii Debeaux, enhancing its efficacy and reducing toxicity (21). The ethanol extract of Scutellaria barbata D. Don enhanced the retention of rhodamine, the substrate of ABC transporter in 5-FU-tolerant CRC cells, leading to the inhibition of cell proliferation and cell apoptosis (22). Cucurbitacin E can inhibit TFAP4/Wnt/β-Catenin signaling, down-regulate the expression of ABCC1 and MDR 1 to increase the chemosensitivity of colorectal cancer cells to 5-FU (23). Quercetin and kaempferol regulated the activity and expression of MRP2, thereby promoting the absorption of drugs in the intestinal tract and inhibiting drug efflux as well as downregulating the expression of ABCB1 (24, 25). Neferine, isolated from lotus seeds, combines with P-gp, and increases the retention of drugs in cancer cells (26). Lignans, a secondary metabolite of Arctium lappa L., regulates the efflux function of P-gp in multidrug-resistant cancer cells (27). Pien Tze Huang, a TCM preparation, decreased the proportion of stem cell-like lateral groups of CRC cells in a dose-dependent manner, affecting the morphology of cancer cells, and the mRNA levels of ABCB1 and ABCG2 (28). Evodiamine inhibits the p50/p65 NF-κB pathway and inhibits ABCG2-mediated oxaliplatin resistance (29). On the basis of homologous protein modeling and molecular docking technology, 837 TCMs were reported to have the potential to inhibit the activity of ABCG2 (30). TCM and its active ingredients have demonstrated a good ability to inhibit ABC transporters, making them favorable candidates for developing new inhibitors.
DNA damage repair mechanisms include base excision repair (BER), mismatch repair (MMR), and homologous recombination (HR). These mechanisms identify and correct DNA damage caused by multiple factors such as reactive oxygen species (ROS), radiation, and chemicals (31, 32). DNA damage repair, not only removes the damaged cells but also assists in drug resistance (Figure 2) (33, 34).
Figure 2 Exogenous and endogenous stresses such as radiotherapy and chemotherapy, metabolic reprogramming and ROS cause DNA damage and repair inhibition of CRC cells, which cause chemoresistance by regulating cell death, tumor microenvironment change and drug inactivation.
For example, 5-FU induces a base mismatch in DNA, which is recognized by MMR protein, leading to programmed cell death. Defective MMR prevents cancer cell death. BER recognizes and removes 5-FU in DNA causing drug resistance (7, 35, 36). In addition, nuclear DNA is the main target of platinum-cytotoxic drugs. These drugs covalently bind to DNA to form a Pt-DNA adduct, which damages the normal transcription and replication functions of DNA. This event activates a variety of signal pathways leading to cell apoptosis. Once the process of platinum damaging DNA is inhibited, the tendency of cell apoptosis will get weakened, resulting in drug resistance of the tumor (37–39). The challenges in anti-cancer platinum drugs are avoiding the precise and efficient DNA damage repair mechanisms, such as the HR repair mechanism of DNA double-strand breaks in cells. The mechanism of DNA damage combined with the inhibition of DNA repair results in sensitizing the cancer cells, thereby improving the therapeutic effect.
The use of TCM and prescriptions in DNA damage, the repair of cancer cells by reversing drug resistance, and alleviating the toxic side-effects of chemotherapeutic drugs are well documented (40–42). It has been reported that shikonin from the root of Lithospermum erythrorhizon enhances DNA damage caused by cisplatin by inducing intracellular oxidative stress and mitochondrial activation leading to the inhibition of HCT116 xenograft tumors in nude mice (43). Compound kushen injection led to the inhibition of cell cycle regulatory protein and DNA repair. It also promoted DNA double-strand break and induced cancer cell apoptosis through multiple pathways (44–46). Curcumin is known to regulate the expression of DNA repair-related genes, inhibit tumor growth, promote apoptosis, and enhance the chemosensitivity of MMR-deficient CRC to 5-FU (47–49). Berberine led to the downregulation of RRM1, RRM2, LIG1, and POLE2, participated in DNA repair and replication, and demonstrated the potential to inhibit DNA repair (50). Ginsenoside Rg3 responds to DNA damage by activating the VRK1/P53BP1 pathway, improving the efficiency of chemotherapy, reducing its side effects, and providing a new idea for cancer prevention and treatment (51). Cantharidin works by upregulating KDM4A and catalyzing the demethylation of histone H3K36me3 and inducing DNA damage, which in turn enhances the chemotherapy sensitivity (52). Cytotoxic drugs often result in serious neurotoxicity and other side effects while killing tumor cells, while Silibinin protects against cisplatin-induced neurotoxicity by reducing DNA damage and apoptosis (42, 53, 54).
The programmed cell death pathway is necessary to maintain normal cellular development, which includes apoptosis, necrosis, cell scorch, iron death, and cell death modes related to autophagy and non-programmed necrosis (55, 56). Several studies have validated that apoptosis, autophagy, and other cell death modes are closely related to chemotherapy resistance (Figure 3). Multiple factors such as hunger and chemotherapy cause stress to the cancer cells. The uncontrolled growth of tumor cells leads to increased metabolic demand, which then activates autophagy, leading to the inhibition of tumors by the removal of damaged proteins/organelles (57). The rapid proliferation of cancer cells increases the nutrition and oxygen demand. Throughout treatment, cancer cells are in hypoxia and nutritionally deficient environments. For survival, the cancer cells are significantly dependent on autophagosomes for metabolism. Thus, autophagy fails to inhibit tumor growth, but instead results in chemotherapy resistance, thereby contributing to the development of MDR and protecting cancer cells from the attack of chemotherapy drugs (58–60).
Figure 3 Tumors keep their sustained growth, metastasis and gain chemoresistance by escaping cell death such as ferroptosis, apoptosis and autophagy etc.
Orderly death is called apoptosis, which is essential in maintaining the internal cell environment. Tumor cells lack the ability of apoptosis (20, 61). Tumor cells adapt to the induction of apoptosis by drugs, which leads to the development of drug resistance. An effective way to overcome drug resistance related to apoptosis of cancer cells is through stimulating and restoring the ability of tumor cells to undergo apoptosis and promoting the death mode independent of apoptosis (62–64).
Ferroptosis is a type of event that involves the accumulation of lipid-ROS (L-ROS) in an iron-dependent manner (65, 66). Glutathione (GSH) depletion inactivates GSH-dependent peroxidase (GPX4). Transferrin containing Fe3+ enters the cell and transforms into Fe2+ to participate in the Fenton reaction. The unsaturated fatty acids, such as arachidonic acid, are metabolized into PE-AA through ACSL4 and LPCAT, which are then oxidized by LOXs, all of which can lead to the accumulation of L-ROS and further ferroptosis of cells (67, 68). The inhibition of ferroptosis can promote the development of tumors, resulting in resistance to several types of chemotherapy (69–71).
Reversing the inhibited cell death mode, developing new cell death modes, and inducing cell death are important means to overcome chemoresistance. Various compounds from TCM have been proven to target a variety of cell death modes and reverse the drug resistance induced by chemotherapy drugs. For instance, the combination of artesunate and autophagy inhibition blocked the protective effect of artesunate-induced autophagy on CRC cells and enhanced the apoptosis induced by artesunate (72). Zuojinwan induced apoptosis of HCT116 cells by the PI3K-Akt-signaling pathway (73). Curcumin upregulates CDX2, thereby inhibiting the Wnt/β-catenin signaling pathway and downregulating the anti-apoptosis signaling. This event ultimately results in reduced cell viability and apoptosis of human colon adenocarcinoma cell SW620 (74–77). Pien Tze Huang inhibits STAT3 and the Notch1 pathways and promotes apoptosis of CRC cells (78, 79). Sanguisorba officinalis L. inhibits the Wnt/β-catenin pathway, downregulates Bcl-2 protein, upregulates pro-apoptotic proteins such as Bax, and inhibits the growth and metastasis of 5-FU-resistant CRC (80). Moreover, cisplatin is a known apoptosis inducer. The combined use of the compound kushen injection and cisplatin enhances cell death of SW480 by inducing exogenous apoptosis (46, 68).
Several studies have reported that Chinese medicinal compounds and their active ingredients are involved in the induction and inhibition of autophagy of cancer cells. Autophagy works by inhibiting tumor growth through the removal of damaged organelles and the induction of autophagy under appropriate conditions. Reynoutria japonica Houtt. extracts induce autophagy and promote the apoptosis of cisplatin-resistant cancer cells (81). A. carmichaelii Debeaux induces apoptosis and autophagy, thereby reversing multidrug resistance (82). The treatment of CRC cells with T33, a compound of Chinese medicine, caused changes in autophagy markers, the activation of autophagy, inhibition of tumor proliferation, and reduction in the transplanted tumor volume (83). At the same time, autophagy is also a protective response to DNA damage after drug treatment (84, 85). The increase in the expression of the autophagy gene enhances the vitality of cancer cells and prevents cancer cells from entering apoptosis (72, 86). Moreover, it exhibits a protective effect on cells, resulting in autophagy-dependent chemotherapy resistance (60). It has been reported that resveratrol and epigallocatechin gallate (EGCG), the active ingredient of green tea, enhanced cell death by blocking the drug-induced protective autophagy flux and demonstrated synergistic anticancer effect, thereby overcoming drug resistance (87–89). Flavonoids from S. baicalensis Georgi inhibited autophagy, promoted cell apoptosis, and exerted anti-CRC activity by regulating the ATF4/sestrin2 pathway (90). Sophora flavescens Aiton extract activated the AMPK/mTOR pathway, increased the autophagy flux of colon cancer, and induced apoptosis (91).
Ferroptosis-assisted reverse chemotherapy resistance can be achieved by regulating the GPX4 pathway, iron metabolism pathway, and lipid metabolism pathway (69, 92). Genome analysis revealed that HMOX1, TP53, and ACSL5 were significantly upregulated in activated ferroptosis. This phenomenon was recorded in Andrographis paniculata-mediated CRC chemical sensitivity to 5-FU (93). Camellia nitidissima C. W. Chi decreased the expression of GPX4 and increased the expression of HMOX1 in vivo and in vitro. It also induced ferroptosis to inhibit CRC progress (94). β-elemene, a zedoary turmeric extract, induced ferroptosis through iron-dependent ROS accumulation, GSH consumption, and upregulation of transferrin. The downregulation of negative regulatory proteins of ferroptosis such as GPX4 and GLS, when combined with cetuximab, sensitized KRAS mutant CRC cells (95). Auriculasin isolated from Flemingia philippinensis promoted ROS production, accumulated intracellular Fe2+, and induced CRC ferroptosis in a concentration-dependent manner (96). MAP30 isolated from Momordica charantia seeds along with cisplatin stimulated cytoplasmic oxidative stress and ROS accumulation, induced ferroptosis, and mediated cancer cell death by increasing intracellular Ca2+, thereby exhibiting effective anticancer and chemoresistance effects (97). Glycyrrhetinic acid nanoparticles increased the ROS level in CRC cells by downregulating GPX4 and enhancing Fe-dependent cytotoxicity to kill CRC cells by Fenton reaction (98).
5-FU is the cornerstone of first-line CRC therapy. However, clinical resistance to 5-FU and its derivatives remains a challenge in CRC treatment (36) (Figure 4). In vivo, 5-FU is transformed into FU deoxynucleotide (F-duMP) via the OPRT-RR and TP-TK pathways, inhibiting thymidylate synthase (TS), preventing dUMP methylation to dTMP, inhibiting DNA synthesis, and arresting the cell cycle in the S phase (7). Several studies have reported that gene polymorphism and TS upregulation in CRC are closely related to 5-FU tolerance (7, 99, 100). Thymidine phosphorylase (TP) is upregulated in CRC, which has a poor prognosis. Capecitabine is one of the most active oral fluoropyrimidines. Capecitabine has no cytotoxicity as a precursor of 5-FU, and it is activated by TP to become cytotoxic 5-FU; hence, its adverse reactions are mild and manageable (101). Increasing TP expression is required for chemotherapy drugs such as capecitabine to exert an anti-tumor effect. However, TP has significant angiogenic activities and anti-apoptosis properties, which promote tumor growth and metastasis (102, 103). A TP inhibitor eliminates the tumorigenic effect of TP and the activation of capecitabine. The dual nature of TP also affects chemical resistance. TAS-102 is an oral FU preparation composed of trifluoropyridine (TFT) and thymidine phosphorylation inhibitor (TPI) (104). TFT can inhibit TS from hindering DNA synthesis, but it can also induce DNA damage and demonstrate a cytotoxic effect by doping its metabolites into DNA. In addition, a TP inhibitor prevents TFT degradation by TP and ensures the TFT biological activity (105). This unique action mechanism makes it suitable for patients with CRC with 5-FU and OXA resistance, hence it can be employed in the clinical treatment of metastatic CRC (106).
Figure 4 Oral and injected chemotherapeutic drugs are transformed into cytotoxic compounds after being activated and metabolized by various enzymes in the body. Once the target is changed and the drug is inactivated, CRC cells escape and become resistant to chemotherapy drugs.
5-FU is excreted before getting metabolized to dihydrofluorouracil by dihydropyrimidine dehydrogenase (DPD). A high DPD activity makes 5-FU inactive and tolerant (107, 108). Past studies have demonstrated that using DPD inhibitors to downregulate DPD in CRC and increase 5-FU bioavailability can effectively reverse 5-FU resistance (109). However, the toxicity of 5-FU caused by low DPD activity renders chemotherapy unsustainable (110).
The CYP450 enzyme mediates the inactivation of several anticancer drugs and the activation of precursor drugs. The overexpression of CYP450 subtypes such as CYP3A4 and CYP3A5 in tumor cells renders chemotherapy drugs, such as irinotecan, ineffective, resulting in chemical resistance (111, 112). CYP1A2 and CYP2A6 were significantly upregulated in 5-FU-resistant CRC cells, while the combination of CYP450 inhibitors restored 5-FU cytotoxicity (113).
GSH-transferase (GST) catalyzes the combination of drugs with GSH and excretes it via bile/urine, causing it to detoxify and inactivate, thereby reducing the efficacy of chemotherapy drugs (7). GST overexpression and gene polymorphism are linked to cancer progression and resistance to chemotherapeutic drugs such as OXA (114, 115). Its inhibitor should therefore be administered to boost chemotherapy’s curative effect and alleviate drug resistance.
The overexpression of TS desensitizes CRC to 5-FU drugs, and the overexpression of drug metabolic enzymes such as the CYP450 enzyme inactivates chemotherapeutic drug metabolism, allowing tumor cells to escape. Inhibiting drug target upregulation and lowering drug metabolic inactivation are essential approaches to increase chemotherapeutic sensitivity. Anti-tumor active ingredients derived from traditional Chinese medicinal plants and animals are gaining popularity. By inhibiting TS, formula HQGGT enhanced the toxic effect of 5-FU on 5-FU-resistant CRC cells and decreased tumor growth in vivo (116). Venenum bufonis extract degrades TS by the proteasome to limit TS expression, induce DNA damage, and hinder tumor cell proliferation (117). The expression of drug resistance-related genes TS and DPD in CRC cells was significantly downregulated after treatment with the formula GCFF, and 5-FU cooperated with the inhibition of cancer cell proliferation (118). Coptidis rhizoma extract can be utilized as an adjuvant to assist 5-FU in significantly inhibiting TS activity overexpressed in HCT116/R cells (119). Apigenin inhibited the TS expression and activity in 5-FU-resistant HCT116 cells as well as improved the 5-FU’s therapeutic efficacy on CRC (100). Emodin exists in a variety of herbs. It has been reported that emodin may raise the expression of TP mRNA and protein in cancer cells treated with capecitabine, enhancing capecitabine activation, and downregulating Rad51 and ERCC1, as well as cooperating with capecitabine-induced cytotoxicity (120). S. baicalensis Georgi, S. flavescens, and Schisandra chinensis (Turcz.) Baill. extracts significantly inhibited cytochrome P450 subtype activity in human liver microsomes (121–124). Curcumae rhizoma and Arisaematis Rhizoma Preparatum are two Chinese medicinal plants that have considerate inhibitory effects on CYP3A4 (125).
Epithelial-mesenchymal transition (EMT) is a reversible process in which polar epithelial cells lose the adhesion polarity of the basement membrane and the ability of the tight junction and cell adhesion due to the action of some factors, transforming the cells into mesenchymal cells that are capable of infiltration and migration (126). EMT in several physiological and pathological processes including wound healing, nerve development, and cancer cell metastasis. EMT is involved in several signaling pathways, which include the Notch, Wnt, and TGF-β signaling pathways (127).The changes in epithelial gene markers like E-cadherin and ZO-1 as well as mesenchymal gene markers such as N-cadherin indicate the occurrence of EMT. In recent years, the relationship between EMT and MDR has grown stronger (Figure 5). It was found that silencing the E-cadherin expression altered the chemoresistance of various CRC cell lines to irinotecan and oxaliplatin (128). EMT induced by the hERG1 ion channel reduces cancer cell sensitivity to cisplatin (129). BMAL1 knockdown increases the expression of epithelial markers in CRC cell lines while decreasing the expression of mesenchymal markers, maintains the balance between epithelium and mesenchymal, stabilizes cancer cells in the epithelial characteristic state, inhibits EMT, and reduces the chemical resistance of colon cancer cells (130). Inhibiting the Wnt signaling pathway delays the progress of EMT and reverses CRC chemical resistance to 5-FU (41). The EMT-induced protein ZEB2 promotes oxaliplatin resistance in CRC by activating the nucleotide excision repair genes ERCC1 and ERCC4 (131).
Figure 5 A variety of signal pathways regulate EMT-related transcription factors and downstream related pathways. EMT and crosstalk with TME, DNA damage repair and so on cause CRC resistance.
Cancer chemoresistance is frequently accompanied by EMT, which generates chemical resistance either directly or indirectly, although the mechanism by which EMT drives chemoresistance remains elusive. EMT-mediated chemoresistance is closely related to ABC transporter, tumor microenvironment, and cancer stem cells (CSC). The ABC transporter gene promoter contains the binding sites for EMT transcription factors such as Snail and ZEB. The overexpression of these sites increases the transporter promoter activity in cancer cells, resulting in transporter overexpression and drug efflux (132). EMT cells contain stem cell-like characteristics similar to CSCs in signal pathways and chemoresistance phenotype (133). The FBXW7-ZEB2 axis connects EMT with the tumor microenvironment to promote stem cells and CRC chemoresistance (134). Cancer-associated fibroblasts (CAFs) in the tumor microenvironment can activate Wnt/β-catenin and promote CRC stemness and 5-FU/L-OHP resistance by secreting exosome miR-92a-3p and transferring it into CRC cells (135). Although it is unclear how EMT contributes to chemoresistance, it is evident that inhibiting EMT is an effective measure to reverse chemoresistance and inhibit tumor growth and metastasis.
TCM and its extracts have had a robust response in this regard. Curcumin can reverse 5-FU resistance in CRC cells by regulating the TET1-NKD-Wnt signaling pathway and inhibiting EMT progression, as well as inhibiting the TGF-β/Smad2/3 signaling pathway and reversing OXA resistance in CRC (136, 137). Curcumin can also sensitize 5-FU-resistant cells by upregulating EMT inhibitory miRNA and downregulating BMI1 and other EMT genes (138). Cinnamaldehyde, derived from Cinnamomum cassia, can inhibit the Wnt/β-catenin pathway induced by hypoxia in conjunction with oxaliplatin, reverse EMT and CRC cells dryness, and improve CRC sensitivity to OXA (139). S. officinalis L. blocked the Wnt pathway, which inhibited the proliferation and metastasis of 5-FU-resistant CRC by downregulating N-cadherin, vimentin, and snail protein and upregulating E-cadherin (80). Danshensu inhibits platelet-induced EMT transformation and chemoresistance in CRC cells (140). Jiedu Sangen Decoction inhibits the AKT/GSK-3β signaling pathway, thereby reversing EMT (141, 142). Resveratrol strongly inhibited the formation of EMT and CSC induced by TNF-β, downregulated cancer-promoting factors such as vimentin and slug, upregulated E-cadherin and claudin-2, increased the tight junction and cell adhesion, inhibited NF-κB activation, and increased 5-FU sensitivity in 5-FU-resistant CRC (143, 144). Resveratrol can also inhibit the EMT progression in LoVo cells via the TGF-β1/Smads pathway (145). Quercetin inhibits TGF-β1-induced EMT by inhibiting Twist1 and regulating E-cadherin (146). β-element combined with cetuximab promoted the epithelial marker expression, decreased mesenchymal gene marker expression, inhibited EMT, re-sensitized KRAS mutant CRC cells against EGFR antibody therapy, and inhibited cancer cell migration (95). By promoting the miR-134 expression, astragaloside IV could downregulate the CREB1 signaling pathway, inhibit EMT, and increase the sensitivity of CRC to OXA (147).
The mechanism of the tumor microenvironment (TME) in the MDR phenotype is becoming clearer. TME is an extremely complex ecosystem composed of tumor-associated macrophages (TAM), immune cells, cancer-associated fibroblasts (CAFs), and other non-cellular components such as secretory products and extracellular matrix (148). The cells and molecules in the tumor microenvironment can be exploited to maintain tumor immunosuppression and to promote immune escape, invasion, and treatment resistance. Cell components enhance tumor drug resistance by recruiting and secreting a variety of protective cytokines; non-cellular components, such as extracellular matrix (ECM) and hypoxia, can mediate drug resistance by building physical barriers and affecting tumor cell growth and metabolism (149–151). TAM secretes IL-6 and MFG-E8, respectively, activates the IL-6R/STAT3 and Hedgehog pathways, induces CSCs, and shields CSCs from the cytotoxic effects of 5-FU and cisplatin, resulting in treatment resistance and recurrence (152–154). CAFs transfer exosomes miR-92a-3p and lncRNA H19 into CRC cells, activate Wnt/β-catenin, mediate EMT, and promote CRC stemness and 5-FU/OXA resistance (135, 155). The hypoxic HOTAIR/miR-1277-5p/ZEB1 axis mediated oxaliplatin resistance in CRC (156, 157). Hypoxia can also interact with other mechanisms, such as activating cytochrome P450 subtype CYP3A6 and accelerating drug metabolism; it can also induce ABC transporter upregulation and promote drug efflux (158). Drugs’ effects are opposed and limited due to the intricacy of the tumor microenvironment. The therapeutic scheme targeting tumor microenvironment is becoming one of the most promising anti-cancer strategies in the future as our understanding of it grows (Figure 6).
Figure 6 Tumor microenvironment in chemoresistant CRC. Extracellular matrix acts as a physical barrier to prevent drugs from entering. The microenvironment of the formation of new blood vessels, hypoxia and acidification contributes to chemoresistance. Cancer-associated fibroblasts (CAFs) and cancer stem cells (CSCs) promote tumor by secreting tumor growth factors and exosomes. Immune cells acquire immunosuppressive phenotype to make the tumor escape.
TCM has been proven in studies to regulate TME-related molecular mechanisms and serve an anti-tumor role (157). Curcumin can enhance the FOLFOX’s cytotoxic effect on colon cancer by regulating EGFR and IGF-1R (159). Formula PHY906 can increase CPT-11’s anti-tumor activity by reducing neutrophil or macrophage infiltration, TNF-α expression, and pro-inflammatory cytokine concentrations, inhibiting NF-κB and lowering irinotecan (CPT-11)’s gastrointestinal toxicity (160). Gegen Qinlian Decoction combined with PD-1 inhibitor could significantly increase CD8+T cells in the peripheral blood and tumor tissues, reshaped the tumor microenvironment, inhibited immune checkpoint, and effectively restored the T cell functions (161). Ginsenosides can regulate the quantity and function of bone marrow immunosuppressive agents, enhance the body’s anti-cancer ability, and inhibit cancer cell growth, metastasis, and recurrence in a tumor microenvironment (162). When CRC cells are in the hypoxia microenvironment, tanshinone IIA inhibits angiogenesis by targeting TGF-β1 and inhibiting the HIF-1α/β-Catenin/VEGF pathway (163). Resveratrol inhibits tumor stem cell-like phenotype induced by TNF-β and increases chemotherapy sensitivity (144). Pien Tze Huang inhibited the Notch1 pathway in vivo to negatively regulate CSCs characteristics, prevent spheroid formation, and diminish tumorigenicity (78). The water extract of Huaier can inhibit the proliferation of CRC stem cells by downregulating the Wnt/β-catenin pathway (164). Berberine, an immunological checkpoint inhibitor, can enhance the immunity of tumor-infiltrating T cells, activate regulatory T cells, and diminish immunosuppressive myeloid-derived suppressor cells (MDSC) (165). Andrographolide, in combination with a PD-1 inhibitor, improved the function of CD4 and CD8 T cells, affected the expression of T cell-related markers, and inhibited the growth of CRC (166). Gallic acid combined with anti-PD-1 antibody can block PD-L1/PD-1 signal transduction, downregulate Foxp3 stability, inhibit regulatory T cells, promote CD8 T cells to secrete IFN-γ, and limit CRC (167). Nano-drugs containing ursolic acid and lentinan can destroy immunogenic cells, promote dendritic cell maturation, repolarize TAM into anti-tumor M1 type, reshape immunosuppressive TME, and render it sensitive to immunotherapy (168).
Despite the continuous development of treatment modalities, chemoresistance remains the most significant barrier to the curative treatment of various cancers, while epigenetics modification also plays a key role in the occurrence and development of chemotherapy resistance in tumors (Figure 7). Epigenetic modification influences cancer cell proliferation, metabolism, and metastasis, leading to anti-cancer drug resistance (169, 170). Histone alteration, such as methylation and deacetylation, interferes with the efficacy of drugs by regulating the expression of multidrug efflux transporters, drug-metabolizing enzymes, and drug targets (171–178). In addition, by regulating DNA damage repair, cancer stem cells, and tumor microenvironment, epigenetic modification protects cells against drug-mediated cell death (179–181). Currently, the emergence of several epigenetic inhibitors, such as DNA methyltransferase inhibitors and histone methyltransferase inhibitors, may make epigenetics a viable treatment strategy for reversing CRC drug resistance. When compared to traditional chemotherapy drugs, epigenetics drugs offer certain hope, although several problems still need to be addressed. DNMT inhibitors azacytidine and decitabine can cause bone marrow suppression and trigger gastrointestinal reactions (182). The performance of natural compounds in inhibiting epigenetic changes attracts people’s attention.
Figure 7 The role of epigenetic modification in chemoresistant CRC. DNA methylation, RNA methylation, histone methylation, deacetylation, etc., lead to CRC chemoesistance by promoting drug efflux, changing drug metabolism and cell death and other ways.
Curcumin has several epigenetic inhibitory activities. In vivo and in vitro, it can suppress the activity and expression of histone deacetylases in CRC, and reactivate the tumor suppressor gene RARβ (183, 184). Curcumin can be utilized to inhibit DNA methyltransferase, demethylate DNA, reverse decitabine tolerance in CRC (185). The combination of emodin and decitabine can significantly increase DNMT1 and DNMT3a expressions, improve the demethylation of tumor suppressor genes P16, RASSF1A, and ppENK, and play an anti-cancer role (186). Cinnamic acid derivatives inhibit histone deacetylase, which causes CRC cell death (187). Berberine lowered m6A via inhibiting β-catenin and increased FTO expression, which suppressed the stemness and tumorigenicity of CSCs in CRC (188). BBR binds to CSN5, blocking its ubiquitination activity, causing PD-L1 to be ubiquitinated and degraded, and preventing cancer cells from passing through immune checkpoints (165). Aidi injection inhibits ubiquitin-proteasome system activity, diminishes cytotoxic protein degradation, and targets mitochondrial apoptosis (189). TCM can target epigenetic modification, transform CRC into a therapeutic response, and prevent or overcome chemoresistance.
Tumor metabolic abnormalities are considered the new hallmark of cancer therapy (190). Metabolic reprogramming, which involves lipid metabolism, oxidative stress, mitochondrial metabolism, and glycolysis, is significant in cancer chemoresistance (191–194). Chemoresistance mediated by tumor metabolic changes is closely related to TME and epigenetic modifications. The high expression of the methylated protein YTHDF1 promotes the high expression and activity of transglutaminase 1, resulting in CRC’s tolerance to cisplatin (177). The methylation of histone causes glutamine synthetase (GS) to be upregulated in adipocytes, and the resultant rise in the glutamine level is a potential resistance mechanism in patients with CRC to 5-FU (195). HIF-1α-induced glucose metabolism reprogramming confers 5-FU resistance to CRC cells (196). The application of drugs and small molecular compounds targeting the abnormal metabolism of tumors plays a decisive role in reversing the tolerance of traditional chemotherapy drugs and inhibiting the growth of tumors, which is the future of cancer treatment.
TCM, as an alternative and complementary medicine, has also demonstrated exceptional efficacy in regulating abnormal tumor metabolism. Moreover, natural compounds have fewer side effects while inhibiting the growth of cancer cells. By downregulating HIF-1α, Worenine and Matrine could reverse the Warburg effect and inhibit the growth of CRC cells (197, 198). Kaempferol upregulates miR-326-hnRNPA1, inhibits glycolysis mediated by PKM2, and reverses tolerance to 5-Fu in CRC (199). Jiedu Sangen decoction inhibits glycolysis in CRC by regulating the PI3K/AKT/HIF-1α signaling pathway and restoring 5-FU sensitivity (200). RA-XII is extracted from Rubia yunnanensis Diels and it inhibits CRC by inhibiting fatty acid synthesis (201).
The role of microbes in regulating drug metabolism is becoming increasingly clear with time. It is also established that microbes play an important role in cancer development and treatment resistance. UroA, a microbial metabolite, can inhibit ABC transporters by regulating the FOXO3-FOXM1 axis and sensitizing 5-FU-resistant CRC (202). Lactobacillus plantarum metabolites restored SMCT1 expression and function in 5-FU-resistant CRC cells, overcoming the double resistance of 5-FU and butyrate (203). The impairment of TGF-β signaling creates an intestinal flora imbalance and CRC resistance to 5-FU (204). Fusobacterium nucleatum promotes non-CSC lipid accumulation in CRC to achieve CSC characteristics, decreases lipid accumulation in CSC to allow it to self-renew, activates autophagy, and induces chemical resistance in CRC (205, 206). The intestinal flora can regulate the anticancer activity of oxaliplatin and cyclophosphamide and induce T-cell reaction and ROS production (207). The role of intestinal flora in chemical resistance is multifaceted, making it the guardian as well as the enemy.
Chinese herbal medicine is closely related to intestinal bacteria and plays a key role in cancer therapy. TCM influences the composition of intestinal flora, whereas intestinal microbes influence the metabolism of Chinese herbal medicines’ active compounds. This interdependent relationship offers fresh possibilities for cancer treatment. Berberine has been demonstrated to inhibit Fusobacterium nucleatum, regulate intestinal flora composition, relieve immunosuppression, and inhibit CRC (208, 209). Wu pill can help prevent colitis-related CRC by regulating the intestinal microbiota (210). However, ferulic acid needs to be metabolized by intestinal flora, and its product has stronger anti-cancer activity than its mother, which is used to treat chemical resistance CRC (211). Yi-Yi-Fu-Zi-Bai-Jiang-San has no direct inhibitory effect on CRC, but it significantly improves the intestinal bacteria composition and tumor immune infiltration of CRC tumor-bearing mice and inhibits the tumor growth after fecal bacteria transplantation treatment with the intestinal flora regulated by this compound (212).
Enhancing treatment sensitivity or overcoming chemoresistance is a pressing issue in the field of cancer. Several studies have reported that TCM participates in inhibiting tumor growth and metastasis, improving tumor microenvironment, regulating cell death, inhibiting CRC progression, increasing the response of drug-resistant CRC to drug treatment, reducing toxic and side effects, improving prognosis, prolonging patient survival time (Table 1). TCM’s auxiliary sensitizing effect on chemotherapy sensitivity is becoming increasingly essential and evident. TCM used in combination with anticancer drugs can increase anticancer drug intracellular accumulation, prevent the original drug tolerance mechanism or mediate a new cell death mechanism, modify the tumor microenvironment, relieve the immunosuppression, erase methylation, and other reversible modifications. We thus believe that TCM has a promising future as an alternative and complementary therapy for clinical cancer treatment, whether used alone or as a sensitizer to other anticancer drugs.
However, the components of TCM and compound prescription are complex, and it is difficult to fully clarify its mechanism. Different from TCM, modern medicine mainly starts from the occurrence and development of cancer in the treatment of cancer, and the treatment mechanism and targeted path are clear. A variety of targeted inhibitors or small molecule drugs have been developed to overcome the chemical resistance of traditional chemotherapy drugs, such as ABC transports inhibitors, iron death inducers, autophagy inhibitors, DNA methylation inhibitors, histone deacetylase inhibitors, etc., and corresponding clinical trials have been carried out. Although these targeted preparations have shown some promising clinical results, their side effects that cannot be ignored also limit their real clinical application. ABC transports has not been approved for the treatment of malignant tumors due to its non-targeted selective inhibition and individual differences (213). The stability and dosage of epigenetic drugs also make their effects in solid tumors need to be improved (214). Under the development trend of precision medicine,TCM’s effective components have become the research focus for Chinese medicine combined with chemotherapy to reverse MDR. Presently, most TCM research on active compounds for reversing CRC MDR is mostly concentrated in flavonoids and phenols, with the greatest study in CRC in combination with traditional drugs (215). In reversing CRC multidrug resistance, Chinese medicine and its active ingredients offer both considerable potential and controversy. Different from the precise targeting characteristics of modern medicine, TCM has the characteristics of multi-component, multi-target and multi-stage in nature. For example, curcumin can inhibit DNA damage repair, inhibit EMT, down-regulate the expression and activity of HDAC and cooperate with DNA demethylation of azacytidine to reverse the resistance of decitabine and 5-FU. This feature also makes it difficult for TCM to create secondary drug resistance or even multi-drug resistance. However, because of the complex components and numerous targets of TCM and compound prescription, as well as the thinking theory and compatibility of dialectical administration in TCM theory, it is difficult to specify the exact method. Presently, the research on compound prescription is limited to a single component and mechanism and is primarily concentrated in a single extract, which does not reflect the TCM’s theory of syndrome differentiation and treatment, and the principle of compatibility among the monarch, minister assistant, and guide. Some scholars studied the optimal proportion of the main active ingredients of Aidi injection, namely cantharidin, calycosin-7-O-β-D-glucoside, ginsenoside Rc, and ginsenoside Rd, and discovered that the latter three can inhibit the degradation of cytotoxic proteins by inhibiting the activity of the ubiquitin-proteasome system, which is enhanced by cantharidin. Synergistic cantharidin can activate PP2A, activate mitochondrial apoptosis, and promote CRC cell death (189). This report fully demonstrates the scientific nature of the compatibility theory of TCM. The reasonable compatibility of TCM is also the subject of TCM modernization research. TCM research as a chemical sensitizer mainly focuses on cell and animal experiments or is based on in vitro research. Although several clinical trials of TCM for CRC have been conducted, there is still a lack of evident clinical randomized controlled trials, and it has not yet become the first line of clinical medication. Weak absorption, low solubility, bioavailability, and unknown side effects have always limited the development of TCM’s global expansion. However, with the development of nanotechnologies, such as liposomes, nanoparticles, and bionic drug delivery systems, the abovementioned defects of active ingredients of TCM have gradually been addressed (216–219). It is critical to accelerate the transformation of TCM experimental research findings into clinical applications by conducting evidence-based medicine and component research to assess efficacy and safety. Currently, the establishment of the State Key Laboratory of Traditional Chinese Medicine Components and its component bank and the Food and Drug Administration’s approval of clinical TCM research have all accelerated the pace of modern TCM research and development.
With an increasing incidence rate of CRC morbidity and mortality, the concerns related to CRC treatment include improving drug sensitivity and reversing chemoresistance. TCM can work in conjunction with anticancer medications to lessen side effects and boost effectiveness, boost intracellular anticancer drug accumulation, block initial drug tolerance, mediate cell death, enhance tumor microenvironment, relieve immunosuppression, erase methylation and other reversible modifications, and produce significant anticancer efficacy. TCM has shown great advantages in the process of increasing chemotherapy sensitivity with its multi-component, multi-target and multi-stage treatment characteristics different from modern medicine. We believe TCM has a promising future as an alternative and complementary therapy for clinical CRC treatment in developing new sensitizers to other anticancer drugs.
XL and XH contributed into the writing and revision of the manuscript. XY, YY and HZ draw figures and tables. All authors contributed to the article and approved the submitted version.
This work was funded by grants from the Administration of Traditional Chinese Medicine of Zhejiang Province, China (No.2021ZZ013 and No.2022ZX006).
The authors would like to thank all workers who participated in the article, as well as MJEditor (www.mjeditor.com) for providing English editing services and the authorization of Figdraw.
The authors declare that the research was conducted in the absence of any commercial or financial relationships that could be construed as a potential conflict of interest.
All claims expressed in this article are solely those of the authors and do not necessarily represent those of their affiliated organizations, or those of the publisher, the editors and the reviewers. Any product that may be evaluated in this article, or claim that may be made by its manufacturer, is not guaranteed or endorsed by the publisher.
1. Wang L, Lo CH, He X, Hang D, Wang M, Wu K, et al. Risk factor profiles differ for cancers of different regions of the colorectum. Gastroenterol (2020) 159(1):241–56 e13. doi: 10.1053/j.gastro.2020.03.054
2. Sung H, Ferlay J, Siegel RL, Laversanne M, Soerjomataram I, Jemal A, et al. Global cancer statistics 2020: GLOBOCAN estimates of incidence and mortality worldwide for 36 cancers in 185 countries. CA Cancer J Clin (2021) 71(3):209–49. doi: 10.3322/caac.21660
3. Dekker E, Tanis PJ, Vleugels JLA, Kasi PM, Wallace MB. Colorectal cancer. Lancet (2019) 394(10207):1467–80. doi: 10.1016/S0140-6736(19)32319-0
4. Wang KW, Dong M. Potential applications of artificial intelligence in colorectal polyps and cancer: Recent advances and prospects. World J Gastroenterol (2020) 26(34):5090–100. doi: 10.3748/wjg.v26.i34.5090
5. Garcia-Alfonso P, Torres G, Garcia G, Gallego I, Ortega L, Sandoval C, et al. FOLFOXIRI plus biologics in advanced colorectal cancer. Expert Opin Biol Ther (2019) 19(5):411–22. doi: 10.1080/14712598.2019.1595580
6. Satapathy SR, Sjolander A. Cysteinyl leukotriene receptor 1 promotes 5-fluorouracil resistance and resistance-derived stemness in colon cancer cells. Cancer Lett (2020) 488:50–62. doi: 10.1016/j.canlet.2020.05.023
7. Vodenkova S, Buchler T, Cervena K, Veskrnova V, Vodicka P, Vymetalkova V. 5-fluorouracil and other fluoropyrimidines in colorectal cancer: Past, present and future. Pharmacol Ther (2020) 206:107447. doi: 10.1016/j.pharmthera.2019.107447
8. Baird RD, Kaye SB. Drug resistance reversal–are we getting closer? Eur J Cancer (2003) 39(17):2450–61. doi: 10.1016/S0959-8049(03)00619-1
9. Xu JF, Wan Y, Tang F, Chen L, Yang Y, Xia J, et al. Emerging significance of ginsenosides as potentially reversal agents of chemoresistance in cancer therapy. Front Pharmacol (2021) 12:720474. doi: 10.3389/fphar.2021.720474
10. Newman DJ, Cragg GM. Natural products as sources of new drugs from 1981 to 2014. J Nat Prod (2016) 79(3):629–61. doi: 10.1021/acs.jnatprod.5b01055
11. Huang YF, Niu WB, Hu R, Wang LJ, Huang ZY, Ni SH, et al. Correction: FIBP knockdown attenuates growth and enhances chemotherapy in colorectal cancer via regulating GSK3beta-related pathways. Oncogenesis (2022) 11(1):36. doi: 10.1038/s41389-022-00407-5
12. Wang Y, Wang Y, Qin Z, Cai S, Yu L, Hu H, et al. The role of non-coding RNAs in ABC transporters regulation and their clinical implications of multidrug resistance in cancer. Expert Opin Drug Metab Toxicol (2021) 17(3):291–306. doi: 10.1080/17425255.2021.1887139
13. Hsu HH, Chen MC, Baskaran R, Lin YM, Day CH, Lin YJ, et al. Oxaliplatin resistance in colorectal cancer cells is mediated via activation of ABCG2 to alleviate ER stress induced apoptosis. J Cell Physiol (2018) 233(7):5458–67. doi: 10.1002/jcp.26406
14. Theile D, Wizgall P. Acquired ABC-transporter overexpression in cancer cells: transcriptional induction or Darwinian selection? Naunyn Schmiedebergs Arch Pharmacol (2021) 394(8):1621–32. doi: 10.1007/s00210-021-02112-3
15. Zhu Y, Huang S, Chen S, Chen J, Wang Z, Wang Y, et al. SOX2 promotes chemoresistance, cancer stem cells properties, and epithelial-mesenchymal transition by beta-catenin and Beclin1/autophagy signaling in colorectal cancer. Cell Death Dis (2021) 12(5):449. doi: 10.1038/s41419-021-03733-5
16. Abdallah HM, Al-Abd AM, El-Dine RS, El-Halawany AM. P-glycoprotein inhibitors of natural origin as potential tumor chemo-sensitizers: A review. J Adv Res (2015) 6(1):45–62. doi: 10.1016/j.jare.2014.11.008
17. Li W, Zhang H, Assaraf YG, Zhao K, Xu X, Xie J, et al. Overcoming ABC transporter-mediated multidrug resistance: Molecular mechanisms and novel therapeutic drug strategies. Drug Resist Updat (2016) 27:14–29. doi: 10.1016/j.drup.2016.05.001
18. Robey RW, Pluchino KM, Hall MD, Fojo AT, Bates SE, Gottesman MM. Revisiting the role of ABC transporters in multidrug-resistant cancer. Nat Rev Cancer (2018) 18(7):452–64. doi: 10.1038/s41568-018-0005-8
19. Mohammad IS, He W, Yin L. Insight on multidrug resistance and nanomedicine approaches to overcome MDR. Crit Rev Ther Drug Carrier Syst (2020) 37(5):473–509. doi: 10.1615/CritRevTherDrugCarrierSyst.2020025052
20. Lin X, Xu L, Tan H, Zhang X, Shao H, Yao L, et al. The potential effects and mechanisms of gegen qinlian decoction in oxaliplatin-resistant colorectal cancer based on network pharmacology. Heliyon (2022) 8(11):e11305. doi: 10.1016/j.heliyon.2022.e11305
21. He Y, Wei Z, Xie Y, Yi X, Zeng Y, Li Y, et al. Potential synergic mechanism of wutou-gancao herb-pair by inhibiting efflux transporter p-glycoprotein. J Pharm Anal (2020) 10(2):178–86. doi: 10.1016/j.jpha.2019.09.004
22. Lin J, Feng J, Yang H, Yan Z, Li Q, Wei L, et al. Scutellaria barbata d. don inhibits 5-fluorouracil resistance in colorectal cancer by regulating PI3K/AKT pathway. Oncol Rep (2017) 38(4):2293–300. doi: 10.3892/or.2017.5892
23. Yang P, Liu W, Fu R, Ding GB, Amin S, Li Z. Cucurbitacin e chemosensitizes colorectal cancer cells via mitigating TFAP4/Wnt/beta-catenin signaling. J Agric Food Chem (2020) 68(48):14148–60. doi: 10.1021/acs.jafc.0c05551
24. Zhang S, Morris ME. Effect of the flavonoids biochanin a and silymarin on the p-glycoprotein-mediated transport of digoxin and vinblastine in human intestinal caco-2 cells. Pharm Res (2003) 20(8):1184–91. doi: 10.1023/A:1025044913766
25. Xiao Y, Xin L, Li L, Li G, Shi X, Ji G, et al. Quercetin and kaempferol increase the intestinal absorption of isorhamnetin coexisting in elaeagnus rhamnoides (L.) a. nelson (Elaeagnaceae) extracts via regulating multidrug resistance-associated protein 2. Phytomedicine (2019) 53:154–62. doi: 10.1016/j.phymed.2018.09.028
26. Kadioglu O, Law BYK, Mok SWF, Xu SW, Efferth T, Wong VKW. Mode of action analyses of neferine, a bisbenzylisoquinoline alkaloid of lotus (Nelumbo nucifera) against multidrug-resistant tumor cells. Front Pharmacol (2017) 8:238. doi: 10.3389/fphar.2017.00238
27. Su S, Cheng X, Wink M. Natural lignans from arctium lappa modulate p-glycoprotein efflux function in multidrug resistant cancer cells. Phytomedicine (2015) 22(2):301–7. doi: 10.1016/j.phymed.2014.12.009
28. Wei L, Chen P, Chen Y, Shen A, Chen H, Lin W, et al. Pien tze Huang suppresses the stem-like side population in colorectal cancer cells. Mol Med Rep (2014) 9(1):261–6. doi: 10.3892/mmr.2013.1760
29. Sui H, Zhou LH, Zhang YL, Huang JP, Liu X, Ji Q, et al. Evodiamine suppresses ABCG2 mediated drug resistance by inhibiting p50/p65 NF-kappaB pathway in colorectal cancer. J Cell Biochem (2016) 117(6):1471–81. doi: 10.1002/jcb.25451
30. Le MT, Hoang VN, Nguyen DN, Bui TH, Phan TV, Huynh PN, et al. Structure-based discovery of ABCG2 inhibitors: A homology protein-based pharmacophore modeling and molecular docking approach. Molecules (2021) 26(11). doi: 10.3390/molecules26113115
31. Tomasini PP, Guecheva TN, Leguisamo NM, Pericart S, Brunac AC, Hoffmann JS, et al. Analyzing the opportunities to target DNA double-strand breaks repair and replicative stress responses to improve therapeutic index of colorectal cancer. Cancers (Basel) (2021) 13(13). doi: 10.3390/cancers13133130
32. Wang M, Xie C. DNA Damage repair and current therapeutic approaches in gastric cancer: A comprehensive review. Front Genet (2022) 13:931866. doi: 10.3389/fgene.2022.931866
33. Wiegering A, Matthes N, Muhling B, Koospal M, Quenzer A, Peter S, et al. Reactivating p53 and inducing tumor apoptosis (RITA) enhances the response of RITA-sensitive colorectal cancer cells to chemotherapeutic agents 5-fluorouracil and oxaliplatin. Neoplasia (2017) 19(4):301–9. doi: 10.1016/j.neo.2017.01.007
34. Marin JJG, Macias RIR, Monte MJ, Herraez E, Peleteiro-Vigil A, Blas BS, et al. Cellular mechanisms accounting for the refractoriness of colorectal carcinoma to pharmacological treatment. Cancers (Basel) (2020) 12(9). doi: 10.1016/j.neo.2017.01.007
35. de Castro EGH, Jesuino Nogueira L, Bencke Grudzinski P, da Costa Ghignatti PV, Guecheva TN, Motta Leguisamo N, et al. Olaparib-mediated enhancement of 5-fluorouracil cytotoxicity in mismatch repair deficient colorectal cancer cells. BMC Cancer (2021) 21(1):448. doi: 10.1186/s12885-021-08188-7
36. Sethy C, Kundu CN. 5-fluorouracil (5-FU) resistance and the new strategy to enhance the sensitivity against cancer: Implication of DNA repair inhibition. BioMed Pharmacother (2021) 137:111285. doi: 10.1016/j.biopha.2021.111285
37. Xian C, Chen H, Xiong F, Fang Y, Huang H, Wu J. Platinum-based chemotherapy via nanocarriers and co-delivery of multiple drugs. Biomater Sci (2021) 9(18):6023–36. doi: 10.1039/D1BM00879J
38. Koberle B, Schoch S. Platinum complexes in colorectal cancer and other solid tumors. Cancers (Basel) (2021) 13(9). doi: 10.3390/cancers13092073
39. Qu J, Sun Z, Peng C, Li D, Yan W, Xu Z, et al. C. tropicalis promotes chemotherapy resistance in colon cancer through increasing lactate production to regulate the mismatch repair system. Int J Biol Sci (2021) 17(11):2756–69. doi: 10.7150/ijbs.59262
40. Huang W, Tang H, Wen F, Lu X, Li Q, Shu P. Jianpi-yangwei decoction inhibits DNA damage repair in the drug resistance of gastric cancer by reducing FEN1 expression. BMC Complement Med Ther (2020) 20(1):196. doi: 10.1186/s12906-020-02983-8
41. Liu Y, Yang S, Wang K, Lu J, Bao X, Wang R, et al. Cellular senescence and cancer: Focusing on traditional Chinese medicine and natural products. Cell Prolif (2020) 53(10):e12894. doi: 10.1111/cpr.12894
42. Wang XL, Lin FL, Xu W, Wang C, Wang QQ, Jiang RW. Silybin b exerts protective effect on cisplatin-induced neurotoxicity by alleviating DNA damage and apoptosis. J Ethnopharmacol (2022) 288:114938. doi: 10.1016/j.jep.2021.114938
43. He G, He G, Zhou R, Pi Z, Zhu T, Jiang L, et al. Enhancement of cisplatin-induced colon cancer cells apoptosis by shikonin, a natural inducer of ROS in vitro and in vivo. Biochem Biophys Res Commun (2016) 469(4):1075–82. doi: 10.1016/j.bbrc.2015.12.100
44. Cui J, Qu Z, Harata-Lee Y, Nwe Aung T, Shen H, Wang W, et al. Cell cycle, energy metabolism and DNA repair pathways in cancer cells are suppressed by compound kushen injection. BMC Cancer (2019) 19(1):103. doi: 10.1186/s12885-018-5230-8
45. Zhang W, Gong W, He X, Wu C, Tu X. A systematic review and meta-analysis on the efficacy of compound kushen injection in 3 kinds of digestive tract tumor. J Gastrointest Oncol (2021) 12(6):2919–29. doi: 10.21037/jgo-21-774
46. Wu X, Lu Y, Qin X. Combination of compound kushen injection and cisplatin shows synergistic antitumor activity in p53-R273H/P309S mutant colorectal cancer cells through inducing apoptosis. J Ethnopharmacol (2022) 283:114690. doi: 10.1016/j.jep.2021.114690
47. Shakibaei M, Buhrmann C, Kraehe P, Shayan P, Lueders C, Goel A. Curcumin chemosensitizes 5-fluorouracil resistant MMR-deficient human colon cancer cells in high density cultures. PloS One (2014) 9(1):e85397. doi: 10.1371/journal.pone.0085397
48. Yang G, Qiu J, Wang D, Tao Y, Song Y, Wang H, et al. Traditional Chinese medicine curcumin sensitizes human colon cancer to radiation by altering the expression of DNA repair-related genes. Anticancer Res (2018) 38(1):131–6. doi: 10.21873/anticanres.12200
49. Li T, Pan DB, Pang QQ, Zhou M, Yao XJ, Yao XS, et al. Diarylheptanoid analogues from the rhizomes of zingiber officinale and their anti-tumour activity. RSC Adv (2021) 11(47):29376–84. doi: 10.1039/D1RA03592D
50. Ni L, Li Z, Ren H, Kong L, Chen X, Xiong M, et al. Berberine inhibits non-small cell lung cancer cell growth through repressing DNA repair and replication rather than through apoptosis. Clin Exp Pharmacol Physiol (2022) 49(1):134–44. doi: 10.1111/1440-1681.13582
51. Liu T, Zuo L, Guo D, Chai X, Xu J, Cui Z, et al. Ginsenoside Rg3 regulates DNA damage in non-small cell lung cancer cells by activating VRK1/P53BP1 pathway. BioMed Pharmacother (2019) 120:109483. doi: 10.1016/j.biopha.2019.109483
52. Wei C, Deng X, Gao S, Wan X, Chen J. Cantharidin inhibits proliferation of liver cancer by inducing DNA damage via KDM4A-dependent histone H3K36 demethylation. Evid Based Complement Alternat Med (2022) 2022:2197071. doi: 10.1155/2022/2197071
53. Alberti P. Platinum-drugs induced peripheral neurotoxicity: Clinical course and preclinical evidence. Expert Opin Drug Metab Toxicol (2019) 15(6):487–97. doi: 10.1080/17425255.2019.1622679
54. Staff NP, Cavaletti G, Islam B, Lustberg M, Psimaras D, Tamburin S. Platinum-induced peripheral neurotoxicity: From pathogenesis to treatment. J Peripher Nerv Syst (2019) 24 Suppl 2:S26–39. doi: 10.1111/jns.12335
55. Su Z, Yang Z, Xu Y, Chen Y, Yu Q. Apoptosis, autophagy, necroptosis, and cancer metastasis. Mol Cancer (2015) 14:48. doi: 10.1186/s12943-015-0321-5
56. Bedoui S, Herold MJ, Strasser A. Emerging connectivity of programmed cell death pathways and its physiological implications. Nat Rev Mol Cell Biol (2020) 21(11):678–95. doi: 10.1038/s41580-020-0270-8
57. Li X, He S, Ma B. Autophagy and autophagy-related proteins in cancer. Mol Cancer (2020) 19(1):12. doi: 10.1186/s12943-020-1138-4
58. Ferro F, Servais S, Besson P, Roger S, Dumas JF, Brisson L. Autophagy and mitophagy in cancer metabolic remodelling. Semin Cell Dev Biol (2020) 98:129–38. doi: 10.1016/j.semcdb.2019.05.029
59. Li YJ, Lei YH, Yao N, Wang CR, Hu N, Ye WC, et al. Autophagy and multidrug resistance in cancer. Chin J Cancer (2017) 36(1):52. doi: 10.1186/s40880-017-0219-2
60. Zamame Ramirez JA, Romagnoli GG, Kaneno R. Inhibiting autophagy to prevent drug resistance and improve anti-tumor therapy. Life Sci (2021) 265:118745. doi: 10.1016/j.lfs.2020.118745
61. Kashyap D, Garg VK, Goel N. Intrinsic and extrinsic pathways of apoptosis: Role in cancer development and prognosis. Adv Protein Chem Struct Biol (2021) 125:73–120. doi: 10.1016/bs.apcsb.2021.01.003
62. Samanta D, Huang TY, Shah R, Yang Y, Pan F, Semenza GL. BIRC2 expression impairs anti-cancer immunity and immunotherapy efficacy. Cell Rep (2020) 32(8):108073. doi: 10.1016/j.celrep.2020.108073
63. Carneiro BA, El-Deiry WS. Targeting apoptosis in cancer therapy. Nat Rev Clin Oncol (2020) 17(7):395–417. doi: 10.1038/s41571-020-0341-y
64. Balaji S, Terrero D, Tiwari AK, Ashby CR Jr., Raman D. Alternative approaches to overcome chemoresistance to apoptosis in cancer. Adv Protein Chem Struct Biol (2021) 126:91–122. doi: 10.1016/bs.apcsb.2021.01.005
65. Jiang X, Stockwell BR, Conrad M. Ferroptosis: Mechanisms, biology and role in disease. Nat Rev Mol Cell Biol (2021) 22(4):266–82. doi: 10.1038/s41580-020-00324-8
66. Zhang H, Deng T, Liu R, Ning T, Yang H, Liu D, et al. CAF secreted miR-522 suppresses ferroptosis and promotes acquired chemo-resistance in gastric cancer. Mol Cancer (2020) 19(1):43. doi: 10.1186/s12943-020-01168-8
67. Battaglia AM, Chirillo R, Aversa I, Sacco A, Costanzo F, Biamonte F. Ferroptosis and cancer: Mitochondria meet the “Iron maiden” cell death. Cells (2020) 9(6). doi: 10.3390/cells9061505
68. Su Y, Zhao B, Zhou L, Zhang Z, Shen Y, Lv H, et al. Ferroptosis, a novel pharmacological mechanism of anti-cancer drugs. Cancer Lett (2020) 483:127–36. doi: 10.1016/j.canlet.2020.02.015
69. Chaudhary N, Choudhary BS, Shah SG, Khapare N, Dwivedi N, Gaikwad A, et al. Lipocalin 2 expression promotes tumor progression and therapy resistance by inhibiting ferroptosis in colorectal cancer. Int J Cancer (2021) 149(7):1495–511. doi: 10.1002/ijc.33711
70. Zhang X, Sui S, Wang L, Li H, Zhang L, Xu S, et al. Inhibition of tumor propellant glutathione peroxidase 4 induces ferroptosis in cancer cells and enhances anticancer effect of cisplatin. J Cell Physiol (2020) 235(4):3425–37. doi: 10.1002/jcp.29232
71. Zhang Q, Deng T, Zhang H, Zuo D, Zhu Q, Bai M, et al. Adipocyte-derived exosomal MTTP suppresses ferroptosis and promotes chemoresistance in colorectal cancer. Adv Sci (Weinh) (2022) 9(28):e2203357. doi: 10.1002/advs.202203357
72. Jiang F, Zhou JY, Zhang D, Liu MH, Chen YG. Artesunate induces apoptosis and autophagy in HCT116 colon cancer cells, and autophagy inhibition enhances the artesunateinduced apoptosis. Int J Mol Med (2018) 42(3):1295–304. doi: 10.3892/ijmm.2018.3712
73. Huang S, Zhang Z, Li W, Kong F, Yi P, Huang J, et al. Network pharmacology-based prediction and verification of the active ingredients and potential targets of zuojinwan for treating colorectal cancer. Drug Des Devel Ther (2020) 14:2725–40. doi: 10.2147/DDDT.S250991
74. Shakibaei M, Kraehe P, Popper B, Shayan P, Goel A, Buhrmann C. Curcumin potentiates antitumor activity of 5-fluorouracil in a 3D alginate tumor microenvironment of colorectal cancer. BMC Cancer (2015) 15:250. doi: 10.1186/s12885-015-1291-0
75. Shakibaei M, Mobasheri A, Lueders C, Busch F, Shayan P, Goel A. Curcumin enhances the effect of chemotherapy against colorectal cancer cells by inhibition of NF-kappaB and src protein kinase signaling pathways. PloS One (2013) 8(2):e57218. doi: 10.1371/journal.pone.0057218
76. Kunnumakkara AB, Diagaradjane P, Anand P, Harikumar KB, Deorukhkar A, Gelovani J, et al. Curcumin sensitizes human colorectal cancer to capecitabine by modulation of cyclin D1, COX-2, MMP-9, VEGF and CXCR4 expression in an orthotopic mouse model. Int J Cancer (2009) 125(9):2187–97. doi: 10.1002/ijc.24593
77. Kunnumakkara AB, Diagaradjane P, Guha S, Deorukhkar A, Shentu S, Aggarwal BB, et al. Curcumin sensitizes human colorectal cancer xenografts in nude mice to gamma-radiation by targeting nuclear factor-kappaB-regulated gene products. Clin Cancer Res (2008) 14(7):2128–36. doi: 10.1158/1078-0432.CCR-07-4722
78. Qi F, Wei L, Shen A, Chen Y, Lin J, Chu J, et al. Pien tze Huang inhibits the proliferation, and induces the apoptosis and differentiation of colorectal cancer stem cells via suppression of the Notch1 pathway. Oncol Rep (2016) 35(1):511–7. doi: 10.3892/or.2015.4378
79. Zhuang Q, Hong F, Shen A, Zheng L, Zeng J, Lin W, et al. Pien tze Huang inhibits tumor cell proliferation and promotes apoptosis via suppressing the STAT3 pathway in a colorectal cancer mouse model. Int J Oncol (2012) 40(5):1569–74. doi: 10.1371/journal.pone.0057218
80. Zhang W, Peng C, Yan J, Chen P, Jiang C, Sang S, et al. Sanguisorba officinalis l. suppresses 5-fluorouracil-sensitive and-resistant colorectal cancer growth and metastasis via inhibition of the wnt/beta-catenin pathway. Phytomedicine (2022) 94:153844. doi: 10.1016/j.phymed.2021.153844
81. Wang YL, Horng CT, Hsieh MT, Chen HC, Huang YS, Yang JS, et al. Autophagy and apoptotic machinery caused by polygonum cuspidatum extract in cisplatinresistant human oral cancer CAR cells. Oncol Rep (2019) 41(4):2549–57.
82. Zhang W, Lu C, Cai S, Feng Y, Shan J, Di L. Aconiti lateralis radix praeparata as potential anticancer herb: Bioactive compounds and molecular mechanisms. Front Pharmacol (2022) 13:870282. doi: 10.3389/fphar.2022.870282
83. Liu YT, Tzang BS, Yow J, Chiang YH, Huang CY, Hsu TC. Traditional Chinese medicine formula T33 inhibits the proliferation of human colorectal cancer cells by inducing autophagy. Environ Toxicol (2022) 37(5):1007–17. doi: 10.1002/tox.23460
84. Chen LH, Loong CC, Su TL, Lee YJ, Chu PM, Tsai ML, et al. Autophagy inhibition enhances apoptosis triggered by BO-1051, an n-mustard derivative, and involves the ATM signaling pathway. Biochem Pharmacol (2011) 81(5):594–605. doi: 10.1016/j.bcp.2010.12.011
85. Xu J, Gewirtz DA. Is autophagy always a barrier to cisplatin therapy? Biomolecules (2022) 12(3). doi: 10.3390/biom12030463
86. Liu Y, Yang B, Zhang L, Cong X, Liu Z, Hu Y, et al. Ginkgolic acid induces interplay between apoptosis and autophagy regulated by ROS generation in colon cancer. Biochem Biophys Res Commun (2018) 498(1):246–53. doi: 10.1016/j.bbrc.2018.01.091
87. Lin CJ, Lee CC, Shih YL, Lin TY, Wang SH, Lin YF, et al. Resveratrol enhances the therapeutic effect of temozolomide against malignant glioma in vitro and in vivo by inhibiting autophagy. Free Radic Biol Med (2012) 52(2):377–91. doi: 10.1016/j.freeradbiomed.2011.10.487
88. Meng J, Chang C, Chen Y, Bi F, Ji C, Liu W. EGCG overcomes gefitinib resistance by inhibiting autophagy and augmenting cell death through targeting ERK phosphorylation in NSCLC. Onco Targets Ther (2019) 12:6033–43. doi: 10.2147/OTT.S209441
89. Rai G, Mishra S, Suman S, Shukla Y. Resveratrol improves the anticancer effects of doxorubicin in vitro and in vivo models: A mechanistic insight. Phytomedicine (2016) 23(3):233–42. doi: 10.1016/j.phymed.2015.12.020
90. Liu L, Liu T, Tao W, Liao N, Yan Q, Li L, et al. Flavonoids from scutellaria barbata d. don exert antitumor activity in colorectal cancer through inhibited autophagy and promoted apoptosis via ATF4/sestrin2 pathway. Phytomedicine (2022) 99:154007. doi: 10.1016/j.phymed.2022.154007
91. Sun D, Tao W, Zhang F, Shen W, Tan J, Li L, et al. Trifolirhizin induces autophagy-dependent apoptosis in colon cancer via AMPK/mTOR signaling. Signal Transduct Target Ther (2020) 5(1):174. doi: 10.1038/s41392-020-00281-w
92. Zhang C, Liu X, Jin S, Chen Y, Guo R. Ferroptosis in cancer therapy: A novel approach to reversing drug resistance. Mol Cancer (2022) 21(1):47. doi: 10.1186/s12943-022-01530-y
93. Sharma P, Shimura T, Banwait JK, Goel A. Andrographis-mediated chemosensitization through activation of ferroptosis and suppression of beta-catenin/Wnt-signaling pathways in colorectal cancer. Carcinogenesis (2020) 41(10):1385–94. doi: 10.1093/carcin/bgaa090
94. Chen Y, Zhang F, Du Z, Xie J, Xia L, Hou X, et al. Proteome analysis of camellia nitidissima chi revealed its role in colon cancer through the apoptosis and ferroptosis pathway. Front Oncol (2021) 11:727130. doi: 10.3389/fonc.2021.727130
95. Chen P, Li X, Zhang R, Liu S, Xiang Y, Zhang M, et al. Combinative treatment of beta-elemene and cetuximab is sensitive to KRAS mutant colorectal cancer cells by inducing ferroptosis and inhibiting epithelial-mesenchymal transformation. Theranostics (2020) 10(11):5107–19. doi: 10.7150/thno.44705
96. Wang CX, Chen LH, Zhuang HB, Shi ZS, Chen ZC, Pan JP, et al. Auriculasin enhances ROS generation to regulate colorectal cancer cell apoptosis, ferroptosis, oxeiptosis, invasion and colony formation. Biochem Biophys Res Commun (2022) 587:99–106. doi: 10.1016/j.bbrc.2021.11.101
97. Chan DW, Yung MM, Chan YS, Xuan Y, Yang H, Xu D, et al. MAP30 protein from momordica charantia is therapeutic and has synergic activity with cisplatin against ovarian cancer in vivo by altering metabolism and inducing ferroptosis. Pharmacol Res (2020) 161:105157. doi: 10.1016/j.phrs.2020.105157
98. Li Q, Su R, Bao X, Cao K, Du Y, Wang N, et al. Glycyrrhetinic acid nanoparticles combined with ferrotherapy for improved cancer immunotherapy. Acta Biomater (2022) 144:109–20. doi: 10.1016/j.actbio.2022.03.030
99. Durinikova E, Plava J, Tyciakova S, Skvara P, Vojs Stanova A, Kozovska Z, et al. Cytotoxic response of 5-fluorouracil-resistant cells to gene- and cell-directed enzyme/prodrug treatment. Cancer Gene Ther (2018) 25(11-12):285–99. doi: 10.1038/s41417-018-0030-5
100. Yang C, Song J, Hwang S, Choi J, Song G, Lim W. Apigenin enhances apoptosis induction by 5-fluorouracil through regulation of thymidylate synthase in colorectal cancer cells. Redox Biol (2021) 47:102144. doi: 10.1016/j.redox.2021.102144
101. Furukawa T, Tabata S, Yamamoto M, Kawahara K, Shinsato Y, Minami K, et al. Thymidine phosphorylase in cancer aggressiveness and chemoresistance. Pharmacol Res (2018) 132:15–20. doi: 10.1016/j.phrs.2018.03.019
102. Bronckaers A, Gago F, Balzarini J, Liekens S. The dual role of thymidine phosphorylase in cancer development and chemotherapy. Med Res Rev (2009) 29(6):903–53. doi: 10.1002/med.20159
103. Zhang Q, Zhang Y, Hu X, Qin Y, Zhong W, Meng J, et al. Thymidine phosphorylase promotes metastasis and serves as a marker of poor prognosis in hepatocellular carcinoma. Lab Invest (2017) 97(8):903–12. doi: 10.1038/labinvest.2017.51
104. Cecchini M, Kortmansky JS, Cui C, Wei W, Thumar JR, Uboha NV, et al. A phase 1b expansion study of TAS-102 with oxaliplatin for refractory metastatic colorectal cancer. Cancer (2021) 127(9):1417–24. doi: 10.1002/cncr.33379
105. Chakrabarti S, Wintheiser G, Tella SH, Oxencis C, Mahipal A. TAS-102: A resurrected novel fluoropyrimidine with expanding role in the treatment of gastrointestinal malignancies. Pharmacol Ther (2021) 224:107823. doi: 10.1016/j.pharmthera.2021.107823
106. Yoshida Y, Yamada T, Kamiyama H, Kosugi C, Ishibashi K, Yoshida H, et al. Combination of TAS-102 and bevacizumab as third-line treatment for metastatic colorectal cancer: TAS-CC3 study. Int J Clin Oncol (2021) 26(1):111–7. doi: 10.1007/s10147-020-01794-8
107. Chai J, Dong W, Xie C, Wang L, Han DL, Wang S, et al. MicroRNA-494 sensitizes colon cancer cells to fluorouracil through regulation of DPYD. IUBMB Life (2015) 67(3):191–201. doi: 10.1002/iub.1361
108. Salonga D, Danenberg KD, Johnson M, Metzger R, Groshen S, Tsao-Wei DD, et al. Colorectal tumors responding to 5-fluorouracil have low gene expression levels of dihydropyrimidine dehydrogenase, thymidylate synthase, and thymidine phosphorylase. Clin Cancer Res (2000) 6(4):1322–7.
109. Zhang YH, Luo DD, Wan SB, Qu XJ. S1PR2 inhibitors potently reverse 5-FU resistance by downregulating DPD expression in colorectal cancer. Pharmacol Res (2020) 155:104717. doi: 10.1016/j.phrs.2020.104717
110. Omura K. Clinical implications of dihydropyrimidine dehydrogenase (DPD) activity in 5-FU-based chemotherapy: Mutations in the DPD gene, and DPD inhibitory fluoropyrimidines. Int J Clin Oncol (2003) 8(3):132–8. doi: 10.1007/s10147-003-0330-z
111. Buck E, Sprick M, Gaida MM, Grullich C, Weber TF, Herpel E, et al. Tumor response to irinotecan is associated with CYP3A5 expression in colorectal cancer. Oncol Lett (2019) 17(4):3890–8. doi: 10.3892/ol.2019.10043
112. Olszewski U, Liedauer R, Ausch C, Thalhammer T, Hamilton G. Overexpression of CYP3A4 in a COLO 205 colon cancer stem cell model in vitro. Cancers (Basel) (2011) 3(1):1467–79. doi: 10.3390/cancers3011467
113. Untereiner AA, Pavlidou A, Druzhyna N, Papapetropoulos A, Hellmich MR, Szabo C. Drug resistance induces the upregulation of H2S-producing enzymes in HCT116 colon cancer cells. Biochem Pharmacol (2018) 149:174–85. doi: 10.1016/j.bcp.2017.10.007
114. Pljesa-Ercegovac M, Savic-Radojevic A, Matic M, Coric V, Djukic T, Radic T, et al. Glutathione transferases: Potential targets to overcome chemoresistance in solid tumors. Int J Mol Sci (2018) 19(12). doi: 10.3390/ijms19123785
115. Singh RR, Reindl KM. Glutathione s-transferases in cancer. Antioxid (Basel) (2021) 10(5). doi: 10.3390/antiox10050701
116. Liu H, Liu H, Zhou Z, Parise RA, Chu E, Schmitz JC. Herbal formula Huang qin Ge gen tang enhances 5-fluorouracil antitumor activity through modulation of the E2F1/TS pathway. Cell Commun Signal (2018) 16(1):7. doi: 10.1186/s12964-018-0218-1
117. Yang A, Wu Q, Chen Q, Yang J, Li H, Tao Y, et al. Cinobufagin restrains the growth and triggers DNA damage of human hepatocellular carcinoma cells via proteasome-dependent degradation of thymidylate synthase. Chem Biol Interact (2022) 360:109938. doi: 10.1016/j.cbi.2022.109938
118. Yu C, Liu SL, Qi MH, Zou X, Wu J, Zhang J. Herbal medicine guan Chang fu fang enhances 5-fluorouracil cytotoxicity and affects drug-associated genes in human colorectal carcinoma cells. Oncol Lett (2015) 9(2):701–8. doi: 10.3892/ol.2014.2766
119. Kang YH, Lee JS, Lee NH, Kim SH, Seo CS, Son CG. Coptidis rhizoma extract reverses 5-fluorouracil resistance in HCT116 human colorectal cancer cells via modulation of thymidylate synthase. Molecules (2021) 26(7). doi: 10.3390/molecules26071856
120. Ko JC, Tsai MS, Kuo YH, Chiu YF, Weng SH, Su YC, et al. Modulation of Rad51, ERCC1, and thymidine phosphorylase by emodin result in synergistic cytotoxic effect in combination with capecitabine. Biochem Pharmacol (2011) 81(5):680–90. doi: 10.1016/j.bcp.2010.12.008
121. Chen L, Cai J, Wang S, Hu L, Yang X. Effect of radix sophorae flavescentis on activity of CYP450 isoforms in rats. Int J Clin Exp Med (2015) 8(11):21365–71.
122. Wang B, Yang S, Hu J, Li Y. Multifaceted interaction of the traditional Chinese medicinal herb schisandra chinensis with cytochrome P450-mediated drug metabolism in rats. J Ethnopharmacol (2014) 155(3):1473–82. doi: 10.1016/j.jep.2014.07.026
123. Yim D, Kim MJ, Shin Y, Lee SJ, Shin JG, Kim DH. Inhibition of cytochrome P450 activities by sophora flavescens extract and its prenylated flavonoids in human liver microsomes. Evid Based Complement Alternat Med (2019) 2019:2673769. doi: 10.1155/2019/2673769
124. Ueng YF, Shyu CC, Lin YL, Park SS, Liao JF, Chen CF. Effects of baicalein and wogonin on drug-metabolizing enzymes in C57BL/6J mice. Life Sci (2000) 67(18):2189–200. doi: 10.1016/S0024-3205(00)00809-2
125. Wei W, Li Z, Li HJ, An Y, Qu H, Yao C, et al. The inhibitory effect of 225 frequently-used traditional Chinese medicines for CYP3A4 metabolic enzyme by isoform-specific probe. Fitoterapia (2021) 152:104858. doi: 10.1016/j.fitote.2021.104858
126. Babaei G, Aziz SG, Jaghi NZZ. EMT. Cancer stem cells and autophagy; the three main axes of metastasis. BioMed Pharmacother (2021) 133:110909. doi: 10.1016/j.biopha.2020.110909
127. Cho ES, Kang HE, Kim NH, Yook JI. Therapeutic implications of cancer epithelial-mesenchymal transition (EMT). Arch Pharm Res (2019) 42(1):14–24. doi: 10.1007/s12272-018-01108-7
128. Skarkova V, Skarka A, Manethova M, Stefanidi AA, Rudolf E. Silencing of e-cadherin expression leads to increased chemosensitivity to irinotecan and oxaliplatin in colorectal cancer cell lines. Hum Exp Toxicol (2021) 40(12):2063–73. doi: 10.1177/09603271211021479
129. Fortunato A. The role of hERG1 ion channels in epithelial-mesenchymal transition and the capacity of riluzole to reduce cisplatin resistance in colorectal cancer cells. Cell Oncol (Dordr) (2017) 40(4):367–78. doi: 10.1007/s13402-017-0328-6
130. Zhang Y, Devocelle A, Desterke C, de Souza LEB, Hadadi E, Acloque H, et al. BMAL1 knockdown leans epithelial-mesenchymal balance toward epithelial properties and decreases the chemoresistance of colon carcinoma cells. Int J Mol Sci (2021) 22(10). doi: 10.3390/ijms22105247
131. Sreekumar R, Al-Saihati H, Emaduddin M, Moutasim K, Mellone M, Patel A, et al. The ZEB2-dependent EMT transcriptional programme drives therapy resistance by activating nucleotide excision repair genes ERCC1 and ERCC4 in colorectal cancer. Mol Oncol (2021) 15(8):2065–83. doi: 10.1002/1878-0261.12965
132. Erin N, Grahovac J, Brozovic A, Efferth T. Tumor microenvironment and epithelial mesenchymal transition as targets to overcome tumor multidrug resistance. Drug Resist Updat (2020) 53:100715. doi: 10.1016/j.drup.2020.100715
133. Roy S, Sunkara RR, Parmar MY, Shaikh S, Waghmare SK. EMT imparts cancer stemness and plasticity: new perspectives and therapeutic potential. Front Biosci (Landmark Ed) (2021) 26(2):238–65. doi: 10.2741/4893
134. Li N, Babaei-Jadidi R, Lorenzi F, Spencer-Dene B, Clarke P, Domingo E, et al. An FBXW7-ZEB2 axis links EMT and tumour microenvironment to promote colorectal cancer stem cells and chemoresistance. Oncogenesis (2019) 8(3):13. doi: 10.1038/s41389-019-0125-3
135. Hu JL, Wang W, Lan XL, Zeng ZC, Liang YS, Yan YR, et al. CAFs secreted exosomes promote metastasis and chemotherapy resistance by enhancing cell stemness and epithelial-mesenchymal transition in colorectal cancer. Mol Cancer (2019) 18(1):91. doi: 10.1186/s12943-019-1019-x
136. Lu Y, Zhang R, Zhang X, Zhang B, Yao Q. Curcumin may reverse 5-fluorouracil resistance on colonic cancer cells by regulating TET1-NKD-Wnt signal pathway to inhibit the EMT progress. BioMed Pharmacother (2020) 129:110381. doi: 10.1016/j.biopha.2020.110381
137. Yin J, Wang L, Wang Y, Shen H, Wang X, Wu L. Curcumin reverses oxaliplatin resistance in human colorectal cancer via regulation of TGF-beta/Smad2/3 signaling pathway. Onco Targets Ther (2019) 12:3893–903. doi: 10.2147/OTT.S199601
138. Toden S, Okugawa Y, Jascur T, Wodarz D, Komarova NL, Buhrmann C, et al. Curcumin mediates chemosensitization to 5-fluorouracil through miRNA-induced suppression of epithelial-to-mesenchymal transition in chemoresistant colorectal cancer. Carcinogenesis (2015) 36(3):355–67. doi: 10.1093/carcin/bgv006
139. Wu CE, Zhuang YW, Zhou JY, Liu SL, Wang RP, Shu P. Cinnamaldehyde enhances apoptotic effect of oxaliplatin and reverses epithelial-mesenchymal transition and stemnness in hypoxic colorectal cancer cells. Exp Cell Res (2019) 383(1):111500. doi: 10.1016/j.yexcr.2019.111500
140. Cao Y, Lu K, Xia Y, Wang Y, Wang A, Zhao Y. Danshensu attenuated epithelial-mesenchymal transformation and chemoresistance of colon cancer cells induced by platelets. Front Biosci (Landmark Ed) (2022) 27(5):160. doi: 10.31083/j.fbl2705160
141. Yuan L, Zhang K, Zhou MM, Wasan HS, Tao FF, Yan QY, et al. Jiedu sangen decoction reverses epithelial-to-mesenchymal transition and inhibits invasion and metastasis of colon cancer via AKT/GSK-3beta signaling pathway. J Cancer (2019) 10(25):6439–56. doi: 10.7150/jca.32873
142. Zhang K, Peng T, Yan Q, Sun L, Miao H, Yuan L, et al. Jiedu sangen decoction inhibits migration and invasion of colon cancer SW480 cells via suppressing epithelial mesenchymal transition. Evid Based Complement Alternat Med (2018) 2018:1495768. doi: 10.1155/2018/1495768
143. Buhrmann C, Shayan P, Kraehe P, Popper B, Goel A, Shakibaei M. Resveratrol induces chemosensitization to 5-fluorouracil through up-regulation of intercellular junctions, epithelial-to-mesenchymal transition and apoptosis in colorectal cancer. Biochem Pharmacol (2015) 98(1):51–68. doi: 10.1016/j.bcp.2015.08.105
144. Buhrmann C, Yazdi M, Popper B, Shayan P, Goel A, Aggarwal BB, et al. Resveratrol Chemosensitizes TNF-β-Induced Survival of 5-FU-Treated Colorectal Cancer Cells. Resveratrol Chemosensitizes TNF-beta-Induced Survival 5-FU-Treated Colorectal Cancer Cells Nutrients (2018) 10(7):888. doi: 10.3390/nu10070888
145. Ji Q, Liu X, Han Z, Zhou L, Sui H, Yan L, et al. Resveratrol suppresses epithelial-to-mesenchymal transition in colorectal cancer through TGF-beta1/Smads signaling pathway mediated Snail/E-cadherin expression. BMC Cancer (2015) 15:97. doi: 10.1186/s12885-015-1119-y
146. Feng J, Song D, Jiang S, Yang X, Ding T, Zhang H, et al. Quercetin restrains TGF-beta1-induced epithelial-mesenchymal transition by inhibiting Twist1 and regulating e-cadherin expression. Biochem Biophys Res Commun (2018) 498(1):132–8. doi: 10.1016/j.bbrc.2018.02.044
147. Ye Q, Su L, Chen D, Zheng W, Liu Y. Astragaloside IV induced miR-134 expression reduces EMT and increases chemotherapeutic sensitivity by suppressing CREB1 signaling in colorectal cancer cell line SW-480. Cell Physiol Biochem (2017) 43(4):1617–26. doi: 10.1159/000482025
148. Jarosz-Biej M, Smolarczyk R, Cichon T, Kulach N. Tumor microenvironment as a “Game changer” in cancer radiotherapy. Int J Mol Sci (2019) 20(13). doi: 10.3390/ijms20133212
149. Riera-Domingo C, Audige A, Granja S, Cheng WC, Ho PC, Baltazar F, et al. Immunity, hypoxia, and metabolism-the menage a trois of cancer: Implications for immunotherapy. Physiol Rev (2020) 100(1):1–102. doi: 10.1152/physrev.00018.2019
150. Roma-Rodrigues C, Mendes R, Baptista PV, Fernandes AR. Targeting tumor microenvironment for cancer therapy. Int J Mol Sci (2019) 20(4). doi: 10.3390/ijms20040840
151. Zhang S, Ji WW, Wei W, Zhan LX, Huang X. Long noncoding RNA Meg3 sponges miR-708 to inhibit intestinal tumorigenesis via SOCS3-repressed cancer stem cells growth. Cell Death Dis (2021) 13(1):25. doi: 10.1038/s41419-021-04470-5
152. Bharti R, Dey G, Mandal M. Cancer development, chemoresistance, epithelial to mesenchymal transition and stem cells: A snapshot of IL-6 mediated involvement. Cancer Lett (2016) 375(1):51–61. doi: 10.1016/j.canlet.2016.02.048
153. Chen Y, Tan W, Wang C. Tumor-associated macrophage-derived cytokines enhance cancer stem-like characteristics through epithelial-mesenchymal transition. Onco Targets Ther (2018) 11:3817–26. doi: 10.2147/OTT.S168317
154. Jinushi M, Chiba S, Yoshiyama H, Masutomi K, Kinoshita I, Dosaka-Akita H, et al. Tumor-associated macrophages regulate tumorigenicity and anticancer drug responses of cancer stem/initiating cells. Proc Natl Acad Sci U S A (2011) 108(30):12425–30. doi: 10.1073/pnas.1106645108
155. Ren J, Ding L, Zhang D, Shi G, Xu Q, Shen S, et al. Carcinoma-associated fibroblasts promote the stemness and chemoresistance of colorectal cancer by transferring exosomal lncRNA H19. Theranostics (2018) 8(14):3932–48. doi: 10.7150/thno.25541
156. Weng X, Liu H, Ruan J, Du M, Wang L, Mao J, et al. HOTAIR/miR-1277-5p/ZEB1 axis mediates hypoxia-induced oxaliplatin resistance via regulating epithelial-mesenchymal transition in colorectal cancer. Cell Death Discovery (2022) 8(1):310. doi: 10.1038/s41420-022-01096-0
157. Zhang Y, Lou Y, Wang J, Yu C, Shen W. Research status and molecular mechanism of the traditional Chinese medicine and antitumor therapy combined strategy based on tumor microenvironment. Front Immunol (2020) 11:609705. doi: 10.3389/fimmu.2020.609705
158. Duan Y, Zhu J, Yang J, Gu W, Bai X, Liu G, et al. A decade’s review of miRNA: A center of transcriptional regulation of drugmetabolizing enzymes and transporters under hypoxia. Curr Drug Metab (2021) 22(9):709–25. doi: 10.2174/1389200222666210514011313
159. Patel BB, Sengupta R, Qazi S, Vachhani H, Yu Y, Rishi AK, et al. Curcumin enhances the effects of 5-fluorouracil and oxaliplatin in mediating growth inhibition of colon cancer cells by modulating EGFR and IGF-1R. Int J Cancer (2008) 122(2):267–73. doi: 10.1002/ijc.23097
160. Lam W, Bussom S, Guan F, Jiang Z, Zhang W, Gullen EA, et al. The four-herb Chinese medicine PHY906 reduces chemotherapy-induced gastrointestinal toxicity. Sci Transl Med (2010) 2(45):45ra59. doi: 10.1126/scitranslmed.3001270
161. Lv J, Jia Y, Li J, Kuai W, Li Y, Guo F, et al. Gegen qinlian decoction enhances the effect of PD-1 blockade in colorectal cancer with microsatellite stability by remodelling the gut microbiota and the tumour microenvironment. Cell Death Dis (2019) 10(6):415. doi: 10.1038/s41419-019-1638-6
162. Liu J, Wang Y, Qiu Z, Lv G, Huang X, Lin H, et al. Impact of TCM on tumor-infiltrating myeloid precursors in the tumor microenvironment. Front Cell Dev Biol (2021) 9:635122. doi: 10.3389/fcell.2021.635122
163. Sui H, Zhao J, Zhou L, Wen H, Deng W, Li C, et al. Tanshinone IIA inhibits beta-catenin/VEGF-mediated angiogenesis by targeting TGF-beta1 in normoxic and HIF-1alpha in hypoxic microenvironments in human colorectal cancer. Cancer Lett (2017) 403:86–97. doi: 10.1016/j.canlet.2017.05.013
164. Zhang T, Wang K, Zhang J, Wang X, Chen Z, Ni C, et al. Huaier aqueous extract inhibits colorectal cancer stem cell growth partially via downregulation of the wnt/beta-catenin pathway. Oncol Lett (2013) 5(4):1171–6. doi: 10.3892/ol.2013.1145
165. Liu Y, Liu X, Zhang N, Yin M, Dong J, Zeng Q, et al. Berberine diminishes cancer cell PD-L1 expression and facilitates antitumor immunity via inhibiting the deubiquitination activity of CSN5. Acta Pharm Sin B (2020) 10(12):2299–312. doi: 10.1016/j.apsb.2020.06.014
166. Liu W, Fan T, Li M, Zhang G, Guo W, Yang X, et al. Andrographolide potentiates PD-1 blockade immunotherapy by inhibiting COX2-mediated PGE2 release. Int Immunopharmacol (2020) 81:106206. doi: 10.1016/j.intimp.2020.106206
167. Deng B, Yang B, Chen J, Wang S, Zhang W, Guo Y, et al. Gallic Acid induces T-helper-1-like treg cells and strengthens immune checkpoint blockade efficacy. J Immunother Cancer (2022) 10(7). doi: 10.1136/jitc-2021-004037
168. Mao Q, Min J, Zeng R, Liu H, Li H, Zhang C, et al. Self-assembled traditional Chinese nanomedicine modulating tumor immunosuppressive microenvironment for colorectal cancer immunotherapy. Theranostics (2022) 12(14):6088–105. doi: 10.7150/thno.72509
169. Crea F, Nobili S, Paolicchi E, Perrone G, Napoli C, Landini I, et al. Epigenetics and chemoresistance in colorectal cancer: An opportunity for treatment tailoring and novel therapeutic strategies. Drug Resist Updat (2011) 14(6):280–96. doi: 10.1016/j.drup.2011.08.001
170. Li B, Jiang J, Assaraf YG, Xiao H, Chen ZS, Huang C. Surmounting cancer drug resistance: New insights from the perspective of N(6)-methyladenosine RNA modification. Drug Resist Updat (2020) 53:100720. doi: 10.1016/j.drup.2020.100720
171. Isono M, Nakano M, Fukami T, Nakajima M. Adenosine N(6)-methylation upregulates the expression of human CYP2B6 by altering the chromatin status. Biochem Pharmacol (2022) 205:115247. doi: 10.1016/j.bcp.2022.115247
172. Yang Z, Zhao F, Gu X, Feng L, Xu M, Li T, et al. Binding of RNA m6A by IGF2BP3 triggers chemoresistance of HCT8 cells via upregulation of ABCB1. Am J Cancer Res (2021) 11(4):1428–45.
173. Uddin MB, Roy KR, Hosain SB, Khiste SK, Hill RA, Jois SD, et al. An N(6)-methyladenosine at the transited codon 273 of p53 pre-mRNA promotes the expression of R273H mutant protein and drug resistance of cancer cells. Biochem Pharmacol (2019) 160:134–45. doi: 10.1016/j.bcp.2018.12.014
174. Ye P, Xing H, Lou F, Wang K, Pan Q, Zhou X, et al. Histone deacetylase 2 regulates doxorubicin (Dox) sensitivity of colorectal cancer cells by targeting ABCB1 transcription. Cancer Chemother Pharmacol (2016) 77(3):613–21. doi: 10.1007/s00280-016-2979-9
175. Hirukawa A, Singh S, Wang J, Rennhack JP, Swiatnicki M, Sanguin-Gendreau V, et al. Reduction of global H3K27me(3) enhances HER2/ErbB2 targeted therapy. Cell Rep (2019) 29(2):249–57 e8. doi: 10.1016/j.celrep.2019.08.105
176. Ma Y, Yang Y, Wang F, Moyer MP, Wei Q, Zhang P, et al. Long non-coding RNA CCAL regulates colorectal cancer progression by activating wnt/beta-catenin signalling pathway via suppression of activator protein 2alpha. Gut (2016) 65(9):1494–504. doi: 10.1136/gutjnl-2014-308392
177. Chen P, Liu XQ, Lin X, Gao LY, Zhang S, Huang X. Targeting YTHDF1 effectively re-sensitizes cisplatin-resistant colon cancer cells by modulating GLS-mediated glutamine metabolism. Mol Ther Oncolytics (2021) 20:228–39. doi: 10.1016/j.omto.2021.01.001
178. Chen Z, Wu L, Zhou J, Lin X, Peng Y, Ge L, et al. N6-methyladenosine-induced ERRgamma triggers chemoresistance of cancer cells through upregulation of ABCB1 and metabolic reprogramming. Theranostics (2020) 10(8):3382–96. doi: 10.7150/thno.40144
179. Gao Y, Wang H, Chen S, An R, Chu Y, Li G, et al. Single-cell N(6)-methyladenosine regulator patterns guide intercellular communication of tumor microenvironment that contribute to colorectal cancer progression and immunotherapy. J Transl Med (2022) 20(1):197. doi: 10.1186/s12967-022-03395-7
180. Straussman R, Morikawa T, Shee K, Barzily-Rokni M, Qian ZR, Du J, et al. Tumour micro-environment elicits innate resistance to RAF inhibitors through HGF secretion. Nature (2012) 487(7408):500–4. doi: 10.1038/nature11183
181. Wu Y, Wang Z, Han L, Guo Z, Yan B, Guo L, et al. PRMT5 regulates RNA m6A demethylation for doxorubicin sensitivity in breast cancer. Mol Ther (2022) 30(7):2603–17. doi: 10.1016/j.ymthe.2022.03.003
182. Giri AK, Aittokallio T. DNMT inhibitors increase methylation in the cancer genome. Front Pharmacol (2019) 10:385. doi: 10.3389/fphar.2019.00385
183. Guo Y, Shu L, Zhang C, Su ZY, Kong AN. Curcumin inhibits anchorage-independent growth of HT29 human colon cancer cells by targeting epigenetic restoration of the tumor suppressor gene DLEC1. Biochem Pharmacol (2015) 94(2):69–78. doi: 10.1016/j.bcp.2015.01.009
184. Jiang A, Wang X, Shan X, Li Y, Wang P, Jiang P, et al. Curcumin reactivates silenced tumor suppressor gene RARbeta by reducing DNA methylation. Phytother Res (2015) 29(8):1237–45. doi: 10.1002/ptr.5373
185. Hosokawa M, Seiki R, Iwakawa S, Ogawara KI. Combination of azacytidine and curcumin is a potential alternative in decitabine-resistant colorectal cancer cells with attenuated deoxycytidine kinase. Biochem Biophys Res Commun (2021) 578:157–62. doi: 10.1016/j.bbrc.2021.09.041
186. Pan FP, Zhou HK, Bu HQ, Chen ZQ, Zhang H, Xu LP, et al. Emodin enhances the demethylation by 5-Aza-CdR of pancreatic cancer cell tumor-suppressor genes P16, RASSF1A and ppENK. Oncol Rep (2016) 35(4):1941–9. doi: 10.3892/or.2016.4554
187. Anantharaju PG, Reddy DB, Padukudru MA, Chitturi CMK, Vimalambike MG, Madhunapantula SV. Induction of colon and cervical cancer cell death by cinnamic acid derivatives is mediated through the inhibition of histone deacetylases (HDAC). PloS One (2017) 12(11):e0186208. doi: 10.1371/journal.pone.0186208
188. Zhao Z, Zeng J, Guo Q, Pu K, Yang Y, Chen N, et al. Berberine suppresses stemness and tumorigenicity of colorectal cancer stem-like cells by inhibiting m(6)A methylation. Front Oncol (2021) 11:775418. doi: 10.3389/fonc.2021.775418
189. An P, Lu D, Zhang L, Lan H, Yang H, Ge G, et al. Synergistic antitumor effects of compound-composed optimal formula from aidi injection on hepatocellular carcinoma and colorectal cancer. Phytomedicine (2022) 103:154231. doi: 10.1016/j.phymed.2022.154231
190. Pavlova NN, Zhu J, Thompson CB. The hallmarks of cancer metabolism: Still emerging. Cell Metab (2022) 34(3):355–77. doi: 10.1016/j.cmet.2022.01.007
191. Tan Y, Li J, Zhao G, Huang KC, Cardenas H, Wang Y, et al. Metabolic reprogramming from glycolysis to fatty acid uptake and beta-oxidation in platinum-resistant cancer cells. Nat Commun (2022) 13(1):4554. doi: 10.1038/s41467-022-32101-w
192. Yang R, Yi M, Xiang B. Novel insights on lipid metabolism alterations in drug resistance in cancer. Front Cell Dev Biol (2022) 10:875318. doi: 10.3389/fcell.2022.875318
193. Chen X, Chen S, Yu D. Metabolic reprogramming of chemoresistant cancer cells and the potential significance of metabolic regulation in the reversal of cancer chemoresistance. Metabolites (2020) 10(7). doi: 10.3390/metabo10070289
194. Shi T, Ma Y, Cao L, Zhan S, Xu Y, Fu F, et al. B7-H3 promotes aerobic glycolysis and chemoresistance in colorectal cancer cells by regulating HK2. Cell Death Dis (2019) 10(4):308. doi: 10.1038/s41419-019-1549-6
195. Zhang X, Li Q, Du A, Li Y, Shi Q, Chen Y, et al. Adipocytic glutamine synthetase upregulation via altered histone methylation promotes 5FU chemoresistance in peritoneal carcinomatosis of colorectal cancer. Front Oncol (2021) 11:748730. doi: 10.3389/fonc.2021.748730
196. Dong S, Liang S, Cheng Z, Zhang X, Luo L, Li L, et al. ROS/PI3K/Akt and wnt/beta-catenin signalings activate HIF-1alpha-induced metabolic reprogramming to impart 5-fluorouracil resistance in colorectal cancer. J Exp Clin Cancer Res (2022) 41(1):15. doi: 10.1186/s13046-021-02229-6
197. Hong X, Zhong L, Xie Y, Zheng K, Pang J, Li Y, et al. Matrine reverses the warburg effect and suppresses colon cancer cell growth via negatively regulating HIF-1alpha. Front Pharmacol (2019) 10:1437. doi: 10.3389/fphar.2019.01437
198. Ji L, Shen W, Zhang F, Qian J, Jiang J, Weng L, et al. Worenine reverses the warburg effect and inhibits colon cancer cell growth by negatively regulating HIF-1alpha. Cell Mol Biol Lett (2021) 26(1):19. doi: 10.1186/s11658-021-00263-y
199. Wu H, Du J, Li C, Li H, Guo H, Li Z. Kaempferol can reverse the 5-fu resistance of colorectal cancer cells by inhibiting PKM2-mediated glycolysis. Int J Mol Sci (2022) 23(7). doi: 10.3390/ijms23073544
200. Sun LT, Zhang LY, Shan FY, Shen MH, Ruan SM. Jiedu sangen decoction inhibits chemoresistance to 5-fluorouracil of colorectal cancer cells by suppressing glycolysis via PI3K/AKT/HIF-1alpha signaling pathway. Chin J Nat Med (2021) 19(2):143–52. doi: 10.1016/S1875-5364(21)60015-8
201. Wang Y, Guo D, He J, Song L, Chen H, Zhang Z, et al. Inhibition of fatty acid synthesis arrests colorectal neoplasm growth and metastasis: Anti-cancer therapeutical effects of natural cyclopeptide RA-XII. Biochem Biophys Res Commun (2019) 512(4):819–24. doi: 10.1016/j.bbrc.2019.03.088
202. Ghosh S, Singh R, Vanwinkle ZM, Guo H, Vemula PK, Goel A, et al. Microbial metabolite restricts 5-fluorouracil-resistant colonic tumor progression by sensitizing drug transporters via regulation of FOXO3-FOXM1 axis. Theranostics (2022) 12(12):5574–95. doi: 10.7150/thno.70754
203. Kim HJ, An J, Ha EM. Lactobacillus plantarum-derived metabolites sensitize the tumor-suppressive effects of butyrate by regulating the functional expression of SMCT1 in 5-FU-resistant colorectal cancer cells. J Microbiol (2022) 60(1):100–17. doi: 10.1007/s12275-022-1533-1
204. Wang Z, Hopson LM, Singleton SS, Yang X, Jogunoori W, Mazumder R, et al. Mice with dysfunctional TGF-beta signaling develop altered intestinal microbiome and colorectal cancer resistant to 5FU. Biochim Biophys Acta Mol Basis Dis (2021) 1867(10):166179. doi: 10.1016/j.bbadis.2021.166179
205. Liu H, Du J, Chao S, Li S, Cai H, Zhang H, et al. Fusobacterium nucleatum promotes colorectal cancer cell to acquire stem cell-like features by manipulating lipid droplet-mediated numb degradation. Adv Sci (Weinh) (2022) 9(12):e2105222. doi: 10.1002/advs.202105222
206. Yu T, Guo F, Yu Y, Sun T, Ma D, Han J, et al. Fusobacterium nucleatum promotes chemoresistance to colorectal cancer by modulating autophagy. Cell (2017) 170(3):548–63 e16. doi: 10.1016/j.cell.2017.07.008
207. Roy S, Trinchieri G. Microbiota: A key orchestrator of cancer therapy. Nat Rev Cancer (2017) 17(5):271–85. doi: 10.1038/nrc.2017.13
208. Li Y, Li ZX, Xie CY, Fan J, Lv J, Xu XJ, et al. Gegen qinlian decoction enhances immunity and protects intestinal barrier function in colorectal cancer patients via gut microbiota. World J Gastroenterol (2020) 26(48):7633–51. doi: 10.3748/wjg.v26.i48.7633
209. Yu YN, Yu TC, Zhao HJ, Sun TT, Chen HM, Chen HY, et al. Berberine may rescue fusobacterium nucleatum-induced colorectal tumorigenesis by modulating the tumor microenvironment. Oncotarget (2015) 6(31):32013–26. doi: 10.18632/oncotarget.5166
210. Jiang F, Liu M, Wang H, Shi G, Chen B, Chen T, et al. Wu Mei wan attenuates CAC by regulating gut microbiota and the NF-kB/IL6-STAT3 signaling pathway. BioMed Pharmacother (2020) 125:109982. doi: 10.1016/j.biopha.2020.109982
211. Luo Y, Wang CZ, Sawadogo R, Yuan J, Zeng J, Xu M, et al. 4-vinylguaiacol, an active metabolite of ferulic acid by enteric microbiota and probiotics, possesses significant activities against drug-resistant human colorectal cancer cells. ACS Omega (2021) 6(7):4551–61. doi: 10.1021/acsomega.0c04394
212. Sui H, Zhang L, Gu K, Chai N, Ji Q, Zhou L, et al. YYFZBJS ameliorates colorectal cancer progression in Apc(Min/+) mice by remodeling gut microbiota and inhibiting regulatory T-cell generation. Cell Commun Signal (2020) 18(1):113. doi: 10.1186/s12964-020-00596-9
213. Engle K, Kumar G. Cancer multidrug-resistance reversal by ABCB1 inhibition: A recent update. Eur J Med Chem (2022) 239:114542. doi: 10.1016/j.ejmech.2022.114542
214. Hogg SJ, Beavis PA, Dawson MA, Johnstone RW. Targeting the epigenetic regulation of antitumour immunity. Nat Rev Drug Discovery (2020) 19(11):776–800. doi: 10.1038/s41573-020-0077-5
215. de Oliveira Junior RG, Christiane Adrielly AF, da Silva Almeida JRG, Grougnet R, Thiery V, Picot L. Sensitization of tumor cells to chemotherapy by natural products: A systematic review of preclinical data and molecular mechanisms. Fitoterapia (2018) 129:383–400. doi: 10.1016/j.fitote.2018.02.025
216. Zheng Y, Wang Y, Xia M, Gao Y, Zhang L, Song Y, et al. The combination of nanotechnology and traditional Chinese medicine (TCM) inspires the modernization of TCM: review on nanotechnology in TCM-based drug delivery systems. Drug Delivery Transl Res (2022) 12(6):1306–25. doi: 10.1007/s13346-021-01029-x
217. Wei D, Yang H, Zhang Y, Zhang X, Wang J, Wu X, et al. Nano-traditional Chinese medicine: a promising strategy and its recent advances. J Mater Chem B (2022) 10(16):2973–94. doi: 10.1039/D2TB00225F
218. Song JW, Liu YS, Guo YR, Zhong WX, Guo YP, Guo L. Nano-liposomes double loaded with curcumin and tetrandrine: Preparation, characterization, hepatotoxicity and anti-tumor effects. Int J Mol Sci (2022) 23(12). doi: 10.3390/ijms23126858
Keywords: traditional Chinese medicine, colorectal cancer, chemoresistance, sensitizer, chemotherapy
Citation: Lin X, Yang X, Yang Y, Zhang H and Huang X (2023) Research progress of traditional Chinese medicine as sensitizer in reversing chemoresistance of colorectal cancer. Front. Oncol. 13:1132141. doi: 10.3389/fonc.2023.1132141
Received: 26 December 2022; Accepted: 27 January 2023;
Published: 13 March 2023.
Edited by:
Veronika Vymetalkova, Academy of Sciences of the Czech Republic (ASCR), CzechiaReviewed by:
Monika Sramkova, Biomedical Research Center SAS, SlovakiaCopyright © 2023 Lin, Yang, Yang, Zhang and Huang. This is an open-access article distributed under the terms of the Creative Commons Attribution License (CC BY). The use, distribution or reproduction in other forums is permitted, provided the original author(s) and the copyright owner(s) are credited and that the original publication in this journal is cited, in accordance with accepted academic practice. No use, distribution or reproduction is permitted which does not comply with these terms.
*Correspondence: Xuan Huang, aHVhbmd4dWFuMTk3NkAxNjMuY29t
Disclaimer: All claims expressed in this article are solely those of the authors and do not necessarily represent those of their affiliated organizations, or those of the publisher, the editors and the reviewers. Any product that may be evaluated in this article or claim that may be made by its manufacturer is not guaranteed or endorsed by the publisher.
Research integrity at Frontiers
Learn more about the work of our research integrity team to safeguard the quality of each article we publish.