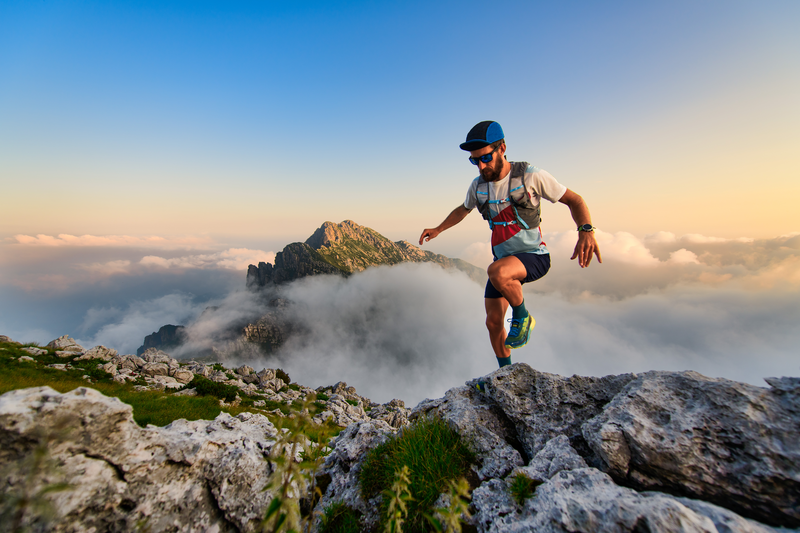
94% of researchers rate our articles as excellent or good
Learn more about the work of our research integrity team to safeguard the quality of each article we publish.
Find out more
REVIEW article
Front. Oncol. , 23 March 2023
Sec. Cancer Metabolism
Volume 13 - 2023 | https://doi.org/10.3389/fonc.2023.1123420
This article is part of the Research Topic Metabolic Regulation under Oxidative Stress in Cancer View all 13 articles
Copper is an essential micronutrient for human body and plays a vital role in various biological processes including cellular respiration and free radical detoxification. Generally, copper metabolism in the body is in a stable state, and there are specific mechanisms to regulate copper metabolism and maintain copper homeostasis. Dysregulation of copper metabolism may have a great connection with various types of diseases, such as Wilson disease causing copper overload and Menkes disease causing copper deficiency. Cancer presents high mortality rates in the world due to the unlimited proliferation potential, apoptosis escape and immune escape properties to induce organ failure. Copper is thought to have a great connection with cancer, such as elevated levels in cancer tissue and serum. Copper also affects tumor progression by affecting angiogenesis, metastasis and other processes. Notably, cuproptosis is a novel form of cell death that may provide novel targeting strategies for developing cancer therapy. Copper chelators and copper ionophores are two copper coordinating compounds for the treatment of cancer. This review will explore the relationship between copper metabolism and cancers, and clarify copper metabolism and cuproptosis for cancer targeted therapy.
Copper (Cu) is an essential micronutrient for the human body. Copper has four oxidation states: metallic copper, Cu+, Cu2 + and Cu3+. As a transition metal, copper plays a key role in many biological processes, such as cellular respiration (1), free radical detoxification (2–5), cellular iron metabolism (6), angiogenesis (7), and neurotransmitter synthesis (8). However, excess intracellular copper ions can be toxic to cells (9). The transfer of electrons will occur in the transfer of copper ions with different valence states, resulting in the formation of reactive oxygen species (ROS).ROS can injury biological organic molecules such as proteins, nucleic acids and lipids, and also interfere with the synthesis of iron sulfur clusters to injury countless essential enzymes in cells. In addition, copper overload is seen in Wilson disease (WD), which is a manifestation of dysregulation of organismal copper homeostasis (10). Deficiency of copper ions results in the reduction of multiple enzyme activities, which is also thought to underlie the pathologies of Menkes disease (MD) (11). Therefore, no matter whether copper ions are excessive or deficient, it may be harmful to human body. Copper homeostasis is essential in organisms and dysregulation of copper metabolism leads to the occurrence of some diseases.
Copper is closely related to cancer. It is well known that copper is also involved in tumor formation and progression. Copper levels are elevated in a variety of malignancies, and high levels of copper ions affect tumor proliferation, angiogenesis, as well as metastasis (12–14). In recent years, a novel form of cell death induced by intracellular copper, discovered by Tsvetkov and co-workers (15), which is distinct from oxidative stress-related cell death, is a type of copper-dependent cell death, termed cuproptosis. This review will explore the relationship between copper metabolism, cuproptosis and cancers, providing references for cancer targeted therapy.
In mammals, copper is required for cellular metabolism, but its excess is toxic to cells. Therefore, copper concentration in cells is tightly regulated (16). There are many components involved in cellular copper homeostasis maintenance, including (1) transporters mediating copper absorption, such as copper transporter receptor 1 (Ctr1) (also called SCL1A1), copper transporter receptor 2 (Ctr2), divalent metal transporter 1 (DMT1); (2) enzymes guiding copper ion efflux, such as copper-transporting ATPase 1 (ATP7A) and copper-transporting ATPase 2 (ATP7B); (3) biomolecules that sequester and store copper, such as metallothionein (MT), glutathione (GSH); (4) copper chaperones, such as copper chaperone for superoxide dismutase (CCS), antioxidant protein 1 (Atox1), cytochrome c oxidase copper chaperone 17 (Cox17), which direct copper to copper dependent enzymes and transport copper to organelles that requiring copper (16).
A major contributor involved in copper uptake in mammals is Ctr1 (17). It is now generally accepted that Ctr1 transports Cu+ in a high affinity manner (18, 19), however in mammalian enterocytes the copper ion is in the form of divalent copper (Cu2+), which can be directly transported by divalent metal transporter 1 (DMT1) but cannot be directly utilized by cells (20). Thus enterocytes produced intracellular reductases such as steap2/3/4 to reduce cell surface Cu2+ to Cu+ and then Cu+ can be transported by Ctr1 (21) (Figure 1).
Figure 1 A diagram of cellular copper transport and metabolism. Extracellularly, copper exists as Cu2+. The cellular reductases protein family Steap proteins (mainly Steap 2/3/4) reduce Cu2+ to Cu+, which is transported into the cell via Ctr1, and a fraction of Cu+ is targeted to cytosolic SOD1 by the copper chaperone CCS to scavenge free radicals. A fraction of Cu+ is delivered by the copper chaperone Cox17 to the mitochondrial Cox to generate ATP. A portion of Cu+ is delivered to ATP7A/B of the trans Golgi network by the copper chaperone Atox1, which promotes cuproproteins (CuPrs) assembly and secretion. The remaining excess Cu+ is sequestered by metallothionein (MT). There are copper sensors in the nucleus that respond to changes in copper concentration through regulating MT1 and MT2 gene transcriptions. In enterocytes, ATP7A migrates to the plasma membrane to pump Cu+ into the blood. In hepatocytes, ATP7B pumps Cu+ into the bile.
Copper ions are transported to specific locations after entering the cell via utilization or detoxification pathways, and excess copper will be sequestered by copper storage proteins such as MT and GSH (22). The intracellular trafficking of copper is mediated by copper chaperones, such as CCS, Atox1, Cox17. These copper chaperones assist copper in reaching vital destinations without inflicting damage or becoming trapped in adventitious binding sites (23) In the cytoplasm, CCS mediates Cu+ loading and activation of superoxide dismutase 1 (SOD1) (24). The chaperone Cox17 mainly functions to transfer copper to Sco1, Sco2, Cox11 (25), and Sco1/2 plays an important role for cytochrome c oxidase (Cox) formation (26). The role of Atox1 is to bind Cu+ and deliver it to P1B type ATPases (27), which includes ATP7A and ATP7B with important roles in the systemic distribution of copper ions.
Excessive copper ion accumulation in cells may generate ROS, causing oxidative damage to cells. Thus, the excess Cu+ will be bound by MT or GSH, maintaining the concentration of intracellular Cu ions within a suitable range (16). The copper ion export in mammals is dependent on large multi transmembrane proteins ATP7A and ATP7B (16). There are multiple routes for copper ions transport out of the cell. For example, copper ions in intestinal epithelial cells enter the blood directly via ATP7A, and ATP7B in hepatocytes pumps copper ions into the bile (16) (Figure 1).
Copper metabolism is meticulously controlled in living organisms to maintain the level of copper in a reasonable range. Defects in molecules involved in copper metabolism will resultin disturbed copper homeostasis and related diseases. WD and MD are two typical copper disorders resulting from dysregulation of copper metabolism (28).
MD is a copper deficiency disease caused by mutations in ATP7A (28). The main function of ATP7A is to transport copper from enterocytes to the blood, where it plays a vital role in intestinal absorption of copper and renal copper reabsorption (29). Defective ATP7A impedes intestinal copper absorption, ultimately leading to severe systemic copper deficiency, as well as deficiency of cuproenzymes in tissues, such as brain. The symptoms exhibited by MD patients include neuropathy, hypopigmentation, seizures, and hypothermia (30, 31). In addition, copper deficiency can lead to impaired energy levels, increased oxidative damage, and changes in immune cell structure and function in living organisms (32).Studies have suggested that copper deficiency may result in a higher frequency of infections as well as a higher risk of cardiovascular disease (33, 34).
WD is a copper overload disease caused by mutations in ATP7B (28). The main functions of ATP7B is to transport copper to the trans Golgi to facilitate assembly and secretion of cuproenzymes (29). In addition to hepatocytes, ATP7B also acts as a copper ion exporter, excreting it into bile. Because of gene mutations, ATP7B dysfunction leads to copper ion accumulation in the liver. When the capacity of the liver for storage is exceeded, copper spills into the circulation and subsequently enters and deposits into other tissues (e.g. eye and brain), causing oxidative stress that damages the tissues (28). The typical pathological features of WD are neurological abnormalities and acute liver failure (35, 36).
Cancer has long been a research hotspot in the life sciences as well as in medicine. The incidence and mortality rates of cancer have been rising rapidly over the past few decades. It has now become the disease responsible for the largest number of deaths in the population worldwide (37). Among these, lung cancer is the most common and associated with the highest mortality in the population. The second highest incidence was for female breast cancer, followed by prostate and colorectal cancer. The top four cancers in order of mortality were lung, colorectal, stomach and liver cancer (37). Copper ion metabolism is also involved in the progression of these cancers (38).
In fact, copper in the human body has a great association with cancer, and there are a large number of medical studies showing that the serum copper levels in cancer patients, as well as in tumor tissues, can be higher or lower (mostly high) compared with normal individuals (Tables 1, 2). When tumor is removed, serum copper return to comparable levels with healthy individuals (13). In several serum medical detections of breast cancer patients, it was found that copper levels were significantly elevated in the serum of breast cancer patients compared with the healthy population (51). Similarly, elevated levels of copper have been reported in the serum of patients with oral cancer (47), gallbladder cancer (46), liver cancer (49), pancreatic cancer (57), and prostate cancer (61). Serum copper levels were found to be decreased in the serum of patients with certain cancers, such as colorectal cancer (56) and endometrial cancer (68). In colorectal and breast cancer, increased serum copper levels correlated with cancer staging and progression (69). And serum elevated copper levels are also associated with hematological malignancies either in relapse or in disease progression (70). Because of the variation of serum copper levels in cancer, it may be used as an indicator to diagnose certain tumors. The Cu/Zn ratio has been widely recognized as an indicator for the auxiliary diagnosis of tumors, and we summarized most up-to-date evidence of Cu/Zn ratio in the diagnosis of cancers (71, 72).
An increasing number of existing studies have demonstrated that copper is critical for the development of cancer not only as a component to maintain cell function, but also as a central hub in cell signaling pathways involving cell proliferation, angiogenesis, and metastasis (73). Cu+ is redox active and is able to promote the production of ROS and thereby activate tumor signaling, leading to tumor proliferation (69). In part, studies of the relationship between co-binding proteins (or chaperones) and cell proliferation identified that Atox1, a copper dependent transcription factor, promoted the expression of genes encoding cell replication (74). In recent studies, copper has also been found to have a specific role in the mitotic signaling pathway of tumorigenesis. Studies using drosophila and mouse models found that copper uptake via Ctr1 activates the mitogen activated protease kinase (MAPK) (75). Among molecules of this pathway, MEK1 is a copper binding protein that, when bound to copper ions, is able to push the MEK1-ERK interaction to promote the phosphorylation of ERK1 and ERK2, ultimately leading to carcinogenesis and promoting tumor growth (76, 77).
Copper is able to induce a number of proangiogenic responses (78), and increases proangiogenic gene expression by stabilizing nuclear hypoxia inducible factor-1 (HIF-1) (79). Copper also activates some angiogenic factors such as basic fibroblast growth factor (bFGF), tumor necrosis factor alpha (TNF-α), IL-1, IL-6 and IL-8 (80). In addition, copper stimulates the proliferation and metastasis of vascular endothelial cells (81). It directly binds to the angiogenic growth factor angiopoietin, enhancing its affinity for endothelial cells (82).
Copper is implicated in epithelial to mesenchymal transition (EMT), which is necessary for cancer metastasis (83). Increasing studies have shown that copper enhances the invasive and metastatic abilities of cancer cells through the activation of metabolic and proliferative enzymes (84, 85). For example, copper is indispensable for the activity of lysine oxidase (LOX) and lysine oxidase like (LOXL) proteins, which catalyze the cross-linking of collagen and elastin in the extracellular matrix (ECM), and create preconditions for tumor development and metastasis (86). It was found that a copper dependent redox protein named memo affected metastasis of breast cancer cells by increasing intracellular ROS levels (87). Recently, the copper chaperone Atox1 has also been shown to be essential in breast cancer cell migration (88, 89).
Copper is redox active and easily interconverts between Cu+ and Cu2+. Many important enzymes utilize this property of copper to exert their functions in redox reactions in living organisms (69). Because copper is capable of generating excess ROS, copper transporters and chaperones have evolved to regulate copper uptake, efflux, and distribution within cells (33). Dysregulation of copper metabolism may lead to oxidative stress, such as decreased SOD1 activity and increased superoxide anion in different animal models (90, 91). Copper deficiency may also increase oxidative stress in mitochondria by inhibiting cytochrome c oxidase activity (92). And the dysregulation of copper metabolism may cause cancer, as copper deficiency may have effects on the oxidative system (33). Copper is a cofactor of SOD1, and the main function of SOD is to scavenge free radicals to prevent cells from oxidative stress injury, especially playing a crucial role in scavenging ROS generated from mitochondria (93, 94). SOD protein has three isoenzymes in humans. In particular, Cu/Zn SOD (SOD1) is a SOD with a bimetallic enzymatic function, which requires copper to catalyze the reaction and zinc to increase catalytic efficiency and enzyme stability (95–98). Copper deficiency leads to reduced SOD1 activity, and reduced SOD1 activity contributes to carcinogenesis (99). Copper deficiency also alters the activity of other enzymes involved in oxidative stress as well as ROS scavengers (e.g. catalase, metallothionein) (33). Alterations in these proteins may cause deregulation of oxidative stress, overproduction of ROS as well as deregulation of oxidative stress in the body may impair DNA repair machinery which is also an important mechanism in cancer development (100).
Ceruloplasmin is involved in copper metabolism, which is the main carrier of copper in the human body, and about 90% of copper in plasma is found in ceruloplasmin. In addition, ceruloplasmin is a multi-copper oxidase that plays an important role in iron homeostasis (101). When Fe2+ exported from ferroportin, the sole iron exporter, ceruloplasmin promotes cellular iron export by oxidizing iron ion from Fe2+ to Fe3+ (102). Although ceruloplasmin synthesis and secretion are not affected by copper levels, copper deficiency may result in decreased ceruloplasmin stability and activity (103). Ceruloplasmin is also closely linked to cancer, and studies have indicated that significant ceruloplasmin gene expression occurs in many tumors and that the overall incidence of cancer is positively correlated with serum ceruloplasmin levels and may be able to serve as a prognostic marker in some cancers (104–107).
Among current treatments for cancer, targeted therapy is considered to be highly promising because its intervention can be selectively performed on molecules and pathways involved in the growth and developmental progression of tumors (108). Considering copper as a nutrient for cancer growth, angiogenesis, and metastasis, it may be an attractive target in cancer therapy (109). Copper metal binding compounds have great potential in cancer therapy. When copper binding compounds are mentioned, copper chelators and copper ionophores come to mind.Currently, copper chelators and copper ionophores have great potential value in cancer targeted therapy.
Copper chelators are able to bind to copper and reduce its bioavailability, thereby inhibiting angiogenesis and hindering cancer growth and metastasis (110). So far, some copper chelation methods have been used in clinical trials and provided some new strategies for the treatment of cancer (111, 112). Copper chelators with anticancer activity are tetrathiomolybdate (TTM), D-penicillamine (D-Pen) and others. There are studies demonstrating TTM exerts significant efficacy in the treatment of squamous cell carcinoma (113), lung (114), breast (115) and prostate cancer (116). It is important to note, however, that copper chelators are simply anticancer and are not sufficient by themselves to kill malignant cells, therefore, it needs to be combined with other drugs to achieve a therapeutic effect that is promising for cancer (117).
Copper ionophore in contrast to copper chelators,is able to increase intracellular copper bioavailability. There are various modes of action of copper ionophores, such as DNA interaction, proteasome inhibition as well as ROS generation (69). Typical copper ionophores are chloroquinol and disulfiram (DSF), and they can release coordinately available copper in the intracellular reducing environment, increasing the bioavailability of copper inside cells (118). Chloroquinol and DSF are able to cause intracellular production of ROS and inhibit the activity of proteasomes in cancer cells, which enables apoptosis (119). Chloroquinol and disulfiram have also been shown to reduce tumor growth in models of prostate and breast cancer (120–122). Copper chelators are able to inhibit cuproptosis, whereas copper ionophores induce cuproptosis. Cuproptosis, already introduced in a previous text, is a copper dependent cytotoxicity with a unique mechanism leading to cell death (123). However, the field of copper metal binding compounds to treat cancer is still in an early stage of development, and although clinical trials have been conducted and are able to give some strategies to treat cancer, they still need to be explored further to overcome their disadvantages. The lack of selectivity for targeting cancer cells is one of the challenges in this field.
In addition, inhibiting the expression of copper transporters may provide some reference for cancer therapy. We searched through the gepia database and analyzed for survival curves between the expression levels of the copper ion transporter SLC31A1 and cancer patient survival. We found that the expression level of copper importer in cancer tissue may have a close relationship with patient survival. Analysis of the survival curves between the expression levels of SLC31A1 and cancer patient survival showed that lower SLC31A1 expression significantly increased overall survival compared with individuals with higher SLC31A1 expression in Adrenocortical carcinoma (ACC), Breast invasive carcinoma (BRCA), Brain Lower Grade Glioma (LGG), Mesothelioma (MESO), Skin Cutaneous Melanoma (SKCM) (Figure 2). The inverse association between SLC31A1 expression and patient survival suggests that excessive copper ion uptake may promote cancer progression and increase patient mortality. This correlation may provide a potential mechanism for developing novel cancer therapies through inhibition SLC31A1 expression or removal of large amounts of copper ions in tumor tissues.
Figure 2 Survival curves for overall survival of high versus low expressing SLC31A1. (A) ACC, adrenocortical carcinoma; (B) BRCA, breast invasive carcinoma; (C) LGG, brain lower grade glioma; (D) MESO, mesothelioma. The overall survival rate of low expression of SLC31A1was significantly higher than that of high expression of SLC31A1. SLC31A1, solute carrier family 31 member 1 (copper ion transporter); HR, hazard rate. (http://gepia.cancer-pku.cn/index.html; Accessed 10 October 2022).
Recently, a novel mode of cell death was discovered by Tsvetkov et al. (15). It is a copper-dependent, regulated, distinct from other known cell death regulatory mechanisms, and this copper-dependent manner of death has been termed “cuproptosis”. Heavy metal overload such as iron will cause deleterious effect on cells. An example is ferroptosis, an iron-dependent form of cell death caused by unrestricted lipid peroxidation (124). Cuproptosis results from mitochondrial stress. Copper can directly bind to ester acylated components of the tricarboxylic acid cycle, with subsequent aggregation of copper bound lipidated mitochondrial enzymes and loss of iron sulfur protein clusters, finally leading to the occurrence of cuproptosis (15).
The exact regulatory mechanism of copper induced cell death still needs further elucidation, although various pathways have been proposed, including induction of apoptosis, induction of reactive oxygen species, inhibition of the ubiquitin proteasome system. Currently in the study of Tsvetkov et al. (15), it was demonstrated that the mechanism of cuproptosis involves a copper ionophore named “elesclomol”, which can bind Cu2+ in the extracellular environment and transport Cu2+ into the cells. Intracellularly, several critical genes are involved in this process. FDX1 gene encodes a reductase ferredoxin 1 reducing Cu2+ to Cu+. DLAT gene encodes an enzyme called dihydrolipoyl transacetylase that is a part of pyruvate dehydrogenase involved in the tricarboxylic acid cycle and is a protein target for lipidation. FDX1 is a key regulator of cuproptosis and an upstream regulator of protein lipoylation. On the one hand, FDX1 promotes lipoylation of pyruvate dehydrogenase, and Cu2+ directly binds to lipoylated proteins (mainly DLAT), followed by aberrant oligomerization of DLAT, resulting in tricarboxylic acid cycle (TCA) inhibition, and on the other hand, FDX1 reduces Cu2+ to Cu+, leading to inhibition of iron sulfur protein clusters synthesis, disruption of iron sulfur protein clusters stability, and ultimately leads to proteotoxic stress and cell death (Figure 3).
Figure 3 A diagram of the simple mechanism of cuproptosis. Elesclomol imports Cu2+ into the cell, and then reduced to Cu+ by FDX1. Cu+ binds to lipoylated components of the mitochondrial TCA cycle, promoting lipoylated protein aggregation followed by a decrease in iron-sulfur cluster proteins, thereby inducing proteotoxic stress, leading to cell death. ES, elesclomol; FDX1, ferredoxin 1; DLAT, dihydrolipoyl transacetylase; TCA, tricarboxylic acid cycle.
Cancer cells can evade the regulated cell death pathway, which is also one of the important hallmarks of cancer. Currently, one of the major challenges in cancer therapies is the escape of cancer cells from cell death pathways. The discovery of cuproptosis will provide a novel target to overcome the resistance of cancer cells for cell death. Existing evidence indicates that the Cu2+ carrier, elesclomol, can kill specific drug-resistant cancer cells (125). In recent studies on lower grade gliomas (LGG), arguing that copper death may serve as a potential therapeutic target for LGG (126). Researches have shown that cuproptosis related genes may play a great role in the diagnosis and prognosis of some types of cancer. In the research of pancreatic adenocarcinoma (PAAD) by Huang et al., the cuproptosis-related gene index (CRGI) was developed through machine algorithm, and its immunological characteristics were studied by exploring its impact on the expression of immune checkpoints, prospective immunotherapeutic response, etc. A new CRGI was identified and verified, and the cuproptosis-related gene was found to be a reliable diagnostic biomarker in PAAD (127). Sha et al., in a study of triple-negative breast cancer (TNBC), identified two clusters of cuproptosis related genes (CRG) with features of immune cell infiltration and demonstrated that the CRG signature may be used to assess tumor immune cell infiltration, clinical features, and prognostic status. Their study has shown the potential effect of CRG on the tumor microenvironment (TME), clinicopathological characteristics, and prognosis of TNBC which are potential tools for predicting patient outcomes (128). In the study of clear cell renal cell carcinoma (ccRCC), Wang et al. found that ccRCC samples had significantly lower FDX1 expression levels than normal tissue samples and lower FDX1 gene expression levels were strongly associated with higher cancer grades and more advanced tumor-node-metastasis stages. The results of multivariate and univariate analyses indicated that ccRCC patients with low FDX1 expression had shorter overall survival (OS) than those with high FDX1 expression. The study illustrates that in ccRCC, reduced FDX1 expression is associated with disease progression, poor prognosis and dysregulated immune cell infiltration which illustrates that the cuproptosis related gene may serve as a potential prognostic predictor for ccRCC patients and may provide new insights into cancer treatment (129, 130). Zhu et al. performed a comprehensive analysis of cuproptosis related molecular patterns in 1274 colorectal cancer specimens based on 16 cuproptosis regulators and revealed a novel cuproptosis related molecular pattern associated with TME phenotypes, and the formation of a cuproptosis score will further enhance the understanding of TME characteristics and instruct a more personalized immunotherapy schedule in colorectal cancer (131). Lv et al. explored the association of cuproptosis related genes with skin cutaneous melanoma (SKCM) prognosis by accessing and analyzing a public database, and found that 11 out 12 genes were upregulated in melanoma tissues and three genes (LIPT1, PDHA1, and SLC31A1) were of predictive value for melanoma prognosis. Further exploration found that LIPT1 expression was increased in melanoma biopsies and was an independent favorable prognostic indicator for melanoma patients (132). Zhang et al. integrated a set of bioinformatics tools to analyze the expression and prognostic significance of FDX1, a key regulator of cuproptosis. The cuproptosis related risk score (CRRS) was derived by correlation analysis. The metabolic features, mutation signatures, and immune profile of CRRS-classified hepatocellular carcinoma (HCC) patients were investigated, and the role of CRRS in treatment guidance was analyzed. FDX1 was found to be significantly downregulated in HCC and its high expression was associated with longer survival time. HCC patients in the high CRRS group had significantly worse OS and enriched in tumor related pathways. Mutational analysis revealed that several tumor suppressors such as tumor protein P53 (TP53) and Breast cancer susceptibility gene 1 (BRCA1) -associated protein 1 (BAP1) were mutated at a higher frequency in high CRRS HCC patients, illustrating that cuproptosis related signatures are helpful in predicting prognosis and guiding the treatment of HCC patients (133). There are also scientists building a liver cancer prognosis model based on cuproptosis related mRNAs and lncRNAs, which can effectively predict the potential survival of liver cancer patients as well as evaluate the infiltration of immune cells, tumor mutation burden, and sensitivity to antitumor drugs in liver cancer (134). Li et al. systematically evaluated cuproptosis patterns in bladder cancer (BLCA) patients based on 46 cuproptosis related genes and correlated these cuproptosis patterns with TME phenotypes and immunotherapy effects. For the evaluation of individual patients, a cuproptosis risk score (CRS) for prognosis and a cuproptosis signature for precise TME phenotypes and immunotherapy efficacy prediction were constructed. Finally, it was demonstrated that CRS and cuproptosis signature have potential roles in predicting prognosis and immunotherapy efficacy in BLCA (135). A pan-cancer analysis revealed that transcription and protein expression of FDX1 was significantly reduced in most cancer types, and furthermore, FDX1 expression levels were closely correlated with immune cell infiltration, immune checkpoint genes, and immune regulatory genes to some extent. Due to its important role in tumorigenesis and tumor immunity, FDX1 can serve as a potential therapeutic target and prognostic marker in various malignancies (136–138). Altogether, putting the discovery of cuproptosis may provide a new strategy for cancer prognosis as well as treatment.
Copper plays an irreplaceable role as a micronutrient in the human body, and both deficiency or overload of copper in the body can negatively affect the human body, therefore, the mechanism of copper metabolism in cells keeps copper at a stable level to achieve copper homeostasis. Copper metabolism is also closely associated with cancer development, and copper is able to affect cancer cell proliferation and metastasis. Intracellular copper has a great connection with cancer, therefore, targeting copper in cancer therapy may play a great role. Currently, using copper complexes for cancer treatment, copper chelators with copper ionophores are two good choices, but the efficacy of copper chelators alone is not significant, and copper ionophores are still in the development stage, which also lack selectivity for targeting cancer cells. Therefore, improving selectivity against cancer cells is a worthy goal of investigation in the future. In a recent study on cell death, a novel concept cuproptosis, which is a copper dependent cell death induced by copper, was proposed, and the exact regulatory mechanism of this novel regulated cell death still needs to be continued to be explored. The proposal of cuproptosis presents a new avenue for the treatment of cancer.
It has a link between copper metabolism and cuproptosis. The dysregulation of copper metabolism in the body, such as copper overload may lead to cuproptosis. In addition, cuproptosis also offer a novel strategy for targeted cancer therapy. Copper metabolism and cuproptosis is worthy of further exploration and application to conquer cancer in clinic.
Conceptualization, JL, YL and JW; investigation, ZW, DJ and SZ; writing—original draft preparation, ZW, DJ and YJ; writing—review and editing, PA, JL, YL and JW; Visualization, ZW, ND and JL; supervision, JL, YL and JW; project administration, YL and JW; funding acquisition, YL and JL. All authors have read and agreed to the published version of the manuscript.
This work was supported by the Beijing Advanced Innovation Center for Food Nutrition and Human Health, the National Natural Science Foundation of China (31970717, 82170429), the Chinese Universities Scientific Fund (2020TC015), and the Beijing Municipal Natural Science Foundation (7222111).
The authors declare that the research was conducted in the absence of any commercial or financial relationships that could be construed as a potential conflict of interest.
All claims expressed in this article are solely those of the authors and do not necessarily represent those of their affiliated organizations, or those of the publisher, the editors and the reviewers. Any product that may be evaluated in this article, or claim that may be made by its manufacturer, is not guaranteed or endorsed by the publisher.
1. Tsukihara T, Aoyama H, Yamashita E, Tomizaki T, Yamaguchi H, Shinzawa-Itoh K, et al. Structures of metal sites of oxidized bovine heart cytochrome c oxidase at 2.8 a. Science (1995) 269(5227):1069–74. doi: 10.1126/science.7652554
2. Clement AM, Nguyen MD, Roberts EA, Garcia ML, Boillée S, Rule M, et al. Wild-type nonneuronal cells extend survival of SOD1 mutant motor neurons in ALS mice. Science (2003) 302(5642):113–7. doi: 10.1126/science.1086071
3. De Freitas JM, Liba A, Meneghini R, Valentine JS, Gralla EB. Yeast lacking Cu-zn superoxide dismutase show altered iron homeostasis. role of oxidative stress in iron metabolism. J Biol Chem (2000) 275(16):11645–9. doi: 10.1074/jbc.275.16.11645
4. Paynter DI, Moir RJ, Underwood EJ. Changes in activity of the Cu-zn superoxide dismutase enzyme in tissues of the rat with changes in dietary copper. J Nutr (1979) 109(9):1570–6. doi: 10.1093/jn/109.9.1570
5. Staveley BE, Phillips JP, Hilliker AJ. Phenotypic consequences of copper-zinc superoxide dismutase overexpression in drosophila melanogaster. Genome (1990) 33(6):867–72. doi: 10.1139/g90-130
6. Chan WY, Rennert OM. The role of copper in iron metabolism. Ann Clin Lab Sci (1980) 10(4):338–44.
7. Siegel RC. Lysyl oxidase. Int Rev Connect Tissue Res (1979) 8:73–118. doi: 10.1016/B978-0-12-363708-6.50009-6
8. Gerbasi V, Lutsenko S, Lewis EJ. A mutation in the ATP7B copper transporter causes reduced dopamine beta-hydroxylase and norepinephrine in mouse adrenal. Neurochem Res (2003) 28(6):867–73. doi: 10.1023/A:1023219308890
9. De Freitas J, Wintz H, Kim JH, Poynton H, Fox T, Vulpe C. Yeast, a model organism for iron and copper metabolism studies. Biometals (2003) 16(1):185–97. doi: 10.1023/A:1020771000746
10. Aggarwal A, Bhatt M. Wilson Disease. Curr Opin Neurol (2020) 33(4):534–42. doi: 10.1097/WCO.0000000000000837
11. Bertini I, Rosato A. Menkes disease. Cell Mol Life Sci (2008) 65(1):89–91. doi: 10.1007/s00018-007-7439-6
12. Finney L, Mandava S, Ursos L, Zhang W, Rodi D, Vogt S, et al. X-Ray fluorescence microscopy reveals large-scale relocalization and extracellular translocation of cellular copper during angiogenesis. Proc Natl Acad Sci USA (2007) 104(7):2247–52. doi: 10.1073/pnas.0607238104
13. Gupte A, Mumper RJ. Elevated copper and oxidative stress in cancer cells as a target for cancer treatment. Cancer Treat Rev (2009) 35(1):32–46. doi: 10.1016/j.ctrv.2008.07.004
14. Ishida S, Andreux P, Poitry-Yamate C, Auwerx J, Hanahan D. Bioavailable copper modulates oxidative phosphorylation and growth of tumors. Proc Natl Acad Sci USA (2013) 110(48):19507–12. doi: 10.1073/pnas.1318431110
15. Tsvetkov P, Coy S, Petrova B, Dreishpoon M, Verma A, Abdusamad M, et al. Copper induces cell death by targeting lipoylated TCA cycle proteins. Science (2022) 375(6586):1254–61. doi: 10.1126/science.abf0529
16. Chen J, Jiang Y, Shi H, Peng Y, Fan X, Li C. The molecular mechanisms of copper metabolism and its roles in human diseases. Pflugers Arch (2020) 472(10):1415–29. doi: 10.1007/s00424-020-02412-2
17. Kim BE, Nevitt T, Thiele DJ. Mechanisms for copper acquisition, distribution and regulation. Nat Chem Biol (2008) 4(3):176–85. doi: 10.1038/nchembio.72
18. Eisses JF, Chi Y, Kaplan JH. Stable plasma membrane levels of hCTR1 mediate cellular copper uptake. J Biol Chem (2005) 280(10):9635–9. doi: 10.1074/jbc.M500116200
19. Lee J, Peña MM, Nose Y, Thiele DJ. Biochemical characterization of the human copper transporter Ctr1. J Biol Chem (2002) 277(6):4380–7. doi: 10.1074/jbc.M104728200
20. Shawki A, Anthony SR, Nose Y, Engevik MA, EJ. Intestinal DMT1 is critical for iron absorption in the mouse but is not required for the absorption of copper or manganese. Am J Physiol Gastrointest Liver Physiol (2015) 309(8):G635–47. doi: 10.1152/ajpgi.00160.2015
21. Ohgami RS, Campagna DR, McDonald A, Fleming MD. The steap proteins are metalloreductases. Blood (2006) 108(4):1388–94. doi: 10.1182/blood-2006-02-003681
22. Lalioti V, Muruais G, Tsuchiya Y, Pulido D, Sandoval IV. Molecular mechanisms of copper homeostasis. Front Biosci (Landmark Ed) (2009) 14(13):4878–903. doi: 10.2741/3575
23. Robinson NJ, Winge DR. Copper metallochaperones. Annu Rev Biochem (2010) 79:537–62. doi: 10.1146/annurev-biochem-030409-143539
24. Wong PC, Waggoner D, Subramaniam JR, Tessarollo L, Bartnikas TB, Culotta VC, et al. Copper chaperone for superoxide dismutase is essential to activate mammalian Cu/Zn superoxide dismutase. Proc Natl Acad Sci USA (2000) 97(6):2886–91. doi: 10.1073/pnas.040461197
25. Maxfield AB, Heaton DN, Winge DR. Cox17 is functional when tethered to the mitochondrial inner membrane. J Biol Chem (2004) 279(7):5072–80. doi: 10.1074/jbc.M311772200
26. Leary SC, Cobine PA, Kaufman BA, Guercin GH, Mattman A, Palaty J, et al. The human cytochrome c oxidase assembly factors SCO1 and SCO2 have regulatory roles in the maintenance of cellular copper homeostasis. Cell Metab (2007) 5(1):9–20. doi: 10.1016/j.cmet.2006.12.001
27. Hamza I, Faisst A, Prohaska J, Chen J, Gruss P, Gitlin JD, et al. The metallochaperone Atox1 plays a critical role in perinatal copper homeostasis. Proc Natl Acad Sci USA (2001) 98(12):6848–52. doi: 10.1073/pnas.111058498
28. Wang X, Garrick MD, Collins JF. Animal models of normal and disturbed iron and copper metabolism. J Nutr (2019) 149(12):2085–100. doi: 10.1093/jn/nxz172
29. Lutsenko S, Barnes NL, Bartee MY, Dmitriev OY. Function and regulation of human copper-transporting ATPases. Physiol Rev (2007) 87(3):1011–46. doi: 10.1152/physrev.00004.2006
30. Kaler SG. ATP7A-related copper transport diseases-emerging concepts and future trends. Nat Rev Neurol (2011) 7(1):15–29. doi: 10.1038/nrneurol.2010.180
32. Keen CL, Hanna LA, Lanoue L, Uriu-Adams JY, Rucker RB, Clegg MS. Developmental consequences of trace mineral deficiencies in rodents: Acute and long-term effects. J Nutr (2003) 133(5 Suppl 1):1477s–80s. doi: 10.1093/jn/133.5.1477S
33. Uriu-Adams JY, Keen CL. Copper, oxidative stress, and human health. Mol Aspects Med (2005) 26(4-5):268–98. doi: 10.1016/j.mam.2005.07.015
34. Bonham M, O'Connor JM, Hannigan BM, Strain JJ. The immune system as a physiological indicator of marginal copper status? Br J Nutr (2002) 87(5):393–403. doi: 10.1079/BJN2002558
35. Członkowska A, Litwin T, Dusek P, Ferenci P, Lutsenko S, Medici V, et al. Wilson Disease. Nat Rev Dis Primers (2018) 4(1):21. doi: 10.1038/s41572-018-0018-3
36. Compston A. Progressive lenticular degeneration: A familial nervous disease associated with cirrhosis of the liver, by s. a. kinnier Wilson, (From the national hospital, and the laboratory of the national hospital, queen square, London) brain 1912: 34; 295-509. Brain (2009) 132(Pt 8):1997–2001. doi: 10.1093/brain/awp193
37. Bray F, Ferlay J, Soerjomataram I, Siegel RL, Torre LA, Jemal A, et al. Global cancer statistics 2018: GLOBOCAN estimates of incidence and mortality worldwide for 36 cancers in 185 countries. CA Cancer J Clin (2018) 68(6):394–424. doi: 10.3322/caac.21492
38. Shanbhag VC, Gudekar N, Jasmer K, Papageorgiou C, Singh K, Petris MJ, et al. Copper metabolism as a unique vulnerability in cancer. Biochim Biophys Acta Mol Cell Res (2021) 1868(2):118893. doi: 10.1016/j.bbamcr.2020.118893
39. Mansouri B, Ramezani Z, Yousefinejad V, Nakhaee S, Azadi N, Khaledi P, et al. Association between trace elements in cancerous and non-cancerous tissues with the risk of breast cancers in western Iran. Environ Sci pollut Res Int (2022) 29(8):11675–84. doi: 10.1007/s11356-021-16549-9
40. Farquharson MJ, Al-Ebraheem A, Falkenberg G, Leek R, Harris AL, Bradley DA, et al. The distribution of trace elements Ca, fe, Cu and zn and the determination of copper oxidation state in breast tumour tissue using muSRXRF and muXANES. Phys Med Biol (2008) 53(11):3023–37. doi: 10.1088/0031-9155/53/11/018
41. Sohrabi M, Gholami A, Azar MH, Yaghoobi M, Shahi MM, Shirmardi S, et al. Trace element and heavy metal levels in colorectal cancer: Comparison between cancerous and non-cancerous tissues. Biol Trace Elem Res (2018) 183(1):1–8. doi: 10.1007/s12011-017-1099-7
42. Xie B, Lin J, Sui K, Huang Z, Chen Z, Hang W, et al. Differential diagnosis of multielements in cancerous and non-cancerous esophageal tissues. Talanta (2019) 196:585–91. doi: 10.1016/j.talanta.2018.12.061
43. Yaman M, Kaya G, Simsek M. Comparison of trace element concentrations in cancerous and noncancerous human endometrial and ovary tissues. Int J Gynecol Cancer (2007) 17(1):220–8. doi: 10.1111/j.1525-1438.2006.00742.x
44. Yaman M, Kaya G, Yekeler H. Distribution of trace metal concentrations in paired cancerous and non-cancerous human stomach tissues. World J Gastroenterol (2007) 13(4):612–8. doi: 10.3748/wjg.v13.i4.612
45. Theophanides T, Anastassopoulou J. Copper and carcinogenesis. Crit Rev Oncol Hematol (2002) 42(1):57–64. doi: 10.1016/S1040-8428(02)00007-0
46. Basu S, Singh MK, Singh TB, Bhartiya SK, Singh SP, Shukla VK, et al. Heavy and trace metals in carcinoma of the gallbladder. World J Surg (2013) 37(11):2641–6. doi: 10.1007/s00268-013-2164-9
47. Baharvand M, Manifar S, Akkafan R, Mortazavi H, Sabour S. Serum levels of ferritin, copper, and zinc in patients with oral cancer. BioMed J (2014) 37(5):331–6. doi: 10.4103/2319-4170.132888
48. Jayadeep A, Pillai Raveendran K, Kannan S, Nalinakumari KR, Mathew B, Nair Krishnan M, et al. Serum levels of copper, zinc, iron and ceruplasmin in oral leukoplakia and squamous cell carcinoma. J Exp Clin Cancer Res (1997) 16(3):295–300.
49. Fang AP, Chen PY, Wang XY, Liu ZY, Zhang DM, Luo Y, et al. Serum copper and zinc levels at diagnosis and hepatocellular carcinoma survival in the guangdong liver cancer cohort. Int J Cancer (2019) 144(11):2823–32. doi: 10.1002/ijc.31991
50. Deshmukh VK, Raman PH, Dhuley JN, Naik SR. Role of ceruloplasmin in inflammation: Increased serum ceruloplasmin levels during inflammatory conditions and its possible relationship with anti-inflammatory agents. Pharmacol Res Commun (1985) 17(7):633–42. doi: 10.1016/0031-6989(85)90070-0
51. Pavithra V, Sathisha TG, Kasturi K, Mallika DS, Amos SJ, Ragunatha S, et al. Serum levels of metal ions in female patients with breast cancer. J Clin Diagn Res (2015) 9(1):BC25–c27. doi: 10.7860/JCDR/2015/11627.5476
52. Feng Y, Zeng JW, Ma Q, Zhang S, Tang J, Feng JF. Serum copper and zinc levels in breast cancer: A meta-analysis. J Trace Elem Med Biol (2020) 62:126629. doi: 10.1016/j.jtemb.2020.126629
53. Kuo HW, Chen SF, Wu CC, Chen DR, Lee JH. Serum and tissue trace elements in patients with breast cancer in Taiwan. Biol Trace Elem Res (2002) 89(1):1–11. doi: 10.1385/BTER:89:1:1
54. Saleh F, Behbehani A, Asfar S, Khan I, Ibrahim G. Abnormal blood levels of trace elements and metals, DNA damage, and breast cancer in the state of Kuwait. Biol Trace Elem Res (2011) 141(1-3):96–109. doi: 10.1007/s12011-010-8724-z
55. Goyal MM, Kalwar AK, Vyas RK, Bhati A. A study of serum zinc, selenium and copper levels in carcinoma of esophagus patients. Indian J Clin Biochem (2006) 21(1):208–10. doi: 10.1007/BF02913100
56. Khoshdel Z, Naghibalhossaini F, Abdollahi K, Shojaei S, Moradi M, Malekzadeh M. Serum copper and zinc levels among Iranian colorectal cancer patients. Biol Trace Elem Res (2016) 170(2):294–9. doi: 10.1007/s12011-015-0483-4
57. Lener MR, Scott RJ, Wiechowska-Kozłowska A, Serrano-Fernández P, Baszuk P, Jaworska-Bieniek K, et al. Serum concentrations of selenium and copper in patients diagnosed with pancreatic cancer. Cancer Res Treat (2016) 48(3):1056–64. doi: 10.4143/crt.2015.282
58. Mao S, Huang S. Zinc and copper levels in bladder cancer: A systematic review and meta-analysis. Biol Trace Elem Res (2013) 153(1-3):5–10. doi: 10.1007/s12011-013-9682-z
59. Linder MC, Hazegh-Azam M. Copper biochemistry and molecular biology. Am J Clin Nutr (1996) 63(5):797s–811s. doi: 10.1093/ajcn/63.5.797
60. Panaiyadiyan S, Quadri JA, Nayak B, Pandit S, Singh P, Seth A, et al. Association of heavy metals and trace elements in renal cell carcinoma: A case-controlled study. Urol Oncol (2022) 40(3):111.e11–111.e18. doi: 10.1016/j.urolonc.2021.11.017
61. Saleh SAK, Adly HM, Abdelkhaliq AA, Nassir AM. Serum levels of selenium, zinc, copper, manganese, and iron in prostate cancer patients. Curr Urol (2020) 14(1):44–9. doi: 10.1159/000499261
62. Shen F, Cai WS, Li JL, Feng Z, Cao J, Xu B. The association between serum levels of selenium, copper, and magnesium with thyroid cancer: a meta-analysis. Biol Trace Elem Res (2015) 167(2):225–35. doi: 10.1007/s12011-015-0304-9
63. Brem S. Angiogenesis and cancer control: From concept to therapeutic trial. Cancer Control (1999) 6(5):436–58. doi: 10.1177/107327489900600502
64. Zhang M, Shi M, Zhao Y. Association between serum copper levels and cervical cancer risk: A meta-analysis. Biosci Rep (2018) 38(4):BSR20180161. doi: 10.1042/BSR20180161
65. Bobrowska B, Skrajnowska D, Tokarz A. Effect of Cu supplementation on genomic instability in chemically-induced mammary carcinogenesis in the rat. J BioMed Sci (2011) 18(1):95. doi: 10.1186/1423-0127-18-95
66. Zhang X, Yang Q. Association between serum copper levels and lung cancer risk: A meta-analysis. J Int Med Res (2018) 46(12):4863–73. doi: 10.1177/0300060518798507
67. Fisher GL, Shifrine M. Hypothesis for the mechanism of elevated serum copper in cancer patients. Oncology (1978) 35(1):22–5. doi: 10.1159/000225249
68. Atakul T, Altinkaya SO, Abas BI, Yenisey C. Serum copper and zinc levels in patients with endometrial cancer. Biol Trace Elem Res (2020) 195(1):46–54. doi: 10.1007/s12011-019-01844-x
69. Denoyer D, Masaldan S, Fontaine La S, Cater MA. Targeting copper in cancer therapy: 'Copper that cancer'. Metallomics (2015) 7(11):1459–76. doi: 10.1039/C5MT00149H
70. Kaiafa GD, Saouli Z, Diamantidis MD, Kontoninas Z, Voulgaridou V, Raptaki M, et al. Copper levels in patients with hematological malignancies. Eur J Intern Med (2012) 23(8):738–41. doi: 10.1016/j.ejim.2012.07.009
71. Kazi Tani LS, Gourlan AT, Dennouni-Medjati N, Telouk P, Dali-Sahi M, Harek Y, et al. Copper isotopes and copper to zinc ratio as possible biomarkers for thyroid cancer. Front Med (Lausanne) (2021) 8:698167. doi: 10.3389/fmed.2021.698167
72. Dragutinović VV, Tatic SB, Nikolic-Mandic S, Savin S, Cvejic D, Dunðerovic D, et al. Matrix metalloproteinase-9 and the Cu/Zn ratio as ancillary diagnostic tools in distinguishing between the classical and follicular variants of papillary thyroid carcinoma. Biol Trace Elem Res (2012) 149(1):29–33. doi: 10.1007/s12011-012-9404-y
73. Grubman A, White AR. Copper as a key regulator of cell signalling pathways. Expert Rev Mol Med (2014) 16:e11. doi: 10.1017/erm.2014.11
74. Itoh S, Kim HW, Nakagawa O, Ozumi K, Lessner SM, Aoki H, et al. Novel role of antioxidant-1 (Atox1) as a copper-dependent transcription factor involved in cell proliferation. J Biol Chem (2008) 283(14):9157–67. doi: 10.1074/jbc.M709463200
75. Turski ML, Brady DC, Kim HJ, Kim BE, Nose Y, Counter CM, et al. A novel role for copper in ras/mitogen-activated protein kinase signaling. Mol Cell Biol (2012) 32(7):1284–95. doi: 10.1128/MCB.05722-11
76. Brady DC, Crowe MS, Turski ML, Hobbs GA, Yao X, Chaikuad A, et al. Copper is required for oncogenic BRAF signalling and tumorigenesis. Nature (2014) 509(7501):492–6. doi: 10.1038/nature13180
77. Dankner M, Rose AAN, Rajkumar S, Siegel PM, Watson IR. Classifying BRAF alterations in cancer: new rational therapeutic strategies for actionable mutations. Oncogene (2018) 37(24):3183–99. doi: 10.1038/s41388-018-0171-x
78. Tisato F, Marzano C, Porchia M, Pellei M, Santini C, et al. Copper in diseases and treatments, and copper-based anticancer strategies. Med Res Rev (2010) 30(4):708–49. doi: 10.1002/med.20174
79. Xie H, Kang YJ. Role of copper in angiogenesis and its medicinal implications. Curr Med Chem (2009) 16(10):1304–14. doi: 10.2174/092986709787846622
80. Suska F, Esposito M, Gretzer C, Källtorp M, Tengvall P, Thomsen P. IL-1alpha, IL-1beta and TNF-alpha secretion during in vivo/ex vivo cellular interactions with titanium and copper. Biomaterials (2003) 24(3):461–8. doi: 10.1016/S0142-9612(02)00359-9
81. De Luca A, Barile A, Arciello M, Rossi L. Copper homeostasis as target of both consolidated and innovative strategies of anti-tumor therapy. J Trace Elem Med Biol (2019) 55:204–13. doi: 10.1016/j.jtemb.2019.06.008
82. Soncin F, Guitton JD, Cartwright T, Badet J. Interaction of human angiogenin with copper modulates angiogenin binding to endothelial cells. Biochem Biophys Res Commun (1997) 236(3):604–10. doi: 10.1006/bbrc.1997.7018
83. Dongre A, Weinberg RA. New insights into the mechanisms of epithelial-mesenchymal transition and implications for cancer. Nat Rev Mol Cell Biol (2019) 20(2):69–84. doi: 10.1038/s41580-018-0080-4
84. Blockhuys S, Wittung-Stafshede P. Roles of copper-binding proteins in breast cancer. Int J Mol Sci (2017) 18(4):871. doi: 10.3390/ijms18040871
85. Shanbhag V, Jasmer-McDonald K, Zhu S, Martin AL, Gudekar N, Khan A, et al. ATP7A delivers copper to the lysyl oxidase family of enzymes and promotes tumorigenesis and metastasis. Proc Natl Acad Sci USA (2019) 116(14):6836–41. doi: 10.1073/pnas.1817473116
86. Farhat A, Ferns GA, Ashrafi K, Arjmand MH. Lysyl oxidase mechanisms to mediate gastrointestinal cancer progression. Gastrointest Tumors (2021) 8(1):33–40. doi: 10.1159/000511244
87. MacDonald G, Nalvarte I, Smirnova T, Vecchi M, Aceto N, Dolemeyer A, et al. Memo is a copper-dependent redox protein with an essential role in migration and metastasis. Sci Signal (2014) 7(329):ra56. doi: 10.1126/scisignal.2004870
88. Blockhuys S, Zhang X, Wittung-Stafshede P. Single-cell tracking demonstrates copper chaperone Atox1 to be required for breast cancer cell migration. Proc Natl Acad Sci USA (2020) 117(4):2014–9. doi: 10.1073/pnas.1910722117
89. Blockhuys S, Brady DC, Wittung-Stafshede P. Evaluation of copper chaperone ATOX1 as prognostic biomarker in breast cancer. Breast Cancer (2020) 27(3):505–9. doi: 10.1007/s12282-019-01044-4
90. Hawk SN, Lanoue L, Keen CL, Kwik-Uribe CL, Rucker RB, Uriu-Adams JY. Copper-deficient rat embryos are characterized by low superoxide dismutase activity and elevated superoxide anions. Biol Reprod (2003) 68(3):896–903. doi: 10.1095/biolreprod.102.009167
91. Lynch SM, Frei B, Morrow JD, Roberts LJ 2nd, Xu A, Jackson T, et al. Vascular superoxide dismutase deficiency impairs endothelial vasodilator function through direct inactivation of nitric oxide and increased lipid peroxidation. Arterioscler Thromb Vasc Biol (1997) 17(11):2975–81. doi: 10.1161/01.ATV.17.11.2975
92. Johnson WT, Thomas AC. Copper deprivation potentiates oxidative stress in HL-60 cell mitochondria. Proc Soc Exp Biol Med (1999) 221(2):147–52. doi: 10.3181/00379727-221-44397
93. Fridovich I. Superoxide radical: An endogenous toxicant. Annu Rev Pharmacol Toxicol (1983) 23:239–57. doi: 10.1146/annurev.pa.23.040183.001323
94. Cadenas E, Davies KJ. Mitochondrial free radical generation, oxidative stress, and aging. Free Radic Biol Med (2000) 29(3-4):222–30. doi: 10.1016/S0891-5849(00)00317-8
95. Banci L, Bertini I, Cabelli DE, Hallewell RA, Tung JW, Viezzoli MS, et al. A characterization of copper/zinc superoxide dismutase mutants at position 124. zinc-deficient proteins. Eur J Biochem (1991) 196(1):123–8. doi: 10.1111/j.1432-1033.1991.tb15794.x
96. Roberts BR, Tainer JA, Getzoff ED, Malencik DA, Anderson SR, Bomben VC, et al. Structural characterization of zinc-deficient human superoxide dismutase and implications for ALS. J Mol Biol (2007) 373(4):877–90. doi: 10.1016/j.jmb.2007.07.043
97. Potter SZ, Zhu H, Shaw BF, Rodriguez JA, Doucette PA, Sohn SH, et al. Binding of a single zinc ion to one subunit of copper-zinc superoxide dismutase apoprotein substantially influences the structure and stability of the entire homodimeric protein. J Am Chem Soc (2007) 129(15):4575–83. doi: 10.1021/ja066690+
98. Boyd SD, Ullrich MS, Skopp A, Winkler DD. Copper sources for Sod1 activation. Antioxidants (Basel) (2020) 9(6):500. doi: 10.3390/antiox9060500
99. Liaw KY, Lee PH, Wu FC, Tsai JS, Lin-Shiau SY. Zinc, copper, and superoxide dismutase in hepatocellular carcinoma. Am J Gastroenterol (1997) 92(12):2260–3.
100. Tang JY, Ou-Yang F, Hou MF, Huang HW, Wang HR, Li KT, et al. Oxidative stress-modulating drugs have preferential anticancer effects - involving the regulation of apoptosis, DNA damage, endoplasmic reticulum stress, autophagy, metabolism, and migration. Semin Cancer Biol (2019) 58:109–17. doi: 10.1016/j.semcancer.2018.08.010
101. Arredondo M, González M, Olivares M, Pizarro F, Araya M. Ceruloplasmin, an indicator of copper status. Biol Trace Elem Res (2008) 123(1-3):261–9. doi: 10.1007/s12011-008-8110-2
102. Drakesmith H, Nemeth E, Ganz T. Ironing out ferroportin. Cell Metab (2015) 22(5):777–87. doi: 10.1016/j.cmet.2015.09.006
103. Hellman NE, Gitlin JD. Ceruloplasmin metabolism and function. Annu Rev Nutr (2002) 22:439–58. doi: 10.1146/annurev.nutr.22.012502.114457
104. Arner E, Forrest AR, Ehrlund A, Mejhert N, Itoh M, Kawaji H, et al. Ceruloplasmin is a novel adipokine which is overexpressed in adipose tissue of obese subjects and in obesity-associated cancer cells. PloS One (2014) 9(3):e80274. doi: 10.1371/journal.pone.0080274
105. Knekt P, Aromaa A, Maatela J, Rissanen A, Hakama M, Aaran RK, et al. Serum ceruloplasmin and the risk of cancer in Finland. Br J Cancer (1992) 65(2):292–6. doi: 10.1038/bjc.1992.58
106. Zhang Y, Chen Z, Chen JG, Chen XF, Gu DH, Liu ZM, et al. Ceruloplasmin overexpression is associated with oncogenic pathways and poorer survival rates in clear-cell renal cell carcinoma. FEBS Open Bio (2021) 11(11):2988–3004. doi: 10.1002/2211-5463.13283
107. Han IW, Jang JY, Kwon W, Park T, Kim Y, Lee KB, et al. Ceruloplasmin as a prognostic marker in patients with bile duct cancer. Oncotarget (2017) 8(17):29028–37. doi: 10.18632/oncotarget.15995
108. Ciavarella S, Milano A, Dammacco F, Silvestris F. Targeted therapies in cancer. BioDrugs (2010) 24(2):77–88. doi: 10.2165/11530830-000000000-00000
109. Michniewicz F, Saletta F, Rouaen JRC, Hewavisenti RV, Mercatelli D, Cirillo G, et al. Copper: An intracellular achilles' heel allowing the targeting of epigenetics, kinase pathways, and cell metabolism in cancer therapeutics. ChemMedChem (2021) 16(15):2315–29. doi: 10.1002/cmdc.202100172
110. Wadhwa S, Mumper RJ. D-penicillamine and other low molecular weight thiols: Review of anticancer effects and related mechanisms. Cancer Lett (2013) 337(1):8–21. doi: 10.1016/j.canlet.2013.05.027
111. Lopez J, Ramchandani D, Vahdat L. Copper depletion as a therapeutic strategy in cancer. Met Ions Life Sci (2019) 19:19:/books/9783110527872/9783110527872-018/9783110527872-018.xml. doi: 10.1515/9783110527872-012
112. Baldari S, Di Rocco G, Toietta G. Current biomedical use of copper chelation therapy. Int J Mol Sci (2020) 21(3):1069. doi: 10.3390/ijms21031069
113. Cox C, Merajver SD, Yoo S, Dick RD, Brewer GJ, Lee JS, et al. Inhibition of the growth of squamous cell carcinoma by tetrathiomolybdate-induced copper suppression in a murine model. Arch Otolaryngol Head Neck Surg (2003) 129(7):781–5. doi: 10.1001/archotol.129.7.781
114. Khan MK, Miller MW, Taylor J, Gill NK, Dick RD, Golen Van K, et al. Radiotherapy and antiangiogenic TM in lung cancer. Neoplasia (2002) 4(2):164–70. doi: 10.1038/sj.neo.7900218
115. Pan Q, Bao LW, Kleer CG, Brewer GJ, Merajver SD. Antiangiogenic tetrathiomolybdate enhances the efficacy of doxorubicin against breast carcinoma. Mol Cancer Ther (2003) 2(7):617–22.
116. van Golen KL, Bao L, Brewer GJ, Pienta KJ, Kamradt JM, Livant DL, et al. Suppression of tumor recurrence and metastasis by a combination of the PHSCN sequence and the antiangiogenic compound tetrathiomolybdate in prostate carcinoma. Neoplasia (2002) 4(5):373–9. doi: 10.1038/sj.neo.7900258
117. Khan MK, Mamou F, Schipper MJ, May KS, Kwitny A, Warnat A, et al. Combination tetrathiomolybdate and radiation therapy in a mouse model of head and neck squamous cell carcinoma. Arch Otolaryngol Head Neck Surg (2006) 132(3):333–8. doi: 10.1001/archotol.132.3.333
118. Park KC, Fouani L, Jansson PJ, Wooi D, Sahni S, Lane DJ, et al. Copper and conquer: copper complexes of di-2-pyridylketone thiosemicarbazones as novel anti-cancer therapeutics. Metallomics (2016) 8(9):874–86. doi: 10.1039/C6MT00105J
119. Denoyer D, Clatworthy SAS, Cater MA. Copper complexes in cancer therapy. Met Ions Life Sci (2018) 18:/books/9783110470734/9783110470734-022/9783110470734-022.xml. doi: 10.1515/9783110470734-022
120. Cater MA, Haupt Y. Clioquinol induces cytoplasmic clearance of the X-linked inhibitor of apoptosis protein (XIAP): therapeutic indication for prostate cancer. Biochem J (2011) 436(2):481–91. doi: 10.1042/BJ20110123
121. Chen D, Cui QC, Yang H, Barrea RA, Sarkar FH, Sheng S, et al. Clioquinol, a therapeutic agent for alzheimer's disease, has proteasome-inhibitory, androgen receptor-suppressing, apoptosis-inducing, and antitumor activities in human prostate cancer cells and xenografts. Cancer Res (2007) 67(4):1636–44. doi: 10.1158/0008-5472.CAN-06-3546
122. Chen D, Cui QC, Yang H, Dou QP. Disulfiram, a clinically used anti-alcoholism drug and copper-binding agent, induces apoptotic cell death in breast cancer cultures and xenografts via inhibition of the proteasome activity. Cancer Res (2006) 66(21):10425–33. doi: 10.1158/0008-5472.CAN-06-2126
123. Duan WJ, He RR. Cuproptosis: Copper-induced regulated cell death. Sci China Life Sci (2022) 65(8):1680–2. doi: 10.1007/s11427-022-2106-6
124. Kazan K, Kalaipandian S. Ferroptosis: Yet another way to die. Trends Plant Sci (2019) 24(6):479–81. doi: 10.1016/j.tplants.2019.03.005
125. Tsvetkov P, Detappe A, Cai K, Keys HR, Brune Z, Ying W, et al. Mitochondrial metabolism promotes adaptation to proteotoxic stress. Nat Chem Biol (2019) 15(7):681–9. doi: 10.1038/s41589-019-0291-9
126. Bao JH, Lu WC, Duan H, Ye YQ, Li JB, Liao WT, et al. Identification of a novel cuproptosis-related gene signature and integrative analyses in patients with lower-grade gliomas. Front Immunol (2022) 13:933973. doi: 10.3389/fimmu.2022.933973
127. Huang X, Zhou S, Tóth J, Hajdu A. Cuproptosis-related gene index: A predictor for pancreatic cancer prognosis, immunotherapy efficacy, and chemosensitivity. Front Immunol (2022) 13:978865. doi: 10.3389/fimmu.2022.978865
128. Sha S, Si L, Wu X, Chen Y, Xiong H, Xu Y, et al. Prognostic analysis of cuproptosis-related gene in triple-negative breast cancer. Front Immunol (2022) 13:922780. doi: 10.3389/fimmu.2022.922780
129. Wang T, Liu Y, Li Q, Luo Y, Liu D, Li B, et al. Cuproptosis-related gene FDX1 expression correlates with the prognosis and tumor immune microenvironment in clear cell renal cell carcinoma. Front Immunol (2022) 13:999823. doi: 10.3389/fimmu.2022.999823
130. Bian Z, Fan R, Xie L. A novel cuproptosis-related prognostic gene signature and validation of differential expression in clear cell renal cell carcinoma. Genes (Basel) (2022) 13(5):851. doi: 10.3390/genes13050851
131. Zhu Z, Zhao Q, Song W, Weng J, Li S, Guo T, et al. A novel cuproptosis-related molecular pattern and its tumor microenvironment characterization in colorectal cancer. Front Immunol (2022) 13:940774. doi: 10.3389/fimmu.2022.940774
132. Lv H, Liu X, Zeng X, Liu Y, Zhang C, Zhang Q, et al. Comprehensive analysis of cuproptosis-related genes in immune infiltration and prognosis in melanoma. Front Pharmacol (2022) 13:930041. doi: 10.3389/fphar.2022.930041
133. Zhang Z, Zeng X, Wu Y, Liu Y, Zhang X, Song Z. Cuproptosis-related risk score predicts prognosis and characterizes the tumor microenvironment in hepatocellular carcinoma. Front Immunol (2022) 13:925618. doi: 10.3389/fimmu.2022.925618
134. Liu Y, Liu Y, Ye S, Feng H, Ma L. Development and validation of cuproptosis-related gene signature in the prognostic prediction of liver cancer. Front Oncol (2022) 12:985484. doi: 10.3389/fonc.2022.985484
135. Li H, Zu X, Hu J, Xiao Z, Cai Z, Gao N, et al. Cuproptosis depicts tumor microenvironment phenotypes and predicts precision immunotherapy and prognosis in bladder carcinoma. Front Immunol (2022) 13:964393. doi: 10.3389/fimmu.2022.964393
136. Yang L, Zhang Y, Wang Y, Jiang P, Liu F, Feng N, et al. Ferredoxin 1 is a cuproptosis-key gene responsible for tumor immunity and drug sensitivity: A pan-cancer analysis. Front Pharmacol (2022) 13:938134. doi: 10.3389/fphar.2022.938134
137. Xiao C, Yang L, Jin L, Lin W, Zhang F, Huang S, et al. Prognostic and immunological role of cuproptosis-related protein FDX1 in pan-cancer. Front Genet (2022) 13:962028. doi: 10.3389/fgene.2022.962028
Keywords: copper, cancer, copper metabolism, cuproptosis, copper complexes, cancer therapeutics
Citation: Wang Z, Jin D, Zhou S, Dong N, Ji Y, An P, Wang J, Luo Y and Luo J (2023) Regulatory roles of copper metabolism and cuproptosis in human cancers. Front. Oncol. 13:1123420. doi: 10.3389/fonc.2023.1123420
Received: 16 December 2022; Accepted: 08 March 2023;
Published: 23 March 2023.
Edited by:
Alessandra di Masi, Roma Tre University, ItalyReviewed by:
Wukun Liu, Nanjing University of Chinese Medicine, ChinaCopyright © 2023 Wang, Jin, Zhou, Dong, Ji, An, Wang, Luo and Luo. This is an open-access article distributed under the terms of the Creative Commons Attribution License (CC BY). The use, distribution or reproduction in other forums is permitted, provided the original author(s) and the copyright owner(s) are credited and that the original publication in this journal is cited, in accordance with accepted academic practice. No use, distribution or reproduction is permitted which does not comply with these terms.
*Correspondence: Jiaping Wang, d2FuZ2ppYXBpbmdfMTExM0AxNjMuY29t; Yongting Luo, bHVvLnlvbmd0aW5nQGNhdS5lZHUuY24=; Junjie Luo, bHVvampAY2F1LmVkdS5jbg==
†These authors have contributed equally to this work
Disclaimer: All claims expressed in this article are solely those of the authors and do not necessarily represent those of their affiliated organizations, or those of the publisher, the editors and the reviewers. Any product that may be evaluated in this article or claim that may be made by its manufacturer is not guaranteed or endorsed by the publisher.
Research integrity at Frontiers
Learn more about the work of our research integrity team to safeguard the quality of each article we publish.