- Institute of Medical Sciences, The Second Hospital of Shandong University, Jinan, China
Currently, cancer has become one of the major public health problems worldwide. Apoptosis is an important anti-cancer defense mechanism, which is used in the development of targeted drugs. Because cancer cells have endogenous resistance to apoptosis,the clinical efficacy of related drugs is not ideal. Therefore, non-apoptotic regulatory cell death may bring new therapeutic strategies for cancer treatment. Cuproptosis is a novel form of regulatory cell death which is copper-dependent, regulated and distinct from other known cell death regulatory mechanisms. FDX1,LIAS,and DLAT named cuproptosis-related genes play an essential role in regulating cuproptosis. Meanwhile, abnormal accumulation of copper can be observed in various malignant tumors. The correlation has been established between elevated copper levels in serum and tissues and the progression of several cancers. Copper transporters, CTR1 and Copper-transporting ATPases(ATP7A and ATP7B), are mainly involved in regulating the dynamic balance of copper concentration to maintain copper homeostasis. Thus,cuproptosis-related genes and copper transporters will be the focus of cancer research in future. This review elaborated the basic functions of cuproptosis-related genes and copper transporters by retrievalling PubMed. And then we analyzed their potential relationship with cancer aiming to provide theoretical support and reference in cancer progression, diagnosis and treatment for future study.
1 Introduction
Cancer has become one of the major public health problems worldwide. According to the 2022 Cancer Progress Report released by the American Association for Cancer Research (AACR), there are 18.1 million new cancers globally in 2020, resulting in 9.89 million patients deaths. It is estimated there will be 28 million new cancers worldwide by 2040 (1). Indeed, the fundamental purpose of cancer therapy is to selectively reduce cancer cells and induce cancer cell death. Therefore, researchers have never stopped exploring and studying any type of cell death.
Cell death is a conservative phenomenon that prokaryotic and eukaryotic cells fail to maintain basic life functions.Generally speaking, cell death is divided into accidental cell death (ACD) and regulated cell death (RCD). ACD is an uncontrollable biological process, while RCD has precise molecular mechanisms and complex signal regulation pathways (2). At present, there are dozens of RCD, in which cell apoptosis, pyroptosis, autophagy, ferroptosis and necroptosis have been extensively studied (3). Apoptosis is a pivotal anti-cancer defense mechanism, which is applied to the development of targeted drugs. Because cancer cells have endogenous resistance to apoptosis, the clinical therapeutic effect of relevant drugs is not ideal (4). Thus, non-apoptotic regulatory cell death may bring novel therapeutic strategies for cancer treatment.
In 2022, Tsvetkov et al. (5) proposed a new form of regulatory cell death called “cuproptosis” in Science. Cuproptosis is triggered by the direct combination of copper ions with the lipoacylation component in the tricarboxylic acid (TCA) cycle.This leads to the aggregation of lipid-acylated- related proteins and downregulation of iron-sulfur cluster proteins,eventually resulting in protein toxicity stress and cell death.The team found ten genes involved in the cuproptosis through the whole genome CRISPR-Cas9 function deletion screening.There are seven positive regulatory genes (ferredoxin 1(FDX1), lipoic acid synthase (LIAS), lipoyl transferase 1 (LIPT1), drolipoamide S- acetyltransferase (DLAT), dihydrolipoamide dehydrogenase (DLD), pyruvate dehydrogenase E1 subunit alpha 1 (PDHA1), pyruvate dehydrogenase E1 subunit beta (PDHB))and three negative regulatory genes (metal-regulatory transcription factor-1 (MTF1),glutaminase (GLS), and cyclin- dependent kinase inhibitor 2A (CDKN2A)). Simultaneously, there is abnormal accumulation of copper in multiple malignant cancers (6–8). A significant role has been played in promoting tumor cell proliferation, metastasis and angiogenesis by copper (7, 9). All this suggests unbalanced copper homeostasis has an impact on the tumor. Copper transporters 1(CTR1) and copper-transporting ATPases(ATP7A and ATP7B) participate in the transport and distribution of copper to maintain copper homeostasis (10, 11). Therefore, it is of great significance to study the implication of cuproptosis-related genes and copper transporters in the development of cancer. In this study, we retrieved the functions of three key cuproptosis-related genes (FDX1, LIAS, DLAT) and copper transporters (CTR1,ATP7A and ATP7B).Then we analyzed their potential relationship with cancer so as to provide theoretical support and reference for future research on cancer progression,diagnosis and treatment.
2 Cuproptosis-related genes and cancer
Actually, Tsvetkov et al. (12) in 2019 found the copper ionophore elesclomol-Cu2+ complex entered cells and FDX1 reduced Cu2+ to Cu+,which ultimately led to a unique copper dependent cell death and could not be blocked by apoptosis inhibitors or ferroptosis inhibitors.However, the mechanism was unclear. In March 2022, based on the above research, Tsvetkov et al. (5) named this copper- dependent cell death as”cuproptosis”. This study demonstrates cuproptosis is closely related to the TCA cycle. Through genome wide CRISPR-Cas9 function deletion screening, it is found that the necessary condition for copper binding is protein lipoylation. And FDX1 is the upstream regulator of protein lipoylation. By knocking down the single genes FDX1 and LIAS, the deletion of FDX1 and LIAS could enhance the resistance to copper-induced cell death. Thus, FDX1 and protein lipoylation are the key regulators of cuproptosis. Additionally, the combination of copper with lipoylated protein DLAT will induce unstable expression of iron-sulfur cluster protein causing protein toxicity stress and cell death. This brand-new form of non-apoptotic regulatory cell death will be a hot spot for cancer treatment in the future. As three key genes in cuproptosis, FDX1, LIAS, and DLAT, are expected to be the targets in cancer therapy. Next, I will summarize the functions of these genes and their current research on cancer.
2.1 FDX1
Iron–sulfur cluster proteins are a kind of protein cofactors that modulates various biochemical processes, including mitochondrial respiration, electron transfer, redox catalysis and biosynthesis (13, 14). The human genome contains two homologous ferredoxins, ferredoxin 1 (FDX1) and ferredoxin 2 (FDX2). FDX1 produces a market effect on steroidogenesis, while FDX2 is involved in the synthesis of Fe -S cluster and heme A (15).
One of the signs of cancer is cellular metabolism disorders (16). It happens that the main function of FDX1 is to regulate substance metabolism. Hence, researchers have explored the association between FDX1 and cancer. Downregulation of FDX1 significantly changes glucose, amino acid, and fatty acid oxidative metabolism in lung adenocarcinoma. Meanwhile, the prognosis of lung adenocarcinoma patients with low expression of FDX1 is even worse (17). This is consistent with the previous studies.The most common manifestation of lung cancer metabolism is abnormal glucose and lactic acid metabolism (18). Besides, the steroid synthesis regulated by FDX1 may be related to the occurrence of tumors. FDX1 is involved in regulating steroid hormone synthesis, receiving electrons transferred from NADPH via Adx reductase, which in turn reduces members of the mitochondrial cytochrome P450 (CYP450). CYP450 mainly includes CYP1, CYP2 and CYP3 families. CYP1B1 in the CYP1 family catalyzes the conversion of estradiol to 4-hydroxy-17-β-estradiol (4-OHE2) (19). The metabolite of estradiol,4-OHE2, is involved in the development of some hormone-related cancers. For example, 4-OHE2 undergoes the redox cycle, which produces reactive oxygen species (ROS) and chemoreactive estrogen semiquinone, thereby promoting the occurrence of breast cancer (20). In endometrial cancer, 4-OHE2 participates in the occurrence of endometrial cancer by inducing PTEN mutation on codon 130/131 (21). So the potential association between FDX1 regulating CYP450 and the pathogenesis of hormone-related cancer deserves further study. From this, we speculate FDX1 may be involved in the metabolic disorder during the occurrence and development of cancer, subsequently changing its phenotype and tumor microenvironment.
With the proposal of cuproptosis, a research conducted systematic bioinformatic database analysis on FDX1. Firstly, the expression of FDX1 at mRNA and protein levels was reduced in most cancers, such as breast invasive carcinoma (BRCA), colon adenocarcinoma (COAD), lung adenocarcinoma (LUAD), thyroid carcinoma (THCA), etc. Secondly, the expression of FDX1 was related to the clinical features, anti-tumor drug sensitivity and drug resistance in some cancer types.Simultaneously, gene-set enrichment analysis showed tight correlation between FDX1 and immune-related pathways, immune cell infiltration, and immunoregulatory genes (22). Although the analysis of public bioinformatic database has some limitations due to the limited clinical and pathological information, it also indicates FDX1 is expected to become a potential target for cancer treatment and a biomarker for evaluating prognosis in the future.
2.2 LIAS
Lipoic acid is an antioxidant synthesized in mitochondria. It can not only clear free radicals,but also participate in regulating mitochondrial energy metabolism and oxidative stress. Lipoic acid synthase (LIAS) belongs to the biotin and lipoic acid synthase family, which is involved in catalyzing the final step of lipoic acid synthesis (23). Relevant studies have shown the lower expression of LIAS can promote the progression of diseases such as diabetes, atherosclerosis and neonatal epilepsy by increasing oxidative stress (24–26). Downregulating LIAS by RNA interference may lead to redox imbalance, mitochondrial dysfunction and inflammation (27). As a result, LIAS is related to mitochondrial energy metabolism and antioxidant defense. Mitochondria participate in electron chain transfer and oxidative phosphorylation through TCA cycle. Some tumors with high level of oxidative phosphorylation (such as breast cancer, melanoma, cholangiocarcinoma, etc) are mainly powered by it.Studies have applied drugs targeting oxidative phosphorylation such as PGC-1α to inhibit the proliferation of tumor cells (28–30). Therefrom, we surmise LIAS may become a latent therapeutic target for mitochondrial respiration-dependent tumors. Moreover, experiments have proved the mutation of LIAS inhibits the activity of proline hydroxylase (PHDs), thereby promoting the activation of HIF-1 (31). It is universally acknowledged that the higher expression of HIF-1 is a prerequisite for tumor cell proliferation and migration, angiogenesis and epithelial-mesenchymal transition (EMT) (32). Consequently, LIAS may have an effect on tumor proliferation and invasion by regulating HIF-1. Finally, the effect of LIAS on oxidative stress and inflammation provides clues to its role in tumor immunotherapy. In the atherosclerotic mouse model with the overexpression of LIAS, it was found that the number of Treg was increased and T cell infiltration was reduced (25). Similarly,in pulmonary fibrosis mice with the overexpression of LIAS, the inhibition of NF-kB relieved the chronic inflammatory response, showing an increase in the number of Treg cells and a decrease in T cell infiltration (33). The latest pan-cancer analysis of the bioinformatics database on LIAS is consistent with the above experimental conclusions. Researchers found the expression of LIAS was correlated with the infiltration of immune cells (34). In the tumor microenvironment, increased Treg cells will inhibit the activation and function of effector T cells, resulting in the immune escape of tumor cells (35). In conclusion, LIAS may have far-reaching effect on the proliferation, angiogenesis and immune escape of tumor.
2.3 DLAT
Pyruvate dehydrogenase complex (PDC) is a multienzyme complex located in mitochondria that catalyzes pyruvate, transfers acetyl groups to coenzyme A through DLAT, finally transforms to acetyl-CoA. DLAT is the E2 subunit of PDC complex, which is critical to TCA cycle (36). So far, a series of studies have demonstrated the role of DLAT in tumors. Wen et al. (37)observed the expression of DLAT was appreciably upregulated in gastric cancer cells, which promoted oxidative phosphorylation and provide energy for tumor cells by catalyzing the conversion of pyruvate to acetyl-CoA. Shan et al. (38) found DLAT promoted tumor cell proliferation and growth by elevating the level of 6PGD lysine acetylation in primary leukemia. According to the research on non-small cell lung cancer, PM2.5 upregulated the expression of DLAT to promote glycolysis through the dual regulation mechanism of Sp1-DLAT and eIF4E-DLAT axis, enhancing the proliferation of tumor cells (39).
Next, we have noticed the association between E4F1 and tumors, which is involved in the modulation of PDC subunits.E4F1 was initially identified as a cellular target of the viral oncoprotein E1A. It regulates DLAT transcription to control the metabolism of pyruvate oxidation pathway (40). Most importantly, E4F1 mediates the ubiquitination of tumor suppressor gene p53, enhancing its transcriptional activity and eventually arresting the cell cycle (41). Accordingly, E4F1 may affect the development of cancer by coordinating p53 and DLAT.
Finally, DLAT is a biological substrate of Sirtuin 4 (SIRT4) lipoamidase activity. SIRT4 induces DLAT hydrolysis to regulate TCA cycle by lipoyl- and biotinyl-lysine modifications of DLAT (42). According to the classical theory “Warburg effect”, most tumor cells obtain energy through aerobic glycolysis. It is considered to be a marker of cancer progression and metastasis (43). It was found that the glycolysis was decreased in overexpressing SIRT4 hepatocellular carcinoma (HCC) cell lines. In other words, the overexpression of SIRT4 will reduce the energy obtained by the tumor (44). To sum up, DLAT may influence tumor development by regulating pyruvate oxidation, TCA cycle and glycolysis.
3 Copper transporters and cancer
On the one hand,Copper is an essential cofactor for enzymes that mediates cell functions. On the other hand, the abnormal accumulation of copper also induce oxidative stress and cytotoxicity (45). Therefore, copper homeostasis is the guarantee to maintain the basic cell functions. Copper transport in vivo involves a variety of copper chaperones and copper transporters. Metal reductase STEAP reduces extracellular Cu2+to Cu+.Then copper transport protein 1 (CTR1) transfers Cu+ from outside the cell into it. Copper ions entering the cell are transported through copper chaperone. The leading pathways are as follows: ① Anti oxidant 1(ATOX1) transports copper to trans-Golgi network (TGN) and then combines with copper-transporting ATPases to expel excessive copper ion. ② Copper enters mitochondria through SLC25A3 for storage.In mitochondria,cytochrome c oxidase 17(COX17) directly delivers copper to cytochrome c oxidase 11 (COX11) and synthesis of cytochrome oxidase protein.COX11 and synthesis of cytochrome oxidase 1(SCO1) are the metallochaperone that inserts copper into the cytochrome c oxidase 1(COX1) and cytochrome c oxidase 2(COX2) respectively. Cytochrome c oxidase assembly factor 6 (COA6) and SCO2 help to maintain the redox balance of SCO1 and in turn its copper binding and delivery to cytochrome c oxidase (COX).Together,these proteins maintain appropriate intracellular copper bioavailability and ensure metallation of copper-dependent enzymes. ③ Copper chaperone for super-oxide dismutase 1(CCS) transports copper to superoxide dismutase 1 (SOD1) so as to remove free radicals (46–50).
Actually,Copper chaperone also has potential role in various tumors. In melanoma, ATOX 1 deficiency reduces MEK levels in copper-binding MAPK signaling and suppresses MAPK signaling activation (51). Li Y et al. (52) found CCS promoted the growth and migration of breast cancer cells by regulating ROS-mediated ERK1/2 activity.In leukemia, COX17 inhibition can increase the level of mitochondrial copper, thereby affecting methionine metabolism and DNA methylation, and reduce the activity of leukemia stem cells (53). SCO2 may synergisticallyinfluence tumor progression by promoting mitochondrial respiration (54).
Next, I will systematically summarize the role of CTR1 and Copper-transporting ATPases in tumorigenesis and development.
3.1 CTR1
CTR1 is a high-affinity copper transporter encoded by SLC31A1. The C terminus of CTR1 is intracellular and the N terminus is extracellular. It is arranged in the form of homotrimer on the cell membrane to facilitate the inflow of copper. In the pregnant mouse model with the deficiency of CTR1, obvious growth and development defects was discovered in embryo. Even worse, some embryos would die in utero in the secong trimester of pregnancy. This demonstrates CTR1 is crucial in embryonic development (55).
Actually, studies have shown CTR1 is highly expressed in melanoma, liver cancer, prostate cancer and other human cancer cell lines (56). Subsequently,many reports investigate the effect of CTR1 on tumor progression from tumor-related signaling pathways, angiogenesis and platinum drug resistance. First and foremost, CTR1 mediates copper causing cell signal cascade disorder indirectly, which leads to the occurrence and development of tumors. Abnormal activation of mitogen-activated protein kinases (MAPK) signaling is a classic hallmark of several malignant tumors. The mutation of RAS and BRAF is the most common (57). In 2014, Counter Research Group (9)confirmed downregulating the expression of CTR1 may influence the combination of MEK1 and copper, thereby inhibiting ERK signaling pathway mediated by BRAF and ultimatingly suppressing the tumor growth. In other words, copper modulated by CTR1 regulates BRAF/MEK/ERK signaling pathway indirectly.
Secondly, angiogenesis is the initial process of tumor proliferation and metastasis (58). Earlier studies have indicated copper is a vital element in promoting angiogenesis. It can not only activate the main factors of angiogenesis by HIF-1, but also combine with angiogenin to promote angiogenesis (59). In human umbilical vein endothelial cells (HUVECs), the downregulation of CTR1 prevented copper from entering vascular endothelial cells and reduced angiogenesis (60). Another experiment is in line with the above conclusion. Three peptides rich in histidine and methionine are designed in the copper-binding region of CTR1, preventing copper from entering cells and inhibiting angiogenesis (61). Hence,the inhibition of CTR1 causing unbalanced copper may become a new idea for anti-angiogenesis.
Last but not least, studies have deeply explored the relationship between chemoresistance to cisplatin and the expression of CTR1. Platinum drugs are one of the most common chemotherapeutic agents for many solid tumors. Resistance of tumor cells to cisplatin is the key reason for the poor effect of chemotherapy. Studies have revealed CTR1 is the major transporter of platinum drugs (62). In addition, patients who had received platinum chemotherapy with stage III non-small cell lung cancer were selected to study their tumor tissues by immunohistochemistry experiments. The results indicated the high expression of CTR1 had a better response to cisplatin treatment and favourable prognosis (63). In human ovarian cancer cells, cisplatin downregulated the levels of CTR1 in time- dependent and concentration-dependent manner (64). Apparently, CTR1 has the potential to be a new target to overcome platinum resistance.
3.2 Copper-transporting ATPases (ATP7A and ATP7B)
ATP7A and ATP7B are two homologous isoforms of copper-transporting P-type ATPases. In order to expel excessive copper from the body, ATP7A transports copper to the basement membrane of extracellular matrix, while ATP7B transports it out of hepatic cells and into bile. The mutation of ATP7A and ATP7B will damage the nervous system, leading to Menkes disease and Wilson disease respectively (65). Additionally, ATP7A and ATP7B also produce a marked effect in tumor progression. First, The members of LOX family modulates extracellular matrix (ECM) remodeling leading to tumor cell migration (66). According to a study applying CRISPR/Cas9 to silence ATP7A,the activity of LOX was inhibited in breast cancer and lung cancer cell lines,suppressing these tumor cell growth and migration (67). Meanwhile, KRAS-mutant colorectal cancer obtained high levels of copper through pinocytosis accompanied by the upregulation of ATP7A (68). Furthermore, The combination of ATP7A and VEGFR2 can prevent the degradation of VEGFR2 to promote angiogenesis through P62-SQSTM1 pathway (69). It is noteworthy ATP7A or ATP7B can bind to platinum drugs to expel it from cancer cells, producing chemotherapy drug resistance. In breast cancer cell MDA-MB-231 with ATP7A knocked out, the ability of cisplatin to reduce cell proliferation was enhanced. This study indicated the overexpression of miR-148a-3p negatively regulates ATP7A, which will increase the sensitivity of breast cancer cells to cisplatin (70). Similarly, targeting ATP7B negatively regulated by miR139 will reduce the chemical resistance of ovarian cancer cells to platinum drugs (71). In conclusion, targeting Copper-transporting ATPases,ATP7A or ATP7B,has broad application space in overcoming the resistance of tumor cells to platinum drugs.
4 Conclusion
Given that copper accumulation occurs in various cancer, it is reasonable to speculate the potential association of cuproptosis–related genes and copper transporters with cancer is multifaceted(Figure 1). Based on the finding copper chelator inhibit copper-induced cell death, it is pivotal to deprive appropriate copper to reduce the level of Cu2+ under overloaded copper conditions. Specifically,using copper chelator or genetically modifying cuproptosis-related genes and copper transporters might be feasible strategies. Additionally, considering the differences in the abundance and respiratory patterns of lipoylated proteins in tumors, it is necessary to study the precise concentration range leading to cytotoxicity in various disease models,and then establish a reasonable personalized treatment.As cuproptosis was identifified recently, there is no reliable biomarker which limits our ability to determine whether cuproptosis is involved in human pathological. Thus, reliable and sensitive biomarkers of cuproptosis are needed to be identifified in different disease settings. FDX1, LIAS and DLAT are upstream regulatory genes in cuproptosis. At the same time, copper transporters,CTR1,ATP7A and ATP7B, play a crucial role in copper homeostasis. This review systematically sorted out the functions of the above five key genes.Based on their effects on cancer,we analyzed their intrinsic values in cancer. We have a preliminary summary in Table 1. By modulating different substance metabolism,FDX1,LIAS and DLAT, exert impact on tumor cell proliferation, migration, angiogenesis, immune arrest.Specifically,FDX1regulates steroid synthesis. Lias regulates mitochondrial energy metabolism and oxidative stress. DLAT regulates pyruvate oxidation, TCA cycle and glycolysis. These principal upstream regulatory genes in cuproptosis are also of great reference for studying downstream regulatory genes in the future. CTR1 and copper-transporting ATPases mainly influence the resistance of tumor cells to platinum drugs. Regretfully, it is unclear whether their mechanism associated with cuproptosis or not. So far, the research on cuproptosis and its related genes in cancer is limited.It is still at the stage of exploring its connection with cancer,which is lack of fundamental experiment study to prove their cause-and-effect. Undeniably,it provides a research direction with enormous potential for cancer treatment.
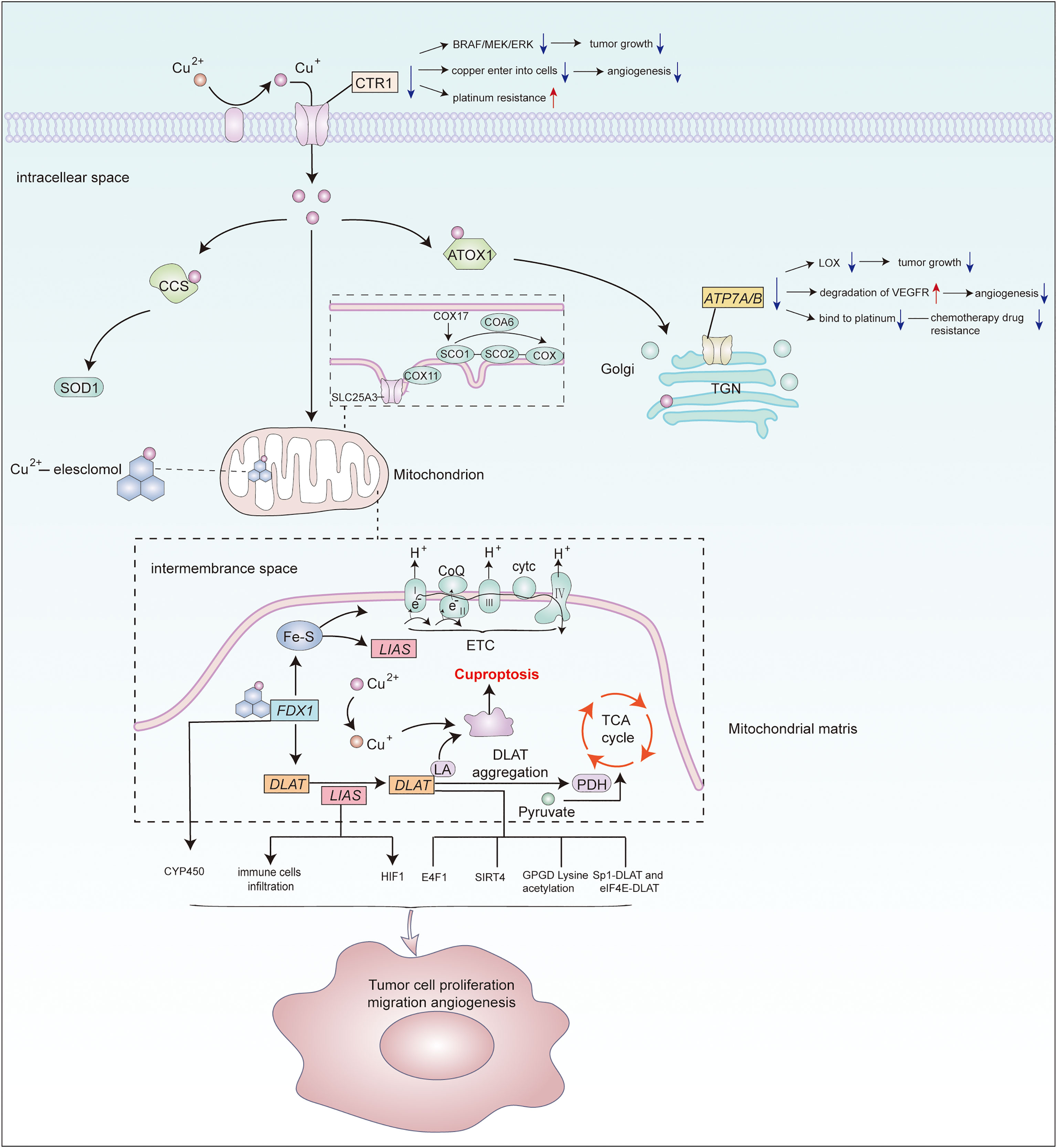
Figure 1 Potential association of cuproptosis–related genes and copper transporters with cancer. Metal reductase STEAP reduces extracellular Cu2+to Cu+,then CTR1 transfers Cu+ from outside the cell into it.Cytoplasmic and mitochondrial metallochaperones (ATOX1, CCS, SCO1, SCO2, COX11, COX17) working in concert to ensure targeted insertion of copper into metalloprotein.In mitochondria,copper enters it through SLC25A3 for storage.COA6 and SCO2 help to maintain the redox balance of SCO1 and in turn its copper binding and delivery to COX.ATP7A and ATP7B perform copper export function.Copper can induce cuproptosis by binding to lipid-acylated TCA cycle components, promoting lipid-acylated protein aggregation, and inducing protein stress.Vital regulatory genes in cuproptosis or copper homeostasis may regulate various molecules or signaling pathway to affect cancer progression. However, the specifific mechanisms require further study.
Author contributions
QZ conceived the framework, searched literature, integrated data, made the table and wrote the manuscript. TQ reviewed the paper and provided fundings. All authors contributed to the article and approved the submitted version.
Funding
This work was supported by National Natural Science Foundation of China (No. 81472766) and project ZR2020MH224 supported by Shandong Provincial Natural Science Foundation.
Conflict of interest
The authors declare that the research was conducted in the absence of any commercial or financial relationships that could be construed as a potential conflict of interest.
Publisher’s note
All claims expressed in this article are solely those of the authors and do not necessarily represent those of their affiliated organizations, or those of the publisher, the editors and the reviewers. Any product that may be evaluated in this article, or claim that may be made by its manufacturer, is not guaranteed or endorsed by the publisher.
References
1. Williams PA, Zaidi SK, Sengupta R. AACR cancer progress report 2022: Decoding cancer complexity, integrating science, and transforming patient outcomes. Clin Cancer Res (2022) 28(19):4178–9. doi: 10.1158/1078-0432.CCR-22-2588
2. Galluzzi L, Vitale I, Aaronson SA, Abrams JM, Adam D, Agostinis P. Molecular mechanisms of cell death: Recommendations of the nomenclature committee on cell death 2018. Cell Death Differ (2018) 25(3):486–541. doi: 10.1038/s41418-017-0012-4
3. Tang D, Kang R, Berghe TV, Vandenabeele P, Kroemer G. The molecular machinery of regulated cell death. Cell Res (2019) 29(5):347–64. doi: 10.1038/s41422-019-0164-5
4. Liang C, Zhang X, Yang M, Dong X. Recent progress in ferroptosis inducers for cancer therapy. Advanced Materials (2019) 31:1904197. doi: 10.1002/adma.201904197
5. Tsvetkov P, Coy S, Petrova B, Dreishpoon M, Verma A, Abdusamad M, et al. Copper induces cell death by targeting lipoylated TCA cycle proteins. Science (2022) 375(6586):1254–61. doi: 10.1126/science.abf0529
6. Margalioth EJ, Schenker JG, Chevion M. Copper and zinc levels in normal and malignant tissues. Cancer (1983) 52:868–72. doi: 10.1002/1097-0142(19830901)52:5<868::AID-CNCR2820520521>3.0.CO;2-K
7. Finney L, Vogt S, Fukai T, Glesne D. Copper and angiogenesis: Unravelling a relationship key to cancer progression. Clin Exp Pharmacol Physiol (2009) 36:88–94. doi: 10.1111/j.1440-1681.2008.04969.x
8. Denoyer D, Masaldan S, La Fontaine S, Cater MA. Targeting copper in cancer therapy: “Copper that cancer”. Metallomics (2015) 7:1459–76. doi: 10.1039/C5MT00149H
9. Brady DC, Crowe MS, Turski ML, Hobbs GA, Yao X, Chaikuad A, et al. Copper is required for oncogenic BRAF signalling and tumorigenesis. Nature (2014) 509(7501):492–6. doi: 10.1038/nature13180
10. Wee NK, Weinstein DC, Fraser ST, Fraser ST, Assinder SJ. The mammalian copper transporters CTR1 and CTR2 and their roles in development and disease. Int J Biochem Cell Biol (2013) 45(5):960–3. doi: 10.1016/j.biocel.2013.01.018
11. Lukanović D, Herzog M, Kobal B, Černe K. The contribution of copper efflux transporters ATP7A and ATP7B to chemoresistance and personalized medicine in ovarian cancer. Biomed Pharmacother (2020) 129:110401. doi: 10.1016/j.biopha.2020.110401
12. Tsvetkov P, Detappe A, Cai K, Keys HR, Brune Z, Ying W, et al. Mitochondrial metabolism promotes adaptation to proteotoxic stress. Nat Chem Biol (2019) 15(7):681–9. doi: 10.1038/s41589-019-0291-9
13. Sheftel AD, Stehling O, Pierik AJ, Elsässer HP, Mühlenhoff U, Webert H, et al. Humans possess two mitochondrial ferredoxins, Fdx1 and Fdx2, with distinct roles in steroidogenesis, heme, and Fe/S cluster biosynthesis. In: Proceedings of the national academy of sciences of the united states of America, vol. 107. (2010). p. 11775–80.
14. Py B, Barras F. Building fe-s proteins: Bacterial strategies. Nat Rev Microbiol (2010) 8:436–46. doi: 10.1038/nrmicro2356
15. Johnson DC, Dean DR, Smith AD, Johnson MK. Structure, function, and formation of biological iron-sulfur clusters. Annu Rev Biochem (2005) 74:247–81. doi: 10.1146/annurev.biochem.74.082803.133518
16. Hanahan D. Hallmarks of cancer: New dimensions. Cancer Discov (2022) 12:31–46. doi: 10.1158/2159-8290.CD-21-1059
17. Zhang Z, Ma Y, Guo X, Du Y, Zhu Q, Wang X, et al. FDX1 can impact the prognosis and mediate the metabolism of lung adenocarcinoma. Front Pharmacol (2021) 12:749134. doi: 10.3389/fphar.2021.749134
18. Hensley CT, Faubert B, Yuan Q, Lev-Cohain N, Jin E, Kim J, et al. Metabolic heterogeneity in human lung tumors. Cell (2016) 164:681–94. doi: 10.1016/j.cell.2015.12.034
19. Zhao F, Hao Z, Zhong Y, Xu Y, Guo M, Zhang B, et al. Discovery of breast cancer risk genes and establishment of a prediction model based on estrogen metabolism regulation. BMC Cancer (2021) 21(1):194. doi: 10.1186/s12885-021-07896-4
20. Park SA, Na HK, Surh YJ. Resveratrol suppresses 4-hydroxyestradiol-induced transformation of human breast epithelial cells by blocking IκB kinaseβ-NF-κB signalling. Free Radical Res (2012) 46(8):1051–7. doi: 10.3109/10715762.2012.671940
21. Ke H, Suzuki A, Miyamoto T, Kashima H, Shiozawa T. 4-hydroxy estrogen induces DNA damage on codon 130/131 of PTEN in endometrial carcinoma cells. Mol Cell Endocrinol (2014) 400:71–7. doi: 10.1016/j.mce.2014.10.027
22. Zhang C, Zeng Y, Guo X, Shen H, Zhang J, Wang K, et al. Pan-cancer analyses confirmed the cuproptosis-related gene FDX1 as an immunotherapy predictor and prognostic biomarker. Front Genet (2022) 13:923737. doi: 10.3389/fgene.2022.923737
23. Krishnamoorthy E, Hassan S, Hanna LE, Padmalayam I, Rajaram R, Viswanathan V. Homology modeling of homo sapiens lipoic acid synthase: Substrate docking and insights on its binding mode. J Theor Biol (2017) 420:259–66. doi: 10.1016/j.jtbi.2016.09.005
24. Xu L, Hiller S, Simington S, Nickeleit V, Maeda N, James LR, et al. Influence of different levels of lipoic acid synthase gene expression on diabetic nephropathy. PloS One (2016) 11(10):e0163208. doi: 10.1371/journal.pone.0163208
25. Tian S, Nakamura J, Hiller S, Simington S, Holley DW, Mota R, et al. New insights into immunomodulation via overexpressing lipoic acid synthase as a therapeutic potential to reduce atherosclerosis. Vascul Pharmacol (2020) 133-134:106777. doi: 10.1016/j.vph.2020.106777
26. Mayr JA, Zimmermann FA, Fauth C, Bergheim C, Meierhofer D, Radmayr D, et al. Lipoic acid synthetase deficiency causes neonatal-onset epilepsy, defective mitochondrial energy metabolism, and glycine elevation. Am J Hum Genet (2011) 89:792–97. doi: 10.1016/j.ajhg.2011.11.011
27. Padmalayam I, Hasham S, Saxena U, Pillarisetti S. Lipoic acid synthase (LASY): a novel role in inflammation, mitochondrial function, and insulin resistance. Diabetes (2009) 58:600–8. doi: 10.2337/db08-0473
28. Wu Q, Ba-Alawi W, Deblois G, Cruickshank J, Duan S, Lima-Fernandes E, et al. GLUT1 inhibition blocks growth of RB1-positive triple negative breast cancer. Nat Commun (2020) 11(1):4205. doi: 10.1038/s41467-020-18020-8
29. Raggi C, Taddei ML, Sacco E, Navari1 N, Correnti M, Piombanti1 B, et al. Mitochondrial OXidative metabolism contributes to a cancer stem cell pheno-type in cholangiocarcinoma. J Hepatol (2021) 74(6):1373–85. doi: 10.1016/j.jhep.2020.12.031
30. Fischer GM, Jalali A, Kircher DA, Lee WC, McQuade JL, Haydu LE, et al. Molecular profiling reveals unique immune and metabolic features of mel-anoma bran metastases. Cancer Discovery (2019) 9(5):628–45. doi: 10.1158/2159-8290.CD-18-1489
31. Burr SP, Costa AS, Grice GL, Timms RT, Lobb IT, Freisinger P, et al. Mitochondrial protein lipoylation and the 2-oxoglutarate dehydrogenase complex controls HIF1a stability in aerobic conditions. Cell Metab (2016) 24(5):740–52. doi: 10.1016/j.cmet.2016.09.01555
32. Hajizadeh F, Okoye I, Esmaily M, Chaleshtari M, Masjedi A, Azizi G, et al. Hypoxia inducible factors in the tumor microenvironment as therapeutic targets of cancer stem cells. Life Sci (2019) 237:116952. doi: 10.1016/j.lfs.2019.116952
33. Zhao Y, Xu G, Li H, Chang M, Guan Y, Li Y, et al. Overexpression of endogenous lipoic acid synthase attenuates pulmonary fibrosis induced by crystalline silica in mice. Toxicol Lett (2020) 323:57–66. doi: 10.1016/j.toxlet.2020.01.023
34. Cai Y, He Q, Liu W, Liang Q, Peng B, Li J, et al. Comprehensive analysis of the potential cuproptosis-related biomarker LIAS that regulates prognosis and immunotherapy of pan-cancers. Front Oncol (2022) 12:952129. doi: 10.3389/fonc.2022.952129
35. Schmidt A, Marabita F, Kiani NA, Gross CC, Johansson HJ, Éliás S, et al. Time-resolved transcriptome and proteome landscape of human regulatory T cell (Treg) differentiation reveals novel regulators of FOXP3. BMC Biol (2018) 16(1):47. doi: 10.1186/s12915-018-0518-3
36. Patel MS, Nemeria NS, Furey W, Jordan F. The pyruvate dehydrogenase complexes: Structure-based function andregulation. J Biol Chem (2014) 289(24):16615–23. doi: 10.1074/jbc.R114.563148
37. Goh WQ, Ow GS, Kuznetsov VA, Chong S, Lim YP. DLAT subunit of the pyruvate dehydrogenase complex is upregulated in gastric cancer-implications in cancer therapy. Am J Trans Res (2015) 7(6):1140–51.
38. Shan C, Elf S, Ji Q, Zhou L, Hitosugi T. Lysine acetylation activates 6-phosphogluconate dehydrogenase to promote tumor growth. Mol Cell (2014) 55(4):S52–565. doi: 10.1016/j.molcel.2014.06.020
39. Chen Q, Wang Y, Yang L, Sun L, Wen Y, Huang Y, et al. PM2.5 promotes NSCLC carcinogenesis through translationally and transcriptionally activating DLAT-mediated glycolysis reprograming. J Exp Clin Cancer Res (2022) 41(1):229. doi: 10.1186/s13046-022-02437-8
40. Lacroix M, Rodier G, Kirsh O, Houles T, Delpech H, Seyran B, et al. E4F1 controls a transcriptional program essential for pyruvate dehydrogenase activity. Proc Natl Acad Sci (2016) 113:10998–1003. doi: 10.1073/pnas.1602754113
41. Le Cam L, Linares LK, Paul C, Julien E, Lacroix M, Hatchi E, et al. E4F1 is an atypical ubiquitin ligase that modulates p53 effector functions independently of degradation. Cell (2006) 127(4):775–88. doi: 10.1016/j.cell.2006.09.031
42. Bai Y, Yang J, Cui Y, Yao Y, Wu F, Liu C, et al. Research progress of Sirtuin4 in cancer. Front Oncol (2021) 10:562950. doi: 10.3389/fonc.2020.562950
43. Ward PS, Thompson CB. Metabolic reprogramming: A cancer hallmark even warburg did not anticipate. Cancer Cell (2012) 21(3):297–308. doi: 10.1016/j.ccr.2012.02.014
44. Chen X, Ding X, Wu Q, Qi J, Zhu M, Miao , et al. Monomethyltransferase SET8 facilitates hepatocellular carcinoma growth by enhancing aerobic glycolysis. Cell Death Disease (2019) 10(4):312. doi: 10.1038/s41419-019-1541-1
45. Que EL, Domaille DW, Chang CJ. Metals in neurobiology: Probing their chemistry and biology with molecular imaging. Chem Rev (2008) 108:1517–49. doi: 10.1021/cr078203u
46. Pierson H, Yang H, Lutsenko S. Copper transport and disease: What can we learn from organoids? Annu Rev Nutr (2019) 39:75–94. doi: 10.1146/annurev-nutr-082018-124242
47. Hartwig C, Méndez GM, Bhattacharjee S, Vrailas-Mortimer AD, Zlatic SA, Freeman AAH, et al. Golgi-dependent copper homeostasis sustains synaptic development and mitochondrial content. J Neurosci (2021) 41(2):215⁃233. doi: 10.1523/JNEUROSCI.1284-20.2020
48. Cobine PA, Pierrel F, Winge DR. Copper trafficking to the mitochondrion and assembly of copper metalloenzymes. Biochim Biophys Acta (2006) 1763(7):759⁃772. doi: 10.1016/j.bbamcr.2006.03.002
49. Cobine PA, Moore SA, Leary SC. Getting out what you put in: Copper in mitochondria and its impacts on human disease. Biochim Biophys Acta Mol Cell Res (2021) 1868:118867. doi: 10.1016/j.bbamcr.2020.118867
50. Swaminathan AB, Gohil VM. The role of COA6 in the mitochondrial copper delivery pathway to cytochrome c oxidase. Biomolecules (2022) 12:125. doi: 10.3390/biom12010125
51. Kim YJ, Bond GJ, Tsang T, Posimo JM, Busino L, Brady DC, et al. Copper chaperone ATOX1is required for MAPK signaling and growth in BRAF mutationpositive melanoma. Metallomics (2019) 11(8):1430–40. doi: 10.1039/c9mt00042a
52. Li Y, Liang R, Zhang X, Wang J, Shan C, Liu S, et al. Copper chaperone for superoxide dismutase promotes breast cancer cell proliferation and migration via ROSmediated MAPK/ERK signaling. Front Pharmacol (2019) 10:356. doi: 10.3389/fphar.2019.00356
53. Singh RP, Jeyaraju DV, Voisin V, Hurren R, Xu C, Hawley JR, et al. Disrupting mitochondrial copper distribution inhibits leukemic stem cell selfrenewal. Cell Stem Cell (2020) 26(6):926–937.e10. doi: 10.1016/j.stem.2020.04.010
54. Du W, Amarachintha S, Wilson AF, Pang Q. SCO2 mediates oxidative stress-induced glycolysis to oxidative phosphorylation switch in hemato poietic stem cells. Stem Cells (2016) 34:960–71. doi: 10.1002/stem.2260
55. Lee J, Prohaska JR, Thiele DJ. Essential role for mammalian copper transporter Ctr1 in copper homeostasis and embryonic development. Proc Natl Acad Sci - PNAS. (2001) 98(12):6842–7. doi: 10.1073/pnas.111058698
56. Kim KI, Jang SJ, Park JH, Lee YJ, Lee TS, Woo KS, et al. Detection of increased 64Cu uptake by human copper transporter 1 gene overexpression using PET with 64CuCl2 in human breast cancer xenograft model. J Nucl Med (2014) 55(10):1692–8. doi: 10.2967/jnumed.114.141127
57. Degirmenci U, Wang M, Hu J. Targeting aberrant RAS/RAF/MEK/ERK signaling for cancer therapy. Cells (2020) 9(1):198. doi: 10.3390/cells9010198
58. Carmeliet P, Jain RK. Angiogenesis in cancer and other diseases. Nature (2000) 407(6801):249–57. doi: 10.1038/35025220
59. Baldari S, Di Rocco G, Toietta G. Current biomedical use of copper chelation therapy. Int J Mol Sci (2020) 21(3):1069. doi: 10.3390/ijms21031069
60. Narayanan G, Bharathidevi SR, Vuyyuru H, Muthuvel B, Konerirajapuram Natrajan S. CTR1 silencing inhibits angiogenesis by limiting copper entry into endothelial cells. PloS One (2013) 8(9):e71982. doi: 10.1371/journal.pone.0071982
61. Narayanan IG, Natarajan SK. Peptides derived from histidine and methionine-rich regions of copper transporter 1 exhibit anti-angiogenic property by chelating extracellular Cu. Chem Biol Drug Design. (2017) 91(3):797–804. doi: 10.1111/cbdd.13145
62. Song I, Savaraj N, Siddik Z, Liu P, Wei Y, Wu CJ, et al. Role of human copper transporter Ctr1 in the transport of platinum-based antitumor agents in cisplatin-sensitive and cisplatin-resistant cells[J]. Mol Cancer Ther (2004) 3(12):1543–9. doi: 10.1158/1535-7163.1543.3.12
63. Chen HH, Yan JJ, Chen WC, Kuo MT, Lai YH, Lai WW, et al. Predictive and prognostic value of human copper transporter 1 (hCtr1) in patients with stage III non-small-cell lung cancer receiving first-line platinum-based doublet chemotherapy. Lung Cancer (2011) 75(2):228–34. doi: 10.1016/j.lungcan.2011.06.011
64. Holzer AK, Katano K, Klomp LW, Howell SB. Cisplatin rapidly down-regulates its own influx transporter hCTR1 in cultured human ovarian carcinoma cells. Clin Cancer Res (2004) 10(19):6744–9. doi: 10.1158/1078-0432.CCR-04-0748
65. Chen J, Jiang Y, Shi H, Peng Y, Fan X, Li C, et al. The molecular mechanisms of copper metabolism and its roles in human diseases. Pflügers Archiv - Eur J Physiol (2020) 472(10):1415–29. doi: 10.1007/s00424-020-02412-2
66. Payne SL, Fogelgren B, Hess AR, Seftor EA, Wiley EL, Fong S, et al. Lysyl oxidase regulates breast cancer cell migration and adhesion through a hydrogen peroxide-mediated mechanism. Cancer Res (Chicago,III.). (2005) 65(24):11429–36. doi: 10.1158/0008-5472.CAN-05-1274
67. Baker AM, Bird D, Lang G, Cox TR, Erler JT. Lysyl oxidase enzymatic function increases stiffness to drive colorectal cancer progression through FAK. Oncogene (2012) 32(14):1863–8. doi: 10.1038/onc.2012.202
68. Aubert L, Nandagopal N, Steinhart Z, Lavoie G, Nourreddine S, Berman J, et al. Copper bioavailability is a KRAS-specific vulnerability in colorectal cancer. Nat Commun (2020) 11(1):3701. doi: 10.1038/s41467-020-17549-y
69. Ash D, Sudhahar V, Youn SW, Okur MN, Das A, O'Bryan JP, et al. The p-type ATPase transporter ATP7A promotes angiogenesis by limiting autophagic degradation of VEGFR2. Nat Commun (2021) 12(1):3091. doi: 10.1038/s41467-021-23408-1
70. Yu Z, Cao W, Ren Y, Zhang Q, Liu J. ATPase copper transporter a, negatively regulated by miR-148a-3p, contributes to cisplatin resistance in breast cancer cells. Clin Trans Med (2020) 10(1):57–73. doi: 10.1002/ctm2.19
Keywords: cancer, cuproptosis, cuproptosis-related genes, copper transporters, non-apoptotic regulatory cell death
Citation: Zhao Q and Qi T (2023) The implications and prospect of cuproptosis-related genes and copper transporters in cancer progression. Front. Oncol. 13:1117164. doi: 10.3389/fonc.2023.1117164
Received: 06 December 2022; Accepted: 17 February 2023;
Published: 28 February 2023.
Edited by:
Valentina Oliveri, University of Catania, ItalyReviewed by:
Jianfa Wu, Shanghai University of Medicine and Health Sciences, ChinaCopyright © 2023 Zhao and Qi. This is an open-access article distributed under the terms of the Creative Commons Attribution License (CC BY). The use, distribution or reproduction in other forums is permitted, provided the original author(s) and the copyright owner(s) are credited and that the original publication in this journal is cited, in accordance with accepted academic practice. No use, distribution or reproduction is permitted which does not comply with these terms.
*Correspondence: Tonggang Qi, tg_qi@sdu.edu.cn