- Department of Oncology, Shanghai Ninth People’s Hospital, Shanghai Jiao Tong University School of Medicine, Shanghai, China
As a relatively rare population of cancer cells existing in the tumor microenvironment, cancer stem cells (CSCs) possess properties of immune privilege to evade the attack of immune system, regulated by the microenvironment of CSCs, the so-called CSCs niche. The bidirectional interaction of CSCs with tumor microenvironment (TME) components favors an immunosuppressive shelter for CSCs’ survival and maintenance. Gastrointestinal cancer stem cells (GCSCs) are broadly regarded to be intimately involved in tumor initiation, progression, metastasis and recurrence, with elevated tumor resistance to conventional therapies, which pose a major hindrance to the clinical efficacy for treated patients with gastrointestinal malignancies. Thus, a multitude of efforts have been made to combat and eradicate GCSCs within the tumor mass. Among diverse methods of targeting CSCs in gastrointestinal malignancies, immunotherapy represents a promising strategy. And the better understanding of GCSCs immunomodulation and immunoresistance mechanisms is beneficial to guide and design novel GCSCs-specific immunotherapies with enhanced immune response and clinical efficacy. In this review, we have gathered available and updated information to present an overview of the immunoevasion features harbored by cancer stem cells, and we focus on the description of immune escape strategies utilized by CSCs and microenvironmental regulations underlying CSCs immuno-suppression in the context of gastrointestinal malignancies. Importantly, this review offers deep insights into recent advances of CSC-targeting immunotherapeutic approaches in gastrointestinal cancers.
1 Introduction
Gastrointestinal malignancies mainly including colorectal cancer, gastric cancer, pancreatic cancer, liver cancer and esophageal cancer, are still serious public health problems with worldwide concern. According to GLOBOCAN 2020 report, colorectal cancer (CRC) ranks as the second greatest cause of cancer-related mortality globally (1). In the United States, CRC has been estimated as the second deadliest cancer type among men and women combined and it ranks third for incidence in both men and women (2). Despite different therapeutic tools like surgery, radiotherapy, and chemotherapy, a certain number of patients still face unremarkable and unfavorable clinical outcomes owing to therapeutic resistance, tumor metastasis and recurrence. Alternatively, immunotherapies such as cancer vaccines, immune checkpoint inhibitors and adoptive cell transfer can stimulate anti-tumor immune responses to acquire long-term efficacy. Therefore, immunotherapy has become an optimal treatment modality for the favorable prognosis of patients with gastrointestinal malignancies.
Cancer stem cells also referred to as tumor initiating cells (TICs) or cancer progenitor cells, are a small group of unlimitedly proliferative tumor cells endowed with tumorigenicity along with stem cell-like traits like self-renewal, proliferation, quiescence and differentiation. It was reported that CSCs were first identified from acute myeloid leukemia (AML) by Bonnet and Dick in the late 1990s (3). Subsequently, researchers further found the existence of CSCs in multiple solid tumor types including cancers of the colorectal (4, 5), pancreas (6), liver (7, 8), gastric (9), brain (10, 11), breast (12–15), prostate (16), lung (17), bladder (18) and melanoma (19). Notably, cancer stem cells in gastrointestinal cancers were first detected in 2007 in colorectal cancer (5). Different biomarkers of gastrointestinal cancer stem cell can be used for identification and antitumor therapies (20–22) (Figure 1). GCSCs are located in a specialized environment called “niche”, which can support and maintain the properties of immune privilege harbored by GCSCs. The properties and the suppressive environment enable GCSCs to be resistant to conventional treatment strategies. According to the accumulated evidence, GCSCs possess therapeutic resistance through several mechanisms including the maintenance of senescence, increased DNA repair capacity and redox capacity, drug efflux, and epithelial-mesenchymal transition (EMT) (23, 24). Therefore, targeting GCSCs is a promising method.
GCSCs are capable of evading immune system impairment through adopting a series of smart strategies and interacting with the tumor microenvironment. In the context of gastrointestinal cancers, enough consideration has been given to elucidating the relevant strategies CSCs adopted to evade immune attacks, which contribute to therapy insensitivity and drug resistance. Additionally, this review offers a current understanding of GCSCs and their niche with a special emphasis on bidirectional crosstalk. To precisely target GCSCs, this review highlights various immune-targeted therapies against gastrointestinal cancers for the purpose of exploring more effective GCSCs-specific targeted therapeutic tools.
2 Immune escape mechanisms of GCSCs
Certain methods have been adopted by GCSCs to circumvent the attack of immune system, enhance their own survival and facilitate tumor initiation, about which we will discuss as the followings (Figure 2). GCSCs can not only downregulate relevant molecules expression to suppress T cells response and NK cells-mediated cytotoxicity, but also exhibit increased levels of immune checkpoint molecules expression to support immune evasion.
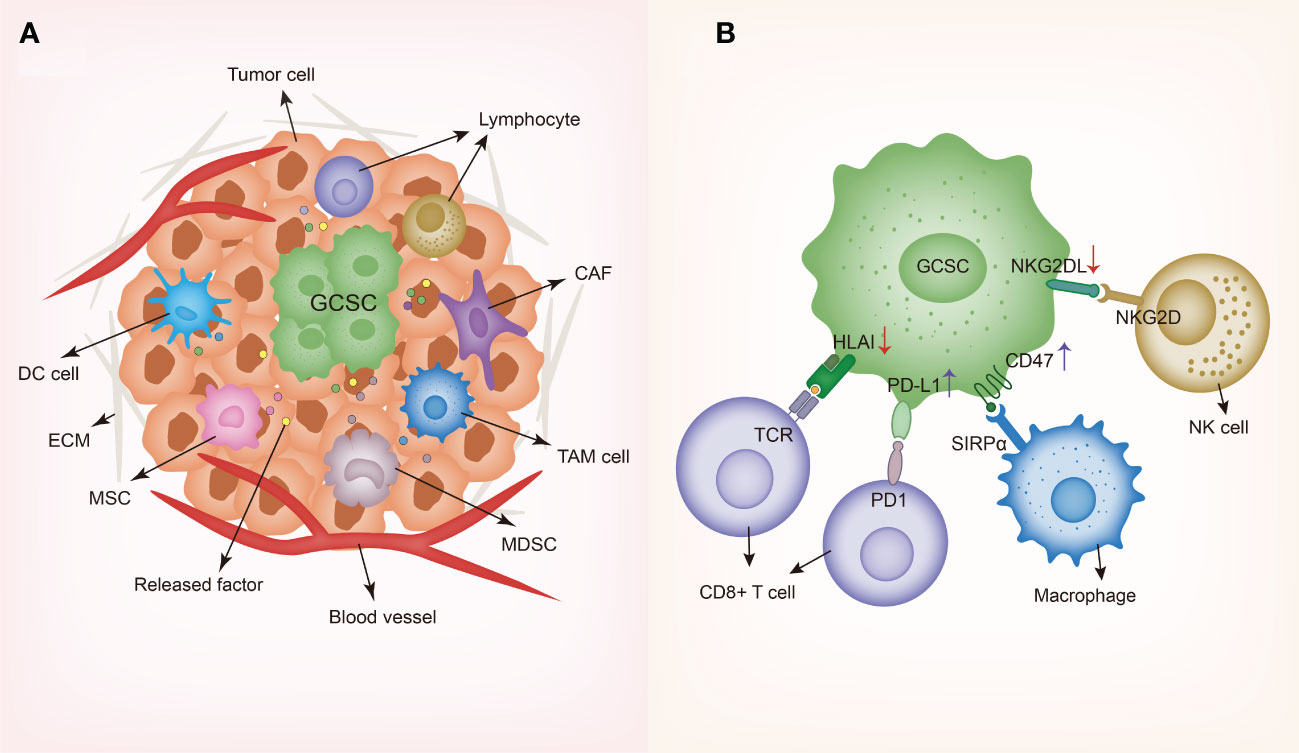
Figure 2 Schematic representing gastrointestinal cancer stem cells and their niche. (A) GCSC niche contains diverse cell types such as dendritic cells (DCs), tumor-associated macrophages (TAMs), cancer-associated fibroblasts (CAFs), myeloid-derived suppressor cells (MDSCs), cancer-associated mesenchymal stem cells (MSCs) and lymphocytes, along with various secreted factors such as cytokines and chemokines. In order to create and maintain an immunosuppressive environment, GCSCs recruit different cells to the niche and modulate the behaviors of these cells through producing a wide variety of soluble factors. (B) Immune evasion mechanisms of GCSC including the downregulation of HLA and NKG2DL and the upregulation of PD-L1 and CD47. GCSC, gastrointestinal cancer stem cell; DC, dendritic cell; TAM, tumor-associated macrophage; CAF, cancer-associated fibroblast; MDSC, myeloid-derived suppressor cell; MSC, mesenchymal stem cell; ECM, extracellular matrix; HLA, human leukocyte antigen-A; SIRPα, signal regulatory protein alpha; TCR, T cell receptor; NKG2D, natural killer group 2D; NKG2DL, natural killer group 2D ligand.
2.1 Reduced expression of MHC-I and natural killer ligands
A growing number of studies highlight that CSCs exhibit reduced expression of the components that participate in antigen processing and presentation, for instance, the major histocompatibility complex class I (MHC- I) molecules (HLA-A, B, C) and the antigen processing machinery (APM) molecules, suggesting CSCs ability to avoid the recognition and activation of CD8+ T lymphocytes. Such a phenomenon was observed in CSCs from glioblastoma (25), melanoma (26), lung cancer (27), head and neck squamous cell carcinoma (28, 29), and colorectal cancer (30, 31), rather than the non-CSCs counterparts. Importantly, CSCs with a decreased level of MHC-I expression are vulnerable to NK cells. For example, CRC CICs characteristic of lower MHC-I expression was found to show higher NK cell susceptibility due to high levels of NK-cell-activating receptor ligands (30).
Nevertheless, CSCs can also evade NK cells-mediated killing by tuning the expression of NK cell activating and inhibitory receptors (32). It was observed that STAT3 could suppress NK cell-mediated immunosurveillance through downregulating natural killer group 2, member D receptor ligands (NKG2DL) in HT29 colorectal cancer cell line, and STAT3 neutralization activated NK cells via the induction of MHC class I chain-related protein A (MICA), which is the recognition receptor of NK cells (33). In addition, one study showed NK cell-mediated cytotoxicity can be attenuated through upregulating carcinoembryonic antigen-related cell adhesion molecule 1 (CEACAM1) of EpCAMhigh liver CSCs (34). Using anti-CEACAM1 antibody can inhibit EpCAMhigh CSCs in hepatocellular carcinoma (HCC).
2.2 Elevated expression of immune checkpoint molecules
It has been found that PD-1 ligand (PD-L1), also known as B7H1 or CD274, is overexpressed on CSCs from CRC (35–37), head and neck squamous cell carcinoma (38), and gastric cancer (39), contributing to immune evasion of CSCs (40). EMT induced PD-L1 expressed on CSCs via EMT/β-catenin/STT3/PD-L1 signaling axis, and targeting EMT/β-catenin/STT3/PD-L1 axis may downregulate PD-L1 (40). In colorectal cancer, PD-L1 can trigger CSC-like characteristics and chemoresistance in CRC cells (41). PD-L1 overexpressed on CD133+ colorectal CSCs and EMT enable CSCs to invade and metastasize (37). Moreover, PD-L1 was found to be overexpressed on CD133+CD44+ colorectal CSCs, and it promoted CSCs expansion and self-renewal via PD-L1- activated HMGA1-dependent signaling pathways (36). In gastric cancer, B7-H1 expressed in Lgr5+ gastric CSCs can enhance CSC proliferation and tumor formation by binding with PD-1 on T cells (39). Besides, B7-H3 was reported to be expressed on ovarian cancer initiating cells (CICs), and applying B7-H3-specific monoclonal antibody 376.96 resulted in the reduction of CICs content in vitro (42).
Based on the existing evidence, the “don’t eat me” signal CD47 has been reported to exhibit elevated levels of CSCs from liver cancer (43, 44), pancreatic cancer (45), esophageal squamous cell carcinoma (ESCC) (46), lung cancer (47) and other cancer types. CD47 can inhibit the phagocytic activity of macrophages to cancer stem cells by interacting with signal regulatory protein α (SIRP-α) on phagocytic cells. In addition, Michele Cioffi et al. (45) demonstrated that anti-CD47 treatment induced pancreatic CSCs apoptosis apart from being phagocytized by macrophages. The upregulation of CD47 assists CSCs to escape destruction from the immune system. In ESCC, researchers found CD47+ CD133+ ESCC cells with CSC-like characteristics can be veritably eliminated after anti-CD47 treatment (46). It has been found that 4-methylumbelliferone (4Mu), the hyaluronan synthesis inhibitor, plays a role in promoting phagocytosis via downregulating CD47 expression on hepatic CSCs, and potentiating cytotoxic-specific T cell response against HCC induced by interleukin-12 (48). Additionally, suppression of CD47 preferentially expressed on liver TICs reduced HCC CSC-like properties including self-renewal and chemoresistance (43).
3 Microenvironmental regulations of GCSCs
As widely regarded, the tumor microenvironment CSCs reside in has been termed as “CSCs niche” and accumulated studies indicate that there exists complicated interplay between CSCs and the tumor microenvironment (TME), playing a pivotal role in tumor invasiveness and progression. Studies have uncovered that different microenvironmental regulation factors collaborate with GCSCs to sustain their immunosuppression properties. Especially, GCSCs interact with different cells in the TME composed of dendritic cells (DCs), tumor-associated macrophages (TAMs), cancer-associated fibroblasts (CAFs), myeloid-derived suppressor cells (MDSCs), cancer-associated mesenchymal stem cells (MSCs), regulatory T cells (Tregs), along with other cell types (Figure 2).
3.1 Dendritic cells
As the most important antigen-presenting cell subtype to T lymphocytes responsible for initiating immune responses, DCs can connect innate immune system with adaptive immune system. However, CSCs alter DC phenotypes and render them immunosuppressive, thus impeding the activation of T cells and anti-tumor immune responses. In colon cancer, a previous study indicated that DCs-secreted CXCL1 enhanced cell migration, EMT and cancer metastatic ability as well as increasing expression of oncogene (PTHLH, TYRP1, FOXO1, TCF4 and ZNF880), CSC-related transcriptional factors (Nanog, Oct4 and Sox2) and miR-105 (49).
3.2 Tumor-associated macrophages
TAMs are divided into M1 pro-inflammatory phenotype and M2 pro-tumorigenic phenotype. In gastrointestinal cancer, CSCs promote TAMs recruitment by secreting CC chemokine family members (CCL2, CCL5), CXC chemokine family members (CXCL5, CXCL12), and soluble proteins like colony-stimulating factor1 (CSF1), MIC-1, LOX and VEGF (50). CSCs can also stimulate TAMs polarization into M2 phenotype by secreting IL-13, IL-34, CSF2, TGF-β, osteoactivin and exosomes (50). In turn, as the predominant immune cell type within CSCs niche, infiltrated TAMs can enhance CSCs properties and facilize CSCs maintenance through the secretion of various cytokines and soluble molecules including CCL8, CCL17, CCL22, IL-6, IL-18, TGF-β, hCAP-18/LL-37, S100A9, MFG-E8 and extracellular vesicles (50). For example, in hepatocellular carcinoma, TGF-β produced by TAMs induced NF-κB, AKT and STAT3 signaling pathways in CSCs, thus enhancing HCC stemness and epithelial to mesenchymal transition (51). Besides, TAMs-secreted IL-6 promoted CD44+ HCC CSCs expansion via STAT3 (52). In colorectal cancer, transforming growth factor-β (TGF-β) can promote stem cell-like properties (53). In pancreatic cancer, CSCs were detected to overexpress TGF-β superfamily members Nodal and Activin, which drive self-renewal and in vivo tumorigenicity (54). TAMs produce leucine leucine-37 (LL-37) to respond to CSCs-secreted TGF-β1, Nodal and Activin A, leading to PDAC progression and metastasis, which can be inhibited by targeting the LL-37 receptors FPR2 and P2X7R (55).
3.3 Cancer-associated fibroblasts
CAFs regulate CSCs properties to support tumor growth through specific factors and pathways in gastrointestinal cancers such as gastric (56), colorectal (57, 58) and liver cancers (59, 60). Reciprocally, CSCs will enhance the proliferation and maintain the immunosuppressive phenotype of CAFs (61). Recently, it has been reported that cancer-associated fibroblasts (CAFs) exert an effect on educating MDSCs and thus promoting stemness of intrahepatic cholangiocarcinoma through the LTB4-BLT2 axis (62). CAFs can enhance the self-renewal and the frequency of PDAC CSCs via integrin-FAK signaling (63). NRG1 secreted by CAFs promotes the self-renewal of gastric CSCs through the activation of NF-κB signaling (64). Furthermore, a recent study uncovered that CAFs can promote cancer stemness through the osteopontin/secreted phosphoprotein 1-CD44 axis in pancreatic cancer (65). CAFs-secreted hepatocyte growth factor (HGF) and IL-6 were reported to enhance the stemness of CD24+ HCC cells through STAT3 signaling (59). CAFs can sustain the stemness of gastric CSCs via acting on TGF-β signaling (56). HGF secreted by CAFs can regulate CSCs stemness of hepatocellular carcinoma and colorectal cancer by activating c-Met/FRA1/HEY1 signaling, Wnt/β-catenin and PI3K signaling, respectively (60, 66).
3.4 Myeloid-derived suppressor cells
MDSCs are categorized into monocytic MDSCs (M-MDSCs) and polymorphonuclear MDSCs (PMN-MDSCs). They are essential cellular components mediating immunosuppression. Substantial evidence has revealed MDSCs are closely linked with cancer stemness maintenance (67, 68). In esophageal squamous cell carcinoma (ESCC), MDSCs can activate NEDD9 to enhance cancer stemness with the involvement of Notch signaling (69). By the activation of STAT3, MDSCs can enlarge ALDH1(Bright) CSCs population derived from pancreatic cancer (70). Additionally, a previous study showed MDSCs can confer breast cancer cells stem-like properties (71).
3.5 Cancer-associated mesenchymal stem cells
MSCs produce diverse cytokines to facilize CSCs restoration in the TME (72, 73). They can support the reacquisition and maintenance of gastric CSCs by the activations of the WNT and TGF-β signaling pathways (72). In addition, MSCs derived from gastric cancer can secrete IL-6 and IL-8 to trigger TAMs polarization into M2 macrophages through the JAK2/STAT3 signaling pathway (74).
3.6 Regulatory T cells
As a subtype of CD4+ T cells, Treg cells are capable of promoting cancer progression by negatively regulating immune response. CSCs recruit Treg cells into the tumor microenvironment through releasing relevant factors such as CCL1, CCL2 and CCL5. In turn, Treg cells contribute to the formation of immunosuppressive tumor environment. In one study of CRC, it has been found that IL-17-expressing Treg cells can induce cancer-initiating cells (75).
It is revealed that CSCs modulate immune cells behaviors by secreting various immunosuppressive cytokines and soluble factors like IL-4, IL-6, IL-8, IL-10, IL-13, and TGF-β (76–78). IL-4 was detected to be overexpressed on CICs isolated from CRC, representing one important actor that affects T lymphocytes-mediated anti-tumor activity (30). IL-4 signaling blockade can be regarded as a useful target of CRC CICs. Similarly, it was demonstrated that disrupting IL-8/CXCR1 signaling involved in pancreatic CSCs stemness would lead to the reduction of CSCs population (76). Furthermore, TGF-β stimulates the differentiation of regulatory T cells (Treg) and promotes fibrosis to make negative effects on effector T-cell proliferation and infiltration (78, 79). In addition to CSCs, TGF-β can also be produced by TAMs, CAFs, lymphocytes and mesenchymal stem cells (MSCs) (80–82). Collectively, this compelling evidence implicates the complex crosstalk between GCSCs and cells in the TME plays a vital part in the regulation of GCSCs.
4 Immunotherapeutic approaches against CSCs in gastrointestinal malignancies
In the last recent decades, cancer immunotherapy has obtained enormous attention. Considering that GCSCs play a critical role in drug resistance and therapeutic failure, immunotherapeutic approaches that target GCSCs can be a promising research field. Diverse anti-GCSC immunotherapeutic approaches have been designed and developed including antibody-mediated immunotherapy, immune cell-based immunotherapy, vaccines and oncolytic virotherapy (Figure 3). Preclinical studies and clinical trials associated with multiple immunotherapies targeting GCSCs have been listed in Tables 1, 2, respectively.
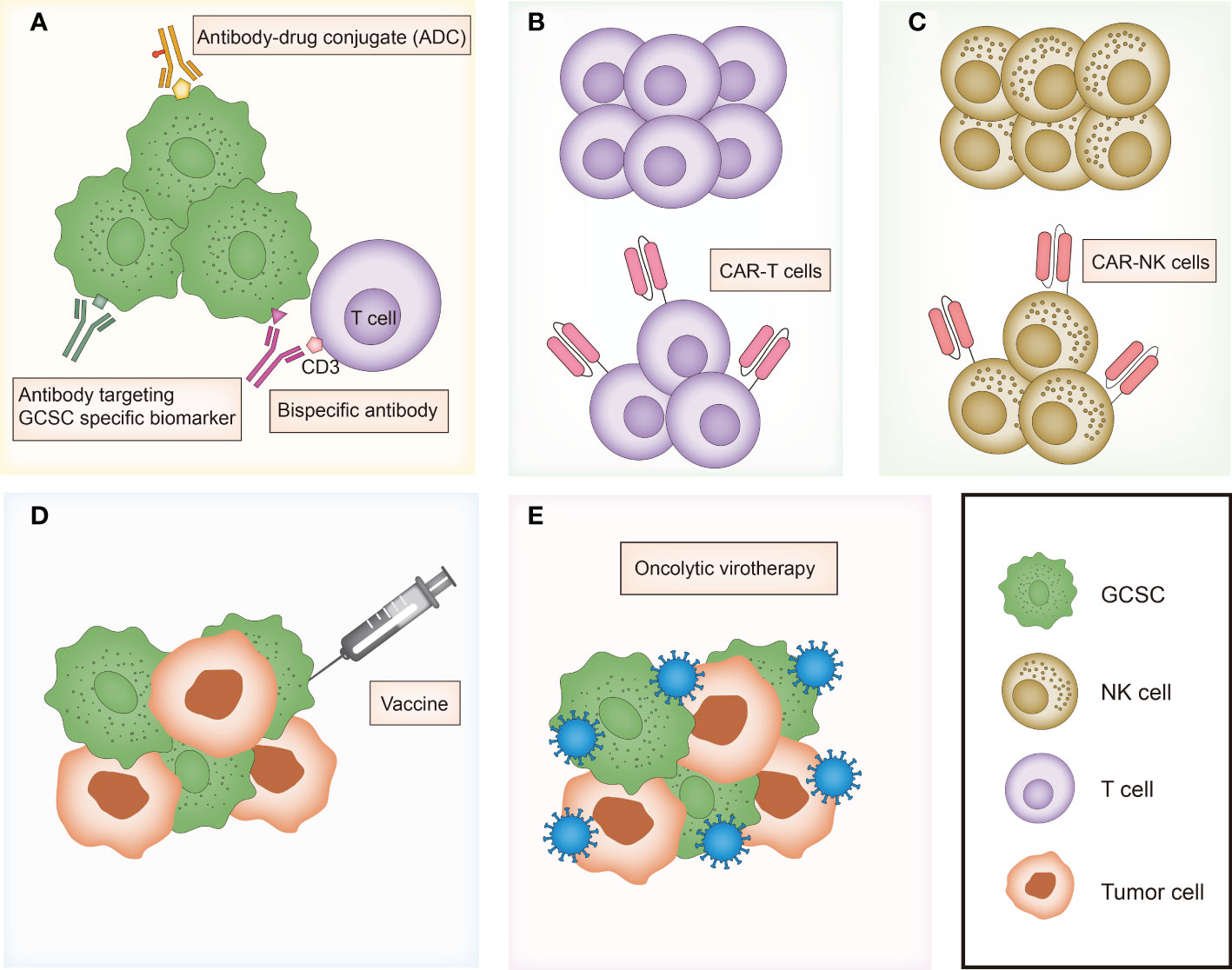
Figure 3 Overview of major immunotherapies targeting gastrointestinal cancer stem cells. (A) Antibody-mediated immunotherapies including antibody targeting GCSC specific biomarker, bispecific antibody and antibody-drug conjugate (ADC). (B) T cell-based immunotherapy. (C) NK cell-based immunotherapy. (D) Vaccines targeting GCSC. (E) Oncolytic virotherapy targeting GCSC. GCSC, gastrointestinal cancer stem cell; CAR, chimeric antigen receptor.
4.1 GCSCs biomarkers
Various biomarkers have been applied for CSCs-targeting therapeutic strategies. Representative biomarkers for CSCs identification in gastrointestinal malignancies are summarized in Figure 1.
CD133 (human prominin-1), a kind of glycoprotein with five transmembrane regions, has been identified as a valid CSC marker in gastrointestinal cancers including gastric, liver, colorectal, pancreatic cancers (106–109) and other cancers like lung (110), brain (10), and prostate cancers (16). In hepatocellular carcinoma, CD133 was reported to facilitate CSC-like features by stabilizing EGFR-AKT signaling (111).
CD24, a mucin-like cell surface glycoprotein, has been regarded as a “don’t eat me” signal (112). By binding to inhibitory receptor sialic-acid-binding Ig-like lectin 10 (Siglec-10), CD24 overexpressed on liver CSCs prevents macrophage phagocytosis. J Ke et al. (113) demonstrated that CD24+ subpopulation of colon cancer cell lines showed CSCs properties like self-renewal capacity and tumorigenicity ability compared to CD24- cancer cells.
The epithelial cell adhesion molecule (EpCAM, CD326) is a multi-functional transmembrane glycoprotein overexpressed in normal epithelial cell and epithelial carcinomas such as cancers of pancreas, colon, stomach, lung, ovarian and so on (114, 115). Functionally, it plays a significant role in modulating intercellular cell-adhesion, cell signaling, proliferation, epithelial-to-mesenchymal transition and stemness of cancer cells (114, 116, 117), known to be a marker for CSCs in liver cancer and colorectal cancer (118, 119). In a recent study, researchers identified a high-risk tumor subtype of hepatocellular carcinoma using intratumoral EpCAM+ cancer stem cell (120).
In addition to well-known identified CSC biomarkers, other novel and potential GCSC biomarkers also have been found. Doublecortin-like kinase1 (DCLK1) is mentioned as a CSC marker of gastrointestinal cancers such as colon cancer (121, 122), pancreatic cancer (123) and liver cancer (124). One recent study stated that DCLK1 can also express on cholangiocarcinoma (CCA) CSC subpopulations of iCCACD133+ and pCCALGR5+ (125). Glypican-3 (GPC3) was reported to mediate the CSC properties like self-renewal, cell cycle progression, and tumor formation via autophagy induction in hepatocellular carcinoma, implicating it as a novel liver CSC marker (126). Moreover, heat shock protein DNAJB8 has been found to be a novel CSC/CIC antigen in CRC (127). As the melanoma antigen gene (MAGE) family number, MAGE-A9 exhibits a higher level of expression in EpCAM+ HCC cells, contributing to the stemness of hepatocellular carcinoma (128).
Notably, CSCs accounting for only a small proportion of cancer tissues have been shown to share several similar biomarker profiles with normal stem cells, indicating that therapeutic agents targeting biomarkers may kill healthy normal tissue stem cells instead of CSCs, which will bring serious problems like drug toxicity and tumor recurrence during anticancer treatment (129–131). For example, LGR5 expression is not restricted to gastric, CRC CSCs, but it can also be expressed in healthy intestine stem cells. Thus, identifying unique markers and specific cell surface antigens of CSCs compared to non-CSCs is of utmost importance to CSC-directed immunotherapies. Multiple cancer testis antigens were preferentially expressed in CSCs rather than non-CSCs (132), suggesting potential antigens for immunotherapy targets.
4.2 Antibody-mediated immunotherapies
With advances in understanding the key role GCSCs plays in gastrointestinal cancers initiation and development, there is a growing concern in targeting GCSCs. In order to diminish and deplete the GCSCs, antibody-mediated treatment modalities have been developed and tested in diverse studies. For example, a first-in-human, phase I, two-arm clinical trial (NCT01358903) involving patients with advanced-stage CD44+ solid tumors showed RG7356 (anti-CD44 monoclonal antibody) can be well tolerated in spite of its modest clinical efficacy (133). Further, nanoparticles can be taken as the drug carrier to enhance the efficacy of antibody immunotherapy. The anti-CD133 antibody-conjugated SN-38-loaded nanoparticles named CD133Ab-NPs-SN-38 has been designed to target CD133+ cells in colorectal cancer, and the results indicated that it could eliminate and suppress cancer growth and recurrence in an HCT116 xenograft model (134).
The combined use of different biomarkers has demonstrated enhanced therapeutic effects. For instance, the bispecific and trifunctional antibody catumaxomab, which can simultaneously bind to T cells, macrophages, DC, and NK-cells with two antigen-binding sites including EpCAM and CD3, has been used to treat CD133+/EpCAM+ epithelial cancer patients suffering from malignant ascites (135–137). A clinical study (NCT00836654) was conducted in EpCAM+ cancer patients suffering from malignant ascites, demonstrating that catumaxomab treatment slowed deterioration in quality of life (QoL) for patients to achieve a prolonged survival period (138). In addition, a phase II study (NCT01504256) indicated the efficacy of catumaxomab in gastric adenocarcinoma patients with peritoneal carcinomatosis, and meanwhile, severe side effects regarding catumaxomab were revealed (139).
Apart from the common antibodies against GCSCs biomarkers, antibody-drug conjugate (ADC)-directed immunotherapy has been developed as a favorable therapeutic approach. Comprised of antibody with exquisite specificity and cytotoxic drug with cell-killing efficiency, ADC is an innovative antitumor weapon to fight against GCSCs, as evidenced by the accumulating studies. L M Smith et al. used anti-CD133 ADC, AC133-vcMMAF to eradicate CD133+ tumor cells including CSCs in hepatocellular and gastric cancers (86). In addition, LGR5-targeted ADCs were effective in the eradication of LGR5+ gastrointestinal cancers and the suppression of tumor progression (87, 140). Trophoblast glycoprotein 5T4 expression exhibits an increased level in colorectal CICs compared with non-CICs, and an anti-5T4 antibody in combination with potent microtubule inhibitor DM4 showed powerful efficacy against colorectal CICs in vitro and in vivo (88). Further, in preclinical models of hepatocellular carcinoma, anti-CD24 ADCs including CD24 antibody-conjugated doxorubicin G7mAb-DOX and the hG7-BM3-VcMMAE conjugates showed antitumor activities in vivo (89, 90).
4.3 Immune cell-based immunotherapies
Immune cell-based immunotherapies such as T cell-based immunotherapy, NK cell-based immunotherapy and cytokine-induced killer (CIK) cell-based immunotherapy have enormous potential for the treatment of gastrointestinal cancers.
4.3.1 T cell-based immunotherapy
CSC-primed T cells targeting CSCs strictly depend on the normal and intact antigen processing and presenting machinery, and the absence of antigens may lead to the failure of targeting CSCs. The olfactory receptor family 7 subfamily C member 1 (OR7C1) was identified as a potential functional marker of colon CICs and its overexpression correlates with poor prognosis of CRC patients (91). A cytotoxic T lymphocytes (CTL) adoptive transfer mouse model applying CTLs specific for OR7C1 peptide was constructed to exert specific cytotoxicity targeting colon CICs. Another study reported that ankyrin repeat and SOCS box protein 4 (ASB4) can elicit CD8+ cytotoxic T cell responses against colorectal CSCs but not non-CSCs because of its specific and unique expression in CSCs (92). In vivo models, adoptive transfer of CTLs specific for ASB4 significantly prevented tumor development.
The chimeric antigen receptor-modified T (CAR-T) cell immunotherapy can specially recognize and kill cancer cells using engineered T lymphocytes with the expression of chimeric antigen receptor (CAR) on their surface. To date, CAR-T immunotherapies based on specific biomarkers have been explored in a variety of preclinical studies and clinical trials. Wei Xia Ang et al. established peritoneal dissemination mouse models of human colorectal cancer in immunodeficient NSG mice, demonstrating that anti-EpCAM CAR-T immunotherapy can suppress and delay the development of peritoneal tumors (93). Another preclinical study of the adoptive transfer of EpCAM CAR-T cells indicated that the growth and formation of colon cancers were delayed in vivo mouse model, without serious adverse effects (141). Clinical trials using EpCAM targeted CAR-T therapy have been conducted in a broad spectrum of gastrointestinal cancers, as depicted in Table 2. A phase I trial (NCT02541370) showed the clinical efficacy and controllable side effects of autologous CAR-T cell directed CD133 termed CART-133 to treat patients with CD133+ advanced metastasis malignancies, and it seemed that CART-133 cells exhibited the “on-target, off-tumor” effect on patients with bile duct stenosis for the reason that it can target CD133 antigen exposed on the bile duct endothelium (142). Kai-chao Feng et al. reported EGFR-specific and CD133-specific CAR-T sequential treatment defined as CAR-T cocktail immunotherapy in a case of advanced cholangiocarcinoma, in which the patient achieved certain clinical response, but the accompanied toxicities should not be ignored (143). In fact, using CAR-T therapy can offer optimistic efficacy for cancer treatment, but we may face much difficulties owing to PD-L1 existence and T cell exhaustion (144, 145).
4.3.2 NK cell-based immunotherapy
NK cells are able to selectively kill GCSCs through modulating the expression of activating and inhibitory receptors. For example, it has been observed that NK cells can kill CD133+ CSCs of colon and gastric cancers (95, 146). In vivo adoptive NK cells transfer model of pancreatic cancer, researchers found NK cells can target CSCs and attack them, leading to the reduction of CSC population and the delayed tumor progression (147). Moreover, Erik Ames and colleagues (148) indicated that the combination of NK cell adoptive immunotherapy with local radiation therapy (RT) had synergistic effects on CSCs elimination and tumor growth suppression in vitro and in vivo. RT can sensitize CSCs derived from solid tumors like pancreatic cancer to NK cells attack by promoting the expression of NK cell ligands on CSCs. This study provided evidence for adoptive cell therapy in conjunction with other cytotoxic standard therapies for complete tumor eradication and long-term clinical benefits in multiple solid tumors.
Compared with CAR-T immunotherapy, CAR-NK cell therapy can reduce on-target toxicity (149, 150). Researchers also constructed EpCAM targeted second-generation CAR and transduced it into NK-92 cells using lentiviral vectors, and they indicated CAR-NK-92 cells can specially kill EpCAM+ colorectal cancer cells and combination with regorafenib can greatly reduce tumor growth in NOD/SCID mice with human colorectal xenograft models (94). A clinical trial (NCT02839954) concerning the immunotherapy of anti-MUC1 CAR-pNK cells has been conducted in patients with MUC1+ solid tumors.
4.3.3 CIK cell-based immunotherapy
Furthermore, cytokine-induced killer (CIK) cells loaded with the anti-CD3/anti-CD133 BsAb (BsAb-CIK) can selectively eliminate CD133high cancer cells of SW1990 pancreatic cancer cell line and Hep3B hepatic cancer cell line (97). Moreover, BsAb-CIK treatment in a mouse model led to the suppression of CD133high tumor growth. A previous study indicated that the combined strategy of DCs loaded with liver cancer stem cells (LCSC) antigens and CIK cells exhibited a significant inhibitory effect on HCC tumor growth and LCSC growth (96).
4.4 Vaccines
Vaccination based on CSC lysates exhibit efficacious antitumor ability in preclinical and clinical studies. Mei Guo et al. suggested that colorectal CSCs lysates-based vaccine served as an effective and safe anti-colorectal cancer vaccine (151). In this study, molecule mucin1 (MUC1), a tumor associated antigen overexpressed in colorectal CSCs in comparison with WT SW620 cells, was reported to be required for colorectal CSCs-based vaccine to exert anti-tumor immunity (151, 152). Furthermore, in a clinical trial carried out among 90 patients with pancreatic adenocarcinoma in 2014, pancreatic CSC vaccine was preliminarily proved to be safe and effective (153).
Another strategy, dendritic cells (DCs) based anti-tumor vaccination is capable of inducing cytotoxic T lymphocytes activities against CSCs. Several DC-based vaccines have been devised to cure patients with gastrointestinal cancers. Different components including RNAs extracted from cancer cells, tumor lysates, and tumor-derived peptides can be used as antigens to induce dendritic cells activities. Vahid Bagheri et al. utilized DCs pulsed with mRNA of gastric CSCs isolated from patients to prime in vitro effective T cell-mediated immune responses (154). Similarly, in 2010, Jian-cong Sun et al. developed a DC-based vaccine loaded with CD133+ HCC cells total RNA, which can generate antigen-specific cytotoxic T lymphocytes response targeting HCC CSCs (155). Compared with RNA-loaded DCs, DCs pulsed with lysates of colon cancer stem cells (CCSCs) isolated from CD44+ CT-26 colon cancer cells can evoke stronger anti-tumor immune responses against CCSCs (156). Apart from that, a recent study applying a D5 melanoma model indicated ALDH 1A1+1A3 dual peptides-DC vaccine can significantly reduce ALDHhigh CSCs and enhance anti-PD-L1 efficacy (157).
Recently, tumor-cell derived autophagosomes (Dribbles), which can serve as a kind of DC-pulsed antigen due to the high immunogenicity, have been used for DC-based vaccination in diverse cancers such as oral squamous cell carcinoma (158), head and neck cancer (159), colorectal carcinoma (98) and hepatocellular carcinoma (160). Of interest, Changhao Fu et al. obtained defective ribosomal products-containing autophagosome-rich blebs from CD44+ colon CSCs and generated DC vaccines pulsed with CCSC-derived Dribbles, demonstrating the efficient cytotoxic T-cells immune responses and tumor growth inhibition in mice model of colorectal carcinoma (98).
4.5 Oncolytic virotherapy
A growing number of evidence has elucidated that oncolytic viruses (OVs) can potentially kill both differentiated cancer cells within tumor bulks and CSCs through multiple ways (161). Several preclinical studies indicated that GCSCs resistant to conventional treatment modalities can be efficiently eradicated by oncolytic virotherapy-mediated killing, which have been indicated in Table 1.
As mentioned above, promising preclinical experiments or clinical trials administering immunotherapy have been conducted and reported to target GCSCs. Indeed, safe and successful clinical use of drugs requires further studies and investigations, and it is equally important to identify specific GCSCs biomarkers. Moreover, eliminating GCSCs can affect tumor growth, but to achieve complete elimination of tumor mass, it is a feasible strategy that combines anti-GCSCs immunotherapy with other anti-tumor therapies such as chemotherapy and radiotherapy.
5 Conclusion
Taken together, GCSCs can evade immune attack through the downregulation of MHC-I and natural killer ligands and the upregulation of immune checkpoint molecules. And the immunosuppressive niche provides a supportive room for them to survive and maintain properties, contributing to the development of malignant cancers. Current efforts aimed at developing GCSCs-targeted immunotherapies in gastrointestinal cancers have shown preclinical and clinical success, but it is always a thought-provoking question to selectively target GCSCs efficiently and accurately. In addition, it is a promising and effective method to combine GCSCs-directed immunotherapy with conventional therapies like chemotherapy and radiotherapy. Despite these advances, a tremendous amount of efforts need to be put into the development of efficacious immunotherapies targeting GCSCs.
Author contributions
JA wrote the manuscript and designed the figures, FL and XH designed the manuscript. All authors contributed to the article and approved the submitted version.
Funding
This work was supported by the Clinical Research Program of 9th People’s Hospital, Shanghai Jiao Tong University School of Medicine (JYLJ202019).
Conflict of interest
The authors declare that the research was conducted in the absence of any commercial or financial relationships that could be construed as a potential conflict of interest.
The reviewer YZ declared a shared affiliation with the authors to the handling editor at the time of review.
Publisher’s note
All claims expressed in this article are solely those of the authors and do not necessarily represent those of their affiliated organizations, or those of the publisher, the editors and the reviewers. Any product that may be evaluated in this article, or claim that may be made by its manufacturer, is not guaranteed or endorsed by the publisher.
References
1. Sung H, Ferlay J, Siegel RL, Laversanne M, Soerjomataram I, Jemal A, et al. Global cancer statistics 2020: Globocan estimates of incidence and mortality worldwide for 36 cancers in 185 countries. CA Cancer J Clin (2021) 71(3):209–49. doi: 10.3322/caac.21660
2. Siegel RL, Miller KD, Wagle NS, Jemal A. Cancer statistics, 2023. CA Cancer J Clin (2023) 73(1):17–48. doi: 10.3322/caac.21763
3. Bonnet D, Dick JE. Human acute myeloid leukemia is organized as a hierarchy that originates from a primitive hematopoietic cell. Nat Med (1997) 3(7):730–7. doi: 10.1038/nm0797-730
4. O'Brien CA, Pollett A, Gallinger S, Dick JE. A human colon cancer cell capable of initiating tumour growth in immunodeficient mice. Nature (2007) 445(7123):106–10. doi: 10.1038/nature05372
5. Ricci-Vitiani L, Lombardi DG, Pilozzi E, Biffoni M, Todaro M, Peschle C, et al. Identification and expansion of human colon-Cancer-Initiating cells. Nature (2007) 445(7123):111–5. doi: 10.1038/nature05384
6. Li C, Heidt DG, Dalerba P, Burant CF, Zhang L, Adsay V, et al. Identification of pancreatic cancer stem cells. Cancer Res (2007) 67(3):1030–7. doi: 10.1158/0008-5472.CAN-06-2030
7. Yang ZF, Ho DW, Ng MN, Lau CK, Yu WC, Ngai P, et al. Significance of Cd90+ cancer stem cells in human liver cancer. Cancer Cell (2008) 13(2):153–66. doi: 10.1016/j.ccr.2008.01.013
8. Shan J, Shen J, Liu L, Xia F, Xu C, Duan G, et al. Nanog regulates self-renewal of cancer stem cells through the insulin-like growth factor pathway in human hepatocellular carcinoma. Hepatology (2012) 56(3):1004–14. doi: 10.1002/hep.25745
9. Quante M, Wang TC. Stem cells in gastroenterology and hepatology. Nat Rev Gastroenterol Hepatol (2009) 6(12):724–37. doi: 10.1038/nrgastro.2009.195
10. Singh SK, Clarke ID, Terasaki M, Bonn VE, Hawkins C, Squire J, et al. Identification of a cancer stem cell in human brain tumors. Cancer Res (2003) 63(18):5821–8.
11. Singh SK, Hawkins C, Clarke ID, Squire JA, Bayani J, Hide T, et al. Identification of human brain tumour initiating cells. Nature (2004) 432(7015):396–401. doi: 10.1038/nature03128
12. Al-Hajj M, Wicha MS, Benito-Hernandez A, Morrison SJ, Clarke MF. Prospective identification of tumorigenic breast cancer cells. Proc Natl Acad Sci U.S.A. (2003) 100(7):3983–8. doi: 10.1073/pnas.0530291100
13. Ponti D, Costa A, Zaffaroni N, Pratesi G, Petrangolini G, Coradini D, et al. Isolation and in vitro propagation of tumorigenic breast cancer cells with Stem/Progenitor cell properties. Cancer Res (2005) 65(13):5506–11. doi: 10.1158/0008-5472.CAN-05-0626
14. Polytarchou C, Iliopoulos D, Struhl K. An integrated transcriptional regulatory circuit that reinforces the breast cancer stem cell state. Proc Natl Acad Sci U.S.A. (2012) 109(36):14470–5. doi: 10.1073/pnas.1212811109
15. Pece S, Tosoni D, Confalonieri S, Mazzarol G, Vecchi M, Ronzoni S, et al. Biological and molecular heterogeneity of breast cancers correlates with their cancer stem cell content. Cell (2010) 140(1):62–73. doi: 10.1016/j.cell.2009.12.007
16. Collins AT, Berry PA, Hyde C, Stower MJ, Maitland NJ. Prospective identification of tumorigenic prostate cancer stem cells. Cancer Res (2005) 65(23):10946–51. doi: 10.1158/0008-5472.CAN-05-2018
17. Kim CF, Jackson EL, Woolfenden AE, Lawrence S, Babar I, Vogel S, et al. Identification of bronchioalveolar stem cells in normal lung and lung cancer. Cell (2005) 121(6):823–35. doi: 10.1016/j.cell.2005.03.032
18. Goodwin Jinesh G, Willis DL, Kamat AM. Bladder cancer stem cells: Biological and therapeutic perspectives. Curr Stem Cell Res Ther (2014) 9(2):89–101. doi: 10.2174/1574888x08666131113123051
19. Schatton T, Murphy GF, Frank NY, Yamaura K, Waaga-Gasser AM, Gasser M, et al. Identification of cells initiating human melanomas. Nature (2008) 451(7176):345–9. doi: 10.1038/nature06489
20. Yang L, Shi P, Zhao G, Xu J, Peng W, Zhang J, et al. Targeting cancer stem cell pathways for cancer therapy. Signal Transduct Target Ther (2020) 5(1):8. doi: 10.1038/s41392-020-0110-5
21. Bisht S, Nigam M, Kunjwal SS, Sergey P, Mishra AP, Sharifi-Rad J. Cancer stem cells: From an insight into the basics to recent advances and therapeutic targeting. Stem Cells Int (2022) 2022:9653244. doi: 10.1155/2022/9653244
22. Li MM, Yuan J, Guan XY, Ma NF, Liu M. Molecular subclassification of gastrointestinal cancers based on cancer stem cell traits. Exp Hematol Oncol (2021) 10(1):53. doi: 10.1186/s40164-021-00246-x
23. Chivu-Economescu M, Necula LG, Matei L, Dragu DL, Neagu AI, Alexiu I, et al. Gastrointestinal cancer stem cells as targets for innovative immunotherapy. World J Gastroenterol (2020) 26(14):1580–93. doi: 10.3748/wjg.v26.i14.1580
24. Stem J, Flickinger JC Jr., Merlino D, Caparosa EM, Snook AE, Waldman SA. Therapeutic targeting of gastrointestinal cancer stem cells. Regener Med (2019) 14(4):331–43. doi: 10.2217/rme-2018-0146
25. Di Tomaso T, Mazzoleni S, Wang E, Sovena G, Clavenna D, Franzin A, et al. Immunobiological characterization of cancer stem cells isolated from glioblastoma patients. Clin Cancer Res (2010) 16(3):800–13. doi: 10.1158/1078-0432.CCR-09-2730
26. Schatton T, Schutte U, Frank NY, Zhan Q, Hoerning A, Robles SC, et al. Modulation of T-cell activation by malignant melanoma initiating cells. Cancer Res (2010) 70(2):697–708. doi: 10.1158/0008-5472.CAN-09-1592
27. Morrison BJ, Steel JC, Morris JC. Reduction of mhc-I expression limits T-Lymphocyte-Mediated killing of cancer-initiating cells. BMC Cancer (2018) 18(1):469. doi: 10.1186/s12885-018-4389-3
28. Grau JJ, Mesia R, de la Iglesia-Vicente M, Williams ES, Taberna M, Caballero M, et al. Enrichment of cells with cancer stem cell-like markers in relapses of chemoresistant patients with locally advanced head and neck squamous cell carcinoma. Oncology (2016) 90(5):267–72. doi: 10.1159/000445118
29. Chikamatsu K, Takahashi G, Sakakura K, Ferrone S, Masuyama K. Immunoregulatory properties of Cd44+ cancer stem-like cells in squamous cell carcinoma of the head and neck. Head Neck (2011) 33(2):208–15. doi: 10.1002/hed.21420
30. Volonte A, Di Tomaso T, Spinelli M, Todaro M, Sanvito F, Albarello L, et al. Cancer-initiating cells from colorectal cancer patients escape from T cell-mediated immunosurveillance in vitro through membrane-bound il-4. J Immunol (2014) 192(1):523–32. doi: 10.4049/jimmunol.1301342
31. Tallerico R, Todaro M, Di Franco S, Maccalli C, Garofalo C, Sottile R, et al. Human nk cells selective targeting of colon cancer-initiating cells: A role for natural cytotoxicity receptors and mhc class I molecules. J Immunol (2013) 190(5):2381–90. doi: 10.4049/jimmunol.1201542
32. Lei MML, Lee TKW. Cancer stem cells: Emerging key players in immune evasion of cancers. Front Cell Dev Biol (2021) 9:692940. doi: 10.3389/fcell.2021.692940
33. Bedel R, Thiery-Vuillemin A, Grandclement C, Balland J, Remy-Martin JP, Kantelip B, et al. Novel role for Stat3 in transcriptional regulation of nk immune cell targeting receptor mica on cancer cells. Cancer Res (2011) 71(5):1615–26. doi: 10.1158/0008-5472.CAN-09-4540
34. Park DJ, Sung PS, Kim JH, Lee GW, Jang JW, Jung ES, et al. Epcam-high liver cancer stem cells resist natural killer cell-mediated cytotoxicity by upregulating Ceacam1. J Immunother Cancer (2020) 8(1):e000301. doi: 10.1136/jitc-2019-000301
35. Wu Y, Chen M, Wu P, Chen C, Xu ZP, Gu W. Increased pd-L1 expression in breast and colon cancer stem cells. Clin Exp Pharmacol Physiol (2017) 44(5):602–4. doi: 10.1111/1440-1681.12732
36. Wei F, Zhang T, Deng SC, Wei JC, Yang P, Wang Q, et al. Pd-L1 promotes colorectal cancer stem cell expansion by activating Hmga1-dependent signaling pathways. Cancer Lett (2019) 450:1–13. doi: 10.1016/j.canlet.2019.02.022
37. Zhi Y, Mou Z, Chen J, He Y, Dong H, Fu X, et al. B7h1 expression and epithelial-to-Mesenchymal transition phenotypes on colorectal cancer stem-like cells. PLos One (2015) 10(8):e0135528. doi: 10.1371/journal.pone.0135528
38. Lee Y, Shin JH, Longmire M, Wang H, Kohrt HE, Chang HY, et al. Cd44+ cells in head and neck squamous cell carcinoma suppress T-Cell-Mediated immunity by selective constitutive and inducible expression of pd-L1. Clin Cancer Res (2016) 22(14):3571–81. doi: 10.1158/1078-0432.CCR-15-2665
39. Yang Y, Wu KE, Zhao E, Li W, Shi L, Xie G, et al. B7-H1 enhances proliferation ability of gastric cancer stem-like cells as a receptor. Oncol Lett (2015) 9(4):1833–8. doi: 10.3892/ol.2015.2949
40. Hsu JM, Xia W, Hsu YH, Chan LC, Yu WH, Cha JH, et al. Stt3-dependent pd-L1 accumulation on cancer stem cells promotes immune evasion. Nat Commun (2018) 9(1):1908. doi: 10.1038/s41467-018-04313-6
41. Min HY, Cho J, Sim JY, Boo HJ, Lee JS, Lee SB, et al. S100a14: A novel negative regulator of cancer stemness and immune evasion by inhibiting Stat3-mediated programmed death-ligand 1 expression in colorectal cancer. Clin Transl Med (2022) 12(7):e986. doi: 10.1002/ctm2.986
42. Fauci JM, Sabbatino F, Wang Y, Londono-Joshi AI, Straughn JM Jr., Landen CN, et al. Monoclonal antibody-based immunotherapy of ovarian cancer: Targeting ovarian cancer cells with the B7-H3-Specific mab 376.96. Gynecol Oncol (2014) 132(1):203–10. doi: 10.1016/j.ygyno.2013.10.038
43. Lee TK, Cheung VC, Lu P, Lau EY, Ma S, Tang KH, et al. Blockade of Cd47-mediated cathepsin S/Protease-activated receptor 2 signaling provides a therapeutic target for hepatocellular carcinoma. Hepatology (2014) 60(1):179–91. doi: 10.1002/hep.27070
44. Castelli G, Pelosi E, Testa U. Liver cancer: Molecular characterization, clonal evolution and cancer stem cells. Cancers (Basel) (2017) 9(9):127. doi: 10.3390/cancers9090127
45. Cioffi M, Trabulo S, Hidalgo M, Costello E, Greenhalf W, Erkan M, et al. Inhibition of Cd47 effectively targets pancreatic cancer stem cells Via dual mechanisms. Clin Cancer Res (2015) 21(10):2325–37. doi: 10.1158/1078-0432.CCR-14-1399
46. Wang JH, Huang ST, Zhang L, Liu ZG, Liang RX, Jiang SW, et al. Combined prognostic value of the cancer stem cell markers Cd47 and Cd133 in esophageal squamous cell carcinoma. Cancer Med (2019) 8(3):1315–25. doi: 10.1002/cam4.1894
47. Liu L, Zhang L, Yang L, Li H, Li R, Yu J, et al. Anti-Cd47 antibody as a targeted therapeutic agent for human lung cancer and cancer stem cells. Front Immunol (2017) 8:404. doi: 10.3389/fimmu.2017.00404
48. Rodriguez MM, Fiore E, Bayo J, Atorrasagasti C, Garcia M, Onorato A, et al. 4mu decreases Cd47 expression on hepatic cancer stem cells and primes a potent antitumor T cell response induced by interleukin-12. Mol Ther (2018) 26(12):2738–50. doi: 10.1016/j.ymthe.2018.09.012
49. Hsu YL, Chen YJ, Chang WA, Jian SF, Fan HL, Wang JY, et al. Interaction between tumor-associated dendritic cells and colon cancer cells contributes to tumor progression Via Cxcl1. Int J Mol Sci (2018) 19(8):2427. doi: 10.3390/ijms19082427
50. Luo S, Yang G, Ye P, Cao N, Chi X, Yang WH, et al. Macrophages are a double-edged sword: Molecular crosstalk between tumor-associated macrophages and cancer stem cells. Biomolecules (2022) 12(6):850. doi: 10.3390/biom12060850
51. Fan QM, Jing YY, Yu GF, Kou XR, Ye F, Gao L, et al. Tumor-associated macrophages promote cancer stem cell-like properties Via transforming growth factor-Beta1-Induced epithelial-mesenchymal transition in hepatocellular carcinoma. Cancer Lett (2014) 352(2):160–8. doi: 10.1016/j.canlet.2014.05.008
52. Wan S, Zhao E, Kryczek I, Vatan L, Sadovskaya A, Ludema G, et al. Tumor-associated macrophages produce interleukin 6 and signal Via Stat3 to promote expansion of human hepatocellular carcinoma stem cells. Gastroenterology (2014) 147(6):1393–404. doi: 10.1053/j.gastro.2014.08.039
53. Nakano M, Kikushige Y, Miyawaki K, Kunisaki Y, Mizuno S, Takenaka K, et al. Dedifferentiation process driven by tgf-beta signaling enhances stem cell properties in human colorectal cancer. Oncogene (2019) 38(6):780–93. doi: 10.1038/s41388-018-0480-0
54. Lonardo E, Hermann PC, Mueller MT, Huber S, Balic A, Miranda-Lorenzo I, et al. Nodal/Activin signaling drives self-renewal and tumorigenicity of pancreatic cancer stem cells and provides a target for combined drug therapy. Cell Stem Cell (2011) 9(5):433–46. doi: 10.1016/j.stem.2011.10.001
55. Sainz B Jr., Alcala S, Garcia E, Sanchez-Ripoll Y, Azevedo MM, Cioffi M, et al. Microenvironmental hcap-18/Ll-37 promotes pancreatic ductal adenocarcinoma by activating its cancer stem cell compartment. Gut (2015) 64(12):1921–35. doi: 10.1136/gutjnl-2014-308935
56. Hasegawa T, Yashiro M, Nishii T, Matsuoka J, Fuyuhiro Y, Morisaki T, et al. Cancer-associated fibroblasts might sustain the stemness of scirrhous gastric cancer cells Via transforming growth factor-beta signaling. Int J Cancer (2014) 134(8):1785–95. doi: 10.1002/ijc.28520
57. Kinugasa Y, Matsui T, Takakura N. Cd44 expressed on cancer-associated fibroblasts is a functional molecule supporting the stemness and drug resistance of malignant cancer cells in the tumor microenvironment. Stem Cells (2014) 32(1):145–56. doi: 10.1002/stem.1556
58. Ren J, Ding L, Zhang D, Shi G, Xu Q, Shen S, et al. Carcinoma-associated fibroblasts promote the stemness and chemoresistance of colorectal cancer by transferring exosomal lncrna H19. Theranostics (2018) 8(14):3932–48. doi: 10.7150/thno.25541
59. Li Y, Wang R, Xiong S, Wang X, Zhao Z, Bai S, et al. Cancer-associated fibroblasts promote the stemness of Cd24(+) liver cells Via paracrine signaling. J Mol Med (Berl) (2019) 97(2):243–55. doi: 10.1007/s00109-018-1731-9
60. Lau EY, Lo J, Cheng BY, Ma MK, Lee JM, Ng JK, et al. Cancer-associated fibroblasts regulate tumor-initiating cell plasticity in hepatocellular carcinoma through c-Met/Fra1/Hey1 signaling. Cell Rep (2016) 15(6):1175–89. doi: 10.1016/j.celrep.2016.04.019
61. Huang TX, Guan XY, Fu L. Therapeutic targeting of the crosstalk between cancer-associated fibroblasts and cancer stem cells. Am J Cancer Res (2019) 9(9):1889–904.
62. Lin Y, Cai Q, Chen Y, Shi T, Liu W, Mao L, et al. Cafs shape myeloid-derived suppressor cells to promote stemness of intrahepatic cholangiocarcinoma through 5-lipoxygenase. Hepatology (2022) 75(1):28–42. doi: 10.1002/hep.32099
63. Begum A, McMillan RH, Chang YT, Penchev VR, Rajeshkumar NV, Maitra A, et al. Direct interactions with cancer-associated fibroblasts lead to enhanced pancreatic cancer stem cell function. Pancreas (2019) 48(3):329–34. doi: 10.1097/MPA.0000000000001249
64. Han ME, Kim HJ, Shin DH, Hwang SH, Kang CD, Oh SO. Overexpression of Nrg1 promotes progression of gastric cancer by regulating the self-renewal of cancer stem cells. J Gastroenterol (2015) 50(6):645–56. doi: 10.1007/s00535-014-1008-1
65. Nallasamy P, Nimmakayala RK, Karmakar S, Leon F, Seshacharyulu P, Lakshmanan I, et al. Pancreatic tumor microenvironment factor promotes cancer stemness Via Spp1-Cd44 axis. Gastroenterology (2021) 161(6):1998–2013.e1997. doi: 10.1053/j.gastro.2021.08.023
66. Todaro M, Gaggianesi M, Catalano V, Benfante A, Iovino F, Biffoni M, et al. Cd44v6 is a marker of constitutive and reprogrammed cancer stem cells driving colon cancer metastasis. Cell Stem Cell (2014) 14(3):342–56. doi: 10.1016/j.stem.2014.01.009
67. Yang T, Liang N, Li J, Hu P, Huang Q, Zhao Z, et al. Mdscs might be "Achilles heel" for eradicating cscs. Cytokine Growth Factor Rev (2022) 65:39–50. doi: 10.1016/j.cytogfr.2022.04.006
68. Cui TX, Kryczek I, Zhao L, Zhao E, Kuick R, Roh MH, et al. Myeloid-derived suppressor cells enhance stemness of cancer cells by inducing Microrna101 and suppressing the corepressor Ctbp2. Immunity (2013) 39(3):611–21. doi: 10.1016/j.immuni.2013.08.025
69. Yue D, Liu S, Zhang T, Wang Y, Qin G, Chen X, et al. Nedd9 promotes cancer stemness by recruiting myeloid-derived suppressor cells Via Cxcl8 in esophageal squamous cell carcinoma. Cancer Biol Med (2021) 18(3):705–20. doi: 10.20892/j.issn.2095-3941.2020.0290
70. Panni RZ, Sanford DE, Belt BA, Mitchem JB, Worley LA, Goetz BD, et al. Tumor-induced Stat3 activation in monocytic myeloid-derived suppressor cells enhances stemness and mesenchymal properties in human pancreatic cancer. Cancer Immunol Immunother (2014) 63(5):513–28. doi: 10.1007/s00262-014-1527-x
71. Peng D, Tanikawa T, Li W, Zhao L, Vatan L, Szeliga W, et al. Myeloid-derived suppressor cells endow stem-like qualities to breast cancer cells through Il6/Stat3 and No/Notch cross-talk signaling. Cancer Res (2016) 76(11):3156–65. doi: 10.1158/0008-5472.CAN-15-2528
72. Nishimura K, Semba S, Aoyagi K, Sasaki H, Yokozaki H. Mesenchymal stem cells provide an advantageous tumor microenvironment for the restoration of cancer stem cells. Pathobiology (2012) 79(6):290–306. doi: 10.1159/000337296
73. Plaks V, Kong N, Werb Z. The cancer stem cell niche: How essential is the niche in regulating stemness of tumor cells? Cell Stem Cell (2015) 16(3):225–38. doi: 10.1016/j.stem.2015.02.015
74. Li W, Zhang X, Wu F, Zhou Y, Bao Z, Li H, et al. Gastric cancer-derived mesenchymal stromal cells trigger M2 macrophage polarization that promotes metastasis and emt in gastric cancer. Cell Death Dis (2019) 10(12):918. doi: 10.1038/s41419-019-2131-y
75. Yang S, Wang B, Guan C, Wu B, Cai C, Wang M, et al. Foxp3+Il-17+ T cells promote development of cancer-initiating cells in colorectal cancer. J Leukoc Biol (2011) 89(1):85–91. doi: 10.1189/jlb.0910506
76. Chen L, Fan J, Chen H, Meng Z, Chen Z, Wang P, et al. The il-8/Cxcr1 axis is associated with cancer stem cell-like properties and correlates with clinical prognosis in human pancreatic cancer cases. Sci Rep (2014) 4:5911. doi: 10.1038/srep05911
77. Todaro M, Alea MP, Di Stefano AB, Cammareri P, Vermeulen L, Iovino F, et al. Colon cancer stem cells dictate tumor growth and resist cell death by production of interleukin-4. Cell Stem Cell (2007) 1(4):389–402. doi: 10.1016/j.stem.2007.08.001
78. Quaglino E, Cavallo F, Conti L. Cancer stem cell antigens as targets for new combined anti-cancer therapies. Int J Biochem Cell Biol (2020) 129:105861. doi: 10.1016/j.biocel.2020.105861
79. Maccalli C, Volonte A, Cimminiello C, Parmiani G. Immunology of cancer stem cells in solid tumours. a review. Eur J Cancer (2014) 50(3):649–55. doi: 10.1016/j.ejca.2013.11.014
80. Mrad M, Imbert C, Garcia V, Rambow F, Therville N, Carpentier S, et al. Downregulation of sphingosine kinase-1 induces protective tumor immunity by promoting M1 macrophage response in melanoma. Oncotarget (2016) 7(44):71873–86. doi: 10.18632/oncotarget.12380
81. Zhuang J, Lu Q, Shen B, Huang X, Shen L, Zheng X, et al. Tgfbeta1 secreted by cancer-associated fibroblasts induces epithelial-mesenchymal transition of bladder cancer cells through lncrna-Zeb2nat. Sci Rep (2015) 5:11924. doi: 10.1038/srep11924
82. Heissig B, Dhahri D, Eiamboonsert S, Salama Y, Shimazu H, Munakata S, et al. Role of mesenchymal stem cell-derived fibrinolytic factor in tissue regeneration and cancer progression. Cell Mol Life Sci (2015) 72(24):4759–70. doi: 10.1007/s00018-015-2035-7
83. Molejon MI, Tellechea JI, Moutardier V, Gasmi M, Ouaissi M, Turrini O, et al. Targeting Cd44 as a novel therapeutic approach for treating pancreatic cancer recurrence. Oncoscience (2015) 2(6):572–5. doi: 10.18632/oncoscience.172
84. Kato Y, Ohishi T, Yamada S, Itai S, Furusawa Y, Sano M, et al. Anti-Cd133 monoclonal antibody cmab-43 exerts antitumor activity in a mouse xenograft model of colon cancer. Monoclon Antib Immunodiagn Immunother (2019) 38(2):75–8. doi: 10.1089/mab.2019.0002
85. Zhao L, Yang Y, Zhou P, Ma H, Zhao X, He X, et al. Targeting Cd133high colorectal cancer cells in vitro and in vivo with an asymmetric bispecific antibody. J Immunother (2015) 38(6):217–28. doi: 10.1097/CJI.0000000000000086
86. Smith LM, Nesterova A, Ryan MC, Duniho S, Jonas M, Anderson M, et al. Cd133/Prominin-1 is a potential therapeutic target for antibody-drug conjugates in hepatocellular and gastric cancers. Br J Cancer (2008) 99(1):100–9. doi: 10.1038/sj.bjc.6604437
87. Junttila MR, Mao W, Wang X, Wang BE, Pham T, Flygare J, et al. Targeting Lgr5+ cells with an antibody-drug conjugate for the treatment of colon cancer. Sci Transl Med (2015) 7(314):314ra186. doi: 10.1126/scitranslmed.aac7433
88. Wang R, Lai Q, Tang L, Tao Y, Yao Y, Liu Y, et al. A novel 5t4-targeting antibody-drug conjugate H6-Dm4 exhibits potent therapeutic efficacy in gastrointestinal tumor xenograft models. Am J Cancer Res (2018) 8(4):610–23.
89. Ma Z, He H, Sun F, Xu Y, Huang X, Ma Y, et al. Selective targeted delivery of doxorubicin Via conjugating to anti-Cd24 antibody results in enhanced antitumor potency for hepatocellular carcinoma both in vitro and in vivo. J Cancer Res Clin Oncol (2017) 143(10):1929–40. doi: 10.1007/s00432-017-2436-0
90. Sun F, Wang T, Jiang J, Wang Y, Ma Z, Li Z, et al. Engineering a high-affinity humanized anti-Cd24 antibody to target hepatocellular carcinoma by a novel cdr grafting design. Oncotarget (2017) 8(31):51238–52. doi: 10.18632/oncotarget.17228
91. Morita R, Hirohashi Y, Torigoe T, Ito-Inoda S, Takahashi A, Mariya T, et al. Olfactory receptor family 7 subfamily c member 1 is a novel marker of colon cancer-initiating cells and is a potent target of immunotherapy. Clin Cancer Res (2016) 22(13):3298–309. doi: 10.1158/1078-0432.CCR-15-1709
92. Miyamoto S, Kochin V, Kanaseki T, Hongo A, Tokita S, Kikuchi Y, et al. The antigen Asb4 on cancer stem cells serves as a target for ctl immunotherapy of colorectal cancer. Cancer Immunol Res (2018) 6(3):358–69. doi: 10.1158/2326-6066.CIR-17-0518
93. Ang WX, Li Z, Chi Z, Du SH, Chen C, Tay JC, et al. Intraperitoneal immunotherapy with T cells stably and transiently expressing anti-epcam car in xenograft models of peritoneal carcinomatosis. Oncotarget (2017) 8(8):13545–59. doi: 10.18632/oncotarget.14592
94. Zhang Q, Zhang H, Ding J, Liu H, Li H, Li H, et al. Combination therapy with epcam-Car-Nk-92 cells and regorafenib against human colorectal cancer models. J Immunol Res (2018) 2018:4263520. doi: 10.1155/2018/4263520
95. Xia P, Xu XY. Dkk3 attenuates the cytotoxic effect of natural killer cells on Cd133(+) gastric cancer cells. Mol Carcinog (2017) 56(7):1712–21. doi: 10.1002/mc.22628
96. Yang T, Zhang W, Wang L, Xiao C, Wang L, Gong Y, et al. Co-Culture of dendritic cells and cytokine-induced killer cells effectively suppresses liver cancer stem cell growth by inhibiting pathways in the immune system. BMC Cancer (2018) 18(1):984. doi: 10.1186/s12885-018-4871-y
97. Huang J, Li C, Wang Y, Lv H, Guo Y, Dai H, et al. Cytokine-induced killer (Cik) cells bound with anti-Cd3/Anti-Cd133 bispecific antibodies target Cd133(High) cancer stem cells in vitro and in vivo. Clin Immunol (2013) 149(1):156–68. doi: 10.1016/j.clim.2013.07.006
98. Fu C, Tian G, Duan J, Liu K, Zhang C, Yan W, et al. Therapeutic antitumor efficacy of cancer stem cell-derived dribble vaccine on colorectal carcinoma. Int J Med Sci (2021) 18(14):3249–60. doi: 10.7150/ijms.61510
99. Yin T, Shi P, Gou S, Shen Q, Wang C. Dendritic cells loaded with pancreatic cancer stem cells (Cscs) lysates induce antitumor immune killing effect in vitro. PLos One (2014) 9(12):e114581. doi: 10.1371/journal.pone.0114581
100. Sato-Dahlman M, Miura Y, Huang JL, Hajeri P, Jacobsen K, Davydova J, et al. Cd133-targeted oncolytic adenovirus demonstrates anti-tumor effect in colorectal cancer. Oncotarget (2017) 8(44):76044–56. doi: 10.18632/oncotarget.18340
101. Mato-Berciano A, Raimondi G, Maliandi MV, Alemany R, Montoliu L, Fillat C. A notch-sensitive upar-regulated oncolytic adenovirus effectively suppresses pancreatic tumor growth and triggers synergistic anticancer effects with gemcitabine and nab-paclitaxel. Oncotarget (2017) 8(14):22700–15. doi: 10.18632/oncotarget.15169
102. Zhang X, Komaki R, Wang L, Fang B, Chang JY. Treatment of radioresistant stem-like esophageal cancer cells by an apoptotic gene-armed, telomerase-specific oncolytic adenovirus. Clin Cancer Res (2008) 14(9):2813–23. doi: 10.1158/1078-0432.CCR-07-1528
103. Yano S, Tazawa H, Hashimoto Y, Shirakawa Y, Kuroda S, Nishizaki M, et al. A genetically engineered oncolytic adenovirus decoys and lethally traps quiescent cancer stem-like cells in S/G2/M phases. Clin Cancer Res (2013) 19(23):6495–505. doi: 10.1158/1078-0432.CCR-13-0742
104. Zhang X, Meng S, Zhang R, Ma B, Liu T, Yang Y, et al. Gp73-regulated oncolytic adenoviruses possess potent killing effect on human liver cancer stem-like cells. Oncotarget (2016) 7(20):29346–58. doi: 10.18632/oncotarget.8830
105. Yoo SY, Bang SY, Jeong SN, Kang DH, Heo J. A cancer-favoring oncolytic vaccinia virus shows enhanced suppression of stem-cell like colon cancer. Oncotarget (2016) 7(13):16479–89. doi: 10.18632/oncotarget.7660
106. Soleimani A, Dadjoo P, Avan A, Soleimanpour S, Rajabian M, Ferns G, et al. Emerging roles of Cd133 in the treatment of gastric cancer, a novel stem cell biomarker and beyond. Life Sci (2022) 293:120050. doi: 10.1016/j.lfs.2021.120050
107. Zhou G, Da Won Bae S, Nguyen R, Huo X, Han S, Zhang Z, et al. An aptamer-based drug delivery agent (Cd133-Apt-Dox) selectively and effectively kills liver cancer stem-like cells. Cancer Lett (2021) 501:124–32. doi: 10.1016/j.canlet.2020.12.022
108. Ren F, Sheng WQ, Du X. Cd133: A cancer stem cells marker, is used in colorectal cancers. World J Gastroenterol (2013) 19(17):2603–11. doi: 10.3748/wjg.v19.i17.2603
109. Hermann PC, Huber SL, Herrler T, Aicher A, Ellwart JW, Guba M, et al. Distinct populations of cancer stem cells determine tumor growth and metastatic activity in human pancreatic cancer. Cell Stem Cell (2007) 1(3):313–23. doi: 10.1016/j.stem.2007.06.002
110. Cortes-Dericks L, Galetta D. Impact of cancer stem cells and cancer stem cell-driven drug resiliency in lung tumor: Options in sight. Cancers (Basel) (2022) 14(2):267. doi: 10.3390/cancers14020267
111. Jang JW, Song Y, Kim SH, Kim JS, Kim KM, Choi EK, et al. Cd133 confers cancer stem-like cell properties by stabilizing egfr-akt signaling in hepatocellular carcinoma. Cancer Lett (2017) 389:1–10. doi: 10.1016/j.canlet.2016.12.023
112. Barkal AA, Brewer RE, Markovic M, Kowarsky M, Barkal SA, Zaro BW, et al. Cd24 signalling through macrophage siglec-10 is a target for cancer immunotherapy. Nature (2019) 572(7769):392–6. doi: 10.1038/s41586-019-1456-0
113. Ke J, Wu X, Wu X, He X, Lian L, Zou Y, et al. A subpopulation of Cd24(+) cells in colon cancer cell lines possess stem cell characteristics. Neoplasma (2012) 59(3):282–8. doi: 10.4149/neo_2012_036
114. Patriarca C, Macchi RM, Marschner AK, Mellstedt H. Epithelial cell adhesion molecule expression (Cd326) in cancer: A short review. Cancer Treat Rev (2012) 38(1):68–75. doi: 10.1016/j.ctrv.2011.04.002
115. Cioffi M, Dorado J, Baeuerle PA, Heeschen C. Epcam/Cd3-bispecific T-cell engaging antibody Mt110 eliminates primary human pancreatic cancer stem cells. Clin Cancer Res (2012) 18(2):465–74. doi: 10.1158/1078-0432.CCR-11-1270
116. Gires O, Pan M, Schinke H, Canis M, Baeuerle PA. Expression and function of epithelial cell adhesion molecule epcam: Where are we after 40 years? Cancer Metastasis Rev (2020) 39(3):969–87. doi: 10.1007/s10555-020-09898-3
117. Brown TC, Sankpal NV, Gillanders WE. Functional implications of the dynamic regulation of epcam during epithelial-to-Mesenchymal transition. Biomolecules (2021) 11(7):956. doi: 10.3390/biom11070956
118. Yamashita T, Honda M, Nakamoto Y, Baba M, Nio K, Hara Y, et al. Discrete nature of epcam+ and Cd90+ cancer stem cells in human hepatocellular carcinoma. Hepatology (2013) 57(4):1484–97. doi: 10.1002/hep.26168
119. Dalerba P, Dylla SJ, Park IK, Liu R, Wang X, Cho RW, et al. Phenotypic characterization of human colorectal cancer stem cells. Proc Natl Acad Sci U.S.A. (2007) 104(24):10158–63. doi: 10.1073/pnas.0703478104
120. Krause J, von Felden J, Casar C, Frundt TW, Galaski J, Schmidt C, et al. Hepatocellular carcinoma: Intratumoral epcam-positive cancer stem cell heterogeneity identifies high-risk tumor subtype. BMC Cancer (2020) 20(1):1130. doi: 10.1186/s12885-020-07580-z
121. Li L, Bellows CF. Doublecortin-like kinase 1 exhibits cancer stem cell-like characteristics in a human colon cancer cell line. Chin J Cancer Res (2013) 25(2):134–42. doi: 10.3978/j.issn.1000-9604.2013.03.02
122. Chandrakesan P, Yao J, Qu D, May R, Weygant N, Ge Y, et al. Dclk1, a tumor stem cell marker, regulates pro-survival signaling and self-renewal of intestinal tumor cells. Mol Cancer (2017) 16(1):30. doi: 10.1186/s12943-017-0594-y
123. Westphalen CB, Takemoto Y, Tanaka T, Macchini M, Jiang Z, Renz BW, et al. Dclk1 defines quiescent pancreatic progenitors that promote injury-induced regeneration and tumorigenesis. Cell Stem Cell (2016) 18(4):441–55. doi: 10.1016/j.stem.2016.03.016
124. Ali N, Chandrakesan P, Nguyen CB, Husain S, Gillaspy AF, Huycke M, et al. Inflammatory and oncogenic roles of a tumor stem cell marker doublecortin-like kinase (Dclk1) in virus-induced chronic liver diseases. Oncotarget (2015) 6(24):20327–44. doi: 10.18632/oncotarget.3972
125. Nevi L, Di Matteo S, Carpino G, Zizzari IG, Samira S, Ambrosino V, et al. Dclk1, a putative stem cell marker in human cholangiocarcinoma. Hepatology (2021) 73(1):144–59. doi: 10.1002/hep.31571
126. Wei W, So SK. Glypican-3-Mediates autophagy and promotes self-renewal and tumor initiation of hepatocellular carcinoma cells. J Stem Cell Res Ther (2014) 04(09):1000229. doi: 10.4172/2157-7633.1000229
127. Morita R, Nishizawa S, Torigoe T, Takahashi A, Tamura Y, Tsukahara T, et al. Heat shock protein Dnajb8 is a novel target for immunotherapy of colon cancer-initiating cells. Cancer Sci (2014) 105(4):389–95. doi: 10.1111/cas.12362
128. Wei Y, Wang Y, Gong J, Rao L, Wu Z, Nie T, et al. High expression of mage-A9 contributes to stemness and malignancy of human hepatocellular carcinoma. Int J Oncol (2018) 52(1):219–30. doi: 10.3892/ijo.2017.4198
129. Huang B, Yan X, Li Y. Cancer stem cell for tumor therapy. Cancers (Basel) (2021) 13(19):4814. doi: 10.3390/cancers13194814
130. Zhang D, Tang DG, Rycaj K. Cancer stem cells: Regulation programs, immunological properties and immunotherapy. Semin Cancer Biol (2018) 52(Pt 2):94–106. doi: 10.1016/j.semcancer.2018.05.001
131. Prasad S, Ramachandran S, Gupta N, Kaushik I, Srivastava SK. Cancer cells stemness: A doorstep to targeted therapy. Biochim Biophys Acta Mol Basis Dis (2020) 1866(4):165424. doi: 10.1016/j.bbadis.2019.02.019
132. Yamada R, Takahashi A, Torigoe T, Morita R, Tamura Y, Tsukahara T, et al. Preferential expression of Cancer/Testis genes in cancer stem-like cells: Proposal of a novel Sub-category, Cancer/Testis/Stem gene. Tissue Antigens (2013) 81(6):428–34. doi: 10.1111/tan.12113
133. Menke-van der Houven van Oordt CW, Gomez-Roca C, van Herpen C, Coveler AL, Mahalingam D, Verheul HM, et al. First-in-Human phase I clinical trial of Rg7356, an anti-Cd44 humanized antibody, in patients with advanced, Cd44-expressing solid tumors. Oncotarget (2016) 7(48):80046–58. doi: 10.18632/oncotarget.11098
134. Ning ST, Lee SY, Wei MF, Peng CL, Lin SY, Tsai MH, et al. Targeting colorectal cancer stem-like cells with anti-Cd133 antibody-conjugated Sn-38 nanoparticles. ACS Appl Mater Interfaces (2016) 8(28):17793–804. doi: 10.1021/acsami.6b04403
135. Seimetz D, Lindhofer H, Bokemeyer C. Development and approval of the trifunctional antibody catumaxomab (Anti-epcam X anti-Cd3) as a targeted cancer immunotherapy. Cancer Treat Rev (2010) 36(6):458–67. doi: 10.1016/j.ctrv.2010.03.001
136. Jager M, Schoberth A, Ruf P, Hess J, Hennig M, Schmalfeldt B, et al. Immunomonitoring results of a phase Ii/Iii study of malignant ascites patients treated with the trifunctional antibody catumaxomab (Anti-epcam X anti-Cd3). Cancer Res (2012) 72(1):24–32. doi: 10.1158/0008-5472.CAN-11-2235
137. Fossati M, Buzzonetti A, Monego G, Catzola V, Scambia G, Fattorossi A, et al. Immunological changes in the ascites of cancer patients after intraperitoneal administration of the bispecific antibody catumaxomab (Anti-Epcamxanti-Cd3). Gynecol Oncol (2015) 138(2):343–51. doi: 10.1016/j.ygyno.2015.06.003
138. Wimberger P, Gilet H, Gonschior AK, Heiss MM, Moehler M, Oskay-Oezcelik G, et al. Deterioration in quality of life (Qol) in patients with malignant ascites: Results from a phase Ii/Iii study comparing paracentesis plus catumaxomab with paracentesis alone. Ann Oncol (2012) 23(8):1979–85. doi: 10.1093/annonc/mds178
139. Knodler M, Korfer J, Kunzmann V, Trojan J, Daum S, Schenk M, et al. Randomised phase ii trial to investigate catumaxomab (Anti-epcam X anti-Cd3) for treatment of peritoneal carcinomatosis in patients with gastric cancer. Br J Cancer (2018) 119(3):296–302. doi: 10.1038/s41416-018-0150-6
140. Gong X, Azhdarinia A, Ghosh SC, Xiong W, An Z, Liu Q, et al. Lgr5-targeted antibody-drug conjugate eradicates gastrointestinal tumors and prevents recurrence. Mol Cancer Ther (2016) 15(7):1580–90. doi: 10.1158/1535-7163.MCT-16-0114
141. Zhang BL, Li D, Gong YL, Huang Y, Qin DY, Jiang L, et al. Preclinical evaluation of chimeric antigen receptor-modified T cells specific to epithelial cell adhesion molecule for treating colorectal cancer. Hum Gene Ther (2019) 30(4):402–12. doi: 10.1089/hum.2018.229
142. Wang Y, Chen M, Wu Z, Tong C, Dai H, Guo Y, et al. Cd133-directed car T cells for advanced metastasis malignancies: A phase I trial. Oncoimmunology (2018) 7(7):e1440169. doi: 10.1080/2162402X.2018.1440169
143. Feng KC, Guo YL, Liu Y, Dai HR, Wang Y, Lv HY, et al. Cocktail treatment with egfr-specific and Cd133-specific chimeric antigen receptor-modified T cells in a patient with advanced cholangiocarcinoma. J Hematol Oncol (2017) 10(1):4. doi: 10.1186/s13045-016-0378-7
144. Becerril-Rico J, Alvarado-Ortiz E, Toledo-Guzman ME, Pelayo R, Ortiz-Sanchez E. The cross talk between gastric cancer stem cells and the immune microenvironment: A tumor-promoting factor. Stem Cell Res Ther (2021) 12(1):498. doi: 10.1186/s13287-021-02562-9
145. Masoumi J, Jafarzadeh A, Abdolalizadeh J, Khan H, Philippe J, Mirzaei H, et al. Cancer stem cell-targeted chimeric antigen receptor (Car)-T cell therapy: Challenges and prospects. Acta Pharm Sin B (2021) 11(7):1721–39. doi: 10.1016/j.apsb.2020.12.015
146. Yin T, Wang G, He S, Liu Q, Sun J, Wang Y. Human cancer cells with stem cell-like phenotype exhibit enhanced sensitivity to the cytotoxicity of il-2 and il-15 activated natural killer cells. Cell Immunol (2016) 300:41–5. doi: 10.1016/j.cellimm.2015.11.009
147. Ames E, Canter RJ, Grossenbacher SK, Mac S, Chen M, Smith RC, et al. Nk cells preferentially target tumor cells with a cancer stem cell phenotype. J Immunol (2015) 195(8):4010–9. doi: 10.4049/jimmunol.1500447
148. Ames E, Canter RJ, Grossenbacher SK, Mac S, Smith RC, Monjazeb AM, et al. Enhanced targeting of stem-like solid tumor cells with radiation and natural killer cells. Oncoimmunology (2015) 4(9):e1036212. doi: 10.1080/2162402X.2015.1036212
149. Donini C, Rotolo R, Proment A, Aglietta M, Sangiolo D, Leuci V. Cellular immunotherapy targeting cancer stem cells: Preclinical evidence and clinical perspective. Cells (2021) 10(3):543. doi: 10.3390/cells10030543
150. Shokouhifar A, Firouzi J, Nouri M, Sarab GA, Ebrahimi M. Nk cell upraise in the dark world of cancer stem cells. Cancer Cell Int (2021) 21(1):682. doi: 10.1186/s12935-021-02400-1
151. Guo M, Luo B, Pan M, Li M, Zhao F, Dou J. Muc1 plays an essential role in tumor immunity of colorectal cancer stem cell vaccine. Int Immunopharmacol (2020) 85:106631. doi: 10.1016/j.intimp.2020.106631
152. Guo M, Luo B, Pan M, Li M, Xu H, Zhao F, et al. Colorectal cancer stem cell vaccine with high expression of Muc1 serves as a novel prophylactic vaccine for colorectal cancer. Int Immunopharmacol (2020) 88:106850. doi: 10.1016/j.intimp.2020.106850
153. Lin M, Yuan YY, Liu SP, Shi JJ, Long XA, Niu LZ, et al. Prospective study of the safety and efficacy of a pancreatic cancer stem cell vaccine. J Cancer Res Clin Oncol (2015) 141(10):1827–33. doi: 10.1007/s00432-015-1968-4
154. Bagheri V, Abbaszadegan MR, Memar B, Motie MR, Asadi M, Mahmoudian RA, et al. Induction of T cell-mediated immune response by dendritic cells pulsed with mrna of sphere-forming cells isolated from patients with gastric cancer. Life Sci (2019) 219:136–43. doi: 10.1016/j.lfs.2019.01.016
155. Sun JC, Pan K, Chen MS, Wang QJ, Wang H, Ma HQ, et al. Dendritic cells-mediated ctls targeting hepatocellular carcinoma stem cells. Cancer Biol Ther (2010) 10(4):368–75. doi: 10.4161/cbt.10.4.12440
156. Fu C, Zhou N, Zhao Y, Duan J, Xu H, Wang Y. Dendritic cells loaded with Cd44(+) ct-26 colon cell lysate evoke potent antitumor immune responses. Oncol Lett (2019) 18(6):5897–904. doi: 10.3892/ol.2019.10952
157. Liao F, Zhang J, Hu Y, Najafabadi AH, Moon JJ, Wicha MS, et al. Efficacy of an aldh peptide-based dendritic cell vaccine targeting cancer stem cells. Cancer Immunol Immunother (2022) 71(8):1959–73. doi: 10.1007/s00262-021-03129-6
158. Dong H, Su H, Chen L, Liu K, Hu HM, Yang W, et al. Immunocompetence and mechanism of the dribble-dcs vaccine for oral squamous cell carcinoma. Cancer Manag Res (2018) 10:493–501. doi: 10.2147/CMAR.S155914
159. Su H, Luo Q, Xie H, Huang X, Ni Y, Mou Y, et al. Therapeutic antitumor efficacy of tumor-derived autophagosome (Dribble) vaccine on head and neck cancer. Int J Nanomedicine (2015) 10:1921–30. doi: 10.2147/IJN.S74204
160. Yi Y, Han J, Zhao L, Wang C, Fang Y, Wei Q, et al. Immune responses of dendritic cells combined with tumor-derived autophagosome vaccine on hepatocellular carcinoma. Chin J Cancer Res (2015) 27(6):597–603. doi: 10.3978/j.issn.1000-9604.2015.12.07
Keywords: cancer stem cells, immunotherapy, gastrointestinal malignancies, anti-tumor therapy, immune evasion
Citation: An J, Hu X and Liu F (2023) Current understanding of cancer stem cells: Immune evasion and targeted immunotherapy in gastrointestinal malignancies. Front. Oncol. 13:1114621. doi: 10.3389/fonc.2023.1114621
Received: 02 December 2022; Accepted: 09 February 2023;
Published: 23 February 2023.
Edited by:
Steven Zheng, Rutgers, The State University of New Jersey, United StatesReviewed by:
Jin Hou, Second Military Medical University, ChinaYan Zhang, Shanghai Jiao Tong University, China
Dainius Characiejus, Vilnius University, Lithuania
Bashdar Mahmud Hussen, Hawler Medical University, Iraq
Copyright © 2023 An, Hu and Liu. This is an open-access article distributed under the terms of the Creative Commons Attribution License (CC BY). The use, distribution or reproduction in other forums is permitted, provided the original author(s) and the copyright owner(s) are credited and that the original publication in this journal is cited, in accordance with accepted academic practice. No use, distribution or reproduction is permitted which does not comply with these terms.
*Correspondence: Feng Liu, bGl1ZmVuZ0BzaHNtdS5lZHUuY24=; Xiaohua Hu, NjExMTAwQHNoOWhvc3BpdGFsLm9yZy5jbg==