- 1Innovative Institute, China Medical University, Shenyang, China
- 2Department of Pharmacology, School of Pharmacy, China Medical University, Shenyang, China
- 3Liaoning Key Laboratory of Molecular Targeted Anti-Tumor Drug Development and Evaluation, Shenyang, China
- 4Liaoning Cancer Immune Peptide Drug Engineering Technology Research Centre, Shenyang, China
- 5Shenyang Kangwei Medical Laboratory Analysis Co. LTD, Shenyang, China
Advanced non-small cell lung cancer (NSCLC) is a severe disease and still has high mortality rate after conventional treatment (e.g., surgical resection, chemotherapy, radiotherapy and targeted therapy). In NSCLC patients, cancer cells can induce immunosuppression, growth and metastasis by modulating cell adhesion molecules of both cancer cells and immune cells. Therefore, immunotherapy is increasingly concerned due to its promising anti-tumor effect and broader indication, which targets cell adhesion molecules to reverse the process. Among these therapies, immune checkpoint inhibitors (mainly anti-PD-(L)1 and anti-CTLA-4) are most successful and have been adapted as first or second line therapy in advanced NSCLC. However, drug resistance and immune-related adverse reactions restrict its further application. Further understanding of mechanism, adequate biomarkers and novel therapies are necessary to improve therapeutic effect and alleviate adverse effect.
Introduction
Nowadays, cancer is the second leading cause of death, the first leading cause of disability-adjusted life years (DALYs) loss and will probably be the first leading cause of death in 2060 (1). According to WHO GLOBOCAN 2020 database, lung cancer is the second most common cancer after breast cancer and causes more dead cases than any other cancer. To make matters worse, smoking and air pollution are still increasing in many developing countries (2). The affordable and accessible cigarettes and chronic health effect of nicotine leads to long-term dependence of smoking and an elevation of lung cancer risk (3). Lung cancer is a kind of highly heterogenous disease and can be categorized into two main groups: NSCLC (85%) and SCLC (15%) (4). In this review, we will mainly talk about advanced NSCLC which is unresectable for most patients. Chemotherapy and radiotherapy also provide modest efficacy (5). Target therapy has been invented and adapted as first-line treatment for certain genotype NSCLC patients, which can inhibit tumor progression and significantly improve prognosis by blocking abnormal signaling pathway (6). However, targeted therapy is not effective forever. For example, NSCLC will develop resistance to EGFR tyrosine kinase inhibitors (TKIs) after a median of 10 to 14 months treatment and effective treatment has not been defined except Osimertinib for the T790M mutation (7, 8).
Studies have showed that tumor immune evasion participates in the whole course of NSCLC rather than only in advanced stage. Immune cells will be activated at early stage with an elevation of anti-tumor cells (e.g., NK cells and CD8 + cells) but soon inhibited at advanced stage (e.g., the transitory activation of CD4 + and CD8 + T cells, from Th1 to Th2, the increase of regulatory T cells (Tregs)) (9, 10). Cell adhesion molecules mediate the contact and binding between cells or between cells and extracellular matrix. They can be divided into five groups: integrins, selectins, cadherins, immunoglobulin superfamily and mucin-like vascular addressin and are crucial for the formation of tumor microenvironment (11). The overexpression of CTLA-4, PD-1, and PD-L1 can negatively regulate anti-tumor immunity (9). Low expression of E-cadherin is associated with poor prognosis and LFA-1 participates in T cell activation and migration (12, 13). In this review, we will mainly talk about how cell adhesion molecules participate in tumor progression and therapies for cell adhesion molecules.
Immune checkpoint inhibitors (ICIs) can blockade negative costimulatory molecules and reverse the tumor-induced immunosuppression. ICI is the only immunotherapy that showed clinical benefit in NSCLC and has showed better long-term survival compared with chemotherapy and radiotherapy (14, 15) (Table 1). Despite of these advantages, ICIs also have its limitations. Primary and secondary resistance to ICIs is common and around 40–50% of lung cancer patients experienced disease deterioration during the first cycles of immunotherapy (16). Various immune-related adverse effects (irAEs) decrease life quality and even leads to treatment failure or death (17). Combined therapy, biomarkers and novel ICIs are promising to increase the response rate and treatment efficacy (18). After adequate treatment, retreatment is possible and may be beneficial for irAE patients who had no treatment response before irAEs onset (19). Cell adhesion molecules are also potential targets for other immunotherapies (e.g., tumor vaccines, CAR-T) which may be helpful to enhance immunotherapy efficacy (20, 21).
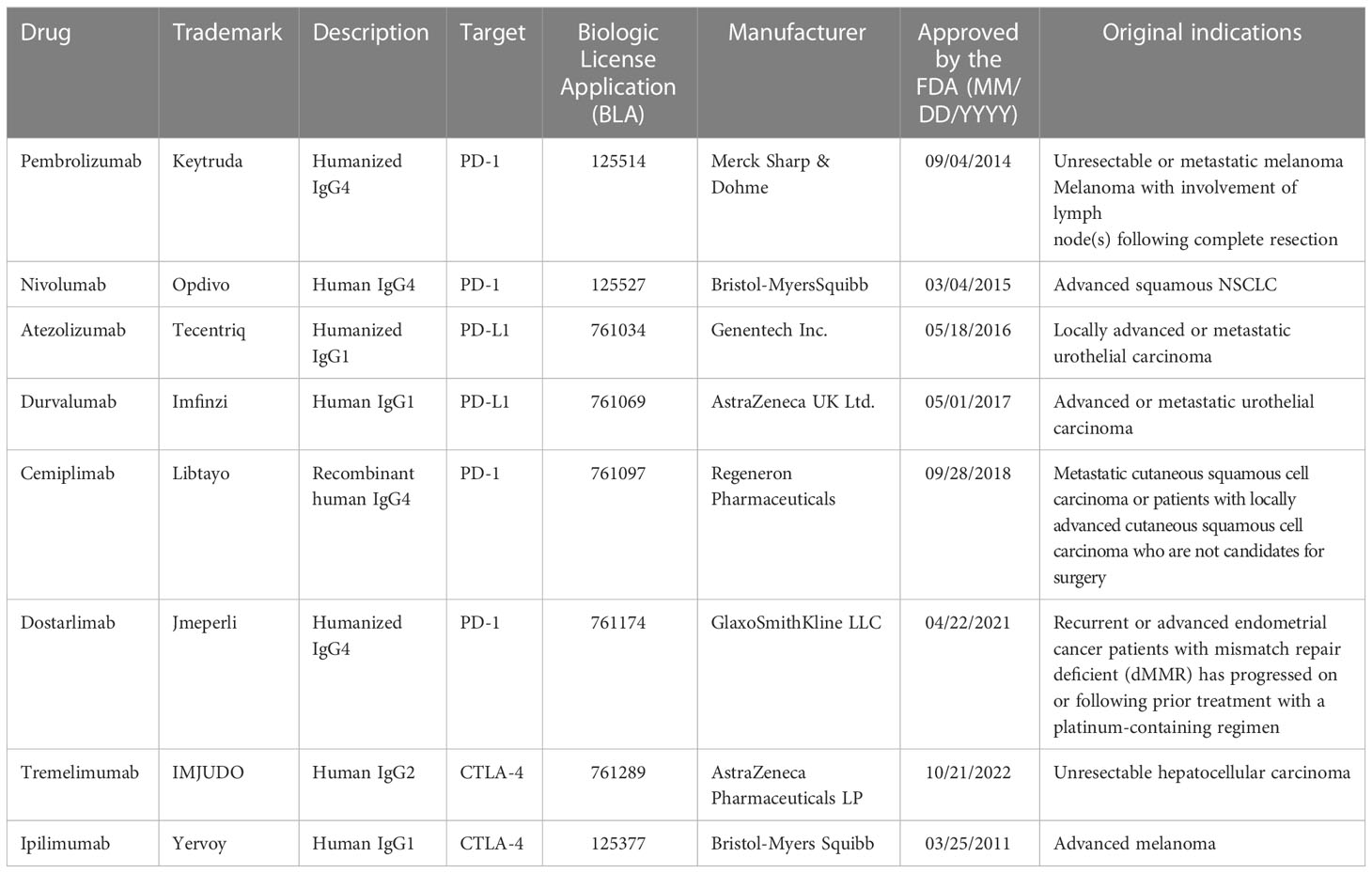
Table 1 Overview of anti-PD-1/PD-L1 and CTLA-4 monoclonal antibodies approved by the FDA with NSCLC indication as of November 2022.
Effects of cell adhesion molecules on the progression of NSCLC
Cell adhesion molecules plays a crucial role in tumor progression. MUC-1 expression on tumor cells promotes metastasis by binding to intercellular adhesion molecule-1 (ICAM-1) in endothelial cells. Integrins increases the number of tumor-associated macrophages (TAMs), and both integrin and TAMs are associated with epithelial-to-mesenchymal transition of epithelial tumor cells, which leads to loss of epithelial markers and increase of mesenchymal markers. As a result, tumor cells are more easily to metastasis (22, 23). Some cell adhesion molecules also mediate tumor immune evasion, such as cytolytic T lymphocyte-associated protein-4 (CTLA-4) and programmed cell death protein-1 (PD-1)/programmed cell death-ligand 1 (PD-L1), which are also called immune checkpoint (24). High expression of certain cell adhesion molecules in Tregs may lead to Tregs infiltration and immunosuppression at tumor site (11). For NSCLC, higher immune score was associated with better prognosis in adenocarcinoma, while this relation was not found in squamous cell carcinoma (25). In this review, we will mainly talk about aberrant cell adhesion molecules in NSCLC.
LFA-1 and ICAM-1
LFA-1 (CD11a/CD18) belongs to integrin family and mainly expressed by blood cells and ICAM-1 is an important ligand for LFA-1. Besides the adhesive function like other integrins, LFA-1 has “outside-in” and “inside-out” bidirectional signaling pathways, it also has different conformations, from folded low-affinity to extended high-affinity. LFA-1 has complex effect in tumor progression. On the one hand, it promotes the differentiation of T cells, mediates cytotoxic anti-tumor response and is necessary for lymphocyte infiltration; on the other hand, leukocytes infiltration may promote tumor progression, such as Tregs (26). Like LFA-1, ICAM-1 is also important in tumor progression. ICAM family belongs to immunoglobulin superfamily and participates in immune responses and intracellular signaling. Currently, there are five known ICAMs (ICAM-1 to ICAM-5) (27).
LFA-1 is a key T cell integrin and participates in regulation of T cell activation and migration (12). LFA-1 rather than CD28 enhanced the impact of TCR clustering, which is consistent with the localization effect of LFA-1 (28, 29). This process is largely dependent on the activation of multiple kinases and adaptor proteins, such as phosphoinositide 3-kinase, Crk protein and ERK pathways (30, 31). The binding of LFA-1 and ICAM-1 participates in T cell differentiation into specific phenotype (32). The binding of LFA-1 with high density ICAM-1 can directly facilitate cytokine secretion by increasing cytoplasmic Ca2+ and ERK phosphorylation in invariant natural killer T cells (33). LFA-1 is also necessary for extracellular ISG15 to mediate IL-10 and IFN-γ secretion (34). PI3Kδ also facilitates T cell activation by increasing LFA-1/ICAM-1 interaction (35). And G-CSF inhibits LFA-1/ICAM-1-mediated CD4 + T cells inflammatory cytokines secretion by down-regulating Lck and ZAP-70 (36). CD8 + T cell has intracellular LFA-1 storage, which can be transported to cell surface after antigenic stimulation and is an important mechanism for LFA-1 to regulate T cell differentiation (37). Although ICAMs is not necessary for Th1 differentiation, LFA-1/ICAM-1 can promotes Th1 polarization through Notch pathway (38, 39). LFA-1 also plays an important role in normal T follicular helper cells function through upregulation of Bcl-6 and DOCK8 (40, 41).
In tumor progression, aberrant ICAM-1 expression and impaired LFA-1/ICAM-1 function has been observed. Increased sICAM-1 concentration in the exhaled breath condensate has been observed in NSCLC patients compared with that of healthy people and COPD patients and sICAM-1 concentration was significantly decreased after resection (63.4± 26.0 ng/mL vs 44.0 ± 17.7 ng/mL, p < 0.01) (42). A meta-analysis revealed that higher concentration of circulating soluble ICAM-1 (sICAM-1) was associated with more advanced disease stage and poorer prognosis in NSCLC. This may be explained by the impaired ICAM-1/LFA-1 interaction due to the binding of sICAM-1 and LFA-1, tissue damage and angiogenesis caused by increased sICAM-1 (43). Galectin can disrupt the formation of functional tumor-infiltrating T cells synapse by preventing LFA-1 recruitment and the affinity regulation (44). ICAM-3 promoted tumor metastasis by binding to LFA-1 in NSCLC cells (45). LFA-1/ICAM-1 also disrupts CD8 + T cells recirculation by promoting tumor tissues aggregation (46). NSCLC cells can adhere to vertebral bone marrow endothelial cells through CX3CL1/ICAM-1/LFA-1 pathway and lead to spinal metastasis (47). On the other hand, LFA-1/ICAM-1 interaction has potential anti-tumor effect because it is an alternative costimulatory signaling to activate anti-tumor cytotoxic T cells (48). NSCLC cells exhibited an IFN-γ-dependent ICAM-1 upregulation activated by EGFR CAR-T cells. This ICAM-1 upregulation permitted EGFR CAR-T cells to move from tumor edge to center, and blockade of ICAM-1/LFA-1 disrupted the CAR-T cell tumor infiltration (49). Impaired ICAM-1 upregulation on alveolar macrophages in patients with NSCLC after IFN-γ stimulation had also been observed, which might disrupt the normal function of alveolar macrophages and more studies are needed due to the small sample size and unclear mechanisms of this study (50). This contradiction has also been observed in other studies. Cannabinoids was reported to inhibit lung cancer cell invasion and metastasis by upregulating ICAM-1 in vitro (51). However, studies found that low level of ICAM-1 is associated with longer progression-free survival (PFS) and overall survival (OS). This may be contributed to the relation between aggressive NSCLC histological subtypes and high ICAM-1 expression. (e.g., adenocarcinomas and undifferentiated carcinomas) (52, 53).
CTLA-4
CTLA-4 (CD152) is a kind of surface molecule in T cells and associated with immune regulation. Both CTLA-4 and CD28 can bind to B7-1 and B7-2. CD28 participates in the co-stimulation of T cells in the interaction between antigen presenting cells and T cells, while CTLA-4 has a higher affinity than CD28 and inhibits the activation of T cells. Therefore, CTLA-4 is highly expressed in activated T cells and Tregs in order to avoid autoimmune disease. However, CTLA-4 is activated in NSCLC and leads to immunosuppression and tumor immune evasion. These indicate that the blockage of CTLA-4 may be a promising immunotherapy target (25).
Ipilimumab is a CTLA-4-targeted monoclonal antibody and had been approved by food and drug administration (FDA) in metastatic melanoma. However, ipilimumab showed less effect compared with anti-PD-1 therapy when combined with radiotherapy in metastatic NSCLS patients (54). A meta-analysis showed that the combination of ipilimumab and chemotherapy didn’t improve OS compared to pure chemotherapy and were associated with more immune-related toxicities (55). Therefore, anti-CTLA-4 therapy is greatly restricted and has not been approved for NSCLC treatment (56).
PD-1/PD-L1
PD-1/PD-L1 is an immune checkpoint which is related to inhibitory immune regulation. PD-1 is expressed in a variety of immune cells especially T cells and has two ligands: PD-L1 and PD-L2. In cancer, overexpression of PD-L1 contributes to T cells hypofunction and apoptosis (57). For T cells, PD-1/PD-L1 ligation inhibits TCR proximal signaling molecules phosphorylation, PI3K–Akt–mTOR pathway and Ras–MEK–ERK pathway, it also contributes to dysfunction of T cell–dendritic cell interaction and metabolic alteration; for cancer cells, PD-L1 leads to antiapoptotic effect, immune evasion, PI3K–Akt–mTOR pathway activation and glycolysis, which facilitate cancer cell survival (Figure 1) (58). Therefore, PD-1/PD-L1 inhibitor blocks this process and shows satisfactory prognosis improvement in NSCLC (14).
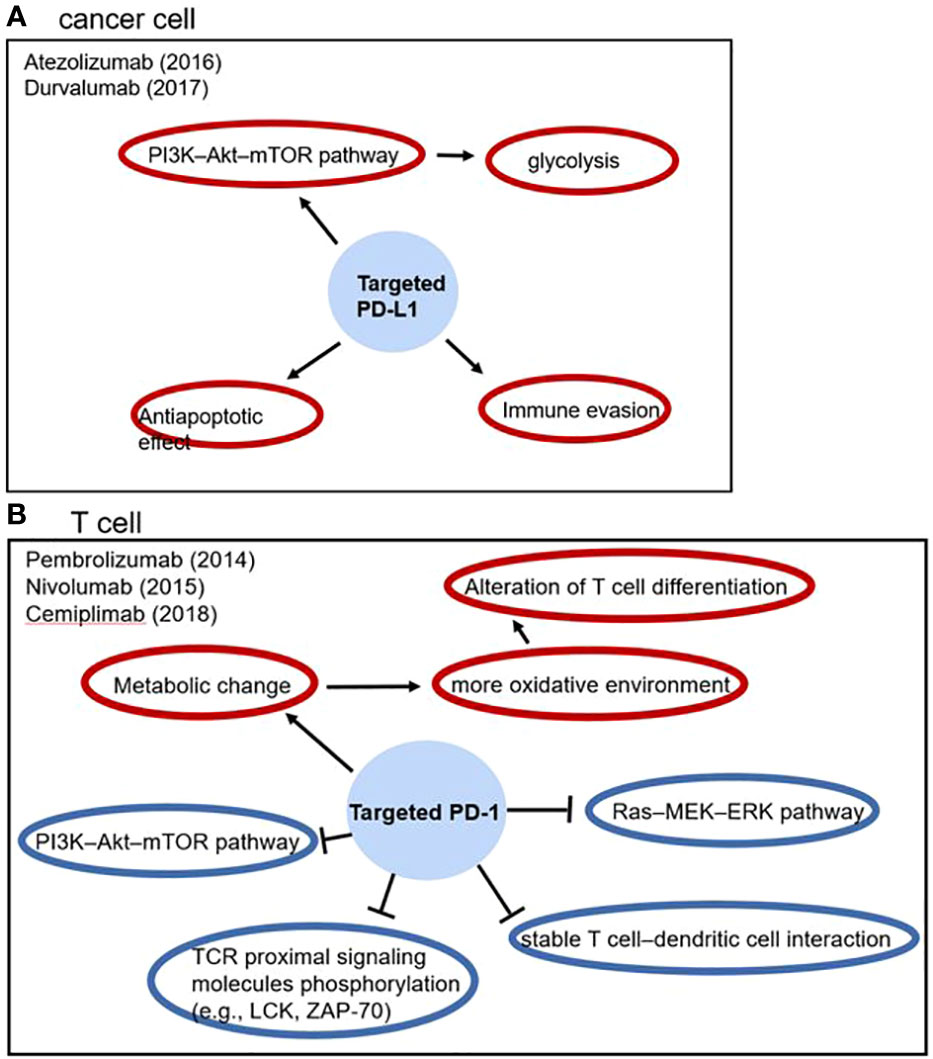
Figure 1 The effect of PD-1/PD-L1 in tumor progression and anti-PD-1/PD-L1 drugs, blue circles represent inhibitory effects and red circles represent stimulatory effects.
PD-1/PD-L1 is a distinct target for NSCLC and has little cross-resistance with other kinds of anti-tumor agents. Clinical benefit of Osimertinib (a third generation EGFR TKI) is independent of PD-L1 expression level (59). PD-1 inhibitors are effective for NSCLCs with high PD-L1 expression regardless of EGFR genotype (60). PD-L1 expression is also failed to be a prognostic factor for advanced NSCLC patients with chemotherapy (61).
E-cadherin
The cadherin family is a class of cell adhesion molecules that mediates intercellular adhesion (62). Cadherin, especially E-cadherin, plays an important role in contact inhibition through Hippo, Wnt, TGF-β, NF-κB and other signal pathways. And downregulation of E-cadherin expression is associated with tumor progression and metastasis (63). Tissue resident memory T cell is a kind of tumor-specific T cells which can binds to E-cadherin and mediates T-cell receptor-dependent cytotoxic effect in NSCLC (64). The upregulation of E-cadherin and downregulation of N-cadherin induced by BRAF-activated non-coding RNA overexpression is also associated with epithelial to mesenchymal transition inhibition in lung cancer (65). And E-cadherin gene promoter hypermethylation is related to an elevated risk of NSCLC (66).
The expression level of E-cadherin and other relevant molecules may be promising biomarkers for NSCLC prognosis. Low expression of E-cadherin is closely associated with poor prognosis and biological behavior such as advanced clinical stage and lymph node metastasis, while high expression of paired related homeobox 1 (PRRX1) and low zinc expression of finger E-box binding homeobox 1 (ZEB1) are related to high E-cadherin expression and low level of epithelial-mesenchymal transition and tumor angiogenesis in NSCLC patients. These findings suggest that PRRX1 and ZEB1 may also be potential prognostic biomarkers and therapeutic targets for NSCLC (13, 67). The modification of E-cadherin can also affect the tumor progression. FUT8 gene encodes α-1,6-fucosyltransferase, which is the only enzyme for core-fucosylation on N-glycoproteins. Upregulation of FUT8 leads to an increase of core fucosylated E-cadherin and inhibits normal E-cadherin function. As a result, cancer metastasis is promoted. This is consistent with the correlation that upregulation of FUT8 is associated with increased tumor metastasis and worse survival in NSCLC, indicating that FUT8 may also be a potential prognostic biomarker for NSCLC (68).
MUC-1
Mucin 1 (MUC-1) is an overexpressed glycoprotein and associated with cancer cell proliferation, migration and invasion in NSCLC. MUC1 consists of an extracellular N-terminal subunit (MUC1-N) and a transmembrane MUC1 C-terminal subunit (MUC1-C). From precancerous lesions to invasive carcinoma, upregulation of MUC-1 expression has been observed in patients with NSCLC (69). Studies showed that MUC1 was regulated by STAT3 and MUC1-C promoted the self-renewal of NSCLC cells via LIN28B/let-7/HMGA2 axis (70, 71). MUC1-C enhanced MYC expression in KRAS mutant NSCLC cells via WNT/β-catenin (CTNNB1) pathway activation, forming MUC1-C/β-catenin/TCF4 complexes on the MYC promoter and recruiting the p300 histone acetylase (EP300) to enhance histone H3 acetylation and MYC gene transcription. And MUC1-C inhibition contributed to an inhibition of MYC gene expression, tumor cell survival and tumor growth in KRAS mutant NSCLC cells (72). MUC1-C suppression was associated with inhibition of epithelial-mesenchymal transition (EMT) and KRAS independence in KRAS mutant NSCLC cells, which contributed to tumor growth inhibition (73). MUC1-C blockade also contributed to EGFR (T790M), AKT and ERK signaling suppression and survival inhibition in NSCLC cells with mutant EGFR (74). Silencing of MUC1 also enhanced the anti-tumor efficacy of paclitaxel against paclitaxel-resistant cell line A549/PR in NSCLC via Bax and Caspase-3 upregulation and Bcl-2 downregulation (75). MUC1 is also associated with tumor associated macrophage-induced lung cancer stem cell progression (76). In vitro, downregulation of MUC-1 was associated with AKT and ERK suppression, decreased VEGF and VEGF-C, tumor cell proliferation inhibition and increased cell apoptosis in NSCLC (77). Upregulation of MUC-1 after EGFR-targeted therapy has been observed, which is associated with PI3K/AKT/mTOR and JAK2/STAT3 pathways. This finding indicates that serum MUC-1 may be a novel biomarker for anti-EGFR therapy monitoring (78).
Other molecules
CD44 is a non-kinase transmembrane glycoprotein and associated with tumor progression, metastasis and drug resistance in NSCLC (79, 80). High CD44v expression in tumor was related to shorter overall survival (p<0.001) and recurrence-free survival (p<0.001) after curative resection for patients with NSCLC (80). CD44s expression was related to lymph node metastases (p=0.007) and CD44v6 expression was more related to tumor size (p=0.0032) in lung adenocarcinoma (81). A study demonstrated that CD44 enhanced PD-L1 expression partly by the cleaved intracytoplasmic domain of CD44 bound to the consensus CD44-ICD binding site on the regulatory region of the PD-L1 locus in NSCLC (82). CD44 is also a downstream molecule upregulated by Notch3 in enhancing stem-like property in NSCLC cells (83). A study showed that CD44 facilitated CD133+CD44+ lung cancer stem cells metastasis via Wnt/β-catenin-FoxM1-Twist signaling (84). In vitro, overexpression of CD44 promoted NSCLC cell proliferation and colony formation and vice versa (85). Serglycin induces NSCLC cell migration by binding to the GAG motif to CD44 (86). The expression of CD44 is more frequent in circulating tumor cells than in brain metastasis. This may be contributed to the importance of CD44 in tumor cell survival in the blood flow, decline of CD44 after chemotherapy and radiotherapy (87). Sp1 is an important transcription factor in NSCLC progression, which increases in the early stage but decreases in the late stage. It can inhibit tumor stemness and metastasis. CD44 expression can be inhibited by Sp1 through inducing the expression of miR-3194-5p, miR-218-5p, miR-193-5p, miR-182-5p and miR-135-5p (88). Hyaluronan-CD44/HA-mediated motility receptor signaling pathway is overexpressed in NSCLC and associated with cell proliferation and survival (89). VCAM-1 also participates in NSCLC progression. miR-26a is downregulated in NSCLC, and it can block IL-2 mediated proliferation and migration in NSCLC by binding to the 3’-UTR binding sequence of VCAM-1 (90). Study also showed that sVCAM1 secreted by CXCR4-overexpressing NSCLC cells promoted osteoclastogenesis (91).
Current and potential applications of cell adhesion molecules on NSCLC
Anti-PD-1/PD-L1
PD-1/PD-L1 is an immune checkpoint and has been targeted by several monoclonal antibodies. Atezolizumab, avelumab and durvalumab are PD-L1-targeted while the other monoclonal antibodies mentioned in this paragraph are PD-1-targeted (Table 2). The addition of atezolizumab to bevacizumab plus chemotherapy significantly improved PFS and OS of metastatic non-squamous NSCLC patients and the Phase 3 IMpower132 study showed that combination of atezolizumab and carboplatin/cisplatin and pemetrexed significantly improved PFS (median 7.6 vs 5.2 months, HR = 0.60, 95%CI: 0.49–0.72, p < 0.0001) of non-squamous NSCLC than that of carboplatin/cisplatin and pemetrexed while statistical difference in OS had not been observed (92, 95). Atezolizumab also outcompeted docetaxel in OS for previously treated NSCLC patients (93, 96). Compared with docetaxel, avelumab failed to prolong OS in patients with platinum-treated PD-L1+ NSCLC. However, after 2 years follow-up, avelumab showed better OS than docetaxel especially in high PD-L1 expression subgroups (PD-L1≥50%) (99, 100). Durvalumab prolonged PFS and OS compared with placebo and this effect was further observed after 3 and 4 years, indicating the long survival benefit of durvalumab (94, 101, 102). However, durvalumab failed to statistically improve OS compared with chemotherapy in metastatic NSCLC first-line treatment (103). Cemiplimab also significantly improved OS and PFS compared with chemotherapy in patients with advanced NSCLC (PD-L1>50%) (97). Compared with docetaxel, nivolumab prolonged OS of previously treated NSCLC in predominantly Chinese patient subgroup after 2 years, which is consistent with the outcome of CheckMate 017 (104, 105). Several studies have showed that pembrolizumab plus chemotherapy improved OS compared with chemotherapy. Pembrolizumab also outcompeted chemotherapy in OS (98, 106–110). Sintilimab and tislelizumab are two Chinese original anti-PD-1 monoclonal antibodies. They are more affordable and cost-effective for Chinese patients compared with foreign drugs (111, 112). They also showed improvement of PFS in combination with chemotherapy compared with chemotherapy alone. However, OS was not obtained and more studies are needed (113, 114).
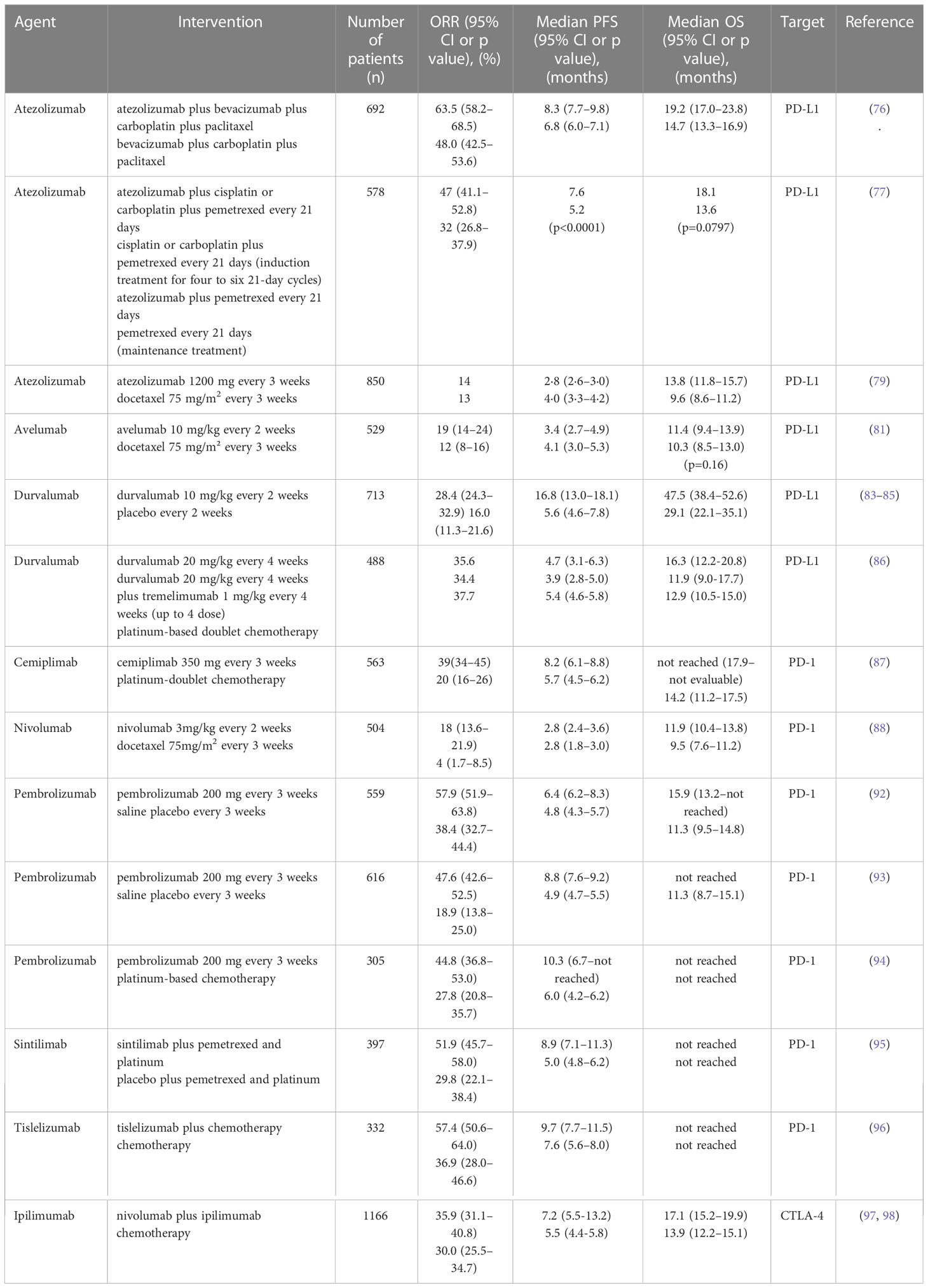
Table 2 Completed phase 3 NSCLC clinical trials of PD-1/PD-L1 and CTLA-4-targeted monoclonal antibodies in recent 5 years.
The expression level of PD-L1 in NSCLC cells is an important criterion for PD-1/PD-L1 treatment. For pembrolizumab, PD-L1 expression≥50% is suitable for first-line therapy and ≥1% for second-line treatment, respectively. tumor is considered as PD-L1 positive if more than 50% tumor cells express PD-L1 and suitable for anti-PD-1/PD-L1 treatment. However, for the combination of chemotherapy and immunotherapy, PD-L1 expression didn’t affect survival benefit and PD-L1 expression examination is unnecessary (115). Training for pathologists may increase reliability for samples around 50% and has very little or no impact on the inter- or intra-observer reproducibility (116).
Anti-CTLA-4
Ipilimumab is a CTLA-4-targeted monoclonal antibody which has applied in melanoma. However, it shows limited therapeutic effect and obvious adverse effect in NSCLC. Currently, all anti-CTLA-4 immunotherapy alone has limited application in NSCLC.
The effect of blocking CTLA-4 remains unclear, which limits the development of new antibodies. Traditionally, it is believed that anti-CTLA-4 antibodies produces anti-tumor effect through blocking the interaction of CTLA-4/B7. Some studies argued that anti-CTLA-4 antibodies produces anti-tumor effect by killing Tregs through antibody-dependent cell-mediated cytotoxicity because Fc domain is essential for ipilimumab anti-tumor function (117, 118). On the other hand, tremelimumab is a new CTLA-4-targeted IgG2 monoclonal antibody with less antibody-dependent cell-mediated cytotoxicity (ADCC) and also showed anti-tumor effect in clinical trials and is supported by a murine surrogate antibodies experiment, which showed that anti-tumor effect was not completely dependent on ADCC (119). Similar CTLA-4 binding properties between ipilimumab and tremelimumab also indicates the importance of CTLA-4/B7 blockage (120). Therefore, anti-CTLA-4 antibodies may produce anti-tumor effect through both CTLA-4/B7 blockage and ADCC, and other unknown mechanisms may also take effect.
Anti-MUC1
Tecemotide (L-BLP25) is a MUC1 antigen-specific tumor vaccine to induce T cell immunity against MUC1. A phase 3 clinical trial (NCT00409188) showed that no statistical difference was observed between tecemotide group (median 25.8 months) and placebo group (median 22.4 months) after chemoradiotherapy in overall survival (HR 0.89, 95% CI 0.77–1.03, P = 0.111) for patients with unresectable stage III NSCLC. However, improvement median survival in tecemotide group was observed in the analysis of concurrent chemoradiotherapy subgroup (29.4 months versus 20.8 months, aHR 0.81, 95% CI 0.68–0.98, P = 0.026). Elevated sMUC1 and ANA might be associated with survival benefit with tecemotide (interaction P = 0.0085 and 0.0022) and might be potential biomarkers (121, 122). TG4010 is a modified vaccinia Ankara encoding MUC1 and human IL-2. Studies showed that clinical benefits of TG4010 was associated with anti-MUC1 T cell responses. And anti-MUC1 responses contributed to epitope spreading against other tumor associated antigens (123). Although there was no statistical difference in health-related quality of life between TG4010 plus chemotherapy group and chemotherapy group in (NCT00415818), TIME study (NCT01383148) showed that TG4010 and chemotherapy group (5.9 months, 95% CI 5.4–6.7) had a prolonged PFS compared with placebo and chemotherapy group (5.1 months, 95% CI 4.2–5.9) (HR=0.74 95% CI 0.55–0.98; one-sided p=0.019) (124, 125). Gatipotuzumab is an anti-tumor-associated epitope of mucin-1 monoclonal antibody and tomuzotuximab is an anti-EGFR antibody. The combination of these two drugs has been tested in the GATTO study (126). A MUC1-targeted dendritic-cell-based vaccine exhibited anti-tumor activity and clinical benefits for patients with MUC1-positive refractory NSCLC. The median survival time and 1-year survival rate for patients who received more than six vaccinations was 9.5 months and 39.3%, while those of patients received initial vaccination was 7.4 months and 25.0%, respectively. Immune-related adverse events occurrence (12.6 months versus 6.7 months, p=0.042) and peripheral lymphocytes count/white blood cells count > 20.0% (12.6 months versus 4.5 months, p=0.014) were associated with longer survival time and might be predictive biomarkers for better clinical benefits (127).
Other molecules
High levels of CD31+ circulating tumor endothelial cells are associated with poor prognosis in anti-angiogenic therapy and may be a predictive factor (128, 129). Downregulation of CD44 is related to inhibition of wild−type EGFR signals, which leads to of cell proliferation inhibition and increased cisplatin sensitivity (130, 131). CD44 overexpression is also associated with sensitivity to PD-1 axis blockade and may be a novel auxiliary biomarker (132). These findings indicate that CD44 blockade may facilitate anti-PD-1 therapy and cisplatin treatment efficacy. The levels of serum VCAM-1 are significantly higher in NSCLC patients, which be a potential auxiliary biomarker (133). High baseline serum levels of VCAM-1 are related to better prognosis in NSCLC patients treated by second line nivolumab and may also be a potential biomarker for anti-PD-1 therapy (134).
Combined therapy
For advanced NSCLC, one therapy alone may be not enough to reach satisfactory therapeutic effect. Therefore, the combination of immune checkpoint blockers and other therapies may improve treatment efficacy and has been carried out by multiple studies (135, 136). The combination of radiotherapy and anti-CTLA-4 therapy activates T cells with TCR against tumor cells repertoires and the combination of immune checkpoint inhibitor and radiation/chemotherapy has been clinically applied in unresectable stage III NSCLC (18, 137). Studies showed that the combination of nivolumab and ipilimumab (dual checkpoint inhibition) significantly improved OS and PFS in NSCLC and especially effective for high mutational burden tumors and PD-L1 expression <1% (138–141). Although nivolumab plus ipilimumab seemed to outcompete other ICI therapies in OS for patients with PD-L1 expression <1%, the risk of Grade 3 or higher treatment-related adverse effects was also significantly increased compared with nivolumab alone (OR=5.80; 95%CI, 1.60-21.0). Therefore, the clinical benefit and tolerance of patient should be evaluated before dual checkpoint inhibition administration and more studies are needed (142). A case report showed that cryotherapy is a feasible treatment for ICI resistant metastasis (143). The combination of nivolumab and ipilimumab (dual immune blockage) may be more efficient than nivolumab or ipilimumab alone (144). The combination of low-dose apatinib (VEGFR2-TKI) and anti–PD-1 also improved treatment efficacy in NSCLC mouse model and patients (145). Evodiamine inhibits the MUC1-C/PD-L1 axis and enhances CD8+ T cells function, which may be combined with ICIs in NSCLC to enhance treatment efficacy (146). A network meta-analysis of 7155 NSCLC patients showed that the combination of chemotherapy plus PD-L1 inhibitors plus dexamethasone pretreatment may be a candidate for the first-line treatment of NSCLC patients (147). Combined therapy is also a potential method to overcome ICI resistance. The combination of oncolytic viruses and ICIs showed to overcome ICI resistance in a syngeneic mouse model and a case report (148, 149). T-cell immunoglobulin domain and mucin domain-3 (TIM-3) is an inhibitory receptor associated with PD-1 and may also participate in anti-PD-1 drug resistance. A study showed that high TIM-3 level on tumor infiltrating lymphocytes was related to poor prognosis in NSCLC (150). Therefore, the dual blockade of PD-1 and TIM-3 may overcome anti-PD-1 drug resistance. A clinical trial of the combination of sabatolimab (anti-TIM-3 antibody) and spartalizumab (anti-PD-1 antibody) has also been carried out and showed some signs of anti-tumor immunity (151).
Chimeric antigen receptor-T cell (CAR-T) expresses a modified protein containing three regions: extracellular region which can specifically bind to tumor cells, transmembrane region and intracellular region which can induce stimulatory signal transduction. This unique receptor leads to the robust anti-tumor effect (152). The combination of checkpoint inhibitors and CAR-T cell therapy may further activate anti-tumor immunity and are being applied in many clinical trials (153). However, CAR-T cell therapy has limited efficacy for most solid tumors. For NSCLC, it has following shortcomings: toxicity induced by CAR-T cell; lack of adequate tumor-specific antigens as targets and poor anti-tumor effect (154). EGFR and VEGFR2 may be potential target antigens for CAR-T cell therapy against NSCLC (155). CAR-T cells with constitutively anti-PD-1 secretion also showed better anti-tumor activity in mouse model (20).
The objective of tumor vaccine is to promote an activate adaptive anti-tumor immune response (156). However, outcomes of NSCLC vaccine clinical trials were disappointing. NSCLC vaccines should overcome the inhibition of tumor microenvironment, negative checkpoint signals and the low availability of effector T cells to tumor sites (157). Neoantigens is a class of tumor-specific antigens caused by non-synonymous mutations of tumor cells. Unlike tumor-associated antigen, it is not expressed in normal cells and induces stronger immune reactions, which may be a promising peptide target for individualized tumor vaccine (158, 159). The combination of ICIs and tumor vaccine may enhance treatment efficacy and has been used in clinical trials (156).
Not all combined therapies are adequate. The combination of immunotherapy (except immune checkpoint inhibitors) and conventional treatment didn’t improve prognosis and might increase incidence of adverse events (15). Several clinical trials also failed to show a clinical benefit of PD-1/PD-L1 monoclonal antibodies in patients with EGFR-mutant NSCLC (160). These studies indicate that combined therapy should be used carefully in order to reach a balance between clinical benefit and adverse events.
Adverse effects and drug resistance in immunotherapy
Adverse effects
Adverse effects also happen in anti-PD-1/PD-L1 immunotherapy. Interestingly, according to a small sample study, the incidence rate of irAEs was significantly higher in responders than non-responders (65.2% vs. 19.3%, P < 0.01), indicating that irAEs may be related to better therapeutic effect (161). Whether anti-PD-1 or anti-PD-L1 antibodies has less adverse events remains controversial and studies showed contradictory outcomes (17, 162–164) A study showed that HLADRB1*11:01 and pruritus as well as a nominally significant additive association between HLA-DQB1*03:01 and colitis, indicating the role of genetic diversity in adverse events of immune checkpoint inhibitors (165). Cardiovascular toxicity is a rare but serious adverse event of immune checkpoint inhibitors treatment, including myocarditis, pericarditis, heart failure, acute myocardial infarction, etc (166). Pneumonitis is also a common adverse effect in anti-PD-1/anti-PD-L1 treatment for NSCLC patients with high mortality and poor treatment (94, 167, 168). Interstitial lung disease is a rare (1–5%) but severe adverse effect (50–60% mortality rate). A study indicated that performance status≥ 2 and ≥ 50 pack-year were independent risk factors of ICI-induced interstitial lung disease of grade≥ 3 and all grades (169). Different therapy showed different distribution of irAEs. Atezolizumab plus platinum may be related to a higher incidence rate of colitis, while pembrolizumab may be related to a higher incidence of pneumonitis and hepatitis (170). Irrational drug use also increases the frequency of adverse effects. A study showed that the use of osimertinib immediately after nivolumab is associated with higher incidence of grade 3 or higher hepatotoxicity in patients with advanced NSCLC harboring EGFR mutation acquired T790M resistance (171). Sintilimab monotherapy showed a higher incidence of fatal adverse effects (0 to 2.5%) than average (172). Lower dose and frequency of ipilimumab seems to improve the safety of combined therapy (139). Hyperprogression is defined as an unexpected acceleration of cancer growth after immunotherapy and is a severe adverse effect with bad prognosis. NSCLC has a relatively high incidence rate of hyperprogression (14%) and no biomarker has been identified (173). High level of IL-10 and low pretreatment neutrophil-to-lymphocyte ratio may be predictive factors for irAEs monitor (174, 175).
Drug resistance
Primary or secondary drug resistance has been observed in NSCLC anti-PD-1/PD-L1 therapy. ORRs are no more than 20–30% and no biomarker (except PD-L1) has been identified. Underlying mechanism may include tumor neoantigen loss, impaired IFN-γ signaling, upregulation of other immune checkpoint receptors, tumor microenvironment, epigenetic modulation, etc (176). Secretive PD-L1 may also be one of the resistance mechanisms by combining with anti-PD-L1 antibody (177). An autopsy of two immunotherapy failure patients also showed a sharply decrease of PD-L1 expression after immunotherapy (178). Acquired EGFR exon 21 L858R has been observed in a nivolumab resistant patient, which may also be a possible mechanism of secondary ICI resistance (179) (Figure 2).
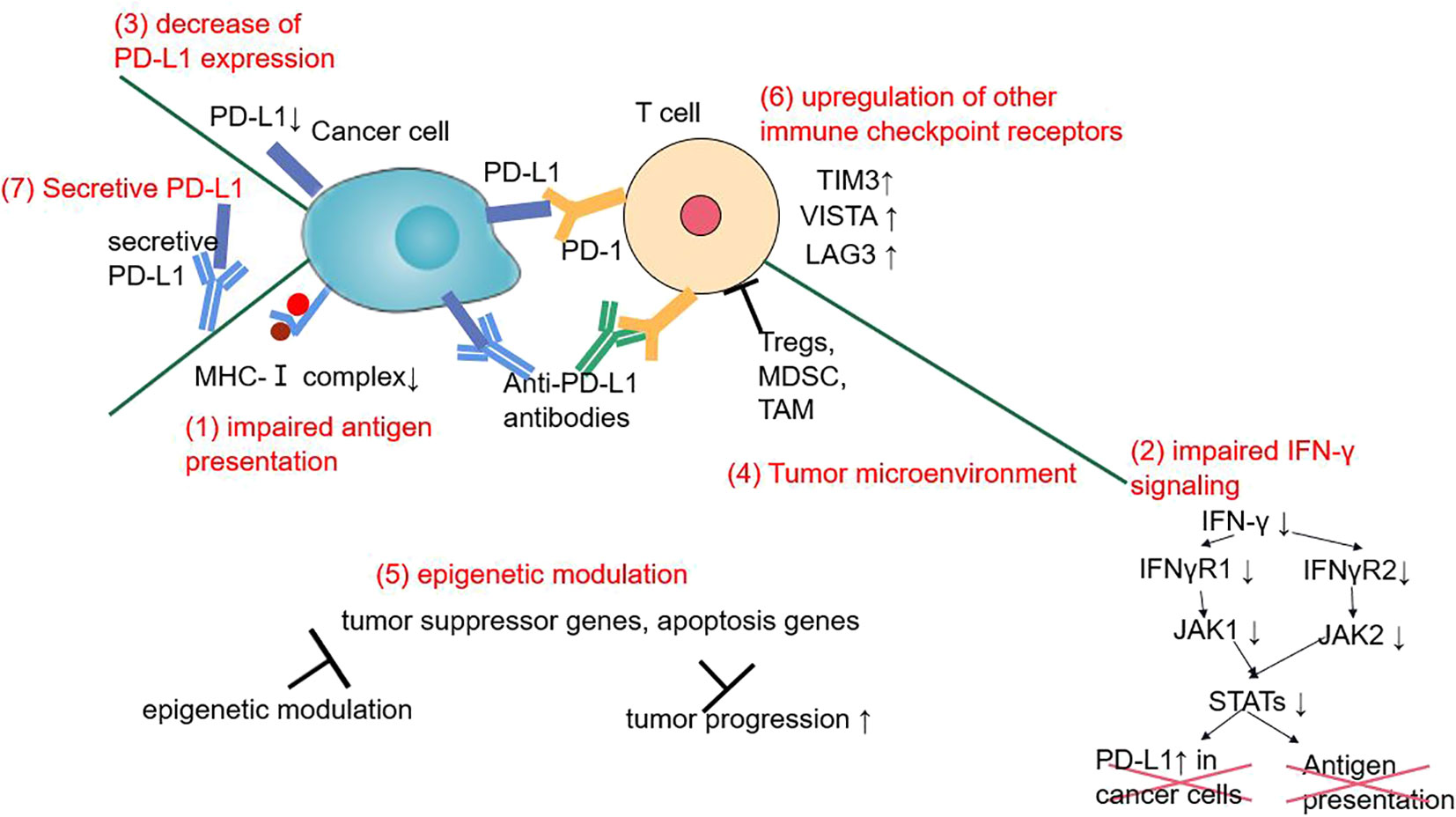
Figure 2 The mechanisms of primary or secondary ICI resistance in NSCLC during anti-PD-1/PD-L1 therapy.
There are some characteristics in anti-PD-1/PD-L1 resistance and may be future biomarkers to predict treatment efficacy. Tumor Mutation Burden (TMB) and EGFR/ALK mutations are two promising biomarkers (180, 181). Several studies have showed that patients with high tissue TMB have a higher ORR with immune checkpoint inhibitors than those with low tissue TMB (182, 183). EGFR-mutated NSCLC, on the other hand, is related to lower PD-L1 expression, low TMB, and increased risk of hyperprogression and pulmonary toxicity (184). ALK-/EGFR-positive tumors are negative prognostic factors in immunotherapy and immunosuppressive microenvironment has also been observed in ALK/EGFR-positive tumors (185). Whether KRAS is a good prognostic factor in ICI therapy remains controversial (186). Low blood serum amyloid A may also indicate better survival outcomes for pembrolizumab in advanced NSCLC treatment (187). High levels of serum hypoxanthine and histidine may be associated with better anti-PD-1 response (188). Activation of Hedgehog pathway, increasing of plasma Wnt1 protein, TGFBR2 mutation and CDKN2A loss−of−function are also associated with ICIs resistance in NSCLC (189–191). A study showed that high level of circulating monocytic myeloid-derived suppressive-like cells was related to primary resistance to immunotherapy (192). Interestingly, smoking patients with NSCLC seemed to have better responses to anti-PD-1/PD-L1, anti-CTLA-4, and anti-MUC1 drugs compared with non-smokers, which might affect therapy selection for smokers with NSCLC (193).
Prospect
Aberrant cell adhesion molecule expression plays a crucial role in tumor microenvironment formation and tumor immune evasion which can be utilized in immunotherapy. Upregulation of PD-1/PD-L1/CTLA-4 leads to tumor immunosuppression in NSCLC. Downregulation of E-cadherin facilitates tumor metastasis and LFA-1 plays a complex role in tumor progression. MUC1 upregulation affects various signaling pathways to enhance the proliferation, migration and invasion ability of NSCLC. Currently, ICIs has made great progress while other immunotherapies showed disappointing efficacy in NSCLC. Primary or secondary drug resistance and irAEs are still major barriers for ICIs.
For immune checkpoint inhibitors, the expression level of target molecules is important for drug selection. Novel laboratory technologies such as On‐chip Sort (a microfluidic chip cell sorter) can separate and analyze circulating cancer cells, which is convenient, non-invasive and more sensitive compared with biopsy (194). The delivery strategy of anti-tumor drugs is associated with treatment efficacy and adverse effects, and cell adhesion molecules may be a promising target (195). αVβ3 integrin overexpression in tumor is a sign of angiogenesis. Therefore, RGD peptides may be peptide-drug conjugate because it can selectively bind to cancer cells with αVβ3 and αVβ5 integrins highly expressed (196). The combination of immunotherapy and other treatments may improve therapeutic effect. However, combined therapy may also lead to increased adverse effect and should be used carefully on the basis of evidence-based medicine. Dual immune checkpoint inhibitors (the combination of anti-PD-1 and anti-CTLA-4 therapy) is considered as a promising therapy to enhance ICI efficacy and showed improved prognosis in melanoma and NSCLC (139, 197). CAR-T cell immunotherapy is mainly used in hematological tumors and not being clinically applied in NSCLC. Future CAR-T cell immunotherapy for NSCLC should be more specific in tumor antigens selection and TCR designation in order to robustly inducing anti-tumor effect and decreasing the incidence adverse effects (20, 198, 199). ICAM-1 specific CAR-T cell may also increase its efficacy and safety (200). The combination of ICI (exogenous or secretive) and CAR-T cell therapy may further activate anti-tumor immunity (20, 153).
Despite of great progression, NSCLC is still a deadly and highly heterogenous disease. One or two therapies can hardly control disease progression and drug resistance is easily developed. Therefore, individualized and combined therapy are further trends for NSCLC immunotherapy. To overcome the drawbacks in immunotherapy, understanding the mechanisms of cell adhesion molecule interaction; new biomarkers for diagnosis, monitor and follow-up; novel technologies and therapies; and accumulation of clinical experience are necessary for a wider application of NSCLC immunotherapy.
Author contributions
HY, YM designed and conceptualized the review. ZY, MW and XJ provided precious advice and corrections. All authors contributed to the article and approved the submitted version.
Funding
This work was supported by the NSFC joint fund for regional innovation and development (No. U20A20413).
Conflict of interest
Author XJ was employed by Shenyang Kangwei Medical Laboratory Analysis Co. LTD.
The remaining authors declare that the research was conducted in the absence of any commercial or financial relationships that could be construed as a potential conflict of interest.
Publisher’s note
All claims expressed in this article are solely those of the authors and do not necessarily represent those of their affiliated organizations, or those of the publisher, the editors and the reviewers. Any product that may be evaluated in this article, or claim that may be made by its manufacturer, is not guaranteed or endorsed by the publisher.
References
1. Mattiuzzi C, Lippi G. Current cancer epidemiology. J Epidemiol Glob Health (2019) 9:217–22. doi: 10.2991/jegh.k.191008.001
2. Bray F, Ferlay J, Soerjomataram I, Siegel RL, Torre LA, Jemal A. Global cancer statistics 2018: GLOBOCAN estimates of incidence and mortality worldwide for 36 cancers in 185 countries. CA Cancer J Clin (2018) 68:394–424. doi: 10.3322/caac.21492
3. West R. Tobacco smoking: Health impact, prevalence, correlates and interventions. Psychol Health (2017) 32:1018–36. doi: 10.1080/08870446.2017.1325890
4. Inamura K. Lung cancer: Understanding its molecular pathology and the 2015 wHO classification. Front Oncol (2017) 7:193. doi: 10.3389/fonc.2017.00193
5. Cheema PK, Rothenstein J, Melosky B, Brade A, Hirsh V. Perspectives on treatment advances for stage III locally advanced unresectable non-Small-Cell lung cancer. Curr Oncol (2019) 26:37–42. doi: 10.3747/co.25.4096
6. Majeed U, Manochakian R, Zhao Y, Lou Y. Targeted therapy in advanced non-small cell lung cancer: Current advances and future trends. J Hematol Oncol (2021) 14. doi: 10.1186/s13045-021-01121-2
7. Westover D, Zugazagoitia J, Cho BC, Lovly CM, Paz-Ares L. Mechanisms of acquired resistance to first-and second-generation EGFR tyrosine kinase inhibitors. Ann Oncol (2018) 29:i10–9. doi: 10.1093/annonc/mdx703
8. Wu SG, Shih JY. Management of acquired resistance to EGFR TKI-targeted therapy in advanced non-small cell lung cancer. Mol Cancer (2018) 17. doi: 10.1186/s12943-018-0777-1
9. Schreiber RD, Old LJ, Smyth MJ. Cancer immunoediting: Integrating immunity’s roles in cancer suppression and promotion Science (2011). 331(6024):1565-70.
10. Anichini A, Perotti VE, Sgambelluri F, Mortarini R. Immune escape mechanisms in non small cell lung cancer. Cancers (Basel) (2020) 12:1–20. doi: 10.3390/cancers12123605
11. Harjunpää H, Asens ML, Guenther C, Fagerholm SC. Cell adhesion molecules and their roles and regulation in the immune and tumor microenvironment. Front Immunol (2019) 10:1078. doi: 10.3389/fimmu.2019.01078
12. Walling BL, Kim M. LFA-1 in T cell migration and differentiation. Front Immunol (2018) 9:952. doi: 10.3389/fimmu.2018.00952
13. Chao D, Hu G, Li Q. Clinicopathological significance and prognostic value of e-cadherin expression in non-small cell lung cancer: A protocol for systematic review and meta-analysis. Medicine (2021) 100:e24748. doi: 10.1097/MD.0000000000024748
14. Xia L, Liu Y, Wang Y. PD-1/PD-L1 blockade therapy in advanced non-Small-Cell lung cancer: Current status and future directions. Oncologist (2019) 24:S31–41. doi: 10.1634/theoncologist.2019-io-s1-s05
15. Zhu J, Li R, Tiselius E, Roudi R, Teghararian O, Suo C, et al. Immunotherapy (excluding checkpoint inhibitors) for stage I to III non-small cell lung cancer treated with surgery or radiotherapy with curative intent. Cochrane Database Sys. Rev (2017) 2017. doi: 10.1002/14651858.CD011300.pub2
16. Błach J, Wojas-Krawczyk K, Nicoś M, Krawczyk P. Failure of immunotherapy–the molecular and immunological origin of immunotherapy resistance in lung cancer. Int J Mol Sci (2021) 22. doi: 10.3390/ijms22169030
17. Wang Y, Zhou S, Yang F, Qi X, Wang X, Guan X, et al. Treatment-related adverse events of PD-1 and PD-L1 inhibitors in clinical trials: A systematic review and meta-analysis. JAMA Oncol (2019) 5:1008–19. doi: 10.1001/jamaoncol.2019.0393
18. Breen WG, Leventakos K, Dong H, Merrell KW. Radiation and immunotherapy: Emerging mechanisms of synergy. J Thorac Dis (2020) 12:7011–23. doi: 10.21037/jtd-2019-cptn-07
19. Santini FC, Rizvi H, Plodkowski AJ, Ni A, Lacouture ME, Gambarin-Gelwan M, et al. Safety and efficacy of re-treating with immunotherapy after immune-related adverse events in patients with NSCLC. Cancer Immunol Res (2018) 6:1093–9. doi: 10.1158/2326-6066.CIR-17-0755
20. Zhang L, Li H, Zhang F, Wang S, Li G. Car-t immunotherapy and non-small cell lung cancer: Bottleneck and dawn. Chin J Lung Cancer (2020) 23:916–20. doi: 10.3779/j.issn.1009-3419.2020.103.10
21. Villanueva N, Bazhenova L. New strategies in immunotherapy for lung cancer: Beyond PD-1/PD-L1. Ther Adv Respir Dis (2018) 12:1–29. doi: 10.1177/1753466618794133
22. Paolillo M, Schinelli S. Extracellular matrix alterations in metastatic processes. Int J Mol Sci (2019) 20. doi: 10.3390/ijms20194947
23. Palomba R, Parodi A, Evangelopoulos M, Acciardo S, Corbo C, de Rosa E, et al. Biomimetic carriers mimicking leukocyte plasma membrane to increase tumor vasculature permeability. Sci Rep (2016) 6. doi: 10.1038/srep34422
24. Rowshanravan B, Halliday N, Sansom DM. CTLA-4: A moving target in immunotherapy. Blood (2018) 131:58–67. doi: 10.1182/blood-2017-06-741033
25. Öjlert ÅK, Halvorsen AR, Nebdal D, Lund-Iversen M, Solberg S, Brustugun OT, et al. The immune microenvironment in non-small cell lung cancer is predictive of prognosis after surgery. Mol Oncol (2019) 13:1166–79. doi: 10.1002/1878-0261.12475
26. Reina M, Espel E. Role of LFA-1 and ICAM-1 in cancer. Cancers (Basel) (2017) 9. doi: 10.3390/cancers9110153
27. Xiao X, Murk DD, Cheng CY. Intercellular adhesion molecules (ICAMs) and spermatogenesis. Hum Reprod Update (2013) 19:167–86. doi: 10.1093/humupd/dms049
28. Benard E, Nunès JA, Limozin L, Sengupta K. T Cells on engineered substrates: The impact of TCR clustering is enhanced by LFA-1 engagement. Front Immunol (2018) 9:2085. doi: 10.3389/fimmu.2018.02085
29. Siokis A, Robert PA, Demetriou P, Dustin ML, Meyer-Hermann M. F-Actin-Driven CD28-CD80 localization in the immune synapse. Cell Rep (2018) 24:1151–62. doi: 10.1016/j.celrep.2018.06.114
30. Roy NH, Kim SHJ, Buffone A, Blumenthal D, Huang B, Agarwal S, et al. LFA-1 signals to promote actin polymerization and upstream migration in T cells. J Cell Sci (2020) 133. doi: 10.1242/jcs.248328
31. Roy NH, MacKay JL, Robertson TF, Hammer DA, Burkhardt JK. Crk adaptor proteins mediate actin-dependent T cell migration and mechanosensing induced by the integrin LFA-1. Sci Signal (2018) 11. doi: 10.1126/scisignal.aat3178
32. Verma NK, Kelleher D. Not just an adhesion molecule: LFA-1 contact tunes the T lymphocyte program. J Immunol (2017) 199:1213–21. doi: 10.4049/jimmunol.1700495
33. Sharma A, Lawry SM, Klein BS, Wang X, Sherer NM, Zumwalde NA, et al. LFA-1 ligation by high-density ICAM-1 is sufficient to activate IFN-γ release by innate T lymphocytes. J Immunol (2018) 201:2452–61. doi: 10.4049/jimmunol.1800537
34. Swaim CD, Scott AF, Canadeo LA, Huibregtse JM. Extracellular ISG15 signals cytokine secretion through the LFA-1 integrin receptor. Mol Cell (2017) 68:581–590.e5. doi: 10.1016/j.molcel.2017.10.003
35. Garçon F, Okkenhaug K. PI3Kδ promotes CD4+ T-cell interactions with antigen-presenting cells by increasing LFA-1 binding to ICAM-1. Immunol Cell Biol (2016) 94:486–95. doi: 10.1038/icb.2016.1
36. Zhao S, Gu Z, Wang L, Guan L, Wang F, Yang N, et al. G-CSF inhibits LFA-1-mediated CD4 + T cell functions by inhibiting lck and ZAP-70. Oncotarget (2017) 8:51578–90. doi: 10.18632/oncotarget.18194
37. Capece T, Walling BL, Lim K, Kim KD, Bae S, Chung HL, et al. A novel intracellular pool of LFA-1 is critical for asymmetric CD8+ T cell activation and differentiation. J Cell Biol (2017) 216:3817–29. doi: 10.1083/jcb.201609072
38. Feigelson SW, Solomon A, Biram A, Hatzav M, Lichtenstein M, Regev O, et al. ICAMs are not obligatory for functional immune synapses between naive CD4 T cells and lymph node DCs. Cell Rep (2018) 22:849–59. doi: 10.1016/j.celrep.2017.12.103
39. Verma NK, Fazil MHUT, Ong ST, Chalasani MLS, Low JH, Kottaiswamy A, et al. LFA-1/ICAM-1 ligation in human T cells promotes Th1 polarization through a GSK3β signaling–dependent notch pathway. J Immunol (2016) 197:108–18. doi: 10.4049/jimmunol.1501264
40. Meli AP, Fontés G, Avery DT, Leddon SA, Tam M, Elliot M, et al. The integrin LFA-1 controls T follicular helper cell generation and maintenance. Immunity (2016) 45:831–46. doi: 10.1016/j.immuni.2016.09.018
41. Janssen E, Tohme M, Butts J, Giguere S, Sage PT, Velázquez FE, et al. DOCK8 is essential for LFA-1–dependent positioning of T follicular helper cells in germinal centers. JCI Insight (2020) 5. doi: 10.1172/jci.insight.134508
42. Zhou F, Chen J, Tao G, Zhu M, Xie W, Cao X. Increased levels of exhaled sICAM1, sVCAM1, and sE-selectin in patients with non-small cell lung cancer. Respir Med (2014) 108:1670–6. doi: 10.1016/j.rmed.2014.08.003
43. Gu X, Ma C, Yuan D, Song Y. Circulating soluble intercellular adhesion molecule-1 in lung cancer: A systematic review. Transl Lung Cancer Res (2012) 1:36–44. doi: 10.3978/j.issn.2218-6751.08.01
44. Petit AE, Demotte N, Scheid B, Wildmann C, Bigirimana R, Gordon-Alonso M, et al. A major secretory defect of tumour-infiltrating T lymphocytes due to galectin impairing LFA-1-mediated synapse completion. Nat Commun (2016) 7. doi: 10.1038/ncomms12242
45. Shen W, Zhang X, Du R, Fan Y, Luo D, Bao Y, et al. ICAM3 mediates tumor metastasis via a LFA-1-ICAM3-ERM dependent manner. Biochim Biophys Acta Mol Basis Dis (2018) 1864:2566–78. doi: 10.1016/j.bbadis.2018.05.002
46. Yanguas A, Garasa S, Teijeira Á, Aubá C, Melero I, Rouzaut A. ICAM-1-LFA-1 dependent CD8+ T-lymphocyte aggregation in tumor tissue prevents recirculation to draining lymph nodes. Front Immunol (2018) 9:2084. doi: 10.3389/fimmu.2018.02084
47. Wang K, Jiang L, Hu A, Sun C, Zhou L, Huang Y, et al. Vertebral-specific activation of the CX3CL1/ICAM-1 signaling network mediates non-small-cell lung cancer spinal metastasis by engaging tumor cell-vertebral bone marrow endothelial cell interactions. Theranostics (2021) 11:4770–89. doi: 10.7150/thno.54235
48. Basingab FS, Ahmadi M, Morgan DJ. IFNg-dependent interactions between ICAM-1 and LFA-1 counteract prostaglandin E2-mediated inhibition of antitumor CTL responses. Cancer Immunol Res (2016) 4:400–11. doi: 10.1158/2326-6066.CIR-15-0146
49. Kantari-Mimoun C, Barrin S, Vimeux L, Haghiri S, Gervais C, Joaquina S, et al. CAR T-cell entry into tumor islets is a two-step process dependent on IFNg and ICAM-1. Cancer Immunol Res (2021) 9:1425–38. doi: 10.1158/2326-6066.CIR-20-0837
50. Browska M, Grubek-Jaworska H, Hoser G, Domagal [ A-Kulawik J, Krenke R, Chazan R. Effect of IFN-stimulation on expression of intercellular adhesion molecule-1 (ICAM-1) on alveolar macrophages in patients with non-small cell lung cancer. J Interferon Cytokine Res (2006), 26(3):190–5. doi: 10.1089/jir.2006.26.190
51. Haustein M, Ramer R, Linnebacher M, Manda K, Hinz B. Cannabinoids increase lung cancer cell lysis by lymphokine-activated killer cells via upregulation of ICAM-1. Biochem Pharmacol (2014) 92:312–25. doi: 10.1016/j.bcp.2014.07.014
52. Gkogkou P, Peponi E, Ntaskagiannis D, Demou A, Ioakeim E, Evangelos B, et al. ICAM-1 expression in patients with advanced non-small cell lung cancer treated with radiotherapy. JBUON (2020) 25:1779–83.
53. Sui X, Jiang L, Teng H, Mi L, Li B, Shi A, et al. Prediction of clinical outcome in locally advanced non-small cell lung cancer patients treated with chemoradiotherapy by plasma markers. Front Oncol (2021) 10:625911. doi: 10.3389/fonc.2020.625911
54. Chen D, Menon H, Verma V, Guo C, Ramapriyan R, Barsoumian H, et al. Response and outcomes after anti-CTLA4 versus anti-PD1 combined with stereotactic body radiation therapy for metastatic non-small cell lung cancer: Retrospective analysis of two single-institution prospective trials. J Immunother Cancer (2020) 8. doi: 10.1136/jitc-2019-000492
55. Zhang H, Shen J, Yi L, Zhang W, Luo P, Zhang J. Efficacy and safety of ipilimumab plus chemotherapy for advanced lung cancer: A systematic review and meta-analysis. J Cancer (2018) 9:4556–67. doi: 10.7150/jca.27368
56. Sanmamed MF, Chen L. A paradigm shift in cancer immunotherapy: From enhancement to normalization. Cell (2018) 175:313–26. doi: 10.1016/j.cell.2018.09.035
57. Zak KM, Grudnik P, Magiera K, Dömling A, Dubin G, Holak TA. Structural biology of the immune checkpoint receptor PD-1 and its ligands PD-L1/PD-L2. Structure (2017) 25:1163–74. doi: 10.1016/j.str.2017.06.011
58. Boussiotis VA. Molecular and biochemical aspects of the PD-1 checkpoint pathway. New Engl J Med (2016) 375:1767–78. doi: 10.1056/nejmra1514296
59. Brown H, Vansteenkiste J, Nakagawa K, Cobo M, John T, Barker C, et al. Programmed cell death ligand 1 expression in untreated EGFR mutated advanced NSCLC and response to osimertinib versus comparator in FLAURA. J Thorac Oncol (2020) 15:138–43. doi: 10.1016/j.jtho.2019.09.009
60. Masuda K, Horinouchi H, Tanaka M, Higashiyama R, Shinno Y, Sato J, et al. Efficacy of anti-PD-1 antibodies in NSCLC patients with an EGFR mutation and high PD-L1 expression. J Cancer Res Clin Oncol (2021) 147:245–51. doi: 10.1007/s00432-020-03329-0
61. Auliac JB, Guisier F, Bizieux A, Assouline P, Bernardini M, Lamy R, et al. Impact of programmed death ligand 1 expression in advanced non-small-cell lung cancer patients, treated by chemotherapy (GFPC 06- 2015 study). Onco. Targets Ther (2020) 13:13299–305. doi: 10.2147/OTT.S288825
62. Cao ZQ, Wang Z, Leng P. Aberrant n-cadherin expression in cancer. Biomed. Pharmacother. (2019) 118. doi: 10.1016/j.biopha.2019.109320
63. Mendonsa AM, Na TY, Gumbiner BM. E-cadherin in contact inhibition and cancer. Oncogene (2018) 37:4769–80. doi: 10.1038/s41388-018-0304-2
64. Corgnac S, Boutet M, Kfoury M, Naltet C, Mami-Chouaib F. The emerging role of CD8+ tissue resident memory T (TRM) cells in antitumor immunity: A unique functional contribution of the CD103 integrin. Front Immunol (2018) 9:1904. doi: 10.3389/fimmu.2018.01904
65. Liu XF, Hao JL, Xie T, Pant OP, Lu CB, Lu CW, et al. The BRAF activated non-coding RNA: A pivotal long non-coding RNA in human malignancies. Cell Prolif (2018) 51. doi: 10.1111/cpr.12449
66. Sun Z, Liu G, Xu N. Does hypermethylation of CpG island in the promoter region of the e-cadherin gene increase the risk of lung cancer? a meta-analysis. Thorac Cancer (2019) 10:54–9. doi: 10.1111/1759-7714.12900
67. Yang R, Liu Y, Wang Y, Wang X, Ci H, Song C, et al. Low PRRX1 expression and high ZEB1 expression are significantly correlated with epithelial-mesenchymal transition and tumor angiogenesis in non-small cell lung cancer. Med (United States) (2021) 100. doi: 10.1097/MD.0000000000024472
68. Bastian K, Scott E, Elliott DJ, Munkley J. Fut8 alpha-(1,6)-fucosyltransferase in cancer. Int J Mol Sci (2021) 22:1–22. doi: 10.3390/ijms22010455
69. Saltos A, Khalil F, Smith M, Li J, Schell M, Antonia SJ, et al. Clinical associations of mucin 1 in human lung cancer and precancerous lesions. Oncotarget (2018), 9(86):35666–75. doi: 10.18632/oncotarget.26278
70. Gao J, Mcconnell MJ, Yu B, Li J, Balko JM, Black EP, et al. MUC1 is a downstream target of STAT3 and regulates lung cancer cell survival and invasion. Int J Oncol 35(2):337–45.
71. Alam M, Ahmad R, Rajabi H, Kufe D. MUC1-c induces the LIN28B!LET-7!HMGA2 axis to regulate self-renewal in NSCLC. Mol Cancer Res (2015) 13:449–60. doi: 10.1158/1541-7786.MCR-14-0363
72. Bouillez A, Rajabi H, Pitroda S, Jin C, Alam M, Kharbanda A, et al. Inhibition of MUC1-c suppresses MYC expression and attenuates malignant growth in KRAS mutant lung adenocarcinomas. Cancer Res (2016) 76:1538–48. doi: 10.1158/0008-5472.CAN-15-1804
73. Kharbanda A, Rajabi H, Jin C, Alam M, Wong K-K, Kufe D. MUC1-c confers EMT and KRAS independence in mutant KRAS lung cancer cells. Oncotarget (2014) 5(19):8893–905. doi: 10.18632/oncotarget.2360
74. Kharbanda A, Rajabi H, Jin C, Tchaicha J, Kikuchi E, Wong KK, et al. Targeting the oncogenic MUC1-c protein inhibits mutant EGFR-mediated signaling and survival in non-small cell lung cancer cells. Clin Cancer Res (2014) 20:5423–34. doi: 10.1158/1078-0432.CCR-13-3168
75. Xu H, Gao H, Li H, Li D, Yuan W, Zhang L, et al. Downregulated mucin 1 alleviates paclitaxel resistance in non-small cell lung cancer cells. Mol Med Rep (2020) 22:2966–72. doi: 10.3892/mmr.2020.11349
76. Huang W-C, Chan M-L, Chen M-J, Tsai T-H, Chen Y-J. Modulation of macrophage polarization and lung cancer cell stemness by MUC1 and development of a related small-molecule inhibitor pterostilbene. Oncotarget (2016) 7(26):39363–75. doi: 10.18632/oncotarget.8101
77. Xu T, Li D, Wang H, Zheng T, Wang G, Xin Y. MUC1 downregulation inhibits non-small cell lung cancer progression in human cell lines. Exp Ther Med (2017) 14:4443–7. doi: 10.3892/etm.2017.5062
78. de Boer HR, Pool M, Joosten E, Everts M, Samplonius DF, Helfrich W, et al. Quantitative proteomics analysis identifies MUC1 as an effect sensor of EGFR inhibition. Oncogene (2019) 38:1477–88. doi: 10.1038/s41388-018-0522-7
79. Li G, Gao Y, Cui Y, Zhang T, Cui R, Jiang Y, et al. Overexpression of CD44 is associated with the occurrence and migration of non-small cell lung cancer. Mol Med Rep (2016) 14:3159–67. doi: 10.3892/mmr.2016.5636
80. Nagashima T, Oshima T, Hiroshima Y, Yokose T, Woo T, Rino Y, et al. Clinical significance of tumour CD44v and MIST1 expression in patients with non-small-cell lung cancer. Anticancer Res (2020) 40:6407–16. doi: 10.21873/anticanres.14662
81. Affy AM, Tate S, Durbin-Johnson B, Rocke DM, Konia T. Expression of CD44s and CD44v6 in lung cancer and their correlation with prognostic factors. Int J Biol Markers (2011) 26:50–7. doi: 10.5301/JBM.2011.6291
82. Kong T, Ahn R, Yang K, Zhu X, Fu Z, Morin G, et al. CD44 promotes PD-L1 expression and its tumor-intrinsic function in breast and lung cancers. Cancer Res (2020) 80:444–57. doi: 10.1158/0008-5472.CAN-19-1108
83. Ma Y, Li M, Si J, Xiong Y, Lu F, Zhang J, et al. Blockade of Notch3 inhibits the stem-like property and is associated with ALDH1A1 and CD44 via autophagy in non-small lung cancer. Int J Oncol (2016) 48:2349–58. doi: 10.3892/ijo.2016.3464
84. Su J, Wu S, Wu H, Li L, Guo T. CD44 is functionally crucial for driving lung cancer stem cells metastasis through wnt/β-catenin-FoxM1-Twist signaling. Mol Carcinog. (2016) 55:1962–73. doi: 10.1002/mc.22443
85. Hu B, Ma Y, Yang Y, Zhang L, Han H, Chen J. CD44 promotes cell proliferation in non-small cell lung cancer. Oncol Lett (2018) 15:5627–33. doi: 10.3892/ol.2018.8051
86. Guo JY, Chiu CH, Wang MJ, Li FA, Chen JY. Proteoglycan serglycin promotes non-small cell lung cancer cell migration through the interaction of its glycosaminoglycans with CD44. J BioMed Sci (2020) 27. doi: 10.1186/s12929-019-0600-3
87. Loreth D, Schuette M, Zinke J, Mohme M, Piffko A, Schneegans S, et al. Cd74 and cd44 expression on ctcs in cancer patients with brain metastasis. Int J Mol Sci (2021) 22. doi: 10.3390/ijms22136993
88. Young MJ, Chen YC, Wang SA, Chang HP, Yang WB, Lee CC, et al. Estradiol-mediated inhibition of Sp1 decreases miR-3194-5p expression to enhance CD44 expression during lung cancer progression. J BioMed Sci (2022) 29. doi: 10.1186/s12929-022-00787-1
89. Song JM, Im J, Nho RS, Han YH, Upadhyaya P, Kassie F. Hyaluronan-CD44/RHAMM interaction-dependent cell proliferation and survival in lung cancer cells. Mol Carcinog. (2019) 58:321–33. doi: 10.1002/mc.22930
90. Li L, Li D, Chen Y. MiRNA-26a blocks interleukin-2-mediated migration and proliferation of non-small cell lung cancer cells via vascular cell adhesion molecule-1. Transl Cancer Res (2020) 9:1768–78. doi: 10.21037/tcr.2020.02.36
91. Liao T, Chen W, Sun J, Zhang Y, Hu X, Yang S, et al. CXCR4 accelerates osteoclastogenesis induced by non-small cell lung carcinoma cells through self-potentiation and VCAM1 secretion. Cell Physiol Biochem (2018) 50:1100–12. doi: 10.1159/000494533
92. Socinski MA, Jotte RM, Cappuzzo F, Orlandi F, Stroyakovskiy D, Nogami N, et al. Atezolizumab for first-line treatment of metastatic nonsquamous NSCLC. New Engl J Med (2018) 378:2288–301. doi: 10.1056/nejmoa1716948
93. Rittmeyer A, Barlesi F, Waterkamp D, Park K, Ciardiello F, von Pawel J, et al. Atezolizumab versus docetaxel in patients with previously treated non-small-cell lung cancer (OAK): A phase 3, open-label, multicentre randomised controlled trial. Lancet (2017) 389:255–65. doi: 10.1016/S0140-6736(16)32517-X
94. Antonia SJ, Villegas A, Daniel D, Vicente D, Murakami S, Hui R, et al. Durvalumab after chemoradiotherapy in stage III non–Small-Cell lung cancer. New Engl J Med (2017) 377:1919–29. doi: 10.1056/nejmoa1709937
95. Nishio M, Barlesi F, West H, Ball S, Bordoni R, Cobo M, et al. Atezolizumab plus chemotherapy for first-line treatment of nonsquamous NSCLC: Results from the randomized phase 3 IMpower132 trial. J Thorac Oncol (2021) 16:653–64. doi: 10.1016/j.jtho.2020.11.025
96. Mazieres J, Rittmeyer A, Gadgeel S, Hida T, Gandara DR, Cortinovis DL, et al. Atezolizumab versus docetaxel in pretreated patients with NSCLC: Final results from the randomized phase 2 POPLAR and phase 3 OAK clinical trials. J Thorac Oncol (2021) 16:140–50. doi: 10.1016/j.jtho.2020.09.022
97. Sezer A, Kilickap S, Gümüş M, Bondarenko I, Özgüroğlu M, Gogishvili M, et al. Cemiplimab monotherapy for first-line treatment of advanced non-small-cell lung cancer with PD-L1 of at least 50%: a multicentre, open-label, global, phase 3, randomised, controlled trial. Lancet (2021) 397:592–604. doi: 10.1016/S0140-6736(21)00228-2
98. Langer CJ, Gadgeel SM, Borghaei H, Papadimitrakopoulou VA, Patnaik A, Powell SF, et al. Carboplatin and pemetrexed with or without pembrolizumab for advanced, non-squamous non-small-cell lung cancer: A randomised, phase 2 cohort of the open-label KEYNOTE-021 study. Lancet Oncol (2016) 17:1497–508. doi: 10.1016/S1470-2045(16)30498-3
99. Park K, Özgüroğlu M, Vansteenkiste J, Spigel D, Yang JCH, Ishii H, et al. Avelumab versus docetaxel in patients with platinum-treated advanced NSCLC: 2-year follow-up from the JAVELIN lung 200 phase 3 trial. J Thorac Oncol (2021) 16:1369–78. doi: 10.1016/j.jtho.2021.03.009
100. Barlesi F, Vansteenkiste J, Spigel D, Ishii H, Garassino M, de Marinis F, et al. Avelumab versus docetaxel in patients with platinum-treated advanced non-small-cell lung cancer (JAVELIN lung 200): An open-label, randomised, phase 3 study. Lancet Oncol (2018) 19:1468–79. doi: 10.1016/S1470-2045(18)30673-9
101. Gray JE, Villegas A, Daniel D, Vicente D, Murakami S, Hui R, et al. Three-year overall survival with durvalumab after chemoradiotherapy in stage III NSCLC–update from PACIFIC. J Thorac Oncol (2020) 15:288–93. doi: 10.1016/j.jtho.2019.10.002
102. Faivre-Finn C, Vicente D, Kurata T, Planchard D, Paz-Ares L, Vansteenkiste JF, et al. Four-year survival with durvalumab after chemoradiotherapy in stage III NSCLC–an update from the PACIFIC trial. J Thorac Oncol (2021) 16:860–7. doi: 10.1016/j.jtho.2020.12.015
103. Rizvi NA, Cho BC, Reinmuth N, Lee KH, Luft A, Ahn MJ, et al. Durvalumab with or without tremelimumab vs standard chemotherapy in first-line treatment of metastatic non-small cell lung cancer: The MYSTIC phase 3 randomized clinical trial. JAMA Oncol (2020) 6:661–74. doi: 10.1001/jamaoncol.2020.0237
104. Lu S, Wang J, Cheng Y, Mok T, Chang J, Zhang L, et al. Nivolumab versus docetaxel in a predominantly Chinese patient population with previously treated advanced non-small cell lung cancer: 2-year follow-up from a randomized, open-label, phase 3 study (CheckMate 078). Lung Cancer (2021) 152:7–14. doi: 10.1016/j.lungcan.2020.11.013
105. Brahmer J, Reckamp KL, Baas P, Crinò L, Eberhardt WEE, Poddubskaya E, et al. Nivolumab versus docetaxel in advanced squamous-cell non–Small-Cell lung cancer. New Engl J Med (2015) 373:123–35. doi: 10.1056/nejmoa1504627
106. Mazieres J, Kowalski D, Luft A, Vicente D, Tafreshi A, Laktionov K, et al. Health-related quality of life with carboplatin-paclitaxel or nab-paclitaxel with or without pembrolizumab in patients with metastatic squamous non-Small-Cell lung cancer. J Clin Oncol (2019) 37:271-280. doi: 10.1200/JCO.19
107. Paz-Ares L, Luft A, Vicente D, Tafreshi A, Gümüş M, Mazières J, et al. Pembrolizumab plus chemotherapy for squamous non–Small-Cell lung cancer. New Engl J Med (2018) 379:2040–51. doi: 10.1056/nejmoa1810865
108. Gandhi L, Rodríguez-Abreu D, Gadgeel S, Esteban E, Felip E, de Angelis F, et al. Pembrolizumab plus chemotherapy in metastatic non–Small-Cell lung cancer. New Engl J Med (2018) 378:2078–92. doi: 10.1056/nejmoa1801005
109. Horinouchi H, Nogami N, Saka H, Nishio M, Tokito T, Takahashi T, et al. Pembrolizumab plus pemetrexed-platinum for metastatic nonsquamous non–small-cell lung cancer: KEYNOTE-189 Japan study. Cancer Sci (2021) 112:3255–65. doi: 10.1111/cas.14980
110. Reck M, Rodríguez-Abreu D, Robinson AG, Hui R, Csőszi T, Fülöp A, et al. Pembrolizumab versus chemotherapy for PD-L1–positive non–Small-Cell lung cancer. New Engl J Med (2016) 375:1823–33. doi: 10.1056/nejmoa1606774
111. Li X, Li X, Han S, Ma A, Chen P, Wang X, et al. Economic evaluation of sintilimab plus chemotherapy vs. pembrolizumab plus chemotherapy for the treatment of first-line advanced or metastatic squamous NSCLC. Front Public Health (2022) 10:956792. doi: 10.3389/fpubh.2022.956792
112. Gong J, Su D, Shang J, Xu S, Tang L, Sun Z, et al. Cost-effectiveness of tislelizumab versus docetaxel for previously treated advanced non-Small-Cell lung cancer in China. Front Pharmacol (2022) 13:830380. doi: 10.3389/fphar.2022.830380
113. Yang Y, Wang Z, Fang J, Yu Q, Han B, Cang S, et al. Efficacy and safety of sintilimab plus pemetrexed and platinum as first-line treatment for locally advanced or metastatic nonsquamous NSCLC: A randomized, double-blind, phase 3 study (Oncology pRogram by InnovENT anti-PD-1-11). J Thorac Oncol (2020) 15:1636–46. doi: 10.1016/j.jtho.2020.07.014
114. Lu S, Wang J, Yu Y, Yu X, Hu Y, Ai X, et al. Tislelizumab plus chemotherapy as first-line treatment for locally advanced or metastatic nonsquamous NSCLC (RATIONALE 304): A randomized phase 3 trial. J Thorac Oncol (2021) 16:1512–22. doi: 10.1016/j.jtho.2021.05.005
115. Incorvaia L, Fanale D, Badalamenti G, Barraco N, Bono M, Corsini LR, et al. Programmed death ligand 1 (PD-L1) as a predictive biomarker for pembrolizumab therapy in patients with advanced non-Small-Cell lung cancer (NSCLC). Adv Ther (2019) 36(10):2600–17. doi: 10.6084/m9.figshare.9135527
116. Cooper WA, Russell PA, Cherian M, Duhig EE, Godbolt D, Jessup PJ, et al. Intra- and interobserver reproducibility assessment of PD-L1 biomarker in non–small cell lung cancer. Clin Cancer Res (2017) 23:4569–77. doi: 10.1158/1078-0432.CCR-17-0151
117. Vargas FA, Furness AJS, Litchfield K, Joshi K, Rosenthal R, Ghorani E, et al. Fc effector function contributes to the activity of human anti-CTLA-4 antibodies. Cancer Cell (2018) 33:649–663.e4. doi: 10.1016/j.ccell.2018.02.010
118. Ingram JR, Blomberg OS, Rashidian M, Ali L, Garforth S, Fedorov E, et al. Anti-CTLA-4 therapy requires an fc domain for efficacy. Proc Natl Acad Sci USA (2018) 115:3912–7. doi: 10.1073/pnas.1801524115
119. Schofield DJ, Percival-Alwyn J, Rytelewski M, Hood J, Rothstein R, Wetzel L, et al. Activity of murine surrogate antibodies for durvalumab and tremelimumab lacking effector function and the ability to deplete regulatory T cells in mouse models of cancer. MAbs (2021) 13. doi: 10.1080/19420862.2020.1857100
120. He M, Chai Y, Qi J, Zhang CWH, Tong Z, Shi Y, et al. Remarkably similar CTLA-4 binding properties of therapeutic ipilimumab and tremelimumab antibodies. Oncotarget (2017) 8(40):67129–39. doi: 10.18632/oncotarget.18004
121. Butts C, Socinski MA, Mitchell PL, Thatcher N, Havel L, Krzakowski M, et al. Tecemotide (L-BLP25) versus placebo after chemoradiotherapy for stage III non-small-cell lung cancer (START): A randomised, double-blind, phase 3 trial. Lancet Oncol (2014) 15:59–68. doi: 10.1016/S1470-2045(13)70510-2
122. Mitchell PL, Thatcher N, Socinski MA, Wasilewska-Tesluk E, Horwood K, Szczesna A, et al. Tecemotide in unresectable stage III non-small-cell lung cancer in the phase III START study: Updated overall survival and biomarker analyses. Ann Oncol (2015) 26:1134–42. doi: 10.1093/annonc/mdv104
123. Tosch C, Bastien B, Barraud L, Grellier B, Nourtier V, Gantzer M, et al. Viral based vaccine TG4010 induces broadening of specific immune response and improves outcome in advanced NSCLC. J Immunother Cancer (2017) 5. doi: 10.1186/s40425-017-0274-x
124. Rotonda C, Anota A, Mercier M, Bastien B, Lacoste G, Limacher JM, et al. Impact of TG4010 vaccine on health-related quality of life in advanced non-small-cell lung cancer: Results of a phase IIB clinical trial. PloS One (2015) 10. doi: 10.1371/journal.pone.0132568
125. Quoix E, Lena H, Losonczy G, Forget F, Chouaid C, Papai Z, et al. TG4010 immunotherapy and first-line chemotherapy for advanced non-small-cell lung cancer (TIME): Results from the phase 2b part of a randomised, double-blind, placebo-controlled, phase 2b/3 trial. Lancet Oncol (2016) 17:212–23. doi: 10.1016/S1470-2045(15)00483-0
126. Ochsenreither S, Fiedler WM, Conte GD, Macchini M, Matos I, Habel B, et al. Safety and preliminary activity results of the GATTO study, a phase ib study combining the anti-TA-MUC1 antibody gatipotuzumab with the anti-EGFR tomuzotuximab in patients with refractory solid tumors. ESMO Open (2022) 7. doi: 10.1016/j.esmoop.2022.100447
127. Teramoto K, Ozaki Y, Hanaoka J, Sawai S, Tezuka N, Fujino S, et al. Predictive biomarkers and effectiveness of MUC1-targeted dendritic-cell-based vaccine in patients with refractory non-small cell lung cancer. Ther Adv Med Oncol (2017) 9:147–57. doi: 10.1177/1758834016678375
128. Liu Z, Wang J, Meng Z, Wang X, Zhang C, Qin T, et al. CD31-labeled circulating endothelial cells as predictor in anlotinib-treated non-small-cell lung cancer: Analysis on ALTER-0303 study. Cancer Med (2018) 7:3011–21. doi: 10.1002/cam4.1584
129. Zhang T, Zhang L, Gao Y, Wang Y, Liu Y, Zhang H, et al. Role of aneuploid circulating tumor cells and CD31+ circulating tumor endothelial cells in predicting and monitoring anti-angiogenic therapy efficacy in advanced NSCLC. Mol Oncol (2021) 15:2891–909. doi: 10.1002/1878-0261.13092
130. Yin J, Zhang H, Wu X, Zhang Y, Li J, Shen J, et al. CD44 inhibition attenuates EGFR signaling and enhances cisplatin sensitivity in human EGFR wild-type non-small-cell lung cancer cells. Int J Mol Med (2020) 45:1783–92. doi: 10.3892/ijmm.2020.4562
131. Quan YH, Lim JY, Choi BH, Choi Y, Choi YH, Park JH, et al. Self-targeted knockdown of CD44 improves cisplatin sensitivity of chemoresistant non-small cell lung cancer cells. Cancer Chemother Pharmacol (2019) 83:399–410. doi: 10.1007/s00280-018-3737-y
132. Moutafi MK, Molero M, Martinez Morilla S, Baena J, Vathiotis IA, Gavrielatou N, et al. Spatially resolved proteomic profiling identifies tumor cell CD44 as a biomarker associated with sensitivity to PD-1 axis blockade in advanced non-small-cell lung cancer. J Immunother Cancer (2022) 10. doi: 10.1136/jitc-2022-004757
133. Cai D, Xu Y, Ding R, Qiu K, Zhang R, Wang H, et al. Extensive serum biomarker analysis in patients with non-small-cell lung carcinoma. Cytokine (2020) 126. doi: 10.1016/j.cyto.2019.154868
134. Carbone F, Ministrini S, Bonaventura A, Vecchié A, Minetti S, Bardi N, et al. Serum levels of VCAM-1 are associated with survival in patients treated with nivolumab for NSCLC. Eur J Clin Invest (2022) 52. doi: 10.1111/eci.13668
135. Longo V, Brunetti O, Azzariti A, Galetta D, Nardulli P, Leonetti F, et al. Strategies to improve cancer immune checkpoint inhibitors efficacy, other than abscopal effect: A systematic review. Cancers (Basel) (2019) 11. doi: 10.3390/cancers11040539
136. Cheng Y, Li H, Zhang L, Liu JJ, Yang CL, Zhang S. Current and future drug combination strategies based on programmed death-1/programmed death-ligand 1 inhibitors in non-small cell lung cancer. Chin Med J (Engl) (2021) 134:1780–8. doi: 10.1097/CM9.0000000000001560
137. Formenti SC, Rudqvist NP, Golden E, Cooper B, Wennerberg E, Lhuillier C, et al. Radiotherapy induces responses of lung cancer to CTLA-4 blockade. Nat Med (2018) 24:1845–51. doi: 10.1038/s41591-018-0232-2
138. Hellmann MD, Ciuleanu T-E, Pluzanski A, Lee JS, Otterson GA, Audigier-Valette C, et al. Nivolumab plus ipilimumab in lung cancer with a high tumor mutational burden. New Engl J Med (2018) 378:2093–104. doi: 10.1056/nejmoa1801946
139. Hellmann MD, Paz-Ares L, Bernabe Caro R, Zurawski B, Kim S-W, Carcereny Costa E, et al. Nivolumab plus ipilimumab in advanced non–Small-Cell lung cancer. New Engl J Med (2019) 381:2020–31. doi: 10.1056/nejmoa1910231
140. Ready N, Hellmann MD, Awad MM, Otterson GA, Gutierrez M, Gainor JF, et al. First-line nivolumab plus ipilimumab in advanced non-small-cell lung cancer (CheckMate 568): Outcomes by programmed death ligand 1 and tumor mutational burden as biomarkers. J Clin Oncol (2019) 37(12):992–1000. doi: 10.1200/JCO.18.01042
141. Osipov A, Lim SJ, Popovic A, Azad NS, Laheru DA, Zheng L, et al. Tumor mutational burden, toxicity, and response of immune checkpoint inhibitors targeting PD(L)1, CTLA-4, and combination: A meta-regression analysis. Clin Cancer Res (2020) 26:4842–51. doi: 10.1158/1078-0432.CCR-20-0458
142. Wang D, Shaver LG, Shi F, Wei J, Qin T, Wang S, et al. Comparative efficacy and safety of PD-1/PD-L1 immunotherapies for non-small cell lung cancer: A network meta-analysis. Eur Rev Med Pharmacol Sci (2021) 25(7):2866–84. doi: 10.26355/eurrev_202104_25541
143. Adam LC, Raja J, Ludwig JM, Adeniran A, Gettinger SN, Kim HS. Cryotherapy for nodal metastasis in NSCLC with acquired resistance to immunotherapy. J Immunother Cancer (2018) 6. doi: 10.1186/s40425-018-0468-x
144. Reck M, Borghaei H, O’Byrne KJ. Nivolumab plus ipilimumab in non-small-cell lung cancer. Future Oncol (2019) 15:2287–302. doi: 10.2217/fon-2019-0031
145. Zhao S, Ren S, Jiang T, Zhu B, Li X, Zhao C, et al. Low-dose apatinib optimizes tumor microenvironment and potentiates antitumor effect of PD-1/PD-L1 blockade in lung cancer. Cancer Immunol Res (2019) 7:630–43. doi: 10.1158/2326-6066.CIR-17-0640
146. Jiang ZB, Huang JM, Xie YJ, Zhang YZ, Chang C, Lai HL, et al. Evodiamine suppresses non-small cell lung cancer by elevating CD8+ T cells and downregulating the MUC1-C/PD-L1 axis. J Exp Clin Cancer Res (2020) 39. doi: 10.1186/s13046-020-01741-5
147. Li Y, He F, Liu S, Zhang Y, Li L, Wang B, et al. Effect of pretreatment with dexamethasone on the efficacy and immune-related adverse events of immunotherapy in first-line treatment for advanced non-small cell lung cancer: A network meta-analysis of randomized control trials. Am J Clin Exp Immunol (2021) 10(4):93–102.
148. Yan X, Wang L, Zhang R, Pu X, Wu S, Yu L, et al. Overcoming resistance to anti-PD immunotherapy in a syngeneic mouse lung cancer model using locoregional virotherapy. Oncoimmunology (2018) 7. doi: 10.1080/2162402X.2017.1376156
149. Zhang QN, Li Y, Zhao Q, Tian M, Chen LL, Miao LY, et al. Recombinant human adenovirus type 5 (Oncorine) reverses resistance to immune checkpoint inhibitor in a patient with recurrent non-small cell lung cancer: A case report. Thorac Cancer (2021) 12:1617–9. doi: 10.1111/1759-7714.13947
150. Jia K, He Y, Dziadziuszko R, Zhao S, Zhang X, Deng J, et al. T Cell immunoglobulin and mucin-domain containing-3 in non-small cell lung cancer. Transl Lung Cancer Res (2019) 8:895–906. doi: 10.21037/tlcr.2019.11.17
151. Curigliano G, Gelderblom H, Mach N, Doi T, Tai D, Forde PM, et al. Phase I/Ib clinical trial of sabatolimab, an anti–TIM-3 antibody, alone and in combination with spartalizumab, an anti–PD-1 antibody, in advanced solid tumors. Clin Cancer Res (2021) 27:3620–9. doi: 10.1158/1078-0432.CCR-20-4746
152. D’Aloia MM, Zizzari IG, Sacchetti B, Pierelli L, Alimandi M. CAR-T cells: The long and winding road to solid tumors review-article. Cell Death Dis (2018) 9. doi: 10.1038/s41419-018-0278-6
153. Ma S, Li X, Wang X, Cheng L, Li Z, Zhang C, et al. Current progress in car-t cell therapy for solid tumors. Int J Biol Sci (2019) 15:2548–60. doi: 10.7150/ijbs.34213
154. Qu J, Mei Q, Chen L, Zhou J. Chimeric antigen receptor (CAR)-t-cell therapy in non-small-cell lung cancer (NSCLC): Current status and future perspectives. Cancer Immunol. Immunother. (2021) 70:619–31. doi: 10.1007/s00262-020-02735-0
155. Zeltsman M, Dozier J, McGee E, Ngai D, Adusumilli PS. CAR T-cell therapy for lung cancer and malignant pleural mesothelioma. Trans Res (2017) 187:1–10. doi: 10.1016/j.trsl.2017.04.004
156. Oliveres H, Caglevic C, Passiglia F, Taverna S, Smits E, Rolfo C. Vaccine and immune cell therapy in non-small cell lung cancer. J Thorac Dis (2018) 10:S1602–14. doi: 10.21037/jtd.2018.05.134
157. Vermaelen K. Vaccine strategies to improve anticancer cellular immune responses. Front Immunol (2019) 10:8. doi: 10.3389/fimmu.2019.00008
158. Peng M, Mo Y, Wang Y, Wu P, Zhang Y, Xiong F, et al. Neoantigen vaccine: An emerging tumor immunotherapy. Mol Cancer (2019) 18. doi: 10.1186/s12943-019-1055-6
159. Sahin U, Türeci Ö. Personalized vaccines for cancer immunotherapy. Science 359(6382):1355–60. doi: 10.1126/science.aar7112
160. Liang H, Liu X, Wang M. Immunotherapy combined with epidermal growth factor receptor-tyrosine kinase inhibitors in non-small-cell lung cancer treatment. Onco. Targets Ther (2018) 11:6189–96. doi: 10.2147/OTT.S178497
161. Akamatsu H, Murakami E, Oyanagi J, Shibaki R, Kaki T, Takase E, et al. Immune-related adverse events by immune checkpoint inhibitors significantly predict durable efficacy even in responders with advanced non-small cell lung cancer. Oncologist (2020) 25:e679–83. doi: 10.1634/theoncologist.2019-0299
162. Shao J, Wang C, Ren P, Jiang Y, Tian P, Li W. Treatment- and immune-related adverse events of immune checkpoint inhibitors in advanced lung cancer. Biosci Rep (2020) 40. doi: 10.1042/BSR20192347
163. Ai Q, Chen W, Li Y, Li G. Upper gastrointestinal tract IrAEs: A case report about sintilimab-induced acute erosive hemorrhagic gastritis. Front Immunol (2022) 13:840916. doi: 10.3389/fimmu.2022.840916
164. Bi H, Ren D, Wang Q, Ding X, Wang H. Immune checkpoint inhibitor-induced myocarditis in lung cancer patients: A case report of sintilimab-induced myocarditis and a review of the literature. Ann Palliat. Med (2021) 10:793–802. doi: 10.21037/apm-20-2449
165. Hasan Ali O, Berner F, Bomze D, Fässler M, Diem S, Cozzio A, et al. Human leukocyte antigen variation is associated with adverse events of checkpoint inhibitors. Eur J Cancer (2019) 107:8–14. doi: 10.1016/j.ejca.2018.11.009
166. Lyon AR, Yousaf N, Battisti NML, Moslehi J, Larkin J. Immune checkpoint inhibitors and cardiovascular toxicity. Lancet Oncol (2018) 19:e447–58. doi: 10.1016/S1470-2045(18)30457-1
167. Wu J, Hong D, Zhang X, Lu X, Miao J. PD-1 inhibitors increase the incidence and risk of pneumonitis in cancer patients in a dose-independent manner: A meta-analysis. Sci Rep (2017) 7. doi: 10.1038/srep44173
168. Tone M, Izumo T, Awano N, Kuse N, Inomata M, Jo T, et al. High mortality and poor treatment efficacy of immune checkpoint inhibitors in patients with severe grade checkpoint inhibitor pneumonitis in non-small cell lung cancer. Thorac Cancer (2019) 10:2006–12. doi: 10.1111/1759-7714.13187
169. Okada N, Matsuoka R, Sakurada T, Goda M, Chuma M, Yagi K, et al. Risk factors of immune checkpoint inhibitor-related interstitial lung disease in patients with lung cancer: A single-institution retrospective study. Sci Rep (2020) 10. doi: 10.1038/s41598-020-70743-2
170. Zhang W, Gu J, Bian C, Huang G. Immune-related adverse events associated with immune checkpoint inhibitors for advanced non-small cell lung cancer: A network meta-analysis of randomized clinical trials. Front Pharmacol (2021) 12:686876. doi: 10.3389/fphar.2021.686876
171. Yamaguchi O, Kaira K, Kawasaki T, Mouri A, Hashimoto K, Shiono A, et al. Severe hepatotoxicity due to osimertinib after nivolumab therapy in patients with non-small cell lung cancer harboring EGFR mutation. Thorac Cancer (2020) 11:1045–51. doi: 10.1111/1759-7714.13363
172. Zhang L, Lin W, Tan F, Li N, Xue Q, Gao S, et al. Sintilimab for the treatment of non-small cell lung cancer. biomark Res (2022) 10. doi: 10.1186/s40364-022-00363-7
173. Grecea M, Marabelle A, Ammari S, Massard C, Champiat S. Managing hyperprogressive disease in the era of programmed cell death protein 1/Programmed death-ligand 1 blockade: A case discussion and review of the literature. Oncologist (2020) 25:369–74. doi: 10.1634/theoncologist.2019-0671
174. Wang H, Zhou F, Zhao C, Cheng L, Zhou C, Qiao M, et al. Interleukin-10 is a promising marker for immune-related adverse events in patients with non-small cell lung cancer receiving immunotherapy. Front Immunol (2022) 13:840313. doi: 10.3389/fimmu.2022.840313
175. Fujimoto A, Toyokawa G, Koutake Y, Kimura S, Kawamata Y, Fukuishi K, et al. Association between pretreatment neutrophil-to-lymphocyte ratio and immune-related adverse events due to immune checkpoint inhibitors in patients with non-small cell lung cancer. Thorac Cancer (2021) 12:2198–204. doi: 10.1111/1759-7714.14063
176. Lee YJ, Lee JB, Ha SJ, Kim HR. Clinical perspectives to overcome acquired resistance to anti–programmed death-1 and anti–programmed death ligand-1 therapy in non-small cell lung cancer. Mol Cells (2021) 44:363–73. doi: 10.14348/molcells.2021.0044
177. Gong B, Kiyotani K, Sakata S, Nagano S, Kumehara S, Baba S, et al. Secreted PD-L1 variants mediate resistance to PD-L1 blockade therapy in non-small cell lung cancer. J Exp Med (2019) 216:982–1000. doi: 10.1084/jem.20180870
178. Takahashi T, Tateishi A, Bychkov A, Fukuoka J. Remarkable alteration of PD-L1 expression after immune checkpoint therapy in patients with non-small-cell lung cancer: Two autopsy case reports. Int J Mol Sci (2019) 20. doi: 10.3390/ijms20102578
179. Xia C, Zeng F, Zhang Y. EGFR exon 21 L858R as an acquired resistance mechanism to nivolumab in a lung cancer patient originally driver gene-negative. Thorac Cancer (2019) 10:1256–9. doi: 10.1111/1759-7714.13023
180. Lantuejoul S, Damotte D, Hofman V, Adam J. Programmed death ligand 1 immunohistochemistry in non-small cell lung carcinoma. J Thorac Dis (2019) 11:S89–S101. doi: 10.21037/jtd.2018.12.103
181. Maung TZ, Ergin HE, Javed M, Inga EE, Khan S. Immune checkpoint inhibitors in lung cancer: Role of biomarkers and combination therapies. Cureus (2020) 12(5):e8095. doi: 10.7759/cureus.8095
182. Nan Z, Guoqing W, Xiaoxu Y, Yin M, Xin H, Xue L, et al. The predictive efficacy of tumor mutation burden (TMB) on nonsmall cell lung cancer treated by immune checkpoint inhibitors: A systematic review and meta-analysis. BioMed Res Int (2021) 2021. doi: 10.1155/2021/1780860
183. Berland L, Heeke S, Humbert O, Macocco A, Long-Mira E, Lassalle S, et al. Current views on tumor mutational burden in patients with nonsmall cell lung cancer treated by immune checkpoint inhibitors. J Thorac Dis (2019) 11:S71–80. doi: 10.21037/jtd.2018.11.102
184. To KKW, Fong W, Cho WCS. Immunotherapy in treating EGFR-mutant lung cancer: Current challenges and new strategies. Front Oncol (2021) 11:635007. doi: 10.3389/fonc.2021.635007
185. Budczies J, Kirchner M, Kluck K, Kazdal D, Glade J, Allgäuer M, et al. Deciphering the immunosuppressive tumor microenvironment in ALK- and EGFR-positive lung adenocarcinoma. Cancer Immunol. Immunother. (2022) 71:251–65. doi: 10.1007/s00262-021-02981-w
186. Uras IZ, Moll HP, Casanova E. Targeting KRAS mutant non-small-cell lung cancer: Past, present and future. Int J Mol Sci (2020) 21:1–30. doi: 10.3390/ijms21124325
187. di Noia V, D’Argento E, Pilotto S, Vita E, Ferrara MG, Damiano P, et al. Blood serum amyloid a as potential biomarker of pembrolizumab efficacy for patients affected by advanced non-small cell lung cancer overexpressing PD-L1: Results of the exploratory “FoRECATT” study. Cancer Immunol. Immunother. (2021) 70:1583–92. doi: 10.1007/s00262-020-02788-1
188. Nie X, Xia L, Gao F, Liu L, Yang Y, Chen Y, et al. Serum metabolite biomarkers predictive of response to PD-1 blockade therapy in non-small cell lung cancer. Front Mol Biosci (2021) 8:678753. doi: 10.3389/fmolb.2021.678753
189. Mehlman C, Kamga PT, Costantini A, Julié C, Dumenil C, Dumoulin J, et al. Baseline hedgehog pathway activation and increase of plasma Wnt1 protein are associated with resistance to immune checkpoint inhibitors in advanced non-Small-Cell lung cancer. Cancers (Basel) (2021) 13(5):1107. doi: 10.3390/cancers
190. Li T, Wang H, Xu J, Li C, Zhang Y, Wang G, et al. TGFBR2 mutation predicts resistance to immune checkpoint inhibitors in patients with non-small cell lung cancer. Ther Adv Med Oncol (2021) 13. doi: 10.1177/17588359211038477
191. Gutiontov SI, Turchan WT, Spurr LF, Rouhani SJ, Chervin CS, Steinhardt G, et al. CDKN2A loss-of-function predicts immunotherapy resistance in non-small cell lung cancer. Sci Rep (2021) 11. doi: 10.1038/s41598-021-99524-1
192. Bronte G, Petracci E, de Matteis S, Canale M, Zampiva I, Priano I, et al. High levels of circulating monocytic myeloid-derived suppressive-like cells are associated with the primary resistance to immune checkpoint inhibitors in advanced non-small cell lung cancer: An exploratory analysis. Front Immunol (2022) 13:866561. doi: 10.3389/fimmu.2022.866561
193. Wang L, Liu F, Li J, Ma L, Feng H, Liu Q, et al. From anti-pd-1/pd-l1 to ctla-4 and to muc1–is the better response to treatment in smokers of cancer patients drug specific? J Pers Med (2021) 11. doi: 10.3390/jpm11090914
194. Watanabe M, Kenmotsu H, Ko R, Wakuda K, Ono A, Imai H, et al. Isolation and molecular analysis of circulating tumor cells from lung cancer patients using a microfluidic chip type cell sorter. Cancer Sci (2018) 109:2539–48. doi: 10.1111/cas.13692
195. Guo Q, Jiang C. Delivery strategies for macromolecular drugs in cancer therapy. Acta Pharm Sin B (2020) 10:979–86. doi: 10.1016/j.apsb.2020.01.009
196. Moral MEG, Siahaan TJ. Conjugates of cell adhesion peptides for therapeutics and diagnostics against cancer and autoimmune diseases. Curr Top Med Chem (2018) 17:3425–43. doi: 10.2174/1568026618666180118154514
197. Larkin J, Chiarion-Sileni V, Gonzalez R, Grob J-J, Rutkowski P, Lao CD, et al. Five-year survival with combined nivolumab and ipilimumab in advanced melanoma. New Engl J Med (2019) 381:1535–46. doi: 10.1056/nejmoa1910836
198. Schmidts A, Maus MV. Making CAR T cells a solid option for solid tumors. Front Immunol (2018) 9:2593. doi: 10.3389/fimmu.2018.02593
199. Leko V, Rosenberg SA. Identifying and targeting human tumor antigens for T cell-based immunotherapy of solid tumors. Cancer Cell (2020) 38:454–72. doi: 10.1016/j.ccell.2020.07.013
Keywords: non-small cell lung cancer, monoclonal antibody, immunotherapy, immune checkpoint inhibitor, cell adhesion molecules
Citation: Yang H, Miao Y, Yu Z, Wei M and Jiao X (2023) Cell adhesion molecules and immunotherapy in advanced non-small cell lung cancer: Current process and potential application. Front. Oncol. 13:1107631. doi: 10.3389/fonc.2023.1107631
Received: 25 November 2022; Accepted: 07 February 2023;
Published: 21 February 2023.
Edited by:
Keqiang Chen, National Cancer Institute at Frederick (NIH), United StatesCopyright © 2023 Yang, Miao, Yu, Wei and Jiao. This is an open-access article distributed under the terms of the Creative Commons Attribution License (CC BY). The use, distribution or reproduction in other forums is permitted, provided the original author(s) and the copyright owner(s) are credited and that the original publication in this journal is cited, in accordance with accepted academic practice. No use, distribution or reproduction is permitted which does not comply with these terms.
*Correspondence: Xue Jiao, eGppYW9AY211LmVkdS5jbg==; Minjie Wei, bWp3ZWlAY211LmVkdS5jbg==