- 1Department of Anesthesiology, The Affiliated Hospital of Xuzhou Medical University, Xuzhou, China
- 2Jiangsu Province Key Laboratory of Anesthesiology, Xuzhou Medical University, Xuzhou, China
- 3Department of Anesthesiology, Xinhua Hospital Affiliated to Shanghai Jiao Tong University School of Medicine, Shanghai, China
- 4Department of Anesthesiology, The First People’s Hospital of Changde City, Changde, China
Background: Neutrophil extracellular traps (NETs) can enhance the metastasis of non-small cell lung cancer (NSCLC). As biomarkers of tumor metastasis, metalloproteinases (MMPs) and vascular endothelial growth factor (VEGF) together with NETs are essential to endothelial-to-mesenchymal transition (EMT). We hypothesized that intravenous infusion of lidocaine and dexmedetomidine could reduce the production of NETs and biomarkers of tumor metastasis after video-assisted thoracic surgery (VATS) in NSCLC patients.
Method: The trial included 132 NSCLC patients undergoing VATS. The patients were equally randomized to a placebo group (Group C), a lidocaine group (Group L, intravenous lidocaine 8 mg/kg/h for 15 minutes before anesthesia, 2 mg/kg/h during surgery, and 1 mg/kg/h until 24 hours after surgery), a dexmedetomidine group (Group D, intravenous dexmedetomidine 2 μg/kg/h for 15 minutes before anesthesia, 0.5 μg/kg/h during surgery, and 0.25 μg/kg/h until 24 hours after surgery), and a dexmedetomidine plus lidocaine group (Group LD, combination use of lidocaine and dexmedetomidine). The primary outcome was the production of myeloperoxidase (MPO) and citrullinated histone-3 (H3Cit), biomarkers of NETs, on postoperative day (POD) 1. MMP-3, MMP-9, and VEGF-α, as biomarkers of tumor metastasis, were also evaluated on POD 1.
Results: The baseline patient characteristics and perioperative data did not differ between the study groups. MPO was significantly decreased in Groups L, D, and LD (-197.08 ± 34.01, -137.37 ± 32.41, and -189.45 ± 33.73 U/ml, P<0.001, respectively) compared with Group C (-106.51 ± 25.44 U/ml). H3Cit was also lessened in Groups L, D, and LD (-49.51 ± 9.11, -34.80 ± 10.37, and -51.82 ± 8.98 ng/ml, P<0.001, respectively) compared with Group C (-24.73 ± 7.65 ng/ml). Lidocaine and dexmedetomidine also reduced MMP-3 (-69.08 ± 13.22, -52.84 ± 13.78, -85.34 ± 12.59 vs. -40.55 ± 10.71 ng/ml in Group L, D, LD vs. Group C, P<0.001, respectively), MMP-9 (-8.46 ± 1.68, -6.07 ± 1.82, -9.67 ± 1.43 vs. -4.28 ± 1.29 ng/ml in Group L, D, LD vs. Group C, P<0.001, respectively), and VEGF-α (-95.55 ± 22.53, -71.65 ± 18.77, -104.89 ± 15.49 vs. -51.73 ± 16.27 pg/ml in Group L, D, LD vs. Group C, P<0.001, respectively) on POD 1.
Conclusion: In NSCLC patients, continuous perioperative intravenous infusion of lidocaine and dexmedetomidine significantly reduced the production of NETs and tumor metastasis biomarkers on POD 1. Meanwhile, it also decreased inflammation, protected cellular immune function, reduced pain and opioid consumption, and improved the quality of postoperative recovery.
Clinical trial registration: chictr.org.cn, identifier: 187049.
Foundations
1. National Natural Science Foundation of China, 82270059;
2. Jiangsu Provincial Health Commission Medical Research Key Project, K2019003;
3. Jiangsu Natural Science Foundation, BK20221222; China Primary Health Care Foundation, YLGX-MZ-2022004;
4. The foundation of Xuzhou Science and Technology Department, KC21154.
1 Introduction
Non-small cell lung cancer (NSCLC) is the leading cause of cancer death worldwide due mainly to recurrence and metastasis (1). Surgery as the optimal curative option for early-stage NSCLC patients could paradoxically increase the development of metastases (2). Perioperative inflammation and sympathetic nervous system (SNS) activation are closely related to tumor metastatic progression (3). Anesthesia interventions are an essential part of the perioperative period and have been shown to be a potential way to decrease tumor metastasis risk by reducing stress and inflammation levels and preserving immune system function in NSCLC patients (4).
Neutrophil extracellular traps (NETs) are sticky substances containing DNA and various proteases released during the apoptosis of neutrophils (5). NETs have emerged recently as a new biomarker of tumor metastasis (6). NETs can not only enhance metastases of NSCLC (7) or other cancers (8, 9) but also awaken dormant cancer cells (10). NETs, along with metalloproteinases (MMP) and vascular endothelial growth factor (VEGF), promote vascular leakage and endothelial-to-mesenchymal transition (EMT) (11).
The amide local anesthetic lidocaine (Lido) has anti-inflammatory effects and has been widely used clinically. Recent studies proved that perioperative intravenous administration of lidocaine could reduce NETs in pancreatic cancer patients (12) and peripheral circulation levels of VEGF in breast cancer patients (13). Dexmedetomidine (Dex), as a highly selective α2-adrenergic receptor agonist, plays an important role in anesthesia and has been commonly used during thoracic surgical anesthesia. It has been demonstrated that preoperative administration of Dex can not only improve intraoperative oxygenation, lung mechanics, and the quality of postoperative recovery (14, 15) but also alleviate SNS activation and the inflammatory response, immunosuppression, and postoperative pain (16). It is not known whether Lido and Dex minimize the production of perioperative NETs in NSCLC patients.
The primary purpose of our study was to investigate whether the continuous intravenous infusion of Lido and Dex during the perioperative period could reduce the production of NETs in patients with NSCLC. Several studies have shown that it is feasible to detect markers of NET formation in serum (e.g., myeloperoxidase (MPO) and citrullinated histone-3 (H3Cit)) (13, 17), so we selected MPO and H3Cit as alternative indicators of NET formation in our study. This study will evaluate tumor metastasis biomarkers (VEGF-A, MMP-3, MMP-9), inflammatory factors, immune function and clinical outcomes.
2 Materials and methods
This study was a prospective, single-center, randomized, double-blinded clinical trial. The protocol was approved by the Ethics Committee of the Affiliated Hospital of Xuzhou Medical University (XYFY2022-KL254-01). Before the inclusion of the first patient, the trial was registered at the Chinese Clinical Trial Registry (ChiCTR2100050796; leading researcher: Zhiping Wang). This study was carried out between July 22, 2022, and October 2, 2022. Written informed consent was obtained from patients before they participated in the study.
2.1 Patients
Inclusion criteria were as follows: 18 to 80 years of age, American Society of Anesthesiologists (ASA) physical status I, II, or III, scheduled for elective video-assisted thoracic surgery, consent to receive postoperative patient-controlled analgesia (PCA), early-stage lung cancer without distant dissemination (clinical stage ≤ II), and willing to accept follow-up. The preoperative diagnosis of early-stage lung cancer was based on assessment by thoracic surgeons and findings from computed tomography and positron emission tomography-computed tomography. The final diagnosis of early-stage lung cancer depended on the results of intraoperative rapid frozen pathology and postoperative routine pathological examination. The exclusion criteria were preoperative adjuvant chemotherapy or radiotherapy, pregnancy, previous treatment with beta-blockers, hepatic or renal insufficiency or dialysis, obstructive pneumonia or other acute infections not well controlled, opioid abuse, mental health problems or cognitive impairment, history of epilepsy and taking antiepileptic drugs, chronic inflammatory or autoimmune disease and glucocorticoids or other inflammatory immunosuppressive therapy taken within the past month. Patients were removed from the trial if treated with glucocorticoids perioperatively or transferred to the ICU.
2.2 Randomization, concealment, and blinding
Patients were randomly assigned to one of the four groups using IBM SPSS 24.0 software (SPSS Inc., IBM, Chicago, IL, USA) in a 1:1:1:1 ratio by investigators not involved in the trial. The experimental groups were the placebo group (C), the placebo plus Lido group (L), the DEX plus placebo group (D), and the DEX plus Lido group (LD).
The pharmacy personnel performed the blinding by writing a random number in a sealed and opaque envelope. The medication was applied according to the protocol in the envelope. The drugs handed over to the anesthesiologist were labeled only with the method of administration. The pumping rate was set consistently at a rate of 0.4 ml/kg/h 15 minutes before anesthesia and 0.1 ml/kg/h after induction of anesthesia. Specifically, Lido was pumped at 8 mg/kg/h for 15 minutes before anesthesia, 2 mg/kg/h during surgery, and 1 mg/kg/h until 24 hours after surgery. Dex was pumped at 2 μg/kg/h for 15 minutes before anesthesia, 0.5 μg/kg/h during surgery, and 0.25 μg/kg/h until 24 hours after surgery.
The researcher was blinded to the grouping of patients. All trial personnel received standardized training, and the same surgeons performed all procedures.
2.3 Protocol and intervention
All patients routinely fasted for 6 to 8 hours. Once the patient arrived in the operating room, the anesthesiologist established peripheral venous access and performed a standardized monitoring process. Monitored parameters and procedures of monitoring included noninvasive blood pressure (NIBP), ECG, pulse oximetry, invasive blood pressure (IBP), bispectral index (BIS, VISTA™ Monitoring System, Aspect Medical Systems Inc., Norwood, MA, USA) and a train-of-four stimulation (TOF) (BeneVision N17/N15 OR, Mindray Medical International Co., Guangdong, China).
A standardized anesthesia process was implemented for each patient. The intervention described above was started before the induction of anesthesia. General anesthesia was induced using a combination of sufentanil 0.3-0.6 μg/kg, etomidate 0.2-0.4 mg/kg, and cis-atracurium 0.15-0.25 mg/kg. Tracheal intubation was performed under visual laryngoscopy after induction. The ventilator parameters were set as follows: tidal volume 6–8 mL/kg; respiratory rate 12–16 times/min; ratio of aspiration to expiration 1:1.5; fraction of inspired oxygen 60-100%; and positive end-expiratory pressure 0-6 cmH2O. Anesthesia was maintained with propofol 2-10 mg/kg/h and remifentanil 0.1-0.8 μg/kg/min, with propofol dose adjustment based on BIS values (fluctuating between 40-50) and remifentanil adjustment based on intraoperative hemodynamic parameters (fluctuating ±10% from preoperative level); cisatracurium was infused intraoperatively at 1-2 μg/kg/min.
At the end of the surgery, the lungs were bilaterally aspirated, and the lung on the operative side was treated with pulmonary resuscitation (repeated puffing at a pressure of 15-25 cmH2O until no bubbles were visible from the negative pressure drainage device). All anesthetic drugs were discontinued except for the experimental drugs. Then, the patients were transferred to the postanesthesia care unit for further observation and subsequently transferred back to the surgical ward.
All patients were treated with an electronic PCA pump (sufentanil 100 μg plus tropisetron 10 mg) for postoperative analgesia. The PCA background infusion rate was initially set at 2 ml/h, and an additional 0.5 ml of solution was given per compression. The PCA adjustment was performed by trained thoracic nursing staff. PCA was suspended at 72 hours postoperatively.
2.4 Collection of blood samples
The patient’s preoperative blood samples (5 ml) were collected before anesthesia was performed, and postoperative blood samples were collected 24 hours after the operation. Blood samples were obtained from the patient’s median cubital vein and injected into a procoagulation tube. After being left to coagulate for 1 hour at room temperature, the blood was centrifuged at 3000 rpm for 15 minutes. Subsequently, 2 ml of serum was acquired from the upper layer of the blood and transferred to sterile EP tubes, which were then stored at -80°C in a refrigerator for further enzyme-linked immunosorbent assay (ELISA) experiments.
2.5 Neutrophil extracellular trapping and other tests
MPO, H3Cit, MMP-3, MMP-9, and VEGF-α were measured using commercially available ELISA kits (Shanghai Lanpai Biotechnology Co.). All test procedures were carried out strictly according to the ELISA kits’ instructions. Due to financial constraints, repeat measurements were not performed. Inflammatory factors, including IL-1β, IL-2, IL-4, IL-6, IFN-γ and TNF-α, were tested. The numbers of neutrophils, lymphocytes, and lymphocyte subsets, including the percentages of CD4+ T cells, CD8+ T cells, and NK cells, were also investigated perioperatively. The hospital laboratory department measured inflammatory factor levels, blood cell counts, and lymphocyte subsets. Blood sample collection was performed by the staff responsible for blood sample collection in this study. We also investigated the Th1:Th2 balance by evaluating the IFN-γ:IL-4 ratio.
2.6 Outcome measures
The primary outcomes were the levels of NET-specific markers (MPO and H3Cit) on postoperative day (POD) 1. Secondary outcomes included tumor metastasis biomarkers (VEGF-α, MMP-3, MMP-9), inflammatory factors (IL-1β, IL-2, IL-4, IL-6, IFN-γ, and TNF-α), immune function (the counts of macrophages, neutrophils, and lymphocytes, the percentages of CD4+ T cells, CD8+ T cells, NK cells, the ratio of CD4+:CD8+ T cells, and the ratio of IFN-γ:IL-4), intraoperative remifentanil consumption, time to the first use of PCA, time to the first exhaust and defecation, drainage removal time, bed-leaving time, length of hospital stay (LOS), sufentanil consumption in the first 3 days postoperatively, VAS scores at rest and activity during the 3 days after surgery, postoperative quality of recovery based on the 40-item Quality of Recovery (QoR-40) questionnaire administered during the first 3 days after surgery, incidence of postoperative pulmonary complications (PPCs) during hospitalization, all-cause mortality within 30 days postoperatively, and rate of readmission due to complications within 30 days postoperatively.
2.7 Sample-size calculation
The sample size was calculated using PASS software (version 15.0.5, NCSS, LCC, USA). The difference in serum levels of MPO before and after surgery, the measurement of which required a larger sample size than H3Cit testing, was chosen for calculation based on the results of the pre-experiment ELISA. The results showed that the differences in MPO in the C group, L group, D group, and LD group were -86.9 ± 22.26, -177.8 ± 27.04, -137.2 ± 16.32 and -218.4 ± 20.16 U/L, respectively. All pairwise types in multiple comparisons in PASS software were selected for sample size calculation. The minimum detectable difference of the mean from the results was 40.6 U/L, and a maximum standard deviation of 27.04 U/L was chosen. Assuming a type I error of 0.05 and a type II error of 0.1, 30 patients per group were needed to ensure 90% power. Considering the possibility of drop-out, 33 patients were eventually required for each group.
2.8 Statistical analysis
All statistical analyses were conducted using IBM SPSS 24.0 software, and plots were generated using GraphPad Prism 8 (GraphPad Software, LLC, San Diego, CA, USA). The normality and homogeneity of the data were assessed using the Kolmogorov−Smirnov test, and Levene’s test was used for continuous data. Normally distributed data were expressed as the mean ± standard deviation and examined using one-way ANOVA. If the homogeneity of variance was not achieved, then the Kruskal−Wallis test was performed.
A generalized linear model (GLM) was applied to test the differences in metastasis biomarkers, inflammation factors, peripheral blood cells, and cellular immune functions. The preoperative level was chosen as a covariable during analysis. The nonnormally distributed data are presented as the median (IQR) and were tested with the Kruskal−Wallis test. Categorical variables are reported as frequencies (%) and were analyzed using Fisher’s exact test.
Repeatedly measured data (VAS scores and QoR-40 scores) were analyzed using a generalized estimating equation (GEE) with a robust estimation as the covariance matrix. An unstructured working correlation matrix was selected during the GEE process. The Kaplan−Meier test was applied to describe and analyze the differences in time to the first use of PCA, time to the first exhaustion and defecation, drainage removal time, and bed-leaving time. The Bonferroni method was applied to correct P values when multiple comparisons were conducted. The effect size expressed as a partial η2 was also calculated.
Tumor metastasis biomarkers, inflammatory factors, and cellular immune function were included as independent variables, and NETs were taken as dependent variables in the multifactorial regression. The stepwise method was chosen when the probability corresponding to the F value of the independent variable was included if <0.05 and excluded if >0.10. All statistical analyses were two-tailed tests, and a P value < 0.05 was recognized as indicative of significant difference.
3 Results
Between July 22, 2002, and October 2, 2022, 203 subjects were enrolled, and 132 were randomized into four groups (Figure 1). Eventually, 33 patients from each group were included in the final analysis. There was no loss to follow-up one month after surgery. The last patient was admitted on October 2.
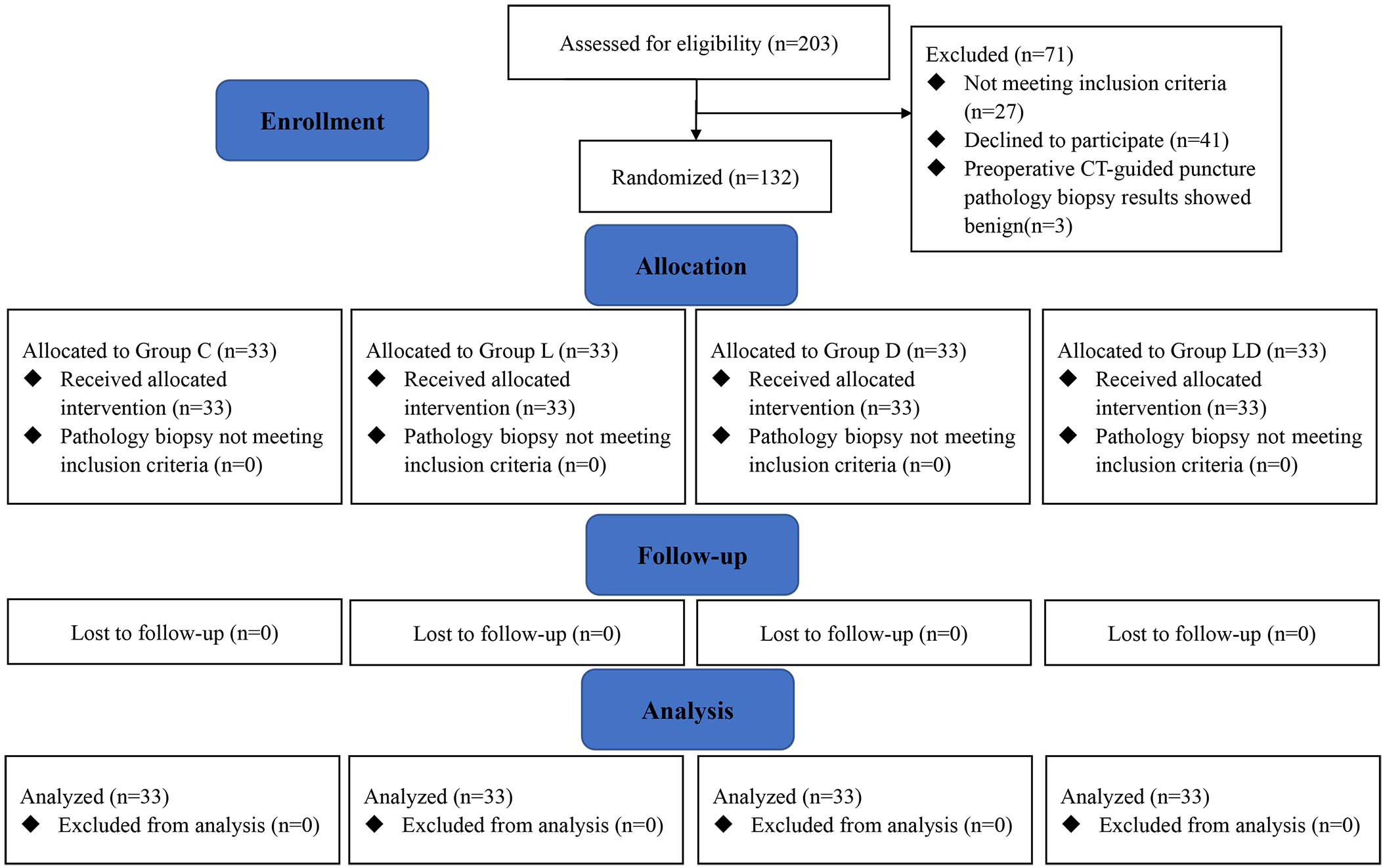
Figure 1 Study flow diagram based on the Consolidated Standards of Reporting Trials (CONSORT) statement. Group C, placebo group; Group L, placebo plus lidocaine group; Group L, dexmedetomidine plus placebo group; Group LD, dexmedetomidine plus lidocaine group.
3.1 Participants
The baseline patient demographic and clinical characteristics in the four groups were comparable (Table 1). The mean arterial pressure remained constant during anesthesia and surgery (Table 2). There was no dose difference between perioperative Lido and Dex (P=0.147) in the drug-applying group (Table 2). Other intraoperative and surgical-related characteristics were identical among the four groups, except for the differences in intraoperative remifentanil dosage (P<0.001) and propofol dosage (P=0. 008) (Table 2).
3.2 Primary outcomes
The biomarkers of NETs differed among the four groups. All the results were obtained by subtracting the preoperative level from the postoperative level. MPO was significantly decreased in Groups L, D, and LD (-197.08 ± 34.01, -137.37 ± 32.41, and -189.45 ± 33.73 U/ml, P<0.001, respectively) compared with Group C (-106.51 ± 25.44 U/ml). H3Cit was also lessened in Groups L, D, and LD (-49.51 ± 9.11, -34.80 ± 10.37, and -51.82 ± 8.98 ng/ml, P<0.001, respectively) compared with Group C (-24.73 ± 7.65 ng/ml) (Table 3, Figure 2A).
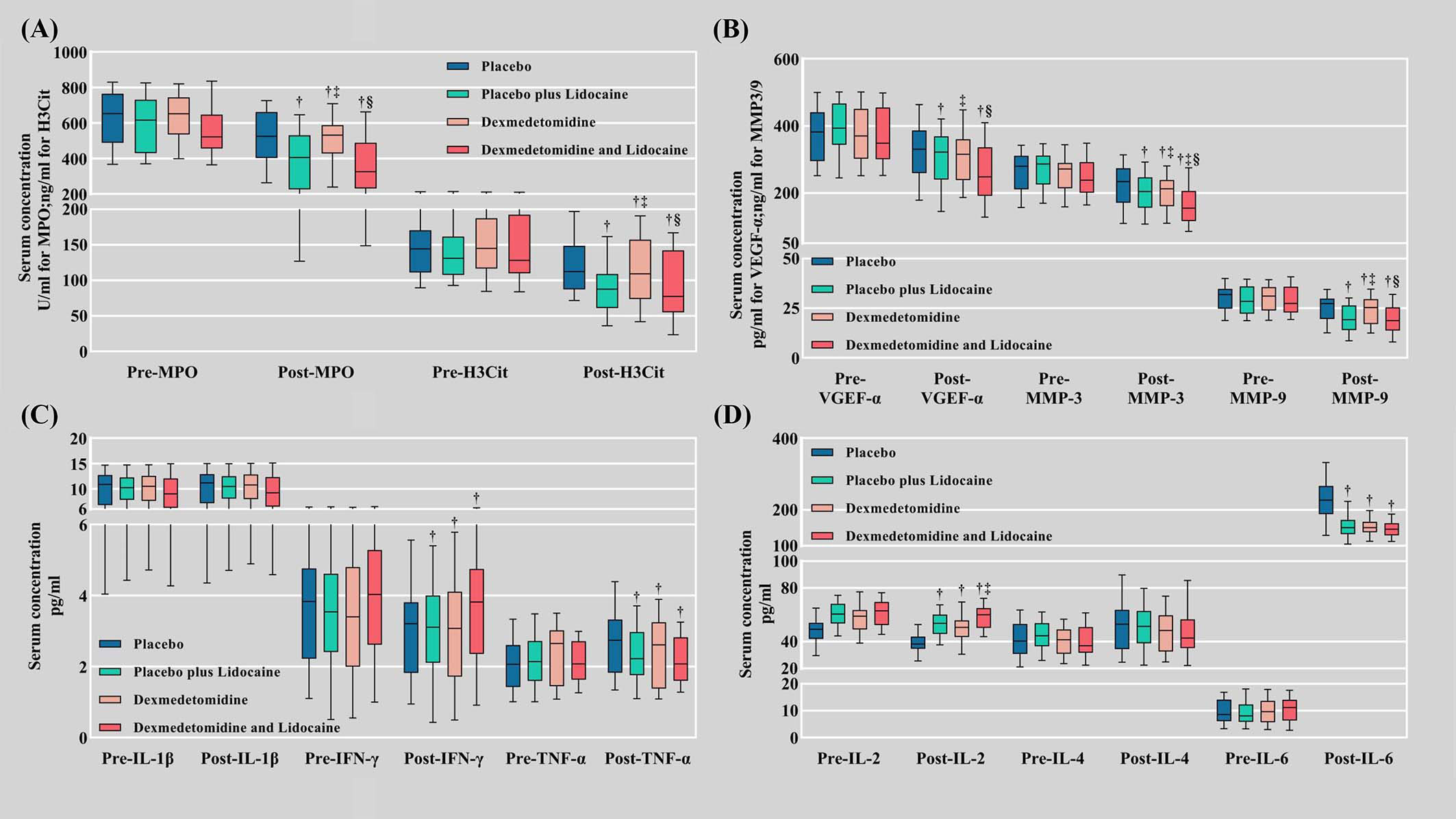
Figure 2 Serum biomarker levels. (A) Biomarkers of NET formation (MPO and H3Cit); (B) Metastasis biomarkers (VEGF-α, MMP-3, and MMP-9); (C) Inflammatory factors (IL-1β, IFN-γ, TNF-α); (D) Inflammatory factors (IL-2, IL-4, and IL-6). Pre, preoperative; Post, postoperative; NETs, neutrophil extracellular traps; MPO, myeloperoxidase; H3Cit, citrullinated histone 3; VEGF-A, vascular endothelial growth factor-A; MMP-3, matrix metalloproteinase-3; MMP-9, matrix metalloproteinase-9. † Compared with the placebo group (C), the difference was statistically significant. ‡ Compared with the placebo plus lidocaine group (L), the difference was statistically significant. § Compared with the dexmedetomidine plus placebo group (D), the difference was statistically significant.
3.3 Secondary outcomes
3.3.1 Biomarkers of tumor metastasis
On POD 1, serological biomarkers of tumor metastasis MMP-3 (-69.08 ± 13.22, -52.84 ± 13.78, -85.34 ± 12.59 ng/ml vs. -40.55 ± 10.71 ng/ml in Group L, D, LD vs. Group C, P<0.001, respectively), MMP-9 (-8.46 ± 1.68, -6.07 ± 1.82, -9.67 ± 1.43 ng/ml in Group L, D, LD vs. Group C, P<0.001, respectively), and VEGF-α (-95.55 ± 22.53, -71.65 ± 18.77, -104.89 ± 15.49 vs. -51.73 ± 16.27 pg/ml in Group L, D, LD vs. Group C, P<0.001, respectively) were also decreased in the Lido and Dex groups (Table 3, Figure 2B).
3.3.2 Inflammatory factors
Among the inflammatory indicators, IL-1β and IL-4 did not differ significantly between the four groups at 24 hours postoperatively. The postoperative elevation of IL-6 (P<0.001) and TNF-α (P<0.001) was markedly suppressed in the pharmacological intervention groups (L, D, and LD) compared to group C. In contrast, the postoperative decrease in IL-2 (P<0.001) and IFN-γ (P<0.001) was dramatically retarded in the pharmacological intervention group compared to group C (Table 3, Figures 2C, D).
3.3.3 Cellular immune function
Postoperative neutrophil counts showed no difference in elevation between groups, while lymphocyte counts (P<0.001) decreased in the pharmacological intervention groups. Postoperative macrophage elevation was not significantly different between groups (Figure 3A). The postoperative decrease in the percentage of NK cells was slight in groups L, D, and LD versus C (P<0.001) (Table 3) (Figure 3B). The trend of CD4+ T-cell changes (P<0.001) mirrored the pattern of lymphocyte counts (Figure 3B), whereas an examination of the numbers of CD8+ T cells (Figure 3B) and the ratio of CD4+:CD8+ T cells (Figure 3C) revealed no significant difference between groups. The postoperative decrease in the IFN-γ:IL-4 ratio in the pharmacological intervention group was markedly slowed, which was significantly different from that in group C (P<0.001) (Figure 3C).
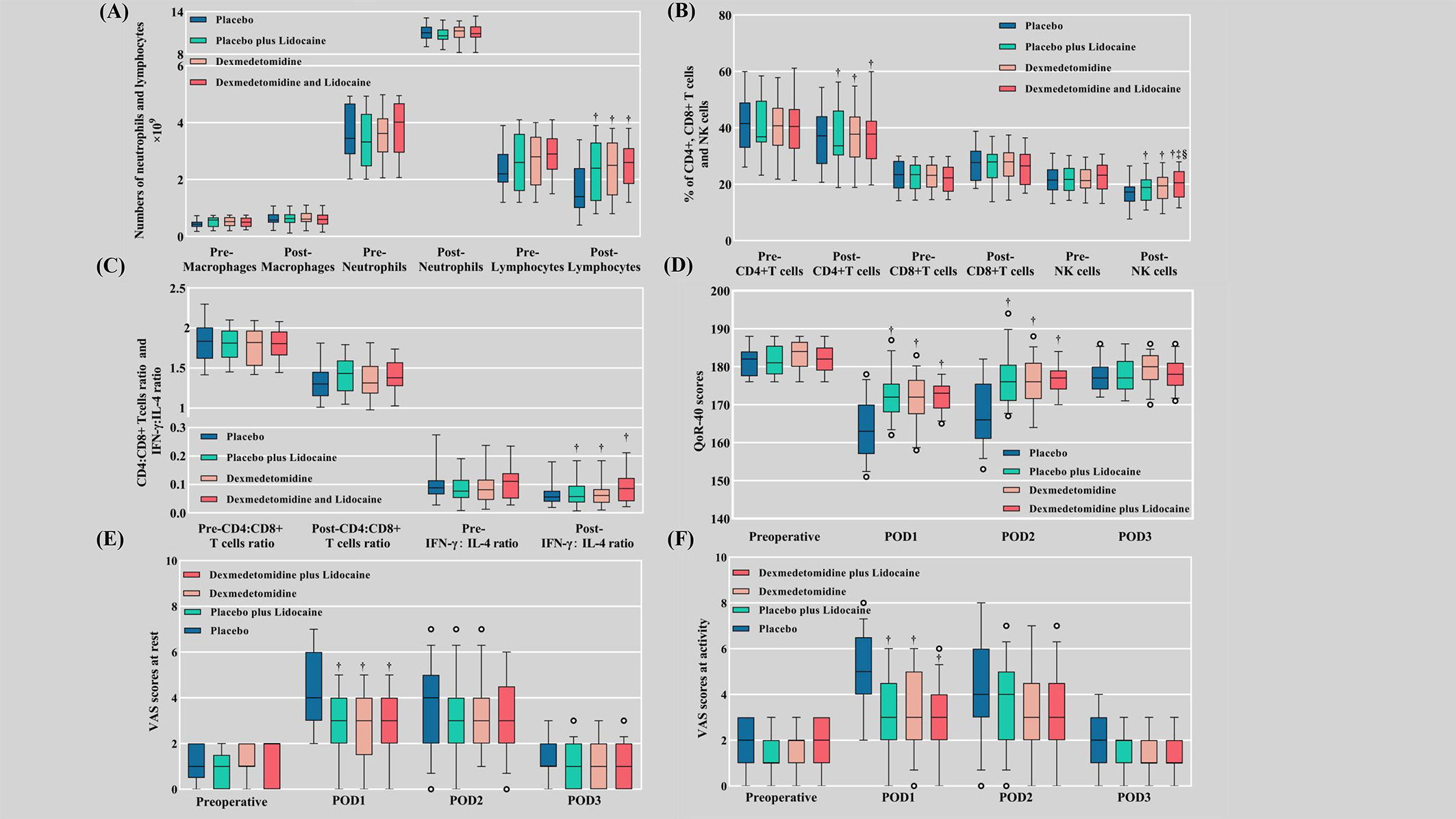
Figure 3 Cellular immune function, VAS scores, and QoR-40 scores. (A) Numbers of macrophages, neutrophils, and lymphocytes; (B) Percentages of CD4+ T cells, CD8+ T cells, and NK cells; (C) The ratios of CD4+:CD8+ T cells and IFN-γ:IL-4; (D) QoR-40 scores; (E) VAS scores at rest; (F) VAS scores at activity. Pre, preoperative; Post, postoperative; VAS, visual analog scale; QoR-40, Quality of Recovery 40; POD, postoperative day. † Compared with the placebo group (C), the difference was statistically significant. ‡ Compared with the placebo plus lidocaine group (L), the difference was statistically significant. § Compared with the dexmedetomidine plus placebo group (D), the difference was statistically significant.
3.3.4 VAS and QoR-40
The consumption of sufentanil at 3 days postoperatively was the lowest in the LD group, followed by the L, D, and C groups sequentially (P<0.001). There were no differences in preoperative VAS scores and QoR-40 scores between groups (Figures 3D–F). Both at-rest VAS scores (P<0.001) and at-activity VAS scores (P<0.001) were inferior in the pharmacological intervention group compared to group C at POD 1, while there was no discrepancy between the four groups at POD 2 and POD 3. QoR-40 scores were better in the pharmacological intervention group than in group C at POD 1 (P<0.001) and POD 2 (P<0.001), while there was no difference between the four groups at POD 3 (Supplementary Table 1).
3.3.5 Other postoperative observations
There were significant differences between the four groups concerning the time to the first PCA (P<0.001), exhaustion (P<0.001), defecation (P<0.001), bed-leaving (P<0.001), and drainage tube removal (P<0.001) (Figures 4A–E). The incidence of PPCs during hospitalization (P=0.804), the severity of PPCs according to the Clavien−Dindo classification (P=0.485), and the length of stay (P=0.630) did not differ significantly between groups (Supplementary Table 1).
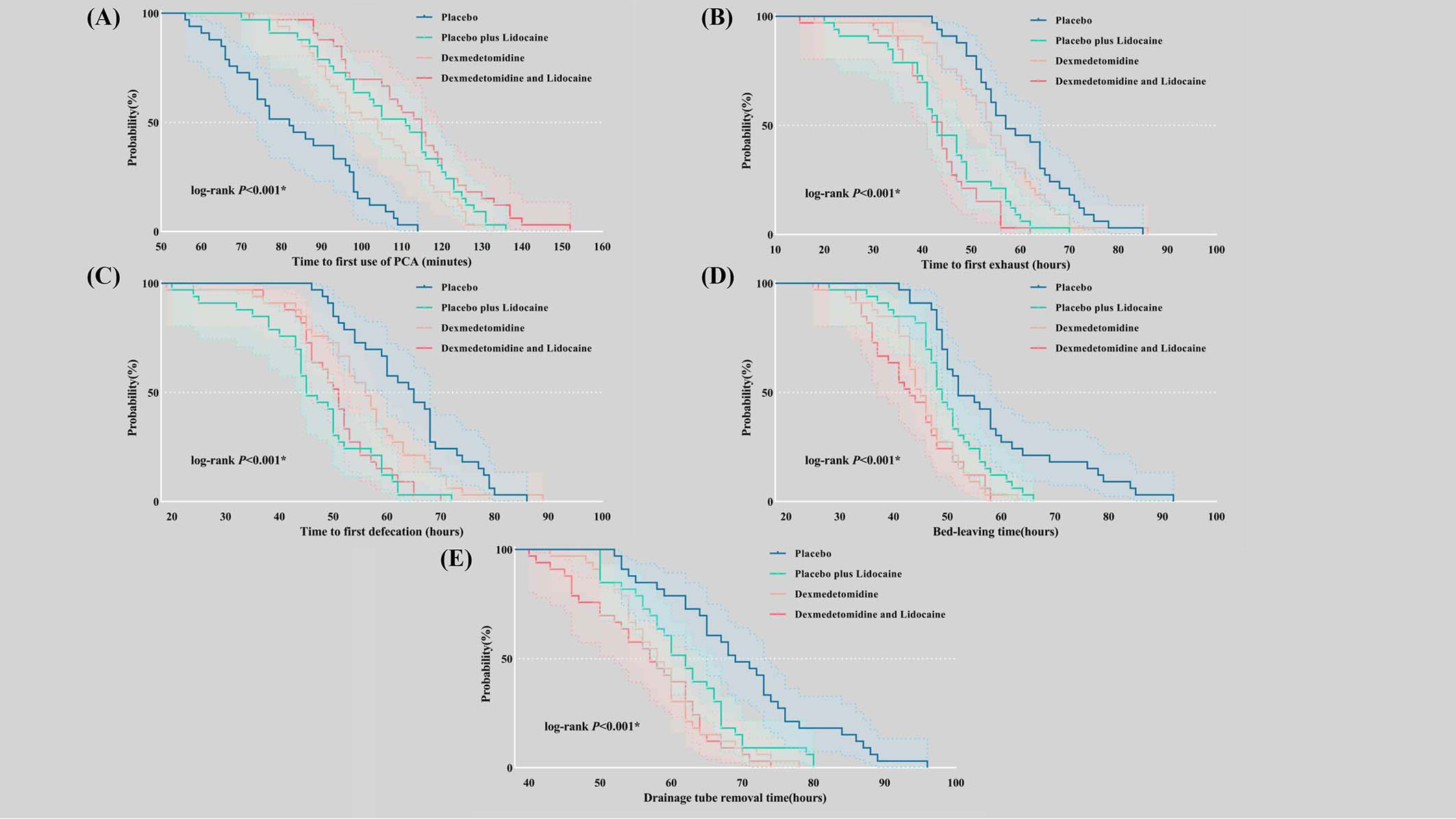
Figure 4 Kaplan−Meier curves were used to describe time-related outcomes during the postoperative period. (A) Time to first use of PCA; (B) Time to first exhaust; (C) Time to first defecation; (D) Bed-leaving time; (E) Drainage tube removal time. PCA, patient-controlled analgesia.
3.3.6 Short-term outcomes
No deaths occurred within 30 days after surgery in our study, and no patients were readmitted due to surgical complications (Supplementary Table 1).
3.4 Subgroup analysis of NETs
Subgroup analysis investigating the effect of intravenous Lido and Dex on differences in NETs between the perioperative and postoperative periods were conducted. The subgroup categories were based on age (≤ 60 years vs. > 60 years), sex (female vs. male), cancer stage (T1-2 vs. Tis), surgical type (lobectomy vs. nonlobectomy) and presence of PPCs (Yes vs. No). There was no interaction between grouping factors and subgroup classifications except the presence of PPCs (P=0.003). (Supplementary Table 2).
3.5 Multivariable linear regression analysis of NETs
The effects of tumor metastasis biomarkers, inflammatory factors, and cellular immune function on NETs were explored. There was a positive correlation between VEGF-α, MMP-3, MMP-9, and NETs. IL-6 was positively correlated with MPO, while CD4+ T cells were negatively correlated with H3Cit (Supplementary Table 3, Supplementary Figure 1).
3.6 Exploring the correlation between NETs and clinical outcomes
Postoperative MPO levels were positively correlated with the incidence of PPCs (β=0.392, P<0.001). MPO and H3Cit were positively correlated with active VAS scores at POD 1 (β=0.217, P=0.014; β=0.216, P=0.015, respectively) and negatively correlated with QoR-40 scores at POD 1 (β=-0.226, P=0.011; β=-0.177, P=0.047, respectively). MPO and H3Cit were positively correlated with the time to first exhaust (β=0.363, P<0.001; β=0.298, P<0.001, respectively) and defecation (β=0.328, P<0.001; β=0.256, P=0.004, respectively). The patients who suffered postoperative infectious adverse events had a more significant reduction in MPO values in the trial groups (-81.78 ± 13.54, -156.99.70 ± 13.92, -129.09 ± 16.45, and -172.00 ± 40.78 in Group C, L, D, and LD, P<0.001, respectively).
4 Discussion
In this single-center, double-blind, randomized trial, we evaluated the effect of intraoperative intravenous administration of Lido and Dex on perioperative serum levels of biomarkers of NETs (MPO and H3Cit) as well as other biomarkers contributing to invasion of cancer in early-stage NSCLC patients. H3Cit, as the critical biomarker of NET formation, predicts the risk of high mortality in patients with cancer (18). We selected 24 hours postoperatively to measure NET biomarkers because previous studies have shown that NETs and MPO formation occur at highest levels on the first postoperative day (12). Higher postoperative levels of NETs are associated with disease progression in cancer surgery (19). Previous studies have revealed that NETs could promote NSCLC metastasis through the NF-κB/NLRP3 inflammatory pathway (7) and facilitate metastasis of circulating lung carcinoma cells to the liver after surgery (8). Our results suggested that both Lido and Dex reduce the formation of NETs. The underlying mechanism might be that Lido and Dex inhibit the activation of the TLR2/NF-κB/NLRP3 pathway, high mobility group box-1 (HMGB-1), and granulocyte-colony stimulating factor (G-CSF) as suggested from the results of inflammation studies (20–22). HMGB-1 and G-CSF are crucial mediators in the initial formation of NETs (23). Another possible mechanism might be the activation by Dex of α2-adrenergic receptors expressed on neutrophils and the consequent desensitization of neutrophils to cytokine activation (24).
In the study by Hunter T et al., continuous perioperative intravenous Lido was shown to reduce NET formation directly (25). In contrast, the impact of Dex on NETs was not immediate (26). These results might explain why Dex alone did not reduce MPO and H3Cit levels as much as Lido alone. Evidence suggests that the intraoperative use of propofol rather than sevoflurane in breast cancer patients reduces the production of NETs, and the addition of Lido reduces NETs even more (13). Our study demonstrated that Lido and Dex could reduce the production of NETs despite the decreased usage of intraoperative propofol.
Lido has been proven to inhibit lung cancer growth through multiple mechanisms in vitro or in vivo (27). Piegeler et al. (28) proved that Lido at clinically relevant concentrations diminished lung adenocarcinoma cell invasion and MMP-9 secretion by vitiated SRC-dependent inflammatory signaling conduction. Our trial revealed that either Lido or Dex decreased the serum levels of MMP-3 and MMP-9 after surgery, and their usage in combination significantly decreased MMP-3. In agreement with our speculation, decreased concentrations of both MMP-3 and MMP-9 were observed, seemingly because the suppression of MMP-3 and TNF-α levels led to a decrease in MMP-9 (29). Favorably for us, Galoș et al. (13) demonstrated that intravenous Lido could promote the postsurgical decrease in MMP-3 expression and NET formation in breast cancer patients. Also in line with our results, Zhang et al. (12) confirmed that intravenous Lido infusion reduced the appearance of NETs and MPO-DNA complexes in blood on POD 1 and POD 3 in pancreatic cancer patients. Furthermore, Zhang’s results ultimately suggested no effects of intravenous Lido perioperatively on the patients’ overall survival (OS) and disease-free survival (DFS). In our findings, Dex did not decrease the level of VEGF-α, but Lido did.
Recent studies have proven that Dex could paradoxically impact lung cancer. Lavon et al. (30) found that Dex increased tumor cell retention and growth of metastases of Lewis lung carcinoma in BALB/c mice. A similar perspective could be seen in Wang’s study (31). However, other investigations proved that treatment with clonidine, an α2- adrenoreceptor agonist, was not associated with shorter DFS or OS after lung cancer surgery (32). In our study, Dex reduced the formation of NETs and the expression of MMP-3 and MMP-9, which might retard the EMT of cancer clinically. However, without long-term follow-up, it is not known whether there was any effect on the patients’ DFS or OS.
The change in IL-6 expression has been proven to be used as an indicator of postoperative stress level (33). It is closely related to the occurrence of cancer-related pain and prognosis in NSCLC patients (34). Our results suggested that intravenous infusion of Lido and Dex could significantly reduce the serum level of IL-6.
Tumor cells rely on immune evasion to augment survival and often benefit from postoperative immunosuppressive states, which are closely related to tumor metastasis (35). Our trial implied that Lido and Dex had a significant impact on the equilibrium of the IFN-γ:IL4 ratio, which was a substitute indicator of the Th1:Th2 ratio. This equilibrium has been recognized as influencing the preservation of cellular immune function and is essential in suppressing tumor metastasis (36). Lido and Dex also increased the CD4+ T-cell ratio, but they did not significantly affect the equilibrium of CD4+ and CD8+ T cells, a result which is similar to the findings of another study (37). The lymphocyte count generally decreased significantly after surgery, and this trend was mitigated by Lido and Dex in this study.
Reducing suppression of immune function in the perioperative period curtails tumor metastasis, and NK cells can eradicate circulating cells that act as critical inhibitors of metastatic spread (38). Previous studies have shown that the use of opioids decreased NK cell function in patients one day after surgery (39). The stability of IFN-γ levels is closely related to the ordinary operation of NK cells (40). The results of our trial showed that Lido and Dex could preserve more NK cells, enhance IFN-γ status, and increase IL-2 levels postoperatively, which was strongly associated with better survival of NSCLC patients (41). This effect might be attributed to lower levels of stress, less consumption of opioids (42) and increased expression of NKG2D receptors on NK cells (37, 43, 44). Our study revealed that Lido and Dex could reduce the dosage of opioids required perioperatively. Studies have shown that intraoperative opioid exposure is associated with poor OS in early-stage NSCLC patients (45), which implies that Lido and Dex might have the ability to improve OS in NSCLC patients.
Our results suggested that in the Lido and Dex groups, the quality of recovery was briefly improved on POD 1 and POD 2, and the VAS scores at rest and at activity were both ephemerally ameliorated on POD 1 as observed in previous trials (12, 46). Once Lido and Dex were discontinued, the pain relief effect disappeared quickly, and the improvement in recovery quality lasted only up to 24 hours after discontinuation. Postoperative pumping of Lido and Dex could not only replace the analgesic effects of opioids but also reduce the frequency and total consumption of postoperative opioids. The opioid-sparing effect observed in our study could bring many benefits for NSCLC patients, such as early recovery of the gastrointestinal tract, early bed-leaving, and early drainage tube removal, without significantly increasing the occurrence of adverse events; this has been indicated by the results of a previous study as well (47).
A previous study proved that the 5-year overall and relapse-free survival rates were significantly worse in NSCLC patients with PPCs than in those without PPCs (48). Our results suggested that the trial group showed a better reduction in NETs in patients who developed PPCs. Although we did not observe differences in PPCs between groups, the initial confirmation of a positive correlation between higher levels of postoperative MPO and PPCs may provide some clues for future studies. In contrast to the results of our study, a previous study showed that intraoperative lidocaine infusion reduces postoperative PPCs (49). The reason for the difference in results may be the different definition of PPCs and the sample size of the study. Like a previous study (50), meaningful results regarding all-cause mortality and readmission rates were not observed in our study due to complications within 30 days after surgery.
While exploring the effects of tumor metastasis biomarkers, inflammatory factors, and cellular immunity on NETs, we found that VEGF-α, MMP-3, and MMP-9 were positively correlated with NETs, which is mechanistically plausible based on previous studies (51, 52). A positive correlation between IL-6 and NETs has been demonstrated in many studies (53, 54). Previous studies have shown that elevated NETs can increase Treg cells (55), and Treg cells decrease the proportion of CD4+ T cells, thereby weakening the body’s antitumor cellular immune function (56). Our results show a negative correlation between CD4+ T cells and NETs in agreement with these studies.
Above all, we hypothesized that adding Lido and Dex to general anesthesia may affect the metastasis and recurrence of NSCLC. This speculation is worthy of further evaluation in a large randomized clinical trial. At the same time, whether NETs can be used as an independent risk factor to predict the prognosis of NSCLC patients, especially those with concomitant postoperative infectious complications or PPCs, deserves further investigation.
5 Limitations
Our study has some limitations. First, the direct effects of Lido and Dex on tumor cells or tissues were not tested, and we have no long-term follow-up data to prove whether Lido and Dex have impacts on the OS or DFS of NSCLC patients. Second, blood samples were not collected at the end of surgery and tested. In addition, intraoperative tumor metastasis biomarkers and stress levels were not evaluated. Meanwhile, the impact of NETs and other tumor metastasis biomarkers on the survival of NSCLC patients remains unknown, and the current evidence is mainly derived from basic experiments. Also, the upstream factor VEGF-α, i.e., the hypoxia-induced factor, was not assessed.
6 Conclusions
This trial is the first study designed to investigate the effect of the perioperative application of Lido and Dex on NETs in NSCLC patients. Perioperative continuous intravenous infusion of Lido and Dex significantly reduced the production of NETs (MPO and H3Cit) and the expression of tumor metastasis biomarkers (VEGF-α, MMP-3, and MMP-9) in the peripheral blood of NSCLC patients. At the same time, Lido and Dex could also decrease inflammation, protect cellular immune function, reduce pain and opioid consumption, and improve the quality of postoperative recovery in NSCLC patients. It would be fruitful to pursue further research about whether the intravenous infusion of Lido and Dex can affect metastasis and recurrence in patients with NSCLC to improve the prognosis of such patients.
Data availability statement
The original contributions presented in the study are included in the article/Supplementary Material. Further inquiries can be directed to the corresponding author.
Ethics statement
The studies involving human participants were reviewed and approved by Ethics Committee of the Affiliated Hospital of Xuzhou Medical University. The patients/participants provided their written informed consent to participate in this study.
Author contributions
BR, MC and CL contributed equally to this work and shared first authorship. They worked on the generation of article ideas, the elaboration of experimental protocols, the implementation of experiments and the process of writing articles. HZ, JYZ, WC and JS were involved in the collection of trial data and clinical samples. JWZ, TL, and RW were involved in the statistical processing of the experimental data and the collection of trial data and clinical samples. ZW was the general manager of the experiment and was involved in the development of the test protocol, the revision of the article and the supervision of the quality of the implementation of the experiment and the implementation process. All authors contributed to the article and approved the submitted version.
Funding
This study was supported by 1. National Natural Science Foundation of China, 82270059; 2. Jiangsu Provincial Health Commission Medical Research Key Project, K2019003; 3. Jiangsu Natural Science Foundation, BK20221222; China Primary Health Care Foundation, YLGX-MZ-2022004; 4. The foundation of Xuzhou Science and Technology Department, KC21154.
Conflict of interest
The authors declare that the research was conducted in the absence of any commercial or financial relationships that could be construed as a potential conflict of interest.
The reviewer FS declared a shared parent affiliation with the author MC to the handling editor at the time of review.
Publisher’s note
All claims expressed in this article are solely those of the authors and do not necessarily represent those of their affiliated organizations, or those of the publisher, the editors and the reviewers. Any product that may be evaluated in this article, or claim that may be made by its manufacturer, is not guaranteed or endorsed by the publisher.
Supplementary material
The Supplementary Material for this article can be found online at: https://www.frontiersin.org/articles/10.3389/fonc.2023.1101449/full#supplementary-material
Supplementary Figure 1 | Linear regression (A) with MPO as the dependent variable; (B) with H3Cit as the dependent variable. Dif., difference; MPO, myeloperoxidase; H3Cit, citrullinated histone 3; VEGF-A, vascular endothelial growth factor-A; MMP-3, matrix metalloproteinase-3; MMP-9, matrix metalloproteinase-9.
References
1. Bray F, Ferlay J, Soerjomataram I, Siegel RL, Torre LA, Jemal A. Global cancer statistics 2018: Globocan estimates of incidence and mortality worldwide for 36 cancers in 185 countries. CA: Cancer J Clin (2018) 68(6):394–424. doi: 10.3322/caac.21492
2. Tohme S, Simmons RL, Tsung A. Surgery for cancer: A trigger for metastases. Cancer Res (2017) 77(7):1548–52. doi: 10.1158/0008-5472.Can-16-1536
3. Cole SW, Nagaraja AS, Lutgendorf SK, Green PA, Sood AK. Sympathetic nervous system regulation of the tumour microenvironment. Nat Rev Cancer (2015) 15(9):563–72. doi: 10.1038/nrc3978
4. Watanabe K, Masuda H, Noma D. Anesthetic and analgesic techniques and perioperative inflammation may affect the timing of recurrence after complete resection for non-Small-Cell lung cancer. Front Surg (2022) 9:886241. doi: 10.3389/fsurg.2022.886241
5. Brinkmann V, Reichard U, Goosmann C, Fauler B, Uhlemann Y, Weiss DS, et al. Neutrophil extracellular traps kill bacteria. Sci (New York NY) (2004) 303(5663):1532–5. doi: 10.1126/science.1092385
6. Demkow U. Neutrophil extracellular traps (Nets) in cancer invasion, evasion and metastasis. Cancers (2021) 13(17):4495. doi: 10.3390/cancers13174495
7. Wang Y, Liu F, Chen L, Fang C, Li S, Yuan S, et al. Neutrophil extracellular traps (Nets) promote non-small cell lung cancer metastasis by suppressing lncrna Mir503hg to activate the nf-Kb/Nlrp3 inflammasome pathway. Front Immunol (2022) 13:867516. doi: 10.3389/fimmu.2022.867516
8. Cools-Lartigue J, Spicer J, McDonald B, Gowing S, Chow S, Giannias B, et al. Neutrophil extracellular traps sequester circulating tumor cells and promote metastasis. J Clin Invest (2013) 123(8):3446–58. doi: 10.1172/jci67484
9. Xiao Y, Cong M, Li J, He D, Wu Q, Tian P, et al. Cathepsin c promotes breast cancer lung metastasis by modulating neutrophil infiltration and neutrophil extracellular trap formation. Cancer Cell (2021) 39(3):423–37.e7. doi: 10.1016/j.ccell.2020.12.012
10. Albrengues J, Shields MA, Ng D, Park CG, Ambrico A, Poindexter ME, et al. Neutrophil extracellular traps produced during inflammation awaken dormant cancer cells in mice. Sci (New York NY) (2018) 361(6409):eaao4227. doi: 10.1126/science.aao4227
11. Pieterse E, Rother N, Garsen M, Hofstra JM, Satchell SC, Hoffmann M, et al. Neutrophil extracellular traps drive endothelial-to-Mesenchymal transition. Arteriosclerosis thrombosis Vasc Biol (2017) 37(7):1371–9. doi: 10.1161/atvbaha.117.309002
12. Zhang H, Qu M, Guo K, Wang Y, Gu J, Wu H, et al. Intraoperative lidocaine infusion in patients undergoing pancreatectomy for pancreatic cancer: A mechanistic, multicentre randomised clinical trial. Br J anaesthesia (2022) 129(2):244–53. doi: 10.1016/j.bja.2022.03.031
13. Galoş EV, Tat TF, Popa R, Efrimescu CI, Finnerty D, Buggy DJ, et al. Neutrophil extracellular trapping and angiogenesis biomarkers after intravenous or inhalation anaesthesia with or without intravenous lidocaine for breast cancer surgery: A prospective, randomised trial. Br J Anaesthesia (2020) 125(5):712–21. doi: 10.1016/j.bja.2020.05.003
14. Lee SH, Kim N, Lee CY, Ban MG, Oh YJ. Effects of dexmedetomidine on oxygenation and lung mechanics in patients with moderate chronic obstructive pulmonary disease undergoing lung cancer surgery: A randomised double-blinded trial. Eur J anaesthesiol (2016) 33(4):275–82. doi: 10.1097/eja.0000000000000405
15. Lee SH, Lee CY, Lee JG, Kim N, Lee HM, Oh YJ. Intraoperative dexmedetomidine improves the quality of recovery and postoperative pulmonary function in patients undergoing video-assisted thoracoscopic surgery: A consort-prospective, randomized, controlled trial. Medicine (2016) 95(7):e2854. doi: 10.1097/md.0000000000002854
16. Miao Z, Wu P, Wang J, Zhou FC, Lin Y, Lu XY, et al. Whole-course application of dexmedetomidine combined with ketorolac in nonnarcotic postoperative analgesia for patients with lung cancer undergoing thoracoscopic surgery: A randomized control trial. Pain physician (2020) 23(2):E185–93.
17. Dicker AJ, Crichton ML, Pumphrey EG, Cassidy AJ, Suarez-Cuartin G, Sibila O, et al. Neutrophil extracellular traps are associated with disease severity and microbiota diversity in patients with chronic obstructive pulmonary disease. J Allergy Clin Immunol (2018) 141(1):117–27. doi: 10.1016/j.jaci.2017.04.022
18. Grilz E, Mauracher LM, Posch F, Königsbrügge O, Zöchbauer-Müller S, Marosi C, et al. Citrullinated histone H3, a biomarker for neutrophil extracellular trap formation, predicts the risk of mortality in patients with cancer. Br J haematol (2019) 186(2):311–20. doi: 10.1111/bjh.15906
19. Erpenbeck L, Schön MP. Neutrophil extracellular traps: Protagonists of cancer progression? Oncogene (2017) 36(18):2483–90. doi: 10.1038/onc.2016.406
20. Wang L, Wang M, Li S, Wu H, Shen Q, Zhang S, et al. Nebulized lidocaine ameliorates allergic airway inflammation Via downregulation of Tlr2. Mol Immunol (2018) 97:94–100. doi: 10.1016/j.molimm.2018.03.010
21. Zheng X, Li J, Fan Q, Zhao X, Chen K. Dexmedetomidine alleviates myocardial Ischemia/Reperfusion-induced injury and Ca(2+) overload Via the microrna-346-3p/Camkiid axis. Int J Cardiol (2021) 338:185–95. doi: 10.1016/j.ijcard.2021.03.016
22. Wang HL, Liu YY, Yan HD, Wang XS, Huang R, Lei WF. Intraoperative systemic lidocaine inhibits the expression of Hmgb1 in patients undergoing radical hysterectomy. Int J Clin Exp Med (2014) 7(10):3398–403.
23. Snoderly HT, Boone BA, Bennewitz MF. Neutrophil extracellular traps in breast cancer and beyond: Current perspectives on net stimuli, thrombosis and metastasis, and clinical utility for diagnosis and treatment. Breast Cancer Res BCR (2019) 21(1):145. doi: 10.1186/s13058-019-1237-6
24. Herrera-García AM, Domínguez-Luis MJ, Arce-Franco M, Armas-González E, Álvarez de la Rosa D, Machado JD, et al. Prevention of neutrophil extravasation by A2-Adrenoceptor-Mediated endothelial stabilization. J Immunol (Baltimore Md 1950) (2014) 193(6):3023–35. doi: 10.4049/jimmunol.1400255
25. Finnerty DT, Buggy DJ. A novel role for lidocaine in covid-19 patients? Br J anaesthesia (2020) 125(4):e391–4. doi: 10.1016/j.bja.2020.07.015
26. Corriden R, Schmidt BE, Olson J, Okerblom J, Masso-Silva JA, Nizet V, et al. Dexmedetomidine does not directly inhibit neutrophil extracellular trap production. Br J Anaesthesia (2022) 128(2):e51–4. doi: 10.1016/j.bja.2021.11.015
27. Freeman J, Crowley PD, Foley AG, Gallagher HC, Iwasaki M, Ma D, et al. Effect of perioperative lidocaine, propofol and steroids on pulmonary metastasis in a murine model of breast cancer surgery. Cancers (2019) 11(5):613. doi: 10.3390/cancers11050613
28. Piegeler T, Schläpfer M, Dull RO, Schwartz DE, Borgeat A, Minshall RD, et al. Clinically relevant concentrations of lidocaine and ropivacaine inhibit tnfα-induced invasion of lung adenocarcinoma cells in vitro by blocking the activation of akt and focal adhesion kinase. Br J Anaesthesia (2015) 115(5):784–91. doi: 10.1093/bja/aev341
29. Tu YA, Chou CH, Yang PK, Shun CT, Wen WF, Tsao PN, et al. Intentional endometrial injury enhances angiogenesis through increased production and activation of mmp-9 by tnf-A and mmp-3 in a mouse model. Mol Hum Reprod (2021) 27(10):gaab055. doi: 10.1093/molehr/gaab055
30. Lavon H, Matzner P, Benbenishty A, Sorski L, Rossene E, Haldar R, et al. Dexmedetomidine promotes metastasis in rodent models of breast, lung, and colon cancers. Br J Anaesthesia (2018) 120(1):188–96. doi: 10.1016/j.bja.2017.11.004
31. Wang C, Datoo T, Zhao H, Wu L, Date A, Jiang C, et al. Midazolam and dexmedetomidine affect neuroglioma and lung carcinoma cell biology in vitro and in vivo. Anesthesiology (2018) 129(5):1000–14. doi: 10.1097/aln.0000000000002401
32. Forget P, Berlière M, Poncelet A, De Kock M. Effect of clonidine on oncological outcomes after breast and lung cancer surgery. Br J Anaesthesia (2018) 121(1):103–4. doi: 10.1016/j.bja.2018.04.020
33. Angka L, Khan ST, Kilgour MK, Xu R, Kennedy MA, Auer RC. Dysfunctional natural killer cells in the aftermath of cancer surgery. Int J Mol Sci (2017) 18(8):1787. doi: 10.3390/ijms18081787
34. Liu Y, Gao Y, Lin T. Expression of interleukin-1 (Il-1), il-6, and tumor necrosis factor-A (Tnf-A) in non-small cell lung cancer and its relationship with the occurrence and prognosis of cancer pain. Ann palliative Med (2021) 10(12):12759–66. doi: 10.21037/apm-21-3471
35. Horowitz M, Neeman E, Sharon E, Ben-Eliyahu S. Exploiting the critical perioperative period to improve long-term cancer outcomes. Nat Rev Clin Oncol (2015) 12(4):213–26. doi: 10.1038/nrclinonc.2014.224
36. Wada H, Seki S, Takahashi T, Kawarabayashi N, Higuchi H, Habu Y, et al. Combined spinal and general anesthesia attenuates liver metastasis by preserving Th1/Th2 cytokine balance. Anesthesiology (2007) 106(3):499–506. doi: 10.1097/00000542-200703000-00014
37. Wang K, Wu M, Xu J, Wu C, Zhang B, Wang G, et al. Effects of dexmedetomidine on perioperative stress, inflammation, and immune function: Systematic review and meta-analysis. Br J Anaesthesia (2019) 123(6):777–94. doi: 10.1016/j.bja.2019.07.027
38. Ackerman RS, Luddy KA, Icard BE, Piñeiro Fernández J, Gatenby RA, Muncey AR. The effects of anesthetics and perioperative medications on immune function: A narrative review. Anesth Analgesia (2021) 133(3):676–89. doi: 10.1213/ane.0000000000005607
39. Narahara H, Kadoi Y, Hinohara H, Kunimoto F, Saito S. Comparative effects of flurbiprofen and fentanyl on natural killer cell cytotoxicity, lymphocyte subsets and cytokine concentrations in post-surgical intensive care unit patients: Prospective, randomized study. J Anesth (2013) 27(5):676–83. doi: 10.1007/s00540-013-1597-5
40. Ahlers O, Nachtigall I, Lenze J, Goldmann A, Schulte E, Höhne C, et al. Intraoperative thoracic epidural anaesthesia attenuates stress-induced immunosuppression in patients undergoing major abdominal surgery. Br J anaesthesia (2008) 101(6):781–7. doi: 10.1093/bja/aen287
41. Neuner A, Schindel M, Wildenberg U, Muley T, Lahm H, Fischer JR. Prognostic significance of cytokine modulation in non-small cell lung cancer. Int J Cancer (2002) 101(3):287–92. doi: 10.1002/ijc.10604
42. Beilin B, Shavit Y, Hart J, Mordashov B, Cohn S, Notti I, et al. Effects of anesthesia based on Large versus small doses of fentanyl on natural killer cell cytotoxicity in the perioperative period. Anesth Analgesia (1996) 82(3):492–7. doi: 10.1097/00000539-199603000-00011
43. Cata JP, Ramirez MF, Velasquez JF, Di AI, Popat KU, Gottumukkala V, et al. Lidocaine stimulates the function of natural killer cells in different experimental settings. Anticancer Res (2017) 37(9):4727–32. doi: 10.21873/anticanres.11879
44. Ramirez MF, Tran P, Cata JP. The effect of clinically therapeutic plasma concentrations of lidocaine on natural killer cell cytotoxicity. Regional Anesth Pain Med (2015) 40(1):43–8. doi: 10.1097/aap.0000000000000191
45. Connolly JG, Tan KS, Mastrogiacomo B, Dycoco J, Caso R, Jones GD, et al. Intraoperative opioid exposure, tumour genomic alterations, and survival differences in people with lung adenocarcinoma. Br J Anaesthesia (2021) 127(1):75–84. doi: 10.1016/j.bja.2021.03.030
46. Foo I, Macfarlane AJR, Srivastava D, Bhaskar A, Barker H, Knaggs R, et al. The use of intravenous lidocaine for postoperative pain and recovery: International consensus statement on efficacy and safety. Anaesthesia (2021) 76(2):238–50. doi: 10.1111/anae.15270
47. Wang L, Sun J, Zhang X, Wang G. The effect of lidocaine on postoperative quality of recovery and lung protection of patients undergoing thoracoscopic radical resection of lung cancer. Drug Design Dev Ther (2021) 15:1485–93. doi: 10.2147/dddt.S297642
48. Okada S, Shimomura M, Ishihara S, Ikebe S, Furuya T, Inoue M. Clinical significance of postoperative pulmonary complications in elderly patients with lung cancer. Interactive Cardiovasc Thorac Surg (2022) 35(2):ivac153. doi: 10.1093/icvts/ivac153
49. Wang X, Guo K, Zhao Y, Li T, Yang Y, Xu L, et al. Lung-protective effects of lidocaine infusion on patients with intermediate/ high risk of postoperative pulmonary complications: A double-blind randomized controlled trial. Drug Design Dev Ther (2022) 16:1041–53. doi: 10.2147/dddt.S358609
50. Hou YH, Shi WC, Cai S, Liu H, Zheng Z, Qi FW, et al. Effect of intravenous lidocaine on serum interleukin-17 after video-assisted thoracic surgery for non-Small-Cell lung cancer: A randomized, double-blind, placebo-controlled trial. Drug Design Dev Ther (2021) 15:3379–90. doi: 10.2147/dddt.S316804
51. Jaillon S, Ponzetta A, Di Mitri D, Santoni A, Bonecchi R, Mantovani A. Neutrophil diversity and plasticity in tumour progression and therapy. Nat Rev Cancer (2020) 20(9):485–503. doi: 10.1038/s41568-020-0281-y
52. Poto R, Cristinziano L, Modestino L, de Paulis A, Marone G, Loffredo S, et al. Neutrophil extracellular traps, angiogenesis and cancer. Biomedicines (2022) 10(2):431. doi: 10.3390/biomedicines10020431
53. Ohyama A, Osada A, Kawaguchi H, Kurata I, Nishiyama T, Iwai T, et al. Specific increase in joint neutrophil extracellular traps and its relation to interleukin 6 in autoimmune arthritis. Int J Mol Sci (2021) 22(14):7633. doi: 10.3390/ijms22147633
54. Navrátilová A, Bečvář V, Baloun J, Damgaard D, Nielsen CH, Veigl D, et al. S100a11 (Calgizzarin) is released Via netosis in rheumatoid arthritis (Ra) and stimulates il-6 and tnf secretion by neutrophils. Sci Rep (2021) 11(1):6063. doi: 10.1038/s41598-021-85561-3
55. Wang H, Zhang H, Wang Y, Brown ZJ, Xia Y, Huang Z, et al. Regulatory T-cell and neutrophil extracellular trap interaction contributes to carcinogenesis in non-alcoholic steatohepatitis. J Hepatol (2021) 75(6):1271–83. doi: 10.1016/j.jhep.2021.07.032
Keywords: lidocaine, dexmedetomidine, tumor, metastasis, lung cancer, neutrophil extracellular traps
Citation: Ren B, Cheng M, Liu C, Zheng H, Zhang J, Chen W, Song J, Zhuang J, Liu T, Wang R and Wang Z (2023) Perioperative lidocaine and dexmedetomidine intravenous infusion reduce the serum levels of NETs and biomarkers of tumor metastasis in lung cancer patients: A prospective, single-center, double-blinded, randomized clinical trial. Front. Oncol. 13:1101449. doi: 10.3389/fonc.2023.1101449
Received: 17 November 2022; Accepted: 14 February 2023;
Published: 24 February 2023.
Edited by:
Peixin Dong, Hokkaido University, JapanReviewed by:
Stephen Gowing, University of Manitoba, United StatesF. Sun, Shanghai Jiao Tong University, China
Copyright © 2023 Ren, Cheng, Liu, Zheng, Zhang, Chen, Song, Zhuang, Liu, Wang and Wang. This is an open-access article distributed under the terms of the Creative Commons Attribution License (CC BY). The use, distribution or reproduction in other forums is permitted, provided the original author(s) and the copyright owner(s) are credited and that the original publication in this journal is cited, in accordance with accepted academic practice. No use, distribution or reproduction is permitted which does not comply with these terms.
*Correspondence: Zhiping Wang, emhwc3F4dEAxMjYuY29t
†These authors have contributed equally to this work and share first authorship