- 1Department of Health Science, South College, Nashville, TN, United States
- 2Department of Surgery, Vanderbilt University Medical Center, Nashville, TN, United States
- 3Vanderbilt-Ingram Cancer Center, Vanderbilt University Medical Center, Nashville, TN, United States
Gastrointestinal (GI) cancers that include all cancers of the digestive tract organs are generally associated with obesity, lack of exercising, smoking, poor diet, and heavy alcohol consumption. Treatment of GI cancers typically involves surgery followed by chemotherapy and/or radiation. Unfortunately, intrinsic or acquired resistance to these therapies underscore the need for more effective targeted therapies that have been proven in other malignancies. The aggressive features of GI cancers share distinct signaling pathways that are connected to each other by the overexpression and activation of AXL receptor tyrosine kinase. Several preclinical and clinical studies involving anti-AXL antibodies and small molecule AXL kinase inhibitors to test their efficacy in solid tumors, including GI cancers, have been recently carried out. Therefore, AXL may be a promising therapeutic target for overcoming the shortcomings of standard therapies in GI cancers.
Introduction
Increasing cancer risk factors linked to emerging economy and globalization have aggravated the global cancer burden with an expected 47% increase of incidence in 2040 relative to 2020 (1). The rising disease burden caused by the malignancies of the digestive system has become one of the major public health challenges. Particularly, colorectal (10%) stomach (5.6%), esophageal (3.1%), liver (8.3%), and pancreatic (4.7%) cancers are among the most diagnosed malignancies after female breast and lung cancers (11.7% and 11.4% accordingly) (1, 2). Therefore, there is a critical need for identifying reliable molecular markers and targets for gastrointestinal (GI) oncotherapies. For the last decade, AXL receptor tyrosine kinase, also known as UFO, attracted a substantial interest in cancer biology because of the progressively accumulated data demonstrating the ability of this protein to regulate cell survival, proliferation, and motility in normal and cancer tissues (3–8). The selective overexpression of AXL in GI malignancies is associated with a poor clinical prognosis (9–11), proliferation (10, 12, 13), metastasis (14), immunosuppressive tumor microenvironment (3, 15, 16), and drug resistance (17, 18). This review provides a comprehensive update on the current research initiatives highlighting AXL as a promising therapeutic target and a novel diagnostic and prognostic marker of GI cancers. The research findings from preclinical and clinical studies on the evaluation of drugs in targeting the AXL-mediated signaling pathways in GI cancers are reviewed.
AXL function and signaling
AXL protein (100 - 140 kDa) belongs to the receptor tyrosine kinase (RTK) subfamily of transmembrane receptors TAM, which comprises TYRO3 (19), AXL (20), and MER (21–23). Initially, AXL was identified as a transforming gene in patients with chronic myelogenous leukemia (24). Later, the names AXL (from the Greek “anexelekto”, meaning “uncontrolled”) and UFO were given concurrently to the same cDNA encoding an RTK overexpressed in human myeloid leukemia cells (25, 26) and NIH3T3 mouse fibroblasts transfected with DNA from a patient with a chronic myeloproliferative disorder (20, 27). TAM family of RTKs is characterized by a combination of two immunoglobin-like domains and fibronectin type III domains in the extracellular (N-terminal) region. AXL also has an intracellular (C-terminal) tyrosine kinase domain, which plays an essential role in signal transduction (28). The vitamin k-dependent growth arrest-specific protein 6 (Gas6) (29) serves as a high affinity ligand for AXL (21, 30, 31). Gas6 binding to AXL primes the homodimerization of receptor with another Gas6/AXL ligand-receptor complex and autophosphorylation of three tyrosine residues (32). This set of reactions initiates the recruitment of p85 subunit of phosphoinositide-3 kinase (PI3K), phospholipase C-γ (PLCγ), or growth factor receptor–bound protein 2 (Grb2) and activate the relevant downstream signaling pathways involved in survival, proliferation, or migration (33, 34). Notably, the activation of AXL is negatively regulated by the binding of its soluble form sAXL to Gas6 (34, 35). Important physiological functions of the Gas6/AXL pathway include cell migration and survival (36), adhesion (37), and suppression of apoptosis (38) in inflammatory, endothelial, and smooth muscle cells. Additionally, the Gas6/AXL signaling plays an important role in the activation of macrophages and phagocytosis (39).
AXL expression in GI cancers (esophagus, stomach, pancreas, liver and colon)
Since genetic modifications of AXL gene, such as rearrangement, amplification, or mutations, are relatively rare (40, 41), the AXL functions in GI cancers are likely determined by the level of its expression. High expression of AXL has been reported in a variety of primary GI tumors and metastases and linked to poor clinical prognosis (Table 1) (11, 42–45). Invasive esophageal adenocarcinoma (EAC) frequently progresses from a premalignant condition, gastroesophageal reflux disease-associated Barrett’s esophagus (BE). AXL expression is linked to adverse prognosis in EAC (11) as well as poor prognosis and distant metastases in esophageal squamous cell carcinoma (43). Particularly, serial analysis of gene expression (SAGE) indicated a significant upregulation of AXL “tags” in metachronous mucosal biopsy samples obtained from a patient progressed from BE to EAC (11). Moreover, both univariate and multivariate analyses of 92 surgically resected sections of EAC demonstrated a positive correlation of AXL overexpression with decreased median survival of the patients (11). Elevated expression of AXL and p-AXL (Y779) proteins was detected by immunoblot analysis in human EAC cell lines SK-GT-4, FLO-1, and JH-EsoAd1 as compared to normal esophageal squamous epithelial cell lines (18). Immunohistochemical (IHC) staining with anti-AXL specific antibody of tissue microarrays indicated AXL overexpression in 51.8% of EAC tumors relative to normal esophageal squamous tissue specimens (18). The results of a further IHC analysis performed on tissue microarrays including 53 human EAC and 11 normal esophageal tissues revealed that AXL as well as another potentially prooncogenic molecule non-receptor tyrosine kinase c-ABL were overexpressed in 55% and 66% of EAC samples, respectively, as compared to normal tissue specimens (46). Moreover, co-overexpression of AXL and c-ABL was detected in 49% of EAC samples (46).
High mRNA and protein expression of Gas6 and AXL has been reported in human gastric cancer cell lines and tissues (45). Notably, Gas6 expression was significantly correlated with lymph node metastases (45).
The immunohistochemical evaluation of expression of AXL protein in a panel of 99 archival pancreatic cancers revealed AXL expression in 54 out of 99 specimens (55%); and positive AXL expression in pancreatic cancer was significantly associated with lymph node metastases and a shorter median survival (12 as opposed to 18 months) as compared to AXL-negative tumor samples (50). Frequent overexpression of both molecules, Gas6 and AXL, has been detected in Pancreatic Ductal Adenocarcinoma (PDA) cells and was linked to a poor prognosis in patients with stage II PDA (13).
Additionally, high AXL mRNA and protein expression levels were associated with poor overall survival in early-stage colorectal cancer (CRC) tissues (42). Particularly, the statistical analysis of CRC microarray dataset, available through the Gene Expression Omnibus (GEO) (52), showed a significant association between high AXL mRNA expression and decreased disease-specific survival in a cohort of 177 patients diagnosed with an early-stage (stage II/III) CRC (42). Furthermore, AXL overexpression in colorectal adenocarcinoma as compared to normal colon tissues was demonstrated by IHC in tissue microarray resection specimens of primary tumors collected from 509 patients with colorectal adenocarcinoma (stage I-IV) at the National University Hospital of Singapore between 1990 and 1999 (42). Likewise, the overexpression of AXL and GAS6 was shown by IHC in 76,7% and 73.5%, respectively, in 223 human CRC specimens, while the amplification of AXL gene was detected by fluorescence in situ hybridization (FISH) in 8 out of 146 cases (5,4%) of CRC samples (51). The increased expression of AXL and GAS6 proteins was correlated with less differentiated histological grading, tumor stage and lymph nodes involvement (51). Given that majority of patients with high-risk stage II/III CRC tend to relapse (53) and progress to the advanced stages, AXL could be used as a prognostic biomarker for the distal part of GI tract.
While all known methods to evaluate AXL expression include tissue extraction, in some forms of hepatic neoplasm, it is possible to assess clinical outcome by evaluating plasma levels of soluble AXL (sAXL). It is an 85 kDa N-terminal product of extracellular ADAM metalloproteases-dependent proteolytic cleavage of AXL, which has GAS6 ligand-binding abilities and serving as a decoy receptor (26, 54, 55). This circulating sAXL has a promising potential as a specific serum marker of cirrhosis and early stages of hepatocellular carcinoma (HCC) (49). It has been documented that serum concentrations of sAXL were elevated at early (82.57 ng/mL) and later stages (114.50 ng/mL) of HCC in comparison with healthy controls (40.15 ng/mL) (49). Notably, sAXL levels were not altered in patients with chronic liver disease, liver adenomas and cholangiocarcinomas (49). These data suggest that elevated concentration of sAXL is a valuable biomarker of liver neoplastic transformation that could be noninvasively detected in plasma. In another study analyzing the diagnostic potential of this molecule, sAXL levels were evaluated in 311 HCC and 237 control serum samples collected from clinical centers in Europe and China (56). Average concentrations of sAXL were significantly higher in the serum of HCC patients (18.575 ng/mL) as compared to healthy (13.388 ng/mL) or cirrhotic (12.169 ng/mL) controls (56). Levels of sAxl remained unchanged in the serum of individuals diagnosed with primary ovarian, colorectal and breast carcinomas, or secondary colon-derived hepatic malignancies. Consequently, the soluble form of AXL was suggested as highly specific and accurate diagnostic marker for alpha-fetoprotein-negative HCC patients (56). Additionally, sAXL was proposed as a biomarker for early diagnosis of PADC based on the studies Martinez-Bosch, N. et al, 2022, which demonstrated increased sAXL levels in plasma of PDAC group as compared to healthy controls or chronic pancreatitis (CP) patients. Immunohistochemical analysis revealed higher protein expression in tissues samples obtained from PDAC and precancerous lesions as compared to CP or healthy control specimens. The immunohistochemistry data was confirmed by RNA expression analysis from TCGA database. It was noted that patients with high levels of AXL have a lower overall survival. Importantly, ROC statistical analysis of the plasma levels of sAXL, GAS6, or CA19-9 (a marker of pancreatic cancer) in two studied cohorts revealed that sAXL outperformed CA19-9 for discriminating between CP and PDAC (57). The data showing increased AXL expression in GI cancer tissues are summarized in Table 1.
The mechanisms leading to AXL overexpression are tissue-specific and may vary depending on local tissue microenvironment. Irrespective of AXL localization, the alteration of patterns of this molecule expression could be considered a hallmark of GI carcinogenesis. The specific roles of AXL in the alteration of basic cell functions in GI cancers are discussed below.
Proliferation and survival
Initial steps in carcinogenesis are associated with uncontrolled proliferation and survival of transformed or cancer stem cells (58). Gas6-AXL signaling pathway has been shown to enhance cell survival and suppress apoptosis in gastric cancer cells through activation of the AKT pathway (45). In another study, YAP-dependent cell survival and proliferation required AXL expression and activation of ERK1/2 signaling cascade in human HCC (59). Additionally, the proliferation of a metastatic HCC in vitro and in vivo was markedly suppressed by tunicamycin-induced de-glycosylation and downregulation of AXL (60).
In pancreatic ductal adenocarcinoma, the upregulation of AXL has been associated with a poor clinical prognosis and increased cell proliferation (12, 13), while stable knockdown of AXL resulted in a significant reduction in cell viability and anchorage-independent growth in pancreatic cancer cells (50). In a preclinical study, treatment with S49076, an ATP-competitive tyrosine kinase inhibitor of MET, AXL, and FGFR1, significantly inhibited colony formation in soft agar by HCC cells overexpressing AXL and FGFR2 (61). The lack of sensitivity to S49076 in the same cell lines cultured in monolayer (61) suggests a key role of AXL in extracellular matrix anchorage-independent growth and survival. Indeed, the results of currently available preclinical and clinical studies suggest that the primary roles of AXL and other TAM RTKs may be mostly related to the mechanisms of survival, motility, and drug resistance rather than functioning as oncogenic drivers (62, 63).
Epithelial–mesenchymal transition and metastasis
The epithelial–mesenchymal transition (EMT) is a process by which epithelial cells undergo morphological and functional changes towards a mesenchymal phenotype (64). During this process of trans-differentiation, epithelial cells lose their polarity as well as cell-cell adhesion properties and acquire characteristics of mesenchymal stem cells (65). Cancer cells detach from the primary tumor location, migrate through the extracellular matrix, and intravasate into blood vessels, promoting metastases (66, 67). Importantly, residual metastatic disease from the primary tumor remains the major reason of recurrence and greater than 90% of cancer-related death (68). In cancers of the digestive system, AXL overexpression in tumors and metastases indicates adverse clinical prognosis in patients (9, 10, 42–44, 51, 69).
EMT associated with intrahepatic metastasis is a typical feature of HCC (48). Several studies in HCC have demonstrated elevated levels of AXL transcript and protein in association with EMT (48, 56, 59). For example, AXL mRNA overexpression and correlation with EMT has been documented in 28 HCC cell lines and 373 RNA-seq tissue datasets in comparison with cirrhotic and normal liver samples (70). A crucial role of AXL in transforming growth factor beta (TGF-β)-dependent HCC progression was proposed based on the studies revealing upregulation and activation of AXL in EMT-altered hepatoma cells (48). At the same time, AXL activation by Gas-6 increased TGF-β1 mRNA, while AXL knockdown dramatically reduced resistance to TGF-β-dependent growth inhibition by abrogating invasion and trans-endothelial migration of mesenchymal HCC cells (48). Notably, AXL overexpression triggered metastatic colonization of epithelial hepatoma cells in vivo. Immunohistochemical analysis of AXL expression in tumor tissues collected from 133 HCC patients demonstrated a correlation of increased AXL expression with advanced tumor stages, augmented vessel invasion of HCC cells, elevated risk of cancer relapse after liver transplantation, and a poor clinical prognosis (48). One of the most severe metastatic complications in HCC is portal vein tumor thrombus (PVTT). It has been shown that co-implantation of human umbilical vein endothelial cells (HUVECs) overexpressing AXL with HCC cells in xenograft nude mice and patient-derived xenograft (PDX) nude mice substantially enhanced tumor growth, hepatic metastasis, and vessel metastasis of HCC (71). These effects were suppressed by an AXL inhibitor R428, also known as BGB324 or bemcentinib (71).
Several studies suggested AXL as a potential therapeutic target in pancreatic cancer (13, 50, 72, 73). Thus, the immunohistochemical assessment of 99 pancreatic cancer specimens revealed a higher number of lymph node metastases and a shorter median survival of patients with AXL-positive tumors (12 versus 18 months) in contrast to the AXL-negative group (50). Stable knockdown of endogenous AXL in pancreatic cancer cells resulted in a significant decrease of mRNA levels of matrix metalloproteinase (MMP)-9 and EMT-associated transcription factors twist, snail, and slug (50). Moreover, AXL knockdown cells exhibited reduction in cell viability, migration, and invasion (50). The role of AXL signaling in progression and metastasis of pancreatic cancer was confirmed in a study using low-dose warfarin, a vitamin K “antagonist” to inhibit Gas6-dependent AXL activation (72). Treatment with low-dose warfarin reduced AXL-mediated human pancreatic cancer cells migration, invasiveness, and proliferation, while increasing apoptosis and sensitivity to chemotherapy. Additionally, warfarin decreased primary tumor growth and suppressed metastases in a murine model of pancreatic ductal adenocarcinoma (PDAC) (72). On a molecular level, low-dose warfarin treatment blocked TGFβ-induced expression of AXL, and markedly reduced expression levels of mesenchymal markers, Zeb1 and nuclear β-catenin in Panc-1 pancreatic epithelioid carcinoma cell line. Consistently, warfarin inhibited expression of vimentin and increased levels of E-cadherin in AXL-positive Panc-1 xenografts (72).
AXL signaling axis is also implicated in EMT of GI cancers. For instance, high levels of Gas6 and AXL mRNA and proteins were revealed in human gastric cancer cell lines and tissue samples, and Gas6 expression was significantly correlated with metastases to lymph nodes (45). The in vitro experiments using recombinant Gas6 and a decoy-receptor of AXL showed that activation of Gas6-AXL signaling axis leads to the inhibition of apoptosis and exacerbation of AKT-dependent survival and invasion of gastric cancer cells (45). In another study, inhibition of AXL-NF-κB signaling pathway by ursolic acid markedly inhibited cell migration and reduced the expression of mesenchymal markers and EMT-related transcription factors in gastric cancer cells and xenografts (74). In EAC cells, genetic silencing of AXL attenuated invasion, migration, and in vivo engraftment. Furthermore, pharmacological inhibition of AXL with small molecule agent R428 has shown similar functional effects in EAC cells (11). Our studies in EAC cell lines demonstrated that increased expression of AXL facilitates peripheral distribution of lysosomes leading to activation of cell invasion signaling cascade through the regulation of cathepsin B secretion (75). Besides, we found that these processes were caused by extracellular acidification because of AXL-induced secretion of lactate through AKT-NF-κB–dependent synthesis of lactate transporter MCT-1 (75).
Recently, dual inhibition of TGFβ and AXL signaling pathways was proposed as a novel therapy for human colorectal adenocarcinoma with mesenchymal phenotype (CMS4), a very aggressive CRC characterized by resistance to standard chemotherapies, low survival rate and high risk of recurrence (76, 77). In fact, overexpression of AXL and TGFβ receptors in CMS4 tumors correlated with higher risk of post-surgical relapse in stage II/III CRC and decreased survival (76). In CRC cell lines, treatment with the TGFβ inhibitor, galunisertib, and the AXL inhibitor, R428, markedly reduced colony formation and migration of cancer cells, and demonstrated potent anti-tumor activity in 3D spheroid cultures obtained from individuals with advanced CRC (76). Additionally, multitarget tyrosine kinase inhibitor (TKI) cabozantinib and AXL/c-MET selective inhibitor R428 both decreased AXL phosphorylation and TGFβ-induced E-cadherin expression (marker of EMT), cell viability, migration, and tumor growth in esophageal squamous cell carcinoma (ESCC) cells and xenograft models (78).
Interestingly, AXL expression was upregulated by Long non-coding RNA (lncRNA) CALIC in complex with RNA-binding protein hnRNP-L in colon cancer cells, while knockdown of either CALIC or AXL inhibited metastases in vivo (14). Application of AXL expression as a marker of poor prognosis and a crucial mediator of cell invasion was proposed for early-stage CRC, specifically in the adjuvant disease in the cases of unsuccessful EGFR/VEGF–targeted therapies (42).
AXL in angiogenesis
Angiogenesis is the formation of new blood vessels that often promote tumor growth and progression. AXL regulates many angiogenic activities such as proliferation and migration of vascular smooth muscle cells (VSMCs) and endothelial cells (ECs) (36), tube formation in vitro and angiogenesis in vivo (3). Vascular endothelial growth factor (VEGF) is secreted in high levels by most types of cancer cells (79). Proliferation and migration of VSMC are necessary for tumor angiogenesis (80). In fact, VSMCs express Gas6, and exogenous Gas6 promotes proliferation and migration of VSMCs (36). AXL also is expressed by tumor stromal cells, including ECs (7, 81). Knockdown of AXL or Gas6 expression markedly inhibited migration of HUVECs, while AXL overexpression enhanced cell growth and tubes formation (3). Notably, overexpression of AXL expression was observed in HCC-tumor-derived endothelial cells (TECs), although not in the tumor cells of HCC patients with portal vein tumor thrombus (PVTT) type of metastases. These data were associated with poor overall survival and disease-free survival of HCC patients with PVTT (71). Moreover, elevated expression of AXL was associated with the expression of a marker of endothelial cells CD 31 in vitro and in vivo (71).
Interestingly, Axl-null mice exhibited an impaired angiogenesis and vascular permeability in response to VEGF-A treatment (82). Therefore, it has been proposed that AXL could be one of the essential mediators of VEGF-A-dependent activation of pro-angiogenic PI3K/AKT signaling pathway (82). Accordingly, using AXL inhibitors in addition to anti-VEGF therapeutics could be an effective strategy targeting neovascularization in GI cancers.
AXL in the immune response to tumors
Inflammation is a one of the hallmarks of carcinogenesis, and it has been proven that chronic inflammation caused by autoimmune gastritis and Helicobacter pylori infection increases a risk of developing gastric cancer (83). In fact, more than 90% of gastric adenocarcinomas originate from epithelial cells of gastric mucosa because of chronic inflammation (83). Tumor intrinsic and immunosuppressive mechanisms contribute to conventional chemotherapy resistance (84). TAM family of RTKs, including AXL, are key regulators of immune response (85). Following their activation by Gas6 ligand – activator of all TAM family members, and Protein S ligand -activator of both MER and TYRO3, these receptors promote the resolution of inflammation by suppressing activation of cells of the innate immune system (86). Remarkably, studies on Tyro3−/−Axl−/−Mer−/− triple mutant mice (TAM TKOs) have demonstrated that loss of function of the three receptors, Tyro3, Axl, and Mer, dysregulates the immune system, presented by a severe lymphoproliferative disorder accompanied by a broad-spectrum autoimmune disease (39, 87). In a cancer setting, TAM receptors regulate the initiation and progression of tumorigenesis and, simultaneously, the anti-tumor functions of immune cells (85). Tumor progression is considerably affected by the tumor microenvironment (TME), comprised of all host cells and tissue components surrounding the cancer cells (88). On the other hand, programmed cell death through apoptosis maintains tissue homeostasis and prevents oncogenic transformation. Clearance of cell debris is the last stage of apoptosis. Uncleared products of this process might induce necrosis, thereby promoting inflammation and autoimmunity (89). Externalized phosphatidylserine (PS) acts as “eat-me” signal on apoptotic cells, stressed cells, exosomes, and liposomes. Importantly, endogenous ligands Gas6 and Protein S link externalized PS molecules with TAMs, activating those RTKs and promoting clearance of apoptotic cells (90, 91).
Studies on animal models have shown that TAM family receptors are involved in the clearance of apoptotic cells by macrophages and dendritic cells (DC) (92, 93). In fact, treatment with dextran sulfate sodium (DSS) salt blocked clearance of apoptotic neutrophils in the lamina propria of large intestine and promoted colitis in Axl−/−Mer−/− double mutant mice (94). The Authors demonstrated that the observed inflammatory phenotype is associated with the knockout of Axl and Mer genes in radioresistant population of macrophages residing specifically in the intestinal tissues, while loss of Axl and Mer in the radiosensitive bone marrow–derived hematopoietic cells was not linked to exacerbated colitis (94). As such, AXL and MERTK inhibitors might induce adverse effects at the systemic level, and physiological effects of the alteration of AXL and MERTK signaling could be highly tissue-specific and depend on tumor microenvironment.
Tumor-associated macrophages are abundant in the TME and contribute to immunosuppression and tumor progression (92). In human and murine macrophage cultures, AXL activation has been shown to mediate Interferon α induction of Twist, a transcriptional repressor of inflammatory cytokine tumor necrosis factor α (TNFα) (95). Altering AXL expression and downstream activation of Twist highlights a promising approach to control inflammation, which is the hallmark of oncogenesis. In several studies, activation of TAM receptors not only decreased severe inflammatory responses (96), but also induced efferocytosis and macrophage polarization towards a pro-tumor M2-like phenotype, accompanied by the increased production of immunosuppressive cytokines (92, 97–99). AXL induced TANK binding kinase 1 (TBK1)-NF-κB signaling pathway and innate immune suppression in the TME in pancreatic cancer (73), while inhibition of AXL with small molecule R428 enhanced immune stimulatory microenvironment (73). Immune checkpoint blockade (PD-1) is a novel popular approach in cancer immunotherapies. Unfortunately, some of the tumors are resistant to PD-1 inhibitors and considered to be immunologically “cold,” because of the lack of tumor antigen-specific primed cytotoxic T cells (99). It has been shown that Sitravatinib, a broad-spectrum tyrosine kinase inhibitor (TKI) targeting MET, TAM, and members of VEGFR, platelet-derived growth factor receptor (PDGFR), and Eph families is highly effective in various cancer models, including CT1B-A5, an isogenic pancreatic cancer cell line, that could be partially attributed to altering the TME and restoring the efficacy of immune checkpoint blockade (PD-1) (99). Therefore, AXL is one of the major drivers of immune suppression in the TME. Although AXL-mediated pathway is an attractive candidate for inhibition in GI cancers to reverse the immunosuppressive TME, further investigations are needed as this therapeutic approach may cause adverse systemic effects like inflammation and autoimmunity.
AXL in resistance to anti-cancer therapies
One of the major problems of anti-cancer therapies is that many cancers are initially responsive to treatment, but ultimately develop drug resistance that may lead to an unfavorable clinical outcome (100). AXL overexpression in GI cancers has been associated with resistance to both targeted and non-targeted anti-cancer therapies. Particularly, EAC is characterized by resistance to chemotherapy and poor prognosis (18). Notably, AXL overexpression has been shown to mediate resistance to epirubicin by upregulation of c-MYC transcription via AKT-β-catenin signaling pathway in EAC cells (17). Additionally, AXL has been proposed as a promising therapeutic target to sensitize GI cancers to DNA-damaging chemotherapy drugs. In fact, genetic silencing of endogenous AXL abrogates cisplatin resistance through inhibition of the pro-apoptotic c-ABL/p73β signaling pathway in human EAC cells (18). AXL expression also promotes resistance to TNF-related apoptosis-inducing ligand (TRAIL) mediated by death receptor 5 (DR5) activity in EAC cells (47). Specifically, AXL and DR5 protein interaction blocks the recruitment of caspase-8 to the death-inducing signaling complex (DISC), resulting in enhanced cell survival, and decreased apoptosis. Sensitivity to TRAIL was restored in EAC cells after genetic silencing of endogenous AXL (47).
Resistance to the chemotherapy drug gemcitabine in PDAC was attributed to the function of YWHAZ/14-3-3 zeta/delta (14-3-3ζ) protein, which was isolated from monocyte-derived macrophage cultures (101). In mice bearing orthotopic PDAC xenografts, the antitumor activity of gemcitabine was significantly enhanced by pharmacological inhibition of AXL, which is a binding protein partner of 14-3-3ζ (48, 101). Therefore, it was suggested that apoptosis induced by chemotherapy might in turn activate a survival pathway through 14-3-3ζ/AXL and AKT phosphorylation cascade (101). Treatment of PDAC cells with BGB324, a selective small molecule inhibitor of AXL, promotes epithelial differentiation, stimulatory immune microenvironment, and high expression of nucleoside transporters, enhancing the response to gemcitabine (73). Of note, BGB324 treatment also improved survival and gemcitabine sensitivity in mice with advanced PDAC (73).
Based on a large body of evidence (9, 102, 103), cancer progression is frequently associated with acquired resistance to the inhibitors of EGF receptor (EGFR) mediated by the enhanced AXL expression as a bypass mechanism. For instance, increased AXL mRNA levels were found in 5 out of 7 CRC patients following anti-EGFR therapy (9). Moreover, resistance to anti-EGFR drugs accompanied by high AXL expression was demonstrated in three-dimensional CRC cell cultures derived from an AXL-positive, RAS wild-type patient after anti-EGFR treatment (9). Furthermore, AXL overexpression in CRC cell lines led to the resistance to EGFR inhibition. The role of AXL in EGFR inhibition resistance was established by analysis of AXL expression in tumor xenograft mice and in CRC patients after anti-EGFR treatment (9). Overexpression and activation of AXL upregulates PI3K/mammalian target of rapamycin (mTOR) and MAPK signaling pathways, enhancing cell survival, cell growth, invasion, and migration (104). Particularly, AXL mediates resistance to PI3Kα inhibition through activation of EGFR/PKC/mTOR cascade in head and neck (H&N) carcinomas as well in ESCC (105). The study suggested simultaneous EGFR and PI3Kα inhibition as a prospective therapeutic approach to overcome AXL-dependent resistance to PI3Kα inhibitors in patients with esophageal and H&N squamous cell carcinomas (105).
AXL plays a major role in promoting resistance to several common chemotherapeutics and targeted anti-cancer therapies. For example, treatment with AXL inhibitor S49076 markedly decreased tumor resistance to bevacizumab, a VEGF/VEGFR blocker, in a colon carcinoma xenograft model and attenuated colony formation of FGFR1/2- and AXL-positive hepatocarcinoma cells (61). In addition, cabozantinib, a dual inhibitor of MET and AXL, decreased cell growth in both in vitro and in vivo models of HER2-amplified gastric cancer with acquired resistance to afatinib, a pan-HER inhibitor (106). Studies in ESCC cell model have demonstrated a synergistic effect of combinatory treatment with HER2 inhibitor lapatinib and AXL inhibitor foretinib (43). Notably, in esophageal tissue of patients diagnosed with operable primary ESCC, the cumulative expression of AXL and HER2 was associated with unfavorable clinical outcome (43). Therefore, drug resistance to lapatinib could be potentially overcome by the inhibition of AXL. In HCC cell lines, AXL inhibition with RNA-interference or R428 compound improved sensitivity to sorafenib associated with increased phosphorylation level of AXL (70). Elevated level of AXL expression and its activation have been implicated in the resistance to imatinib in gastrointestinal stromal tumors (GIST) (107). Since GI cancer mesenchymal cells exhibit high levels of AXL expression, this RTK is potentially a promising therapeutic target for overcoming chemoresistance and improving the efficacy of current cancer therapies.
Targeting AXL in GI cancers
With the development of personalized medicine and targeted therapy, including tyrosine kinase inhibitors, GI cancer treatment continues to progress. Preclinical studies demonstrated that small-molecule TAM inhibitors, such as R428 (73) and RXDX106 (108), display anti-cancer activity in GI organs. As AXL has been associated with various stages of carcinogenesis and the inhibition of its expression and activity demonstrated promising results, AXL-specific inhibitors are currently being evaluated in clinical studies. BGB324 (BerGenBio); also known as R428 (Rigel Pharmaceuticals), is an oral selective small molecule AXL inhibitor that is currently being investigated in phase II clinical trials of pancreatic neoplasms (Table 2). BGB324 enhanced the efficacy of gemcitabine in preclinical studies in vivo through the stimulation of immune cellular response, expression of nucleoside transporters and promotion of epithelial cells differentiation in PDAC (73). Additionally, BGB324 is currently being tested in clinical trials as a monotherapy and in combination with chemo-, targeted-, and immunotherapy in various cancers (acute myeloid leukemia (AML), NCT02488408; non-small cell lung cancer (NSCLC), NCT02424617, NCT02922777; melanoma, NCT02872259). Particularly, combinations with nab-paclitaxel, gemcitabine, or cisplatin have shown encouraging results of clinical activity in patients with metastatic pancreatic cancer (NCT03649321).
AVB-500 (AVB-S6-500, Batiraxcept; Aravive, Inc.) is a novel high affinity Fc-sAXL fusion protein, which acts as an AXL decoy receptor by binding Gas6 and blocking AXL signaling (109). Preclinical data demonstrated inhibition of Gas6-induced AXL and Src phosphorylation, tumor vessel density, tumor growth, and metastatic burden in renal cell carcinoma (6, 110, 111) and ovarian cancer (109, 112) in response to treatment with AVB-500. Compared with chemotherapy alone, AVB-500 in combination with carboplatin and/or paclitaxel attenuated ovarian cancer cell survival in vitro and tumor growth in vivo (112). AVB-500 is currently investigated in Phase I/II clinical trials for patients with platinum-resistant or recurrent ovarian, fallopian tube, or peritoneal cancers as a combination therapy (Clinical Trial Identification #s: NCT03639246, NCT04019288) (Table 2). Also, a Phase 1b/2 study of AVB-500 safety and efficacy as a monotherapy or in combination with cabozantinib or nivolumab in patients with advanced or metastatic clear cell renal cell carcinoma is in progress (Clinical Trial Identification # NCT04300140). Three other studies are currently active and recruiting patients for ovarian cancer, advanced urothelial carcinoma, and pancreatic adenocarcinoma to assess AVB-500 efficacy as a combination therapy either with paclitaxel, or cabozantinib, or nab-paclitaxel/gemcitabine, correspondingly (Clinical Trial Identification #s: NCT04729608, NCT04004442, NCT04983407) (113).
A promising approach using a conditionally active biologic (CAB) AXL-targeted antibody drug conjugate BA3011 CAB-AXL-ADC, BioAtla, LLC, Table 2, NCT 03425279) alone and in combination with a PD-1 inhibitor Nivolumab in patients with advanced solid tumors is currently getting tested in Phase I, and in adult and adolescent patients with advanced, refractory sarcoma is investigated in Phase II. BA3011 is a product of fusion of anti-AXL antibodies with anti-mitotic compound monomethyl auristatin E (MMAE). The binding of antibody part of BA3011 to AXL initiates intracellular translocation of antibody-drug conjugate (ADC) complex followed by the release of MMAE ultimately leading to cancer cell death. Patients with advanced solid tumors, including NSCLC, prostate cancer, and pancreatic cancer are currently recruited.
Cabozantinib (Cabometix, XL184, BMS-907351, Cabometyx™, BMS-907351) is an oral small molecule TKI that targets AXL, c-Met and VEGFR (Table 2). This inhibitor has been preclinically investigated in ESCC (78) and liver cancer (114, 115). Currently, the evaluation of cabozantinib in combination with durvalumab (anti-programmed cell death protein 1 (PD-L1) inhibitor) in patients with advanced gastroesophageal adenocarcinoma, gastric cancer, hepatocellular carcinoma, and colorectal cancer is undergoing phase I/II open label, multi-cohort trial to determine safety, tolerability, and efficacy of the treatment (Clinical Trial Identification #: NCT03539822). The investigators propose that cabozantinib in combination with checkpoint-based immunotherapeutics like durvalumab will result in synergistic effect by altering the TME. Cabozantinib has been clinically approved for patients with sorafenib-resistant HCC (116) (Clinical Trial Identification #: NCT01908426). The data from the randomized phase III CELESTIAL trial revealed a significant improvement in progression-free survival and overall survival vs. placebo in a cohort of patients with previously treated advanced HCC (116). The patients who were not included in CELESTIAL trial are currently enrolled in another trial evaluating the therapeutic effect of cabozantinib in the patients with HCC intolerant to sorafenib treatment or first line treatment different from sorafenib (Clinical Trial Identification #: NCT04316182, Phase II). Another trial at stage 2 is ongoing to determine the outcome of cabozantinib treatment in patients with recurrent HCC and who had received a liver transplant as a part of a previous therapy (Clinical Trial Identification #: NCT04204850). Safety and efficacy of the treatment combination of cabozantinib and atezolizumab in comparison with the standard care (treatment with sorafenib) in patients with advanced HCC, who have not received prior systemic anti-cancer treatment, is being investigated in Phase III clinical trial (NCT03755791). The clinical benefits of cabozantinib in a cohort of patients with metastatic disease or unresectable locally advanced malignancy are being assessed as a part of MegaMOST clinical study (NCT04116541).
The clinical study of SLC-391 (SignalChem Lifesciences Corporation), a novel, potent and selective small molecule inhibitor of AXL, is currently ongoing in Canada, and recruiting patients with solid tumors to determine safety and tolerability of the drug (NCT03990454). Notably, the clinical outcome of SLC-391 in combination with the anti-PD-1 therapy pembrolizumab (Keytruda®) will be evaluated in SKYLITE trial, a phase II study for patients with NSCLC carried by Merck (MSD) and British Columbia-based SignalChem Lifesciences. TP-0903 (Sumitomo Dainippon Pharma Oncology, Inc), a novel oral inhibitor of AXL kinase that reverses the mesenchymal cancer phenotype, is currently investigated in Phases 1a/1b clinical trial for advanced solid tumors (Clinical Trial Identification #: NCT02729298). Commonly used anticoagulant Warfarin prevents the Gas6 interaction with externalized phosphatidylserine on the surface of apoptotic cells and cell debris through inhibition of vitamin K-dependent gamma-carboxylation of the γ-carboxyglutamic acid-rich (Gla) domain of Gas6 (117). A preclinical study reported that low-dose warfarin blocks the progression and spread of pancreatic cancer (72). A Phase I study of the effect of escalating doses of Warfarin on circulating biomarkers of AXL pathways (phosphoGas6 and sAXL) in patients with pancreatic adenocarcinoma (Table 2, Clinical Trial Identification #: NCT03536208) was initiated in 2019, but the study was withdrawn in 2021 because of lack of accrual.
MGCD516 (Sitravatinib, Mirati Therapeutics Inc.) is a small molecule spectrum selective TKI of several closely related receptor tyrosine kinases, including TAM and members of the VEGFR, PDGFR, DDR2, TRK and Eph families (118, 119). Anti-tumorigenic and anti-angiogenic activities of MGCD516 have been demonstrated in preclinical models of soft tissue sarcoma (119) and metastatic models of anti-angiogenic therapy resistance (118). Additionally, MGCD516 treatment enhances the immune checkpoint blockade by lowering the number of tumor-associated immunosuppressive myeloid cells and expanding the populations of CD4+ T cells and proliferating CD8+ T cells in the TME (99). MGCD516 therapy is currently investigated in patients with advanced solid tumors (Table 2, Clinical Trial Identification #: NCT02219711).
BPI-9016M (Betta Pharmaceuticals Co., Ltd.), is a novel highly potent dual-target inhibitor of c-Met/AXL. Preclinical studies in a lung adenocarcinoma model demonstrated strong activity of BPI-9016M in vitro and in vivo against c-Met/AXL kinases and their downstream pathways, leading to reduced tumor cell growth, migration, and invasion (120). A clinical trial at Phase I (NCT02478866) is currently assessing pharmacokinetics, safety, and anti-tumor activity of the inhibitor in patients with advanced solid tumors (121). Crizotinib (XALKORI®, PF-02341066, Pfizer Inc.) is a multitargeted, ATP-competitive, small molecule and orally available tyrosine kinase inhibitor that inhibits c-Met, AXL, ALK, and Ron (113). Preclinically, this compound reduced cell growth and induced apoptosis in human gastric carcinoma cells (122). Additionally, crizotinib in combination with mitomycin C increased apoptosis in CRC (123). A Phase II clinical trial is ongoing for patients with hematologic cancers, solid tumors, and metastatic cancer to determine the efficacy and the safety of crizotinib in 23 cohorts of patients with identified activating molecular alterations in the crizotinib target genes (Table 2, Clinical Trial Identification #: NCT02034981). Overall, there are 168 clinical studies associated with crizotinib in the ClinicalTrials.gov database. INCB081776 as a monotherapy or in combination with INCMGA00012 is undergoing Phase I trial for the safety and tolerability, pharmacokinetics, pharmacodynamics, and early clinical activity in patients with advanced solid tumors (Clinical Trial Identification #: NCT03522142). AXL-targeted therapies either as particular agents or in combination with conventional chemotherapy or other small molecule inhibitors have a promising opportunity to increase the survival rate of cancer patients. Nonetheless, more studies of AXL signaling pathways and physiological effects of their alteration are essential to identify the specific cohorts of patients who would be more responsive to the treatments with fewer adverse effects.
Chimeric antigen receptor (CAR)-T cell therapy targeting the B-cell antigen CD19 has proven clinically very successful in hematologic cancers [Clinical Trial Identification #: NCT02435849, NCT02445248, and NCT02348216 (124, 125)]. However, the development of CAR-T cell therapies for solid tumors has been slow because of the unique challenges associated with tumor microenvironment (126, 127). Preclinical studies indicated that CAR-T cell therapy targeting AXL induced in vitro cytotoxicity in triple negative breast cancer cells (TNBC) and reduced tumor growth in a TNBC xenograft mouse model (128). Preclinical and clinical studies are needed to investigate AXL-CAR-T cell therapy approach in GI cancers with high AXL expression.
Conclusions and future perspectives
Collectively and based on the current literature, AXL has been associated with GI cancer development and progression and its inhibition provides a novel therapeutic approach in the fight against GI cancers (Figure 1). Targeting AXL alone or with other TAM receptor tyrosine kinases could stimulate antitumor immunity, reduce cancer cell survival, enhance chemosensitivity and markedly attenuate metastatic tumor burden (129, 130). AXL might potentially become a valuable therapeutic target in GI cancers, and targeted anti-AXL therapies could further improve the standard first line of therapies with the objective to improve the prognosis and clinical outcome in patients with GI cancers or other AXL-expressing tumors.
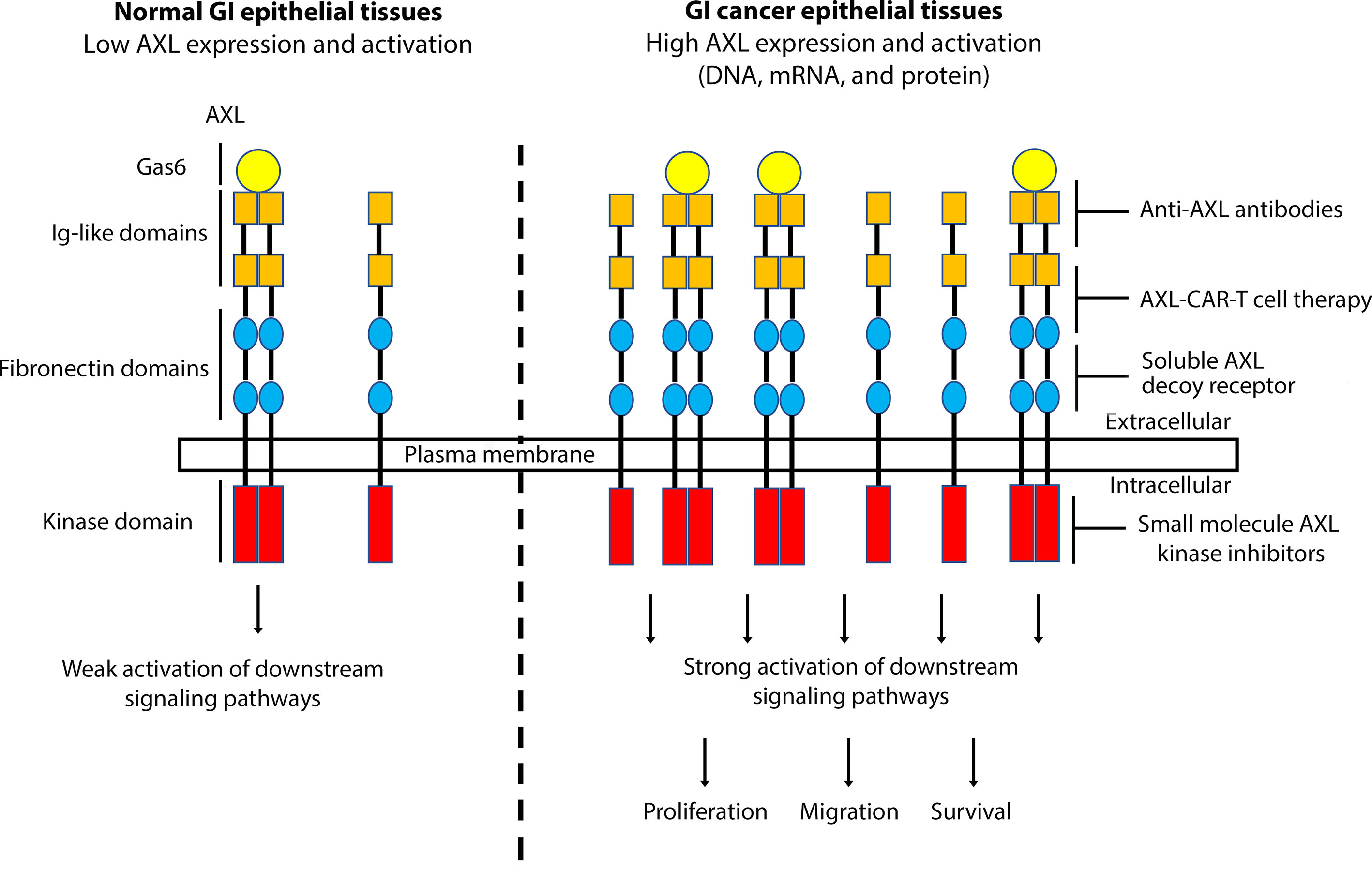
Figure 1 A schematic representation depicting the role of AXL overexpression and activation in GI cancers. Overexpression of AXL, induced by DNA amplification or high mRNA and protein levels, in GI epithelial tissues leads to strong activation of downstream signaling pathways, promoting cell proliferation, migration, and survival, hallmarks of GI carcinogenesis. Targeting AXL with specific monoclonal antibodies, small molecule kinase inhibitors, soluble AXL decoy receptor, or CAR-T cell therapy could be effective as a targeted therapeutic approach in GI cancers.
Author contributions
NP, writing and conception of the manuscript. AB, editing the manuscript and securing the funding. All authors contributed to the article and approved the submitted version.
Funding
This work was supported by the National Cancer Institute of the National Institutes of Health under Award Number RO1CA193219 and Vanderbilt-Ingram Cancer Center (VICC) Specialized Project of Research Excellence (SPORE) in GI cancer (P50CA95103). The content is solely the responsibility of the authors and does not necessarily represent the official views of the National Institutes of Health and Vanderbilt University Medical Center.
Conflict of interest
The authors declare that the research was conducted in the absence of any commercial or financial relationships that could be construed as a potential conflict of interest.
Publisher’s note
All claims expressed in this article are solely those of the authors and do not necessarily represent those of their affiliated organizations, or those of the publisher, the editors and the reviewers. Any product that may be evaluated in this article, or claim that may be made by its manufacturer, is not guaranteed or endorsed by the publisher.
References
1. Sung H, Ferlay J, Siegel RL, Laversanne M, Soerjomataram I, Jemal A, et al. Global cancer statistics 2020: GLOBOCAN estimates of incidence and mortality worldwide for 36 cancers in 185 countries. CA Cancer J Clin (2021) 71(3):209–49. doi: 10.3322/caac.21660
2. (WHO), W.H.O. Global health estimates 2020: deaths by cause, age, sex, by country and by region 2000-2019 (2020). WHO (Accessed December 11, 2020).
3. Holland SJ, Powell MJ, Franci C, Chan EW, Friera AM, Atchison RE, et al. Multiple roles for the receptor tyrosine kinase axl in tumor formation. Cancer Res (2005) 65(20):9294–303. doi: 10.1158/0008-5472.CAN-05-0993
4. Holland SJ, Pan A, Franci C, Hu Y, Chang B, Li W, et al. R428, a selective small molecule inhibitor of axl kinase, blocks tumor spread and prolongs survival in models of metastatic breast cancer. Cancer Res (2010) 70(4):1544–54. doi: 10.1158/0008-5472.CAN-09-2997
5. Onken J, Vajkoczy P, Torka R, Hempt C, Patsouris V, Heppner FL, et al. Phospho-AXL is widely expressed in glioblastoma and associated with significant shorter overall survival. Oncotarget (2017) 8(31):50403–14. doi: 10.18632/oncotarget.18468
6. Rankin EB, Fuh KC, Castellini L, Viswanathan K, Finger EC, Diep AN, et al. Direct regulation of GAS6/AXL signaling by HIF promotes renal metastasis through SRC and MET. Proc Natl Acad Sci U.S.A. (2014) 111(37):13373–8. doi: 10.1073/pnas.1404848111
7. Tanaka M, Siemann DW. Axl signaling is an important mediator of tumor angiogenesis. Oncotarget (2019) 10(30):2887–98. doi: 10.18632/oncotarget.26882
8. Tanaka M, Siemann DW. Gas6/Axl signaling pathway in the tumor immune microenvironment. Cancers (Basel) (2020) 12(7):1850. doi: 10.3390/cancers12071850
9. Cardone C, Blauensteiner B, Moreno-Viedma V, Martini G, Simeon V, Vitiello PP, et al. AXL is a predictor of poor survival and of resistance to anti-EGFR therapy in RAS wild-type metastatic colorectal cancer. Eur J Cancer (2020) 138:1–10. doi: 10.1016/j.ejca.2020.07.010
10. He L, Lei Y, Hou J, Wu J, Lv G. Implications of the receptor tyrosine kinase axl in gastric cancer progression. Onco Targets Ther (2020) 13:5901–11. doi: 10.2147/OTT.S257606
11. Hector A, Montgomery EA, Karikari C, Canto M, Dunbar KB, Wang JS, et al. The axl receptor tyrosine kinase is an adverse prognostic factor and a therapeutic target in esophageal adenocarcinoma. Cancer Biol Ther (2010) 10(10):1009–18. doi: 10.4161/cbt.10.10.13248
12. Bae CA, Ham IH, Oh HJ, Lee D, Woo J, Son SY, et al. Inhibiting the GAS6/AXL axis suppresses tumor progression by blocking the interaction between cancer-associated fibroblasts and cancer cells in gastric carcinoma. Gastric Cancer (2020) 23(5):824–36. doi: 10.1007/s10120-020-01066-4
13. Song X, Wang H, Logsdon CD, Rashid A, Fleming JB, Abbruzzese JL, et al. Overexpression of receptor tyrosine kinase axl promotes tumor cell invasion and survival in pancreatic ductal adenocarcinoma. Cancer (2011) 117(4):734–43. doi: 10.1002/cncr.25483
14. Kawasaki Y, Miyamoto M, Oda T, Matsumura K, Negishi L, Nakato R, et al. The novel lncRNA CALIC upregulates AXL to promote colon cancer metastasis. EMBO Rep (2019) 20(8):e47052. doi: 10.15252/embr.201847052
15. Davra V, Kumar S, Geng K, Calianese D, Mehta D, Gadiyar V, et al. Axl and mertk receptors cooperate to promote breast cancer progression by combined oncogenic signaling and evasion of host antitumor immunity. Cancer Res (2021) 81(3):698–712. doi: 10.1158/0008-5472.CAN-20-2066
16. Rothlin CV, Ghosh S, Zuniga EI, Oldstone MB, Lemke G. TAM receptors are pleiotropic inhibitors of the innate immune response. Cell (2007) 131(6):1124–36. doi: 10.1016/j.cell.2007.10.034
17. Hong J, Maacha S, Belkhiri A. Transcriptional upregulation of c-MYC by AXL confers epirubicin resistance in esophageal adenocarcinoma. Mol Oncol (2018) 12(12):2191–208. doi: 10.1002/1878-0261.12395
18. Hong J, Peng D, Chen Z, Sehdev V, Belkhiri A. ABL regulation by AXL promotes cisplatin resistance in esophageal cancer. Cancer Res (2013) 73(1):331–40. doi: 10.1158/0008-5472.CAN-12-3151
19. Polvi A, Armstrong E, Lai C, Lemke G, Huebner K, Spritz RA, et al. The human TYRO3 gene and pseudogene are located in chromosome 15q14-q25. Gene (1993) 134(2):289–93. doi: 10.1016/0378-1119(93)90109-G
20. Janssen JW, Schulz AS, Steenvoorden AC, Schmidberger M, Strehl S, Ambros PF, et al. A novel putative tyrosine kinase receptor with oncogenic potential. Oncogene (1991) 6(11):2113–20.
21. Nagata K, Ohashi K, Nakano T, Arita H, Zong C, Hanafusa H, et al. Identification of the product of growth arrest-specific gene 6 as a common ligand for axl, sky, and mer receptor tyrosine kinases. J Biol Chem (1996) 271(47):30022–7. doi: 10.1074/jbc.271.47.30022
22. Antony J, Huang RY. AXL-driven EMT state as a targetable conduit in cancer. Cancer Res (2017) 77(14):3725–32. doi: 10.1158/0008-5472.CAN-17-0392
23. Uehara H, Shacter E. Auto-oxidation and oligomerization of protein s on the apoptotic cell surface is required for mer tyrosine kinase-mediated phagocytosis of apoptotic cells. J Immunol (2008) 180(4):2522–30. doi: 10.4049/jimmunol.180.4.2522
24. Liu E, Hjelle B, Bishop JM. Transforming genes in chronic myelogenous leukemia. Proc Natl Acad Sci U.S.A. (1988) 85(6):1952–6. doi: 10.1073/pnas.85.6.1952
25. Neubauer A, Fiebeler A, Graham DK, O'Bryan JP, Schmidt CA, Barckow P, et al. Expression of axl, a transforming receptor tyrosine kinase, in normal and malignant hematopoiesis. Blood (1994) 84(6):1931–41. doi: 10.1182/blood.V84.6.1931.1931
26. O’Bryan JP, Frye RA, Cogswell PC, Neubauer A, Kitch B, Prokop C, et al. Axl, a transforming gene isolated from primary human myeloid leukemia cells, encodes a novel receptor tyrosine kinase. Mol Cell Biol (1991) 11(10):5016–31. doi: 10.1128/mcb.11.10.5016
27. Gay CM, Balaji K, Byers LA. Giving AXL the axe: targeting AXL in human malignancy. Br J Cancer (2017) 116(4):415–23. doi: 10.1038/bjc.2016.428
28. Korshunov VA. Axl-dependent signalling: a clinical update. Clin Sci (Lond) (2012) 122(8):361–8. doi: 10.1042/CS20110411
29. Manfioletti G, Brancolini C, Avanzi G, Schneider C. The protein encoded by a growth arrest-specific gene (gas6) is a new member of the vitamin K-dependent proteins related to protein s, a negative coregulator in the blood coagulation cascade. Mol Cell Biol (1993) 13(8):4976–85. doi: 10.1128/mcb.13.8.4976
30. Stitt TN, Conn G, Gore M, Lai C, Bruno J, Radziejewski C, et al. The anticoagulation factor protein s and its relative, Gas6, are ligands for the tyro 3/Axl family of receptor tyrosine kinases. Cell (1995) 80(4):661–70. doi: 10.1016/0092-8674(95)90520-0
31. Varnum BC, Young C, Elliott G, Garcia A, Bartley TD, Fridell YW, et al. Axl receptor tyrosine kinase stimulated by the vitamin K-dependent protein encoded by growth-arrest-specific gene 6. Nature (1995) 373(6515):623–6. doi: 10.1038/373623a0
32. Sasaki T, Knyazev PG, Clout NJ, Cheburkin Y, Gohring W, Ullrich A, et al. Structural basis for Gas6-axl signalling. EMBO J (2006) 25(1):80–7. doi: 10.1038/sj.emboj.7600912
33. Braunger J, Schleithoff L, Schulz AS, Kessler H, Lammers R, Ullrich A, et al. Intracellular signaling of the Ufo/Axl receptor tyrosine kinase is mediated mainly by a multi-substrate docking-site. Oncogene (1997) 14(22):2619–31. doi: 10.1038/sj.onc.1201123
34. Scaltriti M, Elkabets M, Baselga J. Molecular pathways: AXL, a membrane receptor mediator of resistance to therapy. Clin Cancer Res (2016) 22(6):1313–7. doi: 10.1158/1078-0432.CCR-15-1458
35. Ekman C, Stenhoff J, Dahlback B. Gas6 is complexed to the soluble tyrosine kinase receptor axl in human blood. J Thromb Haemost (2010) 8(4):838–44. doi: 10.1111/j.1538-7836.2010.03752.x
36. Fridell YW, Villa J Jr., Attar EC, Liu ET. GAS6 induces axl-mediated chemotaxis of vascular smooth muscle cells. J Biol Chem (1998) 273(12):7123–6. doi: 10.1074/jbc.273.12.7123
37. McCloskey P, Fridell YW, Attar E, Villa J, Jin Y, Varnum B, et al. GAS6 mediates adhesion of cells expressing the receptor tyrosine kinase axl. J Biol Chem (1997) 272(37):23285–91. doi: 10.1074/jbc.272.37.23285
38. Lee WP, Liao Y, Robinson D, Kung HJ, Liu ET, Hung MC. Axl-gas6 interaction counteracts E1A-mediated cell growth suppression and proapoptotic activity. Mol Cell Biol (1999) 19(12):8075–82. doi: 10.1128/MCB.19.12.8075
39. Lemke G, Lu Q. Macrophage regulation by tyro 3 family receptors. Curr Opin Immunol (2003) 15(1):31–6. doi: 10.1016/S0952-7915(02)00016-X
40. Bailey MH, Tokheim C, Porta-Pardo E, Sengupta S, Bertrand D, Weerasinghe A, et al. Comprehensive characterization of cancer driver genes and mutations. Cell (2018) 174(4):1034–5. doi: 10.1016/j.cell.2018.07.034
41. Gao J, Aksoy BA, Dogrusoz U, Dresdner G, Gross B, Sumer SO, et al. Integrative analysis of complex cancer genomics and clinical profiles using the cBioPortal. Sci Signal (2013) 6(269):pl1. doi: 10.1126/scisignal.2004088
42. Dunne PD, McArt DG, Blayney JK, Kalimutho M, Greer S, Wang T, et al. AXL is a key regulator of inherent and chemotherapy-induced invasion and predicts a poor clinical outcome in early-stage colon cancer. Clin Cancer Res (2014) 20(1):164–75. doi: 10.1158/1078-0432.CCR-13-1354
43. Hsieh MS, Yang PW, Wong LF, Lee JM. The AXL receptor tyrosine kinase is associated with adverse prognosis and distant metastasis in esophageal squamous cell carcinoma. Oncotarget (2016) 7(24):36956–70. doi: 10.18632/oncotarget.9231
44. Liu J, Wang K, Yan Z, Xia Y, Li J, Shi L, et al. Axl expression stratifies patients with poor prognosis after hepatectomy for hepatocellular carcinoma. PloS One (2016) 11(5):e0154767. doi: 10.1371/journal.pone.0154767
45. Sawabu T, Seno H, Kawashima T, Fukuda A, Uenoyama Y, Kawada M, et al. Growth arrest-specific gene 6 and axl signaling enhances gastric cancer cell survival via akt pathway. Mol Carcinog (2007) 46(2):155–64. doi: 10.1002/mc.20211
46. Hong J, Abid F, Phillips S, Salaria SN, Revetta FL, Peng D, et al. Co-Overexpression of AXL and c-ABL predicts a poor prognosis in esophageal adenocarcinoma and promotes cancer cell survival. J Cancer (2020) 11(20):5867–79. doi: 10.7150/jca.47318
47. Hong J, Belkhiri A. AXL mediates TRAIL resistance in esophageal adenocarcinoma. Neoplasia (2013) 15(3):296–304. doi: 10.1593/neo.122044
48. Reichel P, Dengler M, van Zijl F, Huber H, Fuhrlinger JG., Reichl C, et al. Axl activates autocrine transforming growth factor-beta signaling in hepatocellular carcinoma. Hepatology (2015) 61(3):930–41. doi: 10.1002/hep.27492
49. Dengler M, Staufer K, Huber H, Stauber R, Bantel H, Weiss KH, et al. Soluble axl is an accurate biomarker of cirrhosis and hepatocellular carcinoma development: results from a large scale multicenter analysis. Oncotarget (2017) 8(28):46234–48. doi: 10.18632/oncotarget.17598
50. Koorstra JB, Karikari CA, Feldmann G, Bisht S, Rojas PL, Offerhaus GJ, et al. The axl receptor tyrosine kinase confers an adverse prognostic influence in pancreatic cancer and represents a new therapeutic target. Cancer Biol Ther (2009) 8(7):618–26. doi: 10.4161/cbt.8.7.7923
51. Martinelli E, Martini G, Cardone C, Troiani T, Liguori G, Vitagliano D, et al. AXL is an oncotarget in human colorectal cancer. Oncotarget (2015) 6(27):23281–96. doi: 10.18632/oncotarget.3962
52. Barrett T, Troup DB, Wilhite SE, Ledoux P, Evangelista C, Kim IF, et al. NCBI GEO: archive for functional genomics data sets–10 years on. Nucleic Acids Res (2011) 39(Database issue):D1005–10. doi: 10.1093/nar/gkq1184
53. Bockelman C, Engelmann BE, Kaprio T, Hansen TF, Glimelius B. Risk of recurrence in patients with colon cancer stage II and III: a systematic review and meta-analysis of recent literature. Acta Oncol (2015) 54(1):5–16. doi: 10.3109/0284186X.2014.975839
54. Orme JJ, Du Y, Vanarsa K, Mayeux J, Li L, Mutwally A, et al. Heightened cleavage of axl receptor tyrosine kinase by ADAM metalloproteases may contribute to disease pathogenesis in SLE. Clin Immunol (2016) 169:58–68. doi: 10.1016/j.clim.2016.05.011
55. Miller MA, Oudin MJ, Sullivan RJ, Wang SJ, Meyer AS, Im H, et al. Reduced proteolytic shedding of receptor tyrosine kinases is a post-translational mechanism of kinase inhibitor resistance. Cancer Discovery (2016) 6(4):382–99. doi: 10.1158/2159-8290.CD-15-0933
56. Reichl P, Fang M, Starlinger P, Staufer K, Nenutil R, Muller P, et al. Multicenter analysis of soluble axl reveals diagnostic value for very early stage hepatocellular carcinoma. Int J Cancer (2015) 137(2):385–94. doi: 10.1002/ijc.29394
57. Martinez-Bosch N, Cristobal H, Iglesias M, Gironella M, Barranco L, Visa L, et al. Soluble AXL is a novel blood marker for early detection of pancreatic ductal adenocarcinoma and differential diagnosis from chronic pancreatitis. EBioMedicine (2022) 75:103797. doi: 10.1016/j.ebiom.2021.103797
58. Feitelson MA, Arzumanyan A, Kulathinal RJ, Blain SW, Holcombe RF, Mahajna J, et al. Sustained proliferation in cancer: mechanisms and novel therapeutic targets. Semin Cancer Biol (2015) 35 Suppl:S25–54. doi: 10.1016/j.semcancer.2015.02.006
59. Xu MZ, Chan SW, Liu AM, Wong KF, Fan ST, Chen J, et al. AXL receptor kinase is a mediator of YAP-dependent oncogenic functions in hepatocellular carcinoma. Oncogene (2011) 30(10):1229–40. doi: 10.1038/onc.2010.504
60. Li J, Jia L, Ma ZH, Ma QH, Yang XH, Zhao YF. Axl glycosylation mediates tumor cell proliferation, invasion and lymphatic metastasis in murine hepatocellular carcinoma. World J Gastroenterol (2012) 18(38):5369–76. doi: 10.3748/wjg.v18.i38.5369
61. Burbridge MF, Bossard CJ, Saunier C, Fejes I, Bruno A, Leonce S, et al. S49076 is a novel kinase inhibitor of MET, AXL, and FGFR with strong preclinical activity alone and in association with bevacizumab. Mol Cancer Ther (2013) 12(9):1749–62. doi: 10.1158/1535-7163.MCT-13-0075
62. Graham DK, DeRyckere D, Davies KD, Earp HS. The TAM family: phosphatidylserine sensing receptor tyrosine kinases gone awry in cancer. Nat Rev Cancer (2014) 14(12):769–85. doi: 10.1038/nrc3847
63. Li Y, Ye X, Tan C, Hongo JA, Zha J, Liu J, et al. Axl as a potential therapeutic target in cancer: role of axl in tumor growth, metastasis and angiogenesis. Oncogene (2009) 28(39):3442–55. doi: 10.1038/onc.2009.212
64. Hay ED. An overview of epithelio-mesenchymal transformation. Acta Anat (Basel) (1995) 154(1):8–20. doi: 10.1159/000147748
65. Babaei G, Aziz SG, Jaghi NZZ. EMT, cancer stem cells and autophagy; the three main axes of metastasis. BioMed Pharmacother (2021) 133:110909. doi: 10.1016/j.biopha.2020.110909
66. Gjerdrum C, Tiron C, Hoiby T, Stefansson I, Haugen H, Sandal T, et al. Axl is an essential epithelial-to-mesenchymal transition-induced regulator of breast cancer metastasis and patient survival. Proc Natl Acad Sci USA (2010) 107(3):1124–9. doi: 10.1073/pnas.0909333107
67. Nieto MA, Huang RY, Jackson RA, Thiery JP. Emt: 2016. Cell (2016) 166(1):21–45. doi: 10.1016/j.cell.2016.06.028
68. Ganesh K, Massague J. Targeting metastatic cancer. Nat Med (2021) 27(1):34–44. doi: 10.1038/s41591-020-01195-4
69. Niu Z-S, Niu X-J, Wang W-H. Role of the receptor tyrosine kinase axl in hepatocellular carcinoma and its clinical relevance. Future Oncol (2019) 15(6):653–62. doi: 10.2217/fon-2018-0528
70. Pinato DJ, Brown MW, Trousil S, Aboagye EO, Beaumont J, Zhang H, et al. Integrated analysis of multiple receptor tyrosine kinases identifies axl as a therapeutic target and mediator of resistance to sorafenib in hepatocellular carcinoma. Br J Cancer (2019) 120(5):512–21. doi: 10.1038/s41416-018-0373-6
71. Chai ZT, Zhang XP, Ao JY, Zhu XD, Wu MC, Lau WY, et al. AXL overexpression in tumor-derived endothelial cells promotes vessel metastasis in patients with hepatocellular carcinoma. Front Oncol (2021) 11:650963. doi: 10.3389/fonc.2021.650963
72. Kirane A, Ludwig KF, Sorrelle N, Haaland G, Sandal T, Ranaweera R, et al. Warfarin blocks Gas6-mediated axl activation required for pancreatic cancer epithelial plasticity and metastasis. Cancer Res (2015) 75(18):3699–705. doi: 10.1158/0008-5472.CAN-14-2887-T
73. Ludwig KF, Du W, Sorrelle NB, Wnuk-Lipinska K, Topalovski M, Toombs JE, et al. Small-molecule inhibition of axl targets tumor immune suppression and enhances chemotherapy in pancreatic cancer. Cancer Res (2018) 78(1):246–55. doi: 10.1158/0008-5472.CAN-17-1973
74. Li J, Dai C, Shen L. Ursolic acid inhibits epithelial-mesenchymal transition through the Axl/NF-kappaB pathway in gastric cancer cells. Evid Based Complement Alternat Med (2019) 2019:2474805. doi: 10.1155/2019/2474805
75. Maacha S, Hong J, von Lersner A, Zijlstra A, Belkhiri A. AXL mediates esophageal adenocarcinoma cell invasion through regulation of extracellular acidification and lysosome trafficking. Neoplasia (2018) 20(10):1008–22. doi: 10.1016/j.neo.2018.08.005
76. Ciardiello D, Blauensteiner B, Matrone N, Belli V, Mohr T, Vitiello PP, et al. Dual inhibition of TGFbeta and AXL as a novel therapy for human colorectal adenocarcinoma with mesenchymal phenotype. Med Oncol (2021) 38(3):24. doi: 10.1007/s12032-021-01464-3
77. Van der Jeught K, Xu HC, Li YJ, Lu XB, Ji G. Drug resistance and new therapies in colorectal cancer. World J Gastroenterol (2018) 24(34):3834–48. doi: 10.3748/wjg.v24.i34.3834
78. Yang PW, Liu YC, Chang YH, Lin CC, Huang PM, Hua KT, et al. Cabozantinib (XL184) and R428 (BGB324) inhibit the growth of esophageal squamous cell carcinoma (ESCC). Front Oncol (2019) 9:1138. doi: 10.3389/fonc.2019.01138
79. Grepin R, Pages G. Molecular mechanisms of resistance to tumour anti-angiogenic strategies. J Oncol 2010. (2010) p:835680. doi: 10.1155/2010/835680
80. Owens GK. Molecular control of vascular smooth muscle cell differentiation and phenotypic plasticity. Novartis Found Symp (2007) 283:174–91; discussion 191-3, 238-41. doi: 10.1002/9780470319413.ch14
81. Fedeli C, Torriani G, Galan-Navarro C, Moraz ML, Moreno H, Gerold G, et al. Axl can serve as entry factor for lassa virus depending on the functional glycosylation of dystroglycan. J Virol (2018) 92(5). doi: 10.1128/JVI.01613-17
82. Ruan GX, Kazlauskas A. Axl is essential for VEGF-a-dependent activation of PI3K/Akt. EMBO J (2012) 31(7):1692–703. doi: 10.1038/emboj.2012.21
83. Bockerstett KA, DiPaolo RJ. Regulation of gastric carcinogenesis by inflammatory cytokines. Cell Mol Gastroenterol Hepatol (2017) 4(1):47–53. doi: 10.1016/j.jcmgh.2017.03.005
84. Zitvogel L, Kepp O, Kroemer G. Immune parameters affecting the efficacy of chemotherapeutic regimens. Nat Rev Clin Oncol (2011) 8(3):151–60. doi: 10.1038/nrclinonc.2010.223
85. Paolino M, Penninger JM. The role of TAM family receptors in immune cell function: implications for cancer therapy. Cancers (Basel) (2016) 8(10):97. doi: 10.3390/cancers8100097
86. Caraux A, Lu Q, Fernandez N, Riou S, Di Santo JP, Raulet DH, et al. Natural killer cell differentiation driven by Tyro3 receptor tyrosine kinases. Nat Immunol (2006) 7(7):747–54. doi: 10.1038/ni1353
87. Lu Q, Lemke G. Homeostatic regulation of the immune system by receptor tyrosine kinases of the tyro 3 family. Science (2001) 293(5528):306–11. doi: 10.1126/science.1061663
88. Valkenburg KC, de Groot AE, Pienta KJ. Targeting the tumour stroma to improve cancer therapy. Nat Rev Clin Oncol (2018) 15(6):366–81. doi: 10.1038/s41571-018-0007-1
89. Hochreiter-Hufford A, Ravichandran KS. Clearing the dead: apoptotic cell sensing, recognition, engulfment, and digestion. Cold Spring Harb Perspect Biol (2013) 5(1):a008748. doi: 10.1101/cshperspect.a008748
90. Kasikara C, Davra V, Calianese D, Geng K, Spires TE, Quigley M, et al. Pan-TAM tyrosine kinase inhibitor BMS-777607 enhances anti-PD-1 mAb efficacy in a murine model of triple-negative breast cancer. Cancer Res (2019) 79(10):2669–83. doi: 10.1158/0008-5472.CAN-18-2614
91. Kasikara C, Kumar S, Kimani S, Tsou WI, Geng K, Davra V, et al. Phosphatidylserine sensing by TAM receptors regulates AKT-dependent chemoresistance and PD-L1 expression. Mol Cancer Res (2017) 15(6):753–64. doi: 10.1158/1541-7786.MCR-16-0350
92. Myers KV, Amend SR, Pienta KJ. Targeting Tyro3, axl and MerTK (TAM receptors): implications for macrophages in the tumor microenvironment. Mol Cancer (2019) 18(1):94. doi: 10.1186/s12943-019-1022-2
93. Seitz HM, Camenisch TD, Lemke G, Earp HS, Matsushima GK. Macrophages and dendritic cells use different Axl/Mertk/Tyro3 receptors in clearance of apoptotic cells. J Immunol (2007) 178(9):5635–42. doi: 10.4049/jimmunol.178.9.5635
94. Bosurgi L, Bernink JH, Delgado Cuevas V, Gagliani N, Joannas L, Schmid ET, et al. Paradoxical role of the proto-oncogene axl and mer receptor tyrosine kinases in colon cancer. Proc Natl Acad Sci USA (2013) 110(32):13091–6. doi: 10.1073/pnas.1302507110
95. Sharif MN, Sosic D, Rothlin CV, Kelly E, Lemke G, Olson EN, et al. Twist mediates suppression of inflammation by type I IFNs and axl. J Exp Med (2006) 203(8):1891–901. doi: 10.1084/jem.20051725
96. Alciato F, Sainaghi PP, Sola D, Castello L, Avanzi GC. TNF-alpha, IL-6, and IL-1 expression is inhibited by GAS6 in monocytes/macrophages. J Leukoc Biol (2010) 87(5):869–75. doi: 10.1189/jlb.0909610
97. Kim SY, Lim EJ, Yoon YS, Ahn YH, Park EM, Kim HS, et al. Liver X receptor and STAT1 cooperate downstream of Gas6/Mer to induce anti-inflammatory arginase 2 expression in macrophages. Sci Rep (2016) 6:29673. doi: 10.1038/srep29673
98. Shen Y, Cui X, Rong Y, Zhang Z, Xiao L, Zhou T, et al. Exogenous Gas6 attenuates silica-induced inflammation on differentiated THP-1 macrophages. Environ Toxicol Pharmacol (2016) 45:222–6. doi: 10.1016/j.etap.2016.05.029
99. Du W, Huang H, Sorrelle N, Brekken RA. Sitravatinib potentiates immune checkpoint blockade in refractory cancer models. JCI Insight (2018) 3(21):e124184. doi: 10.1172/jci.insight.124184
100. Engelman JA, Settleman J. Acquired resistance to tyrosine kinase inhibitors during cancer therapy. Curr Opin Genet Dev (2008) 18(1):73–9. doi: 10.1016/j.gde.2008.01.004
101. D’Errico G, Alonso-Nocelo M, Vallespinos M, Hermann PC, Alcala S, Garcia CP, et al. Tumor-associated macrophage-secreted 14-3-3zeta signals via AXL to promote pancreatic cancer chemoresistance. Oncogene (2019) 38(27):5469–85. doi: 10.1038/s41388-019-0803-9
102. Park K, Chang GC, Curigliano G, Lim WT, Soo RA, Molina-Vila MA, et al. Phase I results of S49076 plus gefitinib in patients with EGFR TKI-resistant non-small cell lung cancer harbouring MET/AXL dysregulation. Lung Cancer (2021) 155:127–35. doi: 10.1016/j.lungcan.2021.03.012
103. Zhang Z, Lee JC, Lin L, Olivas V, Au V, LaFramboise T, et al. Activation of the AXL kinase causes resistance to EGFR-targeted therapy in lung cancer. Nat Genet (2012) 44(8):852–60. doi: 10.1038/ng.2330
104. Verma A, Warner SL, Vankayalapati H, Bearss DJ, Sharma S. Targeting axl and mer kinases in cancer. Mol Cancer Ther (2011) 10(10):1763–73. doi: 10.1158/1535-7163.MCT-11-0116
105. Elkabets M, Pazarentzos E, Juric D, Sheng Q, Pelossof RA, Brook S, et al. AXL mediates resistance to PI3Kalpha inhibition by activating the EGFR/PKC/mTOR axis in head and neck and esophageal squamous cell carcinomas. Cancer Cell (2015) 27(4):533–46. doi: 10.1016/j.ccell.2015.03.010
106. Yoshioka T, Shien K, Takeda T, Takahashi Y, Kurihara E, Ogoshi Y, et al. Acquired resistance mechanisms to afatinib in HER2-amplified gastric cancer cells. Cancer Sci (2019) 110(8):2549–57. doi: 10.1111/cas.14089
107. Mahadevan D, Cooke L, Riley C, Swart R, Simons B, Della Croce K, et al. A novel tyrosine kinase switch is a mechanism of imatinib resistance in gastrointestinal stromal tumors. Oncogene (2007) 26(27):3909–19. doi: 10.1038/sj.onc.1210173
108. Kim JE, Kim Y, Li G, Kim ST, Kim K, Park SH, et al. MerTK inhibition by RXDX-106 in MerTK activated gastric cancer cell lines. Oncotarget (2017) 8(62):105727–34. doi: 10.18632/oncotarget.22394
109. Fuh KC, Bookman MA, Liu JF, Coleman RL, Herzog TJ, Thaker PH, et al. Phase 1b study of AVB-500 in combination with paclitaxel or pegylated liposomal doxorubicin platinum-resistant recurrent ovarian cancer. Gynecol Oncol (2021) 163(2):254–61. doi: 10.1016/j.ygyno.2021.08.020
110. Rankin EB, Giaccia AJ. The receptor tyrosine kinase AXL in cancer progression. Cancers (Basel) (2016) 8(11):103. doi: 10.3390/cancers8110103
111. Xiao Y, Zhao H, Tian L, Nolley R, Diep AN, Ernst A, et al. S100A10 is a critical mediator of GAS6/AXL-induced angiogenesis in renal cell carcinoma. Cancer Res (2019) 79(22):5758–68. doi: 10.1158/0008-5472.CAN-19-1366
112. Mullen MM, Lomonosova E, Toboni MD, Oplt A, Cybulla E, Blachut B, et al. GAS6/AXL inhibition enhances ovarian cancer sensitivity to chemotherapy and PARP inhibition through increased DNA damage and enhanced replication stress. Mol Cancer Res (2022) 20(2):265–79.. doi: 10.1158/1541-7786.MCR-21-0302
113. Tanaka M, Siemann DW. Therapeutic targeting of the Gas6/Axl signaling pathway in cancer. Int J Mol Sci (2021) 22(18):9953. doi: 10.3390/ijms22189953
114. Wang DD, Chen Y, Chen ZB, Yan FJ, Dai XY, Ying MD, et al. CT-707, a novel FAK inhibitor, synergizes with cabozantinib to suppress hepatocellular carcinoma by blocking cabozantinib-induced FAK activation. Mol Cancer Ther (2016) 15(12):2916–25. doi: 10.1158/1535-7163.MCT-16-0282
115. Xiang QF, Zhang DM, Wang JN, Zhang HW, Zheng ZY, Yu DC, et al. Cabozantinib reverses multidrug resistance of human hepatoma HepG2/adr cells by modulating the function of p-glycoprotein. Liver Int (2015) 35(3):1010–23. doi: 10.1111/liv.12524
116. Abou-Alfa GK, Meyer T, Cheng AL, El-Khoueiry AB, Rimassa L, Ryoo BY, et al. Cabozantinib in patients with advanced and progressing hepatocellular carcinoma. N Engl J Med (2018) 379(1):54–63. doi: 10.1056/NEJMoa1717002
117. Lew ED, Oh J, Burrola PG, Lax I, Zagorska A, Traves PG, et al. Differential TAM receptor-ligand-phospholipid interactions delimit differential TAM bioactivities. Elife (2014) 3:9953. doi: 10.7554/eLife.03385
118. Dolan M, Mastri M, Tracz A, Christensen JG, Chatta G, Ebos JML. Enhanced efficacy of sitravatinib in metastatic models of antiangiogenic therapy resistance. PloS One (2019) 14(8):e0220101. doi: 10.1371/journal.pone.0220101
119. Patwardhan PP, Ivy KS, Musi E, de Stanchina E, Schwartz GK. Significant blockade of multiple receptor tyrosine kinases by MGCD516 (Sitravatinib), a novel small molecule inhibitor, shows potent anti-tumor activity in preclinical models of sarcoma. Oncotarget (2016) 7(4):4093–109. doi: 10.18632/oncotarget.6547
120. Zhang P, Li S, Lv C, Si J, Xiong Y, Ding L, et al. BPI-9016M, a c-met inhibitor, suppresses tumor cell growth, migration and invasion of lung adenocarcinoma via miR203-DKK1. Theranostics (2018) 8(21):5890–902. doi: 10.7150/thno.27667
121. Hu X, Zheng X, Yang S, Wang L, Hao X, Cui X, et al. First-in-human phase I study of BPI-9016M, a dual MET/Axl inhibitor, in patients with non-small cell lung cancer. J Hematol Oncol (2020) 13(1):6. doi: 10.1186/s13045-019-0834-2
122. Zou HY, Li Q, Lee JH, Arango ME, McDonnell SR, Yamazaki S, et al. An orally available small-molecule inhibitor of c-met, PF-2341066, exhibits cytoreductive antitumor efficacy through antiproliferative and antiangiogenic mechanisms. Cancer Res (2007) 67(9):4408–17. doi: 10.1158/0008-5472.CAN-06-4443
123. Lev A, Deihimi S, Shagisultanova E, Xiu J, Lulla AR, Dicker DT, et al. Preclinical rationale for combination of crizotinib with mitomycin c for the treatment of advanced colorectal cancer. Cancer Biol Ther (2017) 18(9):694–704. doi: 10.1080/15384047.2017.1364323
124. June CH, O'Connor RS, Kawalekar OU, Ghassemi S, Milone MC. CAR T cell immunotherapy for human cancer. Science (2018) 359(6382):1361–5. doi: 10.1126/science.aar6711
125. Yang X, Wang GX, Zhou JF. CAR T cell therapy for hematological malignancies. Curr Med Sci (2019) 39(6):874–82. doi: 10.1007/s11596-019-2118-z
126. Bagley SJ, O’Rourke DM. Clinical investigation of CAR T cells for solid tumors: lessons learned and future directions. Pharmacol Ther (2020) 205:107419. doi: 10.1016/j.pharmthera.2019.107419
127. Dees S, Ganesan R, Singh S, Grewal IS. Emerging CAR-T cell therapy for the treatment of triple-negative breast cancer. Mol Cancer Ther (2020) 19(12):2409–21. doi: 10.1158/1535-7163.MCT-20-0385
128. Wei J, Sun H, Zhang A, Wu X, Li Y, Liu J, et al. A novel AXL chimeric antigen receptor endows T cells with anti-tumor effects against triple negative breast cancers. Cell Immunol (2018) 331:49–58. doi: 10.1016/j.cellimm.2018.05.004
129. Khalaf N, El-Serag HB, Abrams HR, Thrift AP. Burden of pancreatic cancer: from epidemiology to practice. Clin Gastroenterol Hepatol (2021) 19(5):876–84. doi: 10.1016/j.cgh.2020.02.054
Keywords: GI cancers, targeted therapy, small molecule inhibitors, anti-AXL antibodies, Gas6
Citation: Pidkovka N and Belkhiri A (2023) Altered expression of AXL receptor tyrosine kinase in gastrointestinal cancers: a promising therapeutic target. Front. Oncol. 13:1079041. doi: 10.3389/fonc.2023.1079041
Received: 24 October 2022; Accepted: 31 May 2023;
Published: 04 July 2023.
Edited by:
João Pessoa, University of Coimbra, PortugalReviewed by:
Douglas K. Graham, Emory University, United StatesIsabel Ben-Batalla, German Cancer Research Center (DKFZ), Germany
Copyright © 2023 Pidkovka and Belkhiri. This is an open-access article distributed under the terms of the Creative Commons Attribution License (CC BY). The use, distribution or reproduction in other forums is permitted, provided the original author(s) and the copyright owner(s) are credited and that the original publication in this journal is cited, in accordance with accepted academic practice. No use, distribution or reproduction is permitted which does not comply with these terms.
*Correspondence: Abbes Belkhiri, abbes.belkhiri@vanderbilt.edu