- 1Department of General Surgery, Guangzhou Red Cross Hospital, Jinan University, Guangzhou, China
- 2Institute of Reproductive Health, Tongji Medical College, Huazhong University of Science and Technology, Wuhan, China
Hepatocellular carcinoma (HCC) is a highly malignant tumor and ranked as the fourth cause of cancer-related mortality. The poor clinical prognosis is due to an advanced stage and resistance to systemic treatment. There are no obvious clinical symptoms in the early stage and the early diagnosis rate remains low. Novel effective biomarkers are important for early diagnosis and tumor surveillance to improve the survival of HCC patients. Circulating tumor cells (CTCs) are cancer cells shed from primary or metastatic tumor and extravasate into the blood system. The number of CTCs is closely related to the metastasis of various solid tumors. CTCs escape from blood vessels and settle in target organs, then form micro-metastasis. Epithelial-mesenchymal transformation (EMT) plays a crucial role in distant metastasis, which confers strong invasiveness to CTCs. The fact that CTCs can provide complete cellular biological information, which allows CTCs to be one of the most promising liquid biopsy targets. Recent studies have shown that CTCs are good candidates for early diagnosis, prognosis evaluation of metastasis or recurrence, and even a potential therapeutic target in patients with HCC. It is a new indicator for clinical application in the future. In this review, we introduce the enrichment methods and mechanisms of CTCs, and focus on clinical application in patients with HCC.
Introduction
Hepatocellular carcinoma (HCC) accounts for about 90% of all primary hepatic malignancies and is one of the most common malignancies. HCC ranks as the sixth most common tumor and its mortality ranks fourth in cancer-related death worldwide, with a high incidence in Asia and Africa (1). Patients with early-stage HCC may undergo curative therapies such as surgical liver resection (LR), liver transplantation (LT) and local ablation. On the other hand, patients with advanced-stage HCC usually accept non-curative therapies such as transcatheter arterial chemoembolization, radiotherapy and systemic therapies (2). Due to the absence of obvious clinical symptoms in the early stage of HCC, most patients are diagnosed with advanced HCC for the first time. The existing methods are difficult to effectively prolong the survival of patients, with a 5-year survival rate of less than 20%. Therefore, the key to improving the prognosis of HCC patients are to find effective means for early diagnosis, surveillance response of treatment, and early intervention (3).
Currently, the diagnosis of HCC mainly depends on serum markers, imaging examination and liver biopsy. Serum alpha-fetoprotein (AFP) is the most widely used tumor marker for early screening and surveillance progression of HCC, nevertheless, it has unsatisfactory performance with a sensitivity of only 60% and specificity of only 80% (4, 5). The updated American Association for the Study of Liver Disease (AASLD) guidelines no longer recommended AFP testing as part of HCC diagnostic criteria (6). Other serum markers, such as osteopontin, Golgi protein-73 or glypican-3 may offer information about the biological aggressiveness of HCC, but they are not erratic and accurate enough to form part of a screening strategy (7–9). Medical imaging methods including B-ultrasound, computed tomography (CT), and magnetic resonance imaging (MR) are recommended for the diagnosis of HCC, however, those methods are difficult to detect early-stage liver cancer with a diameter of less than 1 cm. Although liver biopsy can provide definitely a pathological diagnosis, there is a debate on the widespread use of liver biopsy due to the risks of bleeding and tumor seeding (10). Therefore, we need to find effective markers for early diagnosis and monitoring of recurrence and metastasis in patients with HCC.
In recent years, liquid biopsy technology has gradually emerged and has become one of the most promising methods for early diagnosis and real-time progress assessment of tumors with the advantages of non-invasive and repeated sampling. CTCs, which are the most concerned tumor detection method in liquid biopsy, is obtained from the peripheral blood of patients and carry much comprehensive tumor information for early diagnosis and monitoring of tumors. CTCs were originally discovered in the blood of breast cancer patients by Australian physicians Thomas Ashworth (11). CTCs are a very scarce sub-population of cancer cells released from primary solid tumors or metastatic sites into the peripheral circulation and eventually form metastatic lesions in other target organs (12). The number of CTCs in the circulating system is extremely rare (only a few CTCs in billions of blood cells), a range of 0 − 86 CTCs were detected in 5 mL of blood in HCC patients (13).
In the early stages, little is known about the characteristics and phenotypes of CTCs, and the limitation of enrichment technique leads to a big challenge for the detection of CTCs. With the emergence of new technologies, the improvement of existing technologies and the deepening of understanding of oncology, the detection rate of CTCs has been greatly improved. Currently, CTCs detection has been applied in clinical trials on a small scale. The CellSearch system for CTCs detection, the only approved technology by the US Food and Drug Administration, has been used for breast, lung, prostate and colon cancer (14–16). In recent years, CTCs detection plays an increasingly important role in HCC clinical management.
CTCs can not only provide information about abnormal protein expression, genomic mutation and mRNA variation of solid tumors, but also help people understand the mechanism of tumorigenesis, metastasis and drug resistance from aspects of cell morphology, migration ability and drug response (17–19). Correspondingly, CTCs detection can be used for early diagnosis, individualized treatment, and monitoring of prognosis and recurrence (20). In HCC, rich blood is present around the immediate vicinity of the tumor, allowing thousands of CTCs to be released into the blood circulation daily. CTCs carry a large amount of tumor information and are used as a clinical biomarker for HCC. In the present review, we introduce the enrichment methods and different phenotypes of CTCs and focus on clinical application in patients with HCC.
Enrichment and identification of CTCs
About 106 CTCs fall off into the peripheral blood circulation per gram of tumor primary or metastatic tissue daily, but more than 99.99% of CTCs lose their activity under the attack of the human immune defense system, and only <0.01% of CTCs can survive (21). Every milliliter of blood contains only a few of CTCs, while there are billions of normal blood cells, various proteins, nucleic acids, carbohydrates and other substances. The sensitivity of the enrichment effect is greatly affected by the background interference. Most of the CTCs travel as individual cell, but some as clusters, also known as micro-emboli. Micro-emboli are more easily infiltrate distant tissues and form metastases than individual cell in cancers (22–24). In addition, some CTCs can interact with platelets in the blood and accomplish the phenotypic transformation of tumor cells, resulting in poor prognosis (25, 26). A series of techniques recently have been developed to separate and enrich CTCs from complex background. The capture methods can be divided into the physical techniques and biological techniques according to the properties of CTCs.
Physical methods capture CTCs from erythrocytes and leukocytes through the physical properties of CTCs, including size, density, deformability, and electrical charge. Density gradient centrifugation is based on the difference in density and sedimentation rate between CTCs and blood cells. CTCs and blood components are distributed to specific positions under a certain centrifugal force (27). This method is simple to operate, but is not widely used due to its low sensitivity, specificity and time-consuming. Microfiltration is a physical method based on the assumption that the diameter of tumor cells is too larger than erythrocytes and leukocytes to pass through a filter with lots of small pores (28, 29). However, some studies showed the cell-size based microfiltration loss amount of small size CTCs, resulting in false negative results (30–32). The CTCs ≤ 5μm are particularly observed in a majority of HCC CTCs, which is lost during microfiltration (30). Dielectrophoresis is a method to separate CTCs from blood according to the difference of charge between CTCs and other blood cells (33). Other physical separation and enrichment techniques include microfluidic, acoustophoresis, et al. (34, 35). CTCs enrichment based on physical properties enables high-throughput, but these methods possess a low specificity and a higher false-positive rate due to some blood cells may exhibit similar physical properties to CTCs.
Biological methods capture CTCs based on cellular immune characteristics that specific antibodies or ligands target to the antigens presented on the cell membrane of CTCs. The affinity ligands or antibodies are immobilized on microdevices or magnetic beads to bind with antigens on the cell membrane of CTCs, which achieve efficient and high-purity enrichment (36). Biological methods can be divided into two subcategories: positive techniques and negative techniques. Cellsearch system is the most representative of positive enrichment methods, which is only approved by the US Food and Drug Administration for the detection of CTCs (16). This system employs immunomagnetic beads coated with an antibody specifically against the epithelial Cell adhesion molecule (EpCAM) antigens on CTCs cell surface, and has already been applied in HCC (37–44). However, several types of tumor cells express low level of EpCAM, and some CTCs may lose the expression of EpCAM during the process of EMT (Figure 1). This largely limits the sensitivity of Cellsearch system for EpCAM negative tumor cells (45, 46). The negative enrichment method employs immunomagnetic beads coated with CD45 antibodies to bind the antigens on the surface of leukocytes, and then the binding leukocytes are removed with the beads (47, 48). This indirectly enriching CTCs method makes up for the limitation that the Cellsearch system is highly dependent on EpCAM. Additionally, many research studies show that CTCs detection with a single marker is inefficient. The combination of multiple markers and a physical enrichment method could increase the sensitivity and specificity of CTCs detection.
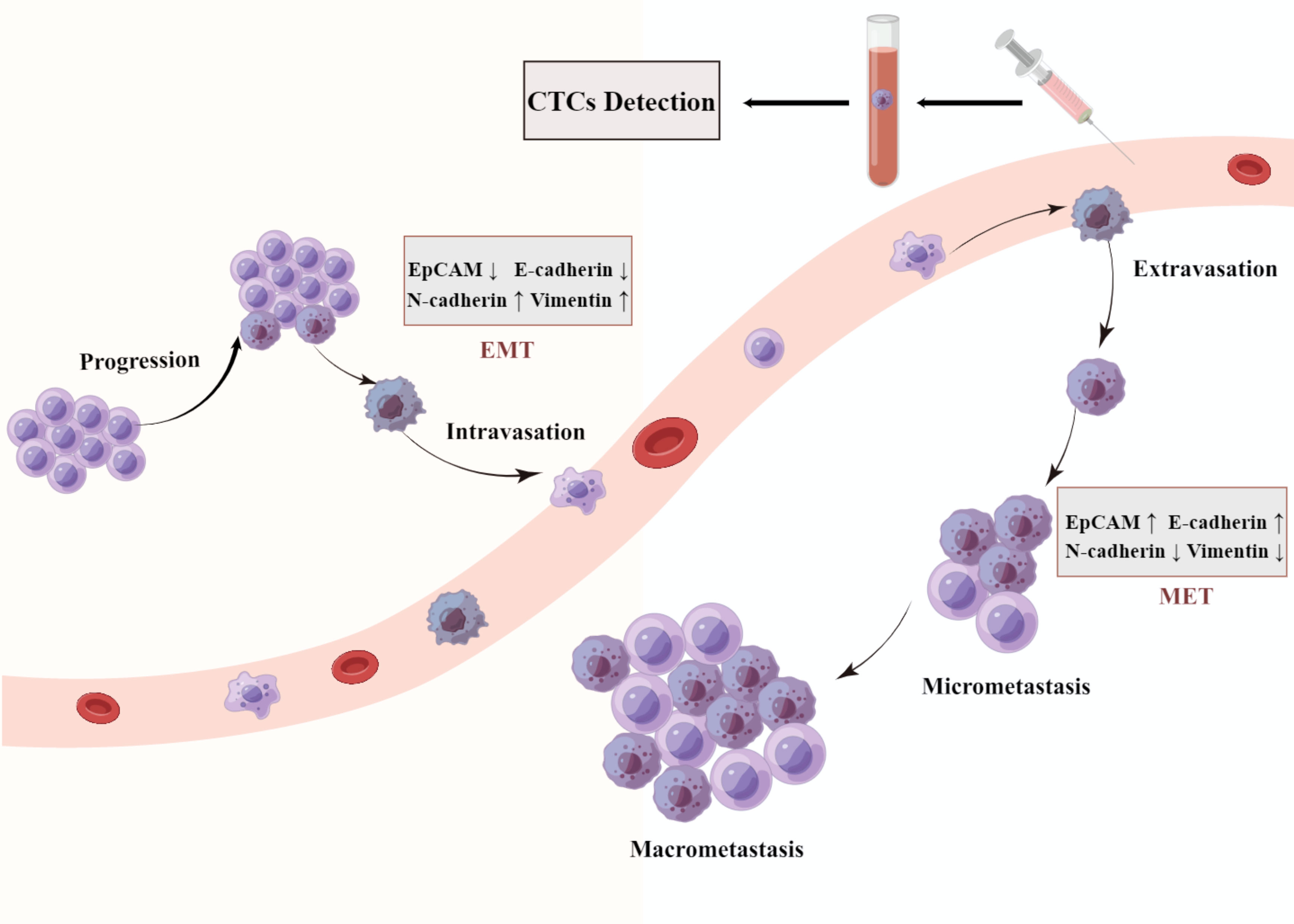
Figure 1 A schematic representation of epithelial mesenchymal transition (EMT). Cancer cells detach from the basement membrane and intravasate to the nearby blood vessels as CTC and travel through blood vessels to a secondary site in a process described as metastasis. They get lodged in different organs by a process termed as mesenchymal epithelial transition (MET).
The most common method to identify CTCs are through immunofluorescence (IF), which provides the size and morphology of CTCs (49). Other identification methods includes flow cytometry (50), quantitative real-time PCR (qRT-PCR) (51) and immunofluorescence in situ hybridization (iFISH) (52). Now multiple detection methods have been used together to improve enrichment efficiency. Microfluidic chips achieve a high sensitivity and specificity in the separation of CTCs by the combination of cell size and immunoaffinity (53, 54). The CanPatrol system combines microfiltration and RNA in situ hybridization, which can simultaneously identify CTCs with EMT phenotype and has been widely used in various tumors including HCC (13, 22, 52, 55–61). A novel integrated strategy, subtraction enrichment (SE)-iFISH, improved the detection of HCC CTCs (30). EpCAM+ CTCs and EpCAM− CTCs, each with different ploidy of chromosome 8, were effectively detected in patients with hepatobiliary malignances.
EMT and CTCs
Many evidence shows a strong relationship between EMT and CTC. EMT is a dynamic process in which the epithelial phenotype cells transition into mesenchymal phenotype cells with the downregulation of epithelial markers (E-cadherin, EpCAM) and upregulation of mesenchymal markers (N-cadherin, vimentin) (62, 63). EMT is critical for embryonic development and tissue repair under physiological conditions, however, it is also thought to play a crucial role in tumor progression by promoting tissue infiltration and metastases (64, 65). EMT is a complex process involving multiple signaling pathways and transcription factor regulation, the up-regulation of mesenchymal genes and suppression of epithelial genes lead to the transformation of cell morphology and acquisition of stronger invasive migration capability (66). Many changes in cellular shape and vitality, such as loss of cellular polarity, the disappearance of intercellular adhesion, and the acquisition of enhanced motility, which promote cells to fall off from tumor tissues and invade into blood vessels (64, 67, 68). EMT process facilitates the secretion of matrix metalloproteinase (MMP), which degrades the extracellular matrix to cause cell migration, tissue invasion and blood vessel infiltration (69). EMT is believed to play an important role in tumor occurrence and progression. The studies have demonstrated that the expression of EMT markers is associated with an aggressive malignant phenotype and with poor prognosis in cancer patients (65, 67, 70).
Recent studies found that tumor cells undergoing EMT are associated with the acquisition of stem cell characteristics (71), immunosuppression (72), resistance to radiotherapy (73) and chemotherapy (74, 75). A recent study in patients with HCC reported that the presence of mesenchymal phenotype CTCs was significantly correlated with high AFP levels, multiple tumors, advanced TNM stages, presence of embolus or micro-embolus, and earlier recurrence (57). Another study showed that mesenchymal phenotype CTCs were an independent risk factor for early recurrence, indicating a shorter postoperative disease-free survival in patients with HCC (60).
EMT promotes the spread of cancer cells from the primary tumor into the blood. Upon arrival at a suitable secondary location, CTCs undergo the MET transformation from the non-proliferative or low-proliferative migratory phenotype to a proliferative type, resulting in distant metastasis (64, 67, 76). To form micro-metastases or develop into metastatic cancers, CTCs are considered to have a corresponding colonization ability in the blood circulation (77, 78). The hypothesis proposes that there may be a reversible switch between epithelial-mesenchymal transition (EMT) and mesenchymal-epithelial transition (MET) (79, 80). Contrary to the physiological process of EMT, mesenchymal phenotype cells transform into epithelial phenotype cells by recovery of intercellular adhesion and reduced mobility during MET, providing the ability of distant colonization and establishment of metastases.
Biomarkers of CTCs in HCC
The biomarkers only expressed on the surface of liver tumor cells and rarely or not expressed in blood cells, which are used for CTCs detection in HCC patients. The biomarkers include epithelial markers, EMT-related markers, hepatocytes and HCC specific markers, and cancer stem-cell markers, as shown in Table 1.
Epithelial markers
EpCAM is one of the most commonly used epithelial markers on the cell membrane, and is widely used for isolating CTCs from peripheral blood. EpCAM+ CTCs enriched by CellSearch system were detected in a cohort of 29 patients, including 20 in the HCC group and 9 in the control group (39). A total of 7/20 (35%) HCC patients had more than two CTCs per 7.5 mL in peripheral blood. Although no patients with non-malignant liver diseases were detected with CTCs, the presence of CTCs was associated with AFP level and vascular invasion. Sequence analysis of CTCs DNA showed that low frequency variants exist in EpCAM+ CTCs. Another study consists of 197 HCC patients who underwent curative surgical resection and show that EpCAM+ CTCs counts (≥3) were associated with postoperative extrahepatic metastases and shorter median overall survival. The HCC patients with high number of CTCs need more careful surveillance in early interventions (44). EpCAM-based enrichment methods are not able to be used in the enrichment of CTCs which express low level of EpCAM (95) or lose expression of EpCAM during the process of EMT (96, 97).
EMT-related markers
According to the expression patterns during EMT process, biomarkers are largely categorized into epithelial markers and mesenchymal markers. Epithelial markers contain E-cadherin, EpCAM and CK8/18/19. Mesenchymal markers include N-cadherin, vimentin and some transcription factors such as ZEB1, ZEB2, twist, slug and Snail1 (98). Based on microfiltration enrichment and RNA in situ hybridization, the CanPatrol system captures CTCs and classifies them into epithelial phenotype, mesenchymal phenotype and mixed phenotype (52). A retrospective cohort study consists of 165 HCC patients who underwent curative surgical resection, and show that more than two preoperative CTCs were present in 117/165 (70.9%) of HCC patients and the elevated CTC counts were correlated with poor clinicopathological features (57). The presence of preoperative mesenchymal CTCs was significantly correlated with multiple tumors, high AFP levels, presence of embolus or micro-embolus, advanced TNM and BCLC stage, and earlier recurrence. Another recent study consists of 112 HCC patients who underwent surgical resection, 12 HBV patients and 20 healthy volunteers (59). A total of 101/112 (90.18%) HCC patients and 2/12 (16.67%) HBV patients were detected with CTCs. Two of HBV patients who were isolated CTCs and then were detected with small HCC within five months, nevertheless, none of the healthy volunteers were isolated CTCs. Preoperatively total CTCs or mesenchymal CTCs were closely correlated with early recurrence, lung metastasis and multi-intrahepatic recurrence. Mesenchymal positive CTCs are a high risk of early recurrence. The combination of epithelial and mesenchymal markers increases the sensitivity and specificity of CTCs detection. CTCs expressing EMT-related markers are significantly correlated with clinicopathological features, which allows adequate stratification for HCC patients’ management.
Hepatocytes and HCC specific markers
Serum AFP is a predictor of prognostic evaluation because the high level of AFP is closely associated with HCC recurrence and metastasis (2, 99). AFP is common biomarker for HCC screening, however, in early HCC the detection rate of AFP is only 25-65% (100). There is an urgent need of HCC specific biomarkers in early detection. A number of hepatocytes and HCC specific markers, including sialoglycoprotein receptor (ASGPR), hepatocyte paraffin 1 (Hep Par 1), and glypican-3 (GPC3), show a diagnostic and prognostic value (47, 50, 53, 86–88, 90).
A study consist of 85 HCC patients, 37 patients with benign liver diseases, 14 patients with other cancers, and 20 healthy volunteers (86). The immunomagnetic enrichment of ASGPR+ CTCs and subsequent identification by immunofluorescence staining with Hep Par 1 antibody were illustrated to be an effective strategy to identify CTCs from peripheral blood of HCC patients. ASGPR is a protein expressed in the outer membrane of hepatocytes and HCC cells. Hep Par 1 is a protein expressed on the mitochondrial membrane of hepatocytes. By this method, CTCs were present in 69/85 (81%) HCC patients, and no CTCs were detected in benign liver diseases, healthy volunteers, or other cancer groups. The counts of CTCs were significantly associated with tumor size, portal vein tumor thrombus and TNM stage. Another study consists of 32 HCC patients, 40 patients with benign liver diseases, 20 healthy volunteers and 20 healthy volunteers (47). The negative enrichment method with CD45 antibodies to remove leukocytes and subsequent identification using a combination of ASGPR and CPS1 antibodies were conducted to identify CTCs from peripheral blood of HCC patients. By this strategy, CTCs with positive ASGPR and CPS1 were detected in 29/32 (91%) of HCC patients, nevertheless, no CTCs were found in healthy volunteers, benign liver diseases and other cancer groups. Recently, Zhu and his colleagues designed and developed a microfluidic Synergetic-Chip, which combined physical size separation and immunological recognition (53). EpCAM and ASGPR antibodies were respectively coated in two parallel channels to capture CTCs, subsequently, enriched CTCs were identified by immunofluorescence staining. By this method, EpCAM or ASGPR positive CTCs were identified in 45/45 (100%) of HCC patients, and the sensitivity and specificity achieve 97.8% and 100%. The specific markers combined with EpCAM were also used and proved to be efficient in CTCs detection (53, 101).
Cancer stem-cell markers
Cancer stem cells (CSCs) are a specific sub-population of cancer cells in tumor tissue, which play an important role in the occurrence and metastasis of carcinoma. The origin of cancer stem cells remains controversial. One of the possible origins is the transformation through mutation and de-differentiation (102). These phenotypic changes are primarily the result of fundamental changes in gene expression concomitant with a diminished transcription of the relevant liver-specific genes, and can be interpreted as a ‘de-differentiation’ of hepatocytes. During the de-differentiation process, the overexpression of oncogenes and inactivation of tumor suppressor genes lead to uncontrolled excessive proliferation of cells and expression of stem cell markers (103). Currently, it is difficult to distinguish CTCs and CSCs due to the lack of a suitable markers. HCC cells and liver cancer stem cells are also derived from the de-differentiation of mature hepatocytes (103–105). Liver cancer stem cells are a unique subset of hepatocellular carcinoma cells with stem cell features and contribute to the disease recurrence, drug resistance and death (98).
Circulating CSCs are a few subpopulations of CTCs, which exhibit unlimited self-renewal and proliferation potential. They are associate with increased aggressiveness and poor prognosis (106). Some CTCs of peripheral blood of HCC patients expressed stem cell markers such as CD44, CD90, and CD133 (91–93). CD133+ CD44+ CSCs had been proved to be associated with elevated serum AFP, serum transaminases and poorer prognosis in HCC patients (107). Based on the detection of CD90+ CD44+ CSCs by multicolor flow cytometry, the number of preoperative CSCs in patients with recurrence were higher compared with the patients without recurrence (91). Patients with CD90+ CD44+ CSCs had lower RFS and OS. Thus, preoperative CSCs can be used to predict post-hepatectomy recurrence of HCC. EpCAM1+ CTCs of peripheral blood of HCC patients showed high tumorigenic capacity and low apoptotic tendency and expressed CSCs markers CD133+ and ABCG2+ (92). Through physical enrichment by Labyrinth chip and immunofluorescence staining using GPC3, CD44, and HepPar-1 antibodies, CTCs were detected in 37/42 (88.1%) of HCC patients, and CD44+ CTCs were identified in 30/42 (71.4%) of HCC patients (93). The detection rate of CD44+ CTCs was significantly higher in patients with advanced stages than those with early stages.
Clinical application of CTCs in HCC
CTCs may transfer through hematogenous metastasis in the early stage, so some HCC patients have distant metastasis before receiving treatment, which leads to a great challenge for HCC management. Therefore, early diagnosis of HCC is the key to receiving the effective treatment. Accumulated evidence indicates that CTCs detection is of great significance for early diagnosis HCC. Related detection markers, methods and clinical application of CTCs are shown in Figure 2.
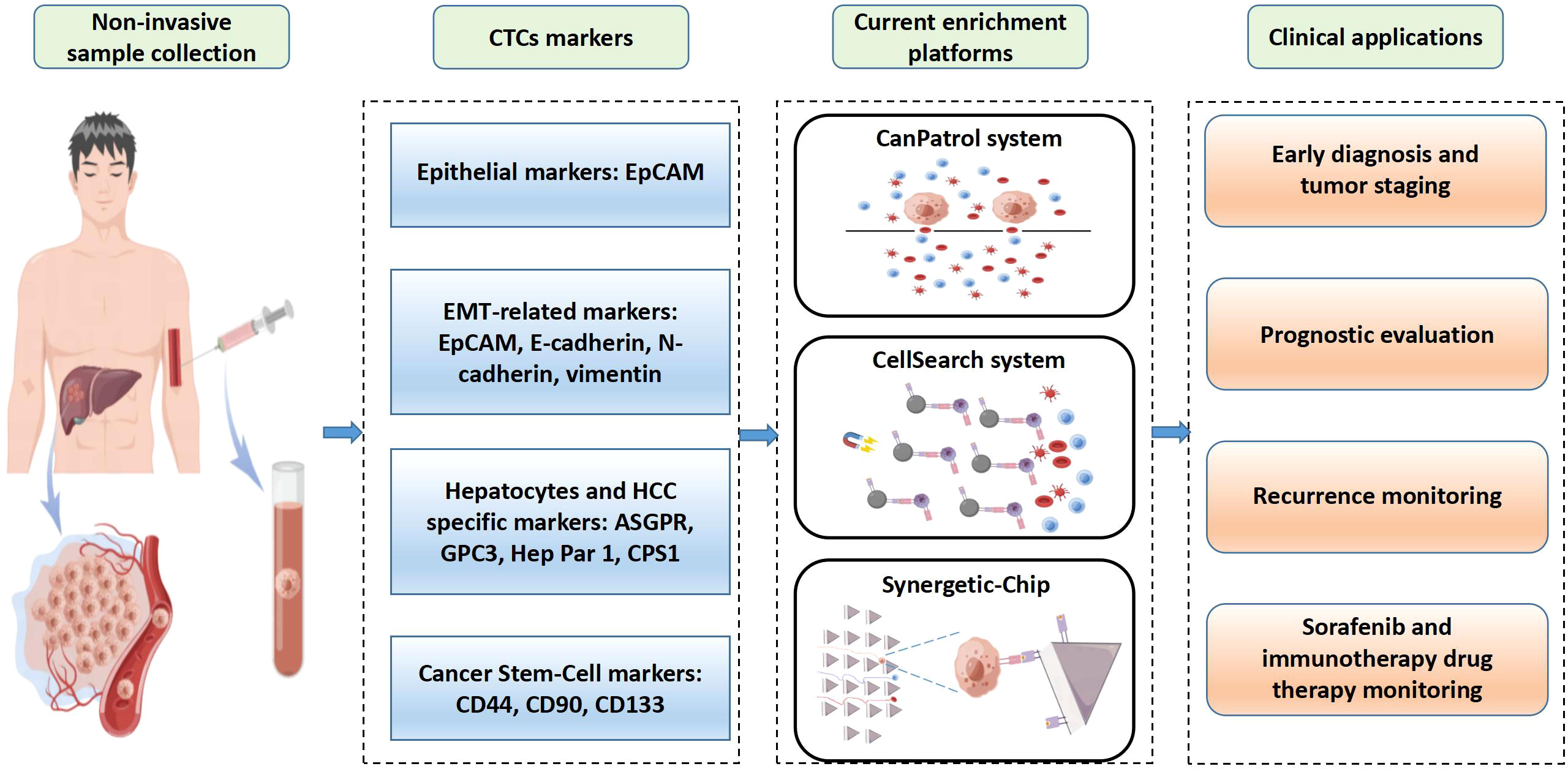
Figure 2 Overview of clinical applications of circulating tumor cells (CTCs) in hepatocellular carcinoma (HCC). CTCs are obtained from patients’ blood samples in a non-invasive way. HCC CTCs are primarily isolated based on their unique biological markers. CellSearch is the only FDA-approved system for CTCs detection used clinically. CanPatol and CTC-chip are other CTCs detection systems. CTCs represent an independent factor for early diagnosis and tumor staging, prognostic evaluation, recurrence monitoring, and drug therapy monitoring.
Diagnosis and tumor staging
Many evidence indicates that CTCs detection with EMT-related markers are useful for early diagnosis and staging. EpCAM+ CTCs were detected in 18/59 (30.5%) HCC patients and 1/19 (5.3%) individuals in the control group. The CTCs detection rates of patients with different BCLC stages were significant differences: stages A 1/9 (11.1%), B 6/31 (19.3%) and C 11/19 (57.9%) (38). CTCs were detected in 101/112 (90.12%) of HCC patients, even at an early stage (59). Patients with late BCLC stage had a higher mesenchymal phenotype CTCs counts than those in early stage. Mesenchymal phenotype CTCs counts (≥1) could be used to distinguish BCLC stage in HCC patients (61). The combination of total CTCs and AFP improve the sensitivity and specificity of 61.95% and 89.47%, respectively. Twist+ vimentin+ CTCs were detected in 33/46 (69.6%) of HCC patients. The CTCs detection rates were closely associated with tumor size, portal vein tumor thrombus, and TNM classification (82). CTCs were detected in 185/195 (95%) of HCC patients. The counts, mesenchymal and mixed phenotype of CTCs were correlated with the clinicopathologic features, such as ages, BCLC stages and AFP levels (13, 22, 52, 57, 58, 61).
HCC specific markers are efficient to detect CTCs clinically. CTCs were identified in 24/27 (89%) HCC patients by specific markers ASGPR, CPS1 and P-CK, and no CTCs were detected in other test subjects (87). ASGPR+CPS1+ CTCs were detected in 29/32 (91%) of HCC patients, and no CTCs were found in healthy volunteers, benign liver diseases and other cancers groups (47). ASGPR+ EpCAM+ CTCs were detected in 45/45 (100%) of HCC patients (53). Some study showed that CTCs counts through HCC specific markers or combination with other markers were correlated with tumor size, differentiation status, portal vein tumor thrombus and TNM stage (50, 53, 86, 88).
Prognostic evaluation and recurrence monitoring
The prognosis of HCC is closely related to the clinical stage. The common clinical prognostic models of HCC include the BCLC staging system and TNM staging system. BCLC staging system is based on tumor burden, liver function and physical status. The parameters of tumor burden are composed of the number and diameter of tumor, portal vein invasion and extrahepatic spread (108). TNM staging is evaluated by the number and diameter of tumors, vascular invasion, adjacent organs invasion, regional lymph nodes metastases and distant metastases (109, 110). Studies have shown that the counts of CTCs are associated with the parameters of BCLC staging and TNM staging in HCC patients (13, 52, 53, 59). Analysis of the CTCs by RNA in situ hybridization (RNA-ISH) revealed that positive rate of CTCs was 83.6% (46/55) and 96.5% (55/57) in patients with BCLC stage 0–A and stage B–C tumors (59). A meta-analysis study of twenty-three published studies showed that CTC positivity were also significantly associated with TNM Stage (RR 1.30, 95% CI: [1.02-1.65]; p=0.03), tumor size (RR 1.36, 95% CI: [1.09-1.69]; p=0.006), vascular invasion (RR 1.99, 95% CI: [1.43-2.77]; p<0.0001), portal vein tumor thrombus (RR 1.73, 95% CI: [1.42-2.11]; p=0.0001), serum AFP level (RR 2.05, 95% CI: [1.18-3.54]; p=0.01), suggesting a strong prognostic value of CTC in HCC (111).
The counts of CTCs make a difference in peripheral blood of HCC patients before and after treatment. Thus, CTCs detection is used to monitor the response of therapy and help clinicians to adjust their treatment plans. Recent study reported the relationship between ΔCTC and recurrence of HCC after hepatectomy. ΔCTC is defined as the changes of CTCs counts after surgery. The positive ΔCTC was correlated with higher recurrence and lower survival in HCC patients after surgical liver resection (84). HCC patients with postoperative EpCAM+ CTCs counts < 2 had significantly longer disease-free survival and overall survival than the patients with CTCs counts ≥2, suggesting increased postoperative EpCAM+ CTCs counts were related to a poor clinical outcome after surgical liver resection (41). Some studies provided evidence that mesenchymal phenotype CTCs could predict early recurrence, metastasis, shorter disease-free survival and overall survival (13, 52, 56, 57, 59, 82, 83). EMT-related phenotype CTCs play an important role in predicting metastasis and recurrence. A study reported that Twist+ CTCs were associated with higher metastasis, recurrence and mortality rate (58). Another study found that mesenchymal phenotype CTCs were independent risk factors for early recurrence. HCC patients with postoperative mesenchymal phenotype CTCs counts ≥1 had a shorter disease-free survival after surgery (60). The preoperative GPC3+CTCs were significantly correlated with the clinical outcome of HCC patients. GPC3+ CTCs counts >5 were an independent risk factor for microscopic portal vein invasion. HCC patients with GPC3+ CTCs counts >5 had lower disease-free survival and overall survival after hepatectomy (50). The preoperative CD90+ CD44+ CSCs were proved to be associated with intrahepatic and extrahepatic recurrence of patients after surgical resection. HCC patients with CSCs > 0.01% (the optimal cut-off point for gating percentages of circulating CSCs by calculating the AUROC) had lower disease-free survival and overall survival (91). HCC patients with PD-L1+ CTCs had shorter overall survival than those patients without PD-L1+ CTCs (94).
Drug therapy monitoring
Drug resistance is also an important factor affecting clinical prognosis. The acquired drug resistance of HCC cells leads to poor effects of systemic therapy (112). Sorafenib is a small molecule multi-kinase inhibitor with multiple antitumor effects and is the first-line targeted drug for the treatment of HCC in the advanced stage (113). Numerous studies have shown that the inactivation of Ras/Raf/ERK pathway and the activation of PI3K/Akt/mTOR pathway plays a crucial role in sorafenib resistance (114–117). CTCs are released from tumor tissue to the bloodstream and carry drug resistance information of the primary tumor or metastases, therefore pERK/pAkt phenotype of CTCs can be used to monitor the therapeutic effect and drug resistance of sorafenib (48). A study reported the association between pERK+ pAkt− CTCs and the sensibility of sorafenib in HCC patients (48). During sorafenib treatment, the CTC counts showed a marked decrease in patients with pERK+ pAkt− CTCs. HCC patients with pERK+ pAkt− CTCs were sensitive to sorafenib-targeted therapy, whereas patients with other phenotype CTCs showed resistance to sorafenib therapy. So, they suggested the percentage of pERK+ pAkt− CTCs could serve as an independent predictive factor for HCC patients treated with sorafenib.
The programmed cell death protein 1 (PD-1) and its ligand (PD-L1) axes is an inhibitory immune checkpoint that is involved in suppressing antitumor immunity. The overexpression of PD-L1 of tumor cells binding to PD-1 on the surface of T cells can induce T-cell anergy or apoptosis, which enables evasion of immune-mediated tumor surveillance (112). Inhibitors targeting PD-1 or PD-L1 can restrain this pathway and restore antitumor immunity (118). The advanced melanoma patients with PD-L1+ CTCs showed a good response to PD-1 inhibitor immunotherapy, whereas those patients with the PD-L1− CTCs exhibited drug resistance (119). PD-L1+ CTCs which were predominantly found in advanced-stage patients could accurately discriminate early-stage from advanced-stage HCC patients (94). Subsequently, they followed up 10 HCC patients who received immunotherapy (PD-1 blockade), and found that 5 patients with PD-L1+ CTCs had a good response to immunotherapy, and only 1 of 5 non-responders had PD-L1+ CTCs. CTCs with specific phenotypes have the potentials to predict drug resistance to targeted therapy and immunotherapy, which provide clinical application in guiding individualized medicine.
Conclusions
The detection of CTCs is of great value in the early diagnosis and tumor staging, evaluation of recurrence and prognosis, and even prediction of drug resistance of targeted therapy and immunotherapy in patients with HCC. However, CTCs detection is not widely used in the clinical application of HCC due to several limitations, such as lack of standardized technical procedures, limitations of detection efficiency, extremely rare of CTCs in blood, and absence of external validation in many clinical studies. If technological advances could overcome the shortcomings of CTCs detection in the future, CTCs detection will be a breakthrough in HCC clinical management, especially in early diagnosis offering an opportunity for curative surgery before metastasis. EMT plays an important role in various pathophysiological processes of CTCs, such as tumor cells shedding and migrating, vascular invasion and distant implantation. More mechanisms of EMT in CTCs should be investigated, which is expected to provide new insight into HCC clinical management.
Author contributions
YY, JD, JH, and BW reviewed the literature. YH and YY drafted the manuscript and designed the figures. YY and ZL revised the manuscript. All authors read and approved the manuscript.
Funding
This work was supported by Guangdong Basic and Applied Basic Research Foundation (2021A1515011261).
Conflict of interest
The authors declare that the research was conducted in the absence of any commercial or financial relationships that could be construed as a potential conflict of interest.
Publisher’s note
All claims expressed in this article are solely those of the authors and do not necessarily represent those of their affiliated organizations, or those of the publisher, the editors and the reviewers. Any product that may be evaluated in this article, or claim that may be made by its manufacturer, is not guaranteed or endorsed by the publisher.
Abbreviations
AFP, alpha-fetoprotein; ASGPR, sialoglycoprotein receptor; BCLC, Barcelona clinic liver cancer; CD44, cluster of differentiation 44; CD45, cluster of differentiation 45; CD90, cluster of differentiation 90; CD133, cluster of differentiation 133; CPS1, carbamoyl phosphate synthetase 1; CSCs, circulating cancer stem cells; CTCs, Circulating tumor cells; EMT, Epithelial-mesenchymal transformation; EpCAM, epithelial Cell adhesion molecule; GPC3, glypican-3; HCC, Hepatocellular carcinoma; Hep Par 1, hepatocyte paraffin 1; IF, immunofluorescence; iFISH, immunofluorescence in situ hybridization; MET, mesenchymal-epithelial transition; MMP, matrix metalloproteinase; pAkt, phosphorylated protein kinase B; PD-L1, programmed cell death ligand 1; pERK, phosphorylated extracellular signal-regulated kinase; qRT-PCR, quantitative real-time PCR; TNM, tumor-node-metastasis; AUC, area under the curve; BLD, benign liver disease; CTCs, circulating tumor cells; DFS, disease-free survival; EMT, epithelial to mesenchymal transition; HCC, hepatocellular carcinoma; HV, healthy volunteers; IF, immunofluorescence; LTx, liver transplantation; OS, overall survival; PFS, progression-free survival; RFS, recurrence-free survival; TACE, transcatheter arterial chemoembolization; TNM, tumor-node-metastasis
References
1. Bray F, Ferlay J, Soerjomataram I, Siegel RL, Torre LA, Jemal A. Global cancer statistics 2018: GLOBOCAN estimates of incidence and mortality worldwide for 36 cancers in 185 countries. CA Cancer J Clin (2018) 68(6):394–424. doi: 10.3322/caac.21492
2. Marrero JA, Kulik LM, Sirlin CB, Zhu AX, Finn RS, Abecassis MM, et al. Diagnosis, staging, and management of hepatocellular carcinoma: 2018 practice guidance by the American association for the study of liver diseases. Hepatology (2018) 68(2):723–50. doi: 10.1002/hep.29913
3. Pelizzaro F, Vitale A, Sartori A, Vieno A, Penzo B, Russo FP, et al. Surveillance as determinant of long-term survival in non-transplanted hepatocellular carcinoma patients. Cancers (Basel) (2021) 13(4):897. doi: 10.3390/cancers13040897
4. Forner A, Reig M, Bruix J. Hepatocellular carcinoma. Lancet (2018) 391(10127):1301–14. doi: 10.1016/S0140-6736(18)30010-2
5. Galle PR, Foerster F, Kudo M, Chan SL, Llovet JM, Qin S, et al. Biology and significance of alpha-fetoprotein in hepatocellular carcinoma. Liver Int (2019) 39(12):2214–29. doi: 10.1111/liv.14223
6. Bruix J, Sherman M, American Association for the Study of Liver D. Management of hepatocellular carcinoma: an update. Hepatology (2011) 53(3):1020–2. doi: 10.1002/hep.24199
7. Khan IM, Gjuka D, Jiao J, Song X, Wang Y, Wang J, et al. A novel biomarker panel for the early detection and risk assessment of hepatocellular carcinoma in patients with cirrhosis. Cancer Prev Res (Phila) (2021) 14(6):667–74. doi: 10.1158/1940-6207.CAPR-20-0600
8. Zhao S, Long M, Zhang X, Lei S, Dou W, Hu J, et al. The diagnostic value of the combination of golgi protein 73, glypican-3 and alpha-fetoprotein in hepatocellular carcinoma: a diagnostic meta-analysis. Ann Transl Med (2020) 8(8):536. doi: 10.21037/atm.2020.02.89
9. Gatselis NK, Tornai T, Shums Z, Zachou K, Saitis A, Gabeta S, et al. Golgi protein-73: A biomarker for assessing cirrhosis and prognosis of liver disease patients. World J Gastroenterol (2020) 26(34):5130–45. doi: 10.3748/wjg.v26.i34.5130
10. Russo FP, Imondi A, Lynch EN, Farinati F. When and how should we perform a biopsy for HCC in patients with liver cirrhosis in 2018? a review. Dig Liver Dis (2018) 50(7):640–6. doi: 10.1016/j.dld.2018.03.014
11. Li J, Han X, Yu X, Xu Z, Yang G, Liu B, et al. Clinical applications of liquid biopsy as prognostic and predictive biomarkers in hepatocellular carcinoma: circulating tumor cells and circulating tumor DNA. J Exp Clin Cancer Res (2018) 37(1):213. doi: 10.1186/s13046-018-0893-1
12. Yin CQ, Yuan CH, Qu Z, Guan Q, Chen H, Wang FB. Liquid biopsy of hepatocellular carcinoma: Circulating tumor-derived biomarkers. Dis Markers (2016) 2016:1427849. doi: 10.1155/2016/1427849
13. Chen J, Cao SW, Cai Z, Zheng L, Wang Q. Epithelial-mesenchymal transition phenotypes of circulating tumor cells correlate with the clinical stages and cancer metastasis in hepatocellular carcinoma patients. Cancer biomark (2017) 20(4):487–98. doi: 10.3233/CBM-170315
14. Dirix L, Buys A, Oeyen S, Peeters D, Liegeois V, Prove A, et al. Circulating tumor cell detection: A prospective comparison between CellSearch(R) and RareCyte(R) platforms in patients with progressive metastatic breast cancer. Breast Cancer Res Treat (2022) 193(2):437–44. doi: 10.1007/s10549-022-06585-5
15. Tamminga M, de Wit S, van de Wauwer C, van den Bos H, Swennenhuis JF, Klinkenberg TJ, et al. Analysis of released circulating tumor cells during surgery for non-small cell lung cancer. Clin Cancer Res (2020) 26(7):1656–66. doi: 10.1158/1078-0432.CCR-19-2541
16. Riethdorf S, O'Flaherty L, Hille C, Pantel K. Clinical applications of the CellSearch platform in cancer patients. Adv Drug Delivery Rev (2018) 125:102–21. doi: 10.1016/j.addr.2018.01.011
17. Heidrich I, Ackar L, Mossahebi Mohammadi P, Pantel K. Liquid biopsies: Potential and challenges. Int J Cancer (2021) 148(3):528–45. doi: 10.1002/ijc.33217
18. Cui K, Ou Y, Shen Y, Li S, Sun Z. Clinical value of circulating tumor cells for the diagnosis and prognosis of hepatocellular carcinoma (HCC): A systematic review and meta-analysis. Med (Baltimore) (2020) 99(40):e22242. doi: 10.1097/MD.0000000000022242
19. Hurtado P, Martinez-Pena I, Pineiro R. Dangerous liaisons: Circulating tumor cells (CTCs) and cancer-associated fibroblasts (CAFs). Cancers (Basel) (2020) 12(10):2861. doi: 10.3390/cancers12102861
20. Agashe R, Kurzrock R. Circulating tumor cells: From the laboratory to the cancer clinic. Cancers (Basel) (2020) 12(9):2361. doi: 10.3390/cancers12092361
21. Massague J, Obenauf AC. Metastatic colonization by circulating tumour cells. Nature (2016) 529(7586):298–306. doi: 10.1038/nature17038
22. Wu S, Liu S, Liu Z, Huang J, Pu X, Li J, et al. Classification of circulating tumor cells by epithelial-mesenchymal transition markers. PloS One (2015) 10(4):e0123976. doi: 10.1371/journal.pone.0123976
23. Aceto N, Bardia A, Miyamoto DT, Donaldson MC, Wittner BS, Spencer JA, et al. Circulating tumor cell clusters are oligoclonal precursors of breast cancer metastasis. Cell (2014) 158(5):1110–22. doi: 10.1016/j.cell.2014.07.013
24. Gkountela S, Castro-Giner F, Szczerba BM, Vetter M, Landin J, Scherrer R, et al. Circulating tumor cell clustering shapes DNA methylation to enable metastasis seeding. Cell (2019) 176(1-2):98–112 e14. doi: 10.1016/j.cell.2018.11.046
25. Yu M, Bardia A, Wittner BS, Stott SL, Smas ME, Ting DT, et al. Circulating breast tumor cells exhibit dynamic changes in epithelial and mesenchymal composition. Science (2013) 339(6119):580–4. doi: 10.1126/science.1228522
26. Heeke S, Mograbi B, Alix-Panabieres C, Hofman P. Never travel alone: The crosstalk of circulating tumor cells and the blood microenvironment. Cells (2019) 8(7):714. doi: 10.3390/cells8070714
27. Hou HW, Warkiani ME, Khoo BL, Li ZR, Soo RA, Tan DS, et al. Isolation and retrieval of circulating tumor cells using centrifugal forces. Sci Rep (2013) 3:1259. doi: 10.1038/srep01259
28. Low WS, Wan Abas WA. Benchtop technologies for circulating tumor cells separation based on biophysical properties. BioMed Res Int (2015) 2015:239362. doi: 10.1155/2015/239362
29. Chen K, Amontree J, Varillas J, Zhang J, George TJ, Fan ZH. Incorporation of lateral microfiltration with immunoaffinity for enhancing the capture efficiency of rare cells. Sci Rep (2020) 10(1):14210. doi: 10.1038/s41598-020-71041-7
30. Wang L, Li Y, Xu J, Zhang A, Wang X, Tang R, et al. Quantified postsurgical small cell size CTCs and EpCAM(+) circulating tumor stem cells with cytogenetic abnormalities in hepatocellular carcinoma patients determine cancer relapse. Cancer Lett (2018) 412:99–107. doi: 10.1016/j.canlet.2017.10.004
31. Ito H, Yamaguchi N, Onimaru M, Kimura S, Ohmori T, Ishikawa F, et al. Change in number and size of circulating tumor cells with high telomerase activity during treatment of patients with gastric cancer. Oncol Lett (2016) 12(6):4720–6. doi: 10.3892/ol.2016.5239
32. Dolfus C, Piton N, Toure E, Sabourin JC. Circulating tumor cell isolation: the assets of filtration methods with polycarbonate track-etched filters. Chin J Cancer Res (2015) 27(5):479–87. doi: 10.3978/j.issn.1000-9604.2015.09.01
33. Russo GI, Musso N, Romano A, Caruso G, Petralia S, Lanzano L, et al. The role of dielectrophoresis for cancer diagnosis and prognosis. Cancers (Basel) (2021) 14(1):198. doi: 10.3390/cancers14010198
34. Renier C, Pao E, Che J, Liu HE, Lemaire CA, Matsumoto M, et al. Label-free isolation of prostate circulating tumor cells using vortex microfluidic technology. NPJ Precis Oncol (2017) 1(1):15. doi: 10.1038/s41698-017-0015-0
35. Undvall Anand E, Magnusson C, Lenshof A, Ceder Y, Lilja H, Laurell T. Two-step acoustophoresis separation of live tumor cells from whole blood. Anal Chem (2021) 93(51):17076–85. doi: 10.1021/acs.analchem.1c04050
36. Hoshino K, Huang YY, Lane N, Huebschman M, Uhr JW, Frenkel EP, et al. Microchip-based immunomagnetic detection of circulating tumor cells. Lab Chip (2011) 11(20):3449–57. doi: 10.1039/c1lc20270g
37. Kuai JH, Wang Q, Zhang AJ, Zhang JY, Chen ZF, Wu KK, et al. Epidermal growth factor receptor-targeted immune magnetic liposomes capture circulating colorectal tumor cells efficiently. World J Gastroenterol (2018) 24(3):351–9. doi: 10.3748/wjg.v24.i3.351
38. Schulze K, Gasch C, Staufer K, Nashan B, Lohse AW, Pantel K, et al. Presence of EpCAM-positive circulating tumor cells as biomarker for systemic disease strongly correlates to survival in patients with hepatocellular carcinoma. Int J Cancer (2013) 133(9):2165–71. doi: 10.1002/ijc.28230
39. Kelley RK, Magbanua MJ, Butler TM, Collisson EA, Hwang J, Sidiropoulos N, et al. Circulating tumor cells in hepatocellular carcinoma: a pilot study of detection, enumeration, and next-generation sequencing in cases and controls. BMC Cancer (2015) 15:206. doi: 10.1186/s12885-015-1195-z
40. von Felden J, Schulze K, Krech T, Ewald F, Nashan B, Pantel K, et al. Circulating tumor cells as liquid biomarker for high HCC recurrence risk after curative liver resection. Oncotarget (2017) 8(52):89978–87. doi: 10.18632/oncotarget.21208
41. Yu JJ, Xiao W, Dong SL, Liang HF, Zhang ZW, Zhang BX, et al. Effect of surgical liver resection on circulating tumor cells in patients with hepatocellular carcinoma. BMC Cancer (2018) 18(1):835. doi: 10.1186/s12885-018-4744-4
42. Zhou KQ, Sun YF, Cheng JW, Du M, Ji Y, Wang PX, et al. Effect of surgical margin on recurrence based on preoperative circulating tumor cell status in hepatocellular carcinoma. EBioMedicine (2020) 62:103107. doi: 10.1016/j.ebiom.2020.103107
43. Wang PX, Sun YF, Zhou KQ, Cheng JW, Hu B, Guo W, et al. Circulating tumor cells are an indicator for the administration of adjuvant transarterial chemoembolization in hepatocellular carcinoma: A single-center, retrospective, propensity-matched study. Clin Transl Med (2020) 10(3):e137. doi: 10.1002/ctm2.137
44. Sun YF, Wang PX, Cheng JW, Gong ZJ, Huang A, Zhou KQ, et al. Postoperative circulating tumor cells: An early predictor of extrahepatic metastases in patients with hepatocellular carcinoma undergoing curative surgical resection. Cancer Cytopathol (2020) 128(10):733–45. doi: 10.1002/cncy.22304
45. Lowes LE, Allan AL. Circulating tumor cells and implications of the epithelial-to-Mesenchymal transition. Adv Clin Chem (2018) 83:121–81. doi: 10.1016/bs.acc.2017.10.004
46. Joosse SA, Gorges TM, Pantel K. Biology, detection, and clinical implications of circulating tumor cells. EMBO Mol Med (2015) 7(1):1–11. doi: 10.15252/emmm.201303698
47. Liu HY, Qian HH, Zhang XF, Li J, Yang X, Sun B, et al. Improved method increases sensitivity for circulating hepatocellular carcinoma cells. World J Gastroenterol (2015) 21(10):2918–25. doi: 10.3748/wjg.v21.i10.2918
48. Li J, Shi L, Zhang X, Sun B, Yang Y, Ge N, et al. pERK/pAkt phenotyping in circulating tumor cells as a biomarker for sorafenib efficacy in patients with advanced hepatocellular carcinoma. Oncotarget (2016) 7(3):2646–59. doi: 10.18632/oncotarget.6104
49. Wang PX, Xu Y, Sun YF, Cheng JW, Zhou KQ, Wu SY, et al. Detection of circulating tumour cells enables early recurrence prediction in hepatocellular carcinoma patients undergoing liver transplantation. Liver Int (2021) 41(3):562–73. doi: 10.1111/liv.14734
50. Hamaoka M, Kobayashi T, Tanaka Y, Mashima H, Ohdan H. Clinical significance of glypican-3-positive circulating tumor cells of hepatocellular carcinoma patients: A prospective study. PloS One (2019) 14(5):e0217586. doi: 10.1371/journal.pone.0217586
51. Guo W, Yang XR, Sun YF, Shen MN, Ma XL, Wu J, et al. Clinical significance of EpCAM mRNA-positive circulating tumor cells in hepatocellular carcinoma by an optimized negative enrichment and qRT-PCR-based platform. Clin Cancer Res (2014) 20(18):4794–805. doi: 10.1158/1078-0432.CCR-14-0251
52. Zhang J, Peng H, Wang B, Luo L, Cheng Y, He G, et al. Efficacy of postoperative adjuvant transcatheter arterial chemoembolization in hepatocellular carcinoma patients with mesenchymal circulating tumor cell. J Gastrointest Surg (2021) 25(7):1770–8. doi: 10.1007/s11605-020-04755-8
53. Zhu L, Lin H, Wan S, Chen X, Wu L, Zhu Z, et al. Efficient isolation and phenotypic profiling of circulating hepatocellular carcinoma cells via a combinatorial-Antibody-Functionalized microfluidic synergetic-chip. Anal Chem (2020) 92(22):15229–35. doi: 10.1021/acs.analchem.0c03936
54. Lin Z, Luo G, Du W, Kong T, Liu C, Liu Z. Recent advances in microfluidic platforms applied in cancer metastasis: Circulating tumor cells' (CTCs) isolation and tumor-On-A-Chip. Small (2020) 16(9):e1903899. doi: 10.1002/smll.201903899
55. Zhong Y, Ma T, Qiao T, Hu H, Li Z, Luo K, et al. Role of phenotypes of circulating tumor cells in the diagnosis and treatment of colorectal cancer. Cancer Manag Res (2021) 13:7077–85. doi: 10.2147/CMAR.S316544
56. Liu YK, Hu BS, Li ZL, He X, Li Y, Lu LG. An improved strategy to detect the epithelial-mesenchymal transition process in circulating tumor cells in hepatocellular carcinoma patients. Hepatol Int (2016) 10(4):640–6. doi: 10.1007/s12072-016-9732-7
57. Ou H, Huang Y, Xiang L, Chen Z, Fang Y, Lin Y, et al. Circulating tumor cell phenotype indicates poor survival and recurrence after surgery for hepatocellular carcinoma. Dig Dis Sci (2018) 63(9):2373–80. doi: 10.1007/s10620-018-5124-2
58. Yin LC, Luo ZC, Gao YX, Li Y, Peng Q, Gao Y. Twist expression in circulating hepatocellular carcinoma cells predicts metastasis and prognoses. BioMed Res Int (2018) 2018:3789613. doi: 10.1155/2018/3789613
59. Qi LN, Xiang BD, Wu FX, Ye JZ, Zhong JH, Wang YY, et al. Circulating tumor cells undergoing EMT provide a metric for diagnosis and prognosis of patients with hepatocellular carcinoma. Cancer Res (2018) 78(16):4731–44. doi: 10.1158/0008-5472.CAN-17-2459
60. Wang Z, Luo L, Cheng Y, He G, Peng B, Gao Y, et al. Correlation between postoperative early recurrence of hepatocellular carcinoma and mesenchymal circulating tumor cells in peripheral blood. J Gastrointest Surg (2018) 22(4):633–9. doi: 10.1007/s11605-017-3619-3
61. Cheng Y, Luo L, Zhang J, Zhou M, Tang Y, He G, et al. Diagnostic value of different phenotype circulating tumor cells in hepatocellular carcinoma. J Gastrointest Surg (2019) 23(12):2354–61. doi: 10.1007/s11605-018-04067-y
62. Meng J, Chen S, Han JX, Qian B, Wang XR, Zhong WL, et al. Twist1 regulates vimentin through Cul2 circular RNA to promote EMT in hepatocellular carcinoma. Cancer Res (2018) 78(15):4150–62. doi: 10.1158/0008-5472.CAN-17-3009
63. Pal M, Bhattacharya S, Kalyan G, Hazra S. Cadherin profiling for therapeutic interventions in epithelial mesenchymal transition (EMT) and tumorigenesis. Exp Cell Res (2018) 368(2):137–46. doi: 10.1016/j.yexcr.2018.04.014
64. Yang J, Antin P, Berx G, Blanpain C, Brabletz T, Bronner M, et al. Guidelines and definitions for research on epithelial-mesenchymal transition. Nat Rev Mol Cell Biol (2020) 21(6):341–52. doi: 10.1038/s41580-020-0237-9
66. Dongre A, Weinberg RA. New insights into the mechanisms of epithelial-mesenchymal transition and implications for cancer. Nat Rev Mol Cell Biol (2019) 20(2):69–84. doi: 10.1038/s41580-018-0080-4
67. Greaves D, Calle Y. Epithelial mesenchymal transition (EMT) and associated invasive adhesions in solid and haematological tumours. Cells (2022) 11(4):649. doi: 10.3390/cells11040649
68. Nieto MA, Huang RY, Jackson RA, Thiery JP. Emt: 2016. Cell (2016) 166(1):21–45. doi: 10.1016/j.cell.2016.06.028
69. Scheau C, Badarau IA, Costache R, Caruntu C, Mihai GL, Didilescu AC, et al. The role of matrix metalloproteinases in the epithelial-mesenchymal transition of hepatocellular carcinoma. Anal Cell Pathol (Amst) (2019) 2019:9423907. doi: 10.1155/2019/9423907
70. Stavropoulou V, Kaspar S, Brault L, Sanders MA, Juge S, Morettini S, et al. MLL-AF9 expression in hematopoietic stem cells drives a highly invasive AML expressing EMT-related genes linked to poor outcome. Cancer Cell (2016) 30(1):43–58. doi: 10.1016/j.ccell.2016.05.011
71. Beerling E, Seinstra D, de Wit E, Kester L, van der Velden D, Maynard C, et al. Plasticity between epithelial and mesenchymal states unlinks EMT from metastasis-enhancing stem cell capacity. Cell Rep (2016) 14(10):2281–8. doi: 10.1016/j.celrep.2016.02.034
72. Terry S, Savagner P, Ortiz-Cuaran S, Mahjoubi L, Saintigny P, Thiery JP, et al. New insights into the role of EMT in tumor immune escape. Mol Oncol (2017) 11(7):824–46. doi: 10.1002/1878-0261.12093
73. Yu X, Wang Q, Liu B, Zhang N, Cheng G. Vitamin d enhances radiosensitivity of colorectal cancer by reversing epithelial-mesenchymal transition. Front Cell Dev Biol (2021) 9:684855. doi: 10.3389/fcell.2021.684855
74. Zhang N, Ng AS, Cai S, Li Q, Yang L, Kerr D. Novel therapeutic strategies: targeting epithelial-mesenchymal transition in colorectal cancer. Lancet Oncol (2021) 22(8):e358–68. doi: 10.1016/S1470-2045(21)00343-0
75. Zhang B, Li Y, Wu Q, Xie L, Barwick B, Fu C, et al. Acetylation of KLF5 maintains EMT and tumorigenicity to cause chemoresistant bone metastasis in prostate cancer. Nat Commun (2021) 12(1):1714. doi: 10.1038/s41467-021-21976-w
76. Bakir B, Chiarella AM, Pitarresi JR, Rustgi AK. EMT, MET, plasticity, and tumor metastasis. Trends Cell Biol (2020) 30(10):764–76. doi: 10.1016/j.tcb.2020.07.003
77. Ocana OH, Corcoles R, Fabra A, Moreno-Bueno G, Acloque H, Vega S, et al. Metastatic colonization requires the repression of the epithelial-mesenchymal transition inducer Prrx1. Cancer Cell (2012) 22(6):709–24. doi: 10.1016/j.ccr.2012.10.012
78. Tsai JH, Donaher JL, Murphy DA, Chau S, Yang J. Spatiotemporal regulation of epithelial-mesenchymal transition is essential for squamous cell carcinoma metastasis. Cancer Cell (2012) 22(6):725–36. doi: 10.1016/j.ccr.2012.09.022
79. Pei D, Shu X, Gassama-Diagne A, Thiery JP. Mesenchymal-epithelial transition in development and reprogramming. Nat Cell Biol (2019) 21(1):44–53. doi: 10.1038/s41556-018-0195-z
80. Jolly MK, Ware KE, Gilja S, Somarelli JA, Levine H. Emt and Met: necessary or permissive for metastasis? Mol Oncol. (2017) 11(7):755–69. doi: 10.1002/1878-0261.12083
81. Fang ZT, Zhang W, Wang GZ, Zhou B, Yang GW, Qu XD, et al. Circulating tumor cells in the central and peripheral venous compartment - assessing hematogenous dissemination after transarterial chemoembolization of hepatocellular carcinoma. Onco Targets Ther (2014) 7:1311–8. doi: 10.2147/OTT.S62605
82. Li YM, Xu SC, Li J, Han KQ, Pi HF, Zheng L, et al. Epithelial-mesenchymal transition markers expressed in circulating tumor cells in hepatocellular carcinoma patients with different stages of disease. Cell Death Dis (2013) 4:e831. doi: 10.1038/cddis.2013.347
83. Sun YF, Guo W, Xu Y, Shi YH, Gong ZJ, Ji Y, et al. Circulating tumor cells from different vascular sites exhibit spatial heterogeneity in epithelial and mesenchymal composition and distinct clinical significance in hepatocellular carcinoma. Clin Cancer Res (2018) 24(3):547–59. doi: 10.1158/1078-0432.CCR-17-1063
84. Ha Y, Kim TH, Shim JE, Yoon S, Jun MJ, Cho YH, et al. Circulating tumor cells are associated with poor outcomes in early-stage hepatocellular carcinoma: a prospective study. Hepatol Int (2019) 13(6):726–35. doi: 10.1007/s12072-019-09994-9
85. Takahashi K, Ofuji K, Hiramatsu K, Nosaka T, Naito T, Matsuda H, et al. Circulating tumor cells detected with a microcavity array predict clinical outcome in hepatocellular carcinoma. Cancer Med (2021) 10(7):2300–9. doi: 10.1002/cam4.3790
86. Xu W, Cao L, Chen L, Li J, Zhang XF, Qian HH, et al. Isolation of circulating tumor cells in patients with hepatocellular carcinoma using a novel cell separation strategy. Clin Cancer Res (2011) 17(11):3783–93. doi: 10.1158/1078-0432.CCR-10-0498
87. Li J, Chen L, Zhang X, Zhang Y, Liu H, Sun B, et al. Detection of circulating tumor cells in hepatocellular carcinoma using antibodies against asialoglycoprotein receptor, carbamoyl phosphate synthetase 1 and pan-cytokeratin. PloS One (2014) 9(4):e96185. doi: 10.1371/journal.pone.0096185
88. Ogle LF, Orr JG, Willoughby CE, Hutton C, McPherson S, Plummer R, et al. Imagestream detection and characterisation of circulating tumour cells - a liquid biopsy for hepatocellular carcinoma? J Hepatol (2016) 65(2):305–13. doi: 10.1016/j.jhep.2016.04.014
89. Ye X, Li G, Han C, Han Q, Shang L, Su H, et al. Circulating tumor cells as a potential biomarker for postoperative clinical outcome in HBV-related hepatocellular carcinoma. Cancer Manag Res (2018) 10:5639–47. doi: 10.2147/CMAR.S175489
90. Pang Y, Wang C, Xiao R, Sun Z. Dual-selective and dual-enhanced SERS nanoprobes strategy for circulating hepatocellular carcinoma cells detection. Chemistry (2018) 24(27):7060–7. doi: 10.1002/chem.201801133
91. Fan ST, Yang ZF, Ho DW, Ng MN, Yu WC, Wong J. Prediction of posthepatectomy recurrence of hepatocellular carcinoma by circulating cancer stem cells: a prospective study. Ann Surg (2011) 254(4):569–76. doi: 10.1097/SLA.0b013e3182300a1d
92. Sun YF, Xu Y, Yang XR, Guo W, Zhang X, Qiu SJ, et al. Circulating stem cell-like epithelial cell adhesion molecule-positive tumor cells indicate poor prognosis of hepatocellular carcinoma after curative resection. Hepatology (2013) 57(4):1458–68. doi: 10.1002/hep.26151
93. Wan S, Kim TH, Smith KJ, Delaney R, Park GS, Guo H, et al. New labyrinth microfluidic device detects circulating tumor cells expressing cancer stem cell marker and circulating tumor microemboli in hepatocellular carcinoma. Sci Rep (2019) 9(1):18575. doi: 10.1038/s41598-019-54960-y
94. Winograd P, Hou S, Court CM, Lee YT, Chen PJ, Zhu Y, et al. Hepatocellular carcinoma-circulating tumor cells expressing PD-L1 are prognostic and potentially associated with response to checkpoint inhibitors. Hepatol Commun (2020) 4(10):1527–40. doi: 10.1002/hep4.1577
95. Nicolazzo C, Gradilone A, Loreni F, Raimondi C, Gazzaniga P. EpCAM(low) circulating tumor cells: Gold in the waste. Dis Markers (2019) 2019:1718920. doi: 10.1155/2019/1718920
96. Giannelli G, Koudelkova P, Dituri F, Mikulits W. Role of epithelial to mesenchymal transition in hepatocellular carcinoma. J Hepatol (2016) 65(4):798–808. doi: 10.1016/j.jhep.2016.05.007
97. Espejo-Cruz ML, Gonzalez-Rubio S, Zamora-Olaya J, Amado-Torres V, Alejandre R, Sanchez-Frias M, et al. Circulating tumor cells in hepatocellular carcinoma: A comprehensive review and critical appraisal. Int J Mol Sci (2021) 22(23):13073. doi: 10.3390/ijms222313073
98. Chen F, Zhong Z, Tan HY, Wang N, Feng Y. The significance of circulating tumor cells in patients with hepatocellular carcinoma: Real-time monitoring and moving targets for cancer therapy. Cancers (Basel) (2020) 12(7):1734. doi: 10.3390/cancers12071734
99. Sberna AL, Bouillet B, Rouland A, Brindisi MC, Nguyen A, Mouillot T, et al. European Association for the study of the liver (EASL), European association for the study of diabetes (EASD) and European association for the study of obesity (EASO) clinical practice recommendations for the management of non-alcoholic fatty liver disease: evaluation of their application in people with type 2 diabetes. Diabetes Med (2018) 35(3):368–75. doi: 10.1111/dme.13565
100. Tzartzeva K, Obi J, Rich NE, Parikh ND, Marrero JA, Yopp A, et al. Surveillance imaging and alpha fetoprotein for early detection of hepatocellular carcinoma in patients with cirrhosis: A meta-analysis. Gastroenterology (2018) 154(6):1706–1718 e1. doi: 10.1053/j.gastro.2018.01.064
101. Court CM, Hou S, Winograd P, Segel NH, Li QW, Zhu Y, et al. A novel multimarker assay for the phenotypic profiling of circulating tumor cells in hepatocellular carcinoma. Liver Transpl (2018) 24(7):946–60. doi: 10.1002/lt.25062
102. Lv D, Chen L, Du L, Zhou L, Tang H. Emerging regulatory mechanisms involved in liver cancer stem cell properties in hepatocellular carcinoma. Front Cell Dev Biol (2021) 9:691410. doi: 10.3389/fcell.2021.691410
103. Walcher L, Kistenmacher AK, Suo H, Kitte R, Dluczek S, Strauss A, et al. Cancer stem cells-origins and biomarkers: Perspectives for targeted personalized therapies. Front Immunol (2020) 11:1280. doi: 10.3389/fimmu.2020.01280
104. Holczbauer A, Factor VM, Andersen JB, Marquardt JU, Kleiner DE, Raggi C, et al. Modeling pathogenesis of primary liver cancer in lineage-specific mouse cell types. Gastroenterology (2013) 145(1):221–31. doi: 10.1053/j.gastro.2013.03.013
105. Mu X, Espanol-Suner R, Mederacke I, Affo S, Manco R, Sempoux C, et al. Hepatocellular carcinoma originates from hepatocytes and not from the progenitor/biliary compartment. J Clin Invest (2015) 125(10):3891–903. doi: 10.1172/JCI77995
106. Mishra L, Banker T, Murray J, Byers S, Thenappan A, He AR, et al. Liver stem cells and hepatocellular carcinoma. Hepatology (2009) 49(1):318–29. doi: 10.1002/hep.22704
107. Zahran AM, Abdel-Rahim MH, Refaat A, Sayed M, Othman MM, Khalak LMR, et al. Circulating hematopoietic stem cells, endothelial progenitor cells and cancer stem cells in hepatocellular carcinoma patients: contribution to diagnosis and prognosis. Acta Oncol (2020) 59(1):33–9. doi: 10.1080/0284186X.2019.1657940
108. Reig M, Forner A, Rimola J, Ferrer-Fabrega J, Burrel M, Garcia-Criado A, et al. BCLC strategy for prognosis prediction and treatment recommendation: The 2022 update. J Hepatol (2022) 76(3):681–93. doi: 10.1016/j.jhep.2021.11.018
109. Amin MB, Greene FL, Edge SB, Compton CC, Gershenwald JE, Brookland RK, et al. The eighth edition AJCC cancer staging manual: Continuing to build a bridge from a population-based to a more "personalized" approach to cancer staging. CA Cancer J Clin (2017) 67(2):93–9. doi: 10.3322/caac.21388
110. Kamarajah SK, Frankel TL, Sonnenday C, Cho CS, Nathan H. Critical evaluation of the American joint commission on cancer (AJCC) 8th edition staging system for patients with hepatocellular carcinoma (HCC): A surveillance, epidemiology, end results (SEER) analysis. J Surg Oncol (2018) 117(4):644–50. doi: 10.1002/jso.24908
111. Fan JL, Yang YF, Yuan CH, Chen H, Wang FB. Circulating tumor cells for predicting the prognostic of patients with hepatocellular carcinoma: A meta analysis. Cell Physiol Biochem (2015) 37(2):629–40. doi: 10.1159/000430382
112. Xing R, Gao J, Cui Q, Wang Q. Strategies to improve the antitumor effect of immunotherapy for hepatocellular carcinoma. Front Immunol (2021) 12:783236. doi: 10.3389/fimmu.2021.783236
113. Lang L. FDA Approves sorafenib for patients with inoperable liver cancer. Gastroenterology (2008) 134(2):379. doi: 10.1053/j.gastro.2007.12.037
114. Ma Y, Xu R, Liu X, Zhang Y, Song L, Cai S, et al. LY3214996 relieves acquired resistance to sorafenib in hepatocellular carcinoma cells. Int J Med Sci (2021) 18(6):1456–64. doi: 10.7150/ijms.51256
115. Qi F, Qin W, Zhang Y, Luo Y, Niu B, An Q, et al. Sulfarotene, a synthetic retinoid, overcomes stemness and sorafenib resistance of hepatocellular carcinoma via suppressing SOS2-RAS pathway. J Exp Clin Cancer Res (2021) 40(1):280. doi: 10.1186/s13046-021-02085-4
116. Liu H, Zhao L, Wang M, Yang K, Jin Z, Zhao C, et al. FNDC5 causes resistance to sorafenib by activating the PI3K/Akt/Nrf2 pathway in hepatocellular carcinoma cells. Front Oncol (2022) 12:852095. doi: 10.3389/fonc.2022.852095
117. Wang L, Zhan Y, Wu Z, Lin M, Jin X, Jiang L, et al. A novel multitarget kinase inhibitor BZG with potent anticancer activity in vitro and vivo enhances efficacy of sorafenib through PI3K pathways in hepatocellular carcinoma cells. BioMed Pharmacother (2020) 125:110033. doi: 10.1016/j.biopha.2020.110033
118. Ribas A, Wolchok JD. Cancer immunotherapy using checkpoint blockade. Science (2018) 359(6382):1350–5. doi: 10.1126/science.aar4060
Keywords: circulating tumor cells, hepatocellular carcinoma, epithelial-mesenchymal transformation, clinical application, detection methods
Citation: Hua Y, Dong J, Hong J, Wang B, Yan Y and Li Z (2022) Clinical applications of circulating tumor cells in hepatocellular carcinoma. Front. Oncol. 12:968591. doi: 10.3389/fonc.2022.968591
Received: 14 June 2022; Accepted: 01 August 2022;
Published: 24 August 2022.
Edited by:
Tao Sun, Nankai University, ChinaReviewed by:
Evin Iscan, Dokuz Eylül University, TurkeyJiang Chen, Zhejiang University, China
P. Lin, Cytelligen, United States
Copyright © 2022 Hua, Dong, Hong, Wang, Yan and Li. This is an open-access article distributed under the terms of the Creative Commons Attribution License (CC BY). The use, distribution or reproduction in other forums is permitted, provided the original author(s) and the copyright owner(s) are credited and that the original publication in this journal is cited, in accordance with accepted academic practice. No use, distribution or reproduction is permitted which does not comply with these terms.
*Correspondence: Zhiming Li, bHptbGVvQHhtdS5lZHUuY24=; Yong Yan, MjI0NjgyNzA3MEBxcS5jb20=