- 1Clinical Nursing Teaching and Research Section, The Second Xiangya Hospital, Central South University, Changsha, China
- 2Department of Kidney Transplantation, The Second Xiangya Hospital of Central South University, Changsha, China
- 3Department of Pathology, Nihon University, Tokyo, Japan
B cells play a critical role in adaptive immune responses mainly due to antigen presentation and antibody production. Studies about the tumor-infiltrating immune cells so far demonstrated that the function of B cells in tumor immunity is quite different among various tumor types. The antigen presentation of B cells is mainly anti-tumoral, while the role of antibody production is controversial. Moreover, the immunosuppressive regulatory B cells are detrimental to anti-tumor immunity via the secretion of various anti-inflammatory cytokines. This review briefly summarizes the different roles of B cells classified by the primary function of B cells, antigen presentation, antibody production, and immunity regulation. Further, it discusses the potential therapeutic target of B cells in tumor immunity.
Introduction
Cancer is still a threat to humanity due to its high death rate (1). Various therapies have been developed to treat cancer, including surgery, radiotherapy, chemotherapy, immunotherapy, etc. Among these therapies, immunotherapy has become more and more attractive for researchers, companies, and clinicians in recent years (2). T cell-based immunotherapy is critical and effective in cancer therapy, and the promising outcome of the antibodies targeting immune checkpoints in the treatment of cancer created a grave impact on immunotherapy (2, 3). Though the CD8+ T cells have an irreplaceable role in the cytotoxicity in the tumor microenvironment (TME) (4) and immune checkpoint inhibitors (ICIs) are quite efficient in many cancer types, most patients are still resistant to ICIs (5). Increasing studies demonstrated the function of other immune cells in the development of cancer in recent years (6, 7), which might be additional and optimal targets for the treatment of cancer.
B cells are involved in adaptive immunity as the antigen-presenting cells (APCs) and antibody-secreting cells (ASCs), while the function of B cells in cancer immunity is controversial. B cell depletion in mice by anti-IgM treatment from birth showed resistance to syngeneic fibrosarcoma and reduced incidence of pulmonary metastasis (8). In contrast, the lung adenocarcinoma cell inoculation in µMT mice failed to show any difference to WT mice (9), yet the µMT mice had faster tumor growth than WT mice when the tumor cell line was transfected with B cell-specific neoantigen (9). The function of B cells in tumor growth seems to vary among different tumor cell lines. Moreover, antibody production from B cells is not always beneficial. For example, antibody-dependent cellular cytotoxicity (ADCC) is a critical mechanism of the antibody in the anti-tumor effect of B cells (10), while the immune complexes in circulation or TME are correlated with poor clinical outcomes (11).
In this review, we will briefly discuss the immunological mechanism of B cells in cancer immunity to elucidate the controversial phenomenon in various tumor types and potential therapeutic targets of B cells in different tumor types. This review is classified by the basic functions of B cells, but not anti- and pro- tumoral functions of B cells, which is already discussed in other reviews (12).
Antigen-presenting cells
B cells are efficient APCs in T cell-dependent (TD) antigen-induced humoral immunity. TD antigens are recognized and engulfed by B cells through B cell receptor (BCR), degraded in lysosome, and presented to CD4+ cells, resulting in CD4+ T cells and further CD8+ cells activation (13). Several studies demonstrated the antigen presentation of B cells plays a critical role in tumor-specific CD4+ and CD8+ T cell activation. B cells undoubtedly present antigen to induce T cell activation in virus-induced tumor growth (14). In the syngeneic B16 melanoma cell line transfer system, B cell depletion by anti-CD20 antibody treatment resulted in a two-fold bigger tumor volume and impaired interferon-γ (IFN- γ) and tumor necrosis factor (TNF-α) production from CD4+ T cells and CD8+ T cells (15).
A recent study elucidated how antigen presentation of B cells plays a role in tumor immunity. T follicular helper (TFH) cells are involved in B cell maturation and activation. Germinal center (GC) B cells could be activated by TFH-B interaction and further differentiate into short-term living plasma cells, long-term living plasmablasts, and memory B cells. The single-cell RNA sequencing result of tumor-infiltrating lymphocytes in many studies revealed the presence of GC B cells in the TME (9, 16), yet the role of GC B cells is not well known. The study done by Cui et al. in lung adenocarcinoma patients elucidated that GC B cells facilitate the function of CD8+ T cells in anti-tumor immunity via the TFH-GC B cell interaction in a neoantigen-dependent manner (9). They utilized a lung adenocarcinoma cell line (KP) with limited somatic mutations, which means that there are few or no neoantigen expression and weak B/T cell responses so that B cell or T cell depletion doesn’t affect the tumor growth. With the transfection of HELLO fusion protein, which contains HEL, GP33, and GP66 that can be recognized by MD4 transgenic BCR, GP33-specific CD4 TCR, and GP66-specific CD8 TCR, respectively, KP-HELLO cells are able to activate specific B/T cells. The inoculation of KP-HELLO cells in B cell knockout or TFH knockout mice showed much faster tumor growth and weaker CD8+ T cell function compared to tumor growth inoculated in WT mice, suggesting that the GC B cells that recognize the neoantigen and further interact with activated CD4+ T cells are able to support CD8+ T cells function in TME. Further results demonstrated that interaction between neoantigen-specific TFH and GC B cells and interleukin-21 (IL-21) secreted by TFH cells are necessary for the cytotoxicity of CD8+ T cells (9).
Antibody-secreting cells
B cells play an essential role in the adaptive immune responses by producing antibodies (17). At the same time, the role of antibody-secreting B cells is a double-edged sword in tumor immunity. Once the B cells are activated by recognizing the neoantigen, B cells participate in a two-pathway differentiation process that induces both short-lived plasmablasts and long-lived plasma cells and memory B cells (17). Therefore, these plasmablasts, plasma cells, memory B cells, and the secreted antibodies are neoantigen-specific. Both BCR signaling that provides binding to the antigen, and the B-T cell interaction are essential in the TD antigen-involved long-term antibody production (17).
Commonly, the antibodies are thought to be anti-tumoral. Antibodies with high FcγR affinity and target neoantigens expressed on tumor cell surface induce ADCC, antibody-dependent cellular phagocytosis (ADCP), and complement-dependent cytotoxicity (CDC), which are significant mechanisms of antibody drugs for cancer therapy. For example, the Fc domain of the monoclonal antibody (mAb) has a different affinity to different FcγR expressed on various immune cells (18), among which natural killer (NK) cell is involved in ADCC and is discussed in many mAb treatments in cancer (10, 19). Several mAbs have been used in the clinic based on their cytotoxicities, such as anti-GD2 mAb for melanoma and neuroblastoma treatment (20–23) and chimeric anti-CD20 mAb and anti-CD22 mAb for leukemia treatment (24–27).
Unfortunately, not all of the antibodies contribute to anti-tumoral immunity. Antibodies bind to various antigens released by tumor cells and form circulating immune complexes (CICs), which correlate with poor outcomes (11). In the squamous cell carcinoma mouse model, CICs accumulate in the dermal stroma of neoplastic tissue, activate FcγR on residents, and recruit pro-tumoral and angiogenic myeloid cells (especially mast cells and macrophages) to facilitate tumor cell survival and angiogenesis (28).
Except for IgG, IgA is also a double-edged sword for tumor growth. Many studies have found the accumulation of IgA-producing B cells in TME (29, 30), yet the role of IgA in tumor growth is still controversial. In ovarian cancer patients, tumor-infiltrating B cell-derived IgA dampens tumor growth through the unspecific transcytosis and neoantigen-specific phagocytosis (29). Yet the function of IgA in other cancers is entirely different. Several cancer types have shown that the proportion of IgA-producing cells is highly associated with poor outcomes (31–33). IgA is pro-tumoral in these cases and has the following mechanisms. Firstly, the IgA production is not induced by neoantigen presentation but by the immunosuppressive microenvironment, and the IgA cannot mediate ADCC (34, 35). Secondly, IgA is immunosuppressive in mucosal immunity (36). IgA deficiency leads to a higher risk of inflammation (37–39), and the interaction between IgA and marginal zone B and B1 cell-specific protein (MZB1) may be an important factor (36). What’s more, IgA induces anti-inflammatory cytokine interleukin-10 (IL-10) production from monocytes and further inhibits the immune system (40).
Regulatory B cells
The discovery of a population of the suppressive function of B cells can be retrospect to 1974 since B cells could delay hypersensitivity (41, 42). Subsequently, more and more papers found that some B cells inhibit the development of various diseases such as experimental autoimmune encephalomyelitis (EAE) (43), allograft rejection (44, 45), lupus nephritis (LN) (46), type 1 diabetes (T1D) (47, 48), anti-neutrophil cytoplasmic antibody (ANCA)-associated vasculitis (AAV) (49) and so on. These B cells regulate immune responses by secreting anti-inflammatory cytokines such as IL-10 (50–54), IL-35 (55–57), and transforming growth factor-β (TGF-β) (58, 59) to dampen CD4+ T cells (60), CD8+ T cells (53), antibody production (61) and facilitate regulatory T (Treg) cells (62, 63). These B cells are so-called Breg cells. Breg cells are not restricted to a specific B cell phenotype. Therefore, IL-10-producing B cells, for example, are usually utilized to detect Breg cells. Since Breg cells vary in various phenotypes, those types of B cells all have an inhibitory function in immune responses. The phenotype of Breg cells mainly includes transitional B cells (CD19+CD24hiCD38hi) (64) and plasmablasts (CD19+CD27intCD38+) (65) in human, follicular B cells (CD19+CD23+CD21int), marginal zone B cells (CD19+CD23-CD21hi), plasma/plasmablasts (CD19+/B220lo/-CD138+), transitional B cells and B10 cells (CD19+/B220lo/-CD1d+CD5+) in mice (50).
Breg cells can not only impair immune responses in TME by secreting antibodies as described above but many anti-inflammatory cytokines production and pathways also contribute to immunosuppression in TME. IL-10 is the most important anti-inflammatory cytokine defining the Breg cells, several pathways are involved in IL-10 production (66, 67). For example, IL-10 production is increased from B cells when stimulated with LPS or CpG (68–70), and MyD88, the downstream of TLR, is necessary for IL-10 production from B cells under LPS stimulation (71), suggesting that TLR activation is able to induce Breg cells differentiation. CD40 and BCR signaling are also related to IL-10 production, as anti-CD40 antibody treatment in vivo and in vitro expands the IL-10+ B cells, and antigen-stimulated B cells transfer in the EAE mouse model rescued IL-10 production in a CD40-dependent manner (72, 73). B cell-derived IL-10 is a strong immunosuppressive cytokine in various autoimmune diseases, it is also important in tumor growth. B cell-deficient mice showed slower tumor growth than WT mice when the mice bearing MC38 carcinoma and EL4 thymoma, and this effect is related to the B cell-derived IL-10 (74, 75). IFN- γ production reduced from B cell-knock out splenic cells when cocultured with WT B cells, and IL-10 production from B cells increased after coculturing with irradiated melanoma cells, not sarcoma cells, indicating that Breg cells suppress the anti-tumor immunity to certain tumors (75). IL-10 production from B cells impairs inflammatory cytokines, including TNF-α and IFN-γ, secretion from cytotoxic T cells to promote tumor growth. While in the chemical carcinogenesis of skin, TNF-α is a promoter for tumor growth, IL-10 produced by B cells facilitates tumor growth in a TNF-α-dependent manner (76). Moreover, IL-10-producing B cells are also being found to promote tumor growth in non-Hodgkin B cell lymphoma (77).
TGF-β is another critical anti-inflammatory cytokine secreted by Breg cells. In the breast tumor model, TGF-β is highly expressed on tumor-infiltrating B cells and associated with the conversion of resting CD4+ T cells to Treg cells (78, 79). Furthermore, IL-35 produced by Breg cells also plays a promotion role in pancreatic tumor growth (80, 81). Altogether, Breg cells suppress anti-tumor immunity via the secretion of anti-inflammatory cytokines such as IL-10, TGF-β and IL-35.
Anti- and pro-tumorigenic factors secreted by B cells
Except for the antibodies and cytokines described above, B cells also secrete some other factors that affect tumor growth. Lymphotoxin α1β2 (LTα1β2) plays a critical role in the lymphoid organ development and especially in ectopic tertiary lymphoid organs (82–84). Indeed, the presence of B cells in tertiary lymphoid organs is associated with better anti-tumor immunity in lung cancer (85). Though the remodeling of lymphoid organs contributes to the anti-tumor immunity, some studies found that lymphotoxin derived from B cells supports tumor growth. Androgen promotes prostate cancer (CaP) growth by binding to the androgen receptor expressed on both normal and cancerous prostate cancer cells. Androgen ablation by castration induces cell death of cancer cells and lymphocyte infiltration in TME, and it is effective for androgen-dependent CaP patients, while many patients are castration-resistant (CR). B cells are abundant in TME of CaP, and the B cell-derived lymphotoxin in TME activates IKKα, which is involved in nuclear factor κB (NF-κB) signaling and promotes metastasis, and STAT3, leading to CR-CaP and prostate tumor growth (86, 87).
In addition, a recent study found that γ-Aminobutyric acid (GABA) derived from B cells promotes tumor growth by facilitating IL10+ macrophages in TME (88). In the study of MC38 colon cancer cell line inoculation in vivo, which is reported that B cells suppress anti-tumor T cell responses in this cell line (89, 90), and B cells secreted GABA promotes tumor growth by facilitating IL-10 production from macrophages. Though GABA production is not restricted to B cells, GABA production from B cells is much more than other immune cells in draining lymph nodes. In addition, B cell-specific GABA depletion restored anti-tumor immunity (88). Therefore, the metabolism network of tumor-infiltrating immune cells could be a valuable target for therapy.
Discussion
The function of B cells in cancer development is controversial. Different B cell phenotypes play a different role in various cancer (Figure 1). When the tumor cells express neoantigens containing BCR epitope, B cells can present these neoantigens and interact with neoantigen-activated TFH cells to facilitate the cytotoxicity of CD8+ T cells. Activated B cells further differentiate into ASCs. The IgG antibodies secreted by ASCs induce ADCC, ADCP, and CDC to promote anti-tumor immunity. Immunosuppressive IgA production in TME supports tumor growth. In addition, CIC accumulation is associated with poor outcomes. IL-10+ IgA-producing B cells could be categorized as a part of Breg cells, which suppress the anti-tumor immunity, other Breg cells such as TGF-β-producing B cells or IL-21-producing B cells also limit anti-tumor immunity. Moreover, B cells-derived lymphotoxin supports lymphoid organ development but promotes tumor growth and relapse by inducing angiogenesis. And GABA produced by B cells in TME impairs tumor growth by supporting IL-10+ macrophages.
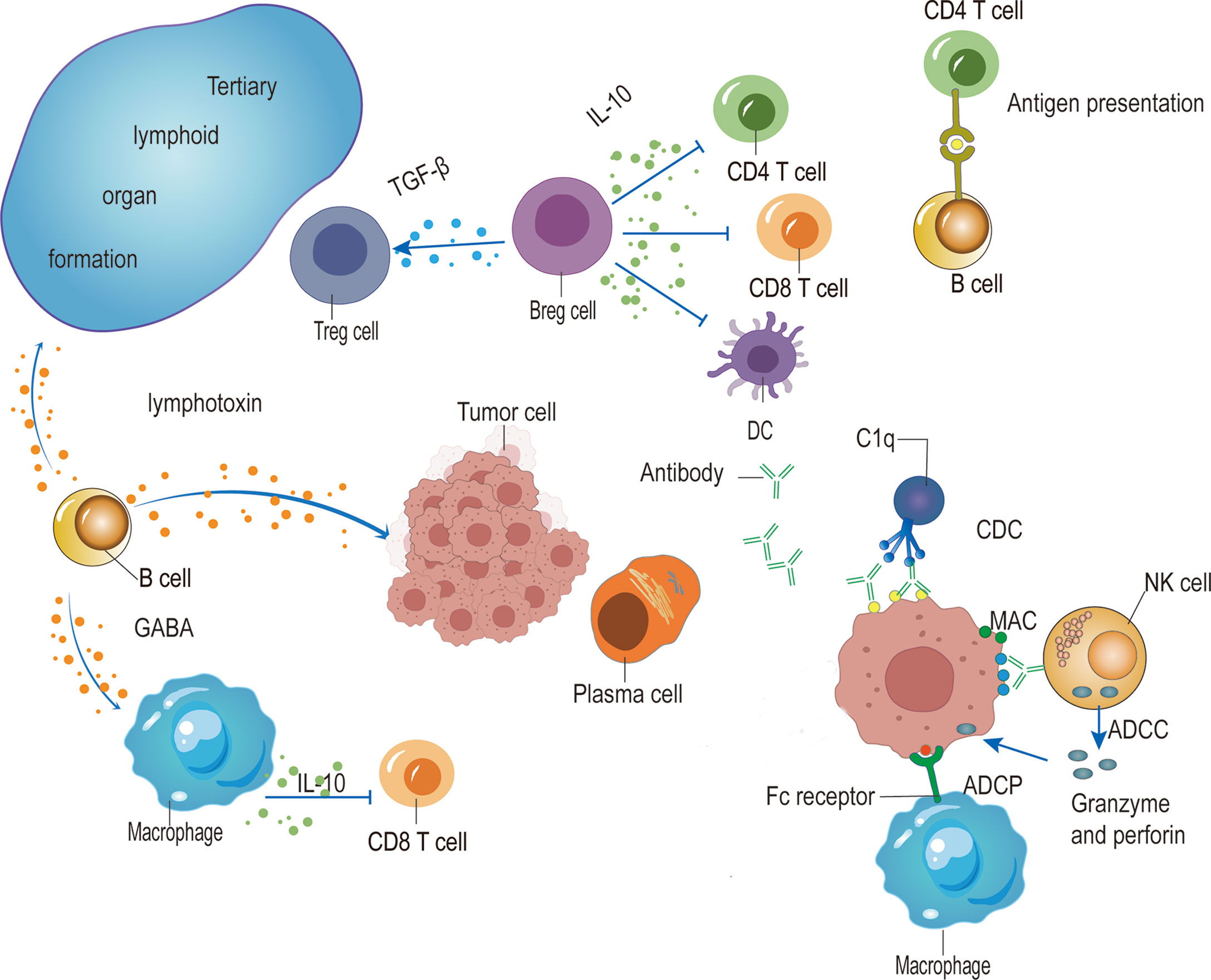
Figure 1 The role of B cells in tumor immunity. The antibodies produced by plasma cells induce ADCC mediated by NK cells, ADCP by macrophages, and CDC mediated by C1q, which target and kill tumor cells. IgA-expressing Breg cells dampen anti-tumor immunity by secreting anti-inflammatory cytokines such as IL-10 and TGF-β to suppress CD4+ T cells, CD8+ T cells and dendritic cells (DCs), and facilitate Treg cells. B cells also promote anti-tumor immunity by presenting antigen to CD4+ T cells and further interacting with activated T cells to induce TFH cells, thus promoting the function of CD8+ T cells. In addition, the production of lymphotoxin from B cells enhances anti-tumor immunity by facilitating tertiary lymphoid organ formation while promoting tumor growth by the induction of angiogenesis. Moreover, B cells produce GABA to impair anti-tumor immunity by facilitating IL-10-producing macrophages.
Though there are many controversial functions of B cells in tumor immunity, the role of B cells in different tumor types is different. Therefore, it is still possible to look for an adequate B cell-based therapy in some specific tumors. For example, IgA+ Breg cells express PDL1, secrete IL-10 in TME and suppress local immune responses in several cancer types, such as human prostate and liver cancer (91, 92). PD-L1/PD-1 blockade can restore the anti-tumor immunity by reactivating CD8+ T cells since Breg cells suppress CD8+ T cells by producing anti-inflammatory cytokine IL-10. Simply depleting B cells couldn’t well demonstrate the function of B cells in a specific tumor cell type, thus, further studies may be needed to elucidate which phenotype of B cells or which mechanism is predominant. Yet, if the depletion of B cells largely impairs tumor growth, it can still be considered a potential treatment. Breg cells play a critical role in suppressing tumor immunity in some cases. Therefore, for these tumor cells, it is valuable to deplete Breg cells. However, since there is no good marker for Breg cells, it is challenging to deplete Breg cells specifically. In the case that B cell deficiency promotes tumor growth, antibody production, and antigen presentation might be essential. Therefore, B cell activation seems feasible in those BCR epitope-containing neoantigen expressing tumor cells. Though STAT3 activation and CD5+ B cell proportion are correlated with poor outcomes in B16 skin tumor cell lines (93, 94), adoptive transfer of activated B cells in tumor cell inoculated mice leads to slower tumor growth (95).
In summary, increasing studies found that B cell-targeted therapy could be a prospective candidate in immunotherapy. However, based on the mouse experiment, B cell-targeted therapy may not be as efficient as T cell-based therapy. Therefore, the combination of B cell and T cell-targeted therapy could be promising in cancer therapy.
Author contributions
RT and WL drafted the manuscript. RT generated the figure. MN and WL revised the manuscript. WL designed the outline of the manuscript and revised the manuscript. All authors contributed to the article and approved the submitted version.
Funding
This work is supported by Education Reform Research of Central South University (2022jy147).
Conflict of interest
The authors declare that the research was conducted in the absence of any commercial or financial relationships that could be construed as a potential conflict of interest.
Publisher’s note
All claims expressed in this article are solely those of the authors and do not necessarily represent those of their affiliated organizations, or those of the publisher, the editors and the reviewers. Any product that may be evaluated in this article, or claim that may be made by its manufacturer, is not guaranteed or endorsed by the publisher.
References
1. Siegel RL, Miller KD, Fuchs HE, Jemal A. Cancer statistics, 2021. CA Cancer J Clin (2021) 71(1):7–33. doi: 10.3322/caac.21654
2. Kottschade LA. The future of immunotherapy in the treatment of cancer. Semin Oncol Nurs (2019) 35(5):150934. doi: 10.1016/j.soncn.2019.08.013
3. Choi Y, Shi Y, Haymaker CL, Naing A, Ciliberto G, Hajjar J. T-Cell agonists in cancer immunotherapy. J Immunother Cancer (2020) 8(2):e000966. doi: 10.1136/jitc-2020-000966
4. Farhood B, Najafi M, Mortezaee K. CD8(+) cytotoxic t lymphocytes in cancer immunotherapy: a review. J Cell Physiol (2019) 234(6):8509–21. doi: 10.1002/jcp.27782
5. Hirsch L, Zitvogel L, Eggermont A, Marabelle A. PD-loma: a cancer entity with a shared sensitivity to the PD-1/PD-L1 pathway blockade. Br J Cancer (2019) 120(1):3–5. doi: 10.1038/s41416-018-0294-4
6. Long W, Chen J, Gao C, Lin Z, Xie X, Dai H. Brief review on the roles of neutrophils in cancer development. J Leukoc Biol (2021) 109(2):407–13. doi: 10.1002/JLB.4MR0820-011R
7. Cendrowicz E, Sas Z, Bremer E, Rygiel TP. The role of macrophages in cancer development and therapy. Cancers (Basel) (2021) 13(8):1946. doi: 10.3390/cancers13081946
8. Brodt P, Gordon J. Anti-tumor immunity in b lymphocyte-deprived mice. i. immunity to a chemically induced tumor. J Immunol (1978) 121(1):359–62.
9. Cui C, Wang J, Fagerberg E, Chen PM, Connolly KA, Damo M, et al. Neoantigen-driven b cell and CD4 T follicular helper cell collaboration promotes anti-tumor CD8 T cell responses. Cell (2021) 184(25):6101–18 e13. doi: 10.1016/j.cell.2021.11.007
10. Wang W, Erbe AK, Hank JA, Morris ZS, Sondel PM. NK cell-mediated antibody-dependent cellular cytotoxicity in cancer immunotherapy. Front Immunol (2015) 6:368. doi: 10.3389/fimmu.2015.00368
11. Gunderson AJ, Coussens LM. B cells and their mediators as targets for therapy in solid tumors. Exp Cell Res (2013) 319(11):1644–9. doi: 10.1016/j.yexcr.2013.03.005
12. Yuen GJ, Demissie E, Pillai S. B lymphocytes and cancer: a love-hate relationship. Trends Cancer (2016) 2(12):747–57. doi: 10.1016/j.trecan.2016.10.010
13. Chen XJ, Jensen PE. The role of b lymphocytes as antigen-presenting cells. Archivum Immunol Et Therapiae Experimentalis (2008) 56(2):77–83. doi: 10.1007/s00005-008-0014-5
14. Schultz KR, Klarnet JP, Gieni RS, HayGlass KT, Greenberg PD. The role of b cells for in vivo T cell responses to a friend virus-induced leukemia. Science (1990) 249(4971):921–3. doi: 10.1126/science.2118273
15. DiLillo DJ, Yanaba K, Tedder TF. B cells are required for optimal CD4(+) and CD8(+) T cell tumor immunity: Therapeutic b cell depletion enhances B16 melanoma growth in mice. J Immunol (2010) 184(7):4006–16. doi: 10.4049/jimmunol.0903009
16. Hu Q, Hong Y, Qi P, Lu G, Mai X, Xu S, et al. Atlas of breast cancer infiltrated b-lymphocytes revealed by paired single-cell RNA-sequencing and antigen receptor profiling. Nat Commun (2021) 12(1):2186. doi: 10.1038/s41467-021-22300-2
17. LeBien TW, Tedder TF. B lymphocytes: how they develop and function. Blood (2008) 112(5):1570–80. doi: 10.1182/blood-2008-02-078071
18. Bruhns P. Properties of mouse and human IgG receptors and their contribution to disease models. Blood (2012) 119(24):5640–9. doi: 10.1182/blood-2012-01-380121
19. Alderson KL, Sondel PM. Clinical cancer therapy by NK cells via antibody-dependent cell-mediated cytotoxicity. J BioMed Biotechnol (2011) 2011:379123. doi: 10.1155/2011/379123
20. Mujoo K, Kipps TJ, Yang HM, Cheresh DA, Wargalla U, Sander DJ, et al. Functional-properties and effect on growth suppression of human neuro-blastoma tumors by isotype switch variants of monoclonal antiganglioside gd2 antibody 14.18. Cancer Res (1989) 49(11):2857–61.
21. Handgretinger R, Baader P, Dopfer R, Klingebiel T, Reuland P, Treuner J, et al. A phase-i study of neuroblastoma with the antiganglioside gd2 antibody 14.g2a. Cancer Immunol Immunother (1992) 35(3):199–204. doi: 10.1007/BF01756188
22. Frost JD, Hank JA, Reaman GH, Frierdich S, Seeger RC, Gan J, et al. A phase I/IB trial of murine monoclonal anti-GD2 antibody 14.G2a plus interleukin-2 in children with refractory neuroblastoma: a report of the children’s cancer group. Cancer (1997) 80(2):317–33. doi: 10.1002/(sici)1097-0142(19970715)80:2<317::aid-cncr21>3.0.co;2-w
23. Handgretinger R, Anderson K, Lang P, Dopfer R, Klingebiel T, Schrappe M, et al. A phase I study of human/mouse chimeric antiganglioside GD2 antibody ch14.18 in patients with neuroblastoma. Eur J Cancer (1995) 31A(2):261–7. doi: 10.1016/0959-8049(94)00413-y
24. Cartron G, Dacheux L, Salles G, Solal-Celigny P, Bardos P, Colombat P, et al. Therapeutic activity of humanized anti-CD20 monoclonal antibody and polymorphism in IgG fc receptor FcgammaRIIIa gene. Blood (2002) 99(3):754–8. doi: 10.1182/blood.V99.3.754
25. Romee R, Foley B, Lenvik T, Wang Y, Zhang B, Ankarlo D, et al. NK cell CD16 surface expression and function is regulated by a disintegrin and metalloprotease-17 (ADAM17). Blood (2013) 121(18):3599–608. doi: 10.1182/blood-2012-04-425397
26. Goede V, Fischer K, Busch R, Engelke A, Eichhorst B, Wendtner CM, et al. Obinutuzumab plus chlorambucil in patients with cll and coexisting conditions. New Engl J Med (2014) 370(12):1101–10. doi: 10.1056/NEJMoa1313984
27. Haso W, Lee DW, Shah NN, Stetler-Stevenson M, Yuan CM, Pastan IH, et al. Anti-CD22-chimeric antigen receptors targeting b-cell precursor acute lymphoblastic leukemia. Blood (2013) 121(7):1165–74. doi: 10.1182/blood-2012-06-438002
28. Andreu P, Johansson M, Affara NI, Pucci F, Tan T, Junankar S, et al. FcRgamma activation regulates inflammation-associated squamous carcinogenesis. Cancer Cell (2010) 17(2):121–34. doi: 10.1016/j.ccr.2009.12.019
29. Biswas S, Mandal G, Payne KK, Anadon CM, Gatenbee CD, Chaurio RA, et al. IgA transcytosis and antigen recognition govern ovarian cancer immunity. Nature (2021) 591(7850):464–70. doi: 10.1038/s41586-020-03144-0
30. Zhong ZW, Nan K, Weng ML, Yue Y, Zhou WC, Wang ZQ, et al. Pro- and anti- effects of immunoglobulin a- producing b cell in tumors and its triggers. Front Immunol (2021) 12. doi: 10.3389/fimmu.2021.765044
31. Jia X, Liu H, Xu C, Han S, Shen Y, Miao X, et al. MiR-15a/16-1 deficiency induces IL-10-producing CD19(+) TIM-1(+) cells in tumor microenvironment. J Cell Mol Med (2019) 23(2):1343–53. doi: 10.1111/jcmm.14037
32. Bosisio FM, Wilmott JS, Volders N, Mercier M, Wouters J, Stas M, et al. Plasma cells in primary melanoma. prognostic significance and possible role of IgA. Modern Pathol (2016) 29(4):347–58. doi: 10.1038/modpathol.2016.28
33. Chiaruttini G, Mele S, Opzoomer J, Crescioli S, Ilieva KM, Lacy KE, et al. B cells and the humoral response in melanoma: The overlooked players of the tumor microenvironment. Oncoimmunology (2017) 6(4):e1294296. doi: 10.1080/2162402X.2017.1294296
34. Pinto D, Montani E, Bolli M, Garavaglia G, Sallusto F, Lanzavecchia A, et al. A functional BCR in human IgA and IgM plasma cells. Blood (2013) 121(20):4110–4. doi: 10.1182/blood-2012-09-459289
35. Sharonov GV, Serebrovskaya EO, Yuzhakova DV, Britanova OV, Chudakov DM. B cells, plasma cells and antibody repertoires in the tumour microenvironment. Nat Rev Immunol (2020) 20(5):294–307. doi: 10.1038/s41577-019-0257-x
36. Xiong E, Min Q, Wang JY. MZB1 promotes the secretion of J chain-containing dimeric IgA and is critical for the suppression of gut inflammation. Eur J Immunol (2019) 49:1365–6. doi: 10.1073/pnas.1904204116
37. Fadlallah J, El Kafsi H, Sterlin D, Juste C, Parizot C, Dorgham K, et al. Microbial ecology perturbation in human IgA deficiency. Sci Transl Med (2018) 10(439):eaan1217. doi: 10.1126/scitranslmed.aan1217
38. Jorgensen GH, Gardulf A, Sigurdsson MI, Sigurdardottir ST, Thorsteinsdottir I, Gudmundsson S, et al. Clinical symptoms in adults with selective iga deficiency: a case-control study. J Clin Immunol (2013) 33(4):742–7. doi: 10.1007/s10875-012-9858-x
39. Koskinen S. Long-term follow-up of health in blood donors with primary selective IgA deficiency. J Clin Immunol (1996) 16(3):165–70. doi: 10.1007/BF01540915
40. Pilette C, Detry B, Guisset A, Gabriels J, Sibille Y. Induction of interleukin-10 expression through fc alpha receptor in human monocytes and monocyte-derived dendritic cells: role of p38 MAPKinase. Immunol Cell Biol (2010) 88(4):486–93. doi: 10.1038/icb.2009.120
41. Katz SI, Parker D, Turk JL. B-cell suppression of delayed-hypersensitivity reactions. Nature (1974) 251(5475):550–1. doi: 10.1038/251550a0
42. Neta R, Salvin SB. Specific suppression of delayed hypersensitivity: the possible presence of a suppressor b cell in the regulation of delayed hypersensitivity. J Immunol (1974) 113(6):1716–25.
43. Wolf SD, Dittel BN, Hardardottir F, Janeway CA Jr. Experimental autoimmune encephalomyelitis induction in genetically b cell-deficient mice. J Exp Med (1996) 184(6):2271–8. doi: 10.1084/jem.184.6.2271
44. Lal G, Nakayama Y, Sethi A, Singh AK, Burrell BE, Kulkarni N, et al. Interleukin-10 from marginal zone precursor b-cell subset is required for costimulatory blockade-induced transplantation tolerance. Transplantation (2015) 99(9):1817–28. doi: 10.1097/TP.0000000000000718
45. Lal G, Kulkarni N, Nakayama Y, Singh AK, Sethi A, Burrell BE, et al. IL-10 from marginal zone precursor b cells controls the differentiation of Th17, tfh and tfr cells in transplantation tolerance. Immunol Lett (2016) 170:52–63. doi: 10.1016/j.imlet.2016.01.002
46. Yap DYH, Chan TM. B cell abnormalities in systemic lupus erythematosus and lupus nephritis-role in pathogenesis and effect of immunosuppressive treatments. Int J Mol Sci (2019) 20(24):6231. doi: 10.3390/ijms20246231
47. Kleffel S, Vergani A, Tezza S, Ben Nasr M, Niewczas MA, Wong S, et al. Interleukin-10+ regulatory b cells arise within antigen-experienced CD40+ b cells to maintain tolerance to islet autoantigens. Diabetes (2015) 64(1):158–71. doi: 10.2337/db13-1639
48. Boldison J, Da Rosa LC, Davies J, Wen L, Wong FS. Dendritic cells license regulatory b cells to produce IL-10 and mediate suppression of antigen-specific CD8 T cells. Cell Mol Immunol (2020) 17(8):843–55. doi: 10.1038/s41423-019-0324-z
49. Todd SK, Pepper RJ, Draibe J, Tanna A, Pusey CD, Mauri C, et al. Regulatory b cells are numerically but not functionally deficient in anti-neutrophil cytoplasm antibody-associated vasculitis. Rheumatol (Oxford) (2014) 53(9):1693–703. doi: 10.1093/rheumatology/keu136
50. Scapini P, Lamagna C, Hu YM, Lee K, Tang QZ, DeFranco AL, et al. B cell-derived IL-10 suppresses inflammatory disease in Lyn-deficient mice. Proc Natl Acad Sci United States America (2011) 108(41):E823–E32. doi: 10.1073/pnas.1107913108
51. Knippenberg S, Peelen E, Smolders J, Thewissen M, Menheere P, Cohen Tervaert JW, et al. Reduction in IL-10 producing b cells (Breg) in multiple sclerosis is accompanied by a reduced naive/memory breg ratio during a relapse but not in remission. J Neuroimmunol (2011) 239(1-2):80–6. doi: 10.1016/j.jneuroim.2011.08.019
52. Saussine A, Tazi A, Feuillet S, Rybojad M, Juillard C, Bergeron A, et al. Active chronic sarcoidosis is characterized by increased transitional blood b cells, increased IL-10-producing regulatory b cells and high BAFF levels. PloS One (2012) 7(8):e43588. doi: 10.1371/journal.pone.0043588
53. Das A, Ellis G, Pallant C, Lopes AR, Khanna P, Peppa D, et al. IL-10-producing regulatory b cells in the pathogenesis of chronic hepatitis b virus infection. J Immunol (2012) 189(8):3925–35. doi: 10.4049/jimmunol.1103139
54. Liu F, Dai W, Li C, Lu X, Chen Y, Weng D, et al. Role of IL-10-producing regulatory b cells in modulating T-helper cell immune responses during silica-induced lung inflammation and fibrosis. Sci Rep (2016) 6:28911. doi: 10.1038/srep28911
55. Wang K, Gong H, Chai R, Yuan H, Chen Y, Liu J. Aberrant frequency of IL-35 producing b cells in colorectal cancer patients. Cytokine (2018) 102:206–10. doi: 10.1016/j.cyto.2017.10.011
56. Yu CR, Choi JK, Uche AN, Egwuagu CE. Production of IL-35 by bregs is mediated through binding of BATF-IRF-4-IRF-8 complex to il12a and ebi3 promoter elements. J Leukoc Biol (2018) 104(6):1147–57. doi: 10.1002/JLB.3A0218-071RRR
57. Dambuza IM, He C, Choi JK, Yu CR, Wang R, Mattapallil MJ, et al. IL-12p35 induces expansion of IL-10 and IL-35-expressing regulatory b cells and ameliorates autoimmune disease. Nat Commun (2017) 8(1):719. doi: 10.1038/s41467-017-00838-4
58. Tian J, Zekzer D, Hanssen L, Lu Y, Olcott A, Kaufman DL. Lipopolysaccharide-activated b cells down-regulate Th1 immunity and prevent autoimmune diabetes in nonobese diabetic mice. J Immunol (2001) 167(2):1081–9. doi: 10.4049/jimmunol.167.2.1081
59. Parekh VV, Prasad DV, Banerjee PP, Joshi BN, Kumar A, Mishra GC. B cells activated by lipopolysaccharide, but not by anti-ig and anti-CD40 antibody, induce anergy in CD8+ T cells: role of TGF-beta 1. J Immunol (2003) 170(12):5897–911. doi: 10.4049/jimmunol.170.12.5897
60. Carter NA, Vasconcellos R, Rosser EC, Tulone C, Muñoz-Suano A, Kamanaka M, et al. Mice lacking endogenous IL-10-producing regulatory b cells develop exacerbated disease and present with an increased frequency of Th1/Th17 but a decrease in regulatory T cells. J Immunol (2011) 186(10):5569–79. doi: 10.4049/jimmunol.1100284
61. van de Veen W, Stanic B, Yaman G, Wawrzyniak M, Sollner S, Akdis DG, et al. IgG4 production is confined to human IL-10-producing regulatory b cells that suppress antigen-specific immune responses. J Allergy Clin Immunol (2013) 131(4):1204–12. doi: 10.1016/j.jaci.2013.01.014
62. Park MJ, Lee SH, Kim EK, Lee EJ, Park SH, Kwok SK, et al. Myeloid-derived suppressor cells induce the expansion of regulatory b cells and ameliorate autoimmunity in the sanroque mouse model of systemic lupus erythematosus. Arthritis Rheumatol (2016) 68(11):2717–27. doi: 10.1002/art.39767
63. Mutnal MB, Hu S, Schachtele SJ, Lokensgard JR. Infiltrating regulatory b cells control neuroinflammation following viral brain infection. J Immunol (2014) 193(12):6070–80. doi: 10.4049/jimmunol.1400654
64. Liu Y, Cheng LS, Wu SD, Wang SQ, Li L, She WM, et al. IL-10-producing regulatory b-cells suppressed effector T-cells but enhanced regulatory T-cells in chronic HBV infection. Clin Sci (Lond) (2016) 130(11):907–19. doi: 10.1042/CS20160069
65. Matsumoto M, Baba A, Yokota T, Nishikawa H, Ohkawa Y, Kayama H, et al. Interleukin-10-producing plasmablasts exert regulatory function in autoimmune inflammation. Immunity (2014) 41(6):1040–51. doi: 10.1016/j.immuni.2014.10.016
66. Wasik M, Nazimek K, Bryniarski K. Regulatory b cell phenotype and mechanism of action: the impact of stimulating conditions. Microbiol Immunol (2018) 62(8):485–96. doi: 10.1111/1348-0421.12636
67. Catalán D, Mansilla MA, Ferrier A, Soto L, Oleinika K, Aguillón JC, et al. Immunosuppressive mechanisms of regulatory b cells. Front Immunol (2021) 12:611795. doi: 10.3389/fimmu.2021.611795
68. Yanaba K, Bouaziz JD, Matsushita T, Tsubata T, Tedder TF. The development and function of regulatory b cells expressing IL-10 (B10 cells) requires antigen receptor diversity and TLR signals. J Immunol (2009) 182(12):7459–72. doi: 10.4049/jimmunol.0900270
69. Lampropoulou V, Hoehlig K, Roch T, Neves P, Calderon Gomez E, Sweenie CH, et al. TLR-activated b cells suppress T cell-mediated autoimmunity. J Immunol (2008) 180(7):4763–73. doi: 10.4049/jimmunol.180.7.4763
70. Barr TA, Brown S, Ryan G, Zhao J, Gray D. TLR-mediated stimulation of APC: Distinct cytokine responses of b cells and dendritic cells. Eur J Immunol (2007) 37(11):3040–53. doi: 10.1002/eji.200636483
71. Neves P, Lampropoulou V, Calderon-Gomez E, Roch T, Stervbo U, Shen P, et al. Signaling via the MyD88 adaptor protein in b cells suppresses protective immunity during salmonella typhimurium infection. Immunity (2010) 33(5):777–90. doi: 10.1016/j.immuni.2010.10.016
72. Fillatreau S, Sweenie CH, McGeachy MJ, Gray D, Anderton SM. B cells regulate autoimmunity by provision of IL-10. Nat Immunol (2002) 3(10):944–50. doi: 10.1038/ni833
73. Blair PA, Chavez-Rueda KA, Evans JG, Shlomchik MJ, Eddaoudi A, Isenberg DA, et al. Selective targeting of b cells with agonistic anti-CD40 is an efficacious strategy for the generation of induced regulatory T2-like b cells and for the suppression of lupus in MRL/lpr mice. J Immunol (2009) 182(6):3492–502. doi: 10.4049/jimmunol.0803052
74. Shah S, Divekar AA, Hilchey SP, Cho HM, Newman CL, Shin SU, et al. Increased rejection of primary tumors in mice lacking b cells: inhibition of anti-tumor CTL and TH1 cytokine responses by b cells. Int J Cancer (2005) 117(4):574–86. doi: 10.1002/ijc.21177
75. Inoue S, Leitner WW, Golding B, Scott D. Inhibitory effects of b cells on antitumor immunity. Cancer Res (2006) 66(15):7741–7. doi: 10.1158/0008-5472.CAN-05-3766
76. Schioppa T, Moore R, Thompson RG, Rosser EC, Kulbe H, Nedospasov S, et al. B regulatory cells and the tumor-promoting actions of TNF-alpha during squamous carcinogenesis. Proc Natl Acad Sci U S A (2011) 108(26):10662–7. doi: 10.1073/pnas.1100994108
77. Horikawa M, Minard-Colin V, Matsushita T, Tedder TF. Regulatory b cell production of IL-10 inhibits lymphoma depletion during CD20 immunotherapy in mice. J Clin Invest (2011) 121(11):4268–80. doi: 10.1172/JCI59266
78. Olkhanud PB, Damdinsuren B, Bodogai M, Gress RE, Sen R, Wejksza K, et al. Tumor-evoked regulatory b cells promote breast cancer metastasis by converting resting CD4(+) T cells to T-regulatory cells. Cancer Res (2011) 71(10):3505–15. doi: 10.1158/0008-5472.CAN-10-4316
79. Zhang Y, Morgan R, Chen C, Cai Y, Clark E, Khan WN, et al. Mammary-tumor-educated b cells acquire LAP/TGF-beta and PD-L1 expression and suppress anti-tumor immune responses. Int Immunol (2016) 28(9):423–33. doi: 10.1093/intimm/dxw007
80. Pylayeva-Gupta Y, Das S, Handler JS, Hajdu CH, Coffre M, Koralov SB, et al. IL35-producing b cells promote the development of pancreatic neoplasia. Cancer Discovery (2016) 6(3):247–55. doi: 10.1158/2159-8290.CD-15-0843
81. Mirlekar B, Michaud D, Searcy R, Greene K, Pylayeva-Gupta Y. IL35 hinders endogenous antitumor t-cell immunity and responsiveness to immunotherapy in pancreatic cancer. Cancer Immunol Res (2018) 6(9):1014–24. doi: 10.1158/2326-6066.CIR-17-0710
82. Shen P, Fillatreau S. Antibody-independent functions of b cells: a focus on cytokines. Nat Rev Immunol (2015) 15(7):441–51. doi: 10.1038/nri3857
83. Luther SA, Lopez T, Bai W, Hanahan D, Cyster JG. BLC expression in pancreatic islets causes b cell recruitment and lymphotoxin-dependent lymphoid neogenesis. Immunity (2000) 12(5):471–81. doi: 10.1016/S1074-7613(00)80199-5
84. Schrama D, thor Straten P, Fischer WH, McLellan AD, Brocker EB, Reisfeld RA, et al. Targeting of lymphotoxin-alpha to the tumor elicits an efficient immune response associated with induction of peripheral lymphoid-like tissue. Immunity (2001) 14(2):111–21. doi: 10.1016/S1074-7613(01)00094-2
85. Germain C, Gnjatic S, Tamzalit F, Knockaert S, Remark R, Goc J, et al. Presence of b cells in tertiary lymphoid structures is associated with a protective immunity in patients with lung cancer. Am J Respir Crit Care Med (2014) 189(7):832–44. doi: 10.1164/rccm.201309-1611OC
86. Ammirante M, Luo JL, Grivennikov S, Nedospasov S, Karin M. B-cell-derived lymphotoxin promotes castration-resistant prostate cancer. Nature (2010) 464(7286):302–5. doi: 10.1038/nature08782
87. Luo JL, Tan W, Ricono JM, Korchynskyi O, Zhang M, Gonias SL, et al. Nuclear cytokine-activated IKKalpha controls prostate cancer metastasis by repressing maspin. Nature (2007) 446(7136):690–4. doi: 10.1038/nature05656
88. Zhang B, Vogelzang A, Miyajima M, Sugiura Y, Wu Y, Chamoto K, et al. B cell-derived GABA elicits IL-10(+) macrophages to limit anti-tumour immunity. Nature (2021) 599(7885):471–6. doi: 10.1038/s41586-021-04082-1
89. Zhang Y, Morgan R, Podack ER, Rosenblatt J. B cell regulation of anti-tumor immune response. Immunol Res (2013) 57(1-3):115–24. doi: 10.1007/s12026-013-8472-1
90. Akrami M, Menzies R, Chamoto K, Miyajima M, Suzuki R, Sato H, et al. Circulation of gut-preactivated naive CD8(+) T cells enhances antitumor immunity in b cell-defective mice. Proc Natl Acad Sci U S A (2020) 117(38):23674–83. doi: 10.1073/pnas.2010981117
91. Shalapour S, Font-Burgada J, Di Caro G, Zhong Z, Sanchez-Lopez E, Dhar D, et al. Immunosuppressive plasma cells impede T-cell-dependent immunogenic chemotherapy. Nature (2015) 521(7550):94–8. doi: 10.1038/nature14395
92. Shalapour S, Lin XJ, Bastian IN, Brain J, Burt AD, Aksenov AA, et al. Inflammation-induced IgA+ cells dismantle anti-liver cancer immunity. Nature (2017) 551(7680):340–5. doi: 10.1038/nature24302
93. Zhang C, Xin H, Zhang W, Yazaki PJ, Zhang Z, Le K, et al. CD5 binds to interleukin-6 and induces a feed-forward loop with the transcription factor STAT3 in b cells to promote cancer. Immunity (2016) 44(4):913–23. doi: 10.1016/j.immuni.2016.04.003
94. Yang C, Lee H, Pal S, Jove V, Deng J, Zhang W, et al. B cells promote tumor progression via STAT3 regulated-angiogenesis. PloS One (2013) 8(5):e64159. doi: 10.1371/journal.pone.0064159
Keywords: B cell, tumor, tumor immunity, cancer, immunotherapy, tumor microenvironment
Citation: Tan R, Nie M and Long W (2022) The role of B cells in cancer development. Front. Oncol. 12:958756. doi: 10.3389/fonc.2022.958756
Received: 01 June 2022; Accepted: 18 July 2022;
Published: 11 August 2022.
Edited by:
Catherine Sautes-Fridman, INSERM U1138 Centre de Recherche des Cordeliers (CRC), FranceReviewed by:
Dharmendra Kumar Yadav, Gachon University, KoreaSotirios Sotiriou, University of Thessaly, Greece
Copyright © 2022 Tan, Nie and Long. This is an open-access article distributed under the terms of the Creative Commons Attribution License (CC BY). The use, distribution or reproduction in other forums is permitted, provided the original author(s) and the copyright owner(s) are credited and that the original publication in this journal is cited, in accordance with accepted academic practice. No use, distribution or reproduction is permitted which does not comply with these terms.
*Correspondence: Wang Long, bG9uZ2ltbTE5OTFAb3V0bG9vay5jb20=; Manhua Nie, bmllbWFuaHVhNzdAY3N1LmVkdS5jbg==
†These authors share last authorship
‡Lead author