- 1Department of Gastrointestinal Colorectal and Anal Surgery, The China-Japan Union Hospital of Jilin University, Changchun, China
- 2College of Food Engineering, Jilin Engineering Normal University, Changchun, China
- 3Department of Thyroid Surgery, The Second Hospital of Jilin University, Changchun, China
- 4Department of Burns Surgery, The First Hospital of Jilin University, Changchun, China
Colorectal cancer (CRC) is a common type of malignant digestive tract tumor with a high incidence rate worldwide. Currently, the clinical treatment of CRC predominantly include surgical resection, postoperative chemotherapy, and radiotherapy. However, these treatments contain severe limitations such as drug side effects, the risk of recurrence and drug resistance. Some natural compounds found in plants, fungi, marine animals, and bacteria have been shown to inhibit the occurrence and development of CRC. Although the explicit molecular mechanisms underlying the therapeutic effects of these compounds on CRC are not clear, classical signaling transduction pathways such as NF-kB and Wnt/β-catenin are extensively regulated. In this review, we have summarized the specific mechanisms regulating the inhibition and development of CRC by various types of natural compounds through nine signaling pathways, and explored the potential therapeutic values of these natural compounds in the clinical treatment of CRC.
Introduction
Natural compounds are chemical substances found in plants, fungi, marine animals, and bacteria that exhibit significant pharmacological effects. Natural compounds can be classified according to their chemical structure as follows: proteins, polypeptides, amino acids, nucleic acids, various enzymes, saccharides, resins, colloids, lignin, vitamins, fats, oils, waxes, alkaloids, volatile oils, flavonoids, glycosides, terpenoids, organic acids, phenols, quinones, lactones, steroids, tannins, antibiotics, and other naturally occurring chemical components. Some natural compounds have the ability to modulate signaling pathways and regulate the expression of genes involved in cell cycle regulation, cell differentiation, and apoptosis (1). One of the most widely researched of these functions is the anti-tumor effect of natural compounds (1).
Colorectal cancer (CRC) is a malignant tumor originating from the colorectal mucosa. It is one of the most common clinical malignant tumors worldwide, with more than 387,600 new cases and 187,100 CRC-related deaths in China, representing the fifth (8.01%) and fourth (9.87%) highest mortality and incidence rates among all cancers in 2015 (2). Thus, reducing the incidence and mortality of CRC is an urgent and important clinical issue. Current CRC treatment methods are based on surgery and chemotherapy, with the most common chemotherapeutic agents being platinum derivatives (oxaliplatin), antimetabolites (capecitabine, 5-fluorouracil (5-FU)), topoisomerase inhibitors (irinotecan), and Tegafur/uracil (UFT) (3). Among these, the first-line chemotherapy drug for CRC treatment is 5-FU (4); however, the overall response rate to 5-FU monotherapy in advanced CRC is limited to 10–15% (5). Moreover, chemotherapy involves different degrees of side effects. The most common adverse effects of 5-FU include vomiting, diarrhea, mucositis of the oral cavity, headaches, skin pruritus, anemia, cardiotoxicity, agranulocytosis, alopecia (hair loss), photosensitivity, hand-foot syndrome, depression, and anxiety (6).
Therefore, the development of anti-tumor drugs that exhibit low toxicity and less potential to develop drug resistance has become the focus of current CRC research, with many researchers turning their attention to natural compounds. Abundant previous research has shown that various types of natural compounds can inhibit CRC. In this review, we discuss the specific mechanisms by which natural compounds inhibit CRC by describing the overall role of several classical signaling pathways in the application of natural compounds to CRC. The anti-cancer activity and clinical potential of natural compounds are reviewed at cellular, animal, and clinical levels.
Nuclear factor kappa B signaling pathway
Polyphenolic compounds
Epigallocatechin gallate (EGCG) is a polyhydroxy phenolic compound extracted from tea, which mainly exists in green tea and raw pu-erh tea. Modern medicine has confirmed that EGCG can prevent the occurrence of cardiovascular disease and inhibit the growth and metabolism of tumors (7). EGCG induces p21 expression by enhancing p21 promoter activity (8), releases adenosine by disrupting the folic acid cycle (9), and inhibits CRC cell proliferation (10) and migration (11) by the NF-κB signaling pathway. miRNAs have the ability to regulate biological processes and play a crucial role in the occurrence and development of cancer (12). EGCG can enhance the sensitivity of CRC cells to 5-FU chemotherapy through the NF-κB/miR-155-5p/MDR1 axis (13). According to previous animal models, EGCG inhibits N,N’-dimethylhydrazine-induced colon tumor development through its antioxidant and anti-inflammatory potential (10, 14). In addition, EGCG can be used in combination with sodium butyrate to induce CRC cell cycle arrest and DNA damage (15). Notably, a clinical study involving 32 volunteers reported that daily oral administration of EGCG (800 mg) regulates targeted biomarkers associated with CRC, including NF-κB. (16). These studies reveal the need for further investigation into EGCG as a potential chemopreventive agent for CRC.
As another polyphenol, the main sources of natural resveratrol (RES) are Polygonum cuspidatum and Vitis; however, RES is also a bioactive ingredient in wine and grape juice, and easily absorbed orally. RES exhibits various pharmacological effects such as anti-tumor, anti-cardiovascular disease, anti-inflammatory, antioxidant, and nervous system protective effects, and has great medicinal value and market prospects (17). According to bioinformatic analysis based on molecular docking and molecular dynamics simulations, the molecular interaction between NF-κB and RES can be applied to develop cancer therapies (18). RES also has inhibitory effects on CRC cell lines by regulating NF-κB, which includes increasing sensitivity to 5-FU chemotherapy (19, 20), inhibiting focal adhesion kinase (FAK) activity and enhancing anti-invasive activity (21), or inducing apoptosis through PD-L1 (22). In addition, there is evidence that RES combined with piceatannol (PIC), a polyphenolic compound also found in grapes, can inhibit iNOS expression, inhibit NF-κB activation (23), and further upregulate PD-L1 (22). Recently, with the popularization of nanotechnology in the pharmaceutical field, silica nanoparticles have been used to encapsulate RES, forming MCM-48-Resveratrol. Compared with pure RES, MCM-48-Resveratrol can more effectively induce CRC apoptosis (24).
Curcumin (CCM) is a diketone hydrophobic polyphenol derived from the dried rhizome of the ginger plant, Curcuma longa L. CCM has long played an important role in the treatment of inflammatory-mediated diseases and begun to attract additional attention because of its low toxicity effects in multiple animal and clinical experiments (25, 26). A number of studies have shown that CCM can significantly inhibit CRC through NF-κB during in vivo and in vitro, for example, by inducing apoptosis and inhibiting metastasis (27). Its therapeutic effect is comparable to that of 5-FU (28), and its combined use with conventional chemotherapeutic drugs such as 5-FU can enhance the effect of chemotherapy (29); thus, it has substantial potential as an anti-chemotherapy-resistant drug for colon cancer treatment. However, high hydrophobicity, poor oral absorption, and fast metabolism limit the clinical use of CCM. To solve these problems, researchers have used nanotechnology to treat CCM, generating a variety of derivatives that retain or even enhance its anti-cancer activity, such as Theracurmin, which boasts improved water solubility (30). These derivatives have greatly expanded the clinical application prospects of CCM (31). Notably, regulation of the NF-κB pathway by CMM was confirmed in a clinical trial in which the combined use of anthocyanins and CCM significantly decreased the expression of NF-κB (32).
Flavonoids
Kaempferol, extracted from Prunus mume, is a flavonoid compound that has attracted widespread attention because of its anti-cancer, anti-inflammatory, antioxidant, anti-bacterial, anti-viral, and other effects. According to both animal and cellular experiments, kaempferol significantly inhibits the growth, migration, and invasion of CRC cells through the RelA/NF-κB signaling pathway, as determined by network pharmacology and molecular docking (33). Moreover, kaempferol can regenerate chemosensitivity in 5-FU-resistant LS174-R cells (34), making it a potentially effective therapy for CRC. Moreover, delphinidin, which is a type of flavonoid abundant in blueberries, inhibits the proliferation of human colon cancer cells (HCT-116) through the NF-κB pathway (35). Furthermore, silibinin can reduce the protein expression levels of various NF-κB regulatory molecules such as Bcl-2, COX-2, iNOS, VEGF, and matrix metalloproteinases (MMPs) (36). Baicalin, a flavonoid compound extracted from the traditional Chinese herbal medicine Scutellaria baicalensis Georgi, can exert therapeutic effects by mediating oxidative stress, inflammation-induced downstream apoptosis, and immune response pathways (37).
Other chemicals
As a quinonemethide triterpenoid extracted from Celastraceae and Hippocrateaceae species, pristimerin has potent anti-cancer effects (38). Specifically, pristimerin has significant cytotoxic and proliferation inhibitory effects on CRC cell lines. Cell cycle analysis revealed that pristimerin can induce G1 phase arrest, which is closely associated with the reduced expression of cyclin D1 and cyclin-dependent kinases (CDK4 and CDK6) and the induction of p21 (39, 40). Furthermore, the results of Azoxymethane (AOM)/Dextran sulfate sodium (DSS)-induced animal models showed that pristimerin inhibits tumor growth mainly by inhibiting NF-κB activity in tumor tissues (41). Among the triterpenoids, Raddeanin A from the sea anemone Raddeana Regel also shows the potential to inhibit the invasion and metastasis of CRC through the NF-κB pathway (42). Wang (43) reported that Raddeanin A inhibits the NF-κB pathway by reducing the phosphorylation of IKBα, thereby inducing the subsequent mitochondrial apoptosis pathway.
Organosulfur compounds in garlic also induce apoptosis in colon cancer cells by modulating NF-κB (44). In SW480 cells and an AOM/DSS-induced mouse CRC model, diallyl disulfide inhibits Glycogen synthase kinase-3β (GSK-3β), a positive regulator of NF-κB, thereby suppressing inflammation and tumors (45). Diallyl sulfide, diallyl disulfide, and diallyl trisulfide also inhibit the migration and invasion of COLO205 cells, with diallyl sulfide exhibiting the greatest effect (46). There have also been reports that allicin increases CRC cell sensitivity to X-ray radiotherapy (47).
Summary
The relationship between the transcription factor NF-κB pathway, inflammation, and cancer has been extensively evaluated (48–50). In colon cancer, chronic inflammation is an important predisposing factor. Therefore, the NFκB signaling pathway plays an important role in tumor biology, regulating key processes in the occurrence and development of various cancers. These processes include promoting cell proliferation (by regulating cyclin D, c-Myc, and IL-6, which regulate growth-promoting signals), inhibiting apoptosis (by inhibiting apoptotic genes including Bcl-2 and BclxL transcription), promoting angiogenesis (inducing VEGF expression), promoting tumor invasion (through E-selectin and MMPs), promoting generation of the epithelial mesenchymal transition (EMT) and colon cancer stem cells (CSCs), and mediating tumor drug resistance.
Some studies have explored the upstream or downstream target of the NFκB pathway and produced more in-depth reports on the anti-cancer mechanism of some natural products (Figure 1, created with BioRender.com). For example, RES inhibits the NF-κB pathway and promotes the expression of Sirt1 by inhibiting the activity of FAK (21); EGCG can downregulate glucose-regulated protein 78 (GRP78) expression and activate the NF-κB/mir-155-5p pathway, therefore inhibiting the resistance of colon cancer cells to chemotherapeutic drugs (13); and pristimerin can induce apoptosis and inhibit cell proliferation by inhibiting the Akt/forkhead box protein O (FOXO3a) pathway (41).
However, as these natural products move from the laboratory to the clinic, we also need to consider whether their effective concentrations are acceptable to humans. For example, PIC, although it exhibits G1/S cell cycle arrest and apoptosis-inducing activity in ex vivo cultured CRC cell lines at an effective concentration of 50 μM, this concentration is unattainable under physiological conditions (22).
Wnt/β-catenin signaling pathway
Flavonoids
Luteolin, a flavonoid component detected in several Chinese herbal medicines, can promote the cycle arrest of HCT-15 cells in the G2/M phase and induce apoptosis (51). Moreover, luteolin was shown to inhibit β-catenin, GSK-3β, and cyclin D1 in a mouse model (52). Apigenin is a flavonoid that is widely distributed in vegetables and fruits grown in the warm tropics, especially celery. Apigenin significantly inhibits the entry of β-catenin into the nucleus, as well as the proliferation, migration, invasion, and organoid growth of CRC cells (53). Apigenin also regulates Akt/mTOR signaling-induced β-catenin degradation during Wnt signaling via the autophagy-lysosomal system (54). Genistein shows anti-cancer activity and mainly exists in species of the legume family, such as Sophora japonica and Shandou root. However, it has exhibited contradictory performance in previous studies on colon cancer and the Wnt/β-catenin signaling pathway. Cellular-level experiments using RKO and DLD1 cell lines revealed that genistein alone cannot inhibit Wnt signaling (55), whereas another report demonstrated that genistein, as an inhibitor of the Wnt/β-catenin signaling pathway, can prevent the development of early colon cancer in animals (56).
Two flavonoid compounds, derricin and derricidin, both extracted from Lonchocarpus sericeus, also exhibit anti-colon cancer activity. These compounds can affect the β-catenin distribution and strongly inhibit activity of the canonical Wnt pathway in the CRC cell line (57). Isobavachalcone, a flavonoid compound discovered in Psoralea corylifolia, inhibits cell proliferation and induces apoptosis by inhibiting the Akt/GSK-3/β-catenin pathway in CRC (58). Furthermore, taxifolin, a flavonol nonanol widely present in olive oil, grapes, citrus fruits, and onions, can reduce the expression of β-catenin, Akt, and Survivin (59), thereby showing potential as a novel anti-Wnt/β-catenin drug.
Alkaloids
Piperine, which can be extracted from the dried near-ripe or ripe fruit of Piper nigrum L., has been clinically used as a broad-spectrum anti-convulsant and shows low toxicity and safety in clinical applications (60). Piperine can also inhibit β-catenin nuclear translocation and cell proliferation in the HCT116 CRC cell line (61). Meanwhile, piperine combined with celecoxib combination therapy can synergistically modulate the Wnt/β-catenin pathway, downregulate stemness markers, and enhance the treatment of CT26 homologous Balb/c mice (62). Jatrorrhizine, an alkaloid found in the traditional Chinese herbal medicine Coptis chinensis, can inhibit the Wnt signaling pathway by decreasing β-catenin and increasing GSK-3β expression. Wang verified this effect of jatrorrhizine in an HCT-116 nude mouse xenograft model (63). Sanguinarine, an alkaloid of papaveraceae, exhibits negative effects on expression of the Wnt/β-catenin pathway and EMT markers in LoVo cells. The anti-metastatic potential exhibited by sanguinarine may also be attributed to inhibition of the Wnt/β-catenin signaling pathway, suggesting that sanguinarine may be a potential treatment for metastatic CRC (64).
Terpenoids
Several terpenoids with anti-CRC activity have been discovered in animal models and at the cellular level. RNA-Seq data showed that primimerin can inhibit the Wnt/β-catenin signaling pathway by activating GSK3β (65). Cucurbitacin E, which is widely distributed in dietary plants, can reduce the expression of β-catenin when combined with 5-FU; moreover, transcription factor activating enhancer-binding protein 4 (TFAP4) is the intracellular target of cucurbitacin E (66). Diterpenoid 11α, 12α-epoxyleukamenin E (EPLE), extracted from Salvia miltiorrhiza, is a novel Wnt signaling inhibitor and a potential candidate for further preclinical evaluation as a CRC treatment option (67). EPLE mediates the downregulation of Wnt by inhibiting the target genes c-Myc, Axin2, and Survivin, with Wnt signaling identified as the main and specific target of EPLE. Furthermore, the triterpenoid Raddeanin A, may downregulate Wnt/β-catenin signaling via inhibition of p-lipoprotein receptor-related protein 6 (LRP6), activation of Akt inactivation, removal of GSK-3β inhibition, and suppression of β-catenin (43).
Other chemicals
In addition to the aforementioned major classes of compounds, other natural active products show considerable inhibition of Wnt/β-catenin signaling, such as sulforaphane (SFN) and phenethyl isothiocyanate, which are rich in cruciferous vegetables. SFN can inhibit β-catenin degradation and induce to form closed chromatin associated nuclear β-catenin (68). Moreover, phenethyl isothiocyanate can become a potent inhibitor of colorectal cancer stem cells (CSCs) by targeting the Wnt/β-catenin pathway (69). Parthenolide, found in feverfew, inhibits the activity of Wnt signaling by directly acting on ubiquitin-specific peptidase 7 and destabilizing β-catenin, which indicates that parthenolide is a target anti-cancer agent for aberrant ubiquitin-specific peptidase 7/Wnt signaling (70). Physalin F, a steroid in Physalis, can inhibit Wnt/β-catenin signaling by modulating the ubiquitination and degradation of β-catenin in a Yes-associated protein (YAP)-dependent manner (71). Another compound in Physalis, 4β-Hydroxywithanolide E, can significantly inhibit tumor growth in HCT116 xenografts by suppressing the Wnt/β-catenin signaling; this compound also has potential as a novel Wnt signaling inhibitor (72).
Summary
The Wnt/β-catenin signaling pathway transmits growth-stimulating signals. Approximately 90% of CRC cases are associated with genetic mutations in the adenomatous polyposis coli (APC) gene or other key components of the Wnt signaling pathway (73), and β-catenin accumulation in the nucleus is associated with poor prognosis in patients with CRC (74).
The most common targets of the natural compounds reviewed in this section involve the regulation of cytoplasmic proteins, including β-catenin, GSK-3β, Axin, and APC (Figure 2, created with BioRender.com). Almost all the natural compounds mentioned above exhibit inhibitory activity against β-catenin, with some compounds able to regulate the expression of GSK-3β including flavonoids (luteolin, apigenin) and alkaloids (jatrorrhizine, sanguinarine). Compounds that inhibit the expression of Axin include the diterpenoid EPLE and the alkaloid sanguinarine.
In addition to the targets present in this pathway, previous studies have also reported the anti-cancer effects of some natural compounds through upstream or downstream targets. For example, parthenolide can directly interact with ubiquitin-specific peptidase 7 to inhibit the Wnt pathway (70). Moreover, Physalin F is involved in the degradation of β-catenin according to YAP, a transcriptional co-activator in the Hippo pathway (71). Furthermore, cucurbitacin E is a potential inhibitor of the transcription factor TFAP4 that can block the overexpression of β-catenin (66), and Raddeanin A can inhibit β-catenin transcriptional activation through inactivation of the Wnt coreceptor LRP6 (43).
In the process of developing natural products as drugs, the effective concentration is an important consulting index. Among the literature reviewed here, the natural products capable of inhibiting CRC cell proliferation at low effective concentrations include EPLE (2 μM), which dose-dependently inhibits endogenous Wnt signaling and induces apoptosis (67), cucurbitacin E (1 μM), which can increase the apoptosis rate of cancer cells combined with oxaliplatin chemotherapy from 44.36% to 85.3% (66), and Raddeanin A (1.2 μM), which significantly reduces the nuclear level of β-catenin after 6 h of treatment (43). However, these concentrations are at the cellular level. In the future, more specific pharmacological experiments are required to determine the effective concentration of these compounds for CRC inhibition at the animal level.
Phosphatidylinositol-3 kinase/Akt/mTOR signaling pathway
Polyphenolic compounds
RES exhibits anti-proliferative and apoptosis-inducing activities on CRC cells by upregulating bone morphogenetic proteins (BMP7) and tensin homolog (PTEN) (75, 76), as well as enhanced sensitivity of CRC cells to cetuximab chemotherapy by upregulating connexin-43 (77). In addition, RES exerts anti-cancer activity through the miR-34a/E2F3/Sirt1 axis (78). A bioinformatics analysis study also found that RES induces the expression of Raf-1 kinase inhibitory protein at the protein level (79).
CCM can regulate the activity of the PI3K/Akt signaling pathway by reducing the phosphorylation of Akt, thereby inhibiting the proliferation of CRC cells and promoting apoptosis (80). The targets and pathways of CCM in the treatment of CRC have been predicted through bioinformatics analysis and molecular docking technology (81). The core targets are AKT1, the epidermal growth factor receptor (EGFR), and STAT3, with AKT1 exhibiting the strongest binding ability to CCM. According to experiments in A/J mice, the combined use of CCM and salsalate more effectively inhibits activation of the PI3K/Akt/mTOR pathway, attenuates the abnormal proliferation of colonic mucosa, and reduces tumor proliferation, whereas CCM or salsalate alone do not inhibit abnormal crypt cell proliferation or tumor multiplicity (82). According to our current knowledge of colonic CSCs, CCM can act as a chemosensitizing drug targeting CSCs, and the combination therapy of chemotherapeutic drugs and CCM may be more effective than single chemotherapeutic drugs. This type of treatment can be achieved by developing an efficient delivery system that can treat CRC via nanotechnology-based approaches (83, 84).
EGCG inhibits the activation of the PI3K/Akt signaling pathway in Caco-2 cells by disrupting lipid raft integrity and promoting EGFR degradation (85). Cyclin D1 and p21 have been identified as molecular targets of EGCG in human CRC cells in a dose-dependent manner (8). Moreover, researchers have confirmed the effect of EGCG on PI3K/Akt signaling pathway regulation in dimethylhydrazine-induced rat CRC models, xenografts of nude mice, and mice transplanted with HCT116 cells (10, 86, 87). Compared with EGCG, epicatechin gallate, which is also present in green tea has received relatively little research attention, with only a few studies reporting its ability to induce mitochondrial dysfunction and PI3K/Akt pathway inactivation in CRC cells (88). Both procyanidin B2, a procyanidin in grape seeds (89), and anthocyanins (90) can downregulate the PI3K/Akt pathway and suppress cell proliferation in CRC cells.
Flavonoids
Several studies have reported various herb-based traditional Chinese medicine formulations (including the JianPi Fu Recipe (91), Fuzheng-Jiedu Decoction (92), Huangqin-Baishao herb pair (93), and Fufang Yiliu Yin (94)), which exhibit the ability to resist colon cancer. Network pharmacology, which predicts active ingredients and potential targets, revealed that the predicted effective ingredients contain a variety of flavonoids, such as kaempferol, isorhamnetin, calycosin, quercetin, wogonin, and nobiletin. Kaempferol can inhibit cell proliferation and induce apoptosis in CRC cells by inhibiting thymidylate synthase or attenuating p-Akt activation (95). Moreover, the combined treatment of Kaempferol and 5-FU can greatly inhibit activation of the PI3K/Akt pathway (34).
Wogonin is the main component of Scutellaria baicalensis, and is isolated from the roots and leaves of this plant. It has recently been reported to exhibit a growth inhibitory effect by inhibiting PI3K/Akt in SW48 cells (96). Moreover, wogonin may induce apoptosis in HT-29 colon cancer cells in a p53-dependent manner via Akt activation (97). Wogonin also downregulates the expression and glycolysis of HIF-1α by inhibiting the PI3K/Akt signaling pathway, thereby reversing hypoxia-induced drug resistance (98). In a clinical trial, researchers (99) noted that Silybin and regorafenib combination therapy exerts synergistic anti-proliferative and apoptotic effects by blocking the PI3K/Akt/mTOR intracellular pathway, suggesting that such combination therapy may increase the clinical efficacy of regorafenib.
Other chemicals
Soybean B-group triterpenoid saponins reportedly inhibit Akt activity and induce macroautophagy in human colon cancer cells at physiological concentrations (100, 101). Pristimerin, which is also a triterpenoid, reportedly induces apoptosis and regulates the PI3K/Akt/FOXO3a signaling pathway (39, 41).
According to a previous study, combining salinomycin and SFN in CRC cell lines and xenograft models effectively enhances apoptosis and inhibits the proliferation, migration, and invasion of CRC by inhibiting the PI3K/Akt pathway (102). Therefore, this new combination of salsalate and SFN may also provide a potential strategy for the treatment of CRC. Diallyl sulfide, diallyl disulfide, and diallyl trisulfide in garlic can inhibit COLO205 cell proliferation through multiple pathways, including the PI3K/Akt pathway (46). FraC (RGD-peptide fragacea toxin C) contained in strawberry anemone is significantly toxic to HCT-116 cells harboring the Phosphatidylinositol-4,5-bisphosphate 3-kinase, catalytic subunit alpha (PIK3CA)/Kirsten rat sarcoma viral oncogene homolog (KRAS) mutation, but less toxic to normal cells, which may suggest a new CRC treatment option based on FraC-peptide (103). Recent research on the anti-cancer effects of traditional Chinese herbal medicines has determined that Huangqin-Baishao Herb Pair and Zuojinwan have the potential to inhibit the development of CRC through the PI3K/Akt/mTOR pathway; however, the effective components that may inhibit the development of CRC have not yet been determined (93, 104).
Summary
Different upstream and downstream genes of the signaling pathway are associated with the development of CRC. In colon cancer, an abnormally activated PI3K/Akt pathway can inhibit p53-independent apoptosis by phosphorylating mTOR and its downstream regulators p70S6K, 4EBP1, etc. (105); this pathway can also involve in regulating VEGR expression in tumor angiogenesis (106) and the expression of cyclinD1 and p21 (107).
Multiple natural active products can inhibit Akt phosphorylation, including polyphenols (RES, EGCG, CCM), flavonoids (Kaempferol, wogonin, etc.), and triterpenoid (Figure 3, created with BioRender.com).
Mitogen-activated protein kinase signaling pathway
Flavonoids
Chemopreventive activity of apigenin in CRC cell lines may be mediated by its ability to modulate the MAPK pathway (108). For example, Zhang observed synergistic inhibition of the proliferation and metastasis of CRC cells by apigenin in combination with chrysin, which is abundant in poplar plants, and inhibited activity of the P38-MAPK/Akt pathway by downregulating p-P38 and p-Akt (109). Kaempferol can also modulate multiple signaling pathways, including MAPK, and can be used alone or in combination with 5-FU to overcome colon cancer drug resistance in cell-level assays and bioinformatics analysis studies (34, 110). Meanwhile, Choi (111) confirmed that kaempferol increases the activation of p-P38 MAPK in HCT116 and HCT15 cells. Anthocyanin, an important class of flavonoids, can potentially mediate CRC cell apoptosis by activating P38-MAPK (112) and inhibiting MMP-2 and MMP-9 activity (90). Silibinin, a flavonoid compound with good anti-cancer ability, can produce anti-cancer effects by regulating the expression of genes related to apoptosis, inflammation, and MAPK pathway proteins, and exhibits higher efficacy when combined with rutin, which is another flavonoid active substance (113). The combination of 5-FU and silibinin can inhibit the dual activation of PI3K/MAPK and CD44v6 to attenuate the activity of colon CSCs, thereby exhibiting potential as a molecular targeted drug (114).
Polyphenolic compounds
RES can exhibit anti-CRC activity through the MAPK pathway, for example, by activating the P38-MAPK in a bone morphogenetic protein receptor (BMPR)-dependent manner (115), and can decrease CRC cell survival through the P38-MAPK/c-Myc pathway (22). Bioinformatics analysis has revealed that RES can induce the expression of Raf-1 kinase inhibitory protein, thereby inhibiting the MAPK pathway (79). Mohapatra reported the synergistic effect of RES with 5-FU (116), and Wang found that RES can sensitize CRC to cetuximab by upregulating connexin-43 (77).
Studies have also revealed the anti-cancer effect of EGCG in the MAPK pathway. For example, EGCG induces apoptosis and P38-MAPK phosphorylation, thereby downregulating EGFR expression in colon cancer cells (117, 118). Furthermore, Inaba suggested that EGCG-induced P38-MAPK phosphorylation inhibits CRC cell growth by mediating apoptosis rather than regulating the cell cycle (119). Another study showed the involvement of c-Jun N-terminal kinase (JNK) in EGCG-induced apoptosis (120).
CCM inhibits colon cancer cell migration and invasion by inhibiting heparinase expression and P38 phosphorylation, which might be related to the P38-MAPK signaling pathway (121, 122). Moreover, CCM can inhibit insulin-stimulated MAPK and MEK phosphorylation in CRC cells. CCM can also mediate CRC cell apoptosis through JNK signaling, but not through P38 or ERK signaling (123). Furthermore, Sarco/endoplasmic reticulum calcium transport ATPases (SERCA) enzymes play important roles in several signal transduction pathways controlling proliferation, differentiation, and apoptosis (124). Among these enzymes, SERCA2 is involved in the malignant progression of CRC. Furthermore, the CCM analog F36 shows strong anti-cancer activity in CRC cells by targeting SERCA2 (125). Similarly, 6-gingerol, another class of compounds found in Ginger, has potential as a chemotherapeutic drug as it can inhibit the phosphorylation of ERK1/2 and JNK MAP kinases, as well as the activation of AP-1 transcription factors (126).
Other chemicals
Previous studies on colon cancer cell lines and nude mouse xenograft models have found that α-mangostin has an anti-cancer effect through regulation of the MAPK/ERK1/2 signal pathway (78, 127). Additionally, norcantharidin (NCTD) is a derivative of cantharidin, the main anti-cancer component of traditional Chinese medicine, Cantharides, which is hydrolyzed to remove dimethyl. Studies have found that NCTD has anti-colon cancer activity and can regulate the MAPK signaling pathway (128). Chen used a CRC xenograft model to show that NCTD reduces the expression of p-JNK and p-ERK in the MAPK pathway and exhibits anti-metastatic and anti-angiogenic activities (129). Moreover, Zhang discovered that NCTD inhibits tumor angiogenesis by blocking VEGFR2/MEK/ERK signaling but has little effect on the phosphorylation of P38-MAPK, Akt, or Cox-2 expression (130). This may indicate that NCTD could play a role in anti-cancer therapy as an anti-metastatic and anti-angiogenic agent.
Alantolactone, which is a plant-derived sesquiterpene lactone, exhibits various pharmacological activities, such as anti-bacterial, anti-inflammatory, and anti-viral effects. Alantolactone also shows anti-proliferative and apoptosis-promoting potential by activating the MAPK-JNK/c-Jun signaling pathway in human CRC cells (131). Moreover, the combined treatment of alantolactone and oxaliplatin shows improved anti-tumor activity (132), making it a potential treatment strategy for colon cancer.
Researchers have also discovered active ingredients from a variety of Chinese herbal medicines that can regulate MAPK signaling and inhibit colon cancer. For example, imperatorin can inhibit HIF-1α protein synthesis by downregulating mTOR/p70S6K/4EBP1 and MAPK pathways (133). Honokiol, which is extracted from the bark and leaves of the Chinese magnolia tree, can downregulate the activity of MAPK, PI3K/Akt, and dynamin-related protein 1 signaling and overcome acquired resistance to cetuximab (134). As the main active compound in many medicinal herbs, oleanolic acid significantly inhibits the activation of PI3K/Akt, mTOR/p70S6K and MAPK signaling (135). Taiwanin E, which is abundant in Taiwania cryptomerioides, is an estrogen analog that has recently been shown to inhibit CRC cell migration through the P38-MAPK pathway (136). In addition, the polysaccharide of Ganoderma lucidum has been confirmed to induce apoptosis in CRC cells by activating the MAPK/ERK pathway (137) and disruption of autophagosome-lysosome fusion (138).
Among several rocaglaol derivatives isolated from Dysoxylum gotadhora, 11k significantly inhibit the MAPK pathway in HCT116 cells (139). Furthermore, Torilis japonica extract not only modulates the P38-MAPK signaling pathway, but also induces apoptosis and modulates apoptosis-related proteins in the HCT 116 xenograft model (140). Moreover, the Boletus edulis ribonucleic acid fraction in Boletus edulis is able to inhibit DNA synthesis in HT-29 cells and effectively silence signal transduction of the MAPK/ERK pathway (141).
Summary
The MAPK cascade is a key pathway for human colon cancer cell survival, dissemination, and drug resistance (142). MAPK signaling can be transduced through three main pathways, namely the ERK, JNK and P-38 (143). In colon cancer cells, all three MAPK signaling pathways are involved in inducing apoptosis in different ways. In addition, the MAPK signaling pathway is involved in cell adhesion, angiogenesis, invasion, and metastasis of colon cancer (144).
However, the mechanism for developing anti-cancer active components from natural products based on this pathway remains unclear. Although a considerable number of active components have recently been reportedly related to the MAPK pathway, only a few studies have been dedicated to molecular mechanism. In addition, very few studies have provided new insights through the correlation of natural products with downstream targets, which allow us to gain a deeper understanding of the mechanisms by which natural products exert anti-CRC effects through the MAPK pathway. We have discussed these newly discovered pathway mechanisms above.
Janus kinase-signal transducer and activator of the transcription signaling pathway
Polyphenolic compounds
CCM has preventive and therapeutic effects on CRC through multiple targets and multiple pathways, and has also been studied in the JAK/STAT pathway. A recent study found that CCM can inhibit the expression of Nicotinamide N-methyltransferase (NNMT) and p-STAT3 in CRC cells and attenuate NNMT-induced resistance to 5-FU (145). Bioinformatics analysis identified AKT1, EGFR, and STAT3 as the core targets of CCM in the treatment of CRC (81). In addition, feruloylacetone, which is naturally degraded after CCM heating, can also significantly inhibit STAT3 phosphorylation and protein expression levels in CRC cells (146).
The anti-proliferative and anti-migratory effects of EGCG on CRC can be partially achieved by downregulating the expression of STAT3 (147). Moreover, the combination of CCM and EGCG not only attenuates the transition from normal endothelial cells to tumor endothelial cells induced by tumor conditioning medium by inhibiting the JAK/STAT3 signaling pathway, but also significantly reduces tumor growth and angiogenesis in a PDX mouse model of CRC; in this study, the combined anti-angiogenic effect was superior to that of CCM or EGCG alone (148). Among polyphenolic compounds, RES also reduces the level of activated STAT1 in the nucleus (149). However, there are no other studies of the mechanism by which the JAK/STAT pathway might be regulated in colon cancer.
Other chemicals
Diallyl disulfide induces apoptosis by upregulating the expression of STAT1 in COLO205 cells (150). The molecular mechanism by which allicin promotes apoptosis and inhibits cell proliferation in HCT116 cells has been linked to STAT3 signaling inhibition (151). Among the flavonoids, wogonin (96) and kaempferol (34) both regulate the JAK/STAT3 signaling pathway in CRC cell lines. In addition, a cinnamaldehyde derivative (CB-PIC) was found to suppress P-glycoprotein expression by inhibiting JAK/STAT3 and PI3K/Akt signaling to overcome drug resistance in chemo-resistant cancer cells, thereby becoming an effective chemosensitizer (152). Furthermore, tea polysaccharides can attenuate the progression of colitis-associated cancer by inhibiting the JAK/STAT3 pathway and the expression of downstream genes, such as IL-6 (153). Dihydrotansinone is a diterpenoid anthraquinone compound contained in traditional Chinese herbal medicines that can downregulate the expression of EGFR mRNA and STAT family proteins, leading to anti-proliferative effects (154). The use of cucurbitacin B in CRC cell lines reduces the expression level of pSTAT3 (155). Furthermore, propolis can enhance the apoptosis of butyrate-sensitive CRC cells and resensitize butyrate-resistant CRC cells by inhibiting PI3K/Akt and JAK/STAT pathway (156).
Summary
In colon cancer, aberrant IL-6/STAT/SOCS3 signaling may be critical for CRC development and progression (157). A dysfunctional JAK2/STAT3 signaling pathway can induce cancer and inhibit apoptosis (158), promote cancer cell migration by regulating E-cadherin and vimentin expression (159), and promote EMT (160). STAT3 plays a particularly crucial role in the regulation of inflammation (161). Most of the natural products discussed in this review also play a role through STAT3, such as allicin, CCM, EGCG, feruloylacetone, wogonin, kaempferol, dihydrotanshinone, cucurbitacin B, CB-PIC, tea polysaccharides, and propolis. Some natural products such as RES and diallyl disulfide can also resist cancer by interacting with the STAT1 protein.
TGF-β/SMAD signaling pathway
Polyphenolic compounds
CCM also exhibits anti-cancer properties via regulation of the TGF-β/SMADs signaling pathway. Moreover, CCM can reverse oxaliplatin resistance in CRC by inhibiting TGF-β/SMADs signaling at cellular and animal levels (162). CCM exhibits therapeutic potential to inhibit CRC cell metastasis via the interaction of stromal cells and tumor cells (163). Furthermore, CCM can upregulate the expression of TGF-β1 and HES-1 (164). Moreover, RES can inhibit EMT by mediating Snail/E-cadherin expression (165), as well as oncogenic processes and tumor growth, by regulating TGF expression (166). Oxyresveratrol, a natural derivative of RES, can also inhibit human colon cancer cell migration by regulating EMT and miRNA (167). In addition, EGCG can lead to a transient increase in TGF-β2 expression in CRC cells (168).
Other chemicals
Several other classes of compounds have been reported in recent years. For example, ursolic acid can inhibit CRC cell invasion by modulating the TGF-β1/zinc finger E-box-binding homeobox/miR-200c signaling network (169). SFN can reduce the protein expression and enzymatic activity of ornithine decarboxylase by inducing TGF-β/SMAD signaling (170). Baicalin inhibits endogenous and exogenous TGFβ1-induced EMT in CRC cells by inhibiting the TGFβ/SMAD pathway (171). Oxymatrine, an alkaloid extracted from the Chinese herbal medicine Sophora flavescens Ait, has been shown to inhibit the growth of CRC, and regulate the expression of TGF-β1, Smad4, and pSmad2 (172). Finally, tetrandrine is a bisbenzylisoquinoline alkaloid, whose anti-cancer activity has been demonstrated to inhibit PI3K/Akt signaling by upregulating TGF-β1 and reduce PTEN phosphorylation (173).
Summary
In colon cancer, the TGF-β/SMAD pathway has dual roles. In the early inflammatory response associated with CRC, TGF-β has an inhibitory effect on cancer development. Then, once CRC is identified, the TGF-β pathway promotes the development of cancer (162). An abnormal TGF-β/SMAD pathway can promote the migration of cancer cells by evading immune surveillance, inhibit apoptosis, promote tumor angiogenesis by regulating the expression of VEGF, and induce EMT in late tumor stages (165). Moreover, EMT regulated by the TGF-β/SMAD signaling pathway has been gradually recognized as an important mechanism of tumor chemoresistance. The anti-cancer activity of natural products through the TGF-β/SMAD pathway is most strongly manifested in the inhibition of EMT. Approximately half of the natural products have reported effects, including CCM, RES, oxyresveratrol, baicalin, and oxymatrine.
Notch signaling pathway
Natural compounds
CCM combined with cisplatin can promote the apoptosis of HT29 cells by regulating the expression of related apoptosis genes and inhibiting activation of the Notch1 signaling pathway (174). Quercetin and ionizing radiation combination therapy exhibits anti-cancer effects by targeting CSCs and inhibiting Notch1 signaling (175). Cucurbitacin B or cucurbitacin I can inhibit the growth of colon cancer by inhibiting the Notch signaling pathway (176). (-)-gossypol (177) and gossypolone (178), both downregulate Notch/Wnt signaling and inhibit the growth of CRC cells by inhibiting the RNA-binding protein Musashi-1. Moreover, genistein can inhibit the proliferation of several cancer cells and induce apoptosis. In CRC, genistein can inhibit colon cancer cell migration by reversing EMT through inhibition of the Notch1/NF-κB/slug/E-cadherin pathway (179), suggesting its potential as an antimetabolite for colon cancer.
Other chemicals
Secondary metabolites of lichens are promising biological resources for candidate anti-cancer drugs. For example, physciosporin, a compound isolated from Pseudocyphellaria granulata, inhibits colon cancer cells through the Notch signaling pathway (180). Moreover, withaferin-A, a bioactive compound derived from Withania somnifera, inhibits Notch-mediated prosurvival signaling, which promotes c-Jun-NH(2)-kinase-mediated apoptosis (181).
Summary
In colon cancer, Notch signaling is dysregulated throughout CRC tumorigenesis, with both Notch receptors and Notch ligands found to be dysregulated (182–184). Almost 80% of CRC cases have reported high expression of Notch1, Notch2, HES1, Delta1, and Jagged1 proteins (185). Moreover, abnormally high expression of the Jagged1 protein can promote cell proliferation and migration by promoting the expression of Cyclin D1, Cyclin E, and c-myc (186), as well as inducing EMT (187). Similarly, aberrant expression of Notch2 can promote tumor metastasis and EMT. miR-139-5p and its target gene Notch1 modulate CRC sensitivity to 5-FU by regulating the expression of its downstream MRP-1 and Bcl-2 (188), and Notch1 can also promote cancer development by inhibiting the expression of PTEN (189).
Hedgehog signaling pathway
The HH pathway plays a crucial role in embryonic development, tissue homeostasis, and carcinogenesis (190, 191). Recent studies have found that it also contributes to the resistance of cancer cells to various chemotherapeutic drugs (192). Considering current CRC treatment options, it is crucial to identify new drugs and strategies to help overcome HH-mediated drug resistance.
To date, only a few compounds are reported to inhibit cancer through the HH pathway. Inoscavin A, a pyrone compound isolated from Sanghuangporus vaninii extract, inhibits the activity of the HH pathway by inhibiting Smo, thereby inhibiting cell proliferation, promoting apoptosis, and exerting an anti-cancer effect (193). Physciosporin also inhibits colon cancer cells through the HH signaling pathway (180). Garcinone C, a natural xanthone derivative found in the Garcinia mangostana, can prevent the growth of colon tumors in both cells and animals through non-canonical HH signaling dependent on Gli1 (194). This suggests that garcinone C may be a good chemopreventive agent against the growth of colon tumors.
Hippo signaling pathway
As the Hippo pathway plays a critical role in cell proliferation, differentiation, apoptosis, and tumorigenesis, it has become a research hotspot in recent years. Components of the Hippo pathway are deregulated in various tumors, and the expression levels of its major signal transmitters are considered to be one of the prognostic factors in CRC (195). Moreover, EGCG inhibits colon cancer tumor growth by downregulating the sonic hedgehog protein (86).
A few natural compounds have been linked to this pathway in recent years. Chai revealed a novel therapeutic mechanism of cucurbitacin B, namely, inhibition of the proliferation and invasion of CRC cells and promotion of apoptosis via inhibition of Hippo-YAP signaling and its downstream target genes Cyr 61 and c-Myc in CRC cells (196). Xue found that fucoidan can activate the Hippo pathway and downregulate the β-catenin pathway to induce tumor cell apoptosis and inhibit tumor growth, and that fucoidan can prevent CRC by regulating intestinal microbiology (197). Additionally, lappaol F, a natural lignan from Arctium lappa Linné, inhibits tumor cell growth by inducing cell cycle arrest. Lappaol F was recently recognized as an inhibitor of YAP to exert anti-CRC activity (198). Ginsenoside compound K, a component of ginseng, exhibits an anti-CRC effect by inhibiting the expression of adipocyte-specific phospholipase A2, which could promote the progression of CRC by inhibiting the Hippo signaling pathway (199).
Concluding remarks
Among polyphenols, CCM, EGCG, and RES exhibit proven anti-cancer effects through various signaling pathways (Table 1). Through the regulation of various pathways by EGCG, CCM, and RES, we can gain a deeper understanding of the crosstalk between these pathways and the overall mechanism by which natural compounds exert anti-cancer effects. These three compounds are still widely studied by researchers, especially in relation to NF-κB and PI3K/Akt pathways. With advances in bioinformation technology, many studies have verified the correlation between these three compounds and the pathways discussed in this review, for example, through bioinformatics analysis and molecular docking, with all reporting positive correlations. In addition, researchers have focused on the analogs or metabolites of these three substances, and attempted to discover the smallest amounts of these natural products that can exert anti-cancer effects, which can contribute to the future development of novel anti-cancer drugs based on natural active products. Another large class of natural active products is flavonoids. At present, new flavonoids are extracted from plants every year and subsequently investigated via research into anti-cancer treatments. Thus, the articles discussed in this review report a relatively large number of flavonoids. In addition to the safety of natural compounds in the human body, clinical trials have also proven the ability of such compounds to inhibit cancer.
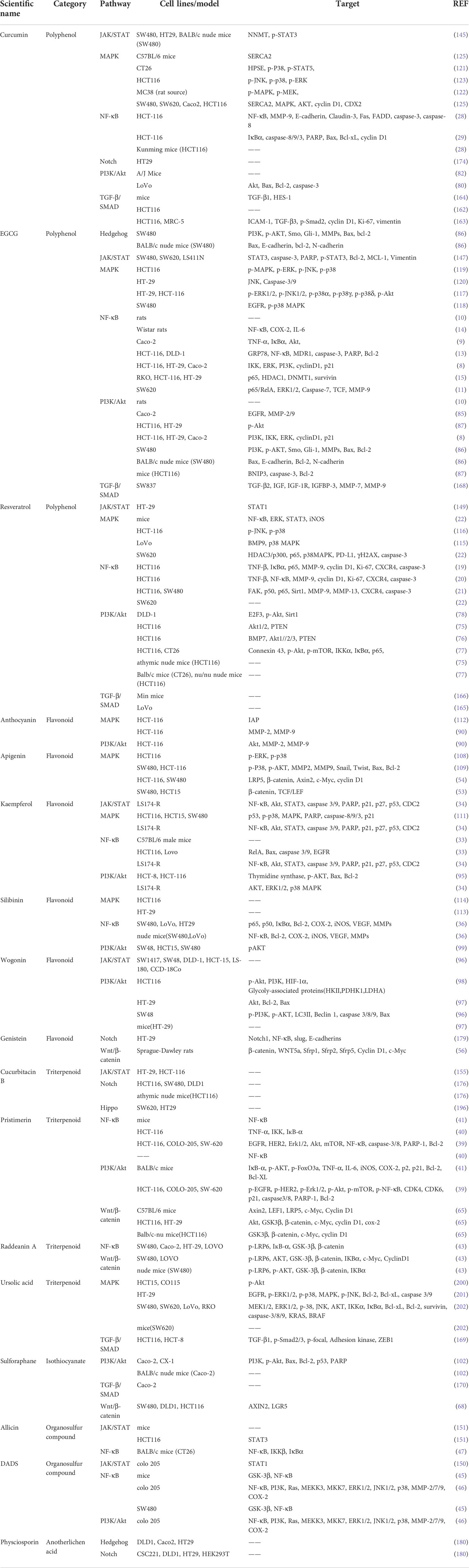
Table 1 Summary of main natural compounds involved in different pathways with therapeutic approach to colorectal cancer.
Among the many natural compounds, clinical studies on CCM are particularly abundant. For example, a phase II trial study reported that oral CCM is a safe adjunctive drug with added benefits in FOLFOX chemotherapy for patients with metastatic CRC (203). Moreover, treating CCM with liposomes can improve its high hydrophobicity. Another phase I clinical trials have also shown the safety of intravenous liposomal CCM (204). A study using micronized RES, which is called SRT501, found that it was well tolerated in patients with liver metastases from CRC, and had certain clinical benefits (205). A group of clinical trials recruiting patients with colon cancer resection and polypectomy showed that continuous long-term treatment with a combination of daily standard doses of apigenin (20 mg) and EGCG (20 mg) can reduce the recurrence rate of tumors (206). In clinical trials on the efficacy of EGCG, researchers have mostly employed oral green tea preparations. Indeed, oral green tea extract (EGCG, 800 mg/day) or green tea polyphenol preparation Poly E (EGCG, 780 mg/day) is well tolerated by people at high risk of CRC (207). Oral green tea extract can also reduce DNA methyltransferase (DNMT1) and NF-κB mRNA in CRC tissues (16), whereas oral Poly E administration for six months failed to reduce rectal abnormal crypt foci numbers in afflicted subjects (207). However, as this evidence is insufficient to describe the role of EGCG in preventing CRC, longer-term clinical trials are required. The combined use of natural compounds with traditional chemotherapy drugs is worthy of further research attention. Natural compounds have an ability to reduce the side effects and improve the efficacy of conventional chemotherapy, which places a considerable burden on the patient’s body (Table 2).
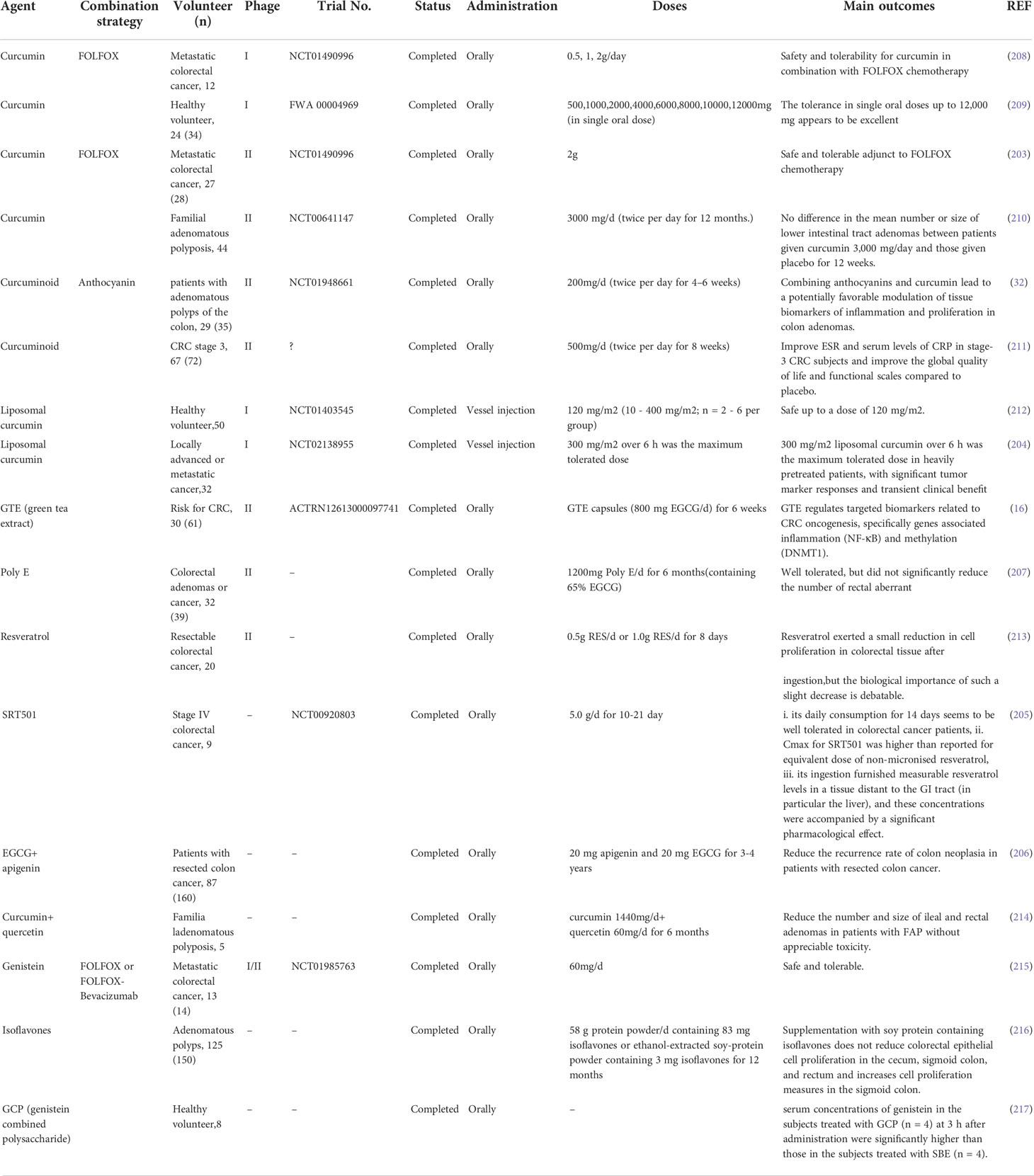
Table 2 Clinical trials studied in natural compound for chemoprevention and treatment of colorectal cancer.
It is worth mentioning that immunotherapy by inhibiting immune checkpoints as an anti-cancer method has attracted much attention in recent years. It is regarded as an effective treatment to improve the survival rate of patients with advanced or/and metastatic cancer (218). However, the serious adverse reactions that may be caused by immunotherapy, and the cancer cells themselves may not respond to immunotherapy, seriously restrict the clinical application of immunotherapy. At present, natural compounds have been shown to modulate intracellular biochemical reactions including the regulation of immune checkpoints such as PD-L1 and cytotoxic T-lymphocyte antigen 4 (CTLA-4), through a variety of signaling pathways. These effects have been confirmed in multiple cancer types including colon cancer (219). Previous studies have revealed that NF-κB, PI3K and MAPK signaling pathways were involved in the regulation of CRC via PD-L1 by resveratrol, piceatannol and panaxadiol (22, 220). Therefore, it might be predicted that natural compounds combined with immunotherapy will bring us new ideas for cancer therapy. Future clinical trials may validate the synergy of the natural compounds and immune checkpoint inhibitors.
Natural compounds and their potential for clinical applications undoubtedly have many advantages. Nevertheless, many limitations hinder the industrialization of many types of natural compounds:
1. The excellent safety and tolerable safe dose to the human body are certainly advantages of natural compounds; however, in most natural compounds, their safety is ascribed to their easy degradation by the human digestive system. As such, it is difficult to achieve a sufficient blood drug concentration via oral administration. Moreover, their decomposition efficiency in the digestive system is difficult to calculate, which further increases the difficulty of selecting an appropriate dose for clinical applications.
2. Most of the natural compounds discussed in this review are derived from food. However, according to epidemiological statistical reports, patients with CRC that ingest these natural compounds through diet alone do not report anti-CRC effects, particularly after surgery or during chemotherapy. Therefore, a method of extracting these natural compounds from animals and plants must be found, and the cost of such extraction must also be considered.
3. The anti-cancer mechanism of many natural compounds has not been well studied, the downstream genes remain unclear, and in vivo experiments are lacking. Currently, only research into RES, EGCG, CCM, genistein, and quercetin has achieved clinical levels.
4. The combined use of natural products and existing chemotherapeutic drugs has been the focus of many studies; however, nanotechnology advances in recent years have opened up new prospects for the application of natural compounds. Specifically, combining natural compounds with nanomaterials could overcome several of the shortcomings of natural products, such as high hydrophobicity and rapid degradation, and enable the development of new application forms based on natural compounds, such as intravenous injection.
Author contributions
XL and YJ designed the study. MW and XL wrote the manuscript. TC, XC, HX and XM edited the paper. YJ supervised the whole paper. All authors contributed to the article and approved the submitted version.
Funding
This study was supported by Department of Science and Technology of Jilin Provincial (no. 20200201430JC), Jilin Province Development and Reform Commission (no. 2021C043-9), Education Department of Jilin Province (no. JJKH20220194KJ), and the PhD Research Project of Jilin Engineering Normal University (no. BSKJ201923).
Acknowledgments
We would like to thank the Editage team for English language editing.
Conflict of interest
The authors declare that the research was conducted in the absence of any commercial or financial relationships that could be construed as a potential conflict of interest.
Publisher’s note
All claims expressed in this article are solely those of the authors and do not necessarily represent those of their affiliated organizations, or those of the publisher, the editors and the reviewers. Any product that may be evaluated in this article, or claim that may be made by its manufacturer, is not guaranteed or endorsed by the publisher.
References
1. Dias DA, Urban S, Roessner U. A historical overview of natural products in drug discovery. Metabolites (2012) 2(2):303–36. doi: 10.3390/metabo2020303
2. Wu CX, Gu K, Gong YM, Zheng YS, Wang SM, Chen R. Analysis of incidence and mortality of colorectal in China, 2015. Zhongguo Ai Zheng Za Zhi (2020) 3(04):241–5. doi: 10.19401/j.cnki.1007-3639.2020.04.001
3. Xie YH, Chen YX, Fang JY. Comprehensive review of targeted therapy for colorectal cancer. Signal Transduct Target Ther (2020) 5(1):22. doi: 10.1038/s41392-020-0116-z
4. Xian Z, Hu B, Wang T, Zeng J, Cai J, Zou Q, et al. Lncrna uca1 contributes to 5-fluorouracil resistance of colorectal cancer cells through mir-23b-3p/znf281 axis. Onco Targets Ther (2020) 13:7571–83. doi: 10.2147/OTT.S258727
5. Pardini B, Kumar R, Naccarati A, Novotny J, Prasad RB, Forsti A, et al. 5-Fluorouracil-Based chemotherapy for colorectal cancer and mthfr/mtrr genotypes. Br J Clin Pharmacol (2011) 72(1):162–3. doi: 10.1111/j.1365-2125.2010.03892.x
6. de Gramont A, Figer A, Seymour M, Homerin M, Hmissi A, Cassidy J, et al. Leucovorin and fluorouracil with or without oxaliplatin as first-line treatment in advanced colorectal cancer. J Clin Oncol (2000) 18(16):2938–47. doi: 10.1200/JCO.2000.18.16.2938
7. Singh BN, Shankar S, Srivastava RK. Green tea catechin, epigallocatechin-3-gallate (egcg): mechanisms, perspectives and clinical applications. Biochem Pharmacol (2011) 82(12):1807–21. doi: 10.1016/j.bcp.2011.07.093
8. Zhang X, Min KW, Wimalasena J, Baek SJ. Cyclin d1 degradation and p21 induction contribute to growth inhibition of colorectal cancer cells induced by epigallocatechin-3-gallate. J Cancer Res Clin Oncol (2012) 138(12):2051–60. doi: 10.1007/s00432-012-1276-1
9. Navarro-Perán E, Cabezas-Herrera J, Sánchez-del-Campo L, García-Cánovas F, Rodríguez-López JN. The anti-inflammatory and anti-cancer properties of epigallocatechin-3-gallate are mediated by folate cycle disruption, adenosine release and nf-κb suppression. Inflammation Res (2008) 57(10):472–8. doi: 10.1007/s00011-008-8013-x
10. Wang Y, Jin HY, Fang MZ, Wang XF, Chen H, Huang SL, et al. Epigallocatechin gallate inhibits dimethylhydrazine-induced colorectal cancer in rats. World J Gastroenterol (2020) 26(17):2064–81. doi: 10.3748/wjg.v26.i17.2064
11. Zhou F, Zhou H, Wang T, Mu Y, Wu B, Guo DL, et al. Epigallocatechin-3-gallate inhibits proliferation and migration of human colon cancer sw620 cells in vitro. Acta Pharmacol Sin (2012) 33(1):120–6. doi: 10.1038/aps.2011.139
12. Garzon R, Fabbri M, Cimmino A, Calin GA, Croce CM. Microrna expression and function in cancer. Trends Mol Med (2006) 12(12):580–7. doi: 10.1016/j.molmed.2006.10.006
13. La X, Zhang L, Li Z, Li H, Yang Y. (-)-Epigallocatechin gallate (egcg) enhances the sensitivity of colorectal cancer cells to 5-fu by inhibiting grp78/nf-kappab/mir-155-5p/mdr1 pathway. J Agric Food Chem (2019) 67(9):2510–8. doi: 10.1021/acs.jafc.8b06665
14. Afzal SM, Vafa A, Rashid S, Shree A, Islam J, Ali N, et al. Amelioration of n,n′-dimethylhydrazine induced colon toxicity by epigallocatechin gallate in wistar rats. Hum Exp Toxicol (2021) 40(9):1558–71. doi: 10.1177/09603271211002884
15. Saldanha SN, Kala R, Tollefsbol TO. Molecular mechanisms for inhibition of colon cancer cells by combined epigenetic-modulating epigallocatechin gallate and sodium butyrate. Exp Cell Res (2014) 324(1):40–53. doi: 10.1016/j.yexcr.2014.01.024
16. Hu Y, McIntosh GH, Le Leu RK, Somashekar R, Meng XQ, Gopalsamy G, et al. Supplementation with brazil nuts and green tea extract regulates targeted biomarkers related to colorectal cancer risk in humans. Br J Nutr (2016) 116(11):1901–11. doi: 10.1017/S0007114516003937
17. Aziz MH, Kumar R, Ahmad N. Cancer chemoprevention by resveratrol: in vitro and in vivo studies and the underlying mechanisms (review). Int J Oncol (2003) 23(1):17–28. doi: 10.3892/ijo.23.1.17
18. Dariya B, Behera SK, Srivani G, Farran B, Alam A, Nagaraju GP. Computational analysis of nuclear factor-κb and resveratrol in colorectal cancer. J Biomol Struct Dyn (2021) 39(8):2914–22. doi: 10.1080/07391102.2020.1757511
19. Buhrmann C, Yazdi M, Popper B, Shayan P, Goel A, Aggarwal BB, et al. Evidence that tnf-beta induces proliferation in colorectal cancer cells and resveratrol can down-modulate it. Exp Biol Med (Maywood) (2019) 244(1):1–12. doi: 10.1177/1535370218824538
20. Buhrmann C, Yazdi M, Popper B, Shayan P, Goel A, Aggarwal BB, et al. Resveratrol chemosensitizes tnf-beta-induced survival of 5-fu-treated colorectal cancer cells. Nutrients (2018) 10(7):888–907. doi: 10.3390/nu10070888
21. Buhrmann C, Shayan P, Goel A, Shakibaei M. Resveratrol regulates colorectal cancer cell invasion by modulation of focal adhesion molecules. Nutrients (2017) 9(10):1073. doi: 10.3390/nu9101073
22. Lucas J, Hsieh TC, Halicka HD, Darzynkiewicz Z, Wu JM. Upregulation of pdl1 expression by resveratrol and piceatannol in breast and colorectal cancer cells occurs via hdac3/p300mediated nfkappab signaling. Int J Oncol (2018) 53(4):1469–80. doi: 10.3892/ijo.2018.4512
23. Youn J, Lee JS, Na HK, Kundu JK, Surh YJ. Resveratrol and piceatannol inhibit inos expression and nf-kappab activation in dextran sulfate sodium-induced mouse colitis. Nutr Cancer (2009) 61(6):847–54. doi: 10.1080/01635580903285072
24. Summerlin N, Qu Z, Pujara N, Sheng Y, Jambhrunkar S, McGuckin M, et al. Colloidal mesoporous silica nanoparticles enhance the biological activity of resveratrol. Colloids Surf B Biointerfaces (2016) 144:1–7. doi: 10.1016/j.colsurfb.2016.03.076
25. Menon VP, Sudheer AR. Antioxidant and anti-inflammatory properties of curcumin. Adv Exp Med Biol (2007) 595:105–25. doi: 10.1007/978-0-387-46401-5_3
26. Unlu A, Nayir E, Dogukan Kalenderoglu M, Kirca O, Ozdogan M. Curcumin (Turmeric) and Cancer J BUON (2016) 21(5):1050–60.
27. Ismail NI, Othman I, Abas F, HL N, Naidu R. Mechanism of apoptosis induced by curcumin in colorectal cancer. Int J Mol Sci (2019) 20(10):2454–88. doi: 10.3390/ijms20102454
28. Xiang L, He B, Liu Q, Hu D, Liao W, Li R, et al. Antitumor effects of curcumin on the proliferation, migration and apoptosis of human colorectal carcinoma hct116 cells. Oncol Rep (2020) 44(5):1997–2008. doi: 10.3892/or.2020.7765
29. Shakibaei M, Mobasheri A, Lueders C, Busch F, Shayan P, Goel A. Curcumin enhances the effect of chemotherapy against colorectal cancer cells by inhibition of nf-kappab and src protein kinase signaling pathways. PLoS One (2013) 8(2):e57218. doi: 10.1371/journal.pone.0057218
30. Adachi S, Hamoya T, Fujii G, Narita T, Komiya M, Miyamoto S, et al. Theracurmin inhibits intestinal polyp development in apc-mutant mice by inhibiting inflammation-related factors. Cancer Sci (2020) 111(4):1367–74. doi: 10.1111/cas.14329
31. Wong KE, Ngai SC, Chan KG, Lee LH, Goh BH, Chuah LH. Curcumin nanoformulations for colorectal cancer: a review. Front Pharmacol (2019) 10:152. doi: 10.3389/fphar.2019.00152
32. Briata IM, Paleari L, Rutigliani M, Petrera M, Caviglia S, Romagnoli P, et al. A presurgical study of curcumin combined with anthocyanin supplements in patients with colorectal adenomatous polyps. Int J Mol Sci (2021) 22(20):11024–38 . doi: 10.3390/ijms222011024
33. Zhou M, Li J, Luo D, Zhang H, Yu Z, Chen Y, et al. Network pharmacology and molecular docking-based investigation: prunus mume against colorectal cancer via silencing rela expression. Front Pharmacol (2021) 12:761980. doi: 10.3389/fphar.2021.761980
34. Riahi-Chebbi I, Souid S, Othman H, Haoues M, Karoui H, Morel A, et al. The phenolic compound kaempferol overcomes 5-fluorouracil resistance in human resistant ls174 colon cancer cells. Sci Rep (2019) 9(1):195. doi: 10.1038/s41598-018-36808-z
35. Yun JM, Afaq F, Khan N, Mukhtar H. Delphinidin, an anthocyanidin in pigmented fruits and vegetables, induces apoptosis and cell cycle arrest in human colon cancer hct116 cells. Mol Carcinog (2009) 48(3):260–70. doi: 10.1002/mc.20477
36. Raina K, Agarwal C, Agarwal R. Effect of silibinin in human colorectal cancer cells: Targeting the activation of nf-kappab signaling. Mol Carcinog (2013) 52(3):195–206. doi: 10.1002/mc.21843
37. Hu Q, Zhang W, Wu Z, Tian X, Xiang J, Li L, et al. Baicalin and the liver-gut system: pharmacological bases explaining its therapeutic effects. Pharmacol Res (2021) 165:105444. doi: 10.1016/j.phrs.2021.105444
38. Li JJ, Yan YY, Sun HM, Liu Y, Su CY, Chen HB, et al. Anti-cancer effects of pristimerin and the mechanisms: a critical review. Front Pharmacol (2019) 10:746. doi: 10.3389/fphar.2019.00746
39. Yousef BA, Guerram M, Hassan HM, Hamdi AM, Zhang LY, Jiang ZZ. Pristimerin demonstrates anticancer potential in colorectal cancer cells by inducing g1 phase arrest and apoptosis and suppressing various pro-survival signaling proteins. Oncol Rep (2016) 35(2):1091–100. doi: 10.3892/or.2015.4457
40. Yousef BA, Hassan HM, Zhang LY, Jiang ZZ. Pristimerin exhibits in vitro and in vivo anticancer activities through inhibition of nuclear factor-small ka, cyrillicb signaling pathway in colorectal cancer cells. Phytomedicine (2018) 40:140–7. doi: 10.1016/j.phymed.2018.01.008
41. Park JH, Kim JK. Pristimerin, a naturally occurring triterpenoid, attenuates tumorigenesis in experimental colitis-associated colon cancer. Phytomedicine (2018) 42:164–71. doi: 10.1016/j.phymed.2018.03.033
42. Naz I, Ramchandani S, Khan MR, Yang MH, Ahn KS. Anticancer potential of raddeanin a, a natural triterpenoid isolated from anemone raddeana regel. Molecules (2020) 25(5):1035–56. doi: 10.3390/molecules25051035
43. Wang Y, Bao X, Zhao A, Zhang J, Zhang M, Zhang Q, et al. Raddeanin a inhibits growth and induces apoptosis in human colorectal cancer through downregulating the wnt/beta-catenin and nf-kappab signaling pathway. Life Sci (2018) 207:532–49. doi: 10.1016/j.lfs.2018.06.035
44. Ban JO, Yuk DY, Woo KS, Kim TM, Lee US, Jeong HS, et al. Inhibition of cell growth and induction of apoptosis via inactivation of nf-kappab by a sulfurcompound isolated from garlic in human colon cancer cells. J Pharmacol Sci (2007) 104(4):374–83. doi: 10.1254/jphs.fp0070789
45. Saud SM, Li W, Gray Z, Matter MS, Colburn NH, Young MR, et al. Diallyl disulfide (dads), a constituent of garlic, inactivates nf-kappab and prevents colitis-induced colorectal cancer by inhibiting gsk-3beta. Cancer Prev Res (Phila) (2016) 9(7):607–15. doi: 10.1158/1940-6207.CAPR-16-0044
46. Lai KC, Hsu SC, Kuo CL, Yang JS, Ma CY, Lu HF, et al. Diallyl sulfide, diallyl disulfide, and diallyl trisulfide inhibit migration and invasion in human colon cancer colo 205 cells through the inhibition of matrix metalloproteinase-2, -7, and -9 expressions. Environ Toxicol (2013) 28(9):479–88. doi: 10.1002/tox.20737
47. Huang WL, Wu SF, Xu ST, Ma YC, Wang R, Jin S, et al. Allicin enhances the radiosensitivity of colorectal cancer cells via inhibition of nf-kappab signaling pathway. J Food Sci (2020) 85(6):1924–31. doi: 10.1111/1750-3841.15156
48. DiDonato JA, Mercurio F, Karin M. Nf-kappab and the link between inflammation and cancer. Immunol Rev (2012) 246(1):379–400. doi: 10.1111/j.1600-065X.2012.01099.x
49. Ben-Neriah Y, Karin M. Inflammation meets cancer, with nf-kappab as the matchmaker. Nat Immunol (2011) 12(8):715–23. doi: 10.1038/ni.2060
50. Naugler WE, Karin M. Nf-kappab and cancer-identifying targets and mechanisms. Curr Opin Genet Dev (2008) 18(1):19–26. doi: 10.1016/j.gde.2008.01.020
51. Pandurangan AK, Dharmalingam P, Sadagopan SK, Ramar M, Munusamy A, Ganapasam S. Luteolin induces growth arrest in colon cancer cells through involvement of wnt/beta-catenin/gsk-3beta signaling. J Environ Pathol Toxicol Oncol (2013) 32(2):131–9. doi: 10.1615/jenvironpatholtoxicoloncol.2013007522
52. Ashokkumar P, Sudhandiran G. Luteolin inhibits cell proliferation during azoxymethane-induced experimental colon carcinogenesis via wnt/beta-catenin pathway. Invest New Drugs (2011) 29(2):273–84. doi: 10.1007/s10637-009-9359-9
53. Xu M, Wang S, Song YU, Yao J, Huang K, Zhu X. Apigenin suppresses colorectal cancer cell proliferation, migration and invasion via inhibition of the wnt/beta-catenin signaling pathway. Oncol Lett (2016) 11(5):3075–80. doi: 10.3892/ol.2016.4331
54. Lin CM, Chen HH, Lin CA, Wu HC, Sheu JJ, Chen HJ. Apigenin-induced lysosomal degradation of beta-catenin in wnt/beta-catenin signaling. Sci Rep (2017) 7(1):372. doi: 10.1038/s41598-017-00409-z
55. Pintova S, Planutis K, Planutiene M, Holcombe RF. Me-143 is superior to genistein in suppression of wnt signaling in colon cancer cells. Anticancer Res (2017) 37(4):1647–53. doi: 10.21873/anticanres.11495
56. Zhang Y, Li Q, Zhou D, Chen H. Genistein, a soya isoflavone, prevents azoxymethane-induced up-regulation of wnt/beta-catenin signalling and reduces colon pre-neoplasia in rats. Br J Nutr (2013) 109(1):33–42. doi: 10.1017/S0007114512000876
57. Fonseca BF, Predes D, Cerqueira DM, Reis AH, Amado NG, Cayres MC, et al. Derricin and derricidin inhibit wnt/beta-catenin signaling and suppress colon cancer cell growth in vitro. PLoS One (2015) 10(3):e0120919. doi: 10.1371/journal.pone.0120919
58. Li Y, Qin X, Li P, Zhang H, Lin T, Miao Z, et al. Isobavachalcone isolated from psoralea corylifolia inhibits cell proliferation and induces apoptosis via inhibiting the akt/gsk-3beta/beta-catenin pathway in colorectal cancer cells. Drug Des Devel Ther (2019) 13:1449–60. doi: 10.2147/DDDT.S192681
59. Razak S, Afsar T, Ullah A, Almajwal A, Alkholief M, Alshamsan A, et al. Taxifolin, a natural flavonoid interacts with cell cycle regulators causes cell cycle arrest and causes tumor regression by activating wnt/beta-catenin signaling pathway. BMC Cancer (2018) 18(1):1043. doi: 10.1186/s12885-018-4959-4
60. Mishra A, Punia JK, Bladen C, Zamponi GW, Goel RK. Anticonvulsant mechanisms of piperine, a piperidine alkaloid. Channels (Austin) (2015) 9(5):317–23. doi: 10.1080/19336950.2015.1092836
61. de Almeida GC, Oliveira LFS, Predes D, Fokoue HH, Kuster RM, Oliveira FL, et al. Piperine suppresses the wnt/beta-catenin pathway and has anti-cancer effects on colorectal cancer cells. Sci Rep (2020) 10(1):11681. doi: 10.1038/s41598-020-68574-2
62. Srivastava S, Dewangan J, Mishra S, Divakar A, Chaturvedi S, Wahajuddin M, et al. Piperine and celecoxib synergistically inhibit colon cancer cell proliferation via modulating wnt/beta-catenin signaling pathway. Phytomedicine (2021) 84:153484. doi: 10.1016/j.phymed.2021.153484
63. Wang P, Gao XY, Yang SQ, Sun ZX, Dian LL, Qasim M, et al. Jatrorrhizine inhibits colorectal carcinoma proliferation and metastasis through wnt/beta-catenin signaling pathway and epithelial-mesenchymal transition. Drug Des Devel Ther (2019) 13:2235–47. doi: 10.2147/DDDT.S207315
64. Zhu M, Gong Z, Wu Q, Shi X, Su Q, Zhang Y. Sanguinarine suppresses migration and metastasis in colorectal carcinoma associated with the inversion of emt through the wnt/beta-catenin signaling. Clin Transl Med (2020) 10(1):1–12. doi: 10.1002/ctm2.1
65. Zhao Q, Bi Y, Zhong J, Ren Z, Liu Y, Jia J, et al. Pristimerin suppresses colorectal cancer through inhibiting inflammatory responses and wnt/beta-catenin signaling. Toxicol Appl Pharmacol (2020) 386:114813. doi: 10.1016/j.taap.2019.114813
66. Yang P, Liu W, Fu R, Ding GB, Amin S, Li Z. Cucurbitacin e chemosensitizes colorectal cancer cells via mitigating tfap4/wnt/beta-catenin signaling. J Agric Food Chem (2020) 68(48):14148–60. doi: 10.1021/acs.jafc.0c05551
67. Ye Q, Yao G, Zhang M, Guo G, Hu Y, Jiang J, et al. A novel ent-kaurane diterpenoid executes antitumor function in colorectal cancer cells by inhibiting wnt/beta-catenin signaling. Carcinogenesis (2015) 36(3):318–26. doi: 10.1093/carcin/bgv003
68. Bernkopf DB, Daum G, Bruckner M, Behrens J. Sulforaphane inhibits growth and blocks Wnt/Beta-catenin signaling of colorectal cancer cells. Oncotarget (2018) 9(74):33982–94. doi: 10.18632/oncotarget.26125
69. Chen Y, Li Y, Wang XQ, Meng Y, Zhang Q, Zhu JY, et al. Phenethyl isothiocyanate inhibits colorectal cancer stem cells by suppressing wnt/beta-catenin pathway. Phytother Res (2018) 32(12):2447–55. doi: 10.1002/ptr.6183
70. Li X, Kong L, Yang Q, Duan A, Ju X, Cai B, et al. Parthenolide inhibits ubiquitin-specific peptidase 7 (Usp7), wnt signaling, and colorectal cancer cell growth. J Biol Chem (2020) 295(11):3576–89. doi: 10.1074/jbc.RA119.011396
71. Chen C, Zhu D, Zhang H, Han C, Xue G, Zhu T, et al. Yap-dependent ubiquitination and degradation of beta-catenin mediates inhibition of wnt signalling induced by physalin f in colorectal cancer. Cell Death Dis (2018) 9(6):591. doi: 10.1038/s41419-018-0645-3
72. Ye ZN, Yuan F, Liu JQ, Peng XR, An T, Li X, et al. Physalis peruviana-derived 4beta-hydroxywithanolide e, a novel antagonist of wnt signaling, inhibits colorectal cancer in vitro and in vivo. Molecules (2019) 24(6):1146–59. doi: 10.3390/molecules24061146
73. White BD, Chien AJ, Dawson DW. Dysregulation of wnt/beta-catenin signaling in gastrointestinal cancers. Gastroenterology (2012) 142(2):219–32. doi: 10.1053/j.gastro.2011.12.001
74. Baldus SE, Monig SP, Huxel S, Landsberg S, Hanisch FG, Engelmann K, et al. Muc1 and nuclear beta-catenin are coexpressed at the invasion front of colorectal carcinomas and are both correlated with tumor prognosis. Clin Cancer Res (2004) 10(8):2790–6. doi: 10.1158/1078-0432.ccr-03-0163
75. Liu YZ, Wu K, Huang J, Liu Y, Wang X, Meng ZJ, et al. The pten/pi3k/akt and wnt/beta-catenin signaling pathways are involved in the inhibitory effect of resveratrol on human colon cancer cell proliferation. Int J Oncol (2014) 45(1):104–12. doi: 10.3892/ijo.2014.2392
76. Zeng YH, Zhou LY, Chen QZ, Li Y, Shao Y, Ren WY, et al. Resveratrol inactivates pi3k/akt signaling through upregulating bmp7 in human colon cancer cells. Oncol Rep (2017) 38(1):456–64. doi: 10.3892/or.2017.5662
77. Wang Y, Wang W, Wu X, Li C, Huang Y, Zhou H, et al. Resveratrol sensitizes colorectal cancer cells to cetuximab by connexin 43 upregulation-induced akt inhibition. Front Oncol (2020) 10:383. doi: 10.3389/fonc.2020.00383
78. Kumazaki M, Noguchi S, Yasui Y, Iwasaki J, Shinohara H, Yamada N, et al. Anti-cancer effects of naturally occurring compounds through modulation of signal transduction and mirna expression in human colon cancer cells. J Nutr Biochem (2013) 24(11):1849–58. doi: 10.1016/j.jnutbio.2013.04.006
79. Dariya B, Behera SK, Srivani G, Aliya S, Alam A, Nagaraju GP. Resveratrol binds and activates rkip protein in colorectal cancer. Amino Acids (2020) 52(9):1299–306. doi: 10.1007/s00726-020-02889-2
80. Jiang QG, Li TY, Liu DN, Zhang HT. Pi3k/Akt pathway involving into apoptosis and invasion in human colon cancer cells lovo. Mol Biol Rep (2014) 41(5):3359–67. doi: 10.1007/s11033-014-3198-2
81. Han X, Yang C, Guo C, Xu Y, Liu X, Xie R, et al. Bioinformatics analysis to screen key targets of curcumin against colorectal cancer and the correlation with tumor-infiltrating immune cells. Evid Based Complement Alternat Med (2021) 2021:9132608. doi: 10.1155/2021/9132608
82. Wu X, Koh GY, Huang Y, Crott JW, Bronson RT, Mason JB. The combination of curcumin and salsalate is superior to either agent alone in suppressing pro-cancerous molecular pathways and colorectal tumorigenesis in obese mice. Mol Nutr Food Res (2019) 63(8):e1801097. doi: 10.1002/mnfr.201801097
83. Kasdagly M, Radhakrishnan S, Reddivari L, Veeramachaneni DN, Vanamala J. Colon carcinogenesis: influence of western diet-induced obesity and targeting stem cells using dietary bioactive compounds. Nutrition (2014) 30(11-12):1242–56. doi: 10.1016/j.nut.2014.02.016
84. Ramasamy TS, Ayob AZ, Myint HH, Thiagarajah S, Amini F. Targeting colorectal cancer stem cells using curcumin and curcumin analogues: insights into the mechanism of the therapeutic efficacy. Cancer Cell Int (2015) 15:96. doi: 10.1186/s12935-015-0241-x
85. Zhu W, Li MC, Wang FR, Mackenzie GG, Oteiza PI. The inhibitory effect of ecg and egcg dimeric procyanidins on colorectal cancer cells growth is associated with their actions at lipid rafts and the inhibition of the epidermal growth factor receptor signaling. Biochem Pharmacol (2020) 175:113923. doi: 10.1016/j.bcp.2020.113923
86. Ding F, Yang S. Epigallocatechin-3-gallate inhibits proliferation and triggers apoptosis in colon cancer via the hedgehog/phosphoinositide 3-kinase pathways. Can J Physiol Pharmacol (2021) 99(9):910–20. doi: 10.1139/cjpp-2020-0588
87. Khiewkamrop P, Surangkul D, Srikummool M, Richert L, Pekthong D, Parhira S, et al. Epigallocatechin gallate triggers apoptosis by suppressing de novo lipogenesis in colorectal carcinoma cells. FEBS Open Bio (2022) 12(5):937–58. doi: 10.1002/2211-5463.13391
88. Gao X, Li X, Ho CT, Lin X, Zhang Y, Li B, et al. Cocoa tea (camellia ptilophylla) induces mitochondria-dependent apoptosis in hct116 cells via ros generation and pi3k/akt signaling pathway. Food Res Int (2020) 129:108854. doi: 10.1016/j.foodres.2019.108854
89. Zhang R, Yu Q, Lu W, Shen J, Zhou D, Wang Y, et al. Grape seed procyanidin b2 promotes the autophagy and apoptosis in colorectal cancer cells via regulating pi3k/akt signaling pathway. Onco Targets Ther (2019) 12:4109–18. doi: 10.2147/OTT.S195615
90. Shin DY, Lu JN, Kim GY, Jung JM, Kang HS, Lee WS, et al. Anti-invasive activities of anthocyanins through modulation of tight junctions and suppression of matrix metalloproteinase activities in hct-116 human colon carcinoma cells. Oncol Rep (2011) 25(2):567–72. doi: 10.3892/or.2010.1104
91. Lu X, Wu X, Jing L, Tao L, Zhang Y, Huang R, et al. Network pharmacology analysis and experiments validation of the inhibitory effect of jianpi fu recipe on colorectal cancer lovo cells metastasis and growth. Evid Based Complement Alternat Med (2020) 2020:4517483. doi: 10.1155/2020/4517483
92. Shi H, Tian S, Tian H. Network pharmacology interpretation of fuzheng-jiedu decoction against colorectal cancer. Evid Based Complement Alternat Med (2021) 2021:4652492. doi: 10.1155/2021/4652492
93. Xu T, Wang Q, Liu M. A network pharmacology approach to explore the potential mechanisms of huangqin-baishao herb pair in treatment of cancer. Med Sci Monit (2020) 26:e923199. doi: 10.12659/MSM.923199
94. Dong B, Yang Z, Ju Q, Zhu S, Wang Y, Zou H, et al. Anticancer effects of fufang yiliu yin formula on colorectal cancer through modulation of the pi3k/akt pathway and bcl-2 family proteins. Front Cell Dev Biol (2020) 8:704. doi: 10.3389/fcell.2020.00704
95. Li Q, Wei L, Lin S, Chen Y, Lin J, Peng J. Synergistic effect of kaempferol and 5fluorouracil on the growth of colorectal cancer cells by regulating the pi3k/akt signaling pathway. Mol Med Rep (2019) 20(1):728–34. doi: 10.3892/mmr.2019.10296
96. Tan H, Li X, Yang WH, Flavone KYA. Wogonin from scutellaria baicalensis inhibits the proliferation of human colorectal cancer cells by inducing of autophagy, apoptosis and g2/m cell cycle arrest via modulating the Pi3k/Akt and Stat3 signalling pathways. J BUON (2019) 24(3):1143–9.
97. Kim SJ, Kim HJ, Kim HR, Lee SH, Cho SD, Choi CS, et al. Antitumor actions of baicalein and wogonin in ht-29 human colorectal cancer cells. Mol Med Rep (2012) 6(6):1443–9. doi: 10.3892/mmr.2012.1085
98. Wang H, Zhao L, Zhu LT, Wang Y, Pan D, Yao J, et al. Wogonin reverses hypoxia resistance of human colon cancer hct116 cells via downregulation of hif-1alpha and glycolysis, by inhibiting pi3k/akt signaling pathway. Mol Carcinog (2014) 53 Suppl 1:E107–18. doi: 10.1002/mc.22052
99. Belli V, Sforza V, Cardone C, Martinelli E, Barra G, Matrone N, et al. Regorafenib in combination with silybin as a novel potential strategy for the treatment of metastatic colorectal cancer. Oncotarget (2017) 8(40):68305–16. doi: 10.18632/oncotarget.20054
100. Ellington AA, Berhow MA, Singletary KW. Inhibition of akt signaling and enhanced erk1/2 activity are involved in induction of macroautophagy by triterpenoid b-group soyasaponins in colon cancer cells. Carcinogenesis (2006) 27(2):298–306. doi: 10.1093/carcin/bgi214
101. Ellington AA, Berhow M, Singletary KW. Induction of macroautophagy in human colon cancer cells by soybean b-group triterpenoid saponins. Carcinogenesis (2005) 26(1):159–67. doi: 10.1093/carcin/bgh297
102. Liu F, Lv RB, Liu Y, Hao Q, Liu SJ, Zheng YY, et al. Salinomycin and sulforaphane exerted synergistic antiproliferative and proapoptotic effects on colorectal cancer cells by inhibiting the pi3k/akt signaling pathway in vitro and in vivo. Onco Targets Ther (2020) 13:4957–69. doi: 10.2147/OTT.S246706
103. Sarkhosh-Inanlou R, Imani M, Sam MR. The response of pik3ca/ kras-mutant colorectal cancer stem-like cells to rgd-peptide frac produced by the strawberry anemone: a promising water-soluble peptide-based inhibitor of metastasis-driver gene cxcr4, stem cell regulatory genes and self-renewal. BioMed Pharmacother (2020) 132:110807. doi: 10.1016/j.biopha.2020.110807
104. Huang S, Zhang Z, Li W, Kong F, Yi P, Huang J, et al. Network pharmacology-based prediction and verification of the active ingredients and potential targets of zuojinwan for treating colorectal cancer. Drug Des Devel Ther (2020) 14:2725–40. doi: 10.2147/DDDT.S250991
105. Johnson SM, Gulhati P, Arrieta I, Wang X, Uchida T, Gao T, et al. Curcumin inhibits proliferation of colorectal carcinoma by modulating Akt/mTOR signaling. Anticancer Res (2009) 29(8):3185–90.
106. Matsumura A, Kubota T, Taiyoh H, Fujiwara H, Okamoto K, Ichikawa D, et al. Hgf regulates vegf expression via the c-met receptor downstream pathways, pi3k/akt, mapk and stat3, in ct26 murine cells. Int J Oncol (2013) 42(2):535–42. doi: 10.3892/ijo.2012.1728
107. Zhang Y, Wang S, Qian W, Ji D, Wang Q, Zhang Z, et al. Uc.338 targets p21 and cyclin d1 via pi3k/akt pathway activation to promote cell proliferation in colorectal cancer. Oncol Rep (2018) 40(2):1119–28. doi: 10.3892/or.2018.6480
108. Van Dross R, Xue Y, Knudson A, Pelling JC. The chemopreventive bioflavonoid apigenin modulates signal transduction pathways in keratinocyte and colon carcinoma cell lines. J Nutr (2003) 133(11 Suppl 1):3800S–4S. doi: 10.1093/jn/133.11.3800S
109. Zhang X, Zhang W, Chen F, Lu Z. Combined effect of chrysin and apigenin on inhibiting the development and progression of colorectal cancer by suppressing the activity of p38-mapk/akt pathway. IUBMB Life (2021) 73(5):774–83. doi: 10.1002/iub.2456
110. Wang X, Li L, Zhang G. A proteomic study on the protective effect of kaempferol pretreatment against deoxynivalenol-induced intestinal barrier dysfunction in a Caco-2 cell model. Food Funct (2020) 11(8):7266–79. doi: 10.1039/d0fo01579b
111. Choi JB, Kim JH, Lee H, Pak JN, Shim BS, Kim SH. Reactive oxygen species and p53 mediated activation of p38 and caspases is critically involved in kaempferol induced apoptosis in colorectal cancer cells. J Agric Food Chem (2018) 66(38):9960–7. doi: 10.1021/acs.jafc.8b02656
112. Shin DY, Lee WS, Lu JN, Kang MH, Ryu CH, Kim GY, et al. Induction of apoptosis in human colon cancer hct-116 cells by anthocyanins through suppression of akt and activation of p38-mapk. Int J Oncol (2009) 35(6):1499–504. doi: 10.3892/ijo_00000469
113. Nafees S, Mehdi SH, Zafaryab M, Zeya B, Sarwar T, Rizvi MA. Synergistic interaction of rutin and silibinin on human colon cancer cell line. Arch Med Res (2018) 49(4):226–34. doi: 10.1016/j.arcmed.2018.09.008
114. Patel S, Waghela B, Shah K, Vaidya F, Mirza S, Patel S, et al. Silibinin, a natural blend in polytherapy formulation for targeting cd44v6 expressing colon cancer stem cells. Sci Rep (2018) 8(1):16985. doi: 10.1038/s41598-018-35069-0
115. Yuan SX, Wang DX, Wu QX, Ren CM, Li Y, Chen QZ, et al. Bmp9/P38 mapk is essential for the antiproliferative effect of resveratrol on human colon cancer. Oncol Rep (2016) 35(2):939–47. doi: 10.3892/or.2015.4407
116. Mohapatra P, Preet R, Choudhuri M, Choudhuri T, Kundu CN. 5-fluorouracil increases the chemopreventive potentials of resveratrol through dna damage and mapk signaling pathway in human colorectal cancer cells. Oncol Res (2011) 19(7):311–21. doi: 10.3727/096504011x13079697132844
117. Cerezo-Guisado MI, Zur R, Lorenzo MJ, Risco A, Martin-Serrano MA, Alvarez-Barrientos A, et al. Implication of akt, erk1/2 and alternative p38mapk signalling pathways in human colon cancer cell apoptosis induced by green tea egcg. Food Chem Toxicol (2015) 84:125–32. doi: 10.1016/j.fct.2015.08.017
118. Adachi S, Shimizu M, Shirakami Y, Yamauchi J, Natsume H, Matsushima-Nishiwaki R, et al. (-)-Epigallocatechin gallate downregulates egf receptor via phosphorylation at ser1046/1047 by p38 mapk in colon cancer cells. Carcinogenesis (2009) 30(9):1544–52. doi: 10.1093/carcin/bgp166
119. Inaba H, Nagaoka Y, Kushima Y, Kumagai A, Matsumoto Y, Sakaguchi M, et al. Comparative examination of anti-proliferative activities of (-)-epigallocatechin gallate and (–)-epigallocatechin against hct116 colorectal carcinoma cells. Biol Pharm Bull (2008) 31(1):79–84. doi: 10.1248/bpb.31.79
120. Chen C, Shen G, Hebbar V, Hu R, Owuor ED, Kong AN. Epigallocatechin-3-gallate-induced stress signals in ht-29 human colon adenocarcinoma cells. Carcinogenesis (2003) 24(8):1369–78. doi: 10.1093/carcin/bgg091
121. Li S, Fu H, Wang Y, Wang L, Jia B, Bian Y. Curcumin inhibits ct26 cells metastasis by decreasing heparanase expression. J Leukoc Biol (2020) 108(6):1727–33. doi: 10.1002/JLB.1MA0620-357R
122. Fenton JI, McCaskey SJ. Curcumin and docosahexaenoic acid block insulin-induced colon carcinoma cell proliferation. Prostaglandins Leukot Essent Fatty Acids (2013) 88(3):219–26. doi: 10.1016/j.plefa.2012.11.010
123. Collett GP, Campbell FC. Curcumin induces c-jun n-terminal kinase-dependent apoptosis in hct116 human colon cancer cells. Carcinogenesis (2004) 25(11):2183–9. doi: 10.1093/carcin/bgh233
124. Brouland JP, Valleur P, Papp B. [Expression of serca pumps during cell differentiation and tumorigenesis: application to colonic carcinogenesis]. Ann Pathol (2006) 26(3):159–72. doi: 10.1016/s0242-6498(06)70701-5
125. Fan L, Li A, Li W, Cai P, Yang B, Zhang M, et al. Novel role of sarco/endoplasmic reticulum calcium atpase 2 in development of colorectal cancer and its regulation by f36, a curcumin analog. BioMed Pharmacother (2014) 68(8):1141–8. doi: 10.1016/j.biopha.2014.10.014
126. Radhakrishnan EK, Bava SV, Narayanan SS, Nath LR, Thulasidasan AK, Soniya EV, et al. [6]-gingerol induces caspase-dependent apoptosis and prevents pma-induced proliferation in colon cancer cells by inhibiting mapk/ap-1 signaling. PLoS One (2014) 9(8):e104401. doi: 10.1371/journal.pone.0104401
127. Lee HN, Jang HY, Kim HJ, Shin SA, Choo GS, Park YS. Antitumor and apoptosis-inducing effects of α-mangostin extracted from the pericarp of the mangosteen fruit (Garcinia mangostana L.) in YD-15 tongue mucoepidermoid carcinoma cells. Int J Mol Med (2016) 37(4):939–48. doi: 10.3892/ijmm.2016.2517
128. Yang PY, Chen MF, Tsai CH, Hu DN, Chang FR, Wu YC. Involvement of caspase and mapk activities in norcantharidin-induced colorectal cancer cell apoptosis. Toxicol In Vitro (2010) 24(3):766–75. doi: 10.1016/j.tiv.2009.12.025
129. Chen YJ, Tsai YM, Kuo CD, Ku KL, Shie HS, Liao HF. Norcantharidin is a small-molecule synthetic compound with anti-angiogenesis effect. Life Sci (2009) 85(17-18):642–51. doi: 10.1016/j.lfs.2009.09.003
130. Zhang L, Ji Q, Liu X, Chen X, Chen Z, Qiu Y, et al. Norcantharidin inhibits tumor angiogenesis via blocking vegfr2/mek/erk signaling pathways. Cancer Sci (2013) 104(5):604–10. doi: 10.1111/cas.12120
131. Ren Y, Lv C, Zhang J, Zhang B, Yue B, Luo X, et al. Alantolactone exhibits antiproliferative and apoptosis-promoting properties in colon cancer model via activation of the mapk-jnk/c-jun signaling pathway. Mol Cell Biochem (2021) 476(12):4387–403. doi: 10.1007/s11010-021-04247-6
132. Cao P, Xia Y, He W, Zhang T, Hong L, Zheng P, et al. Enhancement of oxaliplatin-induced colon cancer cell apoptosis by alantolactone, a natural product inducer of ros. Int J Biol Sci (2019) 15(8):1676–84. doi: 10.7150/ijbs.35265
133. Mi C, Ma J, Wang KS, Zuo HX, Wang Z, Li MY, et al. Imperatorin suppresses proliferation and angiogenesis of human colon cancer cell by targeting hif-1alpha via the mtor/p70s6k/4e-bp1 and mapk pathways. J Ethnopharmacol (2017) 203:27–38. doi: 10.1016/j.jep.2017.03.033
134. Pearson HE, Iida M, Orbuch RA, McDaniel NK, Nickel KP, Kimple RJ, et al. Overcoming resistance to cetuximab with honokiol, a small-molecule polyphenol. Mol Cancer Ther (2018) 17(1):204–14. doi: 10.1158/1535-7163.MCT-17-0384
135. Li L, Wei L, Shen A, Chu J, Lin J, Peng J. Oleanolic acid modulates multiple intracellular targets to inhibit colorectal cancer growth. Int J Oncol (2015) 47(6):2247–54. doi: 10.3892/ijo.2015.3198
136. Hsu HH, Kuo WW, Day CH, Shibu MA, Li SY, Chang SH, et al. Taiwanin e inhibits cell migration in human lovo colon cancer cells by suppressing mmp-2/9 expression via p38 mapk pathway. Environ Toxicol (2017) 32(8):2021–31. doi: 10.1002/tox.22379
137. Liang Z, Yi Y, Guo Y, Wang R, Hu Q, Xiong X. Chemical characterization and antitumor activities of polysaccharide extracted from ganoderma lucidum. Int J Mol Sci (2014) 15(5):9103–16. doi: 10.3390/ijms15059103
138. Pan H, Wang Y, Na K, Wang Y, Wang L, Li Z, et al. Autophagic flux disruption contributes to ganoderma lucidum polysaccharide-induced apoptosis in human colorectal cancer cells via mapk/erk activation. Cell Death Dis (2019) 10(6):456. doi: 10.1038/s41419-019-1653-7
139. Yang HJ, Li YN, Yan C, Yang J, Zeng YR, Yi P, et al. Discovery and synthesis of rocaglaol derivatives inducing apoptosis in hct116 cells via suppression of mapk signaling pathway. Fitoterapia (2021) 151:104876. doi: 10.1016/j.fitote.2021.104876
140. Kim GT, Lee SH, Kim YM. Torilis japonica extract-generated intracellular ros induces apoptosis by reducing the mitochondrial membrane potential via regulation of the ampk-p38 mapk signaling pathway in hct116 colon cancer. Int J Oncol (2016) 49(3):1088–98. doi: 10.3892/ijo.2016.3578
141. Lemieszek MK, Ribeiro M, Marques G, Nunes FM, Pozarowski P, Rzeski W. New insights into the molecular mechanism of boletus edulis ribonucleic acid fraction (be3) concerning antiproliferative activity on human colon cancer cells. Food Funct (2017) 8(5):1830–9. doi: 10.1039/c6fo01626j
142. De Luca A, Maiello MR, D’Alessio A, Pergameno M, Normanno N. The ras/raf/mek/erk and the pi3k/akt signalling pathways: role in cancer pathogenesis and implications for therapeutic approaches. Expert Opin Ther Targets (2012) 16 Suppl 2:S17–27. doi: 10.1517/14728222.2011.639361
143. Wagner EF, Nebreda AR. Signal integration by jnk and p38 mapk pathways in cancer development. Nat Rev Cancer (2009) 9(8):537–49. doi: 10.1038/nrc2694
144. Fang JY, Richardson BC. The mapk signalling pathways and colorectal cancer. Lancet Oncol (2005) 6(5):322–7. doi: 10.1016/S1470-2045(05)70168-6
145. Li G, Fang S, Shao X, Li Y, Tong Q, Kong B, et al. Curcumin reverses nnmt-induced 5-fluorouracil resistance Via increasing ros and cell cycle arrest in colorectal cancer cells. Biomolecules (2021) 11(9):1295–314. doi: 10.3390/biom11091295
146. Chou YT, Koh YC, Nagabhushanam K, Ho CT, Pan MH. A natural degradant of curcumin, feruloylacetone inhibits cell proliferation via inducing cell cycle arrest and a mitochondrial apoptotic pathway in Hct116 colon cancer cells. Molecules (2021) 26(16):4884–900. doi: 10.3390/molecules26164884
147. Luo KW, Xia J, Cheng BH, Gao HC, Fu LW, Luo XL. Tea polyphenol egcg inhibited colorectal-cancer-cell proliferation and migration via downregulation of stat3. Gastroenterol Rep (Oxf) (2021) 9(1):59–70. doi: 10.1093/gastro/goaa072
148. Jin G, Yang Y, Liu K, Zhao J, Chen X, Liu H, et al. Combination curcumin and (-)-epigallocatechin-3-gallate inhibits colorectal carcinoma microenvironment-induced angiogenesis by jak/stat3/il-8 pathway. Oncogenesis (2017) 6(10):e384. doi: 10.1038/oncsis.2017.84
149. Serra D, Rufino AT, Mendes AF, Almeida LM, Dinis TC. Resveratrol modulates cytokine-induced jak/stat activation more efficiently than 5-aminosalicylic acid: an in vitro approach. PLoS One (2014) 9(10):e109048. doi: 10.1371/journal.pone.0109048
150. Lu HF, Yang JS, Lin YT, Tan TW, Ip SW, Li YC, et al. Diallyl disulfide induced signal transducer and activator of transcription 1 expression in human colon cancer colo 205 cells using differential display rt-pcr. Cancer Genomics Proteomics (2007) 4(2):93–7.
151. Li X, Ni J, Tang Y, Wang X, Tang H, Li H, et al. Allicin inhibits mouse colorectal tumorigenesis through suppressing the activation of stat3 signaling pathway. Nat Prod Res (2019) 33(18):2722–5. doi: 10.1080/14786419.2018.1465425
152. Yun M, Lee D, Park MN, Kim EO, Sohn EJ, Kwon BM, et al. Cinnamaldehyde derivative (cb-pic) sensitizes chemo-resistant cancer cells to drug-induced apoptosis via suppression of mdr1 and its upstream stat3 and akt signalling. Cell Physiol Biochem (2015) 35(5):1821–30. doi: 10.1159/000373993
153. Liu LQ, Nie SP, Shen MY, Hu JL, Yu Q, Gong D, et al. Tea polysaccharides inhibit colitis-associated colorectal cancer via interleukin-6/stat3 pathway. J Agric Food Chem (2018) 66(17):4384–93. doi: 10.1021/acs.jafc.8b00710
154. Su YS, Kuo MZ, Kuo YT, Huang SW, Lee CJ, Su ZY, et al. Diterpenoid anthraquinones as chemopreventive agents altered microrna and transcriptome expressions in cancer cells. BioMed Pharmacother (2021) 136:111260. doi: 10.1016/j.biopha.2021.111260
155. Yar Saglam AS, Alp E, Elmazoglu Z, Menevse S. Treatment with cucurbitacin b alone and in combination with gefitinib induces cell cycle inhibition and apoptosis via egfr and jak/stat pathway in human colorectal cancer cell lines. Hum Exp Toxicol (2016) 35(5):526–43. doi: 10.1177/0960327115595686
156. Drago E, Bordonaro M, Lee S, Atamna W, Lazarova DL. Propolis augments apoptosis induced by butyrate via targeting cell survival pathways. PLoS One (2013) 8(9):e73151. doi: 10.1371/journal.pone.0073151
157. Li Y, de Haar C, Chen M, Deuring J, Gerrits MM, Smits R, et al. Disease-related expression of the il6/stat3/socs3 signalling pathway in ulcerative colitis and ulcerative colitis-related carcinogenesis. Gut (2010) 59(2):227–35. doi: 10.1136/gut.2009.184176
158. Shoaib A, Tabish M, Ali S, Arafah A, Wahab S, Almarshad FM, et al. Dietary phytochemicals in cancer signalling pathways: role of mirna targeting. Curr Med Chem (2021) 28(39):8036–67. doi: 10.2174/0929867328666210420101605
159. Xu JH, Zhang C, Tang B, Hao YX, Chen J, Liu T, et al. [Effect of Jak2/Stat3/vimentin signaling pathway on proliferation and migration of human colon cancer cells]. Zhonghua Wei Chang Wai Ke Za Zhi (2010) 13(4):282–5. doi: 10.3760/cma.j.issn.1671-0274.2010.04.015
160. Wei C, Yang C, Wang S, Shi D, Zhang C, Lin X, et al. Crosstalk between cancer cells and tumor associated macrophages is required for mesenchymal circulating tumor cell-mediated colorectal cancer metastasis. Mol Cancer (2019) 18(1):64. doi: 10.1186/s12943-019-0976-4
161. Guthrie GJ, Roxburgh CS, Horgan PG, McMillan DC. Does interleukin-6 link explain the link between tumour necrosis, local and systemic inflammatory responses and outcome in patients with colorectal cancer? Cancer Treat Rev (2013) 39(1):89–96. doi: 10.1016/j.ctrv.2012.07.003
162. Yin J, Wang L, Wang Y, Shen H, Wang X, Wu L. Curcumin reverses oxaliplatin resistance in human colorectal cancer via regulation of tgf-beta/smad2/3 signaling pathway. Onco Targets Ther (2019) 12:3893–903. doi: 10.2147/OTT.S199601
163. Buhrmann C, Kraehe P, Lueders C, Shayan P, Goel A, Shakibaei M. Curcumin suppresses crosstalk between colon cancer stem cells and stromal fibroblasts in the tumor microenvironment: potential role of emt. PLoS One (2014) 9(9):e107514. doi: 10.1371/journal.pone.0107514
164. Bounaama A, Djerdjouri B, Laroche-Clary A, Le Morvan V, Robert J. Short curcumin treatment modulates oxidative stress, arginase activity, aberrant crypt foci, and tgf-beta1 and hes-1 transcripts in 1,2-dimethylhydrazine-colon carcinogenesis in mice. Toxicology (2012) 302(2-3):308–17. doi: 10.1016/j.tox.2012.08.014
165. Ji Q, Liu X, Han Z, Zhou L, Sui H, Yan L, et al. Resveratrol suppresses epithelial-to-mesenchymal transition in colorectal cancer through tgf-beta1/smads signaling pathway mediated snail/e-cadherin expression. BMC Cancer (2015) 15:97. doi: 10.1186/s12885-015-1119-y
166. Schneider Y, Duranton B, Gosse F, Schleiffer R, Seiler N, Raul F. Resveratrol inhibits intestinal tumorigenesis and modulates host-defense-related gene expression in an animal model of human familial adenomatous polyposis. Nutr Cancer (2001) 39(1):102–7. doi: 10.1207/S15327914nc391_14
167. Lin TA, Lin WS, Chou YC, Nagabhushanam K, Ho CT, Pan MH. Oxyresveratrol inhibits human colon cancer cell migration through regulating epithelial-mesenchymal transition and microrna. Food Funct (2021) 12(20):9658–68. doi: 10.1039/d1fo01920a
168. Shimizu M, Deguchi A, Hara Y, Moriwaki H, Weinstein IB. Egcg inhibits activation of the insulin-like growth factor-1 receptor in human colon cancer cells. Biochem Biophys Res Commun (2005) 334(3):947–53. doi: 10.1016/j.bbrc.2005.06.182
169. Zhang L, Cai QY, Liu J, Peng J, Chen YQ, Sferra TJ, et al. Ursolic acid suppresses the invasive potential of colorectal cancer cells by regulating the tgf-beta1/zeb1/mir-200c signaling pathway. Oncol Lett (2019) 18(3):3274–82. doi: 10.3892/ol.2019.10604
170. Kaminski BM, Loitsch SM, Ochs MJ, Reuter KC, Steinhilber D, Stein J, et al. Isothiocyanate sulforaphane inhibits protooncogenic ornithine decarboxylase activity in colorectal cancer cells via induction of the tgf-beta/smad signaling pathway. Mol Nutr Food Res (2010) 54(10):1486–96. doi: 10.1002/mnfr.201000105
171. Yang B, Bai H, Sa Y, Zhu P, Liu P. Inhibiting emt, stemness and cell cycle involved in baicalin-induced growth inhibition and apoptosis in colorectal cancer cells. J Cancer (2020) 11(8):2303–17. doi: 10.7150/jca.37242
172. Wang X, Liu C, Wang J, Fan Y, Wang Z, Wang Y. Oxymatrine inhibits the migration of human colorectal carcinoma rko cells via inhibition of pai-1 and the tgf-beta1/smad signaling pathway. Oncol Rep (2017) 37(2):747–53. doi: 10.3892/or.2016.5292
173. Chen QZ, Li Y, Shao Y, Zeng YH, Ren WY, Liu RX, et al. Tgf-Beta1/Pten/Pi3k signaling plays a critical role in the anti-proliferation effect of tetrandrine in human colon cancer cells. Int J Oncol (2017) 50(3):1011–21. doi: 10.3892/ijo.2017.3875
174. Chen GP, Zhang Y, Xu ZY, Yu JF, Wei X. Curcumin combined with cis-platinum promote the apoptosis of human colorectal cancer ht29 cells and mechanism. Int J Clin Exp Pathol (2017) 10(12):11496–505.
175. Li Y, Wang Z, Jin J, Zhu SX, He GQ, Li SH, et al. Quercetin pretreatment enhances the radiosensitivity of colon cancer cells by targeting notch-1 pathway. Biochem Biophys Res Commun (2020) 523(4):947–53. doi: 10.1016/j.bbrc.2020.01.048
176. Dandawate P, Subramaniam D, Panovich P, Standing D, Krishnamachary B, Kaushik G, et al. Cucurbitacin b and i inhibits colon cancer growth by targeting the notch signaling pathway. Sci Rep (2020) 10(1):1290. doi: 10.1038/s41598-020-57940-9
177. Lan L, Appelman C, Smith AR, Yu J, Larsen S, Marquez RT, et al. Natural product (-)-gossypol inhibits colon cancer cell growth by targeting rna-binding protein musashi-1. Mol Oncol (2015) 9(7):1406–20. doi: 10.1016/j.molonc.2015.03.014
178. Lan L, Liu H, Smith AR, Appelman C, Yu J, Larsen S, et al. Natural product derivative gossypolone inhibits musashi family of rna-binding proteins. BMC Cancer (2018) 18(1):809. doi: 10.1186/s12885-018-4704-z
179. Zhou P, Wang C, Hu Z, Chen W, Qi W, Li A. Genistein induces apoptosis of colon cancer cells by reversal of epithelial-to-mesenchymal via a notch1/nf-kappab/slug/e-cadherin pathway. BMC Cancer (2017) 17(1):813. doi: 10.1186/s12885-017-3829-9
180. Yang Y, Nguyen TT, Pereira I, Hur JS, Kim H. Lichen secondary metabolite physciosporin decreases the stemness potential of colorectal cancer cells. Biomolecules (2019) 9(12):797–810. doi: 10.3390/biom9120797
181. Koduru S, Kumar R, Srinivasan S, Evers MB, Damodaran C. Notch-1 inhibition by withaferin-a: a therapeutic target against colon carcinogenesis. Mol Cancer Ther (2010) 9(1):202–10. doi: 10.1158/1535-7163.MCT-09-0771
182. Wu WK, Wang XJ, Cheng AS, Luo MX, Ng SS, To KF, et al. Dysregulation and crosstalk of cellular signaling pathways in colon carcinogenesis. Crit Rev Oncol Hematol (2013) 86(3):251–77. doi: 10.1016/j.critrevonc.2012.11.009
183. Chu D, Zhang Z, Zhou Y, Wang W, Li Y, Zhang H, et al. Notch1 and notch2 have opposite prognostic effects on patients with colorectal cancer. Ann Oncol (2011) 22(11):2440–7. doi: 10.1093/annonc/mdq776
184. Serafin V, Persano L, Moserle L, Esposito G, Ghisi M, Curtarello M, et al. Notch3 signalling promotes tumour growth in colorectal cancer. J Pathol (2011) 224(4):448–60. doi: 10.1002/path.2895
185. Jin HY, Zhang HY, Wang X, Xu J, Ding Y. Expression and clinical significance of notch signaling genes in colorectal cancer. Tumour Biol (2012) 33(3):817–24. doi: 10.1007/s13277-011-0301-3
186. Dai Y, Wilson G, Huang B, Peng M, Teng G, Zhang D, et al. Silencing of jagged1 inhibits cell growth and invasion in colorectal cancer. Cell Death Dis (2014) 5:e1170. doi: 10.1038/cddis.2014.137
187. Chen J, Zhang H, Chen Y, Qiao G, Jiang W, Ni P, et al. Mir-598 inhibits metastasis in colorectal cancer by suppressing jag1/notch2 pathway stimulating emt. Exp Cell Res (2017) 352(1):104–12. doi: 10.1016/j.yexcr.2017.01.022
188. Liu H, Yin Y, Hu Y, Feng Y, Bian Z, Yao S, et al. Mir-139-5p sensitizes colorectal cancer cells to 5-fluorouracil by targeting notch-1. Pathol Res Pract (2016) 212(7):643–9. doi: 10.1016/j.prp.2016.04.011
189. Xiong Y, Zhang YY, Wu YY, Wang XD, Wan LH, Li L, et al. Correlation of over-expressions of mir-21 and notch-1 in human colorectal cancer with clinical stages. Life Sci (2014) 106(1-2):19–24. doi: 10.1016/j.lfs.2014.04.017
190. Kong JH, Siebold C, Rohatgi R. Biochemical mechanisms of vertebrate hedgehog signaling. Development (2019) 146(10):dev166892–909. doi: 10.1242/dev.166892
191. Yang L, Xie G, Fan Q, Xie J. Activation of the hedgehog-signaling pathway in human cancer and the clinical implications. Oncogene (2010) 29(4):469–81. doi: 10.1038/onc.2009.392
192. Liang Y, Yang L, Xie J. The role of the hedgehog pathway in chemoresistance of gastrointestinal cancers. Cells (2021) 10(8):2030–40. doi: 10.3390/cells10082030
193. Qiu P, Liu J, Zhao L, Zhang P, Wang W, Shou D, et al. Inoscavin a, a pyrone compound isolated from a sanghuangporus vaninii extract, inhibits colon cancer cell growth and induces cell apoptosis via the hedgehog signaling pathway. Phytomedicine (2022) 96:153852. doi: 10.1016/j.phymed.2021.153852
194. Chen J, Qiu S, Kim JT, Cho JS, Moon JH, Zhou Y, et al. Garcinone c suppresses colon tumorigenesis through the gli1-dependent hedgehog signaling pathway. Phytomedicine (2020) 79:153334. doi: 10.1016/j.phymed.2020.153334
195. Wierzbicki PM, Rybarczyk A. The hippo pathway in colorectal cancer. Folia Histochem Cytobiol (2015) 53(2):105–19. doi: 10.5603/FHC.a2015.0015
196. Chai Y, Xiang K, Wu Y, Zhang T, Liu Y, Liu X, et al. Cucurbitacin b inhibits the hippo-yap signaling pathway and exerts anticancer activity in colorectal cancer cells. Med Sci Monit (2018) 24:9251–8. doi: 10.12659/MSM.911594
197. Xue M, Liang H, Ji X, Zhou Z, Liu Y, Sun T, et al. Effects of fucoidan on gut flora and tumor prevention in 1,2-dimethylhydrazine-induced colorectal carcinogenesis. J Nutr Biochem (2020) 82:108396. doi: 10.1016/j.jnutbio.2020.108396
198. Li X, Lin YY, Tan JY, Liu KL, Shen XL, Hu YJ, et al. An anticancer agent, inhibits yap via transcriptional and post-translational regulation. Pharm Biol (2021) 59(1):619–28. doi: 10.1080/13880209.2021.1923759
199. Yang L, Zheng L, Xie X, Luo J, Yu J, Zhang L, et al. Targeting pla2g16, a lipid metabolism gene, by ginsenoside compound k to suppress the malignant progression of colorectal cancer. J Adv Res (2022) 36:265–76. doi: 10.1016/j.jare.2021.06.009
200. Xavier CP, Lima CF, Preto A, Seruca R, Fernandes-Ferreira M, Pereira-Wilson C, et al. Luteolin, quercetin and ursolic acid are potent inhibitors of proliferation and inducers of apoptosis in both kras and braf mutated human colorectal cancer cells. Cancer Lett (2009) 281(2):162–70. doi: 10.1016/j.canlet.2009.02.041
201. Shan JZ, Xuan YY, Zheng S, Dong Q, Zhang SZ. Ursolic acid inhibits proliferation and induces apoptosis of Ht-29 colon cancer cells by inhibiting the Egfr/Mapk pathway. J Zhejiang Univ Sci B (2009) 10(9):668–74. doi: 10.1631/jzus.B0920149
202. Shan J, Xuan Y, Zhang Q, Zhu C, Liu Z, Zhang S. Ursolic acid synergistically enhances the therapeutic effects of oxaliplatin in colorectal cancer. Protein Cell (2016) 7(8):571–85. doi: 10.1007/s13238-016-0295-0
203. Howells LM, Iwuji COO, Irving GRB, Barber S, Walter H, Sidat Z, et al. Curcumin combined with folfox chemotherapy is safe and tolerable in patients with metastatic colorectal cancer in a randomized phase IIA trial. J Nutr (2019) 149(7):1133–9. doi: 10.1093/jn/nxz029
204. Greil R, Greil-Ressler S, Weiss L, Schonlieb C, Magnes T, Radl B, et al. A phase 1 dose-escalation study on the safety, tolerability and activity of liposomal curcumin (Lipocurc()) in patients with locally advanced or metastatic cancer. Cancer Chemother Pharmacol (2018) 82(4):695–706. doi: 10.1007/s00280-018-3654-0
205. Howells LM, Berry DP, Elliott PJ, Jacobson EW, Hoffmann E, Hegarty B, et al. Phase i randomized, double-blind pilot study of micronized resveratrol (Srt501) in patients with hepatic metastases–safety, pharmacokinetics, and pharmacodynamics. Cancer Prev Res (Phila) (2011) 4(9):1419–25. doi: 10.1158/1940-6207.CAPR-11-0148
206. Hoensch H, Groh B, Edler L, Kirch W. Prospective cohort comparison of flavonoid treatment in patients with resected colorectal cancer to prevent recurrence. World J Gastroenterol (2008) 14(14):2187–93. doi: 10.3748/wjg.14.2187
207. Sinicrope FA, Viggiano TR, Buttar NS, Song L, Schroeder KW, Kraichely RE, et al. Randomized phase ii trial of polyphenon e versus placebo in patients at high risk of recurrent colonic neoplasia. Cancer Prev Res (Phila) (2021) 14(5):573–80. doi: 10.1158/1940-6207.CAPR-20-0598
208. James MI, Iwuji C, Irving G, Karmokar A, Higgins JA, Griffin-Teal N, et al. Curcumin inhibits cancer stem cell phenotypes in ex vivo models of colorectal liver metastases, and is clinically safe and tolerable in combination with folfox chemotherapy. Cancer Lett (2015) 364(2):135–41. doi: 10.1016/j.canlet.2015.05.005
209. Lao CD, Ruffin MTt, Normolle D, Heath DD, Murray SI, Bailey JM, et al. Dose escalation of a curcuminoid formulation. BMC Complement Altern Med (2006) 6(10):1–4. doi: 10.1186/1472-6882-6-10
210. Cruz-Correa M, Hylind LM, Marrero JH, Zahurak ML, Murray-Stewart T, Casero RA Jr., et al. Efficacy and safety of curcumin in treatment of intestinal adenomas in patients with familial adenomatous polyposis. Gastroenterology (2018) 155(3):668–73. doi: 10.1053/j.gastro.2018.05.031
211. Panahi Y, Saberi-Karimian M, Valizadeh O, Behnam B, Saadat A, Jamialahmadi T, et al. Effects of curcuminoids on systemic inflammation and quality of life in patients with colorectal cancer undergoing chemotherapy: A randomized controlled trial. Adv Exp Med Biol (2021) 1328:1–9. doi: 10.1007/978-3-030-73234-9_1
212. Storka A, Vcelar B, Klickovic U, Gouya G, Weisshaar S, Aschauer S, et al. Safety, tolerability and pharmacokinetics of liposomal curcumin in healthy humans. Int J Clin Pharmacol Ther (2015) 53(1):54–65. doi: 10.5414/CP202076
213. Patel KR, Brown VA, Jones DJ, Britton RG, Hemingway D, Miller AS, et al. Clinical pharmacology of resveratrol and its metabolites in colorectal cancer patients. Cancer Res (2010) 70(19):7392–9. doi: 10.1158/0008-5472.CAN-10-2027
214. Cruz-Correa M, Shoskes DA, Sanchez P, Zhao R, Hylind LM, Wexner SD, et al. Combination treatment with curcumin and quercetin of adenomas in familial adenomatous polyposis. Clin Gastroenterol Hepatol (2006) 4(8):1035–8. doi: 10.1016/j.cgh.2006.03.020
215. Pintova S, Dharmupari S, Moshier E, Zubizarreta N, Ang C, Holcombe RF. Genistein combined with folfox or folfox-bevacizumab for the treatment of metastatic colorectal cancer: Phase I/Ii pilot study. Cancer Chemother Pharmacol (2019) 84(3):591–8. doi: 10.1007/s00280-019-03886-3
216. Adams KF, PD L, KM N, JT Y, Feld A, Myerson D, et al. Soy protein containing isoflavones does not decrease colorectal epithelial cell proliferation in a randomized controlled trial. Am J Clin Nutr (2005) 82(3):620–6. doi: 10.1093/ajcn.82.3.620
217. Miura T, Yuan L, Sun B, Fujii H, Yoshida M, Wakame K, et al. Isoflavone aglycon produced by culture of soybean extracts with basidiomycetes and its anti-angiogenic activity. Biosci Biotechnol Biochem (2002) 66(12):2626–31. doi: 10.1271/bbb.66.2626
218. Morad G, Helmink BA, Sharma P, Wargo JA. Hallmarks of response, resistance, and toxicity to immune checkpoint blockade. Cell (2021) 184(21):5309–37. doi: 10.1016/j.cell.2021.09.020
219. Lee J, Han Y, Wang W, Jo H, Kim H, Kim S, et al. Phytochemicals in cancer immune checkpoint inhibitor therapy. Biomolecules (2021) 11(8):1107–41. doi: 10.3390/biom11081107
Keywords: colorectal cancer, natural compounds, signaling pathway, flavonoids, polyphenol
Citation: Wang M, Liu X, Chen T, Cheng X, Xiao H, Meng X and Jiang Y (2022) Inhibition and potential treatment of colorectal cancer by natural compounds via various signaling pathways. Front. Oncol. 12:956793. doi: 10.3389/fonc.2022.956793
Received: 02 June 2022; Accepted: 15 July 2022;
Published: 08 September 2022.
Edited by:
Jiang-Jiang Qin, Chinese Academy of Sciences (CAS), ChinaReviewed by:
Zhigang Xiao, Hunan Provincial People’s Hospital, ChinaHong Zhang, ShengJing Hospital of China Medical University, China
Copyright © 2022 Wang, Liu, Chen, Cheng, Xiao, Meng and Jiang. This is an open-access article distributed under the terms of the Creative Commons Attribution License (CC BY). The use, distribution or reproduction in other forums is permitted, provided the original author(s) and the copyright owner(s) are credited and that the original publication in this journal is cited, in accordance with accepted academic practice. No use, distribution or reproduction is permitted which does not comply with these terms.
*Correspondence: Yang Jiang, amlhbmd5YW5nQGpsdS5lZHUuY24=
†These authors have contributed equally to this work