- 1School of Basic Medical Sciences, Qingdao Medical College, Qingdao University, Qingdao, China
- 2College of New Materials and Chemical Engineering, Beijing Key Laboratory of Enze Biomass Fine Chemicals, Beijing Institute of Petrochemical Technology, Beijing, China
- 3Institute for Translational Medicine, The Affiliated Hospital of Qingdao University, Qingdao Medical College, Qingdao University, Qingdao, China
Cancer is one of the most frequently diagnosed malignant diseases worldwide, posing a serious, long-term threat to patients’ health and life. Systemic chemotherapy remains the first-line therapeutic approach for recurrent or metastatic cancer patients after surgery, with the potential to effectively extend patient survival. However, the development of drug resistance seriously limits the clinical efficiency of chemotherapy and ultimately results in treatment failure and patient death. A large number of studies have shown that non-coding RNAs (ncRNAs), particularly microRNAs, long non-coding RNAs, and circular RNAs, are widely involved in the regulation of cancer drug resistance. Their dysregulation contributes to the development of cancer drug resistance by modulating the expression of specific target genes involved in cellular apoptosis, autophagy, drug efflux, epithelial-to-mesenchymal transition (EMT), and cancer stem cells (CSCs). Moreover, some ncRNAs also possess great potential as efficient, specific biomarkers in diagnosis and prognosis as well as therapeutic targets in cancer patients. In this review, we summarize the recent findings on the emerging role and underlying mechanisms of ncRNAs involved in cancer drug resistance and focus on their clinical applications as biomarkers and therapeutic targets in cancer treatment. This information will be of great benefit to early diagnosis and prognostic assessments of cancer as well as the development of ncRNA-based therapeutic strategies for cancer patients.
Introduction
Cancer is the second leading cause of death after cardiovascular disease globally, representing a serious threat to patients’ life and health (1, 2). Based on recent statistics from the International Agency for Research, approximately 19.3 million new cancer cases and more than 10.0 million deaths occurred in 2020 (3). Currently, surgical resection, radiation, endocrine therapy, targeted therapy, and systemic chemotherapy are the main methods of cancer treatment. Among them, systemic chemotherapy is the most effective therapeutic option for all stages of cancer, with the potential to improve patients’ prognosis in the short term (4–6). It has been reported that chemotherapy could extend the overall survival (OS) of patients with advanced cancer by 6.7 months compared to patients only treated with best supportive care (7). However, the emergency of drug resistance significantly limits the clinical application of chemotherapeutic agents, ultimately resulting in treatment failure and patient death. Drug resistance has become an immense obstacle in cancer treatment (8). The underlying mechanisms involved in drug resistance are considerably complex and have not been fully elucidated. Therefore, a better understanding of the mechanisms responsible for drug resistance will provide opportunities for the development of precise therapeutic strategies for cancer patients.
Non-coding RNAs (ncRNAs), such as microRNAs (miRNAs), long non-coding RNAs (lncRNAs), and circular RNAs (circRNAs), are a large group of transcripts that have no protein coding potential. They were recognized as by-products of transcription without biological function in the past long period of time (9). In recent years, an increasing amount of evidence has suggested that ncRNAs are crucial regulators in almost all cellular processes, such as transcription, apoptosis, proliferation, and differentiation (10, 11). They play crucial roles in the regulation of a variety of physiological and pathological processes. The dysregulation of ncRNAs has been shown to be closely associated with a variety of diseases, particularly cancer (12–14). For instance, the overexpression of miRNA-200a-3p was found to significantly facilitate cell proliferation, migration, and invasion as well as induce apoptosis in gastric cancer (GC) by directly targeting DLC-1 (15). LncRNA ITGB8-AS1 was found to promote cell proliferation, colony formation, and tumor growth in colorectal cancer (CRC) by upregulating ITGA3 and ITGB3 via sponging miR-33b-5p and let-7c-5p/let-7d-5p (16). Furthermore, circRNA C190 overexpression facilitated the proliferation, and migration of non-small cell lung carcinoma (NSCLC) cell lines by targeting CDK1 and CDK6 via sequestrating miR-142-5p (17). Notably, ncRNA dysregulation contributes to the development of cancer drug resistance via various mechanisms, such as the inhibition of apoptosis, enhancement of epithelial-to-mesenchymal transition (EMT), and induction of autophagy (18–20). In addition, the differential expression patterns of ncRNAs endow them with great potential as biomarkers and therapeutic targets for cancer patients.
In this review, we summarize the recent findings on the regulatory mechanisms of ncRNAs in cancer drug resistance and highlight their clinical applications as promising biomarkers and therapeutic targets for cancer patients. A better understanding of the underlying mechanisms of ncRNAs in drug resistance may offer an opportunity to develop ncRNA-based therapeutic strategies for cancer patients against drug resistance.
Overview of ncRNAs
Classification of ncRNAs
It has been reported that ncRNAs make up about 98% of the human genome (21). With the continuous development of high-throughput sequencing technologies, an increasing number of ncRNAs are being identified in eukaryotic cells. According to distinguished classification standards, ncRNAs can be divided into a variety of categories. For instance, ncRNAs are classified into housekeeping ncRNAs (e.g., rRNAs and tRNAs) and regulatory ncRNAs (e.g., miRNAs, circRNAs and lncRNAs) based on their cellular functions. According to their transcript size, ncRNAs are divided into lncRNAs (> 200 nucleotides) and small ncRNAs (< 200 nucleotides), including miRNAs, small interfering RNAs (siRNAs), and piwi-interacting RNAs (piRNAs) (22, 23). Besides, lncRNAs are sorted into two categories, linear lncRNAs and circular lncRNAs based on their structure (24). Moreover, according to the role of lncRNAs in gene expression regulation, they are classified as cis-lncRNAs or trans-lncRNAs (25). In addition, ncRNAs can also be divided into distinct categories based on their subcellular localization (e.g., small nuclear RNAs and cytoplasm-located siRNAs) and genomic origins (including sense or antisense ncRNAs, bidirectional ncRNAs, intronic ncRNAs, and intergenic ncRNAs) (26). Collectively, scientific and systematic classification will be of great benefit in better understanding the characteristics of ncRNAs.
Biogenesis of ncRNAs
The mechanisms of ncRNA biogenesis are extremely complicated, and individual ncRNA categories possess unique characteristics (Figure 1). For instance, both miRNAs and lncRNAs are transcribed by RNA polymerase II (Pol II) from genomic loci. Primary miRNAs (pri-miRNAs) are subsequently catalyzed by a microprocessor complex consisting of DiGeorge syndrome critical region 8 (DGCR8) and Drosha to generate precursor miRNAs (pre-miRNAs). Pre-miRNAs are translocated from the nucleus to the cytoplasm, and then processed into double-stranded miRNAs by the Dicer/TRBP/PACT complex. Finally, the double-stranded miRNAs are processed into mature miRNAs by a series of regulators, including helicase and the RNA-induced silencing complex (RISC) (27). Different from miRNAs, lncRNAs contain 5’ caps and 3’ poly(A) tails. Most lncRNAs undergo a canonical mechanism similar to the biogenesis of mRNAs, by which they are often capped by 7-methyl guanosine at the 5’ end of Pol II transcripts, polyadenylated at their 3′ ends, and spliced similarly to mRNAs (28). CircRNAs are a novel type of ncRNAs characterized by the formation of covalently closed-loop structures without 5’ caps and 3’ tails. CircRNAs are mainly produced from precursor mRNAs via a unique mechanism called back-splicing reaction, in which a downstream splice donor site binds to an upstream splice acceptor site to form a single-strand, covalently closed-loop structure (29).
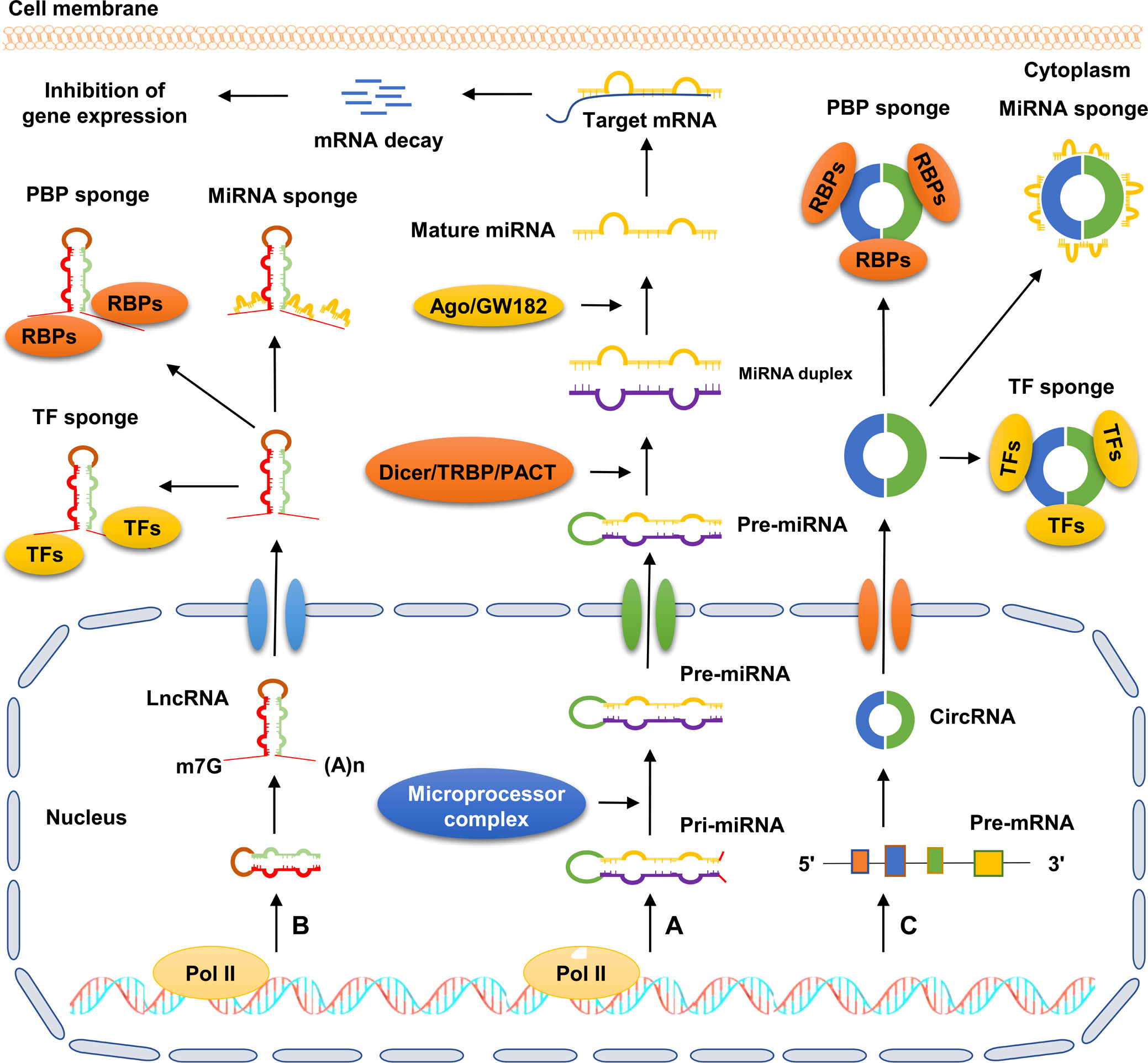
Figure 1 Schematic diagram of ncRNA biogenesis and action patterns. (A) Pri-miRNA is transcribed by RNA polymerasel II from genomic loci and further processed into pre-miRNA by microprocessor complex. Subsequently, pre-miRNA is exported to the cytoplasm and further processed into double-stranded miRNA via the Dicer/TRBP/PACT complex. Next, with the help of Ago/GW182, the double-stranded miRNA is processed into mature miRNA, which directly binds to the 3’-UTR of target mRNA, and then facilitates its degradation. (B) LncRNA transcribed by RNA polymerase II is exported to the cytoplasm. Subsequently, lncRNA exerts its biological role by acting as sponges of miRNAs, RBPs, and TFs. (C) CircRNA is mainly derived from precursor mRNAs via back-splicing reaction, by which the single strand of circRNA forms a covalently closed-loop structure. CircRNA plays crucial roles in cellular processes by serving as sponges of miRNAs, RBPs, and TFs.
The biogenesis of ncRNAs is widely regulated by various factors, such as trans-acting factors, RNA binding proteins (RBPs), and epigenetic modifications. For instance, the overexpression of poly(A)-binding protein nuclear 1 (PABPN1) was found to facilitate the turnover of non-coding transcripts via a polyadenylation-dependent mechanism, indicating its negative role in modulating the processing of certain ncRNAs (30). Alternative splicing factor1/pre-mRNA splicing factor SF2 (ASF/SF2) is a classical RBP encoded by the SFRS1 gene. Wu et al. showed that SF2/ASF overexpression facilitated the maturation process of a series of miRNAs, including miR-7, miR-29b, miR-221, and miR-222. Consistent with this, the knockdown of SF2/ASF resulted in a decreased level of mature miR-7 (31). N6-methyladenosine (m6A) is a well-studied RNA modification that plays crucial roles in distinct processes modulating RNA metabolism, such as the splicing, stability, and translation of mRNA (32). Timoteo et al. revealed that specific m6As promoted circRNA back-splicing reaction in a METTL3- and YTHDC1-dependent manner, whereas the mutation of the m6A sites significantly decreased the circRNA levels, which was paralleled by a strong increase in the precursor RNA (33). Although some progress has been made in recent years, ncRNA biogenesis and its regulatory mechanisms are still not fully understood. Continuous in-depth studies will be beneficial not only in differentiating ncRNAs from protein-coding RNAs but also in deciphering their functional significance.
Patterns of ncRNA action
A large amount of evidence suggests that ncRNAs are involved in almost all physiological and pathological processes, including tissue development, cancer progression, and drug resistance. They play crucial roles in these processes via distinct molecular mechanisms, such as regulating the expression of specific target genes, altering the function and activity of proteins, and targeting related signaling pathways (34–36). All these mechanisms are mainly based on the interaction of ncRNAs with DNA, RNA, and proteins (Figure 1). For instance, miRNAs are 19–25 nucleotides in length and play crucial roles in pivotal cellular processes by regulating specific gene expression at the post-transcriptional level (37, 38). They inhibit the expression of specific genes by directly binding to 3′ untranslated regions (UTRs) of their target mRNAs. One single miRNA can simultaneously control the expression of multiple target genes involved in distinct cellular processes (e.g., invasion, metastasis, and cell cycle), while one gene can also be regulated by several miRNAs (39). LncRNAs and circRNAs have been shown to exert their biological functions by acting as sponges or molecular sinks for miRNAs, RBPs, and transcription factors to specifically modulate their target gene expression. These ncRNAs are also called intracellular competitive endogenous RNAs (ceRNAs) (40, 41). For instance, lncRNA SLC25A25-AS1 was found to promote proliferation, migration, and invasion, and induced apoptosis in NSCLC A549 and H460 cells by upregulating integrin α2 via sponging miR-195-5p (42). Due to their central role in physiological and pathological processes, the dysregulation of ncRNAs is closely associated with the occurrence and development of many diseases including cancer. In fact, the aberrant expression of ncRNAs has been observed in cancer tissues and cell lines. They are involved in the regulation of cancer progression by serving as oncogenes or tumor suppressors (10). In addition, ncRNAs are also crucial regulators in the development of cancer drug resistance (10). In-depth investigations of the underlying mechanism of ncRNAs in cancer drug resistance could contribute to the precise treatment of cancer patients, particularly those with poor response to chemotherapy.
Mechanisms of ncRNAs in mediating cancer drug resistance
Chemotherapy remains the most effective first-line therapeutic approach for all stages of cancer and can effectively improve the clinical outcomes of patients in the short term. However, its long-term role in extending the OS of cancer patients is extremely restricted due to the emergence of drug resistance (43). Drug resistance is classified into single drug resistance and multidrug resistance (MDR). Of these, MDR is the main cause of mortality for most patients (44). Emerging evidence has shown that ncRNAs are closely associated with cancer drug resistance (Table 1). Their dysregulation contributes to the development of cancer drug resistance via distinct mechanisms, including inhibition of apoptosis, activation of protective autophagy, enhancement of drug efflux, induction of EMT, and enhancement of cancer stem cells (CSCs) stemness (Figure 2). However, the exact mechanisms are still not fully clarified. In this section, the involvement of ncRNAs in cancer drug resistance is outlined.
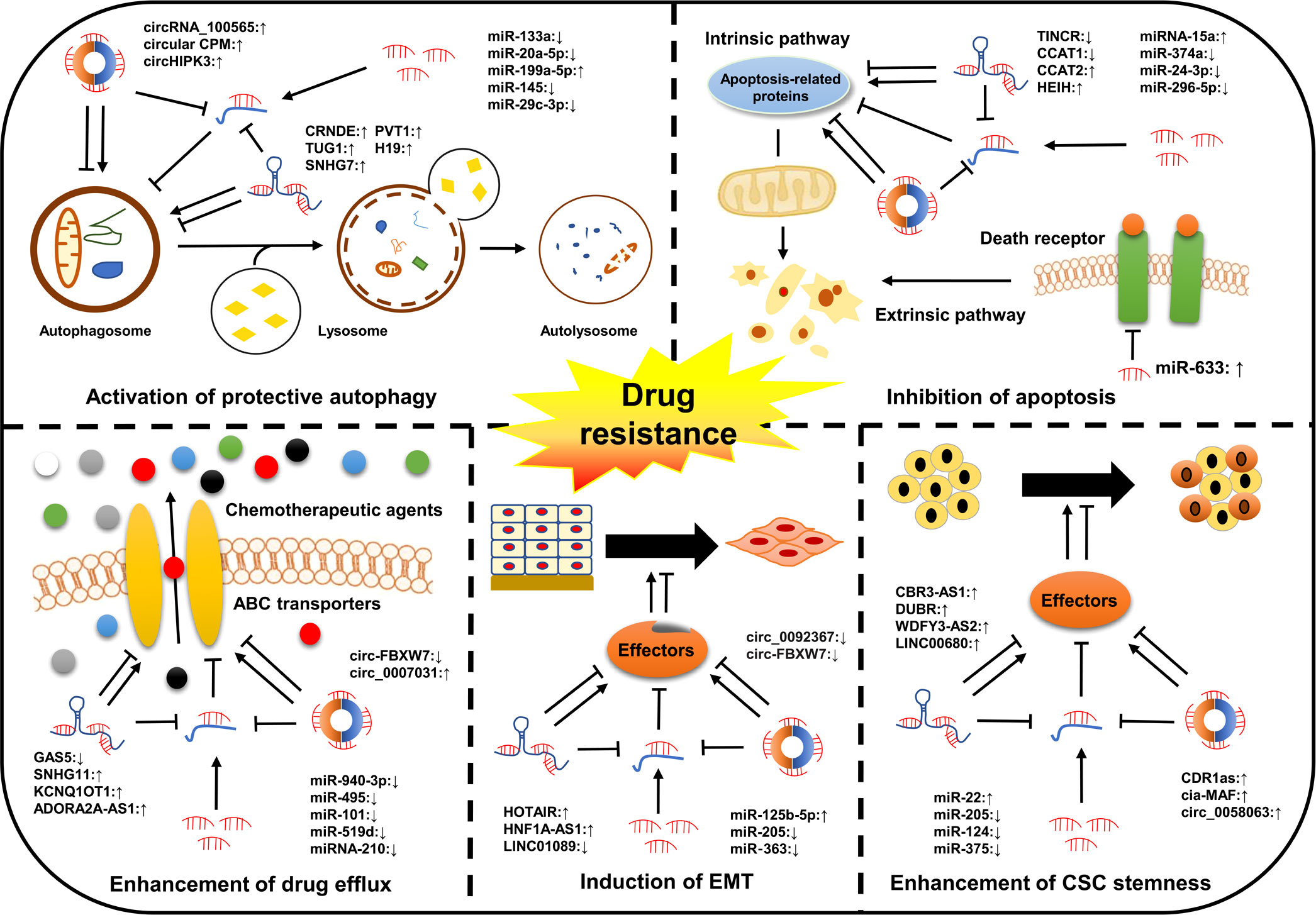
Figure 2 Classical mechanisms of ncRNAs in cancer drug resistance. The dysregulation of ncRNAs contributes to the development of cancer drug resistance by modulating multiple cellular processes of cancer cells, such as drug efflux, cell apoptosis, autophagy, and EMT as well as the acquisition of CSC characteristics.
MiRNAs and cancer drug resistance
MiRNAs affect drug-induced apoptosis by targeting apoptosis-related proteins or drug-resistance pathways
The inhibition of drug-induced apoptosis is one of the main mechanisms contributing to cancer drug resistance. Apoptosis can be divided into two categories: the extrinsic pathways mediated by death receptors and intrinsic (mitochondrial) pathways associated with apoptosis-related proteins such as B-cell lymphoma-2 (Bcl-2) (135). MiRNAs have been shown to influence cancer drug resistance by manipulating apoptosis-related proteins (27, 136). For instance, in our previous work, miR-633 was found to be significantly upregulated in GC tissues and cells, and its upregulation in GC samples was closely associated with the downregulation of Fas-associated protein with death domain (FADD), an adaptor involved in the extrinsic pathway of apoptosis. Mechanistic analysis revealed that miR-633 inhibited doxorubicin (DOX)/cisplatin (CDDP)-induced apoptosis in SGC-7901 and AGS cells by downregulating FADD via directly targeting its 3′-UTR (137). In another study, Yang et al. found that miR‐92a-3p was upregulated in both cervical cancer (CC) tissues and CDDP-resistant CC cell lines HeLa and SiHa. The overexpression of miR‐92a-3p inhibited the CDDP-induced apoptosis of HeLa and SiHa cells by targeting the expression of Krüppel-like factor 4 (KLF4), leading to the enhancement of CDDP resistance in CC. Consistent with this, miR‐92a-3p knockdown increased the sensitivity of HeLa and SiHa cells to CDDP (138).
The Bcl-2 family consisting of anti-apoptotic proteins (e.g., Bcl-2, Mcl-1, and Bcl-xl) and pro-apoptotic proteins (e.g., Bax, Bim, and Bak) plays crucial roles in the mitochondrial apoptotic pathway (139). It has been reported that the ratio between anti-apoptotic proteins and BH3-only proteins (a subtype of pro-apoptotic proteins) can alter the outer mitochondrial membrane permeability by regulating the activation of pore-forming proteins, thereby inducing apoptosis of the cancer cells (140). Zhong et al. showed that miR-625-3p overexpression significantly inhibited the CDDP-induced apoptosis of high-grade serous ovarian cancer (OC) cells OVCAR3 and OVCAR4 by targeting Bcl-2 and Bax expression, resulting in the inhibition of CDDP sensitivity in these cells (141). Ashofteh et al. revealed that miRNA-15a promoted cellular apoptosis by downregulating the mRNA levels of Mcl-1 and Bcl-2 in chronic lymphocytic leukemia (CLL), thereby enhancing the sensitivity of CLL-CII leukemia cells to fludarabine (142). In addition, Sun et al. demonstrated that miR‐374a was downregulated in A2780 cells by propofol. The overexpression miR‐374a suppressed the apoptosis of A2780 cells by decreasing the expression of Bim, p27, and FOXO1, leading to the enhancement of CDDP resistance in OC (143).
The Wnt/β-catenin signaling pathway is involved in the modulation of various cellular processes of cancer cells, such as apoptosis, proliferation, and metastasis. The dysregulation of this pathway has been shown to contribute to the development of cancer drug resistance by influencing the apoptotic pathways (144). For instance, Han et al. found that miR-199b-3p knockdown enhanced the sensitivity of cetuximab-resistant OC cells SW480 and HCT116 to cetuximab by promoting cell apoptosis. Mechanistically, silencing miR-199b-3p could enhance cetuximab-induced apoptosis in cetuximab-resistant SW480 and HCT116 cells by activating the Wnt/β-catenin signaling pathway via downregulating CRIM1 (145). Liu et al. showed that miR-217 was significantly reduced in CDDP-resistant OC COC1 cells compared with in CDDP-sensitive COC1 cells. The overexpression of miR-217 in COC1 cells facilitated CDDP-induced apoptosis and enhanced CDDP sensitivity by inhibiting the activation of the Wnt/β-catenin signaling pathway (146). In addition, multiple miRNAs, such as miR-323a-3p, miR-6727-5p, and miRNA-223-3p, have also been found to contribute to the development of cancer drug resistance by regulating apoptotic pathways via targeting other drug resistance-related signaling pathways, including the phosphatidylinositol 3-kinase (PI3K)/AKT, mitogen-activated protein kinase (MAPK), and nuclear factor kappa B (NF-κB) signaling pathways (147–149). Collectively, these findings indicate that targeting the apoptotic pathways is a common regulation mechanism for miRNAs in cancer drug resistance. Further investigating the mechanisms of miRNAs in drug-induced apoptosis may provide new insights on therapeutic strategies against cancer drug resistance.
MiRNAs and drug efflux in cancer drug resistance
Excessive drug efflux is considered a critical mechanism contributing to cancer drug resistance, in which the efficiency of anticancer drugs is significantly limited due to the reduction in drug concentration in cancer cells (150). It has been reported that excessive drug efflux is a result of the upregulation of drug efflux pumps, including ATP-binding cassette (ABC) transporters. Several members of the ABC family, such as ABCB1, ABCC2, and ABCG2, have been shown to contribute to the development of MDR in a variety of cancers (151). Increasing evidence suggest that miRNAs participate in the modulation of drug efflux in cancer cells by altering the expression ABC transporters (152). For instance, Zou et al. showed that the overexpression of miR-495 significantly reduced the drug efflux in MDR OC cell line A2780DX5 and GC cell line SGC7901R by directly targeting ABCB1, thereby enhancing the sensitivity of cancer cells to DOX and paclitaxel (PTX) (153). ABCB1 is also a target of miR-101 in GC. The overexpression of miR-101 in drug-resistant SGC7901 cells significantly decreased ABCB1 expression at the mRNA and protein levels (154). Tian et al. found that miR-940-3p negatively modulated ABCC2 expression in CDDP-resistant OVCAR3 and SKOV3 cells by directly binding to its 3’-UTR region, leading to the enhancement of CDDP sensitivity in OC (155). Additionally, Tsai et al. revealed that miR-519d was downregulated in human osteosarcoma cells MG-63 and U-2 by CCN family member 2, and its reduction facilitated drug resistance by upregulating the ABCG2 levels (156). Moreover, Amponsah et al. demonstrated that miR-210 overexpression decreased ABCC5 mRNA levels in pancreatic cancer (PC) cell lines (ASAN-PaCa, AsPC-1 and MIA-PaCa2) by targeting its 3’-UTR, leading to the enhancement of gemcitabine sensitivity in PC (157). In addition, several miRNAs, such as miR-34a, miR-7-5p, and miR-325-3p, have also been shown to play a role in cancer drug resistance by influencing drug efflux via targeting ABC transporters in a variety of cancers, including colon cancer, glioblastoma, and hepatocellular carcinoma (HCC) (158–160). Taken together, these studies strongly suggest that miRNAs are involved in the development of cancer drug resistance by altering the function of drug efflux pumps. However, the detailed mechanisms are still inconclusive and need to be further elucidated.
MiRNAs are involved in the development of cancer drug resistance by modulating autophagy
Autophagy is a lysosomal degradation process that is essential for cellular survival, differentiation, and homeostasis. Protective autophagy has been recognized as one of the main mechanisms resulting in cancer drug resistance, by which cancer cells eliminate the cytotoxicity of chemotherapeutic drugs (161). Therefore, targeting autophagy may be an effective therapeutic strategy for improving the poor prognosis of cancer patients. MiRNAs have been shown to participate in cancer drug resistance by regulating autophagy-related genes (162). For instance, Zhou et al. found that miR-133a was significantly downregulated in CDDP-resistant OC cell lines A2780 and SKOV3. The overexpression of miR-133a significantly enhanced the CDDP sensitivity in CDDP-resistant A2780 and SKOV3 cells by inhibiting autophagy via directly targeting YES1 (45). Li et al. showed that miR-20a-5p inhibited autophagy in CDDP-resistant OC cells. Mechanistically, miR-20a-5p suppressed the expression of RBP1 in CDDP-resistant A2780 and COC1 by promoting DNMT3B-mediated RBP1 methylation, resulting in the inhibition of autophagy and CDDP resistance in OC (163). H446/EP was a MDR small cell lung cancer (SCLC) cell line that was developed from H446. Li et al. demonstrated that miR-199a-5p was significantly upregulated in H446/EP cells compared to non-drug-resistant H446 cells. MiR-199a-5p overexpression decreased the CDDP sensitivity in H446 cells by enhancing the autophagy activity via directly targeting p62 (164). Moreover, Zhao et al. revealed that miR-145 was downregulated in CRC tissues and cell lines (HCT116, SW620, and HCT-8). The overexpression of miR-145 enhanced 5-fluorouracil (5-FU) sensitivity in 5-FU-resistant HCT116, SW620, and HCT-8 cells by enhancing 5-FU-induced apoptosis and reducing autophagy. Mechanistically, miR-145 activated p53 by directly targeting HDAC4, thereby inhibiting 5-FU resistance in CRC (165). Collectively, these studies indicate that protective autophagy induced by miRNA dysregulation is a crucial factor resulting in the occurrence of cancer drug resistance. Moreover, miRNAs may simultaneously target apoptotic pathways and autophagy. Thus, it is a valuable strategy to comprehensively identify miRNAs associated with these death pathways to help patients overcome cancer drug resistance.
MiRNAs alter stemness characteristics and EMT in cancer cells
CSCs, also known as tumor-initiating cells (TICs), are a unique subset of tumor cells exhibiting capabilities of self-renewal, differentiation, and tumor initiation. CSCs have been recognized as the main cause of drug resistance, metastasis, and recurrence of cancer (166, 167). Growing evidence suggests that miRNAs are involved in cancer drug resistance by altering the characteristics of CSCs (168). For instance, Zhang et al. found that miR-132 was upregulated in the Lrg5+ gastric CSCs isolated from MKN45 and MKN28 cells. High miR-132 expression was closely associated with chemo-resistance in GC patients. Mechanistic assays revealed that miR-132 facilitated CDDP resistance in Lrg5+ gastric CSCs by upregulating ABCG2 via directly targeting SIRT1 (169). Feng et al. showed that miR-25 was upregulated in liver CSCs (LCSCs) isolated from HepG2, Huh7, and PLC cells compared with the non-CSCs. The knockdown of miR-25 significantly enhanced the sensitivity of the LCSCs to tumor necrosis factor-related apoptosis-inducing ligand (TRAIL)-induced apoptosis by inhibiting Bad phosphorylation via upregulating phosphatase and tensin homologue (PTEN), a PI3K inhibitor (170). In addition, Ni et al. revealed that miR-375 suppressed the stemness of GC cells BGC-823 and SGC-7901 by triggering ferroptosis via directly targeting SLC7A11 (171). Epithelial mesenchymal transition (EMT) is a morphogenetic process that endows epithelial cells with migratory and invasive characteristics. The aberrant activation of EMT has been shown to facilitate the development of cancer drug resistance by enabling the conversion of non-CSCs into CSCs (172, 173). MiRNAs can participate in the development of cancer drug resistance by targeting the EMT process. For instance, Hirao et al. showed that the overexpression of miR-125b-5p in HCC cell lines (PLC/PRF5-R1/R2) enhanced sorafenib resistance. Mechanistically, miR-125b-5p promoted the EMT process and conferred stemness characteristics in PLC/PRF5-R1/R2 cells by targeting ATXN1, leading to the reduction of sorafenib sensitivity in HCC. Consistent with this, ATXN1 knockdown in HCC cells exhibited a higher CSC population and an EMT phenotype (174). Chaudhary et al. revealed that miR-205 was highly downregulated in gemcitabine-resistant MIA PaCa-2R cells compared to gemcitabine-sensitive MIA PaCa-2 cells. The overexpression of miR-205 resulted in a reduction in EMT, CSCs, and chemo-resistance markers in MIA PaCa-2R cells, suggesting that miR-205 can enhance the sensitivity of gemcitabine-resistant PC cells to gemcitabine (175). In addition, the overexpression of miR‐363 in drug-resistant OC cell lines (A2780cp and C13) restores CDDP sensitivity by directly targeting Snail (a mesenchymal marker). Consistent with this, Snail overexpression dramatically suppressed the effect of miR‐363 on CDDP resistance of A2780cp and C13 cells, indicating that miR‐363 regulates CDDP resistance in OC through Snail‐induced EMT (176). In summary, understanding the effect of miRNAs on stemness properties and EMT in the development of cancer drug resistance may provide new insights into the development of therapeutic strategies for patients with a poor response to chemotherapeutic agents.
MiRNAs are involved in the regulation of inflammation by targeting T cells
Chronic inflammation triggered by infections, aberrant immune reactions or environmental factors is an uncontrolled inflammatory response and contributes to cancer progression by influencing various biological behaviors of cancer cells, including cellular proliferation, invasion, angiogenesis, metastasis, and drug resistance (177). T cells are the major effector cells in cellular immunity. They are involved in inflammation by producing cytokines in immune responses (178). The immune evasion and immune tolerance induced by the dysregulation of T cell function have shown to be the main causes of drug resistance development (27). Therefore, targeting T cells is an effective way to improve drug sensitivity for cancer patients. An increasing amount of evidence suggests that miRNAs are crucial regulators of T cell functions. For example, Yan et al. discovered that miR-181a was upregulated in T-cell lymphoblastic lymphoma Jurkat and H9 cells treated with DOX, CDDP, cyclophosphamide, and cytarabine. Knockdown of miR-181a in Jurkat and H9 cells significantly enhanced the sensitivity of these chemotherapeutic drugs (179). Ning et al. demonstrated that miR-208b was upregulated in exosomes from CRC cell lines NCM460, SW480, and oxaliplatin (OXA)-resistant SW480. Exosomal miR-208b facilitated regulatory T cells expansion by targeting programmed cell death factor 4, thereby enhancing OXA resistance in CRC (180). Xu et al. showed that miR-424 (322) reversed drug resistance in OC by activating T cell immune response, resulting in the inhibition of immune evasion in drug-resistant OC. Mechanistically, miR-424 (322) inhibited IFN-γ-induced apoptosis in PD-L1-associated CD8+ T cells and altered T cell cytokine secretions by downregulating PD-L1, resulting in the enhancement of chemotherapy efficacy in Skov3 (CP) cells (181). In addition, the downregulation of miR-145 by CDDP in A2780 cells increased PD-L1 levels by directly targeting c-Myc, leading to the induction of T cell apoptosis and enhancement of CDDP resistance in OC (182), indicating that miR-145 dysregulation contributes to the development CDDP resistance via T cell dysfunction-mediated immune tolerance. All these findings support the hypothesis that miRNAs are involved in the regulation of cancer drug resistance by targeting T cells. Therefore, in-depth investigations are required to clarify the detailed mechanisms of miRNAs in regulating T cells, which may provide new insights into the development of miRNA-based therapeutic strategies for cancer patients, particularly those with a poor response to chemotherapy.
LncRNAs and cancer drug resistance
LncRNAs control the cellular death pathways in cancer drug resistance
The dysregulation of lncRNAs has been shown to participate in the development of cancer drug resistance through interference with cellular apoptosis or proliferation pathways (18, 183). For instance, Li et al. revealed that lncRNA TINCR was significantly increased in CDDP-resistant choroidal melanoma (CM) tissues and cells. TINCR overexpression in OCM-1 cells promoted proliferation and inhibited apoptosis by upregulating ERK-2 via sponging miR-19b-3p, leading to the enhancement of CDDP resistance in CM (184). Zhou et al. found that lncRNA CCAT2 was upregulated in breast cancer (BC) tissues and 5-FU-resistant BC cell lines (MDA‐MB‐231, SKBR‐3, MCF‐7, and HCC‐1937) after chemotherapy. CCAT2 overexpression in 5-FU-resistant MDA‐MB‐231, MCF‐7 cells inhibited apoptosis and increased proliferation by activating the mTOR signaling pathway, resulting in a reduction in 5-FU sensitivity (185). Guo et al. demonstrated that lncRNA HEIH was upregulated in PTX-resistant endometrial cancer Ishikawa and HHUA cells. The overexpression of HEIH in Ishikawa and HHUA cells enhanced PTX resistance by depressing cell apoptosis and enhancing cell proliferation and viability via activating the MAPK signaling pathway (186). Zhu et al. showed that LINC00942 was significantly upregulated in drug-resistant GC cell lines SGC7901 and BGC823, and its overexpression in SGC7901 and BGC823 cells facilitated drug resistance by suppressing cellular apoptosis and enhancing their stemness features. Mechanistically, LINC00942 upregulated MSI2 by inhibiting its degradation via preventing its interaction with SCFβ-TRCP E3 ubiquitin ligase, thereby stabilizing c-Myc mRNA in an m6A-dependent manner (187). In addition, several oncogenic lncRNAs, such as APOC1P1-3, PRLB, and WDFY3-AS2, have also been reported to promote drug resistance by targeting the apoptotic pathways in distinct cancer types (53, 188, 189).
Recent studies indicate that the activation of autophagy by chemotherapeutic agents can protect cancer cells from drug-induced apoptosis (161, 190). Zhang et al. showed that exosomal lncRNA SNHG7 was highly expressed in docetaxel-resistant lung adenocarcinoma (LUAD) H1299 and SPC-A1 cells. The knockdown of SNHG7 in docetaxel-resistant H1299 and SPC-A1 cells significantly inhibited cell proliferation and autophagy and enhanced docetaxel sensitivity. Mechanistically, SNHG7 upregulation facilitated autophagy of H1299 and SPC-A1 cells by stabilizing autophagy-related genes autophagy related 5 (ATG5) and autophagy related 12 (ATG12) via recruiting human antigen R (HuR), resulting in the enhancement of docetaxel resistance in LUAD. Moreover, the transmission of exosomal SNHG7 from docetaxel-resistant H1299 and SPC-A1 cells to parental H1299 and SPC-A1 cells also promoted docetaxel resistance (191). In another study, lncRNA TUG1 was found to be upregulated in CRC tissues. The overexpression of TUG1 in LoVo and HCT15 cells enhanced CDDP resistance. Functional assays revealed that TUG1 promoted the proliferation and autophagy of LoVo and HCT15 cells by activating the HDGF/DDX/β-catenin axis via sequestrating miR-195-5p, leading to the enhancement of CDDP resistance in CRC (192). In addition, Chen et al. found that CRNDE triggered autophagy in HepG2 and Hep3B cells by increasing ATG4B levels via sponging miR-543. CRNDE silencing enhanced the sorafenib sensitivity of HepG2 and Hep3B cells, indicating that CRNDE may promote sorafenib resistance in HCC by driving ATG4B-mediated autophagy (193). High-mobility group box 1 (HMGB1) is a classical non-histone protein closely associated with autophagy (194). Chen et al. revealed that lncRNA H19 overexpression in CDDP-resistant TU-177 and AMC-HN-8 cells significantly facilitated autophagy by upregulating HMGB1 via sequestrating miR-107, resulting in the enhancement of CDDP resistance in laryngeal squamous cell carcinoma (LSCC). Consistent with this, the knockdown of H19 in CDDP-resistant TU-177 cells inhibited autophagy and CDDP resistance (195). Taken together, these findings strongly suggest that lncRNAs are widely involved in the development of cancer drug resistance by targeting cellular death pathways. However, the detailed mechanisms are still not fully understood; additional investigations are required to fully uncover the regulatory role of lncRNAs in cellular death pathways.
LncRNAs modulate ABC transporter-mediated drug efflux in cancer cells
The upregulation of ABC transporters is considered a main cause of MDR development in cancer. An increasing amount of evidence has shown that lncRNAs are involved in cancer drug resistance by regulating ABC transporter-mediated drug efflux (196). For instance, Chen et al. revealed that lncRNA GAS5 overexpression in BC cells significantly enhanced the adriamycin (ADR) sensitivity by inhibiting ABCB1-mediated drug efflux. Mechanistically, GAS5 suppressed the expression of ABCB1 in ADR-resistant MCF-7 cells by activating the Wnt/β-catenin signaling pathway via miR-221-3p/DKK2 axis (197). In another study, lncRNA ADORA2A-AS1 was found to be upregulated in chronic myeloid leukemia (CML). ADORA2A-AS1 knockdown in K562 and KCL22 cells significantly enhanced the imatinib sensitivity of cells. Functional assays showed that ADORA2A-AS1 facilitated ABCC2 expression in K562 and KCL22 cells via sponging miR-665, indicating that ADORA2A-AS1 may contribute to the development of imatinib resistance by driving ABCC2-mediated drug efflux in CML (198). Moreover, Wang et al. demonstrated that lncRNA KCNQ1OT1 significantly increased in temozolomide (TMZ)-resistant U251 and U87 cells compared to TMZ-sensitive U251 and U87 cells. KCNQ1OT1 overexpression in TMZ-resistant U251/TMZ and U87/TMZ cells significantly upregulated the expression of ABCB1, c-Myc, and survivin by increasing PIM1 expression via sponging miR-761, leading to the enhancement of TMZ resistance (199). Shen et al. found that lncARSR was upregulated in ADR-resistant osteosarcoma U2OS and MG63 cells and accompanied by acquired MDR against PTX and CDDP. Mechanistically, lncARSR overexpression in ADR-resistant U2OS and MG63 cells significantly promoted cell rhodamine 123 efflux, survival, and migration by upregulating ABCB1, survivin, and matrix metalloproteinase-2 (MMP2) via activating AKT. Consistent with this, lncARSR knockdown in these ADR-resistant osteosarcoma cells facilitated cell rhodamine 123 retention and apoptosis (200). In addition, Li et al. showed that lncRNA HOTTIP was highly expressed in serum from esophageal cancer (EC) patients. Extracellular vesicles-containing HOTTIP contributed to ADR resistance in EC Eca109 cells by positively activating ABCG2 (201). Collectively, these studies indicate that the dysregulation of lncRNAs contributes to the development of cancer drug resistance by modulating ABC transporter-mediated drug efflux via targeting miRNAs. The exact mechanisms of the lncRNA/miRNA axis in drug efflux need to be further elucidated.
LncRNAs manipulate malignant features of cancer cells
LncRNAs have been shown to regulate the stemness of cancer cells, thereby demonstrating their regulatory roles in cancer drug resistance. For instance, Xie et al. found that lncRNA CBR3-AS1 was significantly upregulated in CRC cell lines (HCT116, HT29, SW620, and SW480) compared to normal colon epithelial FHC cells. CBR3-AS1 knockdown in OXA-resistant HCT116 and SW480 cells notably enhanced OXA sensitivity. Mechanistically, CBR3-AS1 knockdown inhibited the stem-like properties of HCT116 and SW480 cells by downregulating Nanog, Sox2, and Oct4 (stem cell markers) via sponging miR-145-5p, resulting in the reduction of OXA resistance in CRC (202). Liu et al. showed that lncROPM promoted the drug resistance of breast CSCs (BCSCs) isolated from BT-549, Hs578T, and MCF-7 cells by upregulating PLA2G16 via increasing its mRNA stability. Moreover, lncROPM contributed to the maintenance of BCSC stemness by facilitating phospholipid metabolism and the production of free fatty acid (such as arachidonic acid) via increasing the PLA2G16 levels (203). Cheng et al. revealed that lncRNA SNHG7 significantly increased in PC cells (PANC-1 and AsPC-1) co-cultured with mesenchymal stem cells (MSCs). The upregulation of SNHG7 induced by the MSCs in PANC-1 and AsPC-1 cells facilitated stemness of cells and Folfirinox resistance by activating the Notch1/Jagged1/Hes-1 signaling pathway via increasing Notch1 expression (204). In addition, Liu et al. demonstrated that lncRNA DUBR was highly expressed in HCC tissues and liver CSCs isolated from MHCC-97H, SNU-368 and MIHA, and its high expression was closely associated with poor chemotherapy response. DUBR overexpression in SNU-368 and MHCC-97H cells promoted the stemness of cancer cells and OXA resistance. Functional assays revealed that DUBR activated the Notch1 signaling pathway by upregulating cancerous inhibitor of protein phosphatase 2A (CIP2A) levels via sponging miR-520d-5p, leading to the enhancement of the stemness characteristics of the HCC cells and drug resistance (205).
LncRNA dysregulation contributes to the development of cancer drug resistance by altering T cell activity. For instance, KCNQ1OT1 was found to be upregulated in sorafenib‐resistant HCC tissues and cells, and its knockdown in sorafenib‐resistant SK-HEP-1 and Huh-7 cells co-cultured with T cells significantly inhibited immune escape by enhancing the immune surveillance ability of T cells. Mechanistically, KCNQ1OT1 upregulated PD‐L1 levels in sorafenib‐resistant SK-HEP-1 and Huh-7 cells by sponging miR‐506, thereby reducing the apoptosis of CD8+ T cells (206). In another study, LINC00184 overexpression in docetaxel-resistant DU145 and PC3 cells facilitated cell immune escape by upregulating PD-L1 via sponging miR-105-5p, resulting in the enhancement of docetaxel resistance in PCa (207). In addition, HCG18 could inhibit CD8+ T cells activity by increasing PD-L1 levels via sponging miR-20b-5p, leading to the promotion of cetuximab resistance in CRC cells (208). LncRNAs can also act as the recruiters of epigenetic modifiers to play a role in cancer drug resistance. For instance, Li et al. found that PCAT-1 was upregulated in CDDP-resistant GC tissues and cell lines. PCAT-1 knockdown resensitized CDDP-resistant BGC823 and SGC790 cells to CDDP. Functional assays revealed that PCAT-1 epigenetically silenced PTEN by increasing H3K27me3 via recruiting the histone methyltransferase enhancer of zeste homolog 2 (EZH2), resulting in the enhancement of CDDP resistance in GC (209). Si et al. showed that H19 was highly expressed in PTX-resistant BC cells. H19 upregulation in PTX-resistant MCF-7 and ZR-75-1 cells facilitated the recruitment of EZH2 to the BIK gene promoter, increasing H3K27me3 modification and suppressing BIK gene expression (210). In addition, Lin et al. revealed that LINC00261 was downregulated in 5-FU-resistant EC tissues. The overexpression of LINC00261 dramatically inhibited resistance to apoptosis in 5-FU-resistant TE-1 and -5 cells, whereas LINC00261 knockdown observed the opposite effect. Mechanistically, LINC00261 significantly decreased the levels of dihydropyrimidine dehydrogenase by increasing the methylation of its promoter through the recruitment of DNA methyltransferase, thereby enhancing 5-FU sensitivity in EC (211).
LncRNAs are able to govern the EMT process and malignant features of cancer cells to play a role in cancer drug resistance. Li et al. discovered that HOTTIP overexpression in glioma A172 and LN229 cells significantly increased cell proliferation, migration, and metastasis. Further, HOTTIP facilitated the EMT process in TMZ-resistant A172 and LN229 cells by decreasing E-cadherin expression and increasing Zeb1/Zeb2 (mesenchymal markers) via upregulating miR-10b, resulting in the enhancement of TMZ resistance in glioma. Consistent with this, miR-10b knockdown in HOTTIP-overexpressing A172 and LN229 cells reversed the EMT with associated TMZ sensitization (212). Zhang et al. demonstrated that HOTAIR facilitated migration, proliferation, and the resistance of HeLa and Siha cells to CDDP, PTX, and docetaxel. Mechanistically, HOTAIR enhanced the EMT process in HeLa and Siha cells by activating the PTEN/PI3K axis via sequestrating miR-29b, leading to the enhancement of MDR in CC (213). Jiang et al. revealed that HNF1A-AS1 facilitated 5-FU resistance in GC cells (MKN-45 and HGC-27) by enhancing the EMT process via increasing EIF5A2 levels. HNF1A-AS1 served as a sponge of miR-30b-5p to upregulate EIF5A2 (71). Moreover, Zhao et al. showed that DLX6-AS1 promoted proliferation, migration, invasion, and secondary CDDP resistance in LSCC cell lines SK-MES-1 and NCIH226. Mechanistically, DLX6-AS1 increased the expression of CUGBP, Elav-like family member 1 by sponging miR-181a-5p and miR-382-5p, resulting in the secondary CDDP resistance of LSCC cells (214). In addition, multiple lncRNAs, such as LINC01089, CYTOR, and H19, have also been shown to demonstrate their roles in cancer drug resistance by targeting the EMT process and altering malignant characteristics, such as proliferation, invasion, and metastasis (215–217). Altogether, these findings suggest that the underlying mechanisms of lncRNAs in cancer drug resistance involve their modulation of CSC expansion, T cell activity, EMT process, and malignant characteristics. In-depth investigations are required to fully elucidate the exact mechanisms behind lncRNA-mediated cancer drug resistance, which will be of great benefit in the development of lncRNA-based therapeutic strategies for cancer patients exhibiting a poor response to chemotherapy.
CircRNAs and cancer drug resistance
In recent years, the role of circRNAs in cancer progression has become a research hotspot, but the investigation of the contribution of circRNAs to cancer drug resistance is still at an initial stage (20, 218, 219). Emerging evidence indicates that the dysregulation of circRNAs is involved in cancer drug resistance via distinct mechanisms, such as drug transportation, cell death, DNA repair, and cancer stemness (220).
CircRNAs mainly act as miRNA sponges to play regulatory roles in cancer drug resistance. For instance, Xu et al. showed that circ-FBXW7 was downregulated in OXA-resistant CRC tissues and cells. Exosomal transfer of circ-FBXW7 enhanced the sensitivity of the OXA-resistant SW480 and HCT116 cells to OXA by inhibiting OXA efflux, elevating the OXA-induced apoptosis, and suppressing OXA-induced EMT via sponging miR-18b-5p (100). Another circRNA, circRNA_101277, was found to be highly expressed in CRC tissues and cells, and its overexpression in SW620 and SW480 cells facilitated CDDP resistance by upregulating IL-6 via sequestering miR-370 (221). Furthermore, Zhong et al. revealed that circRNA_100565 was upregulated in CDDP-resistant NSCLC tissues and cells. CircRNA_100565 knockdown in the drug-resistant A549 and H1299 cells reduced CDDP resistance by enhancing cell apoptosis and inhibiting proliferation and autophagy. Mechanistically, circRNA_100565 exerted its anti-drug resistant role by upregulating ADAM28 expression via sponging miR-377-3p in CDDP-resistant A549 and H1299 cells (92). Additionally, Huang et al. demonstrated that circAKT3 was highly expressed in CDDP-resistant GC tissues and cells compared to CDDP-sensitive samples. The upregulation of circAKT3 was closely associated with aggressive characteristics in GC patients receiving CDDP treatment. Functional assays demonstrated that circAKT3 upregulated PIK3R1 via sequestrating miR-198, thereby enhancing CDDP resistance by facilitating DNA damage repair and inhibiting the apoptosis of CDDP-resistant SGC7901 and BGC823 cells (222). CircRNA CDR1as was found to contribute to the development of CDDP resistance in NSCLC by altering the stemness characteristics of NSCLC cells. The overexpression of circRNA CDR1as in CDDP-sensitive NSCLC cells (A549, H1299, and Calu6) significantly increased the expression of stemness signatures (e.g., Sox2, Oct4 and Nanog) by upregulating HOXA9 via sponging miR-641, leading to the enhancement of CDDP resistance. Consistent with this, circRNA CDR1as knockdown in CDDP-resistant A549, H1299, and Calu6 cells suppressed the stemness of cancer cells (223). Moreover, Huang et al. demonstrated that circ_0001598 was highly expressed in trastuzumab-resistant BC samples, and its overexpression facilitated immune escape and trastuzumab-resistance of SKBR-3 and BT474 cells by upregulating PD-L1 levels via sponging PD-L1 (224). In addition, Chen et al. showed that high circUSP7 levels are closely associated with CD8+ T cell dysfunction in NSCLC patients. Exosomal circUSP7 inhibited CD8+ T cell activity by upregulating Src homology region 2 (SH2)-containing protein tyrosine phosphatase 2 via sponging miR-934, resulting in enhanced resistance to anti-PD1 immunotherapy in NSCLC patients (225). There is no doubt that circRNAs have multifaceted functions in cancer drug resistance due to the broad involvement of miRNAs.
CircRNAs can also participate in cancer drug resistance by combining with other molecules. For instance, Wei et al. found circ0008399 enhanced CDDP resistance in bladder cancer EJ and T24T cells by upregulating TNF alpha-induced protein 3 (TNFAIP3) via directly binding to Wilms’ tumor 1-associating protein (WTAP). Mechanistically, circ0008399 interacted with WTAP to promote the formation of the WTAP/METTL3/METTL14 m6A methyltransferase complex, thereby upregulating TNFAIP3 expression in an m6A-dependent manner. Consistent with this, targeting the circ0008399/WTAP/TNFAIP3 axis promoted CDDP sensitivity in EJ and T24T cells (226). Hu et al. showed that circFARP1 was involved in the regulation of stemness and gemcitabine resistance in pancreatic ductal adenocarcinoma by altering the ability of cancer-associated fibroblasts via leukemia inhibitory factor (LIF). Functional assays revealed that circFARP1 directly interacted with caveolin 1 to inhibit its degradation by blocking the binding of caveolin 1 to its ubiquitin E3 ligase zinc and ring finger 1 (ZNRF1), thereby enhancing LIF secretion (227). In addition, Chen et al. demonstrated that the overexpression of circRNA cia-MAF drove LCSC propagation, self-renewal, and metastasis by facilitating MAFF expression via recruiting the TIP60 complex to its promoter, indicating that cia-MAF may contribute to the drug resistance of liver cancer by modulating CSCs (228). Particular circRNAs may participate in cancer drug resistance by altering the key regulators during cancer progression. Further investigations are required to fully understand the detailed mechanisms of circRNAs in cancer drug resistance. In addition, the circRNA/miRNA axis associated with chemotherapeutic responsiveness in cancer should be clarified.
PiRNAs and cancer drug resistance
PiRNAs are a novel class of short chain ncRNAs (26-30 nucleotides) involved in a wide variety of physiological and pathological processes. They can regulate the expression of somatic genes through various mechanisms, including DNA methylation, chromatin modification and transposon silencing (229, 230). An increasing amount of evidence suggests that piRNAs are key regulators in the development of cancer drug resistance (231–233). For instance, Tan et al. discovered that piRNA-36,712 was significantly downregulated in BC tissues. The overexpression of piRNA-36,712 in MCF-7 and ZR75-1 cells significantly enhanced the sensitivity of cells to PTX and DOX. Correspondingly, piRNA-36,712 knockdown obtained the opposite effects. Mechanistically, piRNA-36,712 directly interacted with SEPW1P RNA (SEPW1 pseudogene), thereby suppressing SEPW1 expression by facilitating miR-7 and miR-324 to target SEPW1 RNA, resulting in the enhancement of PTX and DOX sensitivity in BC (231). Mai et al. showed that piRNA-54265 was upregulated in CRC tissues. The overexpression of piRNA-54265 in HCT116 and LoVo cells promoted the formation of PIWIL2/STAT3/p-SRC complex by directly binding to PIWIL2, thereby activating the STAT3 signaling pathway, leading to the resistance of CRC cells to 5-FU and OXA (232). In addition, Wang et al. demonstrated that piR-L-138 was upregulated in CDDP-treated LSCC cells and patient-derived xenograft treated with CDDP. The knockdown of piR-L-138 in H157 and SKMES-1 cells enhanced CDDP sensitivity by directly binding to p60-MDM2 (233). Collectively, these findings indicate that piRNAs play vital roles in the regulation of cancer drug resistance, but the detailed mechanisms remain largely unknown. In-depth investigation may bring great benefits to the development of piRNA-based therapeutic strategies for cancer patients, particularly those with a poor response to chemotherapy.
Clinical implications of ncRNAs in cancer drug resistance
NcRNAs as biomarkers for the diagnosis and prognosis of cancer patients
It has been reported that approximately 50% of cancer patients are diagnosed at an advanced stage, with poor response rates and a low chance of cure (3). This is the main factor leading to the poor survival of cancer patients. Moreover, it is difficult for most cancer patients to obtain accurate individualized therapeutic strategies due to the lack of effective methods for prognostic assessment in clinical practice. In recent years, several protein biomarkers, such as carcinoembryonic antigen, carbohydrate antigen 15-3, and human epidermal growth factor receptor-2, have been applied in the early diagnosis and prognostic assessment of cancer patients. However, the unsatisfactory sensitivity and specificity of these biomarkers restricts their further utilization (234–236). Thus, it is urgent to develop new biomarkers with high sensitivity and specificity for cancer patients, particularly those with a poor response to chemotherapy.
NcRNAs can be secreted in actively packed particles (e.g., exosomes, microvesicles, or apoptotic bodies) and freely circulate in the blood, and their concentrations are almost the same as those in primary tumors (237, 238). Moreover, they also exhibit some unique characteristics, such as differently expressed patterns, high stability, and high detectability (239). These features strongly suggest that ncRNAs possess great potential as ideal diagnostic and prognostic biomarkers for cancer patients in clinical treatment. In fact, a large number of ncRNAs, particularly miRNAs and lncRNAs, have been identified as diagnostic and/or prognostic biomarkers of cancer (Table 2). For instance, Pan et al. showed that the levels of miR-33a-5p and miR-128-3p in whole blood were significantly downregulated in lung cancer patients or early-stage lung cancer patients compared to healthy controls. Further prospective study revealed that the area under the curve (AUC) value for the combination of miR-33a-5p and miR-128-3p was 0.9511, which was higher than that for CYFR21-1 (0.5856), NSE (0.6189), and CA72-4 (0.5206), indicating that the combination of the two miRNAs can serve as novel biomarkers for the early detection of lung cancer (284). In another study, Lu et al. developed a 21-miRNA-based diagnostic model and a 3-miRNA-based prognostic model that can be used to predict the prognosis of uterus corpus endometrial cancer patients and their response to chemotherapy and immunotherapy. The AUC values for the diagnostic panels were 0.911 in the training set, 0.827 in the test set, and 0.878 in the entire set. The diagnostic panel was closely associated with tumor mutation burden, PDL1 expression, and the infiltration of immune cells. Moreover, the prognostic risk signature of the prognostic panel can be used to predict the response to some commonly used chemotherapy regimens (285).
In a recent study by Xu et al., they found that the plasma levels of ZFAS1, SNHG11, LINC00909 and LINC00654 were significantly downregulated in postoperative CRC patients compared to preoperative CRC patients. The combination of these four lncRNAs exhibited high diagnostic performance for CRC (AUC = 0.937), especially early-stage disease (AUC = 0.935). Moreover, SNHG11 exhibited the greatest diagnostic ability to distinguish precancerous lesions from early-stage tumor formation (286). Besides, Meng et al. showed that lncRNA BCAR4 overexpression was closely associated with lymph node metastasis (p < 0.001), high tumor stage (p < 0.001), and distant metastasis (p < 0.001). Cancer patients with upregulated lncRNA BCAR4 exhibited poor OS (p < 0.001), suggesting that lncRNA BCAR4 is a promising prognostic biomarker in cancer patients (287). CircRNAs are also promising biomarker candidates in cancer treatment. Liu et al. revealed that hsa_circRNA_101237 was significantly upregulated in multiple myeloma (MM) cells, bortezomib-resistant MM cells, and the bone marrow tissues of MM patients. The high expression of hsa_circRNA_101237 reduced the sensitivity of the MM patients to bortezomib. Further, the AUC value for hsa_circRNA_101237 was 0.92 (p < 0.0001). MM patients with upregulated hsa_circRNA_101237 also demonstrated shorter OS and progression-free survival (PFS). This data indicated that hsa_circRNA_101237 possessed great potential as a diagnostic and prognostic biomarker for MM (288). Collectively, these studies strongly suggest that ncRNAs are valuable biomarkers for diagnosis, prognosis, and predicting drug response in cancer treatment. However, larger patient cohorts are required to further validate their potential as biomarkers in clinical applications.
Therapeutic potential of ncRNAs in cancer drug resistance
The poor response of patients to chemotherapy and the emergence of drug resistance are still the most critical obstacles in clinical cancer treatment. A large number of studies have confirmed the essential roles of ncRNAs in the development of cancer drug resistance (289). They may act as oncogenes or tumor suppressors to play dual roles in cancer progression, depending on their diverse downstream targets (290). These characteristics endow ncRNAs with great potential as promising therapeutic targets or therapeutic agents in cancer treatment. Therapeutic strategies that make use of ncRNAs or directly target ncRNAs may bring great benefits to the precise treatment of cancer patients, particularly those demonstrating a poor response to chemotherapy. The delivery of tumor-suppressive ncRNAs to target cancer cells is considered a promising strategy to improve cancer intervention. For instance, miRNA-3662 was found to be downregulated in pancreatic ductal adenocarcinoma (PDAC) tissues and cell lines. MiRNA-3662 overexpression enhanced gemcitabine sensitivity and inhibited aerobic glycolysis in the PDAC cells by decreasing hypoxia-inducible factor 1α (HIF-1α) expression (126). LncRNA ENSG0000254615 was found to be highly expressed in 5-FU-sensitive CRC cells. ENSG0000254615 overexpression inhibited cell proliferation and 5-FU resistance by upregulating p21 and downregulating Cyclin D1 in CRC (291). Circ‐G004213 was significantly upregulated in CDDP-sensitive liver cancer cells and its high expression was positively associated with the prognosis of patients with liver cancer. Further analysis revealed that circ‐G004213 suppressed CDDP resistance by upregulating PRPF39 via sponging miR‐513b‐5p (292). Therefore, the upregulation of tumor-suppressive ncRNAs, such as miRNA-3662, ENSG0000254615, and circ‐G004213, may represent an effective way to inhibit cancer progression and reverse drug resistance. Targeting oncogenic ncRNAs could be another effective strategy to overcome cancer drug resistance. For instance, miR-192 was significantly increased in CDDP-resistant lung cancer cells compared to non-resistant cancer cells. The overexpression of miR-192 activated the NF-κB signaling pathway by directly targeting NF-κB repressing factor, resulting in the inhibition of apoptosis, promotion of proliferation, and enhancement of CDDP resistance in the lung cancer cells. MiR-192 knockdown obtained the opposite effect (293). In another study, circFBXL5 was found to be highly expressed in BC tissues and 5-FU-resistant BC cells. CircFBXL5 knockdown enhanced the 5-FU sensitivity in the BC cells by suppressing cell migration and invasion and facilitating apoptosis. Mechanistically, circFBXL5 promoted 5-FU resistance by upregulating HMGA2 via sequestrating miR-216b (294). Oncogenic ncRNAs, such as miRNA-3662 and circFBXL5, might be used as ideal candidates for therapeutic targets. These findings strongly suggest that the activation of tumor-suppressive ncRNAs or the inactivation of oncogenic ncRNAs are critical mechanisms that restore cancer drug sensitivity. A better understanding of the molecular mechanism of ncRNAs involved in cancer progression and drug resistance will substantially contribute to the precise treatment of cancer patients. However, there are still some challenges that need to be addressed, such as, low bioavailability, side effects, and off-target effects.
Conclusion and perspective
Cancer is one of the most common and fatal malignant diseases worldwide, with high rates of metastasis and recurrence. Chemotherapy remains the best choice for all stages of cancer, and it can effectively improve patients’ prognosis. However, the emergency of drug resistance seriously restricts the clinical efficiency of chemotherapy, and ultimately results in treatment failure. Therefore, a better understanding of the mechanisms responsible for cancer drug resistance will be of great benefit to the development of precise therapeutic strategies for cancer patients, particularly those demonstrating a poor response to chemotherapy. With the rapid development of high-throughput sequencing techniques, a large number of ncRNAs, particularly miRNAs, lncRNAs, and circRNAs, have been found to be aberrantly expressed in cancer tissues and cell lines. These aberrantly expressed ncRNAs are closely associated with cancer progression and drug resistance. It is well established that ncRNAs participate in the development of cancer drug resistance via distinct mechanisms, including the suppression of cell death pathways, induction of excessive drug efflux, facilitation of autophagy, regulation of CSC features, and enhancement of the EMT. Aberrant levels of ncRNAs have been observed in cancer patients’ blood, tissue, and even urine (295). Furthermore, the aberrant expression of ncRNAs was found to be closely associated with some pathological characteristics of cancer patients, including OS and PFS (288). These features endow ncRNAs with great potential as ideal biomarkers for the diagnosis and prognosis of cancer patients. In addition, due to the crucial roles of ncRNAs in cancer progression and drug resistance, they are considered to be promising therapeutic targets or therapeutic agents for cancer patients (Figure 3). The direct delivery of tumor-suppressive ncRNAs to target cancer cells is a promising way to improve cancer intervention. On the other hand, silencing oncogenic ncRNAs is also an effective strategy to overcome cancer drug resistance. Therefore, it is urgent to develop efficient and non-toxic delivery systems and ncRNA silencing technologies. Although some progress has been made in this area, overcoming resistance to chemotherapeutic drugs remains a large challenge. More clinical trials need to be launched to advance the development of ncRNA-based therapeutic strategies to benefit cancer patients.
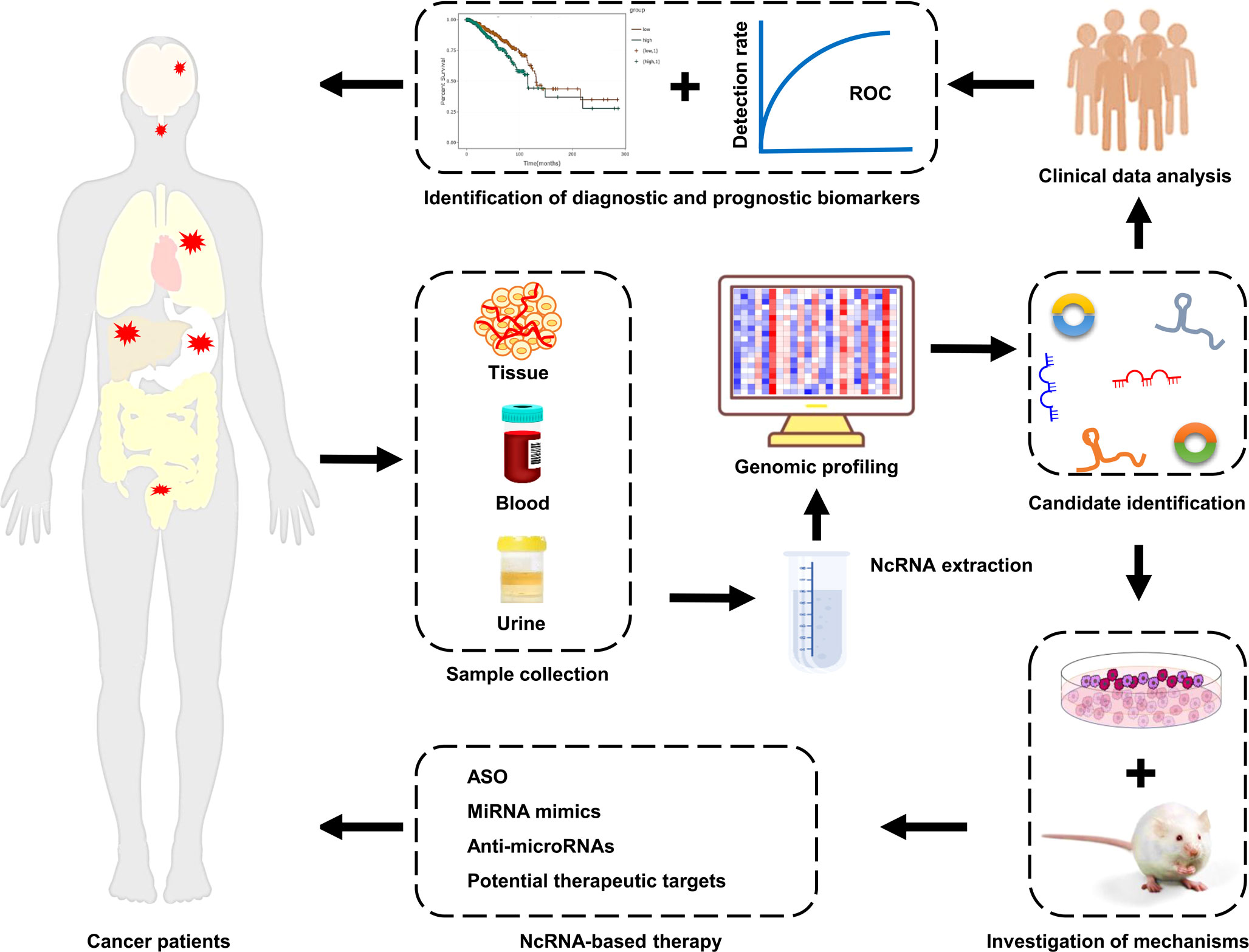
Figure 3 Clinical implications of ncRNAs in cancer drug resistance. NcRNAs are enriched in tissue, blood, and urine samples from cancer patients with drug resistance. The expression profiles of ncRNAs are mapped using high-throughput sequencing technologies. Next, the differentially expressed ncRNAs are screened and identified by bioinformatics analysis. Subsequently, the mechanisms of ncRNAs in cancer drug resistance are elucidated using cell and animal models. The aberrantly expressed ncRNAs that possessed great potential as biomarkers and/or therapeutic targets are identified. Finally, cancer patients, particularly those with drug resistance, receive the individualized precision treatment strategies.
Author contributions
XZ: original draft preparation and writing—review and editing. XA: data curation and funding acquisition. ZJ: data curation. YiwL: data curation. SK: data curation. CD: data curation. JZ: data curation. JW: data curation. YinL: writing—conceptualization, original draft preparation, and writing—review and editing. All authors contributed to the article and approved the submitted version.
Funding
All authors are supported by Qingdao Medical College, Qingdao University. This work was funded by the China Postdoctoral Science Foundation (2018M642607).
Conflict of interest
The authors declare that the research was conducted in the absence of any commercial or financial relationships that could be construed as a potential conflict of interest.
Publisher’s note
All claims expressed in this article are solely those of the authors and do not necessarily represent those of their affiliated organizations, or those of the publisher, the editors and the reviewers. Any product that may be evaluated in this article, or claim that may be made by its manufacturer, is not guaranteed or endorsed by the publisher.
References
1. Yin W, Wang J, Jiang L, James Kang Y. Cancer and stem cells. Exp Biol Med (Maywood) (2021) 246(16):1791–801. doi: 10.1177/15353702211005390
2. Wang M, Yu F, Zhang Y, Chang W, Zhou M. The effects and mechanisms of flavonoids on cancer prevention and therapy: Focus on gut microbiota. Int J Biol Sci (2022) 18(4):1451–75. doi: 10.7150/ijbs.68170
3. Sung H, Ferlay J, Siegel RL, Laversanne M, Soerjomataram I, Jemal A, et al. Global cancer statistics 2020: Globocan estimates of incidence and mortality worldwide for 36 cancers in 185 countries. CA: Cancer J Clin (2021) 71(3):209–49. doi: 10.3322/caac.21660
4. Hirsch FR, Walker J, Higgs BW, Cooper ZA, Raja RG, Wistuba II. The combiome hypothesis: Selecting optimal treatment for cancer patients. Clin Lung Cancer (2022) 23(1):1–13. doi: 10.1016/j.cllc.2021.08.011
5. Lara-Velazquez M, Mehkri Y, Panther E, Hernandez J, Rao D, Fiester P, et al. Current advances in the management of adult craniopharyngiomas. Curr Oncol (2022) 29(3):1645–71. doi: 10.3390/curroncol29030138
6. Zuo YB, Zhang YF, Zhang R, Tian JW, Lv XB, Li R, et al. Ferroptosis in cancer progression: Role of noncoding rnas. Int J Biol Sci (2022) 18(5):1829–43. doi: 10.7150/ijbs.66917
7. Wagner AD, Syn NL, Moehler M, Grothe W, Yong WP, Tai BC, et al. Chemotherapy for advanced gastric cancer. Cochrane Database Syst Rev (2017) 8:CD004064. doi: 10.1002/14651858.CD004064.pub4
8. Liu Y, Ao X, Zhou X, Du C, Kuang S. The regulation of pbxs and their emerging role in cancer. J Cell Mol Med (2022) 26(5):1363–79. doi: 10.1111/jcmm.17196
9. Zhang Y, Wei YJ, Zhang YF, Liu HW, Zhang YF. Emerging functions and clinical applications of exosomal ncrnas in ovarian cancer. Front Oncol (2021) 11:765458. doi: 10.3389/fonc.2021.765458
10. Jiang Y, Zhao L, Wu Y, Deng S, Cao P, Lei X, et al. The role of ncrnas to regulate immune checkpoints in cancer. Front Immunol (2022) 13:853480. doi: 10.3389/fimmu.2022.853480
11. Wang M, Chen X, Yu F, Ding H, Zhang Y, Wang K. Extrachromosomal circular dnas: Origin, formation and emerging function in cancer. Int J Biol Sci (2021) 17(4):1010–25. doi: 10.7150/ijbs.54614
12. Najafi S, Tan SC, Raee P, Rahmati Y, Asemani Y, Lee EHC, et al. Gene regulation by antisense transcription: A focus on neurological and cancer diseases. Biomed Pharmacother = Biomed Pharmacotherapie (2022) 145:112265. doi: 10.1016/j.biopha.2021.112265
13. Zhang YF, Shan C, Wang Y, Qian LL, Jia DD, Zhang YF, et al. Cardiovascular toxicity and mechanism of bisphenol a and emerging risk of bisphenol s. Sci Total Environ (2020) 723:137952. doi: 10.1016/j.scitotenv.2020.137952
14. Shan P, Yang F, Qi H, Hu Y, Zhu S, Sun Z, et al. Alteration of Mdm2 by the small molecule Yf438 exerts antitumor effects in triple-negative breast cancer. Cancer Res (2021) 81(15):4027–40. doi: 10.1158/0008-5472.CAN-20-0922
15. Li Z, Wang Y, Liu S, Li W, Wang Z, Jia Z, et al. Mir-200a-3p promotes gastric cancer progression by targeting dlc-1. J Mol Histol (2022) 53(1):39–49. doi: 10.1007/s10735-021-10037-7
16. Lin X, Zhuang S, Chen X, Du J, Zhong L, Ding J, et al. Lncrna Itgb8-As1 functions as a cerna to promote colorectal cancer growth and migration through integrin-mediated focal adhesion signaling. Mol Ther (2022) 30(2):688–702. doi: 10.1016/j.ymthe.2021.08.011
17. Ishola AA, Chien CS, Yang YP, Chien Y, Yarmishyn AA, Tsai PH, et al. Oncogenic circrna C190 promotes non-small cell lung cancer Via modulation of the Egfr/Erk pathway. Cancer Res (2022) 82(1):75–89. doi: 10.1158/0008-5472.CAN-21-1473
18. Chen B, Dragomir MP, Yang C, Li Q, Horst D, Calin GA. Targeting non-coding rnas to overcome cancer therapy resistance. Signal Transduction Targeted Ther (2022) 7(1):121. doi: 10.1038/s41392-022-00975-3
19. Yamaguchi K, Yamamoto T, Chikuda J, Shirota T, Yamamoto Y. Impact of non-coding rnas on chemotherapeutic resistance in oral cancer. Biomolecules (2022) 12(2):284. doi: 10.3390/biom12020284
20. Wang M, Yu F, Li P, Wang K. Emerging function and clinical significance of exosomal circrnas in cancer. Mol Ther Nucleic Acids (2020) 21:367–83. doi: 10.1016/j.omtn.2020.06.008
21. Mattick JS. Non-coding rnas: The architects of eukaryotic complexity. EMBO Rep (2001) 2(11):986–91. doi: 10.1093/embo-reports/kve230
22. Nair J, Maheshwari A. Non-coding rnas in necrotizing enterocolitis- a new frontier? Curr Pediatr Rev (2022) 18(1):25–32. doi: 10.2174/1573396317666211102093646
23. Liu Y, Ao X, Jia Y, Li X, Wang Y, Wang J. The foxo family of transcription factors: Key molecular players in gastric cancer. J Mol Med (Berl) (2022) 100(7):997–1015. doi: 10.1007/s00109-022-02219-x
24. Dahariya S, Paddibhatla I, Kumar S, Raghuwanshi S, Pallepati A, Gutti RK. Long non-coding rna: Classification, biogenesis and functions in blood cells. Mol Immunol (2019) 112:82–92. doi: 10.1016/j.molimm.2019.04.011
25. Ma L, Bajic VB, Zhang Z. On the classification of long non-coding rnas. RNA Biol (2013) 10(6):925–33. doi: 10.4161/rna.24604
26. Dozmorov MG, Giles CB, Koelsch KA, Wren JD. Systematic classification of non-coding rnas by epigenomic similarity. BMC Bioinf (2013) 14 Suppl 14:S2. doi: 10.1186/1471-2105-14-S14-S2
27. Liu Y, Ao X, Ji G, Zhang Y, Yu W, Wang J. Mechanisms of action and clinical implications of micrornas in the drug resistance of gastric cancer. Front Oncol (2021) 11:768918. doi: 10.3389/fonc.2021.768918
28. Liu Y, Ding W, Yu W, Zhang Y, Ao X, Wang J. Long non-coding rnas: Biogenesis, functions, and clinical significance in gastric cancer. Mol Ther Oncolytics (2021) 23:458–76. doi: 10.1016/j.omto.2021.11.005
29. Liu Y, Ao X, Yu W, Zhang Y, Wang J. Biogenesis, functions, and clinical implications of circular rnas in non-small cell lung cancer. Mol Ther Nucleic Acids (2022) 27:50–72. doi: 10.1016/j.omtn.2021.11.013
30. Hurschler BA, Harris DT, Grosshans H. The type ii poly(a)-binding protein pabp-2 genetically interacts with the let-7 mirna and elicits heterochronic phenotypes in caenorhabditis elegans. Nucleic Acids Res (2011) 39(13):5647–57. doi: 10.1093/nar/gkr145
31. Wu H, Sun S, Tu K, Gao Y, Xie B, Krainer AR, et al. A splicing-independent function of Sf2/Asf in microrna processing. Mol Cell (2010) 38(1):67–77. doi: 10.1016/j.molcel.2010.02.021
32. Akhtar J, Lugoboni M, Junion G. M(6)a rna modification in transcription regulation. Transcription (2021) 12(5):266–76. doi: 10.1080/21541264.2022.2057177
33. Di Timoteo G, Dattilo D, Centron-Broco A, Colantoni A, Guarnacci M, Rossi F, et al. Modulation of circrna metabolism by M(6)a modification. Cell Rep (2020) 31(6):107641. doi: 10.1016/j.celrep.2020.107641
34. Galicia J, Khan AA. Non-coding rnas in endodontic disease. Semin Cell Dev Biol (2022) 124:82–4. doi: 10.1016/j.semcdb.2021.07.006
35. Chen X, Li X, Wei C, Zhao C, Wang S, Gao J. High expression of Setdb1 mediated by mir-29a-3p associates with poor prognosis and immune invasion in breast invasive carcinoma. Transl Cancer Res (2021) 10(12):5065–75. doi: 10.21037/tcr-21-1527
36. Gao XQ, Liu CY, Zhang YH, Wang YH, Zhou LY, Li XM, et al. The circrna cneacr regulates necroptosis of cardiomyocytes through Foxa2 suppression. Cell Death Differ (2022) 29(3):527–39. doi: 10.1038/s41418-021-00872-2
37. Zhang L, Zhang Y, Zhao Y, Wang Y, Ding H, Xue S, et al. Circulating mirnas as biomarkers for early diagnosis of coronary artery disease. Expert Opin Ther Pat (2018) 28(8):591–601. doi: 10.1080/13543776.2018.1503650
38. Zhang L, Zhang Y, Xue S, Ding H, Wang Y, Qi H, et al. Clinical significance of circulating micrornas as diagnostic biomarkers for coronary artery disease. J Cell Mol Med (2020) 24(1):1146–50. doi: 10.1111/jcmm.14802
39. Ghanbarian H, Yildiz MT, Tutar Y. Microrna targeting. Methods Mol Biol (2022) 2257:105–30. doi: 10.1007/978-1-0716-1170-8_6
40. Gan J, Gu T, Yang H, Ao Z, Cai G, Hong L, et al. Non-coding rnas regulate spontaneous abortion: A global network and system perspective. Int J Mol Sci (2022) 23(8):4214. doi: 10.3390/ijms23084214
41. Zhang L, Wang Y, Yu F, Li X, Gao H, Li P. Circhipk3 plays vital roles in cardiovascular disease. Front Cardiovasc Med (2021) 8:733248. doi: 10.3389/fcvm.2021.733248
42. Chen J, Gao C, Zhu W. Long non-coding rna Slc25a25-As1 exhibits oncogenic roles in non-small cell lung cancer by regulating the microrna-195-5p/Itga2 axis. Oncol Lett (2021) 22(1):529. doi: 10.3892/ol.2021.12790
43. Bahar E, Han SY, Kim JY, Yoon H. Chemotherapy resistance: Role of mitochondrial and autophagic components. Cancers (2022) 14(6):1462. doi: 10.3390/cancers14061462
44. Liu Y, Ao X, Wang Y, Li X, Wang J. Long non-coding rna in gastric cancer: Mechanisms and clinical implications for drug resistance. Front Oncol (2022) 12:841411. doi: 10.3389/fonc.2022.841411
45. Zhou Y, Wang C, Ding J, Chen Y, Sun Y, Cheng Z. Mir-133a targets Yes1 to reduce cisplatin resistance in ovarian cancer by regulating cell autophagy. Cancer Cell Int (2022) 22(1):15. doi: 10.1186/s12935-021-02412-x
46. Hu Z, Cai M, Zhang Y, Tao L, Guo R. Mir-29c-3p inhibits autophagy and cisplatin resistance in ovarian cancer by regulating Foxp1/Atg14 pathway. Cell Cycle (2020) 19(2):193–206. doi: 10.1080/15384101.2019.1704537
47. Cai Y, An B, Yao D, Zhou H, Zhu J. Microrna mir-30a inhibits cisplatin resistance in ovarian cancer cells through autophagy. Bioengineered (2021) 12(2):10713–22. doi: 10.1080/21655979.2021.2001989
48. Guo J, Pan H. Long noncoding rna Linc01125 enhances cisplatin sensitivity of ovarian cancer Via mir-1972. Med Sci Monitor Int Med J Exp Clin Res (2019) 25:9844–54. doi: 10.12659/MSM.916820
49. Xiao L, Shi XY, Li ZL, Li M, Zhang MM, Yan SJ, et al. Downregulation of Linc01508 contributes to cisplatin resistance in ovarian cancer Via the regulation of the hippo-yap pathway. J Gynecol Oncol (2021) 32(5):e77. doi: 10.3802/jgo.2021.32.e77
50. Zhao Z, Ji M, Wang Q, He N, Li Y. Circular rna Cdr1as upregulates scai to suppress cisplatin resistance in ovarian cancer Via mir-1270 suppression. Mol Ther Nucleic Acids (2019) 18:24–33. doi: 10.1016/j.omtn.2019.07.012
51. Huang W, Chen L, Zhu K, Wang D. Oncogenic microrna-181d binding to ogt contributes to resistance of ovarian cancer cells to cisplatin. Cell Death Discovery (2021) 7(1):379. doi: 10.1038/s41420-021-00715-6
52. Wang J, Liu L. Mir-149-3p promotes the cisplatin resistance and emt in ovarian cancer through downregulating Timp2 and Cdkn1a. J Ovarian Res (2021) 14(1):165. doi: 10.1186/s13048-021-00919-5
53. Wu Y, Wang T, Xia L, Zhang M. Lncrna Wdfy3-As2 promotes cisplatin resistance and the cancer stem cell in ovarian cancer by regulating hsa-Mir-139-5p/Sdc4 axis. Cancer Cell Int (2021) 21(1):284. doi: 10.1186/s12935-021-01993-x
54. Wang DY, Li N, Cui YL. Long non-coding rna Ccat1 sponges mir-454 to promote chemoresistance of ovarian cancer cells to cisplatin by regulation of surviving. Cancer Res Treat (2020) 52(3):798–814. doi: 10.4143/crt.2019.498
55. Liu X, Yin Z, Wu Y, Zhan Q, Huang H, Fan J. Circular rna lysophosphatidic acid receptor 3 (Circ-Lpar3) enhances the cisplatin resistance of ovarian cancer. Bioengineered (2022) 13(2):3739–50. doi: 10.1080/21655979.2022.2029109
56. Cao Y, Xie X, Li M, Gao Y. Circhipk2 contributes to ddp resistance and malignant behaviors of ddp-resistant ovarian cancer cells both in vitro and in vivo through Circhipk2/Mir-338-3p/Chtop cerna pathway. Onco Targets Ther (2021) 14:3151–65. doi: 10.2147/OTT.S291823
57. Nakamura K, Sawada K, Miyamoto M, Kinose Y, Yoshimura A, Ishida K, et al. Downregulation of mir-194-5p induces paclitaxel resistance in ovarian cancer cells by altering Mdm2 expression. Oncotarget (2019) 10(6):673–83. doi: 10.18632/oncotarget.26586
58. Li M, Zhang S, Ma Y, Yang Y, An R. Role of Hsamir105 during the pathogenesis of paclitaxel resistance and its clinical implication in ovarian cancer. Oncol Rep (2021) 45(5):84. doi: 10.3892/or.2021.8035
59. Lin H, Shen L, Lin Q, Dong C, Maswela B, Illahi GS, et al. Snhg5 enhances paclitaxel sensitivity of ovarian cancer cells through sponging mir-23a. Biomed Pharmacother = Biomed Pharmacotherapie (2020) 123:109711. doi: 10.1016/j.biopha.2019.109711
60. Zhang M, Liu S, Fu C, Wang X, Zhang M, Liu G, et al. Lncrna kb-1471a8.2 overexpression suppresses cell proliferation and migration and antagonizes the paclitaxel resistance of ovarian cancer cells. Cancer Biother Radiopharm (2019) 34(5):316–24. doi: 10.1089/cbr.2018.2698
61. Zheng Y, Li Z, Yang S, Wang Y, Luan Z. Circexoc6b suppresses the proliferation and motility and sensitizes ovarian cancer cells to paclitaxel through mir-376c-3p/Foxo3 axis. Cancer Biother Radiopharm (2020). doi: 10.1089/cbr.2020.3739
62. Zhao H, Wang A, Zhang Z. Lncrna Sdhap1 confers paclitaxel resistance of ovarian cancer by regulating Eif4g2 expression Via mir-4465. J Biochem (2020) 168(2):171–81. doi: 10.1093/jb/mvaa036
63. Huang B, Wei M, Hong L. Long noncoding rna hulc contributes to paclitaxel resistance in ovarian cancer Via mir-137/Itgb8 axis. Open Life Sci (2021) 16(1):667–81. doi: 10.1515/biol-2021-0058
64. Zhu J, Luo JE, Chen Y, Wu Q. Circ_0061140 knockdown inhibits tumorigenesis and improves ptx sensitivity by regulating mir-136/Cbx2 axis in ovarian cancer. J Ovarian Res (2021) 14(1):136. doi: 10.1186/s13048-021-00888-9
65. Wei S, Qi L, Wang L. Overexpression of Circ_Celsr1 facilitates paclitaxel resistance of ovarian cancer by regulating mir-149-5p/Sik2 axis. Anticancer Drugs (2021) 32(5):496–507. doi: 10.1097/CAD.0000000000001058
66. Li LQ, Pan D, Chen Q, Zhang SW, Xie DY, Zheng XL, et al. Sensitization of gastric cancer cells to 5-fu by microrna-204 through targeting the Tgfbr2-mediated epithelial to mesenchymal transition. Cell Physiol Biochem (2018) 47(4):1533–45. doi: 10.1159/000490871
67. Wang CQ. Mir-195 reverses 5-fu resistance through targeting Hmga1 in gastric cancer cells. Eur Rev Med Pharmacol Sci (2019) 23(9):3771–8. doi: 10.26355/eurrev_201905_17803
68. Jiang L, Zhang Y, Guo L, Liu C, Wang P, Ren W. Exosomal microrna-107 reverses chemotherapeutic drug resistance of gastric cancer cells through Hmga2/Mtor/P-gp pathway. BMC Cancer (2021) 21(1):1290. doi: 10.1186/s12885-021-09020-y
69. Wang XY, Zhou YC, Wang Y, Liu YY, Wang YX, Chen DD, et al. Mir-149 contributes to resistance of 5-fu in gastric cancer Via targeting Trem2 and regulating beta-catenin pathway. Biochem Biophys Res Commun (2020) 532(3):329–35. doi: 10.1016/j.bbrc.2020.05.135
70. Hu J, Huang L, Ding Q, Lv J, Chen Z. Long noncoding rna haglr sponges mir-338-3p to promote 5-fu resistance in gastric cancer through targeting the ldha-glycolysis pathway. Cell Biol Int (2022) 46(2):173–84. doi: 10.1002/cbin.11714
71. Jiang L, Zhang Y, Su P, Ma Z, Ye X, Kang W, et al. Long non-coding rna Hnf1a-As1 induces 5-fu resistance of gastric cancer through mir-30b-5p/Eif5a2 pathway. Transl Oncol (2022) 18:101351. doi: 10.1016/j.tranon.2022.101351
72. Fang L, Lv J, Xuan Z, Li B, Li Z, He Z, et al. Circular cpm promotes chemoresistance of gastric cancer Via activating Prkaa2-mediated autophagy. Clin Transl Med (2022) 12(1):e708. doi: 10.1002/ctm2.708
73. Xu G, Li M, Wu J, Qin C, Tao Y, He H. Circular rna Circnrip1 sponges microrna-138-5p to maintain hypoxia-induced resistance to 5-fluorouracil through hif-1alpha-Dependent glucose metabolism in gastric carcinoma. Cancer Manag Res (2020) 12:2789–802. doi: 10.2147/CMAR.S246272
74. Chen Z, Gao YJ, Hou RZ, Ding DY, Song DF, Wang DY, et al. Microrna-206 facilitates gastric cancer cell apoptosis and suppresses cisplatin resistance by targeting Mapk2 signaling pathway. Eur Rev Med Pharmacol Sci (2019) 23(1):171–80. doi: 10.26355/eurrev_201901_16761
75. Ren N, Jiang T, Wang C, Xie S, Xing Y, Piao D, et al. Lncrna Adamts9-As2 inhibits gastric cancer (Gc) development and sensitizes chemoresistant gc cells to cisplatin by regulating mir-223-3p/Nlrp3 axis. Aging (2020) 12(11):11025–41. doi: 10.18632/aging.103314
76. Sun G, Li Z, He Z, Wang W, Wang S, Zhang X, et al. Circular rna Mctp2 inhibits cisplatin resistance in gastric cancer by mir-99a-5p-Mediated induction of Mtmr3 expression. J Exp Clin Cancer Res (2020) 39(1):246. doi: 10.1186/s13046-020-01758-w
77. Zhang J, Zha W, Qian C, Ding A, Mao Z. Circular rna Circ_0001017 sensitizes cisplatin-resistant gastric cancer cells to chemotherapy by the mir-543/Phlpp2 axis. Biochem Genet (2022) 60(2):558–75. doi: 10.1007/s10528-021-10110-6
78. Lee SD, Yu D, Lee DY, Shin HS, Jo JH, Lee YC. Upregulated microrna-193a-3p is responsible for cisplatin resistance in Cd44(+) gastric cancer cells. Cancer Sci (2019) 110(2):662–73. doi: 10.1111/cas.13894
79. Miao X, Liu Y, Fan Y, Wang G, Zhu H. Lncrna bancr attenuates the killing capacity of cisplatin on gastric cancer cell through the Erk1/2 pathway. Cancer Manag Res (2021) 13:287–96. doi: 10.2147/CMAR.S269679
80. Sun H, Wu P, Zhang B, Wu X, Chen W. Mcm3ap-As1 promotes cisplatin resistance in gastric cancer cells Via the mir-138/Foxc1 axis. Oncol Lett (2021) 21(3):211. doi: 10.3892/ol.2021.12472
81. Liu Y, Xu J, Jiang M, Ni L, Ling Y. Circrna donson contributes to cisplatin resistance in gastric cancer cells by regulating mir-802/Bmi1 axis. Cancer Cell Int (2020) 20:261. doi: 10.1186/s12935-020-01358-w
82. Wu Q, Wang H, Liu L, Zhu K, Yu W, Guo J. Hsa_Circ_0001546 acts as a mirna-421 sponge to inhibit the chemoresistance of gastric cancer cells Via Atm/Chk2/P53-dependent pathway. Biochem Biophys Res Commun (2020) 521(2):303–9. doi: 10.1016/j.bbrc.2019.10.117
83. Song W, Qian Y, Zhang MH, Wang H, Wen X, Yang XZ, et al. The long non-coding rna Ddx11-As1 facilitates cell progression and oxaliplatin resistance Via regulating mir-326/Irs1 axis in gastric cancer. Eur Rev Med Pharmacol Sci (2020) 24(6):3049–61. doi: 10.26355/eurrev_202003_20669
84. Zhong Y, Wang D, Ding Y, Tian G, Jiang B. Circular rna Circ_0032821 contributes to oxaliplatin (Oxa) resistance of gastric cancer cells by regulating Sox9 Via mir-515-5p. Biotechnol Lett (2021) 43(2):339–51. doi: 10.1007/s10529-020-03036-3
85. Liu X, Zhou X, Chen Y, Huang Y, He J, Luo H. Mir-186-5p targeting Six1 inhibits cisplatin resistance in non-Small-Cell lung cancer cells (Nsclcs). Neoplasma (2020) 67(1):147–57. doi: 10.4149/neo_2019_190511N420
86. Cui D, Feng Y, Qian R. Up-regulation of microrna mir-101-3p enhances sensitivity to cisplatin Via regulation of small interfering rna (Sirna) anti-human Agt4d and autophagy in non-Small-Cell lung carcinoma (Nsclc). Bioengineered (2021) 12(1):8435–46. doi: 10.1080/21655979.2021.1982274
87. Ye Y, Gu J, Liu P, Wang H, Jiang L, Lei T, et al. Long non-coding rna Spry4-It1 reverses cisplatin resistance by downregulating mpzl-1 Via suppressing emt in nsclc. Onco Targets Ther (2020) 13:2783–93. doi: 10.2147/OTT.S232769
88. Zhu C, Jiang X, Xiao H, Guan J. Circ_0030998 restrains cisplatin resistance through mediating mir-1323/Pdcd4 axis in non-small cell lung cancer. Biochem Genet (2022). doi: 10.1007/s10528-022-10220-9
89. Sun B, Hu N, Cong D, Chen K, Li J. Microrna-25-3p promotes cisplatin resistance in non-Small-Cell lung carcinoma (Nsclc) through adjusting Pten/Pi3k/Akt route. Bioengineered (2021) 12(1):3219–28. doi: 10.1080/21655979.2021.1939577
90. Ge P, Cao L, Zheng M, Yao Y, Wang W, Chen X. Lncrna Snhg1 contributes to the cisplatin resistance and progression of nsclc Via mir-330-5p/Dclk1 axis. Exp Mol Pathol (2021) 120:104633. doi: 10.1016/j.yexmp.2021.104633
91. Xiao S, Sun L, Ruan B, Li J, Chen J, Xiong J, et al. Long non-coding rna Linc01224 promotes progression and cisplatin resistance in non-small lung cancer by sponging mir-2467. Pulm Pharmacol Ther (2021) 70:102070. doi: 10.1016/j.pupt.2021.102070
92. Zhong Y, Lin H, Li Q, Liu C, Shen J. Circrna_100565 contributes to cisplatin resistance of nsclc cells by regulating proliferation, apoptosis and autophagy Via mir-337-3p/Adam28 axis. Cancer biomark (2021) 30(2):261–73. doi: 10.3233/CBM-201705
93. Xu F, Ye ML, Zhang YP, Li WJ, Li MT, Wang HZ, et al. Microrna-375-3p enhances chemosensitivity to 5-fluorouracil by targeting thymidylate synthase in colorectal cancer. Cancer Sci (2020) 111(5):1528–41. doi: 10.1111/cas.14356
94. Jiang Z, Li L, Hou Z, Liu W, Wang H, Zhou T, et al. Lncrna Hand2-As1 inhibits 5-fluorouracil resistance by modulating mir-20a/Pdcd4 axis in colorectal cancer. Cell Signalling (2020) 66:109483. doi: 10.1016/j.cellsig.2019.109483
95. Ren TJ, Liu C, Hou JF, Shan FX. Circddx17 reduces 5-fluorouracil resistance and hinders tumorigenesis in colorectal cancer by regulating mir-31-5p/Kank1 axis. Eur Rev Med Pharmacol Sci (2020) 24(4):1743–54. doi: 10.26355/eurrev_202002_20351
96. Wu S, Zhou Y, Liu P, Zhang H, Wang W, Fang Y, et al. Microrna-29b-3p promotes 5-fluorouracil resistance <Em>Via</Em> suppressing Traf5-mediated necroptosis in human colorectal cancer. Eur J Histochem (2021) 65(2):3247. doi: 10.4081/ejh.2021.3247
97. Ma YN, Hong YG, Yu GY, Jiang SY, Zhao BL, Guo A, et al. Lncrna Lbx2-As1 promotes colorectal cancer progression and 5-fluorouracil resistance. Cancer Cell Int (2021) 21(1):501. doi: 10.1186/s12935-021-02209-y
98. He X, Ma J, Zhang M, Cui J, Yang H. Circ_0007031 enhances tumor progression and promotes 5-fluorouracil resistance in colorectal cancer through regulating mir-133b/Abcc5 axis. Cancer biomark (2020) 29(4):531–42. doi: 10.3233/CBM-200023
99. Wu YZ, Lin HY, Zhang Y, Chen WF. Mir-200b-3p mitigates oxaliplatin resistance Via targeting Tubb3 in colorectal cancer. J Gene Med (2020) 22(7):e3178. doi: 10.1002/jgm.3178
100. Xu Y, Qiu A, Peng F, Tan X, Wang J, Gong X. Exosomal transfer of circular rna Fbxw7 ameliorates the chemoresistance to oxaliplatin in colorectal cancer by sponging mir-18b-5p. Neoplasma (2021) 68(1):108–18. doi: 10.4149/neo_2020_200417N414
101. Qian XL, Zhou F, Xu S, Jiang J, Chen ZP, Wang SK, et al. Mir-454-3p promotes oxaliplatin resistance by targeting pten in colorectal cancer. Front Oncol (2021) 11:638537. doi: 10.3389/fonc.2021.638537
102. Gao R, Fang C, Xu J, Tan H, Li P, Ma L. Lncrna Cacs15 contributes to oxaliplatin resistance in colorectal cancer by positively regulating Abcc1 through sponging mir-145. Arch Biochem Biophys (2019) 663:183–91. doi: 10.1016/j.abb.2019.01.005
103. Li TT, Mou J, Pan YJ, Huo FC, Du WQ, Liang J, et al. Microrna-138-1-3p sensitizes sorafenib to hepatocellular carcinoma by targeting Pak5 mediated beta-Catenin/Abcb1 signaling pathway. J BioMed Sci (2021) 28(1):56. doi: 10.1186/s12929-021-00752-4
104. Dong ZB, Wu HM, He YC, Huang ZT, Weng YH, Li H, et al. Mirna-124-3p.1 sensitizes hepatocellular carcinoma cells to sorafenib by regulating Foxo3a by targeting Akt2 and Sirt1. Cell Death Dis (2022) 13(1):35. doi: 10.1038/s41419-021-04491-0
105. Sui C, Dong Z, Yang C, Zhang M, Dai B, Geng L, et al. Lncrna Foxd2-As1 as a competitive endogenous rna against mir-150-5p reverses resistance to sorafenib in hepatocellular carcinoma. J Cell Mol Med (2019) 23(9):6024–33. doi: 10.1111/jcmm.14465
106. Tan W, Lin Z, Chen X, Li W, Zhu S, Wei Y, et al. Mir-126-3p contributes to sorafenib resistance in hepatocellular carcinoma Via downregulating Spred1. Ann Trans Med (2021) 9(1):38. doi: 10.21037/atm-20-2081
107. Liu Y, Chen L, Yuan H, Guo S, Wu G. Lncrna dancr promotes sorafenib resistance Via activation of il-6/Stat3 signaling in hepatocellular carcinoma cells. Onco Targets Ther (2020) 13:1145–57. doi: 10.2147/OTT.S229957
108. Weng H, Zeng L, Cao L, Chen T, Li Y, Xu Y, et al. Circfoxm1 contributes to sorafenib resistance of hepatocellular carcinoma cells by regulating Mecp2 Via mir-1324. Mol Ther Nucleic Acids (2021) 23:811–20. doi: 10.1016/j.omtn.2020.12.019
109. Yang Y, Yang Z, Zhang R, Jia C, Mao R, Mahati S, et al. Mir-27a-3p enhances the cisplatin sensitivity in hepatocellular carcinoma cells through inhibiting Pi3k/Akt pathway. Biosci Rep (2021) 41(12):BSR20192007. doi: 10.1042/BSR20192007
110. Zhao P, Cui X, Zhao L, Liu L, Wang D. Overexpression of growth-Arrest-Specific transcript 5 improved cisplatin sensitivity in hepatocellular carcinoma through sponging mir-222. DNA Cell Biol (2020) 39(4):724–32. doi: 10.1089/dna.2019.5282
111. Yang Y, Shi L, Zhang D, Wu D, An Y, Zhang Y, et al. Long non-coding rna Fgd5-As1 contributes to cisplatin resistance in hepatocellular carcinoma Via sponging microrna-153-3p by upregulating twinfilin actin binding protein 1 (Twf1). Bioengineered (2021) 12(1):6713–23. doi: 10.1080/21655979.2021.1971484
112. Li P, Song R, Yin F, Liu M, Liu H, Ma S, et al. Circmrps35 promotes malignant progression and cisplatin resistance in hepatocellular carcinoma. Mol Ther (2022) 30(1):431–47. doi: 10.1016/j.ymthe.2021.08.027
113. Li D, Wang X, Yang M, Kan Q, Duan Z. Mir3609 sensitizes breast cancer cells to adriamycin by blocking the programmed death-ligand 1 immune checkpoint. Exp Cell Res (2019) 380(1):20–8. doi: 10.1016/j.yexcr.2019.03.025
114. Liang Y, Song X, Li Y, Su P, Han D, Ma T, et al. Circkdm4c suppresses tumor progression and attenuates doxorubicin resistance by regulating mir-548p/Pbld axis in breast cancer. Oncogene (2019) 38(42):6850–66. doi: 10.1038/s41388-019-0926-z
115. Yin Y, Wang X, Li T, Ren Q, Li L, Sun X, et al. Microrna-221 promotes breast cancer resistance to adriamycin Via modulation of Pten/Akt/Mtor signaling. Cancer Med (2020) 9(4):1544–52. doi: 10.1002/cam4.2817
116. Zheng R, Jia J, Guan L, Yuan H, Liu K, Liu C, et al. Long noncoding rna lnc-Loc645166 promotes adriamycin resistance Via nf-Kappab/Gata3 axis in breast cancer. Aging (2020) 12(10):8893–912. doi: 10.18632/aging.103012
117. Chen J, Shi P, Zhang J, Li Y, Ma J, Zhang Y, et al. Circrna_0044556 diminishes the sensitivity of triplenegative breast cancer cells to adriamycin by sponging Mir145 and regulating nras. Mol Med Rep (2022) 25(2):51. doi: 10.3892/mmr.2021.12567
118. Shi YF, Lu H, Wang HB. Downregulated lncrna Adamts9-As2 in breast cancer enhances tamoxifen resistance by activating microrna-130a-5p. Eur Rev Med Pharmacol Sci (2019) 23(4):1563–73. doi: 10.26355/eurrev_201902_17115
119. Li H, Li Q, He S. Hsa_Circ_0025202 suppresses cell tumorigenesis and tamoxifen resistance Via mir-197-3p/Hipk3 axis in breast cancer. World J Surg Oncol (2021) 19(1):39. doi: 10.1186/s12957-021-02149-x
120. Han X, Li Q, Liu C, Wang C, Li Y. Overexpression mir-24-3p repressed bim expression to confer tamoxifen resistance in breast cancer. J Cell Biochem (2019) 120(8):12966–76. doi: 10.1002/jcb.28568
121. Liu Y, Li M, Yu H, Piao H. Lncrna cytor promotes tamoxifen resistance in breast cancer cells Via sponging Mir125a5p. Int J Mol Med (2020) 45(2):497–509. doi: 10.3892/ijmm.2019.4428
122. Shi F, Su J, Liu Z, Wang J, Wang T. Mir-144 reverses cisplatin resistance in cervical cancer Via targeting Lhx2. J Cell Biochem (2019) 120(9):15018–26. doi: 10.1002/jcb.28763
123. Hou H, Yu R, Zhao H, Yang H, Hu Y, Hu Y, et al. Lncrna Otud6b-As1 induces cisplatin resistance in cervical cancer cells through up-regulating cyclin D2 Via mir-206. Front Oncol (2021) 11:777220. doi: 10.3389/fonc.2021.777220
124. Shen Z, Zhou L, Zhang C, Xu J. Reduction of circular rna Foxo3 promotes prostate cancer progression and chemoresistance to docetaxel. Cancer Lett (2020) 468:88–101. doi: 10.1016/j.canlet.2019.10.006
125. Zhang H, Li M, Zhang J, Shen Y, Gui Q. Exosomal circ-xiap promotes docetaxel resistance in prostate cancer by regulating mir-1182/Tpd52 axis. Drug Des Devel Ther (2021) 15:1835–49. doi: 10.2147/DDDT.S300376
126. Liu A, Zhou Y, Zhao T, Tang X, Zhou B, Xu J. Mirna-3662 reverses the gemcitabine resistance in pancreatic cancer through regulating the tumor metabolism. Cancer Chemother Pharmacol (2021) 88(2):343–57. doi: 10.1007/s00280-021-04289-z
127. Yu S, Wang M, Zhang H, Guo X, Qin R. Circ_0092367 inhibits emt and gemcitabine resistance in pancreatic cancer Via regulating the mir-1206/Esrp1 axis. Genes (2021) 12(11):1701. doi: 10.3390/genes12111701
128. Wu Y, Xu W, Yang Y, Zhang Z. Mirna-93-5p promotes gemcitabine resistance in pancreatic cancer cells by targeting the pten-mediated Pi3k/Akt signaling pathway. Ann Clin Lab Sci (2021) 51(3):310–20.
129. Zhou C, Yi C, Yi Y, Qin W, Yan Y, Dong X, et al. Lncrna Pvt1 promotes gemcitabine resistance of pancreatic cancer Via activating Wnt/Beta-catenin and autophagy pathway through modulating the mir-619-5p/Pygo2 and mir-619-5p/Atg14 axes. Mol Cancer (2020) 19(1):118. doi: 10.1186/s12943-020-01237-y
130. Liu Y, Xia L, Dong L, Wang J, Xiao Q, Yu X, et al. Circhipk3 promotes gemcitabine (Gem) resistance in pancreatic cancer cells by sponging mir-330-5p and targets Rassf1. Cancer Manag Res (2020) 12:921–9. doi: 10.2147/CMAR.S239326
131. Luo G, Zhang Y, Wu Z, Zhang L, Liang C, Chen X. Exosomal Linc00355 derived from cancer-associated fibroblasts promotes bladder cancer cell resistance to cisplatin by regulating mir-34b-5p/Abcb1 axis. Acta Biochim Biophys Sin (Shanghai) (2021) 53(5):558–66. doi: 10.1093/abbs/gmab023
132. Sun M, Liu X, Zhao W, Zhang B, Deng P. Circ_0058063 contributes to cisplatin-resistance of bladder cancer cells by upregulating B2m through acting as rna sponges for mir-335-5p. BMC Cancer (2022) 22(1):313. doi: 10.1186/s12885-022-09419-1
133. Sekino Y, Sakamoto N, Sentani K, Oue N, Teishima J, Matsubara A, et al. Mir-130b promotes sunitinib resistance through regulation of pten in renal cell carcinoma. Oncology (2019) 97(3):164–72. doi: 10.1159/000500605
134. Huang KB, Pan YH, Shu GN, Yao HH, Liu X, Zhou M, et al. Circular rna Circsnx6 promotes sunitinib resistance in renal cell carcinoma through the mir-1184/Gpcpd1/ lysophosphatidic acid axis. Cancer Lett (2021) 523:121–34. doi: 10.1016/j.canlet.2021.10.003
135. Yuan L, Xu ZY, Ruan SM, Mo S, Qin JJ, Cheng XD. Long non-coding rnas towards precision medicine in gastric cancer: Early diagnosis, treatment, and drug resistance. Mol Cancer (2020) 19(1):96. doi: 10.1186/s12943-020-01219-0
136. Liu Y, Ao X, Ding W, Ponnusamy M, Wu W, Hao X, et al. Critical role of Foxo3a in carcinogenesis. Mol Cancer (2018) 17(1):104. doi: 10.1186/s12943-018-0856-3
137. Pang X, Zhou Z, Yu Z, Han L, Lin Z, Ao X, et al. Foxo3a-dependent mir-633 regulates chemotherapeutic sensitivity in gastric cancer by targeting fas-associated death domain. RNA Biol (2019) 16(2):233–48. doi: 10.1080/15476286.2019.1565665
138. Yang J, Hai J, Dong X, Zhang M, Duan S. Microrna-92a-3p enhances cisplatin resistance by regulating kruppel-like factor 4-mediated cell apoptosis and epithelial-to-Mesenchymal transition in cervical cancer. Front Pharmacol (2021) 12:783213. doi: 10.3389/fphar.2021.783213
139. Suraweera CD, Banjara S, Hinds MG, Kvansakul M. Metazoans and intrinsic apoptosis: An evolutionary analysis of the bcl-2 family. Int J Mol Sci (2022) 23(7):3691. doi: 10.3390/ijms23073691
140. Warren CFA, Wong-Brown MW, Bowden NA. Bcl-2 family isoforms in apoptosis and cancer. Cell Death Dis (2019) 10(3):177. doi: 10.1038/s41419-019-1407-6
141. Zhong L, Liu X, Wang L, Liu Y, Zhang D, Zhao Y. Microrna-625-3p improved proliferation and involved chemotherapy resistance Via targeting pten in high grade ovarian serous carcinoma. J Ovarian Res (2022) 15(1):7. doi: 10.1186/s13048-021-00939-1
142. Ashofteh N, Amini R, Molaee N, Karami H, Baazm M. Mirna-mediated knock-down of bcl-2 and mcl-1 increases fludarabine-sensitivity in cll-cii cells. Asian Pac J Cancer Prev (2021) 22(7):2191–8. doi: 10.31557/APJCP.2021.22.7.2191
143. Sun Y, Peng YB, Ye LL, Ma LX, Zou MY, Cheng ZG. Propofol inhibits proliferation and cisplatin resistance in ovarian cancer cells through regulating the Microrna374a/Forkhead box O1 signaling axis. Mol Med Rep (2020) 21(3):1471–80. doi: 10.3892/mmr.2020.10943
144. Hiremath IS, Goel A, Warrier S, Kumar AP, Sethi G, Garg M. The multidimensional role of the Wnt/Beta-catenin signaling pathway in human malignancies. J Cell Physiol (2022) 237(1):199–238. doi: 10.1002/jcp.30561
145. Han H, Li Y, Qin W, Wang L, Yin H, Su B, et al. Mir-199b-3p contributes to acquired resistance to cetuximab in colorectal cancer by targeting Crim1 Via Wnt/Beta-catenin signaling. Cancer Cell Int (2022) 22(1):42. doi: 10.1186/s12935-022-02460-x
146. Liu HR, Zhao J. Effect and mechanism of mir-217 on drug resistance, invasion and metastasis of ovarian cancer cells through a regulatory axis of Cul4b gene Silencing/Inhibited Wnt/Beta-catenin signaling pathway activation. Eur Rev Med Pharmacol Sci (2021) 25(1):94–107. doi: 10.26355/eurrev_202101_24353
147. Zhang Y, Liang S, Xiao B, Hu J, Pang Y, Liu Y, et al. Mir-323a regulates Erbb3/Egfr and blocks gefitinib resistance acquisition in colorectal cancer. Cell Death Dis (2022) 13(3):256. doi: 10.1038/s41419-022-04709-9
148. Liu H, Song M, Sun X, Zhang X, Miao H, Wang Y. T-Box transcription factor Tbx1, targeted by microrna-6727-5p, inhibits cell growth and enhances cisplatin chemosensitivity of cervical cancer cells through akt and mapk pathways. Bioengineered (2021) 12(1):565–77. doi: 10.1080/21655979.2021.1880732
149. Yuan J, Zhang Q, Wu S, Yan S, Zhao R, Sun Y, et al. Mirna-223-3p modulates ibrutinib resistance through regulation of the Chuk/Nf-kappab signaling pathway in mantle cell lymphoma. Exp Hematol (2021) 103:52–9.e2. doi: 10.1016/j.exphem.2021.08.010
150. Adhikari S, Bhattacharya A, Adhikary S, Singh V, Gadad SS, Roy S, et al. The paradigm of drug resistance in cancer: An epigenetic perspective. Biosci Rep (2022) 42(4):BSR20211812. doi: 10.1042/BSR20211812
151. Robey RW, Pluchino KM, Hall MD, Fojo AT, Bates SE, Gottesman MM. Revisiting the role of abc transporters in multidrug-resistant cancer. Nat Rev Cancer (2018) 18(7):452–64. doi: 10.1038/s41568-018-0005-8
152. Gomes BC, Rueff J, Rodrigues AS. Micrornas and cancer drug resistance. Methods Mol Biol (2016) 1395:137–62. doi: 10.1007/978-1-4939-3347-1_9
153. Zou Z, Zou R, Zong D, Shi Y, Chen J, Huang J, et al. Mir-495 sensitizes mdr cancer cells to the combination of doxorubicin and taxol by inhibiting Mdr1 expression. J Cell Mol Med (2017) 21(9):1929–43. doi: 10.1111/jcmm.13114
154. Bao J, Xu Y, Wang Q, Zhang J, Li Z, Li D, et al. Mir-101 alleviates chemoresistance of gastric cancer cells by targeting Anxa2. Biomed Pharmacother = Biomed Pharmacotherapie (2017) 92:1030–7. doi: 10.1016/j.biopha.2017.06.011
155. Tian J, Xu YY, Li L, Hao Q. Mir-490-3p sensitizes ovarian cancer cells to cisplatin by directly targeting Abcc2. Am J Transl Res (2017) 9(3):1127–38.
156. Tsai HC, Chang AC, Tsai CH, Huang YL, Gan L, Chen CK, et al. Ccn2 promotes drug resistance in osteosarcoma by enhancing Abcg2 expression. J Cell Physiol (2019) 234(6):9297–307. doi: 10.1002/jcp.27611
157. Amponsah PS, Fan P, Bauer N, Zhao Z, Gladkich J, Fellenberg J, et al. Microrna-210 overexpression inhibits tumor growth and potentially reverses gemcitabine resistance in pancreatic cancer. Cancer Lett (2017) 388:107–17. doi: 10.1016/j.canlet.2016.11.035
158. Li Y, Gong P, Hou JX, Huang W, Ma XP, Wang YL, et al. Mir-34a regulates multidrug resistance Via positively modulating Oaz2 signaling in colon cancer cells. J Immunol Res (2018) 2018:7498514. doi: 10.1155/2018/7498514
159. Gajda E, Godlewska M, Mariak Z, Nazaruk E, Gawel D. Combinatory treatment with mir-7-5p and drug-loaded cubosomes effectively impairs cancer cells. Int J Mol Sci (2020) 21(14):5039. doi: 10.3390/ijms21145039
160. Li R, Xu T, Wang H, Wu N, Liu F, Jia X, et al. Dysregulation of the mir-325-3p/Dpagt1 axis supports hbv-positive hcc chemoresistance. Biochem Biophys Res Commun (2019) 519(2):358–65. doi: 10.1016/j.bbrc.2019.08.116
161. Wang M, Yu F, Chen X, Li P, Wang K. The underlying mechanisms of noncoding rnas in the chemoresistance of hepatocellular carcinoma. Mol Ther Nucleic Acids (2020) 21:13–27. doi: 10.1016/j.omtn.2020.05.011
162. Shahverdi M, Hajiasgharzadeh K, Sorkhabi AD, Jafarlou M, Shojaee M, Jalili Tabrizi N, et al. The regulatory role of autophagy-related mirnas in lung cancer drug resistance. Biomed Pharmacother = Biomed Pharmacotherapie (2022) 148:112735. doi: 10.1016/j.biopha.2022.112735
163. Li H, Lei Y, Li S, Li F, Lei J. Microrna-20a-5p inhibits the autophagy and cisplatin resistance in ovarian cancer Via regulating Dnmt3b-mediated DNA methylation of Rbp1. Reprod Toxicol (2022) 109:93–100. doi: 10.1016/j.reprotox.2021.12.011
164. Li T, Zhang H, Wang Z, Gao S, Zhang X, Zhu H, et al. The regulation of autophagy by the mir-199a-5p/P62 axis was a potential mechanism of small cell lung cancer cisplatin resistance. Cancer Cell Int (2022) 22(1):120. doi: 10.1186/s12935-022-02505-1
165. Zhao L, Chen H, Zhang Q, Ma J, Hu H, Xu L. Atf4-mediated microrna-145/Hdac4/P53 axis affects resistance of colorectal cancer cells to 5-fluorouracil by regulating autophagy. Cancer Chemother Pharmacol (2022) 89(5):595–607. doi: 10.1007/s00280-021-04393-0
166. Liu Y. Targeting the non-canonical akt-Foxo3a axis: A potential therapeutic strategy for oral squamous cell carcinoma. EBioMedicine (2019) 49:6–8. doi: 10.1016/j.ebiom.2019.10.020
167. Ao X, Ding W, Zhang Y, Ding D, Liu Y. Tcf21: A critical transcription factor in health and cancer. J Mol Med (Berl) (2020) 98(8):1055–68. doi: 10.1007/s00109-020-01934-7
168. Saha T, Lukong KE. Breast cancer stem-like cells in drug resistance: A review of mechanisms and novel therapeutic strategies to overcome drug resistance. Front Oncol (2022) 12:856974. doi: 10.3389/fonc.2022.856974
169. Zhang L, Guo X, Zhang D, Fan Y, Qin L, Dong S, et al. Upregulated mir-132 in Lgr5(+) gastric cancer stem cell-like cells contributes to cisplatin-resistance Via Sirt1/Creb/Abcg2 signaling pathway. Mol Carcinog (2017) 56(9):2022–34. doi: 10.1002/mc.22656
170. Feng X, Jiang J, Shi S, Xie H, Zhou L, Zheng S. Knockdown of mir-25 increases the sensitivity of liver cancer stem cells to trail-induced apoptosis Via Pten/Pi3k/Akt/Bad signaling pathway. Int J Oncol (2016) 49(6):2600–10. doi: 10.3892/ijo.2016.3751
171. Ni H, Qin H, Sun C, Liu Y, Ruan G, Guo Q, et al. Mir-375 reduces the stemness of gastric cancer cells through triggering ferroptosis. Stem Cell Res Ther (2021) 12(1):325. doi: 10.1186/s13287-021-02394-7
172. Niu G, Hao J, Sheng S, Wen F. Role of T-box genes in cancer, epithelial-mesenchymal transition, and cancer stem cells. J Cell Biochem (2022) 123(2):215–30. doi: 10.1002/jcb.30188
173. Liu Y, Ding W, Ge H, Ponnusamy M, Wang Q, Hao X, et al. Foxk transcription factors: Regulation and critical role in cancer. Cancer Lett (2019) 458:1–12. doi: 10.1016/j.canlet.2019.05.030
174. Hirao A, Sato Y, Tanaka H, Nishida K, Tomonari T, Hirata M, et al. Mir-125b-5p is involved in sorafenib resistance through ataxin-1-Mediated epithelial-mesenchymal transition in hepatocellular carcinoma. Cancers (2021) 13(19):4917. doi: 10.3390/cancers13194917
175. Chaudhary AK, Mondal G, Kumar V, Kattel K, Mahato RI. Chemosensitization and inhibition of pancreatic cancer stem cell proliferation by overexpression of microrna-205. Cancer Lett (2017) 402:1–8. doi: 10.1016/j.canlet.2017.05.007
176. Cao L, Wan Q, Li F, Tang CE. Mir-363 inhibits cisplatin chemoresistance of epithelial ovarian cancer by regulating snail-induced epithelial-mesenchymal transition. BMB Rep (2018) 51(9):456–61. doi: 10.5483/BMBRep.2018.51.9.104
177. Singh N, Baby D, Rajguru JP, Patil PB, Thakkannavar SS, Pujari VB. Inflammation and cancer. Ann Afr Med (2019) 18(3):121–6. doi: 10.4103/aam.aam_56_18
178. Dong C. Cytokine regulation and function in T cells. Annu Rev Immunol (2021) 39:51–76. doi: 10.1146/annurev-immunol-061020-053702
179. Yan ZX, Zheng Z, Xue W, Zhao MZ, Fei XC, Wu LL, et al. Microrna181a is overexpressed in T-cell Leukemia/Lymphoma and related to chemoresistance. BioMed Res Int (2015) 2015:197241. doi: 10.1155/2015/197241
180. Ning T, Li J, He Y, Zhang H, Wang X, Deng T, et al. Exosomal mir-208b related with oxaliplatin resistance promotes treg expansion in colorectal cancer. Mol Ther (2021) 29(9):2723–36. doi: 10.1016/j.ymthe.2021.04.028
181. Xu S, Tao Z, Hai B, Liang H, Shi Y, Wang T, et al. Mir-424(322) reverses chemoresistance Via T-cell immune response activation by blocking the pd-L1 immune checkpoint. Nat Commun (2016) 7:11406. doi: 10.1038/ncomms11406
182. Sheng Q, Zhang Y, Wang Z, Ding J, Song Y, Zhao W. Cisplatin-mediated down-regulation of mir-145 contributes to up-regulation of pd-L1 Via the c-myc transcription factor in cisplatin-resistant ovarian carcinoma cells. Clin Exp Immunol (2020) 200(1):45–52. doi: 10.1111/cei.13406
183. Chae Y, Roh J, Kim W. The roles played by long non-coding rnas in glioma resistance. Int J Mol Sci (2021) 22(13):6834. doi: 10.3390/ijms22136834
184. Li W, Nie A, Jin L, Cui Y, Xie N, Liang G. Long non-coding rna terminal differentiation-induced non-coding rna regulates cisplatin resistance of choroidal melanoma by positively modulating extracellular signal-regulated kinase 2 Via sponging microrna-19b-3p. Bioengineered (2022) 13(2):3422–33. doi: 10.1080/21655979.2021.2014618
185. Zhou D, Gu J, Wang Y, Luo B, Feng M, Wang X. Long noncoding rna Ccat2 reduces chemosensitivity to 5-fluorouracil in breast cancer cells by activating the mtor axis. J Cell Mol Med (2022) 26(5):1392–401. doi: 10.1111/jcmm.17041
186. Guo JL, Tang T, Li JH, Yang YH, Zhang L, Quan Y. Lncrna heih enhances paclitaxel-tolerance of endometrial cancer cells Via activation of mapk signaling pathway. Pathol Oncol Res (2020) 26(3):1757–66. doi: 10.1007/s12253-019-00718-w
187. Zhu Y, Zhou B, Hu X, Ying S, Zhou Q, Xu W, et al. Lncrna Linc00942 promotes chemoresistance in gastric cancer by suppressing Msi2 degradation to enhance c-myc mrna stability. Clin Transl Med (2022) 12(1):e703. doi: 10.1002/ctm2.703
188. Lu Q, Wang L, Gao Y, Zhu P, Li L, Wang X, et al. Lncrna Apoc1p1-3 promoting anoikis-resistance of breast cancer cells. Cancer Cell Int (2021) 21(1):232. doi: 10.1186/s12935-021-01916-w
189. Zhao Y, Hong L. Lncrna-prlb confers paclitaxel resistance of ovarian cancer cells by regulating Rsf1/Nf-kappab signaling pathway. Cancer Biother Radiopharm (2021) 36(2):202–10. doi: 10.1089/cbr.2019.3363
190. He J, Zhu S, Liang X, Zhang Q, Luo X, Liu C, et al. Lncrna as a multifunctional regulator in cancer multi-drug resistance. Mol Biol Rep (2021) 48(8):1–15. doi: 10.1007/s11033-021-06603-7
191. Zhang K, Chen J, Li C, Yuan Y, Fang S, Liu W, et al. Exosome-mediated transfer of Snhg7 enhances docetaxel resistance in lung adenocarcinoma. Cancer Lett (2022) 526:142–54. doi: 10.1016/j.canlet.2021.10.029
192. Xia C, Li Q, Cheng X, Wu T, Gao P, Gu Y. Insulin-like growth factor 2 mrna-binding protein 2-stabilized long non-coding rna taurine up-regulated gene 1 (Tug1) promotes cisplatin-resistance of colorectal cancer Via modulating autophagy. Bioengineered (2022) 13(2):2450–69. doi: 10.1080/21655979.2021.2012918
193. Chen L, Sun L, Dai X, Li T, Yan X, Zhang Y, et al. Lncrna crnde promotes Atg4b-mediated autophagy and alleviates the sensitivity of sorafenib in hepatocellular carcinoma cells. Front Cell Dev Biol (2021) 9:687524. doi: 10.3389/fcell.2021.687524
194. Xu T, Jiang L, Wang Z. The progression of Hmgb1-induced autophagy in cancer biology. Onco Targets Ther (2019) 12:365–77. doi: 10.2147/OTT.S185876
195. Chen L, Xu Z, Zhao J, Zhai X, Li J, Zhang Y, et al. H19/Mir-107/Hmgb1 axis sensitizes laryngeal squamous cell carcinoma to cisplatin by suppressing autophagy in vitro and in vivo. Cell Biol Int (2021) 45(3):674–85. doi: 10.1002/cbin.11520
196. Liu K, Gao L, Ma X, Huang JJ, Chen J, Zeng L, et al. Long non-coding rnas regulate drug resistance in cancer. Mol Cancer (2020) 19(1):54. doi: 10.1186/s12943-020-01162-0
197. Chen Z, Pan T, Jiang D, Jin L, Geng Y, Feng X, et al. The lncrna-Gas5/Mir-221-3p/Dkk2 axis modulates Abcb1-mediated adriamycin resistance of breast cancer Via the Wnt/Beta-catenin signaling pathway. Mol Ther Nucleic Acids (2020) 19:1434–48. doi: 10.1016/j.omtn.2020.01.030
198. Liu Y, Li H, Zhao Y, Li D, Zhang Q, Fu J, et al. Knockdown of Adora2a antisense rna 1 inhibits cell proliferation and enhances imatinib sensitivity in chronic myeloid leukemia. Bioengineered (2022) 13(2):2296–307. doi: 10.1080/21655979.2021.2024389
199. Wang W, Han S, Gao W, Feng Y, Li K, Wu D. Long noncoding rna Kcnq1ot1 confers gliomas resistance to temozolomide and enhances cell growth by retrieving Pim1 from mir-761. Cell Mol Neurobiol (2022) 42(3):695–708. doi: 10.1007/s10571-020-00958-4
200. Shen P, Cheng Y. Long noncoding rna lncarsr confers resistance to adriamycin and promotes osteosarcoma progression. Cell Death Dis (2020) 11(5):362. doi: 10.1038/s41419-020-2573-2
201. Li Y, Liu Y, Chen G, Liu H, Wu Y, Liu J, et al. Hottip is upregulated in esophageal cancer and triggers the drug resistance. J BUON (2021) 26(3):1056–61.
202. Xie L, Cui G, Li T. Long noncoding rna Cbr3-As1 promotes stem-like properties and oxaliplatin resistance of colorectal cancer by sponging mir-145-5p. J Oncol (2022) 2022:2260211. doi: 10.1155/2022/2260211
203. Liu S, Sun Y, Hou Y, Yang L, Wan X, Qin Y, et al. A novel lncrna ropm-mediated lipid metabolism governs breast cancer stem cell properties. J Hematol Oncol (2021) 14(1):178. doi: 10.1186/s13045-021-01194-z
204. Cheng D, Fan J, Qin K, Zhou Y, Yang J, Ma Y, et al. Lncrna Snhg7 regulates mesenchymal stem cell through the Notch1/Jagged1/Hes-1 signaling pathway and influences folfirinox resistance in pancreatic cancer. Front Oncol (2021) 11:719855. doi: 10.3389/fonc.2021.719855
205. Liu S, Bu X, Kan A, Luo L, Xu Y, Chen H, et al. Sp1-induced lncrna dubr promotes stemness and oxaliplatin resistance of hepatocellular carcinoma Via E2f1-Cip2a feedback. Cancer Lett (2022) 528:16–30. doi: 10.1016/j.canlet.2021.12.026
206. Zhang J, Zhao X, Ma X, Yuan Z, Hu M. Kcnq1ot1 contributes to sorafenib resistance and programmed Deathligand1mediated immune escape Via sponging Mir506 in hepatocellular carcinoma cells. Int J Mol Med (2020) 46(5):1794–804. doi: 10.3892/ijmm.2020.4710
207. Zhang W, Xin J, Lai J, Zhang W. Lncrna Linc00184 promotes docetaxel resistance and immune escape Via mir-105-5p/Pd-L1 axis in prostate cancer. Immunobiology (2022) 227(1):152163. doi: 10.1016/j.imbio.2021.152163
208. Xu YJ, Zhao JM, Ni XF, Wang W, Hu WW, Wu CP. Lncrna Hcg18 suppresses Cd8(+) T cells to confer resistance to cetuximab in colorectal cancer Via mir-20b-5p/Pd-L1 axis. Epigenomics (2021) 13(16):1281–97. doi: 10.2217/epi-2021-0130
209. Li H, Ma X, Yang D, Suo Z, Dai R, Liu C. Pcat-1 contributes to cisplatin resistance in gastric cancer through epigenetically silencing pten Via recruiting Ezh2. J Cell Biochem (2020) 121(2):1353–61. doi: 10.1002/jcb.29370
210. Si X, Zang R, Zhang E, Liu Y, Shi X, Zhang E, et al. Lncrna H19 confers chemoresistance in eralpha-positive breast cancer through epigenetic silencing of the pro-apoptotic gene bik. Oncotarget (2016) 7(49):81452–62. doi: 10.18632/oncotarget.13263
211. Lin K, Jiang H, Zhuang SS, Qin YS, Qiu GD, She YQ, et al. Long noncoding rna Linc00261 induces chemosensitization to 5-fluorouracil by mediating methylation-dependent repression of dpyd in human esophageal cancer. FASEB J (2019) 33(2):1972–88. doi: 10.1096/fj.201800759R
212. Li Z, Li M, Xia P, Lu Z. Hottip mediated therapy resistance in glioma cells involves regulation of emt-related mir-10b. Front Oncol (2022) 12:873561. doi: 10.3389/fonc.2022.873561
213. Zhang W, Wu Q, Liu Y, Wang X, Ma C, Zhu W. Lncrna hotair promotes chemoresistance by facilitating epithelial to mesenchymal transition through mir-29b/Pten/Pi3k signaling in cervical cancer. Cells Tissues Organs (2022) 211(1):16–29. doi: 10.1159/000519844
214. Zhao X, Wang J, Zhu R, Zhang J, Zhang Y. Dlx6-As1 activated by H3k4me1 enhanced secondary cisplatin resistance of lung squamous cell carcinoma through modulating mir-181a-5p/Mir-382-5p/Celf1 axis. Sci Rep (2021) 11(1):21014. doi: 10.1038/s41598-021-99555-8
215. Sun J, Zheng X, Wang B, Cai Y, Zheng L, Hu L, et al. Lncrna limt (Linc01089) contributes to sorafenib chemoresistance Via regulation of mir-665 and epithelial to mesenchymal transition in hepatocellular carcinoma cells. Acta Biochim Biophys Sin (Shanghai) (2022) 54(2):261–70. doi: 10.3724/abbs.2021019
216. Yang J, Ma Q, Zhang M, Zhang W. Lncrna cytor drives l-ohp resistance and facilitates the epithelial-mesenchymal transition of colon carcinoma cells Via modulating mir-378a-5p/Serpine1. Cell Cycle (2021) 20(14):1415–30. doi: 10.1080/15384101.2021.1934626
217. Cai J, Sun H, Zheng B, Xie M, Xu C, Zhang G, et al. Curcumin attenuates lncrna H19induced epithelialmesenchymal transition in&Nbsp;Tamoxifenresistant breast cancer cells. Mol Med Rep (2021) 23(1):13. doi: 10.3892/mmr.2020.11651
218. Zhang Y, Jia DD, Zhang YF, Cheng MD, Zhu WX, Li PF, et al. The emerging function and clinical significance of circrnas in thyroid cancer and autoimmune thyroid diseases. Int J Biol Sci (2021) 17(7):1731–41. doi: 10.7150/ijbs.55381
219. Wen ZJ, Xin H, Wang YC, Liu HW, Gao YY, Zhang YF. Emerging roles of circrnas in the pathological process of myocardial infarction. Mol Ther Nucleic Acids (2021) 26:828–48. doi: 10.1016/j.omtn.2021.10.002
220. Cui C, Yang J, Li X, Liu D, Fu L, Wang X. Functions and mechanisms of circular rnas in cancer radiotherapy and chemotherapy resistance. Mol Cancer (2020) 19(1):58. doi: 10.1186/s12943-020-01180-y
221. Lv Q, Xia Q, Li A, Wang Z. Circrna_101277 influences cisplatin resistance of colorectal cancer cells by modulating the mir-370/Il-6 axis. Genet Res (Camb) (2022) 2022:4237327. doi: 10.1155/2022/4237327
222. Huang X, Li Z, Zhang Q, Wang W, Li B, Wang L, et al. Circular rna Akt3 upregulates Pik3r1 to enhance cisplatin resistance in gastric cancer Via mir-198 suppression. Mol Cancer (2019) 18(1):71. doi: 10.1186/s12943-019-0969-3
223. Zhao Y, Zheng R, Chen J, Ning D. Circrna Cdr1as/Mir-641/Hoxa9 pathway regulated stemness contributes to cisplatin resistance in non-small cell lung cancer (Nsclc). Cancer Cell Int (2020) 20:289. doi: 10.1186/s12935-020-01390-w
224. Huang L, Ma J, Cui M. Circular rna Hsa_Circ_0001598 promotes programmed death-Ligand-1-Mediated immune escape and trastuzumab resistance Via sponging mir-1184 in breast cancer cells. Immunol Res (2021) 69(6):558–67. doi: 10.1007/s12026-021-09237-w
225. Chen SW, Zhu SQ, Pei X, Qiu BQ, Xiong D, Long X, et al. Cancer cell-derived exosomal Circusp7 induces Cd8(+) T cell dysfunction and anti-Pd1 resistance by regulating the mir-934/Shp2 axis in nsclc. Mol Cancer (2021) 20(1):144. doi: 10.1186/s12943-021-01448-x
226. Wei W, Sun J, Zhang H, Xiao X, Huang C, Wang L, et al. Circ0008399 interaction with wtap promotes assembly and activity of the M(6)a methyltransferase complex and promotes cisplatin resistance in bladder cancer. Cancer Res (2021) 81(24):6142–56. doi: 10.1158/0008-5472.CAN-21-1518
227. Hu C, Xia R, Zhang X, Li T, Ye Y, Li G, et al. Circfarp1 enables cancer-associated fibroblasts to promote gemcitabine resistance in pancreatic cancer Via the Lif/Stat3 axis. Mol Cancer (2022) 21(1):24. doi: 10.1186/s12943-022-01501-3
228. Chen Z, Lu T, Huang L, Wang Z, Yan Z, Guan Y, et al. Circular rna cia-maf drives self-renewal and metastasis of liver tumor-initiating cells Via transcription factor maff. J Clin Invest (2021) 131(19):e148020. doi: 10.1172/JCI148020
229. Jia DD, Jiang H, Zhang YF, Zhang Y, Qian LL, Zhang YF. The regulatory function of Pirna/Piwi complex in cancer and other human diseases: The role of DNA methylation. Int J Biol Sci (2022) 18(8):3358–73. doi: 10.7150/ijbs.68221
230. Riquelme I, Perez-Moreno P, Letelier P, Brebi P, Roa JC. The emerging role of piwi-interacting rnas (Pirnas) in gastrointestinal cancers: An updated perspective. Cancers (2021) 14(1):202. doi: 10.3390/cancers14010202
231. Tan L, Mai D, Zhang B, Jiang X, Zhang J, Bai R, et al. Piwi-interacting rna-36712 restrains breast cancer progression and chemoresistance by interaction with Sepw1 pseudogene Sepw1p rna. Mol Cancer (2019) 18(1):9. doi: 10.1186/s12943-019-0940-3
232. Mai D, Ding P, Tan L, Zhang J, Pan Z, Bai R, et al. Piwi-interacting rna-54265 is oncogenic and a potential therapeutic target in colorectal adenocarcinoma. Theranostics (2018) 8(19):5213–30. doi: 10.7150/thno.28001
233. Wang Y, Gable T, Ma MZ, Clark D, Zhao J, Zhang Y, et al. A pirna-like small rna induces chemoresistance to cisplatin-based therapy by inhibiting apoptosis in lung squamous cell carcinoma. Mol Ther Nucleic Acids (2017) 6:269–78. doi: 10.1016/j.omtn.2017.01.003
234. Matsuoka T, Yashiro M. Biomarkers of gastric cancer: Current topics and future perspective. World J Gastroenterol (2018) 24(26):2818–32. doi: 10.3748/wjg.v24.i26.2818
235. Umelo IA, Costanza B, Castronovo V. Innovative methods for biomarker discovery in the evaluation and development of cancer precision therapies. Cancer Metastasis Rev (2018) 37(1):125–45. doi: 10.1007/s10555-017-9710-0
236. Liu Y, Wang Y, Li X, Jia Y, Wang J, Ao X. Foxo3a in cancer drug resistance. Cancer Lett (2022) 540:215724. doi: 10.1016/j.canlet.2022.215724
237. Beermann J, Piccoli MT, Viereck J, Thum T. Non-coding rnas in development and disease: Background, mechanisms, and therapeutic approaches. Physiol Rev (2016) 96(4):1297–325. doi: 10.1152/physrev.00041.2015
238. Aurilia C, Donati S, Palmini G, Miglietta F, Falsetti I, Iantomasi T, et al. Are non-coding rnas useful biomarkers in parathyroid tumorigenesis? Int J Mol Sci (2021) 22(19):10465. doi: 10.3390/ijms221910465
239. Zhang L, Zhang Y, Wang Y, Zhao Y, Ding H, Li P. Circular rnas: Functions and clinical significance in cardiovascular disease. Front Cell Dev Biol (2020) 8:584051. doi: 10.3389/fcell.2020.584051
240. Liang M, Li Q, Shi S, Tian YN, Feng Y, Yang Y, et al. Overexpression of mir-138-5p sensitizes taxol-resistant epithelial ovarian cancer cells through targeting cyclin-dependent kinase 6. Gynecol Obstet Invest (2021) 86(6):533–41. doi: 10.1159/000518510
241. Duan L, Yan Y, Wang G, Xing YL, Sun J, Wang LL. Muir-182-5p functions as a tumor suppressor to sensitize human ovarian cancer cells to cisplatin through direct targeting the cyclin dependent kinase 6 (Cdk6). J BUON (2020) 25(5):2279–86.
242. Chen YY, Tai YC. Hsa_Circ_0006404 and Hsa_Circ_0000735 regulated ovarian cancer response to docetaxel treatment Via regulating p-gp expression. Biochem Genet (2022) 60(1):395–414. doi: 10.1007/s10528-021-10080-9
243. Shi X, Xiao L, Mao X, He J, Ding Y, Huang J, et al. Mir-205-5p mediated downregulation of pten contributes to cisplatin resistance in C13k human ovarian cancer cells. Front Genet (2018) 9:555. doi: 10.3389/fgene.2018.00555
244. Tan WX, Sun G, Shangguan MY, Gui Z, Bao Y, Li YF, et al. Novel role of lncrna chrf in cisplatin resistance of ovarian cancer is mediated by mir-10b induced emt and Stat3 signaling. Sci Rep (2020) 10(1):14768. doi: 10.1038/s41598-020-71153-0
245. Luo Y, Gui R. Circulating exosomal Circfoxp1 confers cisplatin resistance in epithelial ovarian cancer cells. J Gynecol Oncol (2020) 31(5):e75. doi: 10.3802/jgo.2020.31.e75
246. Xu ZH, Yao TZ, Liu W. Mir-378a-3p sensitizes ovarian cancer cells to cisplatin through targeting Mapk1/Grb2. Biomed Pharmacother = Biomed Pharmacotherapie (2018) 107:1410–7. doi: 10.1016/j.biopha.2018.08.132
247. Chen Y, Zhao XH, Zhang DD, Zhao Y. Mir-513a-3p inhibits emt mediated by Hoxb7 and promotes sensitivity to cisplatin in ovarian cancer cells. Eur Rev Med Pharmacol Sci (2020) 24(20):10391–402. doi: 10.26355/eurrev_202010_23389
248. Ni MW, Zhou J, Zhang YL, Zhou GM, Zhang SJ, Feng JG, et al. Downregulation of Linc00515 in high-grade serous ovarian cancer and its relationship with platinum resistance. biomark Med (2019) 13(7):535–43. doi: 10.2217/bmm-2018-0382
249. Wang Y, Bao W, Liu Y, Wang S, Xu S, Li X, et al. Mir-98-5p contributes to cisplatin resistance in epithelial ovarian cancer by suppressing mir-152 biogenesis Via targeting Dicer1. Cell Death Dis (2018) 9(5):447. doi: 10.1038/s41419-018-0390-7
250. Jiang J, Wang S, Wang Z, Cai J, Han L, Xie L, et al. Hotair promotes paclitaxel resistance by regulating Chek1 in ovarian cancer. Cancer Chemother Pharmacol (2020) 86(2):295–305. doi: 10.1007/s00280-020-04120-1
251. Xia B, Zhao Z, Wu Y, Wang Y, Zhao Y, Wang J. Circular rna Circtnpo3 regulates paclitaxel resistance of ovarian cancer cells by mir-1299/Nek2 signaling pathway. Mol Ther Nucleic Acids (2020) 21:780–91. doi: 10.1016/j.omtn.2020.06.002
252. Jin L, Zhang Z. Serum mir-3180-3p and mir-124-3p may function as noninvasive biomarkers of cisplatin resistance in gastric cancer. Clin Lab (2020) 66(12). doi: 10.7754/Clin.Lab.2020.200302
253. Li Y, Lv S, Ning H, Li K, Zhou X, Xv H, et al. Down-regulation of Casc2 contributes to cisplatin resistance in gastric cancer by sponging mir-19a. Biomed Pharmacother = Biomed Pharmacotherapie (2018) 108:1775–82. doi: 10.1016/j.biopha.2018.09.181
254. Lv X, Li P, Wang J, Gao H, Hei Y, Zhang J, et al. Hsa_Circ_0000520 influences herceptin resistance in gastric cancer cells through Pi3k-akt signaling pathway. J Clin Lab Anal (2020) 34(10):e23449. doi: 10.1002/jcla.23449
255. Gao H, Ma J, Cheng Y, Zheng P. Exosomal transfer of macrophage-derived mir-223 confers doxorubicin resistance in gastric cancer. Onco Targets Ther (2020) 13:12169–79. doi: 10.2147/OTT.S283542
256. Zhang Z, Li M, Zhang Z. Lncrna Malat1 modulates oxaliplatin resistance of gastric cancer Via sponging mir-22-3p. Onco Targets Ther (2020) 13:1343–54. doi: 10.2147/OTT.S196619
257. Zhang Z, Yu X, Zhou B, Zhang J, Chang J. Circular rna Circ_0026359 enhances cisplatin resistance in gastric cancer Via targeting mir-1200/Pold4 pathway. BioMed Res Int (2020) 2020:5103272. doi: 10.1155/2020/5103272
258. Zhou Y, Ding BZ, Lin YP, Wang HB. Mir-34a, as a suppressor, enhance the susceptibility of gastric cancer cell to luteolin by directly targeting Hk1. Gene (2018) 644:56–65. doi: 10.1016/j.gene.2017.10.046
259. Pang K, Song J, Bai Z, Zhang Z. Mir-15a-5p targets Phlpp2 in gastric cancer cells to modulate platinum resistance and is a suitable serum biomarker for oxaliplatin resistance. Neoplasma (2020) 67(5):1114–21. doi: 10.4149/neo_2020_190904N861
260. Luo Y, Zheng S, Wu Q, Wu J, Zhou R, Wang C, et al. Long noncoding rna (Lncrna) Eif3j-dt induces chemoresistance of gastric cancer Via autophagy activation. Autophagy (2021) 17(12):4083–101. doi: 10.1080/15548627.2021.1901204
261. Zhen Q, Zhang Y, Gao L, Wang R, Chu W, Zhao X, et al. Mir-519d-3p enhances the sensitivity of non-Small-Cell lung cancer to tyrosine kinase inhibitors. Mamm Genome (2021) 32(6):508–16. doi: 10.1007/s00335-021-09919-8
262. Gu G, Hu C, Hui K, Zhang H, Chen T, Zhang X, et al. Exosomal mir-136-5p derived from anlotinib-resistant nsclc cells confers anlotinib resistance in non-small cell lung cancer through targeting Ppp2r2a. Int J Nanomed (2021) 16:6329–43. doi: 10.2147/IJN.S321720
263. Chen ZY, Liu HY, Jiang N, Yuan JM. Lncrna Host2 enhances gefitinib-resistance in non-small cell lung cancer by down-regulating mirna-621. Eur Rev Med Pharmacol Sci (2019) 23(22):9939–46. doi: 10.26355/eurrev_201911_19560
264. Li Q, Wang Y, He J. Mir-133a-3p attenuates resistance of non-small cell lung cancer cells to gefitinib by targeting Spag5. J Clin Lab Anal (2021) 35(7):e23853. doi: 10.1002/jcla.23853
265. Li X, Zhang X, Yang C, Cui S, Shen Q, Xu S. The lncrna Rhpn1-As1 downregulation promotes gefitinib resistance by targeting mir-299-3p/Tnfsf12 pathway in nsclc. Cell Cycle (2018) 17(14):1772–83. doi: 10.1080/15384101.2018.1496745
266. Xu YH, Tu JR, Zhao TT, Xie SG, Tang SB. Overexpression of lncrna Egfras1 is associated with a poor prognosis and promotes chemotherapy resistance in nonsmall cell lung cancer. Int J Oncol (2019) 54(1):295–305. doi: 10.3892/ijo.2018.4629
267. Song HM, Meng D, Wang JP, Zhang XY. Circrna Hsa_Circ_0005909 predicts poor prognosis and promotes the growth, metastasis, and drug resistance of non-Small-Cell lung cancer Via the mirna-338-3p/Sox4 pathway. Dis Markers (2021) 2021:8388512. doi: 10.1155/2021/8388512
268. Zhang L, Chen H, Song Y, Gu Q, Zhang L, Xie Q, et al. Mir-325 promotes oxaliplatin-induced cytotoxicity against colorectal cancer through the Hspa12b/Pi3k/Akt/Bcl-2 pathway. Dig Dis Sci (2021) 66(8):2651–60. doi: 10.1007/s10620-020-06579-7
269. Wang H, Li H, Zhang L, Yang D. Overexpression of Meg3 sensitizes colorectal cancer cells to oxaliplatin through regulation of mir-141/Pdcd4 axis. Biomed Pharmacother = Biomed Pharmacotherapie (2018) 106:1607–15. doi: 10.1016/j.biopha.2018.07.131
270. Sun L, Fang Y, Wang X, Han Y, Du F, Li C, et al. Mir-302a inhibits metastasis and cetuximab resistance in colorectal cancer by targeting nfib and Cd44. Theranostics (2019) 9(26):8409–25. doi: 10.7150/thno.36605
271. Hong S, Yan Z, Song Y, Bi M, Li S. Lncrna Agap2-As1 augments cell viability and mobility, and confers gemcitabine resistance by inhibiting mir-497 in colorectal cancer. Aging (2020) 12(6):5183–94. doi: 10.18632/aging.102940
272. Zhang Y, Li C, Liu X, Wang Y, Zhao R, Yang Y, et al. Circhipk3 promotes oxaliplatin-resistance in colorectal cancer through autophagy by sponging mir-637. EBioMedicine (2019) 48:277–88. doi: 10.1016/j.ebiom.2019.09.051
273. Wu H, Gu J, Zhou D, Cheng W, Wang Y, Wang Q, et al. Linc00160 mediated paclitaxel-and doxorubicin-resistance in breast cancer cells by regulating Tff3 Via transcription factor C/Ebpbeta. J Cell Mol Med (2020) 24(15):8589–602. doi: 10.1111/jcmm.15487
274. Zhang M, Wang Y, Jiang L, Song X, Zheng A, Gao H, et al. Lncrna Cbr3-As1 regulates of breast cancer drug sensitivity as a competing endogenous rna through the Jnk1/Mek4-mediated mapk signal pathway. J Exp Clin Cancer Res (2021) 40(1):41. doi: 10.1186/s13046-021-01844-7
275. Wang L, Zhou Y, Jiang L, Lu L, Dai T, Li A, et al. Circwac induces chemotherapeutic resistance in triple-negative breast cancer by targeting mir-142, upregulating Wwp1 and activating the Pi3k/Akt pathway. Mol Cancer (2021) 20(1):43. doi: 10.1186/s12943-021-01332-8
276. Shu G, Su H, Wang Z, Lai S, Wang Y, Liu X, et al. Linc00680 enhances hepatocellular carcinoma stemness behavior and chemoresistance by sponging mir-568 to upregulate Akt3. J Exp Clin Cancer Res (2021) 40(1):45. doi: 10.1186/s13046-021-01854-5
277. Xu J, Ji L, Liang Y, Wan Z, Zheng W, Song X, et al. Circrna-sore mediates sorafenib resistance in hepatocellular carcinoma by stabilizing Ybx1. Signal Transduction Targeted Ther (2020) 5(1):298. doi: 10.1038/s41392-020-00375-5
278. Zhou S, Wei J, Wang Y, Liu X. Cisplatin resistance-associated Circrna_101237 serves as a prognostic biomarker in hepatocellular carcinoma. Exp Ther Med (2020) 19(4):2733–40. doi: 10.3892/etm.2020.8526
279. Lu H, Lu S, Yang D, Zhang L, Ye J, Li M, et al. Mir-20a-5p regulates gemcitabine chemosensitivity by targeting Rrm2 in pancreatic cancer cells and serves as a predictor for gemcitabine-based chemotherapy. Biosci Rep (2019) 39(5):BSR20181374. doi: 10.1042/BSR20181374
280. Okazaki J, Tanahashi T, Sato Y, Miyoshi J, Nakagawa T, Kimura T, et al. Microrna-296-5p promotes cell invasion and drug resistance by targeting Bcl2-related ovarian killer, leading to a poor prognosis in pancreatic cancer. Digestion (2020) 101(6):794–806. doi: 10.1159/000503225
281. Liu Y, Wang J, Dong L, Xia L, Zhu H, Li Z, et al. Long noncoding rna Hcp5 regulates pancreatic cancer gemcitabine (Gem) resistance by sponging hsa-Mir-214-3p to target hdgf. Onco Targets Ther (2019) 12:8207–16. doi: 10.2147/OTT.S222703
282. Wang H, Wu B, Wang J, Hu Y, Dai X, Ye L, et al. Methylation associated mir-1246 contributes to poor prognosis in gliomas treated with temozolomide. Clin Neurol Neurosurg (2021) 200:106344. doi: 10.1016/j.clineuro.2020.106344
283. Luo Y, Gui R. Circulating exosomal circmyc is associated with recurrence and bortezomib resistance in patients with multiple myeloma. Turk J Haematol (2020) 37(4):248–62. doi: 10.4274/tjh.galenos.2020.2020.0243
284. Pan J, Zhou C, Zhao X, He J, Tian H, Shen W, et al. A two-mirna signature (Mir-33a-5p and mir-128-3p) in whole blood as potential biomarker for early diagnosis of lung cancer. Sci Rep (2018) 8(1):16699. doi: 10.1038/s41598-018-35139-3
285. Lu N, Liu J, Ji C, Wang Y, Wu Z, Yuan S, et al. Mirna based tumor mutation burden diagnostic and prognostic prediction models for endometrial cancer. Bioengineered (2021) 12(1):3603–20. doi: 10.1080/21655979.2021.1947940
286. Xu W, Zhou G, Wang H, Liu Y, Chen B, Chen W, et al. Circulating lncrna Snhg11 as a novel biomarker for early diagnosis and prognosis of colorectal cancer. Int J Cancer (2020) 146(10):2901–12. doi: 10.1002/ijc.32747
287. Meng Y, Liu YL, Li K, Fu T. Prognostic value of long non-coding rna breast cancer anti-estrogen resistance 4 in human cancers: A meta-analysis. Med (Baltimore) (2019) 98(21):e15793. doi: 10.1097/MD.0000000000015793
288. Liu X, Tang H, Liu J, Wang X. Hsa_Circrna_101237: A novel diagnostic and prognostic biomarker and potential therapeutic target for multiple myeloma. Cancer Manag Res (2020) 12:2109–18. doi: 10.2147/CMAR.S241089
289. Zhang X, Xie K, Zhou H, Wu Y, Li C, Liu Y, et al. Role of non-coding rnas and rna modifiers in cancer therapy resistance. Mol Cancer (2020) 19(1):47. doi: 10.1186/s12943-020-01171-z
290. Yan H, Bu P. Non-coding rna in cancer. Essays Biochem (2021) 65(4):625–39. doi: 10.1042/EBC20200032
291. Fu Y, Huang R, Li J, Xie X, Deng Y. Lncrna Ensg00000254615 modulates proliferation and 5-fu resistance by regulating P21 and cyclin D1 in colorectal cancer. Cancer Invest (2021) 39(9):696–710. doi: 10.1080/07357907.2021.1923727
292. Qin L, Zhan Z, Wei C, Li X, Zhang T, Li J. Hsacircrnag004213 promotes cisplatin sensitivity by regulating Mir513b5p/Prpf39 in liver cancer. Mol Med Rep (2021) 23(6):421. doi: 10.3892/mmr.2021.12060
293. Li Y, Zu L, Wu H, Zhang F, Fan Y, Pan H, et al. Mir-192/Nkrf axis confers lung cancer cell chemoresistance to cisplatin Via the nf-kappab pathway. Thorac Cancer (2022) 13(3):430–41. doi: 10.1111/1759-7714.14278
294. Zhu M, Wang Y, Wang F, Li L, Qiu X. Circfbxl5 promotes the 5-fu resistance of breast cancer Via modulating mir-216b/Hmga2 axis. Cancer Cell Int (2021) 21(1):384. doi: 10.1186/s12935-021-02088-3
Keywords: non-coding RNA, cancer, drug resistance, biomarker, therapeutic target
Citation: Zhou X, Ao X, Jia Z, Li Y, Kuang S, Du C, Zhang J, Wang J and Liu Y (2022) Non-coding RNA in cancer drug resistance: Underlying mechanisms and clinical applications. Front. Oncol. 12:951864. doi: 10.3389/fonc.2022.951864
Received: 24 May 2022; Accepted: 02 August 2022;
Published: 17 August 2022.
Edited by:
Takeo Tatsuta, Tohoku Medical and Pharmaceutical University, JapanReviewed by:
Jayant Maini, Manav Rachna International Institute of Research and Studies (MRIIRS), IndiaHamed Shoorei, Birjand University of Medical Sciences, Iran
Copyright © 2022 Zhou, Ao, Jia, Li, Kuang, Du, Zhang, Wang and Liu. This is an open-access article distributed under the terms of the Creative Commons Attribution License (CC BY). The use, distribution or reproduction in other forums is permitted, provided the original author(s) and the copyright owner(s) are credited and that the original publication in this journal is cited, in accordance with accepted academic practice. No use, distribution or reproduction is permitted which does not comply with these terms.
*Correspondence: Ying Liu, liuying_hero@163.com
†These authors have contributed equally to this work