- 1Hematology and Medical Oncology Division, Department of Internal Medicine, Cipto Mangunkusumo National General Hospital, Jakarta, Indonesia
- 2Faculty of Medicine, University of Indonesia, Jakarta, Indonesia
- 3Hospital Medicine, Bhakti Medicare Hospital, Cicurug, Sukabumi, Indonesia
Patient populations, including those with hematological malignancies, have different responses to COVID-19 vaccines. This study aimed to quantitatively analyze the efficacy and safety of COVID-19 mRNA vaccines in patients with hematological malignancies. Studies reporting on the efficacy and safety of COVID-19 mRNA vaccines in cohorts with hematological malignancies compared to healthy controls were systematically searched in four databases. Meta-analysis and subgroup analyses were performed to generate quantitative synthesis. Fifteen studies with 2,055 cohorts with hematological malignancies and 1,105 healthy subjects as control were included. After two doses of COVID-19 vaccination, only 60% of cohorts with hematological malignancies were seroconverted compared to healthy controls (RR 0.60; 95%CI 0.50–0.71). A single dose of the vaccine resulted in a significantly lower seroconversion rate (RR 0.30; 95%CI 0.16–0.54). Non-Hodgkin lymphoma cohorts had the lowest rate of seroconversion (RR 0.5; 95%CI 0.35–0.71) and those who received active treatments had lower immunological responses (RR 0.59; 95%CI 0.46–0.75). Antibody titers were lower in cohorts with hematological malignancies without any differences in adverse effects in both groups. In conclusion, cohorts with hematological malignancies showed a lower seroconversion rate and antibody titers after receiving COVID-19 mRNA vaccines. The type of malignancy and the status of treatment had a significant impact on the response to vaccination. The vaccines were shown to be safe for both patients with hematological malignancies and healthy controls. Booster doses and stricter health protocols might be beneficial for patient populations.
Introduction
The severe acute respiratory syndrome coronavirus 2 (SARS-CoV-2) virus has spread worldwide since its first case in 2019, with 500 million infected people with coronavirus disease 2019 (COVID-19) and more than 6.1 million deaths (1). The virus has caused great difficulty across the globe, compromising service in healthcare facilities and in different public sectors. Actions towards managing the pandemic have been implemented, starting with social distancing measures in numerous countries. Social distancing itself has been associated with reduction of COVID-19 incidence by 29% and a 35% reduction of COVID-19 mortality, and even almost reached zero cases in China in 2020, prior to the emergence of cases due to new mutant variants (2, 3). The vaccination program, started in 2020, has been considered the key approach for ending the pandemic. In total, more than 11 billion doses have been administered with around 59% of the world’s population fully vaccinated (4). A total of 46 trials have been conducted on the COVID-19 vaccine, from messenger ribonucleic acid (mRNA) vaccines, inactivated vaccines, protein subunit vaccines, to viral vector vaccines (5). The vaccination program has reported a good outcome, with an efficacy of 61%–95%, thus decreasing the mortality rate, the incidence of severe diseases and the incidence of infection to almost 90% after the implementation of the vaccination program, despite growing concerns of short-term immunity, especially concerning the mRNA vaccines (6–9).
Despite the success of COVID-19 vaccines with its high efficacy on the healthy population, concerns on the efficacy and safety of COVID-19 vaccines in the immunocompromised population remain unresolved. The seroconversion rate has been found to be significantly lower in immunocompromised patients, especially organ transplant recipients, with only one third achieving seroconversion status, followed by patients with immune-mediated inflammatory disorders (75%), and malignancies or cancers ranging from 63% –90% achieving seroconversion (7, 10). The same concern is also directed at patients with hematological malignancies, especially due to their high risk of being immunosuppressed due to the pathogenesis and molecular mechanism of the disease. One study has mentioned the lower positive seropositivity rate ranging from 62%–66% in patients with hematological malignancies, those with B-cell malignancies, and those undergoing active monoclonal antibody treatment (11). The response rate of COVID-19 vaccines in hematological malignancies is also lower than the solid cancer group by almost two fold (12). RNA vaccines are considered the vaccine with the highest efficacy, despite their short-term immunogenicity. The BNT162b2 and mRNA-1273 have been shown to be 95.3% and 84.4% effective, respectively, in healthy populations (13). Therefore, the mRNA vaccine could be suitable as an option for immunosuppressed patients (6). Previous cumulative data on the efficacy and safety of mRNA vaccines have been collected in immunosuppressed patients (14), and in patients with hematological malignancies by Cavanna et al. (15) and Sakuraba et al. (10). However, due to the small number of included studies and the wide confidence interval, the results might seem insignificant. Thus, this systematic review and meta-analysis was conducted to evaluate the efficacy of mRNA vaccines on hematological malignancies to include the most recent studies on the topic.
Methods
This study was conducted in accordance with Preferred Reporting Items for Systematic Review and Meta-Analysis (PRISMA) guidelines (16). This systematic review was also subject to the Assessing the Methodological Quality of Systematic Reviews (AMSTAR) 2 checklist (17).
Search protocol registration
The protocol of this study was registered in the PROSPERO database (CRD42022316188).
Ethical approval
This systematic review used studies and the grey literature published in several medical databases. Ethics approval was not required for this study.
Search strategy
Studies related to the efficacy and safety of the COVID-19 mRNA vaccine in cohorts with hematological malignancy were systematically searched in databases including PubMed, Scopus, ScienceDirect, and EBSCOHost. General search terms used included: ‘COVID-19’, ‘SARS-CoV-2’, ‘nCOV-19’, ‘mRNA vaccine’, ‘BNT162’, ‘mRNA-1273’, ‘malignancy’, ‘neoplasm’, and ‘cancer’. The search was carried out on March 15, 2022 with detailed keywords used for each database, as shown in Table 1. The search was limited to literature in English, but no limitation was used on the publication period. Manual searching of references was used to identify additional suitable studies. Duplicate entries were then removed, followed by screening of titles and abstracts. Full-text reviews were conducted to identify potentially eligible titles and abstracts by each investigator. The search and screening were conducted independently by five investigators (IR, IW, JR, LW, and SP). Reasons for excluding studies were listed.
Study eligibility criteria
The following criteria were used to select the studies to be included in the analysis: 1) prospective cohort studies or clinical trials of COVID-19 vaccines in cohorts with hematological malignancies or sub-analysis of hematological malignancies, 2) vaccination with any formulation of COVID-19 mRNA vaccine with standard number of dosing, 3) healthy subjects without any malignancy as controls, 4) the results of the studies included the seroconversion rate, antibody titer level, and adverse effects (AEs). The criteria for the studies to be excluded were: 1) a study other than a prospective cohort or clinical trial (i.e., cross-sectional studies, case reports, review articles, commentaries, or correspondence letters), 2) studies without available full-text articles; 3) single-arm studies or without healthy subjects as controls, 4) a cohort receiving stem cell transplant as active therapy.
Data extraction and risk of bias assessment
The following data were extracted from each study: 1) first author and publication year; 2) study characteristics, such as study location, study design, and sample size; 3) characteristics of hematological malignancy cohorts, such as type of cancer, mean age, sex proportion, and type of active treatment received; 4) intervention details, such as type of vaccine, number of doses and follow up duration; 5) characteristics of healthy subject controls, such as control size, mean age, and sex proportion, and 6) outcomes presented. Primary outcomes were the seropositive rate, antibody titer level, and reported AEs, while other available outcomes were extracted as secondary outcomes. The risk of bias of each selected study was assessed using suitable appraisal tools. The Joanna Briggs Institute (JBI) checklist was used for nonrandomized intervention studies of intervention (e.g., cohort studies), while randomized clinical trials were evaluated using the Cochrane Risk of Bias 2.0 tool (18, 19). Any discrepancies among the five investigators (IR, IW, JR, LW, and SP) were resolved by discussion with an independent investigator (KW). The cutoff point values of the JBI checklist used to determine the level of bias of each study were the following: study with yes in equal or more than 70% of the questions labeled as low risk, 50%–69% of the questions labeled as moderate risk, and less than 50% of the questions labeled as high risk (20, 21).
Data synthesis and statistical analysis
Quantitative analysis was conducted to calculate the cumulative outcome of positive seropositivity of mRNA vaccines in patients with hematological malignancies. A total of 15 studies were included in the quantitative analysis. The analysis was performed using Review Manager 5.4 (Cochrane). The analysis was based on dichotomous data with Mantel–Haenszel statistical method with random effects analysis model. The effect measures used on the analysis were risk ratio (RR) on the seropositivity rate comparison between the hematological malignancies group and its control group counterpart. The comparison was conducted on the first dose and second dose of vaccine, as well as the comparison between active treatment and non-active treatment. Subgroup analyses were used to explore cumulative outcomes of each hematological cancer type. The analyses were set on 95% confidence intervals (CI) with heterogeneity analysis using I2 as the outcome. The I2 cutoff of <50% indicated no significant heterogeneity, 50%–70% indicated considerable heterogeneity, 70%–90% high heterogeneity, and >90% indicated extremely high heterogeneity (22). The cumulative results were then extrapolated into Forest plots to describe the cumulative RR of the efficacy of the mRNA vaccine.
Results
Study selection
Searching the literature in four databases retrieved 1,381 articles, 182 of which were removed due to duplication. The titles and abstracts of 1,199 papers were selected and 1,064 papers were excluded due to irrelevancy. Full text reviews were omitted leaving 135 papers, and 120 papers were removed based on the exclusion criteria. Nine relevant studies were obtained through manual search, although they were later excluded based on the exclusion criteria. The remaining 15 papers were included in qualitative and quantitative synthesis. The summary of the study selection is presented in the PRISMA diagram (see Figure 1).
Study characteristics and risk of bias
The 15 included studies were published between 2021 and 2022 in various countries and were used for the analysis. Most included studies were cohort prospective in design, except for one study which was an open-label, nonrandomized prospective clinical trial. A total of 2,055 patients with hematological malignancies were included of which 58.7% were men, with a median age range between 51 and 73 years. The control groups consisted of 1,105 healthy subjects and only 11 studies mentioned a detailed description of their age and sex proportion. Among the remaining studies, one matched age and sex, one matched age only, one matched sex only, and one did not match age and sex to the cohort with hematological malignancies. There were 5 studies that included multiple hematological malignancies in their cohorts and the other 10 studies included only a specific type of hematological malignancies. All the included studies had patients in different stages of the disease (i.e., active, chronic phase, remission, or relapse) and different stages of treatment (i.e., treatment naive, actively receiving various types of therapy, or refractory). Two doses of the BNT162b2 mRNA vaccine (Pfizer-Bio NTech) were used in all studies, except for a few studies (i.e., 3 studies with ChAdOx1 nCoV-19/AZD1222/Oxford-AstraZeneca vaccine and 3 studies with the mRNA-1273/Moderna vaccine). The duration of follow-up was also different between studies, ranging from 14 days to 72 days from the second dose. Table 2 summarizes the baseline characteristics for all 15 studies included in this systematic review and meta-analysis.
Four articles did not clearly identify the confounders and five articles did not clearly mention their strategies to deal with the confounders. Two papers mentioned confounding factors but did not mention the strategies used to deal with them. Furthermore, in two papers the baseline data (especially those related to the outcome) of their cohort were not clearly defined. One paper showed different baseline antibody data between the population and control groups. Despite the limitations mentioned above, all the included studies had low levels of bias after being assessed using the JBI checklist. The detailed scoring based on the JBI checklist is provided in Table 3.
Study outcomes
Comparison of the rate of seroconversion after the COVID-19 mRNA vaccine
All 15 studies reported the seroconversion rate after SARS-CoV-2 mRNA vaccination, hence were all included in the qualitative and quantitative analysis (see Table 4). All cohorts with hematological malignancies showed lower rates of seroconversion after two doses of vaccination compared to healthy controls, although statistical significance was only mentioned in 8 studies. The pooled proportions of the seroconversion rate were 61.8% for the hematological malignancies cohort and 97.2% for the healthy controls. Meta-analysis of all included studies showed that after two doses of COVID-19 vaccination, only 60% of patients with hematological malignancy were seroconverted compared to healthy controls (RR=0.60; 95%CI 0.50–0.71; P<0.0001) as shown in Figure 2. There was high heterogeneity (I2 = 96%), which was statistically significant.
An effort was made to minimize heterogeneity by performing a sub-analysis based on the type of hematological malignancy (see Figure 3). In 14 studies, nine different types of hematological malignancies were mentioned (Cavanna et al. (15) did not mention the type of malignancy). The results showed that patients with non-Hodgkin lymphoma (NHL) had the lowest rate of seroconversion (RR 0.5; 95%CI 0.35–0.71; P=0.0001), followed by chronic lymphocytic leukemia (CLL) (RR 0.56; 95%CI 0.46–0.69; P<0.0001), Waldenstrom macroglobulinemia (WM) (RR 0.5; 95%CI 0.35–0.71; P=0.0001), acute leukemia (RR 0.72; 95%CI 0.32–1.60; P=0.42), multiple myeloma (MM) (RR 0.74; 95%CI 0.61–0.90; P=0.003), myeloproliferative neoplasms (MPN) (RR 0.84; 95%CI 0.76–0.94; P=0.002), Hodgkin lymphoma (HL) (RR 0.87; 95%CI 0.71–1.07; P=0.19), chronic myeloid leukemia (CML) (RR 0.94; 95%CI 0.86–1.02; P=0.14), and myelodysplastic syndrome (MDS) (RR 0.95; 95%CI 0.83–1.08; P=0.40). Figure 4 summarizes the risk ratio for each type of hematological malignancy. Significant heterogeneity (I2 above 35%) was found in five types of hematological malignancy (i.e., MM, CLL, NHL, HL, and CML). The sub-analysis of the acute leukemia and MPN groups did not show high heterogeneity, whereas it was not calculable in the MDS and WM groups.
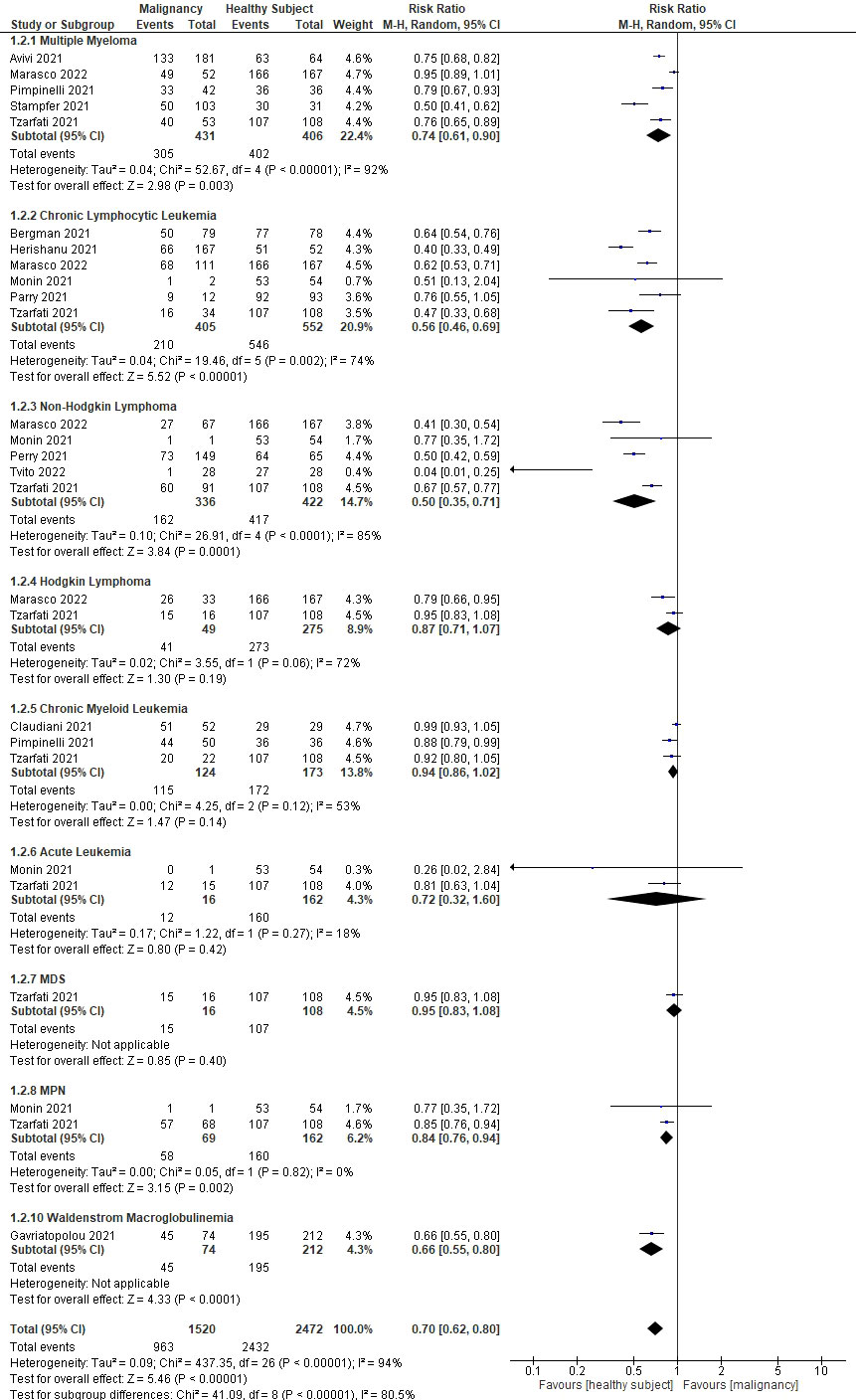
Figure 3 Sub-analyses showing the rate of seroconversion between different types of hematological malignancies.
Another sub-analysis was performed to compare the rate of seroconversion in patients with active treatments with patients who were treatment naive or had completed their treatments (see Figure 5). Data on treatment status were provided in 7 studies. Patients with active treatments had statistically significantly lower immunological responses toward the COVID-19 vaccination compared to patients not in active treatment (RR 0.59, 95%CI 0.46–0.75, P<0.0001). However, heterogeneity was also high in this sub-analysis (I2 = 80%).
Our of 15 studies, eight reported the rate of seroconversion after the first dose and compared rates after the second dose. All studies showed a marked improvement in the rate of seroconversion after the second dose, although they were still lower than the healthy control group. A sub-analysis was performed to quantitatively measure RR when receiving only one dose of COVID-19 mRNA vaccine in relation to the rate of seroconversion (see Figure 6). Receiving only one dose had a lower RR, which also indicated a lower rate of seroconversion, which was approximately half that of receiving two complete doses of the vaccine (RR 0.30; 95%CI 0.16–0.54; P<0.0001; I2 = 98%).
Comparison of antibody titers after two doses of the COVID-19 mRNA vaccine
Eleven studies measured and reported antibody titers after two doses of SARS-CoV-2 mRNA vaccination and therefore were included in the qualitative analysis. Similar to the seroconversion rate, all studies found lower antibody titers in patients with hematological malignancies when compared with healthy controls (Table 4). Herishanu et al. (27) showed that their CLL cohorts had more than a 1,000-fold lower median antibody titers and even the responding subgroup of CLL cohorts had a 7-fold lower median antibody titers than the healthy controls. According to other studies, Tzarfati et al. (24) (cohorts with various types of malignancy) and Gavriatopoulou et al. (30) (Waldenstrom macroglobulinemia cohorts) found lower antibody titers in cohorts of hematological malignancies by 2- and 3-fold, respectively. Marasco et al. (36) showed a larger gap of antibody titers (5 fold) between cohorts with various malignancies and healthy controls (207.5 U/mL vs. 1078 U/mL). Conversely, Claudiani et al. (25) found statistically insignificant differences in antibody titers between CML patients and healthy controls in their analysis.
Other studies also mentioned the median antibody titers between subgroups of their cohorts. Active treatment of patients in a study by Perry et al. (26) achieved lower antibodies when compared with treatment naive patients, although they were still lower compared to the healthy controls (13.7 U/mL, 1008 U/mL, and 1332 U/mL, respectively). Pimpinelli et al. (28) presented differences in antibody titers between different types of malignancies (MM 106.7 AU/mL vs. MPM 172.9 AU/mL). Different stages of the disease also had a significant impact on antibody titers. Stampfer et al. (31) and Avivi et al. (29) compared antibody titers for active MM and smoldering MM (early asymptomatic stage); cohorts with smoldering MM had 3 times higher antibody titers in the Stampfer et al. study and 9 times higher in the Avivi et al. study.
Some studies also measured antibody titers between the first and second dose of the vaccine, allowing comparisons to be made. MM patients in the study by Stampfer et al. (31) had a 15-fold increase in antibody titers after receiving the second dose. The study by Parry et al. (23) showed that the median antibody titers between CLL and healthy controls was 104 times lower after the first dose, which then decreased to 74 times lower after the second dose. Getting the second dose of vaccination also significantly increased antibody titers 2-fold in WM cohorts in the study by Gavriatopoulou et al. (30) and more than 100 times in CML cohorts in the study by Claudiani et al. (25).
Comparison of safety and adverse events after COVID-19 mRNA vaccine
Only 8 studies reported AEs observed after the SARS-CoV-2 mRNA vaccination. These studies showed that the COVID-19 the COVID-19 mRNA vaccines were safe for patients with hematological malignancies and healthy subjects. The analysis of Perry et al. (26) showed that there were no statistically significant differences in the types and severity of AEs between NHL patients and healthy controls. In contrast, in the studies by Avivi et al. (29) and Monin et al. (33), the incidence of AEs among healthy controls across all studies ranged from 20%–50%, most of which were mild localized reactions and resolved without any complication. Gavriatopoulou et al. (30) added that between the first and second doses, there was no difference in the occurrence of mild localized reactions (37% vs. 38%) but there was a difference in systemic adverse reactions (13% vs. 24%).
Pain at injection sites and local erythema or swelling were the most common mild AEs mentioned in these studies (26, 27, 33, 34). Commonly occurring systemic AEs were fatigue or weakness, muscle pain, headache, and fever (26, 27). Mild transient lymphadenopathy was found only in a small percentage of the subjects, about 1%–2.5%, cumulatively. However, in these studies there were also some moderate and severe AEs (26, 29). Pimpinelli et al. (28) observed the appearance of moderate AEs (e.g., malaise, fever, myalgia) in 9% and severe pain in 2% of their cohorts, especially after receiving the second dose of the vaccine. There were six moderate adverse reactions in the study by Bergman et al. (32), but they were unlikely to be related to vaccination and five of these resolved. Malard et al. (34) also reported the appearance of grade 3 AEs in 8.4% of their cohorts. Lastly, the analysis by Herishanu et al. (27) showed that the AEs were not correlated with a positive serologic response to the vaccine or with the treatment status.
Discussion
COVID-19 has been the focus of researchers worldwide for the last 2 years. It has impacted the quality of life of the entire global population with its high transmissibility, morbidity, and mortality (37). Some populations have been shown to have higher susceptibility to this disease, including those with malignancies. Immunodeficiency in individuals with malignancies can be caused by the properties of the disease itself and by the effects of the radiation or chemotherapy (10). A study with 507,307 patients with COVID-19 showed that cancer patients who also received anticancer treatment within 3 months before the diagnosis of COVID-19 had an increased risk of hospitalization, admission to intensive care units, and death (38). Furthermore, COVID-19 vaccines have been developed and are considered essential to end the pandemic. Global efforts to create herd immunity are still in progress (39). Unfortunately, vulnerable individuals with malignancies seem to receive less protection from these vaccines. The meta-analysis by Sakuraba et al. (10) showed that cancer patients had a lower response to COVID-19 vaccinations compared to healthy individuals, especially in those with hematological cancer. Their finding is highly concerning, as hematological diseases are believed to have the highest level of immunosuppression and are associated with a 3- to 4-fold higher rate of severe COVID-19 disease, and even mortality (40–42). This systematic review and meta-analysis examining the efficacy and safety of the COVID-19 mRNA vaccine, specifically for people with hematological malignancies, is intended to help solve the problem.
In this systematic review, we identified 8 out of 15 studies reporting a seroconversion rate after administering the first dose of the COVID-19 mRNA vaccine (RR 0.30). However, the seroconversion rate was lower than that of the healthy control group. The antibody response improved significantly after the second dose (RR 0.60). Our findings also showed that patients with active treatments had a significantly lower immunological response than patients not receiving active treatment (treatment naive or had completed their treatment) (RR 0.59). The types of treatment could have a large impact on the response to vaccination, as all lymphoma cohorts that received anti-CD20 monoclonal antibodies in the study by Tvito et al. (35) did not show any serologic response. The seroconversion rate was also shown in MM, CLL, NHL, HL, CML, acute leukemia, MDS, MPN and WM. Receiving one dose of the COVID-19 mRNA vaccine showed a lower rate of seroconversion compared to two completed doses of the vaccine. Therefore, our findings recommend that patients with malignancy receive two doses of the COVID-19 mRNA vaccine. It can be speculated that disease-specific immunosuppression (such as hypoglobulinemia, B-cell dysfunction, and T-cell exhaustion) adds to treatment-related variables (27). In hematological malignancies such as CLL, the anti-SARS-CoV-2 antibody response to COVID-19 infection has already been reported to be low (67%); similar data have also been published for patients with MM convalescent to COVID-19, but data on anti-Ig for spike protein after COVID-19 vaccination have been limited so far (30, 31). Our data on vaccinated patients are consistent with three recently published observations in patients with CLL and MM (27, 28, 32).
This study emphasized lower antibody titers in hematological cancer patients compared to healthy populations. Hematological cancer patients were known to have two to seven times lower antibody titer levels, although a study could not conclude a significant difference (11). A breakdown of studies showed that patients who underwent active treatments also had lower antibody titer levels. Additionally, characteristics such as types of malignancy, stage of cancer, and number of vaccination doses also contribute to antibody titer levels in patients with hematological malignancy.
The results of this study were consistent with the findings of Teh et al. (11), which found a seropositivity rate of 37%–51% and 62%–65% after the first and second dose of the COVID-19 vaccine, respectively. The same study found that the lowest and highest conversion rates were found in CLL and acute leukemia with a 51% and a 93% rate, respectively. Furthermore, active treatment with targeted therapy and anti-CD20 monoclonal antibody showed the poorest immune response. The included studies also showed that patients receiving anti-CD20 monoclonal antibody-containing therapy (e.g., rituximab and obinutuzumab) had the lowest seroconversion rate (between 0% and 36%) (24, 26, 31). Another study suggested that the administration of rituximab could delay the immune response to COVID-19 after vaccination for a minimum of 6 months (43). Patients receiving a daratumumab-containing regimen (anti-CD38 monoclonal antibody) had higher seroconversion rate (range 58–69%) (28, 29). Regarding targeted therapies, patients receiving Bruton’s tyrosine kinase inhibitor (BTKi; for example, ibrutinib) or B-cell lymphoma-2 (BCL-2) inhibitor (e.g., venetoclax) had the lowest seroconversion rate (range 12.5%–52% and 13%–52%, respectively) (23, 24, 27, 32, 36). Therefore, considerations of active treatment should be made before administering the COVID-19 vaccine, especially in patients receiving targeted therapy and anti-CD20 monoclonal antibody.
Prophylactic agents are being developed to provide additional protection against COVID-19 in populations at high-risk for severe COVID-19 and vaccine nonresponders. Prophylactic agents available include the monoclonal antibody sotrovimab, tixagevimab-cilgavimab, casirivimab-imdevimab, and bamlanivimab-etesevimab (44). Intramuscular injection of tixagevimab-cilgavimab reduced the risk of symptomatic COVID-19 by 76.7% without any significant AE, as shown in the study by Levin et al. (45). The use of these prophylactic agents could become a solution to the low seroconversion rate in patients with hematological malignancies.
In regard to the safety issue, approximately half of all participants with hematological malignancy reported experiencing mild to moderate AEs. Mild AEs include pain at the injection site, swelling, and erythema followed by general fatigue and muscle pain or arthralgia. Of the moderate AES, most participants experienced fever, malaise, and lymphadenopathy. The hematological malignancy group reported significantly more AEs compared to the control group. However, there were no significant differences in AEs among treatment status, type of cancer, and disease status of patients. Similar results have also been reported by Teh et al. (11) and Fendler et al. (46), who reported mild AEs after vaccination in up to 40%–50% of patients.
To our knowledge, this study is by far the largest systematic review and meta-analysis of SARS-CoV-2 mRNA vaccination for cohorts specifically with hematological malignancies with 15 studies being included. Only studies with healthy subjects as control were included to clearly establish a cause and effect relationship of the independent variable (i.e., having hematological malignancies). Studies including patients with stem cell therapies were also excluded to reduce the chance of heterogeneity, because stem cell therapies are known to have immunomodulatory effects (47). Furthermore, the 15 included studies were found to have a low level of bias when evaluated using the JBI checklist.
Nonetheless, there are also some limitations to this study. This study was limited to a single type of vaccine (mRNA vaccine) to reduce heterogeneity, although there are approximately nine different vaccines currently available. Further research is encouraged to study the efficacy of the other types of vaccines in cohorts with hematological malignancies. Another limitation comes from the heterogeneity found in the meta-analysis. Several sub-analyses, conducted in an effort to explain heterogeneity found in the main meta-analysis, also showed significant heterogeneity and several factors could have caused the heterogeneity in this meta-analysis. Most studies evaluated the BNT 162b2 vaccine (Pfizer), although some also used mRNA-1273 (Moderna) and AZD1222 (AstraZeneca) vaccines, which could have produced different efficacy and safety profiles. The effect of different chemotherapy drugs and the disease status were not quantitatively measured due to the lack of data and the small number of sample sizes. Lastly, there were also differences in the methods used for measurement and cutoff for seropositivity. Although most studies used the Roche Elecsys anti-SARS-CoV-2 immunoassay method with a cutoff of 0.8 U/mL, some used different kits or methods and some even presented their data in different units (see Table 4). The data for control groups were incomplete in some studies; hence, some sub-analysis comparisons were conducted using the overall controls instead of the subgroup-specific controls.
Conclusions
In this systematic review and meta-analysis of 15 studies, including 2,055 patients with hematological malignancies and 1,105 healthy controls, only 61.8% of hematological malignancies seroconverted after two doses of the SARS-CoV-2 vaccine mRNA compared to 97.2% for healthy controls (RR=0.60; 95%CI 0.50–0.71). Our sub-analyses showed that the type of malignancy affected the rate of seroconversion, because NHL patients had the lowest rate (RR 0.5; 95%CI 0.35–0.71), while patients with myelodysplastic syndrome had the highest rate (RR 0.95; 95%CI 0.83–1.08). Additionally, patients actively treated at the time of vaccination had a lower seroconversion rate (RR 0.59; 95%CI 0.46–0.75). Cohorts with hematological malignancies also showed inferior results in quantitative measurement of antibody titers compared to healthy controls. Fortunately, no increased AEs of vaccination were observed in the cohort of hematological malignancies, with most of the AEs being mild and eventually resolved. Thus, despite the increasing number of AEs due to the COVID-19 vaccination, the vaccine is still considerably safe for patients with hematological malignancies.
Undoubtedly, additional studies including larger sample sizes, a standardized measurement method and cutoff, and improved analyzing methods are needed. Observing increased seroconversion rates and antibody titers after the second dose, it will be intriguing to study the benefits of booster doses for specific groups of individuals, including those with hematological malignancies. Furthermore, we hope that the results of this meta-analysis could help policy makers devise better protection strategies for individuals with hematological malignancies, and even for patients who have already been vaccinated, through stricter health protocols, better personal hygiene practices, and further trials of prophylactic agents.
Data availability statement
The original contributions presented in the study are included in the article/supplementary material. Further inquiries can be directed to the corresponding author.
Author contributions
All authors have contributed substantially to the writing of this manuscript, with detailed contribution as follow: Conceptualization, Methodology, Supervision, and Project Administration by IR; Investigation, Writing – Original Draft Preparation, and Visualization by SP, LW, JT, and IW; Writing – Review and Editing by KW. All authors have read and agreed to the published version of the manuscript.
Acknowledgments
The authors wish to extend our gratitude towards all authors of the included articles as the data source for this article.
Conflict of interest
The authors declare that the research was conducted in the absence of any commercial or financial relationships that could be construed as a potential conflict of interest.
Publisher’s note
All claims expressed in this article are solely those of the authors and do not necessarily represent those of their affiliated organizations, or those of the publisher, the editors and the reviewers. Any product that may be evaluated in this article, or claim that may be made by its manufacturer, is not guaranteed or endorsed by the publisher.
References
1. World Health Organization. WHO coronavirus (COVID-19) dashboard with vaccination data . Available at: https://covid19.who.int/.
2. VoPham T, Weaver MD, Hart JE, Ton M, White E, Newcomb PA. Effect of social distancing on COVID-19 incidence and mortality in the US. medRxiv (2020). doi: 10.1101/2020.06.10.20127589
3. Qian M, Jiang J. COVID-19 and social distancing. Z Fur Gesundheitswissenschaften. (2022) 30(1):259. doi: 10.1007/s10389-020-01321-z
4. Mathieu E, Ritchie H, Ortiz-Ospina E, Roser M, Hasell J, Appel C, et al. A global database of COVID-19 vaccinations. Nat Hum Behav (2021) 5(7):947–53. doi: 10.1038/s41562-021-01122-8
5. Korang SK, von Rohden E, Veroniki AA, Ong G, Ngalamika O, Siddiqui F, et al. Vaccines to prevent COVID-19: A living systematic review with trial sequential analysis and network meta-analysis of randomized clinical trials. PloS One (2022) 17(1):e0260733. doi: 10.1371/journal.pone.0260733
6. Hall V, Foulkes S, Insalata F, Kirwan P, Saei A, Atti A, et al. Protection against SARS-CoV-2 after covid-19 vaccination and previous infection. N Engl J Med (2022) 386(13):1207–20. doi: 10.1056/NEJMoa2118691
7. Lee ARYB, Wong SY, Chai LYA, Lee SC, Lee MX, Muthiah MD, et al. Efficacy of covid-19 vaccines in immunocompromised patients: systematic review and meta-analysis. BMJ. (2022) 376:e068632. doi: 10.1136/bmj-2021-068632
8. Ghazy RM, Ashmawy R, Hamdy NA, Elhadi YAM, Reyad OA, Elmalawany D, et al. Efficacy and effectiveness of SARS-CoV-2 vaccines: A systematic review and meta-analysis. Vaccines (2022) 10(3):350. doi: 10.3390/vaccines10030350
9. McDonald I, Murray SM, Reynolds CJ, Altmann DM, Boyton RJ. Comparative systematic review and meta-analysis of reactogenicity, immunogenicity and efficacy of vaccines against SARS-CoV-2. NPJ Vaccines (2021) 6(1):1–14. doi: 10.1038/s41541-021-00336-1
10. Sakuraba A, Luna A, Micic D. Serologic response following SARS-COV2 vaccination in patients with cancer: a systematic review and meta-analysis. J Hematol Oncol (2022) 15(1):15–37. doi: 10.1186/s13045-022-01233-3
11. Teh JSK, Coussement J, Neoh ZCF, Spelman T, Lazarakis S, Slavin MA, et al. Immunogenicity of COVID-19 vaccines in patients with hematologic malignancies: a systematic review and meta-analysis. Blood Adv (2022) 6(7):2014–34. doi: 10.1182/bloodadvances.2021006333
12. Agha M, Blake M, Chilleo C, Wells A, Haidar G. Suboptimal response to COVID-19 mRNA vaccines in hematologic malignancies patients. medRxiv. (2021). doi: 10.1101/2021.04.06.21254949
13. Rotshild V, Hirsh-Raccah B, Miskin I, Muszkat M, Matok I. Comparing the clinical efficacy of COVID-19 vaccines: a systematic review and network meta-analysis. Sci Rep (2021) 11:22777. doi: 10.1038/s41598-021-02321-z
14. Mehrabi Nejad MM, Moosaie F, Dehghanbanadaki H, Haji Ghadery A, Shabani M, Tabary M, et al. Immunogenicity of COVID-19 mRNA vaccines in immunocompromised patients: a systematic review and meta-analysis. Eur J Med Res (2022) 27:23–36. doi: 10.1186/s40001-022-00648-5
15. Cavanna L, Citterio C, Toscani I. Covid-19 vaccines in cancer patients. seropositivity and safety. systematic review and meta-analysis. Vaccines. (2021) 9:1048–1057. doi: 10.3390/vaccines9091048
16. Page MJ, McKenzie JE, Bossuyt PM, Boutron I, Hoffmann TC, Mulrow CD, et al. The PRISMA 2020 statement: an updated guideline for reporting systematic reviews. BMJ. (2021) 372:n71. doi: 10.1136/bmj.n71
17. Shea BJ, Reeves BC, Wells G, Thuku M, Hamel C, Moran J, et al. AMSTAR 2: a critical appraisal tool for systematic reviews that include randomised or non-randomised studies of healthcare interventions, or both. BMJ. (2017) 358:4008. doi: 10.1136/bmj.j4008
18. Joanna Briggs Institute. Checklist for cohort studies (2017). Available at: https://jbi.global/critical-appraisal-tools.
19. Sterne JAC, Savović J, Page MJ, Elbers RG, Blencowe NS, Boutron I, et al. RoB 2: a revised tool for assessing risk of bias in randomised trials. BMJ. (2019) 366:l4898. doi: 10.1136/bmj.l4898
20. Goplen CM, Verbeek W, Kang SH, Jones CA, Voaklander DC, Churchill TA, et al. Preoperative opioid use is associated with worse patient outcomes after total joint arthroplasty: a systematic review and meta-analysis. BMC Musculoskelet Disord (2019) 20(1):234–246. doi: 10.1186/s12891-019-2619-8
21. Melo G, Dutra KL, Rodrigues Filho R, Ortega AOL, Porporatti AL, Dick B, et al. Association between psychotropic medications and presence of sleep bruxism: A systematic review. J Oral Rehabil. (2018) 45(7):545–54. doi: 10.1111/joor.12633
22. Borenstein M, Higgins JPT, Hedges LV, Rothstein HR. Basics of meta-analysis: I 2 is not an absolute measure of heterogeneity. Res Synth Methods (2017) 8(1):5–18. doi: 10.1002/jrsm.1230
23. Parry H, McIlroy G, Bruton R, Ali M, Stephens C, Damery S, et al. Antibody responses after first and second covid-19 vaccination in patients with chronic lymphocytic leukaemia. Blood Cancer J (2021) 11(7):136–144. doi: 10.1038/s41408-021-00528-x
24. Tzarfati KH, Gutwein O, Apel A, Rahimi-Levene N, Sadovnik M, Harel L, et al. BNT162b2 COVID-19 vaccine is significantly less effective in patients with hematologic malignancies. Am J Hematol (2021) 96(10):1195–203. doi: 10.1002/ajh.26284
25. Claudiani S, Apperley JF, Parker EL, Marchesin F, Katsanovskaja K, Palanicawandar R, et al. Durable humoral responses after the second anti-SARS-CoV-2 vaccine dose in chronic myeloid leukaemia patients on tyrosine kinase inhibitors. Br J Haematol (2022) 197(1):e1–4. doi: 10.1111/bjh.18001
26. Perry C, Luttwak E, Balaban R, Shefer G, Morales MM, Aharon A, et al. Efficacy of the BNT162b2 mRNA COVID-19 vaccine in patients with b-cell non-Hodgkin lymphoma. Blood Adv (2021) 5(16):3053–61. doi: 10.1182/bloodadvances.2021005094
27. Herishanu Y, Avivi I, Aharon A, Shefer G, Levi S, Bronstein Y, et al. Efficacy of the BNT162b2 mRNA COVID-19 vaccine in patients with chronic lymphocytic leukemia. Blood. (2021) 137(23):3165. doi: 10.1182/blood.2021011568
28. Pimpinelli F, Marchesi F, Piaggio G, Giannarelli D, Papa E, Falcucci P, et al. Fifth-week immunogenicity and safety of anti-SARS-CoV-2 BNT162b2 vaccine in patients with multiple myeloma and myeloproliferative malignancies on active treatment: preliminary data from a single institution. J Hematol Oncol (2021) 14(1):86–93. doi: 10.1186/s13045-021-01090-6
29. Avivi I, Balaban R, Shragai T, Sheffer G, Morales M, Aharon A, et al. Humoral response rate and predictors of response to BNT162b2 mRNA COVID19 vaccine in patients with multiple myeloma. Br J Haematol (2021) 195(2):186–93. doi: 10.1111/bjh.17608
30. Gavriatopoulou M, Terpos E, Ntanasis-Stathopoulos I, Briasoulis A, Gumeni S, Malandrakis P, et al. Poor neutralizing antibody responses in 106 patients with WM after vaccination against SARS-CoV-2: A prospective study. Blood Adv (2021) 5(21):4398–405. doi: 10.1182/bloodadvances.2021005444
31. Stampfer SD, Goldwater MS, Jew S, Bujarski S, Regidor B, Daniely D, et al. Response to mRNA vaccination for COVID-19 among patients with multiple myeloma. Leukemia. (2021) 35(12):3534–41. doi: 10.1038/s41375-021-01354-7
32. Bergman P, Blennow O, Hansson L, Mielke S, Nowak P, Chen P, et al. Safety and efficacy of the mRNA BNT162b2 vaccine against SARS-CoV-2 in five groups of immunocompromised patients and healthy controls in a prospective open-label clinical trial. EBioMedicine. (2021) 74:103705. doi: 10.1016/j.ebiom.2021.103705
33. Monin L, Laing AG, Muñoz-Ruiz M, McKenzie DR, del Molino del Barrio I, Alaguthurai T, et al. Safety and immunogenicity of one versus two doses of the COVID-19 vaccine BNT162b2 for patients with cancer: interim analysis of a prospective observational study. Lancet Oncol (2021) 22(6):765–78. doi: 10.1016/S1470-2045(21)00213-8
34. Malard F, Gaugler B, Gozlan J, Bouquet L, Fofana D, Siblany L, et al. Weak immunogenicity of SARS-CoV-2 vaccine in patients with hematologic malignancies. Blood Cancer J (2021) 11(8):142-150. doi: 10.1038/s41408-021-00534-z
35. Tvito A, Ronson A, Ghosheh R, Kharit M, Ashkenazi J, Magen S, et al. Anti-CD20 monoclonal antibodies inhibit seropositive response to covid-19 vaccination in non-Hodgkin lymphoma patients within 6 months after treatment. Exp Hematol (2022) 107:20–3. doi: 10.1016/j.exphem.2021.12.396
36. Marasco V, Carniti C, Guidetti A, Farina L, Magni M, Miceli R, et al. T-Cell immune response after mRNA SARS-CoV-2 vaccines is frequently detected also in the absence of seroconversion in patients with lymphoid malignancies. Br J Haematol (2022) 196(3):548–58. doi: 10.1111/bjh.17877
37. Chowdhury SD, Oommen AM. Epidemiology of COVID-19. J Dig Endosc. (2020) 11(1):3. doi: 10.1055/s-0040-1712187
38. Chavez-Macgregor M, Lei X, Zhao H, Scheet P, Giordano SH. Evaluation of COVID-19 mortality and adverse outcomes in US patients with or without cancer. JAMA Oncol (2022) 8(1):69–78. doi: 10.1001/jamaoncol.2021.5148
39. World Health Organization. COVID-19 vaccines (2022). Available at: https://www.who.int/emergencies/diseases/novel-coronavirus-2019/covid-19-vaccines.
40. Chemaly RF, Ghosh S, Bodey GP, Rohatgi N, Safdar A, Keating MJ, et al. Respiratory viral infections in adults with hematologic malignancies and human stem cell transplantation recipients: a retrospective study at a major cancer center. Med (Baltimore). (2006) 85(5):278–87. doi: 10.1097/01.md.0000232560.22098.4e
41. Richardson S, Hirsch JS, Narasimhan M, Crawford JM, McGinn T, Davidson KW, et al. Presenting characteristics, comorbidities, and outcomes among 5700 patients hospitalized with COVID-19 in the new York city area. JAMA. (2020) 323(20):2052–9. doi: 10.1001/jama.2020.6775
42. Wu Z, McGoogan JM. Characteristics of and important lessons from the coronavirus disease 2019 (COVID-19) outbreak in China: Summary of a report of 72 314 cases from the Chinese center for disease control and prevention. JAMA. (2020) 323(13):1239–42. doi: 10.1001/jama.2020.2648
43. Cattaneo C, Cancelli V, Imberti L, Dobbs K, Sottini A, Pagani C, et al. Production and persistence of specific antibodies in COVID-19 patients with hematologic malignancies: role of rituximab. Blood Cancer J (2021) 11(9):1–7. doi: 10.1038/s41408-021-00546-9
44. Owen C, Robinson S, Christofides A, Sehn LH. A Canadian perspective: Monoclonal antibodies for pre- and post-exposure protection from COVID-19 in vulnerable patients with hematological malignancies. Curr Oncol (2022) 29(6):3940. doi: 10.3390/curroncol29060315
45. Levin MJ, Ustianowski A, De Wit S, Launay O, Avila M, Templeton A, et al. Intramuscular AZD7442 (Tixagevimab–cilgavimab) for prevention of covid-19. N Engl J Med (2022) 386(23):2188–200. doi: 10.1056/NEJMoa2116620
46. Fendler A, de Vries EGE, GeurtsvanKessel CH, Haanen JB, Wörmann B, Turajlic S, et al. COVID-19 vaccines in patients with cancer: immunogenicity, efficacy and safety. Nat Rev Clin Oncol (2022) 19:385–401. doi: 10.1038/s41571-022-00610-8
Keywords: COVID-19, mRNA vaccine, hematologic malignancies, seroconversion rates, antibody titers, adverse effects
Citation: Rinaldi I, Pratama S, Wiyono L, Tandaju JR, Wardhana IL and Winston K (2022) Efficacy and safety profile of COVID-19 mRNA vaccine in patients with hematological malignancies: Systematic review and meta-analysis. Front. Oncol. 12:951215. doi: 10.3389/fonc.2022.951215
Received: 23 May 2022; Accepted: 11 July 2022;
Published: 05 August 2022.
Edited by:
Giuseppe Maurizio Campo, University of Messina, ItalyCopyright © 2022 Rinaldi, Pratama, Wiyono, Tandaju, Wardhana and Winston. This is an open-access article distributed under the terms of the Creative Commons Attribution License (CC BY). The use, distribution or reproduction in other forums is permitted, provided the original author(s) and the copyright owner(s) are credited and that the original publication in this journal is cited, in accordance with accepted academic practice. No use, distribution or reproduction is permitted which does not comply with these terms.
*Correspondence: Ikhwan Rinaldi, aWtod2FucmluYWxkaUBnbWFpbC5jb20=