- Department of Gastrointestinal Surgery, The Second Hospital, Cheeloo College of Medicine, Shandong University, Jinan, China
Primary liver cancer is the sixth most frequently diagnosed cancer worldwide and the third leading cause of cancer-related death. The majority of the primary liver cancer cases are hepatocellular carcinoma and intrahepatic cholangiocarcinoma. Worldwide, there is an increasing incidence of primary liver cancer cases due to multiple risk factors ranging from parasites and viruses to metabolic diseases and lifestyles. Often, patients are diagnosed at advanced stages, depriving them of surgical curability benefits. Moreover, the efficacy of the available chemotherapeutics is limited in advanced stages. Furthermore, tumor metastases and recurrence make primary liver cancer management exceptionally challenging. Thus, exploring the molecular mechanisms for the development and progression of primary liver cancer is critical in improving diagnostic, treatment, prognostication, and surveillance modalities. These mechanisms facilitate the discovery of specific targets that are critical for novel and more efficient treatments. Consequently, the Hippo signaling pathway executing a pivotal role in organogenesis, hemostasis, and regeneration of tissues, regulates liver cells proliferation, and apoptosis. Cell polarity or adhesion molecules and cellular metabolic status are some of the biological activators of the pathway. Thus, understanding the mechanisms exhibited by the Hippo pathway is critical to the development of novel targeted therapies. This study reviews the advances in identifying therapeutic targets and prognostic markers of the Hippo pathway for primary liver cancer in the past six years.
Introduction
Worldwide, primary liver cancer is the sixth most frequently diagnosed malignancy and the third leading cause of cancer-related mortality. Primary liver malignancies comprise hepatocellular carcinoma, which accounts for 75-85% of the cases, and cholangiocarcinoma (CCA), accounting for 10-15% of the cases, plus other rare subtypes. Several risk factors for primary liver malignancies have been pointed out, ranging from metabolic diseases, viral infections, parasitic infections, food toxins, and lifestyle (1). Of note, primary liver cancer has seen a rapidly growing pace with the reported aggressive nature of the disease and difficulties in treatment (2). Most patients with primary liver cancer present with advanced disease due to the asymptomatic nature of disease. Moreover, primary liver cancers have diverse and complex molecular pathogenetic patterns that render the disease hard to treat and with high recurrences.
The Hippo pathway regulates cell proliferation and programmed cell death and maintains tissue homeostasis and stem cell function. Several upstream and downstream regulators comprising a kinase cascade chain are responsible for the functionality of the Hippo signaling system (3, 4). The core kinases include the mammalian STE20-like protein kinase (MST1/2) and the large tumor suppressor kinase 1/2 (LATS1/2). The two primary downstream regulators of the Hippo pathway are Yes-associated protein (YAP) and PDZ binding motif (TAZ). In contrast, the upstream effectors include Kidney and brain expressed protein (KIBRA), Ajuba, FAT1-4, Ras association domain family (RASSF), and Merlin. When MST1/2 binds to human Salvador 1 (SAV1), it causes phosphorylation and activation of LATS1/2. Subsequently, LATS1/2 phosphorylates and secludes YAP and TAZ by facilitating YAP/TAZ association with 14-3-3 proteins in the cytoplasm. Furthermore, MST1/2 can interact with the Monopolar spindle 1-binder kinase (MOB1), which also regulates LATS1/2 activity. YAP1 is phosphorylated and retained in the cytoplasm when the Hippo pathway is activated. Contrary, the Hippo pathway’s repression causes YAP/TAZ dephosphorylation and their translocation into the nucleus. Eventually, Yap1/TAZ interact with other transcription factors such as TEA domain (TEAD), Runt-related transcription factor (RUNX), and SMAD to induce the expression of genes such as CTGF, Survivin, CYR61, and JAG1, that facilitate migration and proliferation of tumor cells, and inhibition of apoptosis (5–12).
YAP overexpression has been associated with poor survival rates, intrahepatic metastases, vascular invasion, tumor size, diversity, and liver cirrhosis in patients with primary liver cancers (13, 14). Furthermore, among HCC experimental models, YAP/TAZ peritumoral activity tends to exhibit tumor-suppressive roles, and dual suppression of PI3KCA/YAP expression has been associated with the death of HCC and CCA cells (15, 16). Thus, this study examines publications on the Hippo signaling pathway identifying novel targets with prognostic and therapeutic potential for HCC and cholangiocarcinoma in the past six years.
Hepatocellular adenocarcinoma
YAP/TAZ optimization for therapeutic purposes
Reorganizing YAP/TAZ signaling and targeting Yap has been proposed to abrogate Sorafenib resistance. YAP/TAZ promotes Sorafenib-induced ferroptosis resistance via a TEAD-dependent route by three mechanisms, which include induced expression of SLC7A11, increased activating transcription factor 4 (ATF4) activity, and upregulation of Survivin gene expression (17, 18). Furthermore, targeting TAZ, which also regulates the BCL2L12 gene, represents a promising drug target among patients with c-myc-induced HCC patients (19). Amino acid metabolism is an essential aspect of cancer biologics. YAP and TAZ have been reported to control cancer metabolism by increasing the uptake of amino acids via SLC38A1 and SLC7A5 transporters, which are thought to be potential treatment targets (20). It has also been observed that, among YAP-positive malignancies, targeting α2β1 integrin and NUAK family kinase 2 (NUAK2) expression blocks tumor progression by inhibiting the MST-YAP cascade and actin-myosin activity (21–23).
Interestingly, some scientists observed that dual suppression of YAP and TAZ expression in hypoxic carcinoma cells results in increased apoptosis of cancer cells (24). The targeting of tumor lineage plasticity mechanism of HCC involving an interactive axis (CLDN6/TJP2/YAP1) has shown improved antitumor efficacy of a de novo anti-CLDN6 (claudin 6) monoclonal antibody conjugated to a cytotoxic agent, Mertansine DM1 (CLDN6-DM1) as a monotherapy or combined with Sorafenib (25). Lastly, the repression of TAZ expression by Diosgenin, a phytosteroid sapogenin, is reportedly an effective antitumor therapy working via apoptosis induction, cell migration/invasion repression, and cell proliferation inhibition (26) (Table 1).
Significant long chain noncodingRNAs (lncRNAs) in HCC treatment
Long-chain noncoding RNAs play crucial roles in regulating microRNAs that are involved in carcinogenesis. The distortion of their activity as regulators of cancer predisposes to cancer growth. Thus, novel lncRNAs mediating their effects by CUL4A-mediated ubiquitination of large tumor suppressor kinase 1 (LATS1), enhancing YAPS127 phosphorylation, and activating the tumor-suppressive Hippo pathway (miR-106b-5p/LATS1) have been deemed potential HCC treatment options leading to tumor growth inhibition (27, 28) (Table 1).
Drugs and plant derivatives with therapeutic actions on HCC
Drugs such as Metformin, Artemisinin, Evodiamine, tankyrase inhibitors, Xiaoping, Wogonin, statin, and Dercusin have been deemed promising agents in treating HCC. These drugs exert their antitumor effects via mechanisms such as inhibition of IL-2 and LATS1 expression, Mst1/2 activation, and upregulation of LATS1 phosphorylation, N-cadherin-Snail-E-cadherin axis regulation, proliferation suppression, apoptosis induction, angiomotin-like protein 1/2 (AMOTL1/2) upregulation, Hippo, Wnt, hedgehog pathway, and cell cycle inhibition (Figure 1). Statins consumption, in particular, is associated with prolonged recurrence-free survival (29–36). In research reports from other investigators, several drug combinations with improved antitumor efficacy exhibited tumor growth suppression and enhanced apoptosis, such as a pan-inhibitor of Aurora Kinases (SNS-314) and Hippo pathway inhibitors and Hypocrellin A and Oleanolic acid, have been proposed (37, 38). Furthermore, in myc/Ras-induced HCC, a combination of tadalafil (a phosphodiesterase 5 inhibitor) and JQ1 (Bromodomain and Extra-Terminal domain inhibitor) evades the BET inhibitor’s resistance influenced by YAP/TAZ expression (39). The antitumor activity of Cisplatin in HCC improved when it was administered with Melatonin due to downregulation of YAP and caspase-3 and poly ADO-ribosome polymerase cleavage (40).
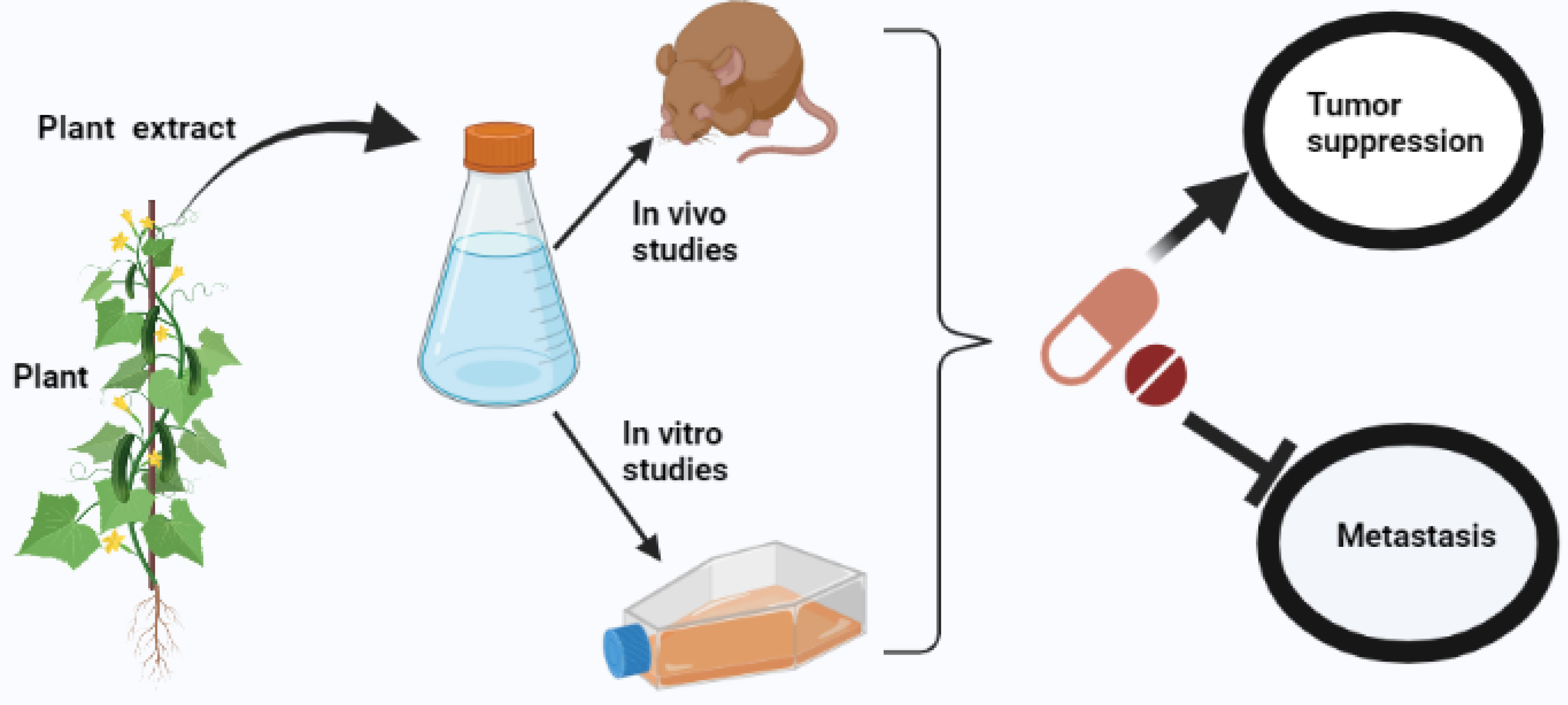
Figure 1 Illustrating the activity of antitumor drugs in a mouse model and human primary liver cancer cells.
Moreover, targeting the S100A1 gene seemed to enhance Cisplatin’s inhibitory effects (41). Reportedly, reactive oxidative species (H2O2)-induced actuation of YAP1 by the c-Myc pathway represents a possible treatment option that rejuvenates the unfolded protein pathway (42) (Table 2). Recently, α-hederin has been reported as an agonist of the Hippo pathway that augments effects such as apoptosis, proliferation inhibition, YAP nuclear levels reduction, and upregulates the Hippo pathway-related proteins and genes. Notably, α-hederin suppressed tumor growth and weight in the mouse model (43). Finally, targeting RNA-binding protein Dnd1 suppresses spheroid formation and expression of stemness-related genes and enhances Sorafenib sensitivity making it a probable drug target for HCC treatment (44) (Table 2).
Significant interacting pathways for HCC treatment
Interacting pathways play critical roles in hepatocellular carcinogenesis, and their optimization provides opportunities for developing targeted therapeutic strategies. The inhibition of YAP/AKT in the Hippo/PI3K-PTEN-mTOR pathways and regulation of the Hippo/YAP and PI3K/AKT pathways are associated with induced apoptosis and suppressed tumor growth by FR5 compound and poplar propolis extract (45, 46). Blockage of a feedback loop involving CD44S and YAP1 (CD44S regulates YAP expression via PI3K/AKT pathway and YAP/TEAD axis regulates CD44S) inhibits vascular invasion and more severe form of liver cirrhosis (47). Furthermore, utilizing genetic or pharmacologic blockage involving HMGP1/YAP/HIGF1α, MTA2-FRDM6-Hippo, COX-2-PGE2-EP2-Gαs-β-catenin-YAP-COX-2, and their respective targets (i.e., high mobility group box one protein (HMGB1), metastatic associated protein 2 (MTA2), cyclooxygenase 2 (COX-2) &YAP) prevents tumorigenesis, excessive glycolysis, and metastases. Lastly, utilizing a FUS-LATS1/2 axis inhibited HCC progression by activating the Hippo pathway (48–51) (Table 1).
MicroRNAs in HCC treatment
The upregulation of some microRNAs is associated with tumor proliferation, epithelial-mesenchymal transition, and metastases. Some recently documented mechanisms for these events include inactivation of the Hippo/Yap via paired box 5 (PAX5), Protein tyrosine phosphatase receptor type B (PTPRB) downregulation, and LATS2 inhibition. Thus, targeting specific miRNA molecules (i.e. miR-1254, miR-665, miR-103) propagating tumorigenesis provides novel drug options against HCC (52–54). Nonetheless, the overexpression of other downregulated miRNAs is associated with inhibiting hepatocarcinogenesis via downregulation of YAP1, DNA methyltransferase 3 beta (DNMT3B) upregulation leading to LATS1 methylation, S-phase arrest through upregulating p21 and p27 expression, and inhibiting PI3K/AKT pathway (55–57). Reportedly, a microRNA-590-5p represses Adriamycin chemoresistance via Yap expression regulation (58). Noteworthy, miR-21 deficiency is associated with tumorigenesis through increased oncogenes expression and minute dysregulation of the Hippo signaling pathway, signal transducer and activator of transcription factor 3 (STAT3), and mitogen-activated protein kinase (MAPK) pathways. Hence, prudence is recommended in adopting miR-21 inhibitors in treating liver cancer (59) (Table 1).
Proposed therapeutic targets for HCC
Seemingly, Septin 6 (SEPT6), Frizzled-2, MORC Family cw-Type Zinc Finger 2 (MORC2), Ubiquitin-specific protease 11 (USP11), Yki/Yap-Src42A/SRC positive feedback loop, m6A methyltransferase 3 (METTL3), and family with sequence similarity 83 member D (FAM83D) play crucial roles in HCC tumorigenesis by promoting vascular mimicry, cell stemness, migration, invasion, and silencing of the Hippo pathway by DNA methylation-dependent mechanism. Targeting these genes shows prospective benefits such as preventing disease progression, recurrence post-transplantation, and metastases. Besides, USP11 overexpression is linked with a 5-fold risk of all-cause-related mortality (60–65). Furthermore, other crucial targets and their regulatory loops representing potential therapeutic options for HCC have been reported, including Phospholipase 1 inhibited by T-box transcription factor 3 (TBX3), YAP-dependent monocyte chemoattractant protein 1 (MCP-1) in a protumoral microenvironment, Meis homeobox 2C/D (MEIS2C/D) activating Wnt/β-catenin and inhibiting Hippo pathway, and the YAP/nuclear receptor 4A1 (NR4A1) (66–69) (Table 1).
Strategically, the combined use of inhibitors of YAP and epidermal growth factor receptor (EGFR) targeting the EGFR-PI3K-PDK1 pathway shows improved cytotoxicity for HCC cells (70). Optimizing the tumor-suppressive effects of potassium channel tetramerization domain containing 11 (KCTD11) (by p21 activation and suppression of cell cycle proteins) and LATS1 overexpression with YAP1 nucleocytoplasmic translocation by tumor growth factor-beta 1 (TGF- β1) inhibit HCC cells growth and development (71, 72). Further, the suppression of LIM domain only 3 (LMO3) expression exerting its actions via the LATS1/Hippo pathway evades invasion and metastasis by cancer cells (73). Otherwise, actinin alpha 1 (ACTN1) expression, acyl-CoA dehydrogenase Long-chain (ACADL)/YAP, and YAP/Forkhead Box M1 (FOXM1) are proposed targets for preventing tumor growth and early recurrence of HCC (74–76) (Table 1).
The inhibition of cell growth, migration, and proliferation, as well as disruption of cytoskeleton function, prevent tumorigenesis. Accordingly, several investigators have revealed that targeting NatB-mediated protein N-α-terminal acetylation (NATB) expression, Neuronally Expressed Developmentally Downregulated 4 (NEDD4)/LATS1 pathway, Herpesvirus-associated ubiquitin-specific protease (HAUSP) expression, Cip1-interacting zinc finger protein 1 (CIZ1) expression, and phosphorylated-ezrin (p-Ezrin) effectively arrested carcinogenesis in HCC (77–81). Further, YAP targeting has shown suppression of cancer cell growth in patients with hypoxia-mediated HCC metabolism and HBX-induced HCC (82, 83). Specific targeting of tumor-initiating cells (TICs) seems to overcome resistance to antiangiogenic therapy in HCC. Moreover, TICs have been shown to recruit tumor-infiltrated type II macrophages in the early phase; thus, suppressing TICs via YAP or M2 macrophages is a valuable treatment option in HCC (84, 85) (Table 1).
Several other targets inhibit HCC cells proliferation, growth, and migration and induce apoptosis through different mechanisms such as RSPO2/Hippo/Yap and S1P2-induced Yap activation, EGF-induced Erb-B2 receptor Tyrosine Kinase 2 (ErBB2) and PI3K/AKT activation, and Junctional Cadherin 5 Associated with coronary artery disease (JCAD)/LATS1 interaction (86–89). Furthermore, targeting Hepatitis B X-interacting protein (HBXIP), which potentiates its effects by upregulating YAP through the transcription factor c-myb coactivation in HCC cells, prevents cancer cell proliferation (90). Succinctly, Hypoxia-inducible factor-2α is a potential antitumor target that facilitates NASH-induced hepatocarcinogenesis progression, and HIF-2α inhibitors reportedly block this activity (91). Recently, suppression of STK25 expression in HCC cell lines has been proposed as a new treatment target among cancers expressing miR-4800-3p (92). Lastly, exploring potential Scrib agonists may provide potent antitumor drugs as Scrib expression inhibits tumor cell proliferation via repression of Yap, c-Myc, and cyclin D1 (93) (Table 1).
Prognostic markers for progression, survival, recurrence or metastasis
Cytoplasmic YAP and nuclear TAZ expression in Keratin 19 negative HCC patients is associated with poor overall and disease-free survival (94). The overexpression of YAP leads to Plasminogen activating inhibitor-1 (PAI-1) overexpression, which is associated with poor survival and early recurrence rates (23). High YAP and low glutathione peroxidase 4 (GPX4) expression are associated with Sorafenib treatment’s increased survival. Further, YAP signaling modifications present a potential biomarker for tumor ferroptosis-induced response prediction (95). Nevertheless, YAP and Src homology phosphotyrosine phosphatase 2 (SHP2) expression represents unfavorable prognostic indicators with poor overall and recurrence-free survival (96). Other scientists also observed that overexpression of c-Src had a negative correlation with patient survival (97). A group of investigators noted that Yap/TAZ expression in HCC was associated with high serum alpha-fetoprotein levels, increased proliferation activity, microvascular invasion, and stemness and epithelial-mesenchymal transition-related expression markers such as SMAD2/3, CAIX, and p53. Consequently, they proposed consideration for the status of the hypoxia markers when using YAP/TAZ to determine the behavior of HCC (98). Notably, a prognostic nomogram based on five Hippo-related genes (i.e., the master regulator of cell cycle and proliferative metabolism (MYC), neurofibromatosis 2 (NF2), misshapen-like kinase 1 (MINK1), baculoviral IAP repeat-containing 3 (BIRC3), and casein kinase 1 epsilon (CSNK1E)) has been proposed, outperforming available clinical parameters in the prognostication of HCC (99). PDZ and LIM domain 1 (PDLIM1), ACTN1, mitochondrial ACADL, and matricellular spondin-2 (SPON2) expressions involved in mechanisms such as enhancing the Hippo pathway activity, reducing RhoA GTPases activity, repressing cell proliferation, repressing tumor growth, facilitating interaction with MOB1, decreasing phosphorylation of LATS1 and YAP, M1-macrophage recruitment facilitation, and suppression hepatocellular carcinoma metastases have been implicated in prognosticating HCC (75, 100–102) (Table 1).
Among HBV-induced HCC patients, Plasminogen expression inhibits cell apoptosis and enhances cell line growth through upregulation of the SRC gene and the inhibition of the Hippo signaling pathway (103). Expression of a genetic variant of LATS1 (LATS1 rs7317471) in HCC patients exhibiting age below 53 years, female gender, smoking, alcohol drinking, and Barcelona clinic liver cancer stage B is associated with decreased risk of death (104). Low AT-Rich interaction Domain 1A (ARID1A), downregulation of Retinal dehydrogenase 5 (visual cycle enzyme), and repressed Mitochondrial Amidoxime Reducing Component 2 (MARC2) expression have been associated with poor overall and disease-free survival, metastasis, and disease progression. These observations were mediated via mechanisms, including; immune activity regulation, regulation of genes related to HCC development, regulation of the epithelia-mesenchymal-transition process, regulation of p27 levels, and regulation of HNF4A expression (105–107). Loss of Liver kinase B1 expression correlates with migration and invasion of liver cancer cells via the ZEB1-induced Yap signaling (108).
Meanwhile, overexpression of genes related to immune infiltration, actin stress fiber congregation, cell migration, and invasion leads to poor overall clinical outcomes and disease-free survival (109, 110). TNF Alpha Induced Protein 8 (TNFAIP8), novel enhancer RNA (SPRY4-AS1), TEA Domain Transcription factor 1 (TEAD), DNMT3B, and Stathmin overexpression were associated with recurrence, poor disease-free, progression-free, relapse-free, and overall survival. These genes propagated the poor outcomes via mechanisms such as inhibition of YAP phosphorylation, decreasing LATS1 phosphorylation, increasing iron accumulation and consequent oxidative injury, and activity of several infiltrating immune cells (111–115). Researchers noted that FAM83D and NEK2 genes were related to high recurrences, poor survival, and metastases following liver transplantation and hepatectomy. Apart from MAPK and TGF-beta, FAM83D enhanced CD44 expression and CD44-cancer stem cell malignancy. Regarding NEK2, mechanistic studies revealed that EMT was essential in NEK2-induced HCC cell invasion (116, 117). Furthermore, an interplay between YAP and FOXM1 leading to chromosomal instability has been associated with poor survival, and early recurrences. Otherwise, inactivation of the Hippo pathway has been linked to overall poor prognosis (76, 118) (Table 1).
The overexpression of Rac GTPase activating protein 1 is reportedly associated with shorter survival from enhanced cytokinesis and suppressed apoptosis. Aurora A/B co-overexpression is associated with the worst prognosis among HCC patients (37, 119). Monoacylglycerol lipase overexpression has been linked with proliferation and invasion of HCC cells via Prostaglandin E2 and Lysophosphatidic acid mechanisms (120). Mps one binder kinase activator-like 2 (MOB2) expression influences migration and invasion of cancer cells and may represent a valuable marker of disease progression (121). Notably, silencing of Hippo signature (SOH) is associated with a poorer prognosis than non-silencing in HCC patients. SOH was determined as an independent predictor of poor prognosis on multivariate analysis (118). Nonetheless, the expression of WW and C2 Domain Containing 2 (WWC2) is associated with better 5-year overall survival among HCC patients (122) (Table 1).
Cholangiocarcinoma
Proposed therapeutic targets for CCA treatment
Identifying new molecular targets that may potentially improve the treatment of cholangiocarcinoma has been an unceasing expedition (123). Furthermore, molecules that propagate cancer cell growth, migration, metastasis, or proliferation are indispensable in achieving intent to cure goals. As such, investigators observed that depleting myeloid leukemia 1 (Mcl-1) expression is associated with increased cell death in CCA cells. Accordingly, administering a pan-Fibroblast growth factor receptor (FGFR) inhibitor in YAP expressing cells was associated with cancer cell death. Similar effects were observed with the inhibition of the platelet-derived growth factor receptor (PDGFR) in YAP-expressing cells. Thus, YAP expression may be adopted in assessing FGFR therapies response, and the PDGFR-SFK cascade regulating YAP activation presents a novel treatment strategy (124, 125). Targeting LncRNA MNX1-AS1 expression, which exerts its actions via MNX1-AS1/c-Myc and MAZ/MNX1/Ajuba/Hippo pathway, correlates with tumor growth, and migration, and metastasis inhibition (126). Moreover, inhibiting genes that downregulate the miR-29-3p family or upregulation of transcription factor SP1 may prevent the malignant transformation of ICC cells by expressing ITGA6/ITGB1 genes (127) (Table 2).
The inactivation of a mechanosensitive ion channel Piezo 1 (acting through the Hippo/YAP axis) and its downstream effectors, and the inhibition of expression of several other genes, including; FOXM1 (regulator of CIN25 gene), Agrin, histone methyltransferase G9a, Microfibril associated protein 5 (MFAP5) (transcriptional target of YAP/TEAD), and TAZ appears to prevent metastases, induce cell death, and suppress proliferation. Moreover, targeting these molecules inhibited colony formation, migration, invasion, tumor angiogenesis, and enhanced vitamin D3-sensitivity via the p53/CYP24A1 pathway. Some of the mechanisms identified include decreased H3K9me2, restoration of LATS1, YAP activity inhibition, and TAZ inhibition (128–133). Furthermore, inhibition of a Hippo-pathway-related long noncoding RNA that interacts with mTORC1 subunit Raptor is associated with suppression of tumor growth, and YAP/TAZ-directed therapies have shown benefits in treating CCA patients with chromosomal instability (134, 135) (Table 2).
Drugs and plant derivatives for tumor growth suppression and metastasis prevention
Licochalcone A compound and antiparasitic macrolide lactones (AML) combined with TGF- β pathway inhibitor repress Yap expression and transcriptional tendency via separate mechanisms that ultimately prevent tumor growth. Licochalcone A suppresses PES1 expression and nuclear localization while AML targets YAP/TAZ activity (136, 137). A group of investigators reported improved chemosensitivity of conventional therapies for CCA when administered concomitantly with histone deacetylase (HDAC) inhibitor that also allowed dose reduction. The drugs used in the study were gemcitabine, cisplatin, 5-fluorouracil (5-FU), oxaliplatin, or gemcitabine plus cisplatin (138),. Further, decreased phosphatase SPH2 activity in cholangiocarcinoma patients can induce chemotherapy resistance through the MCL1-mediated pathway. Thus, targeting the MCL1 pathway provides promising treatment alternatives for patients exhibiting chemotherapy resistance from low SPH2 (139) (Table 3).
Prognostic markers for survival, recurrence, and metastases
Among post-radical CCA resection patients, high Lymphocyte-specific protein tyrosine kinase (LCK) and Circular Alpha-actinin-4 (circACTN4) expression are related to early tumor recurrence and worse prognosis, respectively. High circACTN4 expression is associated with a worse prognosis as it enhances proliferation and metastases by molecular miR-424-5p sponging and interacts with Y-box homolog protein 1 (74, 140). Furthermore, YAP/TAZ dual positivity following tumor resection correlates with poor overall and disease-free survival typified by worse TNM stages, poor tissue differentiation, and high CA19-9 levels (141). Besides, YAP overexpression in CCA is associated with poor overall survival (142, 143). Lastly, DEP Domain Containing 1 (DEPDC), Fucosyltransferase 4 FUT4, Midkine (MDK), Phosphofurin acidic cluster sorting protein 1 (PACS1), Piwi-like protein 4-Homo sapiens 1 (PIWIL4) genes, miR-22, miR-551b, and cg27362525 and cg26597242 CpG have been proposed as potential prognostic markers for cholangiocarcinoma (144) (Table 2).
Conclusion
Although primary liver cancer remains challenging in its management, the newly proposed targets potentiating their effects via tumor cell viability, proliferation, migration, and apoptosis holds promising outcomes in treating and prognosticating the disease. Furthermore, targeting the identified molecules in this study led to inhibition of tumor cell growth and migration and activated the apoptosis of the tumor cells. Additionally, the new targets effectively predicted the prognosis of patients with primary liver cancer in terms of metastases risk, disease-free, progression-free, and overall survival. Currently, YAP and TAZ expression serve that purpose. The discovery of new targets should be coupled with developing novel nomograms that are key to predicting the prognosis in primary liver cancer patients. These nomograms should incorporate individual risk factors likely to influence treatment outcomes in different patients. Furthermore, it is commendable to validate further the significance and applicability of these new targets identified as a critical phase towards their drugability trials.
Author contributions
GM designed and drafted the manuscript. GM, Z-PX, J-JL, TW, and YD discussed and revised the manuscript. All authors contributed to the article and approved the submitted version.
Acknowledgments
The authors extend their gratitude to the working staff of the Gastrointestinal Surgery Department of The Second Hospital of Shandong University.
Conflict of interest
The authors declare that the research was conducted in the absence of any commercial or financial relationships that could be construed as a potential conflict of interest.
Publisher’s note
All claims expressed in this article are solely those of the authors and do not necessarily represent those of their affiliated organizations, or those of the publisher, the editors and the reviewers. Any product that may be evaluated in this article, or claim that may be made by its manufacturer, is not guaranteed or endorsed by the publisher.
Glossary
References
1. Sung H, Ferlay J, Siegel RL, Laversanne M, Soerjomataram I, Jemal A, et al. Global cancer statistics 2020: GLOBOCAN estimates of incidence and mortality worldwide for 36 cancers in 185 countries. CA Cancer J Clin (2021) 71:209–49. doi: 10.3322/caac.21660
2. Anstee QM, Reeves HL, Kotsiliti E, Govaere O, Heikenwalder M. From NASH to HCC: Current concepts and future challenges. Nat Rev Gastroenterol Hepatol (2019) 16:411–28. doi: 10.1038/s41575-019-0145-7
3. Meng P, Zhang YF, Zhang W, Chen X, Xu T, Hu S, et al. Identification of the atypical cadherin FAT1 as a novel glypican-3 interacting protein in liver cancer cells. Sci Rep (2021) 11:40. doi: 10.1038/s41598-020-79524-3
4. Meng Z, Moroishi T, Guan KL. Mechanisms of hippo pathway regulation. Genes Dev (2016) 30:1–17. doi: 10.1101/gad.274027.115
5. Lee NH, Kim SJ, Hyun J. MicroRNAs regulating hippo-YAP signaling in liver cancer. Biomedicines (2021) 9(4):347. doi: 10.3390/biomedicines9040347
6. Shan L, Jiang H, Ma L, Yu Y. Yes-associated protein: A novel molecular target for the diagnosis, treatment and prognosis of hepatocellular carcinoma. Oncol Lett (2017) 14:3291–6. doi: 10.3892/ol.2017.6622
7. Qian X, Zhang W, Yang H, Zhang L, Kang N, Lai J. Role of yes-associated protein-1 in gastrointestinal cancers and hepatocellular carcinoma. Explor Res Hypothesis Med (2021) 6:110–7. doi: 10.14218/ERHM.2021.00017
8. Quan Y, Li Z, Zhu K, Liang J. Transcatheter arterial chemoembolization combined with Hippo/YAP inhibition significantly improve the survival of rats with transplanted hepatocellular carcinoma. Lipids Health Dis (2021) 20:74. doi: 10.1186/s12944-021-01486-w
9. Cunningham R, Hansen Carsten G. The hippo pathway in cancer: YAP/TAZ and TEAD as therapeutic targets in cancer. Clin Sci (2022) 136:197–222. doi: 10.1042/CS20201474
10. Liu Y, Wang X, Yang Y. Hepatic hippo signaling inhibits development of hepatocellular carcinoma. Clin Mol Hepatol (2020) 26:742–50. doi: 10.3350/cmh.2020.0178
11. Ma J, Huang X. Research progress in role of hippo signaling pathway in diagnosis and treatment for hepatocellular carcinoma. Zhong Nan Da Xue Xue Bao Yi Xue Ban (2021) 46:637–43. doi: 10.11817/j.issn.1672-7347.2021.200243
12. Zhang S, Zhou D. Role of the transcriptional coactivators YAP/TAZ in liver cancer. Curr Opin Cell Biol (2019) 61:64–71. doi: 10.1016/j.ceb.2019.07.006
13. Wu H, Liu Y, Jiang XW, Li WF, Guo G, Gong JP, et al. Clinicopathological and prognostic significance of yes-associated protein expression in hepatocellular carcinoma and hepatic cholangiocarcinoma. Tumour Biol (2016) 37:13499–508. doi: 10.1007/s13277-016-5211-y
14. LaQuaglia MJ, Grijalva JL, Mueller KA, Perez-Atayde AR, Kim HB, Sadri-Vakili G. YAP subcellular localization and hippo pathway transcriptome analysis in pediatric hepatocellular carcinoma. Sci Rep (2016) 6:30238. doi: 10.1038/srep30238
15. Moya IM, Castaldo SA, Van den Mooter L, Soheily S, Sansores-Garcia L, Jacobs J, et al. Peritumoral activation of the hippo pathway effectors YAP and TAZ suppresses liver cancer in mice. Science (2019) 366:1029–34. doi: 10.1126/science.aaw9886
16. Li X, Tao J, Cigliano A, Sini M, Caideraro J, Azoulay D, et al. Co-Activation of PIK3CA and yap promotes development of hepatocellular and cholangiocellular tumors in mouse and human liver. Oncotarget (2015) 6:10102–15. doi: 10.18632/oncotarget.3546
17. Gao R, Kalathur RKR, Coto-Llerena M, Ercan C, Buechel D, Shuang S, et al. YAP/TAZ and ATF4 drive resistance to sorafenib in hepatocellular carcinoma by preventing ferroptosis. EMBO Mol Med (2021) 13:e14351. doi: 10.15252/emmm.202114351
18. Sun T, Mao W, Peng H, Wang Q, Jiao L. YAP promotes sorafenib resistance in hepatocellular carcinoma by upregulating survivin. Cell Oncol (Dordr) (2021) 44:689–99. doi: 10.1007/s13402-021-00595-z
19. Wang H, Zhang S, Zhang Y, Jia J, Wang J, Liu X, et al. TAZ is indispensable for c-MYC-induced hepatocarcinogenesis. J Hepatol (2022) 76:123–34. doi: 10.1016/j.jhep.2021.08.021
20. Park YY, Sohn BH, Johnson RL, Kang MH, Kim SB, Shim JJ, et al. Yes-associated protein 1 and transcriptional coactivator with PDZ-binding motif activate the mammalian target of rapamycin complex 1 pathway by regulating amino acid transporters in hepatocellular carcinoma. Hepatology (2016) 63:159–72. doi: 10.1002/hep.28223
21. Yuan WC, Pepe-Mooney B, Galli GG, Dill MT, Huang HT, Hao M, et al. NUAK2 is a critical YAP target in liver cancer. Nat Commun (2018) 9:4834. doi: 10.1038/s41467-018-07394-5
22. Wong KF, Liu AM, Hong W, Xu Z, Luk JM. Integrin α2β1 inhibits MST1 kinase phosphorylation and activates yes-associated protein oncogenic signaling in hepatocellular carcinoma. Oncotarget (2016) 7:77683–95. doi: 10.18632/oncotarget.12760
23. Marquard S, Thomann S, Weiler SME, Bissinger M, Lutz T, Sticht C, et al. Yes-associated protein (YAP) induces a secretome phenotype and transcriptionally regulates plasminogen activator inhibitor-1 (PAI-1) expression in hepatocarcinogenesis. Cell Commun Signal (2020) 18:166. doi: 10.1186/s12964-020-00634-6
24. Yan B, Li T, Shen L, Zhou Z, Liu X, Wang X, et al. Simultaneous knockdown of YAP and TAZ increases apoptosis of hepatocellular carcinoma cells under hypoxic condition. Biochem Biophys Res Commun (2019) 515:275–81. doi: 10.1016/j.bbrc.2019.05.143
25. Kong FE, Li GM, Tang YQ, Xi SY, Loong JHC, Li MM, et al. Targeting tumor lineage plasticity in hepatocellular carcinoma using an anti-CLDN6 antibody-drug conjugate. Sci Transl Med (2021) 13(579):eabb6282. doi: 10.1126/scitranslmed.abb6282
26. Chen Z, Xu J, Wu Y, Lei S, Liu H, Meng Q, et al. Diosgenin inhibited the expression of TAZ in hepatocellular carcinoma. Biochem Biophys Res Commun (2018) 503:1181–5. doi: 10.1016/j.bbrc.2018.07.022
27. Ni W, Zhang Y, Zhan Z, Ye Feng, Liang Y, Huang J, et al. A novel lncRNA uc.134 represses hepatocellular carcinoma progression by inhibiting CUL4A-mediated ubiquitination of LATS1. J Hematol Oncol (2017) 10:91. doi: 10.1186/s13045-017-0449-4
28. Zeng Y, Xu Q, Xu N. Long non-coding RNA LOC107985656 represses the proliferation of hepatocellular carcinoma cells through activation of the tumor-suppressive hippo pathway. Bioengineered (2021) 12:7964–74. doi: 10.1080/21655979.2021.1984005
29. Zhao D, Xia L, Geng W, Xu D, Zhong C, Zhang J, et al. Metformin suppresses interleukin-22 induced hepatocellular carcinoma by upregulating hippo signaling pathway. J Gastroenterol Hepatol (2021) 36:3469–76. doi: 10.1111/jgh.15674
30. Li Y, Lu J, Chen Q, Han S, Shao H, Chen P, et al. Artemisinin suppresses hepatocellular carcinoma cell growth, migration and invasion by targeting cellular bioenergetics and hippo-YAP signaling. Arch Toxicol (2019) 93:3367–83. doi: 10.1007/s00204-019-02579-3
31. Zhao S, Xu K, Jiang R, Li D, Guo X, Zhou P, et al. Evodiamine inhibits proliferation and promotes apoptosis of hepatocellular carcinoma cells via the hippo-Yes-Associated protein signaling pathway. Life Sci (2020) 251:117424. doi: 10.1016/j.lfs.2020.117424
32. Jia J, Qiao Y, Pilo MG, Cigliano A, Liu X, Shao Z, et al. Tankyrase inhibitors suppress hepatocellular carcinoma cell growth via modulating the hippo cascade. PloS One (2017) 12:e0184068. doi: 10.1371/journal.pone.0184068
33. Zhan J, Shi LL, Wang Y, Wei B, Yang SL. In vivo study on the effects of xiaoaiping on the stemness of hepatocellular carcinoma cells. Evid Based Complement Alternat Med (2019) 2019:4738243. doi: 10.1155/2019/4738243
34. Wu K, Teng M, Zhou W, Lu F, Zhou Y, Zeng J, et al. Wogonin induces cell cycle arrest and apoptosis of hepatocellular carcinoma cells by activating hippo signalling. Anticancer Agents Med Chem (2021) 22(8):1551–60. doi: 10.2174/1871520621666210824105915
35. Higashi T, Hayashi H, Kitano Y, Yamamura K, Kaida T, Arima K, et al. Statin attenuates cell proliferative ability via TAZ (WWTR1) in hepatocellular carcinoma. Med Oncol (2016) 33:123. doi: 10.1007/s12032-016-0845-6
36. Li J, Wang H, Wang L, Tan R, Zhu M, Zhong X, et al. Decursin inhibits the growth of HepG2 hepatocellular carcinoma cells via Hippo/YAP signaling pathway. Phytother Res (2018) 32:2456–65. doi: 10.1002/ptr.6184
37. Liu F, Wang G, Wang X, Che Z, Dong W, Guo X, et al. Targeting high aurora kinases expression as an innovative therapy for hepatocellular carcinoma. Oncotarget (2017) 8:27953–65. doi: 10.18632/oncotarget.15853
38. Wang L, Wang J, Cao Y, Li W, Wang Y, Xu J, et al. Molecular evidence for better efficacy of hypocrellin a and oleanolic acid combination in suppression of HCC growth. Eur J Pharmacol (2019) 842:281–90. doi: 10.1016/j.ejphar.2018.10.042
39. Kong D, Jiang Y, Miao X, Wu Z, Liu H, Gong W. Tadalafil enhances the therapeutic efficacy of BET inhibitors in hepatocellular carcinoma through activating hippo pathway. Biochim Biophys Acta Mol Basis Dis (2021) 1867:166267. doi: 10.1016/j.bbadis.2021.166267
40. Mi L, Kuang H. Melatonin regulates cisplatin resistance and glucose metabolism through hippo signaling in hepatocellular carcinoma cells. Cancer Manag Res (2020) 12:1863–74. doi: 10.2147/CMAR.S230466
41. Guo Q, Wang J, Cao Z, Tang C, Huang F. Interaction of S100A1 with LATS1 promotes cell growth through regulation of the hippo pathway in hepatocellular carcinoma. Int J Oncol (2018) 53:592–602. doi: 10.3892/ijo.2018.4431
42. Cho Y, Park MJ, Kim K, Kim SW, Kim W, Oh S, et al. Reactive oxygen species-induced activation of yes-associated protein-1 through the c-myc pathway is a therapeutic target in hepatocellular carcinoma. World J Gastroenterol (2020) 26:6599–613. doi: 10.3748/wjg.v26.i42.6599
43. Chen T, Sun D, Wang Q, Zhou T, Tan J, Xu C, et al. α-hederin inhibits the proliferation of hepatocellular carcinoma cells via hippo-Yes-Associated protein signaling pathway. Front Oncol (2022) 12:839603. doi: 10.3389/fonc.2022.839603
44. Xu W, Gong F, Zhang T, Chi B, Wang J. RNA-Binding protein Dnd1 inhibits epithelial-mesenchymal transition and cancer stem cell-related traits on hepatocellular carcinoma cells. Biotechnol Lett (2017) 39:1359–67. doi: 10.1007/s10529-017-2375-5
45. Zhang Y, Li K, Ying Y, Chen B, Hao K, Chen B, et al. C21 steroid-enriched fraction refined from marsdenia tenacissima inhibits hepatocellular carcinoma through the coordination of hippo-yap and PTEN-PI3K/AKT signaling pathways. Oncotarget (2017) 8:110576–91. doi: 10.18632/oncotarget.22833
46. Liu H, Li J, Yuan W, Hao S, Wang M, Wang F, et al. Bioactive components and mechanisms of poplar propolis in inhibiting proliferation of human hepatocellular carcinoma HepG2 cells. BioMed Pharmacother (2021) 144:112364. doi: 10.1016/j.biopha.2021.112364
47. Fan Z, Xia H, Xu H, Ma J, Zhou S, Hou W, et al. Standard CD44 modulates YAP1 through a positive feedback loop in hepatocellular carcinoma. BioMed Pharmacother (2018) 103:147–56. doi: 10.1016/j.biopha.2018.03.042
48. Chen R, Zhu S, Fan XG, Wang H, Lotze MT, Zeh HJ 3rd, et al. High mobility group protein B1 controls liver cancer initiation through yes-associated protein -dependent aerobic glycolysis. Hepatology (2018) 67:1823–41. doi: 10.1002/hep.29663
49. Guan C, Chang Z, Gu X, Liu R. MTA2 promotes HCC progression through repressing FRMD6, a key upstream component of hippo signaling pathway. Biochem Biophys Res Commun (2019) 515:112–8. doi: 10.1016/j.bbrc.2019.05.025
50. Xu G, Wang Y, Li W, et al. COX-2 forms regulatory loop with YAP to promote proliferation and tumorigenesis of hepatocellular carcinoma cells. Neoplasia (2018) 20:324–34. doi: 10.1016/j.neo.2017.12.004
51. Bao L, Yuan L, Li P, Bu Q, Guo A, Zhang H, et al. A FUS-LATS1/2 axis inhibits hepatocellular carcinoma progression via activating hippo pathway. Cell Physiol Biochem (2018) 50:437–51. doi: 10.1159/000494155
52. Lu X, Yang C, Hu Y, Xu J, Shi C, Rao J, et al. Upregulation of miR-1254 promotes hepatocellular carcinoma cell proliferation, migration, and invasion via inactivation of the hippo-YAP signaling pathway by decreasing PAX5. J Cancer (2021) 12:771–89. doi: 10.7150/jca.49680
53. Hu Y, Yang C, Yang S, Cheng F, Rao J, Wang X. miR-665 promotes hepatocellular carcinoma cell migration, invasion, and proliferation by decreasing hippo signaling through targeting PTPRB. Cell Death Dis (2018) 9:954. doi: 10.1038/s41419-018-0978-y
54. Han LL, Yin XR, Zhang SQ. miR-103 promotes the metastasis and EMT of hepatocellular carcinoma by directly inhibiting LATS2. Int J Oncol (2018) 53:2433–44. doi: 10.3892/ijo.2018.4580
55. Ruan T, He X, Yu J, Hang Z. MicroRNA-186 targets yes-associated protein 1 to inhibit hippo signaling and tumorigenesis in hepatocellular carcinoma. Oncol Lett (2016) 11:2941–5. doi: 10.3892/ol.2016.4312
56. Wu H, Zhang W, Wu Z, Liu Y, Shi Y, Gong J, et al. miR-29c-3p regulates DNMT3B and LATS1 methylation to inhibit tumor progression in hepatocellular carcinoma. Cell Death Dis (2019) 10:48. doi: 10.1038/s41419-018-1281-7
57. Bie B, Sun J, Li J, Guo Y, Jiang W, Huang C, et al. Baicalein, a natural anti-cancer compound, alters MicroRNA expression profiles in Bel-7402 human hepatocellular carcinoma cells. Cell Physiol Biochem (2017) 41:1519–31. doi: 10.1159/000470815
58. Chen M, Wu L, Tu J, Zhao Z, Fan X, Mao J, et al. miR-590-5p suppresses hepatocellular carcinoma chemoresistance by targeting YAP1 expression. EBioMedicine (2018) 35:142–54. doi: 10.1016/j.ebiom.2018.08.010
59. Correia de Sousa M, Calo N, Sobolewski C, Gjorgjieva M, Clement S, Maeder C, et al. Mir-21 suppression promotes mouse hepatocarcinogenesis. Cancers (Basel) (2021) 13(19):4983. doi: 10.3390/cancers13194983
60. Fan Y, Du Z, Ding Q, Zhang J, Winkel MOD, Gerbes AL, et al. SEPT6 drives hepatocellular carcinoma cell proliferation, migration and invasion via the Hippo/YAP signaling pathway. Int J Oncol (2021) 58(6):25. doi: 10.3892/ijo.2021.5205
61. Ou H, Chen Z, Xiang L, Fang Y, Xu Y, Liu Q, et al. Frizzled 2-induced epithelial-mesenchymal transition correlates with vasculogenic mimicry, stemness, and hippo signaling in hepatocellular carcinoma. Cancer Sci (2019) 110:1169–82. doi: 10.1111/cas.13949
62. Wang T, Qin ZY, Wen LZ, Guo Y, Liu Q, Lei ZJ, et al. Epigenetic restriction of hippo signaling by MORC2 underlies stemness of hepatocellular carcinoma cells. Cell Death Differ (2018) 25:2086–100. doi: 10.1038/s41418-018-0095-6
63. Zhang S, Xie C, Li H, Zhang K, Li J, Wang X, et al. Ubiquitin-specific protease 11 serves as a marker of poor prognosis and promotes metastasis in hepatocellular carcinoma. Lab Invest (2018) 98:883–94. doi: 10.1038/s41374-018-0050-7
64. Ding Y, Wang G, Zhan M, Sun X, Deng Y, Zhao Y, et al. Hippo signaling suppresses tumor cell metastasis via a yki-Src42A positive feedback loop. Cell Death Dis (2021) 12:1126. doi: 10.1038/s41419-021-04423-y
65. Qiao K, Liu Y, Xu Z, Zhang H, Zhang H, Zhang C, et al. RNA m6A methylation promotes the formation of vasculogenic mimicry in hepatocellular carcinoma via hippo pathway. Angiogenesis (2021) 24:83–96. doi: 10.1007/s10456-020-09744-8
66. Liang B, Zhou Y, Qian M, Xu M, Wang J, Zhang Y, et al. TBX3 functions as a tumor suppressor downstream of activated CTNNB1 mutants during hepatocarcinogenesis. J Hepatol (2021) 75:120–31. doi: 10.1016/j.jhep.2021.01.044
67. Kim W, Khan SK, Liu Y, Xu R, Park O, He Y, et al. Hepatic hippo signaling inhibits protumoural microenvironment to suppress hepatocellular carcinoma. Gut (2018) 67:1692–703. doi: 10.1136/gutjnl-2017-314061
68. Guan L, Li T, Ai N, Wang W, He B, Bai Y, et al. MEIS2C and MEIS2D promote tumor progression via wnt/β-catenin and hippo/YAP signaling in hepatocellular carcinoma. J Exp Clin Cancer Res (2019) 38:417. doi: 10.1186/s13046-019-1417-3
69. He L, Yuan L, Yu W, Sun Y, Jiang D, Wang X, et al. A regulation loop between YAP and NR4A1 balances cell proliferation and apoptosis. Cell Rep (2020) 33:108284. doi: 10.1016/j.celrep.2020.108284
70. Xia H, Dai X, Yu H, Zhou S, Fan Z, Wei G, et al. EGFR-PI3K-PDK1 pathway regulates YAP signaling in hepatocellular carcinoma: the mechanism and its implications in targeted therapy. Cell Death Dis (2018) 9:269. doi: 10.1038/s41419-018-0302-x
71. Tong R, Yang B, Xiao H, Peng C, Hu W, Weng X, et al. KCTD11 inhibits growth and metastasis of hepatocellular carcinoma through activating hippo signaling. Oncotarget (2017) 8:37717–29. doi: 10.18632/oncotarget.17145
72. Zhang X, Fan Q, Li Y, Yang Z, Yang L, Zong Z, et al. Transforming growth factor-beta1 suppresses hepatocellular carcinoma proliferation via activation of hippo signaling. Oncotarget (2017) 8:29785–94. doi: 10.18632/oncotarget.14523
73. Cheng Y, Hou T, Ping J, Chen T, Yin B. LMO3 promotes hepatocellular carcinoma invasion, metastasis and anoikis inhibition by directly interacting with LATS1 and suppressing hippo signaling. J Exp Clin Cancer Res (2018) 37:228. doi: 10.1186/s13046-018-0903-3
74. Chen Q, Wang H, Li Z, Li F, Liang L, Zou Y, et al. Circular RNA ACTN4 promotes intrahepatic cholangiocarcinoma progression by recruiting YBX1 to initiate FZD7 transcription. J Hepatol (2022) 76:135–47. doi: 10.1016/j.jhep.2021.08.027
75. Zhao X, Qin W, Jiang Y, Yang Z, Yuan B, Dai R, et al. ACADL plays a tumor-suppressor role by targeting Hippo/YAP signaling in hepatocellular carcinoma. NPJ Precis Oncol (2020) 4:7. doi: 10.1038/s41698-020-0111-4
76. Weiler SME, Pinna F, Wolf T, Luitz T, Geldiyev A, Sticht C, et al. Induction of chromosome instability by activation of yes-associated protein and forkhead box M1 in liver cancer. Gastroenterology (2017) 152:2037–2051.e2022. doi: 10.1053/j.gastro.2017.02.018
77. Neri L, Lasa M, Elosegui-Artola A, D'Avola D, Carte B, Gazquez C, et al. NatB-mediated protein n-α-terminal acetylation is a potential therapeutic target in hepatocellular carcinoma. Oncotarget (2017) 8:40967–81. doi: 10.18632/oncotarget.17332
78. Zheng H, Ke X, Li D, Wang Q, Wang J, Liu X, et al. NEDD4 promotes cell growth and motility in hepatocellular carcinoma. Cell Cycle (2018) 17:728–38. doi: 10.1080/15384101.2018.1440879
79. Sun X, Ding Y, Zhan M, Li Y, Gao D, Wang G, et al. Usp7 regulates hippo pathway through deubiquitinating the transcriptional coactivator yorkie. Nat Commun (2019) 10:411. doi: 10.1038/s41467-019-08334-7
80. Lei L, Wu J, Gu D, Liu H, Wang S. CIZ1 interacts with YAP and activates its transcriptional activity in hepatocellular carcinoma cells. Tumour Biol (2016) 37:11073–9. doi: 10.1007/s13277-016-4866-8
81. Xue Y, Bhushan B, Mars WM, Bowen W, Tao J, Orr A, et al. Phosphorylated ezrin (Thr567) regulates hippo pathway and yes-associated protein (Yap) in liver. Am J Pathol (2020) 190:1427–37. doi: 10.1016/j.ajpath.2020.03.014
82. Zhang X, Li Y, Ma Y, Yang L, Wang T, Meng X, et al. Yes-associated protein (YAP) binds to HIF-1α and sustains HIF-1α protein stability to promote hepatocellular carcinoma cell glycolysis under hypoxic stress. J Exp Clin Cancer Res (2018) 37:216. doi: 10.1186/s13046-018-0892-2
83. Wu Y, Zhang J, Zhang H, Zhai Y. Hepatitis b virus X protein mediates yes-associated protein 1 upregulation in hepatocellular carcinoma. Oncol Lett (2016) 12:1971–4. doi: 10.3892/ol.2016.4885
84. Castven D, Czauderna C, Becker D, et al. Acquired resistance to antiangiogenic therapies in hepatocellular carcinoma is mediated by yes-associated protein 1 activation and transient expansion of stem-like cancer cells. Hepatol Commun (2021) 6(5):1140–56. doi: 10.1002/hep4.1869
85. Guo X, Zhao Y, Yan H, Yang Y, Shen S, Dai X, et al. Single tumor-initiating cells evade immune clearance by recruiting type II macrophages. Genes Dev (2017) 31:247–59. doi: 10.1101/gad.294348.116
86. Conboy CB, Vélez-Reyes GL, Tschida BR, Hu H, Kaufmann G, Koes N, et al. R-spondin 2 drives liver tumor development in a yes-associated protein-dependent manner. Hepatol Commun (2019) 3:1496–509. doi: 10.1002/hep4.1422
87. Cheng JC, Wang EY, Yi Y, Thakur A, Tsai SH, Hoodless PA, et al. S1P stimulates proliferation by upregulating CTGF expression through S1PR2-mediated YAP activation. Mol Cancer Res (2018) 16:1543–55. doi: 10.1158/1541-7786.MCR-17-0681
88. Wang EY, Cheng JC, Thakur A, Yi Y, Tsai SH, Hoodless PA. YAP transcriptionally regulates ErbB2 to promote liver cell proliferation. Biochim Biophys Acta Gene Regul Mech (2018) S1874-9399(18)30039-7. doi: 10.1016/j.bbagrm.2018.07.004
89. Ye J, Li TS, Xu G, Zhao YM, Zhang NP, Fan J, et al. JCAD promotes progression of nonalcoholic steatohepatitis to liver cancer by inhibiting LATS2 kinase activity. Cancer Res (2017) 77:5287–300. doi: 10.1158/0008-5472.CAN-17-0229
90. Wang Y, Fang R, Cui M, Zhang W, Bai X, Wang H, et al. The oncoprotein HBXIP up-regulates YAP through activation of transcription factor c-myb to promote growth of liver cancer. Cancer Lett (2017) 385:234–42. doi: 10.1016/j.canlet.2016.10.018
91. Foglia B, Sutti S, Cannito S, Rosso C, Maggiora M, Autelli R, et al. Hepatocyte-specific deletion of HIF2α prevents NASH-related liver carcinogenesis by decreasing cancer cell proliferation. Cell Mol Gastroenterol Hepatol (2022) 13:459–82. doi: 10.1016/j.jcmgh.2021.10.002
92. Lin H, Peng J, Zhu T, Zhang R, Lei L. Exosomal miR-4800-3p aggravates the progression of hepatocellular carcinoma via regulating the hippo signaling pathway by targeting STK25. Front Oncol (2022) 12:759864. doi: 10.3389/fonc.2022.759864
93. Kapil S, Sharma BK, Patil M, Elattar S, Yuan J, Hou S, et al. The cell polarity protein scrib functions as a tumor suppressor in liver cancer. Oncotarget (2017) 8:26515–31. doi: 10.18632/oncotarget.15713
94. Van Haele M, Moya IM, Karaman R, Rens G, Snoeck J, Govaere O, et al. YAP and TAZ heterogeneity in primary liver cancer: An analysis of its prognostic and diagnostic role. Int J Mol Sci (2019) 20(3):638 doi: 10.3390/ijms20030638
95. Qin Y, Pei Z, Feng Z, Lin P, Wang S, Li Y, et al. Oncogenic activation of YAP signaling sensitizes ferroptosis of hepatocellular carcinoma via ALOXE3-mediated lipid peroxidation accumulation. Front Cell Dev Biol (2021) 9:751593. doi: 10.3389/fcell.2021.751593
96. Kim MK, Park JY, Kang YN. Tumorigenic role of YAP in hepatocellular carcinogenesis is involved in SHP2 whose function is different in vitro and in vivo. Pathol Res Pract (2018) 214:1031–9. doi: 10.1016/j.prp.2018.04.010
97. Yang J, Zhang X, Liu L, Yang X, Qian Q, Du B, et al. C-src promotes the growth and tumorigenesis of hepatocellular carcinoma via the hippo signaling pathway. Life Sci (2021) 264:118711. doi: 10.1016/j.lfs.2020.118711
98. Park H, Lee Y, Lee K, Lee H, Yoo JE, Ahn S. The clinicopathological significance of YAP/TAZ expression in hepatocellular carcinoma with relation to hypoxia and stemness. Pathol Oncol Res (2021) 27:604600. doi: 10.3389/pore.2021.604600
99. Pan Q, Qin F, Yuan H, He B, Yang N, Zhang Y, et al. Normal tissue adjacent to tumor expression profile analysis developed and validated a prognostic model based on hippo-related genes in hepatocellular carcinoma. Cancer Med (2021) 10:3139–52. doi: 10.1002/cam4.3890
100. Huang Z, Zhou JK, Wang K, Chen H, Qin S, Liu J, et al. PDLIM1 inhibits tumor metastasis through activating hippo signaling in hepatocellular carcinoma. Hepatology (2020) 71:1643–59. doi: 10.1002/hep.30930
101. Chen Q, Zhou XW, Zhang AJ, He K. ACTN1 supports tumor growth by inhibiting hippo signaling in hepatocellular carcinoma. J Exp Clin Cancer Res (2021) 40:23. doi: 10.1186/s13046-020-01821-6
102. Zhang YL, Li Q, Yang XM, Fang F, Li J, Wang YH, et al. SPON2 promotes M1-like macrophage recruitment and inhibits hepatocellular carcinoma metastasis by distinct integrin-rho GTPase-hippo pathways. Cancer Res (2018) 78:2305–17. doi: 10.1158/0008-5472.CAN-17-2867
103. Hu ZG, Chen YB, Huang M, Tu JB, Tu SJ, Pan YJ, et al. PLG inhibits hippo signaling pathway through SRC in the hepatitis b virus-induced hepatocellular-carcinoma progression. Am J Transl Res (2021) 13:515–31.
104. Shen L, Wen J, Zhao T, Hu Z, Song C, Gu D, et al. A genetic variant in large tumor suppressor kinase 2 of hippo signaling pathway contributes to prognosis of hepatocellular carcinoma. Onco Targets Ther (2016) 9:1945–51. doi: 10.2147/OTT.S100699
105. Yim SY, Kang SH, Shin JH, Jeong YS, Sohn BH, Um SH, et al. Low ARID1A expression is associated with poor prognosis in hepatocellular carcinoma. Cells (2020) 9(9):2002. doi: 10.3390/cells9092002
106. Hu H, Xu L, Luo SJ, Xiang T, Chen Y, Cao ZR, et al. Retinal dehydrogenase 5 (RHD5) attenuates metastasis via regulating HIPPO/YAP signaling pathway in hepatocellular carcinoma. Int J Med Sci (2020) 17:1897–908. doi: 10.7150/ijms.46091
107. Wu D, Wang Y, Yang G, Zhang S, Liu Y, Zhou S, et al. A novel mitochondrial amidoxime reducing component 2 is a favorable indicator of cancer and suppresses the progression of hepatocellular carcinoma by regulating the expression of p27. Oncogene (2020) 39:6099–112. doi: 10.1038/s41388-020-01417-6
108. Qiu B, Wei W, Zhu J, Fu G, Lu D. EMT induced by loss of LKB1 promotes migration and invasion of liver cancer cells through ZEB1-induced YAP signaling. Oncol Lett (2018) 16:6465–71. doi: 10.3892/ol.2018.9445
109. Kong W, Wu Z, Yang M, Zuo X, Yin G, Chen W. LMNB2 is a prognostic biomarker and correlated with immune infiltrates in hepatocellular carcinoma. IUBMB Life (2020) 72:2672–85. doi: 10.1002/iub.2408
110. Weiler SME, Lutz T, Bissinger M, Sticht C, Knaub M, Gretz N, et al. TAZ target gene ITGAV regulates invasion and feeds back positively on YAP and TAZ in liver cancer cells. Cancer Lett (2020) 473:164–75. doi: 10.1016/j.canlet.2019.12.044
111. Dong Q, Fu L, Zhao Y, Xie C, Li Q, Wang E. TNFAIP8 interacts with LATS1 and promotes aggressiveness through regulation of hippo pathway in hepatocellular carcinoma. Oncotarget (2017) 8:15689–703. doi: 10.18632/oncotarget.14938
112. Ye M, Wang S, Qie JB, Sun PL. SPRY4-AS1, a novel enhancer RNA, is a potential novel prognostic biomarker and therapeutic target for hepatocellular carcinoma. Front Oncol (2021) 11:765484. doi: 10.3389/fonc.2021.765484
113. Ren X, Wang X, Yan Y, Chen X, Cai Y, Liang Q, et al. Integrative bioinformatics and experimental analysis revealed TEAD as novel prognostic target for hepatocellular carcinoma and its roles in ferroptosis regulation. Aging (Albany NY) (2022) 14:961–74. doi: 10.18632/aging.203853
114. Liu YP, Pan LL, Kong CC. Stathmin 1 promotes the progression of liver cancer through interacting with YAP1. Eur Rev Med Pharmacol Sci (2020) 24:7335–44. doi: 10.26355/eurrev_202007_21900
115. Dong G, Qiu F, Liu C, Wu H, Liu Y. [High expression of DNMT3B promotes proliferation and invasion of hepatocellular carcinoma cells via hippo signaling pathway]. Nan Fang Yi Ke Da Xue Xue Bao (2019) 39:1443–52. doi: 10.12122/j.issn.1673-4254.2019.12.08
116. Lin B, Chen T, Zhang Q, Lu X, Zheng Z, Ding J, et al. FAM83D associates with high tumor recurrence after liver transplantation involving expansion of CD44+ carcinoma stem cells. Oncotarget (2016) 7:77495–507. doi: 10.18632/oncotarget.12715
117. Zhang Y, Wang W, Wang Y, Huang X, Zhang Z, Chen B, et al. NEK2 promotes hepatocellular carcinoma migration and invasion through modulation of the epithelial-mesenchymal transition. Oncol Rep (2018) 39:1023–33. doi: 10.3892/or.2018.6224
118. Sohn BH, Shim JJ, Kim SB, Jang KY, Kim SM, Kim JH, et al. Inactivation of hippo pathway is significantly associated with poor prognosis in hepatocellular carcinoma. Clin Cancer Res (2016) 22:1256–64. doi: 10.1158/1078-0432.CCR-15-1447
119. Yang XM, Cao XY, He P, Li J, Feng MX, Zhang YL, et al. Overexpression of rac GTPase activating protein 1 contributes to proliferation of cancer cells by reducing hippo signaling to promote cytokinesis. Gastroenterology (2018) 155:1233–1249.e1222. doi: 10.1053/j.gastro.2018.07.010
120. Zhang J, Liu Z, Lian Z, Liao R, Chen Y, Qin Y, et al. Monoacylglycerol lipase: A novel potential therapeutic target and prognostic indicator for hepatocellular carcinoma. Sci Rep (2016) 6:35784. doi: 10.1038/srep35784
121. Zhang W, Shen J, Gu F, Zhang Y, Wu W, Weng J, et al. Monopolar spindle-one-binder protein 2 regulates the activity of large tumor suppressor/yes-associated protein to inhibit the motility of SMMC-7721 hepatocellular carcinoma cells. Oncol Lett (2018) 15:5375–83. doi: 10.3892/ol.2018.7952
122. Zhang Y, Yan S, Chen J, Gan C, Chen D, Li Y, et al. WWC2 is an independent prognostic factor and prevents invasion via hippo signalling in hepatocellular carcinoma. J Cell Mol Med (2017) 21:3718–29. doi: 10.1111/jcmm.13281
123. Cigliano A, Zhang S, Ribback S, Steinmann S, Sini M, Ament CE. The hippo pathway effector TAZ induces intrahepatic cholangiocarcinoma in mice and is ubiquitously activated in the human disease. J Exp Clin Cancer Res (2022) 41:192. doi: 10.1186/s13046-022-02394-2
124. Rizvi S, Yamada D, Hirsova P, Bronk SF, Werneburg NW, Krishnan A, et al. A hippo and fibroblast growth factor receptor autocrine pathway in cholangiocarcinoma. J Biol Chem (2016) 291:8031–47. doi: 10.1074/jbc.M115.698472
125. Smoot RL, Werneburg NW, Sugihara T, Hernandez M, Yang L, Mehner C, et al. Platelet-derived growth factor regulates YAP transcriptional activity via src family kinase dependent tyrosine phosphorylation. J Cell Biochem (2018) 119:824–36. doi: 10.1002/jcb.26246
126. Li F, Chen Q, Xue H, Zhang L, Wang K, Shen F. LncRNA MNX1-AS1 promotes progression of intrahepatic cholangiocarcinoma through the MNX1/Hippo axis. Cell Death Dis (2020) 11:894. doi: 10.1038/s41419-020-03029-0
127. Hozaka Y, Seki N, Tanaka T, Asai S, Moriya S, Idichi T, et al. Molecular pathogenesis and regulation of the miR-29-3p-Family: Involvement of ITGA6 and ITGB1 in intra-hepatic cholangiocarcinoma. Cancers (Basel) (2021) 13(11):2804. doi: 10.3390/cancers13112804
128. Zhu B, Qian W, Han C, Bai T, Hou X. Piezo 1 activation facilitates cholangiocarcinoma metastasis via Hippo/YAP signaling axis. Mol Ther Nucleic Acids (2021) 24:241–52. doi: 10.1016/j.omtn.2021.02.026
129. Rizvi S, Fischbach SR, Bronk SF, Hirsova P, Krishnan A, Dhanasekaran R, et al. YAP-associated chromosomal instability and cholangiocarcinoma in mice. Oncotarget (2018) 9:5892–905. doi: 10.18632/oncotarget.23638
130. He M, Cheng C, Tu J, Ji SS, Lou D, Bai B. Agrin expression is correlated with tumor development and poor prognosis in cholangiocarcinoma. J Int Med Res (2021) 49:3000605211009722. doi: 10.1177/03000605211009722
131. Ma W, Han C, Zhang J, Song K, Chen W, Kwon H, et al. The histone methyltransferase G9a promotes cholangiocarcinogenesis through regulation of the hippo pathway kinase LATS2 and YAP signaling pathway. Hepatology (2020) 72:1283–97. doi: 10.1002/hep.31141
132. Marti P, Stein C, Blumer T, Abraham Y, Dill MT, Pikiolek M, et al. YAP promotes proliferation, chemoresistance, and angiogenesis in human cholangiocarcinoma through TEAD transcription factors. Hepatology (2015) 62:1497–510. doi: 10.1002/hep.27992
133. Xiao H, Tong R, Yang B, Lv Z, Du C, Peng C, et al. TAZ regulates cell proliferation and sensitivity to vitamin D3 in intrahepatic cholangiocarcinoma. Cancer Lett (2016) 381:370–9. doi: 10.1016/j.canlet.2016.08.013
134. Zhang S, Liang S, Wu D, Guo H, Ma K, Liu L, et al. LncRNA coordinates hippo and mTORC1 pathway activation in cancer. Cell Death Dis (2021) 12:822. doi: 10.1038/s41419-021-04112-w
135. Tóth M, Wehling L, Thiess L, Rose F, Schmitt J, Weiler SME, et al. Co-Expression of YAP and TAZ associates with chromosomal instability in human cholangiocarcinoma. BMC Cancer (2021) 21:1079. doi: 10.1186/s12885-021-08794-5
136. Xu KD, Miao Y, Li P, Li PP, Liu J, Li J, et al. Licochalcone a inhibits cell growth through the downregulation of the hippo pathway via PES1 in cholangiocarcinoma cells. Environ Toxicol (2022) 37:564–73. doi: 10.1002/tox.23422
137. Nishio M, Sugimachi K, Goto H, Wang J, Morikawa T, Miyachi Y, et al. Dysregulated YAP1/TAZ and TGF-β signaling mediate hepatocarcinogenesis in Mob1a/1b-deficient mice. Proc Natl Acad Sci U S A (2016) 113:E71–80. doi: 10.1073/pnas.1517188113
138. Jung DE, Park SB, Kim K, Kim C, Song SY. CG200745, an HDAC inhibitor, induces anti-tumour effects in cholangiocarcinoma cell lines via miRNAs targeting the hippo pathway. Sci Rep (2017) 7:10921. doi: 10.1038/s41598-017-11094-3
139. Buckarma EH, Werneburg NW, Conboy CB, Kabashima A, O'Brien DR, Wang C, et al. The YAP-interacting phosphatase SHP2 can regulate transcriptional coactivity and modulate sensitivity to chemotherapy in cholangiocarcinoma. Mol Cancer Res (2020) 18:1574–88. doi: 10.1158/1541-7786.MCR-20-0165
140. Sugihara T, Werneburg NW, Hernandez MC, Yang L, Kabashima A, Hirsova P, et al. YAP tyrosine phosphorylation and nuclear localization in cholangiocarcinoma cells are regulated by LCK and independent of LATS activity. Mol Cancer Res (2018) 16:1556–67. doi: 10.1158/1541-7786.MCR-18-0158
141. Liu Y, Wu Y, Ouyang YS, He JJ, Shen LL, Qi H, et al. [The combination of YAP/TAZ predicts the clinical prognosis in patients with cholangiocarcinoma after radical resection]. Zhonghua Nei Ke Za Zhi (2021) 60:637–43. doi: 10.3760/cma.j.cn112138-20210201-00091
142. Sugimachi K, Nishio M, Aishima S, Kuroda Y, Iguchi T, Komatsu H, et al. Altered expression of hippo signaling pathway molecules in intrahepatic cholangiocarcinoma. Oncology (2017) 93:67–74. doi: 10.1159/000463390
143. García P, Rosa L, Vargas S, Weber H, Espinoza JA, Suarez F, et al. Hippo-YAP1 is a prognosis marker and potentially targetable pathway in advanced gallbladder cancer. Cancers (Basel) (2020) 12(4):778. doi: 10.3390/cancers12040778
Keywords: hippo signalling pathway, therapeutic markers, prognostic markers, hepatocellular carcinoma, cholangiocarcinoma
Citation: Mranda GM, Xiang Z-P, Liu J-J, Wei T and Ding Y (2022) Advances in prognostic and therapeutic targets for hepatocellular carcinoma and intrahepatic cholangiocarcinoma: The hippo signaling pathway. Front. Oncol. 12:937957. doi: 10.3389/fonc.2022.937957
Received: 09 May 2022; Accepted: 13 July 2022;
Published: 12 August 2022.
Edited by:
Xiangsong Wu, Shanghai Jiao Tong University School of Medicine, ChinaReviewed by:
Weiqi Rong, Chinese Academy of Medical Sciences and Peking Union Medical College, ChinaShaocheng Lyu, Beijing Chaoyang Hospital, Capital Medical University, China
Copyright © 2022 Mranda, Xiang, Liu, Wei and Ding. This is an open-access article distributed under the terms of the Creative Commons Attribution License (CC BY). The use, distribution or reproduction in other forums is permitted, provided the original author(s) and the copyright owner(s) are credited and that the original publication in this journal is cited, in accordance with accepted academic practice. No use, distribution or reproduction is permitted which does not comply with these terms.
*Correspondence: Yinlu Ding, dingyinlu@126.com; celestinajohn60@gmail.com