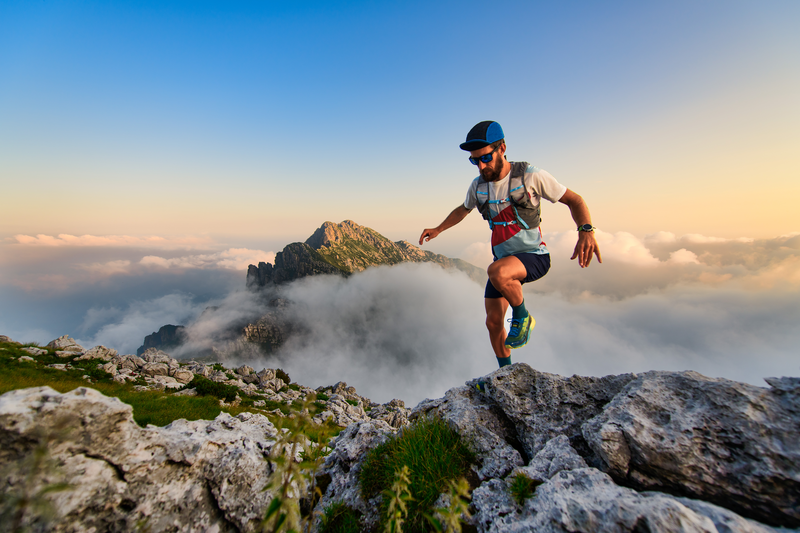
94% of researchers rate our articles as excellent or good
Learn more about the work of our research integrity team to safeguard the quality of each article we publish.
Find out more
REVIEW article
Front. Oncol. , 12 October 2022
Sec. Neuro-Oncology and Neurosurgical Oncology
Volume 12 - 2022 | https://doi.org/10.3389/fonc.2022.925560
This article is part of the Research Topic Advances in Brain Tumors Diagnosis and Treatment View all 27 articles
Background: Glioblastoma (GBM) is a malignant brain tumor associated with high morbidity and mortality rates with a poor prognosis. In recent years, studies on prognostic markers such as programmed death ligand 1 (PD-L1) have increased; however, their conclusions remain controversial. Here, relevant literature was reviewed and a meta-analysis was performed to clarify the correlation between PD-L1 expression and overall survival (OS) in GBM.
Methods: The non-foundational literature on PD-L1 expression associated with OS in GBM up to February 2022 was searched in the PubMed, Metstr, Cochrane, and Web of Science databases. Literature was rigorously screened according to inclusion and exclusion criteria, the total hazard ratio (HR), and corresponding 95% confidence intervals (CIs).
Results: Calculating the combined HR value and corresponding 95% CI of HR=1.124 (95% CI: 1.047–1.201, P=0.000, I2 (I-squared)=48.8%), it was shown that PD-L1 expression was significantly associated with low OS in GBM patients. Although I2 = 48.8% < 50%, to make the results more credible, in the cutoff values ≥10% subgroup HR=1.37 (95% CI: 1.07–1.67, P=0.000, I2 = 0%), which was also the result found in the first meta-analysis. In contrast, in the cutoff value ≥5% subgroup HR=1.14 (95% CI: 0.98–1.30, P=0.000, I2 = 59.8%) and in the cutoff value median PD-L1 expression levels subgroup HR=1.05 (95% CI: 0.92–1.18, P=0.000, I2 = 0%), indicating that PD-L1 expression was not associated with low OS in GBM. Furthermore, in four studies, we found no significant correlation between PD-L1 expression and the progression-free survival of GBM (HR=1.14, 95% CI:0.40–1.88, P=0.03, I2 = 29.3%).
Conclusion: PD-L1 expression was significantly associated with low OS in GBM patients; however, this result needs to be interpreted with caution and requires a large, multicenter clinical study in patients with similar baseline data for further evaluation.
Glioma, a primary malignant central nervous system tumor, has high morbidity and mortality rates (1). The higher the pathological grade, the more malignant the tumor and the worse the prognosis; glioblastoma is considered the most malignant central nervous system tumor, with an overall 5-year survival rate of only 9.8% (2). The prognosis is very poor, even with a range of conventional treatments available such as surgical resection combined with postoperative radiotherapy (3). Fortunately, in recent years, treatments including the blockade of immune checkpoints (stimulatory or inhibitory factors that generate immune responses) and chimeric antigen receptor T-cell therapy have offered hope for patients (4, 5). Studies have shown that various malignancies are groundbreaking in this regard (6); for example, blocking programmed death 1 (PD-1)/programmed death ligand 1 (PD-L1) immune checkpoints can modify the prognosis of non-small cell lung cancer (NSCLC), gastric cancer, head and neck squamous cell carcinoma, and melanoma to some extent (7–11). With the successful treatment of melanoma and NSCLC with monoclonal antibodies against PD-1/PD-L1, immunotherapy may become a promising approach for the treatment of glioma (12, 13). Although PD-1/PD-L1 expression has been validated in GBM (14–27), the conclusions are controversial and require further investigation.
In this study, a meta-analysis based on previous studies was conducted to verify the correlation between high/positive PD-L1 expression and overall survival (OS) in GBM. Conclusions differing from previous studies were obtained, demonstrating the need for continued in-depth studies of PD-L1 to predict a prognosis in GBM.
This meta-analysis was conducted based on the recommendations and criteria developed by the Preferred Reporting Items for Systematic Reviews and Meta-Analyses (PRISMA) (28). By using keywords and free words (“GBM” or “glioblastoma”) and (“PD-L1,” “CD274,” or “B7-H1”) in the PubMed, Metstr (http://fmrs.metstr.com/index.aspx), Cochrane, and Web of Science data searches, all literature up to February 2022 was evaluated manually and screened for usable literature (G and J), and senior reviewers (Z) resolved any disputes therein. Finally, basic data from the included literature were extracted, such as the year of publication, first author, country, sample size, cutoff, material, assay method, staining pattern, presence of 1p/19q codeletion, O6 -methylguanine-DNA-methyltransferase (MGMT) methylation status, IDH mutation status, analysis methOS rate and 95% confidence interval (CI), progression-free survival (PFS), and 95% CI. The extraction of results was prioritized under multifactorial analysis and for the literature without a corresponding OS hazard ratio (HR) and 95% CI but with corresponding Kaplan–Meier curves. The interrupted point-taking method of Engauge Digitizer version 12.1 was used to extract the survival rates and transform them into HR and 95% CI.
Inclusion and exclusion criteria were followed for article selection. The inclusion criteria were as follows: 1) the study investigated the prognostic value of PD-L1 expression in GBM; 2) literature was from the CGGA- and TCGA-published databases; 3) pathological findings were present confirming GBM in all patients with GBM; and 4) all patients were initially diagnosed with GBM. The exclusion criteria were as follows: 1) identical literature in different databases; 2) literature dealing with GBM recurrence; 3) similar meta-analyses; and 4) reviews, letters, and basic trials.
Two independent reviewers (G and J) assessed the quality of the included studies using the Newcastle–Ottawa Quality Assessment Scale (NOS) (29), which included three main entries (Table 1, 14–27). According to the relevant literature, an NOS score ≥5 was defined as high quality based on the literature (29).
The extracted HR values and 95% CIs were combined and displayed in deep forest plots, and the vertical line passing through one was defined as an invalid line that would suggest that PD-L1 expression does not predict a GBM prognosis. P<0.05 was defined as statistically significant. Furthermore, in the Cochrane Handbook criteria, heterogeneity is expressed by the Higgins I-squared (I2) statistic, and when I2 >50% indicates significant heterogeneity (30), a random-effects model was chosen to represent the final combined results; when it was <50%, a fixed-effects model was chosen. When there was significant heterogeneity, subgroup analysis, sensitivity analysis, and meta-regression were used to identify the sources of heterogeneity. Finally, funnel plots were used to detect the presence of publication bias, and when the funnel plots were asymmetric, Egger’s linear regression and Begg’s rank were used for further quantitative evaluation. The aforementioned data analyses were performed using Stata 16.0.
A total of 319 publications were initially identified based on the search strategy. Of these, 305 were subsequently excluded for the following reasons: meta-analysis (n=3); GBM recurrence (5); letters, reviews, and base trials (20); and other (277), of which one was excluded for providing HR and 95% CI but the total number of patients for which HR was calculated was unclear (31); another did not provide HR and CI (32) (Figure 1). In total, 14 papers, containing 19 studies, were finally identified for inclusion (Table 1). The 19 studies included 2,943 patients; 8 studies assessed PD-L1 expression from gene expression, and the remaining 11 assessed PD-L1 expression from protein expression. A total of 10 cases showed PD-L1 expression detected by immunohistochemistry (IHC), and 1 case was detected by the immunofluorescence histochemistry (IFC) method. Of these, PFS values were present in four studies. The cutoff values for the percentage of PD-L1 expression–positive cells varied among the included studies, with eight studies having a cutoff value of ≥5%, five studies did not have a cutoff defined, three studies had a median PD-L1 expression level, two studies had a cutoff value of ≥10%, and one study had a cutoff defined as 0.37. Among the analysis methods, eight studies were multifactorial analyses and the others did not mention the analysis type.
The combined HR and corresponding 95% CI were calculated as HR=1.124 (95% CI: 1.047–1.201, P=0.000, I2 = 48.8%), indicating that PD-L1 expression was significantly associated with OS in GBM (Figure 2). Although I2 = 48.8% < 50%, to make the results more credible, in the cutoff values ≥10% subgroup HR=1.37 (95% CI: 1.07–1.67, P=0.000, I2 = 0%), which was also the result found in the first meta-analysis. In contrast, in the cutoff value ≥5% subgroup HR=1.14 (95% CI:0.98–1.30, P=0.000, I2 = 59.8%) and in the cutoff value median PD-L1 expression levels subgroup HR=1.05 (95% CI: 0.92–1.18, P=0.000, I2 = 0%), indicating that PD-L1 expression was not associated with low OS in GBM (Figure 3). Furthermore, in four studies, we found no significant correlation between PD-L1 expression and PFS in GBM (HR=1.14, 95% CI:0.40–1.88, P=0.03, I2 = 29.3%) (Figure 4).
To further explore the sources of heterogeneity, the included literature data were analyzed using a sensitivity analysis; results showed that single studies were not associated with heterogeneity (Figure 5). Second, a subgroup analysis was performed based on gene and protein expression; results showed that in the gene expression subgroup HR=1.12 (95% CI: 1.03–1.20, P=0.000, I2 = 53.0%) and in the protein expression subgroup HR=1.15 (95% CI: 0.99–1.32, P=0.000, I2 = 50.4%), there were no reductions of heterogeneity (Figure 6); Meta-regression was used to test whether the country, year, age, Karnofsky Performance Score (KPS), MGMT methylation status, IDH mutation status, assay, and method of analysis affected heterogeneity. The results of the meta-regression showed that the inclusion of four variables (eight variables) resulted in a heterogeneity between the studies of Tau 2 = 0, which was 0.0303 less than the previous value of 0.0303 in Figure 2, implying that these factors could be used to explain 48.8% of the heterogeneity between studies (Table 2).
Funnel plots were used to assess the presence of publication bias (Appendix A Figures A1); when the funnel plots were asymmetric, Egger and Begg tests were used for quantitative assessment (Appendix: Figures A2, A3). As shown in Table 3, P=0.18 > 0.05, 95% CI: –.5499226–2.712886, indicating the absence of publication bias in the included studies.
PD-L1 is expressed by a variety of cell types, including macrophages, T cells, B cells, and a subset of non-hematopoietic cell types such as vascular endothelial cells (33). Aberrant expression is present not only in glioma cell lines but also in other tissue specimens (34–36). To date, little is known about the mechanisms regulating PD-L1 expression, although one study suggested that it is regulated by two basic mechanisms: innate immune resistance–mediated structural expression and acquired immune resistance–mediated inducible expression (37). In tumor cells, interferon gamma (IFN-g-), which responds to an antitumor immune activity, is a major regulator of PD-L1; PD-L1 expression in tumors can also be activated by oncogenic mutations, such as the deletion of phosphatase and tensin homologue in gliomas (38–40).
PD-L1 expression has been observed in cancers such as NSCLC, melanoma, and colorectal cancer (41–43). In addition, PD-L1 expression has been found in gliomas, but the significance in predicting its expression in glioma patients remains controversial. For example, a study by Knudsen et al. (20) on 163 patients with glioblastoma showed that high median PD-L1 expression levels were not significantly related to prognosis, both under univariate and multifactorial analyses (univariate: HR=0.89, 95% CI: 0.66–1.23, P=0.58; multifactorial: HR=1.05, 95% CI: 0.8–1.5, P=0.8). In contrast, Han et al. (18) performed an immunohistochemical analysis of pathological sections from 54 glioma patients and defined at least 5% of cells detected by membrane staining as PD-L1 positive (without regard to staining intensity); high PD-L1 expression was related to gliomas and OS under multifactorial analysis (HR=4.958, 95% CI:1.557–15.79). Although there have been an increasing number of studies on the role of PD-L1 in predicting glioma prognosis in recent years, the conclusions remain mixed. Even though the index, patients, materials, assay, staining pattern, cutoff date, and analysis methods were the same as Han et al. (18), a study by Lee KS (19) showed that PD-L1 expression did not predict prognosis in glioma patients (HR=1.204,95% CI: 0.584–2.485, P=0.615).
Because of this inconsistency, this study reviewed and analyzed all the published literature on the role of PD-L1 expression in predicting OS in GBM patients. The results showed a correlation between PD-L1 expression and low OS in GBM patients. Further subgroup analysis showed that PD-L1 did not correlate with low OS in GBM patients in terms of protein expression, which was inconsistent with the findings of Wang H (44). Through discussion, it was found that the HR values included in this study were multifactorial, whereas the study by Wang et al. was univariate. In addition, this meta-analysis found a statistically significant heterogeneity of 0% (P=0.000) in the subgroup with a PD-L1 cutoff ≥10% for the first time, although further studies are needed to demonstrate this due to the paucity of literature. In the meta-regression, differences in the country, year, age, KPS, MGMT methylation status, IDH mutation status, assay, and method of analysis affected heterogeneity.
Fortunately, even though no immune checkpoint inhibitors have been approved for glioma (24), the efficacy of PD-1/PD-L1 inhibitors has been validated in preclinical glioma models; PD-1/PD-L1 inhibitors have been shown to restore antitumor T-cell activity and improve survival, which provides a theoretical basis for clinical trials (45–50). For example, in a study by Reiss SN (51), pembrolizumab prolonged PFS in some patients despite a low response rate. In addition, Lim (52) confirmed that nivolumab was well tolerated by newly diagnosed GBM patients, the incidence of adverse events was consistent with other reported neurological frequencies, and no deaths due to drug toxicity were reported; however, survival data require further follow-up. Therefore, there is a need to further investigate the relationship between PD-L1 expression and low OS in GBM patients. In addition, it has been shown that PD-L1 mRNA is expressed in all glioma grades and shows grade dependency (25, 53), possibly due to the association of expression with the vascular endothelial growth factor, matrix metalloproteinase 9, and KI-67. Further studies are needed to better understand these relationships.
Although the present study showed a correlation between PD-L1 expression and low OS in GBM patients, there are some limitations. First, although we analyzed the effect of the MGMT methylation status and IDH mutation status on heterogeneity, we could not further analyze the prognosis of PD-L1 expression in the MGMT methylation status or IDH mutation status only because the MGMT methylation status or IDH mutation status is not mentioned in part of the literature. Second, this study was retrospective and, as such, it was not possible to define GBM according to the latest WHO pathological classification (54), and only four studies mentioned PFS values. In addition, the impact of treatment on the prognosis of GBM patients could not be further explored because some treatment options were not mentioned. Finally, despite the low heterogeneity of the present study, PD-L1 in protein expression was not shown to be associated with low OS in GBM patients in the subgroup analysis, and despite the analysis of the reasons for this, further studies are needed to demonstrate the relationship between PD-L1 and the prognosis of GBM patients.
In conclusion, despite the limitations of this study, a correlation was found between PD-L1 expression and poor OS in GBM. Statistically significant heterogeneity was found in the subgroup with a PD-L1 cutoff of ≥10% after pooled analysis, providing a theoretical basis for prospective clinical studies. Furthermore, meta-regression analysis demonstrated that the country, year, age, KPS, MGMT methylation status, IDH mutation status, assay, and method of analysis-affected heterogeneity were sources of heterogeneity. After controlling for these, the correlation between PD-L1 expression and low OS in GBM patients will become clearer, and interventions aimed at improving patient prognosis will become more defined.
XG is responsible for article screening, writing and analysis; YZ selects the article direction and guides the article framework; HJ is responsible for article screening and data analysis; XM is responsible for article embellishment and guides article revision, submission and payment of page charges.
This study was supported by a grant (No. 2022SF-166) from the Shaanxi Province Key R&D Program Projects.
The authors declare that the content of the article was composed without any commercial or financial relationsh ip and without any conflict of interest.
All claims expressed in this article are solely those of the authors and do not necessarily represent those of their affiliated organizations, or those of the publisher, the editors and the reviewers. Any product that may be evaluated in this article, or claim that may be made by its manufacturer, is not guaranteed or endorsed by the publisher.
The Supplementary Material for this article can be found online at: https://www.frontiersin.org/articles/10.3389/fonc.2022.925560/full#supplementary-material
1. Uyttenhove C, Pilotte L, Théate I, Stroobant V, Colau D, Parmentier N, et al. Evidence for a tumoral immune resistance mechanism based on tryptophan degradation by indoleamine 2,3-dioxygenase. Nat Med (2003) 9(10):1269–74. doi: 10.1038/nm934
2. Tran B, Rosenthal MA. Survival comparison between glioblastoma multiforme and other incurable cancers. J Clin Neurosci (2010) 17:417–21. doi: 10.1016/j.jocn.2009.09.004
3. Luo H, Tao C, Long X, Huang K, Zhu X. A risk signature of four aging-related genes has clinical prognostic value and is associated with a tumor immune microenvironment in glioma. Aging (Albany NY) (2021) 13(12):16198–218. doi: 10.18632/aging.203146
4. Boussiotis VA, Charest A. Immunotherapies for malignant glioma. Oncogene (2018) 37(9):1121–41. doi: 10.1038/s41388-017-0024-z
5. Korman AJ, Peggs KS, Allison JP. Checkpoint blockade in cancer immunotherapy. Adv Immunol (2006) 90:297–339. doi: 10.1016/S0065-2776(06)90008-X
6. Riess JW, Lara PN Jr, Gandara DR. Theory meets practice for immune checkpoint blockade in small-cell lung cancer. J Clin Oncol (2016) 34(31):3717–8. doi: 10.1200/JCO.2016.69.0040
7. Ledford H, Else H, Warren M. Cancer immunologists scoop medicine Nobel prize. Nature (2018) 562(7725):20–1. doi: 10.1038/d41586-018-06751-0
8. Rotte A, D’Orazi G, Bhandaru M. Nobel Committee honors tumor immunologists. J Exp Clin Cancer Res (2018) 37(1):262. doi: 10.1186/s13046-018-0937-6
9. Chen YS, Shen CR. Immune checkpoint blockade therapy: The 2014 tang prize in biopharmaceutical science. BioMed J (2015) 38(1):5–8. doi: 10.4103/2319-4170.151150
10. Xu F, Jin T, Zhu Y, Dai C. Immune checkpoint therapy in liver cancer. J Exp Clin Cancer Res (2018) 37(1):110. doi: 10.1186/s13046-018-0777-4
11. Faghfuri E, Faramarzi MA, Nikfar S, Abdollahi M. Nivolumab and pembrolizumab as immune-modulating monoclonal antibodies targeting the PD-1 receptor to treat melanoma. Expert Rev Anticancer Ther (2015) 15(9):981–93. doi: 10.1586/14737140.2015.1074862
12. Hellmann MD, Paz-Ares L, Bernabe Caro R, Zurawski B, Kim SW, Carcereny Costa E, et al. Nivolumab plus ipilimumab in advanced non-Small-Cell lung cancer. N Engl J Med (2019) 381(21):2020–31. doi: 10.1056/NEJMoa1910231
13. Wolchok JD, Chiarion-Sileni V, Gonzalez R, Rutkowski P, Grob J-J, Cowey CL, et al. Overall survival with combined nivolumab and ipilimumab in advanced melanoma. N Engl J Med (2017) 377(14):1345–56. doi: 10.1056/NEJMoa1709684
14. Liu Y, Carlsson R, Ambjørn M, Hasan M, Badn W, Darabi A, et al. PD-L1 expression by neurons nearby tumors indicates better prognosis in glioblastoma patients. J Neurosci (2013) 33(35):14231–45. doi: 10.1523/JNEUROSCI.5812-12.2013
15. Nduom EK, Wei J, Yaghi NK, Hasan M, Badn W, Darabi A, et al. PD-L1 expression and prognostic impact in glioblastoma. Neuro Oncol (2016) 18(2):195–205. doi: 10.1093/neuonc/nov172
16. Berghoff AS, Kiesel B, Widhalm G, Rajky O, Ricken G, Wöhrer A, et al. Programmed death ligand 1 expression and tumor-infiltrating lymphocytes in glioblastoma. Neuro Oncol (2015) 17(8):1064–75. doi: 10.1093/neuonc/nou307
17. Zeng J, Zhang XK, Chen HD, Zhong ZH, Wu QL, Lin SX. Expression of programmed cell death-ligand 1 and its correlation with clinical outcomes in gliomas. Oncotarget (2016) 7(8):8944–55. doi: 10.18632/oncotarget.6884
18. Han J, Hong Y, Lee YS. PD-L1 expression and combined status of PD-L1/PD-1-Positive tumor infiltrating mononuclear cell density predict prognosis in glioblastoma patients. J Pathol Transl Med (2017) 51(1):40–8. doi: 10.4132/jptm.2016.08.31
19. Lee KS, Lee K, Yun S, Moon S, Park Y, Han JH, et al. Prognostic relevance of programmed cell death ligand 1 expression in glioblastoma. J Neurooncol (2018) 136(3):453–61. doi: 10.1007/s11060-017-2675-6
20. Knudsen AM, Rudkjøbing SJ, Sørensen MD, Dahlrot RH, Kristensen BW. Expression and prognostic value of the immune checkpoints galectin-9 and PD-L1 in glioblastomas. J Neuropathol Exp Neurol (2021) 80(6):541–51. doi: 10.1093/jnen/nlab041
21. Heiland DH, Haaker G, Delev D, Mercas B, Masalha W, Heynckes S, et al. Comprehensive analysis of PD-L1 expression in glioblastoma multiforme. Oncotarget (2017) 8(26):42214–25. doi: 10.18632/oncotarget.15031
22. Jan CI, Tsai WC, Harn HJ, Shyu WC, Liu MC, Lu HM, et al. Predictors of response to autologous dendritic cell therapy in glioblastoma multiforme. Front Immunol (2018) 9:727. doi: 10.3389/fimmu.2018.00727
23. Wang Z, Zhang C, Liu X, Wang Z, Sun L, Li G, et al. Molecular and clinical characterization of PD-L1 expression at transcriptional level via 976 samples of brain glioma. Oncoimmunology (2016) 5(11):e1196310. doi: 10.1080/2162402X.2016.1196310
24. Pratt D, Dominah G, Lobel G, Obungu A, Lynes J, Sanchez V, et al. Programmed death ligand 1 is a negative prognostic marker in recurrent isocitrate dehydrogenase-wildtype glioblastoma. Neurosurgery (2019) 85(2):280–9. doi: 10.1093/neuros/nyy268
25. Zhu Z, Zhang H, Chen B, Liu X, Zhang S, Zong Z, et al. PD-L1-Mediated immunosuppression in glioblastoma is associated with the infiltration and M2-polarization of tumor-associated macrophages. Front Immunol (2020) 11:588552. doi: 10.3389/fimmu.2020.588552
26. Su L, Guo W, Lou L, Nie S, Zhang Q, Liu Y, et al. EGFR-ERK pathway regulates CSN6 to contribute to PD-L1 expression in glioblastoma. Mol Carcinog (2020) 59(5):520–32. doi: 10.1002/mc.23176
27. Takashima Y, Kawaguchi A, Kanayama T, Hayano A, Yamanaka R. Correlation between lower balance of Th2 helper T-cells and expression of PD-L1/PD-1 axis genes enables prognostic prediction in patients with glioblastoma. Oncotarget (2018) 9(27):19065–78. doi: 10.18632/oncotarget.24897
28. Moher D, Liberati A, Tetzlaff J, Altman DG, PRISMA Group. Preferred reporting items for systematic reviews and meta-analyses: The PRISMA statement. PLoSMed (2009) 6:e1000097. doi: 10.1371/journal.pmed.1000097
29. Stang A. Critical evaluation of the Newcastle-Ottawa scale for the assessment of the quality of nonrandomized studies in meta-analyses. Eur J Epidemiol (2010) 25(9):603–5. doi: 10.1007/s10654-010-9491-z
30. Higgins JP, Thompson SG. Quantifying heterogeneity in a meta-analysis. Stat Med (2002) 21:1539–58. doi: 10.1002/sim.1186
31. Mei J, Cai Y, Xu R, Yang X, Zhou W, Wang H, et al. Characterization of the clinical significance of PD-1/PD-Ls expression and methylation in patients with low-grade glioma. Technol Cancer Res Treat (2021) 20:15330338211011970. doi: 10.1177/15330338211011970
32. Long S, Li M, Liu J, Yang Y, Li G. Identification of immunologic subtype and prognosis of GBM based on TNFSF14 and immune checkpoint gene expression profiling. Aging (Albany NY) (2020) 12(8):7112–28. doi: 10.18632/aging.103065
33. Sharpe AH, Wherry EJ, Ahmed R, Freeman GJ. The function of programmed cell death 1 and its ligands in regulating autoimmunity and infection. Nat Immunol (2007) 8(3):239–45. doi: 10.1038/ni1443
34. Wintterle S, Schreiner B, Mitsdoerffer M, Schneider D, Chen L, Meyermann R, et al. Expression of the B7-related molecule B7-H1 by glioma cells: A potential mechanism of immune paralysis. Cancer Res (2003) 63(21):7462–7.
35. Wilmotte R, Burkhardt K, Kindler V, Belkouch MC, Dussex G, Tribolet N, et al. B7-homolog 1 expression by human glioma: A new mechanism of immune evasion. Neuroreport (2005) 16(10):1081–5. doi: 10.1097/00001756-200507130-00010
36. Baral A, Ye HX, Jiang PC, Yao Y, Mao Y. B7-H3 and B7-H1 expression in cerebral spinal fluid and tumor tissue correlates with the malignancy grade of glioma patients. Oncol Lett (2014) 8(3):1195–201. doi: 10.3892/ol.2014.2268
37. Momtaz P, Postow MA. Immunologic checkpoints in cancer therapy: focus on the programmed death-1 (PD-1) receptor pathway. Pharmgenomics Pers Med (2014) 7:357–65. doi: 10.2147/PGPM.S53163
38. Francisco LM, Sage PT, Sharpe AH. The PD-1 pathway in tolerance and autoimmunity. Immunol Rev (2010) 236:219–42. doi: 10.1111/j.1600-065X.2010.00923.x
39. Parsa AT, Waldron JS, Panner A, Crane CA, Parney IF, Barry JJ, et al. Loss of tumor suppressor PTEN function increases B7-H1 expression and immunoresistance in glioma. Nat Med (2007) 13(1):84–8. doi: 10.1038/nm1517
40. Broekman ML, Maas SLN, Abels ER, Mempel TR, Krichevsky AM, Breakefield XO. Multidimensional communication in the microenvirons of glioblastoma. Nat Rev Neurol (2018) 14:482–95. doi: 10.1038/s41582-018-0025-8
41. Brahmer JR, Tykodi SS, Chow LQ, Hwu WJ, Topalian SL, Hwu P, et al. Safety and activity of anti-PD-L1 antibody in patients with advanced cancer. N Engl J Med (2012) 366(26):2455–65. doi: 10.1056/NEJMoa1200694
42. Topalian SL, Hodi FS, Brahmer JR, Gettinger SN, Smith DC, McDermott DF, et al. Safety, activity, and immune correlates of anti-PD-1 antibody in cancer. N Engl J Med (2012) 366(26):2443–54. doi: 10.1056/NEJMoa1200690
43. Taube JM, Klein A, Brahmer JR, Xu H, Pan X, Kim JH, et al. Association of PD-1, PD-1 ligands, and other features of the tumor immune microenvironment with response to anti-PD-1 therapy. Clin Cancer Res (2014) 20(19):5064–74. doi: 10.1158/1078-0432.CCR-13-3271
44. Wang H, Xiao Y, Ren X, Wan D. Prognostic value of programmed death ligand 1 (PD-L1) in glioblastoma: A systematic review, meta-analysis and validation based on dataset. Bioengineered (2021) 12(2):10366–78. doi: 10.1080/21655979.2021.1996515
45. Reardon DA, Gokhale PC, Klein SR, et al. Glioblastoma eradication following immune checkpoint blockade in an orthotopic, immunocompetent model. Cancer Immunol Res (2016) 4(2):124–35. doi: 10.1158/2326-6066
46. Huang BY, Zhan YP, Zong WJ, Yu CJ, Li JF, Qu YM, et al. The PD-1/B7-H1 pathway modulates the natural killer cells versus mouse glioma stem cells. PloS One (2015) 10(8):e0134715. doi: 10.1371/journal.pone.0134715
47. Wainwright DA, Chang AL, Dey M, Balyasnikova IV, Kim CK, Tobias A, et al. Durable therapeutic efficacy utilizing combinatorial blockade against IDO, CTLA-4, and PD-L1 in mice with brain tumors. Clin Cancer Res (2014) 20(20):5290–301. doi: 10.1158/1078-0432.CCR-14-0514
48. Ladomersky E, Zhai L, Lenzen A, Lauing KL, Qian J, Scholtens DM, et al. IDO1 inhibition synergizes with radiation and PD-1 blockade to durably increase survival against advanced glioblastoma. Clin Cancer Res (2018) 24(11):2559–73. doi: 10.1158/1078-0432.CCR-17-3573
49. Zeng J, See AP, Phallen J, Jackson CM, Belcaid Z, Ruzevick J, et al. Anti-PD-1 blockade and stereotactic radiation produce long-term survival in mice with intracranial gliomas. Int J Radiat Oncol Biol Phys (2013) 86(2):343–9. doi: 10.1016/j.ijrobp.2012.12.025
50. Kim JE, Patel MA, Mangraviti A, Kim ES, Theodros D, Velarde E, et al. Combination therapy with anti-PD-1, anti-TIM-3, and focal radiation results in regression of murine gliomas. Clin Cancer Res (2017) 23(1):124–36. doi: 10.1158/1078-0432.CCR-15-1535
51. Reiss SN, Yerram P, Modelevsky L, Grommes C. Retrospective review of safety and efficacy of programmed cell death-1 inhibitors in refractory high grade gliomas. J Immunother Cancer (2017) 5(1):99. doi: 10.1186/s40425-017-0302-x
52. Lim M, Omuro A, Vlahovic G, Reardon DA, Sahebjam S, Cloughesy T, et al. Nivolumab (nivo) in combination with radiotherapy (RT) ± temozolomide (TMZ): updated safety results from CheckMate 143 in pts with methylated or unmethylated newly diagnosed glioblastoma (GBM). Ann Oncol (2017) 28(suppl 5):v109–21. doi: 10.1093/annonc/mdx366
53. Xue S, Hu M, Li P, Ma J, Xie L, Teng F, et al. Relationship between expression of PD-L1 and tumor angiogenesis, proliferation, and invasion in glioma. Oncotarget (2017) 8(30):49702–12. doi: 10.18632/oncotarget.17922
Keywords: glioma, glioblastoma, PD-L1, CD274, B7-H1, prognostic glioma, a primary
Citation: Guo X, Zhang Y, Jiao H and Miao X (2022) The prognostic significance of PD-L1 expression in patients with glioblastoma: A meta-analysis. Front. Oncol. 12:925560. doi: 10.3389/fonc.2022.925560
Received: 21 April 2022; Accepted: 19 August 2022;
Published: 12 October 2022.
Edited by:
Enrico Franceschi, IRCCS Institute of Neurological Sciences of Bologna (ISNB), ItalyReviewed by:
Marica Eoli, IRCCS Carlo Besta Neurological Institute Foundation, ItalyCopyright © 2022 Guo, Zhang, Jiao and Miao. This is an open-access article distributed under the terms of the Creative Commons Attribution License (CC BY). The use, distribution or reproduction in other forums is permitted, provided the original author(s) and the copyright owner(s) are credited and that the original publication in this journal is cited, in accordance with accepted academic practice. No use, distribution or reproduction is permitted which does not comply with these terms.
*Correspondence: Xingyu Miao, bWlhb3h5dUAxNjMuY29t
Disclaimer: All claims expressed in this article are solely those of the authors and do not necessarily represent those of their affiliated organizations, or those of the publisher, the editors and the reviewers. Any product that may be evaluated in this article or claim that may be made by its manufacturer is not guaranteed or endorsed by the publisher.
Research integrity at Frontiers
Learn more about the work of our research integrity team to safeguard the quality of each article we publish.