- General Surgery, Cancer Center, Department of Colorectal Surgery, Zhejiang Provincial People’s Hospital (Affiliated People’s Hospital, Hangzhou Medical College), Hangzhou, China
Hypoxia-mediated tumor progression is a major problem in colorectal cancer (CRC). MicroRNA (miR)-200b-3p can attenuate tumorigenesis in CRC, while exosomal miRNAs derived from cancer-associated fibroblasts (CAFs) can promote cancer progression. Nevertheless, the function of exosomal miR-200b-3p derived from CAFs in CRC remains unclear. In this study, CAFs and normal fibroblasts (NFs) were isolated from CRC and adjacent normal tissues. Next, exosomes were isolated from the supernatants of CAFs cultured under normoxia and hypoxia. Cell viability was tested using the cell counting kit-8 assay, and flow cytometry was used to assess cell apoptosis. Cell invasion and migration were evaluated using the transwell assay. Dual-luciferase was used to investigate the relationship between miR-200b-3p and high-mobility group box 3 (HMBG3). Reverse transcription-quantitative polymerase chain reaction (RT-qPCR) was performed to determine the miR-200b-3p and HMBG3 level. Our results found that the miR-200b-3p level was sharply reduced in CRC tissues compared to adjacent normal tissues. Additionally, the miR-200b-3p level was reduced in exosomes derived from hypoxic CAFs compared to exosomes derived from CAFs under normoxia. Exosomes derived from hypoxic CAFs weakened the sensitivity of CRC cells to 5-fluorouracil (5-FU) compared to hypoxic CAFs-derived exosomes. However, hypoxic CAFs-derived exosomes with upregulated miR-200b-3p increased the sensitivity of CRC cells to 5-fluorouracil (5-FU) compared to hypoxic CAFs-derived exosomes. In addition, HMBG3 was identified as the downstream target of miR-200b-3p in CRC cells, and its overexpression partially reversed the anti-tumor effect of the miR-200b-3p agomir on CRC via the mediation of the β-catenin/c-Myc axis. Furthermore, compared to exosomes derived from normoxia CAFs, exosomes derived from hypoxic CAFs weakened the therapeutic effects of 5-FU on CRC in vivo via the upregulation of HMGB3 levels. Collectively, the loss of exosomal miR-200b-3p in hypoxia CAFs reduced the sensitivity to 5-FU in CRC by targeting HMGB3. Thus, our research outlines a novel method for the treatment of CRC.
Introduction
Colorectal cancer (CRC) is the third most common malignancy worldwide, exhibiting high incidence and mortality rates (1, 2). Although surgical resection and adjuvant therapies have been used to treat CRC, the overall 5-year survival rate of patients with CRC remains low (3–5). For CRC, 5-fluorouracil (5-FU) is used as a chemotherapeutic agent; however, drug resistance caused by its long-term use decreases the clinical efficacy of 5-FU (6, 7). Therefore, it is imperative to increase the sensitivity of CRC cells to 5-FU for effective treatment.
A hypoxic tumor microenvironment (TME) is associated with tumor progression and chemoresistance (8–10). Hypoxia can contribute to aggressive tumor behavior and induce distant metastasis (11, 12). Cancer-associated fibroblasts (CAFs) are key players in the TME, which could promote chemoresistance during cancer progression (13–15). Hypoxic cancer cells can promote CAF activation, which, in turn, leads to increased invasion and stemness of tumor cells (16). Meanwhile, hypoxic CAFs can promote endothelial cell angiogenesis, facilitating the metastasis of malignant tumors (17). Thus, hypoxic CAFs may play vital roles in chemoresistance and metastasis during tumor progression.
Crosstalk between CAFs and tumor cells is often mediated by exosomes (18). Exosomes (40–100 nm) contain different nucleic acids including miRNAs that can modulate the communication between CAFs and cancer cells (19, 20). It has been shown that CAF-secreted exosomal miR−181d−5p could inhibit CRC cell sensitivity to 5-FU (21). Chen et al. suggested that exosomal miR-590-3p from CAFs could confer radioresistance to CRC cells (22). Furthermore, the miR-200b-3p level was reduced in CRC, and it can mitigate oxaliplatin resistance by targeting tubulin beta 3 class III (23). Nevertheless, whether exosomal miR-200b-3p derived from CAFs could affect the sensitivity of CRC cells to 5-FU remains unclear.
In this study, we aimed to investigate the function of exosomal miR-200b-3p derived from CAFs in CRC. We hope that our research provides new strategies for increasing the sensitivity of CRC cells to 5-FU.
Materials and methods
Ethics statement
This study was approved by the Ethics Committee of the Zhejiang Provincial People’s Hospital (No. 20211009ZPPH).
Tissue collection
Five pairs of CRC and adjacent normal tissues were collected from patients with CRC who had undergone surgery at the Zhejiang Provincial People’s Hospital. Primary human CAFs were isolated from tumor tissues, and NFs were extracted from the adjacent normal tissues (24). This study was approved by the Ethics Committee of the Zhejiang Provincial People’s Hospital. Isolated CAFs and NFs were identified by fibroblast activation protein (FAP) staining, as previously described (25).
Cell culture and transfection
CAFs and NFs were maintained in Dulbecco’s minimal essential medium/Ham’s F12 (DMEM/F12) containing fetal bovine serum (FBS; 10%) at 37°C. For hypoxic stimulation, CAFs were maintained in 1% O2 for 24 h. Human CRC cells (SW480 and SW620) were purchased from the American Type Culture Collection and cultured in the DMEM medium (Invitrogen) containing FBS (10%) in the presence of 5% CO2 at 37°C.
The miR-200b-3p agomir and negative control (NC) were purchased from RIBOBIO (Guangzhou, China). Before hypoxic stimulation, CAFs were transfected with the NC or miR-200b-3p agomir for 48 h using Lipofectamine 2000 (Invitrogen).
In addition, pcDNA3.1-HMGB3 and pcDNA3.1 control were purchased from RIBOBIO. CAFs were transfected with pcDNA3.1-HMGB3 or pcDNA3.1 control for 48 h.
RT-qPCR assay
TRIpure Reagent (ELK Biotechnology) was used to extract the RNA from cells, and the EntiLink 1st Strand cDNA Synthesis Kit (ELK Biotechnology) was used to reverse-transcribe RNA into cDNA. Next, qPCR was conducted on the StepOne Real-Time PCR System using the EnTurbo SYBR Green PCR SuperMix Kit (ELK Biotechnology). U6 served as an internal control for miR-200b-3p. β-actin served as an internal control for HMGB3. The 2−ΔΔCq method was used for the quantification of data. U6, forward 5′-CTCGCTTCGGCAGCACAT-3′, reverse 5′-AACGCTTCACGAATTTGCGT-3′; miR-200b-3p, forward 5′-GGCCCTAATACTGCCTGGTA-3′, reverse 5′-CTCAACTGGTGTCGTGGAGTC-3′; β-actin, forward 5′-GTCCACCGCAAATGCTTCTA-3′, reverse 5′-TGCTGTCACCTTCACCGTTC-3′; HMGB3, forward 5′-CTATGATCGGGAAATGAAGGATTAT-3′, reverse 5′-TTCTCATACTTCTCCTTCAGCTTTG-3′.
Western blotting
BCA kit was used to determine the protein concentration. Proteins were then electrophoresed on sodium dodecyl sulfate-polyacrylamide gels (10%) and transferred to a polyvinylidene fluoride membrane. Primary antibodies against HMGB3 (1:1000; Proteintech Group), CD63 (1:5000; Proteintech Group), tumor susceptibility 101 (TSG101; 1:2000; Proteintech Group), β-catenin (1:2000; Proteintech Group), c-Myc (1:2000; Proteintech Group), non-phospho (active) β-Catenin (1:1000; Abcam), and β-actin (1:1000; Proteintech Group) were used to incubate the membranes overnight at 4°C. Then, the membranes were incubated with the appropriate secondary antibodies for 1 h. An ECL kit was used for protein detection.
Cell apoptosis detection
CRC cells were trypsinized and resuspended. Next, 5 μl of Annexin V and 5 μl of propidium iodide were applied to stain the cells in the dark for 15 min. Flow cytometry (BD Biosciences, Franklin Lake, NJ, USA) was used to analyze the apoptotic cells. FlowJo (v10.6.2; BD Biosciences) was used to quantify the data.
Trypan blue staining
First, 90 µl of the cell suspension was transferred to a cryovial. Trypan blue staining buffer (0.4%, 10 μl) was mixed with the cell suspension and incubated for 5 min. Finally, the number of dead cells (stained blue) were counted and observed under a conventional light microscope.
Exosome isolation and identification
CAFs were cultured under both normoxic (CAFs-N) and hypoxic (CAFs-H) conditions. Exosomes were purified from CAFs-N (CAFs-N-Exo) or CAFs-H (CAFs-H-Exo) via ultracentrifugation, as previously described (26). Exosomes were then resuspended and stored immediately at −80°C. For NTA, the size distribution of exosomes was determined using a ZetaView nanoparticle tracking analyzer (Particle Metrix). For transmission electron microscopy (TEM), the exosomes were fixed, loaded, and stained with 1% phosphotungstic acid. The morphology of the exosomes was then observed using a transmission electron microscope.
Exosome uptake
PKH26 dye (Sigma) was used to label CAFs-N- and CAFs-H-derived exosomes (CAFs-N-Exo and CAFs-H-Exo, respectively) for 30 min. Later on, the labeled exosomes (red) were co-cultured with SW480 cells at 37°C. Subsequently, fluorescence microscopy was used to observe the labeled exosomes.
Cell counting kit (CCK)-8 assay
SW480 cells (5000 cells/well) were seeded onto a 96-well plate overnight. After that, each well was exposed to a CCK-8 reagent (10 μl; Beyotime). After 2 h of incubation, the absorbance at 450 nm was assessed using a microplate reader.
Co-culture system
CAFs-N or CAFs-H cells were seeded into Transwell polyester permeable supports. SW480 cells were seeded into the bottom chamber. SW480 cells (bottom chamber) were co-cultured with transfected CAFs-N or CAFs-H cells (upper chamber) for 48 h. Subsequently, a CCK-8 assay was used to determine cell viability.
Transwell assays
Cell migration and invasion assays were performed using Transwell assays in 24-well Transwell chambers (Corning). SW480 cells suspended in 100 µl of serum-free DMEM were plated onto the upper chamber, and 500 μl of DMEM plus 10% FBS was added to the lower chamber. After 24 h of incubation, the cells that passed through the filter were stained with 0.2% crystal violet. Subsequently, cells were observed under a microscope. For the invasion assay, the lower chamber was pre-coated with Matrigel (BD Biosciences).
Luciferase reporter assay
The wild-type or mutant 3′-untranslated region (UTR) of HMGB3 was inserted into the pGL6-miR‐based luciferase reporter vector (Beyotime). SW480 cells were co-transfected with HMGB3 wild-type or mutant plasmids and the miR-200b-3p agomir or agomir NC using Lipofectamine 2000 for 48 h. Next, the luciferase activity was detected with the Dual Luciferase Reporter Assay System.
Animal study
Four-week-old BALB/c nude mice were purchased from Weitonglihua, China. SW480 cells (107 cells) were injected into the left flank of nude mice subcutaneously. When the tumors reached approximately 200 mm3 in size, the animals were divided randomly into four groups: control, 5-FU, 5-FU + CAFs-N/NC-Exo, and 5-FU + CAFs-H/NC-Exo. Animals were injected with 5-FU (25 mg/kg) intraperitoneally once a week. Meanwhile, PBS, CAFs-N/NC-Exo, or CAFs-H/NC-Exo was injected intratumorally twice weekly for 3 weeks. The tumor volume was measured every week using the following formula: volume = (length × width2)/2. The mice were sacrificed on day 21 and the tumors were removed. Subsequently, the tumor volumes and tumor weights were measured. Cell apoptosis was assessed using the APO-BrdU TUNEL assay kit. The animal study was approved by the Ethics Committee of the Zhejiang Provincial People’s Hospital.
Statistical analysis
Data are expressed as the mean ± standard deviation. Group comparisons were performed using one-way analysis of variance and Tukey’s test. Differences were considered statistically significant at p < 0.05. All data were analyzed in triplicate.
Results
MiR-200b-3p can be transferred from hypoxic CAFs to SW480 cells via exosomes
Tumor cell growth is closely related to the TME, in which hypoxia and CAFs are two major factors (27, 28). Thus, CAFs and NFs were isolated from the CRC and adjacent normal tissues, respectively. As shown in Figure 1A, the level of FAP (a specific fibroblast marker) was much higher in CAFs than in NFs, indicating the successful isolation of CAFs. To investigate whether hypoxic CAFs could affect 5-FU sensitivity in CRC cells, SW480 cells were co-cultured with CAFs-N or CAFs-H. As shown in Figures 1B, C, 5-FU sharply reduced CRC cell viability, whereas CAFs-H notably reversed that change, suggesting that CAFs-H significantly reduced the sensitivity of CRC cells to 5-FU.
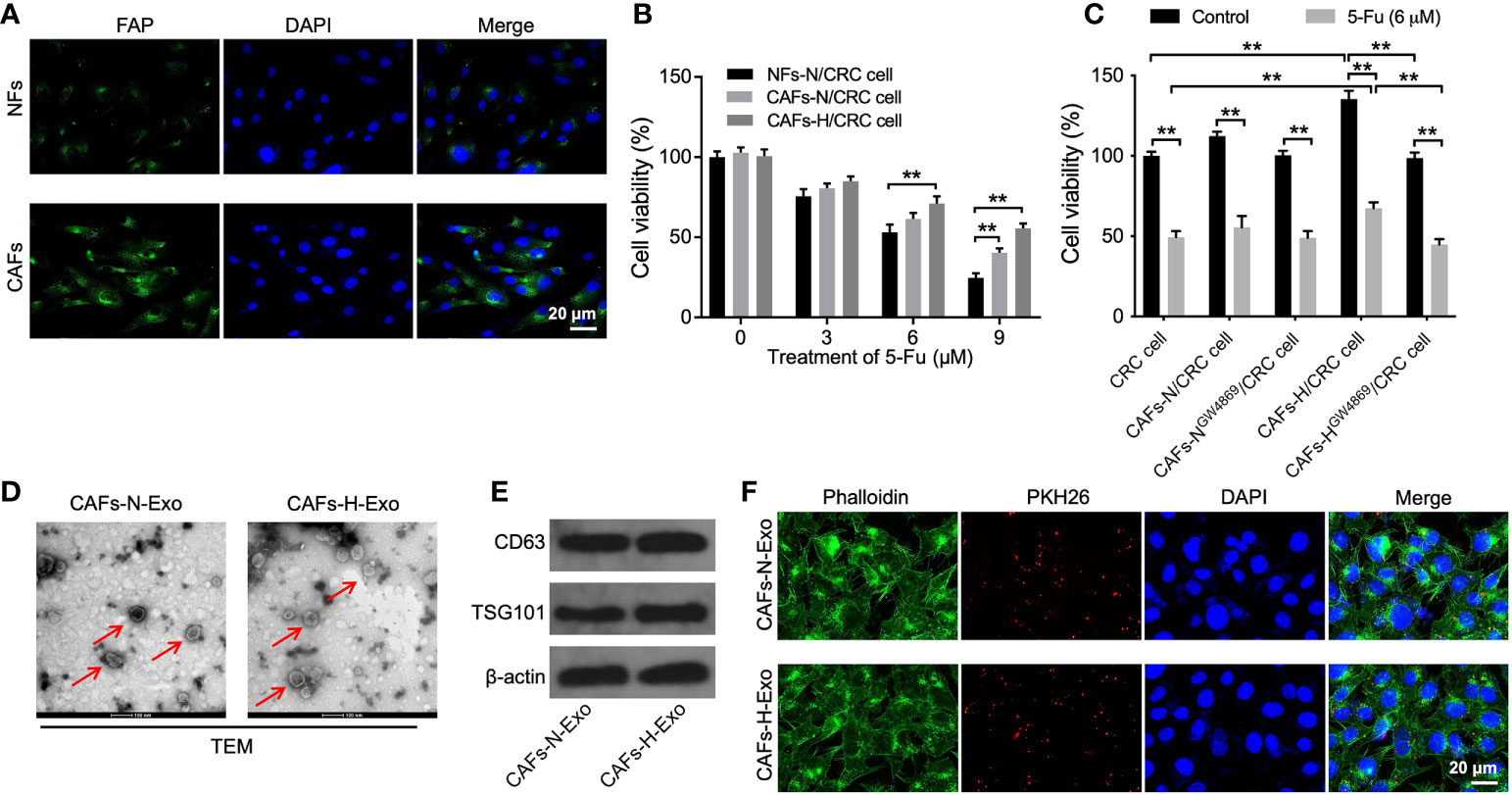
Figure 1 Hypoxic CAFs-derived exosomes can be transferred to SW480 cells. (A) NFs and CAFs were isolated from CRC tissues and adjacent normal tissues, respectively. IF staining for FAP expression in CAFs and NFs. (B) CRC cells were treated with 0, 3, 6, or 9 μM 5-FU for 48 h. Then, CRC cells were co-cultured with NFs-N, CAFs-N, or CAFs-H. The viability of CRC cells was tested using the CCK8 assay. (C) CRC cells were treated with 6 μM 5-FU for 48 h. Then, CRC cells were co-cultured with CAFs-N, CAFs-H, or GW4869-treated CAFs-N or CAFs-H. The viability of CRC cells was tested by CCK8 assay. (D) Identification of exosomes (CAFs-N-Exo and CAFs-H-Exo) derived from CAFs cultured under normoxia and hypoxia by TEM. Red arrow points indicate exosomes. (E) Western blot analysis of exosome surface markers CD63 and TSG101 in CAFs-N-Exo and CAFs-H-Exo. (F) Fluorescence images showed the internalization of PKH26-labeled CAFs-N-Exo or CAFs-H-Exo (red) by SW480 cells. **p < 0.01.
It has been shown that exosomes carrying miRNAs could modulate the communication between CAFs and cancer cells (19, 20). Thus, to explore whether hypoxic CAFs-derived exosomes result in this effect, exosome inhibitor GW4869 was used (29). As indicated in Figure 1C, reducing the exosome secretion of hypoxic CAFs by GW4869 inhibited the ability of hypoxic CAFs to decrease sensitivity to 5-FU in CRC cells. These results showed that exosomes play a key role in mediating the communication between hypoxic CAFs and SW480 cells.
Next, CAFs-N-Exo and CAFs-H-Exo were isolated from the supernatants of CAFs-N or CAFs-H, respectively. These two vesicles were identified as round and cup-shaped membrane-coated particles using TEM (Figure 1D). In addition, these two vesicles expressed the exosomal markers CD63 and TSG101 (Figure 1E). Meanwhile, PKH26-labeled exosomes were observed in SW480 cells (Figure 1F), suggesting that exosomes could be absorbed by SW480 cells.
As shown in Supplementary Figure 1A, the miR-200b-3p level was significantly downregulated in CRC tissues. Additionally, the miR-200b-3p level in SW480 cells was markedly upregulated by the miR-200b-3p agomir (Supplementary Figure 1B). Meanwhile, compared to exosomes derived from miRNA NC-transfected normoxia CAFs (CAFs-N/NC-Exo), the miR-200b-3p level was significantly downregulated in exosomes derived from miRNA NC-transfected hypoxic CAFs (CAFs-H/NC-Exo) (Supplementary Figure 1C). Furthermore, miR-200b-3p levels in CRC cells were notably elevated by exosomes derived from normoxia or hypoxic CAFs that were transfected with the miR-200b-3p agomir (CAFs-N/miR-200b-3p agomir-Exo or CAFs-H/miR-200b-3p agomir-Exo) (Supplementary Figure 1D). Thus, miR-200b-3p can be transferred from hypoxic CAFs to SW480 cells via exosomes.
Exosomal miR-200b-3p derived from hypoxic CAFs increases the sensitivity of CRC cells to 5-FU
To investigate the function of hypoxic CAFs-derived exosomal miR-200b-3p in CRC, CCK-8, trypan blue staining, transwell, and flow cytometry assays were performed. As indicated in Figures 2A–E, 5-FU treatment remarkably reduced CRC cell viability, migration, and invasion and promoted cell death and apoptosis, whereas CAFs-N/NC-Exo or CAFs-H/NC-Exo significantly reversed these phenomena, suggesting that normoxia or hypoxic CAFs could reduce the sensitivity of CRC cells to 5-FU. Significantly, compared to CAFs-H/NC-Exo, CAFs-H/miR-200b-3p agomir-Exo reduced CRC cell viability, migration, and invasion and increased cell death (Figures 2A–E). In summary, exosomal miR-200b-3p derived from hypoxic CAFs could increase the sensitivity of CRC cells to 5-FU.
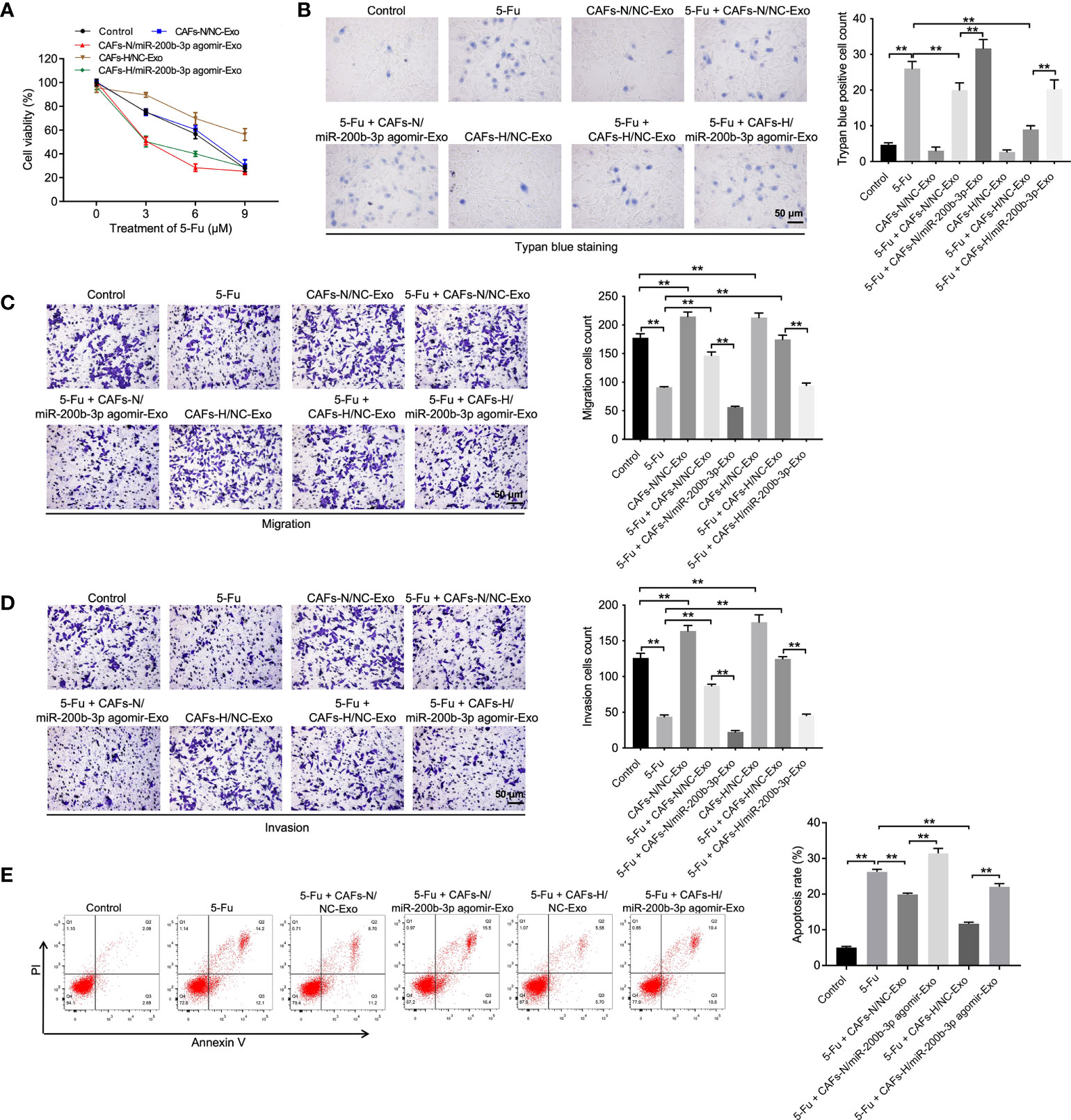
Figure 2 Exosomal miR-200b-3p derived from hypoxic CAFs increases the sensitivity of CRC cells to 5-FU. CRC cells were treated with 3, 6, or 9 μM 5-FU for 48 h. Then, CRC cells were treated with CAFs-N/NC-Exo, CAFs-N/miR-200b-3p agomir-Exo, CAFs-H/NC-Exo, or CAFs-H/miR-200b-3p agomir-Exo. (A) The viability of CRC cells was tested using the CCK8 assay. (B) The death of CRC cells was tested using trypan blue staining. (C, D) The migration and invasion of CRC cells was detected using the transwell assay. (E) The apoptosis of CRC cells was tested by flow cytometry. **p < 0.01.
HMGB3 is the target mRNA of miR-200b-3p
TargetScan (https://www.targetscan.org/vert_71/) was used to identify the downstream targets of miR-200b-3p. As shown in Figure 3A, the 3′UTR of HMGB3 mRNA contained a complementary miR-200b-3p binding sequence, suggesting that HMGB3 might be a downstream target of miR-200b-3p. In addition, a dual luciferase reporter assay was performed to validate whether HMGB3 is indeed a target of miR-200b-3p. As indicated in Figure 3B, the relative luciferase activity in WT-HMGB3 was significantly reduced by the miR-200b-3p agomir. Moreover, compared with the NC group, the HMGB3 level was significantly upregulated in SW480 cells transfected with pcDNA3.1-HMGB3 (Figure 3C). Meanwhile, the HMGB3 level in CRC cells was significantly inhibited by the miR-200b-3p agomir, while this effect was reversed by HMGB3 overexpression (Figures 3D, E). Furthermore, CAFs-H/NC-Exo markedly increased the HMGB3 level in CRC cells compared to the control group (Figure 3E). Taken together, HMGB3 is a direct binding target mRNA of miR-200b-3p.
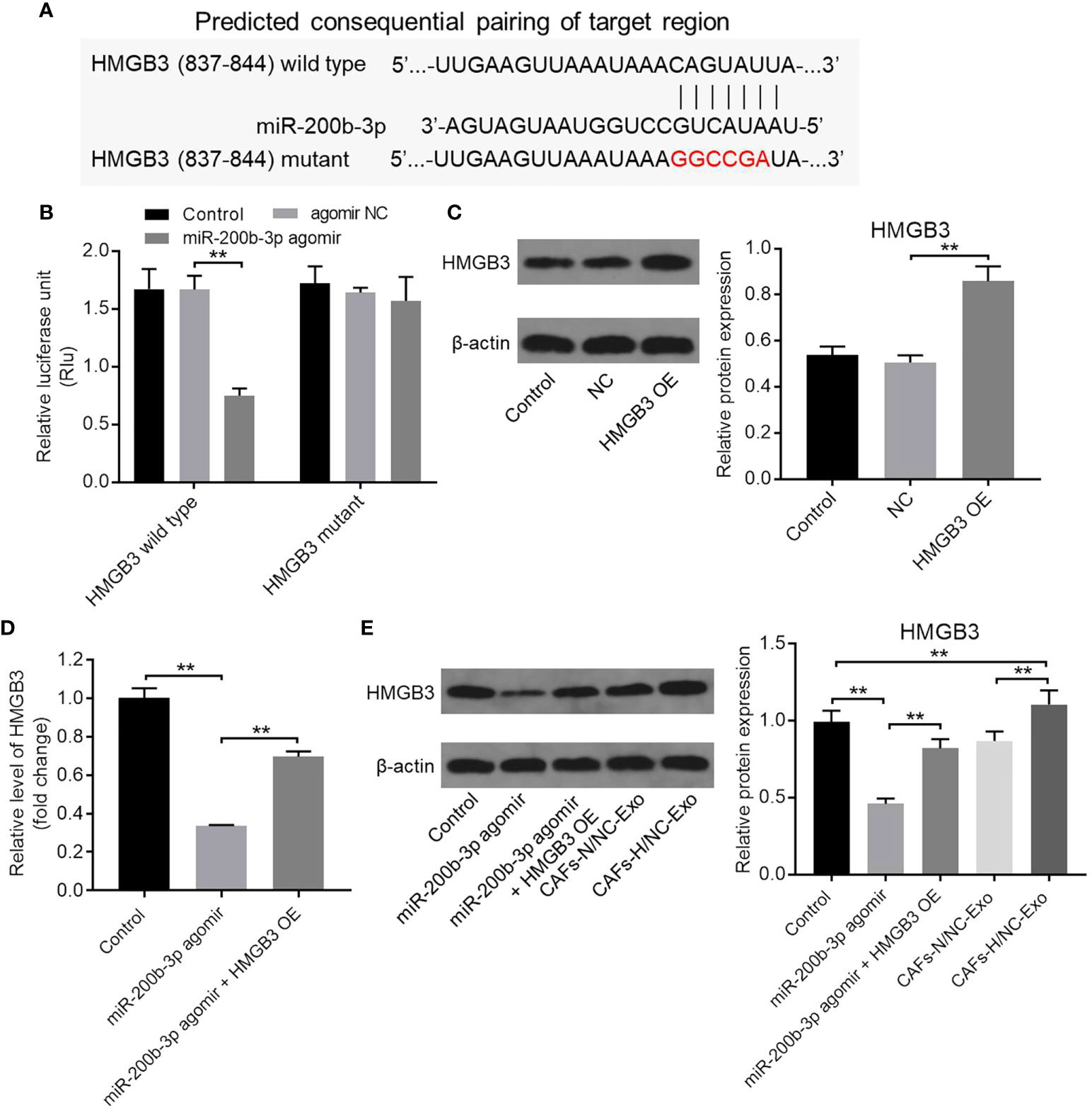
Figure 3 HMGB3 was identified to be the target mRNA of miR-200b-3p. (A) Targetscan was used to predict the downstream mRNA of miR-200b-3p. (B) The relative luciferase activity in WT/MT-HMGB3 was tested using the dual luciferase report assay. (C) SW480 cells were transfected with NC or pcDNA3.1-HMGB3 for 48 h. The HMGB3 level in SW480 cells was investigated by Western blot. The relative expression was quantified by normalizing to β-actin. (D) The HMGB3 level in CRC cells was evaluated using RT-qPCR. (E) SW480 cells were treated with the miR-200b-3p agomir, miR-200b-3p agomir + HMGB3 OE, CAFs-N/NC-Exo or CAFs-H/NC-Exo for 48 h. The level of HMGB3 in CRC cells was investigated by Western blot. **P < 0.01.
Overexpression of miR-200b-3p increases the sensitivity of CRC cells to 5-FU by inhibiting HMGB3
To investigate the mechanism by which the miR-200b-3p agomir increases the sensitivity of CRC cells to 5-FU, CCK-8, and trypan blue staining assays were performed. As shown in Figures 4A, B and Supplementary Figure 2, the anti-tumor effects of 5-FU in CRC cells were enhanced by the miR-200b-3p agomir; however, these changes were notably reversed by HMGB3 overexpression. In addition, the level of active β-catenin, total β-catenin, and c-Myc in SW480 cells was notably decreased by 5-FU (Figure 4C). As expected, the miR-200b-3p agomir further reduced the active and total β-catenin level and c-Myc level in SW480 cells compared with the 5-FU group (Figure 4C). However, the effects of miR-200b-3p on these proteins were notably abolished by HMGB3 overexpression (Figure 4C). These results indicated that miR-200b-3p overexpression could increase the sensitivity of CRC cells to 5-FU by inhibiting HMGB3/β-catenin/c-Myc signaling.
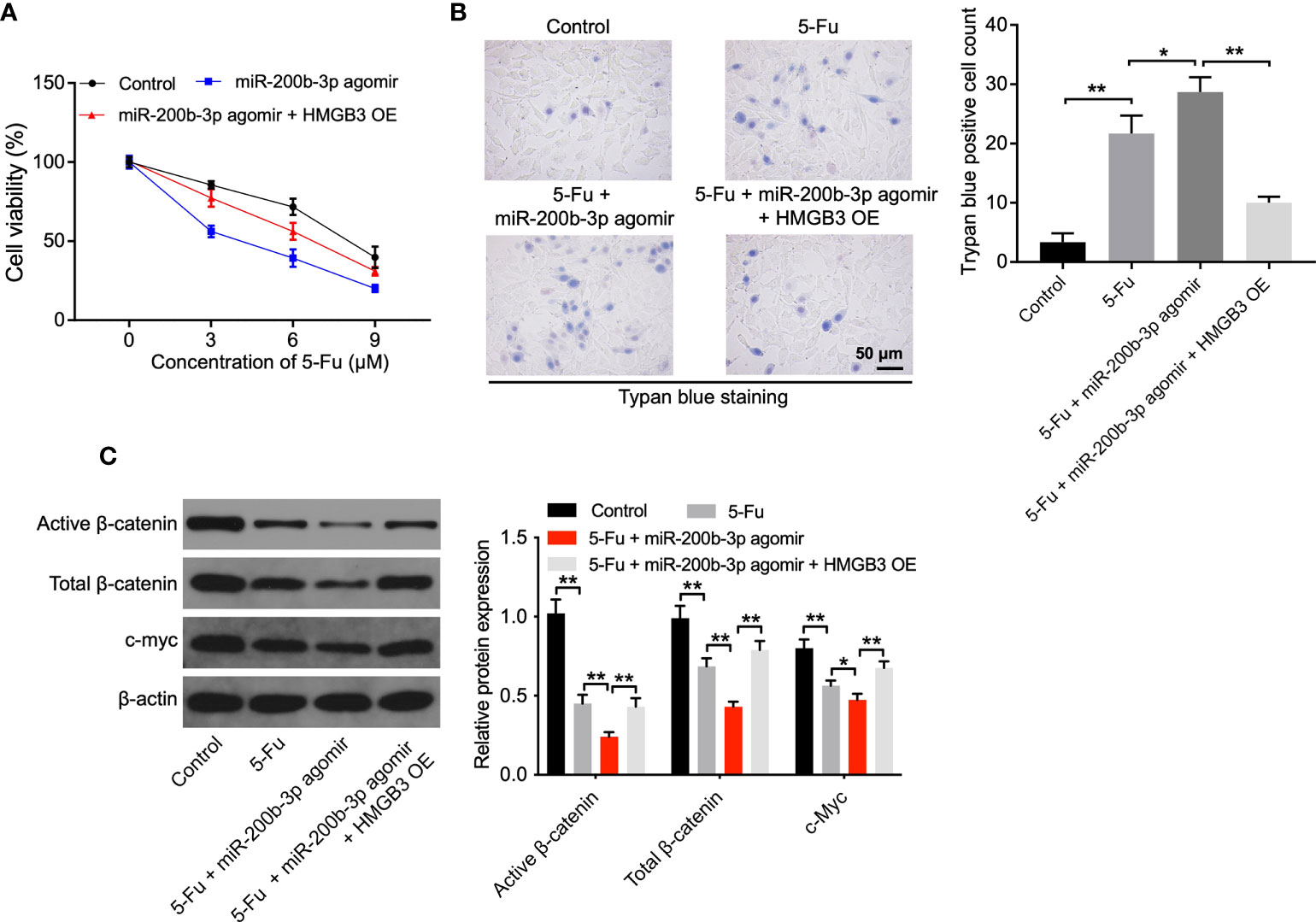
Figure 4 The miR-200b-3p agomir increases the sensitivity of CRC cells to 5-FU via inhibiting HMGB3. (A) SW480 cells were treated with 0, 3, 6, or 9 μM 5-FU for 48 h. Then, cells were treated with the miR-200b-3p agomir or miR-200b-3p agomir + HMGB3 OE. The viability of SW480 cells was tested by CCK8 assay. (B) The death of SW480 cells was tested using trypan blue staining. (C) The expressions of active β-catenin, total β-catenin, and c-Myc in SW480 cells were detected by Western blot. *p < 0.05, **p < 0.01.
Loss of miR-200b-3p in hypoxic CAFs-derived exosomes weakened the anti-tumor effects of 5-FU on CRC cells in vivo
Finally, we investigated the effect of CAFs-H-Exo on the tumorigenicity of CRC in vivo. As shown in Figures 5A, B, 5-FU treatment obviously reduced the tumor volume and tumor weight, whereas CAFs-N/NC-Exo or CAFs-H/NC-Exo treatment partially reversed these changes. In addition, CAFs-H/NC-Exo notably reduced the apoptotic effect of 5-FU on tumor tissues (Figure 5C). Moreover, 5-FU significantly downregulated the HMGB3, c-Myc, and β-catenin level in tumor tissues; however, these changes were reversed by CAFs-N/NC-Exo or CAFs-H-Exo (Figure 5D). Meanwhile, compared with the CAFs-N/NC-Exo group, CAFs-H/NC-Exo further weakened the anti-tumor effects of 5-FU on CRC in vivo (Figures 5A–D). Significantly, the miR-200b-3p level was significantly downregulated in CAFs-H/NC-Exo, compared to CAFs-N/NC-Exo (Supplementary Figure 1C). These data show that the loss of miR-200b-3p in hypoxic CAFs-derived exosomes could reduce the sensitivity of SW480 cells to 5-FU in vivo via upregulating HMGB3/β-catenin/c-Myc signaling.
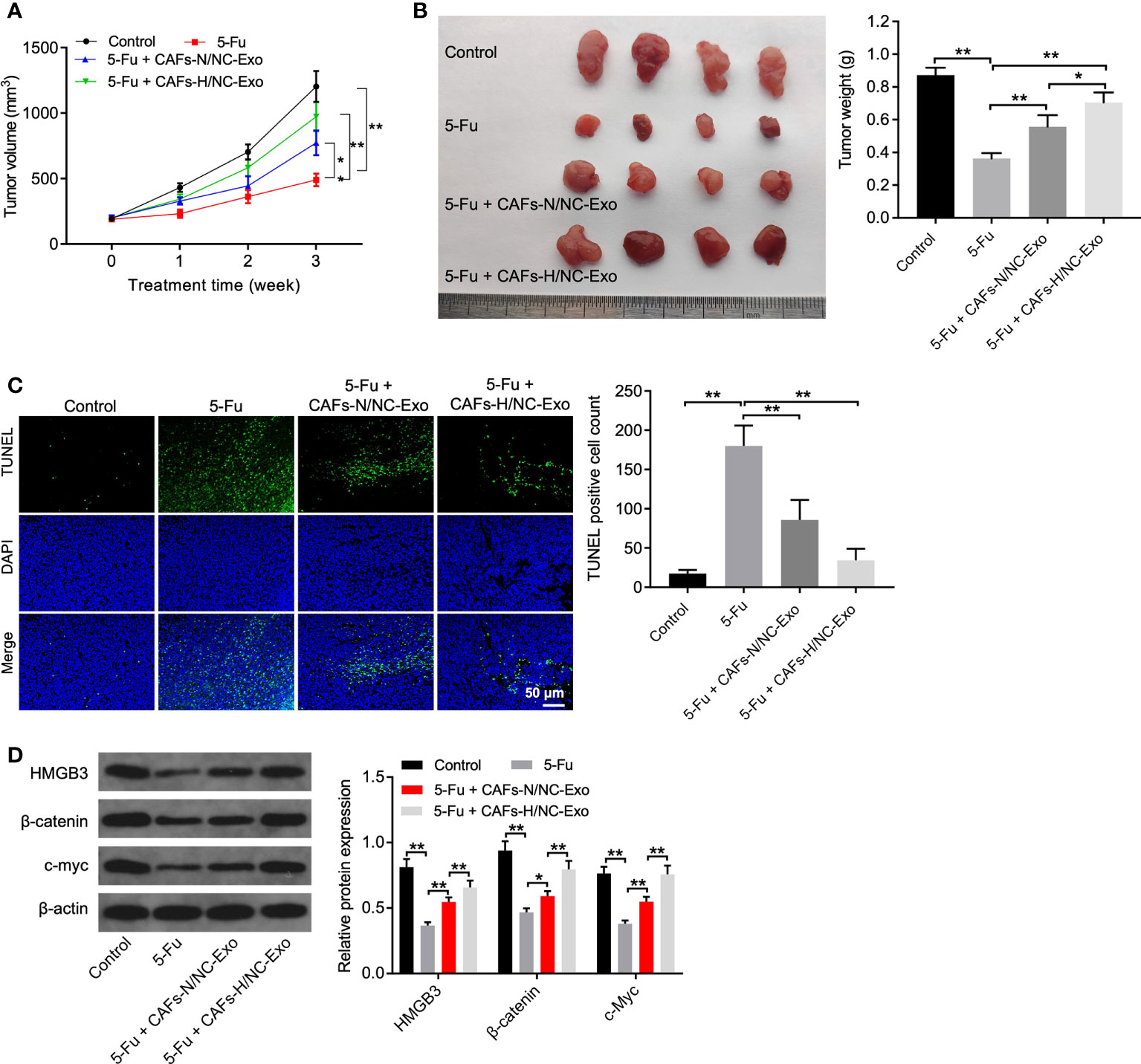
Figure 5 Loss of miR-200b-3p in hypoxic CAFs-derived exosomes weakened the anti-tumor effects of 5-FU on CRC in vivo. (A, B) Tumor volume and tumor weight of xenograft tumors. (C) TUNEL analysis of cell apoptosis in tumor tissues. (D) Western blot analysis of HMGB3, β-catenin, and c-Myc expressions in tumor tissues. *p < 0.05, **p < 0.01.
Discussion
Hypoxia plays a crucial role in tumor progression (30). CAFs can regulate cancer progression, acting as key modulators in the TME (31, 32). Exosomes are important mediators of cell-to-cell crosstalk between tumor and surrounding cells via transmitting miRNAs (33). Jin et al. suggested that CAF-derived exosomal miR-3656 could promote the progression and development of esophageal squamous cell carcinoma (34), and Fan et al. suggested that exosomal miR-26a from CAFs could facilitate endometrial cancer progression by targeting the signal transducer and activator of the transcription 3/chitinase 3-like 1 signaling pathway (35). However, the specific role of hypoxia in CAFs requires further exploration. Thus, we explored the crosstalk between hypoxic CAFs and CRC cells. Our results showed that compared with hypoxic CAF-derived exosomes, hypoxic CAF-derived exosomes with the miR-200b-3p agomir can increase the sensitivity of CRC cells to 5-FU, and this is the first study to explore the function of exosomal miR-200b-3p in CRC. In contrast, Chen et al. found that exosomal miR-590-3p derived from CAFs was able to confer radioresistance to CRC cells (22), and Yin et al. suggested that CAF-derived exosomes with upregulated miR-135b-5p levels can promote CRC cell growth by inhibiting thioredoxin-interacting protein (36). These results showed that miRNAs involved in the communication between hypoxic CAFs and CRC cells.
Exosome-mediated transfer of miRNAs from CAFs to cancer cells can induce chemoresistance during cancer progression (33, 37). Exosomal miR-200b was found to act as a biomarker in human cancers including CRC (38–40). In addition, the miR-200b level was found to be downregulated in CRC (41). Thus, we investigated the role of exosomal miR-200b-3p in CRC. In our study, compared to normoxia CAFs-derived exosomes, the miR-200b-3p level was significantly downregulated in hypoxic CAF-derived exosomes. Meanwhile, CAFs-H/NC-Exo weakened the anti-tumor effects of 5-FU on CRC in vitro and in vivo, compared with the CAFs-N/NC-Exo group. These results showed that compared to normoxia CAFs-derived exosomes, loss of exosomal miR-200b-3p in hypoxia CAFs could reduce the sensitivity of CRC cells to 5-FU. Additionally, miR-200b-3p can be transferred from hypoxic CAFs to CRC cells via exosomes. Normoxia or hypoxic CAF-derived exosomes reduced the sensitivity of CRC cells to 5-FU, while these effects were reversed by normoxia or hypoxic CAF-derived exosomes with upregulated miR-200b-3p levels, respectively. These data showed that increasing the exosomal miR-200b-3p level derived from hypoxic CAFs could reverse the effect of hypoxic CAF-derived exosomes on 5-FU sensitivity in CRC cells.
HMGB3 acts as the downstream mRNA of miR-200b-3p in CRC. HMGB3 is a crucial mediator of cancer development and associated with chemoresistance during cancer progression (42, 43). Yu et al. found that lncRNA OIP5-AS1 could facilitate the chemoresistance of breast cancer cells to trastuzumab via the upregulation of HMGB3 (43). Additionally, HMGB3 could facilitate CRC progression via activating WNT/β-catenin/c-Myc signaling (44). β-catenin is a vital modulator of Wnt signaling and plays important roles in cancer metastasis and chemoresistance (45, 46). Meanwhile, c-Myc is a downstream target of β-catenin, which could contribute to chemoresistance in CRC (47–49). In this study, we found that hypoxic CAFs-derived exosomes could increase the HMGB3 level in CRC cells. Meanwhile, hypoxic CAFs-derived exosomes could reverse 5-FU-induced HMGB3, c-Myc, and β-catenin downregulation in tumor tissues, suggesting that hypoxic CAFs-derived exosomes could reduce the sensitivity of CRC cells to 5-FU via activating HMGB3/β-catenin/c-Myc signaling. In addition, the miR-200b-3p agomir could increase the sensitivity of 5-FU to CRC cells by the inhibition of HMGB3/β-catenin/c-Myc signaling. Collectively, exosomes with upregulated miR-200b-3p levels derived from hypoxic CAFs could increase the sensitivity of 5-FU to CRC cells via inhibiting the HMGB3/β-catenin/c-Myc axis.
This study has some limitations. First, other target mRNAs of miR-200b-3p in CRC were not explored. One miRNA has been found to target multiple genes (50). Evidence has shown that miR-200b could target fascin actin-bundling protein 1 (FSCN1), and p70S6K1 and these two target genes (FSCN1 and p70S6K1) are involved in the chemoresistance of cancers (51, 52). Thus, further studies are needed to investigate whether exosomal miR-200b-3p could modulate CRC progression via targeting other genes (e.g. FSCN1 and p70S6K1). Second, other signaling pathways involved in exosomal miR-200b-3p-mediated CRC progression could not be investigated. Future studies should focus on addressing these limitations for more novel insights.
Conclusion
Our results revealed that the loss of exosomal miR-200b-3p in hypoxia CAFs could reduce the sensitivity of CRC cells to 5-FU by upregulating HMGB3. Thus, our research may be beneficial for future studies aiming at the development of possible therapeutic strategies for CRC.
Data availability statement
The original contributions presented in the study are included in the article/Supplementary Materials. Further inquiries can be directed to the corresponding author.
Ethics statement
The studies involving human participants were reviewed and approved by the ethics committee of Zhejiang Provincial People’s Hospital (No. 20211009ZPPH). The patients/participants provided their written informed consent to participate in this study. The animal study was reviewed and approved by the ethics committee of Zhejiang Provincial People’s Hospital.
Author contributions
The study was conceived and designed by HY, BC, RC, and ST, and the experiments were performed by WG, ZW, and BZ. Data were analyzed, and the paper was written by XH, YG, SG, QD, and PY. All authors have reviewed and agreed to the submission of the manuscript.
Funding
This study was financially supported by the Natural Science Foundation of Zhejiang Province (No. LY18H160041, LY17H160064, and LQ21H160042), the Funding Project of Health and Family Planning Commission of Zhejiang Province (2018KY217), and the Funding Project Administration of Traditional Chinese Medicine of Zhejiang Province (2018ZA009).
Conflict of interest
The authors declare that the research was conducted in the absence of any commercial or financial relationships that could be construed as a potential conflict of interest.
Publisher’s note
All claims expressed in this article are solely those of the authors and do not necessarily represent those of their affiliated organizations, or those of the publisher, the editors and the reviewers. Any product that may be evaluated in this article, or claim that may be made by its manufacturer, is not guaranteed or endorsed by the publisher.
Supplementary material
The Supplementary Material for this article can be found online at: https://www.frontiersin.org/articles/10.3389/fonc.2022.920131/full#supplementary-material
Supplementary Figure 1 | MiR-200b-3p was downregulated in CRC tissues. (A) The level of miR-200b-3p in CRC tissues or adjacent normal tissues was tested by RT-qPCR. (B) CAFs cells were transfected with the NC or miR-200b-3p agomir. The level of miR-200b-3p in CAFs was investigated by RT-qPCR. (C) The level of miR-200b-3p in CAFs-N/NC-Exo and CAFs-H/NC-Exo was evaluated by RT-qPCR. (D) CRC cells were treated with CAFs-N/NC-Exo, CAFs-N/miR-200b-3p agomir-Exo, CAFs-H/NC-Exo, or CAFs-H/miR-200b-3p agomir-Exo. The level of miR-200b-2p in CRC cells was evaluated by RT-qPCR. **p < 0.01.
Supplementary Figure 2 | The miR-200b-3p agomir increases the sensitivity of SW620 cells to 5-FU. SW620 cells were treated with 6 μM 5-FU for 48 h. Then, cells were treated with the miR-200b-3p agomir or miR-200b-3p agomir + HMGB3 OE. The viability of SW620 cells was tested by CCK8 assay.
References
1. Balacescu O, Sur D, Cainap C, Visan S, Cruceriu D, Manzat-Saplacan R, et al. The impact of miRNA in colorectal cancer progression and its liver metastases. Int J Mol Sci (2018) 19(12):3711. doi: 10.3390/ijms19123711
2. Wang L, Cho KB, Li Y, Tao G, Xie Z, Guo B. Long noncoding RNA (lncRNA)-mediated competing endogenous RNA networks provide novel potential biomarkers and therapeutic targets for colorectal cancer. Int J Mol Sci (2019) 20(22):5758. doi: 10.3390/ijms20225758
3. Piawah S, Venook AP. Targeted therapy for colorectal cancer metastases: A review of current methods of molecularly targeted therapy and the use of tumor biomarkers in the treatment of metastatic colorectal cancer. Cancer (2019) 125(23):4139–47. doi: 10.1002/cncr.32163
4. Kuipers EJ, Grady WM, Lieberman D, Seufferlein T, Sung JJ, Boelens PG, et al. Colorectal cancer. Nat Rev Dis Primers (2015) 1:15065. doi: 10.1038/nrdp.2015.65
5. Bashir B, Snook AE. Immunotherapy regimens for metastatic colorectal carcinomas. Hum Vaccin Immunother (2018) 14(2):250–4. doi: 10.1080/21645515.2017.1397244
6. Balintova L, Matuskova M, Gabelova A. The evaluation of the efficacy and potential genotoxic hazard of combined SAHA and 5-FU treatment in the chemoresistant colorectal cancer cell lines. Mutat Res Genet Toxicol Environ Mutagen (2022) 874-875:503445. doi: 10.1016/j.mrgentox.2022.503445
7. Fakhr E, Zare F, Azadmanesh K, Teimoori-Toolabi L. LEF1 silencing sensitizes colorectal cancer cells to oxaliplatin, 5-FU, and irinotecan. BioMed Pharmacother (2021) 143:112091. doi: 10.1016/j.biopha.2021.112091
8. McKeown SR. Defining normoxia, physoxia and hypoxia in tumours-implications for treatment response. Br J Radiol (2014) 87(1035):20130676. doi: 10.1259/bjr.20130676
9. Krishnamachary B, Mironchik Y, Jacob D, Goggins E, Kakkad S, Ofori F, et al. Hypoxia theranostics of a human prostate cancer xenograft and the resulting effects on the tumor microenvironment. Neoplasia (2020) 22(12):679–88. doi: 10.1016/j.neo.2020.10.001
10. Meng W, Hao Y, He C, Li L, Zhu G. Exosome-orchestrated hypoxic tumor microenvironment. Mol Cancer (2019) 18(1):57. doi: 10.1186/s12943-019-0982-6
11. Silvoniemi A, Suilamo S, Laitinen T, Forsback S, Löyttyniemi E, Vaittinen S, et al. Repeatability of tumour hypoxia imaging using [(18)F]EF5 PET/CT in head and neck cancer. Eur J Nucl Med Mol Imaging (2018) 45(2):161–9. doi: 10.1007/s00259-017-3857-3
12. Qin Y, Liu HJ, Li M, Zhai DH, Tang YH, Yang L, et al. Salidroside improves the hypoxic tumor microenvironment and reverses the drug resistance of platinum drugs via HIF-1α signaling pathway. EBioMedicine (2018) 38:25–36. doi: 10.1016/j.ebiom.2018.10.069
13. Wu HJ, Hao M, Yeo SK, Guan JL. FAK signaling in cancer-associated fibroblasts promotes breast cancer cell migration and metastasis by exosomal miRNAs-mediated intercellular communication. Oncogene (2020) 39(12):2539–49. doi: 10.1038/s41388-020-1162-2
14. Sazeides C, Le A. Metabolic relationship between cancer-associated fibroblasts and cancer cells. Adv Exp Med Biol (2018) 1063:149–65. doi: 10.1007/978-3-319-77736-8_11
15. Su S, Chen J, Yao H, Liu J, Yu S, Lao L, et al. CD10(+)GPR77(+) cancer-associated fibroblasts promote cancer formation and chemoresistance by sustaining cancer stemness. Cell (2018) 172(4):841–56.e16. doi: 10.1016/j.cell.2018.01.009
16. Deep G, Panigrahi GK. Hypoxia-induced signaling promotes prostate cancer progression: Exosomes role as messenger of hypoxic response in tumor microenvironment. Crit Rev Oncog (2015) 20(5-6):419–34. doi: 10.1615/CritRevOncog.v20.i5-6.130
17. Kugeratski FG, Atkinson SJ, Neilson LJ, Lilla S, Knight JRP, Serneels J, et al. Hypoxic cancer-associated fibroblasts increase NCBP2-AS2/HIAR to promote endothelial sprouting through enhanced VEGF signaling. Sci Signal (2019) 12(567):eann8247. doi: 10.1126/scisignal.aan8247
18. Wang H, Wei H, Wang J, Li L, Chen A, Li Z. MicroRNA-181d-5p-Containing exosomes derived from CAFs promote EMT by regulating CDX2/HOXA5 in breast cancer. Mol Ther Nucleic Acids (2020) 19:654–67. doi: 10.1016/j.omtn.2019.11.024
19. Ge Q, Zhou Y, Lu J, Bai Y, Xie X, Lu Z. miRNA in plasma exosome is stable under different storage conditions. Molecules (2014) 19(2):1568–75. doi: 10.3390/molecules19021568
20. Richards KE, Zeleniak AE, Fishel ML, Wu J, Littlepage LE, Hill R. Cancer-associated fibroblast exosomes regulate survival and proliferation of pancreatic cancer cells. Oncogene (2017) 36(13):1770–8. doi: 10.1038/onc.2016.353
21. Pan S, Deng Y, Fu J, Zhang Y, Zhang Z, Qin X. N6methyladenosine upregulates miR181d5p in exosomes derived from cancerassociated fibroblasts to inhibit 5FU sensitivity by targeting NCALD in colorectal cancer. Int J Oncol (2022) 60(2). doi: 10.3892/ijo.2022.5304
22. Chen X, Liu Y, Zhang Q, Liu B, Cheng Y, Zhang Y, et al. Exosomal miR-590-3p derived from cancer-associated fibroblasts confers radioresistance in colorectal cancer. Mol Ther Nucleic Acids (2021) 24:113–26. doi: 10.1016/j.omtn.2020.11.003
23. Wu YZ, Lin HY, Zhang Y, Chen WF. miR-200b-3p mitigates oxaliplatin resistance via targeting TUBB3 in colorectal cancer. J Gene Med (2020) 22(7):e3178. doi: 10.1002/jgm.3178
24. Li YY, Zhou CX, Gao Y. Interaction between oral squamous cell carcinoma cells and fibroblasts through TGF-β1 mediated by podoplanin. Exp Cell Res (2018) 369(1):43–53. doi: 10.1016/j.yexcr.2018.04.029
25. Dai X, Xie Y, Dong M. Cancer-associated fibroblasts derived extracellular vesicles promote angiogenesis of colorectal adenocarcinoma cells through miR-135b-5p/FOXO1 axis. Cancer Biol Ther (2022) 23(1):76–88. doi: 10.1080/15384047.2021.2017222
26. Zeng Z, Li Y, Pan Y, Lan X, Song F, Sun J, et al. Cancer-derived exosomal miR-25-3p promotes pre-metastatic niche formation by inducing vascular permeability and angiogenesis. Nat Commun (2018) 9(1):5395. doi: 10.1038/s41467-018-07810-w
27. Wanandi SI, Ningsih SS, Asikin H, Hosea R, Neolaka GMG. Metabolic interplay between tumour cells and cancer-associated fibroblasts (CAFs) under hypoxia versus normoxia. Malays J Med Sci (2018) 25(3):7–16. doi: 10.21315/mjms2018.25.3.2
28. Petrova V, Annicchiarico-Petruzzelli M, Melino G, Amelio I. The hypoxic tumour microenvironment. Oncogenesis (2018) 7(1):10. doi: 10.1038/s41389-017-0011-9
29. Yan W, Li T, Yin T, Hou Z, Qu K, Wang N, et al. M2 macrophage-derived exosomes promote the c-KIT phenotype of vascular smooth muscle cells during vascular tissue repair after intravascular stent implantation. Theranostics (2020) 10(23):10712–28. doi: 10.7150/thno.46143
30. Noman MZ, Messai Y, Carré T, Akalay I, Méron M, Janji B, et al. Microenvironmental hypoxia orchestrating the cell stroma cross talk, tumor progression and antitumor response. Crit Rev Immunol (2011) 31(5):357–77. doi: 10.1615/critrevimmunol.v31.i5.10
31. Zhao H, Yang L, Baddour J, Achreja A, Bernard V, Moss T, et al. Tumor microenvironment derived exosomes pleiotropically modulate cancer cell metabolism. Elife (2016) 5:e10250. doi: 10.7554/eLife.10250
32. Monteran L, Erez N. The dark side of fibroblasts: Cancer-associated fibroblasts as mediators of immunosuppression in the tumor microenvironment. Front Immunol (2019) 10:1835. doi: 10.3389/fimmu.2019.01835
33. Yang F, Yan Y, Yang Y, Hong X, Wang M, Yang Z, et al. MiR-210 in exosomes derived from CAFs promotes non-small cell lung cancer migration and invasion through PTEN/PI3K/AKT pathway. Cell Signal (2020) 73:109675. doi: 10.1016/j.cellsig.2020.109675
34. Jin Y, Meng Q, Zhang B, Xie C, Chen X, Tian B, et al. Cancer-associated fibroblasts-derived exosomal miR-3656 promotes the development and progression of esophageal squamous cell carcinoma via the ACAP2/PI3K-AKT signaling pathway. Int J Biol Sci (2021) 17(14):3689–701. doi: 10.7150/ijbs.62571
35. Fan JT, Zhou ZY, Luo YL, Luo Q, Chen SB, Zhao JC, et al. Exosomal lncRNA NEAT1 from cancer-associated fibroblasts facilitates endometrial cancer progression via miR-26a/b-5p-mediated STAT3/YKL-40 signaling pathway. Neoplasia (2021) 23(7):692–703. doi: 10.1016/j.neo.2021.05.004
36. Yin H, Yu S, Xie Y, Dai X, Dong M, Sheng C, et al. Cancer-associated fibroblasts-derived exosomes upregulate microRNA-135b-5p to promote colorectal cancer cell growth and angiogenesis by inhibiting thioredoxin-interacting protein. Cell Signal (2021) 84:110029. doi: 10.1016/j.cellsig.2021.110029
37. Qin X, Guo H, Wang X, Zhu X, Yan M, Wang X, et al. Exosomal miR-196a derived from cancer-associated fibroblasts confers cisplatin resistance in head and neck cancer through targeting CDKN1B and ING5. Genome Biol (2019) 20(1):12. doi: 10.1186/s13059-018-1604-0
38. Zhang Z, Xing T, Chen Y, Xiao J. Exosome-mediated miR-200b promotes colorectal cancer proliferation upon TGF-β1 exposure. BioMed Pharmacother (2018) 106:1135–43. doi: 10.1016/j.biopha.2018.07.042
39. Pan C, Stevic I, Müller V, Ni Q, Oliveira-Ferrer L, Pantel K, et al. Exosomal microRNAs as tumor markers in epithelial ovarian cancer. Mol Oncol (2018) 12(11):1935–48. doi: 10.1002/1878-0261.12371
40. Shen L, Chen G, Xia Q, Shao S, Fang H. Exosomal miR-200 family as serum biomarkers for early detection and prognostic prediction of cholangiocarcinoma. Int J Clin Exp Pathol (2019) 12(10):3870–6.
41. Suliman MA, Zhang Z, Na H, Ribeiro AL, Zhang Y, Niang B, et al. Niclosamide inhibits colon cancer progression through downregulation of the notch pathway and upregulation of the tumor suppressor miR-200 family. Int J Mol Med (2016) 38(3):776–84. doi: 10.3892/ijmm.2016.2689
42. Yang M, Zheng E, Ni J, Xu X, Jiang X, Zhao G. Circular RNA circFOXO3 facilitate non-small cell lung cancer progression through upregulating HMGB3 via sponging miR-545-3p/miR-506-3p. Tissue Cell (2021) 75:101702. doi: 10.1016/j.tice.2021.101702
43. Yu Q, Li Y, Peng S, Li J, Qin X. Exosomal-mediated transfer of OIP5-AS1 enhanced cell chemoresistance to trastuzumab in breast cancer via up-regulating HMGB3 by sponging miR-381-3p. Open Med (Wars) (2021) 16(1):512–25. doi: 10.1515/med-2021-0249
44. Zhang Z, Chang Y, Zhang J, Lu Y, Zheng L, Hu Y, et al. HMGB3 promotes growth and migration in colorectal cancer by regulating WNT/β-catenin pathway. PloS One (2017) 12(7):e0179741. doi: 10.1371/journal.pone.0179741
45. Yan R, Fan X, Xiao Z, Liu H, Huang X, Liu J, et al. Inhibition of DCLK1 sensitizes resistant lung adenocarcinomas to EGFR-TKI through suppression of wnt/beta-catenin activity and cancer stemness. Cancer Lett (2022) 531:83–97. doi: 10.1016/j.canlet.2022.01.030
46. Quan Y, Li S, Wang Y, Liu G, Lv Z, Wang Z. Propofol and sevoflurane alleviate malignant biological behavior and cisplatin resistance of xuanwei lung adenocarcinoma by modulating the wnt/beta-catenin pathway and PI3K/AKT pathway. Anticancer Agents Med Chem (2021) 22(11):2098–108. doi: 10.2174/1871520621666211026092405
47. Ripple MJ, Parker Struckhoff A, Trillo-Tinoco J, Li L, Margolin DA, McGoey R, et al. Activation of c-myc and cyclin D1 by JCV T-antigen and β-catenin in colon cancer. PloS One (2014) 9(9):e106257. doi: 10.1371/journal.pone.0106257
48. Xu J, Chen Y, Huo D, Khramtsov A, Khramtsova G, Zhang C, et al. β-catenin regulates c-myc and CDKN1A expression in breast cancer cells. Mol Carcinog (2016) 55(5):431–9. doi: 10.1002/mc.22292
49. Huang TH, Mokgautsi N, Huang YJ, Wu ATH, Huang HS. Comprehensive omics analysis of a novel small-molecule inhibitor of chemoresistant oncogenic signatures in colorectal cancer cell with antitumor effects. Cells (2021) 10(8):1970. doi: 10.3390/cells10081970
50. Kabekkodu SP, Shukla V, Varghese VK, DS J, Chakrabarty S, Satyamoorthy K. Clustered miRNAs and their role in biological functions and diseases. Biol Rev Camb Philos Soc (2018) 93(4):1955–86. doi: 10.1111/brv.12428
51. Gao R, Zhang N, Yang J, Zhu Y, Zhang Z, Wang J, et al. Long non-coding RNA ZEB1-AS1 regulates miR-200b/FSCN1 signaling and enhances migration and invasion induced by TGF-β1 in bladder cancer cells. J Exp Clin Cancer Res (2019) 38(1):111. doi: 10.1186/s13046-019-1102-6
Keywords: miR-200b-3p directly targets HMGB3 CAFs, exosome, hypoxia, miR-200b-3p, HMGB3
Citation: Yuan H, Chen B, Chai R, Gong W, Wan Z, Zheng B, Hu X, Guo Y, Gao S, Dai Q, Yu P and Tu S (2022) Loss of exosomal micro-RNA-200b-3p from hypoxia cancer-associated fibroblasts reduces sensitivity to 5-flourouracil in colorectal cancer through targeting high-mobility group box 3. Front. Oncol. 12:920131. doi: 10.3389/fonc.2022.920131
Received: 14 April 2022; Accepted: 09 September 2022;
Published: 05 October 2022.
Edited by:
Daniele Vergara, University of Salento, ItalyReviewed by:
Elizabeth Ortiz Sánchez, National Institute of Cancerology (INCAN), MexicoSara Sergio, University of Salento, Italy
Copyright © 2022 Yuan, Chen, Chai, Gong, Wan, Zheng, Hu, Guo, Gao, Dai, Yu and Tu. This is an open-access article distributed under the terms of the Creative Commons Attribution License (CC BY). The use, distribution or reproduction in other forums is permitted, provided the original author(s) and the copyright owner(s) are credited and that the original publication in this journal is cited, in accordance with accepted academic practice. No use, distribution or reproduction is permitted which does not comply with these terms.
*Correspondence: Shiliang Tu, dHVzaGlsaWFuZzAyMTFAMTYzLmNvbQ==
†These authors have contributed equally to this work