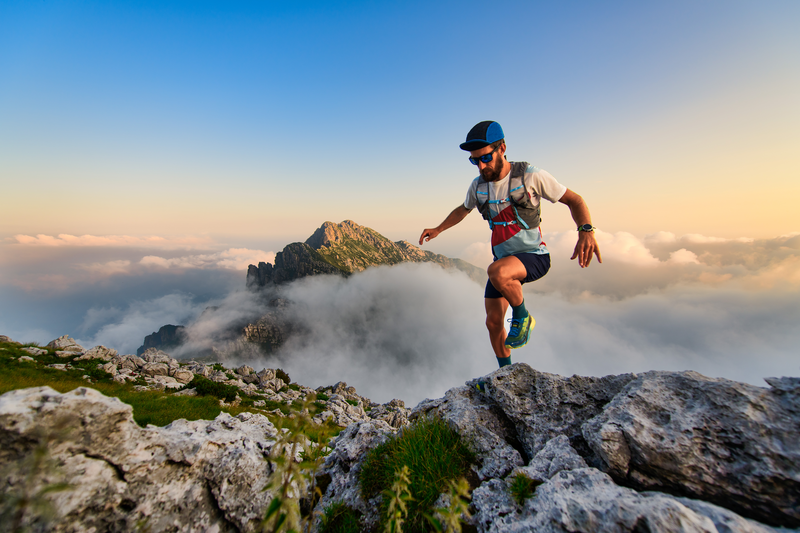
94% of researchers rate our articles as excellent or good
Learn more about the work of our research integrity team to safeguard the quality of each article we publish.
Find out more
ORIGINAL RESEARCH article
Front. Oncol. , 08 July 2022
Sec. Gynecological Oncology
Volume 12 - 2022 | https://doi.org/10.3389/fonc.2022.917834
This article is part of the Research Topic Identification of Immunotherapy-Related Biomarkers for Gynecological Cancers View all 14 articles
In conventional T cells, OX40 has been identified as a major costimulating receptor augmenting survival and clonal expansion of effector and memory T cell populations. In regulatory T cells, (Treg) OX40 signaling suppresses cellular activity and differentiation. However, clinical trials investigating OX40 agonists to enhance anti-tumor immunity, showed only limited success so far. Here we show that platelets from breast cancer patients express relevant levels of OX40L and platelet OX40L (pOX40L) inversely correlates with platelet-expressed immune checkpoint molecules GITRL (pGITRL) and TACI (pTACI). While high expression of pOX40L correlates with T and NK cell activation, elevated pOX40L levels identify patients with higher tumor grades, the occurrence of metastases, and shorter recurrence-free survival (RFS). Of note, OX40 mRNA levels in breast cancer correlate with enhanced expression of anti-apoptotic, immune-suppressive, and tumor-promoting mRNA gene signatures. Our data suggest that OX40L on platelets might play counteracting roles in cancer and anti-tumor immunity. Since pOX40L reflects disease relapse better than the routinely used predictive markers CA15-3, CEA, and LDH, it could serve as a novel biomarker for refractory disease in breast cancer.
Immune checkpoints are crucial parts of inhibitory or activating immune pathways, regulating self-tolerance and immune responses. Particularly in the context of malignant disease, immune-inhibitory receptors including PD-1 or CTLA-4, are triggered to dampen anti-tumor immune responses (1–3). In contrast, co-stimulatory signaling pathways like CD27, CD40, or OX40 have been described to play a crucial role in T cell activation, proliferation, and tumor immune surveillance (4–6). An increasing number of therapeutic strategies have been exploited to block or activate the respective pathways and reinforce anti-tumor immunity (6–9). Strategies to improve co-stimulatory pathways like OX40 have shown promising anti-tumor activity in preclinical mouse models (10–12). The immune checkpoint molecule OX40 is a member of the tumor necrosis factor receptor (TNFR) superfamily. It is expressed on effector T lymphocytes following activation and promotes their differentiation, proliferation, and survival while simultaneously inhibiting the suppressive activity of regulatory T cells (13). Although monoclonal antibodies targeting OX40 are currently being investigated in early clinical trials, a successful translation of the promising preclinical data is still pending (14, 15). Of note, the expression of the cognate ligand (OX40L), also known as tumor necrosis factor superfamily member 4 (TNFSF4) on platelets has been described to be involved in cardiac inflammation and solid tumors (16, 17).
Platelets play a critical role in tumor progression, formation of metastasis, tumor immunosurveillance, and immunotherapy via a manifold of mechanisms, including coating of tumor cells, the release of soluble factors, e.g. TGF-ß, VEGF, and expression of immunoregulatory molecules like PD-L1, GITRL, CD40L and TACI (18–25). Here we show that the immune checkpoint molecule OX40L is frequently expressed on platelets in breast cancer and is negatively correlated with the expression of the immune checkpoint molecules GITRL and TACI on the platelet surface. In breast cancer, high levels of pOX40L were found to be associated with immune cell activation, higher tumor grades, formation of metastasis, and reduced recurrence-free survival (RFS), indicating that pOX40L might play a controversial role in tumor biology via orchestrating immune activation and tumorigenesis. We demonstrate that pOX40L is superior in predicting disease progression compared to routinely used markers in breast cancer.
Although the expression of several immune checkpoint molecules on platelets has recently been described, their role in cancer is only poorly understood (23–25). In an effort to further investigate the impact of platelet-expressed immune checkpoints in solid tumors, we characterized the concurrent expression of the three TNF members pGITRL (pTNFSF18), pTACI (pTNFRSF13B), and pOX40L (pTNFSF4) in a cohort of breast cancer patients (Figure 1A). Interestingly we found a positive correlation of pGITRL and pTACI, two recently identified platelet-expressed immune checkpoint molecules with predictive value in breast cancer (24, 25). Despite the great homology between OX40 and GITR (26), the ligand of the immune stimulatory co-receptor OX40 on platelets was shown to be negatively correlated with pGITRL and pTACI expression (Figures 1B,C). This observation indicates that platelets express a plethora of different immune checkpoint molecules and the platelet immune phenotype (PIP) seems to be specifically regulated, especially in cancer. In in a previous study and in line with the different roles of GITR in NK cells and T cells, pGITRL was found to be associated with T cell but inversely correlated with NK cell activation in breast cancer (9, 24, 27). The fact that highest pGITRL level were present in intermediate tumor stages may support the notion that stimulation of T cell derived GITR contributes to tumor immunosurveillance of breast cancer but will lose its impact in furthering tumor progression in later stages. Expression of the receptor TACI on platelets is also specifically upregulated in breast cancer and is inversely correlated with metastasis and advanced tumor stage. It is not yet understood how pTACI may be involved in the (immune) regulation in breast cancer. One could speculate that pTACI induces reverse signaling into ligand-expressing cells such as breast cancer cells (28–30), but the existence of such signaling still warrants proof (31). Additionally, it was found that TACI is expressed on both, macrophages and tumor cells, particularly in breast cancer cases with poor prognosis (30). Thus, another explanation could be a transfer of TACI between platelets and malignant cells - which has been shown for other pathophysiologically relevant molecules like MHC class I and PD-L1 (19, 23) - or immune cells.
Figure 1 pOX40L in breast cancer patients is associated with a distinct platelet immunophenotype and activation of cytotoxic lymphocytes. (A–C) Comparative analysis of the platelet immune checkpoint molecules pOX40L, pTACI and pGITRL (n = 65). The gating strategy used to analyze pOX40L expression on platelets is given in Supplementary Figure 3. Co-expression of TACI (B) or GITRL (c) on pOX40Llow and pOX40Lhigh platelets, respectively. (D–J) Level of pOX40L in healthy donors (D) and breast cancer patients (G) and expression of CD69 (E, F) and CD25 (H, I) on NK cells and T cells of the respective donors (n = 10 each). (J–Q) Co-regulation of CD69/CD25 on NK/T cells and pOX40L in healthy donors and breast cancer patients (n = 10 each). r-t Comparative analysis of platelet activation in the presence or absence of TRAP-6 (10 µM) with regard to pOX40L status. Representative platelet aggregation studies (R, S) were performed in pOX40L high and low expressing platelets derived from breast cancer patients. Aggregation studies (T) were performed in platelets derived from 5 healthy donors (green) and 10 breast cancer patients (pOX40L low in blue, pOX40L high in red). (U) Expression of CD62P and OX40L on platelets from HD and breast cancer patients ex vivo (n = 10 each). (V) Expression of OX40L on platelets from healthy donors and the complete breast cancer cohort ex vivo (n = 25 and n = 65, respectively). (W) pOX40L level in healthy donors (HD), (left panel) and breast cancer patients (right panel) with regard to CD62P expression (n = 25 and n = 65, respectively). (X) Correlation of pTACI expression and platelet count (left panel) and platelet size (right panel). (B, C), (E, F), (H, I) Each dot represents a single patient. Boxes represent median and 25th to 75th percentiles, whiskers are minimum to maximum.
It is poorly understood how surface levels of immune regulatory molecules on platelets are regulated. Surface expression of some immunoregulative molecules like PD-L1 (23) and CD40L (32) for example are dependent on platelet activation. Changed expression levels in breast cancer however, might be regulated via trogocytosis, reprogrammed megakaryopoiesis (33) or protein synthesis in platelets which can occur at low levels (34). In vitro data show that soluble factors from tumor cells alter megekaryopoiesis in the MEG-01 cell line model and thus the megakaryocyte immunophenotype with regard to GITRL expression. One contributing factor appeared to be TGF-β which is known to be involved in the regulation of GITRL expression on dendritic cells (35). Alike, OX40L is expressed among others on antigen presenting cells where it is upregulated upon antigen presentation and costimulation including interferon gamma (IFN-γ) (36), and following stimulations with prostaglandin E2 (37), IL-18 (38) and thymic stromal lymphopoietin (TSLP) (39). Further studies are needed to study whether these factors also play a role in pOX40L regulation in the context of breast cancer.
Targeting of the co-stimulatory receptor OX40 is recently under evaluation as a novel strategy in cancer immunotherapy, and its expression on CD8+ cytotoxic T cells of breast cancer patients was found to be upregulated upon neo-adjuvant chemotherapy (40). When comparatively analyzing pOX40L and the immunophenotype in a screening cohort of healthy donors and breast cancer patients, we found higher pOX40L levels to be associated with increased T and NK cell activation levels (Figures 1D–I). Especially in breast cancer patients, CD69 and CD25 expression on T and NK cells were strongly correlated with the expression of OX40L on platelets (Figures 1J–Q). This is particularly interesting since previous studies report on an enlarged CD4+ FOXP3+ T cells population in breast cancer (41, 42). Yet, further work is required to evaluate whether pOX40L-dependent signaling contributes to the expansion of regulatory T cells. Taking the known immune-activating role of OX40L on other cell types, including B cells, mast cells or endothelial cells into account, our observation might point to pOX40L as a novel and underestimated player mediating immune cell activation and anti-tumor immunity.
As it is well established that the platelet surface proteome, as well as the PIP, is modulated via platelet activation, we further analyzed pOX40L in the context of platelet reactivity. Platelet aggregometry showed comparable functional capabilities in the presence of the PAR-1 agonist TRAP-6 independent of pOX40L expression (Figures 1R–T). This confirms previous data showing that the expression of immune checkpoint molecules on platelets is not associated with platelet reactivity (23, 24). In contrast to previous observations, platelet activation ex vivo did not result in an expression change of pOX40L on the platelet surface (Figure 1U). Confirming this observation ex vivo, we found no significant correlation of pOX40L and CD62P in our entire cohort of healthy donors and breast cancer patients (Figures 1V, W). Since pOX40L surface expression seems to be rarely regulated during platelet activation, complex adjustment algorithms to model surface expression in vivo are subsequently not required. This highlights pOX40L as a novel putative biomarker candidate in breast cancer. We found that pOX40L, similar to pTACI and pGITRL, was negatively correlated with platelet count and associated with larger platelet size (Figure 1X). This might suggest a complex interplay of anti-cancer treatment, platelet regeneration, and tumor homeostasis, resulting in a subsequent chronic inflammatory environment might be involved in PIP regulation, including pOX40L (43, 44).
In the next step, we studied the association of pOX40L with clinical parameters and disease progression. While pOX40L status was irrespective of the tumor stage (T) and lymph node invasion (N), it was associated with histological grading (G) (p = 0.03), whereas most pOX40Lhigh patients were found in the G3 group (Figures 2A–C). In line, the proliferation index (Ki67) of tumors and formation of metastasis, which both constitute negative prognostic markers for patients, were directly associated with higher pOX40L levels in breast cancer patients (p = 0.005 and p = 0.008), respectively (Figures 2D, E). Accordingly, with a median time to metastasis (TTM) of 17 months compared to 76 months, recurrence-free survival (RFS) was significantly shorter in patients with a high pOX40L expression compared to patients showing low levels of pOX40L (p=0.02) (Figure 3F). Interestingly, this observation seems to be in contrast to the proposed role of pOX40L as an immune-stimulating player promoting anti-tumor effects. Of note, we did not observe any correlation of pOX40L expression and different systemic treatments or number of received treatment regimens (Supplementary Figure1 A-C).
We observed that pOX40L status was inversely correlated with the number of metastatic organs. While patients with high levels of pOX40L showed only one metastatic site, low pOX40L levels were associated with a higher metastatic burden in multiple organs (Figure 2G). These findings led to the hypothesis that pOX40L might be involved in tumor immune cell interactions. Interestingly, pOX40L expression was shown to be independent of treatment modality, kind of treatment and number of treatment regimen (Supplementary Figure 1 A-C). When analyzing the distribution of metastasis in our cohort we observed that patients with high expression of pOX40L frequently developed lung metastasis (Figure 2H). Following this observation, we further investigated putative OX40/OX40L signaling in malignant cells. We correlated OX40 mRNA expression in a TCGA breast cancer data set (TCGA, Firehose Legacy) and gene-expression signatures in breast cancer associated with lung metastasis (45). Remarkably, the main genes involved, e. g. MMP1, MMP2, SPARC, VCMAM1, CXCL1, and CXCR4 were found to be significantly associated in with high OX40 mRNA expression (Figure 2I). In addition, these genes clustered with the expression of anti-apoptotic and tumor-promoting factors (Figures 2I,J). Finally, we studied whether additional immune checkpoint molecules might be co-regulated with OX40 mRNA in breast cancer cells. We found a strong correlation of OX40 and PD-L1, CD80, CD86, VISTA, HVEM, and TGFß, hypothesizing that OX40 expression on tumor cells might be associated with an immune-inhibitory phenotype (Figures 2K–P).
Figure 2 | pOX40L predicts disease relapse and metastasis in breast cancer. (A) pOX40L expression in different tumor stages (T), (n = 65) (B) Association of pOX40L expression and lymph node invasion (N), (n = 65). (C) pOX40L level in patients with different tumor grading (G), (n = 65). (D) Correlation between pOX40L expression on platelets of 65 BC patients and the Ki67 positive cells (%). (E) pOX40L expression in patients with and without metastatic disease (M), (n = 65). (F) Kaplan–Meier curves estimates of RFS (months) in patients with a pOX40L > mean (pOX40Lhigh = red) and pOX40L level < mean (pOX40Llow = blue), (n = 65). Median time to metastasis (pOX40Lhigh = red, pOX40Llow = blue). (G) pOX40L expression in patients with different number of metastasis (n = 65). (H) Mean expression of pOX40L in different metastatic organs (n = 20). (I) Heatmap depicting the relative mRNA level OX40 and a gene set associated with lung metastasis, anti-apoptotic and tumor-promoting signatures in breast tumors (TCGA-Firehose legacy), (n = 65). (J) Spearman correlation mRNA gene signatures and OX40 mRNA. (K–P) Scatter plot of OX40 mRNA and PD-L1 (K), CD80 (l), CD86 (M), VISTA (N), HVEM (O) and TGFß (P) mRNA level in breast cancer. Each dot represents a single patient. (A–E, G, K–P) Each dot represents a single patient. (A–E, G) Data are mean ± SEM.
Our data suggest that OX40L and OX40 are expressed on various cell types in the tumor microenvironment, and OX40/OX40L signaling in cancer might be much more complex than previously known. This might be reflected by the limited success of OX40 targeting therapies so far (10–12). As a result, further comprehensive studies investigating both, OX40 signaling in tumor cells and the functional role of pOX40L on platelets are needed. Since expression of OX40 on breast cancer cells has already been shown to be associated with an aggressive cancer phenotype (46), expression of OX40 on tumor tissue as well as determination of pOX40L might be useful to stratify patients who might benefit from an immunotherapy targeting the OX40 pathway.
Since pOX40L is not regulated upon platelet activation and was shown to predict PFS in our cohort, we finally investigated the potential of pOX40L as a prognostic marker in breast cancer. We comparatively analyzed pOX40L expression and commonly used clinical markers (47–49) to predict disease relapse in breast cancer (Figure 3A). Whereas pOX40L expression, as well as CA15-3, CEA and LDH was independent of the respective subtype (Figure 3B; Supplementary Figures 2A–C), we observed a positive correlation of pOX40L expression and the conventional tumor markers CEA and LDH (Figures 3C–E). This in line with our observation that pOX40L was also associated with higher Ki67 level and higher tumor grading. Of note, with a sensitivity of 0.78 (95% CI: 0.55 – 0.91) and specificity of 0.77 (95% CI: 0.63 – 0.86) pOX40L showed the highest accuracy in predicting disease relapse (Figures 3F–K). Since ER/HER2 status represents an indispensable part of the management of breast cancer patients we additionally analyzed the influence of ER and HER2 status on pOX40L expression in our cohort. Interestingly we didn’t observe any correlation of hormone receptor or HER2 status and expression of OX40L on platelets (Supplementary Figures 2D, E). However, taking into account that triple negative and HER2-positive breast cancer patients present the highest levels of tumor infiltrating lymphocytes (TILs) (50), which is prerequisite for the successful use of immunotherapy, pOX40L expression as well as HER2 and hormone receptor status might be useful predictive tools for OX40 agonists. Of note, additional data are needed to further investigate such an approach.
Figure 3 Predictive value of pOX40L in breast cancer relapse. (A) Comparative analysis of pOX40L and the clinical markers HER2, ER, CA 15-3, CEA and LDH. (B) Association of pOX40L expression and different breast cancer subtypes. (C-E) Correlation of pOX40L and conventional tumor marker including CA15-3 (C), CEA (D) and LDH (E). (F–K) Predictive value of pOX40L (F), HER2 (G), ER (H), CA15-3 (I), CEA (J) and LDH (K) expression for disease relapse was analyzed using ROC (Area under the ROC curve) analysis.
Nevertheless, our exploratory study suffers from some limitations. Due to the fact that we used an exploratory study design our breast cancer cohort constitutes an unequal representation of tumor stages, different treatments and different cycles of various treatment regimens. Even if pOX40L expression seems to be independent of tumor stages, lymph node invasion, treatment modalities and number of treatment regimen (Supplementary Figures 1A–C), further consecutive analysis in a balanced, prospective study cohort are needed.
In addition, our exploratory study was not powered to compare the determination of pOX40L with conventional screening methods including mammogram/MRI or determination of CEA or CA15-3. Further prospective data are needed to verify the predictive role of pOX40L in breast cancer. Nevertheless, since high level of pOX40L were associated with a shorter RFS and TTM, determination of pOX40L might be a useful, noninvasive and cost-effective method allowing for longitudinal follow-up analysis. In patients with high levels of pOX40L follow-up care might be intensified and supplemented by specific screening for metastases.
In conclusion, our explorative data not only highlight the prognostic potential of the platelet-expressed immunoregulatory molecule OX40L as a biomarker in breast cancer progression. They further indicate that OX40/OX40L signaling in cancer seems to be complex and might play a controversial role in tumor biology via orchestrating immune activation and tumorigenesis, an approach which requires further functional and clinical validation.
During 2019-2021, 65 breast cancer patients treated at the Department of Obstetrics and Gynecology and the Department of Medical Oncology and Pneumology were included in our prospective study. The study was approved by IRB (ethics committee of the Faculty of Medicine of the Eberhard Karls Universitaet Tuebingen) and of the University Hospital (13/2007V). Written informed consent in accordance with the Helsinki protocol was given in all cases. The patient characteristics in detail are given in Supplementary Table 1.
Paraformaldehyde was from Affymetrix (Santa Clara, CA, USA). Anti-OX40L antibodies were from Ancell Corporation (Bayport, MN, USA). Anti-human TACI, anti-human GITRL antibody and the respective isotype control were from R&D Systems (Minneapolis, MN). CD41a-PeCy5, and CD62P-FITC were from BD Pharmingen, CD3-APC/Fire, CD69-PE and CD25-FITC and CD56-PECy7 were obtained from BioLegend. The goat anti-mouse PE conjugate was from Dako (Glostrup, Denmark). Dead cells were excluded using Fixable Aqua (Invitrogen, Carlsbad, CA, USA) after extracellular staining according to the manufacturer’s instructions. Bicoll Separating Solution was purchased from Biochrom AG (Berlin, Germany).
Platelet aggregation was analyzed using the four-channel light transmission platelet aggregometer APACT 4004 (Elitech, Puteaux, France) according to the manufacturer’s instructions. For platelet activation ex vivo 10 µM of Thrombin Receptor Activator Peptide 6 (TRAP-6) was added to the platelets.
PBMCs were obtained from patients and healthy donors after informed writing consent. Preparation of PBMCs was performed as previously described (19). Peripheral blood platelets were collected in citrate buffer and briefly centrifuged for 20 min at 120×g. For the generation of platelet-rich plasma, platelets were washed with citrate wash buffer (128 mmol/L NaCl, 11 mmol/L glucose, 7.5 mmol/L Na2HPO4, 4.8 mmol/L sodium citrate, 4.3 mmol/L NaH2PO4, 2.4 mmol/L citric acid, and 0.35% bovine serum albumin).
Flow cytometry was performed as described previously (24). The percentage of positive cells was calculated as follows: “% surface expression obtained with specific antibody” − “% surface expression obtained with isotype control”. T cells were selected by positivity for CD3, and NK cells by a CD56+CD3− phenotype. Platelets were selected by CD41a+ and CD62P− (resting) or CD62P+ (activated).
For continuous variables student’s t test, Mann-Whitney U test, one-way ANOVA and Kruskal-Wallis test was used. For multiple comparison Dunn’s multiple comparison test was used. For categorical data we used chi‐squared test or Fisher’s exact test. Correlation of pOX40L expression and CD69/CD25 expression on T and NK cells, platelet size and platelet volume as well as Ki67 level and pOX40L expression was analyzed using simple linear regression analysis. Correlation of mRNA expression was performed using simple linear regression analysis. Recurrence free survival (RFS) were calculated using the Kaplan‐Meier method. Hazard ratios (HRs) were determined using Cox regression analysis. High pOX40L expression was defined as follows: pOX40L high > mean p=OX40L, pOX40L low < mean pOX40L. The predictive value of pOX40L was evaluated by examining the area under the receiver‐operator characteristic (ROC) with a confidence interval of 95%. All statistical tests were considered statistically significant when P was below 0.05. Statistical analysis was performed using GraphPadPrism (v.8.4.0).
The genome dataset presented in this study can be found in online repositories (The Cancer Genome Atlas Database (TCGA), https://www.cbioportal.org). The data that support the findings of this study are available from the corresponding author, SM upon reasonable request.
The study was approved by IRB (ethics committee of the Faculty of Medicine of the Eberhard Karls Universitaet Tuebingen) and of the University Hospital (13/2007V). The patients/participants provided their written informed consent to participate in this study.
CH, SM, LZ, and HS conceived and designed the study. ML, KC, and YZ conducted in vitro experiments. AK and AH conducted patient data and sample collection as well as medical evaluation and analysis. SR, MH, KK, SM, and CH analyzed data and performed statistical analyses. SR, CH, and SM prepared figures and tables; SR, SM, and CH wrote the first draft of the manuscript. HS, LZ, and AH contributed to data interpretation and manuscript edit. All authors critically reviewed, read, and approved the final manuscript.
This work was funded by the Deutsche Forschungsgemeinschaft (DFG, German Research Foundation) under Germany’s Excellence Strategy - EXC 2180 - 39090067 and DFG, project number 374031971–TRR 240/project B05. SM was supported by the Institutional Strategy of the University of Tuebingen (Deutsche Forschungsgemeinschaft, ZUK 63) and the Deutsche Forschungsgemeinschaft, MA 8774/1-1. HS was funded by Deutsche Forschungsgemeinschaft, SA1360/7-3, Wilhelm Sander-Stiftung, 2007.115.3, and Deutsche Krebshilfe 70112914. SR is participant in the BIH Charité Clinician Scientist Program funded by the Charité – Universitaetsmedizin Berlin, and the Berlin Institute of Health at Charité (BIH).
The authors declare that the research was conducted in the absence of any commercial or financial relationships that could be construed as a potential conflict of interest.
All claims expressed in this article are solely those of the authors and do not necessarily represent those of their affiliated organizations, or those of the publisher, the editors and the reviewers. Any product that may be evaluated in this article, or claim that may be made by its manufacturer, is not guaranteed or endorsed by the publisher.
Flow cytometry sample acquisition was performed on shared instruments of the Flow Cytometry Core Facility Tuebingen.
The Supplementary Material for this article can be found online at: https://www.frontiersin.org/articles/10.3389/fonc.2022.917834/full#supplementary-material
Supplementary Figure 1 | Association of pOX40L and breast cancer treatment. (A) Comparative analysis of pOX40L and different breast cancer subtypes. (B) Association of pOX40L and endocrine therapy. (C) Correlation of pOX40L and different numbers of treatment regimen.
Supplementary Figure 2 | pOX40L expression in different breast cancer subtypes Expression of CA15-3 (A), CEA (B) and LDH (C) in different breast cancer subtypes. (D) pOX40L expression in HER2 positive and negative patients. (E) pOX40L expression in ER positive vs. ER negative patients. pos. = positive, neg. = negative.
Supplementary Figure 3 | Gating strategy used to analyze pOX40L expression on platelets pOX40L levels were assessed by flow cytometry. Platelets were defined as CD41a-positive subcellular fragments and specific staining for OX40L level was analyzed in association with the platelet activation marker P-selectin (CD62P). If not indicated otherwise, data reflect pOX40L expression on all CD41+ platelets, i.e. activated (CD62P+) and resting (CD62P-) platelets.
Supplementary Table 1 | Patient characteristics. Other immune checkpoint molecules in parts of this cohort have been published previously (24, 25, 51).
1. McDermott DF, Atkins MB. PD-1 as a Potential Target in Cancer Therapy. Cancer Med (2013) 2(5):662–73. doi: 10.1002/cam4.106
2. Leach DR, Krummel MF, Allison JP. Enhancement of Antitumor Immunity by CTLA-4 Blockade. Science (1996) 271(5256):1734–6. doi: 10.1126/science.271.5256.1734
3. Hiam-Galvez KJ, Allen BM, Spitzer MH. Systemic Immunity in Cancer. Nat Rev Cancer (2021) 21(6):345–59. doi: 10.1038/s41568-021-00347-z
4. Aspeslagh S, Postel-Vinay S, Rusakiewicz S, Soria J-C, Zitvogel, Aurélien Marabelle, et al. Rationale for Anti-OX40 Cancer Immunotherapy. Eur J Cancer (2016) 52:50–66. doi: 10.1016/j.ejca.2015.08.021
5. Qin S, Xu L, Yi M, Yu S, Wu K, Luo S. Novel Immune Checkpoint Targets: Moving Beyond PD-1 and CTLA-4. Mol Cancer (2019) 18(1):155. doi: 10.1186/s12943-019-1091-2
6. Buchan SL, Rogel A, Al-Shamkhani A. The Immunobiology of CD27 and OX40 and Their Potential as Targets for Cancer Immunotherapy. Blood (2018) 131(1):39–48. doi: 10.1182/blood-2017-07-741025
7. Weiss JM, Wiltout RH. Multifaceted Antitumor Responses to Activating Anti-CD40 Antibody Therapy Combined With Immunomodulatory or Targeted Agents. Oncoimmunology (2014) 3(8):e954483. doi: 10.4161/21624011.2014.954483
8. Ansell SM, Flinn I, Taylor MH, Sikic BI, Brody J, Nemunaitis J, et al. Safety and Activity of Varlilumab, a Novel and First-in-Class Agonist Anti-CD27 Antibody, for Hematologic Malignancies. Blood Adv (2020) 4(9):1917–26. doi: 10.1182/bloodadvances.2019001079
9. Marin-Acevedo JA, Dholaria B, Soyano AE, Knutson KL, Chumsri S, Lou Y. Next Generation of Immune Checkpoint Therapy in Cancer: New Developments and Challenges. J Hematol Oncol (2018) 11(1):39. doi: 10.1186/s13045-018-0582-8
10. Ma Y, Li J, Wang H, Chiu Y, Kingsley CV, Fry D, et al. Combination of PD-1 Inhibitor and OX40 Agonist Induces Tumor Rejection and Immune Memory in Mouse Models of Pancreatic Cancer. Gastroenterology (2020) 159(1):306–319.e12. doi: 10.1053/j.gastro.2020.03.018
11. Kuang Z, Pu P, Wu M, Wu Z, Wang L, Li Y, et al. A Novel Bispecific Antibody With PD-L1-Assisted OX40 Activation for Cancer Treatment. Mol Cancer Ther (2020) 19(12):2564–74. doi: 10.1158/1535-7163.MCT-20-0226
12. Fromm G, de Silva S, Johannes K, Patel A, Hornblower JC, Schreiber TH. Agonist Redirected Checkpoint, PD1-Fc-OX40L, for Cancer Immunotherapy. J Immunother Cancer (2018) 6(1):149. doi: 10.1186/s40425-018-0454-3
13. Croft M. Control of Immunity by the TNFR-Related Molecule OX40 (Cd134). Annu Rev Immunol (2010) 28:57–78. doi: 10.1146/annurev-immunol-030409-101243
14. Diab A, Hamid O, Thompson JA, Ros W, Eskens FALM, Doi T, et al. A Phase I, Open-Label, Dose-Escalation Study of the OX40 Agonist Ivuxolimab in Patients With Locally Advanced or Metastatic Cancers. Clin Cancer Res (2022) 28(1):71–83. doi: 10.1158/1078-0432.CCR-21-0845
15. Gutierrez M, Moreno V, Heinhuis KM, Olszanski AJ, Spreafico A, Ong M, et al. OX40 Agonist BMS-986178 Alone or in Combination With Nivolumab and/or Ipilimumab in Patients With Advanced Solid Tumors. Clin Cancer Res (2021) 27(2):460–72. doi: 10.1158/1078-0432.CCR-20-1830
16. Clar KL, Hinterleitner C, Schneider P, Salih HR, Maurer S. Inhibition of NK Reactivity Against Solid Tumors by Platelet-Derived RANKL. Cancers (Basel) (2019) 11(3):277. doi: 10.3390/cancers11030277
17. Liu DM, Yan JC, Wang CP, Chen GH, Ding S, Liu PJ, et al. The Clinical Implications of Increased OX40 Ligand Expression in Patients With Acute Coronary Syndrome. Clin Chim Acta (2008) 397(1-2):22–6. doi: 10.1016/j.cca.2008.07.003
18. Gay LJ, Felding-Habermann B. Contribution of Platelets to Tumour Metastasis. Nat Rev Cancer (2011) 11(2):123–34. doi: 10.1038/nrc3004
19. Placke T, Örgel M, Schaller M, Jung G, Rammensee HG, et al. Platelet-Derived MHC Class I Confers a Pseudonormal Phenotype to Cancer Cells That Subverts the Antitumor Reactivity of Natural Killer Immune Cells. Cancer Res (2012) 72(2):440–8. doi: 10.1158/0008-5472.CAN-11-1872
20. Maurer S, Kropp KN, Klein G, Steinle A, Haen SP, Walz JS, et al. Platelet-Mediated Shedding of NKG2D Ligands Impairs NK Cell Immune-Surveillance of Tumor Cells. Oncoimmunology (2018) 7(2):e1364827. doi: 10.1080/2162402X.2017.1364827
21. Amirkhosravi A, Amaya M, Desai H, Francis JL. Platelet-CD40 Ligand Interaction With Melanoma Cell and Monocyte CD40 Enhances Cellular Procoagulant Activity. Blood Coagul Fibrinolysis (2002) 13(6):505–12. doi: 10.1097/00001721-200209000-00005
22. Lutz MS, Klimovich B, Maurer S, Heitmann JS, Märklin M, Zekri L, et al. Platelets Subvert Antitumor Efficacy of T Cell-Recruiting Bispecific Antibodies. J Immunother Cancer (2022) 10(2):e003655. doi: 10.1136/jitc-2021-003655
23. Hinterleitner C, Strähle J, Malenke E, Hinterleitner M, Henning M, Seehawer M, et al. Platelet PD-L1 Reflects Collective Intratumoral PD-L1 Expression and Predicts Immunotherapy Response in Non-Small Cell Lung Cancer. Nat Commun (2021) 12(1):7005. doi: 10.1038/s41467-021-27303-7
24. Zhou Y, Heitmann JS, Clar KL, Kropp KN, Hinterleitner M, Engler T, et al. Platelet-Expressed Immune Checkpoint Regulator GITRL in Breast Cancer. Cancer Immunol Immunother (2021) 70(9):2483–96. doi: 10.1007/s00262-021-02866-y
25. Hinterleitner C, Zhou Y, Tandler C, Heitmann JS, Kropp KN, Hinterleitner M, et al. Platelet-Expressed TNFRSF13B (TACI) Predicts Breast Cancer Progression. Front Oncol (2021) 11:642170. doi: 10.3389/fonc.2021.642170
26. Riccardi C, Ronchetti S, Nocentini G. Glucocorticoid-Induced TNFR-Related Gene (GITR) as a Therapeutic Target for Immunotherapy. Expert Opin Ther Targets (2018) 22(9):783–97. doi: 10.1080/14728222.2018.1512588
27. Baltz KM, Krusch M, Baessler T, Schmiedel BJ, Bringmann A, Brossart P, Salih HR. Neutralization of Tumor-Derived Soluble Glucocorticoid-Induced TNFR-Related Protein Ligand Increases NK Cell Anti-Tumor Reactivity. Blood (2008) 112(9):3735–43. doi: 10.1182/blood-2008-03-143016
28. Lee WH, Seo D, Lim SG, Suk K. Reverse Signaling of Tumor Necrosis Factor Superfamily Proteins in Macrophages and Microglia: Superfamily Portrait in the Neuroimmune Interface. Front Immunol (2019) 10:262. doi: 10.3389/fimmu.2019.00262
29. Lim SG, Kim JK, Suk K, Lee WH. Crosstalk Between Signals Initiated From TLR4 and Cell Surface BAFF Results in Synergistic Induction of Proinflammatory Mediators in THP-1 Cells. Sci Rep (2017) 7:45826. doi: 10.1038/srep45826
30. Kampa M, Notas G, Stathopoulos EN, Tsapis A, Castanas E. The TNFSF Members APRIL and BAFF and Their Receptors TACI, BCMA, and BAFFR in Oncology, With a Special Focus in Breast Cancer. Front Oncol (2020) 10:827. doi: 10.3389/fonc.2020.00827
31. Eslami M, Schneider P. Function, Occurrence and Inhibition of Different Forms of BAFF. Curr Opin Immunol (2021) 71:75–80. doi: 10.1016/j.coi.2021.06.009
32. Cognasse F, Duchez AC, Audoux E, Ebermeyer T, Arthaud CA, Prier A, et al. Platelets as Key Factors in Inflammation: Focus on CD40L/Cd40. Front Immunol (2022) 13:825892. doi: 10.3389/fimmu.2022.825892
33. Plantureux L, Mège D, Crescence L, Carminita E, Robert S, Cointe S, et al. The Interaction of Platelets With Colorectal Cancer Cells Inhibits Tumor Growth But Promotes Metastasis. Cancer Res (2020) 80(2):291–303. doi: 10.1158/0008-5472.CAN-19-1181
34. Panes O, Matus V, Sáez CG, Quiroga T, Pereira J, Mezzano D. Human Platelets Synthesize and Express Functional Tissue Factor. Blood (2007) 109(12):5242–50. doi: 10.1182/blood-2006-06-030619
35. Ni XY, Sui HX, Liu Y, Ke SZ, Wang YN, Gao FG. TGF-Beta of Lung Cancer Microenvironment Upregulates B7H1 and GITRL Expression in Dendritic Cells and is Associated With Regulatory T Cell Generation. Oncol Rep (2012) 28(2):615–21. doi: 10.3892/or.2012.1822
36. Webb GJ, Hirschfield GM, Lane PJ. OX40, OX40L and Autoimmunity: A Comprehensive Review. Clin Rev Allergy Immunol (2016) 50(3):312–32. doi: 10.1007/s12016-015-8498-3
37. Krause P, Bruckner M, Uermösi C, Singer E, Groettrup M, Legler DF. Prostaglandin E(2) Enhances T-Cell Proliferation by Inducing the Costimulatory Molecules OX40L, CD70, and 4-1BBL on Dendritic Cells. Blood (2009) 113(11):2451–60. doi: 10.1182/blood-2008-05-157123
38. Maxwell JR, Yadav R, Rossi RJ, Ruby CE, Weinberg AD, Aguila HL, et al. IL-18 Bridges Innate and Adaptive Immunity Through IFN-Gamma and the CD134 Pathway. J Immunol (2006) 177(1):234–45. doi: 10.4049/jimmunol.177.1.234
39. Ito T, Wang YH, Duramad O, Hori T, Delespesse GJ, Watanabe N, et al. TSLP-Activated Dendritic Cells Induce an Inflammatory T Helper Type 2 Cell Response Through OX40 Ligand. J Exp Med (2005) 202(9):1213–23. doi: 10.1084/jem.20051135
40. Wesolowski R, Stiff A, Quiroga D, McQuinn C, Li Z, Nitta H, et al. Exploratory Analysis of Immune Checkpoint Receptor Expression by Circulating T Cells and Tumor Specimens in Patients Receiving Neo-Adjuvant Chemotherapy for Operable Breast Cancer. BMC Cancer (2020) 20(1):445. doi: 10.1186/s12885-020-06949-4
41. Wang L, Simons DL, Lu X, Tu TY, Solomon S, Wang R, et al. Connecting Blood and Intratumoral Treg Cell Activity in Predicting Future Relapse in Breast Cancer. Nat Immunol (2019) 20(9):1220–30. doi: 10.1038/s41590-019-0429-7
42. Sun S, Fei X, Mao Y, Wang X, Garfield DH, Huang O, et al. PD-1(+) Immune Cell Infiltration Inversely Correlates With Survival of Operable Breast Cancer Patients. Cancer Immunol Immunother (2014) 63(4):395–406. doi: 10.1007/s00262-014-1519-x
43. Korniluk A, Koper-Lenkiewicz OM, Kamińska J, Kemona H, Dymicka-Piekarska V. Mean Platelet Volume (MPV): New Perspectives for an Old Marker in the Course and Prognosis of Inflammatory Conditions. Mediators Inflamm (2019) 2019:9213074. doi: 10.1155/2019/9213074
44. Kraytman M. Platelet Size in Thrombocytopenias and Thrombocytosis of Various Origin. Blood (1973) 41(4):587–98. doi: 10.1182/blood.V41.4.587.587
45. Minn AJ, Kang Y, Serganova I, Gupta GP, Giri DD, Doubrovin M, et al. Distinct Organ-Specific Metastatic Potential of Individual Breast Cancer Cells and Primary Tumors. J Clin Invest (2005) 115(1):44–55. doi: 10.1172/JCI22320
46. Xie F, Wang Q, Chen Y, Gu Y, Mao H, Zeng W, et al. Costimulatory Molecule OX40/OX40L Expression in Ductal Carcinoma in Situ and Invasive Ductal Carcinoma of Breast: An Immunohistochemistry-Based Pilot Study. Pathol Res Pract (2010) 206(11):735–9. doi: 10.1016/j.prp.2010.05.016
47. Ménard S, Fortis S, Castiglioni F, Agresti R, Balsari A. HER2 as a Prognostic Factor in Breast Cancer. Oncology (2001) 61 Suppl 2:67–72. doi: 10.1159/000055404
48. Liu D, Wang D, Wu C, Zhang L, Mei Q, Hu G, et al. Prognostic Significance of Serum Lactate Dehydrogenase in Patients With Breast Cancer: A Meta-Analysis. Cancer Manag Res (2019) 11:3611–9. doi: 10.2147/CMAR.S199260
49. Shao Y, Sun X, He Y, Liu C, Liu H. Elevated Levels of Serum Tumor Markers CEA and CA15-3 Are Prognostic Parameters for Different Molecular Subtypes of Breast Cancer. PloS One (2015) 10(7):e0133830. doi: 10.1371/journal.pone.0133830
50. Loi S, Sirtaine N, Piette F, Salgado R, Viale G, Van Eenoo F, et al. Prognostic and Predictive Value of Tumor-Infiltrating Lymphocytes in a Phase III Randomized Adjuvant Breast Cancer Trial in Node-Positive Breast Cancer Comparing the Addition of Docetaxel to Doxorubicin With Doxorubicin-Based Chemotherapy: BIG 02-98. J Clin Oncol (2013) 31(7):860–7. doi: 10.1200/JCO.2011.41.0902
Keywords: breast cancer, biomarker, immunotherapy, platelets, prognosis, OX40L
Citation: Rittig SM, Lutz MS, Clar KL, Zhou Y, Kropp KN, Koch A, Hartkopf AD, Hinterleitner M, Zender L, Salih HR, Maurer S and Hinterleitner C (2022) Controversial Role of the Immune Checkpoint OX40L Expression on Platelets in Breast Cancer Progression. Front. Oncol. 12:917834. doi: 10.3389/fonc.2022.917834
Received: 11 April 2022; Accepted: 08 June 2022;
Published: 08 July 2022.
Edited by:
Jinhui Liu, Nanjing Medical University, ChinaReviewed by:
Dong Li, University of Kentucky, United StatesCopyright © 2022 Rittig, Lutz, Clar, Zhou, Kropp, Koch, Hartkopf, Hinterleitner, Zender, Salih, Maurer and Hinterleitner. This is an open-access article distributed under the terms of the Creative Commons Attribution License (CC BY). The use, distribution or reproduction in other forums is permitted, provided the original author(s) and the copyright owner(s) are credited and that the original publication in this journal is cited, in accordance with accepted academic practice. No use, distribution or reproduction is permitted which does not comply with these terms.
*Correspondence: Stefanie Maurer, c3RlZmFuaWUubWF1cmVyQG1zc20uZWR1
†ORCID: Kim L. Clar, orcid.org/0000-0003-1454-9830
Helmut R. Salih, orcid.org/0000-0002-6719-1847
Disclaimer: All claims expressed in this article are solely those of the authors and do not necessarily represent those of their affiliated organizations, or those of the publisher, the editors and the reviewers. Any product that may be evaluated in this article or claim that may be made by its manufacturer is not guaranteed or endorsed by the publisher.
Research integrity at Frontiers
Learn more about the work of our research integrity team to safeguard the quality of each article we publish.