- 1Department of Hematology, The First Affiliated Hospital of Guangdong Pharmaceutical University, Guangzhou, China
- 2Department of Hematology, Tongji Hospital, TongJi Medical College, Huazhong University of Science and Technology, Wuhan, China
With the success of chimeric antigen receptor-modified (CAR) T-cell therapy for relapsed/refractory (r/r) B-cell malignancies, severe complications after CAR T-cell infusion have emerged as nonnegligible prognosis-related factors. However, the prognosis of patients with CAR T-cell-related hyperferritinaemia (HFA) is unclear. We report the efficacy and safety of CAR T-cell therapy in 16 r/r B-cell malignancy patients with CAR T-cell-related HFA. The rates of serum ferritin levels above 10,000 ng/ml during CAR T-cell therapy were 6.2% and 14.3% in B-cell non-Hodgkin’s lymphoma (B-NHL) and acute B lymphocyte leukemia (B-ALL), respectively. These patients were characterized by an extremely high tumor burden and a high rate of extranodal involvement. In lymphoma, the complete remission (CR) rate was 37.5% (3/8), which was lower than that in the control group with the lowest value of ferritin (CR was 87.5% (7/8), P=0.0406), and it could also be seen that the OS of the control group (1-year OS rate 100%) had a better trend than HFA group (1-year OS rate 50%). In the B-ALL patients, the OS of the control group (1-year OS rate 100%) was higher than HFA group (1-year OS rate 45%, P=0.0189), although there was no significant difference in CR rate. High-grade CRS (≥3) occurred in 56.25% of the patients, and the mortality rate was 56.25%, which was significantly higher than control group (12.5% and 12.5%, P=0.009). The peak serum ferritin level in the patients who died of CRS was significantly higher than others (P=0.0168). Regardless of whether the CAR T-related MAS diagnostic criteria were met, there was no significant difference in ORR and OS in HFA group, however patients with MAS showed a higher rate of high-grade CRS. Interestingly, in our study, glucocorticoid intervention in HFA group showed little impact on expansion of CAR-T cells, whether compared with control group or compared within HFA group by dividing patients into high and low dosage subgroups based on the median dose of glucocorticoid. High mortality was observed in patients with CAR T-cell-related HFA. Early glucocorticoid intervention might be worth trying to improve the safety of CAR T therapy in these patients.
Introduction
Immunotherapy using genetically engineered T-cells to express a chimeric antigen receptor (CAR) is rapidly emerging as a promising new treatment for hematological malignancies. Treatment with chimeric antigen receptor-engineered (CAR) T-cells has demonstrated higher complete remission (CR) rates and improved survival compared with chemotherapy for relapsed/refractory B-cell malignancies (1–3). However, CAR T-cell therapy is associated with unique acute toxicities, which can be severe or even fatal, such as cytokine release syndrome (CRS) and immune effector cell-associated neurotoxicity syndrome (ICANS), which occur as a result of T-cell overactivation (1, 4).
During CAR T-cell therapy, macrophage-activation syndrome (MAS), characterized by severe immune activation, lymph histiocytic infiltration, and immune response-mediated multiorgan failure, can occur (5). A total of 32.8% of ALL patients who received CD22-targeted CAR T-cell therapy experienced hemophagocytic lymphohistiocytosis (HLH)/MAS (6). However, in another multicenter retrospective study, the rate of MAS following CAR T-cell therapy was only 3.48% (7/201) (7). Fulminant and refractory HLH/MAS was observed in ~1% of all patients treated with CAR T-cell therapy, and special therapy, anti-IL-6 therapy, and corticosteroid treatment are necessary in addition to supportive care (4). If not treated promptly, refractory HLH/MAS is associated with high mortality (8). However, the diagnosis of MAS is difficult in the context of CRS, and many diagnostic criteria of MAS are not specific, such as fever and pancytopenia.
Monitoring ferritin levels can be helpful in the diagnosis of CAR T-cell-related MAS, and it is an essential condition of diagnosis that patients must have peak ferritin levels of >10,000 ng/ml during the CRS phase (4, 9). CAR T-cell-activated macrophages that contribute to highly elevated ferritin also function as antitumor agents (10, 11). Furthermore, higher serum ferritin levels may be a possible indication of a prolonged ICU hospitalization of patients treated with axi-cel (CAR19) and may be significantly associated with a higher grade of neurotoxicity (12, 13). However, the prognosis of patients with CAR T-cell-related HFA is unclear.
At our center, sequential infusion of CAR19/22 T cells was efficacious and reduced the rate of antigen-escape relapse in B-cell malignancies (14). However, the clinical features and outcomes of patients with CAR T-cell-related HFA (above 10,000 ng/ml) remain unclear. This study focused on the incidence, clinical characteristics, and therapeutic strategy of CAR T-cell-related ferritin levels above 10,000 ng/ml and these patient’s response to CAR T therapy.
Materials and methods
Patient characteristics
From January 17, 2018, to April 30, 2020, 129 patients with refractory/relapsed (r/r) B-cell non-Hodgkin’s lymphoma (B-NHL) and 56 patients with r/r acute B lymphoblastic leukemia (B-ALL) were enrolled in a clinical trial of sequential infusion of CAR19/22 T-cell therapy (Registry number ChiCTR-OPN-16008526 at http://www.chictr.org.cn). Among them, 8 lymphoma patients (8/129, 6.2%) and 8 leukemia patients (8/56, 14.3%), because of CRS, had high levels of ferritin (greater than 10,000 ng/ml [normal range, 30-400 ng/ml]). In this study, ferritin levels greater than 10,000 ng/ml were defined as HFA. Meanwhile, 16 cases with the lowest peak values of ferritin were grouped as controls, including 8 B-NHL and 8 B-ALL patients. This study was approved by Tongji Hospital, Tongji Medical College, Huazhong University of Science and Technology, in compliance with the Declaration of Helsinki. For details of this trial, refer to our previous study (14).
All patients were diagnosed based on the World Health Organization classification for hematological malignancies. Tumor burden was assessed by bone marrow examination, B ultrasound, positron emission tomography/computed tomography (PET/CT) or CT. Bulky disease was defined as a tumor mass 7.5 cm in maximal diameter (15). Ann Arbor stage and the International Prognostic Index (IPI) were evaluated for lymphoma patients. Next-generation sequencing (NGS), karyotype analysis, fusion gene detection, and fluorescence in situ hybridization (FISH) were performed for molecular genetic evaluation. In B-ALL, a complex karyotype was defined as ≥5 chromosome abnormalities (16). The date of the last follow-up was June 20, 2021.
Generation of CAR T-cells and CAR T regimen
T cells were obtained from the patients. The lentiviral vector encoded anti-CD19 or anti-CD22 single-chain variable fragments, CD28, 4-1BB costimulatory domains and CD3-z signaling domains. Transfection, expansion and examination for effectiveness in vitro were implemented before infusion. The details were described in our previous study (14). An FC regimen (fludarabine 25 mg/m2 and cyclophosphamide 20 mg/kg daily for 3 days) was administered for lymphodepletion before CAR T-cell therapy. Anti-CD22 CAR T-cells were intravenously infused, followed by an infusion of anti-CD19 CAR T-cells the next day.
Response assessment and monitoring
The treatment response was assessed after infusion according to the Lugano response criteria (17). The severity of cytokine release syndrome (CRS) and CAR T-cell-related encephalopathy syndrome (ICANS) was graded based on the CAR T-cell-therapy-associated TOXicity (CARTOX) Working Group Consensus (4) and the Common Terminology Criteria for Adverse Events (CTCAE) version 5.0 (18). The diagnostic criteria of CAR T-cell-related MAS are based on the recommendations of the CARTOX Working Group (4) and meet three of the following conditions: 1) The peak time of ferritin occurred during the CRS stage. 2) There was no obvious infection when a fever was present. 3) There was no evidence of primary disease progression during the peak of ferritin.
Detection of CAR copy number in the peripheral blood
CAR gene copies were detected by droplet digital polymerase chain reaction (ddPCR) (19).
Statistical analysis
Statistical analysis was performed with SPSS 18.0 software (IBM, Armonk, NY, USA). Continuous data are described as medians with ranges, while categorical data are described as frequencies (percentages). The independent t-test was used to compare continuous data between two groups. In contrast, the chi-square test or Fisher’s exact test was used to compare categorical data. GraphPad Prism 8.0 software (GraphPad Software Inc., San Diego, CA, USA) was used to plot the diagrams. Survival was analyzed with the log-rank test. P < 0.05 was considered statistically significant.
Results
Clinical characteristics
The clinical baseline characteristics of the B-NHL and B-ALL cohorts (each cohort included 8 patients with HFA and 8 control cases) are shown in Tables 1, 2.
The median age was 50 years (34-63 years) and 41.5 years (27-66 years) for the HFA and control cohorts of B-NHL patients, respectively, while the sex ratio (male/female) was 1.00 and 3.00. Among the 8 B-NHL patients with HFA, 3 had diffuse large B-cell lymphoma not otherwise specified (DLBCL NOS), 1 had DLBCL transformed from follicular lymphoma (FL), 2 had high-grade B-NHL (HGBL), 1 had FL, and 1 had marginal zone lymphoma (MZL). For the B-NHL control group, there were 5 DLBCL NOS, 1 DLBCL transformed from FL, 1 DLBCL transformed from MZL and 1 HGBL. All HFA patients (8/8, 100%) and 6 control cases (6/8, 75%) were in the advanced stage (Ann Arbor stage IV). All patients in the HFA group had an IPI score of 3 or more, while in the control group, half of the patients had an IPI score of 1-2. Five out of 8 HFA patients (62.5%) had high-risk molecular abnormalities, including double-/triple-hit rearrangements (2/8, 25.0%) and TP53 deletion/mutation (3/8, 37.5%). In the control group, the number was 5 (62.5%) with 1 double-/triple-hit rearrangement (12.5%) and 4 TP53 deletions/mutations (50.0%). Bulky disease was seen in 4 HFA patients (4/8, 50.0%) and in 1 case (12.5%) in the control group. Extranodal lesions, including bone marrow (3/8, 37.5%), central nervous system (CNS) (2/8, 25.0%), liver (2/8, 25.0%), spleen (1/8, 12.5%), lung (1/8, 12.5%) and gastrointestinal tract (2/8, 25.0%), were observed in most HFA patients (7/8, 87.5%), and 5 of the patients had two or more extranodal sites. In the control group, 3 out of 4 extranodal involved cases had 2 sites of extranodal lesions. All 16 B-NHL patients had received a median of 3 lines of prior treatment, and 3 (3/8, 37.5%) and 1 (1/8, 12.5%) in the HFA and control group underwent fourth-line treatment, respectively.
Among the 16 B-ALL patients, the median age was 34 years (22-50 years) and 34 years (15-58 years) for the HFA and control groups, with sex ratios of 0.60 and 0.125, respectively. All HFA B-ALL patients had high-risk molecular abnormalities, including BCR-ABL (3/8, 37.5%; one of the patients had T315I mutation), ph-like (Ikaros 6) (1/8, 12.5%), E2A/PBX1 fusion gene (1/8, 12.5%) and complex karyotypes (4/8, 50.0%). In the control group, 5 patients (62.5%) had high-risk molecular abnormalities with all 5 BCR-ABL (62.5%), and two of them also had T315I mutations and complex karyotypes. The number of bone marrow blasts in the 8 HFA patients and 8 control cases was 64.0% (6.0% to 99.5%) and 17.5% (0% to 93.9%; the patient with 0% bone marrow blast had an orbital mass and CNS involvement), respectively. Four of 8 HFA patients (50.0%) and 1 of 8 control cases (12.5%) developed CNS infiltration. Patients in both groups had a median prior therapy of 2 lines (range, 2 to 5 lines). Two HFA patients (2/8, 25.0%) and 1 control case (1/8, 12.5%) received at least third-line treatments. One patient in the HFA group and control group previously received allogeneic hematopoietic stem-cell transplantation (allo-HSCT).
The small sample size of this study may be a possible limitation of detecting significant differences between the baseline characteristics of the two groups. However, a tendency could still be observed that compared with the control group, B-NHL patients with HFA had a higher proportion of cases with 3-4 IPI scores (P=0.0769), bulky disease (P=0.2821) and extranodal lesions (P=0.2821), while B-ALL patients with HFA had higher bone marrow blasts (P=0.1723) and more CNS-involved cases (P=0.2821).
Outcome
Among the 8 B-NHL patients with HFA, the objective response rate (ORR) was 62.5%, including 3 with CR (3/8, 37.5%), 2 with partial remission (PR) (2/8, 25%), 1 with stable disease (SD) (1/8, 12.5%) and 2 with progressive disease (PD) (2/8, 25%). In contrast, among the 8 B-NHL patients without HFA, the ORR was 100%, including 7 with CR (7/8, 87.5%) and 1 with partial remission (PR) (1/8, 12.5%) (Figure 1A). The CR rate of patients with HFA was significantly lower than that of patients in the control group (P=0.0406). Among the 8 B-NHL patients with HFA, 3 CR patients achieved a sustained remission. One PR patient received HSCT therapy followed by CAR T therapy and achieved a CR, and the other PR patient’s disease progressed again. In contrast, most patients in the control group (7/8, 87.5%) were still alive, and only one patient died of disease progression (Supplementary Figure 1A).
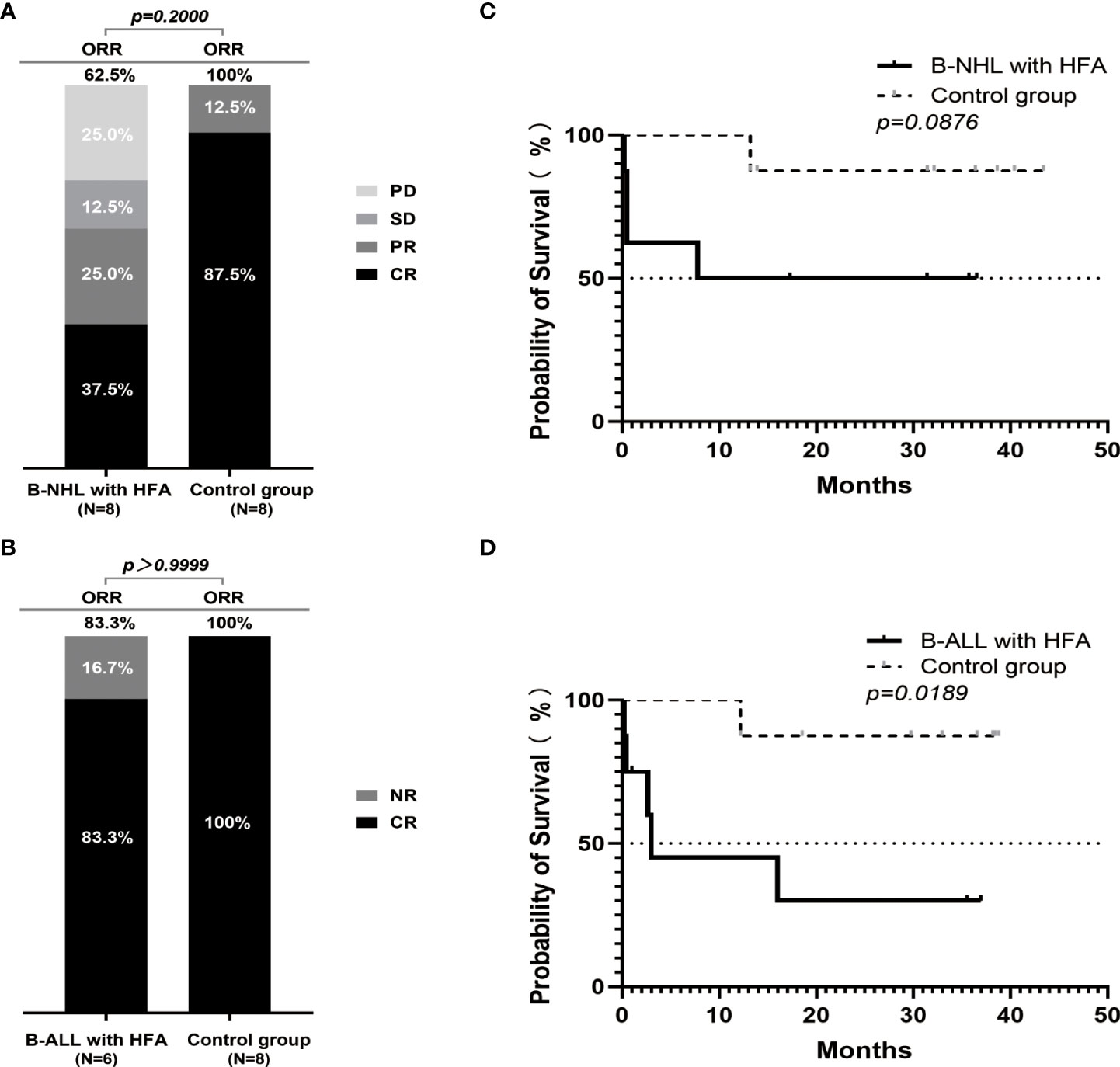
Figure 1 Outcomes of r/r B-NHL and r/r B-ALL cases according to hyperferritinaemia status after the CAR19/22 T-cell therapy. The percentage of patients with CR, PR, SD, PD or CR, NR as the best response in 16 patients with r/r B-NHL (8 in HFA group and 8 in control group) (A) and 14 evaluable patients with r/r B-ALL (6 in HFA group and 8 in control group) (B). Overall survival in the B-NHL (C) and B-ALL (D) cohorts on the basis of hyperferritinaemia status (each cohort included 8 patients with HFA and 8 control cases).
Among the 8 B-ALL patients with HFA, 2 patients died of severe CRS after CAR T-cell infusion before evaluation, 5 of 6 evaluable patients achieved a CR (5/6 83.3%), and only 1 patient had no response. In the control group, all of the patients achieved a CR (Figure 1B). Among the 5 B-ALL patients with HFA who achieved a CR, one was lost to follow-up in the second month after CAR T-cell infusion, and the other 4 underwent three- to thirty-seven-months of follow-up. Three of them received allo-HSCT, one achieved a sustained CR, one relapsed and the last one died of severe infection during transplantation. Conversely, in the control group, only one patient died of severe infection after transplantation, and the others were all alive (Supplementary Figure 1B).
In the B-ALL patients, the OS of the patients without HFA was significantly higher than that of the patients with HFA (P=0.0189). In patients with B-NHL, although there was no significant difference in OS between the patients with and without HFA (P=0.0876), it could also be seen that the OS of the patients without HFA had a better trend than that of the patients with HFA (Figures 1C, D). Meanwhile, B-NHL patients with HFA and bulky disease had a significantly worse OS than those without bulky disease (P=0.0069) (Supplementary Figure 1C). There was no difference in OS between the B-NHL patients with or without high-risk molecular abnormalities (P=0.0511) (Supplementary Figure 1D). However, the proportion of patients with high-risk molecular abnormalities among those with bulky disease was higher than in those with non-bulky disease (P=0.0240) (Supplementary Figure 1E).
Safety
All 16 HFA cases developed CRS within 14 days after CAR T-cell infusion; among them, 7 cases (7/16, 43.8%) were grade 1~2, 5 cases (5/16, 31.3%) were grade 3~4 and 4 cases (4/16, 25.0%) were grade 5. Two patients with lymphoma developed ICANS of grade 3~4. Compared with the control group, patients with HFA had a significantly higher high-grade CRS (≥3) rate in B-NHL (P=0.0256) (Figure 2A). However, no significant difference was found for B-ALL patients or in the distribution of ICANS grades between patients with and without HFA for both lymphoma and leukemia (Figures 2B-D). Nine of 16 patients with HFA (56.25%) died (4 died of CAR T-related MAS, 4 died of tumor progression and 1 died of an infection after allo-HSCT) (Figure 2E). Only two of 16 patients without HFA (12.5%) died (1 died of tumor progression, and 1 died of an infection after allo-HSCT) (Figure 2F).
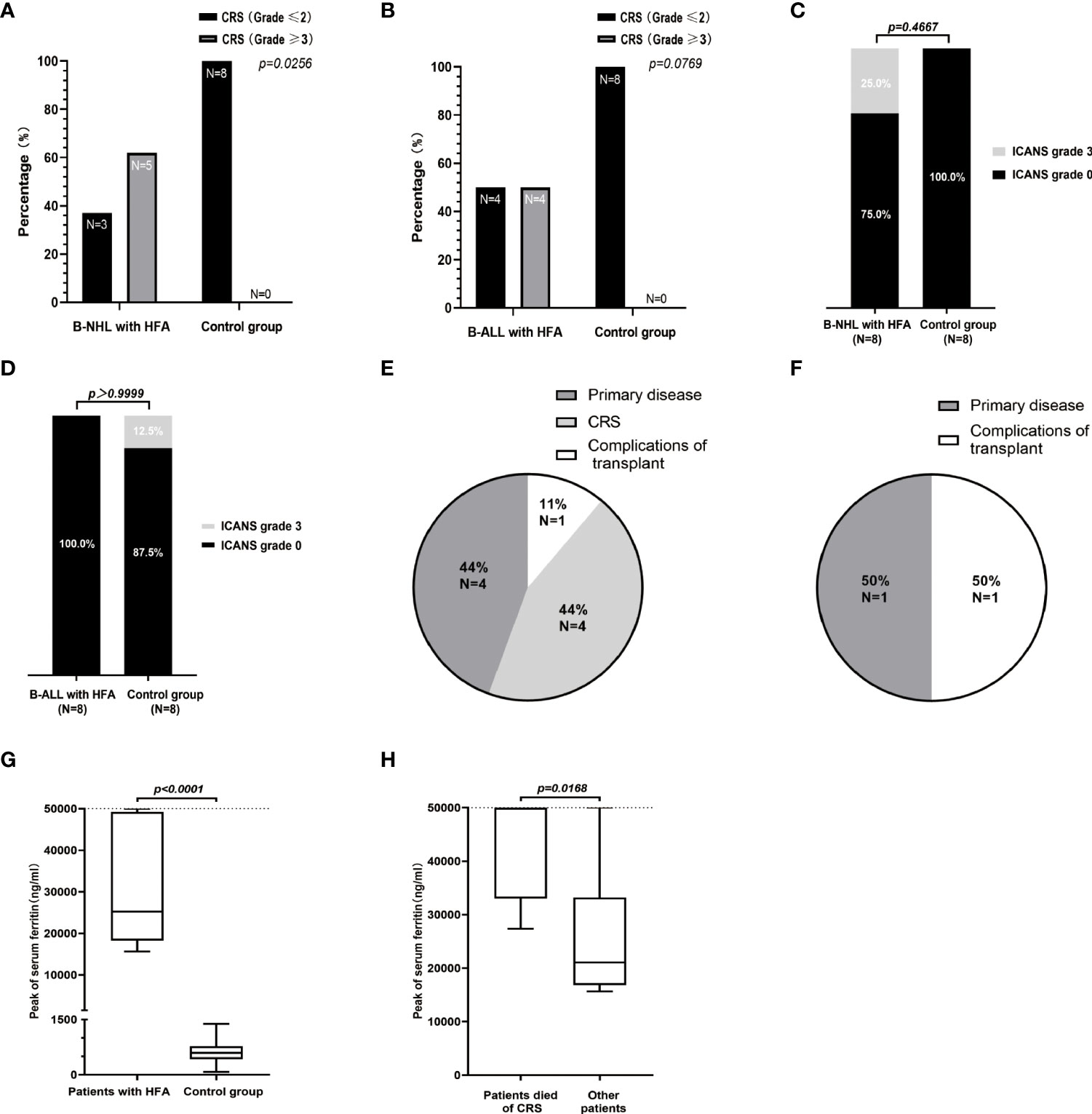
Figure 2 Safety assessments of the 32 patients in B-NHL and B-ALL cohorts. The percentage of patients with high-grade (≥3) and low-grade (≤2) CRS in the B-NHL (A) and B-ALL (B) cohorts on the basis of hyperferritinaemia status. (C, D) ICANS developed in 3 patients (including 2 B-NHL patients in HFA group and 1 B-ALL patient in control group) and all were of grade 3. Causes of death for 9 cases of HFA group (E) and 2 cases of control group (F). (G) The medians of the peak values of serum ferritin in HFA group (n=16) and control group (n=16) were 25257 (range, 15650-50000) ng/ml and 593.1 (range, 77.6-1384) ng/ml, respectively. (H) In the 16 HFA patients, the medians of the peak values of serum ferritin in patients who died of CRS (n=4) and other patients (n=12) were 50000 (range, 27370-50000) ng/ml and 21049.5 (range, 15650-50000) ng/ml, respectively. The horizontal lines in each box represent the median values, and the lower and upper boundaries of each box represent the 25th and the 75th percentiles, respectively, and the whiskers represent the minimum and maximum range. The detection upper limit of serum ferritin was 50000 ng/ml.
The levels of serum ferritin in both HFA group and control group were significantly increased during CRS (Supplementary Figures 2A, B). The levels of serum ferritin and IL-6 in the 16 patients with HFA peaked at a median of 8 days (range 5 to 13 days) and 7 days (range, 3-15 days) after infusion, with median values of 25256.5 ng/ml (normal value 30-400 ng/ml) and 2158.5 pg/ml (normal value < 7 pg/ml), respectively (Supplementary Figures 2C, D). The peak serum ferritin levels in patients with HFA were significantly higher than those in the patients without HFA (P<0.0001), furthermore, among the 16 patients with HFA, the peak serum ferritin levels in the patients who died of CRS were significantly higher than those in the other patients (P=0.0168)(Figures 2G, H).
The MAS-related clinical characteristics of the 16 patients with HFA are shown in Table 3. Among them, 6 lymphoma patients (6/8, 75.0%) and 3 leukemia patients (3/8, 37.5%) met the MAS diagnostic criteria. Thus, the rates of CAR T-cell-related MAS in our center from January 17, 2018, to April 30, 2020, were 4.7% (6/129) in patients with lymphoma and 5.4% (3/56) in patients with leukemia. Seven lymphoma cases (7/8, 87.5%) and 4 leukemia cases (4/8, 50.0%) had ≥ 3-grade liver toxicities. Six lymphoma cases (6/8, 75.0%) and 3 leukemia cases (3/8, 37.5%) had ≥ 3-grade pulmonary edema. In the control group, lymphoma patients, but not leukemia patients, had significantly fewer and less severe lung and liver symptoms than the HFA group (Figures 2E-H). None of the 32 patients had ≥ 3-grade kidney toxicity. Triglycerides were detected in 5 of the 16 patients with HFA; among them, 3 of 4 lymphoma patients had a high serum triglyceride level (> 3 mmol/L), and 1 leukemia patient who had serum triglyceride tests was normal. All of them had high peak values of soluble IL-2 receptor, which were all higher than the upper detection limit (7500 u/ml, normal range 223-710 u/ml). Hypofibrinogenemia (< 1.5 g/L) was found in 87.5% (7/8) of lymphoma patients and 62.5% (5/8) of leukemia patients, and the minimums of fibrinogen after the CAR-T cells infusion of the HFA patients were significantly lower than those in the control group (P=0.0021 for B-NHL cohort and P=0.0162 for B-ALL cohort) (Supplementary Figures 2I, J). For the bone marrow tests, these 16 patients with HFA were critically ill during the CRS phase, and considering that the results could hardly affect the treatment strategy, bone marrow biopsy was not performed.
Among the 16 patients with HFA, there was no difference in OS, ORR, levels of serum ferritin or peaks of CAR transgene copies between patients with and without CAR T-cell-related MAS. However, patients with MAS had a more serious CRS than those without MAS (P=0.0087) (Figures 3A-F).
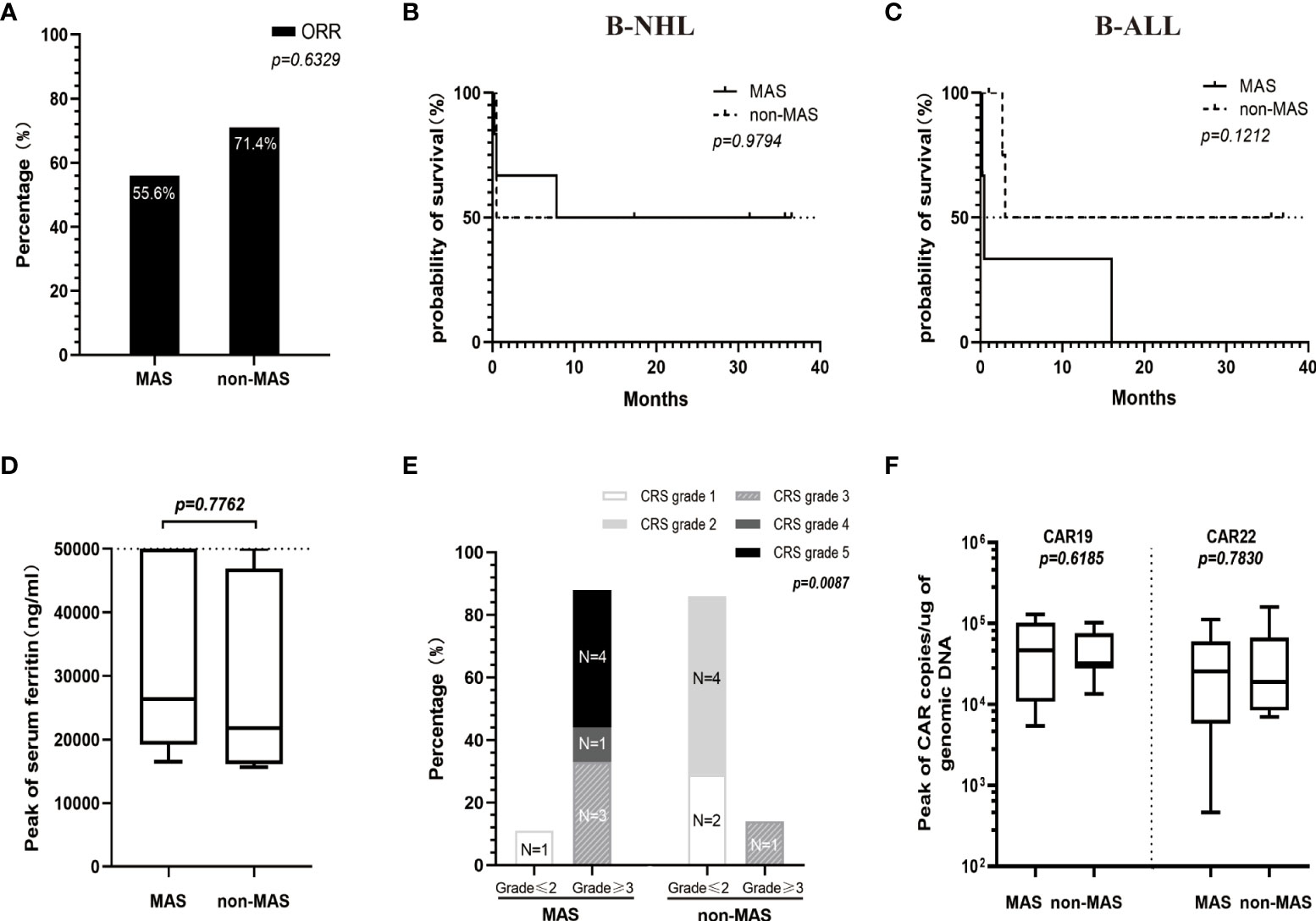
Figure 3 Comparison between patients of the HFA group with MAS (n=9) and without MAS (n=7) in ORR (A), OS (B, C), levels of serum ferritin (D), CRS grade (E) and the peaks of CAR transgene copies (F). (D) The medians of the peak values of serum ferritin in patients with or without MAS were 26328 (range, 16479-50000) ng/ml and 21750 (range, 15650-50000) ng/ml, respectively. (E) Patients with MAS had a significantly higher high-grade CRS (≥3) rate than patients without MAS (P=0.0087). (F) The peak values for CAR19 and CAR22 transgene copies in the MAS group (median 46393, range 5441-129375 copies/µg for CAR19 and median 25688, range 464-112033 copies/µg for CAR22) were not significantly different from those in the non-MAS group (median 31909, range 13473-102707 copies/µg for CAR19 and median 18924, range 6970-159171 copies/µg for CAR22).
Treatment and CAR T-cell kinetics
Methylprednisolone (MP) was administered for CRS in all 16 patients with HFA, and the median of the initial application time was 6 days (range, 3-12 days) after infusion. The median MP dosages were 1276.5 mg (range, 213-4447 mg) for 4 patients who died of serious CRS, 307 mg (range, 146 to 916 mg) for 5 patients who died of other reasons, and 167 mg (range, 40 to 733 mg) for 7 survivors. Notably, the MP dosages in 4 patients with HFA who died of serious CRS were higher than those in the other 12 HFA cases (median 237 mg, range 40-916 mg) (P=0.0173). Only 3 of 16 patients in the control group received MP for CRS (median 53.33 mg, range 16-80 mg). Unsurprisingly, the patients with HFA had significantly higher dosages of MP than those without HFA (P<0.0001) (Figure 4A). Moreover, most HFA patients also received plasmapheresis and hemofiltration, and some of them underwent tocilizumab therapy. Advanced life support, including vasopressor drug pumps, bipaps, invasive mechanical ventilation and oxygen therapy, was performed as needed (Table 3).
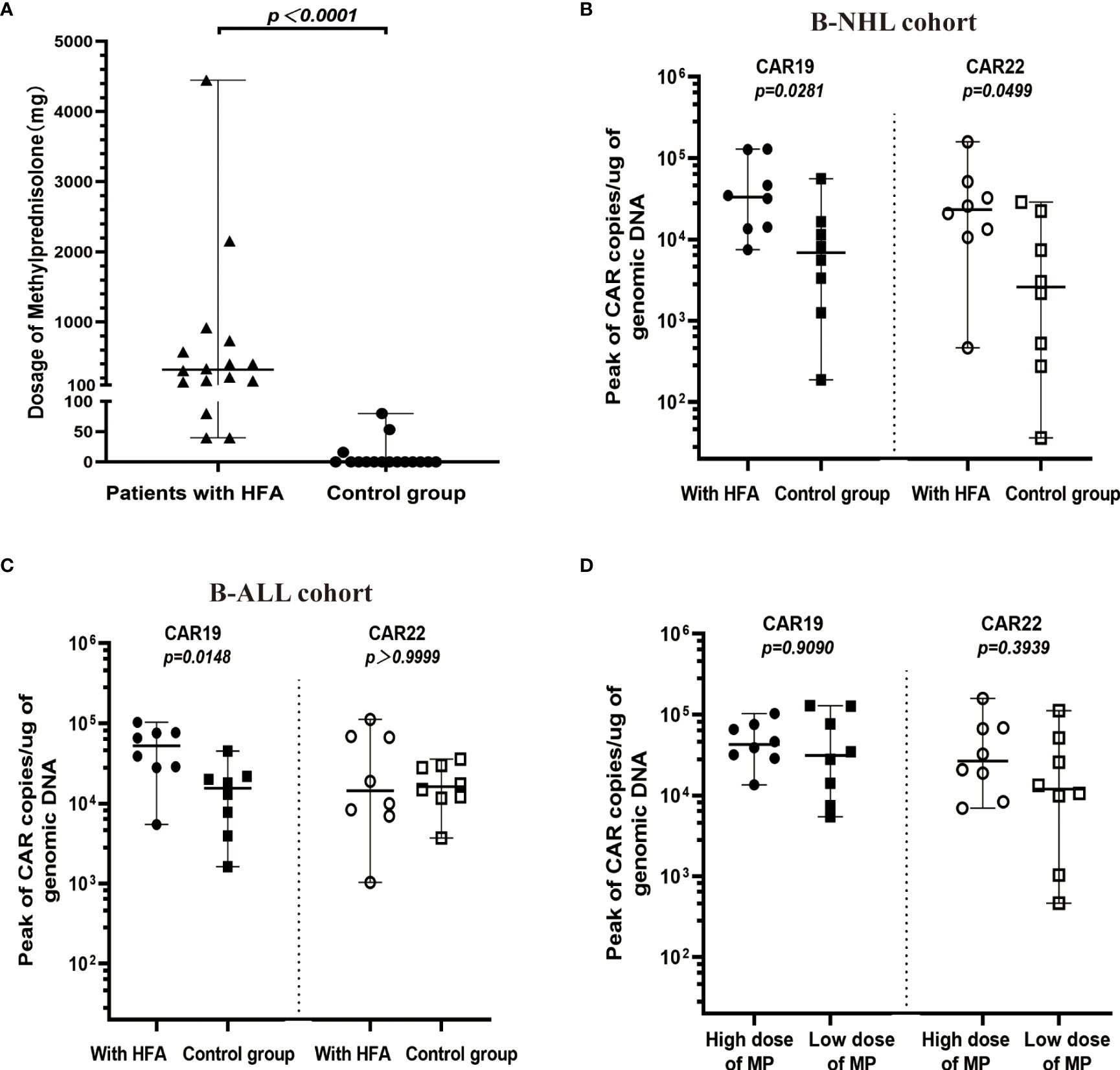
Figure 4 Cellular kinetics of CAR transgenes in peripheral blood. (A) Comparison between HFA group (n=16) and control group (n=16) in dosages of MP (median 320 mg, range 40-4447 mg, and median 0 mg, range 0-80 mg, respectively), only 3 patients in the control group received MP after the CAR-T cell infusion. (B) In B-NHL cohort, the peak values for CAR19 and CAR22 transgene copies in the HFA group (median 33222, range 7458-129375 copies/µg for CAR19 and median 23284, range 464-159171 copies/µg for CAR22) were all significantly higher than those in the control group (median 6864, range 187-55774 copies/µg for CAR19 and median 2588, range 36-28740 copies/µg for CAR22). (C) In B-ALL cohort, the peak values for CAR19 (median 52291, range 5441-102707 copies/µg), but not for CAR22 (median 14433, range 1033-112033 copies/µg) transgene copies in the HFA group were significantly higher than those in the control group (median 15519, range 1615-45078 copies/µg for CAR19 and median 16170, range 3703-35729 copies/µg for CAR22). (D) According to the median dose of MP (320 mg), 16 patients with HFA were divided into a low-dose MP group and a high-dose MP group. The peaks of CAR19 and CAR22 transgene copies in the high-dose MP group (median 31679, range 5441-129375 copies/µg for CAR19 and median 11949, range 464-112033 copies/µg for CAR22) were not significantly different from those in the low-dose MP group (median 42697, range 4770-102707 copies/µg for CAR19 and median 26669, range 6970-159171 copies/µg for CAR22).
Both CAR19 and CAR22 T cells expanded well in all patients with HFA. During the 14 days after CAR T-cell infusion, the peaks for CAR19 and CAR22 in the peripheral blood were 36767 copies/µg (range, 4770-129375) and 23283.5 copies/µg (range, 464-159171), respectively. In the control group, the peaks for CAR19 and CAR22 in the peripheral blood were 11393 copies/µg (range, 1255-55774) and 12141 copies/µg (range, 275-159171), respectively (Supplementary Figures 3A, B). The peaks for CAR19 in all HFA patients and the peaks for CAR22 in HFA patients with B-NHL were significantly higher than those in the control group (Figures 4B, C). All patients with HFA were divided into two groups—a high dose and a low dose of methylprednisolone—based on its median dose of 320 mg. There was no significant difference in the peaks of CAR19 and CAR22 between the two groups (Figure 4D). The peaks of CAR19 (P=0.0905) and CAR22 (P=0.3349) in CR and PR patients were not higher than those in the nonresponding patients (Supplementary Figure 3C). The patients who died of CRS had similar CAR transgene copies to the other patients for CAR19 and CAR22 (Supplementary Figure 3D).
Discussion
In our study, 16 out of 185 patients (129 with lymphoma and 56 with leukemia) had high serum ferritin levels (above 10,000 ng/ml) after CAR T-cell infusion. The incidence of CAR T-related MAS in 185 cases was 4.86% (9/185), and in 16 cases, the incidence of CAR T-related MAS was 56.25% (9/16). Although CAR T-cell-related HFA and MAS are rare adverse events, these adverse events often have very poor outcomes.
For 16 cases with HFA, the CR rates were 37.5% in lymphoma and 83.3% in leukemia, which were lower than those in our previous report (50% and 96%) (14). Poor CR rates may be related to an extremely high tumor burden. For the 8 lymphoma cases, 4 cases had bulky disease, bone marrow (62% BM blast) was involved in one case, one case had primary cutaneous DLBCL-leg type and the other 2 cases had CNS or spine involvement. Three lymphoma patients who had no response to CAR T-cell therapy all had bulky disease, which is similar to previous research, suggesting that a high tumor burden, especially bulky disease, was associated with an inadequate therapeutic response (20–22). Four out of 8 leukemia cases had extramedullary infiltration. The leukemia patient with an extramedullary mass was a nonresponding patient. Some previous reports also implied that leukemia with an extramedullary mass had a poor response to CAR T-cell therapy (23, 24). In terms of the efficacy in our study, patients with high-risk genetic abnormalities were more likely to have a high tumor burden. It was probably that high-risk genetic abnormalities are closely related to chemoresistance (25–27). Compared with the control group, HFA patients also had a higher proportion of IPI scores, large masses, and extranodal involvement; a lower CR rate; and a more serious CRS. Therefore, early delivery of CAR T-cells, especially before chemoresistant clones dominate in the tumor tissue and become bulky disease, may induce a better response in these high-risk cases.
Meanwhile, high tumor burden might bring high CAR T-cell therapy risk. In our study, a higher rate of high-grade CRS was observed in patients with CAR T-related MAS than in patients with CAR T-related HFA only and our previous report (14). 4 patients died of severe CRS, and they all had CAR T-related MAS. The mortality (44.4%, 4/9) in patients with CAR T-related MAS was very high. Both a poor response to CAR T-cell therapy and severe CRS caused a shorter median OS (3 months and not reached in leukemia and lymphoma, respectively) compared with patients without HFA, as in our previous report (31 months for leukemia and 18 months for lymphoma) and other trials (12.9-13.3 months for leukemia and 12-21.1 months for lymphoma) (3, 23, 28, 29). Notably, higher the peaks for CAR19 in all HFA patients than those in the control group indicate a high tumor burden could lead to a rapid expansion of CAR T-cells, which led to severe side effects. Meanwhile, previous studies have also shown that a high tumor burden was positively correlated with CAR T-cell expansion, and these CAR T-cells induced the activation of macrophages. There was more macrophage infiltration in tumor tissue when the patient had a higher tumor burden (30, 31). The severity of CRS after CAR T-cell therapy was closely associated with the expansion and overactivation of CAR T-cells and CAR T-related macrophages (32–34).
Although the HFA patients received a higher dose of glucocorticoids than the control group, the peaks for CAR19 in all HFA patients and the peaks for CAR22 in HFA patients with B-NHL were significantly higher than those in the control group, which might indicate that glucocorticoid intervention had little impact on the expansion of CAR T-cells. However, there are many factors, in addition to glucocorticoids, affect the expansion of CAR T-cells, including the general condition of the patients, tumor burden, and the quality of the CAR T-cells (35–37). Notably, the patients with HFA had similar baseline characteristics and received a high dose of glucocorticoids after CAR T-cell infusion. Glucocorticoids also showed little impact on the expansion of CAR T-cells which was no significant difference between a high dose and a low dose of methylprednisolone. The median time of glucocorticoid use was 6 days after CAR T-cell infusion when the grade of CRS reached 2. The ZUMA 1 cohorts 4 and 6 showed that the occurrence of high-grade CRS was decreased when glucocorticoids were administered early, during CRS grade 1 or preventively before CRS (38). Based on the safety and above data, early glucocorticoid intervention might be useful to improve the safety of CAR T-cell therapy.
In our study, high serum ferritin (≥ 10,000 ng/ml) after CAR T infusion, which was observed in patients with heavy/mass disease burden or high-risk genetic abnormalities, may indicate a poor response and high risk of severe CRS. Early delivery of CAR T-cells and early glucocorticoid intervention during CAR T-cell therapy might improve the outcome of these patients.
Data availability statement
The raw data supporting the conclusions of this article will be made available by the authors, without undue reservation.
Ethics statement
The studies involving human participants were reviewed and approved by the Medical Ethics Committee of the Tongji Hospital, Tongji Medical College, Huazhong University of Science and Technology (TJ-IRB20160310). The patients/participants provided their written informed consent to participate in this study. Written informed consent was obtained in accordance withthe Declaration of Helsinki. This study is registered at www.chictr.org.cn as ChiCTR-OPN-16008526.
Author contributions
LZ, NY, and XZ conceived and coordinated the study, designed, performed, and analyzed the experiments, and wrote the paper. TL, HJ, LJ, DW, and BX carried out the data collection and data analysis and revised the paper. All authors contributed to the article and approved the submitted version.
Funding
This work was supported by the National Natural Science Foundation of China (Grant No. 8217011455).
Acknowledgments
The authors would like to thank all members of the study team for their clinical support.
Conflict of interest
The authors declare that the research was conducted in the absence of any commercial or financial relationships that could be construed as a potential conflict of interest.
Publisher’s note
All claims expressed in this article are solely those of the authors and do not necessarily represent those of their affiliated organizations, or those of the publisher, the editors and the reviewers. Any product that may be evaluated in this article, or claim that may be made by its manufacturer, is not guaranteed or endorsed by the publisher.
Supplementary material
The Supplementary Material for this article can be found online at: https://www.frontiersin.org/articles/10.3389/fonc.2022.912689/full#supplementary-material
References
1. Chou CK, Turtle CJ. Assessment and management of cytokine release syndrome and neurotoxicity following CD19 CAR-T cell therapy. Expert Opin Biol Ther (2020) 20(6):653–64. doi: 10.1080/14712598.2020.1729735
2. Locke FL, Go WY, Neelapu SS. Development and use of the anti-CD19 chimeric antigen receptor T-cell therapy axicabtagene ciloleucel in Large b-cell lymphoma: A review. JAMA Oncol (2020) 6(2):281–90. doi: 10.1001/jamaoncol.2019.3869
3. Schuster SJ, Bishop MR, Tam CS, Waller EK, Borchmann P, McGuirk JP, et al. Tisagenlecleucel in adult relapsed or refractory diffuse Large b-cell lymphoma. New Engl J Med (2019) 380(1):45–56. doi: 10.1056/NEJMoa1804980
4. Neelapu SS, Tummala S, Kebriaei P, Wierda W, Gutierrez C, Locke FL, et al. Chimeric antigen receptor T-cell therapy - assessment and management of toxicities. Nat Rev Clin Oncol (2018) 15(1):47–62. doi: 10.1038/nrclinonc.2017.148
5. Teachey DT, Lacey SF, Shaw PA, Melenhorst JJ, Maude SL, Frey N, et al. Identification of predictive biomarkers for cytokine release syndrome after chimeric antigen receptor T-cell therapy for acute lymphoblastic leukemia. Cancer Discovery (2016) 6(6):664–79. doi: 10.1158/2159-8290.CD-16-0040
6. Shah N, Highfill S, Shalabi H, Yates B, Jin J, Wolters P, et al. CD4/CD8 T-cell selection affects chimeric antigen receptor (CAR) T-cell potency and toxicity: Updated results from a phase I anti-CD22 CAR T-cell trial. J Clin Oncol (2020) 38(17):1938–50. doi: 10.1200/JCO.19.03279
7. Sandler RD, Tattersall RS, Schoemans H, Greco R, Badoglio M, Labopin M, et al. Diagnosis and management of secondary HLH/MAS following HSCT and CAR-T cell therapy in adults; a review of the literature and a survey of practice within EBMT centres on behalf of the autoimmune diseases working party (ADWP) and transplant complications working party (TCWP). Front Immunol (2020) 11:524. doi: 10.3389/fimmu.2020.00524
8. Tamamyan GN, Kantarjian HM, Ning J, Jain P, Sasaki K, Mcclain KL, et al. Malignancy-associated hemophagocytic lymphohistiocytosis in adults: Relation to hemophagocytosis, characteristics, and outcomes. Cancer (2016) 122(18):2856–68. doi: 10.1002/cncr.30084
9. Allen CE, Yu X, Kozinetz CA, McClain KL. Highly elevated ferritin levels and the diagnosis of hemophagocytic lymphohistiocytosis. Pediatr Blood Cancer (2008) 50(6):1227–35. doi: 10.1002/pbc.21423
10. Chmielewski M, Kopecky C, Hombach AA, Abken H. IL-12 release by engineered T cells expressing chimeric antigen receptors can effectively muster an antigen-independent macrophage response on tumor cells that have shut down tumor antigen expression. Cancer Res (2011) 71(17):5697–706. doi: 10.1158/0008-5472.CAN-11-0103
11. Guo Q, Jin Z, Yuan Y, Liu R, Tao X, Wei H, et al. New mechanisms of tumor-associated macrophages on promoting tumor progression: Recent research advances and potential targets for tumor immunotherapy. J Immunol Res (2016) 2016:9720912. doi: 10.1155/2016/9720912
12. Melody M, Rahman ZA, Saunders H, Diaz PL, Gannon N, Rosenthal A, et al. C-reactive protein and ferritin levels and length of intensive care unit stay in patients with b-cell lymphomas treated with axicabtagene ciloleucel. Hematol/oncol Stem Cell Ther (2021) 14(2):141–6. doi: 10.1016/j.hemonc.2020.09.004
13. Karschnia P, Jordan JT, Forst DA, Arrillaga-Romany IC, Batchelor TT, Baehring JM, et al. Clinical presentation, management, and biomarkers of neurotoxicity after adoptive immunotherapy with CAR T cells. Blood (2019) 133(20):2212–21. doi: 10.1182/blood-2018-12-893396
14. Wang N, Hu X, Cao W, Li C, Xiao Y, Cao Y, et al. Efficacy and safety of CAR19/22 T-cell cocktail therapy in patients with refractory/relapsed b-cell malignancies. Blood (2020) 135(1):17–27. doi: 10.1182/blood.2019000017
15. National Comprehensive Cancer Network (NCCN). Clinical practice guidelines in oncology. b-cell lymphomas. version 2 (2019). Available at: https://www.nccn.org/professionals/physician_gls/pdf/b-cell.pdf (Accessed 29 April, 2019).
16. National Comprehensive Cancer Network (NCCN). Clinical practice guidelines in oncology. acute lymphoblastic leukemia. version 2 (2021). Available at: https://www.nccn.org/patients (Accessed 19 July, 2021).
17. Cheson BD, Fisher RI, Barrington SF, Cavalli F, Lister TA. Recommendations for initial evaluation, staging, and response assessment of Hodgkin and non-Hodgkin lymphoma: The lugano classification. J Clin Oncol (2014) 32(27):3059–68. doi: 10.1200/JCO.2013.54.8800
18. National Cancer Institute. Common terminology criteria for adverse events (CTCAE),version 5.0 . Available at: https://ctep.cancer.gov/protocolDevelopment/electronic_applications/docs/CTCAE_v5_Quick_Reference_8.5x11.pdf (Accessed November 27, 2017).
19. Zeng C, Cheng J, Li T, Huang J, Li C, Jiang L, et al. Efficacy and toxicity for CD22/CD19 chimeric antigen receptor T-cell therapy in patients with relapsed/refractory aggressive b-cell lymphoma involving the gastrointestinal tract. Cytotherapy (2020) 22(3):166–71. doi: 10.1016/j.jcyt.2020.01.008
20. Dean EA, Mhaskar RS, Lu H, Mousa MS, Krivenko GS, Lazaryan A, et al. High metabolic tumor volume is associated with decreased efficacy of axicabtagene ciloleucel in large b-cell lymphoma. Blood Adv (2020) 4(14):3268–76. doi: 10.1182/bloodadvances.2020001900
21. Thieblemont C, Meignan M, Gaulard P, Salles G, Briere J, Godmer P, et al. High total metabolic tumor volume at baseline predicts survival independent of response to therapy. Blood (2020) 135(16):1396–405. doi: 10.1182/blood.2019003526
22. Zhou X, Ge T, Li T, Huang L, Cao Y, Xiao Y, et al. CAR19/22 T cell therapy in adult refractory burkitt’s lymphoma. Cancer immunol immunother: CII (2021) 70(8):2379–84. doi: 10.1007/s00262-021-02850-6
23. Park JH, Riviere I, Gonen M, Wang X, Senechal B, Curran KJ, et al. Long-term follow-up of CD19 CAR therapy in acute lymphoblastic leukemia. N Engl J Med (2018) 378(5):449–59. doi: 10.1056/NEJMoa1709919
24. An F, Wang H, Liu Z, Wu F, Zhang J, Tao Q, et al. Influence of patient characteristics on chimeric antigen receptor T cell therapy in b-cell acute lymphoblastic leukemia. Nat Commun (2020) 11(1):5928. doi: 10.1038/s41467-020-19774-x
25. Pascual M, Mena-Varas M, Robles EF, Garcia-Barchino MJ, Panizo C, Hervas-Stubbs S, et al. PD-1/PD-L1 immune checkpoint and p53 loss facilitate tumor progression in activated b-cell diffuse large b-cell lymphomas. Blood (2019) 133(22):2401–12. doi: 10.1182/blood.2018889931
26. Intlekofer AM, Joffe E, Batlevi CL, Hilden P, He J, Seshan VE, et al. Integrated DNA/RNA targeted genomic profiling of diffuse large b-cell lymphoma using a clinical assay. Blood Cancer J (2018) 8(6):60. doi: 10.1038/s41408-018-0089-0
27. Zenz T, Kreuz M, Fuge M, Klapper W, Horn H, Staiger AM, et al. TP53 mutation and survival in aggressive b cell lymphoma. Int J Cancer (2017) 141(7):1381–8. doi: 10.1002/ijc.30838
28. Leahy AB, Elgarten CW, Grupp SA, Maude SL, Teachey DT. Tisagenlecleucel for the treatment of b-cell acute lymphoblastic leukemia. Expert Rev Anticancer Ther (2018) 18(10):959–71. doi: 10.1080/14737140.2018.1512411
29. Abramson J, Palomba M, Gordon L, Lunning M, Wang M, Arnason J, et al. Lisocabtagene maraleucel for patients with relapsed or refractory large b-cell lymphomas (TRANSCEND NHL 001): a multicentre seamless design study. Lancet (London England) (2020) 396(10254):839–52. doi: 10.1016/S0140-6736(20)31366-0
30. Giavridis T, van der Stegen SJC, Eyquem J, Hamieh M, Piersigilli A, Sadelain M. CAR T cell-induced cytokine release syndrome is mediated by macrophages and abated by IL-1 blockade. Nat Med (2018) 24(6):731–8. doi: 10.1038/s41591-018-0041-7
31. Hao Z, Li R, Meng L, Han Z, Hong Z. Macrophage, the potential key mediator in CAR-T related CRS. Exp Hematol Oncol (2020) 9:15. doi: 10.1186/s40164-020-00171-5
32. Frey N, Porter D. Cytokine release syndrome with chimeric antigen receptor T cell therapy. Biol Blood marrow Transplant (2019) 25(4):e123–e7. doi: 10.1016/j.bbmt.2018.12.756
33. Fitzgerald JC, Weiss SL, Maude SL, Barrett DM, Lacey SF, Melenhorst JJ, et al. Cytokine release syndrome after chimeric antigen receptor T cell therapy for acute lymphoblastic leukemia. Crit Care Med (2017) 45(2):e124–e31. doi: 10.1097/CCM.0000000000002053
34. Freyer CW, Porter DL. Cytokine release syndrome and neurotoxicity following CAR T-cell therapy for hematologic malignancies. J Allergy Clin Immunol (2020) 146(5):940–8. doi: 10.1016/j.jaci.2020.07.025
35. Neelapu SS, Locke FL, Bartlett NL, Lekakis LJ, Miklos DB, Jacobson CA, et al. Axicabtagene ciloleucel CAR T-cell therapy in refractory Large b-cell lymphoma. N Engl J Med (2017) 377(26):2531–44. doi: 10.1056/NEJMoa1707447
36. Gardner RA, Ceppi F, Rivers J, Annesley C, Summers C, Taraseviciute A, et al. Preemptive mitigation of CD19 CAR T-cell cytokine release syndrome without attenuation of antileukemic efficacy. Blood (2019) 134(24):2149–58. doi: 10.1182/blood.2019001463
37. Strati P, Ahmed S, Furqan F, Fayad LE, Lee HJ, Iyer SP, et al. Prognostic impact of corticosteroids on efficacy of chimeric antigen receptor T-cell therapy in large b-cell lymphoma. Blood (2021) 137(23):3272–6. doi: 10.1182/blood.2020008865
Keywords: B-cell malignancies, CAR T-cell therapy, hyperferritinaemia, prognosis, clinical characteristics
Citation: Zhou L, Yu N, Li T, Ji H, Jiang L, Wang D, Xu B and Zhou X (2022) Clinical characteristics and prognosis of 16 relapsed/refractory B-cell malignancy patients with CAR T-cell-related hyperferritinaemia. Front. Oncol. 12:912689. doi: 10.3389/fonc.2022.912689
Received: 04 April 2022; Accepted: 21 September 2022;
Published: 12 October 2022.
Edited by:
John Maher, King’s College London, United KingdomReviewed by:
Liang Lin, Cytovia Therapeutics, United StatesYuhua Feng, Central South University, China
Copyright © 2022 Zhou, Yu, Li, Ji, Jiang, Wang, Xu and Zhou. This is an open-access article distributed under the terms of the Creative Commons Attribution License (CC BY). The use, distribution or reproduction in other forums is permitted, provided the original author(s) and the copyright owner(s) are credited and that the original publication in this journal is cited, in accordance with accepted academic practice. No use, distribution or reproduction is permitted which does not comply with these terms.
*Correspondence: Xiaoxi Zhou, Y2VsbG8zMTZAMTYzLmNvbQ==
†These authors have contributed equally to this work and share first authorship