- 1Department of Oncology, Affiliated Hospital of Southwest Medical University, Luzhou, China
- 2Institute of Drug Clinical Trial/GCP Center, Affiliated Hospital of Southwest Medical University, Luzhou, China
Normalizing the tumor microenvironment (TME) is a potential strategy to improve the effectiveness of immunotherapy. Vascular endothelial growth factor (VEGF) and transforming growth factor (TGF)-β pathways play an important role in the development and function of the TME, contributing to the immunosuppressive status of TME. To inhibit VEGF and/or TGF-β pathways can restore TME from immunosuppressive to immune-supportive status and enhance sensitivity to immunotherapy such as programmed death protein-1 (PD-1)/programmed cell death-ligand 1 (PD-L1) inhibitors. In this review, we described the existing preclinical and clinical evidence supporting the use of anti-VEGF and/or anti-TGF-β therapies to enhance cancer immunotherapy. Encouragingly, adopting anti-VEGF and/or anti-TGF-β therapies as a combination treatment with anti-PD-(L)1 therapy have been demonstrated as effective and tolerable in several solid tumors in clinical trials. Although several questions need to be solved, the clinical value of this combination strategy is worthy to be studied further.
Introduction
The tumor microenvironment (TME) is a complex environment characterized by several unique properties including hypoxia, a low PH, an abnormal tumor immune microenvironment (TIME), a high interstitial fluid pressure and vascular abnormalities (1). The treatments targeting TME have been proposed as the promising strategies for cancer treatments, especially immunotherapy and antiangiogenic therapy (1, 2).
Recent years, immunotherapy has shown dramatic effects in the management of various human cancers. The programmed death protein-1 (PD-1)/programmed cell death-ligand 1 (PD-L1) pathway mediates tumor immune evasion. The agents targeting PD-(L)1 have the potential to reverse the inhibition of cytotoxic T cells in the TME, exhibiting promising antitumor activity in various tumor types (2–4). However, 50-80% of patients with tumors do not benefit from PD-(L)1 inhibitors as monotherapies (5). TME has been reported to reduce the effectiveness of immunotherapy (6). Thus, normalizing the TME is regarded as a promising strategy to enhance immunotherapy.
Previous studies have revealed that overexpression of vascular growth factors inhibited immune effector cells and activated immunosuppressive cells directly (1, 5). And the hypoxia TME caused by abnormal tumor vascular structure can prevent immune cell infiltration (1). Thus, tumor vascular structure and vascular growth factors such as vascular endothelial growth factor (VEGF) and its receptor (VEGFR), contributed to the immunosuppressive features of TME (7). Currently, VEGF/VEGFR2 pathway is the predominant target for the development of anti-angiogenesis agents. The efficacy of the combination of anti-PD-(L)1 and anti-VEGF has been evaluated in clinical trials (8, 9).
As a well-studied pleiotropic cytokine, transforming growth factor (TGF)-β plays a vital role in regulating cellular proliferation. Three TGF-β isoforms have been identified in mammals: TGF-β1 (the most common type), TGF-β2, and TGF-β3 (10). In this review, we mainly discussed the function role of TGF-β1. TGF-β in TME has been identified to promote tumor cell invasiveness, migration, and metastasis through mechanisms including tumor angiogenesis and epithelial-mesenchymal transition (EMT) (11). High expression of TGF-β in the TME is reported to be associated with poor clinical outcome (12). TGF-β also has immune-related activities, which works to inhibit cytotoxic T cells, induct peripheral Treg from naive CD4+ cells and increase the survival of myeloid progenitors that differentiate to potent myeloid-derived suppressor cell (MDSCs) (11–13). The activity of TGF-β is reported to promote resistance to anti-PD-L1 therapies (14, 15). Furthermore, TGF-β in TME has been shown to work cooperatively with hypoxia-inducible factor (HIF)-1, which is increased under hypoxic conditions, in inducing the expression of VEGF, driving tumor angiogenesis (16). Thus, inhibition of the TGF-β pathway has been report to overcome immunosuppression, suppress angiogenesis and EMT, and inhibit tumor growth (12).
Previous data supported the hypothesis that anti-PD-(L)1 combined with anti-VEGF and/or anti-TGF-β may offer stronger antitumor activity by synergistically reducing angiogenesis and further enhancing the immune response against malignancy. Therefore, in the present review, the existing evidence supporting the applicability of this treatment strategy was summarized.
Rational for targeting VEGF/VEGFR and/or TGF-β for enhancing anti-PD-(L)1 response
The role of VEGF pathway in TIME
The role of VEGF pathways in TIME has been extensively investigated in tumor models (5). The mechanisms how excessive levels of VEGF induce immunosuppression of TME include at least the following four distinct aspects.
First, VEGF could inhibit dendritic cell (DC) maturation and antigen presentation. DCs are considered as professional and most powerful antigen presenting cells (APCs), which could active T cells and enhance the immune response (17). As early as 1998, Oyama et al. found that VEGF inhibited DC maturation through integrating with its receptor on DC surface (18). Alfaro et al. pointed out that VEGF could inhibit the differentiation of monocytes into DCs (19). Furthermore, Curiel and colleagues found that VEGF can upregulate the expression of PD-L1 on DCs, inhibiting DC antigen presentation function and suppressing the T cells activation (20).
Second, excessive levels of VEGF in TME directly inhibits cytotoxic T lymphocytes (CTL) effector function. The study conducted by Ohm et al. showed an inhibiting effect of VEGF on hematopoietic progenitor cells differentiating to CD8+ and CD4+ T cells, which contributes to tumor-associated immune deficiencies (21). Voron et al. demonstrated that VEGF produced in TME can enhance the expression of PD-1 and other checkpoints involving in CD8+ T cell exhaustion (22). And the treatment targeting VEGF was found to remarkably enhance CD8+ T cell effector function (23, 24).
Third, the excessive levels of VEGF can promote the recruitment of immunosuppressive cells. For example, Ning et al. enrolled 36 patients with renal cell carcinoma (RCC) and observed that VEGF expression was positively associated with the frequency of tumor-infiltrating regulatory T-cells (Tregs) (25). Besides, Terme et al. found that VEGF directly triggers Treg proliferation and anti-VEGF therapy, exerting a promising immunologic effect (26). Courau et al. pointed out that silencing of VEGF resulted in dramatically decreased Tregs and MDSCs in tumor infiltrates, markedly improving the efficacy of anti-PD-1 treatment (13).
Fourth, VEGF has an ability to promote adhesion molecules and chemokines expressions, resulting in abnormal tumor vasculature structures, which can lead to a hypoxia and low PH condition in TME, subsequently, forming a selective immune-cell barrier to reduce the infiltration of different immune cells (1, 27).
Collectively, overexpression of VEGF in TME has a role on suppressing immune effector cells and activating immunosuppressive cells. And VEGF-induced tumor vessels has abnormal vascular structure which can prevent immune cell infiltration. Through these mechanisms, VEGF contributes to an immunosuppressive TME.
The role of TGF-β in TIME
TGF-β is pleiotropic cytokine, produced by activated macrophages, platelets, keratinocytes and fibroblasts (28). TGF-β can regulate fibrosis, EMT, and angiogenesis in tumor growth, it can also exert effect in both innate immune and adaptive immune systems, particularly in immunosuppression (12). The innate immune system is composed by monocytes, macrophages, DCs, granulocytes, and NK cells (29). In the TGF-β-rich TME, the antigen-presenting DCs was reported to shift into a tolerogenic phenotype, with decreased ability to activate T cells (30, 31). The development and differentiation of NK cells is also strongly influenced by TGF-β. Marcoe et al. pointed out that the TGF-β pathway was responsible for NK cell immaturity (32). Viel et al. demonstrated that TGF-β could inhibit the activation of NK cells by inhibiting the mTOR related pathways (33). In myeloid cells, TGF-β was reported to stimulate the survival of monocytes (34). And the inhibition of TGF-β signaling could decrease MDSC differentiation and increase differentiation to proinflammatory macrophages (34). Furthermore, the TGF-β in TME may suppress the inflammatory functions of macrophages, contributing to the immune evasion of cancer cells (35).
In adaptive immune system, TGF-β has been reported to inhibit T cell proliferation, activation, and effector functions. Early studies revealed that TGF-β could inhibit the expression of T-box transcription factor (T-bet) to block Th1 and CD8+ cytotoxic T cells differentiation from naïve T cell (36). Furthermore, Thomas et al. found that TGF-β could specifically inhibit CTL-mediated tumor cytotoxicity through decreasing the levels of cytolytic gene products such as perforin, Fas ligand, granzyme A, granzyme B, and IFNγ (37). And neutralization of systemic TGF-β in mice could restore these cytotoxic gene expression in CTLs, reinforcing tumor clearance (37, 38). These findings suggested an important role of TGF-β pathway in inhibiting tumor antigen-specific T cell priming (38). In addition, Ahmadzadeh and colleague found that TGF-β attenuated the effector function of antigen-specific CD8+ cells obtained (39). TGFβ-rich TME can promote T cells differentiating to a Treg phenotype (40).
Overall, TGF-β in TME acts as an important suppressor of the innate and adaptive immune responses. Targeting TGF-β has been regarded as a novel strategy to enhance the effect of immunotherapy.
The role of VEGF and/or TGF-β in enhancing the sensitivity to anti-PD-(L)1 therapy
Overexpression of VEGF in TME can inhibit immune effector cells and activate immunosuppressive cells, driving immunosuppression in the TME. Blockade of VEGF/VEGFR pathway may enhance the efficacy of anti-PD-(L)1 agents. The data from a number of preclinical studies supported this hypothesis (41–43). And the efficacy and safety of this combination therapy has also been confirmed in several tumor types in clinical trials.
In a previous study, Courau et al. found that blocking of TGF-β pathway could reduce the number of Treg cells, increase the activity of effector T cells, thus restoring the sensitivity to anti-PD-L1 therapy (13). Similarly, Mariathasan et al. found that TGF-β could shape the TME to restrain anti-tumor immunity by restricting T-cell infiltration (44). Therapeutic co-administration of anti-TGF-β to anti-PD-L1 could facilitate T-cell penetration into the center of tumor and enhance anti-tumor immunity (44). These mechanisms provide basis for the combination of anti-TGF-β and anti-PD-(L)1 therapies.
The cross-talk between VEGF and TGF-β pathways has also been identified previously (45). VEGF and TGF-β are usually co-expressed in tumor tissues in which angiogenesis occurs (46). The results from previous studies have shown that TGF-β up-regulated the expression of VEGF (47). Due to the cross-talk between VEGF and TGF-β pathways, the dual inhibition of VEGF and TGF-β may have a stronger anti-angiogenesis effect. Furthermore, the dual inhibition of TGF-β and VEGF signaling can synergistically reduce the number of Tregs and restore sensitivity to anti-PD-1 treatment (13). Thus, the triple inhibition of VEGF/VEGFR, TGF-β and PD-(L)1 seems rational.
Clinical trials about the dual inhibition of VEGF/VEGFR and PD-(L)1
Clinical trials on RCC
IMmotion151 (NCT02420821) was a phase III trial to compare the efficacy of atezolizumab (an anti-PD-L1 agent) plus bevacizumab versus sunitinib in untreated metastatic RCC (mRCC) (48). This trial found that the atezolizumab plus bevacizumab arm had a significantly longer median progression-free survival (PFS) comparing to the sunitinib arm in the PD-L1 positive patient population (11.2 vs. 7.7 months, hazard ratio [HR]=0.74 95% CI: 0.57-0.96, p=0.0217). BTCRC-GU14-003 (NCT02348008) was a phase Ib/II trial to evaluate the potential use of pembrolizumab (a PD-1 inhibitor) combined with bevacizumab in mRCC (49). In the phase II, 48 mRCC patients who were treatment naïve were enrolled. The objective response rate (ORR) of this study was 60.9% (95%CI: 45.4%-74.9%) and the median PFS was 20.7 months (95%CI: 11.3-27.4 months), meeting its primary end point. Thus, the combination of pembrolizumab and bevacizumab is active in mRCC patients as first and subsequent lines of therapy (49).
Axitinib is an oral, potent, small molecule inhibitor of VEGFR 1-3 (50). NCT02853331 was a phase Ib trial that evaluated axitinib in combination with avelumab (an anti-PD-L1 agent) in advanced RCC patients (51). At data cutoff date, 58% of patients achieved complete response (CR)/partial response (PR), showing promising anti-tumor effect (51). Due to these results, a phase III trial (NCT02684006) was conducted. Untreated advanced RCC patients received the treatments of avelumab plus axitinib or the standard-of-care (SOC) sunitinib in a 1:1 ratio at random (52). In PD-L1 positive population, median PFS was 13.8 months vs 7.2 months favoring the avelumab plus axitinib treatment (HR=0.61, 95%CI: 0.47-0.79, p < 0.001). In the overall population, avelumab plus axitinib also resulted in a longer median PFS (13.8 months vs. 8.4 months, HR=0.69, 95%CI: 0.56-0.84; p<0.001) (52). According to these results, the combination of avelumab and axitinib for first-line treatment of clear cell RCC (ccRCC) has been approved by the US Food and Drug Administration (FDA). 861 ccRCC patients received pembrolizumab plus axitinib or sunitinib in a phase III trial named KEYNOTE-426 (NCT02853331) (53). Median PFS was much longer in patients received pembrolizumab plus axitinib than those received sunitinib (15.4 months vs. 11.1 months, HR=0.71, 95%CI: 0.60-0.84, p<0.001). This study demonstrated that pembrolizumab plus axitinib could be used as first-line treatment for ccRCC (53).
Lenvatinib is a multitargeted tyrosine kinase inhibitor (TKI) that mainly inhibits VEGFR1-3 (54). KEYNOTE-146 was a Ib/II study to evaluate lenvatinib combined with pembrolizumab in advanced solid tumors. In the RCC cohort, the ORR at week 24 was 63% (19/30, 95%CI: 43.9%-80.1%), showing encouraging anti-tumor activity in this type of patients (55). In a phase III trial (NCT02811861), 1069 RCC patients were assigned to receive lenvatinib in combination with pembrolizumab, lenvatinib in combination with everolimus, or sunitinib (56). The median PFS was much longer in lenvatinib plus pembrolizumab arm than in sunitinib arm (23.9 months vs. 9.2 months, HR=0.39, 95%CI: 0.32-0.49, P<0.001) (56).
Cabozantinib, is an oral VEGFR1-3 inhibitor. The CheckMate 9ER trial (NCT03141177) compared the efficacy and safety of nivolumab plus cabozantinib and sunitinib in previously untreated advanced RCC (57). Nivolumab plus cabozantinib showed significant benefits over sunitinib in advanced RCC, with median PFS of 16.6 months versus 8.3 months (HR=0.51, 95%CI:0.41-0.64, p<0.001) (57). Furthermore, the combination of cabozantinib with atezolizumab also demonstrated efficacy in patients with advanced RCC in a phase Ib trial (COSMIC-021, NCT03170960) (58).
Famitinib is a multitargeted TKI that mainly against VEGFR, C-Kit, and PDGFR. Famitinib plus camrelizumab (an anti-PD-1 antibody) showed enduring antitumor activity in patients with advanced RCC in a phase II trial (59). The ORR was 84.6% in treatment-naïve patients and 48.0% in pretreated patients, respectively (59). Pazopanib is also a TKI that mainly against VEGFR1-3 (1). Checkmate016 (NCT01472081), a phase I trial, evaluated the efficacy and safety of nivolumab (an anti-PD-1 agent) plus pazopanib or sunitinib in mRCC (60). The results showed that the ORR was 55% in nivolumab + sunitinib arm and 45% in nivolumab + pazopanib arm. For safety, this study showed that the combination of sunitinib or pazopanib and nivolumab caused a high incidence of toxicities which limited the future use of either combination regimen (60). Similarly, a phase I/II study showed that pazopanib plus pembrolizumab had significant hepatotoxicity, despite preliminary signs of efficacy were shown (61).
Clinical trials on non-small-cell lung cancer (NSCLC)
IMpower150 (NCT02366143) was a phase III clinical trial that randomized metastatic non-squamous NSCLC patients to receive atezolizumab plus carboplatin + paclitaxel (ACP), bevacizumab + carboplatin + paclitaxel (BCP), or atezolizumab + BCP (62). The results showed that adding atezolizumab to BCP significantly improved PFS and OS among patients with non-squamous NSCLC (62).
Dual inhibition of VEGFR2 with ramucirumab and PD-1 with pembrolizumab has shown potential anti-tumor effect in NSCLC by JVDF trial (NCT02443324) (63). The study showed that the ORR was 56.3% and 22.2% for patients with high and lower levels of PD-L1 expression, respectively (63). JVDJ (NCT02572687) was a phase Ia/b trial evaluating the safety and efficacy of ramucirumab and durvalumab (an anti-PD-1 antibody) in multi-tumor types (64). In the NSCLC cohort, the ORR, median PFS and OS were, respectively, 11%, 2.7 and 11 months. More prolonged survival could be obtained from patients with high PD-L1 expression (64).
Anlotinib is a novel TKI that mainly targets VEGFR 1-3, epidermal growth factor receptor (EGFR) and FGFR 1-4. A phase Ib trial (NCT03628521) assessed sintilimab (an anti-PD-1 antibody) and anlotinib in the frontline setting for patients with NSCLC, and found that this combination treatment represented a novel chemotherapy-free regimen for unresectable NSCLC without EGFR/ALK/ROS1 mutations, with an ORR of 72.7% and median PFS of 15 months (65). Another phase Ib trial evaluated the combination of anlotinib and PD-1 inhibitors camrelizumab for advanced NSCLC. Anlotinib plus camrelizumab showed promising efficacy for NSCLC, with a median PFS of 8.2 months and a median OS of 12.7 months (66).
Clinical trials on hepatocellular carcinoma (HCC)
IMbrave150 (NCT03434379) was a global, open-label, phase III trial, which assigned unresectable HCC patients to receive either atezolizumab in combination with bevacizumab or sorafenib in a 2:1 ratio (67). In this study, atezolizumab plus bevacizumab arm showed longer OS and PFS than sorafenib arm, as the HR for death was 0.58 (95%CI: 0.42-0.79, p<0.001) and HR for PFS was 0.59 (95%CI: 0.47-0.76, p<0.001) (67). ORIENT-32 (NCT0379440) was a phase II-III study to evaluate sintilimab plus bevacizumab biosimilar IBI305 versus sorafenib as a first-line treatment for unresectable HCC (68). In the phase III part, patients in the sintilimab-IBI305 arm had a much longer median PFS (4.6 months vs 2.8 months, HR=0.56, 95%CI: 0.46-0.70, p<0.0001) and OS (median not reached vs 10.4 months, HR=0.57, 95%CI: 0.43-0.75, p<0.0001) than those in the sorafenib arm (68). Furthermore, this combination regimen showed an acceptable safety profile (68).
A phase Ib trial (NCT03006926) had tried to use lenvatinib plus pembrolizumab in the treatment of unresectable HCC (69). This combination showed promising antitumor activity in HCC, with an ORR of 36.0% and median OS of 22 months (69). And the toxicities of this combination treatment were manageable, with no unexpected safety signals (69). Apatinib is a small molecule VEGFR-2-TKI and camrelizumab is an anti-PD-1 antibody. A phase II study (NCT03463876) showed that camrelizumab plus apatinib had promising efficacy in advanced HCC, with ORR of 34.3% (24/70) in the first-line setting and 22.5% (27/120) in the second-line setting, respectively (70).
Clinical trials on other solid tumors
In addition to RCC, NSCLC and HCC, clinical trials about dual inhibition of VEGF/VEGFR and PD-(L)1 in other types of solid tumors are also abundant.
For instance, lenvatinib plus pembrolizumab showed potential antitumor activity in patients with advanced gastric cancer or advanced endometrial carcinoma (EC) (71, 72). For advanced gastric cancer, a single-arm phase II trial (EPOC1706, NCT03609359) showed that 20 of 29 (69%) patients treated by lenvatinib plus pembrolizumab had an objective response and no serious treatment-related adverse events occurred (71). For advanced EC, patients treated by lenvatinib plus pembrolizumab had an ORR at 24 weeks of 38.0%, as shown in a phase Ib/II study (NCT02501096) (72). Importantly, in patients with microsatellite instability (MSI)-high tumors, the ORR at 24 weeks was as high as 63.6% (72). In the phase III trial (NCT03517449), patients with advanced EC were assigned to receive either lenvatinib plus pembrolizumab or physician’s choice chemotherapy in a 1:1 ratio (73). Lenvatinib plus pembrolizumab obtained a longer PFS and OS comparing to chemotherapy in all patients (PFS: 7.2 vs 3.8 months, HR=0.56, 95%CI: 0.47-0.66, p<0.001; OS: 18.3 vs 11.4 months, HR=0.62, 95% CI: 0.51-0.75, p<0.001) (73).
The results from a multicenter phase II basket trial (NCT03827837) showed that the camrelizumab plus famitinib exhibited antitumor activity in patients with platinum-resistant ovarian cancer (OC) (74). At the cut-off date, 24.3% of patients achieved CR or PR. The median PFS and OS of this group of patients were 4.1 months and 18.9 months, respectively (74). Sintilimab plus anlotinib was demonstrated efficacy as second-line or later therapy for patients with PD-L1-positive advanced cervical cancer in a phase II trial, with an ORR of 54.8% (23/42) (75). In several phase II trials, camrelizumab and apatinib combination showed potenial anti-tumor activity and acceptable toxicity for the treatment of advanced cervical cancer (76), triple-negative breast cancer (TNBC) (77), extensive-stage small-cell lung cancer (SCLC) (78), and advanced osteosarcoma (79). The results of these studies are warranted to be validated by larger randomized controlled trials (RCTs).
The activity of bevacizumab plus PD-(L)1 inhibitors has been evaluated in the gynecological tumors. A phase II trial (NCT02873962) showed that nivolumab plus bevacizumab had potential efficacy in patients with relapsed ovarian cancer OC, especially in the platinum-sensitive setting (80). However, the data from a phase III trial (NCT03038100) did not support the use of atezolizumab in newly diagnosed OC (81). In the OS(ITT) population, the median PFS was 19.5 months in patients treated with atezolizumab plus bevacizumab and platinum-based chemotherapy versus 18.4 months in patients treated with placebo plus bevacizumab and platinum-based chemotherapy, respectively (HR=0.92, 95%CI: 0.79-1.07, p=0.28) (81). Furthermore, a phase II trial (NCT02921269) showed that bevacizumab plus atezolizumab did not improve the ORR in cervical cancer (82).
Conclusions and future directions
In conclusion, the therapy that dual inhibition of VEGF/VEGFR and PD-(L)1, through modulation of both the tumor vasculature and the TIME, has become a key strategy to treat cancer with promising prospect. Table 1 shows selected phase III trials involving VEGF/VEGFR inhibitors combined with anti-PD-(L)1 agents in solid tumors. In several tumor types, this combination therapy increased patient’s survival and response rate beyond the standard treatment, especially in RCC and HCC. However, not all the tumor types are response to this combination, which may be associated with different TIME in different tumor types (1). The addition of other therapies into this combination may help VEGF/VEGFR inhibitors to enhance PD-(L)1 therapeutic effect. And the tolerable safety profile of this combination supports this hypothesis. Furthermore, VEGF/VEGFR inhibitors plus anti-PD-(L)1 agents can not only be studied in advanced/metastatic disease, but also in the neoadjuvant or adjuvant settings. For example, axitinib plus avelumab as neoadjuvant therapy in patients with non-metastatic RCC has been investigated in a phase II trial (NCT03341845). The preliminary results of this ongoing trial has been reported in the 2022 ASCO Genitourinary Cancers Symposium. For non-metastatic high-risk RCC, neoadjuvant avelumab plus axitinib has been demonstrated to lead to PR of the primary tumor in 30% which is associated with DFS (83).
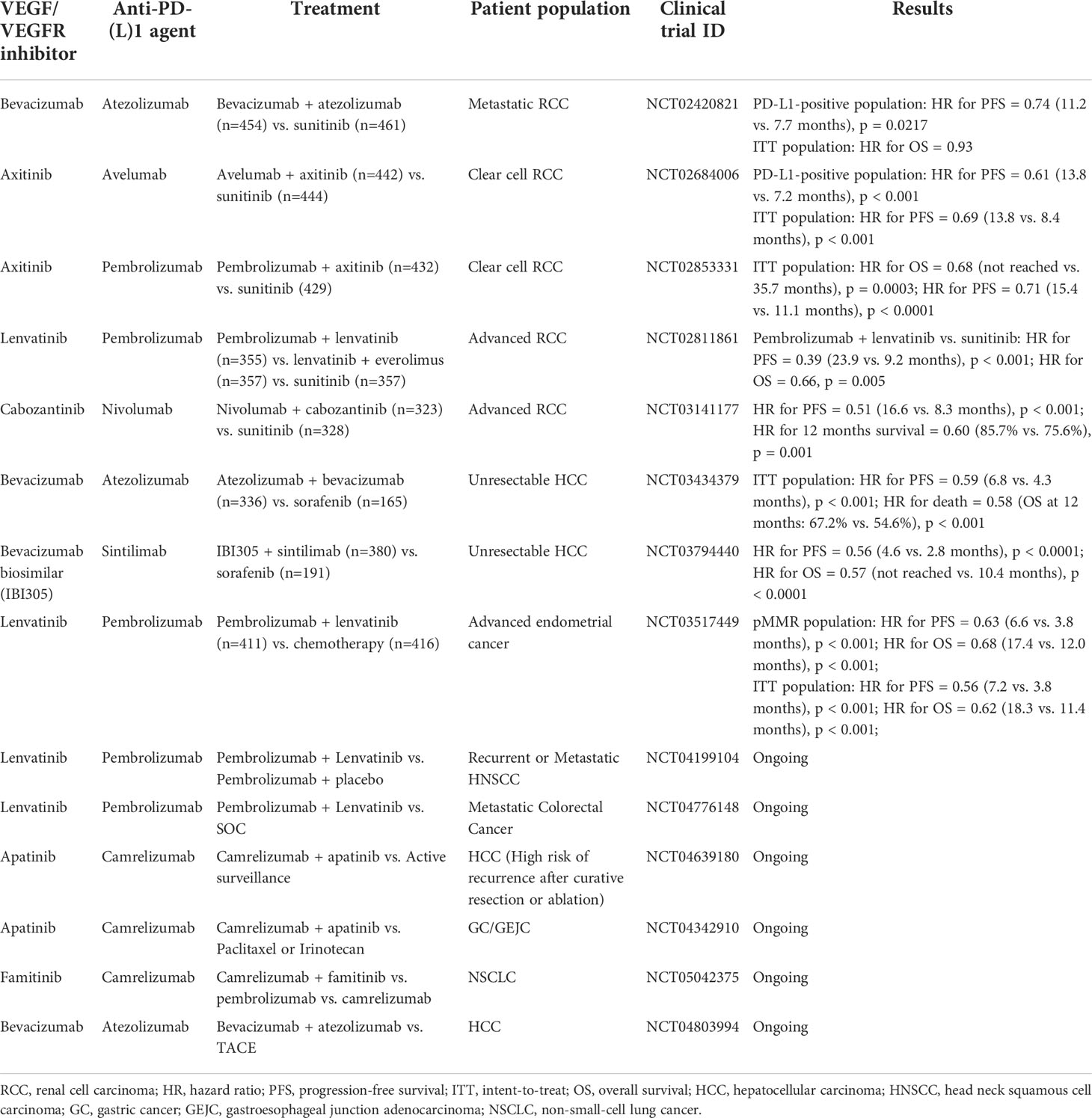
Table 1 Selected phase III clinical trials involving VEGF/VEGFR inhibitors combined with anti-PD-(L)1 agents.
The bispecific antibodies targeting both VEGF/VEGFR and PD-(L)1 pathways is a promising strategy for cancer treatment and is under development. Cui et al. have developed a novel anti-PD-L1/VEGFR bispecific antibody (HB0025) (84). Preclinical data showed that HB0025 could inhibit two pathways concurrently to enhance its anti-cancer activities, supporting further clinical studies (84). Xiong et al. also generated a non-immunogenic, bispecific antibody targeting both VEGF165 and PD-1, serving as a potential anti-tumor agent (85). And the safety and efficacy of another anti-PD-1/VEGF bispecific antibody AK112 are assessed in the several phase I/II trials (NCT04047290, NCT05116007, and NCT04900363). The initial results from study NCT04047290 demonstrated that AK112 had an encouraging anti-tumor activity and a favorable safety profile in patients with platinum-resistant refractory OC (86).
Oral administration offers more convenience to patients. Currently, there are many oral TKIs targeting VEGF/VEGFR available in market, such as lenvatinib, apatinib and famitinib. However, oral immune PD-(L)1 programs are still in their infancy. In comparison with monoclonal antibodies, small-molecule PD-(L)1 inhibitors could overcome several shortcomings of monoclonal antibodies, such as low tumor penetration and high manufacturing costs (87). More importantly, small-molecule PD-(L)1 inhibitors possess the ability to manage immune-related AE due to their shorter pharmacokinetic exposure (88, 89). To our great delight, several oral small-molecule PD-(L)1 inhibitors are under investigation in clinical trials, such as INCB086550 (NCT04629339, NCT04674748 and NCT05101369), CA-170 (NCT02812875), and IMMH-010 (NCT04343859). The combined administration of both VEGF/VEGFR and PD-(L)1 inhibitors orally is worth waiting for.
Clinical trials about the dual inhibition of TGF-β and PD-(L)1
Treatment strategies for the combination of anti-TGF-β and anti-PD-(L)1 agents
The agents inhibit TGF-β pathway usually through the following 3 mechanisms: first, inhibition of TGF-β synthesis directly using antisense molecules such as AP12009; second, blocking TGF-β and its receptors by antibodies or soluble TGF-β decoy receptors (traps) such as fresolimumab (GC1008) and lerdelimumab; third, inhibition of the TGF-β by TKI such as galunisertib (LY2157299) (90).
Currently, several TGF-β inhibitors are under investigation in combination with anti-PD-(L)1 agents. However, the published data are limited. Galunisertib is an oral TKI of type I TGFβ receptor (TGFβRI). Galunisertib plus anti-PD-L1 antibody durvalumab has been assessed in metastatic pancreatic cancer in a phase I trial (NCT02734160) (91). The results showed that galunisertib plus durvalumab had an acceptable tolerability, but clinical activity was limited with an ORR of 3.1% and a DCR of 25.0% (91). The poor efficacy may due to the aggressive nature of pancreatic cancer, as ICIs in pancreatic cancer has shown minimal response in previous studies (91). The safety profile of galunisertib plus durvalumab could lend to this treatment protocol in combination with other treatments in future trials. The combination of galunisertib and anti-PD-1 antibody nivolumab has also been evaluated in a phase I/II trial in patients with solid tumors including recurrent NSCLC and HCC (NCT02423343). The trial has been completed, however, the results have not been published. LY3200882 is a novel, selective TGFβRI inhibitor. Using LY3200882 to inhibit TGFβ and LY3300054 to inhibit PD-L1 has been assessed in solid tumors in a phase I trial (NCT02937272) (92). The efficacy of this combination did not meet the expectations, with an ORR of 7.7% (1/13) and DCR of 39% (5/13) (92). Gemogenovatucel-T is an autologous tumor cell vaccine which specifically decreases the expression of TGF-β1 and TGF-β2 (93). The anti-tumor activity of Gemogenovatucel-T plus aezolizumab (NCT03073525) or durvalumab (NCT02725489) are under investigation in patients with advanced gynecological cancers. SAR439459 is a pan-TGFβ neutralizing antibody (94). In a phase I trial (NCT03192345), the anti-tumor activity of SAR439459, either alone or in combination with PD-1 inhibitor cemiplimab, are under investigation. Vactosertib is a selective small-molecule inhibitor of TGF-βRI (95). Vactosertib plus PD-1 inhibitor pembrolizumab is under investigation in NSCLC (NCT04515979) and metastatic colorectal or gastric cancer (NCT03724851). NIS793 is an anti-TGFβ antibody. The phase I trial (NCT02947165) about the safety of NIS793 as a single agent and in combination with spartalizumab (PDR001, anti-PD-1 antibody) in advanced tumors has been completed. However, the data are not published. Furthermore, the efficacy of NIS793 plus spartalizumab (PDR001) plus SOC in metastatic pancreatic ductal adenocarcinoma (mPDAC) in first-line setting is under investigation in a phase II trial (NCT04390763).
Taken together, most of the clinical trials about anti-TGF-β plus anti-PD-(L)1 agents in solid tumors are still under investigation. From the published data, we could found that this combination provided a manageable safety profile. However, the clinical activity observed with this combination strategy is limited. Some studies have suggested that development of a dual-targeting agent that localize TGF-β inhibition within the TME may be a possible improvement for this situation (12).
Bispecific antibodies against TGF-β and PD-(L)1
Development of bispecific antibody targeting both TGF-β and PD-(L)1 is a hotspot of tumor immunotherapy. Bintrafusp alfa, previously known as M7824, is a bifunctional fusion protein against TGF-βRII and PD-L1 (Figure 1). Preclinical studies showed that bintrafusp alfa reduced TGF-β signaling in TME, resulting in greater tumor volume decrease compared with anti-PD-L1 antibody alone (96). Nineteen patients with advanced solid tumors were treated with bintrafusp alfa in a phase I trial (NCT02517398) (97). In this study, bintrafusp alfa exhibited a manageable safety profile, which was similar to anti-PD-1 monotherapies (97). For efficacy, 1 of 19 patients enrolled experienced a durable CR and 2 patients had a durable PR (97). In the expansion cohort of pretreated advanced NSCLC (NCT02517398), bintrafusp alfa treatment showed an encouraging efficacy with an ORR of 21.3% (17/80) in all patients and 85.7% (6/7) in patients with PD-L1-high (11). In the expansion cohort of heavily pretreated SCCNH (NCT02517398), 4 out of 32 patients received bintrafusp alfa achieved PR (98). Fifty-nine patients with advanced, pretreated human papillomavirus (HPV)-associated cancers (including anal cancer, cervical cancer, and oropharyngeal cancer) from trials of NCT02517398 and NCT03427411, received bintrafusp alfa treatment (99). For full-analysis population, the ORR was 30.5% (including 5 CR) and DCR was 44.1%, supporting further investigation of bintrafusp alfa in such tumor types (99). Several data of a phase I trial conducted in Asian patients (NCT02699515) have also been published. Twenty-three Asian patients (including 9 HCC patients) had received bintrafusp alfa, among them, 8 experienced treatment-related adverse events (TRAEs) (100). In an expansion cohort of advanced gastric/gastroesophageal junction cancer, 31 heavily pretreated patients who received bintrafusp alfa achieved an ORR of 16% and a DCR of 26% (101). Bintrafusp alfa also showed clinical activity in Asian patients with pretreated biliary tract cancer (BTC) in the trial of NCT02699515 (102). The ORR was 20%, with 5 of 6 responses ongoing at the data cut-off. These data prompted progression into phase II and III trials in BTC (NCT03833661 and NCT04066491). Furthermore, the safety profile of bintrafusp alfa shown in two phase I trials (NCT02699515 and NCT02517398) is manageable, with the most common TRAEs of rash maculopapular, pruritus, rash, asthenia, and hypothyroidism. The potential therapeutic effects and safety profile of bintrafusp alfa shown in phase I trials have led to further investigation of bintrafusp alfa.
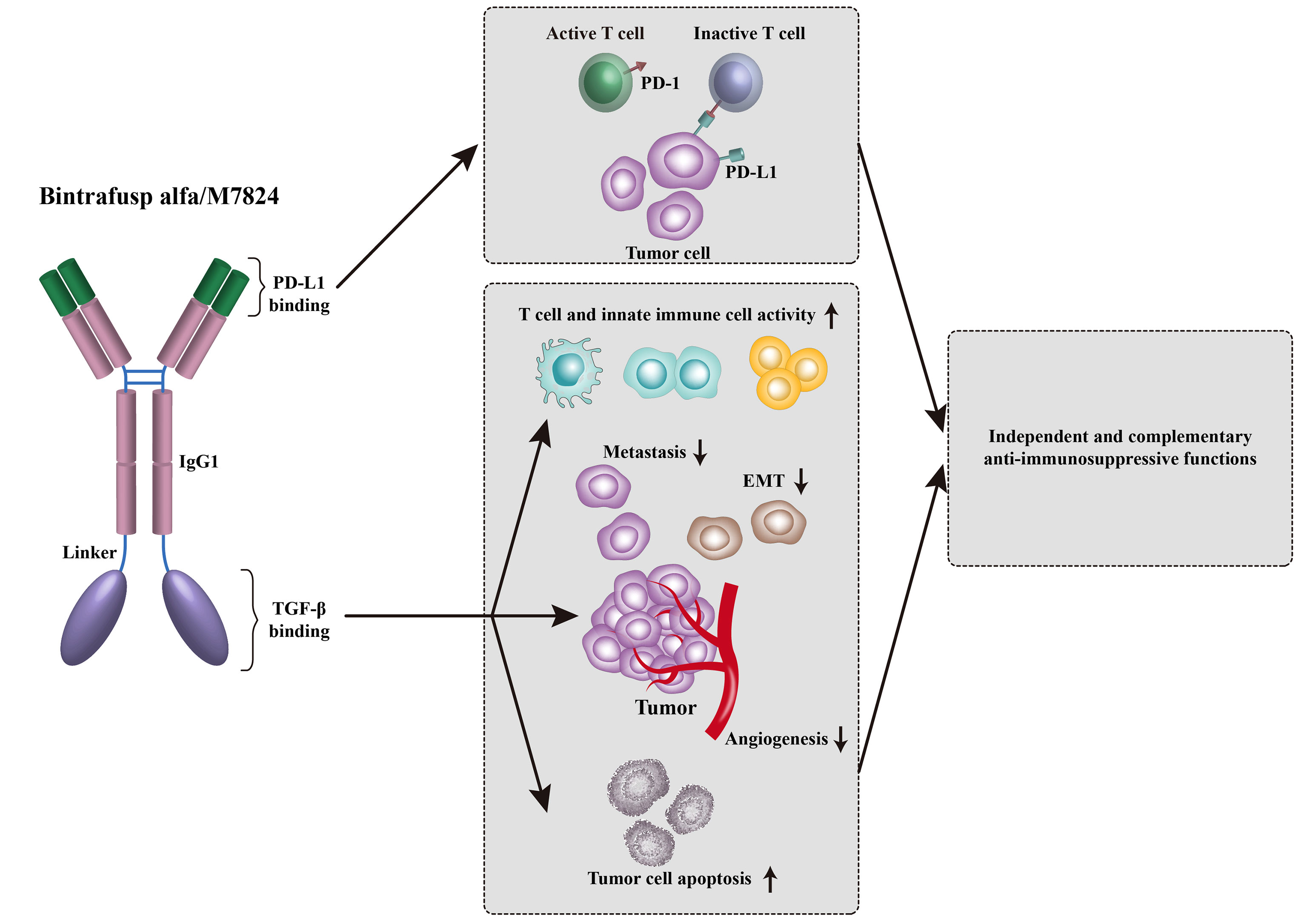
Figure 1 Bintrafusp alfa was designed to simultaneously inhibit PD-1/PD-L1-mediated immunosuppression while decreasing the levels of TGF-β in the TME via a TGF-β ‘trap’ portion.
However, the following phase II and phase III trials failed to meet their primary end points, casting a deep gloom over the development of bintrafusp alfa. In March 2021, the phase II INTR@PID BTC 047 trial (NCT03833661), which examined the efficacy of second-line treatment with bintrafusp alfa for 159 BTC patients, was announced to be failed (103). In August 2021, the phase II/III INTR@PID BTC 055 trial (NCT04066491) was announced to be discontinued based on a review of the data conducted by the Independent Data Monitoring Committee (IDMC), which concluded the trial was unlikely to meet its primary objective (103). In addition, Bintrafusp alfa monotherapy has also shown disappoint results comparing to pembrolizumab in the first-line treatment of patients with NSCLC (NCT03631706) (103). Based on the recommendation of the IDMC, the sponsor decided to discontinue the trial. The disappointed results mentioned above did not lead to the sponsor to give up the development of bintrafusp alfa completely. Currently, over 30 clinical trials about bintrafusp alfa are ongoing or actively recruiting patients according to ClinicalTrials.gov.
Although the disappointed data about bintrafusp alfa in BTC and NSCLC have been revealed, the enthusiasm of other companies to develop TGFβ–PD-(L)1 bifunctional fusion protein have not been reduced. Other PD-(L)1/TGF-β bispecific antibodies, such as SHR-1701, AVID200, TQB2858, TQB2868, PM8001, YM1001 and JS201, are in development. SHR-1701 is a novel bifunctional anti-PD-L1/TGF-βRII agent. The preliminary results of a phase I trial of SHR-1701 (NCT03710265) reported in 2021 ASCO showed that SHR-1701 monotherapy had an acceptable safety profile, no DLT was observed (104). The ORR was 17.8%, with 8 patients achieving PR (104). In the same period, the results from an expansion cohort of a phase I study (NCT03774979) were also reported. SHR-1701 showed encouraging antitumor activity in patients with advanced EGFR-positive NSCLC after failure of at least one line standard EGFR TKI treatment, with an ORR of 16.7% and a DCR of 50.0% (105). SHR-1701 also showed encouraging antitumor activity and controllable safety in patients with pretreated advanced cervical cancer (NCT03774979) (106), pretreated recurrent/refractory (r/r) gastric cancer (NCT03710265) (107), and treatment-naive PD-L1 positive advanced/metastatic NSCLC (NCT03774979) (108). Currently, several clinical trials about SHR-1701 are ongoing. Due to the manageable safety profile of SHR-1701, the combination of SHR-1701 and other therapies has been applied in clinical trials to obtain better clinical efficacy. For example, NCT05020925 is a phase I/II trial to assess the efficacy and safety of SHR-1701 in combination of famitinib in patients with recurrent/metastatic nasopharyngeal carcinoma; NCT05179239 is a phase III trial that evaluates the anti-tumor activity of SHR-1701 or placebo plus chemotherapy with or without BP102 (a bevacizumab biosimilar) patients with recurrent/metastatic cervical cancer. Table 2 summarized the ongoing phase II/III clinical trials of SHR-1701 according to ClinicalTrials.gov.
Conclusions and future directions
TGF-β is an important immune regulator that allows cancer cells to escape immune surveillance (109). Clinical trials have tried to combine anti-TGF-β and anti-PD-(L)1 agents in treating solid tumors. However, the clinical activity observed with this combination strategy is not satisfactory from the published data. A bispecific antibody targeting both pathways, such as bintrafusp alfa and SHR-1701, has the potential for localizing inhibition of TGF-β within the TME, a benefit that is not provided by combining the two independent therapies. The bispecific antibodies show potential therapeutic effects for various tumor types with a manageable safety profile. However, no head-to-head clinical trial has confirmed that the bispecific antibodies against both TGF-β and PD-(L)1 pathways have significant advantages over anti-PD-(L)1 monotherapies or combinations of TGF-β and PD-L1 independent therapies. Furthermore, the biology of TGFβ in TME is not very clear now. Whether targeting TGFβ will enhance the efficacy of cancer immunotherapy should be further confirmed. And better knowing the biology of TGFβ will facilitate the selection of patients that most likely to be benefited from this combination therapy. The manageable safety profile of bispecific antibody against TGF-β and PD-(L)1 allows this therapy plus other modalities such as targeted therapy, chemotherapy and radiation therapy as the SOC potentially in traditionally “cold” tumors. These combinations can not only be studied in advanced/metastatic disease, but also in the adjuvant setting after better knowing the biology of TGFβ.
Clinical trials about the triple inhibition of VEGF/VEGFR, TGF-β and PD-(L)1
It has been reported that TGF-β and VEGF cooperatively control the immunosuppressive TME (13). And the combination of VEGF/VEGFR inhibitors and anti-PD-(L)1 agents or the dual inhibition of TGF-β and PD-(L)1 has shown potential efficacy and manageable safety profile in clinical trials, thus, the triple inhibition of VEGF/VEGFR, TGF-β and PD-(L)1 seems rational. Currently, some clinical trials have attempted to combine PD-(L)1/TGF-β bispecific antibodies and VEGF/VEGFR inhibitors in solid tumors, especially in difficult-to-treat tumors including BTC, pancreatic cancer and SCLC. These studies are all ongoing or in actively recruiting patients status, with a few preliminary results being reported.
The preliminary results of an ongoing exploratory phase II study (ChiCTR2000037927) evaluating the safety and efficacy of SHR-1701 in combination with famitinib in advanced pancreatic cancer and BTC patients who have failed previous standard treatment have been revealed in the 2022 ASCO Gastrointestinal Cancers Symposium (110). Up to 15 Sep 2021, 15 and 9 patients were enrolled in pancreatic cancer and BTC cohorts, respectively. In the pancreatic cancer cohort, the ORR (1 CR and 1 PR) and DCR were 13% and 53%, respectively (110). Among 8 evaluable patients in BTC cohort, the ORR (1 PR) and DCR were 13% and 63%, respectively (110). The most frequently reported TRAEs were proteinuria (58%), hypertension (42%), and blood urine present (42%) (110). These results indicated that HR-1701 plus famitinib had encouraging activity with manageable safety in advanced pancreatic cancer or BTC. SHR-1701 in combination of famitinib in other solid tumors, such as recurrent/metastatic nasopharyngeal carcinoma (NCT05020925), extensive stage SCLC (NCT04884009), advanced or metastatic NSCLC (NCT04699968), are also under investigation. Similarly, SHR-1701 is also attempted to be combined with a bevacizumab biosimilar BP102 in the treatments of solid tumors (NCT04974957, NCT04856787 and NCT05179239).
The efficacy and safety of JS-201, a PD-L1/TGF-β bispecific antibody, combined with lenvatinib in the treatment of SCLC that has failed previous chemotherapy combined with anti-PD-L1 are under investigation in a phase II trial (NCT04951947). TQB2858 is another PD-L1/TGF-β bispecific antibody developed in China. TQB2858 in combination with anlotinib is planned to be evaluated in advanced EC (NCT05121363), recurrent/metastatic nasopharyngeal cancer (NCT05198531) and advanced pancreatic cancer (NCT05193604).
The inhibition of VEGF/VEGFR and TGF-β pathways can overcome microenvironmental resistance to PD-1 blockade (13). Thus, in theory, triple inhibition of VEGF/VEGFR, TGF-β and PD-(L)1 would be a promising strategy to overcome treatment resistance of immunotherapy. Currently, this treatment strategy is under investigation in clinical trials with a bit of data being reported. We await, with interest, more data from the ongoing clinical trials will support the application of this approach in patients with cancers. We also believe that more convenient and effective strategies will be developed in the future to inhibit VEGF/VEGFR, TGF-β and PD-(L)1 pathways synergistically. However, under the condition that the efficacy of the dual inhibition of TGF-β and PD-(L)1 has not been determined, whether triple inhibition of VEGF/VEGFR, TGF-β and PD-(L)1 will have promising efficacy especially in difficult-to-treat tumors is still a problem needed to be solved.
Conclusions
In this review, we described the existing preclinical and clinical evidence supporting the use of anti-VEGF and/or anti-TGF-β therapies to enhance cancer immunotherapy. VEGF/VEGFR and TGF-β pathways play an important role in the development and function of the TME, contributing to the immunosuppressive status of TME. To inhibit VEGF/VEGFR and/or TGF-β pathways can restore TME from immune-suppressive to immune-supportive status and enhance sensitivity to anti-PD-(L)1 treatment. Encouragingly, adopting anti-VEGF and/or anti-TGF-β therapies as a combination treatment with anti-PD-(L)1 therapy have been demonstrated as effective and tolerable in several solid tumors in clinical trials. There is no doubt that anti-VEGF and/or anti-TGF-β therapies have opened a new window for cancer immunotherapy. The clinical value of this combination strategy is worthy to be studied further.
Author contributions
RD designs this review. LL and RD wrote the text and collected related data. QW is responsible for revising the content of the article. All authors contributed to the article and approved the submitted version.
Funding
This work was supported by the Science and Technology Project of the Health Planning Committee of Sichuan Province (No. 21PJ092).
Conflict of interest
The authors declare that the research was conducted in the absence of any commercial or financial relationships that could be construed as a potential conflict of interest.
Publisher’s note
All claims expressed in this article are solely those of the authors and do not necessarily represent those of their affiliated organizations, or those of the publisher, the editors and the reviewers. Any product that may be evaluated in this article, or claim that may be made by its manufacturer, is not guaranteed or endorsed by the publisher.
References
1. Guo F, Cui J. Anti-angiogenesis: Opening a new window for immunotherapy. Life Sci (2020) 258:118163. doi: 10.1016/j.lfs.2020.118163
2. Furukawa K, Nagano T, Tachihara M, Yamamoto M, Nishimura Y. Interaction between immunotherapy and antiangiogenic therapy for cancer. Mol (Basel Switzerland) (2020) 25(17):3900. doi: 10.3390/molecules25173900
3. Daud AI, Wolchok JD, Robert C, Hwu WJ, Weber JS, Ribas A, et al. Programmed death-ligand 1 expression and response to the anti-programmed death 1 antibody pembrolizumab in melanoma. J Clin Oncol (2016) 34(34):4102–9. doi: 10.1200/jco.2016.67.2477
4. Eissler N, Mao Y, Brodin D, Reuterswärd P, Andersson Svahn H, Johnsen JI, et al. Regulation of myeloid cells by activated T cells determines the efficacy of pd-1 blockade. Oncoimmunology (2016) 5(12):e1232222. doi: 10.1080/2162402x.2016.1232222
5. Fukumura D, Kloepper J, Amoozgar Z, Duda DG, Jain RK. Enhancing cancer immunotherapy using antiangiogenics: Opportunities and challenges. Nat Rev Clin Oncol (2018) 15(5):325–40. doi: 10.1038/nrclinonc.2018.29
6. Jain RK. Antiangiogenesis strategies revisited: From starving tumors to alleviating hypoxia. Cancer Cell (2014) 26(5):605–22. doi: 10.1016/j.ccell.2014.10.006
7. Yi M, Jiao D, Qin S, Chu Q, Wu K, Li A. Synergistic effect of immune checkpoint blockade and anti-angiogenesis in cancer treatment. Mol Cancer (2019) 18(1):60. doi: 10.1186/s12943-019-0974-6
8. Wallin JJ, Bendell JC, Funke R, Sznol M, Korski K, Jones S, et al. Atezolizumab in combination with bevacizumab enhances antigen-specific T-cell migration in metastatic renal cell carcinoma. Nat Commun (2016) 7:12624. doi: 10.1038/ncomms12624
9. Choueiri TK, Larkin J, Oya M, Thistlethwaite F, Martignoni M, Nathan P, et al. Preliminary results for avelumab plus axitinib as first-line therapy in patients with advanced clear-cell renal-cell carcinoma (Javelin renal 100): An open-label, dose-finding and dose-expansion, phase 1b trial. Lancet Oncol (2018) 19(4):451–60. doi: 10.1016/s1470-2045(18)30107-4
10. Dahmani A, Delisle JS. Tgf-B in T cell biology: Implications for cancer immunotherapy. Cancers (2018) 10(6):194. doi: 10.3390/cancers10060194
11. Paz-Ares L, Kim TM, Vicente D, Felip E, Lee DH, Lee KH, et al. Bintrafusp Alfa, a bifunctional fusion protein targeting tgf-B and pd-L1, in second-line treatment of patients with nsclc: Results from an expansion cohort of a phase 1 trial. J Thorac Oncol (2020) 15(7):1210–22. doi: 10.1016/j.jtho.2020.03.003
12. Gulley JL, Schlom J, Barcellos-Hoff MH, Wang XJ, Seoane J, Audhuy F, et al. Dual inhibition of tgf-B and pd-L1: A novel approach to cancer treatment. Mol Oncol (2022) 16(11):2117–34. doi: 10.1002/1878-0261.13146
13. Courau T, Nehar-Belaid D, Florez L, Levacher B, Vazquez T, Brimaud F, et al. Tgf-B and vegf cooperatively control the immunotolerant tumor environment and the efficacy of cancer immunotherapies. JCI Insight (2016) 1(9):e85974. doi: 10.1172/jci.insight.85974
14. Ravi R, Noonan KA, Pham V, Bedi R, Zhavoronkov A, Ozerov IV, et al. Bifunctional immune checkpoint-targeted antibody-ligand traps that simultaneously disable tgfβ enhance the efficacy of cancer immunotherapy. Nat Commun (2018) 9(1):741. doi: 10.1038/s41467-017-02696-6
15. Ferris RL, Lenz HJ, Trotta AM, García-Foncillas J, Schulten J, Audhuy F, et al. Rationale for combination of therapeutic antibodies targeting tumor cells and immune checkpoint receptors: Harnessing innate and adaptive immunity through Igg1 isotype immune effector stimulation. Cancer Treat Rev (2018) 63:48–60. doi: 10.1016/j.ctrv.2017.11.008
16. Wilson TJ, Nannuru KC, Futakuchi M, Singh RK. Cathepsin G-mediated enhanced tgf-beta signaling promotes angiogenesis Via upregulation of vegf and mcp-1. Cancer Lett (2010) 288(2):162–9. doi: 10.1016/j.canlet.2009.06.035
17. Sadeghzadeh M, Bornehdeli S, Mohahammadrezakhani H, Abolghasemi M, Poursaei E, Asadi M, et al. Dendritic cell therapy in cancer treatment; the state-of-the-Art. Life Sci (2020) 254:117580. doi: 10.1016/j.lfs.2020.117580
18. Oyama T, Ran S, Ishida T, Nadaf S, Kerr L, Carbone DP, et al. Vascular endothelial growth factor affects dendritic cell maturation through the inhibition of nuclear factor-kappa b activation in hemopoietic progenitor cells. J Immunol (Baltimore Md 1950) (1998) 160(3):1224–32.
19. Alfaro C, Suarez N, Gonzalez A, Solano S, Erro L, Dubrot J, et al. Influence of bevacizumab, sunitinib and sorafenib as single agents or in combination on the inhibitory effects of vegf on human dendritic cell differentiation from monocytes. Br J Cancer (2009) 100(7):1111–9. doi: 10.1038/sj.bjc.6604965
20. Curiel TJ, Wei S, Dong H, Alvarez X, Cheng P, Mottram P, et al. Blockade of B7-H1 improves myeloid dendritic cell-mediated antitumor immunity. Nat Med (2003) 9(5):562–7. doi: 10.1038/nm863
21. Ohm JE, Gabrilovich DI, Sempowski GD, Kisseleva E, Parman KS, Nadaf S, et al. Vegf inhibits T-cell development and may contribute to tumor-induced immune suppression. Blood (2003) 101(12):4878–86. doi: 10.1182/blood-2002-07-1956
22. Voron T, Colussi O, Marcheteau E, Pernot S, Nizard M, Pointet AL, et al. Vegf-a modulates expression of inhibitory checkpoints on Cd8+ T cells in tumors. J Exp Med (2015) 212(2):139–48. doi: 10.1084/jem.20140559
23. de Almeida PE, Mak J, Hernandez G, Jesudason R, Herault A, Javinal V, et al. Anti-vegf treatment enhances Cd8(+) T-cell antitumor activity by amplifying hypoxia. Cancer Immunol Res (2020) 8(6):806–18. doi: 10.1158/2326-6066.cir-19-0360
24. Tada Y, Togashi Y, Kotani D, Kuwata T, Sato E, Kawazoe A, et al. Targeting Vegfr2 with ramucirumab strongly impacts effector/ activated regulatory T cells and Cd8(+) T cells in the tumor microenvironment. J Immunother Cancer (2018) 6(1):106. doi: 10.1186/s40425-018-0403-1
25. Ning H, Shao QQ, Ding KJ, Gao DX, Lu QL, Cao QW, et al. Tumor-infiltrating regulatory T cells are positively correlated with angiogenic status in renal cell carcinoma. Chin Med J (2012) 125(12):2120–5.
26. Terme M, Pernot S, Marcheteau E, Sandoval F, Benhamouda N, Colussi O, et al. Vegfa-vegfr pathway blockade inhibits tumor-induced regulatory T-cell proliferation in colorectal cancer. Cancer Res (2013) 73(2):539–49. doi: 10.1158/0008-5472.can-12-2325
27. Khan KA, Kerbel RS. Improving immunotherapy outcomes with anti-angiogenic treatments and vice versa. Nat Rev Clin Oncol (2018) 15(5):310–24. doi: 10.1038/nrclinonc.2018.9
28. Rujirachotiwat A, Suttamanatwong S. Curcumin upregulates transforming growth factor-B1, its receptors, and vascular endothelial growth factor expressions in an in vitro human gingival fibroblast wound healing model. BMC Oral Health (2021) 21(1):535. doi: 10.1186/s12903-021-01890-9
29. van den Bulk J, de Miranda N, Ten Dijke P. Therapeutic targeting of tgf-B in cancer: Hacking a master switch of immune suppression. Clin Sci (London Engl 1979) (2021) 135(1):35–52. doi: 10.1042/cs20201236
30. Roncarolo MG, Levings MK, Traversari C. Differentiation of T regulatory cells by immature dendritic cells. J Exp Med (2001) 193(2):F5–9. doi: 10.1084/jem.193.2.f5
31. Esebanmen GE, Langridge WHR. The role of tgf-beta signaling in dendritic cell tolerance. Immunol Res (2017) 65(5):987–94. doi: 10.1007/s12026-017-8944-9
32. Marcoe JP, Lim JR, Schaubert KL, Fodil-Cornu N, Matka M, McCubbrey AL, et al. Tgf-B is responsible for nk cell immaturity during ontogeny and increased susceptibility to infection during mouse infancy. Nat Immunol (2012) 13(9):843–50. doi: 10.1038/ni.2388
33. Viel S, Marçais A, Guimaraes FS, Loftus R, Rabilloud J, Grau M, et al. Tgf-B inhibits the activation and functions of nk cells by repressing the mtor pathway. Sci Signaling (2016) 9(415):ra19. doi: 10.1126/scisignal.aad1884
34. Gonzalez-Junca A, Driscoll KE, Pellicciotta I, Du S, Lo CH, Roy R, et al. Autocrine tgfβ is a survival factor for monocytes and drives immunosuppressive lineage commitment. Cancer Immunol Res (2019) 7(2):306–20. doi: 10.1158/2326-6066.cir-18-0310
35. Batlle E, Massagué J. Transforming growth factor-B signaling in immunity and cancer. Immunity (2019) 50(4):924–40. doi: 10.1016/j.immuni.2019.03.024
36. Eshima K, Misawa K, Ohashi C, Iwabuchi K. Role of T-bet, the master regulator of Th1 cells, in the cytotoxicity of murine Cd4(+) T cells. Microbiol Immunol (2018) 62(5):348–56. doi: 10.1111/1348-0421.12586
37. Thomas DA, Massagué J. Tgf-beta directly targets cytotoxic T cell functions during tumor evasion of immune surveillance. Cancer Cell (2005) 8(5):369–80. doi: 10.1016/j.ccr.2005.10.012
38. Donkor MK, Sarkar A, Savage PA, Franklin RA, Johnson LK, Jungbluth AA, et al. T Cell surveillance of oncogene-induced prostate cancer is impeded by T cell-derived tgf-B1 cytokine. Immunity (2011) 35(1):123–34. doi: 10.1016/j.immuni.2011.04.019
39. Ahmadzadeh M, Rosenberg SA. Tgf-beta 1 attenuates the acquisition and expression of effector function by tumor antigen-specific human memory Cd8 T cells. J Immunol (Baltimore Md 1950) (2005) 174(9):5215–23. doi: 10.4049/jimmunol.174.9.5215
40. Moo-Young TA, Larson JW, Belt BA, Tan MC, Hawkins WG, Eberlein TJ, et al. Tumor-derived tgf-beta mediates conversion of Cd4+Foxp3+ regulatory T cells in a murine model of pancreas cancer. J Immunother (Hagerstown Md 1997) (2009) 32(1):12–21. doi: 10.1097/CJI.0b013e318189f13c
41. Yasuda S, Sho M, Yamato I, Yoshiji H, Wakatsuki K, Nishiwada S, et al. Simultaneous blockade of programmed death 1 and vascular endothelial growth factor receptor 2 (Vegfr2) induces synergistic anti-tumour effect in vivo. Clin Exp Immunol (2013) 172(3):500–6. doi: 10.1111/cei.12069
42. Meder L, Schuldt P, Thelen M, Schmitt A, Dietlein F, Klein S, et al. Combined vegf and pd-L1 blockade displays synergistic treatment effects in an autochthonous mouse model of small cell lung cancer. Cancer Res (2018) 78(15):4270–81. doi: 10.1158/0008-5472.can-17-2176
43. Wu FTH, Xu P, Chow A, Man S, Krüger J, Khan KA, et al. Pre- and post-operative anti-Pd-L1 plus anti-angiogenic therapies in mouse breast or renal cancer models of micro- or macro-metastatic disease. Br J Cancer (2019) 120(2):196–206. doi: 10.1038/s41416-018-0297-1
44. Mariathasan S, Turley SJ, Nickles D, Castiglioni A, Yuen K, Wang Y, et al. Tgfβ attenuates tumour response to pd-L1 blockade by contributing to exclusion of T cells. Nature (2018) 554(7693):544–8. doi: 10.1038/nature25501
45. Holderfield MT, Hughes CC. Crosstalk between vascular endothelial growth factor, notch, and transforming growth factor-beta in vascular morphogenesis. Circ Res (2008) 102(6):637–52. doi: 10.1161/circresaha.107.167171
46. Ferrari G, Cook BD, Terushkin V, Pintucci G, Mignatti P. Transforming growth factor-beta 1 (Tgf-Beta1) induces angiogenesis through vascular endothelial growth factor (Vegf)-mediated apoptosis. J Cell Physiol (2009) 219(2):449–58. doi: 10.1002/jcp.21706
47. Chung JY, Chan MK, Li JS, Chan AS, Tang PC, Leung KT, et al. Tgf-B signaling: From tissue fibrosis to tumor microenvironment. Int J Mol Sci (2021) 22(14):7575. doi: 10.3390/ijms22147575
48. Rini BI, Powles T, Atkins MB, Escudier B, McDermott DF, Suarez C, et al. Atezolizumab plus bevacizumab versus sunitinib in patients with previously untreated metastatic renal cell carcinoma (Immotion151): A multicentre, open-label, phase 3, randomised controlled trial. Lancet (London England) (2019) 393(10189):2404–15. doi: 10.1016/s0140-6736(19)30723-8
49. Dudek AZ, Liu LC, Gupta S, Logan TF, Singer EA, Joshi M, et al. Phase Ib/Ii clinical trial of pembrolizumab with bevacizumab for metastatic renal cell carcinoma: Btcrc-Gu14-003. J Clin Oncol (2020) 38(11):1138–45. doi: 10.1200/jco.19.02394
50. Gross-Goupil M, Kwon TG, Eto M, Ye D, Miyake H, Seo SI, et al. Axitinib versus placebo as an adjuvant treatment of renal cell carcinoma: Results from the phase iii, randomized atlas trial. Ann Oncol (2018) 29(12):2371–8. doi: 10.1093/annonc/mdy454
51. Atkins MB, Plimack ER, Puzanov I, Fishman MN, McDermott DF, Cho DC, et al. Axitinib in combination with pembrolizumab in patients with advanced renal cell cancer: A non-randomised, open-label, dose-finding, and dose-expansion phase 1b trial. Lancet Oncol (2018) 19(3):405–15. doi: 10.1016/s1470-2045(18)30081-0
52. Motzer RJ, Penkov K, Haanen J, Rini B, Albiges L, Campbell MT, et al. Avelumab plus axitinib versus sunitinib for advanced renal-cell carcinoma. New Engl J Med (2019) 380(12):1103–15. doi: 10.1056/NEJMoa1816047
53. Chau V, Bilusic M. Pembrolizumab in combination with axitinib as first-line treatment for patients with renal cell carcinoma (Rcc): Evidence to date. Cancer Manage Res (2020) 12:7321–30. doi: 10.2147/cmar.s216605
54. Tohyama O, Matsui J, Kodama K, Hata-Sugi N, Kimura T, Okamoto K, et al. Antitumor activity of lenvatinib (E7080): An angiogenesis inhibitor that targets multiple receptor tyrosine kinases in preclinical human thyroid cancer models. J Thyroid Res (2014) 2014:638747. doi: 10.1155/2014/638747
55. Taylor MH, Lee CH, Makker V, Rasco D, Dutcus CE, Wu J, et al. Phase Ib/Ii trial of lenvatinib plus pembrolizumab in patients with advanced renal cell carcinoma, endometrial cancer, and other selected advanced solid tumors. J Clin Oncol (2020) 38(11):1154–63. doi: 10.1200/jco.19.01598
56. Motzer R, Alekseev B, Rha SY, Porta C, Eto M, Powles T, et al. Lenvatinib plus pembrolizumab or everolimus for advanced renal cell carcinoma. New Engl J Med (2021) 384(14):1289–300. doi: 10.1056/NEJMoa2035716
57. Choueiri TK, Powles T, Burotto M, Escudier B, Bourlon MT, Zurawski B, et al. Nivolumab plus cabozantinib versus sunitinib for advanced renal-cell carcinoma. New Engl J Med (2021) 384(9):829–41. doi: 10.1056/NEJMoa2026982
58. Pal SK, McGregor B, Suárez C, Tsao CK, Kelly W, Vaishampayan U, et al. Cabozantinib in combination with atezolizumab for advanced renal cell carcinoma: Results from the cosmic-021 study. J Clin Oncol (2021) 39(33):3725–36. doi: 10.1200/jco.21.00939
59. Qu YY, Zhang HL, Guo H, Luo H, Zou Q, Xing N, et al. Camrelizumab plus famitinib in patients with advanced or metastatic renal cell carcinoma: Data from an open-label, multicenter phase ii basket study. Clin Cancer Res (2021) 27(21):5838–46. doi: 10.1158/1078-0432.ccr-21-1698
60. Amin A, Plimack ER, Ernstoff MS, Lewis LD, Bauer TM, McDermott DF, et al. Safety and efficacy of nivolumab in combination with sunitinib or pazopanib in advanced or metastatic renal cell carcinoma: The checkmate 016 study. J Immunother Cancer (2018) 6(1):109. doi: 10.1186/s40425-018-0420-0
61. Chowdhury S, Infante JR, Hawkins R, Voss MH, Perini R, Arkenau T, et al. A phase I/Ii study to assess the safety and efficacy of pazopanib and pembrolizumab combination therapy in patients with advanced renal cell carcinoma. Clin Genitourinary Cancer (2021) 19(5):434–46. doi: 10.1016/j.clgc.2021.04.007
62. Socinski MA, Jotte RM, Cappuzzo F, Orlandi F, Stroyakovskiy D, Nogami N, et al. Atezolizumab for first-line treatment of metastatic nonsquamous nsclc. New Engl J Med (2018) 378(24):2288–301. doi: 10.1056/NEJMoa1716948
63. Herbst RS, Arkenau HT, Bendell J, Arrowsmith E, Wermke M, Soriano A, et al. Phase 1 expansion cohort of ramucirumab plus pembrolizumab in advanced treatment-naive nsclc. J Thorac Oncol (2021) 16(2):289–98. doi: 10.1016/j.jtho.2020.10.004
64. Bang YJ, Golan T, Dahan L, Fu S, Moreno V, Park K, et al. Ramucirumab and durvalumab for previously treated, advanced non-Small-Cell lung cancer, Gastric/Gastro-oesophageal junction adenocarcinoma, or hepatocellular carcinoma: An open-label, phase Ia/B study (Jvdj). Eur J Cancer (Oxford Engl 1990) (2020) 137:272–84. doi: 10.1016/j.ejca.2020.06.007
65. Chu T, Zhong R, Zhong H, Zhang B, Zhang W, Shi C, et al. Phase 1b study of sintilimab plus anlotinib as first-line therapy in patients with advanced nsclc. J Thorac Oncol Off Publ Int Assoc Study Lung Cancer (2021) 16(4):643–52. doi: 10.1016/j.jtho.2020.11.026
66. Zhou N, Jiang M, Li T, Zhu J, Liu K, Hou H, et al. Anlotinib combined with anti-Pd-1 antibody, camrelizumab for advanced nsclcs after multiple lines treatment: An open-label, dose escalation and expansion study. Lung Cancer (Amsterdam Netherlands) (2021) 160:111–7. doi: 10.1016/j.lungcan.2021.08.006
67. Finn RS, Qin S, Ikeda M, Galle PR, Ducreux M, Kim TY, et al. Atezolizumab plus bevacizumab in unresectable hepatocellular carcinoma. New Engl J Med (2020) 382(20):1894–905. doi: 10.1056/NEJMoa1915745
68. Ren Z, Xu J, Bai Y, Xu A, Cang S, Du C, et al. Sintilimab plus a bevacizumab biosimilar (Ibi305) versus sorafenib in unresectable hepatocellular carcinoma (Orient-32): A randomised, open-label, phase 2-3 study. Lancet Oncol (2021) 22(7):977–90. doi: 10.1016/s1470-2045(21)00252-7
69. Finn RS, Ikeda M, Zhu AX, Sung MW, Baron AD, Kudo M, et al. Phase ib study of lenvatinib plus pembrolizumab in patients with unresectable hepatocellular carcinoma. J Clin Oncol (2020) 38(26):2960–70. doi: 10.1200/jco.20.00808
70. Xu J, Shen J, Gu S, Zhang Y, Wu L, Wu J, et al. Camrelizumab in combination with apatinib in patients with advanced hepatocellular carcinoma (Rescue): A nonrandomized, open-label, phase ii trial. Clin Cancer Res (2021) 27(4):1003–11. doi: 10.1158/1078-0432.ccr-20-2571
71. Kawazoe A, Fukuoka S, Nakamura Y, Kuboki Y, Wakabayashi M, Nomura S, et al. Lenvatinib plus pembrolizumab in patients with advanced gastric cancer in the first-line or second-line setting (Epoc1706): An open-label, single-arm, phase 2 trial. Lancet Oncol (2020) 21(8):1057–65. doi: 10.1016/s1470-2045(20)30271-0
72. Makker V, Taylor MH, Aghajanian C, Oaknin A, Mier J, Cohn AL, et al. Lenvatinib plus pembrolizumab in patients with advanced endometrial cancer. J Clin Oncol Off J Am Soc Clin Oncol (2020) 38(26):2981–92. doi: 10.1200/jco.19.02627
73. Makker V, Colombo N, Casado Herráez A, Santin AD, Colomba E, Miller DS, et al. Lenvatinib plus pembrolizumab for advanced endometrial cancer. New Engl J Med (2022) 386(5):437–48. doi: 10.1056/NEJMoa2108330
74. Xia L, Peng J, Lou G, Pan M, Zhou Q, Hu W, et al. Antitumor activity and safety of camrelizumab plus famitinib in patients with platinum-resistant recurrent ovarian cancer: Results from an open-label, multicenter phase 2 basket study. J Immunother Cancer (2022) 10(1):e003831. doi: 10.1136/jitc-2021-003831
75. Xu Q, Wang J, Sun Y, Lin Y, Liu J, Zhuo Y, et al. Efficacy and safety of sintilimab plus anlotinib for pd-L1-Positive recurrent or metastatic cervical cancer: A multicenter, single-arm, prospective phase ii trial. J Clin Oncol (2022) 40(16):1795–805. doi: 10.1200/jco.21.02091
76. Lan C, Shen J, Wang Y, Li J, Liu Z, He M, et al. Camrelizumab plus apatinib in patients with advanced cervical cancer (Clap): A multicenter, open-label, single-arm, phase ii trial. J Clin Oncol (2020) 38(34):4095–106. doi: 10.1200/jco.20.01920
77. Liu J, Liu Q, Li Y, Li Q, Su F, Yao H, et al. Efficacy and safety of camrelizumab combined with apatinib in advanced triple-negative breast cancer: An open-label phase ii trial. J Immunother Cancer (2020) 8(1):e000696. doi: 10.1136/jitc-2020-000696
78. Fan Y, Zhao J, Wang Q, Huang D, Li X, Chen J, et al. Camrelizumab plus apatinib in extensive-stage sclc (Passion): A multicenter, two-stage, phase 2 trial. J Thorac Oncol Off Publ Int Assoc Study Lung Cancer (2021) 16(2):299–309. doi: 10.1016/j.jtho.2020.10.002
79. Xie L, Xu J, Sun X, Guo W, Gu J, Liu K, et al. Apatinib plus camrelizumab (Anti-Pd1 therapy, shr-1210) for advanced osteosarcoma (Apfao) progressing after chemotherapy: A single-arm, open-label, phase 2 trial. J Immunother Cancer (2020) 8(1):e000798. doi: 10.1136/jitc-2020-000798
80. Liu JF, Herold C, Gray KP, Penson RT, Horowitz N, Konstantinopoulos PA, et al. Assessment of combined nivolumab and bevacizumab in relapsed ovarian cancer: A phase 2 clinical trial. JAMA Oncol (2019) 5(12):1731–8. doi: 10.1001/jamaoncol.2019.3343
81. Moore KN, Bookman M, Sehouli J, Miller A, Anderson C, Scambia G, et al. Atezolizumab, bevacizumab, and chemotherapy for newly diagnosed stage iii or iv ovarian cancer: Placebo-controlled randomized phase iii trial (Imagyn050/Gog 3015/Engot-Ov39). J Clin Oncol (2021) 39(17):1842–55. doi: 10.1200/jco.21.00306
82. Friedman CF, Snyder Charen A, Zhou Q, Carducci MA, Buckley De Meritens A, Corr BR, et al. Phase ii study of atezolizumab in combination with bevacizumab in patients with advanced cervical cancer. J Immunother Cancer (2020) 8(2):e001126. doi: 10.1136/jitc-2020-001126
83. Bex A, Abu-Ghanem Y, Thienen JVV, Graafland N, Lagerveld B, Zondervan P, et al. Efficacy, safety, and biomarker analysis of neoadjuvant Avelumab/Axitinib in patients (Pts) with localized renal cell carcinoma (Rcc) who are at high risk of relapse after nephrectomy (Neoavax). J Clin Oncol (2022) 40(6_suppl):289–. doi: 10.1200/JCO.2022.40.6_suppl.289
84. Cui X, Jia H, Xin H, Zhang L, Chen S, Xia S, et al. A novel bispecific antibody targeting pd-L1 and vegf with combined anti-tumor activities. Front Immunol (2021) 12:778978. doi: 10.3389/fimmu.2021.778978
85. Xiong C, Mao Y, Wu T, Kang N, Zhao M, Di R, et al. Optimized expression and characterization of a novel fully human bispecific single-chain diabody targeting vascular endothelial growth Factor165 and programmed death-1 in pichia pastoris and evaluation of antitumor activity in vivo. Int J Mol Sci (2018) 19(10):2900. doi: 10.3390/ijms19102900
86. Coward J, Frentzas S, Mislang A, Gao B, Lemech C, Jin X, et al. 427 efficacy and safety of Ak112, an anti-Pd-1/Vegf-a bispecific antibody, in patients with platinum-Resistant/Refractory epithelial ovarian cancer in a phase 1 study. J Immunother Cancer (2021) 9(Suppl 2):A457–A. doi: 10.1136/jitc-2021-SITC2021.427
87. Wu X, Meng Y, Liu L, Gong G, Zhang H, Hou Y, et al. Insights into non-peptide small-molecule inhibitors of the pd-1/Pd-L1 interaction: Development and perspective. Bioorg Med Chem (2021) 33:116038. doi: 10.1016/j.bmc.2021.116038
88. Sasikumar PG, Sudarshan NS, Adurthi S, Ramachandra RK, Samiulla DS, Lakshminarasimhan A, et al. Pd-1 derived Ca-170 is an oral immune checkpoint inhibitor that exhibits preclinical anti-tumor efficacy. Commun Biol (2021) 4(1):699. doi: 10.1038/s42003-021-02191-1
89. Sasikumar PG, Ramachandra M. Small-molecule immune checkpoint inhibitors targeting pd-1/Pd-L1 and other emerging checkpoint pathways. BioDrugs Clin Immunother Biopharmaceut Gene Ther (2018) 32(5):481–97. doi: 10.1007/s40259-018-0303-4
90. Haque S, Morris JC. Transforming growth factor-B: A therapeutic target for cancer. Hum Vaccines Immunother (2017) 13(8):1741–50. doi: 10.1080/21645515.2017.1327107
91. Melisi D, Oh DY, Hollebecque A, Calvo E, Varghese A, Borazanci E, et al. Safety and activity of the tgfβ receptor I kinase inhibitor galunisertib plus the anti-Pd-L1 antibody durvalumab in metastatic pancreatic cancer. J Immunother Cancer (2021) 9(3):e002068. doi: 10.1136/jitc-2020-002068
92. Yap TA, Vieito M, Baldini C, Sepúlveda-Sánchez JM, Kondo S, Simonelli M, et al. First-in-Human phase I study of a next-generation, oral, tgfβ receptor 1 inhibitor, Ly3200882, in patients with advanced cancer. Clin Cancer Res an Off J Am Assoc Cancer Res (2021) 27(24):6666–76. doi: 10.1158/1078-0432.ccr-21-1504
93. Rocconi RP, Grosen EA, Ghamande SA, Chan JK, Barve MA, Oh J, et al. Gemogenovatucel-T (Vigil) immunotherapy as maintenance in frontline stage Iii/Iv ovarian cancer (Vital): A randomised, double-blind, placebo-controlled, phase 2b trial. Lancet Oncol (2020) 21(12):1661–72. doi: 10.1016/s1470-2045(20)30533-7
94. Greco R, Qu H, Qu H, Theilhaber J, Shapiro G, Gregory R, et al. Pan-tgfβ inhibition by Sar439459 relieves immunosuppression and improves antitumor efficacy of pd-1 blockade. Oncoimmunology (2020) 9(1):1811605. doi: 10.1080/2162402x.2020.1811605
95. Jung SY, Yug JS, Clarke JM, Bauer TM, Keedy VL, Hwang S, et al. Population pharmacokinetics of vactosertib, a new tgf-B receptor type I inhibitor, in patients with advanced solid tumors. Cancer Chemother Pharmacol (2020) 85(1):173–83. doi: 10.1007/s00280-019-03979-z
96. Lind H, Gameiro SR, Jochems C, Donahue RN, Strauss J, Gulley JM, et al. Dual targeting of tgf-B and pd-L1 Via a bifunctional anti-Pd-L1/Tgf-Brii agent: Status of preclinical and clinical advances. J Immunother Cancer (2020) 8(1):e000433. doi: 10.1136/jitc-2019-000433
97. Strauss J, Heery CR, Schlom J, Madan RA, Cao L, Kang Z, et al. Phase I trial of M7824 (Msb0011359c), a bifunctional fusion protein targeting pd-L1 and tgfβ, in advanced solid tumors. Clin Cancer Res (2018) 24(6):1287–95. doi: 10.1158/1078-0432.ccr-17-2653
98. Cho BC, Daste A, Ravaud A, Salas S, Isambert N, McClay E, et al. Bintrafusp Alfa, a bifunctional fusion protein targeting tgf-B and pd-L1, in advanced squamous cell carcinoma of the head and neck: Results from a phase I cohort. J Immunother Cancer (2020) 8(2):e000664. doi: 10.1136/jitc-2020-000664
99. Strauss J, Gatti-Mays ME, Cho BC, Hill A, Salas S, McClay E, et al. Bintrafusp Alfa, a bifunctional fusion protein targeting tgf-B and pd-L1, in patients with human papillomavirus-associated malignancies. J Immunother Cancer (2020) 8(2):e001395. doi: 10.1136/jitc-2020-001395
100. Doi T, Fujiwara Y, Koyama T, Ikeda M, Helwig C, Watanabe M, et al. Phase I study of the bifunctional fusion protein bintrafusp Alfa in Asian patients with advanced solid tumors, including a hepatocellular carcinoma safety-assessment cohort. Oncol (2020) 25(9):e1292–e302. doi: 10.1634/theoncologist.2020-0249
101. Kang YK, Bang YJ, Kondo S, Chung HC, Muro K, Dussault I, et al. Safety and tolerability of bintrafusp Alfa, a bifunctional fusion protein targeting tgfβ and pd-L1, in Asian patients with pretreated recurrent or refractory gastric cancer. Clin Cancer Res an Off J Am Assoc Cancer Res (2020) 26(13):3202–10. doi: 10.1158/1078-0432.ccr-19-3806
102. Yoo C, Oh DY, Choi HJ, Kudo M, Ueno M, Kondo S, et al. Phase I study of bintrafusp Alfa, a bifunctional fusion protein targeting tgf-B and pd-L1, in patients with pretreated biliary tract cancer. J Immunother Cancer (2020) 8(1):e000564. doi: 10.1136/jitc-2020-000564
103. Lartigue DJ. New players join quest for a therapeutic foothold against tgfβ (2022). Available at: www.onclive.com/view/new-players-join-quest-for-a-therapeutic-foothold-against-tgf (Accessed January 3, 2022).
104. Liu D, Gong J, Liu T, Li K, Yin X, Liu Y, et al. Phase 1 study of shr-1701, a bifunctional fusion protein targeting pd-L1 and tgf-B, in patients with advanced solid tumors. J Clin Oncol (2021) 39(15_suppl):2503–. doi: 10.1200/JCO.2021.39.15_suppl.2503
105. Shi M, Chen J, Li K, Fang Y, Wen G, Li X, et al. Shr-1701, a bifunctional fusion protein targeting pd-L1 and tgf-B, for advanced nsclc with egfr mutations: Data from a multicenter phase 1 study. J Clin Oncol (2021) 39(15_suppl):9055–. doi: 10.1200/JCO.2021.39.15_suppl.9055
106. Feng J, Wang J, Tang D, Zhou Q, Peng J, Lou H, et al. 776p shr-1701, a bifunctional fusion protein targeting pd-L1 and tgf-B, for pretreated advanced cervical cancer: Data from a clinical expansion cohort of a phase I study. Ann Oncol (2021) 32:S753. doi: 10.1016/j.annonc.2021.08.1218
107. Liu D, Luo S, Li M, Liu T, Ge M, Wang Y, et al. 1375p shr-1701, a novel bifunctional anti-Pd-L1/Tgf-βRii agent, for pretreated Recurrent/Refractory (R/R) gastric cancer (Gc): Data from a first-in-Human phase I study. Ann Oncol (2021) 32:S1042. doi: 10.1016/j.annonc.2021.08.1484
108. Feng J, Chen J, Li K, Li X, Min X, Li B, et al. 1278p shr-1701, a bifunctional fusion protein targeting pd-L1 and tgf-B, as first-line therapy for pd-L1+ Advanced/Metastatic nsclc: Data from a clinical expansion cohort of a phase I study. Ann Oncol (2021) 32:S995. doi: 10.1016/j.annonc.2021.08.1880
109. Larson C, Oronsky B, Carter CA, Oronsky A, Knox SJ, Sher D, et al. Tgf-beta: A master immune regulator. Expert Opin Ther Targets (2020) 24(5):427–38. doi: 10.1080/14728222.2020.1744568
Keywords: tumor microenvironment, VEGF, TGF-β, immunotherapy, PD-1, review
Citation: Li L, Wen Q and Ding R (2022) Therapeutic targeting of VEGF and/or TGF-β to enhance anti-PD-(L)1 therapy: The evidence from clinical trials. Front. Oncol. 12:905520. doi: 10.3389/fonc.2022.905520
Received: 27 March 2022; Accepted: 30 June 2022;
Published: 26 July 2022.
Edited by:
Camillo Porta, University of Bari Aldo Moro, ItalyReviewed by:
Mototsugu Oya, Keio University, JapanMónica Bequet-Romero, Center for Genetic Engineering and Biotechnology (CIGB), Cuba
Copyright © 2022 Li, Wen and Ding. This is an open-access article distributed under the terms of the Creative Commons Attribution License (CC BY). The use, distribution or reproduction in other forums is permitted, provided the original author(s) and the copyright owner(s) are credited and that the original publication in this journal is cited, in accordance with accepted academic practice. No use, distribution or reproduction is permitted which does not comply with these terms.
*Correspondence: Qinglian Wen, d2VucWluZ2xpYW5Ac3dtdS5lZHUuY24=; Ruilin Ding, cmFpbmluZ2RlYW5AMTYzLmNvbQ==
†These authors have contributed equally to this work and share last authorship