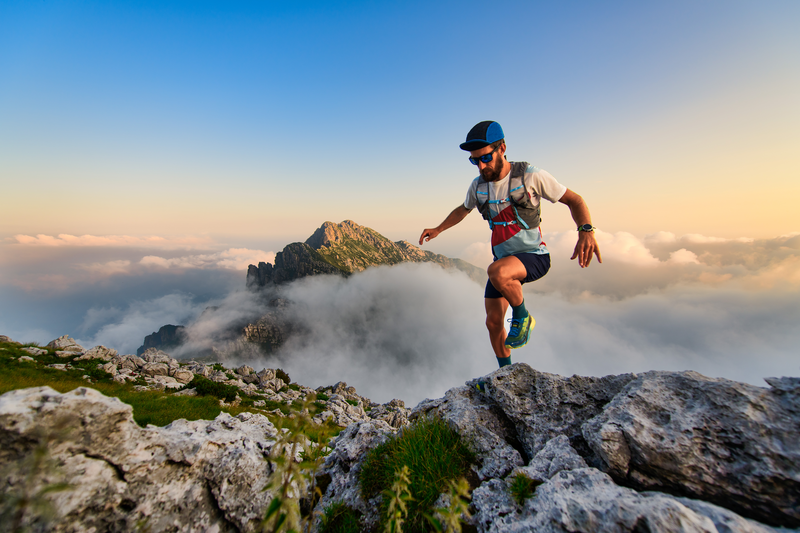
94% of researchers rate our articles as excellent or good
Learn more about the work of our research integrity team to safeguard the quality of each article we publish.
Find out more
ORIGINAL RESEARCH article
Front. Oncol. , 18 July 2022
Sec. Gastrointestinal Cancers: Colorectal Cancer
Volume 12 - 2022 | https://doi.org/10.3389/fonc.2022.900855
Introduction: Chemotherapy-induced cognitive impairment (i.e., “chemobrain”) is a common neurotoxic side-effect experienced by many cancer survivors who undergone chemotherapy. However, the central mechanism underlying chemotherapy-related cognitive impairment is still unclear. The purpose of this study was to investigate the changes of intrinsic brain activity and their associations with cognitive impairment in colorectal cancer (CRC) patients after chemotherapy.
Methods: Resting‐state functional magnetic resonance imaging data of 29 CRC patients following chemotherapy and 29 matched healthy controls (HCs) were collected in this study, as well as cognitive test data including Mini Mental State Exam (MMSE), Montreal Cognitive Assessment (MoCA) and Functional Assessment of Cancer Therapy-Cognitive Function (FACT-Cog). The measure of fractional amplitude of low-frequency fluctuation (fALFF) was calculated and compared between groups. The correlations between the fALFF of impaired brain region and cognitive performance were also analyzed.
Results: Compared with HCs, CRC patients following chemotherapy showed decreased fALFF values in the left anterior cingulate gyrus (ACG) and middle frontal gyrus, as well as increased fALFF values in the left superior frontal gyrus (orbital part) and middle occipital gyrus. Moreover, positive associations were identified between fALFF values of the left ACG and the total scores of MMSE, MoCA and FACT-Cog in the patient group.
Conclusion: These findings indicated that CRC patients after chemotherapy had decreased intrinsic brain activity in the left ACG, which might be vulnerable to the neurotoxic side-effect of chemotherapeutic drugs and related to chemotherapy-induced cognitive impairment.
Colorectal cancer (CRC) is one of the malignant diseases and has high incidence of mortality (1). Approximately 25% of patients newly diagnosed with CRC displayed metastasis, and roughly 50% of those eventually developed metastasis during their lifetime struggling with cancer (2). Advances in early detection, diagnosis and treatment have led to significant improvements in the five-year survival rates of patients with CRC (3). The available treatment options for CRC include surgery, radiotherapy, immunotherapy, targeted therapy (either alone or in combination) and chemotherapy (4). A high number of cancer patients who received chemotherapy had self-reported psychological and cognitive impairment, which had a negative impact on the quality of life (QoL) for cancer patients (5). Chemotherapy related cognitive impairment is called “chemo-brain”, which is a complex treatment-related side effect experienced by cancer survivors during chemotherapy. In addition, cancer related inflammation, anemia, malnutrition and infection, as well as anxiety/depression, negative thoughts, rumination/worry, sleep disorders caused by cancer, might also lead to cognitive impairment (6). Recommended chemotherapeutic drugs for CRC include 5-fluorouracil (5-FU) with leucovorin and irinotecan or oxaliplatin, alone or combined with bevacizumab (7). Although these drugs are effective at improving overall survival (OS) of CRC patients, severe side-effects, such as chemotherapy-related neurotoxicity including toxicity in the central nervous system (CNS), were often found in patients after receiving chemotherapy. The chemotherapy-induced central neurotoxicity were considered to be associated with the endogenous mechanisms, which were named the target and the blood-brain barrier transporter hypotheses (8). Therefore, assessing chemotherapy-related negative impacts on neurocognitive function is crucial for the development of preventive measures and rehabilitation programs after chemotherapy. Additionally, the central pathophysiological mechanism of cognitive impairment associated with chemotherapy is complicated and still unclear.
Cognitive impairment was found in 13%-57% of CRC patients treated with chemotherapy (9), which was similar to the study reporting the prevalence of cognitive impairment ranged from 17% to 75% among patients of various cancer types (10). Another study found that 18-24% of CRC patients had perceived cognitive impairment before treatment based on the Functional Assessment of Cancer Therapy-Cognitive Function (FACT-Cog) while the impaired cognition in those who treated with chemotherapy was aggravated (11). Moreover, the impaired cognition was related to anxiety, depression, fatigue and poorer QOL, which suggested that these negative concomitant symptoms might be associated with cancer-related distress or negative appraisal and decreased tolerance or resilience to manage cancer-related pain and chemotherapy-related side-effects (11–13). A meta-analysis study also found that older CRC patients were more vulnerable to cognitive impairment after chemotherapy, and preventive measures and rehabilitation programs could reduce the risk of developing chemotherapy-induced cognitive impairment (14). A prospective, longitudinal, controlled study showed that 36%-52% CRC patients had cognitive impairment including working memory, attention, verbal memory and processing speed (15). Patients treated with chemotherapy regimens of capecitabine with/without oxaliplatin had high risk of developing cognitive impairment while FOLFOX (folate, 5-FU, oxaliplatin) and oxaliplatin was negatively related to the cognitive impairment (16). In addition, FOLFIRI regimen (folate, 5-FU, irinotecan) caused to increased risk of cognitive impairment in older CRC patients (16). Patients with CRC were also found to have decreased executive function after 12 months of chemotherapy (5-FU with or without oxaliplatin) (17).
Resting-state functional magnetic resonance (rs-fMRI) is a non-invasive imaging method with high spatial resolution, which can be used to detect functional activity during rest by measuring intrinsic blood oxygen level-dependent (BOLD) low-frequency signal fluctuations (18). Therefore, the method of rs-fMRI has been extensively used to explore the central pathophysiological mechanism of cognitive impairment and provide neurophysiological evidence for functional changes in the brain of patients with cognitive impairment (19, 20). Among commonly used measures, the amplitude of low-frequency fluctuation (ALFF) and fractional ALFF (fALFF) with higher test-retest reliability have been applied to measure regional spontaneous brain activity (21, 22). ALFF is calculated as the square root of the power spectrum in low-frequency range, which measures the amplitude of spontaneous brain activity during rest (22). However, the measure of ALFF is sensitive to the physiological noise (23). Therefore, to overcome this limitation, the measure of fALFF measuring the ratio of the low-frequency power spectrum to that of the entire frequency range, is proposed as an improved ALFF indicator, which has been considered as a more robust measure for regional spontaneous brain activity (21). Based on the measure of ALFF, patients with breast cancer receiving one month chemotherapy had increased brain activity in the bilateral precuneus and middle temporal gyrus, left upper and inferior temporal gyrus, which mainly located in the default mode network (DMN) (24). Patients with gastric cancer had decreased ALFF in the bilateral fontal and temporal areas after chemotherapy (capecitabine+oxaliplatin) and decreased fALFF was found in the anterior cingulate gyrus (ACG) and bilateral orbitofrontal gyrus when compared with healthy controls (HCs) (25). Decreased fALFF was identified in the left precuneus of breast cancer patients received a chemotherapy regimen of docetaxel and cyclophosphamide (26). Lung cancer patients before chemotherapy demonstrated decreased ALFF in the right posterior cingulate cortex when compared with patients after chemotherapy including pemetrexed, gemcitabine, cisplatin, nedaplatin, docetaxel and etoposide (27). Therefore, we speculated that fALFF might be a promising imaging indicator for exploring the central pathological mechanism of cognitive impairment in CRC patients after chemotherapy.
To the best of our knowledge, this is the first study to explore the central pathological mechanism involving in the cognitive impairment of CRC patients who had undergone chemotherapy. Based on previous neuroimaging findings, we hypothesized that CRC patients with chemotherapy-related cognitive impairment might have altered functional activity in some brain regions. Therefore, the method of rs-fMRI and the measure of fALFF were applied to explore the possible central mechanism underlying cognitive alternations of CRC patients after chemotherapy in this study.
In total, 29 CRC patients who had undergone chemotherapy were recruited from the Department of Oncology, Jiangsu Cancer Hospital & Jiangsu Institute of Cancer Research & The Affiliated Cancer Hospital of Nanjing Medical University, Nanjing, China. The inclusion criteria of CRC patients were as follows: 1) histological confirmation of CRC adenocarcinoma; 2) received 2 to 3 months of chemotherapy with standard chemotherapeutic agents; 3) right-handed;4)aged from 40 to 70 years; 5) received at least 9 years of education. The exclusion criteria for patients were as follows: 1) terminal stage of CRC (survival period <1 year); 2) history of diagnosis and treatment for any other cancer; 3) presence of brain metastasis; 4) history of radiation therapy before the enrollment; 5) presence of any major medical illness; 6) psychiatric or neurological disorders; 7) history of substance abuse; 8) any contraindication of MRI scans. According the inclusion and exclusion criteria of patients, 29 age, sex, handness and education level-matched HCs with no history of any cancer were enrolled through the online advertisement. The sample size was estimated as follows: δ=(μD-0)/, where δ was the effect size, μD was the difference in means between groups, 0 was the difference in means under the null hypothesis, and δ was the variability in the difference in means (28). In fMRI, μD and δ were typically normalized as percent signal change (i.e. 100×(E-C)/C, where E, patients and C, HCs) (28).
This study was approved by the Research Ethics Committee of Jiangsu Cancer Hospital & Jiangsu Institute of Cancer Research & The Affiliated Cancer Hospital of Nanjing Medical University. All subjects signed informed consent before their participation in the study.
The cognitive function of all participants was measured using the self-report questionnaires including Mini Mental State Exam (MMSE) (attention, memory, language, and visuospatial abilities, as well as orientation to person, place and time) (29), Montreal Cognitive Assessment (MoCA) (attention and concentration, executive function, memory, language, visual structure skills, abstract thinking, calculation and orientation) (30) and Functional Assessment of Cancer Therapy-Cognitive Function (FACT-Cog) (patients’ perceived cognitive impairments, perceived cognitive abilities, noticeability or comments from others, impact of cognitive changes on quality of life) (31).
MRI data were acquired following the complete treatment of chemotherapy (2 to 3 months) with a 3.0 T Philips Aachieva scanner (Figure 1). The details about parameters of MRI acquisition were described in our previous study (Figure 1) (32). The MRI data preprocessing was conducted using the Data Processing Assistant for rs-fMRI advanced edition (DPARSF) in MATLAB software (33). The whole preprocessing procedures were as follows: 1) conversion of MRI data; 2) discarding first 6 images; 3) slice timing; 4) realign; 5) reorientation of images to the Montreal Neurological Institute (MNI) template; 6) segmentation; 7) normalized by T1 image (3×3×3mm3 resolution); 8) smoothing with a 4 mm Gaussian kernel; 9) detrend; 10) nuisance covariates regression including signals from white matter and cerebrospinal fluid and Friston-24 head motion parameters. Participants with head motion >2mm or a spin of >2° in any direction were excluded in this study.
Figure 1 Pipeline of MRI data acquisition and processing, and fALFF calculation. fALFF, fractional amplitude of low-frequency fluctuation; fMRI, functional magnetic resonance; MNI, Montreal Neurological Institute; x, y and z: the coordinates of peak voxel of each cluster in the MNI space.
The measure of fALFF was calculated by the software DPARSF (Figure 1). Firstly, the time course of each voxel in the brain was converted to the frequency domain without band-pass filter by the Fast Fourier transform (FFT) and then the power spectrum was obtained. Secondly, the square root at each frequency of the power spectrum was calculated, and the average square root of the power spectrum of each voxel across 0.01-0.08 Hz was calculated as the ALFF value of voxel. The ratio of the power spectrum in the low-frequency band (0.01-0.08 Hz) to that in the whole frequency range was defined as fALFF value. Finally, to reduce the nonspecific signals, fALFF maps were obtained through fALFF value of each voxel divided by the global mean fALFF value. ALFF is a measure for exploring spontaneous brain activity, however, it is often influenced by physiological noise. Therefore, to overcome the limitations of ALFF, fALFF is proposed to selectively suppress artifacts from non-specific brain areas.
With the software of SPSS (version 23.0; IBM, Armonk, NY, USA), the two-sample t-test was used to analyze the differences of age, educational level, scores of MMSE and MoCA between groups while the Chi-squared test was performed to estimate the differences of gender distribution. Statistical significance was defined as P<0.05.
In addition, to identify the differences of fALFF between groups, the two-sample t-test was conducted by the software of Resting-State fMRI Data Analysis (REST) Toolkit (34). The significance threshold was set at P<0.05 (voxel-level was set at P<0.001 and cluster-level was set at P<0.05; two tailed; the corresponding minimum cluster size was 21 voxels) for multiple comparisons using Gaussian Random Field (GRF) theory.
Finally, Pearson correlation analyses were conducted to examine whether the fALFF values of brain regions showing group-differences were correlated with the total scores of cognitive scales. The significance level was set at P<0.05.
A total of 29 CRC patients (0 in stage I, 3 in stage II, 20 in stage III and 6 in stage IV) were enrolled in this study. In all patients, 5 patients had lesions in the colon while 23 patients had lesions in the rectum. In addition, 5 patients had no metastasis while other cases had metastasis in the lung (2), liver (14), bone (1), as well as intraperitoneal (6) and pelvic metastasis (1). Two chemotherapy regimens were used in this study including CAPOX (capecitabine plus oxaliplatin) (10 patients) and CAPOX plus bevacizumab (19 patients). The drugs in the chemotherapy regimens were administered in accordance with the National Comprehensive Cancer Network (NCCN) guidelines for CRC (35, 36). Moreover, there were no differences in the age, gender and educational level between groups. However, patients had decreased scores of MMSE, MoCA and FACT-Cog when compared with those of HCs. The demographics and clinical information of two groups were presented in Table 1.
The fALFF values of patients after chemotherapy showed a significant decrease in the left ACG and middle frontal gyrus, while displaying a significant increase in the left superior frontal gyrus (orbital part) and middle occipital gyrus when compared with those of HCs (Table 2; Figure 2). In addition, we also compared the differences of fALFF values between patients received different chemotherapy regimen [CAPOX (10 patients) vs CAPOX plus bevacizumab (19 patients)] and details about the participants, methods and results were illustrated in Supplementary Materials.
Figure 2 Brain regions showed altered fALFF in CRC patients. Post-treatment CRC patients showed decreased fALFF in the left anterior cingulate gyrus and middle frontal gyrus, while displaying increased fALFF in the left superior frontal gyrus (orbital part) and middle occipital gyrus. CRC: colorectal cancer. fALFF: fractional amplitude of low-frequency fluctuation. The significance threshold was set at P<0.05 (voxel-level was set at P<0.001 and cluster-level was set at P<0.05; two tailed; the corresponding minimum cluster size was 21 voxels) for multiple comparisons using Gaussian Random Field (GRF) theory. Red indicated regions with increased fALFF while blue indicated regions with decreased fALFF.
As shown in Figure 3, fALFF values of the left ACG were positively correlated with the total scores of MMSE (r=0.42; P=0.024), MoCA (r=0.46; P=0.012) and FACT-Cog (r=0.52; P=0.004).
Figure 3 Correlations between fALFF and scores of cognitive scales. fALFF: fractional amplitude of low-frequency fluctuation. MMSE: Mini Mental State Exam; MoCA: Montreal Cognitive Assessment; FACT-Cog: Functional Assessment of Cancer Therapy-Cognitive Function. The significance level was set at P<0.05.
In this study, we utilized rs-fMRI to investigate the changes of spontaneous brain activities in CRC patients following chemotherapy compared to HCs with the measure of fALFF. We found that patients presented with decreased scores of cognitive function assessment, which suggested that chemotherapy might lead cognitive dysfunction. patients also had decreased fALFF in the left ACG and middle frontal gyrus, and increased fALFF in the left superior frontal gyrus (orbital part) and middle occipital gyrus, which suggested different patterns of spontaneous brain activity between groups in these regions. In addition, we found significant positive correlations between fALFF values of the left ACG and scores of cognitive scales including MMSE, MoCA and FACT-Cog.
Chemotherapy has neurotoxicity on both the central and peripheral nervous system, which manifests as a wide range of clinical syndromes including cognitive impairment (37, 38). Increasing evidence demonstrated that chemotherapeutic drugs (cytotoxic drugs) for non-central nervous system cancer might also lead to cognitive impairment related to chemotherapy (39, 40). The relationship between chemotherapy and cognitive performance of cancer patients who undergone chemotherapy had been explored in previous studies (41–43). In this study, CRC patients had decreased scores of MMSE, MoCA and FACT-Cog, which implied that these patients following chemotherapy had impaired cognitive function. This finding was consistent with the result of previous study, which showed that chemotherapy had a negative effect on the cognitive function of patients (56%) with colon cancer, such as verbal memory (44). Patients with other cancer also had subjective cognitive dysfunction after chemotherapy and the most serious effect was found at one month after chemotherapy, which suggested that the impact of chemotherapy might be an acute rather than a chronic side effect on cognitive function (45).
ACG is one of subregions in the prefrontal cortex, which is considered to be involved in a broad range of processes including cognitive control, emotion processing and attention (46–49). Therefore, it is a critical part of the neural network implicated in cognitive control process, such as attentional control, conflict monitoring and error detection, and it is active when cognitive control is required (50–52). As an important brain region implicated in cognitive control, it has been hypothesized that the activation of ACG increases top-down control (53–55). Reduced functional connectivity was identified in ACG of patients with mild cognitive impairment (MCI), which supported the idea that abnormal activity of ACG might be a useful indicator to understand the central pathological mechanism of cognitive impairment, as well as sensitive neuroimaging biomarker for the early detection of MCI (19, 56, 57). In addition, impaired structure of ACG was also found in patients with MCI and it was paralleled with decreased performance of cognitive function, especially interference and conflict monitoring (58). The structural abnormality of ACG was identified as a predictor of cognitive impairment, which suggested that ACG played a key role in the underlying pathogenesis of cognitive impairment (59). In addition, the impaired cognitive function could be improved by mind-body exercise (movement-based mind-body intervention), which could modulate regional brain activities and structure in ACG (60). The cognitive improvement was also found to be associated with increased functional connectivity of ACG in patients with cognitive impairment (61).
In this study, we found that CRC patients had decreased brain activity in left ACG when compared to HCs. Previous study provided evidence that chemotherapeutic drug, cisplatin, could lead to the abnormality of ACG neurons, which might be associated with cognitive deficits of rats following chemotherapy (62). Cisplatin had been shown to effectively block the expression of local field potential (LTP) at amygdala-ACG synapses and affect the physiological expression of theta oscillation activity (62). These findings suggested that cisplatin might contribute to desynchronization in the amygdala-ACG, which were associated with the abnormalities of learning and memory (62). A pattern of dispersion of theta band activity and reduction in correlation values of cisplatin were found when simultaneously recording local field potentials in ACG and basolateral amygdala (BLA), which might be relevant to the failure of introduction of LTP in the BLA- ACG circuit after administration of cisplatin (62). In human, non-CNS cancer patients after chemotherapy exhibited reduced grey matter volume in ACG, which might contribute to cognitive impairment related to chemotherapy (63). In addition, breast cancer patients received 6 months of chemotherapy showed reduction of grey matter volume in ACG (64). Decreased functional connectivity was also found in breast cancer patients after receiving chemotherapy, which suggested that abnormal function in ACG might be implicated in the cognitive impairment related to chemotherapy of breast cancer patients (65). Importantly, decreased functional connectivity was found in ACG of post-chemotherapy patients with lung cancer (chemotherapy regimen: cisplatin based therapy or carboplatin based therapy for at least six months), and positive association was found between decreased functional connectivity in ACG and reduced MoCA scores of patients (66). Moreover, gray matter density of ACG was also found to decrease in lung cancer patients following platinum-based chemotherapy (67). ACG is considered to play an important role in cognitive control (68). Therefore, these findings supported the view that impaired ACG might play a key role in the development of cognitive impairment in cancer patients treated with chemotherapy. However, it was difficult to make causal relationships between functional abnormalities of ACG and cognitive impairment due to the cross-sectional experimental design. Overall, additional studies with larger sample size were necessary to confirm these results, and longitudinal studies were needed to explore the causal relationships between the functional abnormalities of ACG and chemotherapy-induced cognitive impairment in CRC patients, and to better understand the mechanisms underlying “chemo-brain”.
In summary, we observed chemotherapy-associated cognitive impairment in CRC patients following chemotherapy, which might be associated with decreased fALFF in the left ACG. These findings supported the notion that spontaneous brain activities might serve as neuroimaging biomarkers for cognitive impairment in patients who undergone treatment of chemotherapeutic drugs. Moreover, these results provided an important basis for the developing interventions for cognitive rehabilitation of cancer patients.
The raw data supporting the conclusions of this article will be made available by the authors, without undue reservation.
This study was reviewed and approved by The Research Ethics Committee of Jiangsu Cancer Hospital & Jiangsu Institute of Cancer Research & The Affiliated Cancer Hospital of Nanjing Medical University. The patients/participants provided their written informed consent to participate in this study.
SiL, ShL and XL designed the experiments. SiL, YG, JN, NY, CL, XP, RM and JW contributed to clinical data collection and assessment. SiL, YG, ShL and XL analyzed the results. SiL, YG, ShL and XL wrote the manuscript. SiL, YG, ShL and XL approved the final manuscript. All authors contributed to the article and approved the submitted version.
The work was supported by the grants of: Jiangsu Provincial Natural Science Fund (No. BK20210977); Cadre Health Research Project of Jiangsu Province (No. BJ18033) and Foundation of Jiangsu Cancer Hospital (No. ZM201923).
The authors declare that the research was conducted in the absence of any commercial or financial relationships that could be construed as a potential conflict of interest.
All claims expressed in this article are solely those of the authors and do not necessarily represent those of their affiliated organizations, or those of the publisher, the editors and the reviewers. Any product that may be evaluated in this article, or claim that may be made by its manufacturer, is not guaranteed or endorsed by the publisher.
The Supplementary Material for this article can be found online at: https://www.frontiersin.org/articles/10.3389/fonc.2022.900855/full#supplementary-material
1. Cardoso R, Guo F, Heisser T, Hackl M, Ihle P, De Schutter H, et al. Colorectal cancer incidence, mortality, and stage distribution in European countries in the colorectal cancer screening era: an international population-based study. Lancet Oncol (2021) 22(7):1002–13. doi: 10.1016/S1470-2045(21)00199-6
2. Vatandoust S, Price TJ, Karapetis CS. Colorectal cancer: Metastases to a single organ. World J Gastroenterol (2015) 21(41):11767–76. doi: 10.3748/wjg.v21.i41.11767
3. Dekker E, Tanis PJ, Vleugels JLA, Kasi PM, Wallace MB. Colorectal cancer. Lancet (London Engl) (2019) 394(10207):1467–80. doi: 10.1016/S0140-6736(19)32319-0
4. Biller LH, Schrag D. Diagnosis and treatment of metastatic colorectal cancer: A review. JAMA (2021) 325(7):669–85. doi: 10.1001/jama.2021.0106
5. Lange M, Joly F, Vardy J, Ahles T, Dubois M, Tron L, et al. Cancer-related cognitive impairment: an update on state of the art, detection, and management strategies in cancer survivors. Ann Oncol (2019) 30(12):1925–40. doi: 10.1093/annonc/mdz410
6. André N, Gastinger S, Rébillard A. Chronic fatigue in cancer, brain connectivity and reluctance to engage in physical activity: A mini-review. Front Oncol (2021) 11:774347. doi: 10.3389/fonc.2021.774347
7. Van Cutsem E, Köhne CH, Hitre E, Zaluski J, Chang Chien CR, Makhson A, et al. Cetuximab and chemotherapy as initial treatment for metastatic colorectal cancer. N Engl J Med (2009) 360(14):1408–17. doi: 10.1056/NEJMoa0805019
8. Froklage FE, Reijneveld JC, Heimans JJ. Central neurotoxicity in cancer chemotherapy: pharmacogenetic insights. Pharmacogenomics (2011) 12(3):379–95. doi: 10.2217/pgs.10.197
9. Chan YN, Leak Bryant A, Conklin JL, Girdwood T, Piepmeier A, Hirschey R. Systematic review of cognitive impairment in colorectal cancer survivors who received chemotherapy. Oncol Nurs Forum (2021) 48(6):634–47. doi: 10.1188/21.ONF.634-647
10. Myers JS. Chemotherapy-related cognitive impairment. Clin J Oncol Nurs (2009) 13(4):413–21. doi: 10.1188/09.CJON.413-421
11. Dhillon HM, Tannock IF, Pond GR, Renton C, Rourke SB, Vardy JL. Perceived cognitive impairment in people with colorectal cancer who do and do not receive chemotherapy. J Cancer Surviv Res Pract (2018) 12(2):178–85. doi: 10.1007/s11764-017-0656-6
12. Lv L, Mao S, Dong H, Hu P, Dong R. Pathogenesis, assessments, and management of chemotherapy-related cognitive impairment (CRCI): An updated literature review. J Oncol (2020) 2020:3942439. doi: 10.1155/2020/3942439
13. Eide S, Feng ZP. Doxorubicin chemotherapy-induced "chemo-brain": Meta-analysis. Eur J Pharmacol (2020) 881:173078. doi: 10.1016/j.ejphar.2020.173078
14. Hwang SY, Kim K, Ha B, Lee D, Kim S, Ryu S, et al. Neurocognitive effects of chemotherapy for colorectal cancer: A systematic review and a meta-analysis of 11 studies. Cancer Res Treat (2021) 53(4):1134–47. doi: 10.4143/crt.2020.1191
15. Vardy JL, Dhillon HM, Pond GR, Rourke SB, Bekele T, Renton C, et al. et al. cognitive function in patients with colorectal cancer who do and do not receive chemotherapy: A prospective, longitudinal, controlled study. J Clin Oncol (2015) 33(34):4085–92. doi: 10.1200/JCO.2015.63.0905
16. Kim K, Kim CW, Shin A, Kang H, Jung SJ. Effect of chemotherapy and radiotherapy on cognitive impairment in colorectal cancer: evidence from Korean national health insurance database cohort. Epidemiol Health (2021) 43:e2021093. doi: 10.4178/epih.e2021093
17. Sales MVC, Suemoto CK, Apolinario D, Serrao V, Andrade CS, Conceição DM, et al. Effects of adjuvant chemotherapy on cognitive function of patients with early-stage colorectal cancer. Clin Colorectal Cancer (2019) 18(1):19–27. doi: 10.1016/j.clcc.2018.09.002
18. Lee MH, Smyser CD, Shimony JS. Resting-state fMRI: a review of methods and clinical applications. AJNR Am J Neuroradiol (2013) 34(10):1866–72. doi: 10.3174/ajnr.A3263
19. Ibrahim B, Suppiah S, Ibrahim N, Mohamad M, Hassan HA, Nasser NS, et al. Diagnostic power of resting-state fMRI for detection of network connectivity in alzheimer's disease and mild cognitive impairment: A systematic review. Hum Brain Mapp (2021) 42(9):2941–68. doi: 10.1002/hbm.25369
20. Terry DP, Sabatinelli D, Puente AN, Lazar NA, Miller LS. A meta-analysis of fMRI activation differences during episodic memory in alzheimer's disease and mild cognitive impairment. J Neuroimaging (2015) 25(6):849–60. doi: 10.1111/jon.12266
21. Zou QH, Zhu CZ, Yang Y, Zuo XN, Long XY, Cao QJ, et al. An improved approach to detection of amplitude of low-frequency fluctuation (ALFF) for resting-state fMRI: fractional ALFF. J Neurosci Methods (2008) 172(1):137–41. doi: 10.1016/j.jneumeth.2008.04.012
22. Zang YF, He Y, Zhu CZ, Cao QJ, Sui MQ, Liang M, et al. Altered baseline brain activity in children with ADHD revealed by resting-state functional MRI. Brain Dev (2007) 29(2):83–91. doi: 10.1016/j.braindev.2006.07.002
23. Auer DP. Spontaneous low-frequency blood oxygenation level-dependent fluctuations and functional connectivity analysis of the 'resting' brain. Magn Reson Imaging (2008) 26(7):1055–64. doi: 10.1016/j.mri.2008.05.008
24. Zheng F, Cao P, Zhou J, Li C, Norris J. Study on neurologic and cognitive dysfunction in breast cancer patients undergoing chemotherapy with RS fMRI imaging. World Neurosurg (2020) 149(20):388–96. doi: 10.1016/j.wneu.2020.10.088
25. Kim HG, Shin NY, Bak Y, Kim KR, Jung YC, Han K, et al. Altered intrinsic brain activity after chemotherapy in patients with gastric cancer: A preliminary study. Eur Radiol (2017) 27(7):2679–88. doi: 10.1007/s00330-016-4578-x
26. Chen BT, Jin T, Patel SK, Ye N, Ma H, Wong CW, et al. et al. intrinsic brain activity changes associated with adjuvant chemotherapy in older women with breast cancer: a pilot longitudinal study. Breast Cancer Res Treat (2019) 176(1):181–9. doi: 10.1007/s10549-019-05230-y
27. You J, Hu L, Zhang Y, Chen F, Yin X, Jin M, et al. Altered dynamic neural activity in the default mode network in lung cancer patients after chemotherapy. Med Sci Monit (2020) 26:e921700. doi: 10.12659/MSM.921700
28. Desmond JE, Glover GH. Estimating sample size in functional MRI (fMRI) neuroimaging studies: statistical power analyses. J Neurosci Methods (2002) 118(2):115–28. doi: 10.1016/S0165-0270(02)00121-8
29. Mitrushina M, Satz P. Reliability and validity of the mini-mental state exam in neurologically intact elderly. J Clin Psychol (1991) 47(4):537–43. doi: 10.1002/1097-4679(199107)47:4<537::AID-JCLP2270470411>3.0.CO;2-9
30. Nasreddine ZS, Phillips NA, Bédirian V, Charbonneau S, Whitehead V, Collin I, et al. The Montreal cognitive assessment, MoCA: a brief screening tool for mild cognitive impairment. J Am Geriatr Soc (2005) 53(4):695–9. doi: 10.1111/j.1532-5415.2005.53221.x
31. Cheung YT, Chan A. Linguistic validation of functional assessment of cancer therapy-cognitive function (FACT-cog): methodological concerns. Support Care Cancer (2013) 21(3):655–6. doi: 10.1007/s00520-012-1631-4
32. Liu S, Yin N, Li C, Li X, Ni J, Pan X, et al. Topological abnormalities of pallido-Thalamo-Cortical circuit in functional brain network of patients with nonchemotherapy with non-small cell lung cancer. Front Neurol (2022) 13:821470. doi: 10.3389/fneur.2022.821470
33. Chao-Gan Y, Yu-Feng Z. DPARSF: A MATLAB toolbox for "Pipeline" data analysis of resting-state fMRI. Front Syst Neurosci (2010) 4:13. doi: 10.3389/fnsys.2010.00013
34. Song XW, Dong ZY, Long XY, Li SF, Zuo XN, Zhu CZ, et al. REST: a toolkit for resting-state functional magnetic resonance imaging data processing. PloS One (2011) 6(9):e25031. doi: 10.1371/journal.pone.0025031
35. Benson AB, Venook AP, Al-Hawary MM, Arain MA, Chen YJ, Ciombor KK, et al. NCCN guidelines insights: Rectal cancer, version 6.2020. J Natl Compr Cancer Netw (2020) 18(7):806–15. doi: 10.6004/jnccn.2020.0032
36. Benson AB, Venook AP, Al-Hawary MM, Arain MA, Chen YJ, Ciombor KK, et al. Colon cancer, version 2.2021, NCCN clinical practice guidelines in oncology. J Natl Compr Cancer Netw (2021) 19(3):329–59. doi: 10.6004/jnccn.2021.0012
37. Taillibert S, Le Rhun E, Chamberlain MC. Chemotherapy-related neurotoxicity. Curr Neurol Neurosci Rep (2016) 16(9):81. doi: 10.1007/s11910-016-0686-x
38. Wardill HR, Mander KA, Van Sebille YZ, Gibson RJ, Logan RM, Bowen JM, et al. Cytokine-mediated blood brain barrier disruption as a conduit for cancer/chemotherapy-associated neurotoxicity and cognitive dysfunction. Int J Cancer (2016) 139(12):2635–45. doi: 10.1002/ijc.30252
39. Raffa RB. A proposed mechanism for chemotherapy-related cognitive impairment ('chemo-fog'). J Clin Pharm Ther (2011) 36(3):257–9. doi: 10.1111/j.1365-2710.2010.01188.x
40. Dutta V. Chemotherapy, neurotoxicity, and cognitive changes in breast cancer. J Cancer Res Ther (2011) 7(3):264–9. doi: 10.4103/0973-1482.87008
41. Ono M, Ogilvie JM, Wilson JS, Green HJ, Chambers SK, Ownsworth T, et al. A meta-analysis of cognitive impairment and decline associated with adjuvant chemotherapy in women with breast cancer. Front Oncol (2015) 5:59. doi: 10.3389/fonc.2015.00059
42. Jim HS, Phillips KM, Chait S, Faul LA, Popa MA, Lee YH, et al. Meta-analysis of cognitive functioning in breast cancer survivors previously treated with standard-dose chemotherapy. J Clin Oncol (2012) 30(29):3578–87. doi: 10.1200/JCO.2011.39.5640
43. Lindner OC, Phillips B, McCabe MG, Mayes A, Wearden A, Varese F, et al. A meta-analysis of cognitive impairment following adult cancer chemotherapy. Neuropsychology (2014) 28(5):726–40. doi: 10.1037/neu0000064
44. Cruzado JA, López-Santiago S, Martínez-Marín V, José-Moreno G, Custodio AB, Feliu J. Longitudinal study of cognitive dysfunctions induced by adjuvant chemotherapy in colon cancer patients. Support Care Cancer (2014) 22(7):1815–23. doi: 10.1007/s00520-014-2147-x
45. Kim HJ, Jung SO, Kim E, Abraham I. Association of chemotherapy and subjective cognitive impairment in breast cancer patients: Meta-analysis of longitudinal prospective cohort studies. Eur J Oncol Nurs (2022) 57:102099. doi: 10.1016/j.ejon.2022.102099
46. Apps MA, Rushworth MF, Chang SW. The anterior cingulate gyrus and social cognition: Tracking the motivation of others. Neuron (2016) 90(4):692–707. doi: 10.1016/j.neuron.2016.04.018
47. Gennari SP, Millman RE, Hymers M, Mattys SL. Anterior paracingulate and cingulate cortex mediates the effects of cognitive load on speech sound discrimination. NeuroImage (2018) 178:735–43. doi: 10.1016/j.neuroimage.2018.06.035
48. Gu Z, Lu H, Zhou H, Zhang J, Xing W. The relationship between abnormal cortical activity in the anterior cingulate gyrus and cognitive dysfunction in patients with end- stage renal disease: a fMRI study on the amplitude of low- frequency fluctuations. Ann Palliat Med (2020) 9(6):4187–93. doi: 10.21037/apm-20-2138
49. Pourtois G, Vocat R, N'Diaye K, Spinelli L, Seeck M, Vuilleumier P. Errors recruit both cognitive and emotional monitoring systems: simultaneous intracranial recordings in the dorsal anterior cingulate gyrus and amygdala combined with fMRI. Neuropsychologia (2010) 48(4):1144–59. doi: 10.1016/j.neuropsychologia.2009.12.020
50. Brockett AT, Roesch MR. Anterior cingulate cortex and adaptive control of brain and behavior. Int Rev Neurobiol (2021) 158:283–309. doi: 10.1016/bs.irn.2020.11.013
51. Vassena E, Deraeve J, Alexander WH. Surprise, value and control in anterior cingulate cortex during speeded decision-making. Nat Hum Behav (2020) 4(4):412–22. doi: 10.1038/s41562-019-0801-5
52. Tolomeo S, Christmas D, Jentzsch I, Johnston B, Sprengelmeyer R, Matthews K, et al. A causal role for the anterior mid-cingulate cortex in negative affect and cognitive control. Brain J Neurol (2016) 139(Pt 6):1844–54. doi: 10.1093/brain/aww069
53. White MG, Panicker M, Mu C, Carter AM, Roberts BM, Dharmasri PA, et al. Anterior cingulate cortex input to the claustrum is required for top-down action control. Cell Rep (2018) 22(1):84–95. doi: 10.1016/j.celrep.2017.12.023
54. Silton RL, Heller W, Towers DN, Engels AS, Spielberg JM, Edgar JC, et al. The time course of activity in dorsolateral prefrontal cortex and anterior cingulate cortex during top-down attentional control. NeuroImage (2010) 50(3):1292–302. doi: 10.1016/j.neuroimage.2009.12.061
55. Blair KS, Geraci M, Smith BW, Hollon N, DeVido J, Otero M, et al. Reduced dorsal anterior cingulate cortical activity during emotional regulation and top-down attentional control in generalized social phobia, generalized anxiety disorder, and comorbid generalized social phobia/generalized anxiety disorder. Biol Psychiatry (2012) 72(6):476–82. doi: 10.1016/j.biopsych.2012.04.013
56. Cera N, Esposito R, Cieri F, Tartaro A. Altered cingulate cortex functional connectivity in normal aging and mild cognitive impairment. Front Neurosci (2019) 13:857. doi: 10.3389/fnins.2019.00857
57. Yu E, Liao Z, Tan Y, Qiu Y, Zhu J, Han Z, et al. High-sensitivity neuroimaging biomarkers for the identification of amnestic mild cognitive impairment based on resting-state fMRI and a triple network model. Brain Imaging Behav (2019) 13(1):1–14. doi: 10.1007/s11682-017-9727-6
58. Borsa VM, Della Rosa PA, Catricalà E, Canini M, Iadanza A, Falini A, et al. Interference and conflict monitoring in individuals with amnestic mild cognitive impairment: A structural study of the anterior cingulate cortex. J Neuropsychol (2018) 12(1):23–40. doi: 10.1111/jnp.12105
59. Jeong HJ, Lee YM, Park JM, Lee BD, Moon E, Suh H, et al. Reduced thickness of the anterior cingulate cortex as a predictor of amnestic-mild cognitive impairment conversion to alzheimer's disease with psychosis. J Alzheimers Dis (2021) 84(4):1709–17. doi: 10.3233/JAD-215005
60. Tao J, Liu J, Chen X, Xia R, Li M, Huang M, et al. Mind-body exercise improves cognitive function and modulates the function and structure of the hippocampus and anterior cingulate cortex in patients with mild cognitive impairment. NeuroImage Clin (2019) 23:101834. doi: 10.1016/j.nicl.2019.101834
61. Yin M, Liu Y, Zhang L, Zheng H, Peng L, Ai Y, et al. Effects of rTMS treatment on cognitive impairment and resting-state brain activity in stroke patients: A randomized clinical trial. Front Neural Circuits (2020) 14:563777. doi: 10.3389/fncir.2020.563777
62. Mu L, Wang J, Cao B, Jelfs B, Chan RH, Xu X, et al. Impairment of cognitive function by chemotherapy: association with the disruption of phase-locking and synchronization in anterior cingulate cortex. Mol Brain (2015) 8:32. doi: 10.1186/s13041-015-0125-y
63. Niu R, Du M, Ren J, Qing H, Wang X, Xu G, et al. Chemotherapy-induced grey matter abnormalities in cancer survivors: a voxel-wise neuroimaging meta-analysis. Brain Imaging Behav (2021) 15(4):2215–27. doi: 10.1007/s11682-020-00402-7
64. Jenkins V, Thwaites R, Cercignani M, Sacre S, Harrison N, Whiteley-Jones H, et al. A feasibility study exploring the role of pre-operative assessment when examining the mechanism of 'chemo-brain' in breast cancer patients. SpringerPlus (2016) 5:390. doi: 10.1186/s40064-016-2030-y
65. Tao L, Lin H, Yan Y, Xu X, Wang L, Zhang J, et al. Impairment of the executive function in breast cancer patients receiving chemotherapy treatment: a functional MRI study. Eur J Cancer Care (2017) 26(6):1–8. doi: 10.1111/ecc.12553
66. Zhang Y, Chen YC, Hu L, You J, Gu W, Li Q, et al. Chemotherapy-induced functional changes of the default mode network in patients with lung cancer. Brain Imaging Behav (2020) 14(3):847–56. doi: 10.1007/s11682-018-0030-y
67. Simó M, Root JC, Vaquero L, Ripollés P, Jové J, Ahles T, et al. Cognitive and brain structural changes in a lung cancer population. J Thorac Oncol (2015) 10(1):38–45. doi: 10.1097/JTO.0000000000000345
Keywords: chemotherapy, cognitive impairment, resting-state functional magnetic resonance imaging, fractional amplitude of low-frequency fluctuation, colorectal cancer
Citation: Liu S, Guo Y, Ni J, Yin N, Li C, Pan X, Ma R, Wu J, Li S and Li X (2022) Chemotherapy-induced functional brain abnormality in colorectal cancer patients: a resting‐state functional magnetic resonance imaging study. Front. Oncol. 12:900855. doi: 10.3389/fonc.2022.900855
Received: 21 March 2022; Accepted: 28 June 2022;
Published: 18 July 2022.
Edited by:
Liana Romaniuk, University of Edinburgh, United KingdomReviewed by:
Antino Recio Allen, University of Arkansas for Medical Sciences, United StatesCopyright © 2022 Liu, Guo, Ni, Yin, Li, Pan, Ma, Wu, Li and Li. This is an open-access article distributed under the terms of the Creative Commons Attribution License (CC BY). The use, distribution or reproduction in other forums is permitted, provided the original author(s) and the copyright owner(s) are credited and that the original publication in this journal is cited, in accordance with accepted academic practice. No use, distribution or reproduction is permitted which does not comply with these terms.
*Correspondence: Xiaoyou Li, bHh5Znl4MTE2QDEyNi5jb20=; Shengwei Li, MjQ2MTEyNzhAcXEuY29t
†These authors have contributed equally to this work
Disclaimer: All claims expressed in this article are solely those of the authors and do not necessarily represent those of their affiliated organizations, or those of the publisher, the editors and the reviewers. Any product that may be evaluated in this article or claim that may be made by its manufacturer is not guaranteed or endorsed by the publisher.
Research integrity at Frontiers
Learn more about the work of our research integrity team to safeguard the quality of each article we publish.