- 1The School of Medicine, University of Electronic Science and Technology of China, Chengdu, China
- 2Department of Ultrasound Medical Center, Sichuan Cancer Hospital and Institute, Sichuan Cancer Center, School of Medicine, University of Electronic Science and Technology of China, Chengdu, China
- 3Institute of Materia Medica, North Sichuan Medical College, Nanchong, China
Hepatocellular carcinoma (HCC) is one of the most common malignant tumors. Hepatectomy, liver transplantation, and ablation are the three radical treatments for early-stage hepatocellular carcinoma (ESHCC), but not all patients are fit for or can tolerate surgery; moreover, liver donors are limited. Therefore, ablation plays an important role in the treatment of ESHCC. However, some studies have shown that ablation has a higher local recurrence (LR) rate than hepatectomy and liver transplantation. The specific mechanism is unknown. The latest perspectives on the mechanism of recurrence after ablation of HCC were described and summarized. In this review, we discussed the possible mechanisms of recurrence after ablation of HCC, including epithelial–mesenchymal transition (EMT), activating autophagy, changes in non-coding RNA, and changes in the tumor microenvironment. A systematic and comprehensive understanding of the mechanism will contribute to the research and development of related treatment, combined with ablation to improve the therapeutic effect in patients with ESHCC.
1 Introduction
HCC is a common malignant tumor in China, with the fourth-highest morbidity and the second-highest mortality rate (1, 2); thus, early diagnosis and treatment are important to improve survival, especially for patients with early-stage hepatocellular carcinoma (ESHCC). The main treatments for ESHCC are liver transplantation, hepatectomy, and ablation. Liver transplantation is one of the radical treatments, but there are some limitations such as the limited liver donors and postoperative immune rejection (3). Child-Pugh A grade is required for liver function in HCC patients receiving hepatectomy. Most ESHCC patients have a history of hepatitis B or liver cirrhosis, and the liver reserve is poor, these patients are at high risk for hepatectomy (4). Ablation, as one of the three radical treatments for ESHCC, has the advantage of being minimally invasive and having limited impact on liver function; thus, inoperable ESHCC patients may choose radical treatment with ablation (5–7). Moreover, there was no difference in overall survival (OS) time between ablation and hepatectomy in ESHCC patients (8). However, some studies have shown that ablation has a higher local recurrence (LR) rate than hepatectomy (9, 10). The mechanism is still unclear. In recent years, many studies have explored the possible mechanism behind the higher LR rate in ESHCC after ablation. In this paper, recent perspectives were reviewed.
2 Commonly Used Local Ablation Treatment
2.1 Thermal Ablation
2.1.1 Radiofrequency Ablation
Radiofrequency ablation (RFA) uses radiofrequency alternating current to generate heat between the percutaneous probe and the surrounding tissues, resulting in an increase in temperature between 60°C and 100°C, which is maintained for several minutes, leading to complete coagulative necrosis in the tumor and surrounding tissues (11, 12). RFA has become the main local ablation method for the treatment of ESHCC due to its advantages (it is safer, has less trauma, is less painful, has better tolerance, has fewer adverse reactions, and has quicker recovery) (13). However, the scope of coagulation necrosis caused by RFA is limited; the complete ablation rate of ESHCC with a diameter of more than 3 cm is significantly decreased; and the rate of LR is higher than surgical resection (14). Some studies have reported that RFA combined with transcatheter arterial chemoembolization (TACE) or other treatments could increase the ablation scope and therapeutic efficacy (15). In addition, several studies have reported that RFA combined with chemotherapy drugs or targeted drugs such as sorafenib can improve the therapeutic effect in HCC and prolong the survival time of patients (16). However, the thermal deposition effect [heat-sink effect (HSE); that is, the temperature of the ablation zone is decreased when tumors are located near blood vessels or bronchia] reduces the efficacy of RFA. Therefore, when the tumor is near blood vessels or bronchia (17, 18), the complete ablation rate of RFA will decrease (19). At the same time, RFA may cause skin cauterization, which is one of the major side effects of RFA (20).
2.1.2 Microwave Ablation
Microwave ablation (MWA), another type of thermal ablation, aims to generate an electromagnetic field of 900–2,500 MHz through an antenna placed in the tumor. The electromagnetic field continuously realigns the polar molecules with inherent dipoles in the tissue with the oscillating electric field, and the rotation of the molecules increases their kinetic energy, thus increasing the temperature in the tissues (21, 22). As early as 2001, MWA has been used in the treatment of ESHCC, and several studies have confirmed that MWA has long-term efficacy and could effectively reduce adverse reactions (23, 24). With the continuous progress of MWA technology, MWA has been used from the initial treatment of small HCC to the combination with TACE in the treatment of large HCC and HCC in special areas, such as tumors near the gallbladder, gastrointestinal tract, large vessels, and hilum (24–27). MWA is an important treatment for ESHCC. Liang et al. have led many innovative studies on the treatment of ESHCC with MWA (28, 29) and have formulated the first guidelines on treating ESHCC via MWA (30), laying a theoretical foundation for clinicians to standardize the operation. Compared with RFA, MWA has a lower HSE rate (31, 32) and may result in fewer instances of skin burn (33). Most importantly, MWA has a larger ablation scope than RFA (34, 35), and research has shown that for HCCs that are 5–7 cm in diameter, the complete ablation rate of MWA is 80%, while that of RFA is only 24% (36).
2.2 Other Ablation Treatments
2.2.1 Cryoablation
The goal of cryoablation (CA) is to form ice in the cells and maintain the temperature for a few minutes/cycles, eventually leading to cell death, with a lethal temperature range of −40 °C to −20 °C (37). Compared with RFA and MWA, CA has a lower incidence of pain (38). Due to the different theory of CA, an ice hockey ablation area (IBAZ) can be formed during ablation, which enables CA to monitor the scope and size of the ablation area in real time through imaging devices such as computed tomography (CT), magnetic resonance imaging (MRI), or ultrasound (39–41). However, about 1% of CA may induce hypothermic shock, some of which are fatal (42). In addition, the long-term efficacy of CA in HCC needs more follow-up data (43).
2.2.2 Irreversible Electroporation
Irreversible electroporation (IRE) destroys the tissues and cell membranes by generating a non-thermal electric field on the ablation probe, thus changing the ion-driven homeostasis, resulting in cell death (44). The greatest advantage of IRE is that it can protect the bile ducts and blood vessels around the ablation area from ablation damage (45). Because IRE has a broad transition zone between surviving tissue and necrotic tissue, it may induce LR (46). Meanwhile, as a new form of ablation, the data on IRE’s applicability to other types of diseases and long-term efficacy are limited; thus, it needs more research to verify its therapeutic effect (47, 48).
Although there are many methods of local ablation, RFA and MWA, two forms of thermal ablation, are mainly used for ESHCC in the clinical setting. Next, we will discuss and summarize the mechanism of HCC recurrence caused by thermal ablation.
3 The Mechanism of Recurrence After Ablation of HCC
3.1 EMT
Epithelial–mesenchymal transition (EMT) refers to the transformation from epithelial cells to mesenchymal cells, the molecules of which are remodeled, and phenotypic changes, including malignant tumor progression, enhance the ability of invasion and metastasis in epithelial-derived tumor cells (49). Molecular marker changes in EMT include losing the expression of epithelial markers such as E-cadherin, upregulation of mesenchymal cell markers such as N-cadherin and Vimentin, and the increasing transcriptional regulatory factors such as Snail, Slug, and Twist (50). To investigate whether EMT occurs in recurrence after ablation in HCC, Yoshida et al. simulated thermal ablation in vitro by sublethal heat stress (SHS); that is, hepatoma cells were placed in a water bath at 45°C and 55°C for 10 min, the morphology of the surviving tumor cells was spindle-like on the 5th day, and the expression of CD133, CK7, CK19, and Snail proteins increased, but returned to the baseline level on the 12th day (51). It showed that the surviving hepatoma cells after SHS are capable of EMT, and further research showed that SHS activated the Erk1/2 signal pathway; inhibiting the Erk1/2 pathway could inhibit the proliferation of hepatoma cells. Dong et al. found that SHS-treated hepatoma cells increased the expression of PCNA, N-cadherin, MMP-2, and MMP-9 through activating the Akt/ERK pathway. Furthermore, an animal experiment showed that the risk of lung metastasis was increased with insufficient ablation (52). One research, through an analysis of specimens from recurrent HCC in patients who have undergone ablation, showed that EMT-related markers TGF-β, Twist, and Snail-1 increased compared with patients without ablation. In order to further confirm the role of EMT in recurrence after thermal ablation of HCC, some researchers constructed an in situ incomplete HCC ablation in a xenograft mouse model and found that E-cadherin was downregulated while N-cadherin and β-catenin were upregulated, and that blocking β-catenin, a key protein of EMT, could reduce the EMT phenotype and metastasis in hepatoma cells (53). As a key protein of EMT, flotillin can activate the Akt/Wnt/β-catenin signal pathway to induce EMT and then enhance the ability of proliferation and invasion in residual hepatoma cells after SHS (54). As a key step to obtaining stronger invasion and metastasis, EMT has been confirmed in hepatoma cells, animal models, and samples from HCC patients, and some studies find some key proteins and important signal pathways leading to EMT (Table 1), which provide the direction for basic research and clinical treatment in the future.
3.2 Promoting Angiogenesis Through the HIF-1α/VEGF Signal Pathway
As an important regulator of hypoxic adaptive response, HIF-1α is highly expressed in hypoxic conditions but is maintained at a low concentration under normoxic conditions (55, 56). HIF-1α is usually more obvious in invasive tumors (56) and can be used as an independent predictor for poor prognosis in HCC (57). HIF-1α plays an important role in promoting proliferation, invasion, angiogenesis, and metastasis in tumors (58). Angiogenesis plays a key role in tumor formation and metastasis (59, 60). HIF-1α directly induces a large number of angiogenesis-related genes, such as the VEGF family. The VEGF family, including VEGFA, VEGFB, VEGFC, and VEGFD, are the strongest angiogenic factors expressed in a variety of tumors (61). Studies have shown that VEGF is highly expressed in HCC (62). The expression of VEGF in HCC tissues or serum implies the vascular invasion and metastasis of HCC, which leads to an increase in the recurrence of HCC after treatment and a decrease in the median survival time of patients (63–66). VEGF is the main regulatory factor of angiogenesis. Previous research has shown that VEGF is highly expressed in tumors, and anti-angiogenesis therapy with VEGF inhibitors can inhibit tumor growth (67). Since bevacizumab (Avastin) has been approved in 2004, Avastin has been shown to be effective against a variety of solid tumors, including HCC (68). After the breakthrough of immunotherapy in some solid tumors, studies have shown that immunotherapy plus anti-angiogenesis can further improve efficacy in HCC patients, and has become the first-line treatment for advanced HCC (69). Sorafenib, another anti-angiogenic drug, is used for advanced HCC, which could prolong survival time in patients with advanced HCC (70). A series of retrospective studies have shown that RFA combined with sorafenib can reduce the postoperative LR rate and improve the survival time of patients with HCC (71, 72). However, a randomized, placebo-controlled, double-blind Phase 3 trial showed that there was no significant difference in relapse-free survival time between sorafenib with RFA and RFA with placebo (73). Compared with parent cells, hepatoma cells treated with SHS increase the expression of HIF-1α and VEGF, and enhance angiogenesis, which can be eliminated by Avastin (74). Liu et al. found that hepatoma cells treated with SHS promoted proliferation by the overexpression of CaMKII/ERK-dependent VEGF. Similarly, in the ectopic liver cancer model, it also showed that HIF-1α, VEGF, and microvessel density (MVD) increased after insufficient ablation (75). Animal models confirm that incomplete ablation promotes angiogenesis through the HIF-1α/VEGF signal pathway leading to tumor invasion and metastasis (76). Tan et al. found that incomplete ablation led to the high expression of VEGFR1 and enhanced angiogenesis, and that targeting VEGFR1 could reduce the ability of proliferation and migration in hepatoma cells (77). Analysis of the samples of patients with recurrent HCC after ablation showed that the level of HIF-1α was higher than without RFA. Survival analysis showed that OS was shorter in patients with recurrence after RFA (78). A series of studies have revealed that promoting angiogenesis enrolls in the recurrence of HCC after ablation by activating the HIF-1α/VEGF signal pathway (Table 2). Anti-angiogenesis through targeted HIF-1α / VEGF pathway may be one of the ways to treat recurrence of HCC after ablation, but its clinical use needs more basic researches and clinical trials.
3.3 Activating Autophagy
Autophagy is a self-protective mechanism in cells, which can degrade cytoplasmic substances such as damaged organelles and proteins (79, 80). Recently, there is considerable evidence showing that autophagy plays a key role in many human diseases, including tumors. Inhibiting autophagy can prevent tumor progression (81). The level of LC3-II (a key autophagy marker) is positively correlated with poor prognosis in HCC (82). Chang et al. found that inhibition of autophagy reduced the survival of hepatoma cells (83). In addition, autophagy can help hepatoma cells survive under adverse conditions and promote invasion and migration (84). Peng et al. confirmed that autophagy induced by hypoxia led to resistance to chemotherapeutic drugs in hepatoma cells (85). Thompson et al. found that SHS-treated hepatoma cells activated the PI3K/mTOR/AKT pathway, an important signal pathway of autophagy, resulting in enhanced proliferation and invasion (86). Jondal et al. also showed that the ability of invasion was enhanced in hepatoma cells by SHS by activating the PI3K/mTOR/AKT pathway, and inhibition of the PI3K/mTOR pathway was an effective approach against invasion (87). The autophagy inhibitor chloroquine (CQ) can inhibit the growth of residual hepatoma cells after SHS treatment (88). Zhao et al. confirmed the role of autophagy in an ectopic animal model for the first time by incomplete ablation [insufficient RFA (IRFA)], which promoted proliferation and invasion in residual hepatoma cells. The autophagy inhibitor hydroxychloroquine (HCQ) can also significantly inhibit the growth of hepatoma cells (89). Autophagy is an important mechanism in the tumorigenesis and progression of malignant tumors; activating autophagy also exists in the recurrence of HCC after ablation (Table 3). In vitro and in vivo, it has been shown that autophagy inhibition can inhibit the progression of HCC after ablation.
3.4 Changes in Non-Coding RNA
Long-stranded non-coding RNA (lncRNA) is usually defined as a transcript with more than 200 nucleotides lacking the function of protein coding. In recent years, nevertheless, lncRNA has shown an important regulatory role in cancer biology, including immune response, tumorigenesis, cell development, and metabolism (90, 91). The expression of lncRNA was detected by a gene chip, and hundreds of lncRNA were abnormally expressed in HCC (Figure 1) (91). It was found that the expression of lncRNA and mRNA in hepatoma cells treated with SHS was significantly changed; about 558 lncRNA and 250 mRNA were upregulated, while 224 lncRNA and 1,031 mRNA were downregulated. Further studies showed that the expression of lncRNA FUNDC2P4 in SHS-treated hepatoma cells promoted EMT by reducing the expression of E-cadherin, which promoted proliferation and invasion in hepatoma cells. The downregulation of miR-200C and miR-34a expression promoted EMT and enhanced the invasiveness of SHS-treated hepatoma cells (91). As a current research hotspot, there are some studies that focused on the change of non-coding RNA in the recurrence of HCC after ablation, and more studies are needed to reveal its particular role in the recurrence of HCC after ablation.
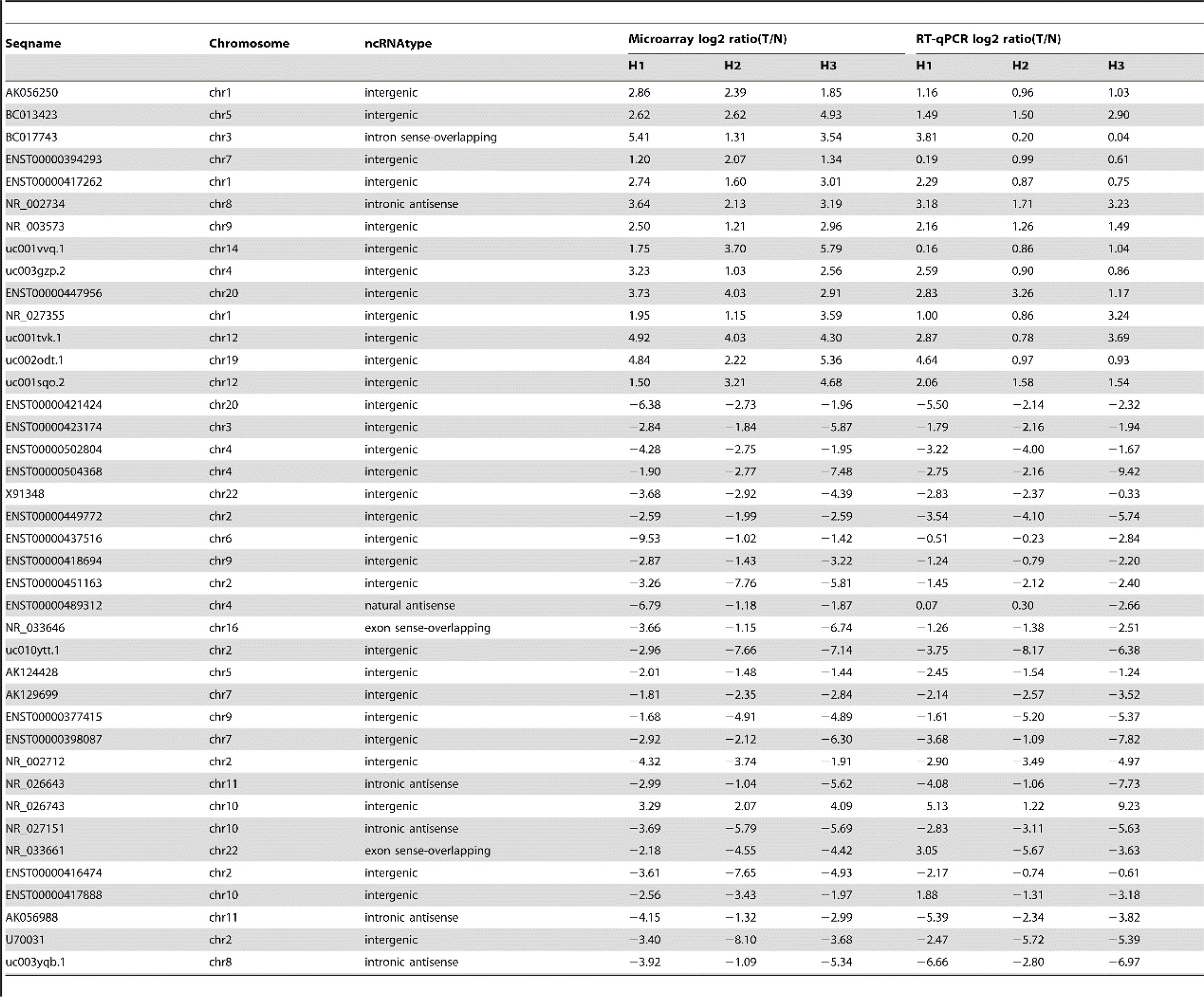
Figure 1 Quantitative RT-PCR confirmation for forty selected lncRNA, cited from Zhu et al. Plos One. 2014 Jul 15;9(7): e101707 (91). .
3.5 Changes in the Tumor Microenvironment
The mechanisms described above are all based on hepatoma cells. However, the tumor microenvironment (TME) also plays an important role in tumorigenesis and tumor progression. TME is rich in activated hepatic stellate cells (HSCs). Evidence shows that the activated HSCs promote hepatoma cells’ proliferation, migration, and invasion (92–95). Periostin (POSTN) is a key protein that activates HSCs and is a perfect anti-fibrosis target (96). The high expression of POSTN can induce EMT and promote proliferation in hepatoma cells (97) and can lead to a worse prognosis in patients with HCC (98). Zhang et al. exposed hepatoma cells to SHS and then cultured them with activated hepatic stellate cell conditioned medium (HSC-CM). Subsequently, there was enhanced invasion of residual hepatoma cells through HSC-CM secreting POSTN. It is proved that POSTN plays a vital role in the recurrence of HCC after ablation (99). It is further found that POSTN induced residual hepatoma cells by SHS, acquiring stem cell characteristics through activating the integrin β-1/AKT/GSK3-β/β-catenin/TCF4/Nanog pathway (100). Zhang et al. also found that the matrix hardness and extracellular matrix type I collagen were involved in the malignant progression in residual hepatoma cells after SHS (101). ERK1/2 inhibitors can reverse ERK phosphorylation induced by extracellular matrix type I collagen (102). TME plays an important role in tumorigenesis and progression, the researches have conducted a preliminary study on the role of TME in recurrence of HCC after ablation (Table 4), but more in-depth studies are needed to find vital proteins and signal pathways.
4 Conclusions
As a minimally invasive, safe, and effective treatment, ablation plays a major role in ESHCC. The mechanism of recurrence of HCC after ablation is mainly focused on EMT, angiogenesis, autophagy activation, and changes in the TME (Figure 2). Current perspectives do not fully elucidate the mechanism of recurrence of HCC after ablation. Most of the studies are in vivo, and some are in vitro. For the establishment of an incomplete tumor ablation model in vitro, most studies used simulated thermal ablation in vitro by SHS. Some scholars doubted whether this method could completely mimic the tumor environment. Furthermore, the temperature and time of the water bath are not the same in different experiments, thus lacking uniform standards (49). In the future, we hope to improve the research method in vitro and in vivo, revealing its distinct mechanism, and providing the theoretical basis for clinical transformation.
Author Contributions
JQY and WG performed the data collection and analysis. JQY, WG, and ML were responsible for the data interpretation. All authors read and approved the final manuscript.
Funding
This study was supported by funds from the National Key Research and Development Program (No. 2019YFE0196700).
Conflict of Interest
The authors declare that the research was conducted in the absence of any commercial or financial relationships that could be construed as a potential conflict of interest.
Publisher’s Note
All claims expressed in this article are solely those of the authors and do not necessarily represent those of their affiliated organizations, or those of the publisher, the editors and the reviewers. Any product that may be evaluated in this article, or claim that may be made by its manufacturer, is not guaranteed or endorsed by the publisher.
Abbreviations
HCC, hepatocellular carcinoma; OS, overall survival; LR, local recurrence; RFA, radiofrequency ablation; HSE, heat-sink effect; MWA, microwave ablation; CA, cryoablation; IBAZ, ice ball ablation zone; IRE, irreversible electroporation; EMT, epithelial–mesenchymal transition; SHS, sublethal heat stress; MVD, microvessel density; CQ, chloroquine; IRFA, insufficient RFA; HCQ, hydroxychloroquine; lncRNA, long non-coding RNA; HSCs, hepatic stellate cells; POSTN, periostin; HSC-CM, hepatic stellate cell conditioned medium.
References
1. Zhou M, Wang H, Zeng X, Yin P, Zhu J, Chen W, et al. Mortality, Morbidity, and Risk Factors in China and its Provinces, 1990-2017: A Systematic Analysis for the Global Burden of Disease Study 2017. Lancet (2019) 394(10204):1145–58. doi: 10.1016/s0140-6736(19)30427-1
2. Zheng RS, Sun KX, Zhang SW, Zeng HM, Zou XN, Chen R, et al. [Report of Cancer Epidemiology in China, 2015]. Zhonghua Zhong Liu Za Zhi (2019) 41(1):19–28. doi: 10.3760/cma.j.issn.0253-3766.2019.01.005
3. Fan J, Yang GS, Fu ZR, Peng ZH, Xia Q, Peng CH, et al. Liver Transplantation Outcomes in 1,078 Hepatocellular Carcinoma Patients: A Multi-Center Experience in Shanghai, China. J Cancer Res Clin Oncol (2009) 135(10):1403–12. doi: 10.1007/s00432-009-0584-6
4. Mohkam K, Dumont PN, Manichon AF, Jouvet JC, Boussel L, Merle P, et al. No-Touch Multibipolar Radiofrequency Ablation vs. Surgical Resection for Solitary Hepatocellular Carcinoma Ranging From 2 to 5 Cm. J Hepatol (2018) 68(6):1172–80. doi: 10.1016/j.jhep.2018.01.014
5. Heimbach JK, Kulik LM, Finn RS, Sirlin CB, Abecassis MM, Roberts LR, et al. AASLD Guidelines for the Treatment of Hepatocellular Carcinoma. Hepatology (2018) 67(1):358–80. doi: 10.1002/hep.29086
6. Salmi A, Turrini R, Lanzani G, Savio A, Anglani L. Efficacy of Radiofrequency Ablation of Hepatocellular Carcinoma Associated With Chronic Liver Disease Without Cirrhosis. Int J Med Sci (2008) 5(6):327–32. doi: 10.7150/ijms.5.327
7. Bruix J, Sherman M. Management of Hepatocellular Carcinoma: An Update. Hepatology (2011) 53(3):1020–2. doi: 10.1002/hep.24199
8. Fang Y, Chen W, Liang X, Li D, Lou H, Chen R, et al. Comparison of Long-Term Effectiveness and Complications of Radiofrequency Ablation With Hepatectomy for Small Hepatocellular Carcinoma. J Gastroenterol Hepatol (2014) 29(1):193–200. doi: 10.1111/jgh.12441
9. Lencioni R, Cioni D, Crocetti L, Franchini C, Pina CD, Lera J, et al. Early-Stage Hepatocellular Carcinoma in Patients With Cirrhosis: Long-Term Results of Percutaneous Image-Guided Radiofrequency Ablation. Radiology (2005) 234(3):961–7. doi: 10.1148/radiol.2343040350
10. Rozenblum N, Zeira E, Scaiewicz V, Bulvik B, Gourevitch S, Yotvat H, et al. Oncogenesis: An "Off-Target" Effect of Radiofrequency Ablation. Radiology (2015) 276(2):426–32. doi: 10.1148/radiol.2015141695
11. Goldberg SN, Gazelle GS, Mueller PR. Thermal Ablation Therapy for Focal Malignancy: A Unified Approach to Underlying Principles, Techniques, and Diagnostic Imaging Guidance. AJR Am J Roentgenol (2000) 174(2):323–31. doi: 10.2214/ajr.174.2.1740323
12. McGahan JP, Gu WZ, Brock JM, Tesluk H, Jones CD. Hepatic Ablation Using Bipolar Radiofrequency Electrocautery. Acad Radiol (1996) 3(5):418–22. doi: 10.1016/s1076-6332(05)80677-4
13. Doyle A, Gorgen A, Muaddi H, Aravinthan AD, Issachar A, Mironov O, et al. Outcomes of Radiofrequency Ablation as First-Line Therapy for Hepatocellular Carcinoma Less Than 3 Cm in Potentially Transplantable Patients. J Hepatol (2019) 70(5):866–73. doi: 10.1016/j.jhep.2018.12.027
14. Mulier S, Ni Y, Jamart J, Ruers T, Marchal G, Michel L. Local Recurrence After Hepatic Radiofrequency Coagulation: Multivariate Meta-Analysis and Review of Contributing Factors. Ann Surg (2005) 242(2):158–71. doi: 10.1097/01.sla.0000171032.99149.fe
15. Zuo TY, Liu FY, Wang MQ, Chen XX. Transcatheter Arterial Chemoembolization Combined With Simultaneous Computed Tomography-Guided Radiofrequency Ablation for Large Hepatocellular Carcinomas. Chin Med J (Engl) (2017) 130(22):2666–73. doi: 10.4103/0366-6999.218002
16. Yang W, Lee JC, Chen MH, Zhang ZY, Bai XM, Yin SS, et al. Thermosensitive Liposomal Doxorubicin Plus Radiofrequency Ablation Increased Tumor Destruction and Improved Survival in Patients With Medium and Large Hepatocellular Carcinoma: A Randomized, Double-Blinded, Dummy-Controlled Clinical Trial in a Single Center. J Cancer Res Ther (2019) 15(4):773–83. doi: 10.4103/jcrt.JCRT_801_18
17. Ghosn M, Solomon SB. Current Management of Oligometastatic Lung Cancer and Future Perspectives: Results of Thermal Ablation as a Local Ablative Therapy. Cancers (Basel) 13(20) (2021). doi: 10.3390/cancers13205202
18. Lu DS, Raman SS, Limanond P, Aziz D, Economou J, Busuttil R, et al. Influence of Large Peritumoral Vessels on Outcome of Radiofrequency Ablation of Liver Tumors. J Vasc Interv Radiol (2003) 14(10):1267–74. doi: 10.1097/01.rvi.0000092666.72261.6b
19. Chu KF, Dupuy DE. Thermal Ablation of Tumours: Biological Mechanisms and Advances in Therapy. Nat Rev Cancer (2014) 14(3):199–208. doi: 10.1038/nrc3672
20. Huffman SD, Huffman NP, Lewandowski RJ, Brown DB. Radiofrequency Ablation Complicated by Skin Burn. Semin Intervent Radiol (2011) 28(2):179–82. doi: 10.1055/s-0031-1280660
21. Lubner MG, Brace CL, Hinshaw JL, Lee FT Jr. Microwave Tumor Ablation: Mechanism of Action, Clinical Results, and Devices. J Vasc Interv Radiol (2010) 21(8 Suppl):S192–203. doi: 10.1016/j.jvir.2010.04.007
22. Carrafiello G, Laganà D, Mangini M, Fontana F, Dionigi G, Boni L, et al. Microwave Tumors Ablation: Principles, Clinical Applications and Review of Preliminary Experiences. Int J Surg (2008) 6 Suppl:1, S65–9. doi: 10.1016/j.ijsu.2008.12.028
23. Lu MD, Chen JW, Xie XY, Liu L, Huang XQ, Liang LJ, et al. Hepatocellular Carcinoma: US-Guided Percutaneous Microwave Coagulation Therapy. Radiology (2001) 221(1):167–72. doi: 10.1148/radiol.2211001783
24. Huang S, Yu J, Liang P, Yu X, Cheng Z, Han Z, et al. Percutaneous Microwave Ablation for Hepatocellular Carcinoma Adjacent to Large Vessels: A Long-Term Follow-Up. Eur J Radiol (2014) 83(3):552–8. doi: 10.1016/j.ejrad.2013.12.015
25. Ni JY, Sun HL, Chen YT, Luo JH, Chen D, Jiang XY, et al. Prognostic Factors for Survival After Transarterial Chemoembolization Combined With Microwave Ablation for Hepatocellular Carcinoma. World J Gastroenterol (2014) 20(46):17483–90. doi: 10.3748/wjg.v20.i46.17483
26. Li M, Li Z, Yu X, Liang P, Gao Y, Han Z, et al. Percutaneous Radio-Frequency Ablation of Hepatocellular Carcinoma Adjacent to the Gastrointestinal Tract. Int J Hyperthermia (2016) 32(6):600–6. doi: 10.3109/02656736.2016.1157904
27. Li M, Yu X, Liang P, Dong B, Liu F. Ultrasound-Guided Percutaneous Microwave Ablation for Hepatic Malignancy Adjacent to the Gallbladder. Int J Hyperthermia (2015) 31(6):579–87. doi: 10.3109/02656736.2015.1014869
28. Zhou Q, Gong N, Zhang D, Li J, Han X, Dou J, et al. Mannose-Derived Carbon Dots Amplify Microwave Ablation-Induced Antitumor Immune Responses by Capturing and Transferring "Danger Signals" to Dendritic Cells. ACS Nano (2021) 15(2):2920–32. doi: 10.1021/acsnano.0c09120
29. Zhou Q, Wu S, Gong N, Li X, Dou J, Mu M, et al. Liposomes Loading Sodium Chloride as Effective Thermo-Seeds for Microwave Ablation of Hepatocellular Carcinoma. Nanoscale (2017) 9(31):11068–76. doi: 10.1039/c7nr02955a
30. Liang P, Wang Y, Yu X, Dong B. Malignant Liver Tumors: Treatment With Percutaneous Microwave Ablation–Complications Among Cohort of 1136 Patients. Radiology (2009) 251(3):933–40. doi: 10.1148/radiol.2513081740
31. Dodd GD 3rd, Soulen MC, Kane RA, Livraghi T, Lees WR, Yamashita Y, et al. Minimally Invasive Treatment of Malignant Hepatic Tumors: At the Threshold of a Major Breakthrough. Radiographics (2000) 20(1):9–27. doi: 10.1148/radiographics.20.1.g00ja019
32. Bhardwaj N, Strickland AD, Ahmad F, Atanesyan L, West K, Lloyd DM. A Comparative Histological Evaluation of the Ablations Produced by Microwave, Cryotherapy and Radiofrequency in the Liver. Pathology (2009) 41(2):168–72. doi: 10.1080/00313020802579292
33. Wright AS, Sampson LA, Warner TF, Mahvi DM, Lee FT Jr. Radiofrequency Versus Microwave Ablation in a Hepatic Porcine Model. Radiology (2005) 236(1):132–9. doi: 10.1148/radiol.2361031249
34. Wright AS, Lee FT Jr., Mahvi DM. Hepatic Microwave Ablation With Multiple Antennae Results in Synergistically Larger Zones of Coagulation Necrosis. Ann Surg Oncol (2003) 10(3):275–83. doi: 10.1245/aso.2003.03.045
35. Yin XY, Xie XY, Lu MD, Xu HX, Xu ZF, Kuang M, et al. Percutaneous Thermal Ablation of Medium and Large Hepatocellular Carcinoma: Long-Term Outcome and Prognostic Factors. Cancer (2009) 115(9):1914–23. doi: 10.1002/cncr.24196
36. Livraghi T, Goldberg SN, Lazzaroni S, Meloni F, Ierace T, Solbiati L, et al. Hepatocellular Carcinoma: Radio-Frequency Ablation of Medium and Large Lesions. Radiology (2000) 214(3):761–8. doi: 10.1148/radiology.214.3.r00mr02761
37. Gage AA, Baust J. Mechanisms of Tissue Injury in Cryosurgery. Cryobiology (1998) 37(3):171–86. doi: 10.1006/cryo.1998.2115
38. Permpongkosol S, Sulman A, Solomon SB, Gong GX, Kavoussi LR. Percutaneous Computerized Tomography Guided Renal Cryoablation Using Local Anesthesia: Pain Assessment. J Urol (2006) 176(3):915–8. doi: 10.1016/j.juro.2006.04.035
39. Gilbert JC, Onik GM, Hoddick WK, Rubinsky B. Real Time Ultrasonic Monitoring of Hepatic Cryosurgery. Cryobiology (1985) 22(4):319–30. doi: 10.1016/0011-2240(85)90179-8
40. Lee FT Jr., Chosy SG, Littrup PJ, Warner TF, Kuhlman JE, Mahvi DM. CT-Monitored Percutaneous Cryoablation in a Pig Liver Model: Pilot Study. Radiology (1999) 211(3):687–92. doi: 10.1148/radiology.211.3.r99jn29687
41. Silverman SG, Sun MR, Tuncali K, Morrison PR, vanSonnenberg E, Shankar S, et al. Three-Dimensional Assessment of MRI-Guided Percutaneous Cryotherapy of Liver Metastases. AJR Am J Roentgenol (2004) 183(3):707–12. doi: 10.2214/ajr.183.3.1830707
42. Seifert JK, Morris DL. World Survey on the Complications of Hepatic and Prostate Cryotherapy. World J Surg (1999) 23(2):109–13; discussion 113-4. doi: 10.1007/pl00013173
43. Xu KC, Niu LZ, Zhou Q, Hu YZ, Guo DH, Liu ZP, et al. Sequential Use of Transarterial Chemoembolization and Percutaneous Cryosurgery for Hepatocellular Carcinoma. World J Gastroenterol (2009) 15(29):3664–9. doi: 10.3748/wjg.15.3664
44. Lee EW, Chen C, Prieto VE, Dry SM, Loh CT, Kee ST. Advanced Hepatic Ablation Technique for Creating Complete Cell Death: Irreversible Electroporation. Radiology (2010) 255(2):426–33. doi: 10.1148/radiol.10090337
45. Seror O. Ablative Therapies: Advantages and Disadvantages of Radiofrequency, Cryotherapy, Microwave and Electroporation Methods, or How to Choose the Right Method for an Individual Patient? Diagn Interv Imaging (2015) 96(6):617–24. doi: 10.1016/j.diii.2015.04.007
46. Cornelis FH, Durack JC, Kimm SY, Wimmer T, Coleman JA, Solomon SB, et al. A Comparative Study of Ablation Boundary Sharpness After Percutaneous Radiofrequency, Cryo-, Microwave, and Irreversible Electroporation Ablation in Normal Swine Liver and Kidneys. Cardiovasc Intervent Radiol (2017) 40(10):1600–8. doi: 10.1007/s00270-017-1692-3
47. Niessen C, Beyer LP, Pregler B, Dollinger M, Trabold B, Schlitt HJ, et al. Percutaneous Ablation of Hepatic Tumors Using Irreversible Electroporation: A Prospective Safety and Midterm Efficacy Study in 34 Patients. J Vasc Interv Radiol (2016) 27(4):480–6. doi: 10.1016/j.jvir.2015.12.025
48. Niessen C, Thumann S, Beyer L, Pregler B, Kramer J, Lang S, et al. Percutaneous Irreversible Electroporation: Long-Term Survival Analysis of 71 Patients With Inoperable Malignant Hepatic Tumors. Sci Rep (2017) 7:43687. doi: 10.1038/srep43687
49. Cheng J, Li M, Lv Y. Sublethal Heat Treatment Promotes Epithelial-Mesenchymal Transition and Enhances the Malignant Potential of Hepatocellular Carcinoma. Hepatology (2014) 59(4):1650. doi: 10.1002/hep.26630
50. Olea-Flores M, Zuñiga-Eulogio MD, Mendoza-Catalán MA, Rodríguez-Ruiz HA, Castañeda-Saucedo E, Ortuño-Pineda C, et al. Extracellular-Signal Regulated Kinase: A Central Molecule Driving Epithelial-Mesenchymal Transition in Cancer. Int J Mol Sci (2019) 20(12). doi: 10.3390/ijms20122885
51. Yoshida S, Kornek M, Ikenaga N, Schmelzle M, Masuzaki R, Csizmadia E, et al. Sublethal Heat Treatment Promotes Epithelial-Mesenchymal Transition and Enhances the Malignant Potential of Hepatocellular Carcinoma. Hepatology (2013) 58(5):1667–80. doi: 10.1002/hep.26526
52. Dong S, Kong J, Kong F, Kong J, Gao J, Ke S, et al. Insufficient Radiofrequency Ablation Promotes Epithelial-Mesenchymal Transition of Hepatocellular Carcinoma Cells Through Akt and ERK Signaling Pathways. J Transl Med (2013) 11:273. doi: 10.1186/1479-5876-11-273
53. Zhang N, Wang L, Chai ZT, Zhu ZM, Zhu XD, Ma DN, et al. Incomplete Radiofrequency Ablation Enhances Invasiveness and Metastasis of Residual Cancer of Hepatocellular Carcinoma Cell HCCLM3 via Activating β-Catenin Signaling. PLoS One (2014) 9(12):e115949. doi: 10.1371/journal.pone.0115949
54. Zhang N, Li H, Qin C, Ma D, Zhao Y, Zhu W, et al. Insufficient Radiofrequency Ablation Promotes the Metastasis of Residual Hepatocellular Carcinoma Cells via Upregulating Flotillin Proteins. J Cancer Res Clin Oncol (2019) 145(4):895–907. doi: 10.1007/s00432-019-02852-z
55. Altun M, Zhao B, Velasco K, Liu H, Hassink G, Paschke J, et al. Ubiquitin-Specific Protease 19 (USP19) Regulates Hypoxia-Inducible Factor 1α (HIF-1α) During Hypoxia. J Biol Chem (2012) 287(3):1962–9. doi: 10.1074/jbc.M111.305615
56. Zagzag D, Zhong H, Scalzitti JM, Laughner E, Simons JW, Semenza GL. Expression of Hypoxia-Inducible Factor 1alpha in Brain Tumors: Association With Angiogenesis, Invasion, and Progression. Cancer (2000) 88(11):2606–18. doi: 10.1002/1097-0142(20000601)88:11<2606::AID-CNCR25>3.0.CO;2-W
57. Xiang ZL, Zeng ZC, Fan J, Tang ZY, Zeng HY, Gao DM. Gene Expression Profiling of Fixed Tissues Identified Hypoxia-Inducible Factor-1α, VEGF, and Matrix Metalloproteinase-2 as Biomarkers of Lymph Node Metastasis in Hepatocellular Carcinoma. Clin Cancer Res (2011) 17(16):5463–72. doi: 10.1158/1078-0432.Ccr-10-3096
58. Vecchietti V, Casagrande C, Ferrari G, Severini Ricca G. New Aporphine Alkaloids of Ocotea Minarum. Farmaco Sci (1979) 34(10):829–40.
59. Bao S, Wu Q, Sathornsumetee S, Hao Y, Li Z, Hjelmeland AB, et al. Stem Cell-Like Glioma Cells Promote Tumor Angiogenesis Through Vascular Endothelial Growth Factor. Cancer Res (2006) 66(16):7843–8. doi: 10.1158/0008-5472.Can-06-1010
60. Carmeliet P, Dor Y, Herbert JM, Fukumura D, Brusselmans K, Dewerchin M, et al. Role of HIF-1alpha in Hypoxia-Mediated Apoptosis, Cell Proliferation and Tumour Angiogenesis. Nature (1998) 394(6692):485–90. doi: 10.1038/28867
61. Li T, Kang G, Wang T, Huang H. Tumor Angiogenesis and Anti-Angiogenic Gene Therapy for Cancer. Oncol Lett (2018) 16(1):687–702. doi: 10.3892/ol.2018.8733
62. Kaseb AO, Hanbali A, Cotant M, Hassan MM, Wollner I, Philip PA. Vascular Endothelial Growth Factor in the Management of Hepatocellular Carcinoma: A Review of Literature. Cancer (2009) 115(21):4895–906. doi: 10.1002/cncr.24537
63. Poon RT, Lau CP, Cheung ST, Yu WC, Fan ST. Quantitative Correlation of Serum Levels and Tumor Expression of Vascular Endothelial Growth Factor in Patients With Hepatocellular Carcinoma. Cancer Res (2003) 63(12):3121–6.
64. Sheen IS, Jeng KS, Shih SC, Kao CR, Chang WH, Wang HY, et al. Clinical Significance of the Expression of Isoform 165 Vascular Endothelial Growth Factor mRNA in Noncancerous Liver Remnants of Patients With Hepatocellular Carcinoma. World J Gastroenterol (2005) 11(2):187–92. doi: 10.3748/wjg.v11.i2.187
65. Guo RP, Zhong C, Shi M, Zhang CQ, Wei W, Zhang YQ, et al. Clinical Value of Apoptosis and Angiogenesis Factors in Estimating the Prognosis of Hepatocellular Carcinoma. J Cancer Res Clin Oncol (2006) 132(9):547–55. doi: 10.1007/s00432-006-0097-5
66. Kaseb AO, Morris JS, Hassan MM, Siddiqui AM, Lin E, Xiao L, et al. Clinical and Prognostic Implications of Plasma Insulin-Like Growth Factor-1 and Vascular Endothelial Growth Factor in Patients With Hepatocellular Carcinoma. J Clin Oncol (2011) 29(29):3892–9. doi: 10.1200/jco.2011.36.0636
67. Schmitt M, Horbach A, Kubitz R, Frilling A, Häussinger D. Disruption of Hepatocellular Tight Junctions by Vascular Endothelial Growth Factor (VEGF): A Novel Mechanism for Tumor Invasion. J Hepatol (2004) 41(2):274–83. doi: 10.1016/j.jhep.2004.04.035
68. Bhoori S, Mazzaferro V. Combined Immunotherapy and VEGF-Antagonist in Hepatocellular Carcinoma: A Step Forward. Lancet Oncol (2020) 21(6):740–1. doi: 10.1016/s1470-2045(20)30211-4
69. Sun X, Zhang Q, Mei J, Yang Z, Chen M, Liang T. Real-World Efficiency of Lenvatinib Plus PD-1 Blockades in Advanced Hepatocellular Carcinoma: An Exploration for Expanded Indications. BMC Cancer (2022) 22(1):293. doi: 10.1186/s12885-022-09405-7
70. Rimassa L, Santoro A. Sorafenib Therapy in Advanced Hepatocellular Carcinoma: The SHARP Trial. Expert Rev Anticancer Ther (2009) 9(6):739–45. doi: 10.1586/era.09.41
71. Fukuda H, Numata K, Moriya S, Shimoyama Y, Ishii T, Nozaki A, et al. Hepatocellular Carcinoma: Concomitant Sorafenib Promotes Necrosis After Radiofrequency Ablation–Propensity Score Matching Analysis. Radiology (2014) 272(2):598–604. doi: 10.1148/radiol.14131640
72. Zhu K, Huang J, Lai L, Huang W, Cai M, Zhou J, et al. Medium or Large Hepatocellular Carcinoma: Sorafenib Combined With Transarterial Chemoembolization and Radiofrequency Ablation. Radiology (2018) 288(1):300–7. doi: 10.1148/radiol.2018172028
73. Bruix J, Takayama T, Mazzaferro V, Chau GY, Yang J, Kudo M, et al. Adjuvant Sorafenib for Hepatocellular Carcinoma After Resection or Ablation (STORM): A Phase 3, Randomised, Double-Blind, Placebo-Controlled Trial. Lancet Oncol (2015) 16(13):1344–54. doi: 10.1016/s1470-2045(15)00198-9
74. Kong J, Kong J, Pan B, Ke S, Dong S, Li X, et al. Insufficient Radiofrequency Ablation Promotes Angiogenesis of Residual Hepatocellular Carcinoma via HIF-1α/VEGFA. PLoS One (2012) 7(5):e37266. doi: 10.1371/journal.pone.0037266
75. Liu Z, Dai H, Jia G, Li Y, Liu X, Ren W. Insufficient Radiofrequency Ablation Promotes Human Hepatoma SMMC7721 Cell Proliferation by Stimulating Vascular Endothelial Growth Factor Overexpression. Oncol Lett (2015) 9(4):1893–6. doi: 10.3892/ol.2015.2966
76. Wu L, Fu Z, Zhou S, Gong J, Liu CA, Qiao Z, et al. HIF-1α and HIF-2α: Siblings in Promoting Angiogenesis of Residual Hepatocellular Carcinoma After High-Intensity Focused Ultrasound Ablation. PLoS One (2014) 9(2):e88913. doi: 10.1371/journal.pone.0088913
77. Tan L, Chen S, Wei G, Li Y, Liao J, Jin H, et al. Sublethal Heat Treatment of Hepatocellular Carcinoma Promotes Intrahepatic Metastasis and Stemness in a VEGFR1-Dependent Manner. Cancer Lett (2019) 460:29–40. doi: 10.1016/j.canlet.2019.05.041
78. Yamada S, Utsunomiya T, Morine Y, Imura S, Ikemoto T, Arakawa Y, et al. Expressions of Hypoxia-Inducible Factor-1 and Epithelial Cell Adhesion Molecule are Linked With Aggressive Local Recurrence of Hepatocellular Carcinoma After Radiofrequency Ablation Therapy. Ann Surg Oncol (2014) 21 Suppl:3, S436–42. doi: 10.1245/s10434-014-3575-z
79. Meng YC, Lou XL, Yang LY, Li D, Hou YQ. Role of the Autophagy-Related Marker LC3 Expression in Hepatocellular Carcinoma: A Meta-Analysis. J Cancer Res Clin Oncol (2020) 146(5):1103–13. doi: 10.1007/s00432-020-03174-1
80. Wang K, Liu R, Li J, Mao J, Lei Y, Wu J, et al. Quercetin Induces Protective Autophagy in Gastric Cancer Cells: Involvement of Akt-mTOR- and Hypoxia-Induced Factor 1α-Mediated Signaling. Autophagy (2011) 7(9):966–78. doi: 10.4161/auto.7.9.15863
81. Yang S, Wang X, Contino G, Liesa M, Sahin E, Ying H, et al. Pancreatic Cancers Require Autophagy for Tumor Growth. Genes Dev (2011) 25(7):717–29. doi: 10.1101/gad.2016111
82. Wu DH, Jia CC, Chen J, Lin ZX, Ruan DY, Li X, et al. Autophagic LC3B Overexpression Correlates With Malignant Progression and Predicts a Poor Prognosis in Hepatocellular Carcinoma. Tumour Biol (2014) 35(12):12225–33. doi: 10.1007/s13277-014-2531-7
83. Chang Y, Yan W, He X, Zhang L, Li C, Huang H, et al. miR-375 Inhibits Autophagy and Reduces Viability of Hepatocellular Carcinoma Cells Under Hypoxic Conditions. Gastroenterology (2012) 143(1):177–87.e8. doi: 10.1053/j.gastro.2012.04.009
84. Peng WX, Xiong EM, Ge L, Wan YY, Zhang CL, Du FY, et al. Egr-1 Promotes Hypoxia-Induced Autophagy to Enhance Chemo-Resistance of Hepatocellular Carcinoma Cells. Exp Cell Res (2016) 340(1):62–70. doi: 10.1016/j.yexcr.2015.12.006
85. Pinheiro C, Longatto-Filho A, Scapulatempo C, Ferreira L, Martins S, Pellerin L, et al. Increased Expression of Monocarboxylate Transporters 1, 2, and 4 in Colorectal Carcinomas. Virchows Arch (2008) 452(2):139–46. doi: 10.1007/s00428-007-0558-5
86. Thompson SM, Callstrom MR, Jondal DE, Butters KA, Knudsen BE, Anderson JL, et al. Heat Stress-Induced PI3K/mTORC2-Dependent AKT Signaling Is a Central Mediator of Hepatocellular Carcinoma Survival to Thermal Ablation Induced Heat Stress. PLoS One (2016) 11(9):e0162634. doi: 10.1371/journal.pone.0162634
87. Jondal DE, Thompson SM, Butters KA, Knudsen BE, Anderson JL, Carter RE, et al. Heat Stress and Hepatic Laser Thermal Ablation Induce Hepatocellular Carcinoma Growth: Role of PI3K/mTOR/AKT Signaling. Radiology (2018) 288(3):730–8. doi: 10.1148/radiol.2018172944
88. Jiang J, Chen S, Li K, Zhang C, Tan Y, Deng Q, et al. Targeting Autophagy Enhances Heat Stress-Induced Apoptosis via the ATP-AMPK-mTOR Axis for Hepatocellular Carcinoma. Int J Hyperthermia (2019) 36(1):499–510. doi: 10.1080/02656736.2019.1600052
89. Zhao Z, Wu J, Liu X, Liang M, Zhou X, Ouyang S, et al. Insufficient Radiofrequency Ablation Promotes Proliferation of Residual Hepatocellular Carcinoma via Autophagy. Cancer Lett (2018) 421:73–81. doi: 10.1016/j.canlet.2018.02.024
90. Schmitt AM, Chang HY. Long Noncoding RNAs in Cancer Pathways. Cancer Cell (2016) 29(4):452–63. doi: 10.1016/j.ccell.2016.03.010
91. Zhu J, Liu S, Ye F, Shen Y, Tie Y, Zhu J, et al. The Long Noncoding RNA Expression Profile of Hepatocellular Carcinoma Identified by Microarray Analysis. PLoS One (2014) 9(7):e101707. doi: 10.1371/journal.pone.0101707
92. Amann T, Bataille F, Spruss T, Mühlbauer M, Gäbele E, Schölmerich J, et al. Activated Hepatic Stellate Cells Promote Tumorigenicity of Hepatocellular Carcinoma. Cancer Sci (2009) 100(4):646–53. doi: 10.1111/j.1349-7006.2009.01087.x
93. Coulouarn C, Corlu A, Glaise D, Guénon I, Thorgeirsson SS, Clément B. Hepatocyte-Stellate Cell Cross-Talk in the Liver Engenders a Permissive Inflammatory Microenvironment That Drives Progression in Hepatocellular Carcinoma. Cancer Res (2012) 72(10):2533–42. doi: 10.1158/0008-5472.Can-11-3317
94. Han S, Han L, Yao Y, Sun H, Zan X, Liu Q. Activated Hepatic Stellate Cells Promote Hepatocellular Carcinoma Cell Migration and Invasion via the Activation of FAK-MMP9 Signaling. Oncol Rep (2014) 31(2):641–8. doi: 10.3892/or.2013.2872
95. Mazzocca A, Dituri F, Lupo L, Quaranta M, Antonaci S, Giannelli G. Tumor-Secreted Lysophostatidic Acid Accelerates Hepatocellular Carcinoma Progression by Promoting Differentiation of Peritumoral Fibroblasts in Myofibroblasts. Hepatology (2011) 54(3):920–30. doi: 10.1002/hep.24485
96. Hong L, Shejiao D, Fenrong C, Gang Z, Lei D. Periostin Down-Regulation Attenuates the Pro-Fibrogenic Response of Hepatic Stellate Cells Induced by TGF-β1. J Cell Mol Med (2015) 19(10):2462–8. doi: 10.1111/jcmm.12636
97. Ruan K, Bao S, Ouyang G. The Multifaceted Role of Periostin in Tumorigenesis. Cell Mol Life Sci (2009) 66(14):2219–30. doi: 10.1007/s00018-009-0013-7
98. Lv Y, Wang W, Jia WD, Sun QK, Li JS, Ma JL, et al. High-Level Expression of Periostin is Closely Related to Metastatic Potential and Poor Prognosis of Hepatocellular Carcinoma. Med Oncol (2013) 30(1):385. doi: 10.1007/s12032-012-0385-7
99. Zhang R, Lin XH, Ma M, Chen J, Chen J, Gao DM, et al. Periostin Involved in the Activated Hepatic Stellate Cells-Induced Progression of Residual Hepatocellular Carcinoma After Sublethal Heat Treatment: Its Role and Potential for Therapeutic Inhibition. J Transl Med (2018) 16(1):302. doi: 10.1186/s12967-018-1676-3
100. Zhang R, Yao RR, Li JH, Dong G, Ma M, Zheng QD, et al. Activated Hepatic Stellate Cells Secrete Periostin to Induce Stem Cell-Like Phenotype of Residual Hepatocellular Carcinoma Cells After Heat Treatment. Sci Rep (2017) 7(1):2164. doi: 10.1038/s41598-017-01177-6
101. Zhang R, Ma M, Dong G, Yao RR, Li JH, Zheng QD, et al. Increased Matrix Stiffness Promotes Tumor Progression of Residual Hepatocellular Carcinoma After Insufficient Heat Treatment. Cancer Sci (2017) 108(9):1778–86. doi: 10.1111/cas.13322
Keywords: hepatocellular carcinoma, early stage, ablation, recurrence, mechanism
Citation: Yang J, Guo W and Lu M (2022) Recent Perspectives on the Mechanism of Recurrence After Ablation of Hepatocellular Carcinoma: A Mini-Review. Front. Oncol. 12:895678. doi: 10.3389/fonc.2022.895678
Received: 14 March 2022; Accepted: 16 May 2022;
Published: 23 August 2022.
Edited by:
Kelvin K.C. Ng, The Chinese University of Hong Kong, Hong Kong SAR, ChinaReviewed by:
Xiaoling Yu, Chinese People’s Liberation Army General Hospital, ChinaCopyright © 2022 Yang, Guo and Lu. This is an open-access article distributed under the terms of the Creative Commons Attribution License (CC BY). The use, distribution or reproduction in other forums is permitted, provided the original author(s) and the copyright owner(s) are credited and that the original publication in this journal is cited, in accordance with accepted academic practice. No use, distribution or reproduction is permitted which does not comply with these terms.
*Correspondence: Man Lu, bnNjbWNwaGR5anFAMTYzLmNvbQ==