- 1The First Affiliated Hospital of University of Science and Technology of China (USTC), Division of Life Sciences and Medicine, University of Science and Technology of China, Hefei, China
- 2Department of Gynecology, Harbin Medical University Cancer Hospital, Harbin, China
- 3Department of Gynecology, Bengbu Medical College Bengbu, Anhui, China
- 4The First Affiliated Hospital of University of Science and Technology of China (USTC), Division of Life Sciences and Medicine, University of Science and Technology of China, Anhui Provincial Cancer Hospital, Hefei, China
Cancer cells typically exhibit a tightly regulated program of metabolic plasticity and epigenetic remodeling to meet the demand of uncontrolled cell proliferation. The metabolic–epigenetic axis has recently become an increasingly hot topic in carcinogenesis and offers new avenues for innovative and personalized cancer treatment strategies. Nicotinamide N-methyltransferase (NNMT) is a metabolic enzyme involved in controlling methylation potential, impacting DNA and histone epigenetic modification. NNMT overexpression has been described in various solid cancer tissues and even body fluids, including serum, urine, and saliva. Furthermore, accumulating evidence has shown that NNMT knockdown significantly decreases tumorigenesis and chemoresistance capacity. Most importantly, the natural NNMT inhibitor yuanhuadine can reverse epidermal growth factor receptor tyrosine kinase inhibitor resistance in lung cancer cells. In this review, we evaluate the possibility of NNMT as a diagnostic biomarker and molecular target for effective anticancer treatment. We also reveal the exact mechanisms of how NNMT affects epigenetics and the development of more potent and selective inhibitors.
Introduction
Cancer is one of the leading causes of death worldwide. GLOBOCAN 2020 has described the most recent changes in cancer burden and the importance of global cancer prevention and care (1). As many cancers lack specific symptoms, many patients are already in the advanced stage when the cancer is detected. Therefore, biomarkers that can be used for early diagnosis and detecting the therapeutic response are extremely important. Recently, the development of immunotherapy and targeted therapy has greatly improved patient prognosis, but acquired drug resistance needs to be addressed (2). Accordingly, more importance has been attached to cancer biological research, which aids the discovery of novel biomarkers, defining more effective molecular targets, and developing new treatment strategies.
Nicotinamide N-methyltransferase (NNMT) was identified as a cytoplasmic enzyme involved in methylating nicotinamide (NAM) and other structurally related compounds such as pyridines. NNMT coverts NAM into 1-MNA (1-methylnicotinamide), which is oxidized to N1-methyl-2-pyridone-5-carboxamide (2PY) and N1-methyl-4-pyridone-3-carboxamide (4PY) by AOX1 and eventually eliminated in the urine (3). However, recent findings have redefined NNMT function in physiology and disease. NNMT-mediated NAM and S-adenosyl methionine (SAM) depletion contributes to metabolic and epigenetic reprogramming by influencing epigenetic modifications and participating in different signaling pathways. This has yielded a broader understanding of various diseases, including metabolic disorder (4, 5), cancer (6–8), neurodegenerative diseases (9), and even endothelial disorder (10).
NNMT increases energy expenditure in a cell-autonomous manner by regulating histone methylation, polyamine flux, and SIRT protein, which suggests that NNMT is a potential target of diet-induced obesity and type 2 diabetes (11). NNMT is overexpressed in the stroma and body fluids and is associated with key prognostic parameters. It could be a novel, sensitive, and specific biomarker for aiding cancer detection (12, 13). Small interfering RNA (siRNA) knockdown of NNMT decreases cancer cell proliferative, migration, invasive, and resistance ability (14, 15). NNMT impairs methylation potential (MP) and decreases DNA and histone methylation, contributing to a wide range of changes in cancer-associated gene expression. Moreover, NNMT has been implicated in regulating autophagy to promote cancer cell survival and maintain cancer stem cell (CSC) stemness (15, 16). In addition to SAM depletion, NNMT enhances esophageal squamous cell chemoresistance by regulating the Warburg effect (6). Based on this, NNMT is a potential therapeutic target in several diseases. However, investigating its exact mechanism and developing efficient NNMT inhibitors remain research challenges.
This review summarizes the diagnostic value of NNMT in various tumors. We also focus on the potential mechanisms of NNMT promotion of cancer progression. Lastly, we introduce recent findings on NNMT inhibitors and their application in cancer therapy. This review provides an effective strategy for clinical transformation, which is a powerful weapon for treatment of patients with cancer.
NNMT Expression and Gene Structure
NNMT is a cytosolic enzyme that was first purified from rat liver in 1951 (17). Subsequently, the NNMT gene was cloned and characterized by PCR, which contributed to revealing the possible molecular genetic mechanism of NNMT regulation (18). It was found that the NNMT gene is located on human chromosome 11q23.1 and mouse chromosome 9, and contains three exons and two introns (19, 20). The NNMT protein is relatively well-conserved across mammals, with 85% amino acid identity between humans and mice (3). The human NNMT gene is ~55.5-kb long and contains two major transcription initiation sites (TISs): TIS201 and TIS203. The product translated from TIS201 and TIS203 is also termed NNMT and consists of 265 amino acid residues. Analysis of the 2000 bp of genomic DNA upstream of TIS201 and TIS203 revealed no TATA box in either 5′ flanking sequence. Nevertheless, there are numerous potential STAT-binding elements, which are likely to regulate STAT3 responsiveness (21). STAT3 activation can improve NNMT expression and promoter activity (22). Hepatocyte nuclear factor 1β (HNF-1β) and interleukin-6 (IL-6) can regulate NNMT levels (22, 23). Combining NNMT and these regulators is likely to improve cancer prognosis. Single-nucleotide polymorphisms (SNPs) in and near the NNMT gene are also associated with many diseases. It is reported that there are 12033 variants across NNMT gene (24). A further illustration of this diversity in the genome comes from the Catalogue of Somatic Mutations in Cancer which lists 101 somatic mutations across the coding sections of NNMT, as a consequence changing or deleting 85 of the predominant amino acid residues (25). Association of SNPs within or near NNMT with cancer and non-cancerous illness, including neurologic illness, liver diseases, heart diseases, spina bifida and obesity has been described in detail. Skin cancers are most associated with somatic mutations across the NNMT coding sections. Furthermore, the NNMT SNP rs694539 increases risk of acute lymphoblastic leukemia (21).
NNMT is expressed mainly in the liver and adipose tissue, and a small amount of NNMT can be detected in the kidney, lung, skeletal muscle, and the heart (26). NNMT expression is altered in metabolic diseases such as obesity and cirrhosis (11). In addition, a wide range of studies have shown that NNMT is elevated in various cancers, including squamous cell carcinoma (SCC) (27), colorectal cancer (12), renal cell carcinoma (28), bladder cancer (29), ovarian cancer (30), cutaneous malignant melanoma (CMM) (31), gastric cancer (32), pancreatic cancer (33, 34), and glioblastoma (GBM) (7). Interestingly, NNMT expression was elevated in atorvastatin- and pravastatin-treated endothelial cells, which elevated nitric oxide and reactive oxygen species (ROS) (35). NNMT expression is usually elevated under metabolic stress and altered mitochondrial activity (36, 37), which may represent a strategy for reacting to oxidative stress.
Association Between NNMT Expression and Poor Cancer Prognosis
Considering that NNMT is elevated in various cancers, most studies on NNMT have focused on its early diagnostic role (27, 38, 39). It is worth noting that some experiments have revealed increased NNMT expression in body fluids, including blood, urine, and saliva (12, 13, 40). NNMT is expected to be a novel serum tumor marker of lung cancer and colorectal cancer (40). Subsequently, Sartini et al. found that patients with oral SCC have higher salivary NNMT protein levels and activity than people without cancer, suggesting that NNMT could be a novel, specific, and low-invasive marker that aids cancer detection (41). It has even been proposed that NNMT be considered as a novel and sensitive serum biomarker for detecting colorectal cancer, outperforming the clinical significance of carcinoembryonic antigen (CEA) (42). Patients with gastric cancer with elevated CEA and NNMT had significantly poorer prognosis than those with only one positive marker, suggesting that combining NNMT with other biomarkers improves clinical diagnostic accuracy (43). It has also been suggested that NNMT expression was significantly higher in the urine of patients with bladder cancer compared with that detected in control specimens (44). Receiver operating characteristic analysis and area under the curve evaluation have demonstrated that NNMT has high sensitivity and specificity (13). Taken together, NNMT is expected to be a novel biomarker that can be applied to early and noninvasive diagnosis and prognosis. Nevertheless, NNMT is actually located in the cytoplasm, and the mechanism by which it is released to body fluids remains unclear.
In addition to being used in early diagnosis, NNMT is also related to poor prognosis. Elevated NNMT levels are typically associated with unfavorable clinical parameters such as tumor size and advanced stage and grade (27, 42). Moreover, NNMT gene silence promotes proliferation, migration, and therapy resistance. We summary association of NNMT expression with clinicopathological characteristics, the result of NNMT gene silence in patients with various cancers and the related signal transduction system (Table 1). Patients with high NNMT levels tend to have poor prognostic parameters and overall survival (OS) and disease-free survival (DFS) (26, 27, 45). We also list number of cases and hazard ratio of NNMT expression in the multivariate analyses. In pancreatic cancer, patients with tumor diameter > 4 cm (p < 0.001) and tumor-node-metastasis (TNM) stage III or IV (p < 0.001) have significantly higher NNMT levels than patients who do not (45). NNMT expression is also associated with CA19-9 level (P=0.005) but it is unclear whether the combination of NNMT and CA19-9 can improve clinical diagnostic accuracy in pancreatic cancers. In addition, NNMT expression in stomach adenocarcinoma correlates positively with the immune infiltration levels of monocytes, M2 macrophages, resting dendritic cells, and neutrophils, but correlates negatively with B cells (32). The NNMT expression score of high-grade serous carcinomas is higher than that in serous borderline tumors and low-grade serous carcinomas (38). Moreover, NNMT gene silence can increase radiosensitivity and chemosensitivity in GBM and CMM, which contributes to combating drug resistance and optimizing combination therapy. Nevertheless, Sartini et al. reported no connection between NNMT activity and non–small cell lung cancer (NSCLC) histologic subtype, grading, and pathological stage (61). It was confirmed that NNMT knockdown contributes to significant suppression of cell proliferation and anchorage-independent cell growth in vitro and in vivo (61). In addition, NNMT expression is not related to grade and stage in renal clear cell carcinoma but correlates negatively with tumor size, suggesting that it may play an important role in the initial malignant conversion phase (49). Unexpectedly, NNMT levels are decreased in human insulinoma and hepatocellular carcinoma (HCC), but the NNMT reduction mechanism remains unclear. In HCC, high NNMT mRNA levels tend to be connected with worse prognosis (26).
Previous studies have mainly focused on NNMT upregulation and downregulation in whole tumor tissue. In fact, NNMT levels vary between the stroma and cancer cells and patients with high stromal NNMT tend to have a poor prognosis (38, 43, 62). Compared with other pathological types, the mesenchymal molecular subtype of ovarian cancer tends to have higher NNMT expression. NNMT expression, which can be used for decisions on prognosis and predicting bevacizumab treatment sensitivity (63). Moreover, high stromal NNMT expression is an independent risk factor of gastric cancer (62). Based on these findings, NNMT overexpression in the stroma aids cancer prognosis prediction.
Biological Roles of the NNMT
Cancer cells and other cells in the tumor microenvironment (TME) have tumor heterogeneity and plasticity, which endows cancer cells with great adaptability to therapy, leading to drug resistance (64). Influenced by pro-tumorigenic cytokines, cancer cells acquire stem cell properties that promote cancer aggressiveness and resistance to therapy. Due to intra- and inter-tumoral heterogeneity, NNMT plays different roles in various cancers and cells. Herein, we review the potential mechanism by which NNMT promotes cancer progression and resistance.
Cancer Cells
NNMT is implicated in regulating tumor proliferation, invasion, and drug resistance by affecting multiple signaling pathways in vivo (Figure 1). The possible mechanism is that NNMT participates in regulating cell cycle progression via many pathways, such as the p27 and PI3K–Akt pathways, enhancing tumorigenesis capacity. NNMT silencing in MGC803 cells has demonstrated a significantly elevated percentage of G2-phase cells (43). On the contrary, downregulating NNMT decreased the proportion of G2- and S-phase cells. Although the results differ, they both suggest that NNMT increases tumorigenesis capacity by regulating the cell cycle. Xie et al. found that 1-MNA can decrease ROS levels, alter the NAD+/NADH ratio, and elevate intracellular ATP levels, suggesting that NNMT maintains cancer cell growth by affecting energy metabolism and promoting cell cycle progression (54). Others have demonstrated that 1-MNA can elevate complex I activity by reducing the degradation of NDUFS3, a complex I subunit. NNMT also can protect complex I from the toxicity of inhibitors such as 1-methyl-4-phenylpyridinium ion and rotenone (65). Lastly, NNMT overexpression unregulated CDK2 and CDK6 expression and downregulated P15, P21, and P27 expression, contributing to the imbalance between CDK and CKI. In this regard, NNMT promotes cancer cell proliferation via the p53–p21 and p27 pathways. Crucially, NNMT is also associated with the phosphorylation status of kinases in the PI3K–Akt and MAPK–ERK pathways (54). Upregulation of NNMT drives the metabolic alteration and sensitizes ovarian cancer cells to mitochondrial metabolic targeting agents without reducing proliferation. It was shown that BRCA1 occupied the NNMT promoter and regulating its expression (8). Specifically, BRCA1 and CDK12 depletion contribute to mitochondrial respiration defects and decreased ATP levels due to the reduced mitochondrial DNA copy number rather than mitochondrial number, which suggest that they stem from decreased flux through electron transport complexes rather than a decrease in mitochondria (8, 66). Furthermore, decreased ATP levels caused by BRCA1 depletion are driven primarily by upregulation of NNMT. Therefore, NNMT is expected to act as an effective cancer therapeutic target in BRCA1-deficient ovary tumor (8).
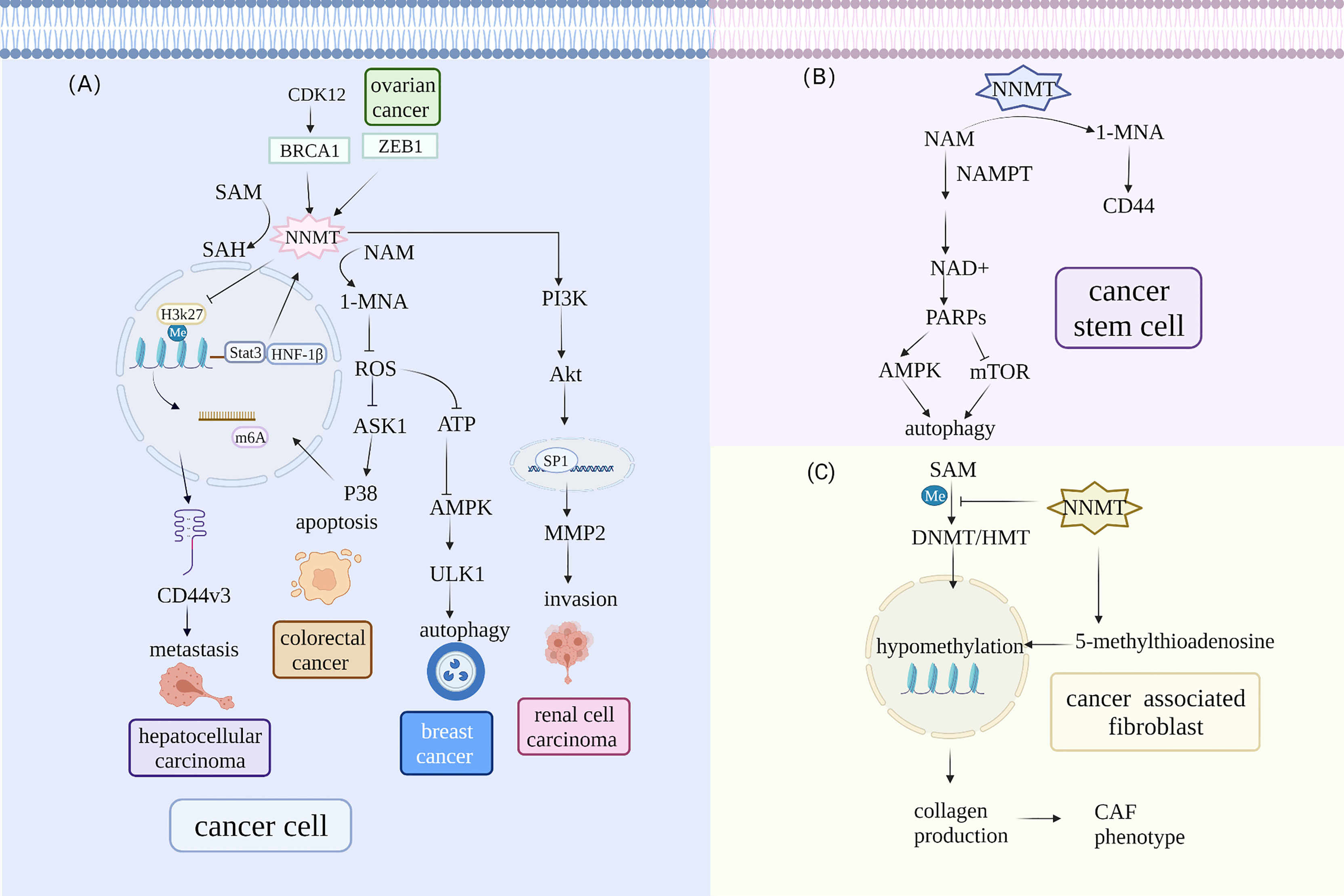
Figure 1 NNMT participates in different signaling pathways in the TME. (A) NNMT expression is directly regulated by transcription factors and subsequently affects metastasis, invasion, apoptosis, and autophagy via a series of downstream signal pathways in cancer cells. Hepatocyte nuclear factor 1β (HNF-1β) and STAT3 can regulate NNMT levels. Moreover, BRCA1 can occupy the NNMT promoter and regulate NNMT expression. In hepatic stellate cells, upregulated NNMT expression alters H3K27 methylation status and affects the N6-methyladenosine (m6A) of CD44, which contributes to tumor metastasis. NNMT promotes clear cell renal cell carcinoma invasion and metastasis by altering matrix metalloproteinase 2 expression. NNMT also lowers the phosphorylation of AKS1 that is an upstream signal of the p38-MAPK pathway and reduces apoptosis in colorectal cancer cells. NNMT can alter protein phosphatase2A methylation status and influence ULK1 activity to regulate autophagy in breast cancer. (B) NNMT regulates autophagy in CSCs by influencing NAD+ metabolism. NNMT decreases the activity of NAD+-dependent enzyme PARP1 that can influence autophagy by upregulation AMPK pathway and downregulation mTOR pathway in CSCs. (C) NNMT overexpression contributes to global hypomethylation and promotes CAF formation. NNMT can elevate 5-methylthioadenosine levels and contribute to global hypomethylation, which alters the methylation status of genes involved in collagen production and promotes Normal fibroblasts acquiring CAF phenotype.
NNMT is also involved in regulating autophagy to maintain cancer cell survival via the mTOR and AMPK pathways. Autophagy is a highly conserved process with a crucial role in cancer status and CSC maintenance by yielding recycled metabolites for growth (67). NNMT knockdown protected hepatic cancer cells from nutrient starvation by promoting autophagy. Mechanistically, NNMT depletion reduced the consumption of methyl donors, increasing protein phosphatase2A (PP2A) methylation. Subsequently, PP2A decreased ULK1 activity through phosphorylated (p)-ULK1 (S638) dephosphorylation (16). ULK1 is an autophagy-related factor that can be regulated negatively by mTOR and positively by AMPK (68). 1-MNA decreases ROS levels, which can regulate autophagy via many signaling pathways (69) (70). Furthermore, a recent study determined that NNMT and 1-MNA regulated autophagy through the ROS-mediated AMPK pathway, protecting breast cancer cells from oxidative stress (71). It is still not clear which signaling pathways NNMT is involved in for regulating autophagy. Nevertheless, these results suggest that NNMT-mediated autophagy may be a new target therapeutic intervention for human cancer.
More importantly, NNMT plays an important role in chemoresistance by participating in the PI3K–Akt and p38–MAPK pathways (15, 72). In NSCLC cell lines with gefitinib resistance, including H292, PC9, H1993 and HCC827 cell lines, NNMT expression is inversely related to that of miR-449a. NNMT stimulates gef-resistant NSCLC cell growth by targeting miR-449a. Knockdown of NNMT (0.5μM) enhanced sensitiveness of H292-Gef cells compared with their control (7.6μM). Mechanistically, NNMT can be upregulated by PI3K–Akt pathway, which is negatively regulated by PTEN. Knockdown of NNMT significantly increased microRNA (miR)-449a levels and decreased the levels of active Akt in vivo in that miR-449a reduced PTEN methylation. This positive feedback loop appears to be a key factor in NNMT overexpression and chemoresistance. Combining NNMT inhibitors and miR-449a enhances antitumor capacity in vivo and in vitro (73). Recently, NNMT overexpression has been found to stabilize SIRT1 protein and reduce the chemotherapy efficacy of adriamycin and paclitaxel in breast cancer cells in an obvious manner. Although SIRT1 mRNA was not significantly changed, SIRT1 protein expression was elevated (15). NNMT regulated glucose, lipid, and cholesterol metabolism in the liver by affecting SIRT1 expression and stability. The results suggest that NNMT and 1-MNA reduce proteasome degradation of SIRT1 by reducing SIRT1 ubiquitination (4). Likewise, a further study suggested that NNMT enhances chemoresistance to 5-FU (5-fluorouracil) in colorectal cancer cells. The possible mechanism is that NNMT decreases 5-FU-induced ROS production in colorectal cancer cells, which lowers the phosphorylation of ASK1, an upstream signal of the p38–MAPK pathway (55). Therefore, NNMT can predict chemotherapy efficacy and represents a potential antitumor target. Nevertheless, the exact mechanisms of NNMT have not been fully investigated and more studies are required to elucidate the molecular mechanism.
Stromal Cells
Previous studies have indicated that NNMT expression is elevated in the stroma of many cancers (56, 74). Immunohistochemistry-based analysis of NNMT in gastric cancer showed that it is mainly expressed in stromal cells (62). Stromal cells play an important role in tumor heterogeneity and promote tumor cell plasticity via metabolic and epigenetic signals. Understanding the reason for the high NNMT expression and its role in stromal cells may contribute to elucidating the mechanism of tumor progression. Hepatic stellate cells had upregulated NNMT expression and altered H3K27 methylation status, which affected the N6-methyladenosine (m6A) of CD44, consequently contributing to the formation of the splice variant CD44v3, which is closely related to tumor metastasis. Meanwhile, 1-MNA reduced the ubiquitin-mediated degradation of CD44 and stabilized CD44 protein, promoting cancer cell invasion and migration (39). Accordingly, NNMT involvement in stromal cell and cancer cell interaction in the mechanical microenvironment may be a reason for the tumor metastasis. A subsequent study has indicated that NNMT promotes clear cell renal cell carcinoma invasion and metastasis by inducing matrix metalloproteinase 2 (MMP2) expression. NNMT alters Akt phosphorylation and affects the association between the transcription factor SP1 and the MMP2 promoter (50). NNMT knockdown in a more aggressive squamous cell line contributed to the downregulation of epithelial–mesenchymal transition (EMT)-related genes, including MMP9, osteopontin (OPN), and versican core protein (VCAN), which are involved in focal adhesion and extracellular matrix (ECM)–receptor interaction (14). Although preliminary, these findings indicate that NNMT is also involved in regulating EMT. Nonetheless, the association between NNMT-induced EMT and tumor invasion and the detailed signaling pathways involved in this process are unclear.
Cancer-associated fibroblasts (CAFs) are a fibroblast subpopulation that secrete pro-oncogenic cytokines and ECM-modifying factors promoting and supporting malignant cell growth and invasion (75). Other cells, such as epithelial cells, can transdifferentiate to CAFs via MMPs, ROS, and tumor-derived transforming growth factor-β (TGF-β) (76). A recent proteomics-based study has revealed that NNMT is a main metabolic regulator of CAFs in ovary cancer (74). NNMT mRNA expression is associated positively with CAF markers (56). In CAFs, NNMT can elevate 5-methylthioadenosine levels, contributing to global hypomethylation. This alters the methylation status of genes involved in collagen production, enhancing collagen contractility (74). Normal fibroblasts acquire the CAF phenotype and secrete many cytokines and oncogenic ECM that contribute to tumor growth and metastasis. Furthermore, NNMT knockdown can convert CAFs to normal fibroblasts, which suggests bidirectional interconversion (74). These results indicate that NNMT drives CAF oncogenic behavior and is also a novel treatment target that can normalize the stroma and attenuate cancer cell invasion.
Cancer Stem Cells
Tumor initiation and progression is driven by CSCs that have the ability to self-renew and differentiate into cells to avoid clone depletion (77). Due to their role in escaping immune surveillance and chemotherapy/apoptosis, more attention should be focused on the potential mechanism. NNMT is elevated in CSCs (7, 78). For example, glioma stem cells (GSCs) have significantly higher NNMT expression than matched differentiated tumor cells. Compared with proneural tumors, NNMT is preferentially expressed in mesenchymal GSCs, a more aggressive subtype. siRNA inhibition of NNMT reduces GSC growth and self-renewal in vitro and is more effective than nicotinamide phosphoribosyltransferase (NAMPT). The findings highlight the possibility of identifying NNMT as a specific GSC phenotype and propose that NNMT is involved in maintaining GSC stemness (7). Regulating methylation can directly lead to metabolic conversion in cancer cells with different phenotypes and even enable cancer cell acquisition of stem cell properties (79). In mesenchymal GSCs, NNMT expression contributes to DNA methylation by participating in nicotinate and methyl donor metabolism (7). As mentioned above, NNMT increases the production of a CD44 variant, a surface marker widely used for identifying CSCs (39). Concordantly, glucose deprivation, which robustly induced NNMT expression in the OVCAR3 cell line, increased the proportion of CD44-expressing cancer stem-like cells. NNMT is elevated with expression of ZEB1, a specific CSC transcription factor, but not all NNMT-positive cells are associated with ZEB1 overexpression. Therefore, NNMT-positive cells may acquire stem cell properties via a ZEB1-independent mechanism (80). The acquisition of such stem cell properties may have a detrimental effect on cancer invasion and drug resistance. Recently, it has been shown that NNMT is likely to regulate autophagy to maintain CSC stemness. Specifically, NNMT can decrease the activity of the PARP1, which is an enzyme to synthesize poly (ADP-ribose) using NAD+ as a substrate (81). PARP1 can influence autophagy by upregulating the AMPK pathway and downregulating the mTOR pathway (67). Such evidence strongly indicates that NNMT might represent a novel antitumor target based on CSC-associated targets.
NNMT Facilitates Energy Metabolism Alterations to Maintain Cancer Cell Survival
Reprogramming energy metabolism is an emerging hallmark of tumor (82). NNMT is a positive regulator of gluconeogenesis and its knockdown in adipose tissue prevented diet-induced obesity in mice (4, 11). Both glucose-6-phosphatase catalytic (G6pc) (20%) and phosphoenolpyruvate carboxykinase 1 cytosolic (Pck1) (40%) expression are significantly decreased in primary hepatocytes with NNMT knockdown. Further studies showed that NNMT can regulate Sirt1 expression and the stability of Sirt1 protein by lowering Sirt1 ubiquitination (4). It has been reported that Sirt1 is a regulator of gluconeogenic/glycolytic pathways (83, 84). The effect of NNMT on gluconeogenesis is mediated by Sirt1 (4). Warburg effect is a phenomenon that even in the presence of oxygen, cancer cells rely on the glycolytic pathway and produce lactate (85). NNMT is a key regulatory element of cancer cell metabolism, especially the Warburg effect. In esophageal squamous cell carcinoma lines, gas chromatography–mass spectrometry analysis has shown that TE1 cells with low sensitivity to 5-FU have higher glycolysis activity and NNMT levels than EC1 and EC109 cells. NNMT knockdown significantly decreased glucose consumption and the expression of glycolysis-related enzymes, including HK2, LDHA, and PAGM1, thereby increasing the 5-FU sensitivity of EC1 cells (6). Silencing PGAM1 upregulated 3-phosphoglycerate (3-PG) and 2-PG levels, inhibiting aerobic glycolysis (86). Moreover, the glycolytic inhibitor 2-deoxyglucose (2‐DG) reversed 5-FU sensitivity in NNMT overexpression cells. Consistent with this, NNMT knockdown increased 2-DG resistance in PANC-1 cells. Recent evidence suggests that in ovarian cancer, NNMT expression is associated positively with PGAM1, which is involved in the Warburg effect, enabling the elucidation of the resistance mechanism of bevacizumab. NNMT is expected to be used for predicting ovarian cancer sensitivity to bevacizumab. Combining an NNMT inhibitor and bevacizumab contributes to improving survival (63). These findings show that NNMT is involved in regulating the Warburg effect enhancement of chemoresistance and is considered a candidate target for cancer therapy. Instead of an energy generation pathway, the Warburg effect presents a mode of energy conversion, generating biosynthetic intermediates to yield precursors for other pathways (87). The exact mechanisms of NNMT regulation of the Warburg effect are not fully understood.
Owing to high NNMT expression, glucose-restricted ovarian cancer cells can acquire metabolic adaptations, using other sugars and some methylated substrates to feed their metabolism. It is possible that NNMT alters histone and DNA methylation status, inducing epigenetic remodeling and metabolism reprogramming (88). Moreover, whether it is in glucose-dependent or glucose-independent ovarian cancer cells, ectopic ZEB1 can increase the expression of NNMT and EMT-associated genes, including MMP2, CTGF, and SPARC. In fact, EMT is a result of phenotypic and metabolic plasticity induced by the ZEB1–NNMT axis (80). NNMT directly increases CTGF expression by reducing CTGF promoter methylation (89). This is a new pathway connecting metabolism and mesenchymal gene expression and contributing to explanation of the mechanisms of cancer cell survival and metastasis in nutrient deficiency.
The Crosstalk Between Tumor Metabolism and Epigenetics
Accumulating evidence has shown that, in cancer, cellular metabolism can also have a profound impact on signaling and transcriptional networks via different mechanisms (87). It is widely accepted that cancer cells reprogram the nutrient procurement and metabolism pathways to meet the demands of uncontrolled cell proliferation (85). Moreover, many intermediary metabolites generated from glycolytic and oxidative phosphorylation serve as cofactors or substrates of epigenetic enzymes involved in post-translational modification and gene expression. Therapies targeting the epigenome have been involved in the potential reinvigoration of antitumor immunity.
NNMT Regulates NAD+ Metabolism to Influence Deacetylases
In addition to metabolism remodeling, epigenomic reprogramming is another main consequence of NNMT overexpression. Chromatin status is driven by the activity and level of chromatin-modifying enzymes such as DNA methyltransferases (DNMT) and histone deacetylases (HDAC) (90) (Figure 2). These enzymes are involved in methylation and acetylation and depend on metabolites as cofactors, integrating the epigenome and transcriptome. NAD+ metabolism is a basis of epigenetic regulatory metabolic reprogramming (91). Under physiological conditions, NAD+ is converted to NAM in NAD+-consuming reactions catalyzed by sirtuins and PARPs (92). NAD+ is required for maintaining the activity of the sirtuin class of HDACs, which NAM inhibits (90). NNMT is activated under NAM saturation conditions because NNMT has low affinity for NAM (3). NNMT prevents NAM accumulation and its inhibitory effect on NAD+-dependent enzymes. Nevertheless, NNMT overexpression is likely to disrupt the balance between NAD+ synthesis and breakdown and decrease NAD+-dependent enzyme activity (79). These enzymes are sensitive to metabolite concentrations and their activity directly influences epigenetic modifications (90). NAM increases SIRT1 activity via NNMT and 1-MNA (4). Moreover, NAM-treated NNMT overexpression mice exhibit fatty liver deterioration because NNMT decreases NAD+ levels and SIRT3 activity, downregulating the expression of genes involved in fatty acid oxidation (5). Therefore, it is important to investigate the influence of NAM concentrations on NNMT-regulated chromatin modification in cancer cells.
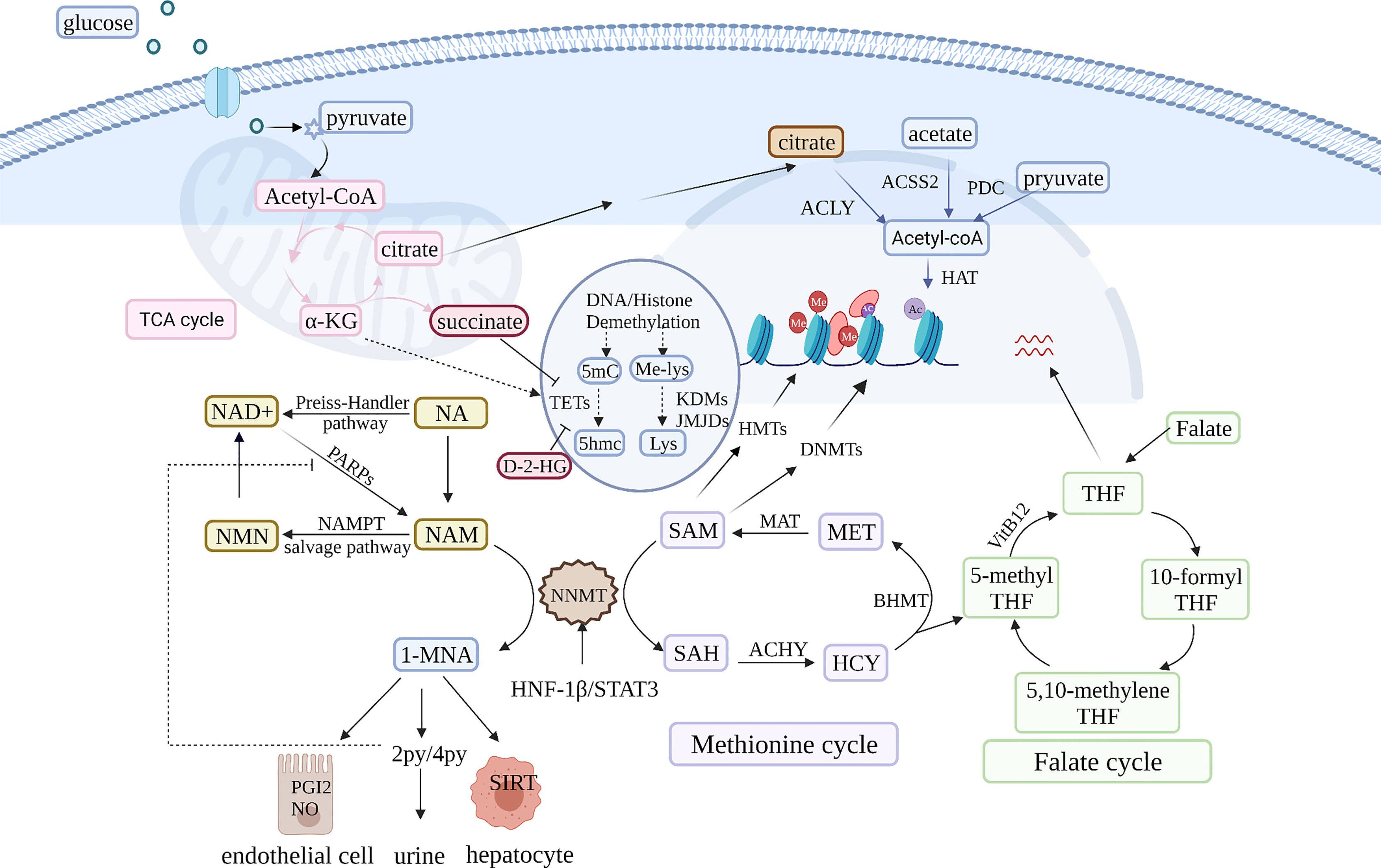
Figure 2 NNMT is pivotal in NAM and SAM metabolism. NNMT uses the universal methyl donor SAM and converts NAM into 1-MNA, which oxidized to 2PY and 4PYand eventually eliminated in the urine. NNMT overexpression leads to a dual drain of NAM and SAM in cancer cells. On one hand, it affects the regeneration of NAD+, a coenzyme of various glucose metabolism enzymes. NNMT over expression decreases NAD+ and contributes to glucose metabolism disorders. The glucose metabolism intermediate α-ketoglutarate (α-KG) is a cofactor of the ten-eleven translocation methylcytosine hydroxylases (TET) and Jumonji C demethylases family (JMJC HDM), which are involved in DNA and histone demethylation, respectively. Furthermore, some glucose metabolism products can enter the nucleus and affect epigenetics. On the other hand, deleting the methyl donor SAM contributes to global hypomethylation and affects the expression of a variety of cancer-associated genes. NNMT also can regulate methyl donor balance by interacting with some enzyme in methionine cycle. In addition to NAM and SAM metabolism, 1-MNA can directly increase Sirt1 protein expression independent of its mRNA level. Sirt1, a regulator of gluconeogenesis and cholesterol synthesis is closely related to NAD+ metabolism. Therefore, NNMT is a pivot in multiple metabolism cycles and influence cancer metabolism from many aspects.
Disruption of Methyl Donor Balance in NNMT Overexpression Cancer Cells
NNMT is also a key node in methyl donor metabolism. NNMT uses the universal methyl donor SAM and produces increased concentrations of 1-MNA, which is further oxidized to 2-PY and 4-PY and eventually excreted in the urine (90). Based on findings that cancer cells have limited capacity for recovering methylation units, Ulanovskaya et al. proposed that 1-MNA is a methylation sink (88). NNMT-mediated SAM depletion can alter cell MP, contributing to the change in global epigenetic histone profiles in cancer (7, 93). For example, NNMT can alter H2K27 methylation status, affecting m6A of CD44, consequently contributing to the formation of CD44v3, which is closely related to tumor metastasis (39). Recent preliminary findings have demonstrated that triple-negative breast cancer (TNBC) cell lines had significantly higher NNMT levels than estrogen receptor-positive cell lines. NNMT overexpression in TNBC cells reduced m6A modification in an obvious manner, contributing to the oncogenic phenotype (94). In addition, NNMT knockdown upregulated tumor suppressor PP2A activity in GBM by increasing methyl availability for leucine carboxyl methyl transferase 1 (LCMT1) and increasing PP2A methylation while concomitantly decreasing the activity of serine/threonine kinases such as Akt and p38 MAPK (95). This aids the understanding of how NNMT affects signaling pathways in cancer cells to promote tumor proliferation, metastasis, and resistance.
Generally, NNMT overexpression decreases SAM and S-adenosyl-L-homocysteine (SAH) levels and alters SAM/SAH in vivo and in vitro in an obvious manner (96). Nevertheless, NNMT knockdown had no effect on SAM/SAH and NAD+ levels in the liver (4). On the contrary, SAM/SAH was significantly decreased in NAM-supplemented NNMT overexpression mice. The possible reason is that NNMT is not a major methyltransferase in the liver. Under the condition of absence of glycine N-methyltransferase (GNMT), NNMT exhibited obvious regulation of MP in normal tissues (5, 88). Multiple factors affect NNMT regulation in hepatic methyl donor balance, which may be related to NNMT downregulation in HCC. In addition to SAM depletion, even inactive NNMT can interact with betaine-homocysteine methyltransferase (BHMT), methionine adenosyltransferase (MAT), and adenosylhomocysteinase (AHCY) to regulate methyl donor balance (96, 97). NNMT promotion of DNA hypomethylation relies on methionine concentrations, a metabolic regulator of methyl donors (7). NNMT over expressing cancer cells that have grown in 20 μM methionine had a more dramatic reduction of SAM levels than those that have grown in 100 μM methionine (88). Furthermore, methionine restriction upregulated NNMT expression and increased mesenchymal gene expression by reducing promoter region methylation. NNMT and methionine restriction both reduced DNMT1 and DNMT3A expression, which may be another DNA hypomethylation mechanism (7).
Development and Application of NNMT in Cancer Therapy
Epigenetic and transcriptomic reprogramming during cancer development is increasingly important and is expected to be an attractive target for cancer treatment. Given the therapeutic hypotheses associated with NNMT, selective, potent, and cell-active inhibitors would be a valuable tool for assessing its therapeutic potential. There are three main NNMT inhibitor types: 1) competitive; 2) bisubstrate; and 3) covalent, with different activities and characteristics (Table 2).
Byproducts of NNMT, SAH and 1-MNA inhibit NNMT activity via a feedback mechanism (107). A study of comprehensive structure–activity relationships (SARs) revealed that quinolinium analogues inhibit NNMT at low concentrations and selectively bind to the NNMT substrate-binding site residues (99). In diet-induced obesity mice, 5-amino-1MQ (5-amino-1-methylquinolinium) exhibited high membrane permeability and caused weight loss by suppressing lipogenesis (100). Based on the primary findings for NNMT in obesity and type 2 diabetes, a recent experiment demonstrated that JBSNF-000088-treated high-fat diet-induced obesity mice exhibited weight loss, improved insulin sensitivity, and normal glucose tolerance (19). However, JBSNF-000088 had no effect on Nnmt knockout mice, suggesting that it is specific to some extent (19). The above findings show that NNMT inhibitors are effective in metabolic disorders, but the potential corrective roles between metabolism and epigenetics in cancer cells are not fully understood. Moreover, bisubstrate inhibitors are designed to target both the substrate and cofactor binding sites to enhance activity and selectivity. This characteristic may contribute to mimicking the ternary transition state of a bireactant enzymatic reaction (102). However, their poor cell permeability has limited their use. SAHH (S-adenosylhomocysteine hydrolase)-coupled assay showed that MS2734 inhibited NNMT at a median inhibitory concentration (IC50) of 14 ± 1.5 μm (102). Presently, NS1 is the most potent and selective NNMT inhibitor in Table 2. Inhibiting alkynyl bisubstrate was a generalizable strategy that is expected to be used for developing probes and inhibitors of other methyltransferases. A naphthalene moiety increased the activity of a bisubstrate inhibitor of NNMT, which was supported by isothermal titration calorimetry binding assays and modeling studies (47). An alpha-chloroacetamide (αCA) compound, RS004 is the first reported covalent inhibitor and inhibits NNMT by covalently binding the noncatalytic active site Cys165 residue (105). The natural product yuanhuadine (YD) significantly inhibits NNMT expression and upregulates miR-449a levels in EGFR-TKI-resistant NSCLC in a concentration-dependent manner. Furthermore, the structure of the NNMT-YD complex had four hydrogen bonds and that YD suppresses NNMT activity via the interacting pocket of the enzyme. These findings aid the development of novel NNMT inhibitors. YD enhances sensitivity to EGFR-TKIs and combining NNMT inhibitors and other traditional drugs improves patient prognosis (73). In addition to the three inhibitor types, N-methyl-4-chloropyridine irreversibly inhibits NNMT based on suicide inhibition, where NNMT promotes its methylation and it subsequently reacts with C159 to inhibit NNMT (108). Currently, it is unclear whether strong or weak NNMT inhibition benefits humans. Experimental studies on the role of NNMT inhibitors in humans are still needed.
Given the urgent demand for NNMT inhibitors, there is a need for a convenient and efficient assay to assess NNMT therapeutic effects. Directly determining the binding affinities of inhibitors using isothermal titration calorimetry and surface plasmon resonance is too costly. SAH-derived chemical proteomic probes and SAH photoreactive probes for profiling NNMT activity have been reported (105, 106). Iyamu et al. designed and synthesized a II138 fluorescent probe (FP) and established an FP-based competition assay to directly detect the molecules that interact with the NNMT active site (109). The authors suggested that this economical and robust assay is appropriate for high-throughput screening applications. However, the FP assay could not detect suicide inhibitors that do not need to bind SAM (109). In short, better tools are needed for identifying and quantifying NNMT inhibitor activity.
At present, many noncancer drugs have shown antitumor effects, including cardiovascular drugs, antibiotics, and psychotropic drugs (110, 111). Drug repurposing is increasingly popular because it lowers cost, has sufficient pharmacological data, and ensures clinical experiment security. Statins affected hepatoma cell migration, invasiveness, and CD44 expression and decreased NNMT levels obviously and in a dose-dependent manner (39). However, a key problem of drug repurposing is the lack of single-agent activity, its feasibility should be examined in further studies (112).
Discussion
As we have summarized, preliminary studies have shown that NNMT plays a key role in various cancers and is expected to be a novel therapeutic antitumor target. In tumor microenvironment, NNMT promotes cancer progress and chemoresistance by regulating cell cycle and autophagy in cancer cells, stromal cells and even cancer stem cells. NNMT in metabolic/epigenetic axis reveals potential mechanism of cancer progress. NNMT can regulate NAD+ Metabolism and disrupt methyl donor balance in cancer cells. Despite the progress in understanding NNMT functions, knowledge gaps remain. Further experiments are required to identify the signals regulating NNMT expression and clarify the exact mechanism and pathways involving NNMT. NNMT and 1-MNA promote tumor progression via various signaling pathways. Nevertheless, it is unclear that whether 2-PY and 4-PY are active and affect the TME via other cell communications, including autocrine or paracrine. The conserved function of NNMT is best reflected by identifying the changes it causes in multiple cancer cell types. Nonetheless, NNMT has different roles in obesity, type 2 diabetes, and hepatic disease (4, 5). Revealing the potential mechanisms is important.
Due to heterogeneity, NNMT metabolic and epigenetic regulation vary between different cancers and specific TME regions. It is unknown whether NNMT is involved in maintaining methyl donor balance in specific diseases and individuals. Specifically, 1-MNA regulates the immune system in ovarian cancers (113). In addition, NNMT expression in stomach adenocarcinoma correlates positively with the immune infiltration levels of monocytes, M2 macrophages, resting dendritic cells, and neutrophils, but correlates negatively with B cells (32). Accordingly, the authors suggested that NNMT can be used for guiding immunotherapy (32). The present review does not cover the role of NNMT in the immune microenvironment. NNMT functions in a specific cell population can be analyzed using single-cell RNA sequencing (scRNA-seq) (114). Organoids are a three-dimensional culture system in vivo that can simulate tissue and organ physical functions (115, 116). Combining organoids and scRNA-seq can identify TMEs and cellular heterogeneity in greater detail, aiding the definition of NNMT functions in different diseases and the development of advanced personalized treatment for patients with metastatic and chemoresistant cancers.
Furthermore, due to the lack of specific and efficient NNMT inhibitors, it remains uncertain whether NNMT can be a novel drug target. NNMT inhibitor development faces challenges. The first crystal structure of human NNMT in a complex with a small-molecular inhibitor formed a solid structural basis for NNMT inhibitor development (102). High selectivity can be achieved by targeting a small binding pocket, such as the NAM-binding pocket. One direction is enhancing bisubstrate inhibitor cellular potency. Inhibitors with high selectivity and efficacy contribute to elucidating the potential mechanism of NNMT and developing novel therapeutic strategies. Combining NNMT inhibitors and traditional targeted drugs, including EGFR-TKIs and bevacizumab, can improve prognosis (63, 73). Therefore, NNMT inhibitors can also be used to combat drug resistance and optimize combination therapy.
We expect that NNMT is a novel biomarker that can be used for early and noninvasive diagnosis. Furthermore, it plays an important role in tumor progression by regulating methyl donor metabolism and participating in multiple signaling pathways. With the development of more studies and clinical trials, we strongly believe that NNMT inhibitors can confer considerable benefit on patients with cancer.
Author Contributions
X-YL collected the related papers and was a major contribution in writing the manuscript. Y-NP made figures and tables. YC and QZ revised the article. B-RX initiated the study and revised the manuscript. All authors read and approved the final manuscript.
Funding
This study was supported by the National Natural Science Foundation of China (No. 81872430), Special Fund in China Postdoctoral Science Foundation (No. 2019T120281, 2019M661304), Heilongjiang Province Postdoctoral Science Foundation (No. LBH-Z18109) and Natural Science Foundation of Heilongjiang Province (No. H2017049) to B-RX.
Conflict of Interest
The authors declare that the research was conducted in the absence of any commercial or financial relationships that could be construed as a potential conflict of interest.
Publisher’s Note
All claims expressed in this article are solely those of the authors and do not necessarily represent those of their affiliated organizations, or those of the publisher, the editors and the reviewers. Any product that may be evaluated in this article, or claim that may be made by its manufacturer, is not guaranteed or endorsed by the publisher.
Acknowledgments
I would like to thank B-RX (The First Affiliated Hospital of USTC, Division of Life Sciences and Medicine, University of Science and Technology of China, Anhui Provincial Cancer Hospital) for advice and useful comments that helped finalize this review article.
Abbreviations
NNMT, nicotinamide N-methyltransferase; NAM, nicotinamide; 1-MNA, 1-methylnicotinamide; 2-py, N1-methyl-2-pyridone-5-carboxamide; 4-py, N1-methyl-4-pyridone-3-carboxamide (4py); AOX, aldehyde oxidase; SAM, S-Adenosyl methionine; SAH, S-adenosyl-L-homocysteine; MP, methylation potential; CSC, cancer stem cell; TISs, transcription initiation sites; HNF-1β, hepatocyte nuclear factor 1β; IL-6, interleukin-6; SNPs, single-nucleotide polymorphisms; TME, the tumor microenvironment; EGFR-TKI, epidermal growth factor receptor tyrosine kinase; m6A, N6-methyladenosine; MMP2, matrix metalloproteinase 2; NSCLC, non–small cell lung cancer; NAMPT, nicotinamide phosphoribosyltransferase; FP, fluorescent probe; YD, yuanhuadine; GNMT, glycine N-methyltransferase; DNMT, DNA methyltransferases; HDAC, histone deacetylases; BHMT, betaine-homocysteine methyltransferase; MAT, methionine adenosyltransferase; 5-amino-1MQ, 5-amino-1-methylquinolinium; HCC, hepatocellular carcinoma; SCC, squamous cell carcinoma; GBM, glioblastoma; CMM, cutaneous malignant melanoma; Ki, inhibition constant.
References
1. Cao W, Chen HD, Yu YW, Li N, Chen WQ. Changing Profiles of Cancer Burden Worldwide and in China: A Secondary Analysis of the Global Cancer Statistics 2020. Chin Med J (Engl) (2021) 134(7):783–91. doi: 10.1097/CM9.0000000000001474
2. Sarmento-Ribeiro AB, Scorilas A, Goncalves AC, Efferth T, Trougakos IP. The Emergence of Drug Resistance to Targeted Cancer Therapies: Clinical Evidence. Drug Resist Update (2019) 47:100646. doi: 10.1016/j.drup.2019.100646
3. Pissios P. Nicotinamide N-Methyltransferase: More Than a Vitamin B3 Clearance Enzyme. Trends Endocrinol Metab (2017) 28(5):340–53. doi: 10.1016/j.tem.2017.02.004
4. Hong S, Moreno-Navarrete JM, Wei X, Kikukawa Y, Tzameli I, Prasad D, et al. Nicotinamide N-Methyltransferase Regulates Hepatic Nutrient Metabolism Through Sirt1 Protein Stabilization. Nat Med (2015) 21(8):887–94. doi: 10.1038/nm.3882
5. Komatsu M, Kanda T, Urai H, Kurokochi A, Kitahama R, Shigaki S, et al. NNMT Activation can Contribute to the Development of Fatty Liver Disease by Modulating the NAD (+) Metabolism. Sci Rep (2018) 8(1):8637. doi: 10.1038/s41598-018-26882-8
6. Cui Y, Yang D, Wang W, Zhang L, Liu H, Ma S, et al. Nicotinamide N-Methyltransferase Decreases 5-Fluorouracil Sensitivity in Human Esophageal Squamous Cell Carcinoma Through Metabolic Reprogramming and Promoting the Warburg Effect. Mol Carcinog (2020) 59(8):940–54. doi: 10.1002/mc.23209
7. Jung J, Kim LJ, Wang X, Wu Q, Sanvoranart T, Hubert CG, et al. Nicotinamide Metabolism Regulates Glioblastoma Stem Cell Maintenance. JCI Insight (2017) 2(10). doi: 10.1172/jci.insight.90019
8. Kanakkanthara A, Kurmi K, Ekstrom TL, Hou X, Purfeerst ER, Heinzen EP, et al. BRCA1 Deficiency Upregulates NNMT, Which Reprograms Metabolism and Sensitizes Ovarian Cancer Cells to Mitochondrial Metabolic Targeting Agents. Cancer Res (2019) 79(23):5920–9. doi: 10.1158/0008-5472.CAN-19-1405
9. Kocinaj A, Chaudhury T, Uddin MS, Junaid RR, Ramsden DB, Hondhamuni G, et al. High Expression of Nicotinamide N-Methyltransferase in Patients With Sporadic Alzheimer's Disease. Mol Neurobiol (2021) 58(4):1769–81. doi: 10.1007/s12035-020-02259-9
10. Fedorowicz A, Mateuszuk L, Kopec G, Skorka T, Kutryb-Zajac B, Zakrzewska A, et al. Activation of the Nicotinamide N-Methyltransferase (NNMT)-1-Methylnicotinamide (MNA) Pathway in Pulmonary Hypertension. Respir Res (2016) 17(1):108. doi: 10.1186/s12931-016-0423-7
11. Kraus D, Yang Q, Kong D, Banks AS, Zhang L, Rodgers JT, et al. Nicotinamide N-Methyltransferase Knockdown Protects Against Diet-Induced Obesity. Nature (2014) 508(7495):258–62. doi: 10.1038/nature13198
12. Roessler M, Rollinger W, Palme S, Hagmann ML, Berndt P, Engel AM, et al. Identification of Nicotinamide N-Methyltransferase as a Novel Serum Tumor Marker for Colorectal Cancer. Clin Cancer Res (2005) 11(18):6550–7. doi: 10.1158/1078-0432.CCR-05-0983
13. Pozzi V, Di Ruscio G, Sartini D, Campagna R, Seta R, Fulvi P, et al. Clinical Performance and Utility of a NNMT-Based Urine Test for Bladder Cancer. Int J Biol Markers (2018) 33(1):94–101. doi: 10.5301/ijbm.5000311
14. Hah YS, Cho HY, Jo SY, Park YS, Heo EP, Yoon TJ. Nicotinamide Nmethyltransferase Induces the Proliferation and Invasion of Squamous Cell Carcinoma Cells. Oncol Rep (2019) 42(5):1805–14. doi: 10.3892/or.2019.7315
15. Wang Y, Zeng J, Wu W, Xie S, Yu H, Li G, et al. Nicotinamide N-Methyltransferase Enhances Chemoresistance in Breast Cancer Through SIRT1 Protein Stabilization. Breast Cancer Res (2019) 21(1):64. doi: 10.1186/s13058-019-1150-z
16. Shin JH, Park CW, Yoon G, Hong SM, Choi KY. NNMT Depletion Contributes to Liver Cancer Cell Survival by Enhancing Autophagy Under Nutrient Starvation. Oncogenesis (2018) 7(8):58. doi: 10.1038/s41389-018-0064-4
17. Cantoni GL. Methylation of Nicotinamide With a Soluble Enzyme System From Rat Liver. J Biol Chem (1951) 189(1):203–16. doi: 10.1016/S0021-9258(18)56110-X
18. Aksoy S, Szumlanski CL, Weinshilboum RM. Human Liver Nicotinamide N-Methyltransferase. cDNA Cloning, Expression, and Biochemical Characterization. J Biol Chem (1994) 269(20):14835–40. doi: 10.1016/s0021-9258(17)36700-5
19. Kannt A, Rajagopal S, Kadnur SV, Suresh J, Bhamidipati RK, Swaminathan S, et al. A Small Molecule Inhibitor of Nicotinamide N-Methyltransferase for the Treatment of Metabolic Disorders. Sci Rep (2018) 8(1):3660. doi: 10.1038/s41598-018-22081-7
20. Aksoy S BB, Ward A, Little PF, Weinshilboum RM. Human Nicotinamide N-Methyltransferase Gene: Molecular Cloning, Structural Characterization and Chromosomal Localization. Genomics (1995) 29(5):555–61. doi: 10.1006/geno.1995.9966
21. Ramsden DB, Waring RH, Parsons RB, Barlow DJ, Williams AC. Nicotinamide N-Methyltransferase: Genomic Connection to Disease. Int J Tryptophan Res (2020) 13:1178646920919770. doi: 10.1177/1178646920919770
22. Tomida M, Ohtake H, Yokota T, Kobayashi Y, Kurosumi M. Stat3 Up-Regulates Expression of Nicotinamide N-Methyltransferase in Human Cancer Cells. J Cancer Res Clin Oncol (2008) 134(5):551–9. doi: 10.1007/s00432-007-0318-6
23. Xu J, Capezzone M, Xu X, Hershman JM. Activation of Nicotinamide N-Methyltransferase Gene Promoter by Hepatocyte Nuclear Factor-1beta in Human Papillary Thyroid Cancer Cells. Mol Endocrinol (2005) 19(2):527–39. doi: 10.1210/me.2004-0215
24. https://www.ensembl.org/Homo_sapiens/Search/Results?q=nnmt;site=ensembl;facet_feature_type=;facet_species=Human.
26. Kim J, Hong SJ, Lim EK, Yu YS, Kim SW, Roh JH, et al. Expression of Nicotinamide N-Methyltransferase in Hepatocellular Carcinoma is Associated With Poor Prognosis. J Exp Clin Cancer Res (2009) 28:20. doi: 10.1186/1756-9966-28-20
27. Akar S, Harmankaya I, Ugras S, Celik C. Expression and Clinical Significance of Nicotinamide N-Methyltransferase in Cervical Squamous Cell Carcinoma. Int J Gynecol Pathol (2020) 39(3):289–95. doi: 10.1097/PGP.0000000000000605
28. Holstein S, Venz S, Junker H, Walther R, Stope MB, Zimmermann U. Nicotinamide N-Methyltransferase and Its Precursor Substrate Methionine Directly and Indirectly Control Malignant Metabolism During Progression of Renal Cell Carcinoma. Anticancer Res (2019) 39(10):5427–36. doi: 10.21873/anticanres.13736
29. Pozzi V, Di Ruscio G, Sartini D, Campagna R, Seta R, Fulvi P, et al. Clinical Performance and Utility of a NNMT-Based Urine Test for Bladder Cancer. Int J Biol Markers (2018) 33(1):94–101. doi: 10.5301/ijbm.5000311
30. Harmankaya I, Akar S, Ugras S, Guler AH, Ezveci H, Aydogdu M, et al. Nicotinamide N-Methyltransferase Overexpression may be Associated With Poor Prognosis in Ovarian Cancer. J Obstet Gynaecol (2020) 41(2):248–53.
31. Ganzetti G, Sartini D, Campanati A, Rubini C, Molinelli E, Brisigotti V, et al. Nicotinamide N-Methyltransferase: Potential Involvement in Cutaneous Malignant Melanoma. Melanoma Res (2018) 28(2):82–8. doi: 10.1097/CMR.0000000000000430
32. Wu M, Hu W, Wang G, Yao Y, Yu XF. Nicotinamide N-Methyltransferase Is a Prognostic Biomarker and Correlated With Immune Infiltrates in Gastric Cancer. Front Genet (2020) 11:580299. doi: 10.3389/fgene.2020.580299
33. Xu Y, Liu P, Zheng DH, Wu N, Zhu L, Xing C, et al. Expression Profile and Prognostic Value of NNMT in Patients With Pancreatic Cancer. Oncotarget (2016) 7(15):19975–81. doi: 10.18632/oncotarget.7891
34. Xu Y, Liu P, Zheng DH, Wu N, Zhu L, Xing C, et al. Expression Profile and Prognostic Value of NNMT in Patients With Pancreatic Cancer. Oncotarget (2016) 7(15):19975–81. doi: 10.18632/oncotarget.7891
35. Dymkowska D, Wrzosek A, Zablocki K. Atorvastatin and Pravastatin Stimulate Nitric Oxide and Reactive Oxygen Species Generation, Affect Mitochondrial Network Architecture and Elevate Nicotinamide N-Methyltransferase Level in Endothelial Cells. J Appl Toxicol (2021) 41(7):1076–88. doi: 10.1002/jat.4094
36. Kanska J, Aspuria PP, Taylor-Harding B, Spurka L, Funari V, Orsulic S, et al. Glucose Deprivation Elicits Phenotypic Plasticity via ZEB1mediated Expression of NNMT. Oncotarget (2017) 8(16):26200–20. doi: 10.18632/oncotarget.15429
37. Song Q, Chen Y, Wang J, Hao L, Huang C, Griffiths A, et al. ER Stress-Induced Upregulation of NNMT Contributes to Alcohol-Related Fatty Liver Development. J Hepatol (2020) 73(4):783–93. doi: 10.1016/j.jhep.2020.04.038
38. Harmankaya I, Akar S, Ugras S, Guler AH, Ezveci H, Aydogdu M, et al. Nicotinamide N-Methyltransferase Overexpression may be Associated With Poor Prognosis in Ovarian Cancer. J Obstet Gynaecol (2021) 41(2):248–53. doi: 10.1080/01443615.2020.1732891
39. Li J, You S, Zhang S, Hu Q, Wang F, Chi X, et al. Elevated N-Methyltransferase Expression Induced by Hepatic Stellate Cells Contributes to the Metastasis of Hepatocellular Carcinoma via Regulation of the CD44v3 Isoform. Mol Oncol (2019) 13(9):1993–2009. doi: 10.1002/1878-0261.12544
40. Tomida M, Mikami I, Takeuchi S, Nishimura H, Akiyama H. Serum Levels of Nicotinamide N-Methyltransferase in Patients With Lung Cancer. J Cancer Res Clin Oncol (2009) 135(9):1223–9. doi: 10.1007/s00432-009-0563-y
41. Sartini D, Pozzi V, Renzi E, Morganti S, Rocchetti R, Rubini C, et al. Analysis of Tissue and Salivary Nicotinamide N-Methyltransferase in Oral Squamous Cell Carcinoma: Basis for the Development of a Noninvasive Diagnostic Test for Early-Stage Disease. Biol Chem (2012) 393(6):505–11. doi: 10.1515/hsz-2012-0112
42. Chen C, Wang X, Huang X, Yong H, Shen J, Tang Q, et al. Nicotinamide N-Methyltransferase: A Potential Biomarker for Worse Prognosis in Gastric Carcinoma. Am J Cancer Res. (2016) 6(3):649–63.
43. Chen C, Wang X, Huang X, Yong H, Shen J, Tang Q, et al. Nicotinamide N-Methyltransferase: A Potential Biomarker for Worse Prognosis in Gastric Carcinoma. Am J Cancer Res (2016) 6(3):649–63.
44. Pozzi V, Di Ruscio G, Sartini D, Campagna R, Seta R, Fulvi P, et al. Clinical Performance and Utility of a NNMT-Based Urine Test for Bladder Cancer. Int J Biol Markers (2018) 33(1):94–101. doi:–10.5301/ijbm.5000311
45. Xu Y, Liu P, Zheng DH, Xu Y, Liu P, Zheng DH, et al. Expression Profile and Prognostic Value of NNMT in Patients With Pancreatic Cancer. Oncotarget (2016) 7(15):19975–81. doi: 10.18632/oncotarget.7891
46. Yu T, Wang YT, Chen P, Li YH, Chen YX, Zeng H, et al. Effects of Nicotinamide N-Methyltransferase on PANC-1 Cells Proliferation, Metastatic Potential and Survival Under Metabolic Stress. Cell Physiol Biochem (2015) 35(2):710–21. doi: 10.1159/000369731
47. Gao Y, van Haren MJ, Moret EE, Rood JJM, Sartini D, Salvucci A, et al. Bisubstrate Inhibitors of Nicotinamide N-Methyltransferase (NNMT) With Enhanced Activity. J Med Chem (2019) 62(14):6597–614. doi: 10.1021/acs.jmedchem.9b00413
48. Liu W, Gou H, Wang X, Li X, Hu X, Su H, et al. TTPAL Promotes Gastric Tumorigenesis by Directly Targeting NNMT to Activate PI3K/AKT Signaling. Oncogene (2021) 40(49):6666–79. doi: 10.1038/s41388-021-01838-x
49. Li J, Yue H, Yu H, Lu X, Xue X. Identification of Nicotinamide N-Methyltransferase as a Novel Tumor Marker for Renal Clear Cell Carcinoma. J Urol (2006) 176(5):2248–54. doi: 10.1016/j.juro.2006.07.046
50. Ravindran Menon D, Hammerlindl H, Torrano J, Schaider H, Fujita M. Nicotinamide N-Methyltransferase Induces Cellular Invasion Through Activating Matrix Metalloproteinase-2 Expression in Clear Cell Renal Cell Carcinoma Cells. Carcinogenesis (2011) 32(2):138–45. doi: 10.1093/carcin/bgq225
51. Zhang J, Xie XY, Yang SW, Wang J, He C. Nicotinamide N-Methyltransferase Protein Expression in Renal Cell Cancer. J Zhejiang Univ Sci B (2010) 11(2):136–43. doi: 10.1631/jzus.B0900249
52. Wu Y, Siadaty MS, Berens ME, Hampton GM, Theodorescu D. Overlapping Gene Expression Profiles of Cell Migration and Tumor Invasion in Human Bladder Cancer Identify Metallothionein 1E and Nicotinamide N-Methyltransferase as Novel Regulators of Cell Migration. Oncogene (2008) 27(52):6679–89. doi: 10.1038/onc.2008.264
53. Wei Y, Guo Y, Zhou J, Dai K, Xu Q, Jin X. Nicotinamide Overcomes Doxorubicin Resistance of Breast Cancer Cells Through Deregulating SIRT1/Akt Pathway. Anticancer Agents Med Chem (2019) 19(5):687–96. doi: 10.2174/1871520619666190114160457
54. El Hout M, Cosialls E, Mehrpour M, Hamai A. Nicotinamide N-Methyltransferase Enhances the Capacity of Tumorigenesis Associated With the Promotion of Cell Cycle Progression in Human Colorectal Cancer Cells. Arch Biochem Biophys (2014) 564:52–66. doi: 10.1016/j.abb.2014.08.017
55. Mowers EE, Sharifi MN, Macleod KF. Nicotinamide N-Methyltransferase Enhances Resistance to 5-Fluorouracil in Colorectal Cancer Cells Through Inhibition of the ASK1-P38 MAPK Pathway. Oncotarget (2016) 7(29):45837–48. doi: 10.18632/oncotarget.9962
56. Lin WJ, Kuang HY. High Stromal Nicotinamide N-Methyltransferase (NNMT) Indicates Poor Prognosis in Colorectal Cancer. Cancer Med (2020) 9(6):2030–8. doi: 10.1002/cam4.2890
57. Yang J, Tong Q, Zhang Y, Yuan S, Gao Y, Deng K, et al. Overexpression of Nicotinamide N-Methyltransferase Mainly Covers Stroma of Colorectal Cancer and Correlates With Unfavorable Survival by its Product 1-MNA. J Cancer (2021) 12(20):6170–81. doi: 10.7150/jca.56419
58. Sartini D, Santarelli A, Rossi V, Goteri G, Rubini C, Ciavarella D, et al. Nicotinamide N-Methyltransferase Upregulation Inversely Correlates With Lymph Node Metastasis in Oral Squamous Cell Carcinoma. Mol Med (2007) 13(7-8):415–21. doi: 10.2119/2007-00035.Sartini
59. Pozzi V, Sartini D, Morganti S, Giuliante R, Di Ruscio G, Santarelli A, et al. RNA-Mediated Gene Silencing of Nicotinamide N-Methyltransferase is Associated With Decreased Tumorigenicity in Human Oral Carcinoma Cells. PloS One (2013) 8(8):e71272. doi: 10.1371/journal.pone.0071272
60. Campagna R, Salvolini E, Pompei V, Pozzi V, Salvucci A, Molinelli E, et al. Nicotinamide N-Methyltransferase Gene Silencing Enhances Chemosensitivity of Melanoma Cell Lines. Pigment Cell Melanoma Res (2021) 34(6):1039–48. doi: 10.1111/pcmr.12993
61. Xie X, Liu H, Wang Y, Zhou Y, Yu H, Li G, et al. Nicotinamide N-Methyltransferase in non-Small Cell Lung Cancer: Promising Results for Targeted Anti-Cancer Therapy. Cell Biochem Biophys (2013) 67(3):865–73. doi: 10.1007/s12013-013-9574-z
62. Eckert MA, Coscia F, Chryplewicz A, Chang JW, Hernandez KM, Pan S, et al. Accumulation of Nicotinamide N-Methyltransferase (NNMT) in Cancer-Associated Fibroblasts: A Potential Prognostic and Predictive Biomarker for Gastric Carcinoma. J Histochem Cytochem (2021) 69(3):165–76. doi: 10.1369/0022155420976590
63. Song M, Li Y, Miao M, Zhang F, Yuan H, Cao F, et al. Patients With Low Nicotinamide N-Methyltransferase Expression Benefit Significantly From Bevacizumab Treatment in Ovarian Cancer. BMC Cancer (2021) 21(1):67. doi: 10.1186/s12885-021-07785-w
64. Tang SW, Yang TC, Lin WC, Chang WH, Wang CC, Lai MK, et al. Epigenetics and Metabolism at the Crossroads of Stress-Induced Plasticity, Stemness and Therapeutic Resistance in Cancer. Theranostics (2020) 10(14):6261–77. doi: 10.7150/thno.42523
65. Itoh G, Chida S, Yanagihara K, Yashiro M, Aiba N, Tanaka M. The Expression of Nicotinamide N-Methyltransferase Increases ATP Synthesis and Protects SH-SY5Y Neuroblastoma Cells Against the Toxicity of Complex I Inhibitors. Biochem J (2011) 436(1):145–55. doi: 10.1042/BJ20101685
66. Yu Y, Xiao CH, Tan LD, Wang QS, Li XQ, Feng YM. BRCA1 Induces Major Energetic Metabolism Reprogramming in Breast Cancer Cells. PloS One (2014) 9(7):e102438. doi: 10.1371/journal.pone.0102438
67. Melzer C, von der Ohe J, Lehnert H, Ungefroren H, Hass R. Crosstalk Between Autophagy and Metabolic Regulation of Cancer Stem Cells. Mol Cancer (2020) 19(1):27. doi: 10.1186/s12943-019-1126-8
68. Pozzi V, Salvolini E, Lucarini G, Salvucci A, Campagna R, Rubini C, et al. Functions of Autophagy in the Tumor Microenvironment and Cancer Metastasis. FEBS J (2018) 285(10):1751–66. doi: 10.1111/febs.14388
69. Novak Kujundzic R, Prpic M, Dakovic N, Dabelic N, Tomljanovic M, Mojzes A, et al. Oxidative Stress Induces Autophagy in Response to Multiple Noxious Stimuli in Retinal Ganglion Cells. Autophagy (2014) 10(10):1692–701. doi: 10.4161/auto.36076
70. Kanska J, Aspuria PP, Taylor-Harding B, Kanska J, Aspuria PP, Taylor-Harding B, et al. Glucose Deprivation Elicits Phenotypic Plasticity via ZEB1-Mediated Expression of NNMT. Oncotarget (2017) 8(16):26200–26220. doi: 10.18632/oncotarget.15429
71. Yu H, Zhou X, Wang Y, Huang X, Yang J, Zeng J, et al. Nicotinamide N-Methyltransferase Inhibits Autophagy Induced by Oxidative Stress Through Suppressing the AMPK Pathway in Breast Cancer Cells. Cancer Cell Int (2020) 20:191. doi: 10.1186/s12935-020-01279-8
72. Xie X, Liu H, Wang Y, Zhou Y, Yu H, Li G, et al. Nicotinamide N-Methyltransferase Enhances Resistance to 5-Fluorouracil in Colorectal Cancer Cells Through Inhibition of the ASK1-P38 MAPK Pathway. Oncotarget (2016) 7(29):45837–48. doi: 10.18632/oncotarget.9962
73. Bach DH, Kim D, Bae SY, Kim WK, Hong JY, Lee HJ, et al. Targeting Nicotinamide N-Methyltransferase and miR-449a in EGFR-TKI-Resistant Non-Small-Cell Lung Cancer Cells. Mol Ther Nucleic Acids (2018) 11:455–67. doi: 10.1016/j.omtn.2018.03.011
74. Eckert MA, Coscia F, Chryplewicz A, Chang JW, Hernandez KM, Pan S, et al. Proteomics Reveals NNMT as a Master Metabolic Regulator of Cancer-Associated Fibroblasts. Nature (2019) 569(7758):723–8. doi: 10.1038/s41586-019-1173-8
75. Itoh G, Chida S, Yanagihara K, Yashiro M, Aiba N, Tanaka M, et al. Cancer-Associated Fibroblasts Induce Cancer Cell Apoptosis That Regulates Invasion Mode of Tumours. Oncogene (2017) 36(31):4434–44. doi: 10.1038/onc.2017.49
76. Yu Y, Xiao CH, Tan LD, Wang QS, Li XQ, Feng YM, et al. Cancer-Associated Fibroblasts Induce Epithelial-Mesenchymal Transition of Breast Cancer Cells Through Paracrine TGF-Beta Signalling. Br J Cancer (2014) 110(3):724–32. doi: 10.1038/bjc.2013.768
77. Melzer C, von derOhe J, Lehnert H, Ungefroren H, Hass R. Cancer Stem Cell Niche Models and Contribution by Mesenchymal Stroma/Stem Cells. Mol Cancer (2017) 16(1):28. doi: 10.1186/s12943-017-0595-x
78. Pozzi V, Salvolini E, Lucarini G, Salvucci A, Campagna R, Rubini C, et al. Cancer Stem Cell Enrichment is Associated With Enhancement of Nicotinamide N-Methyltransferase Expression. IUBMB Life (2020) 72(7):1415–25. doi: 10.1002/iub.2265
79. Novak Kujundzic R, Prpic M, Dakovic N, Dabelic N, Tomljanovic M, Mojzes A, et al. Nicotinamide N-Methyltransferase in Acquisition of Stem Cell Properties and Therapy Resistance in Cancer. Int J Mol Sci (2021) 22(11). doi: 10.3390/ijms22115681
80. Kanska J, Aspuria PP, Taylor-Harding B, Kanska J, Aspuria PP, Taylor-Harding B, et al. Glucose Deprivation Elicits Phenotypic Plasticity via ZEB1-Mediated Expression of NNMT. Oncotarget (2017) 8(16):26200–20. doi: 10.18632/oncotarget.15429
81. Ray Chaudhuri A, Nussenzweig A. The Multifaceted Roles of PARP1 in DNA Repair and Chromatin Remodelling. Nat Rev Mol Cell Biol (2017) 18(10):610–21. doi: 10.1038/nrm.2017.53
82. Hanahan D, Weinberg RA. Hallmarks of Cancer: The Next Generation. Cell (2011) 144(5):646–74. doi: 10.1016/j.cell.2011.02.013
83. El-Khamisy SF, Saifi GM, Weinfeld M, Johansson F, Helleday T, Lupski JR, et al. Defective DNA Single-Strand Break Repair in Spinocerebellar Ataxia With Axonal Neuropathy-1. Nature (2005) 434(7029):108–13. doi: 10.1038/nature03314
84. Liu Y, Dentin R, Chen D, Hedrick S, Ravnskjaer K, Schenk S, et al. A Fasting Inducible Switch Modulates Gluconeogenesis via Activator/Coactivator Exchange. Nature (2008) 456(7219):269–73. doi: 10.1038/nature07349
85. Bose S, Allen AE, Locasale JW. The Molecular Link From Diet to Cancer Cell Metabolism. Mol Cell (2020) 78(6):1034–44. doi: 10.1016/j.molcel.2020.05.018
86. Peng XC, Gong FM, Chen Y, Qiu M, Cheng K, Tang J, et al. Proteomics Identification of PGAM1 as a Potential Therapeutic Target for Urothelial Bladder Cancer. J Proteomics (2016) 132:85–92. doi: 10.1016/j.jprot.2015.11.027
87. Thakur C, Chen F. Connections Between Metabolism and Epigenetics in Cancers. Semin Cancer Biol (2019) 57:52–8. doi: 10.1016/j.semcancer.2019.06.006
88. Ulanovskaya OA, Zuhl AM, Cravatt BF. NNMT Promotes Epigenetic Remodeling in Cancer by Creating a Metabolic Methylation Sink. Nat Chem Biol (2013) 9(5):300–6. doi: 10.1038/nchembio.1204
89. Matei D, Nephew KP. Epigenetic Attire in Ovarian Cancer: The Emperor's New Clothes. Cancer Res (2020) 80(18):3775–85. doi: 10.1158/0008-5472.CAN-19-3837
90. Montellier E, Gaucher J. Targeting the Interplay Between Metabolism and Epigenetics in Cancer. Curr Opin Oncol (2019) 31(2):92–9. doi: 10.1097/CCO.0000000000000501
91. Yaku K, Okabe K, Hikosaka K, Nakagawa T. NAD Metabolism in Cancer Therapeutics. Front Oncol (2018) 8:622. doi: 10.3389/fonc.2018.00622
92. Canto C, Menzies KJ, Auwerx J. NAD(+) Metabolism and the Control of Energy Homeostasis: A Balancing Act Between Mitochondria and the Nucleus. Cell Metab (2015) 22(1):31–53. doi: 10.1016/j.cmet.2015.05.023
93. Li Q, He MD, Mao L, Wang X, Jiang YL, Li M, et al. Nicotinamide N-Methyltransferase Suppression Participates in Nickel-Induced Histone H3 Lysine9 Dimethylation in BEAS-2b Cells. Cell Physiol Biochem (2017) 41(5):2016–26. doi: 10.1159/000475432
94. Deniz N, Dolcen, Harada B, He C, Conzen SD. Role of NNMT-Regulated M6a mRNA Modification in Triple-Negative Breast Cancer Oncogenic Gene Expression. Cancer Res (2020) 80(16Suppl):Abstract nr 1290.
95. Palanichamy K, Kanji S, Gordon N, Thirumoorthy K, Jacob JR, Litzenberg KT, et al. NNMT Silencing Activates Tumor Suppressor PP2A, Inactivates Oncogenic STKs, and Inhibits Tumor Forming Ability. Clin Cancer Res (2017) 23(9):2325–34. doi: 10.1158/1078-0432.CCR-16-1323
96. Hong S, Zhai B, Pissios P. Nicotinamide N-Methyltransferase Interacts With Enzymes of the Methionine Cycle and Regulates Methyl Donor Metabolism. Biochemistry (2018) 57(40):5775–9. doi: 10.1021/acs.biochem.8b00561
97. Portillo F, Vazquez J, Pajares MA. Protein-Protein Interactions Involving Enzymes of the Mammalian Methionine and Homocysteine Metabolism. Biochimie (2020) 173:33–47. doi: 10.1016/j.biochi.2020.02.015
98. van Haren MJ, Taig R, Kuppens J, Sastre Torano J, Moret EE, Parsons RB, et al. Inhibitors of Nicotinamide N-Methyltransferase Designed to Mimic the Methylation Reaction Transition State. Org Biomol Chem (2017) 15(31):6656–67. doi: 10.1039/C7OB01357D
99. Neelakantan H, Wang HY, Vance V, Hommel JD, McHardy SF, Watowich SJ, et al. Structure-Activity Relationship for Small Molecule Inhibitors of Nicotinamide N-Methyltransferase. J Med Chem (2017) 60(12):5015–28. doi: 10.1021/acs.jmedchem.7b00389
100. Neelakantan H, Vance V, Wetzel MD, Wang HL, McHardy SF, Finnerty CC, et al. Selective and Membrane-Permeable Small Molecule Inhibitors of Nicotinamide N-Methyltransferase Reverse High Fat Diet-Induced Obesity in Mice. Biochem Pharmacol (2018) 147:141–52. doi: 10.1016/j.bcp.2017.11.007
101. Kannt A, Rajagopal S, Hallur MS, Swamy I, Kristam R, Dhakshinamoorthy S, et al. Novel Inhibitors of Nicotinamide-N-Methyltransferase for the Treatment of Metabolic Disorders. Molecules (2021) 26(4). doi: 10.3390/molecules26040991
102. Babault N, Allali-Hassani A, Li F, Fan J, Yue A, Ju K, et al. Discovery of Bisubstrate Inhibitors of Nicotinamide N-Methyltransferase (NNMT). J Med Chem (2018) 61(4):1541–51. doi: 10.1021/acs.jmedchem.7b01422
103. Chen D, Li L, Diaz K, Iyamu ID, Yadav R, Noinaj N, et al. Novel Propargyl-Linked Bisubstrate Analogues as Tight-Binding Inhibitors for Nicotinamide N-Methyltransferase. J Med Chem (2019) 62(23):10783–97. doi: 10.1021/acs.jmedchem.9b01255
104. Policarpo RL, Decultot L, May E, Kuzmic P, Carlson S, Huang D, et al. High-Affinity Alkynyl Bisubstrate Inhibitors of Nicotinamide N-Methyltransferase (NNMT). J Med Chem (2019) 62(21):9837–73. doi: 10.1021/acs.jmedchem.9b01238
105. Horning BD, Suciu RM, Ghadiri DA, Ulanovskaya OA, Matthews ML, Lum KM, et al. Chemical Proteomic Profiling of Human Methyltransferases. J Am Chem Soc (2016) 138(40):13335–43. doi: 10.1021/jacs.6b07830
106. Lee HY, Suciu RM, Horning BD, Vinogradova EV, Ulanovskaya OA, Cravatt BF. Covalent Inhibitors of Nicotinamide N-Methyltransferase (NNMT) Provide Evidence for Target Engagement Challenges in Situ. Bioorg Med Chem Lett (2018) 28(16):2682–7. doi: 10.1016/j.bmcl.2018.04.017
107. Swaminathan S, Birudukota S, Thakur MK, Parveen R, Kandan S, Juluri S, et al. Crystal Structures of Monkey and Mouse Nicotinamide N-Methyltransferase (NNMT) Bound With End Product, 1-Methyl Nicotinamide. Biochem Biophys Res Commun (2017) 491(2):416–22. doi: 10.1016/j.bbrc.2017.07.087
108. Sen S, Mondal S, Zheng L, Salinger AJ, Fast W, Weerapana E, et al. Development of a Suicide Inhibition-Based Protein Labeling Strategy for Nicotinamide N-Methyltransferase. ACS Chem Biol (2019) 14(4):613–8. doi: 10.1021/acschembio.9b00211
109. Iyamu ID, Huang R. Development of Fluorescence Polarization-Based Competition Assay for Nicotinamide N-Methyltransferase. Anal Biochem (2020) 604:113833. doi: 10.1016/j.ab.2020.113833
110. Nishihara R, Lochhead P, Kuchiba A, et al. Aspirin Use and Risk of Colorectal Cancer Ac-795 Cording to BRAF Mutation Status. JAMA (2013) 309(24):2563–71. doi: 10.1001/jama.2013.6599
111. Lai JP, Sandhu DS, Moser CD, Cazanave SC, Oseini AM, Shire AM, et al. Additive Effect of Apicidin and Doxorubicin in Sulfatase 1 Expressing Hepatocellular Carcinoma In Vitro and In Vivo. J Hepatol (2009) 50(6):1112–21. doi: 10.1016/j.jhep.2008.12.031
112. Tran AA, Prasad V. Drug Repurposing for Cancer Treatments: A Well-Intentioned, But Misguided Strategy. Lancet Oncol (2020) 21(9):1134–6. doi: 10.1016/S1470-2045(20)30424-1
113. Kilgour MK, MacPherson S, Zacharias LG, Ellis AE, Sheldon RD, Liu EY, et al. 1-Methylnicotinamide is an Immune Regulatory Metabolite in Human Ovarian Cancer. Sci Adv (2021) 7(4):eabe1174. doi: 10.1126/sciadv.abe1174
114. Lee HW, Chung W, Lee HO, Jeong DE, Jo A, Lim JE, et al. Single-Cell RNA Sequencing Reveals the Tumor Microenvironment and Facilitates Strategic Choices to Circumvent Treatment Failure in a Chemorefractory Bladder Cancer Patient. Genome Med (2020) 12(1):47. doi: 10.1186/s13073-020-00741-6
115. Jin MZ, Han RR, Qiu GZ, Ju XC, Lou G, Jin WL, et al. Organoids: An Intermediate Modeling Platform in Precision Oncology. Cancer Lett (2018) 414:174–80. doi: 10.1016/j.canlet.2017.11.021
116. Hubert CG, Rivera M, Spangler LC, Wu Q, Mack SC, Prager BC, et al. A Three-Dimensional Organoid Culture System Derived From Human Glioblastomas Recapitulates the Hypoxic Gradients and Cancer Stem Cell Heterogeneity of Tumors Found In Vivo. Cancer Res (2016) 76(8):2465–77. doi: 10.1158/0008-5472.CAN-15-2402
Keywords: nicotinamide N-methyltransferase (NNMT), tumor biomarker, epigenetics, NNMT inhibitor, cancer therapy, tumor microenvironment
Citation: Li X-Y, Pi Y-N, Chen Y, Zhu Q and Xia B-R (2022) Nicotinamide N-Methyltransferase: A Promising Biomarker and Target for Human Cancer Therapy. Front. Oncol. 12:894744. doi: 10.3389/fonc.2022.894744
Received: 12 March 2022; Accepted: 29 April 2022;
Published: 09 June 2022.
Edited by:
Ubaldo Emilio Martinez-Outschoorn, Thomas Jefferson University, United StatesReviewed by:
Fumiaki Uchiumi, Tokyo University of Science, JapanYu-Chan Chang, National Yang Ming Chiao Tung University, Taiwan
Copyright © 2022 Li, Pi, Chen, Zhu and Xia. This is an open-access article distributed under the terms of the Creative Commons Attribution License (CC BY). The use, distribution or reproduction in other forums is permitted, provided the original author(s) and the copyright owner(s) are credited and that the original publication in this journal is cited, in accordance with accepted academic practice. No use, distribution or reproduction is permitted which does not comply with these terms.
*Correspondence: Bai-Rong Xia, eGlhYmFpcm9uZzk5OTlAMTI2LmNvbQ==
†These authors have contributed equally to this work