- 1Faculty of Health, Queensland University of Technology, Brisbane, QLD, Australia
- 2School of Nursing, Nanjing Medical University, Nanjing, China
- 3Department of Gastric Surgery, The First Affiliated Hospital of Nanjing Medical University, Nanjing, China
- 4Department of Thoracic Surgery, The First Affiliated Hospital of Nanjing Medical University, Nanjing, China
- 5Department of Colorectal Surgery, The First Affiliated Hospital of Nanjing Medical University, Nanjing, China
Background: Gastrointestinal cancers are the most common malignant tumors worldwide. As the improvement of survival by surgical resection alone for cancers is close to the bottleneck, recent neoadjuvant therapy has been emphasized and applied in the treatment. Despite the advantage on improving the prognosis, some studies have reported neoadjuvant therapy could reduce skeletal muscle and therefore affect postoperative outcomes. However, the conclusions are still controversial.
Methods: PubMed, CINAHL, Embase, and Cochrane Library were searched from inception to September 2, 2021. The inclusion criteria were observational studies, published in English, of individuals aged ≥18 years who underwent neoadjuvant therapy with gastrointestinal cancers and were assessed skeletal muscle mass before and after neoadjuvant therapy, with sufficient data on skeletal muscle change or the association with clinical outcomes. Meta-analysis was conducted by using the STATA 12.0 package when more than two studies reported the same outcome.
Results: A total of 268 articles were identified, and 19 studies (1,954 patients) were included in the review. The fixed effects model showed that the risk of sarcopenia increased 22% after receiving neoadjuvant therapy (HR=1.22, 95% CI 1.14, 1.31, Z=4.286, P<0.001). In the random effects model, neoadjuvant therapy was associated with skeletal muscle loss, with a standardized mean difference of -0.20 (95% CI -0.31, -0.09, Z=3.49, P<0.001) and a significant heterogeneity (I2 =62.2%, P<0.001). Multiple meta regression indicated that population, neoadjuvant therapy type, and measuring tool were the potential sources of heterogeneity. The funnel plot revealed that there was no high publication bias in these studies (Begg’s test, P=0.544) and the sensitivity analysis showed stable results when separately excluding studies. For the postoperative outcomes, the results revealed that muscle loss during neoadjuvant therapy was significantly related to overall survival (HR=2,08, 95% CI =1.47, 2.95, Z=4.12, P<0.001, I2 = 0.0%), but not related to disease-free survival and other short-term outcomes.
Conclusions: This systematic review and meta-analysis revealed that skeletal muscle decreased significantly during neoadjuvant therapy in patients with gastrointestinal cancers and skeletal muscle loss was strongly associated with worse overall survival. More high-quality studies are needed to update and valid these conclusions in a more specific or stratified way.
Systematic Review Registration: [https://www.crd.york.ac.uk/PROSPERO/], identifier PROSPERO (CRD42021292118)
Introduction
Gastrointestinal (GI) cancers, which mainly include esophageal, gastric, and colorectal cancers are commonly diagnosed worldwide and have constituted a heavy disease burden. According to the latest statistics, these three cancers account for 18.7% and 22.6% of the newly cancer cases and deaths, respectively (1). Although surgery is still the main treatment for GI tumors, the improvement of survival by surgical resection alone for locally advanced tumors has been close to the bottleneck (2). Over the past decades, the effect of neoadjuvant therapy (NAT) has been gradually emphasized. NAT includes neoadjuvant chemotherapy (NACT), neoadjuvant chemoradiotherapy (NACRT), targeted therapy and even immunotherapy (2). It was first put forward by Frei in 1982 and was a comprehensive treatment model developing gradually, based on postoperative adjuvant radiotherapy and chemotherapy (3). As recommended by National Comprehensive Cancer Network (NCCN) and American Society of Clinical Oncology (ASCO), surgery combined with NAT has gradually become the standard treatment for patients with local advanced GI cancers (4).
Skeletal muscle could be the largest organ of the human body and plays an important role in maintaining fitness and health status (5). Usually, sarcopenia is defined as the progressive loss of muscle mass and function and mainly involves the elderly; however, researchers have found that volumetric muscle loss (VML), which refers to the rapid-onset and focal loss of skeletal muscle, could exert negative effects on other people as well (6, 7). In cancer patients, except for age-related muscle loss, both tumors and tumor-related treatments are vital reasons for muscle homeostasis imbalance and volumetric muscle loss. Cancer-released exosomes could promote proinflammatory cytokines and factors to elicit activation of cascades and inhibit the function of the muscle metabolism pathways; cancer cells may also activate the ubiquitin-proteasome system (UPS) and the myostatin pathway, as well as increase the expression of phosphatase and tensin homolog (PTEN), therefore leading to protein degradation and muscle atrophy (7). In addition, cancer-related treatments, such as chemotherapy, can trigger off a progressive erosion of the muscle. The potential mechanisms lie in that the molecular pathways of muscle metabolism could be modified by chemotherapeutic drugs directly, therefore inducing sarcopenia (8). Furthermore, chemotherapy or radiotherapy may worsen dysphagia, anorexia, and other cancer-related symptoms, detrimentally affecting nutritional status which could lead to reduced body weight and muscle mass (9).
Numerous studies have shown that low skeletal muscle mass can be a predictor of poor outcomes for cancer patients, which is strongly related to a longer hospital stay, more postoperative complications, reduced survival rates and worse, health-related quality of life (7, 10, 11). Due to the increased consumption of nutrients and metabolic disorders, patients with GI tumors are more likely to suffer from muscle depletion (11), which is strongly associated with worsening of the nutritional state and quality of life in these patients (12). Although NAT has the advantage of reducing tumor size and stage, increasing the R0 resection rate and inhibiting micrometastases (13–15), some studies found skeletal muscle mass could decrease significantly after NAT, which may contribute to preoperative sarcopenia and exacerbate postoperative outcomes (16–18). Currently, various assessment tools exist for the characterization and quantification of muscle loss with easy access in the clinical practice (6), and several strategies, such as ghrelin, oral supplementation, and enteral nutrition or exercise have been found effective to improve muscle mass and attenuation induced by chemotherapy (7, 8). Hence, it is necessary to focus on changes of skeletal muscle mass in patients with GI cancers during NAT, which could provide important evidence for the implementation of early individualized rehabilitation interventions.
Previous meta-analyses have demonstrated that sarcopenia could be a predictor of poor postoperative outcomes in cancer patients (19–21). A recent meta-analysis also reported that NAT could significantly increase the prevalence of sarcopenia in patients with esophageal cancer (EC), and overall survival (OS) and disease-free survival (DFS) were worse in EC patients diagnosed with sarcopenia preoperatively than those who were not (22). However, these studies either failed to consider the impact of NAT itself on skeletal muscle mass or confused the effect of pre-existing sarcopenia with NAT-related sarcopenia on postoperative outcomes. Additionally, whether NAT would reduce skeletal muscle mass in patients with GI cancers and, therefore, affect postoperative outcomes are uncertain. Some studies found no statistically significant reduction in skeletal muscle after NAT (23–25), and muscle loss after NAT may not be a predictor for long-term outcomes in cancer patients (26). Furthermore, findings on short-term outcomes like postoperative complications, and mortality were also contradictory (27–30). Therefore, the purpose of this systematic review and meta-analysis was to evaluate the effect of NAT on skeletal muscle mass in patients with GI cancers and to explore the relationship between muscle change during NAT and clinical outcomes.
Methods
This systematic review and meta-analysis followed a predefined protocol registered with PROSPERO (CRD42021292118) (31) and adhered to the latest Preferred Reporting Items for Systematic Reviews and Meta-Analyses (PRISMA) guidelines (32).
Literature Search
A pre-search was conducted in PubMed to identify the subjects, keywords, and synonyms. Then, the PubMed, CINAHL, Embase, and Cochrane Library were searched from inception to September 2, 2021. The search strategies were based on keywords, and the medical subject headings (MeSH) according to PICO framework (Table 1) (33). Reference lists of the selected relevant studies were also scanned to further identify additional studies. Search results were imported into Endnote X7 and duplicates were discarded.
Eligibility Criteria
We included studies that met these criteria: (1) any original observational studies; (2) included patients who were over 18 years and underwent NAT (including chemotherapy and chemoradiotherapy) with gastric, esophageal, or colorectal cancer; (3) patients were assessed skeletal muscle before and after NAT, and the second assessment must have been completed before any additional treatment like surgery and postoperative chemotherapy; (4) reported sufficient data on skeletal muscle change (providing specific pre-NAT and post- NAT value or change rate) with or without the association with clinical outcomes (e.g., complication, length of hospital stay, mortality, survival rate); (5) only published in English. Studies were excluded if full-text of the studies were unavailable. If studies were based on the same patients, the most recent or completed one was chosen.
Study Selection and Data Extraction
Firstly, the title and abstract were screened by XX based on the above criteria. The full-text of potential eligible articles were retrieved and evaluated by two researchers (XX and XJ). Papers that did not meet the criteria were excluded, with the reason(s) for exclusion recorded. Any disagreement would be resolved by discussion until consensus was reached or by consulting a third author (SZ). After that, two researchers extracted data by using a standardized data collection form independently. The following data were collected: the first author’s last name, publication year, country, study design, study aim, sample size, sex, age, cancer type and stage, treatment, measuring tool, muscle change outcomes including the degree of muscle loss during NAT, change rate in muscle mass (mean/range), cut-off value and incidence of severe muscle loss, the prevalence of sarcopenia before and after NAT, and findings on the associations between muscle change and clinical outcomes.
Quality Assessment
The quality of study was independently assessed by two researchers using the National Institutes of Health (NIH) Quality Assessment tool for Observational Cohort and Cross-Sectional Studies. The quality of study was rated as good, fair, or poor (https://www.nhlbi.nih.gov/health-topics/study-quality-assessment-tools).
Statistical Analysis
Meta-analysis was conducted by using the STATA 12.0 package (StataCorp, College Station, TX, USA) when more than two studies reported the same outcome. To compare the change in the prevalence of sarcopenia before and after NAT, hazard risk (HR) was applied. To compare the change of skeletal muscle during NAT, mean and standard deviation (SD) were applied directly from the publication where possible. For studies that only provided the value of median, range, or interquartile range (IQR), method put forward by Wan et al., was applied to estimate the sample mean and SD (34). Due to the various measurement units of muscle mass, the results were pooled by standardized mean difference (SMD). To analyze the relationship between muscle loss and prognosis, odds ratio (OR) or HR for dichotomous or survival variables were pooled using a fixed or random effects model as appropriate. Heterogeneity of the included studies was assessed by I2 test, and regarded as low, moderate, or high when I2 was around 25%, 50% or 75%, respectively. The random effects model was used when I2 >50%; otherwise, a fixed effects model would be applied (35). When I2 >50%, multiple meta regression was performed to explore whether the heterogeneity could be explained by study design, population, cancer type, NAT type, or measuring tool. The following subgroup analyses was used to illustrate the source of heterogeneity identified by meta regression.
Moreover, sensitivity analysis was conducted by sequentially removing each study, and Begg’s test was used to evaluate publication bias. A two-sided P value <0.05 was defined as statistical significance in this study. For those postoperative outcomes that could not be combined, descriptive analysis would be applied.
Results
Search Results and Study Characteristic
A total of 268 articles were found after removal of duplications. Finally, 19 literatures (16–18, 23–27, 29, 30, 36–44) were included in our study with a total sample size of 1,954 participants. The flow diagram of the selection process was presented in Figure 1. Among these studies, there were 3 prospective studies (38, 40, 42), while others are retrospective studies. Thirteen studies (18, 24, 26, 29, 30, 37–44) focused on patients with esophageal cancer, one (27) focused on gastro- esophageal cancer, two (25, 36) focused on gastric cancer and three (16, 17, 23) focused on rectal cancer. Furthermore, more than half of the studies (18, 23–26, 29, 36–38, 41) were conducted in the Asian population, with the remaining nine from non-Asian population (16, 17, 27, 30, 39, 40, 42–44). Most studies have limited NAT to neoadjuvant chemotherapy (NAC) or neoadjuvant chemoradiotherapy (NCRT), while 7 studies (16, 25, 29, 30, 41–43) included both therapies. In addition, computed tomography (CT) was the most used tool to measure muscle mass, and only three studies (18, 24, 38) used bioelectrical impedance analysis (BIA). The characteristics of the included studies are shown in Tables 2, 3.
Quality Assessment
Eight studies were of good quality (16, 18, 25–27, 36, 37, 41), and the others (17, 23, 24, 29, 30, 38–40, 42–44) were regarded as fair. However, it should be noted that all studies did not provide a sample size justification. The quality assessments of the included studies are summarized in Table 4.
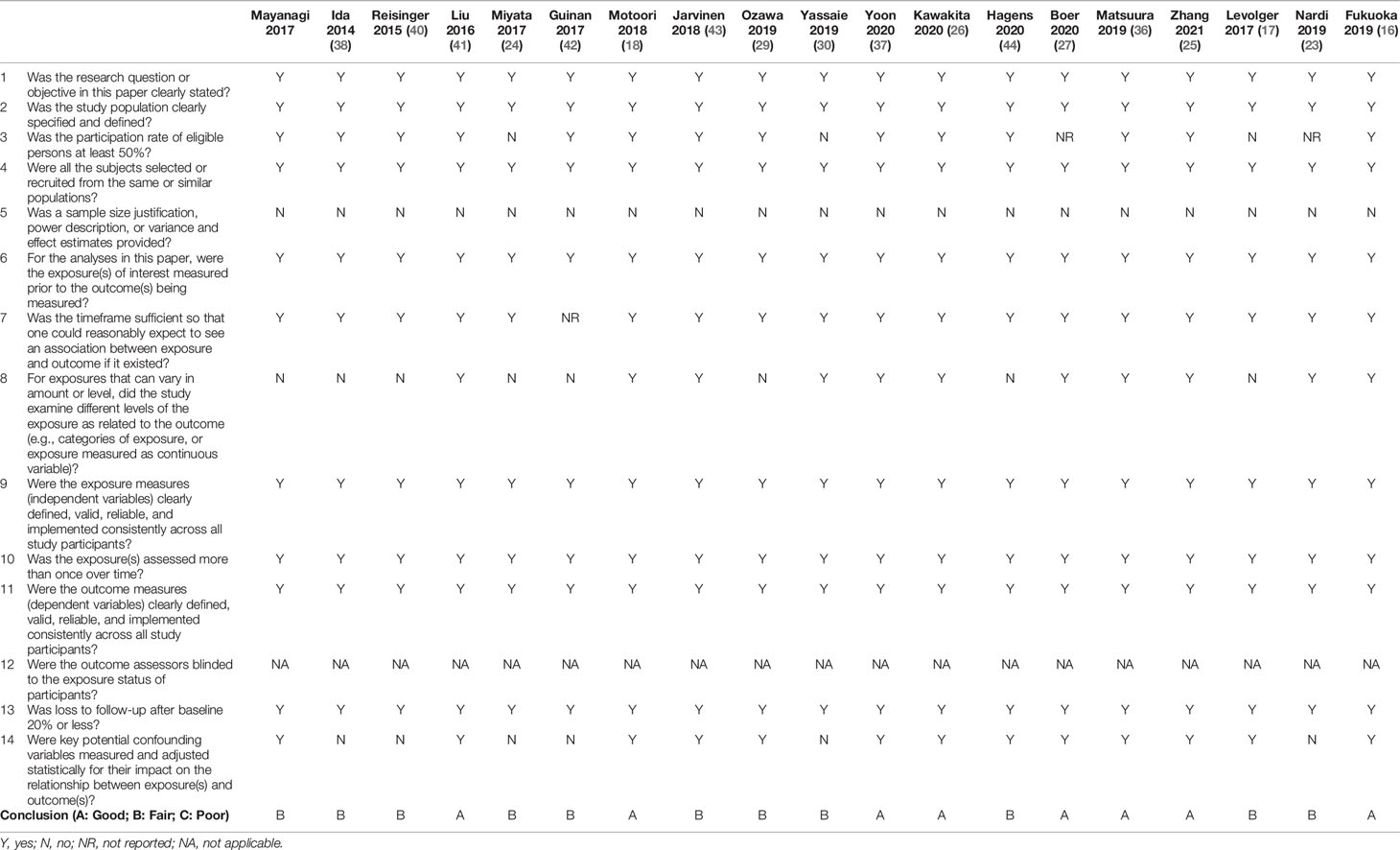
Table 4 Quality assessment for included studies based on NIH Quality Assessment Tool for Observational Cohort and Cross-Sectional Studies (n = 19).
Effect of Neoadjuvant Therapy on Skeletal Muscle Mass
The skeletal muscle indicators involved in the review include skeletal muscle mass (SMM), skeletal muscle index (SMI), skeletal muscle area (SMA), psoas muscle index (PMI), and total psoas muscle area (TPA), and only the study of Yip et al. (39) used fat free mass (FFM) to represent skeletal muscle. In this study, FFM was estimated using a regression equation based on skeletal muscle mass, and thus we included it in our study. In addition, some studies determined the specific cut-off value for the rate of muscle loss to divide patients into severe loss group and no significant muscle loss group. However, the value varied from 3% to 13% due to different calculation methods. Two studies (18, 43) set the value based on previous studies, and four (16, 25, 30, 37) used the ROC curve, with the remaining three studies (26, 27, 41) used specific percentile values to determine the cut-off value.
Nine studies (23, 24, 27, 37, 39, 40, 42–44) reported the change in the prevalence of sarcopenia during NAT, and the meta-analysis showed that the risk of sarcopenia increased 22% after receiving NAT (HR=1.22, 95% CI 1.14, 1.31, Z=4.286, P<0.001) (Figure 2). Meanwhile, seven studies (18, 27, 36, 39–42) reported a significant change of skeletal muscle during NAT, while the other twelve studies observed the opposite result (16, 17, 23–26, 29, 30, 37, 38, 43, 44). Bases on the extracted data, we included sixteen studies (23, 24, 27, 36–40, 42) with 20 groups of sufficient data in our meta-analysis to explore the effect of NAT on skeletal muscle. In the random effects model, NAT was associated with skeletal muscle loss, with a standardized mean difference of -0.20 (95% CI -0.31, -0.09, Z=3.49, P<0.001). However, there was a moderate heterogeneity between the studies (I2 = 62.2%, P<0.001) (Figure 3). As such, we conducted multiple meta regression according to the study design, population, cancer type, neoadjuvant therapy type, and measuring tool. The results indicate that population, neoadjuvant therapy type, and measuring tool were the potential sources of heterogeneity (Table 5). From the following subgroup analysis results (Supplement Figure S1), skeletal muscle mass decreased significantly in the non-Asian population group, NCRT group and CT group (random effect models: SMD = -0.19, 95% CI -0.28, -0.09, Z=3.69, P<0.001; SMD = -0.25, 95% CI -0.44, -0.07, Z=2.66, P=0.008; SMD = -0.23, 95% CI -0.34, -0.12, Z=3.99, P<0.001, respectively).
Relationship Between Muscle Loss During Neoadjuvant Therapy and Postoperative Outcomes
Long-Term Survival Outcomes
Nine studies (16, 17, 23, 26, 37, 39, 41, 43, 44) reported the relationship between muscle loss during NAT and postoperative overall survival. Five studies (16, 37, 41, 43, 44) showed that the group with muscle loss had a poorer overall survival than the group without, while the others did not find a significant difference between them. For disease-free survival, five studies (16, 18, 23, 29, 37) demonstrated that it was associated with the decrease in muscle mass during NAT, but two studies (26, 40) could not observe the difference.
Meanwhile, six studies (16, 17, 29, 37, 41, 43) provided sufficient data on survival outcomes (Table 3). Based on the extracted data, we conducted the meta-analyses. As shown in Figure 4, the results indicate that in the fixed effects model, muscle loss during NAT was significantly related to overall survival (HR=2,08, 95% CI =1.47, 2.95, Z=4.12, P<0.001, I2 = 0.0%). However, in the random effects model, no significant association was found with disease-free survival (HR=1.50, 95% CI =0.68, 3.29, Z=1.00, P=0.317, I2 = 73.0%).
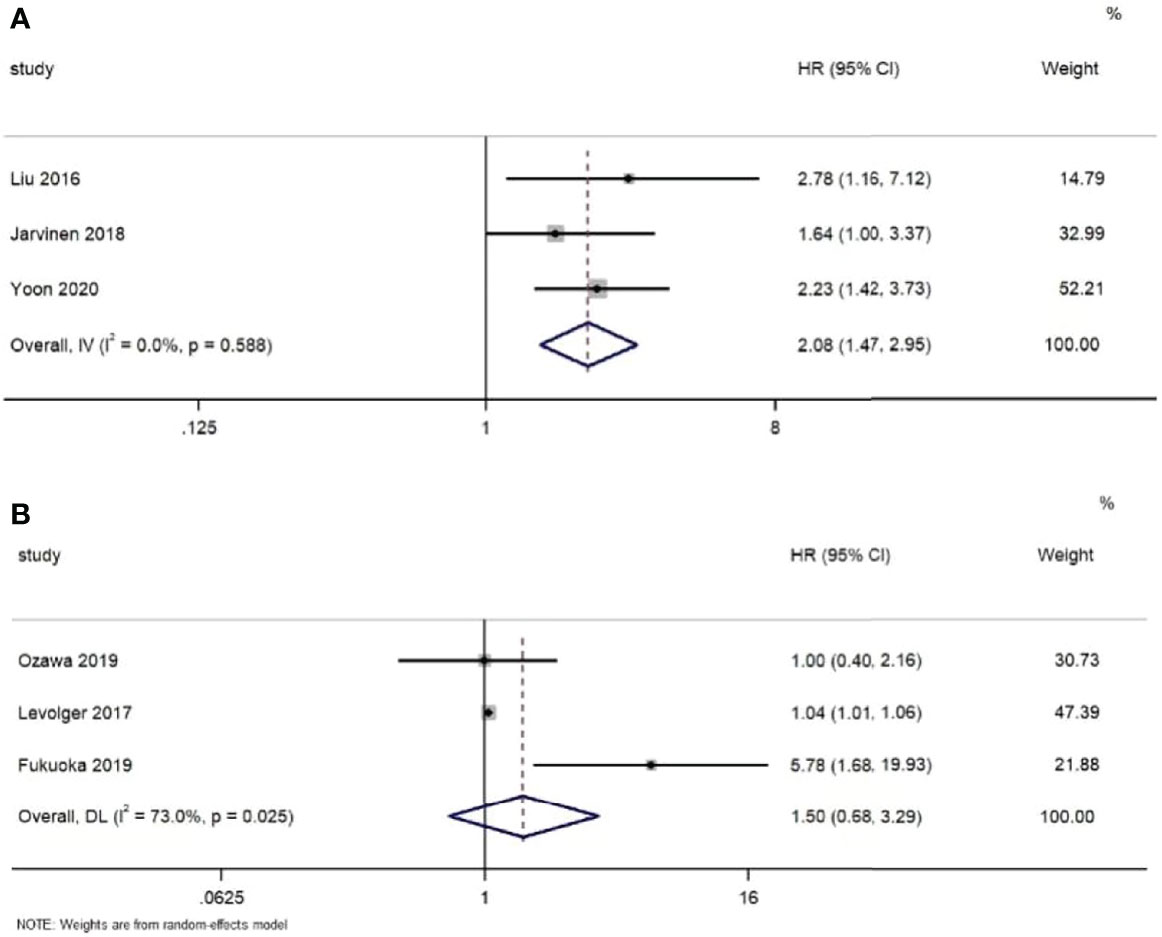
Figure 4 Forest plots of the relationship between muscle loss during neoadjuvant therapy and (A) overall survival and (B) disease-free survival
Postoperative Complications
There were seven studies (16, 25, 27, 29, 38, 41, 43) concerning the effect of muscle loss during NAT on postoperative total complications, while only one study (38) demonstrated that total complications were associated with marked muscle loss and the others showed no significance. Two studies (16, 27) involved severe complications, which was defined as Clavien-Dindo graded 3 (45), though the results were not consistent. Except for total complications and severe complications, some studies also demonstrated specific complications, including blood loss (41), anastomotic leakage (27, 30, 41), pneumonia (27, 29, 30, 41), recurrent nerve paralysis (41) and chylothorax (30, 41), but none of the results had a significant difference. However, when considering postoperative infection, there were two studies showing that muscle loss during NAT was associated with a higher rate of infection (18, 27).
There were five studies (16, 27, 29, 41, 43), 3 (27, 30, 41) and 4 studies (27, 30, 41, 43) separately included in the meta-analyses of the relationship between muscle loss and total complications, pneumonia, and anastomotic leakage (Table 3). The pooled results in the random effects model revealed that there was no significant association between muscle loss and these postoperative outcomes. (Figure 5B (OR=0.88, 95% CI =0.57, 1.35, Z=0.6, P=0.55), Figure 5C (OR=1.05, 95% CI =0.63, 1.77, Z=0.19, P=0.85), Figure 5A (OR=1.62, 95% CI =0.48, 5.48, Z=0.77, P=0.44)
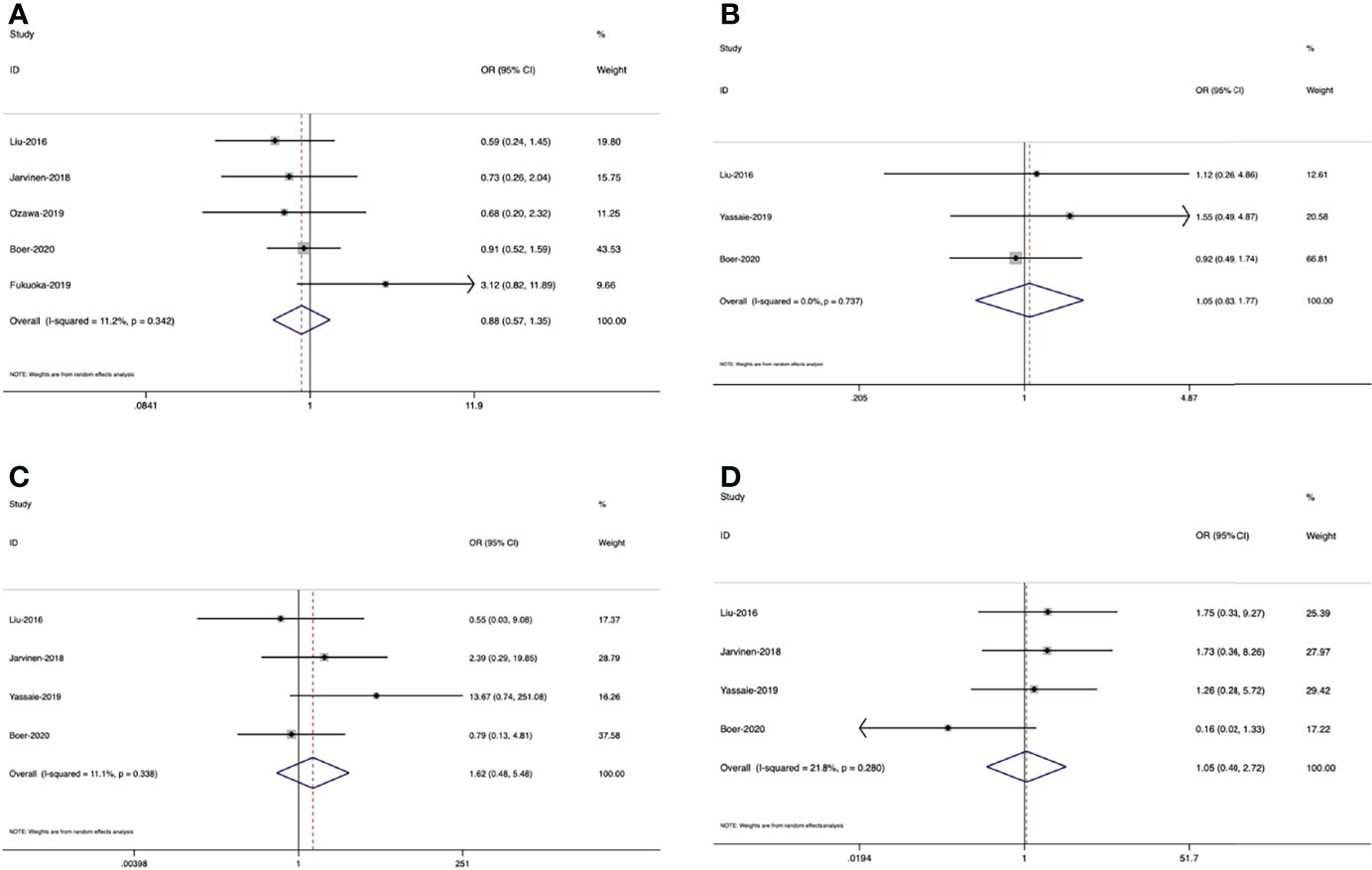
Figure 5 Forest plots of the relationship between muscle loss during neoadjuvant therapy and (A) total complications, (B) anastomotic leakage, (C) pneumonia and (D) mortality
Postoperative 30-d Mortality
There were four studies (27, 30, 40, 41) illustrating the association between loss of muscle mass and postoperative 30-d mortality. One study (30) supported that decreased muscle mass during neoadjuvant therapy could be a predictor of increased mortality in patients receiving esophageal cancer resection, while two studies (27, 41) could not support this conclusion. Another study (40) found that in patients with stage III–IV tumors, there was a significant difference between 30-d mortality and muscle loss, but this difference did not exist in patients with stage I-II tumors. However, the result of meta-analysis demonstrated that muscle loss was not related to postoperative mortality. [Figure 5D (OR=1.05, 95% CI =0.40, 2.72, Z=0.1, P=0.922]
Length of Hospital Stay
Three studies (29, 40, 41) mentioned length of hospital stay and these results all demonstrated that there were no significant differences between the muscle loss and non-muscle loss groups.
Publication Bias and Sensitivity Analysis
The funnel plot revealed that there was no high publication bias in these studies (Begg’s test, P=0.544). Furthermore, we conducted a sensitivity analysis for the meta-analysis of effect of NAT on muscle mass and the results were stable when separately excluding studies. The results are shown in Figure 6.
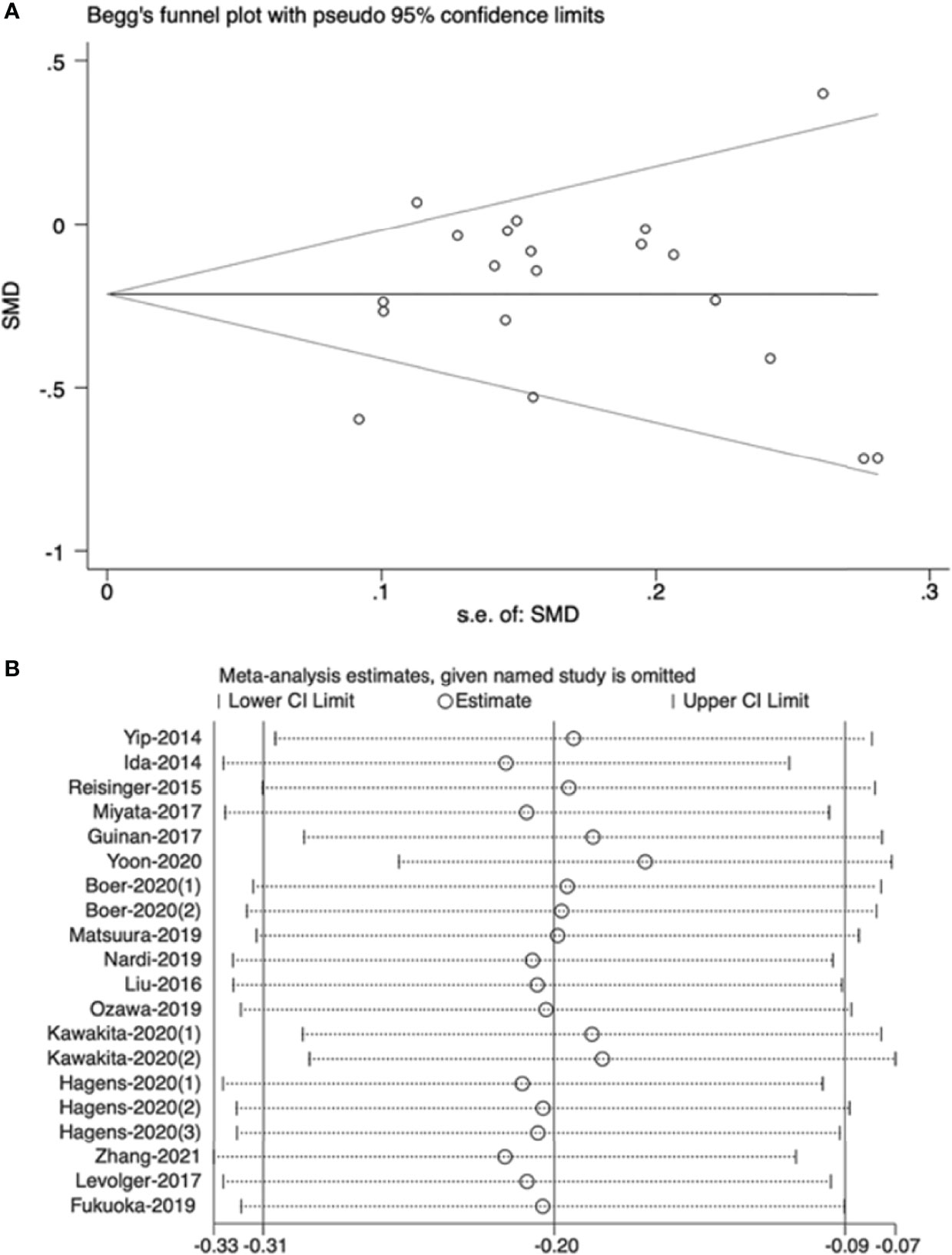
Figure 6 (A) Publication bias of effect of neoadjuvant therapy on muscle mass, (B) Sensitivity analysis of effect of neoadjuvant therapy on muscle mass
Discussion
This systematic review and meta-analysis was the first to investigate the skeletal muscle change during neoadjuvant therapy in patients with gastrointestinal cancers. The pooled results suggest that NAT significantly reduced skeletal muscle mass and muscle loss during NAT could be a prognostic indicator for overall survival.
Body weight (BW) and body mass index (BMI) are the most widely used indicators to evaluate nutrition status, which can be easily assessed in clinical practice (46). However, due to the high prevalence of chemotherapy-induced oedema (47), BW, or BMI may not be the reliable and sensitive indicator for patients receiving NAT. It is notable that most studies included in our study only found the decrease of skeletal muscle mass after NAT without any significant change in BW or BMI. Patients with GI cancers were reported to maintain or even gain body weight during NAT (36, 42), while more than 50% patients experienced skeletal muscle loss (18, 29, 41), and the mean rate of loss was around 5% (16, 26, 39). Our meta-analysis also indicated that NAT was significantly correlated with skeletal muscle loss and the results were proved to be stable without any publication bias. Thus, more attention should be paid to the change of skeletal muscle during NAT. Currently, the detailed mechanisms for skeletal muscle loss during NAT remain uncertain but chemotherapy-related toxicities can be one related reason (24). Toxicities not only cause direct acute damage to mitochondrial function, but also increase the risk of intestinal inflammation including diarrhea, nausea, and vomiting (48–51). As the increasing energy consumption caused by intestinal inflammation, skeletal muscle protein catabolism is enhanced (52). Matsuura et al. (36) found that patients who experienced severe diarrhea showed more skeletal muscle loss. Therefore, prevention of those symptoms may help to preserve muscle mass during NAT. Additionally, the relationship between muscle loss and the effectiveness of NAT should be explored in future study.
In our study, the population was one of the potential sources of heterogeneity based on meta regression. The Asian population has a lower BMI and lower prevalence of sarcopenia than non-Asian individuals (52). As such, the degree of muscle change can vary significantly by population. Moreover, NAT dominates the treatment strategies for GI cancers in western countries, while surgery is still the main method in Asia (53). There are some differences in the neoadjuvant treatment protocols (40, 41). Therefore, future studies need to explore the pattern of muscle change during NAT according to different populations. Meanwhile, the type of NAT was considered as a source of heterogeneity. NAT mainly includes neoadjuvant chemotherapy (NCT), neoadjuvant chemoradiotherapy (NCRT), and NCT combined with NCRT. Although there were no significant differences among these three therapies on improving short-term and long-term outcomes in patients with GI cancers (54, 55), our findings indicate that they had inconsistent impact on skeletal muscle. As such, researchers can further compare different types based on their effect on skeletal muscle and provide evidence for the best choice of treatment regimens. In addition, our results show that measuring tools could cause heterogeneity. Both CT and BIA are effective tools to assess body composition recommended by guidelines (56, 57), but some studies argued the accuracy of skeletal muscle mass assessment by BIA, especially for patients with severe ascites or oedema (58). However, CT requires expensive equipment, has high amount of radiation exposure, and the analysis of body composition from CT necessitates specialist software and training, while BIA is practical, non-invasive, and convenient to perform (59). Therefore, future studies should identify the most suitable tools when assessing muscle change for patients with GI cancers. The assessment of muscle mass should also be included into the regular standard of care for patients who are receiving NAT. We also found that different cancer types were not a potential source of heterogeneity in this study, while previous studies reported that patients with upper-GI cancer, especially esophageal cancer, were at a higher risk of malnutrition (60). The possible reason may be associated with the tumor obstruction in the esophagus (61), leading to the high prevalence of dysphagia and weight loss. Considering this significant syndrome and its negative impact on nutrition condition, researchers could compare the degree of muscle loss during NAT based on different types of GI cancer in the future.
Several systematic reviews have summarized the predictive value of preoperative sarcopenia or sarcopenia at diagnosis in patients with GI cancers (10, 22, 62). However, many included studies reported that the degree of sarcopenia was aggravated and a significant number of patients became sarcopenic during NAT, indicating the continuous change state of body composition and nutrition condition (24, 37, 40, 42). Hence, it is more meaningful to focus on the effect of skeletal muscle changes during NAT rather than pre-NAT or post-NAT muscle mass. We conducted qualitative and quantitative synthesis to explore the impact of muscle loss on both long-term and short-term clinical outcomes. On the aspect of long-term survival, the meta-analysis results showed a significant and strong association between muscle loss during NAT and overall survival, suggesting that skeletal muscle loss during neoadjuvant therapy could be a prognostic indicator for overall survival. However, no correlation with disease-free survival was found. The possible reasons may be related to the limited amount of included articles and high heterogeneity. Conversely, we demonstrated that severe muscle loss during NAT was not associated with short-term postoperative outcomes, including length of hospital stay, postoperative complications, and 30-d morbidity. It is notable that the following curative surgery resection may also reduce skeletal muscle mass and several studies have reported that severe acute surgery-induced muscle loss is related to longer a hospital stay, more postoperative complications, and poorer quality of life (63, 64). As such, worse short-term postoperative outcomes may be attributed to postoperative muscle loss rather than loss during NAT, and more related studies are needed to validate this conclusion. Currently, there is no gold standard to determine severe muscle loss during NAT and the cut-off value varies in our included studies. The identification of cut-off value is one direction of further study, which is beneficial to screen the risk factors for severe muscle loss and implement early detection and intervention.
Due to the close relationship between muscle loss during NAT and worse survival, target intervention should be conducted to prevent severe muscle loss during NAT. As we referred to in the “Introduction”, there are multifactorial causes of muscle loss for patients with GI cancers and patients usually suffer from lower energy intake and higher energy expenditure (65). Symptoms like sarcopenia, dysphagia, malnutrition, and osteoporosis can overlap in the individuals, especially in elderly or frail patients (66). Therefore, comprehensive and multidisciplinary management is crucial in clinical practice and alternative strategies for muscle loss in cancer patients mainly include exercise training, nutritional supplementation, appetite stimulants, and other developing pharmacological agents (67, 68). Among them, exercise training is recommended as an effective non-pharmacological intervention to reduce muscle loss and improve muscle function. One systematic review (69) demonstrated that resistance training could improve mitochondrial density and dynamics, while endurance training was related to increased mitochondrial antioxidant capacity, which can provide strong evidence for clinical target exercise intervention. Currently, there are several drugs being tested in the preclinical or at Phase III stage, which have great possibility to preserve muscle and fat mass and prolong survival (68). As such, a balanced combination of pharmacological and non-pharmacological interventions should be an important goal in the future.
Some limitations should be acknowledged in this study. Firstly, all included studies were observational study in English, and more than half of studies were graded as moderate quality, which may affect the strength of evidence and increase the risk of bias. Secondly, several studies performed Kaplan-Meier method and log-rank test to analyze the effect of muscle loss on clinical outcomes. Therefore, we failed to obtain sufficient data of HRs and ORs for those outcomes. In addition, when the association between muscle loss and outcomes was summarized, only a small number of articles were included in our meta-analysis and subgroup analysis were not stratified.
Conclusion
In summary, this systematic review and meta-analysis revealed that skeletal muscle decreased significantly during neoadjuvant therapy in patients with gastrointestinal cancers and skeletal muscle loss was strongly associated with worse overall survival. Future research should identify the criteria of severe skeletal muscle and further explore the effect on short-term clinical outcomes. Moreover, high-quality prospective studies and randomized controlled trials based on specific population, neoadjuvant treatment, measuring tool and cancer type are needed.
Data Availability Statement
The original contributions presented in the study are included in the article/Supplementary Material. Further inquiries can be directed to the corresponding author.
Author Contributions
QX contributed to study design. X-YX and X-MJ were responsible for literature search, data extraction and analysis and wrote the manuscript. S-QZ contributed to study selection. HX, J-HL, CY, and QX revised and polished the article. All authors contributed to the article and approved the submitted version.
Funding
This study was supported by Project “The exploration of trajectories and intervention program of frailty for gastric cancer survivors based on the health ecology theory” supported by National Natural Science Foundation of China (NSFC) (No.82073407).
Conflict of Interest
The authors declare that the research was conducted in the absence of any commercial or financial relationships that could be construed as a potential conflict of interest.
Publisher’s Note
All claims expressed in this article are solely those of the authors and do not necessarily represent those of their affiliated organizations, or those of the publisher, the editors and the reviewers. Any product that may be evaluated in this article, or claim that may be made by its manufacturer, is not guaranteed or endorsed by the publisher.
Supplementary Material
The Supplementary Material for this article can be found online at: https://www.frontiersin.org/articles/10.3389/fonc.2022.892935/full#supplementary-material
Supplementary Figure 1 | Subgroup analysis of muscle change based on (A) population; (B) NAT type; (C) measuring tool.
References
1. Sung H, Ferlay J, Siegel RL, Laversanne M, Soerjomataram I, Jemal A, et al. Global Cancer Statistics 2020: GLOBOCAN Estimates of Incidence and Mortality Worldwide for 36 Cancers in 185 Countries. CA Cancer J Clin (2021) 71(3):209–49. doi: 10.3322/caac.21660
2. Ao S, Wang Y, Song Q, Ye Y, Lyu G. Current Status and Future Perspectives on Neoadjuvant Therapy in Gastric Cancer. Chin J Cancer Res (2021) 33(2):181–92. doi: 10.21147/j.issn.1000-9604.2021.02.06
3. Frei E 3rd. Clinical Cancer Research: An Embattled Species. Cancer (1982) 50(10):1979–92. doi: 10.1002/1097-0142(19821115)50:10<1979::AID-CNCR2820501002>3.0.CO;2-D
4. Wang XZ, Zeng ZY, Ye X, Sun J, Zhang ZM, Kang WM, et al. Interpretation of the Development of Neoadjuvant Therapy for Gastric Cancer Based on the Vicissitudes of the NCCN Guidelines. World J Gastrointest Oncol (2020) 12(1):37–53. doi: 10.4251/wjgo.v12.i1.37
5. Cespedes Feliciano EM, Kroenke CH, Caan BJ. The Obesity Paradox in Cancer: How Important Is Muscle? Annu Rev Nutr (2018) 38:357–79. doi: 10.1146/annurev-nutr-082117-051723
6. Minetto MA, Busso C, Gamerro G, Lalli P, Massazza G, Invernizzi M. Quantitative Assessment of Volumetric Muscle Loss: Dual-Energy X-Ray Absorptiometry and Ultrasonography. Curr Opin Pharmacol (2021) 57:148–56. doi: 10.1016/j.coph.2021.02.002
7. Invernizzi M, Venetis K, Sajjadi E, Piciotti R, de Sire A, Fusco N. Understanding the Biology of Volumetric Muscle Loss for an Individualized Exercise Rehabilitation Approach in Breast Cancer Patients. Curr Opin Pharmacol (2021) 58:27–34. doi: 10.1016/j.coph.2021.03.007
8. Bozzetti F. Chemotherapy-Induced Sarcopenia. Curr Treat Pptions Oncol (2020) 21(1):7. doi: 10.1007/s11864-019-0691-9
9. Anandavadivelan P, Lagergren P. Cachexia in Patients With Oesophageal Cancer. Nat Rev Clin Oncol (2016) 13(3):185–98. doi: 10.1038/nrclinonc.2015.200
10. Pamoukdjian F, Bouillet T, Lévy V, Soussan M, Zelek L, Paillaud E, et al. Prevalence and Predictive Value of Pre-Therapeutic Sarcopenia in Cancer Patients: A Systematic Review. Clin Nutr (2018) 37(4):1101–13. doi: 10.1016/j.clnu.2017.07.010
11. Hua H, Xu X, Tang Y, Ren Z, Xu Q, Chen L, et al. Effect of Sarcopenia on Clinical Outcomes Following Digestive Carcinoma Surgery: A Meta-Analysis. Support Care Cancer (2019) 27(7):2385–94. doi: 10.1007/s00520-019-04767-4
12. Onishi S, Shiraki M, Nishimura K, Hanai T, Moriwaki H, Shimizu M. Prevalence of Sarcopenia and Its Relationship With Nutritional State and Quality of Life in Patients With Digestive Diseases. J Nutr Sci Vitaminol (2018) 64(6):445–53. doi: 10.3177/jnsv.64.445
13. Kapiteijn E, Marijnen CA, Nagtegaal ID, Putter H, Steup WH, Wiggers T, et al. Preoperative Radiotherapy Combined With Total Mesorectal Excision for Resectable Rectal Cancer. N Engl J Med (2001) 345(9):638–46. doi: 10.1056/NEJMoa010580
14. Schuhmacher C, Gretschel S, Lordick F, Reichardt P, Hohenberger W, Eisenberger CF, et al. Neoadjuvant Chemotherapy Compared With Surgery Alone for Locally Advanced Cancer of the Stomach and Cardia: European Organisation for Research and Treatment of Cancer Randomized Trial 40954. J Clin Oncol (2010) 28(35):5210–8. doi: 10.1200/JCO.2009.26.6114
15. Ando N, Kato H, Igaki H, Shinoda M, Ozawa S, Shimizu H, et al. A Randomized Trial Comparing Postoperative Adjuvant Chemotherapy With Cisplatin and 5-Fluorouracil Versus Preoperative Chemotherapy for Localized Advanced Squamous Cell Carcinoma of the Thoracic Esophagus (JCOG9907). Ann Surg Oncol (2012) 19(1):68–74. doi: 10.1245/s10434-011-2049-9
16. Fukuoka T, Maeda K, Nagahara H, Shibutani M, Iseki Y, Matsutani S, et al. Change in PMI During Neoadjuvant Therapy Is a Predictive Prognostic Marker in Rectal Cancer. Anticancer Res (2019) 39(9):5157–63. doi: 10.21873/anticanres.13711
17. Levolger S, van Vledder MG, Alberda WJ, Verhoef C, de Bruin RWF, JNM IJ, et al. Muscle Wasting and Survival Following Pre-Operative Chemoradiotherapy for Locally Advanced Rectal Carcinoma. Clin Nutr (2018) 37(5):1728–35. doi: 10.1016/j.clnu.2017.06.028
18. Motoori M, Fujitani K, Sugimura K, Miyata H, Nakatsuka R, Nishizawa Y, et al. Skeletal Muscle Loss During Neoadjuvant Chemotherapy Is an Independent Risk Factor for Postoperative Infectious Complications in Patients With Advanced Esophageal Cancer. Oncology (2018) 95(5):281–7. doi: 10.1159/000490616
19. Deng HY, Zha P, Peng L, Hou L, Huang KL, Li XY. Preoperative Sarcopenia Is a Predictor of Poor Prognosis of Esophageal Cancer After Esophagectomy: A Comprehensive Systematic Review and Meta-Analysis. Dis Esophagus (2019) 32(3):doy115. doi: 10.1093/dote/doy115
20. Trejo-Avila M, Bozada-Gutiérrez K, Valenzuela-Salazar C, Herrera-Esquivel J, Moreno-Portillo M. Sarcopenia Predicts Worse Postoperative Outcomes and Decreased Survival Rates in Patients With Colorectal Cancer: A Systematic Review and Meta-Analysis. Int J Colorectal Dis (2021) 36(6):1077–96. doi: 10.1007/s00384-021-03839-4
21. Chen F, Chi J, Liu Y, Fan L, Hu K. Impact of Preoperative Sarcopenia on Postoperative Complications and Prognosis of Gastric Cancer Resection: A Meta-Analysis of Cohort Studies. Arch Gerontol Geriatr (2022) 98:104534. doi: 10.1016/j.archger.2021.104534
22. Jin SB, Tian ZB, Ding XL, Guo YJ, Mao T, Yu YN, et al. The Impact of Preoperative Sarcopenia on Survival Prognosis in Patients Receiving Neoadjuvant Therapy for Esophageal Cancer: A Systematic Review and Meta-Analysis. Front Oncol (2021) 11:619592. doi: 10.3389/fonc.2021.619592
23. De Nardi P, Salandini M, Chiari D, Pecorelli N, Cristel G, Damascelli A, et al. Changes in Body Composition During Neoadjuvant Therapy can Affect Prognosis in Rectal Cancer Patients: An Exploratory Study. Curr Probl Cancer (2020) 44(2):100510. doi: 10.1016/j.currproblcancer.2019.100510
24. Miyata H, Sugimura K, Motoori M, Fujiwara Y, Omori T, Yanagimoto Y, et al. Clinical Assessment of Sarcopenia and Changes in Body Composition During Neoadjuvant Chemotherapy for Esophageal Cancer. Anticancer Res (2017) 37(6):3053–9. doi: 10.21873/anticanres.11660
25. Zhang Y, Li Z, Jiang L, Xue Z, Ma Z, Kang W, et al. Impact of Body Composition on Clinical Outcomes in People With Gastric Cancer Undergoing Radical Gastrectomy After Neoadjuvant Treatment. Nutrition (2021) 85:111135. doi: 10.1016/j.nut.2020.111135
26. Kawakita Y, Motoyama S, Sato Y, Wakita A, Nagaki Y, Imai K, et al. Decreases in the Psoas Muscle Index Correlate More Strongly With Survival Than Other Prognostic Markers in Esophageal Cancer After Neoadjuvant Chemoradiotherapy Plus Esophagectomy. World J Surg (2020) 44(5):1559–68. doi: 10.1007/s00268-019-05344-w
27. den Boer RB, Jones KI, Ash S, van Boxel GI, Gillies RS, O'Donnell T, et al. Impact on Postoperative Complications of Changes in Skeletal Muscle Mass During Neoadjuvant Chemotherapy for Gastro-Oesophageal Cancer. BJS Open (2020) 4(5):847–54. doi: 10.1002/bjs5.50331
28. Mirkin KA, Luke FE, Gangi A, Pimiento JM, Jeong D, Hollenbeak CS, et al. Sarcopenia Related to Neoadjuvant Chemotherapy and Perioperative Outcomes in Resected Gastric Cancer: A Multi-Institutional Analysis. J Gastrointest Oncol (2017) 8(3):589–95. doi: 10.21037/jgo.2017.03.02
29. Ozawa Y, Nakano T, Taniyama Y, Sakurai T, Onodera Y, Kamiya K, et al. Evaluation of the Impact of Psoas Muscle Index, a Parameter of Sarcopenia, in Patients With Esophageal Squamous Cell Carcinoma Receiving Neoadjuvant Therapy. Esophagus (2019) 16(4):345–51. doi: 10.1007/s10388-019-00670-3
30. Yassaie SS, Keane C, French SJH, Al-Herz FAJ, Young MK, Gordon AC. Decreased Total Psoas Muscle Area After Neoadjuvant Therapy is a Predictor of Increased Mortality in Patients Undergoing Oesophageal Cancer Resection. ANZ J Surg (2019) 89(5):515–9. doi: 10.1111/ans.15106
31. Harris JD, Quatman CE, Manring MM, Siston RA, Flanigan DC. How to Write a Systematic Review. Am J sports Med (2014) 42(11):2761–8. doi: 10.1177/0363546513497567
32. Page MJ, McKenzie JE, Bossuyt PM, Boutron I, Hoffmann TC, Mulrow CD, et al. The PRISMA 2020 Statement: An Updated Guideline for Reporting Systematic Reviews. BMJ (Clin Res ed.) (2020) 372:n71. doi: 10.31222/osf.io/v7gm2
33. Schardt C, Adams MB, Owens T, Keitz S, Fontelo P. Utilization of the PICO Framework to Improve Searching PubMed for Clinical Questions. BMC Med Inf Decision Making (2007) 7:16. doi: 10.1186/1472-6947-7-16
34. Wan X, Wang W, Liu J, Tong T. Estimating the Sample Mean and Standard Deviation From the Sample Size, Median, Range and/or Interquartile Range. BMC Med Res Methodol (2014) 14:135. doi: 10.1186/1471-2288-14-135
35. Cumpston M, Li T, Page MJ, Chandler J, Welch VA, Higgins JP, et al. Updated Guidance for Trusted Systematic Reviews: A New Edition of the Cochrane Handbook for Systematic Reviews of Interventions. Cochrane Database Syst Rev (2019) 10:Ed000142. doi: 10.1002/14651858.ED000142
36. Matsuura N, Motoori M, Fujitani K, Nishizawa Y, Komatsu H, Miyazaki Y, et al. Correlation Between Skeletal Muscle Mass and Adverse Events of Neoadjuvant Chemotherapy in Patients With Gastric Cancer. Oncology (2020) 98(1):29–34. doi: 10.1159/000502613
37. Yoon HG, Oh D, Ahn YC, Noh JM, Pyo H, Cho WK, et al. Prognostic Impact of Sarcopenia and Skeletal Muscle Loss During Neoadjuvant Chemoradiotherapy in Esophageal Cancer. Cancers (Basel) (2020) 12(4):925. doi: 10.3390/cancers12040925
38. Ida S, Watanabe M, Karashima R, Imamura Y, Ishimoto T, Baba Y, et al. Changes in Body Composition Secondary to Neoadjuvant Chemotherapy for Advanced Esophageal Cancer are Related to the Occurrence of Postoperative Complications After Esophagectomy. Ann Surg Oncol (2014) 21(11):3675–9. doi: 10.1245/s10434-014-3737-z
39. Yip C, Goh V, Davies A, Gossage J, Mitchell-Hay R, Hynes O, et al. Assessment of Sarcopenia and Changes in Body Composition After Neoadjuvant Chemotherapy and Associations With Clinical Outcomes in Oesophageal Cancer. Eur Radiol (2014) 24(5):998–1005. doi: 10.1007/s00330-014-3110-4
40. Reisinger KW, Bosmans JW, Uittenbogaart M, Alsoumali A, Poeze M, Sosef MN, et al. Loss of Skeletal Muscle Mass During Neoadjuvant Chemoradiotherapy Predicts Postoperative Mortality in Esophageal Cancer Surgery. Ann Surg Oncol (2015) 22(13):4445–52. doi: 10.1245/s10434-015-4558-4
41. Liu J, Motoyama S, Sato Y, Wakita A, Kawakita Y, Saito H, et al. Decreased Skeletal Muscle Mass After Neoadjuvant Therapy Correlates With Poor Prognosis in Patients With Esophageal Cancer. Anticancer Res (2016) 36(12):6677–85. doi: 10.21873/anticanres.11278
42. Guinan EM, Doyle SL, Bennett AE, O'Neill L, Gannon J, Elliott JA, et al. Sarcopenia During Neoadjuvant Therapy for Oesophageal Cancer: Characterising the Impact on Muscle Strength and Physical Performance. Support Care Cancer (2018) 26(5):1569–76. doi: 10.1007/s00520-017-3993-0
43. Järvinen T, Ilonen I, Kauppi J, Salo J, Räsänen J. Loss of Skeletal Muscle Mass During Neoadjuvant treatments Correlates With Worse Prognosis in Esophageal Cancer: A Retrospective Cohort Study. World J Of Surg Oncol (2018) 16(1):27. doi: 10.1186/s12957-018-1327-4
44. Hagens ERC, Feenstra ML, van Egmond MA, van Laarhoven HWM, Hulshof M, Boshier PR, et al. Influence of Body Composition and Muscle Strength on Outcomes After Multimodal Oesophageal Cancer Treatment. J Cachexia Sarcopenia Muscle (2020) 11(3):756–67. doi: 10.1002/jcsm.12540
45. Dindo D, Demartines N, Clavien PA. Classification of Surgical Complications: A New Proposal With Evaluation in a Cohort of 6336 Patients and Results of a Survey. Ann Surg (2004) 240(2):205–13. doi: 10.1097/01.sla.0000133083.54934.ae
46. Bhattacharya A, Pal B, Mukherjee S, Roy SK. Assessment of Nutritional Status Using Anthropometric Variables by Multivariate Analysis. BMC Public Health (2019) 19(1):1045. doi: 10.1186/s12889-019-7372-2
47. Groopman JE, Itri LM. Chemotherapy-Induced Anemia in Adults: Incidence and Treatment. J Natl Cancer Inst (1999) 91(19):1616–34. doi: 10.1093/jnci/91.19.1616
48. Antoun S, Borget I, Lanoy E. Impact of Sarcopenia on the Prognosis and Treatment Toxicities in Patients Diagnosed With Cancer. Curr Opin Support Palliat Care (2013) 7(4):383–9. doi: 10.1097/SPC.0000000000000011
49. Sorensen JC, Petersen AC, Timpani CA, Campelj DG, Cook J, Trewin AJ, et al. BGP-15 Protects Against Oxaliplatin-Induced Skeletal Myopathy and Mitochondrial Reactive Oxygen Species Production in Mice. Front Pharmacol (2017) 8:137. doi: 10.3389/fphar.2017.00137
50. West MA, Loughney L, Lythgoe D, Barben CP, Adams VL, Bimson WE, et al. The Effect of Neoadjuvant Chemoradiotherapy on Whole-Body Physical Fitness and Skeletal Muscle Mitochondrial Oxidative Phosphorylation In Vivo in Locally Advanced Rectal Cancer Patients–An Observational Pilot Study. PLoS One (2014) 9(12):e111526. doi: 10.1371/journal.pone.0111526
51. Gangadharan A, Choi SE, Hassan A, Ayoub NM, Durante G, Balwani S, et al. Protein Calorie Malnutrition, Nutritional Intervention and Personalized Cancer Care. Oncotarget (2017) 8(14):24009–30. doi: 10.18632/oncotarget.15103
52. Shafiee G, Keshtkar A, Soltani A, Ahadi Z, Larijani B, Heshmat R. Prevalence of Sarcopenia in the World: A Systematic Review and Meta- Analysis of General Population Studies. J Diabetes Metab Disord (2017) 16:21. doi: 10.1186/s40200-017-0302-x
53. Yoshida K, Kodera Y, Kochi M, Ichikawa W, Kakeji Y, Sano T, et al. Addition of Docetaxel to Oral Fluoropyrimidine Improves Efficacy in Patients With Stage III Gastric Cancer: Interim Analysis of JACCRO GC-07, A Randomized Controlled Trial. J Clin Oncol (2019) 37(15):1296–304. doi: 10.1200/JCO.18.01138
54. Fan N, Wang Z, Zhou C, Bludau M, Contino G, Zhao Y, et al. Comparison of Outcomes Between Neoadjuvant Chemoradiotherapy and Neoadjuvant Chemotherapy in Patients With Locally Advanced Esophageal Cancer: A Network Meta-Analysis. EClinicalMedicine (2021) 42:101183. doi: 10.1016/j.eclinm.2021.101183
55. von Döbeln GA, Klevebro F, Jacobsen AB, Johannessen HO, Nielsen NH, Johnsen G, et al. Neoadjuvant Chemotherapy Versus Neoadjuvant Chemoradiotherapy for Cancer of the Esophagus or Gastroesophageal Junction: Long-Term Results of a Randomized Clinical Trial. Dis Esophagus (2019) 32(2):10.1093/dote/doy078. doi: 10.1093/dote/doy078
56. Chen LK, Liu LK, Woo J, Assantachai P, Auyeung TW, Bahyah KS, et al. Sarcopenia in Asia: Consensus Report of the Asian Working Group for Sarcopenia. J Am Med Dir Assoc (2014) 15(2):95–101. doi: 10.1016/j.jamda.2013.11.025
57. Cruz-Jentoft AJ, Bahat G, Bauer J, Boirie Y, Bruyère O, Cederholm T, et al. Sarcopenia: Revised European Consensus on Definition and Diagnosis. Age Ageing (2019) 48(4):601. doi: 10.1093/ageing/afz046
58. Ohara M, Suda G, Kimura M, Maehara O, Shimazaki T, Shigesawa T, et al. Computed Tomography, Not Bioelectrical Impedance Analysis, is the Proper Method for Evaluating Changes in Skeletal Muscle Mass in Liver Disease. JCSM Rapid Commun (2020) 3(2):103–14. doi: 10.1002/rco2.20
59. Tojek K, Banaszkiewicz Z, Budzyński J. Body Composition Among Patients Undergoing Surgery for Colorectal Cancer. Prz Gastroenterol (2021) 16(1):47–55. doi: 10.5114/pg.2021.104736
60. Jordan T, Mastnak DM, Palamar N, Kozjek NR. Nutritional Therapy for Patients With Esophageal Cancer. Nutr Cancer (2018) 70(1):23–9. doi: 10.1080/01635581.2017.1374417
61. Anandavadivelan P, Lagergren P. Cachexia in Patients With Oesophageal Cancer. Nature Reviews. Clin Oncol (2016) 13(3):185–98. doi: 10.1038/nrclinonc.2015.200
62. Su H, Ruan J, Chen T, Lin E, Shi L. CT-Assessed Sarcopenia is a Predictive Factor for Both Long-Term and Short-Term Outcomes in Gastrointestinal Oncology Patients: A Systematic Review and Meta-Analysis. Cancer Imaging (2019) 19(1):82. doi: 10.1186/s40644-019-0270-0
63. Huang DD, Ji YB, Zhou DL, Li B, Wang SL, Chen XL, et al. Effect of Surgery-Induced Acute Muscle Wasting on Postoperative Outcomes and Quality of Life. J Surg Res (2017) 218:58–66. doi: 10.1016/j.jss.2017.05.045
64. Shimoda Y, Yamada T, Komori K, Watanabe H, Osakabe H, Kano K, et al. Effect of Muscle Mass Loss After Esophagectomy on Prognosis of Oesophageal Cancer. Anticancer Res (2020) 40(4):2275–81. doi: 10.21873/anticanres.14192
65. Rohm M, Zeigerer A, Machado J, Herzig S. Energy Metabolism in Cachexia. EMBO Rep (2019) 20(4):e47258. doi: 10.15252/embr.201847258
66. de Sire A, Ferrillo M, Lippi L, Agostini F, de Sire R, Ferrara PE, et al. Sarcopenic Dysphagia, Malnutrition, and Oral Frailty in Elderly: A Comprehensive Review. Nutrients (2022) 14(5):982. doi: 10.3390/nu14050982
67. Nascimento CM, Ingles M, Salvador-Pascual A, Cominetti MR, Gomez-Cabrera MC, Viña J. Sarcopenia, Frailty and Their Prevention by Exercise. Free Radical Biol Med (2019) 132:42–9. doi: 10.1016/j.freeradbiomed.2018.08.035
68. Marceca GP, Londhe P, Calore F. Management of Cancer Cachexia: Attempting to Develop New Pharmacological Agents for New Effective Therapeutic Options. Front Oncol (2020) 10:298. doi: 10.3389/fonc.2020.00298
Keywords: skeletal muscle mass (SMM), neoadjuvant therapy (NAT), prognosis, gastrointestinal cancers (GI cancers), meta-analysis
Citation: Xu X-Y, Jiang X-M, Xu Q, Xu H, Luo J-H, Yao C, Ding L-Y and Zhu S-Q (2022) Skeletal Muscle Change During Neoadjuvant Therapy and Its Impact on Prognosis in Patients With Gastrointestinal Cancers: A Systematic Review and Meta-Analysis. Front. Oncol. 12:892935. doi: 10.3389/fonc.2022.892935
Received: 09 March 2022; Accepted: 18 April 2022;
Published: 27 May 2022.
Edited by:
Luigi Marano, University of Siena, ItalyReviewed by:
Marco Invernizzi, University of Eastern Piedmont, ItalyFabio Penna, University of Turin, Italy
Copyright © 2022 Xu, Jiang, Xu, Xu, Luo, Yao, Ding and Zhu. This is an open-access article distributed under the terms of the Creative Commons Attribution License (CC BY). The use, distribution or reproduction in other forums is permitted, provided the original author(s) and the copyright owner(s) are credited and that the original publication in this journal is cited, in accordance with accepted academic practice. No use, distribution or reproduction is permitted which does not comply with these terms.
*Correspondence: Qin Xu, qinxu@njmu.edu.cn
†These authors have contributed equally to this work