- 1Department of Life Sciences and Health, OsloMet − Oslo Metropolitan University, Oslo, Norway
- 2Department of Environmental and Health, Norwegian Institute of Public Health, Oslo, Norway
- 3Department of Research, Cancer Registry of Norway, Oslo, Norway
- 4Department of Informatics, University of Oslo, Oslo, Norway
Patients who develop testicular germ cell tumours (TGCT) are at higher risk to be subfertile than the general population. The conditions are believed to originate during foetal life, however, the mechanisms behind a common aetiology of TGCT and male subfertility remains unknown. Testis-expressed 101 (TEX101) is a glycoprotein that is related to male fertility, and downregulation of the TEX101 gene was shown in pre-diagnostic TGCT patients. In this review, we summarize the current knowledge of TEX101 and its interactome related to fertility and TGCT development. We searched literature and compilation of data from curated databases. There are studies from both human and animals showing that disruption of TEX101 result in abnormal semen parameters and sperm function. Members of the TEX101 interactome, like SPATA19, Ly6k, PICK1, and ODF genes are important for normal sperm function. We found only two studies of TEX101 related to TGCT, however, several genes in its interactome may be associated with TGCT development, such as PLAUR, PRSS21, CD109, and ALP1. Some of the interactome members are related to both fertility and cancer. Of special interest is the presence of the glycosylphosphatidylinositol anchored proteins TEX101 and PRSS21 in basophils that may be coupled to the immune response preventing further development of TGCT precursor cells. The findings of this review indicate that members of the TEX101 interactome could be a part of the link between TGCT and male subfertility.
Introduction
Testis-expressed 101 (TEX101) is a glycoprotein-coding gene essential for spermatogenesis and sperm function, with the protein located on the germ cell surface (1). It is a member of the lymphocyte antigen-6 (Ly6)/urokinase-type plasminogen activator receptor (uPAR) superfamily (2) and is involved in several regulatory networks. The Lymphocyte Antigen 6 Family Member K (Ly6k) gene seems to be of particular importance. This is due to the mutual contributions needed from both TEX101 and Ly6k for their protein expression in testicular germ cells (3). Alterations in the TEX101/Ly6k complex are associated with male subfertility in mice (4).
We have observed that TEX101 is deregulated in pre-diagnostic serum testicular germ cell tumour (TGCT) cases when compared to matched controls (5). A known component of the TEX101 network, the Plasminogen Activator, Urokinase (PLAUR), showed differential expression in some TGCT subtypes compared to normal testis tissue (6). Furthermore, PLAUR is found to be involved in the mechanisms related to the invasion of malignant germ cells and is upregulated in cancer cases (7).
TGCT is a solid cell tumour derived from germ cells, being the most common malignancy among young men in large part of the world, although it accounts for only 1% of all male cancers (8). Poor semen quality is often associated with TGCT (9–11), both being considered as components of a condition called testicular dysgenesis syndrome (TDS), together with the reproductive disorders hypospadias and cryptorchidism (12, 13).
TDS components are believed to originate during foetal life and to have a common aetiology. Risk of developing TDS is thought to be multifactorial, including environmental, lifestyle, and genetic factors, as well as intrauterine growth restrictions (14–16). The mechanisms connecting the male reproductive disorders, including TGCT, are highly complex and are still mainly unknown (17, 18).
Based on its role in male fertility, TEX101 and its interacting network could be a part of the mechanisms linking male subfertility and TGCT. In this review, we present current knowledge of how TEX101 and its network, hereafter referred to as TEX101 interactome, may relate to male subfertility and to TGCT development. We also cover studies connecting TEX101 interactome to other forms of cancer, to acquire knowledge about mechanisms that could be of relevance for TGCT development. Published articles as well as results retrieved from public databases on TEX101 interactome are included.
Methods
Literature Search
We undertook queries of online literature databases including PubMed last updated January 2022 using MeSH terms (online Supplementary File S1). Our primary focus was male infertility, TGCT, cancer, and works including at least one component of the TEX101 interactome.
Database Identification and Search
To determine if a gene or protein is involved in the TEX101 interactome we used several databases and their evidence scores and curation level including the String database v.11.0b (19) provided through Gene Cards v5.6 (20) parsing the top scoring interactants. The minimum confidence score for interactions was set to 0.4, and active interaction sources to be included in the search were: text-mining, curated databases, experiments, gene co-expression, neighbour, gene fusion, and co-occurrence. The average combined score between TEX101 node and a secondary protein node for this String network was 0.91, showing high confidence for the protein-protein interactions involved in the TEX101 interactome.
The database Reactome v.78 (21) was also used for the identification of proteins related to TEX101. With the minimum confidence score set to 0.5, only one additional protein was added to the interactome from this database. The confidence scores for Reactome are taken from the database IntAct v1.0.2 (22). IntAct’s scoring system for valuing the confidence of interactants was given as both a molecular interactions (MI) score and an author score. The MI score was calculated from the weighted sum of the subscores for number of publications, experimental detection methods and interaction types, which was then normalised to a value between 0 and 1.
Genevestigator (23) was also used to determine additional genes in TEX101 interactome, as well as investigating in which cell lines and cancers the presence of TEX101 has been demonstrated. Thresholds for co-expressed genes were set to a Pearson’s correlation coefficient of at least 0.4 calculated by Genevestigator on log2-scaled expression data given in this database.
The database Harmonizome (24) was used to determine associations to TEX101, including functional associations, and was also used to locate miRNAs which target TEX101. Associations in Harmonizome are ranked by a standardised value which indicates the confidence of the association, this absolute standardised value related to the empirical p-value for the association. For this review, we only considered associations with a standardised absolute value over 2, as this indicates an equivalent p-value of 0.01.
The ARCHS4 (25) database was used to gather genes that were co-expressed with TEX101, as well as looking into the functional associations of TEX101. The ARCHS4 database shows predicted human phenotypes of genes, with associated z-scores for confidence values. Like previous databases, in ARCHS4 Pearson’s correlation coefficient determines co-expression of genes, therefore, correlation coefficient of 0.4 was set as a threshold value as this could indicate a positive correlation.
We used the IntAct database (22) to determine additional interactome proteins. For this review, a score of equal to or greater than 0.5 was required for an interaction to be included in the interactome.
BioGRID (26) is a curated repository of interaction data. It does not display confidence scores, but evidence of possible interactions is listed. BioGRID lists both gene-gene interactions and protein-protein interactions.
GENEVESTIGATOR is a commercial database with trial availability. The remaining databases used in this study are freely accessible.
Based on information from these databases, we defined the TEX101 interactome as the genes and proteins listed in Table 1.
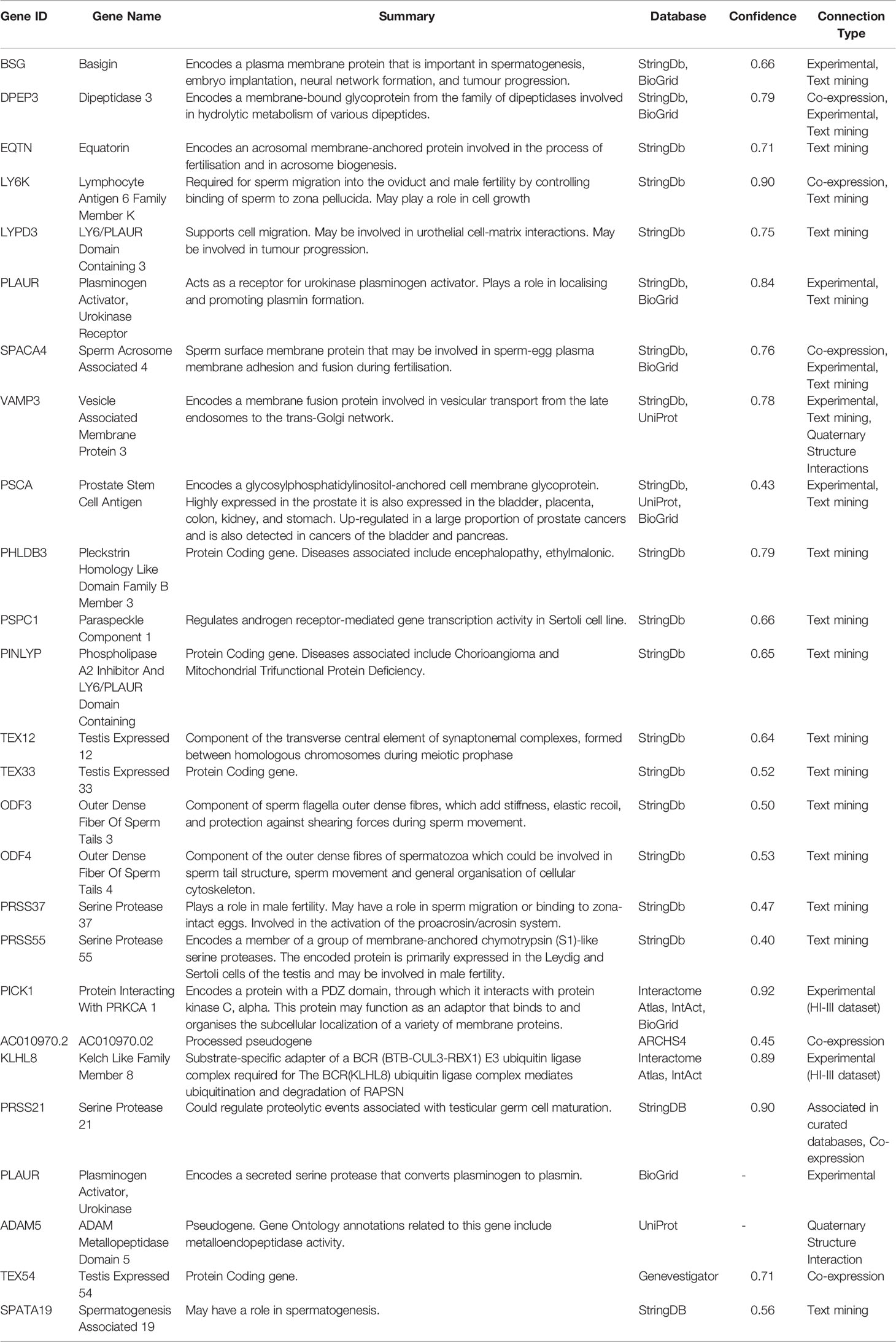
Table 1 List of TEX101 interacting components, as well as the databases the genes or proteins were taken from. Summary of gene function was retrieved from Genecards.
Results
TEX101
TEX101 is a glycosylphosphatidylinositol-anchored protein (GPI-AP) mainly expressed in germ cells, however, lower levels of expression are found elsewhere, such as in basophils (27, 28) and other tissues (Figure 1). While classical GPI-TEX101 is a membrane protein and is cleaved and released to extracellular space after phosphorylation.
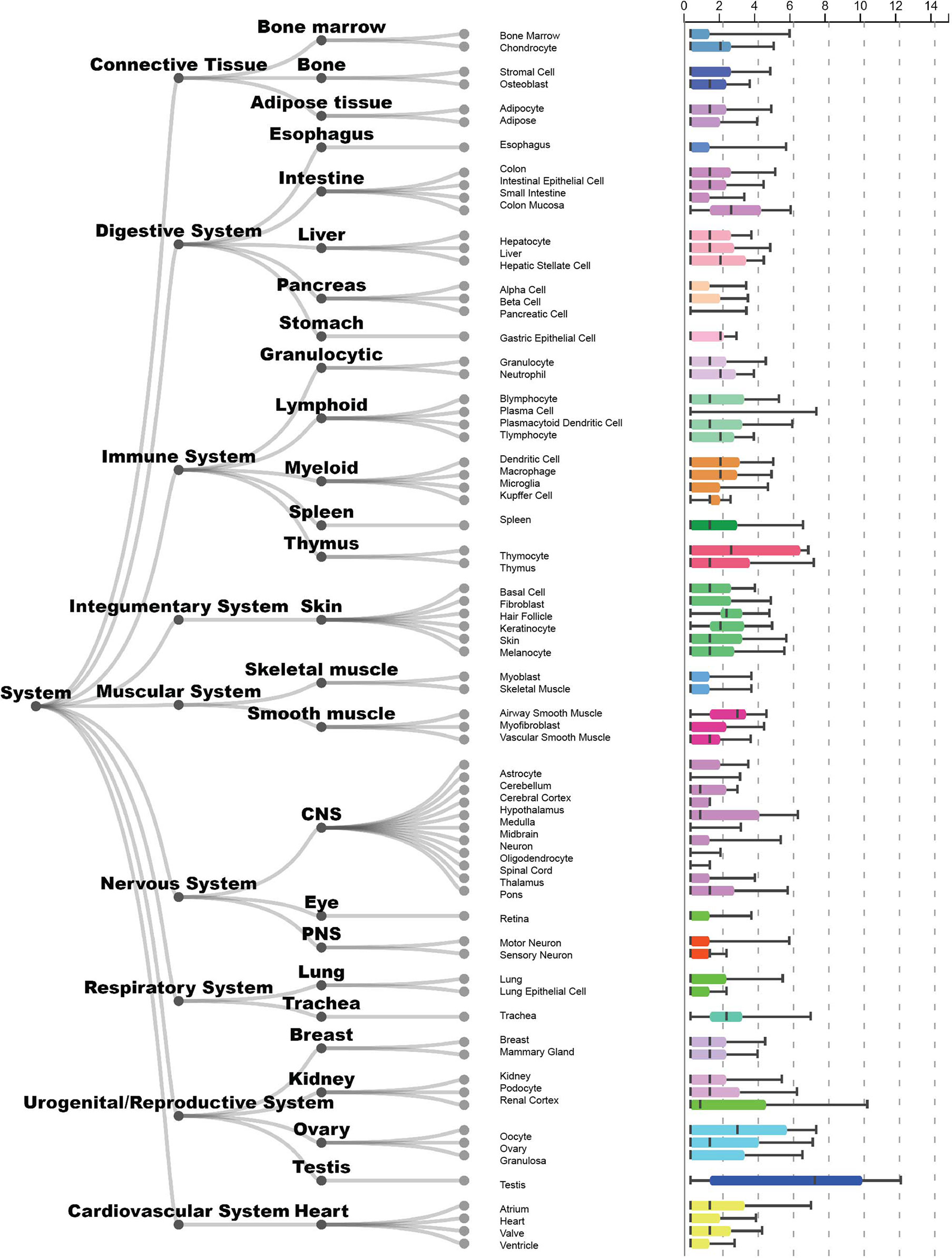
Figure 1 Tissue expression of the gene TEX101 in humans (categorised by system). This figure was constructed and modified from the ARCHS4 database, where expression levels are mined from publicly available data, and can be downloaded by any users. Samples come from many different sources and various subtypes cannot be mixed. Gene expression is normalised across different tissues and given as log transformed counts. Tissues are grouped into levels and cover most cellular contexts. This shows the variability of gene expression in non-homogeneous sample groups. Further information can be found at the TEX101 – ARCHS4 database entry (ARCHS4, n.d.) (29).
GPI-APs are crucial for sperm-egg binding in mice through GPI-AP activity facilitated by testicular angiotensin-converting enzymes (ACE) (30). TEX101 is conserved between human and other organisms, and especially orthologs seen in Pan troglodytes, Canis familiaris, and Mus musculus have high similarity to that in humans (31), indicating an important function across different phyla. Furthermore, the phyletic profile for TEX101, given in the database OrthoDB v10., shows a total of 203 ortholog genes in 111 out of 1274 species, from the eukaryota level up. TEX101 orthologs are present in several model organisms, such as C. elegans, D.melanogaster, M.musculus and D.rerio. The crystal structure of the TEX101 proteins in Homo sapiens and Mus musculus, which has 55% sequence similarity (Uniprot - Supplementary 2), has been compared. Both the overall structure and the electrostatic surface potential showed high similarity between the two, indicating that TEX101 might have similar functions in humans and mice (32).
TEX101 and Germ Cells
In mice, precursors of adult-type germ cells from both sexes express TEX101, which appears after the pregonadal period in males but only transiently in female oocytes before disappearing in the primordial follicle stage (33) In male mice, it can be found in prospermatogonium and neonatal-type undifferentiated spermatogonium, as well as spermatocytes and spermatids before disappearing after post-testicular maturation (34). Within adult mice testis, TEX101 is located on cells of the seminiferous tubules, but not in the interstitial tissues (35).
The localisation of TEX101 in human germ cells has been demonstrated by immunohistochemistry, showing higher TEX101 levels in secondary spermatocytes, spermatids, and testicular spermatozoa (36). It has also been shown to be stable in seminal plasma, where immunoassay was used to assess TEX101 levels in pre- and post-vasectomy patients (37). The presence of TEX101 in seminal plasma may be of relevance in the investigation of male infertility, being a potential biomarker (36). It has also been reported to be highly predictive for the sperm production of men with non-obstructive azoospermia (38).
The TEX101 localisation has also been identified using flow cytometry and monoclonal antibodies and ascertained that it could primarily be found on round germ cells and mature spermatozoa (39). With confocal laser immunofluorescence microscopy, TEX101 was found to be primarily located in the spermatocytes, spermatids, and spermatozoa (40).
TEX101 and Male Fertility
The association between TEX101 and male fertility issues has been described. Mice with a partial disruption of the TEX101 gene where two of the six exons were replaced, showed normal mating behaviours, and possessed normal sperm morphology and sperm motility, but were ultimately infertile (4).
Crucial for fertility, mouse models have shown TEX101 to be involved in chaperoning the ADAM3 protein, protecting it from degradation via proteases in the epididymal fluid (41, 42). A lack of TEX101 was also indicative of an infertile phenotype, due to the loss of the ability for the sperm to migrate into the oviduct (43). Disruption of TEX101 caused a loss of zona-binding ability in spermatozoa, as well as a difficulty in the spermatozoa migration into the oviduct through the uterotubal junction (42).
In human studies, enzyme-linked immunosorbent assay (ELISA) was used to measure TEX101 levels in seminal plasma across several groups of patients, including fertile men, men from couples with unexplained infertility, and men with sperm concentration below the reference range (36). The men from couples with unexplained infertility had sperm concentration within the reference range as well as normal hormone levels, and the female factors were unknown. Several of these men could therefore be fertile. One group of men had no spermatozoa in the ejaculate (azoospermia). Highest TEX101 values were observed in fertile men and in men from couples with unexplained infertility, and far lower levels in patients with reduced sperm concentration. Very low levels were observed in the samples with no spermatozoa.TEX101’s predicted phenotypes include both azoospermia (HP:0000027) and abnormal spermatogenesis (HP:0008669) obtained from the Human Phenotype Ontology database (44), with z-scores of 6.5 and 5.9 respectively. Furthermore, the Gene Ontology biological processes which are predicted for TEX101, include the fusion of sperm to egg plasma membrane, sperm motility, and spermatid development, with z-scores of 9.8, 7.2, and 7.1 respectively. Both these databases of predicted function and phenotypes indicate TEX101’s involvement in male fertility. The database ARCHS4 also showed predicted kinase interactions with the gene TEX101, with the genes PDK3 and PDK5 predicted kinase interactions (KEA) as the top results. PDK3 and PDK4 were also linked to the Sertoli cell function (45).
TEX101 and Cancer
There is a growing body of evidence that TEX101 is associated with multiple forms of cancer (46, 47). Expression of TEX101 has been demonstrated in 38% of basal cell carcinoma tissue samples compared to the absence of expression in normal skin tissue samples (46). This study also noted that TEX101 expression was significantly higher in high-risk basal cell carcinomas than in the low-risk tumours. Alongside these findings they also showed a significant co-expression between TEX101 and ODF2, a gene related to flagellum function of spermatozoa, where ODF2 insufficiency can lead to tailless spermatozoa (48).
A study among patients with chronic myeloid leukaemia showed expression of TEX101 in blood samples of 30% of patients compared to no expression in controls (46). The patients expressing TEX101 had no humoral response to TEX101 and were also mostly in the early stages of CML.
In patients with head and neck squamous cell carcinoma (HNSCC), 81% of patients showed TEX101 expression in cancer cells with an absence of TEX101 in the healthy tissue of controls (47). Initially this study was performed due to the previously known expression of Ly6k in HNSCC, as Ly6k and TEX101 are both part of the same Ly-6/urokinase-type plasminogen activator receptor (LU)-family.
Cancer Cell Line Encyclopaedia (CCLE) (49) also showed TEX101 gene CNVs profiles and expression profiles in many cancer cell lines, including multiple lung cancer cell lines (LXF-289, NCI-H2087, NCI-H889), where TEX101 exhibits both high and low expression associations. For example, in the small cell lung cancer cell line CORL95, TEX101 was one of the most highly expressed genes, with a standardised expression value of 3.4. In a human melanoma cell line HMCB, TEX101 shows one of the lowest expressed genes, with a standardised value of -3.0.
Aside from these connections to various cancer types and to male subfertility, TEX101 has to our knowledge not been associated with other disorders.
TEX101 and TGCT
Our previous study showed a decreased expression of TEX101 in serum samples of patients who later developed TGCT, compared with controls (5). Investigating TEX101 expression in seminoma TGCT tissues demonstrated a similar finding, revealing no expression of TEX101, in contrast to normal testis (50). They hypothesised that the role of TEX101 in cancer progression is due to its possible effect in reducing the function of uPAR, MMPs and cathepsin B. Loss of TEX101 and therefore gain of function of these enzymes could lead to increased cell proliferation and migration of cancer cells in head and neck squamous cell carcinoma as well as in TGCT (47, 50).
Definition of the Interactome of TEX101
The interactome for TEX101 is a complex gene network consisting of genes that have been linked to TEX101 through several methods, including text-mining, co-expression, co-occurrence, association in curated databases, experimental validation, and neighbouring genome location. Amongst the genes involved in the interactome there are a few that directly interact with and possibly depend on TEX101 for action. VAMP3 is used in transporting the de novo TEX101 protein to the cellular membrane where it resides on the cell surface (40). TEX101 and DPEP3 have been observed to directly interact, with the formation of the TEX101-DPEP3 complex, within which, TEX101 can regulate DPEP3’s protease activity (39).
The Interactome of TEX101 and Male Fertility
Several of the genes involved in the TEX101 interactome also have roles in male fertility, such as SPATA19 which is involved in the TEX101 gene network. Although the co-expression score was low (0.06) in StringDB (scored 0 to 1, where 1 is highest likelihood of interaction), co-mentions in PubMed articles scored highly, giving it an overall score of 0.56 (51, 52). SPATA19’s role in male fertility has been investigated using SPATA19 knockout mice, and it was indicated that SPATA19 is involved in sperm motility through the regulation and function of the sperm mitochondria. (53).
Another related gene, not just for male fertility but also for the function of TEX101, is the Ly6k gene. Although the co-expression of putative homologs in Mus musculus and Rattus norvegicus has a low co-expression score of 0.06, its high co-mention score in PubMed articles gives it a score of 0.9 (3, 32, 42). Ly6k is shown to be involved particularly in the migration of the sperm to the oviduct and the binding of the sperm to the ovum’s zona pellucida in mice models (42, 54). Differential proteomic profiling in human spermatozoa showed similar functions of LY6K in humans and mice (55).
PICK1, a gene found to be involved in the interactome through its associations with TEX101 in the database Reactome, has a confidence score of 0.56 with three pieces of evidence found. Its involvement was further validated through IntAct database at a protein level, where the proteins TEX101 and PICK1 were detected to have physical associations through a two hybrid prey pooling approach, two hybrid array, and validated two-hybrid method, giving it an average MI-score of 0.56. PICK1 has been studied in mice models, where PICK1 knockout mice were infertile, with reduced sperm count, severely impaired motility, and fragmented structure of the acrosomes (56).
Finally, the genes ODF3 and ODF4 are both involved in the TEX101 interactome, ODF3 through its co-mentions in PubMed articles, giving it a total score of 0.5 (52, 57). ODF4, though its co-expression of putative homologs in rodents giving it a low co-expression score of 0.06, its co-mentions in PubMed articles, scored highly with a score of 0.5 (46, 52). Outer dense fibres, coded for by the ODF genes, are part of the structure of human sperm tail, protecting it against shear forces. Therefore, it has been observed that defects in these structural fibres are higher in sperm samples with reduced motility (58).
The Interactome of TEX101 and Cancer
There are genes amongst the TEX101 interactome that are also involved in the progression and development of cancers. Examples are the ODF3 and ODF4 genes previously mentioned. The gene ODF4, a cancer/testis tissues associated gene, has previously been seen to be expressed in chronic myeloid leukaemia alongside TEX101 (46). ODF4 also showed expression in the blood samples of 30% of the CML patients in this study and was not observed in any of the control group samples.
BSG is a gene which encodes Basigin, also known as extracellular matrix metalloproteinase inducer (59). BSG has been demonstrated to have a strong association with cancer, through its overexpression in breast, colon, and hepatocellular carcinomas (60). When expressed in non-small cell lung cancer, BSG plays a role in tumour metastasis and invasion (60).
DPEP3 codes for the metalloprotease Dipeptidase 3 and has been shown to have low or no expression in normal cells, except for the testis, where it forms a complex with TEX101 (61). However, high expression is observed in high-grade serous epithelial ovarian carcinoma (EOC) (62). The isolation of the tumour initiating cells subpopulation from patient-derived xenograft EOC models led to the identification of DPEP3 as a tumour initiating cell associated protein.
PICK1 (Protein Interacting With PRKCA 1) codes for a protein which interacts with protein kinase C alpha (PRKCA) (63), and it has been identified as a tumour suppressor gene in astrocytic tumours (64). In metastatic prostate cancer, a decrease of PICK1 expression in the cancer tissue with bone metastasis was observed (65). Furthermore, an upregulation of PICK1 was associated with a decrease in the metastasis potential of prostate cancer cell lines, as well as inhibition of the invasive capabilities of bone metastasis of prostate cancer cells in a mouse intracardial model.
PSCA is a gene that is expressed in the epithelial cells of the prostate, as well as in the bladder, stomach, kidney, skin, and placenta (66). PSCA codes for a GPI-anchored protein which is part of the Thy-1/Ly-6 family, the same family that the TEX101/Ly6k complex belongs to. Although widely expressed in cells throughout the body, upregulation of PSCA is observed in advanced stages of prostate cancer and was associated with malignant progression of pre-malignant prostate lesions. Conversely, decreased expression levels of PSCA have been observed in oesophageal and gastric cancers, and PSCA is thought to have tumour-suppressing function in gastric epithelial cells (66).
Another example of GPI-anchored proteins playing a role in cancer development is the gene OPCML, which has a known role as a cancer suppressor gene and was found to be downregulated in breast and oesophageal cancers (67, 68).
The Interactome of TEX101 and TGCT
Plasminogen Activator, Urokinase Receptor (PLAUR) gene has known protein-protein interactions with TEX101 (StringR DB) and is therefore part of the TEX101 interactome. PLAUR has been found to be upregulated in TGCT tissue and in intratubular germ cell neoplasia (IGCN) tissue (7). PLAUR also showed increased levels, by 6.2-fold, in human seminoma compared to normal testis (6).
PRSS21 which codes for the serine protease testisin, has been included in the interactome due to a confidence score above 0.4 for co-expression given in StringDB. Initially its structural features and expression patterns indicated that it was involved in proteolytic events that led to TGCT development and that expression was restricted to the testis (69, 70). However, despite the involvement of PRSS21 in TGCT development, it was found not to be expressed in embryonal carcinoma cell lines derived from testicular tumours but was found to be expressed in the cervical cancer cell line HeLa and melanoma cell lines. This difference in expression both between normal testis and TGCT tissue as well as TGCT tissue and non-testis cell lines led to the belief that PRSS21 acts as a tumour suppressor in TGCT (69).
CD109 codes for protein belonging to the GPI-AP family, similarly to TEX101 and various other interactome related proteins (71). Increased levels of CD109 transcript expression levels have been seen in the squamous cell cancers of the oesophagus, cervix, and lung (72, 73). Recent germline sequencing research has shown the presence of CD109 in paediatric germ cell tumours arising from gonadal tissues, and several subtypes of paediatric germ cell tumours are shared with testicular germ cell tumours that arise after puberty, for example: teratoma, yolk sac tumour, embryonal carcinoma, and choriocarcinoma (74). A potential autosomal recessive variant of CD109 was discovered in a patient with malignant ovarian teratoma, with an increase in minor allele frequency compared to the control population (74).
ALPI codes for a protein of the same name, an alkaline phosphatase primarily found in the intestinal epithelium (75). Alongside intestinal forms of alkaline phosphatases there are several other alkaline phosphatase isoforms, including Tissue non-specific, Placental, and Germ Cell. Germ cell alkaline phosphatase is located in germ cell neoplasms, and levels of ALPI have been detected in over 75% of individual TGCT samples, primarily located in the cytoplasm or membrane. (https://www.proteinatlas.org/ENSG00000163295-ALPI/pathology/testis+cancer#ihc).
Discussion
Subfertility is associated with an increased risk of TGCT. (76–80). Decreased spermatogenesis has been observed, as well as reduced sperm motility and increased abnormal sperm morphology in TGCT patients before orchiectomy (11). However, reproductive characteristics are less commonly investigated before TGCT treatment than after, and the molecular and genetic relationship between TGCT and subfertility remains unclear.
Based on the present literature, we have shown in Figure 2 how TEX101 and its interactome may be involved in the mechanisms linking male subfertility to the development of TGCT. In addition to TEX101, several of the genes the interactome are associated with sperm production, maturation, and function (42, 53, 56, 58). In particular PRSS21, which is associated with TEX101 in curated databases, has been shown to be involved in initiation and progression of TGCT (Figure 3) and is thought to act as a tumour suppressor (69). It has also been shown that gain in PRSS21 DNA methylation may be used to diagnostic indicator of the presence of TGCT subtype non-seminoma, where the subtype seminoma would show loss of DNA methylation. and loss of PRSS21 mRNA expression could be used to diagnose progression from GCNIS to TGCT (81).
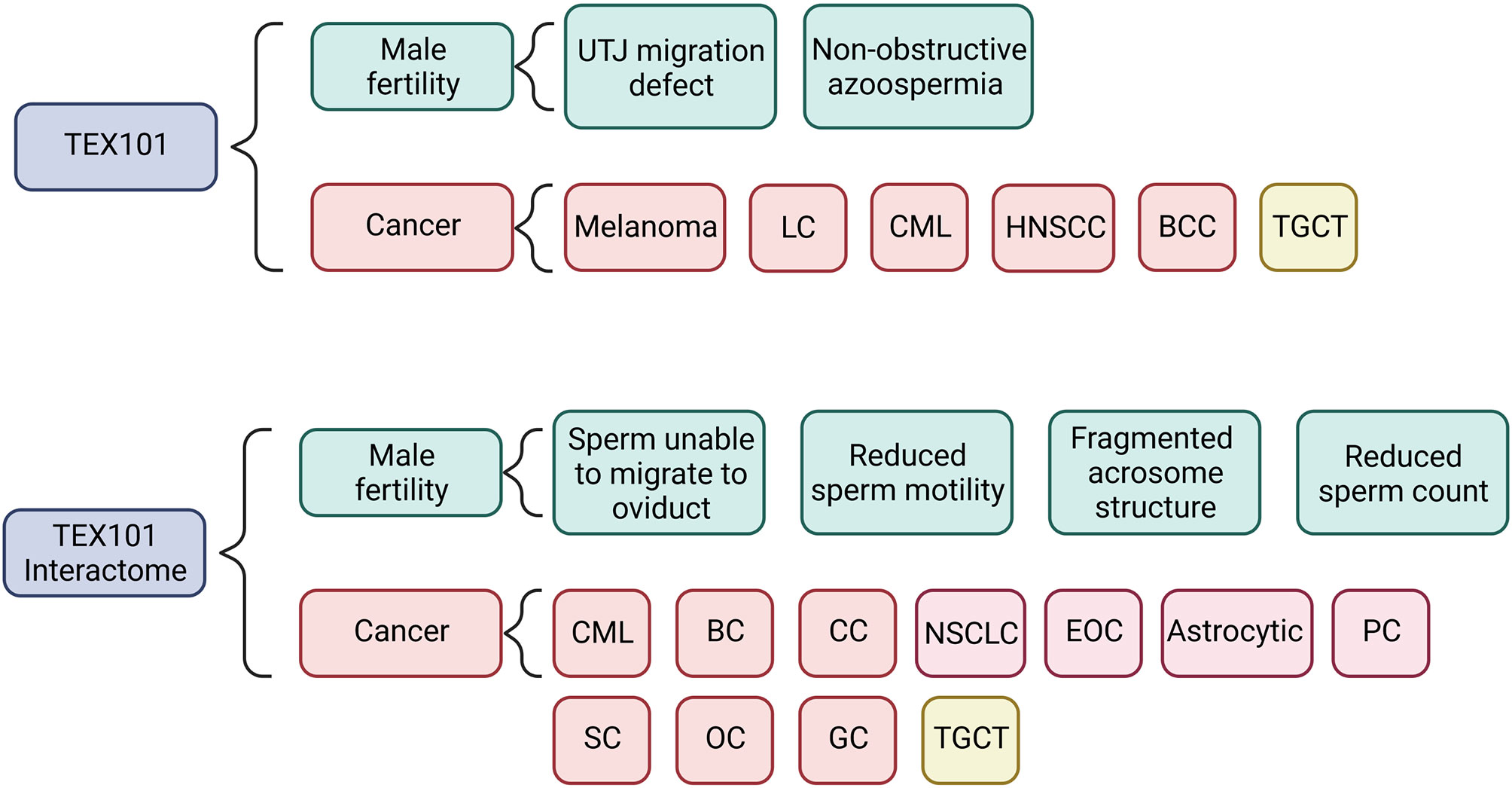
Figure 2 Summary of results for both the TEX101 and the TEX101 interactome literature and database search showing the mechanisms and types of cancer that the respective genes and proteins are involved in. Azoospermia refers to the absence of sperm in ejaculate. LC, Lung Cancer; CML, Chronic Myeloid Leukaemia; HNSCC, Head and Neck Squamous Cell Carcinoma; BCC, Basal Cell Carcinoma; TGCT, Testicular Germ Cell Tumour; CC, Colon Cancer; NSCLC, Non-Small Cell Lung Cancer; EOC, Epithelial Ovarian Cancer; PC, Prostate Cancer; SC, Skin Cancer; OC, Oesophageal Cancer; GC, Gastric Cancer.
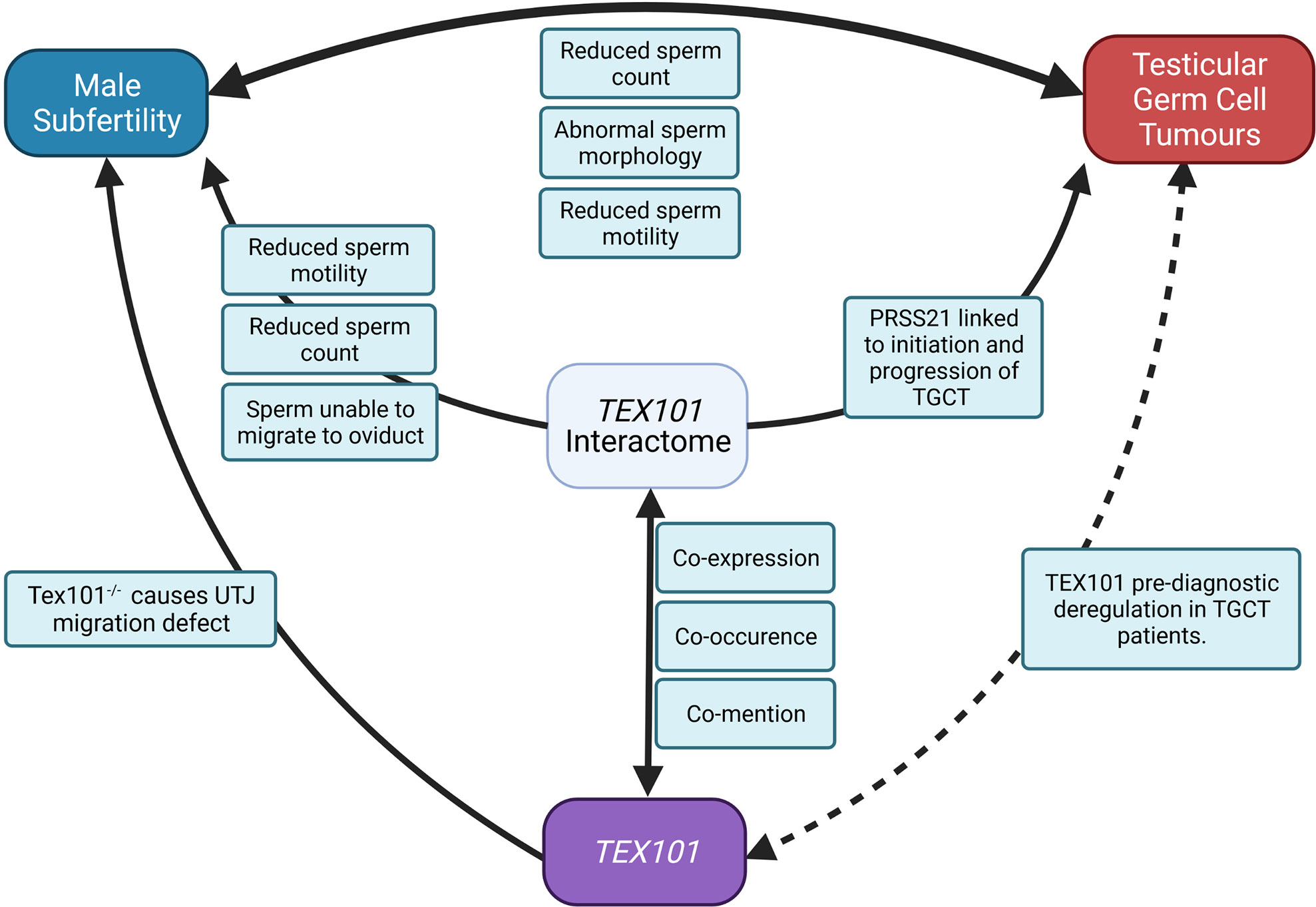
Figure 3 The roles of TEX101 and its interactome in fertility and TGCT (UJT, uterine-tubal junction).
Genome-wide association studies (GWAS) have so far identified 78 susceptibility loci for TGCT (82). Although TGCT development is unique in several aspects, we know from GWAS that there are risk genes for TGCT that are involved in pathways commonly associated with other cancer types. Examples are DNA damage response and telomere length (83). These pathways have broad oncogenic potential as they control key aspects of cell proliferation and are therefore important across multiple cancer types.
Alongside these genes of interest, it has been observed that both TEX101 and many of the interactome genes are associated with various cancer types with specific functional characteristics. The gene OPCML has a known role as a tumour suppressor gene and is downregulated in breast and oesophageal cancers (67, 68). The genes PICK1 and PSCA are also both thought to play tumour suppressing roles in astrocytic and gastric epithelial cell cancers, respectively (64, 66). Both were associated with prostate cancer, though PSCA is associated with advanced stages of prostate cancer, and upregulation of PICK1 is associated with a decrease in the metastatic potential (65). Furthermore, the gene BSG, when present in non-small cell lung cancer is associated with increased metastatic potential (60). The interactome gene CD109 has shown oncogenic potential in some subtypes of TGCT, as well as other types of cancer (74). Thus, some genes within the interactome of TEX101 are related to tumour suppression, and some are associated with oncogenesis.
TEX101 and other genes within the interactome, such as PRSS21 and CD109, belong to GPI-AP, which have been shown to perform a variety of roles throughout the body, including cell-cell adhesion and forming antigens (84). However, they have also been labelled as possible targets for immunotherapy (85–88). Interestingly, GPI-APs were shown to be overexpressed in serum for patients with other types of cancer compared to controls, and they have been suggested as potential non-invasive markers (89). It is possible that the cleaving of TEX101 proteins from the membrane, or other interactome related GPI-APs into serum, could occur upon development of germ cell neoplasia in situ (GCNIS). TEX101 is known to be 47% N-glycosylated in its natural state and has been seen to be expressed as a GPI-AP (90).
Both TEX101 and LY6K are known to be soluble in serum (43, 91), an important characteristic shared with several other GPI-APs that are already useful biomarkers for various cancer types (91–93).
Cancer/testis antigens are expressed in testis, as it is an immune privileged organ (94, 95). TEX101 has been classed as a CT antigen (46), therefore we would expect to see increased levels of TEX101 mRNA in the serum of patients with TGCT compared to controls. However, it was previously observed that TEX101 mRNA in serum showed an average decrease in log2 fold change of around -3 in TGCT patients, compared to controls (5). This could be because, despite TEX101 protein being testis specific, TEX101 mRNA is present in the basophils of control samples as well as in testis (27, 28). One function of basophils is the release of serine proteases into areas of inflammation (96). PRSS21, the previously mentioned serine protease, has also shown tissue specific expression in basophils.
Both PRSS21 and TEX101 are expressed in a subtype of white blood cell known as basophils (28, 97). Basophils have several key immune system functions, including the delivery of histamine, and the detection of early-stage cancer cells. TEX101 and PRSS21 are shown to be present in lower levels in TGCT than controls (5, 69). Our study showed this in serum samples of pre-diagnostic patients, whereas PRSS21 was shown to be lost in TGCT tissue compared to healthy tissue (5, 69). We hypothesise that lower levels of serum TEX101, prior to diagnosis, could indicate a loss of basophil function leading to a reduction of detection systems for early-stage cancers. This, coupled with the immune privilege of the testis, could prevent all immune responses to GCNIS, allowing development into TGCT. The role of the blood testis barrier in GCNIS survival and development should be investigated with further studies.
Many studies of the function of TEX101 are based on animal model organisms, primarily mice, and the results can not immediately be translated to humans. There are few animal models for studying TGCT development, but they show significant differences when compared to human TGCT, possibly due to differences in life length as well as an increased chance of developing different TGCT tumour types (98, 99). Spontaneous tumour growth in testis is unique to humans, and thus far there has been no observed cases of an animal model being able to form the precursor GCNIS cells needed for TGCT growth (100). However, as there are few studies investigating further roles of TEX101 in humans, animal models are important in studying the link between TGCT and subfertility. Interactome studies in general can help bridge the knowledge gap where model organisms could otherwise generate possible hypotheses for further research in this field.
Conclusion
Studies of TEX101 and its interactome indicate an association between subfertility and TGCT via the TEX101. PRSS21 seems to be important in this link, with its shared expression in basophils and GPI-AP role, similar to TEX101. The decrease of PRSS21 in TGCT patients and TEX101 in pre-diagnostic patients could at least partly explain the evasion of GCNIS from early detection from the immune system. Candidates of the TEX101 interactome that are found to be associated with both fertility and TGCT development should be followed up by functional studies in cell or animal models, and the role of the blood-testis barrier in immune response should also be investigated.
Author Contributions
All authors designed the study. JB and MW performed the analyses of the data. All authors drafted the manuscript. All authors discussed the results, contributed to the writing, and approved the final manuscript.
Funding
This work was supported by internal funds of OsloMet—Oslo Metropolitan University and Cancer Registry of Norway.
Conflict of Interest
The authors declare that the research was conducted in the absence of any commercial or financial relationships that could be construed as a potential conflict of interest.
Publisher’s Note
All claims expressed in this article are solely those of the authors and do not necessarily represent those of their affiliated organizations, or those of the publisher, the editors and the reviewers. Any product that may be evaluated in this article, or claim that may be made by its manufacturer, is not guaranteed or endorsed by the publisher.
Acknowledgments
Figure 2 was produced using BioRender software.
Supplementary Material
The Supplementary Material for this article can be found online at: https://www.frontiersin.org/articles/10.3389/fonc.2022.892043/full#supplementary-material
References
1. Djureinovic D, Fagerberg L, Hallström B, Danielsson A, Lindskog C, Uhlén M, et al. The Human Testis-Specific Proteome Defined by Transcriptomics and Antibody-Based Profiling. Mol Hum Reprod (2014) 20(6):476–88. doi: 10.1093/molehr/gau018
2. Loughner CL, Bruford EA, McAndrews MS, Delp EE, Swamynathan S, Swamynathan SK. Organization, Evolution and Functions of the Human and Mouse Ly6/uPAR Family Genes. Hum Genomics (2016) 10(1):10. doi: 10.1186/s40246-016-0074-2
3. Endo S, Yoshitake H, Tsukamoto H, Matsuura H, Kato K, Sakuraba M, et al. TEX101, a Glycoprotein Essential for Sperm Fertility, Is Required for Stable Expression of Ly6k on Testicular Germ Cells. Sci Rep (2016) 6(1):23616. doi: 10.1038/srep23616
4. Fujihara Y, Tokuhiro K, Muro Y, Kondoh G, Araki Y, Ikawa M, et al. Expression of TEX101, Regulated by ACE, Is Essential for the Production of Fertile Mouse Spermatozoa. Proc Natl Acad Sci (2013) 110(20):8111 LP–6. doi: 10.1073/pnas.1222166110
5. Burton J, Umu SU, Langseth H, Grotmol T, Grimsrud TK, Haugen TB, et al. Serum RNA Profiling in the 10-Years Period Prior to Diagnosis of Testicular Germ Cell Tumor. Front Oncol (2020) 10:2207. doi: 10.3389/fonc.2020.574977
6. Ulisse S, Baldini E, Mottolese M, Sentinelli S, Gargiulo P, Valentina B, et al. Increased Expression of Urokinase Plasminogen Activator and Its Cognate Receptor in Human Seminomas. BMC Cancer (2010) 10:151. doi: 10.1186/1471-2407-10-151
7. von Eyben FE, Jacobsen GK, Skotheim RI. Microinvasive Germ Cell Tumor of the Testis. Virchows Archiv (2005) 447(3):610–25. doi: 10.1007/s00428-005-1257-8
8. Trabert B, Chen J, Devesa SS, Bray F, McGlynn KA. International Patterns and Trends in Testicular Cancer Incidence, Overall and by Histologic Subtype 1973-2007. Andrology (2015) 3(1):4–12. doi: 10.1111/andr.293
9. Jacobsen R, Bostofte E, Engholm G, Hansen J, Olsen JH, Skakkebaek NE, et al. Risk of Testicular Cancer in Men With Abnormal Semen Characteristics: Cohort Study. BMJ (Clinical Res Ed.) (2000) 321(7264):789–92. doi: 10.1136/bmj.321.7264.789
10. Walsh TJ, Croughan MS, Schembri M, Chan JM, Turek PJ. Increased Risk of Testicular Germ Cell Cancer Among Infertile Men. Arch Internal Med (2009) 169(4):351–6. doi: 10.1001/archinternmed.2008.562
11. Djaladat H, Burner E, Parikh PM, Beroukhim Kay D, Hays K. The Association Between Testis Cancer and Semen Abnormalities Before Orchiectomy: A Systematic Review. J Adolesc Young Adult Oncol (2014) 3:153–9. doi: 10.1089/jayao.2014.0012
12. Skakkebaek NE, Holm M, Hoei-Hansen C, Jørgensen N, Rajpert-De Meyts E. Association Between Testicular Dysgenesis Syndrome (TDS) and Testicular Neoplasia: Evidence From 20 Adult Patients With Signs of Maldevelopment of the Testis. APMIS: Acta Pathol Microbiol Immunol Scand (2003) 111(1):1–11. doi: 10.1034/j.1600-0463.2003.11101031.x
13. Sharpe RM, Skakkebaek NE. Testicular Dysgenesis Syndrome: Mechanistic Insights and Potential New Downstream Effects. Fertil Steril (2008) 89(2 Suppl):e33–8. doi: 10.1016/j.fertnstert.2007.12.026
14. Xing J-S, Bai Z-M. Is Testicular Dysgenesis Syndrome a Genetic, Endocrine, or Environmental Disease, or an Unexplained Reproductive Disorder? Life Sci (2018) 194:120–9. doi: 10.1016/j.lfs.2017.11.039
15. Skakkebæk NE, Lindahl-Jacobsen R, Levine H, Andersson A-M, Jørgensen N, Main KM, et al. Environmental Factors in Declining Human Fertility. Nature Reviews. Endocrinology (2021) 18(3):139–57. doi: 10.1038/s41574-021-00598-8
16. Skakkebæk NE, Rajpert-De Meyts E, Main KM. Testicular Dysgenesis Syndrome: An Increasingly Common Developmental Disorder With Environmental Aspects: Opinion. Hum Reprod (2001) 16(5):972–8. doi: 10.1093/humrep/16.5.972
17. Rajpert-De Meyts E, McGlynn KA, Okamoto K, Jewett MAS, Bokemeyer C. Testicular Germ Cell Tumours. Lancet (London England) (2016) 387(10029):1762–74. doi: 10.1016/S0140-6736(15)00991-5
18. Xavier R, de Carvalho RC, Fraietta R. Semen Quality From Patients Affected by Seminomatous and Non-Seminomatous Testicular Tumor. Int Braz J Urol: Off J Braz Soc Urol (2021) 47(3):495–502. doi: 10.1590/s1677-5538.ibju.2021.99.01
19. Szklarczyk D, Gable AL, Nastou KC, Lyon D, Kirsch R, Pyysalo S, et al. The STRING Database in 2021: Customizable Protein-Protein Networks, and Functional Characterization of User-Uploaded Gene/Measurement Sets. Nucleic Acids Res (2021) 49(D1):D605–12. doi: 10.1093/nar/gkaa1074
20. Stelzer G, Rosen N, Plaschkes I, Zimmerman S, Twik M, Fishilevich S, et al. The GeneCards Suite: From Gene Data Mining to Disease Genome Sequence Analyses. Curr Protoc Bioinf (2016) 54:1–1. doi: 10.1002/cpbi.5
21. Jassal B, Matthews L, Viteri G, Gong C, Lorente P, Fabregat A, et al. The Reactome Pathway Knowledgebase. Nucleic Acids Res (2020) 48(D1):D498–503. doi: 10.1093/nar/gkz1031
22. Orchard S, Ammari M, Aranda B, Breuza L, Briganti L, Broackes-Carter F, et al. The MIntAct Project–IntAct as a Common Curation Platform for 11 Molecular Interaction Databases. Nucleic Acids Res (2014) 42:D358–63. doi: 10.1093/nar/gkt1115
23. Hruz T, Laule O, Szabo G, Wessendorp F, Bleuler S, Oertle L, et al. Genevestigator V3: A Reference Expression Database for the Meta-Analysis of Transcriptomes. Advances in Bioinformatics. 2008 (2008) 420747. doi: 10.1155/2008/420747
24. Rouillard AD, Gundersen GW, Fernandez NF, Wang Z, Monteiro CD, McDermott MG, et al. The Harmonizome: A Collection of Processed Datasets Gathered to Serve and Mine Knowledge About Genes and Proteins. Database (2016) 2016:baw100. doi: 10.1093/database/baw100
25. Lachmann A, Torre D, Keenan AB, Jagodnik KM, Lee HJ, Wang L, et al. Massive Mining of Publicly Available RNA-Seq Data From Human and Mouse. Nat Commun (2018) 9(1):1366. doi: 10.1038/s41467-018-03751-6
26. Oughtred R, Rust J, Chang C, Breitkreutz B-J, Stark C, Willems A, et al. The BioGRID Database: A Comprehensive Biomedical Resource of Curated Protein, Genetic, and Chemical Interactions. Protein Sci: A Publ Protein Soc (2021) 30(1):187–200. doi: 10.1002/pro.3978
27. The Human Protein Atlas Project. (n.d.-a). TEX101 - Human Protein Atlas. From v21.proteinatlas.org/ENSG00000131126-TEX101 (Accessed February 4, 2022).
28. Uhlén M, Linn F, M HB, Cecilia L, Per O, Adil M, et al. Tissue-Based Map of the Human Proteome. Science (2015) 347(6220):1260419. doi: 10.1126/science.1260419
29. ARCHS4. TEX101 - ARCHS4. Retrieved May 4, 2022, from https://maayanlab.cloud/archs4/gene/TEX101.
30. Kondoh G, Tojo H, Nakatani Y, Komazawa N, Murata C, Yamagata K, et al. Angiotensin-Converting Enzyme is a GPI-Anchored Protein Releasing Factor Crucial for Fertilization. Nat Med (2005) 11(2):160–6. doi: 10.1038/nm1179
31. Kriventseva EV, Kuznetsov D, Tegenfeldt F, Manni M, Dias R, Simão FA, et al. OrthoDB V10: Sampling the Diversity of Animal, Plant, Fungal, Protist, Bacterial and Viral Genomes for Evolutionary and Functional Annotations of Orthologs. Nucleic Acids Res (2019) 47(D1):D807–11. doi: 10.1093/nar/gky1053
32. Masutani M, Sakurai S, Shimizu T, Ohto U. Crystal Structure of TEX101, a Glycoprotein Essential for Male Fertility, Reveals the Presence of Tandemly Arranged Ly6/uPAR Domains. FEBS Lett (2020) 594(18):3020–31. doi: 10.1002/1873-3468.13875
33. Takayama T, Mishima T, Mori M, Ishikawa T, Takizawa T, Goto T, et al. TEX101 Is Shed From the Surface of Sperm Located in the Caput Epididymidis of the Mouse. Zygote (2005) 13(4):325–33. doi: 10.1017/S0967199405003394
34. Kurita A, Takizawa T, Takayama T, Totsukawa K, Matsubara S, Shibahara H, et al. Identification, Cloning, and Initial Characterization of a Novel Mouse Testicular Germ Cell-Specific Antigen1. Biol Reprod (2001) 64(3):935–45. doi: 10.1095/biolreprod64.3.935
35. Yoshitake H, Araki Y. Role of the Glycosylphosphatidylinositol-Anchored Protein TEX101 and Its Related Molecules in Spermatogenesis. Int J Mol Sci (2020) 21:6628–45. doi: 10.3390/ijms21186628
36. Korbakis D, Schiza C, Brinc D, Soosaipillai A, Karakosta TD, Légaré C, et al. Preclinical Evaluation of a TEX101 Protein ELISA Test for the Differential Diagnosis of Male Infertility. BMC Med (2017) 15(1):60. doi: 10.1186/s12916-017-0817-5
37. Korbakis D, Brinc D, Schiza C, Soosaipillai A, Jarvi K, Drabovich AP, et al. Immunocapture-Selected Reaction Monitoring Screening Facilitates the Development of ELISA for the Measurement of Native TEX101 in Biological Fluids. Mol Cell Proteomics: MCP (2015) 14(6):1517–26. doi: 10.1074/mcp.M114.047571
38. Jarvi K, Schlegel P, Schiza C, Drabovich A, Lau S, Soosaipillai A, et al. Semen Biomarker TEX101 Predicts Sperm Retrieval Success for Men With Testicular Failure [Version 1; Peer Review: 2 Approved With Reservations]. F1000Research (2021) 10(569). doi: 10.12688/f1000research.53338.1
39. Schiza C, Korbakis D, Panteleli E, Jarvi K, Drabovich AP, Diamandis EP. Discovery of a Human Testis-Specific Protein Complex TEX101-DPEP3 and Selection of Its Disrupting Antibodies. Mol Cell Proteomics: MCP (2018) 17(12):2480–95. doi: 10.1074/mcp.RA118.000749
40. Tsukamoto H, Yoshitake H, Mori M, Yanagida M, Takamori K, Ogawa H, et al. Testicular Proteins Associated With the Germ Cell-Marker, TEX101: Involvement of Cellubrevin in TEX101-Trafficking to the Cell Surface During Spermatogenesis. Biochem Biophys Res Commun (2006) 345(1):229–38. doi: 10.1016/j.bbrc.2006.04.070
41. Cho C. Testicular and Epididymal ADAMs: Expression and Function During Fertilization. Nat Rev Urol (2012) 9(10):550–60. doi: 10.1038/nrurol.2012.167
42. Fujihara Y, Okabe M, Ikawa M. GPI-Anchored Protein Complex, LY6K/TEX101, is Required for Sperm Migration Into the Oviduct and Male Fertility in Mice. Biol Reprod (2014) 90(3):60. doi: 10.1095/biolreprod.113.112888
43. Schiza CG, Jarv K, Diamandis EP, Drabovich &, A P. An Emerging Role of TEX101 Protein as a Male Infertility Biomarker. EJIFCC (2014) 25(1):9–26.
44. Köhler S, Gargano M, Matentzoglu N, Carmody LC, Lewis-Smith D, Vasilevsky NA, et al. The Human Phenotype Ontology in 2021. Nucleic Acids Res (2021) 49(D1):D1207–17. doi: 10.1093/nar/gkaa1043
45. Regueira M, Artagaveytia SL, Galardo MN, Pellizzari EH, Cigorraga SB, Meroni SB, et al. Novel Molecular Mechanisms Involved in Hormonal Regulation of Lactate Production in Sertoli Cells. Reproduction (2015) 150(4):311–21. doi: 10.1530/REP-15-0093
46. Ghafouri-Fard S, Modarressi MH, Yazarloo F. Expression of Testis-Specific Genes, TEX101 and ODF4, in Chronic Myeloid Leukemia and Evaluation of TEX101 Immunogenicity. Ann Saudi Med (2012) 32(3):256–61. doi: 10.5144/0256-4947.2012.256
47. Yoshitake H, Yokoi H, Ishikawa H, Maruyama M, Endo S, Nojima M, et al. Overexpression of TEX101, a Potential Novel Cancer Marker, in Head and Neck Squamous Cell Carcinoma. Cancer Biomark: Section A Dis Markers (2012) 12(3):141–8. doi: 10.3233/CBM-130302
48. Ito C, Akutsu H, Yao R, Yoshida K, Yamatoya K, Mutoh T, et al. Odf2 Haploinsufficiency Causes a New Type of Decapitated and Decaudated Spermatozoa, Odf2-DDS, in Mice. Sci Rep (2019) 9(1):14249. doi: 10.1038/s41598-019-50516-2
49. Ghandi M, Huang FW, Jané-Valbuena J, Kryukov GV, Lo CC, McDonald ER, et al. Next-Generation Characterization of the Cancer Cell Line Encyclopedia. Nature (2019) 569(7757):503–8. doi: 10.1038/s41586-019-1186-3
50. Shen C-C, Kang Y-H, Yu L, Cui D-D, He Y, Yang J-L, et al. Human Testis-Expressed Sequence 101 is Limitedly Distributed in Germinal Epithelium of Testis and Disappears in Seminoma. Biol Res (2014) 47(1):52. doi: 10.1186/0717-6287-47-52
51. Khan M, Jabeen N, Khan T, Hussain HMJ, Ali A, Khan R, et al. The Evolutionarily Conserved Genes: Tex37, Ccdc73, Prss55 and Nxt2 are Dispensable for Fertility in Mice. Sci Rep (2018) 8(1):4975. doi: 10.1038/s41598-018-23176-x
52. Ghafouri-Fard S, Abbasi A, Moslehi H, Faramarzi N, Taba Taba Vakili S, Mobasheri MB, et al. Elevated Expression Levels of Testis-Specific Genes TEX101 and SPATA19 in Basal Cell Carcinoma and Their Correlation With Clinical and Pathological Features. Br J Dermatol (2010) 162(4):772–9. doi: 10.1111/j.1365-2133.2009.09568.x
53. Mi Y, Shi Z, Li J. Spata19 is Critical for Sperm Mitochondrial Function and Male Fertility. Mol Reprod Dev (2015) 82(11):907–13. doi: 10.1002/mrd.22536
54. Maruyama M, Yoshitake H, Tsukamoto H, Takamori K, Araki Y. Molecular Expression of Ly6k, a Putative Glycosylphosphatidyl-Inositol-Anchored Membrane Protein on the Mouse Testicular Germ Cells. Biochem Biophys Res Commun (2010) 402(1):75–81. doi: 10.1016/j.bbrc.2010.09.117
55. Schiza C, Korbakis D, Jarvi K, Diamandis EP, Drabovich AP. Identification of TEX101-Associated Proteins Through Proteomic Measurement of Human Spermatozoa Homozygous for the Missense Variant Rs35033974. Mol Cell Proteomics MCP (2019) 18(2):338–51. doi: 10.1074/mcp.RA118.001170
56. Xiao N, Kam C, Shen C, Jin W, Wang J, Lee KM, et al. PICK1 Deficiency Causes Male Infertility in Mice by Disrupting Acrosome Formation. J Clin Invest (2009) 119(4):802–12. doi: 10.1172/JCI36230
57. Dianatpour M, Mehdipour P, Nayernia K, Mobasheri M-B, Ghafouri-Fard S, Savad S, et al. Expression of Testis Specific Genes TSGA10, TEX101 and ODF3 in Breast Cancer. Iranian Red Crescent Med J (2012) 14(11):722–6. doi: 10.5812/ircmj.3611
58. Zhao W, Li Z, Ping P, Wang G, Yuan X, Sun F. Outer Dense Fibers Stabilize the Axoneme to Maintain Sperm Motility. J Cell Mol Med (2018) 22(3):1755–68. doi: 10.1111/jcmm.13457
59. Huang W-T, Yang X, He R-Q, Ma J, Hu X-H, Mo W-J, et al. Overexpressed BSG Related to the Progression of Lung Adenocarcinoma With High-Throughput Data-Mining, Immunohistochemistry, In Vitro Validation and in Silico Investigation. Am J Trans Res (2019) 11(8):4835–50.
60. Xiong L, Edwards CK 3rd, Zhou L. The Biological Function and Clinical Utilization of CD147 in Human Diseases: A Review of the Current Scientific Literature. Int J Mol Sci (2014) 15(10):17411–41. doi: 10.3390/ijms151017411
61. Yoshitake H, Yanagida M, Maruyama M, Takamori K, Hasegawa A, Araki Y. Molecular Characterization and Expression of Dipeptidase 3, a Testis-Specific Membrane-Bound Dipeptidase: Complex Formation With TEX101, a Germ-Cell-Specific Antigen in the Mouse Testis. J Reprod Immunol (2011) 90(2):202–13. doi: 10.1016/j.jri.2011.04.010
62. Hamilton E, O’Malley DM, O’Cearbhaill R, Cristea M, Fleming GF, Tariq B, et al. Tamrintamab Pamozirine (SC-003) in Patients With Platinum-Resistant/Refractory Ovarian Cancer: Findings of a Phase 1 Study. Gynecol Oncol (2020) 158(3):640–5. doi: 10.1016/j.ygyno.2020.05.038
63. Staudinger J, Zhou J, Burgess R, Elledge SJ, Olson EN. PICK1: A Perinuclear Binding Protein and Substrate for Protein Kinase C Isolated by the Yeast Two-Hybrid System. J Cell Biol (1995) 128(3):263–71. doi: 10.1083/jcb.128.3.263
64. Cockbill LMR, Murk K, Love S, Hanley JG. Protein Interacting With C Kinase 1 Suppresses Invasion and Anchorage-Independent Growth of Astrocytic Tumor Cells. Mol Biol Cell (2015) 26(25):4552–61. doi: 10.1091/mbc.E15-05-0270
65. Dai Y, Ren D, Yang Q, Cui Y, Guo W, Lai Y, et al. The TGF-β Signalling Negative Regulator PICK1 Represses Prostate Cancer Metastasis to Bone. Br J Cancer (2017) 117(5):685–94. doi: 10.1038/bjc.2017.212
66. Saeki N, Gu J, Yoshida T, Wu X. Prostate Stem Cell Antigen: A Jekyll and Hyde Molecule? Clinical Cancer Research:. Off J Am Assoc Cancer Res (2010) 16(14):3533–8. doi: 10.1158/1078-0432.CCR-09-3169
67. Lian B, Li H, Liu Y, Chai D, Gao Y, Zhang Y, et al. Expression and Promoter Methylation Status of OPCML and Its Functions in the Inhibition of Cell Proliferation, Migration, and Invasion in Breast Cancer. Breast Cancer (Tokyo Japan) (2021) 28(2):448–58. doi: 10.1007/s12282-020-01179-9
68. Singh V, Singh AP, Sharma I, Singh LC, Sharma J, Borthakar BB, et al. Epigenetic Deregulations of Wnt/β-Catenin and Transforming Growth Factor Beta-Smad Pathways in Esophageal Cancer: Outcome of DNA Methylation. J Cancer Res Ther (2019) 15(1):192–203. doi: 10.4103/jcrt.JCRT_634_17
69. Hooper JD, Nicol DL, Dickinson JL, Eyre HJ, Scarman AL, Normyle JF, et al. Testisin, a New Human Serine Proteinase Expressed by Premeiotic Testicular Germ Cells and Lost in Testicular Germ Cell Tumors. Cancer Res (1999) 59(13):3199LP–205.
70. Hooper JD, Bowen N, Marshall H, Cullen LM, Sood R, Daniels R, et al. Localization, Expression and Genomic Structure of the Gene Encoding the Human Serine Protease Testisin the Novel Sequence Data From This Article Have Been Deposited With the DDBJ/Genbank/EMBL Database Under Accession Number AF058301. Biochim Biophys Acta (BBA) - Gene Structure Expression (2000) 1492(1):63–71. doi: 10.1016/S0167-4781(00)00071-3
71. Hagiwara S, Sasaki E, Hasegawa Y, Suzuki H, Nishikawa D, Beppu S, et al. Serum CD109 Levels Reflect the Node Metastasis Status in Head and Neck Squamous Cell Carcinoma. Cancer Med (2021) 10(4):1335–46. doi: 10.1002/cam4.3737
72. Hashimoto M, Ichihara M, Watanabe T, Kawai K, Koshikawa K, Yuasa N, et al. Expression of CD109 in Human Cancer. Oncogene (2004) 23(20):3716–20. doi: 10.1038/sj.onc.1207418
73. Zhang J-M, Hashimoto M, Kawai K, Murakumo Y, Sato T, Ichihara M, et al. CD109 Expression in Squamous Cell Carcinoma of the Uterine Cervix. Pathol Int (2005) 55(4):165–9. doi: 10.1111/j.1440-1827.2005.01807.x
74. Crowgey EL, Soini T, Shah N, Pauniaho S-L, Lahdenne P, Wilson DB, et al. Germline Sequencing Identifies Rare Variants in Finnish Subjects With Familial Germ Cell Tumors. Appl Clin Genet (2020) 13:127–37. doi: 10.2147/TACG.S245093
75. Fawley J, Gourlay DM. Intestinal Alkaline Phosphatase: A Summary of its Role in Clinical Disease. J Surg Res (2016) 202(1):225–34. doi: 10.1016/j.jss.2015.12.008
76. McLachlan RI, Rajpert-De Meyts E, Hoei-Hansen CE, de Kretser DM, Skakkebaek NE. Histological Evaluation of the Human Testis–Approaches to Optimizing the Clinical Value of the Assessment: Mini Review. Hum Reprod (Oxford England) (2007) 22(1):2–16. doi: 10.1093/humrep/del279
77. Peng X, Zeng X, Peng S, Deng D, Zhang J. The Association Risk of Male Subfertility and Testicular Cancer: A Systematic Review. PloS One (2009) 4(5):e5591–1. doi: 10.1371/journal.pone.0005591
78. Guminska A, Oszukowska E, Kuzanski W, Sosnowski M, Wolski JK, Walczak-Jedrzejowska R, et al. Less Advanced Testicular Dysgenesis is Associated by a Higher Prevalence of Germ Cell Neoplasia. Int J Androl (2010) 33(1):e153–62. doi: 10.1111/j.1365-2605.2009.00981.x
79. Tvrda E, Agarwal A, Alkuhaimi N. Male Reproductive Cancers and Infertility: A Mutual Relationship. Int J Mol Sci (2015) 16(4):7230–60. doi: 10.3390/ijms16047230
80. Hanson HA, Anderson RE, Aston KI, Carrell DT, Smith KR, Hotaling JM. Subfertility Increases Risk of Testicular Cancer: Evidence From Population-Based Semen Samples. Fertil Steril (2016) 105(2):322–8.e1. doi: 10.1016/j.fertnstert.2015.10.027
81. Raos D, Krasic J, Masic S, Abramovic I, Coric M, Kruslin B, et al. In Search of TGCT Biomarkers: A Comprehensive In Silico and Histopathological Analysis. Dis Markers (2020) 2020:8841880. doi: 10.1155/2020/8841880
82. Pluta J, Pyle LC, Nead KT, Wilf R, Li M, Mitra N, et al. Identification of 22 Susceptibility Loci Associated With Testicular Germ Cell Tumors. Nat Commun (2021) 12(1):4487. doi: 10.1038/s41467-021-24334-y
83. Pyle LC, Nathanson KL. Genetic Changes Associated With Testicular Cancer Susceptibility. Semin Oncol (2016) 43(5):575–81. doi: 10.1053/j.seminoncol.2016.08.004
84. Paulick MG, Bertozzi CR. The Glycosylphosphatidylinositol Anchor: A Complex Membrane-Anchoring Structure for Proteins. Biochemistry (2008) 47(27):6991–7000. doi: 10.1021/bi8006324
85. Pastan I, Hassan R. Discovery of Mesothelin and Exploiting it as a Target for Immunotherapy. Cancer Res (2014) 74(11):2907–12. doi: 10.1158/0008-5472.CAN-14-0337
86. Deepa N, Pundir CS. An Electrochemical CD59 Targeted Noninvasive Immunosensor Based on Graphene Oxide Nanoparticles Embodied Pencil Graphite for Detection of Lung Cancer. Microchem J (2020) 156:104957. doi: 10.1016/j.microc.2020.104957
87. Katz SC, Burga RA, McCormack E, Wang LJ, Mooring W, Point GR, et al. Phase I Hepatic Immunotherapy for Metastases Study of Intra-Arterial Chimeric Antigen Receptor-Modified T-Cell Therapy for CEA+ Liver Metastases. Clin Cancer Res: Off J Am Assoc Cancer Res (2015) 21(14):3149–59. doi: 10.1158/1078-0432.CCR-14-1421
88. Li B, Lin H, Fan J, Lan J, Zhong Y, Yang Y, et al. CD59 is Overexpressed in Human Lung Cancer and Regulates Apoptosis of Human Lung Cancer Cells. Int J Oncol (2013) 43(3):850–8. doi: 10.3892/ijo.2013.2007
89. Scholler N, Fu N, Yang Y, Ye Z, Goodman GE, Hellström KE, et al. Soluble Member(s) of the Mesothelin/Megakaryocyte Potentiating Factor Family are Detectable in Sera From Patients With Ovarian Carcinoma. Proc Natl Acad Sci (1999) 96(20):11531 LP–36. doi: 10.1073/pnas.96.20.11531
90. Jin H, Yoshitake H, Tsukamoto H, Takahashi M, Mori M, Takizawa T, et al. Molecular Characterization of a Germ-Cell-Specific Antigen, TEX101, From Mouse Testis. Zygote (Cambridge England) (2006) 14(3):201–8. doi: 10.1017/S0967199406003753
91. Ishikawa N, Takano A, Yasui W, Inai K, Nishimura H, Ito H, et al. Cancer-Testis Antigen Lymphocyte Antigen 6 Complex Locus K Is a Serologic Biomarker and a Therapeutic Target for Lung and Esophageal Carcinomas. Cancer Res (2007) 67(24):11601 LP–1. doi: 10.1158/0008-5472.CAN-07-3243
92. Kurosaki A, Hasegawa K, Kato T, Abe K, Hanaoka T, Miyara A, et al. Serum Folate Receptor Alpha as a Biomarker for Ovarian Cancer: Implications for Diagnosis, Prognosis and Predicting Its Local Tumor Expression. Int J Cancer (2016) 138(8):1994–2002. doi: 10.1002/ijc.29937
93. Liu KL, Fan JH, Wu J. Prognostic Role of Circulating Soluble uPAR in Various Cancers: A Systematic Review and Meta-Analysis. Clin Lab (2017) 63(5):871–80. doi: 10.7754/Clin.Lab.2017.170110
94. Gjerstorff MF, Andersen MH, Ditzel HJ. Oncogenic Cancer/Testis Antigens: Prime Candidates for Immunotherapy. Oncotarget (2015) 6(18):15772–87. doi: 10.18632/oncotarget.4694
95. Zhao S, Zhu W, Xue S, Han D. Testicular Defense Systems: Immune Privilege and Innate Immunity. Cell Mol Immunol (2014) 11(5):428–37. doi: 10.1038/cmi.2014.38
96. Yamanishi Y, Miyake K, Iki M, Tsutsui H, Karasuyama H. Recent Advances in Understanding Basophil-Mediated Th2 Immune Responses. Immunol Rev (2017) 278(1):237–45. doi: 10.1111/imr.12548
97. The Human Protein Atlas Project. (n.d.-b). PRSS21 - Human Protein Atlas. Available at: v21.proteinatlas.org/ENSG00000007038-PRSS21 (Accessed February 5, 2022).
98. Olesen IA, Sonne SB, Hoei-Hansen CE, Rajpert-De Meyts E, Skakkebaek NE. Environment, Testicular Dysgenesis and Carcinoma in Situ Testis. Best Practice & Research. Clin Endocrinol Metab (2007) 21(3):462–78. doi: 10.1016/j.beem.2007.04.002
99. Stevens LC, Little CC. Spontaneous Testicular Teratomas in an Inbred Strain of Mice. Proc Natl Acad Sci USA (1954) 40(11):1080–7. doi: 10.1073/pnas.40.11.1080
Keywords: TEX101, interactome, testicular germ cell tumour, male subfertility, male reproductive health
Citation: Burton J, Wojewodzic MW, Rounge TB and Haugen TB (2022) A Role of the TEX101 Interactome in the Common Aetiology Behind Male Subfertility and Testicular Germ Cell Tumor. Front. Oncol. 12:892043. doi: 10.3389/fonc.2022.892043
Received: 08 March 2022; Accepted: 16 May 2022;
Published: 14 June 2022.
Edited by:
Ricardo Leao, University of Coimbra, PortugalReviewed by:
Luís Pedro Rato, Instituto Politécnico da Guarda, PortugalFei Han, Chongqing Medical University, China
Copyright © 2022 Burton, Wojewodzic, Rounge and Haugen. This is an open-access article distributed under the terms of the Creative Commons Attribution License (CC BY). The use, distribution or reproduction in other forums is permitted, provided the original author(s) and the copyright owner(s) are credited and that the original publication in this journal is cited, in accordance with accepted academic practice. No use, distribution or reproduction is permitted which does not comply with these terms.
*Correspondence: Trine B. Haugen, tribha@oslomet.no; Trine B. Rounge, trine.rounge@kreftregisteret.no
†These authors have contributed equally to this work