- 1Innovative Institute of Chinese Medicine and Pharmacy, Shandong University of Traditional Chinese Medicine, Jinan, China
- 2School of Art & Design, Qilu University of Technology (Shandong Academy of Sciences), Jinan, China
Drug resistance, undesirable toxicity and lack of selectivity are the major challenges of conventional cancer therapies, which cause poor clinical outcomes and high mortality in many cancer patients. Development of alternative cancer therapeutics are highly required for the patients who are resistant to the conventional cancer therapies, including radiotherapy and chemotherapy. The success of a new cancer therapy depends on its high specificity to cancer cells and low toxicity to normal cells. Utilization of bacteria has emerged as a promising strategy for cancer treatment. Attenuated or genetically modified bacteria were used to inhibit tumor growth, modulate host immunity, or deliver anti-tumor agents. The bacteria-derived immunotoxins were capable of destructing tumors with high specificity. These bacteria-based strategies for cancer treatment have shown potent anti-tumor effects both in vivo and in vitro, and some of them have proceeded to clinical trials. Pseudomonas aeruginosa, a Gram-negative bacterial pathogen, is one of the common bacteria used in development of bacteria-based cancer therapy, particularly known for the Pseudomonas exotoxin A-based immunotoxins, which have shown remarkable anti-tumor efficacy and specificity. This review concisely summarizes the current knowledge regarding the utilization of P. aeruginosa in cancer treatment, and discusses the challenges and future perspectives of the P. aeruginosa-based therapeutic strategies.
Introduction
Cancer is one of the most dreaded diseases of human, and is the first or second leading cause of death in most countries of the world (1). The hallmark of cancer includes uncontrolled proliferation, resistance to cell death, insensitivity to growth suppressors, sustained angiogenesis, replicative immortality, and abilities of invasion and metastasis (2). The conventional treatment of cancer includes surgery, radiotherapy and chemotherapy, which are well-established and effective in eliminating fast-growing cancer cells (3). However, these conventional cancer therapies have a lot of limitations, including inefficacy in drug-resistant tumors, lack of tumor specificity, undesirable cytotoxicity to normal cells and adverse effects on cancer patients (4). In the past decade, alternative and complementary cancer therapies including nanoparticles, extracellular vesicles for delivering therapeutic agents, gene therapy, targeted therapy, diet therapy, herbal medicine, bacteriotherapy and magnetic hyperthermia have gained a high degree of research attention, and exhibited excellent anti-tumor effects in vitro and in animal models (5, 6). However, most of the therapeutic approaches are currently under preclinical and clinical investigation.
Bacteria-mediated cancer therapy has emerged as a promising approach in cancer treatment, which is capable of overcoming some of the limitations of conventional cancer therapies (7). Many obligate or facultative anaerobic bacterial species including Clostridium sp., Bifidobacterium sp., Salmonella sp., Bacillus sp., Escherichia coli, Listeria monocytogenes and Pseudomonas aeruginosa have been reported to penetrate and replicate in the hypoxic regions of tumors or accumulate in the tumor microenvironment (8, 9). Furthermore, these therapeutic bacteria are able to inhibit tumor growth and metastasis by production of toxins and stimulation of host immune responses (10). In addition, bacteria can be genetically engineered for their accessible genes, and used as vectors to deliver anti-tumor agents or immunomodulatory proteins to tumor sites (11, 12). Importantly, the genetically modified, live attenuated bacteria can be eliminated by antibiotics or triggering and strengthening host immune responses by immunomodulators such as cytokines and host defense peptides after the cancer treatment to prevent unintended infections (13–15).
Pseudomonas aeruginosa is a Gram-negative, aerobic bacteria that is harmless to healthy individuals but causes severe infections in cystic fibrosis patients and immunocompromised individuals (16). Although P. aeruginosa is categorized as an aerobe, it acts as a facultative anaerobe capable of using alternative electron acceptors such as nitrate (NO3−), nitrite (NO2−) and nitrous oxide (N2O) to produce energy under oxygen-limited conditions (17). Live attenuated, inactivated or genetically modified P. aeruginosa have been reported to effectively cause tumor regression in mouse models by inducing cancer cells to undergo programmed cancer cell death (18–21), dampening proliferative signaling (22–24), and activating host anti-tumor responses (25, 26). Furthermore, many P. aeruginosa virulence factors including exotoxin A (ExoA), exoenzyme T (ExoT), azurin, cyclodipeptides, Pa-caspase recruitment domain (Pa-CARD) and rhamnolipids have been found to exert potent cytotoxicity against various cancer cells (27–32). In particular, ExoA is the most toxic virulence factor of P. aeruginosa, and widely applied in construction of immunotoxins for targeted cancer therapy (33). The present review aimed to concisely summarize and discuss the current findings on P. aeruginosa-based cancer therapeutic approaches, including live attenuated or inactivated P. aeruginosa as anti-cancer agents, P. aeruginosa as vaccine vectors for tumor antigen delivery, and P. aeruginosa ExoA-based immunotoxins (Figure 1).
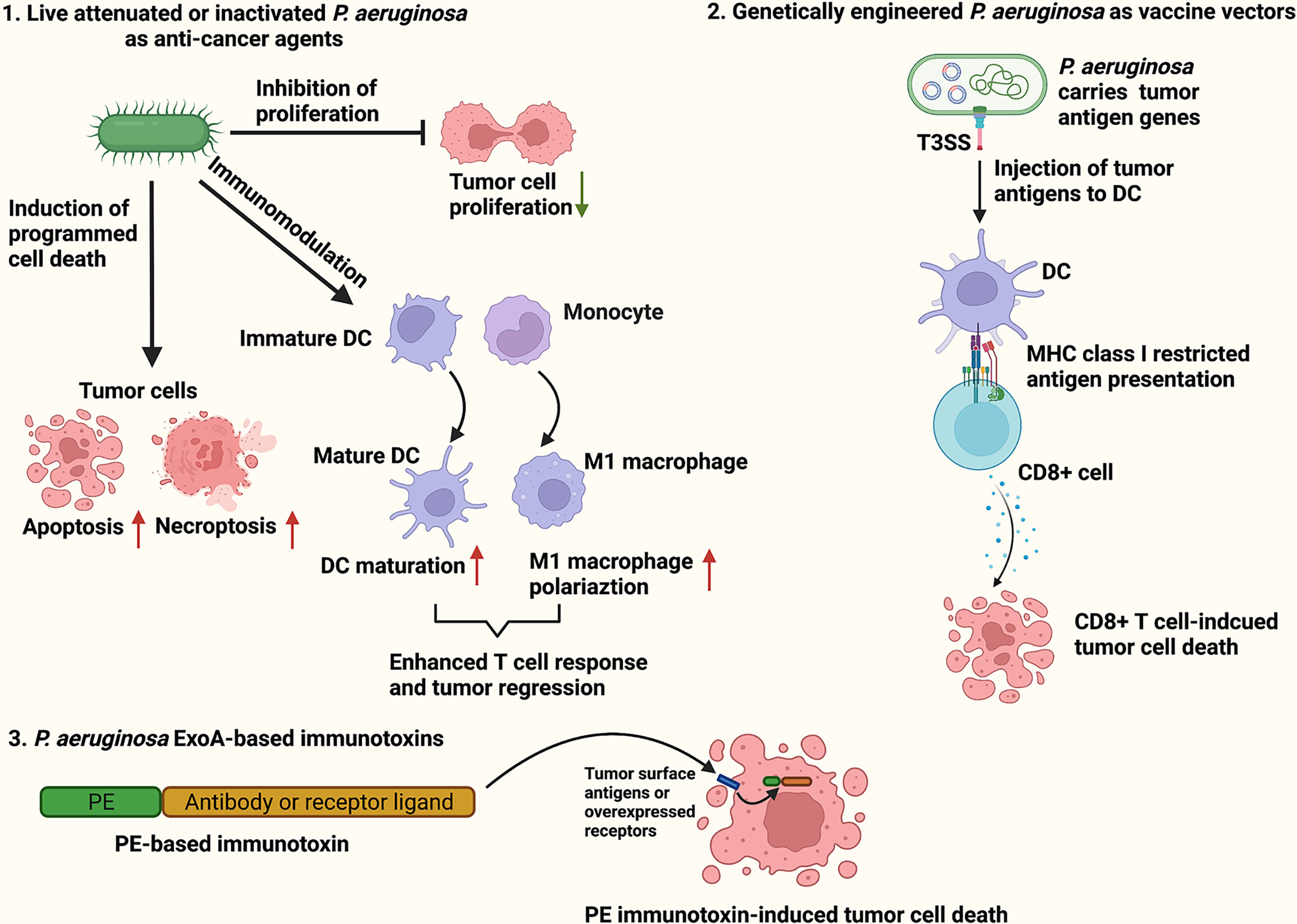
Figure 1 Schematic illustration of P. aeruginosa-based cancer therapies. The P. aeruginosa-based therapeutic strategies for cancer treatment include live attenuated or inactivated P. aeruginosa as anti-cancer agents (1), P. aeruginosa as vaccine vectors for tumor antigen delivery (2), and P. aeruginosa ExoA-based immunotoxins (3).
Live Attenuated or Inactivated P. aeruginosa as Anti-Cancer Agents
The role of bacteria as anti-cancer agents was first identified by German physicians W. Busch and F. Fehleisen who observed tumor regression in the cancer patients suffered from erysipelas caused by Streptococcus pyogenes infection (34). In 1891, William Coley, an American surgeon, inoculated cancer patients with S. pyogenes, which was the first time that bacteria were used to treat cancer (35). Hypoxia is a common feature of solid tumors, which is characterized by insufficient oxygen supply caused by rapid tumor growth. (36). Moreover, the hypoxic tumor microenvironment promotes tumor growth and angiogenesis (37). A number of obligate anaerobes including Clostridium sp. and Bifidobacterium sp. and facultative anaerobes including Salmonella sp., Bacillus sp., E. coli, L. monocytogenes and P. aeruginosa have been reported to colonize and replicate in the hypoxic region of tumors (10). Of note, the bacteria used in cancer treatment are required to be attenuated or genetically modified to reduce their toxicity and the ability to replicate before applying in treatment.
Pseudomonas aeruginosa-mannose sensitive hemagglutinin (PA-MSHA) is a genetically engineered P. aeruginosa strain characterized by high expression of mannose-sensitive hemagglutination (MSHA) fimbriae on its surface, which lowers toxicity by minimizing the exposure of other surface virulence factors such as LPS and flagella (38). Moreover, the MSHA fimbriae has been recognized as a novel ligand of Toll-like receptor 4 (TLR4) (39). Previous studies have shown that PA-MSHA suppressed tumor progression by induction of apoptosis through activating caspase-3, -8 or -9 (18, 19, 40), inhibition of cancer proliferative signaling such as EGFR, NRF2/KEAP1 and hedgehog signaling (22–24), and modulation of host immune responses through enhancing T cell responses, dendritic cell (DC) maturation and M1 macrophage polarization (25, 26, 41). Generally, the live or inactivated PA-MSHA was administered by subcutaneous injection in clinical trials or mouse models, which enhanced host anti-tumor immune responses systemically (19, 26, 42, 43). Moreover, the live PA-MSHA may enter the tumor tissues from blood circulation via passive entrapment in the leaky tumor vasculature or chemotaxis toward the chemicals released by the dying tumor tissue (44, 45). The heat-inactivated PA-MSHA combined with chemotherapy has been applied in clinical trials for treatment of breast cancer, lung cancer and lymphoma in China (42, 43, 46, 47). Most of the clinical studies suggested that the combination of inactivated PA-MSHA and chemotherapy drugs could improve the clinical efficacy of chemotherapy without increasing toxicity to cancer patients. Furthermore, the patients who were more responsive to PA-MSHA stimulation may receive better treatment outcomes. Lv et al. carried out a phase II clinical trial of inactivated PA-MSHA combined with capecitabine for treatment of HER2-negative metastatic breast cancer, and found that the patients with moderate immune-related adverse events (IRAEs) such as fever or skin induration caused by PA-MSHA injection manifested higher survival (25.4 months vs. 16.4 months) and longer progression-free survival (8.2 months vs. 3.1 months) compared to the patients who had no or mild IRAEs (43). One of the challenges for P. aeruginosa-based cancer treatment is that P. aeruginosa is able to induce a self-degradative and recycling process termed autophagy (48–50), which increases the resistance of cancer cells to chemotherapy and radiotherapy (51, 52). Xu et al. identified that PA-MSHA induced autophagy in human breast cancer cells through upregulation the endoplasmic reticulum (ER) stress-activated IRE1 signaling, and treatment of an autophagy inhibitor 3-methyladenosine (3-MA) enhanced the PA-MSHA-induced apoptosis of breast cancer cells in vitro and tumor regression in vivo (53). This study suggests that inhibition of autophagy can increase the effectiveness of P. aeruginosa-induced tumor regression.
In addition to PA-MSHA, the anti-tumor effects of a clinical isolate of P. aeruginosa strain 1409 were examined in vitro and in vivo by Qi et al. (20). The authors demonstrated that P. aeruginosa 1409 induced a programmed necrosis (necroptosis) of TC-1 tumor cells through activation of TLR4-RIP3-MLKL, and the HMGB1 released by the dying tumor cells further induced DC maturation and migration to tumor sites. Subsequently, the mature DC promoted T-cell responses by presenting tumor-associated antigens, thus resulting in remarkable tumor suppression in a TC-1 grafted tumor mouse model (20). This study indicates that the pathogenic clinical strains of P. aeruginosa could induce a potent anti-tumor response by reshaping tumor microenvironment. However, the live pathogenic P. aeruginosa strains must be attenuated or modified to reduce toxicity prior to clinical use due to the weakened immune system of cancer patients, which increases the prevalence of P. aeruginosa infections (54). Furthermore, this bacterial pathogen is resistant to many of the currently available antibiotics such as aminoglycosides, quinolones and β-lactams (55). Thus, clearance of the pathogenic P. aeruginosa strains after treatment is more difficult compared to other therapeutic bacteria.
Pseudomonas aeruginosa as Vaccine Vectors for Tumor Antigen Delivery
Many anaerobic bacteria are recognized as the attractive vectors for the delivery of therapeutic genes to tumors for their ability to internalize and replicate inside tumor cells or grow in the hypoxic tumor microenvironment (56). The therapeutic genes encode anti-tumor agents, cytotoxic peptides, therapeutic molecules or prodrug-converting enzymes (57). The ideal bacterial vectors would be administered systemically, and selectively deliver the therapeutic genes to tumor cells with less toxicity and immunogenicity. Once invading into tumors, the bacteria spread throughout the whole tumor tissues and produce therapeutic agents to inhibit tumor cells (12). Additionally, other than the therapeutic agents, some bacteria capable of surviving in antigen-presenting cells (APCs) or utilizing type III secretion system (T3SS) can be engineered as vaccine vectors that deliver tumor antigens to APCs and induce durable tumor-specific CD8+ T cell responses (58, 59). To date, many bacterial species including Clostridium sporogenes, Salmonella typhimurium, Bifidobacterium longum, E. coli, L. monocytogenes and P. aeruginosa have been genetically modified as vectors for delivery of tumoricidal agents, immunomodulatory proteins or tumor antigens, which showed success in a variety of animal tumor models (60–65).
Gram-negative bacteria utilize T3SS to inject bacterial effectors into host cell cytoplasm (66). In the past decade, the delivery tools based on bacterial T3SS have attracted a lot of research attentions for development of therapeutic cancer vaccines (67). A French research group genetically modified the live attenuated P. aeruginosa strains as vaccine vectors that directly deliver tumor antigens to APCs via T3SS injection and trigger antigen-specific CD8+ T cell responses systemically, leading to long-lasting anti-tumor immune responses (59, 60, 68, 69). For instance, Epaulard et al. generated an attenuated P. aeruginosa strain CHA-OST S54-Ova with deletion of two T3SS toxins, exoenzyme S (ExoS) and ExoT, which were able to induce apoptosis, block production of reactive oxygen species and inhibit the phagocytic activity of host cells (70–73), and this P. aeruginosa strain was genetically modified to express a fusion gene encoding the N-terminal 54 amino acids of ExoS for T3SS-mediated translocation and the C-terminus of ovalbumin (OVA) for immunogenicity (60). Furthermore, the strain CHA-OST S54-Ova was able to elevate the number of OVA-specific CD8+ T cells in vivo, and the mice inoculated with CHA-OST S54-Ova were resistant to the challenge of OVA-expressing mouse melanoma cell line B16 (60). In a separated study, the authors developed another P. aeruginosa strain CHA-OAL by deleting four virulence genes, including exoS, exoT, aroA and lasI, which displayed reduced toxicity and enhanced efficiency for delivering tumor antigens (74). The P. aeruginosa aroA gene encodes an enzyme called 5-enolpyruvylshikimate 3-phosphate synthase, which is essential for synthesis of aromatic amino acids, and deletion of this gene was found to promote the intracellular growth of P. aeruginosa and elicit an increased level of opsonic antibodies in host against P. aeruginosa (75, 76). LasI is an acyl-homoserine lactone synthase that catalyzes the synthesis of N-(3-oxododecanoyl)-L-homoserine lactone (3O-C12-HSL), a quorum sensing signal molecule critical for regulating expression of many P. aeruginosa virulence factors, including ExoA, LasA protease, LasB elastase and alkaline protease (77, 78). Derouazi et al. engineered the P. aeruginosa CHA-OST to express a fusion protein comprising of the N-terminal 54 amino acids of ExoS and a tumor antigen TRP2 epitope, and identified that the TRP2 epitope (125-376) could activate the TRP2-specific CD8+ T cell response, leading to a significant protection of mice against glioma (69). In addition, a killed but metabolically active (KBMA) P. aeruginosa strain OSTAB was created by deletion of ExoS, ExoT and the two subunits of the exonuclease UvrABC, UvrA and UvrB, important for bacterial nucleotide excision repair, and it was subsequently photo-inactivated (79). This KBMA P. aeruginosa strain was incapable of replicating in host but still immunologically active with functional T3SS, which has been suggested to be a promising and safe antigen delivery vector for anti-tumor immunotherapy (68).
P. aeruginosa ExoA-based Immunotoxins for Cancer Treatment
Bacterial toxins in cancer therapy have been extensively studied in the past decade, which effectively change the cellular functions and processes by influencing cell proliferation, differentiation and apoptosis, and eventually kill the tumor cells (57). The theory behinds the bacterial toxin-mediated cancer therapy is creation of chimeric proteins consisting of the catalytic part of a toxin responsible for killing tumor cells and a receptor-binding part such as an antibody or a receptor ligand for specific tumor targeting, and these chimeric proteins are termed as immunotoxins (80). Monoclonal antibodies are commonly used in generation of the tumor cell binding parts in immunotoxin, which bind to the specific molecules that are highly expressed on tumor cell membrane. Upon binding to the target molecules, the immunotoxins are endocytosed and released to host cytosol, ultimately inducing toxin-mediated cell death (81). Moreover, the antigen-binding domain of the antibody part in immunotoxins is usually shortened or modified to reduce immunogenicity (80). The most commonly used bacterial toxins for generation of immunotoxins include Diphtheria toxin and Pseudomonas exotoxin A (PE), which have showed great anti-tumor efficiency both in vivo and in vitro, and some of them are currently under clinical investigation (82, 83).
PE is the most toxic virulence factor in P. aeruginosa which inhibits protein synthesis through ADP-ribosylation of eukaryotic elongation factor 2 (84). It is a single polypeptide chain that can be divided into three functional domains, including receptor binding domain (I), translocation domain (II) and catalytic domain (III) (85). Moreover, the binding of PE receptor binding domain to the low density lipoprotein receptor related protein (LRP1), also known as CD91, on host cell surface mediates uptake of PE via receptor-mediated endocytosis (84). The PE-based immunotoxins were generated by replacing the PE receptor binding domain with the variable fragment (Fv) of a monoclonal antibody or a receptor ligand such as a growth factor or a cytokine, which targets a tumor-specific antigen or a receptor molecule overexpressed on tumor cell surface (86). The representative PE-based immunotoxins discovered and evaluated in the past decade for cancer treatment were summarized in Table 1.
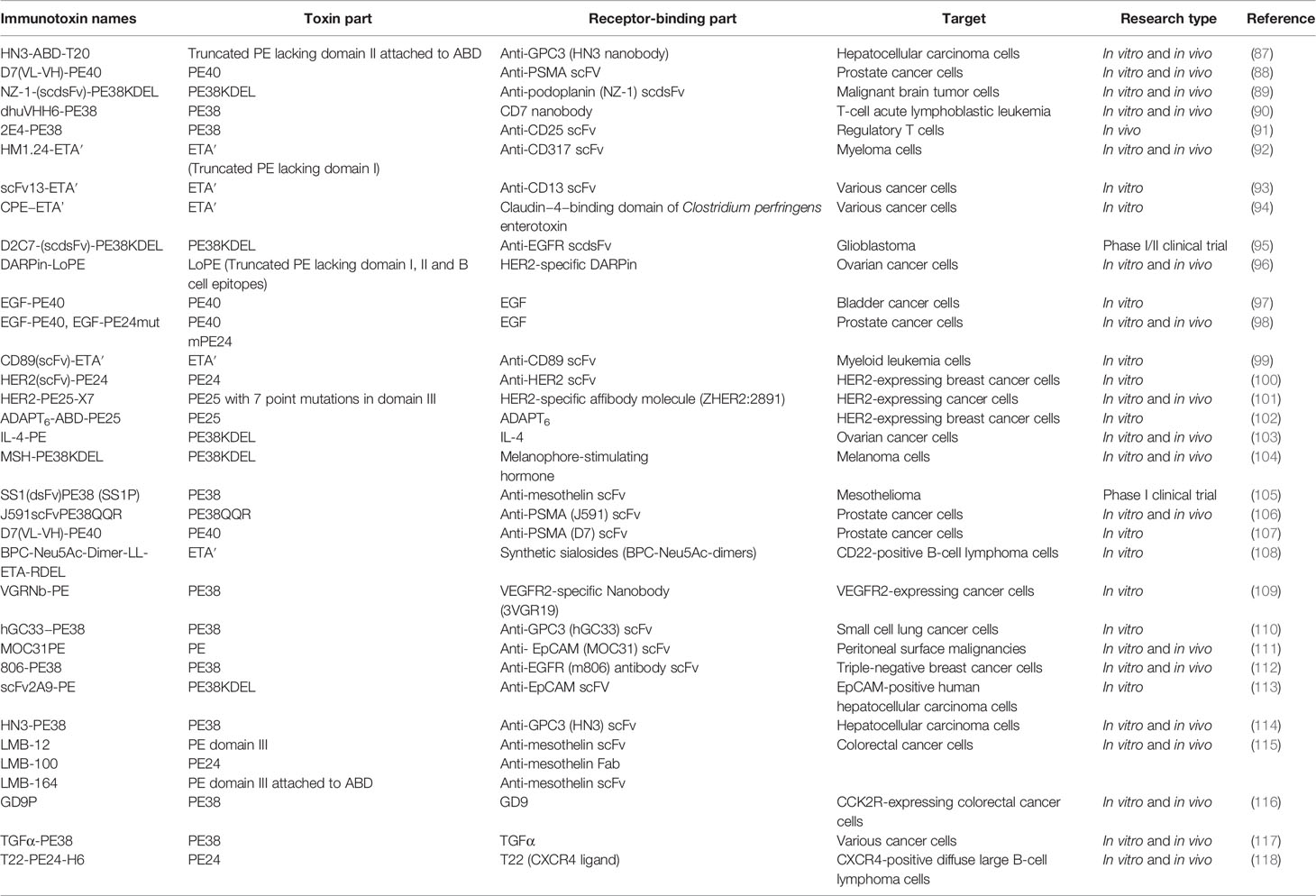
Table 1 A summary of PE-based immunotoxins discovered and evaluated in the past decade for cancer treatment.
The naming of the truncated PE used in construction of the recombinant immunotoxins is usually based on their molecular weight. PE38 and PE40, two truncated forms of PE (38 kDa and 40 kDa, respectively), are most commonly used for immunotoxin construction, and both of them lack the receptor-binding domain (I) (119). Furthermore, as a foreign protein, the immunogenicity of immunotoxins is able to induce production of anti-drug antibodies in host, which neutralize and decrease the efficiency of the immunotoxins (82). Alteration of immunotoxin structure is a feasible strategy to reduce immunogenicity (120). Previous studies have reported that removal of the B cell or T cell epitopes from the PE-based immunotoxins could significantly reduce the immunogenicity and enhanced the anti-tumor efficiency both in vitro and in vivo (121–124). The B cell epitopes in PE38 were mapped by measuring the reactivity of PE38 to the monoclonal antibodies isolated from the mice or patients treated with the PE38-based immunotoxins (125), and they were removed by point mutations of the large hydrophilic amino acids such as arginine, glutamine, glutamic acid and lysine to alanine, serine or glycine, which prevents PE38 from binding to the B cell antigen receptors (124). The T cell epitopes were identified by incubating human peripheral blood mononuclear cells (PBMCs) or mouse splenocytes with whole PE38, and the reacting T-cells were subsequently stimulated with various PE38 peptides (122). Furthermore, the peptides capable of triggering T cell response were determined to contain the T cell epitopes, and the removal of T cell epitopes in PE38 could be achieved by deletion or point mutations (120). To date, many PE-based immunotoxins have been applied in clinical treatment of B-cell lymphoma (126), ovarian cancer (127), mesothelioma (105), breast cancer (128), esophageal cancer (128), brain cancer (95, 129, 130), and pancreatic adenocarcinoma (131). However, most of them are still in the early stage (phase I or II) of clinical trials, and the preliminary data indicated that toxicity and limited efficacy were the major challenges. In addition, the synergistic antitumor activity of PE-based immunotoxins combined with chemotherapeutic agents has been observed in vitro and in mouse tumor models (132–134). However, the combination of PE-based immunotoxins and chemotherapy lacks the clinical evidence for safe use in cancer patients. Alewine et al. demonstrated that the combination of immunotoxin LMB-100 and nab-paclitaxel could amplify the toxic side effects of LMB-100 (131).
Conclusion and Future Perspectives
Tumor resistance to the conventional cancer therapies such as radiotherapy and chemotherapy is major cause of cancer relapse, and has led to a significant barrier in cancer treatment. The bacteria-based cancer therapy has emerged as a promising alternative or complementary strategy for cancer treatment, which exhibited great anti-tumor effects both in vitro and in animal tumor models. Among the therapeutic bacteria, P. aeruginosa takes advantages of large accessible genome, production of virulence factors with potent anti-tumor activities, and expression of various immunogenic molecules on membrane. The peritrichous P. aeruginosa strain PA-MSHA with MSHA fimbriae and low toxicity has been directly used as a therapeutic agent to destroy tumors by inducing tumor cell apoptosis, inhibiting tumor growth, and activating host immune responses. Moreover, the inactivated PA-MSHA combined with chemotherapy has proceeded to clinical trials. However, the PA-MSAH treatment seems to be ineffective to the patients who were tolerant to PA-MSHA stimulation. Therefore, a pre-test of the tolerance to PA-MSHA on cancer patients is recommended, and the patients with moderate adverse reactions will be proceeded for further treatment. The genetically engineered P. aeruginosa strain is able to activate tumor-specific CD8+ T cells by delivering tumor antigens to DCs, inducing long-lasting anti-tumor immunity. Moreover, for safety concern, the live attenuated P. aeruginosa strains should be unable to replicate, and are easily eliminated after treatment. PE is the most widely used P. aeruginosa toxin for construction of recombinant immunotoxins. Although the PE-based immunotoxins have shown significant in vitro and in vivo anti-tumor effects on nearly all types of tumors. However, only a few of them has proceeded to clinical practice, and the low efficiency and unanticipated toxicity to patients remain a big challenge that must be overcome in clinical applications. In future, development of new PE-based immunotoxins with high specificity and less immunogenicity should be one of the major tasks in bacteria-based cancer therapy, which is challenging but rewarding. Overall, the P. aeruginosa-based cancer therapies are promising strategies for cancer treatment, and they are particularly more effective in combination with conventional cancer therapies.
Author Contributions
ZP contributed to the conceptualization, manuscript writing, supervision and funding acquisition. M-DG contributed to manuscript writing. TT made the figures and edited the manuscript. All the authors read and approved the final manuscript.
Funding
This study was funded by the National Natural Science Foundation of China (Grant No. 82002112).
Conflict of Interest
The authors declare that the research was conducted in the absence of any commercial or financial relationships that could be construed as a potential conflict of interest.
Publisher’s Note
All claims expressed in this article are solely those of the authors and do not necessarily represent those of their affiliated organizations, or those of the publisher, the editors and the reviewers. Any product that may be evaluated in this article, or claim that may be made by its manufacturer, is not guaranteed or endorsed by the publisher.
Acknowledgments
The figures in this study were created with BioRender.com.
Abbreviations
ExoA, exotoxin A; CARD, caspase recruitment domain; PA-MSHA, Pseudomonas aeruginosa-mannose sensitive hemagglutinin; MSHA, mannose-sensitive hemagglutination; TLR4, toll-like receptor 4; IRAEs, immune-related adverse events; ER, endoplasmic reticulum; APC, antigen-presenting cell; T3SS, type III secretion system; ExoS, exoenzyme S; ExoT, exoenzyme T; OVA, ovalbumin; 3O-C12-HSL, N-(3-oxododecanoyl)-L-homoserine lactone; KBMA, killed but metabolically active; LRP1, low density lipoprotein receptor related protein; PE, Pseudomonas exotoxin A; Fv, variable fragment; PBMC, peripheral blood mononuclear cell.
References
1. Sung H, Ferlay J, Siegel RL, Laversanne M, Soerjomataram I, Jemal A, et al. Global Cancer Statistics 2020: GLOBOCAN Estimates of Incidence and Mortality Worldwide for 36 Cancers in 185 Countries. CA Cancer J Clin (2021) 71:209–49. doi: 10.3322/caac.21660
2. Hanahan D, Weinberg RA. Hallmarks of Cancer: The Next Generation. Cell (2011) 144:646–74. doi: 10.1016/j.cell.2011.02.013
3. Urruticoechea A, Alemany R, Balart J, Villanueva A, Vinals F, Capella G. Recent Advances in Cancer Therapy: An Overview. Curr Pharm Des (2010) 16:3–10. doi: 10.2174/138161210789941847
4. Chakraborty S, Rahman T. The Difficulties in Cancer Treatment. Ecancermedicalscience (2012) 6:ed16. doi: 10.3332/ecancer.2012
5. Cassileth BR, Deng G. Complementary and Alternative Therapies for Cancer. Oncologist (2004) 9:80–9. doi: 10.1634/theoncologist.9-1-80
6. Pucci C, Martinelli C, Ciofani G. Innovative Approaches for Cancer Treatment: Current Perspectives and New Challenges. Ecancermedicalscience (2019) 13:961. doi: 10.3332/ecancer.2019.961
7. Sedighi M, Zahedi Bialvaei A, Hamblin MR, Ohadi E, Asadi A, Halajzadeh M, et al. Therapeutic Bacteria to Combat Cancer; Current Advances, Challenges, and Opportunities. Cancer Med (2019) 8:3167–81. doi: 10.1002/cam4.2148
8. Azizian K, Pustokhina I, Ghanavati R, Hamblin MR, Amini A, Kouhsari E. The Potential Use of Theranostic Bacteria in Cancer. J Cell Physiol (2021) 236:4184–94. doi: 10.1002/jcp.30152
9. Sznol M, Lin SL, Bermudes D, Zheng LM, King I. Use of Preferentially Replicating Bacteria for the Treatment of Cancer. J Clin Invest (2000) 105:1027–30. doi: 10.1172/JCI9818
10. Drozdz M, Makuch S, Cieniuch G, Wozniak M, Ziolkowski P. Obligate and Facultative Anaerobic Bacteria in Targeted Cancer Therapy: Current Strategies and Clinical Applications. Life Sci (2020) 261:118296. doi: 10.1016/j.lfs.2020.118296
11. Nallar SC, Xu DQ, Kalvakolanu DV. Bacteria and Genetically Modified Bacteria as Cancer Therapeutics: Current Advances and Challenges. Cytokine (2017) 89:160–72. doi: 10.1016/j.cyto.2016.01.002
12. Sawant SS, Patil SM, Gupta V, Kunda NK. Microbes as Medicines: Harnessing the Power of Bacteria in Advancing Cancer Treatment. Int J Mol Sci (2020) 21:7575. doi: 10.3390/ijms21207575
13. Martell EM, Gonzalez-Garcia M, Standker L, Otero-Gonzalez AJ. Host Defense Peptides as Immunomodulators: The Other Side of the Coin. Peptides (2021) 146:170644. doi: 10.1016/j.peptides.2021.170644
14. Netea MG, Kullberg BJ, van der Meer JW. Proinflammatory Cytokines in the Treatment of Bacterial and Fungal Infections. BioDrugs (2004) 18:9–22. doi: 10.2165/00063030-200418010-00002
15. Rommasi F. Bacterial-Based Methods for Cancer Treatment: What We Know and Where We Are. Oncol Ther (2021). doi: 10.1007/s40487-021-00177-x
16. Faure E, Kwong K, Nguyen D. Pseudomonas Aeruginosa in Chronic Lung Infections: How to Adapt Within the Host? Front Immunol (2018) 9:2416. doi: 10.3389/fimmu.2018.02416
17. Arai H. Regulation and Function of Versatile Aerobic and Anaerobic Respiratory Metabolism in Pseudomonas Aeruginosa. Front Microbiol (2011) 2:103. doi: 10.3389/fmicb.2011.00103
18. Cao Z, Shi L, Li Y, Wang J, Wang D, Wang G, et al. Pseudomonas Aeruginosa: Mannose Sensitive Hemagglutinin Inhibits the Growth of Human Hepatocarcinoma Cells via Mannose-Mediated Apoptosis. Dig Dis Sci (2009) 54:2118–27. doi: 10.1007/s10620-008-0603-5
19. Chang L, Xiao W, Yang Y, Li H, Xia D, Yu G, et al. Pseudomonas Aeruginosa-Mannose-Sensitive Hemagglutinin Inhibits Epidermal Growth Factor Receptor Signaling Pathway Activation and Induces Apoptosis in Bladder Cancer Cells In Vitro and In Vivo. Urol Oncol (2014) 32:36.e11–38. doi: 10.1016/j.urolonc.2013.02.013
20. Qi JL, He JR, Jin SM, Yang X, Bai HM, Liu CB, et al. P. Aeruginosa Mediated Necroptosis in Mouse Tumor Cells Induces Long-Lasting Systemic Antitumor Immunity. Front Oncol (2020) 10:610651. doi: 10.3389/fonc.2020.610651
21. Zhao X, Wu X, Yu W, Cai X, Liu Q, Fu X, et al. PA-MSHA Inhibits Proliferation and Induces Apoptosis in Human Non-Small Cell Lung Cancer Cell Lines With Different Genotypes. Mol Med Rep (2016) 14:5369–76. doi: 10.3892/mmr.2016.5869
22. Liu ZB, Hou YF, Zhu J, Hu DL, Jin W, Ou ZL, et al. Inhibition of EGFR Pathway Signaling and the Metastatic Potential of Breast Cancer Cells by PA-MSHA Mediated by Type 1 Fimbriae via a Mannose-Dependent Manner. Oncogene (2010) 29:2996–3009. doi: 10.1038/onc.2010.70
23. Wei Y, Liu D, Jin X, Gao P, Wang Q, Zhang J, et al. PA-MSHA Inhibits the Growth of Doxorubicin-Resistant MCF-7/ADR Human Breast Cancer Cells by Downregulating Nrf2/p62. Cancer Med (2016) 5:3520–31. doi: 10.1002/cam4.938
24. Xiu D, Cheng M, Zhang W, Ma X, Liu L. Pseudomonas Aeruginosa-Mannose-Sensitive Hemagglutinin Inhibits Chemical-Induced Skin Cancer Through Suppressing Hedgehog Signaling. Exp Biol Med (Maywood) (2020) 245:213–20. doi: 10.1177/1535370219897240
25. Wang C, Hu Z, Zhu Z, Zhang X, Wei Z, Zhang Y, et al. The MSHA Strain of Pseudomonas Aeruginosa (PA-MSHA) Inhibits Gastric Carcinoma Progression by Inducing M1 Macrophage Polarization. Tumour Biol (2016) 37:6913–21. doi: 10.1007/s13277-015-4451-6
26. Zhang M, Luo F, Zhang Y, Wang L, Lin W, Yang M, et al. Pseudomonas Aeruginosa Mannose-Sensitive Hemagglutinin Promotes T-Cell Response via Toll-Like Receptor 4-Mediated Dendritic Cells to Slow Tumor Progression in Mice. J Pharmacol Exp Ther (2014) 349:279–87. doi: 10.1124/jpet.113.212316
27. Bernardes N, Ribeiro AS, Abreu S, Vieira AF, Carreto L, Santos M, et al. High-Throughput Molecular Profiling of a P-Cadherin Overexpressing Breast Cancer Model Reveals New Targets for the Anti-Cancer Bacterial Protein Azurin. Int J Biochem Cell Biol (2014) 50:1–9. doi: 10.1016/j.biocel.2014.01.023
28. Goldufsky J, Wood S, Hajihossainlou B, Rehman T, Majdobeh O, Kaufman HL, et al. Pseudomonas Aeruginosa Exotoxin T Induces Potent Cytotoxicity Against a Variety of Murine and Human Cancer Cell Lines. J Med Microbiol (2015) 64:164–73. doi: 10.1099/jmm.0.000003
29. Kwan JM, Fialho AM, Kundu M, Thomas J, Hong CS, Das Gupta TK, et al. Bacterial Proteins as Potential Drugs in the Treatment of Leukemia. Leuk Res (2009) 33:1392–9. doi: 10.1016/j.leukres.2009.01.024
30. Rahimi K, Lotfabad TB, Jabeen F, Mohammad Ganji S. Cytotoxic Effects of Mono- and Di-Rhamnolipids From Pseudomonas Aeruginosa MR01 on MCF-7 Human Breast Cancer Cells. Colloids Surf B Biointerfaces (2019) 181:943–52. doi: 10.1016/j.colsurfb.2019.06.058
31. Vazquez-Rivera D, Gonzalez O, Guzman-Rodriguez J, Diaz-Perez AL, Ochoa-Zarzosa A, Lopez-Bucio J, et al. Cytotoxicity of Cyclodipeptides From Pseudomonas Aeruginosa PAO1 Leads to Apoptosis in Human Cancer Cell Lines. BioMed Res Int (2015) 2015:197608. doi: 10.1155/2015/197608
32. Wolf P, Elsasser-Beile U. Pseudomonas Exotoxin A: From Virulence Factor to Anti-Cancer Agent. Int J Med Microbiol (2009) 299:161–76. doi: 10.1016/j.ijmm.2008.08.003
33. Weldon JE, Pastan I. A Guide to Taming a Toxin–Recombinant Immunotoxins Constructed From Pseudomonas Exotoxin A for the Treatment of Cancer. FEBS J (2011) 278:4683–700. doi: 10.1111/j.1742-4658.2011.08182.x
34. Trivanovic D, Pavelic K, Persuric Z. Fighting Cancer With Bacteria and Their Toxins. Int J Mol Sci (2021) 22:12980. doi: 10.3390/ijms222312980
35. Coley WB. The Treatment of Malignant Tumors by Repeated Inoculations of Erysipelas. With a Report of Ten Original Cases. 262:1893. Clin Orthop Relat Res (1991), 3–11.
36. Emami Nejad A, Najafgholian S, Rostami A, Sistani A, Shojaeifar S, Esparvarinha M, et al. The Role of Hypoxia in the Tumor Microenvironment and Development of Cancer Stem Cell: A Novel Approach to Developing Treatment. Cancer Cell Int (2021) 21:62. doi: 10.1186/s12935-020-01719-5
37. Wang M, Zhao J, Zhang L, Wei F, Lian Y, Wu Y, et al. Role of Tumor Microenvironment in Tumorigenesis. J Cancer (2017) 8:761–73. doi: 10.7150/jca.17648
38. Mu XY. Success in Establishing the MSHA-Positive Pseudomonas Aeruginosa Fimbrial Strain. Wei Sheng Wu Xue Bao (1986) 26:176–9.
39. Mossman KL, Mian MF, Lauzon NM, Gyles CL, Lichty B, Mackenzie R, et al. Cutting Edge: FimH Adhesin of Type 1 Fimbriae is a Novel TLR4 Ligand. J Immunol (2008) 181:6702–6. doi: 10.4049/jimmunol.181.10.6702
40. Liu J, Duan X. PA-MSHA Induces Apoptosis and Suppresses Metastasis by Tumor Associated Macrophages in Bladder Cancer Cells. Cancer Cell Int (2017) 17:76. doi: 10.1186/s12935-017-0445-3
41. Miao ZF, Zhao TT, Miao F, Wang ZN, Xu YY, Mao XY, et al. The Mannose-Sensitive Hemagglutination Pilus Strain of Pseudomonas Aeruginosa Shift Peritoneal Milky Spot Macrophages Towards an M1 Phenotype to Dampen Peritoneal Dissemination. Tumour Biol (2014) 35:4285–93. doi: 10.1007/s13277-013-1559-4
42. Chang J, Liu Y, Han B, Zhou C, Bai C, Li J. Pseudomonas Aeruginosa Preparation Plus Chemotherapy for Advanced Non-Small-Cell Lung Cancer: A Randomized, Multicenter, Double-Blind Phase III Study. Med Oncol (2015) 32:139. doi: 10.1007/s12032-015-0583-1
43. Lv F, Cao J, Liu Z, Wang Z, Zhang J, Zhang S, et al. Phase II Study of Pseudomonas Aeruginosa-Mannose-Sensitive Hemagglutinin in Combination With Capecitabine for Her-2-Negative Metastatic Breast Cancer Pretreated With Anthracycline and Taxane. PloS One (2015) 10:e0118607. doi: 10.1371/journal.pone.0118607
44. Duong MT, Qin Y, You SH, Min JJ. Bacteria-Cancer Interactions: Bacteria-Based Cancer Therapy. Exp Mol Med (2019) 51:1–15. doi: 10.1038/s12276-019-0297-0
45. Lou X, Chen Z, He Z, Sun M, Sun J. Bacteria-Mediated Synergistic Cancer Therapy: Small Microbiome Has a Big Hope. Nanomicro Lett (2021) 13:37. doi: 10.1007/s40820-020-00560-9
46. Chen WD, Tang ZH, Xu F. Application of PA-MSHA Vaccine Adjuvant Therapy and TAC Scheme for Treatment of Breast Carcinoma. Nan Fang Yi Ke Da Xue Xue Bao (2009) 29:1204–7.
47. Li Z, Hao D, Zhang H, Ren L, Yang Y, Li L, et al. A Clinical Study on PA-MSHA Vaccine Used for Adjuvant Therapy of Lymphoma and Lung Cancer. Hua Xi Yi Ke Da Xue Xue Bao (2000) 31:334–7.
48. Junkins RD, Shen A, Rosen K, McCormick C, Lin TJ. Autophagy Enhances Bacterial Clearance During P. Aeruginosa Lung Infection. PloS One (2013) 8:e72263. doi: 10.1371/journal.pone.0072263
49. Pang Z, Xu Y, Zhu Q. Early Growth Response 1 Suppresses Macrophage Phagocytosis by Inhibiting NRF2 Activation Through Upregulation of Autophagy During Pseudomonas Aeruginosa Infection. Front Cell Infect Microbiol (2021) 11:773665. doi: 10.3389/fcimb.2021.773665
50. Yuan K, Huang C, Fox J, Laturnus D, Carlson E, Zhang B, et al. Autophagy Plays an Essential Role in the Clearance of Pseudomonas Aeruginosa by Alveolar Macrophages. J Cell Sci (2012) 125:507–15. doi: 10.1242/jcs.094573
51. Lee JG, Shin JH, Shim HS, Lee CY, Kim DJ, Kim YS, et al. Autophagy Contributes to the Chemo-Resistance of Non-Small Cell Lung Cancer in Hypoxic Conditions. Respir Res (2015) 16:138. doi: 10.1186/s12931-015-0285-4
52. Li L, Liu WL, Su L, Lu ZC, He XS. The Role of Autophagy in Cancer Radiotherapy. Curr Mol Pharmacol (2020) 13:31–40. doi: 10.2174/1874467212666190809154518
53. Xu WH, Liu ZB, Hou YF, Hong Q, Hu DL, Shao ZM. Inhibition of Autophagy Enhances the Cytotoxic Effect of PA-MSHA in Breast Cancer. BMC Cancer (2014) 14:273. doi: 10.1186/1471-2407-14-273
54. Markou P, Apidianakis Y. Pathogenesis of Intestinal Pseudomonas Aeruginosa Infection in Patients With Cancer. Front Cell Infect Microbiol (2014) 3:115. doi: 10.3389/fcimb.2013.00115
55. Pang Z, Raudonis R, Glick BR, Lin TJ, Cheng Z. Antibiotic Resistance in Pseudomonas Aeruginosa: Mechanisms and Alternative Therapeutic Strategies. Biotechnol Adv (2019) 37:177–92. doi: 10.1016/j.biotechadv.2018.11.013
56. Baban CK, Cronin M, O'Hanlon D, O'Sullivan GC, Tangney M. Bacteria as Vectors for Gene Therapy of Cancer. Bioeng Bugs (2010) 1:385–94. doi: 10.4161/bbug.1.6.13146
57. Patyar S, Joshi R, Byrav DS, Prakash A, Medhi B, Das BK. Bacteria in Cancer Therapy: A Novel Experimental Strategy. J BioMed Sci (2010) 17:21. doi: 10.1186/1423-0127-17-21
58. Paterson Y, Guirnalda PD, Wood LM. Listeria and Salmonella Bacterial Vectors of Tumor-Associated Antigens for Cancer Immunotherapy. Semin Immunol (2010) 22:183–9. doi: 10.1016/j.smim.2010.02.002
59. Wang Y, Gouellec AL, Chaker H, Asrih H, Polack B, Toussaint B. Optimization of Antitumor Immunotherapy Mediated by Type III Secretion System-Based Live Attenuated Bacterial Vectors. J Immunother (2012) 35:223–34. doi: 10.1097/CJI.0b013e31824747e5
60. Epaulard O, Toussaint B, Quenee L, Derouazi M, Bosco N, Villiers C, et al. Anti-Tumor Immunotherapy via Antigen Delivery From a Live Attenuated Genetically Engineered Pseudomonas Aeruginosa Type III Secretion System-Based Vector. Mol Ther (2006) 14:656–61. doi: 10.1016/j.ymthe.2006.06.011
61. He L, Yang H, Liu F, Chen Y, Tang S, Ji W, et al. Escherichia Coli Nissle 1917 Engineered to Express Tum-5 can Restrain Murine Melanoma Growth. Oncotarget (2017) 8:85772–82. doi: 10.18632/oncotarget.20486
62. Kim SH, Castro F, Gonzalez D, Maciag PC, Paterson Y, Gravekamp C. Mage-B Vaccine Delivered by Recombinant Listeria Monocytogenes is Highly Effective Against Breast Cancer Metastases. Br J Cancer (2008) 99:741–9. doi: 10.1038/sj.bjc.6604526
63. Liang K, Liu Q, Li P, Luo H, Wang H, Kong Q. Genetically Engineered Salmonella Typhimurium: Recent Advances in Cancer Therapy. Cancer Lett (2019) 448:168–81. doi: 10.1016/j.canlet.2019.01.037
64. Liu SC, Minton NP, Giaccia AJ, Brown JM. Anticancer Efficacy of Systemically Delivered Anaerobic Bacteria as Gene Therapy Vectors Targeting Tumor Hypoxia/Necrosis. Gene Ther (2002) 9:291–6. doi: 10.1038/sj.gt.3301659
65. Yazawa K, Fujimori M, Amano J, Kano Y, Taniguchi S. Bifidobacterium Longum as a Delivery System for Cancer Gene Therapy: Selective Localization and Growth in Hypoxic Tumors. Cancer Gene Ther (2000) 7:269–74. doi: 10.1038/sj.cgt.7700122
66. Coburn B, Sekirov I, Finlay BB. Type III Secretion Systems and Disease. Clin Microbiol Rev (2007) 20:535–49. doi: 10.1128/CMR.00013-07
67. Bai F, Li Z, Umezawa A, Terada N, Jin S. Bacterial Type III Secretion System as a Protein Delivery Tool for a Broad Range of Biomedical Applications. Biotechnol Adv (2018) 36:482–93. doi: 10.1016/j.biotechadv.2018.01.016
68. Chauchet X, Hannani D, Djebali S, Laurin D, Polack B, Marvel J, et al. Poly-Functional and Long-Lasting Anticancer Immune Response Elicited by a Safe Attenuated Pseudomonas Aeruginosa Vector for Antigens Delivery. Mol Ther Oncolytics (2016) 3:16033. doi: 10.1038/mto.2016.33
69. Derouazi M, Wang Y, Marlu R, Epaulard O, Mayol JF, Pasqual N, et al. Optimal Epitope Composition After Antigen Screening Using a Live Bacterial Delivery Vector: Application to TRP-2. Bioeng Bugs (2010) 1:51–60. doi: 10.4161/bbug.1.1.9482
70. Cowell BA, Chen DY, Frank DW, Vallis AJ, Fleiszig SM. ExoT of Cytotoxic Pseudomonas Aeruginosa Prevents Uptake by Corneal Epithelial Cells. Infect Immun (2000) 68:403–6. doi: 10.1128/IAI.68.1.403-406.2000
71. Rangel SM, Logan LK, Hauser AR. The ADP-Ribosyltransferase Domain of the Effector Protein ExoS Inhibits Phagocytosis of Pseudomonas Aeruginosa During Pneumonia. mBio (2014) 5:e01080-01014. doi: 10.1128/mBio.01080-14
72. Sun Y, Karmakar M, Taylor PR, Rietsch A, Pearlman E. ExoS and ExoT ADP Ribosyltransferase Activities Mediate Pseudomonas Aeruginosa Keratitis by Promoting Neutrophil Apoptosis and Bacterial Survival. J Immunol (2012) 188:1884–95. doi: 10.4049/jimmunol.1102148
73. Vareechon C, Zmina SE, Karmakar M, Pearlman E, Rietsch A. Pseudomonas Aeruginosa Effector ExoS Inhibits ROS Production in Human Neutrophils. Cell Host Microbe (2017) 21:611–618.e615. doi: 10.1016/j.chom.2017.04.001
74. Epaulard O, Derouazi M, Margerit C, Marlu R, Filopon D, Polack B, et al. Optimization of a Type III Secretion System-Based Pseudomonas Aeruginosa Live Vector for Antigen Delivery. Clin Vaccine Immunol (2008) 15:308–13. doi: 10.1128/CVI.00278-07
75. Priebe GP, Brinig MM, Hatano K, Grout M, Coleman FT, Pier GB, et al. Construction and Characterization of a Live, Attenuated aroA Deletion Mutant of Pseudomonas Aeruginosa as a Candidate Intranasal Vaccine. Infect Immun (2002) 70:1507–17. doi: 10.1128/IAI.70.3.1507-1517.2002
76. Priebe GP, Meluleni GJ, Coleman FT, Goldberg JB, Pier GB. Protection Against Fatal Pseudomonas Aeruginosa Pneumonia in Mice After Nasal Immunization With a Live, Attenuated aroA Deletion Mutant. Infect Immun (2003) 71:1453–61. doi: 10.1128/IAI.71.3.1453-1461.2003
77. Jimenez PN, Koch G, Thompson JA, Xavier KB, Cool RH, Quax WJ. The Multiple Signaling Systems Regulating Virulence in Pseudomonas Aeruginosa. Microbiol Mol Biol Rev (2012) 76:46–65. doi: 10.1128/MMBR.05007-11
78. Lee J, Zhang L. The Hierarchy Quorum Sensing Network in Pseudomonas Aeruginosa. Protein Cell (2015) 6:26–41. doi: 10.1007/s13238-014-0100-x
79. Le Gouellec A, Chauchet X, Laurin D, Aspord C, Verove J, Wang Y, et al. A Safe Bacterial Microsyringe for In Vivo Antigen Delivery and Immunotherapy. Mol Ther (2013) 21:1076–86. doi: 10.1038/mt.2013.41
80. Zahaf NI, Schmidt G. Bacterial Toxins for Cancer Therapy. Toxins (Basel) (2017) 9:236. doi: 10.3390/toxins9080236
81. Allahyari H, Heidari S, Ghamgosha M, Saffarian P, Amani J. Immunotoxin: A New Tool for Cancer Therapy. Tumour Biol (2017) 39:1010428317692226. doi: 10.1177/1010428317692226
82. Mazor R, Pastan I. Immunogenicity of Immunotoxins Containing Pseudomonas Exotoxin A: Causes, Consequences, and Mitigation. Front Immunol (2020) 11:1261. doi: 10.3389/fimmu.2020.01261
83. Shafiee F, Aucoin MG, Jahanian-Najafabadi A. Targeted Diphtheria Toxin-Based Therapy: A Review Article. Front Microbiol (2019) 10:2340. doi: 10.3389/fmicb.2019.02340
84. Michalska M, Wolf P. Pseudomonas Exotoxin A: Optimized by Evolution for Effective Killing. Front Microbiol (2015) 6:963. doi: 10.3389/fmicb.2015.00963
85. Siegall CB, Chaudhary VK, FitzGerald DJ, Pastan I. Functional Analysis of Domains II, Ib, and III of Pseudomonas Exotoxin. J Biol Chem (1989) 264:14256–61. doi: 10.1016/S0021-9258(18)71671-2
86. Wu T, Zhu J. Recent Development and Optimization of Pseudomonas Aeruginosa Exotoxin Immunotoxins in Cancer Therapeutic Applications. Int Immunopharmacol (2021) 96:107759. doi: 10.1016/j.intimp.2021.107759
87. Fleming BD, Urban DJ, Hall MD, Longerich T, Greten TF, Pastan I, et al. Engineered Anti-GPC3 Immunotoxin, HN3-ABD-T20, Produces Regression in Mouse Liver Cancer Xenografts Through Prolonged Serum Retention. Hepatology (2020) 71:1696–711. doi: 10.1002/hep.30949
88. Michalska M, Schultze-Seemann S, Bogatyreva L, Hauschke D, Wetterauer U, Wolf P. In Vitro and In Vivo Effects of a Recombinant Anti-PSMA Immunotoxin in Combination With Docetaxel Against Prostate Cancer. Oncotarget (2016) 7:22531–42. doi: 10.18632/oncotarget.8001
89. Chandramohan V, Bao X, Kato Kaneko M, Kato Y, Keir ST, Szafranski SE, et al. Recombinant Anti-Podoplanin (NZ-1) Immunotoxin for the Treatment of Malignant Brain Tumors. Int J Cancer (2013) 132:2339–48. doi: 10.1002/ijc.27919
90. Yu Y, Li J, Zhu X, Tang X, Bao Y, Sun X, et al. Humanized CD7 Nanobody-Based Immunotoxins Exhibit Promising Anti-T-Cell Acute Lymphoblastic Leukemia Potential. Int J Nanomed (2017) 12:1969–83. doi: 10.2147/IJN.S127575
91. Onda M, Kobayashi K, Pastan I. Depletion of Regulatory T Cells in Tumors With an Anti-CD25 Immunotoxin Induces CD8 T Cell-Mediated Systemic Antitumor Immunity. Proc Natl Acad Sci USA (2019) 116:4575–82. doi: 10.1073/pnas.1820388116
92. Staudinger M, Glorius P, Burger R, Kellner C, Klausz K, Gunther A, et al. The Novel Immunotoxin HM1.24-ETA' Induces Apoptosis in Multiple Myeloma Cells. Blood Cancer J (2014) 4:e219. doi: 10.1038/bcj.2014.38
93. Grieger E, Gresch G, Niesen J, Woitok M, Barth S, Fischer R, et al. Efficient Targeting of CD13 on Cancer Cells by the Immunotoxin Scfv13-ETA' and the Bispecific scFv [13xds16]. J Cancer Res Clin Oncol (2017) 143:2159–70. doi: 10.1007/s00432-017-2468-5
94. Hashimi SM, Yu S, Alqurashi N, Ipe DS, Wei MQ. Immunotoxin-Mediated Targeting of Claudin-4 Inhibits the Proliferation of Cancer Cells. Int J Oncol (2013) 42:1911–8. doi: 10.3892/ijo.2013.1881
95. Chandramohan V, Pegram CN, Piao H, Szafranski SE, Kuan CT, Pastan IH, et al. Production and Quality Control Assessment of a GLP-Grade Immunotoxin, D2C7-(scdsFv)-PE38KDEL, for a Phase I/II Clinical Trial. Appl Microbiol Biotechnol (2017) 101:2747–66. doi: 10.1007/s00253-016-8063-x
96. Sokolova EA, Shilova ON, Kiseleva DV, Schulga AA, Balalaeva IV, Deyev SM. HER2-Specific Targeted Toxin DARPin-LoPE: Immunogenicity and Antitumor Effect on Intraperitoneal Ovarian Cancer Xenograft Model. Int J Mol Sci (2019) 20:2399. doi: 10.3390/ijms20102399
97. Masilamani AP, Fischer A, Schultze-Seemann S, Kuckuck I, Wolf I, Dressler FF, et al. Epidermal Growth Factor Based Targeted Toxin for the Treatment of Bladder Cancer. Anticancer Res (2021) 41:3741–6. doi: 10.21873/anticanres.15165
98. Fischer A, Wolf I, Fuchs H, Masilamani AP, Wolf P. Pseudomonas Exotoxin A Based Toxins Targeting Epidermal Growth Factor Receptor for the Treatment of Prostate Cancer. Toxins (Basel) (2020) 12:753. doi: 10.3390/toxins12120753
99. Mladenov R, Hristodorov D, Cremer C, Hein L, Kreutzer F, Stroisch T, et al. The Fc-Alpha Receptor is a New Target Antigen for Immunotherapy of Myeloid Leukemia. Int J Cancer (2015) 137:2729–38. doi: 10.1002/ijc.29628
100. Lee S, Park S, Nguyen MT, Lee E, Kim J, Baek S, et al. A Chemical Conjugate Between HER2-Targeting Antibody Fragment and Pseudomonas Exotoxin A Fragment Demonstrates Cytotoxic Effects on HER2-Expressing Breast Cancer Cells. BMB Rep (2019) 52:496–501. doi: 10.5483/BMBRep.2019.52.8.250
101. Guo R, Cao L, Guo W, Liu H, Xu H, Fang Q, et al. HER2-Targeted Immunotoxins With Low Nonspecific Toxicity and Immunogenicity. Biochem Biophys Res Commun (2016) 475:93–9. doi: 10.1016/j.bbrc.2016.05.044
102. Liu H, Lindbo S, Ding H, Altai M, Garousi J, Orlova A, et al. Potent and Specific Fusion Toxins Consisting of a HER2binding, ABDderived Affinity Protein, Fused to Truncated Versions of Pseudomonas Exotoxin A. Int J Oncol (2019) 55:309–19. doi: 10.3892/ijo.2019.4814
103. Green DS, Husain SR, Johnson CL, Sato Y, Han J, Joshi B, et al. Combination Immunotherapy With IL-4 Pseudomonas Exotoxin and IFN-Alpha and IFN-Gamma Mediate Antitumor Effects In Vitro and in a Mouse Model of Human Ovarian Cancer. Immunotherapy (2019) 11:483–96. doi: 10.2217/imt-2018-0158
104. Hui Q, Ma J, Song J, Liu Z, Ren H, Jiang W, et al. In Vitro and In Vivo Studies of Antitumor Effects of the Recombinant Immunotoxin MSH-PE38KDEL on Melanoma. Neoplasma (2014) 61:392–400. doi: 10.4149/neo_2014_048
105. Hassan R, Sharon E, Thomas A, Zhang J, Ling A, Miettinen M, et al. Phase 1 Study of the Antimesothelin Immunotoxin SS1P in Combination With Pemetrexed and Cisplatin for Front-Line Therapy of Pleural Mesothelioma and Correlation of Tumor Response With Serum Mesothelin, Megakaryocyte Potentiating Factor, and Cancer Antigen 125. Cancer (2014) 120:3311–9. doi: 10.1002/cncr.28875
106. Baiz D, Hassan S, Choi YA, Flores A, Karpova Y, Yancey D, et al. Combination of the PI3K Inhibitor ZSTK474 With a PSMA-Targeted Immunotoxin Accelerates Apoptosis and Regression of Prostate Cancer. Neoplasia (2013) 15:1172–83. doi: 10.1593/neo.13986
107. Noll T, Schultze-Seemann S, Kuckuck I, Michalska M, Wolf P. Synergistic Cytotoxicity of a Prostate Cancer-Specific Immunotoxin in Combination With the BH3 Mimetic ABT-737. Cancer Immunol Immunother (2018) 67:413–22. doi: 10.1007/s00262-017-2097-5
108. Schweizer A, Wohner M, Prescher H, Brossmer R, Nitschke L. Targeting of CD22-Positive B-Cell Lymphoma Cells by Synthetic Divalent Sialic Acid Analogues. Eur J Immunol (2012) 42:2792–802. doi: 10.1002/eji.201242574
109. Behdani M, Zeinali S, Karimipour M, Khanahmad H, Schoonooghe S, Aslemarz A, et al. Development of VEGFR2-Specific Nanobody Pseudomonas Exotoxin A Conjugated to Provide Efficient Inhibition of Tumor Cell Growth. N Biotechnol (2013) 30:205–9. doi: 10.3892/ijo.2019.4814
110. Rodakowska E, Walczak-Drzewiecka A, Borowiec M, Gorzkiewicz M, Grzesik J, Ratajewski M, et al. Recombinant Immunotoxin Targeting GPC3 is Cytotoxic to H446 Small Cell Lung Cancer Cells. Oncol Lett (2021) 21:222. doi: 10.3892/ol.2021.12483
111. Flatmark K, Guldvik IJ, Svensson H, Fleten KG, Florenes VA, Reed W, et al. Immunotoxin Targeting EpCAM Effectively Inhibits Peritoneal Tumor Growth in Experimental Models of Mucinous Peritoneal Surface Malignancies. Int J Cancer (2013) 133:1497–506. doi: 10.1002/ijc.28158
112. Simon N, Antignani A, Sarnovsky R, Hewitt SM, FitzGerald D. Targeting a Cancer-Specific Epitope of the Epidermal Growth Factor Receptor in Triple-Negative Breast Cancer. J Natl Cancer Inst (2016) 108:djw028. doi: 10.1093/jnci/djw028
113. Lv M, Qiu F, Li T, Sun Y, Zhang C, Zhu P, et al. Construction, Expression, and Characterization of a Recombinant Immunotoxin Targeting EpCAM. Mediators Inflammation (2015) 2015:460264. doi: 10.1155/2015/460264
114. Gao W, Tang Z, Zhang YF, Feng M, Qian M, Dimitrov DS, et al. Immunotoxin Targeting Glypican-3 Regresses Liver Cancer via Dual Inhibition of Wnt Signalling and Protein Synthesis. Nat Commun (2015) 6:6536. doi: 10.1038/ncomms7536
115. Cerise A, Bera TK, Liu X, Wei J, Pastan I. Anti-Mesothelin Recombinant Immunotoxin Therapy for Colorectal Cancer. Clin Colorectal Cancer (2019) 18:192–199.e191. doi: 10.1016/j.clcc.2019.06.006
116. Chang J, Liu X, Ren H, Lu S, Li M, Zhang S, et al. Pseudomonas Exotoxin A-Based Immunotherapy Targeting CCK2R-Expressing Colorectal Malignancies: An In Vitro and In Vivo Evaluation. Mol Pharm (2021) 18:2285–97. doi: 10.1021/acs.molpharmaceut.1c00095
117. Lim D, Kim KS, Kim H, Ko KC, Song JJ, Choi JH, et al. Anti-Tumor Activity of an Immunotoxin (TGFalpha-PE38) Delivered by Attenuated Salmonella Typhimurium. Oncotarget (2017) 8:37550–60. doi: 10.18632/oncotarget.17197
118. Falgas A, Pallares V, Serna N, Sanchez-Garcia L, Sierra J, Gallardo A, et al. Selective Delivery of T22-PE24-H6 to CXCR4(+) Diffuse Large B-Cell Lymphoma Cells Leads to Wide Therapeutic Index in a Disseminated Mouse Model. Theranostics (2020) 10:5169–80. doi: 10.7150/thno.43231
119. Havaei SM, Aucoin MG, Jahanian-Najafabadi A. Pseudomonas Exotoxin-Based Immunotoxins: Over Three Decades of Efforts on Targeting Cancer Cells With the Toxin. Front Oncol (2021) 11:781800. doi: 10.3389/fonc.2021.781800
120. Mazor R, King EM, Pastan I. Strategies to Reduce the Immunogenicity of Recombinant Immunotoxins. Am J Pathol (2018) 188:1736–43. doi: 10.1016/j.ajpath.2018.04.016
121. Hu X, Zhang M, Zhang C, Long S, Wang W, Yin W, et al. Removal of B-Cell Epitopes for Decreasing Immunogenicity in Recombinant Immunotoxin Against B-Cell Malignancies. J BUON (2016) 21:1374–8.
122. Mazor R, Crown D, Addissie S, Jang Y, Kaplan G, Pastan I. Elimination of Murine and Human T-Cell Epitopes in Recombinant Immunotoxin Eliminates Neutralizing and Anti-Drug Antibodies In Vivo. Cell Mol Immunol (2017) 14:432–42. doi: 10.1038/cmi.2015.91
123. Mazor R, Kaplan G, Park D, Jang Y, Lee F, Kreitman R, et al. Rational Design of Low Immunogenic Anti CD25 Recombinant Immunotoxin for T Cell Malignancies by Elimination of T Cell Epitopes in PE38. Cell Immunol (2017) 313:59–66. doi: 10.1016/j.cellimm.2017.01.003
124. Onda M, Beers R, Xiang L, Nagata S, Wang QC, Pastan I. An Immunotoxin With Greatly Reduced Immunogenicity by Identification and Removal of B Cell Epitopes. Proc Natl Acad Sci USA (2008) 105:11311–6. doi: 10.1073/pnas.0804851105
125. Onda M, Nagata S, FitzGerald DJ, Beers R, Fisher RJ, Vincent JJ, et al. Characterization of the B Cell Epitopes Associated With a Truncated Form of Pseudomonas Exotoxin (PE38) Used to Make Immunotoxins for the Treatment of Cancer Patients. J Immunol (2006) 177:8822–34. doi: 10.4049/jimmunol.177.12.8822
126. Kreitman RJ, Squires DR, Stetler-Stevenson M, Noel P, FitzGerald DJ, Wilson WH, et al. Phase I Trial of Recombinant Immunotoxin RFB4(dsFv)-PE38 (BL22) in Patients With B-Cell Malignancies. J Clin Oncol (2005) 23:6719–29. doi: 10.1200/JCO.2005.11.437
127. Pai LH, Bookman MA, Ozols RF, Young RC, Smith JW, Longo DL, et al. Clinical Evaluation of Intraperitoneal Pseudomonas Exotoxin Immunoconjugate OVB3-PE in Patients With Ovarian Cancer. J Clin Oncol (1991) 9:2095–103. doi: 10.1200/JCO.1991.9.12.2095
128. Pai-Scherf LH, Villa J, Pearson D, Watson T, Liu E, Willingham MC, et al. Hepatotoxicity in Cancer Patients Receiving Erb-38, a Recombinant Immunotoxin That Targets the Erbb2 Receptor. Clin Cancer Res (1999) 5:2311–5.
129. Rainov NG, Heidecke V. Long Term Survival in a Patient With Recurrent Malignant Glioma Treated With Intratumoral Infusion of an IL4-Targeted Toxin (NBI-3001). J Neurooncol (2004) 66:197–201. doi: 10.1023/B:NEON.0000013478.27604.01
130. Sampson JH, Akabani G, Archer GE, Bigner DD, Berger MS, Friedman AH, et al. Progress Report of a Phase I Study of the Intracerebral Microinfusion of a Recombinant Chimeric Protein Composed of Transforming Growth Factor (TGF)-Alpha and a Mutated Form of the Pseudomonas Exotoxin Termed PE-38 (TP-38) for the Treatment of Malignant Brain Tumors. J Neurooncol (2003) 65:27–35. doi: 10.1023/A:1026290315809
131. Alewine C, Ahmad M, Peer CJ, Hu ZI, Lee MJ, Yuno A, et al. Phase I/II Study of the Mesothelin-Targeted Immunotoxin LMB-100 With Nab-Paclitaxel for Patients With Advanced Pancreatic Adenocarcinoma. Clin Cancer Res (2020) 26:828–36. doi: 10.1158/1078-0432.CCR-19-2586
132. Kolyvas E, Rudloff M, Poruchynsky M, Landsman R, Hollevoet K, Venzon D, et al. Mesothelin-Targeted Immunotoxin RG7787 has Synergistic Anti-Tumor Activity When Combined With Taxanes. Oncotarget (2017) 8:9189–99. doi: 10.18632/oncotarget.13984
133. Liu XF, Xiang L, Zhou Q, Carralot JP, Prunotto M, Niederfellner G, et al. Actinomycin D Enhances Killing of Cancer Cells by Immunotoxin RG7787 Through Activation of the Extrinsic Pathway of Apoptosis. Proc Natl Acad Sci USA (2016) 113:10666–71. doi: 10.1073/pnas.1611481113
Keywords: Pseudomonas aeruginosa, cancer therapy, anti-cancer agent, vector, immunotoxin
Citation: Pang Z, Gu M-D and Tang T (2022) Pseudomonas aeruginosa in Cancer Therapy: Current Knowledge, Challenges and Future Perspectives. Front. Oncol. 12:891187. doi: 10.3389/fonc.2022.891187
Received: 07 March 2022; Accepted: 04 April 2022;
Published: 28 April 2022.
Edited by:
Giulio Fracasso, University of Verona, ItalyReviewed by:
Rodolfo Ippoliti, University of L’Aquila, ItalyAchim Rothe, University Hospital of Cologne, Germany
Copyright © 2022 Pang, Gu and Tang. This is an open-access article distributed under the terms of the Creative Commons Attribution License (CC BY). The use, distribution or reproduction in other forums is permitted, provided the original author(s) and the copyright owner(s) are credited and that the original publication in this journal is cited, in accordance with accepted academic practice. No use, distribution or reproduction is permitted which does not comply with these terms.
*Correspondence: Zheng Pang, NjAyMzAwMjFAc2R1dGNtLmVkdS5jbg==; Tong Tang, dGFuZ3RvbmdAcWx1LmVkdS5jbg==