- 1Department of Radiation Oncology, Kaohsiung Medical University Hospital, Kaohsiung, Taiwan
- 2Department of Radiation Oncology, Faculty of Medicine, College of Medicine, Kaohsiung Medical University, Kaohsiung, Taiwan
- 3Department of Radiation Oncology, Kaohsiung Municipal Ta-Tung Hospital, Kaohsiung Medical University, Kaohsiung, Taiwan
- 4Graduate Institute of Medicine, College of Medicine, Kaohsiung Medical University, Kaohsiung, Taiwan
- 5Division of Colorectal Surgery, Department of Surgery, Kaohsiung Medical University Hospital, Kaohsiung Medical University, Kaohsiung, Taiwan
- 6Department of Surgery, Faculty of Medicine, College of Medicine, Kaohsiung Medical University, Kaohsiung, Taiwan
- 7Graduate Institute of Clinical Medicine, College of Medicine, Kaohsiung Medical University, Kaohsiung, Taiwan
- 8Division of Trauma and Surgical Critical Care, Department of Surgery, Kaohsiung Medical University Hospital, Kaohsiung Medical University, Kaohsiung, Taiwan
- 9Department of Emergency Medicine, Faculty of Post-Baccalaureate Medicine, College of Medicine, Kaohsiung Medical University, Kaohsiung, Taiwan
- 10Graduate Institute of Injury Prevention and Control, College of Public Health, Taipei Medical University, Taipei, Taiwan
- 11Center for Cancer Research, Kaohsiung Medical University, Kaohsiung, Taiwan
- 12Pingtung Hospital, Ministry of Health and Welfare, Pingtung, Taiwan
Colorectal cancer (CRC) is a global public health concern because of its high prevalence and mortality. Although radiotherapy is a key method for treating CRC, radioresistance is an obstacle to radiotherapy use. The molecular mechanisms underlying the radioresistance of CRC remain unclear. Increasing evidence has revealed the multiple regulatory functions of non-coding RNAs (ncRNAs) in numerous malignancies, including CRC. Several ncRNAs have been reported to be involved in the determination of radiosensitivity of CRC cells, and some have excellent potential to be prognostic biomarkers or therapeutic targets in CRC treatment. The present review discusses the biological functions and underlying mechanisms of ncRNAs (primarily lncRNA, miRNA, and circRNA) in the regulation of the radiosensitivity of CRC. We also evaluate studies that examined ncRNAs as biomarkers of response to radiation and as therapeutic targets for enhancing radiosensitivity.
Introduction
Colorectal cancer (CRC) has a high incidence and mortality rate. Changes in dietary habits and lifestyle contribute to the increasing incidence of CRC (1). According to various statistics, CRC is the third leading malignancy and second most common cause of cancer-related deaths worldwide (2, 3). Treatment failure is the principal problem in the management of CRC. Treatment methods for rectal cancer are clinically different from those for colon cancer because of the anatomy and high risk of local recurrence associated with rectal cancer. Patients with rectal cancer account for approximately 40% of patients with CRC, and almost half of all patients with rectal cancer are diagnosed with locally advanced rectal cancer (LARC; stages II and III) (4). Neoadjuvant chemoradiotherapy (CRT) followed by radical resection is the standard of care for patients with LARC because this strategy provides improved locoregional control, disease-free survival (DFS), and sphincter-preserving rates (5, 6). For colon cancer, upfront radical resection is the principal therapy, and adjuvant chemotherapy is dependent on pathological features. However, several studies have indicated that neoadjuvant CRT can result in tumor downstaging and even pathological complete response (pCR), thereby facilitating complete tumor resection for locally advanced colon cancer (7, 8).
Because radiotherapy (RT) is one of the primary treatment approaches for CRC, the efficacy of RT can influence the eradication of cancer cells. However, radioresistance is an obstacle to the treatment of CRC. The targeting of nuclear DNA is the major mechanism that causes radiation injury in irradiated cells. Through the direct or indirect interactions between ionizing radiation (IR) and DNA, IR induces various DNA lesions that cause cellular damage and have the potential to kill cells. IR can interact directly with DNA molecules to damage DNA structures or interact indirectly with water molecules to produce reactive oxygen species (ROS) that then destroy DNA (9). Unpaired double-strand breaks (DSBs) are the most lethal form of DNA damage induced by IR (10). Molecules that can increase the number of DSBs or inhibit DNA repair pathways usually improve radiosensitivity (11). DSBs can lead to a series of DNA damage responses (DDRs), which are involved in the detection of DNA lesions, activation of signal transduction, and promotion of DNA repair; they also play a key role in cellular responses to IR (12). DDRs are triggered by the recognition of DNA damage by ataxia-telangiectasia mutated (ATM) and ataxia-telangiectasia- and RAD3-related (ATR) proteins, which are key mediators of DDRs (13). ATM activates the phosphorylation of p53, which then stabilizes itself and increases its accumulation in a nucleus (14). The activation of p53 results in the arrest of the cell cycle and the subsequent promotion of DNA repair or induction of apoptosis through specific pathways. Cell death or recovery is dependent on the level of DNA damage and p53 alterations (15). Two principal methods are involved in the repair of DSBs, namely homologous recombination (HR) and non-homologous end joining (NHEJ) (16). Radiation-induced DSBs principally activate the intrinsic apoptotic pathway. However, the extrinsic apoptotic pathway is also triggered by specific radiation doses (17). These radiation-associated regulatory pathways can be modulated by non-coding RNAs (ncRNAs), which are key mediators in the regulation of radiosensitivity.
The human genome project indicates that less than 2% of the human genome encodes proteins and that more than 90% of the human genome is transcribed into ncRNAs, which are transcripts that lack the potential to code proteins (18, 19). ncRNAs have numerous types, and can generally be classified into two major categories. Transfer RNAs (tRNAs), ribosomal RNAs (rRNAs), and small nucleolar RNAs (snoRNAs) are classified as housekeeping ncRNAs, whereas circular RNAs (circRNAs), long ncRNAs (lncRNAs), microRNAs (miRNAs), tRNA-derived small RNA (tRFs), and small interfering RNAs (siRNAs) are classified as regulatory ncRNAs (20). LncRNAs contain transcripts with more than 200 nucleotides; they can be transcribed and synthesized like mRNAs, but they do not code proteins (21). By contrast, lncRNAs can regulate biological function through interactions with RNA and proteins. miRNAs are small, single-stranded ncRNAs that are approximately 20 nucleotides in length. By targeting mRNAs or other ncRNAs (e.g., lncRNAs and ciriRNAs), miRNAs can execute translational repression and mediate various biological processes (22). Initially, circRNAs were regarded as a by-product of alternative splicing that did not have a biological function (23). However, increasing evidence is indicating that circRNAs can function as alternative splicing mediators, miRNAs sponges, and parental gene expression regulators (24). Taken together, ncRNAs have multiple biological functions, including gene silencing, mRNA processing, chromatin remodeling, and transcriptional and translational regulation. The most frequently studied ncRNAs that are associated with the regulation of radiosensitivity are lncRNAs, miRNAs, and circRNAs. Figure 1 illustrates the mechanisms of ncRNAs regulating radiosensitivity.
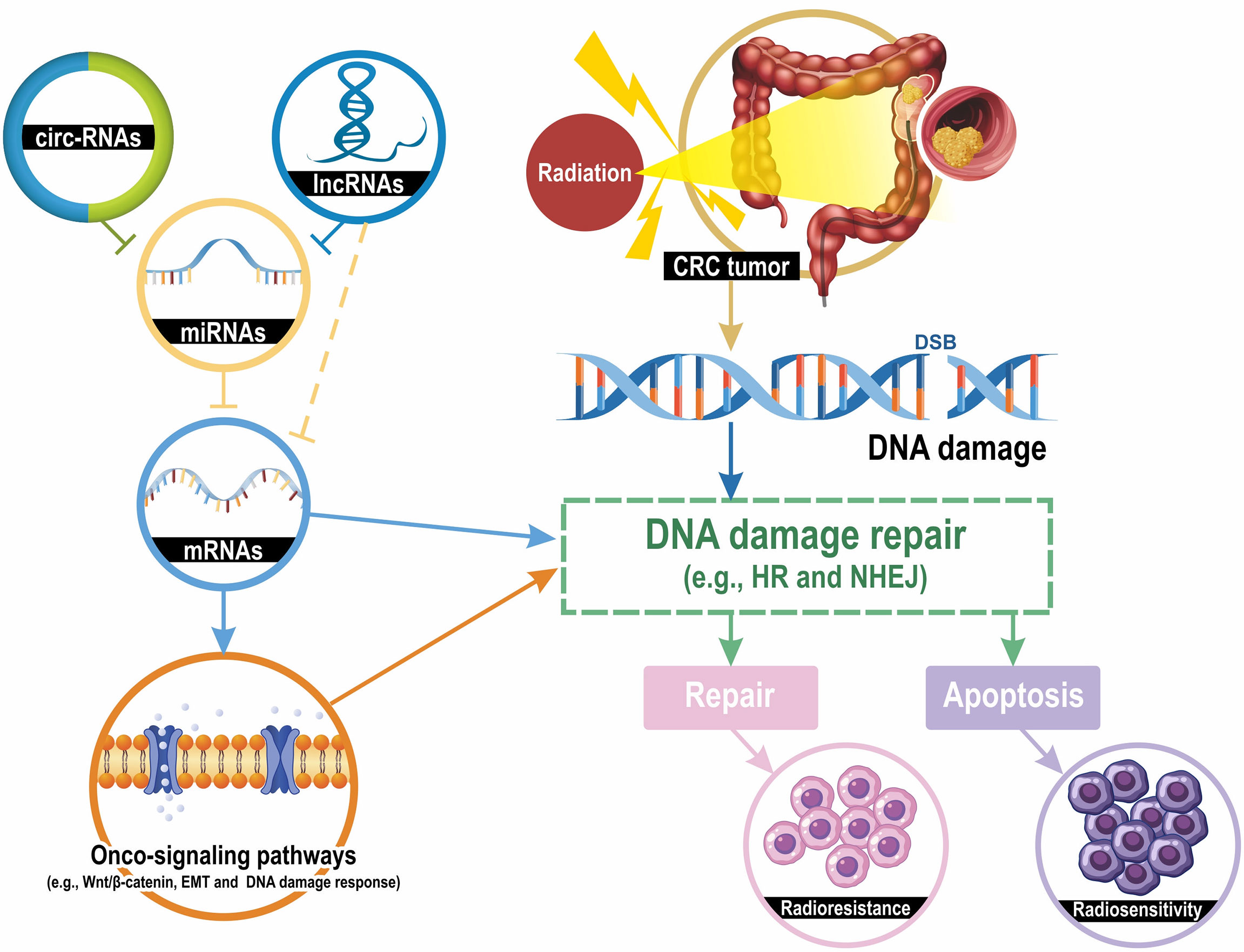
Figure 1 Mechanisms of ncRNAs contributing to CRC cell radiosensitivity. Radiation induces several types of DNA damage, and double-strand breaks (DSBs) are lethal. DNA damage activates the DNA damage repair pathway and the homologous recombination (HR) and the non-homologous end joining (NHEJ) are the most classic mechanisms for DSB repair. DNA damage repair results in two pathways: apoptosis and DNA repair, which lead to radiosensitivity or radioresistance. LncRNAs and circRNAs downregulate the expression levels of miRNA-targeted mRNAs by sponging their targeted miRNAs. mRNAs can directly regulate the DNA damage repair and indirectly modulate repair mechanisms through several onco-signaling pathways, such as Wnt/β-catenin, epithelial-mesenchymal transition (EMT), and DNA damage response. Taken together, the lncRNA-miRNA-mRNA and circRNA-miRNA-mRNA interaction networks modulate the radiosensitivity of CRC cells by regulating DNA damage repair pathways.
Methods
We conducted a search of the databases of PubMed, the Cochrane Central Register of Controlled Trials, and Cochrane Reviews. We did not impose language and regional restrictions and searched the following terms in the format as follows: “non-coding RNA” AND “colorectal cancer “ AND “radiosensitivity” AND “radioresistance” and “miR” AND “colorectal cancer” AND “radiosensitivity” AND”radioresistance.” After searching PubMed with the keywords, we identified 357 relevant journal articles. All relevant studies and bibliographies were thoroughly reviewed to identify additional eligible studies.
LncRNAs and response to radiotherapy—cell line analysis
Long ncRNAs (lncRNA) are characterized as RNAs that have a transcript length of more than 200 nucleotides and function as regulators in various cellular pathways, including DNA damage repair, alteration of cell cycle progression, and induction of resistance to apoptosis. LncRNAs regulate cellular processes by modifying chromatin and transcription, scaffolding for transcriptional factors, modulating mRNA translation, and sponging miRNAs (25). An increasing number of studies have indicated the role of lncRNAs in cancer. Dysregulated lncRNAs have been reported to induce radioresistance in various cancer cells, including CRC cells (26, 27). However, the mechanisms and biological function in CRC have not yet been fully elucidated. Here, we reviewed the literature on the effects of lncRNAs on the regulation of radiosensitivity in CRC. Table 1 displays some lncRNAs that regulate radiosensitivity in CRC, and the targets of the lncRNA and clinical significance.
Yu et al. identified the presence of lnc-TLCD2-1 in radiation-tolerant CCL244 cells and radiation-sensitive HCT116 cells and the presence of correlated lncRNA with radiation resistance. An in vitro study demonstrated that overexpressed lnc-TLCD2-1 induced radioresistance through the regulation of YY1/NF-кB-P65 by directly targeting miR-193a-5p in CRC cells, thus influencing the immune microenvironment of CRC (28). Sun et al. reported that the lncRNA antisense noncoding RNA in the INK4 locus (ANRIL) was upregulated in CRC cells and that the overexpression of ANRIL suppressed radiosensitivity by targeting miR-181a-5p and reversing the functions of chitooligosaccharide, a well-known radiation sensitizer. However, no clinical correlation or in vivo study has validated the effects of ANRIL (29). Pyroptosis (i.e., an inflammatory cell death) is regulated by gasdermins, and it functions as a key mediator in IR-induced damage in normal tissues (37). Su et al. published a study that investigated the role of lncRNA nuclear paraspeckle assembly transcript 1 (NEAT1) in the radiosensitivity of CRC cells. They indicated that lncRNA NEAT1 modulated IR-induced pyroptosis in HCT116 cells through miR−448 inhibition by regulating the expression of gasdermin E, a pyroptosis execution protein (30). Liang et al. reported that lncRNA LINC00958 was upregulated in CRC tissues and cell lines. They demonstrated that LINC00958 inhibited apoptosis and sensitivity to IR in vitro and enhanced tumor growth in vivo. LINC00958 modulated the radiosensitivity of CRC cells by targeting miR-422a, which suppressed MAPK1 expression (26). Li et al. reported that lncRNA eosinophil granule ontogeny transcript (EGOT) was upregulated in rectal cancer tissues and cells. Moreover, EGOT knockdown promoted the radiosensitivity of rectal cancer cells in vitro and in vivo, and EGOT regulated the radiosensitivity of rectal cancer cells by targeting the miR-211-5p/ErbB4 axis (31). Zuo et al. discovered that TTN antisense RNA 1 (TTN-AS1) lncRNA was overexpressed in CRC cells after exposure to IR and that TTN-AS1 knockdown improved the sensitivity of CRC cells to IR and promoted apoptosis by increasing the expression levels of Bax/Bcl2. Their findings suggested that TTN-AS1 lncRNA induced radioresistance by increasing PAK3 expression through the sponging of miR-134-5p and that the p21 and AKT/GSK-3β/β-catenin pathways may be involved in the regulation of radiosensitivity (32). Liu et al. reported that lncRNA homeobox transcript antisense intergenic RNA (HOTAIR) expression was upregulated in CRC tissues and cells and HOTAIR was also highly expressed in the plasma samples from patients with CRC after their RT and CRC cells following IR. HOTAIR knockdown enhanced radiosensitivity in vitro and in vivo through the upregulation of miR-93 and downregulation of ATG12-mediated autophagy (27). Liu et al. reported that the expression of radiation-induced long noncoding RNAs (lnc-RI) was low in radiosensitive CRC cells and that silenced lnc-RI promoted apoptosis caused by cell cycle arrest and induction of DSBs. The experimental results suggested that lnc-RI induced radioresistance by regulating NHEJ repair efficiency through the mediation of the lnc-RI/miR-4727-5p/LIG4 axis (33). Zou et al. investigated the role of lncRNA OIP5 antisense RNA 1 (OIP5-AS1) in modulating the radioresistance of CRC cells and discovered that the expression levels of lncRNA OIP5-AS1 and DYRK1A were low in radioresistant CRC cell lines. They performed functional assays, which verified that lncRNA OIP5-AS1 promoted radiation-induced apoptosis and radiosensitized CRC cells by regulating DYRK1A expression through the sponging of miR-369-3p (34). According to the starBase database (http://starbase.sysu.edu.cn/), LncRNA terminal differentiation-induced noncoding RNA (TINCR) was highly expressed in CRC tissues. Functional assays have verified that the knockdown of TINCR enhanced the radiosensitivity of radioresistant CRC cells and inhibited their stemness by inhibiting TCF4 through the regulation of miR-137 (35). Liu et al. demonstrated that INC00630 was highly expressed in CRC tissues and cells; their functional assays verified that high LINC00630 suppressed BEX1 expression and that the knockdown of LINC00630 promoted the radiosensitivity of CRC cells. Therefore, LINC00630 regulated the radioresistance of CRC cells partially by epigenetically inhibiting BEX1 (36).
LncRNAs and response to radiotherapy—clinical findings
The clinical significance of several lncRNAs has been reported; therefore, they may serve as prognostic markers or therapeutic targets. Lnc-TLCD2-1 was reported to confer radioresistance on CRC cells. Furthermore, the expression levels of lnc-TLCD2-1 were lower in CRC tissues than in normal colon tissues, and patients with CRC who had a high expression of lnc-TLCD2-1 exhibited unfavorable DFS and overall survival (OS) (28). An in vitro study demonstrated that LINC00958 promoted cell proliferation and inhibited apoptosis and sensitivity to radiation. The associations between LINC00958 expression levels and clinicopathological features were analyzed in 63 patients with CRC, and the results revealed that patients with CRC with overexpressed LINC00958 had advanced T-stage, poorly differentiated tumor, and decreased DFS and OS (26). Lin et al. reported that lncRNA EGOT knockdown significantly radiosensitized rectal cancer cells in vivo and in vitro. Among the 40 analyzed patients with rectal cancer, those with upregulated EGOT had poor OS (31). Liu et al. explored the correlations between the levels of LINC00630 in tumor tissues and oncologic outcomes and reported a higher level of LINC00630 expression in the radioresistant cohort than in the radiosensitive cohort. Furthermore, high levels of LINC00630 were significantly associated with shorter OS and recurrence-free survival (36).
MiRNAs and response to radiotherapy—cell line analysis
Radiation is usually combined with chemotherapy preoperatively or postoperatively to reduce locoregional recurrence in CRC. Tumor response to radiation varies considerably, and it involves numerous regulatory pathways (38). miRNAs are one of the key mediators of the radiosensitivity of CRC cells. Table 2 lists several miRNAs that have been shown to be involved in the regulation of radiosensitivity and radioresistance in CRC. Several miRNAs have been identified as biomarkers of radioresistance or radiosensitization. Among radioresistance-related miRNAs, upregulated miR-106b induced radioresistance in vitro and in vivo through the suppression of PTEN/PI3K/AKT pathways and p21 in CRC cells (44). p21 was recognized as a tumor suppressor gene, and it functions as a mediator that can protect cancer cells from DNA damage (53, 54). Wu et al. reported that miR-96-5p induced the radioresistance of rectal cancer cells by inhibiting the GPC3 gene and abnormally triggering the canonical Wnt signaling pathway (43). Liang et al. discovered that the expression of miR-32-5p was upregulated in CRC tissues and that a high level of miR-32-5p was associated with poor oncological outcomes. The suppression of miR-32-5p and the overexpression of TOB1 gene increased the radiosensitivity of CRC cells, and TOB1 was verified to be a direct target gene of miR-32-5p (42).
Several miRNAs are correlated with radiosensitization. Zheng et al. reported that miR-195 sensitized CRC cells (HCT116 and HT29) to radiation by suppressing CARM1 in vitro and in vivo (49). Additionally, they found that the radiosentitizing effects of miR-195 on CRC cells could be reversed by restoring CARM1 expression. The introduction of miR-145 can overcome SNAI1-mediated radioresistance in CRC cells; therefore, miR-145 can be used as a therapeutic agent for targeting cancer stem cells (46). Zhang et al. performed in vitro and in vivo studies and discovered that miR-124 could enhance the radiosensitivity of CRC cells by directly targeting PRRX1, which functioned as an epithelial–mesenchymal transition inducer and stemness mediator (45). miR-185 overexpression suppressed IGF-1R and IGF2 and consequently improved the sensitivity of radioresistant CRC cells to IR (48). IGF‐1R has been associated with cellular resistance to radiation (55, 56). Samadi et al. demonstrated that let‐7e enhanced radiation‐induced apoptosis by directly inhibiting the expression of IGF‐1R in the HCT116 CRC cell line (39). Hu et al. indicated that miR-214 enhanced the radiosensitivity of CRC in vitro and in vivo by inhibiting radiation-induced autophagy and that miR overcame radioresistance by targeting ATG12, which functions as an autophagy-related gene (50). A study reported a higher expression of miR-31 in CRC tissues than in adjacent normal tissues; it also indicated that upregulated miR-31 levels increased the radiosensitivity of CRC cells by inhibiting STK40, which could inhibit the NF-κB signaling pathway (41). Downregulated miR-423-5p levels were identified in acquired radioresistant CRC cells and tissue specimens from patients with radiotherapy-resistant rectal cancer. The overexpression of miR-423-5p sensitized the acquired radioresistant CRC cells by targeting Bcl-xL, which is an antiapoptotic protein (51). Wu et al. discovered that miR-1 was downregulated in CRC tissues and cell lines and that the overexpression of miR-1 enhanced the sensitivity of CRC cells to IR and promoted apoptosis following irradiation (40). Liu et al. discovered that the expression of miR-1587 was lower in CRC tissues and cell lines than in normal colon tissues and cells, and they indicated that miR-1587 overexpression increased the formation of DSBs and radiosensitivity of CRC cells by directly targeting LIG4 (52). Our previous study demonstrated that upregulated miR-148a enhanced radiosensitivity and promoted apoptosis in CRC cells by targeting c-Met in vitro and in vivo (47). A high level of miR-148a was correlated with improved tumor response to neoadjuvant CRT and survival outcomes.
MiRNAs and response to radiotherapy—clinical findings
Because results of the role of miRNAs in the regulation of radiosensitivity in CRC cells have been promising, researchers have evaluated selected miRNAs in patients with CRC to predict RT responses. Differentially expressed miRNA profiling in cancer tissue samples can help identify potential biomarkers of response to RT. miRNA expression profiles were analyzed in tumor tissues collected from 80 rectal cancer patients from a randomized Swedish Rectal Cancer Trial. The decreased expression of miR-302a, miR-105, and miR-888 in the cancer tissues collected from RT and non-RT groups may regulate tumor response to RT in patients with rectal cancer (57). Machackova et al. evaluated tumor biopsy specimens collected from patients with LARC who received neoadjuvant CRT (20 responders and 20 non-responders) and reported that miR-487a-3p expression was significantly higher in the non-responder group than in the responder group (58). Hotchi et al. examined the tumor regression grade in LARC tissue samples and verified that miR-233 and miR-142-3p were associated with treatment response to CRT (59). Kheirelseid et al. reported that miR-16, miR-590-5p, and miR-153 were predictive biomarkers of pCR and that miR-519c-3p and miR-561 were significantly associated with a favorable response to CRT (60).
Although numerous studies have investigated tissue miRNAs to predict response to CRT, their results are highly inconsistent. The methods used to measure response to CRT include comparisons of pCR versus non-pCR or of responders versus non-responders through radiological or pathological downstaging, and this could have contributed to the inconsistencies in reported findings. Another possible explanation is that the studies did not recruit sufficient numbers of patients. Until now, only miR-21 has been identified as a biomarker for predicting pCR (61–63).
Studies have indicated that the stability of circulating miRNAs is excellent; thus, they can perform favorably as minimally invasive biomarkers. The circulating miRNAs obtained from liquid biopsies are promising and accessible predictive markers of response to RT. Guidance from circulating miRNAs can help clinicians in designing tailored therapies. Circulating miRNAs are difficult to measure because of their low concentrations in serum and plasma. However, advances in next-generation sequencing technology have led to the development of techniques for detecting small amounts of miRNAs in blood.
D’Angelo et al. analyzed 38 LARC patients and discovered that miR-125b was highly expressed in both the tissues and serum obtained from non-responders to CRT. They performed a receiver-operating-characteristic curve analysis by examining circulating miR-125b levels and serum carcinoembryonic antigen (CEA) levels and demonstrated that circulating miR-125b performed more favorably than serum CEA in the discrimination of treatment response (64). Hiyoshi et al. collected serum miRNAs from 94 patients with LARC who received neoadjuvant CRT, and they reported a lower expression of serum miR-143 in non-responders than in responders to CRT (65). Yu et al. reported that serum miR-345 expression was significantly lower in responders than in non-responders to CRT in both tissue and serum samples obtained from patients with LARC. In addition, low miR-345 levels were reported to be correlated with improved 3-year local recurrence-free survival (66). Joe et al. analyzed circulating miRNAs from patients with LARC and reported that none of the selected plasma miRNAs was associated with response to CRT. Furthermore, they revealed that the expression levels of circulating microRNAs changed during neoadjuvant CRT; moreover, the expression levels of plasma miRNAs were not always positively correlated with the level of miRNA expression in tumor tissues (67). Similar to the results for cancer tissue miRNAs, the results for circulating miRNAs are considerably inconsistent. Further studies involving the recruitment of large numbers of patients and the application of advanced biotechnology may help reduce this inconsistency.
CircRNAs and response to radiotherapy—cell line analysis
circRNAs are highly stable and exhibit conservative behavior relative to linear RNAs. Increasing evidence has indicated that circRNAs can modulate gene expression at the transcriptional level by regulating the translation or stability of target RNAs through competition with miRNAs (68, 69). circRNAs regulate numerous biological processes, including proliferation, apoptosis, migration, and invasion. Several circRNAs have been discovered to posttranscriptionally modulate gene expression by sponging miRNAs. In the present study, we reviewed studies on the role of circRNAs in regulating the radiosensitivity of CRC. Table 3 summarizes several circRNAs that modulate radiation response in CRC.
Wang et al. identified exosomal circ_0067835 to be upregulated in the serum obtained from patients with CRC who received RT. Circ_0067835 knockdown promoted radiosensitivity in vitro and in vivo by mediating the miR-296-5p/IGF1R axis (72). Liu et al. reported that circ-MFN2 expression was upregulated in CRC tissues and cells and that the knockdown of circ-MFN2 radiosensitized CRC cells in vivo and in vivo. In addition, circ-MFN2 knockdown inhibited cellular proliferation, invasion, and migration. Functionally, circ-MFN2 promoted proliferation and induced the radioresistance of CRC by upregulating miR-574-3p and downregulating IGF1R (73). Exosomal circ_IFT80 was reported to be upregulated in the serum and colon cancer cells of patients with CRC, and the overexpression of circ_IFT80 reduced the radiosensitivity of CRC cells through the inhibition of apoptosis. Exosomal circ_IFT80 could facilitate tumorigenesis and induce radioresistance by regulating the miR-296-5p/MSI1 axis. Furthermore, circ_IFT80 promoted tumor growth in vivo (74). Gao et al. reported that circ_0055625 and musashi homolog 1 (MSI1) were upregulated in CRC tissues and colon cancer cells. Circ_0055625 repression inhibited cell proliferation and promoted radiosensitivity in vitro, and it also suppressed tumor growth and enhanced radiation effects on inhibiting tumor growth in vivo. Circ_0055625 knockdown restrained the progression and radioresistance of CRC cells by downregulating MSI1 through the sponging of miR-338-3p (71). Wang et al. examined CRC tissues and cells and discovered an increase in the expression of circ_0001313 and a decrease in the expression of miR-338-3p. Functionally, circ_0001313 knockdown promoted the radiosensitivity of CRC cells by upregulating miR-338-3p. Moreover, circ_0001313 knockdown increased the caspase-3 activity in CRC cells under irradiation (70). Figure 2 summarizes the relevant signaling pathways of ncRNAs contributing to CRC cell radiosensitivity.
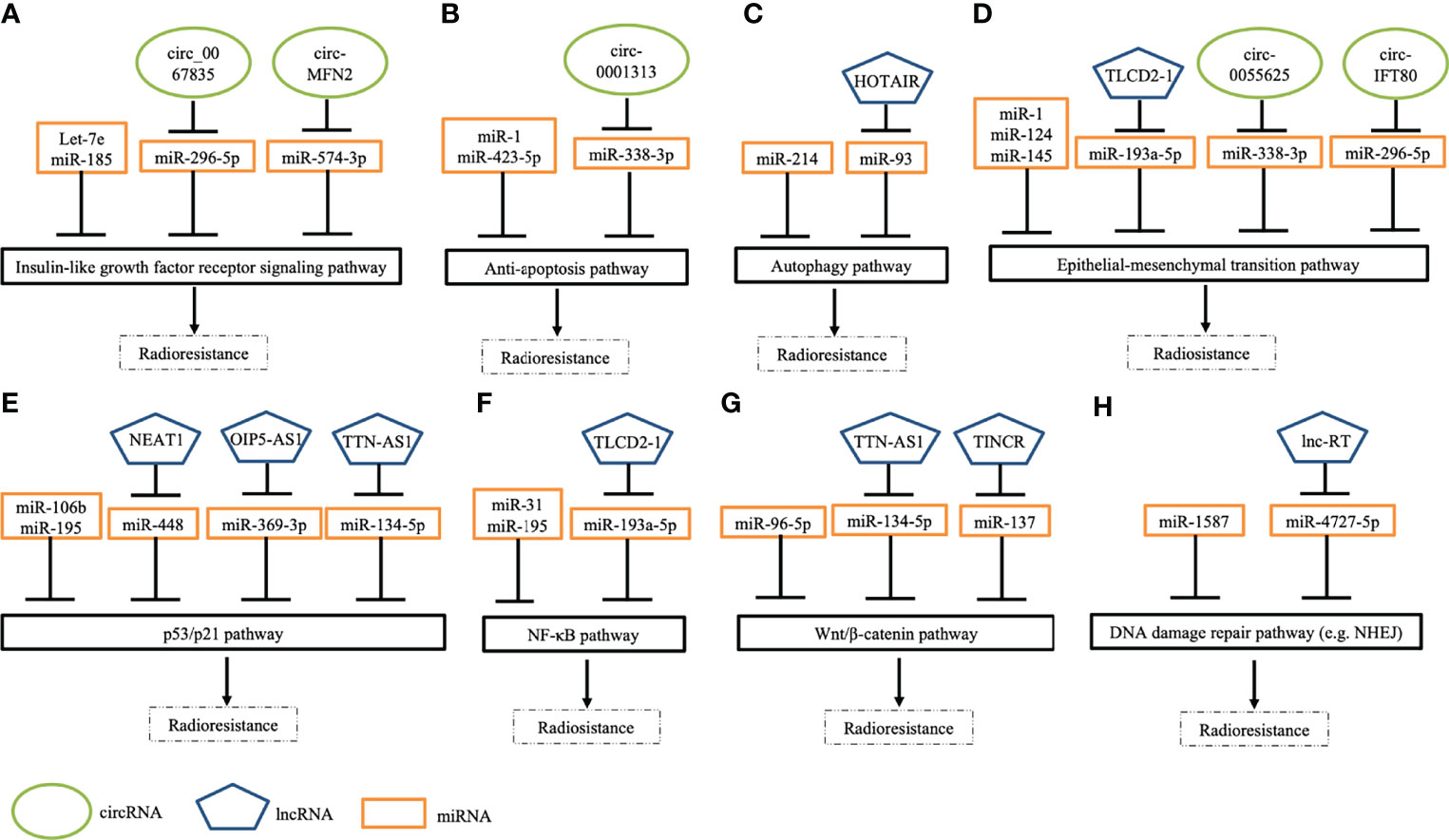
Figure 2 Schematic depicting several pathways of ncRNAs in modulating radiation response to CRC cells. (A) Several ncRNAs regulate radiosensitivity by targeting insulin-like growth factor receptor signaling pathways. (B) A few ncRNAs modulate cell apoptosis by regulating anti-apoptotic pathways. (C) Some ncRNAs modulate response to irradiation by mediating autophagy pathways. (D) Many ncRNAs manipulate epithelial-mesenchymal transition (EMT) by enhancing EMT (e.g., TLCD2-1) or inhibiting EMT (e.g., miR-124). (E) Numerous ncRNAs regulate DNA damage response pathways (e.g., p53 pathways) to modulate radiosensitivity. (F) Specific ncRNAs control NF-κB signaling pathways to overcome radioresistance. (G) Some ncRNAs mediate cellular response to irradiation via targeting Wnt/β-catenin. (H) Several ncRNAs regulate radiosensitivity through mediating DNA damage repair pathways, such as non-homologous end joining (NHEJ).
CircRNAs and response to radiotherapy—clinical findings
Some of the aforementioned circRNAs have clinical significance. Highly expressed circ-MFN2 induced proliferation, metastasis, and radioresistance in CRC cells. In a clinical study, the circ-MFN2 expression levels of 50 patients with CRC were examined, and upregulated circ-MFN2 was revealed to be significantly associated with advanced TNM stage, lymph node metastases, and unfavorable OS (73). Gao et al. discovered that circ_0055625 knockdown inhibited tumorigenesis and enhanced radiosensitivity in CRC cells; they examined 57 patients with CRC and discovered that high circ_0055625 and MSI1 expression levels were associated with a decreased survival rate among such patients (71). The regulatory networks of circRNA-miRNA-mRNA warrant further studies because their role in the regulation of radiosensitivity in CRC remains unclear. Table 4 summarizes the clinical implications of the abovementioned ncRNAs.
Conclusion and future prospects
NcRNAs (primarily lncRNA, miRNA, and circRNA) constitute a complex regulatory network that responds to radiation injury. Through interactions with other ncRNAs, mRNAs, DNA, or proteins, ncRNAs can modulate cellular processes. Our knowledge of ncRNAs is still limited with respect to the variation-related molecular mechanisms for regulating radiosensitivity in patients with CRC. An increasing number of ncRNAs are being classified as oncogenes or tumor suppressor genes, which may modulate the sensitivity of cancer cells to irradiation. Therefore, understanding the underlying mechanism of ncRNAs in tumorigenesis and their regulatory pathways is a key aspect of cancer therapy development. Although ncRNAs have great potential as prognostic biomarkers for guiding precision medicine or as therapeutic targets for improving radiosensitivity in CRC treatments, the use of ncRNAs in clinical practice should be further studied.
With advances in biotechnologies, functional studies of ncRNAs can offer several novel perspectives on cancer treatment. Some ncRNAs have been proved to be associated with tumor stage, treatment efficacy, and prognosis. Several clinical trials have been initiated or are ongoing to investigate the role of ncRNAs in personalized cancer medicine, such as miR-31 (ClinicalTrials.gov Identifier: NCT03362684) and lncRNA CCAT1 (ClinicalTrials.gov Identifer: NCT04269746) in CRC, miR-21 in breast cancer (ClinicalTrials.gov Identifier: NCT05151224), and lncRNA HOTAIR in thyroid cancer (ClinicalTrials.gov Identifer: NCT03469544). The results of these clinical trials are worth looking forward to; moreover, further basic and clinical studies are warranted to investigate the effects of ncRNAs on the regulation of radiosensitivity and to test the clinical applications of ncRNAs in radiotherapy.
Author Contributions
C-MH designed the study and wrote the draft. H-LT, Y-CC, and C-WH collected the related paper. C-CL and W-CS collected the tables and designed them. T-KC, Y-SY, and P-JC assisted in editing. J-YW and M-YH critically reviewed and revised the paper. All authors contributed to the article and approved the submitted version.
Funding
This work was supported by grants through funding from the Ministry of Science and Technology (109-2314-B-037-046-MY3, MOST110-2314-B-037-097, MOST 111-2314-B-037-070-MY3, MOST 111-2314-B-037-049) and the Ministry of Health and Welfare (MOHW111-TDU-B-221-114014) and funded by the health and welfare surcharge of on tobacco products, and the Kaohsiung Medical University Hospital (KMUH110-0R37, KMUH110-0R38, KMUH110-0M34, KMUH110-0M35, KMUH110-0M36, KMUHSA11013, KMUH-DK(C)110010, KMUH-DK(B)110004-3) and KMU Center for Cancer Research (KMU-TC109A04-1) and KMU Office for Industry-Academic Collaboration (S109036), Kaohsiung Medical University. In addition, this study was supported by the Grant of Taiwan Precision Medicine Initiative and Taiwan Biobank, Academia Sinica, Taiwan, R.O.C.
Conflict of Interest
The authors declare that the research was conducted in the absence of any commercial or financial relationships that could be construed as a potential conflict of interest.
Publisher’s Note
All claims expressed in this article are solely those of the authors and do not necessarily represent those of their affiliated organizations, or those of the publisher, the editors and the reviewers. Any product that may be evaluated in this article, or claim that may be made by its manufacturer, is not guaranteed or endorsed by the publisher.
References
1. Keum N, Giovannucci E. Global burden of colorectal cancer: Emerging trends, risk factors and prevention strategies. Nat Rev Gastroenterol Hepatol (2019) 16(12):713–32. doi: 10.1038/s41575-019-0189-8
2. Arnold M, Sierra MS, Laversanne M, Soerjomataram I, Jemal A, Bray F. Global patterns and trends in colorectal cancer incidence and mortality. Gut (2017) 66(4):683–91. doi: 10.1136/gutjnl-2015-310912
3. Schreuders EH, Ruco A, Rabeneck L, Schoen RE, Sung JJ, Young GP, et al. Colorectal cancer screening: A global overview of existing programmes. Gut (2015) 64(10):1637–49. doi: 10.1136/gutjnl-2014-309086
4. Bray F, Ferlay J, Soerjomataram I, Siegel RL, Torre LA, Jemal A. Global cancer statistics 2018: Globocan estimates of incidence and mortality worldwide for 36 cancers in 185 countries. CA: Cancer J Clin (2018) 68(6):394–424. doi: 10.3322/caac.21492
5. van Gijn W, Marijnen CA, Nagtegaal ID, Kranenbarg EM, Putter H, Wiggers T, et al. Preoperative radiotherapy combined with total mesorectal excision for resectable rectal cancer: 12-year follow-up of the multicentre, randomised controlled tme trial. Lancet Oncol (2011) 12(6):575–82. doi: 10.1016/s1470-2045(11)70097-3
6. Sauer R, Becker H, Hohenberger W, Rödel C, Wittekind C, Fietkau R, et al. Preoperative versus postoperative chemoradiotherapy for rectal cancer. N Engl J Med (2004) 351(17):1731–40. doi: 10.1056/NEJMoa040694
7. Huang CM, Huang MY, Ma CJ, Yeh Y, Tsai HL, Huang CW, et al. Neoadjuvant folfox chemotherapy combined with radiotherapy followed by radical resection in patients with locally advanced colon cancer. Radiat Oncol (London England) (2017) 12(1):48. doi: 10.1186/s13014-017-0790-3
8. Chen YC, Tsai HL, Li CC, Huang CW, Chang TK, Su WC, et al. Critical reappraisal of neoadjuvant concurrent chemoradiotherapy for treatment of locally advanced colon cancer. PloS One (2021) 16(11):e0259460. doi: 10.1371/journal.pone.0259460
9. Cook AM, Berry RJ. Direct and indirect effects of radiation: Their relation to growth. Nature (1966) 210(5033):324–5. doi: 10.1038/210324a0
10. Olive PL. The role of DNA single- and double-strand breaks in cell killing by ionizing radiation. Radiat Res (1998) 150(5 Suppl):S42–51. doi: 10.2307/3579807
11. Citrin DE, Mitchell JB. Altering the response to radiation: Sensitizers and protectors. Semin Oncol (2014) 41(6):848–59. doi: 10.1053/j.seminoncol.2014.09.013
12. Goldstein M, Kastan MB. The DNA damage response: Implications for tumor responses to radiation and chemotherapy. Annu Rev Med (2015) 66:129–43. doi: 10.1146/annurev-med-081313-121208
13. Shiloh Y. Atm and related protein kinases: Safeguarding genome integrity. Nat Rev Cancer (2003) 3(3):155–68. doi: 10.1038/nrc1011
14. Enoch T, Norbury C. Cellular responses to DNA damage: Cell-cycle checkpoints, apoptosis and the roles of P53 and atm. Trends Biochem Sci (1995) 20(10):426–30. doi: 10.1016/s0968-0004(00)89093-3
15. Pflaum J, Schlosser S, Müller M. P53 family and cellular stress responses in cancer. Front Oncol (2014) 4:285. doi: 10.3389/fonc.2014.00285
16. Chapman JR, Taylor MR, Boulton SJ. Playing the end game: DNA double-strand break repair pathway choice. Mol Cell (2012) 47(4):497–510. doi: 10.1016/j.molcel.2012.07.029
17. Takasawa R, Nakamura H, Mori T, Tanuma S. Differential apoptotic pathways in human keratinocyte hacat cells exposed to uvb and uvc. Apoptosis Int J Programmed Cell Death (2005) 10(5):1121–30. doi: 10.1007/s10495-005-0901-8
18. Anastasiadou E, Jacob LS, Slack FJ. Non-coding rna networks in cancer. Nat Rev Cancer (2018) 18(1):5–18. doi: 10.1038/nrc.2017.99
19. Wang J, Zhu S, Meng N, He Y, Lu R, Yan GR. Ncrna-encoded peptides or proteins and cancer. Mol Ther J Am Soc Gene Ther (2019) 27(10):1718–25. doi: 10.1016/j.ymthe.2019.09.001
20. Lei B, Tian Z, Fan W, Ni B. Circular rna: A novel biomarker and therapeutic target for human cancers. Int J Med Sci (2019) 16(2):292–301. doi: 10.7150/ijms.28047
21. Statello L, Guo CJ, Chen LL, Huarte M. Author correction: Gene regulation by long non-coding rnas and its biological functions. Nat Rev Mol Cell Biol (2021) 22(2):159. doi: 10.1038/s41580-021-00330-4
22. Chen L, Heikkinen L, Wang C, Yang Y, Sun H, Wong G. Trends in the development of mirna bioinformatics tools. Briefings Bioinf (2019) 20(5):1836–52. doi: 10.1093/bib/bby054
23. Ma Z, Shuai Y, Gao X, Wen X, Ji J. Circular rnas in the tumour microenvironment. Mol Cancer (2020) 19(1):8. doi: 10.1186/s12943-019-1113-0
24. Yin Y, Long J, He Q, Li Y, Liao Y, He P, et al. Emerging roles of circrna in formation and progression of cancer. J Cancer (2019) 10(21):5015–21. doi: 10.7150/jca.30828
25. Gibb EA, Brown CJ, Lam WL. The functional role of long non-coding rna in human carcinomas. Mol Cancer (2011) 10:38. doi: 10.1186/1476-4598-10-38
26. Liang H, Zhao Q, Zhu Z, Zhang C, Zhang H. Long noncoding rna Linc00958 suppresses apoptosis and radiosensitivity of colorectal cancer through targeting mir-422a. Cancer Cell Int (2021) 21(1):477. doi: 10.1186/s12935-021-02188-0
27. Liu Y, Chen X, Chen X, Liu J, Gu H, Fan R, et al. Long non-coding rna hotair knockdown enhances radiosensitivity through regulating microrna-93/Atg12 axis in colorectal cancer. Cell Death Dis (2020) 11(3):175. doi: 10.1038/s41419-020-2268-8
28. Yu Q, Zhang W, Zhou X, Shen W, Xing C, Yang X. Regulation of lnc-Tlcd2-1 on radiation sensitivity of colorectal cancer and comprehensive analysis of its mechanism. Front Oncol (2021) 11:714159. doi: 10.3389/fonc.2021.714159
29. Sun C, Shen C, Zhang Y, Hu C. Lncrna anril negatively regulated chitooligosaccharide-induced radiosensitivity in colon cancer cells by sponging mir-181a-5p. Adv Clin Exp Med Off Organ Wroclaw Med Univ (2021) 30(1):55–65. doi: 10.17219/acem/128370
30. Su F, Duan J, Zhu J, Fu H, Zheng X, Ge C. Long Non−Coding rna nuclear paraspeckle assembly transcript 1 regulates ionizing Radiation−Induced pyroptosis via Microrna−448/Gasdermin e in colorectal cancer cells. Int J Oncol (2021) 59(4):79. doi: 10.3892/ijo.2021.5259
31. Li C, Liu H, Wei R, Liu Z, Chen H, Guan X, et al. Lncrna Egot/Mir-211-5p affected radiosensitivity of rectal cancer by competitively regulating Erbb4. OncoTargets Ther (2021) 14:2867–78. doi: 10.2147/ott.s256989
32. Zuo Z, Ji S, He L, Zhang Y, Peng Z, Han J. Lncrna ttn-As1/Mir-134-5p/Pak3 axis regulates the radiosensitivity of human Large intestine cancer cells through the P21 pathway and Akt/Gsk-3β/β-Catenin pathway. Cell Biol Int (2020) 44(11):2284–92. doi: 10.1002/cbin.11436
33. Liu R, Zhang Q, Shen L, Chen S, He J, Wang D, et al. Long noncoding rna lnc-ri regulates DNA damage repair and radiation sensitivity of crc cells through nhej pathway. Cell Biol Toxicol (2020) 36(5):493–507. doi: 10.1007/s10565-020-09524-6
34. Zou Y, Yao S, Chen X, Liu D, Wang J, Yuan X, et al. Lncrna Oip5-As1 regulates radioresistance by targeting Dyrk1a through mir-369-3p in colorectal cancer cells. Eur J Cell Biol (2018) 97(5):369–78. doi: 10.1016/j.ejcb.2018.04.005
35. Kang Z, Jifu E, Guo K, Ma X, Zhang Y, Yu E. Knockdown of long non-coding rna tincr decreases radioresistance in colorectal cancer cells. Pathol Res Pract (2019) 215(11):152622. doi: 10.1016/j.prp.2019.152622
36. Liu F, Huang W, Hong J, Cai C, Zhang W, Zhang J, et al. Long noncoding rna Linc00630 promotes radio-resistance by regulating Bex1 gene methylation in colorectal cancer cells. IUBMB Life (2020) 72(7):1404–14. doi: 10.1002/iub.2263
37. Lu F, Lan Z, Xin Z, He C, Guo Z, Xia X, et al. Emerging insights into molecular mechanisms underlying pyroptosis and functions of inflammasomes in diseases. J Cell Physiol (2020) 235(4):3207–21. doi: 10.1002/jcp.29268
38. Pajonk F, Vlashi E, McBride WH. Radiation resistance of cancer stem cells: The 4 r's of radiobiology revisited. Stem Cells (Dayton Ohio) (2010) 28(4):639–48. doi: 10.1002/stem.318
39. Samadi P, Afshar S, Amini R, Najafi R, Mahdavinezhad A, Sedighi Pashaki A, et al. Let-7e enhances the radiosensitivity of colorectal cancer cells by directly targeting insulin-like growth factor 1 receptor. J Cell Physiol (2019) 234(7):10718–25. doi: 10.1002/jcp.27742
40. Wu Y, Pu N, Su W, Yang X, Xing C. Downregulation of mir-1 in colorectal cancer promotes radioresistance and aggressive phenotypes. J Cancer (2020) 11(16):4832–40. doi: 10.7150/jca.44753
41. Zhang W, Zhu Y, Zhou Y, Wang J, Jiang P, Xue L. Mirna-31 increases radiosensitivity through targeting Stk40 in colorectal cancer cells. Asia-Pac J Clin Oncol (2022) 18(3):267–78. doi: 10.1111/ajco.13602
42. Liang H, Tang Y, Zhang H, Zhang C. Mir-32-5p regulates radiosensitization, migration and invasion of colorectal cancer cells by targeting Tob1 gene. OncoTargets Ther (2019) 12:9651–61. doi: 10.2147/ott.s228995
43. Wu F, Wu B, Zhang X, Yang C, Zhou C, Ren S, et al. Screening of microrna related to irradiation response and the regulation mechanism of mirna-96-5p in rectal cancer cells. Front Oncol (2021) 11:699475. doi: 10.3389/fonc.2021.699475
44. Zheng L, Zhang Y, Liu Y, Zhou M, Lu Y, Yuan L, et al. Mir-106b induces cell radioresistance Via the Pten/Pi3k/Akt pathways and P21 in colorectal cancer. J Trans Med (2015) 13:252. doi: 10.1186/s12967-015-0592-z
45. Zhang Y, Zheng L, Huang J, Gao F, Lin X, He L, et al. Mir-124 radiosensitizes human colorectal cancer cells by targeting Prrx1. PloS One (2014) 9(4):e93917. doi: 10.1371/journal.pone.0093917
46. Zhu Y, Wang C, Becker SA, Hurst K, Nogueira LM, Findlay VJ, et al. Mir-145 antagonizes Snai1-mediated stemness and radiation resistance in colorectal cancer. Mol Ther J Am Soc Gene Ther (2018) 26(3):744–54. doi: 10.1016/j.ymthe.2017.12.023
47. Huang CM, Huang MY, Chen YC, Chen PJ, Su WC, Chang TK, et al. Mirna-148a enhances the treatment response of patients with rectal cancer to chemoradiation and promotes apoptosis by directly targeting c-met. Biomedicines (2021) 9(10):1371. doi: 10.3390/biomedicines9101371
48. Afshar S, Najafi R, Sedighi Pashaki A, Sharifi M, Nikzad S, Gholami MH, et al. Mir-185 enhances radiosensitivity of colorectal cancer cells by targeting Igf1r and Igf2. Biomed Pharmacother = Biomed Pharmacother (2018) 106:763–9. doi: 10.1016/j.biopha.2018.07.002
49. Zheng L, Chen J, Zhou Z, He Z. Mir-195 enhances the radiosensitivity of colorectal cancer cells by suppressing Carm1. OncoTargets Ther (2017) 10:1027–38. doi: 10.2147/ott.s125067
50. Hu JL, He GY, Lan XL, Zeng ZC, Guan J, Ding Y, et al. Inhibition of Atg12-mediated autophagy by mir-214 enhances radiosensitivity in colorectal cancer. Oncogenesis (2018) 7(2):16. doi: 10.1038/s41389-018-0028-8
51. Shang Y, Wang L, Zhu Z, Gao W, Li D, Zhou Z, et al. Downregulation of mir-423-5p contributes to the radioresistance in colorectal cancer cells. Front Oncol (2020) 10:582239. doi: 10.3389/fonc.2020.582239
52. Liu R, Shen L, Lin C, He J, Wang Q, Qi Z, et al. Mir-1587 regulates DNA damage repair and the radiosensitivity of crc cells via targeting Lig4. Dose-response Publ Int Hormesis Soc (2020) 18(2):1559325820936906. doi: 10.1177/1559325820936906
53. Bene A, Chambers TC. P21 functions in a post-mitotic block checkpoint in the apoptotic response to vinblastine. Biochem Biophys Res Commun (2009) 380(2):211–7. doi: 10.1016/j.bbrc.2009.01.032
54. Gorospe M, Wang X, Guyton KZ, Holbrook NJ. Protective role of P21(Waf1/Cip1) against prostaglandin A2-mediated apoptosis of human colorectal carcinoma cells. Mol Cell Biol (1996) 16(12):6654–60. doi: 10.1128/mcb.16.12.6654
55. Yavari K, Taghikhani M, Maragheh MG, Mesbah-Namin SA, Babaei MH, Arfaee AJ, et al. Sirna-mediated igf-1r inhibition sensitizes human colon cancer Sw480 cells to radiation. Acta Oncol (Stockholm Sweden) (2010) 49(1):70–5. doi: 10.3109/02841860903334429
56. Moreno-Acosta P, Gamboa O, Sanchez de Gomez M, Cendales R, Diaz GD, Romero A, et al. Igf1r gene expression as a predictive marker of response to ionizing radiation for patients with locally advanced Hpv16-positive cervical cancer. Anticancer Res (2012) 32(10):4319–25.
57. Meng WJ, Pathak S, Zhang X, Adell G, Jarlsfelt I, Holmlund B, et al. Expressions of mir-302a, mir-105, and mir-888 play critical roles in pathogenesis, radiotherapy, and prognosis on rectal cancer patients: A study from rectal cancer patients in a Swedish rectal cancer trial of preoperative radiotherapy to big database analyses. Front Oncol (2020) 10:567042. doi: 10.3389/fonc.2020.567042
58. Machackova T, Trachtova K, Prochazka V, Grolich T, Farkasova M, Fiala L, et al. Tumor micrornas identified by small rna sequencing as potential response predictors in locally advanced rectal cancer patients treated with neoadjuvant chemoradiotherapy. Cancer Genomics Proteomics (2020) 17(3):249–57. doi: 10.21873/cgp.20185
59. Hotchi M, Shimada M, Kurita N, Iwata T, Sato H, Morimoto S, et al. Microrna expression is able to predict response to chemoradiotherapy in rectal cancer. Mol Clin Oncol (2013) 1(1):137–42. doi: 10.3892/mco.2012.9
60. Kheirelseid EA, Miller N, Chang KH, Curran C, Hennessey E, Sheehan M, et al. Mirna expressions in rectal cancer as predictors of response to neoadjuvant chemoradiation therapy. Int J Colorectal Dis (2013) 28(2):247–60. doi: 10.1007/s00384-012-1549-9
61. Lopes-Ramos CM, Habr-Gama A, Quevedo Bde S, Felício NM, Bettoni F, Koyama FC, et al. Overexpression of mir-21-5p as a predictive marker for complete tumor regression to neoadjuvant chemoradiotherapy in rectal cancer patients. BMC Med Genomics (2014) 7:68. doi: 10.1186/s12920-014-0068-7
62. Campayo M, Navarro A, Benítez JC, Santasusagna S, Ferrer C, Monzó M, et al. Mir-21, mir-99b and mir-375 combination as predictive response signature for preoperative chemoradiotherapy in rectal cancer. PloS One (2018) 13(11):e0206542. doi: 10.1371/journal.pone.0206542
63. Eriksen AHM, Sørensen FB, Andersen RF, Jakobsen A, Hansen TF. Association between the expression of micrornas and the response of patients with locally advanced rectal cancer to preoperative chemoradiotherapy. Oncol Lett (2017) 14(1):201–9. doi: 10.3892/ol.2017.6141
64. D'Angelo E, Fassan M, Maretto I, Pucciarelli S, Zanon C, Digito M, et al. Serum mir-125b is a non-invasive predictive biomarker of the pre-operative chemoradiotherapy responsiveness in patients with rectal adenocarcinoma. Oncotarget (2016) 7(19):28647–57. doi: 10.18632/oncotarget.8725
65. Hiyoshi Y, Akiyoshi T, Inoue R, Murofushi K, Yamamoto N, Fukunaga Y, et al. Serum mir-143 levels predict the pathological response to neoadjuvant chemoradiotherapy in patients with locally advanced rectal cancer. Oncotarget (2017) 8(45):79201–11. doi: 10.18632/oncotarget.16760
66. Yu J, Li N, Wang X, Ren H, Wang W, Wang S, et al. Circulating serum microrna-345 correlates with unfavorable pathological response to preoperative chemoradiotherapy in locally advanced rectal cancer. Oncotarget (2016) 7(39):64233–43. doi: 10.18632/oncotarget.11649
67. Jo P, Azizian A, Salendo J, Kramer F, Bernhardt M, Wolff HA, et al. Changes of microrna levels in plasma of patients with rectal cancer during chemoradiotherapy. Int J Mol Sci (2017) 18(6):1140. doi: 10.3390/ijms18061140
68. Zhong Y, Du Y, Yang X, Mo Y, Fan C, Xiong F, et al. Circular rnas function as cernas to regulate and control human cancer progression. Mol Cancer (2018) 17(1):79. doi: 10.1186/s12943-018-0827-8
69. Zhang ZJ, Zhang YH, Qin XJ, Wang YX, Fu J. Circular rna Circdennd4c facilitates proliferation, migration and glycolysis of colorectal cancer cells through mir-760/Glut1 axis. Eur Rev Med Pharmacol Sci (2020) 24(5):2387–400. doi: 10.26355/eurrev_202003_20506
70. Wang L, Peng X, Lu X, Wei Q, Chen M, Liu L. Inhibition of Hsa_Circ_0001313 (Circccdc66) induction enhances the radio-sensitivity of colon cancer cells Via tumor suppressor mir-338-3p: Effects of Cicr_0001313 on colon cancer radio-sensitivity. Pathol Res Pract (2019) 215(4):689–96. doi: 10.1016/j.prp.2018.12.032
71. Gao C, Zhang Y, Tian Y, Han C, Wang L, Ding B, et al. Circ_0055625 knockdown inhibits tumorigenesis and improves radiosensitivity by regulating mir-338-3p/Msi1 axis in colon cancer. World J Surg Oncol (2021) 19(1):131. doi: 10.1186/s12957-021-02234-1
72. Wang P, Sun Y, Yang Y, Chen Y, Liu H. Circ_0067835 knockdown enhances the radiosensitivity of colorectal cancer by mir-296-5p/Igf1r axis. OncoTargets Ther (2021) 14:491–502. doi: 10.2147/ott.s281011
73. Liu D, Peng S, Li Y, Guo T. Circ-Mfn2 positively regulates the proliferation, metastasis, and radioresistance of colorectal cancer by regulating the mir-574-3p/Igf1r signaling axis. Front Genet (2021) 12:671337. doi: 10.3389/fgene.2021.671337
Keywords: ncRNAs, colorectal cancer, biomarkers, radiosensitivity, radioresistance
Citation: Huang C-M, Tsai H-L, Chen Y-C, Huang C-W, Li C-C, Su W-C, Chang T-K, Yeh Y-S, Chen P-J, Huang M-Y and Wang J-Y (2022) Role of non-coding RNAs in radiosensitivity of colorectal cancer: A narrative review. Front. Oncol. 12:889658. doi: 10.3389/fonc.2022.889658
Received: 04 March 2022; Accepted: 27 June 2022;
Published: 22 July 2022.
Edited by:
Shuyu Zhang, Sichuan University, ChinaReviewed by:
Arun Renganathan, Washington University in St. Louis, United StatesSrinivas Patnaik, KIIT University, India
Copyright © 2022 Huang, Tsai, Chen, Huang, Li, Su, Chang, Yeh, Chen, Huang and Wang. This is an open-access article distributed under the terms of the Creative Commons Attribution License (CC BY). The use, distribution or reproduction in other forums is permitted, provided the original author(s) and the copyright owner(s) are credited and that the original publication in this journal is cited, in accordance with accepted academic practice. No use, distribution or reproduction is permitted which does not comply with these terms.
*Correspondence: Jaw-Yuan Wang, Y3k2MTQxMTJAbXMxNC5oaW5ldC5uZXQ=; SmF3eXVhbndhbmdAZ21haWwuY29t