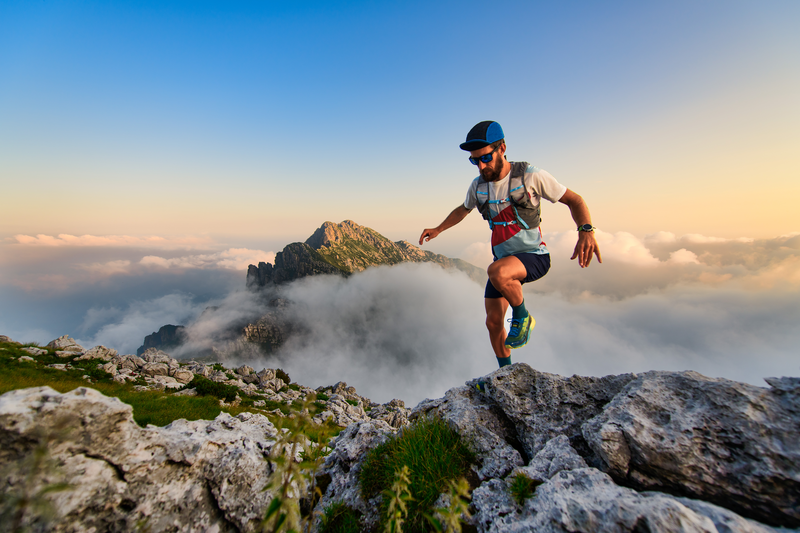
95% of researchers rate our articles as excellent or good
Learn more about the work of our research integrity team to safeguard the quality of each article we publish.
Find out more
METHODS article
Front. Oncol. , 07 July 2022
Sec. Genitourinary Oncology
Volume 12 - 2022 | https://doi.org/10.3389/fonc.2022.888707
This article is part of the Research Topic Advances in Radiotherapy for Prostate Cancer View all 14 articles
Background: Tislelizumab combined with radiotherapy as a salvage treatment for patients with end-stage metastatic castration-resistant prostate cancer (mCRPC) is not reported. This study aimed to describe a protocol to evaluate the safety and efficacy of multisite radiotherapy combined with tislelizumab as a salvage therapy for mCRPC in patients who had at least one second-line treatment failure.
Methods: The study included patients with mCRPC who had at least one lesion suitable for radiotherapy and failed androgen deprivation therapy (ADT), followed by at least one novel second-line endocrine therapy. All patients received tislelizumab monotherapy induction therapy for two cycles, then combined with multisite radiotherapy for one cycle, followed by tislelizumab maintenance therapy, until either disease progressed or the patient developed unacceptable toxicity. Radiation methods and lesions were individually selected according to the specified protocol. Primary endpoints included safety and objective response rate. Secondary endpoints included prostate-specific antigen (PSA) response rate, disease control rate, overall survival, radiographic progression-free survival (rPFS), and biochemical progression-free survival (bPFS). Furthermore, the exploratory endpoints included the identification of the predictive biomarkers and exploration of the correlation between biomarkers and the tumor response to the combined regimen.
Discussion: This study included three treatment stages to evaluate the efficacy of immunotherapy and the combination of immunotherapy and radiotherapy for patients with mCRPC who have had at least second-line treatment failure. Additionally, radiation-related and immune-related early and late toxicities were determined, respectively. Furthermore, the study also aimed to identify the predictive biomarkers associated with immunotherapy for treating mCRPC.
Trial Registration: https://www.chictr.org.cn/showproj.aspx?proj=126359, identifier ChiCTR2100046212.
Prostate cancer (PCa) is the world’s second leading cause of cancer-related mortality in men (1). China has the sixth-highest rate of incidence and mortality due to PCa (2). Androgen deprivation therapy (ADT) is one of the most important therapies for patients with hormone-sensitive PCa. Unfortunately, most patients with PCa eventually develop metastatic castration-resistant prostate cancer (mCRPC) within 2–3 years of undergoing ADT (3, 4). Currently, multiple approved therapies can prolong the survival of patients with mCPRC, including new-generation hormone drugs, such as abiraterone and enzalutamide, and chemotherapeutic drugs, such as docetaxel and cabazitaxel, targeted therapy drugs, and immunotherapy drugs (Sipuleucel-T) (5). However, despite the efficacy of these drugs, cancer cells inevitably develop resistance to them (6). Once patients fail the second-line endocrine therapy, there is a lack of a standard treatment model for subsequent treatments. A clinical trial suggested that a common subset of mCRPC, characterized by defects in DNA repair, could be treated using the poly (ADP-ribose) polymerase (PARP) inhibitor olaparib in patients with mCRPC who had developed resistance to standard treatments (7, 8). However, this subset only accounted for approximately 11.8% of all sporadic mCRPC (2). Most patients could not achieve durable responses with available treatments. Thus, it was a fundamental requirement to identify novel strategies to improve the survival of patients with mCRPC after the failure of second-line endocrine therapy.
The introduction of immunotherapy-targeted programmed death protein 1 (PD-1) and programmed cell death-ligand 1 (PD-L1) has altered the treatment paradigm for various types of malignancies. Unfortunately, immunotherapy has only shown modest efficacy against PCa (9). As per the results of two recently published clinical studies, two anti-PD-1 antibodies, pembrolizumab and atezolizumab were well tolerated and safe in patients with mCRPC. However, complete response (CR) was achieved in only a few patients (10, 11). Tislelizumab, an investigational anti-PD-1 antibody, has been shown to be significantly efficacious in (85.7% objective response rate (ORR)) patients with relapsed/refractory classical Hodgkin’s lymphoma (12). A recently published research demonstrated that tislelizumab showed substantial clinical benefits and an acceptable safety profile in patients with urothelial carcinoma (13). Another study found that tislelizumab had disease stabilization capacity for various tumor types and in patients who had undergone different types of long-term treatments (14). Thus, it was speculated that tislelizumab might act as an effective salvage treatment strategy to improve the outcomes in patients with mCRPC. However, due to the “cold tumor” characteristics of PCa, the response to immunotherapy in PCa might not be as strong compared with other tumors (1). Thus, a combination of immunotherapy and other current treatment strategies, such as chemotherapy, targeted therapy, and radiotherapy (RT), could improve the immune response to “cold tumors.” Previous studies have reported that ipilimumab monotherapy or the addition of atezolizumab to enzalutamide for treating patients with mCRPC could not provide a satisfactory primary endpoint for overall survival (OS) (15, 16). However, using ipilimumab plus RT showed improved outcomes compared with placebo plus RT in patients with postdocetaxel mCRPC (17). Consequently, RT could be a promising strategy for the synergistic enhancement of immunotherapeutic efficacy.
Numerous clinical trials have supported the use of RT in the modification of antitumor immune responses, enhanced expression of antigens on the surface of tumor cells, as well as tumor antigen crosspresentation in the draining lymph nodes, directly resulting in the activation and proliferation of tumor-specific cytotoxic T cells (18–21). Consequently, this might result in a modified tumor microenvironment along with the expansion of immunotherapeutic capacity (22, 23). Multisite stereotactic body radiotherapy (SBRT) has emerged as an altering paradigm for treating solid metastatic tumors (24). A phase I study indicated that multisite SBRT combined with pembrolizumab for solid metastatic tumors was well tolerated with acceptable levels of toxicity (25). However, there is a scarcity of sufficient studies examining the therapeutic effects of combined anti-PD-1 and multisite SBRT in mCRPC treatment, necessitating further research. Another phase II trial demonstrated that avelumab combined with SBRT exhibited elevated activity and acceptable toxicity in treatment-refractory mCRPC (26). Although a study reported that SBRT with a few fractionations was the best choice for the abscopal effect (27), an appropriate RT technique should be chosen based on the symptoms and condition of the patient with mCRPC. Previous studies have demonstrated that low-dose radiation (e.g., doses below 3 Gy) could promote immune cell infiltration into the stroma and the tumor bed of distant tumors, resulting in an improved rate of the systemic response to metastatic disease (28).
Furthermore, low-dose radiotherapy against established metastases has also been shown to significantly enhance the abscopal response to hypofractionated RT plus immune checkpoint inhibitors (29). Low-dose radiation also carries the potential to amplify the antitumor immune effects. Another study suggested that low-dose radiation (a maximum dose of 8–10 Gy/fraction) could induce interferon signaling, resulting in RT-induced abscopal outcomes (30). Several studies also indicated that multiple dose-fractionation schedules of RT resulted in an enhanced abscopal effect compared to a single dose (31, 32). However, further research is required to explore whether the reported doses of RT would exhibit the activation impact in combination with immunotherapy. Thus, combination trials of immunotherapy and RT could be designed to optimize the choice of optimal dose and fractionation.
Here, we aimed to analyze the safety and efficacy of multisite radiotherapy combined with tislelizumab for patients with mCRPC who have experienced failure of at least one second-line treatment. Additionally, we planned to explore the predictive biomarkers of the efficacy of this combined regimen to facilitate clinical studies.
This study is an open-label, single-arm, phase Ib/II prospective study including patients with mCRPC who experienced disease progression after treatment with ADT and had at least one second-line endocrine therapy failure (abiraterone acetate or enzalutamide). This study includes 48 patients, with the entire study (treatment and follow-up phases) lasting approximately 36 months; the maximum duration of tislelizumab treatment has been limited to 2 years. This study protocol has been approved by the Ethics Review Committee of West China Hospital, Sichuan University (Ethical approval number: 2021203). Written informed consent was obtained from all patients. The study has been registered on the Chinese Clinical Trials Registry (Chictr.org.cn) with registration number: ChiCTR2100046212.
All patients who conformed to the inclusion criteria were included (Table 1). Additionally, patients would be able to withdraw from the study if they experience progression of disease (PD), elevated levels of toxicities, are lost to follow-up, die, undergo protocol violation, concomitant disease, or based on the investigator’s decision.
PD was assessed via imaging (CT/MRI/bone scan) as per Prostate Cancer Working Group 3 (PCWG3)–modified RECIST v1.1, a revised version in PCWG3 based on PCWG2.
Figure 1 summarizes the execution outline of this study. Even if patients discontinue treatment due to disease progression, toxicity, or any other reason, they were followed up every 3 months after the end of treatment.
Figure 1 Flowchart of this study. PCWG3, Prostate Cancer Working Group 3; RECIST version 1.1, Response Evaluation Criteria in Solid Tumors version 1.1; irRECIST, Immune-related Response Evaluation Criteria in Solid Tumors.
The enrolled patients were screened within 2 weeks of the initiation of treatment. The following necessary procedures were performed during the screening: a collection of demographic and medical history, physical examination, estimation of PS ECOG, diagnosis and staging of the primary tumor, laboratory examination (blood, liver, kidney, heart, and thyroid function examinations), and imaging analysis. Finally, enrolled patients were required to sign a written informed consent.
Figure 2 shows the therapeutic scheme, which has been divided into three phases, including induction therapy (phase 1), combination therapy (phase 2), and maintenance therapy (phase 3).
Figure 2 Flowchart of the treatment protocol. The therapeutic scheme has been divided into four phases: Induction phase, where patients were scheduled to receive the tislelizumab every 3 weeks (21-day cycle) for two cycles; Combination phase, where patients were scheduled to receive the SBRT (once every 2 days during one cycle) combined with tislelizumab (on day 1 every cycle); and Consolidation and maintenance phases, at 14 days after completing synchronous radiation, where patients were scheduled to receive tislelizumab alone on day 1 of a 21-day cycle until treatment was discontinued. During the follow-up, observing this study’s safety and clinical efficacy were observed. Abbreviations: PD-1, programmed cell death-1; SBRT, stereotactic body radiation therapy. The asterisk indicates that disease progression was confirmed according to the modified RECIST 1.1 of PCWG3. The number sign indicates that patients would receive conventional radiotherapy with 2 Gy every day up to a total dose of 40 or 50 Gy if the surrounding critical organs were at risk around lymph nodes, such as the duodenum, small intestine, and colon.
In phase 1, patients received 1-h intravenous tislelizumab (provided by BeiGene, Beijing, China) at 200 mg every 3 weeks for two cycles. As described in Table 2, tislelizumab was suspended or terminated in the case of severe adverse events.
In phase 2, patients received tislelizumab combined with multisite RT for one cycle. During this phase, tislelizumab was administered at 200 mg once every 3 weeks; the schedule for multisite radiotherapy is presented in Figure 2. RT was performed using the intension-modulated radiotherapy (IMRT) technique under computed tomographic (CT) localization at 6MV-X rays. SBRT was recommended as the method of choice for RT.
The RT was administered to one or three disease sites, selected based on a prioritization order (Table 3). Table 3 also shows the preferred choice of radiotherapy doses/fractions for individual metastases. Furthermore, the exact dose/fraction might be limited by the paracancerous tissue sites in the patient. Thus, based on the actual condition of the patient, we also considered adopting the preferred dose/fraction of RT, as shown in Figure 2.
Lymph nodes with a short diameter ≥1 cm on CT were considered metastatic lymph nodes. Moreover, pelvic wall, retroperitoneal, mediastinum, clavicle, and axilla lymph nodes were preferentially selected as gross tumor volume of lymph nodes (GTVnd) for RT (Figure 2) and without prophylactic irradiation of the lymph node drainage area. Table 4 shows normal tissue dose constraints (33).
Phase 3 was initiated 14 days after completing synchronous radiation, followed by tislelizumab maintenance therapy (200 mg; once every 3 weeks) until either the disease progressed or the patient developed unacceptable toxicity. However, notably, pseudoprogression might occur in the maintenance phase of tislelizumab, which would be required to be distinguished from actual progression by the researchers.
The primary endpoints included safety and ORR. ORR is defined as the proportion of patients who achieved a CR or partial response (PR). Secondary endpoints included the following indicators: prostate-specific antigen (PSA) response rate (PCWG3), disease control rate (DCR), OS, and progression-free survival (PFS) (radiographic PFS (rPFS), biochemical PFS (bPFS)). Here, rPFS is the time between the initial treatment start and radiographic PD, and bPFS is the time between the start of initial treatment and PD (caused by continuous elevation of PSA). We defined the PSA response rate as a 50% decline in PSA levels from baseline to 12 weeks after receiving tislelizumab monotherapy and the DCR as the proportion of patients whose best response was CR, PR, or stable disease (SD).
PFS is the time interval between therapy initiation and radiographic or biochemical PD, or death, whichever comes first. Furthermore, the exploratory purpose of this study was to explore the predictive biomarkers as described in the Discussion and Appendix Table 1 that were related to efficacy and survival, which would help guide toward more individualized therapy.
In this study, laboratory testing and imaging examination were used to evaluate clinical symptoms, tumor response, adverse events (AEs), and biomarkers (Appendix Table 1). A radiological review determined the tumor response every two treatment cycles (6 weeks). If disease progression was indicated based on imaging analysis, subsequent imaging analysis would be done to confirm this at least 4 weeks later. If pseudoprogression was confirmed, the investigator would then decide whether treatment could be continued.
The following data were recorded for safety and ORR assessment: demographics and medical history, physical examination, vital signs, laboratory testing, imaging examination, PSA, AEs, and biomarkers testing. The predictive biomarkers evaluated in this analysis included the following: the expression of DNA mismatch repair protein (MMR), androgen receptor splice variant 7 (AR-V7), tumor PD-L1, tumor-infiltrating lymphocyte (TIL) count, classification of immune cells and subsets (CD4+T, CD8+T, Treg, MDSC, M1-TAM (antitumor M1-like), M2-TAM (protumor M2-like)), the status of homologous recombination repair gene, AR pathway-related genes, and tumor mutation burden (TMB) level in tumors. In addition, we would also determine the classification of immune cells and subsets and TMB levels in peripheral blood.
Patients who successfully completed the interventional treatment were followed up for 30 days, and rAEs were recorded. In the case of no complications, patients were followed up every 2–3 months to collect antitumor treatment data and OS. However, patients who discontinued treatment for reasons other than PD were followed up every 8 weeks, followed up via imaging evaluation. If patients developed PD, they were followed up every 12 weeks to collect OS until death, consent to withdrawal, or the end of the study.
During safety evaluation, we observed and recorded all AEs (including acute and late radiotherapy-related adverse events, immune-related adverse events (irAEs)), serious adverse events (SAEs), laboratory examination, general physical examination, performance status score, electrocardiogram, echocardiogram, thyroid function, myocardial markers, etc. The Common Toxicity Criteria for Adverse Events (CTCAE) v4.0 were used to classify AEs. Additionally, the radiation toxicity criteria of the Radiation Therapy Oncology Group (RTOG) and the European Organization for Research and Treatment of Cancer (EORTC) guidelines were used to assess the acute and late radiotherapy-related toxicities of grade and management (34). Secondly, the irAEs were also graded and managed according to the updated ASCO guidelines (35). Additionally, the “early” (<12 months) and “late” (>12 months) irAEs were categorized based on recent research data (36, 37). In this study, the following SAEs were considered: death, life-threatening AEs, in-patient or prolongation of existing hospitalization, permanent/severe disability, congenital anomalies/birth defects, or any significant medical event requiring intervention. Any AEs were registered during the AE reporting period. In addition, AEs associated with the investigational drug were also registered after reporting. All patients exhibiting SAEs were discontinued immediately, and the investigator reported cases to the sponsor as well as the ethics committee of the hospital within 24 h.
The sample size of this study was calculated according to Simon’s two-stage method (α = 0.05 (bilateral), β = 0.2) and by using efficacy as the estimation index. In a previous KEYNOTE-199 study, ORR was reported to be 5% in 133 patients who were PD-L1 positive in cohort 1 and 3% in 66 patients who were PD-L1 negative in cohort 2. The response rate of these 199 patients in the two cohorts was 4.5% (10). In this study, we hypothesized that the effective rate of radiotherapy combined with tislelizumab would reach 15%. Thus, 48 patients were enrolled and divided into two stages. A stage 1 study included 29 patients; stage 2 consisted of 15 patients when the ORR from stage 1 reached at least 1 (RECIST v1.1). Four patients were then added, considering a 10% loss to follow-up or dropout rate.
The primary efficiency analysis will be performed on the complete analysis set, including all subjects assigned to interventional therapy. Patients who received ≥1 dose of the investigational drug and recorded safety indicators were evaluated for safety analysis. Descriptive statistics were provided using medians (ranges) and means (standard deviations) for continuous variables and frequency (proportions) for categorical variables. The Clopper–Pearson method was used for PSA response rates and 95% CI. The Kaplan–Meier method was used to estimate the PFS and OS; the median values were estimated with a 95% CI. All statistical analyses were two-sided, and p < 0.05 was considered significant. All statistical analyses were done using SPSS software v25.0.
All researchers in this study were responsible for the accuracy of the collected data as well as data management. The data monitoring committee (DMC) conducted regular data monitoring during and after the study.
This study presents the first investigational analysis of the safety and efficacy of tislelizumab combined multisite RT for patients with mCRPC who had experienced failed ADT and at least one second-line endocrine therapy failure. Until now, the poor responses of immunotherapy against PCa might be attributed to its characteristics of low immune infiltration, low tumor mutation load, and low antigen presentation (38). Additionally, PCa evades and inhibits antitumor immunity via elevated expression of PD-L1 and enrichment of Tregs in both tumor and peripheral blood [19-21]. Interestingly, various studies have confirmed that a combination of immunotherapy and RT could constitute a promising strategy for the synergistic enhancement of treatment efficacy. In the last few years, several studies on various types of tumors have explored the combination of radiotherapy and immunotherapy, such as breast cancer, melanoma, nonsmall-cell lung cancer (NSCLC), and esophageal squamous cell carcinoma (39). All studies showed promising antitumor activity and acceptable tolerability. In recent years, there have been significant advances in the treatment of PCa, and several new treatment strategies for mCRPC with clinically proven survival benefits for mCRPC have been developed (10, 11, 40). However, there is still a lack of appropriate strategies for patients with mCRPC who have experienced ADT failure and second-line endocrine therapy. A recent study revealed that avelumab with SABR showed promising activity and acceptable toxicity in treatment-refractory mCRPC (26), indicating that immunotherapy combined with RT was still the best area of research. However, the data were limited to only one combination of tislelizumab and RT, limiting the treatment potential of mCRPC. Therefore, the combination treatment of tislelizumab plus multisite radiotherapy represents a potential approach and needs further investigation for patients with mCRPC who had experienced failure of ADT and second-line endocrine therapy.
The present study has been designed for three treatment phases. Tislelizumab monotherapy aims to observe the efficacy of tislelizumab monotherapy for patients with mCRPC by measuring changes in patients’ PSA levels and symptoms. Due to the “cold tumor” characteristics of PCa, we predict that the 2-cycle efficacy of tislelizumab monotherapy may be insignificant. But it may show effectiveness in some patients who might benefit from immunotherapy in a short period, and those patients are worth being screened for biomarkers for immunomonotherapy.
Some patients with an immediate immune reaction to immunotherapy may result in irAEs. Previous studies reported that patients who experienced irAEs demonstrated marked improvements in immunotherapy efficacy compared to those with low toxicity (41). However, if irAEs occur prematurely (≤8 weeks), immunotherapy is likely to be discontinued due to toxicity. Therefore, we designed two cycles of tislelizumab monotherapy. Furthermore, recent retrospective studies have indicated that “early” irAEs were associated with poor prognosis, and the immunosuppressive treatment for irAEs may hinder anti–PD-1 monotherapy efficacy (42, 43). Therefore, the monotherapy phase would also help understand whether the early irAEs will occur in tislelizumab monotherapy, thus assessing the safety of tislelizumab monotherapy. Followed by the tislelizumab combined RT, comparing the efficacy and safety of tislelizumab monotherapy, the safety and synergistic effect of multisite RT combined with immunotherapy can be better observed. Furthermore, the safety and efficacy of RT can still be fully observed due to a delayed effect from RT, even if entering the tislelizumab monotherapy maintenance phase. Notably, suppose patients with mCRPC obtain a good survival benefit from this study, then the treatment value of tislelizumab as a maintenance therapy method in these patients could obtain preliminary verification.
Until now, there has been a lack of consensus regarding the ideal dose of RT in combination with immunotherapy. SBRT, as a novel RT method, is essential in the treatment of early primary cancer and oligometastatic disease, such as oligometastatic (≤5 lesions) PCa, early-stage nonsmall-cell lung cancer, and liver cancer (44, 45). It has the potential to deliver a small amount of ultra-high doses of radiation to relatively small target lesions, achieving local control with a low risk of toxicity (46). For advanced cancer patients with multiple metastases, the dose of irradiated lesions might be different to achieve excellent local control with a low risk of toxicity and more potent immune activation effects. Therefore, individualized RT will be performed in this study. We will still preferentially select treatment with SBRT, 40 Gy in five fractions, every other day for primary lesions.
On the one hand, the hypofractionated SBRT regimen of 40 Gy/5 is delivered to accommodate the radiation tolerance of organs at risk. On the other hand, the hypofractionated SBRT regimen facilitates immunogenic cell death (ICD), leading to the release of tumor antigens, thus amplifying the efficacy of immunotherapy (47). However, the majority of patients with PCa usually present with multiple distant metastases. In such cases, the dose regimens, guidelines, and normal tissue constraints determined in carefully conducted, high-quality prospective trials should be adopted (44). According to the ASTRO guidelines and the SABR-COMET study (48), 30 Gy in 10 fractions was preferred to treat bone metastases in the present study, which plays a role in palliative pain relief and modulates the immune response microenvironment (49). For liver metastases, 20 Gy in 5 fractions was standard institutional practice. According to the 2011 consensus guidelines, the radiation dose fractionation for lung metastases mainly included 8 Gy in 1 fraction, 20 Gy in 5 fractions, and 30 Gy in 10 fractions (50), and 20 Gy in 5 fractions was preferred for the treatment of bone metastases in the present study.
Furthermore, the present study combined immunotherapy treatment for patients with mCRPC who had failed multiline therapy and had a relatively long survival time. Thus, irAEs are important safety parameters to consider, especially for fatal irAEs such as pneumonitis, neurologic toxicity, colitis/diarrhea, and hepatitis (51) as the significant life-threatening factors for elderly patients. Using the combination of higher radiation doses with anti-PD-1 immunotherapy may cause irAEs to occur in the long term. Therefore, SBRT with relatively low radiation doses was performed based on security considerations in this study.
Tislelizumab is a novel IgG4 anti-PD-1 mAb monoclonal antibody that minimizes binding to FcγR on the surface of macrophages to eliminate antibody-dependent phagocytosis, resulting in a higher affinity for PD-1 compared with pembrolizumab and nivolumab (12). Both clinical literature and pharmacokinetics (PK) analysis have demonstrated that tislelizumab is well tolerated for multiple advanced tumor types and supports fixed dosing (200 mg) (52). Therefore, in the present study, we used a fixed-dose instead of dose-escalation exploration, which avoided the uncertainty caused by dose exploration and improved the effectiveness of this study.
Additionally, the most important aim was to maximize the therapeutic benefits by developing predictive biomarkers of immunotherapy responsiveness. Several biomarkers have been associated with the treatment effect of anti-PD-1 therapy, such as TMB, mismatch repair deficiency (dMMR), PD-1 expression, and TIL number (53). At the same time, these have been reported to be relatively rare in patients with mCRPC. TMB, a biomarker independent of PD-L1 expression, has been revealed to have a significant association with ORR across multiple cancer types (54). However, the application of TMB in mCRPC needs further validation. A previous study suggested that tumors with dMMR are susceptible to PD-1 and PD-L1 inhibitors.
Meanwhile, dMMR tumors exhibit a dense infiltration of CD8+ TILs that have been shown to induce a better and more durable response (55). Several clinical studies have indicated the association between dMMR and immunotherapy-related responses and better prognosis in other solid tumors (55–57). However, this correlation needs further exploration in mCRPC. Numerous clinical trials have investigated that PD-L1 expression is the most widely adopted predictor, and high PD-L1 expression is associated with clinical benefit and response rate improvement in anti-PD-1/anti-PD-L1 therapy (53). TIL is a vital component that influences the tumor immune microenvironment and is used for the prediction of immunotherapy combined with the expression of PD-L1 expression. Elevated levels of baseline TIL and PD-L1 expression in breast cancers were found to be associated with an increased probability of pathologic complete response (58). However, in the immunotherapy combination of multisite RT for mCRPC treatment, the predictive value of PD-L1 expression and TIL counts is vague and deserves further investigation.
Furthermore, studies have demonstrated that genomic alterations might elicit a broad impact on the tumor microenvironment, contributing to the promotion and maintenance of responses to immunotherapy (59–61). Thus, a genomic analysis needs to be performed in this study to determine the impact of genomic alterations (such as mutations in the exonuclease domain of the DNA polymerase epsilon (POLE), high tumor mutational burden, and the presence of biallelic loss of CDK12, among others) on immunotherapy for PCa, for the early detection and identification of novel therapeutic targets. Thus, it would be crucial to establish a comprehensive assessment framework involving multiple biomarkers for interrogating the tumor immune landscape and selecting sensitive patients.
However, this study has several limitations. It is a nonrandomized study with a small sample size. Therefore, the results of this study would provide preliminary support for future randomized, controlled trials to assess the combined therapeutic regimen for patients with mCRPC.
Thus, this study is the first attempt to evaluate the efficacy and safety of tislelizumab with multisite radiotherapy for patients with mCRPC who have failed ADT and second-line endocrine therapy, in an attempt to provide an accurate and effective combined treatment for patients with mCRPC and improve the survival status of patients.
The original contributions presented in the study are included in the article/Supplementary Material. Further inquiries can be directed to the corresponding author.
The studies involving human participants were reviewed and approved by Ethics Review Committee of West China Hospital, Sichuan University (Ethical approval number: 2021203). The patients/participants provided their written informed consent to participate in this study.
KC, YQW, YC, YCW, and ZL were involved in the study conception and design. ZL provided administrative support. KC, YQW, and YC provided materials and samples. KC, JZ, and XQ participated in data collection. KC, YQW, YC, YCW, ZL, JZ, and XH contributed to analysis and interpretation of the data and wrote the manuscript. All authors were involved in the final approval of the manuscript. All authors agreed to be accountable for all aspects of the work and approved the final manuscript.
This study was partly supported by the Sichuan Science and Technology Department Key Research and Development Project (22YFS0336) and 1.3.5 project for disciplines of excellence, West China Hospital, Sichuan University (ZYJC18048).
The authors declare that the research was conducted in the absence of any commercial or financial relationships that could be construed as a potential conflict of interest.
All claims expressed in this article are solely those of the authors and do not necessarily represent those of their affiliated organizations, or those of the publisher, the editors and the reviewers. Any product that may be evaluated in this article, or claim that may be made by its manufacturer, is not guaranteed or endorsed by the publisher.
Thanks are due to all the patients in our study and their family members. Thanks to Beigene for providing the study medication to the patients during the study.
The Supplementary Material for this article can be found online at: https://www.frontiersin.org/articles/10.3389/fonc.2022.888707/full#supplementary-material
Supplementary Table 1 | Assessment timetable. In peripheral blood, the biomarkers and the main detection techniques included immune cells and subsets, such as CD4+T/CD8+T/Treg/MDSC/M1-TAM/M2-TAM (FCM); TMB (NGS). PSA, prostate-specific antigen; AEs, adverse events; SAEs, serious adverse events; MMR, mismatch repair protein; PD-1, programmed death-1; PD-L1, programmed death-ligand 1; PD-L2, programmed death-ligand 2; TILs, tumor-infiltrating lymphocytes; MDSCs, myeloid-derived suppressor cells; M1-TAM, antitumor M1-like; M2-TAM, protumor M2-like; LAG-3, lymphocyte activation gene 3; TIM-3, T-cell immunoglobulin mucin-3; AR-V7, androgen receptor variant 7; TMB, tumor mutation burden. Biomarker detection techniques: IHC, immunohistochemical; HE staining, hematoxylin-eosin staining; FCM, flow cytometry; RNA-seq, RNA sequencing. aIn a tumor, the biomarkers and the main detection techniques were as follows: MMR (IHC), PD-1/PD-L1/PD-L2 (IHC), TIL (HE staining and IHC), immune cells, and subsets, such as CD4+T/CD8+T/Treg/MDSC/M1-TAM/M2-TAM (FCM); costimulatory factor: LAG-3/TIM-3/CD28/CD80/CD137 (GenecastPlex-59 panel), AR-V7 (IHC and NGS), homologous recombination repair genes (NGS), AR pathway-related genes (NGS), and RNA (RNA-seq).
mCRPC, metastatic castration-resistant prostate cancer; ADT, androgen deprivation therapy; PSA, prostate-specific antigen; rPFS, progression-free survival; bPFS, biochemical progression-free survival; PCa, prostate cancer; PD-1, programmed death receptors; PD-L1, programmed death-ligand 1; CR, complete response; RT, radiotherapy; SBRT, stereotactic body radiotherapy; PD, disease progression; PCWG3, Prostate Cancer Working Group 3; OS, overall survival; IMRT, intension-modulated radiotherapy; CT, computed tomographic; ORR, objective response rate; PR, partial response; PSA, prostate-specific antigen; PFS, progression-free survival; SD, stable disease; AEs, adverse events; MMR, mismatch repair protein; AR-V7, androgen receptor splice variant 7; TIL, tumor-infiltrating lymphocyte; M1-TAM, antitumor M1-like; M2-TAM, protumor M2-like; TMB, tumor mutation burden; TC, T cell receptor; irAEs, immune-related adverse events; SAEs, serious adverse events; CTCAE, Common Toxicity Criteria for Adverse Events; NSCLC, nonsmall-cell lung cancer; ICD, immunogenic cell death; dMMR, mismatch repair deficiency; POLE, polymerase epsilon.
1. Baciarello G, Gizzi M, Fizazi K. Advancing Therapies in Metastatic Castration-Resistant Prostate Cancer. Expert Opin Pharmacother (2018) 19(16):1797–804. doi: 10.1080/14656566.2018.1527312
2. Pritchard CC, Mateo J, Walsh MF, De Sarkar N, Abida W, Beltran H, et al. Inherited DNA-Repair Gene Mutations in Men With Metastatic Prostate Cancer. New Engl J Med (2016) 375(5):443–53. doi: 10.1056/NEJMoa1603144
3. Fizazi K, Jenkins C, Tannock IF. Should Docetaxel be Standard of Care for Patients With Metastatic Hormone-Sensitive Prostate Cancer? Pro and Contra. Ann Oncol Off J Eur Soc Med Oncol (2015) 26(8):1660–7. doi: 10.1093/annonc/mdv245
4. Gravis G, Boher JM, Joly F, Soulie M, Albiges L, Priou F, et al. Androgen Deprivation Therapy (ADT) Plus Docetaxel Versus ADT Alone in Metastatic Non Castrate Prostate Cancer: Impact of Metastatic Burden and Long-Term Survival Analysis of the Randomized Phase 3 GETUG-AFU15 Trial. Eur Urol (2016) 70(2):256–62. doi: 10.1016/j.eururo.2015.11.005
5. Mao Y, Hu M, Yang G, Gao E, Chen W. Current Status of Castration-Resistant Prostate Cancer Drug Therapy. Int J Surg: Oncol (2021) 6(1):41–9. doi: 10.29337/ijsonco.126.
6. Alva A, Hussain M. Optimal Pharmacotherapeutic Management of Hormone-Sensitive Metastatic Prostate Cancer. Drugs (2013) 73(14):1517–24. doi: 10.1007/s40265-013-0106-3
7. Mateo J, Carreira S, Sandhu S, Miranda S, Mossop H, Perez-Lopez R, et al. DNA-Repair Defects and Olaparib in Metastatic Prostate Cancer. N Engl J Med (2015) 373(18):1697–708. doi: 10.1056/NEJMoa1506859
8. de Bono J, Mateo J, Fizazi K, Saad F, Shore N, Sandhu S, et al. Olaparib for Metastatic Castration-Resistant Prostate Cancer. New Engl J Med (2020) 382(22):2091–102. doi: 10.1056/NEJMoa1911440
9. Bansal D, Reimers MA, Knoche EM, Pachynski RK. Immunotherapy and Immunotherapy Combinations in Metastatic Castration-Resistant Prostate Cancer. Cancers (2021) 13(2):334. doi: 10.3390/cancers13020334
10. Antonarakis ES, Piulats JM, Gross-Goupil M, Goh J, Ojamaa K, Hoimes CJ, et al. Pembrolizumab for Treatment-Refractory Metastatic Castration-Resistant Prostate Cancer: Multicohort, Open-Label Phase II KEYNOTE-199 Study. J Clin Oncol Off J Am Soc Clin Oncol (2020) 38(5):395–405. doi: 10.1200/JCO.19.01638
11. Petrylak DP, Loriot Y, Shaffer DR, Braiteh F, Powderly J. Safety and Clinical Activity of Atezolizumab in Patients With Metastatic Castration-Resistant Prostate Cancer: A Phase I Study. Clin Cancer Res (2021) 27(12):3360–9. doi: 10.1158/1078-0432.ccr-20-1981
12. Song Y, Gao Q, Fan L, Zou D, Zhang H, Zhou J, et al. Tumor Microenvironment Associated With Complete Response to Tislelizumab Monotherapy in Relapsed/Refractory Classical Hodgkin Lymphoma Reveals a Potentially Different Mechanism of Action. Blood (2020) 136:17. doi: 10.1182/blood-2020-136696
13. Ye D, Liu J, Zhou A, Zou Q, Li H, Fu C, et al. Tislelizumab in Asian Patients With Previously Treated Locally Advanced or Metastatic Urothelial Carcinoma. Cancer Sci (2021) 112(1):305–13. doi: 10.1111/cas.14681
14. Shen L, Guo J, Zhang Q, Pan H, Yuan Y, Bai Y, et al. Tislelizumab in Chinese Patients With Advanced Solid Tumors: An Open-Label, non-Comparative, Phase 1/2 Study. J Immunother Cancer (2020) 8(1):e000437. doi: 10.1136/jitc-2019-000437
15. Beer TM, Kwon ED, Drake CG, Fizazi K, Logothetis C, Gravis G, et al. Randomized, Double-Blind, Phase III Trial of Ipilimumab Versus Placebo in Asymptomatic or Minimally Symptomatic Patients With Metastatic Chemotherapy-Naive Castration-Resistant Prostate Cancer. J Clin Oncol Off J Am Soc Clin Oncol (2017) 35(1):40–7. doi: 10.1200/jco.2016.69.1584
16. Powles T, Yuen KC, Gillessen S, Kadel EE3, Rathkopf D, Matsubara N, et al. Atezolizumab With Enzalutamide Versus Enzalutamide Alone in Metastatic Castration-Resistant Prostate Cancer: A Randomized Phase 3 Trial. Nat Med (2022) 28(1):144–53. doi: 10.1038/s41591-021-01600-6
17. Fizazi K, Drake CG, Beer TM, Kwon ED, Scher HI, Gerritsen WR, et al. Final Analysis of the Ipilimumab Versus Placebo Following Radiotherapy Phase III Trial in Postdocetaxel Metastatic Castration-Resistant Prostate Cancer Identifies an Excess of Long-Term Survivors. Eur Urol (2020) 78(6):822–30. doi: 10.1016/j.eururo.2020.07.032
18. Garnett CT, Palena C, Chakraborty M, Tsang KY, Schlom J, Hodge JW. Sublethal Irradiation of Human Tumor Cells Modulates Phenotype Resulting in Enhanced Killing by Cytotoxic T Lymphocytes. Cancer Res (2004) 64(21):7985–94. doi: 10.1158/0008-5472.CAN-04-1525
19. Sharabi AB, Nirschl CJ, Kochel CM, Nirschl TR, Francica BJ, Velarde E, et al. Stereotactic Radiation Therapy Augments Antigen-Specific PD-1-Mediated Antitumor Immune Responses via Cross-Presentation of Tumor Antigen. Cancer Immunol Res (2015) 3(4):345–55. doi: 10.1158/2326-6066.CIR-14-0196
20. Bernstein MB, Garnett CT, Zhang H, Velcich A, Wattenberg MM, Gameiro SR, et al. Radiation-Induced Modulation of Costimulatory and Coinhibitory T-Cell Signaling Molecules on Human Prostate Carcinoma Cells Promotes Productive Antitumor Immune Interactions. Cancer biother radiopharmaceuticals (2014) 29(4):153–61. doi: 10.1089/cbr.2013.1578
21. Sharabi AB, Tran PT, Lim M, Drake CG, Deweese TL. Stereotactic Radiation Therapy Combined With Immunotherapy: Augmenting the Role of Radiation in Local and Systemic Treatment. Oncol (Williston Park NY) (2015) 29(5):331–40.
22. Antonia SJ, Villegas A, Daniel D, Vicente D, Murakami S, Hui R, et al. Overall Survival With Durvalumab After Chemoradiotherapy in Stage III NSCLC. New Engl J Med (2018) 379(24):2342–50. doi: 10.1056/NEJMoa1809697
23. Deng L, Liang H, Burnette B, Weicheslbaum RR, Fu YX. Radiation and Anti-PD-L1 Antibody Combinatorial Therapy Induces T Cell-Mediated Depletion of Myeloid-Derived Suppressor Cells and Tumor Regression. Oncoimmunology (2014) 3:e28499. doi: 10.4161/onci.28499
24. Zhang JY, Yan YY, Li JJ, Adhikari R, Fu LW. PD-1/PD-L1 Based Combinational Cancer Therapy: Icing on the Cake. Front Pharmacol (2020) 11:722. doi: 10.3389/fphar.2020.00722
25. Luke JJ, Lemons JM, Karrison TG, Pitroda SP, Melotek JM, Zha Y, et al. Safety and Clinical Activity of Pembrolizumab and Multisite Stereotactic Body Radiotherapy in Patients With Advanced Solid Tumors. J Clin Oncol Off J Am Soc Clin Oncol (2018) 36(16):1611–8. doi: 10.1200/JCO.2017.76.2229
26. Kwan EM, Spain L, Anton A, Gan CL, Garrett L, Chang D, et al. Avelumab Combined With Stereotactic Ablative Body Radiotherapy in Metastatic Castration-Resistant Prostate Cancer: The Phase 2 ICE-PAC Clinical Trial. Eur Urol (2021) 81(3):253–62. doi: 10.1016/j.eururo.2021.08.011
27. Ashrafizadeh M, Farhood B, Eleojo Musa A, Taeb S, Rezaeyan A, Najafi M. Abscopal Effect in Radioimmunotherapy. Int Immunopharmacol (2020) 85:106663. doi: 10.1016/j.intimp.2020.106663
28. Herrera FG, Romero P, Coukos G. Lighting Up the Tumor Fire With Low-Dose Irradiation. Trends Immunol (2022) 43(3):173–9. doi: 10.1016/j.it.2022.01.006
29. Yin L, Xue J, Li R, Zhou L, Deng L, Chen L, et al. Effect of Low-Dose Radiation Therapy on Abscopal Responses to Hypofractionated Radiation Therapy and Anti-PD1 in Mice and Patients With Non-Small Cell Lung Cancer. Int J Radiat oncol biol Phys (2020) 108(1):212–24. doi: 10.1016/j.ijrobp.2020.05.002
30. Vanpouille-Box C, Alard A, Aryankalayil MJ, Sarfraz Y, Diamond JM, Schneider RJ. DNA Exonuclease Trex1 Regulates Radiotherapy-Induced Tumour Immunogenicity. Nat Commun (2017) 8:15618. doi: 10.1038/ncomms15618
31. Theelen WS, de Jong MC, Baas P. Synergizing Systemic Responses by Combining Immunotherapy With Radiotherapy in Metastatic non-Small Cell Lung Cancer: The Potential of the Abscopal Effect. Lung Cancer (Amsterdam Netherlands) (2020) 142:106–13. doi: 10.1016/j.lungcan.2020.02.015
32. Dewan MZ, Galloway AE, Kawashima N, Dewyngaert JK, Babb JS, Formenti SC, et al. Fractionated But Not Single-Dose Radiotherapy Induces an Immune-Mediated Abscopal Effect When Combined With Anti-CTLA-4 Antibody. Clin Cancer Res an Off J Am Assoc Cancer Res (2009) 15(17):5379–88. doi: 10.1158/1078-0432.CCR-09-0265
33. Hanna GG, Murray L, Patel R, Jain S, Aitken KL, Franks KN, et al. UK Consensus on Normal Tissue Dose Constraints for Stereotactic Radiotherapy. Clin Oncol (Royal Coll Radiologists (Great Britain)) (2018) 30(1):5–14. doi: 10.1016/j.clon.2017.09.007
34. Cox JD, Stetz J, Pajak TF. Toxicity Criteria of the Radiation Therapy Oncology Group (RTOG) and the European Organization for Research and Treatment of Cancer (EORTC). Int J Radiat oncol biol Phys (1995) 31(5):1341–6. doi: 10.1016/0360-3016(95)00060-C
35. Schneider BJ, Naidoo J. Management of Immune-Related Adverse Events in Patients Treated With Immune Checkpoint Inhibitor Therapy: ASCO Guideline Update. J Clin Oncol (2021) 39(36):4073–126. doi: 10.1200/jco.21.01440
36. Ghisoni E, Wicky A, Bouchaab H, Imbimbo M, Delyon J, Gautron Moura B, et al. Late-Onset and Long-Lasting Immune-Related Adverse Events From Immune Checkpoint-Inhibitors: An Overlooked Aspect in Immunotherapy. Eur J Cancer (Oxford Engl 1990) (2021) 149:153–64. doi: 10.1016/j.ejca.2021.03.010
37. Nigro O, Pinotti G, De Galitiis F, Di Pietro FR, Giusti R, Filetti M, et al. Late Immune-Related Adverse Events in Long-Term Responders to PD-1/PD-L1 Checkpoint Inhibitors: A Multicentre Study. Eur J Cancer (Oxford Engl 1990) (2020) 134:19–28. doi: 10.1016/j.ejca.2020.04.025
38. Liu C, Wang M. Immune Checkpoint Inhibitor Therapy for Bone Metastases: Specific Microenvironment and Current Situation. J Immunol Res (2021) 2021:8970173. doi: 10.1155/2021/8970173
39. Cushman TR, Caetano MS, Welsh JW, Verma V. Overview of Ongoing Clinical Trials Investigating Combined Radiotherapy and Immunotherapy. Immunotherapy (2018) 10(10):851–0. doi: 10.2217/imt-2018-0019
40. Nuhn P, De Bono JS, Fizazi K, Freedland SJ, Grilli M, Kantoff PW, et al. Update on Systemic Prostate Cancer Therapies: Management of Metastatic Castration-Resistant Prostate Cancer in the Era of Precision Oncology. Eur Urol (2019) 75(1):88–99. doi: 10.1016/j.eururo.2018.03.028
41. Das S, Johnson DB. Immune-Related Adverse Events and Antitumor Efficacy of Immune Checkpoint Inhibitors. J immunother Cancer (2019) 7(1):306. doi: 10.1186/s40425-019-0805-8
42. Bai X, Hu J, Betof Warner A. Early Use of High-Dose Glucocorticoid for the Management of irAE Is Associated With Poorer Survival in Patients With Advanced Melanoma Treated With Anti-PD-1 Monotherapy. Clin Cancer Res (2021) 27(21):5993–6000. doi: 10.1158/1078-0432.ccr-21-1283
43. Maslov DV, Tawagi K, Kc M, Simenson V, Yuan H, Parent C, et al. Timing of Steroid Initiation and Response Rates to Immune Checkpoint Inhibitors in Metastatic Cancer. J immunother Cancer (2021) 9(7):e002261. doi: 10.1136/jitc-2020-002261
44. Lo SS, Fakiris AJ, Chang EL, Mayr NA, Wang JZ, Papiez L, et al. Stereotactic Body Radiation Therapy: A Novel Treatment Modality. Nat Rev Clin Oncol (2010) 7(1):44–54. doi: 10.1038/nrclinonc.2009.188
45. Ahmed KA, Barney BM, Davis BJ, Park SS, Kwon ED, Olivier KR. Stereotactic Body Radiation Therapy in the Treatment of Oligometastatic Prostate Cancer. Front Oncol (2012) 2:215. doi: 10.3389/fonc.2012.00215
46. Chen Y, Gao M, Huang Z, Yu J, Meng X. SBRT Combined With PD-1/PD-L1 Inhibitors in NSCLC Treatment: A Focus on the Mechanisms, Advances, and Future Challenges. J Hematol Oncol (2020) 13(1):105. doi: 10.1186/s13045-020-00940-z
47. Demaria S, Guha C, Schoenfeld J, Morris Z, Monjazeb A, Sikora A, et al. Radiation Dose and Fraction in Immunotherapy: One-Size Regimen Does Not Fit All Settings, So How Does One Choose? J Immunother Cancer (2021) 9(4):e002038. doi: 10.1136/jitc-2020-002038
48. Palma DA, Olson R, Harrow S, Gaede S, Louie AV, Haasbeek C, et al. Stereotactic Ablative Radiotherapy for the Comprehensive Treatment of Oligometastatic Cancers: Long-Term Results of the SABR-COMET Phase II Randomized Trial. J Clin Oncol Off J Am Soc Clin Oncol (2020) 38(25):2830–8. doi: 10.1200/JCO.20.00818
49. Lutz S, Balboni T, Jones J, Lo S, Petit J, Rich SE, et al. Palliative Radiation Therapy for Bone Metastases: Update of an ASTRO Evidence-Based Guideline. Pract Radiat Oncol (2017) 7(1):4–12. doi: 10.1016/j.prro.2016.08.001
50. Rodrigues G, Videtic GM, Sur R, Bezjak A, Bradley J, Hahn CA, et al. Palliative Thoracic Radiotherapy in Lung Cancer: An American Society for Radiation Oncology Evidence-Based Clinical Practice Guideline. Pract Radiat Oncol (2011) 1(2):60–71. doi: 10.1016/j.prro.2011.01.005
51. Kennedy LB, Salama AKS. A Review of Cancer Immunotherapy Toxicity. CA: Cancer J Clin (2020) 70(2):86–104. doi: 10.3322/caac.21596
52. Desai J, Deva S, Lee JS, Lin CC, Yen CJ, Chao Y, et al. Phase IA/IB Study of Single-Agent Tislelizumab, an Investigational Anti-PD-1 Antibody, in Solid Tumors. J immunother Cancer (2020) 8(1):e000453. doi: 10.1136/jitc-2019-000453
53. Yi M, Jiao D, Xu H, Liu Q, Zhao W, Han X, et al. Biomarkers for Predicting Efficacy of PD-1/PD-L1 Inhibitors. Mol Cancer (2018) 17(1):129. doi: 10.1186/s12943-018-0864-3
54. Yarchoan M, Hopkins A, Jaffee EM. Tumor Mutational Burden and Response Rate to PD-1 Inhibition. New Engl J Med (2017) 377(25):2500–1. doi: 10.1056/NEJMc1713444
55. Zhao P, Li L, Jiang X, Li Q. Mismatch Repair Deficiency/Microsatellite Instability-High as a Predictor for Anti-PD-1/PD-L1 Immunotherapy Efficacy. J Hematol Oncol (2019) 12(1):54. doi: 10.1186/s13045-019-0738-1
56. Jin Z, Sinicrope FA. Prognostic and Predictive Values of Mismatch Repair Deficiency in Non-Metastatic Colorectal Cancer. Cancers (2021) 13(2):300. doi: 10.3390/cancers13020300
57. Marabelle A, Le DT, Ascierto PA, Di Giacomo AM, De Jesus-Acosta A, Delord JP, et al. Efficacy of Pembrolizumab in Patients With Noncolorectal High Microsatellite Instability/Mismatch Repair-Deficient Cancer: Results From the Phase II KEYNOTE-158 Study. J Clin Oncol Off J Am Soc Clin Oncol (2020) 38(1):1–10. doi: 10.1200/jco.19.02105
58. Pelekanou V, Barlow WE, Nahleh ZA, Wasserman B, Lo YC, von Wahlde MK, et al. Tumor-Infiltrating Lymphocytes and PD-L1 Expression in Pre- and Posttreatment Breast Cancers in the SWOG S0800 Phase II Neoadjuvant Chemotherapy Trial. Mol Cancer Ther (2018) 17(6):1324–31. doi: 10.1158/1535-7163.MCT-17-1005
59. Cao L, Huang C, Cui Zhou D, Hu Y, Lih TM, Savage SR, et al. Proteogenomic Characterization of Pancreatic Ductal Adenocarcinoma. Cell (2021) 184(19):5031–52 e26. doi: 10.1016/j.cell.2021.08.023
60. Antonarakis ES, Isaacsson Velho P, Fu W, Wang H, Agarwal N, Sacristan Santos V, et al. CDK12-Altered Prostate Cancer: Clinical Features and Therapeutic Outcomes to Standard Systemic Therapies, Poly (ADP-Ribose) Polymerase Inhibitors, and PD-1 Inhibitors. JCO Precis Oncol (2020) 4:370–81. doi: 10.1200/po.19.00399
Keywords: metastatic castration-resistant prostate cancer (mCRPC), tislelizumab, PD-1 monoclonal antibodies, combination therapy, study protocol, multisite radiotherapy
Citation: Cheng K, Wang Y, Chen Y, Zhu J, Qi X, Wang Y, Zou Y, Lu Q and Li Z (2022) Multisite Radiotherapy Combined With Tislelizumab for Metastatic Castration-Resistant Prostate Cancer With Second-Line and Above Therapy Failure: Study Protocol for an Open-Label, Single-Arm, Phase Ib/II Study. Front. Oncol. 12:888707. doi: 10.3389/fonc.2022.888707
Received: 03 March 2022; Accepted: 10 June 2022;
Published: 07 July 2022.
Edited by:
Constantinos Zamboglou, German Oncology Center, CyprusReviewed by:
August Sigle, University of Freiburg, GermanyCopyright © 2022 Cheng, Wang, Chen, Zhu, Qi, Wang, Zou, Lu and Li. This is an open-access article distributed under the terms of the Creative Commons Attribution License (CC BY). The use, distribution or reproduction in other forums is permitted, provided the original author(s) and the copyright owner(s) are credited and that the original publication in this journal is cited, in accordance with accepted academic practice. No use, distribution or reproduction is permitted which does not comply with these terms.
*Correspondence: Zhiping Li, bGl6aGlwaW5nNjIwMzEyQDE2My5jb20=
†These authors have contributed equally to this work and share first authorship
Disclaimer: All claims expressed in this article are solely those of the authors and do not necessarily represent those of their affiliated organizations, or those of the publisher, the editors and the reviewers. Any product that may be evaluated in this article or claim that may be made by its manufacturer is not guaranteed or endorsed by the publisher.
Research integrity at Frontiers
Learn more about the work of our research integrity team to safeguard the quality of each article we publish.