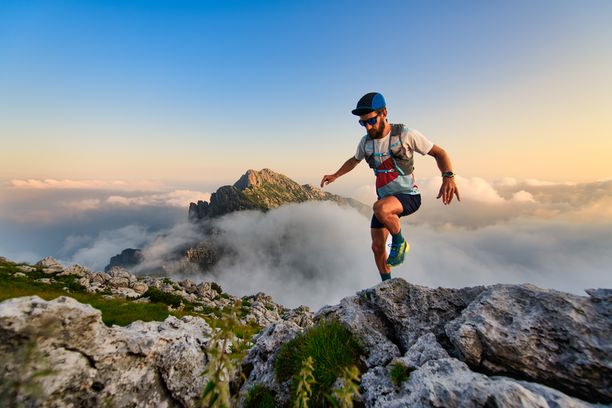
95% of researchers rate our articles as excellent or good
Learn more about the work of our research integrity team to safeguard the quality of each article we publish.
Find out more
REVIEW article
Front. Oncol., 04 July 2022
Sec. Cancer Imaging and Image-directed Interventions
Volume 12 - 2022 | https://doi.org/10.3389/fonc.2022.879697
This article is part of the Research TopicReviews in Cancer Imaging and Image-directed InterventionsView all 17 articles
R0 surgical resection is the preferred treatment for bone and soft tissue sarcoma. However, there is still a lack of precise technology that can visualize bone and soft tissue sarcoma during surgery to assist the surgeon in judging the tumor surgical boundary. Fluorescence imaging technology has been used in the diagnosis of cancer. It is a simple and essentially safe technique that takes no additional time during the operation. Intraoperative fluorescence imaging has potential application prospects in assisting the surgeons in judging the tumor boundary and improving the accuracy of surgical resection. This review mainly starts with clinical studies, animal experimentation, and newly designed probes of intraoperative fluorescence imaging of bone and soft tissue sarcoma, to appraise the application prospects of fluorescence imaging technology in bone and soft tissue sarcoma.
Traditionally, surgeons mainly use preoperative CT(computed tomography) and MRI(magnetic resonance imaging) to assess the tumor boundary within the surgeons’ naked eyes to select the scope of resection during the surgery (1). CT and MRI cannot be used in real-time and have limited tumor specificity (2). Assessments of the resection boundary based on the surgeons’ naked eyes are inaccurate and rely on surgeons’ experience. The intraoperative frozen section reduces surgical efficiency because of the unavoidable extension of the surgery period (3). It is urgent to find an auxiliary examination during the operation to judge the boundary between the tumor tissue and the normal tissue, which can improve the accuracy of the operation together with assistance in finding the tumor satellite foci (4).
The essence of intraoperative tumor fluorescence imaging is to allow fluorescent dyes to accumulate in tumor tissue during the operation so that the surgeons can find the boundary of the tumor. Fluorescent probes have different principles, such as EPR effects (enhanced permeability and retention) and antigen-antibody reactions. To date, fluorescence imaging has exhibited promising advantages in various tumors, such as brain tumors (5), breast cancer (6), and gastric cancer (7).
In recent years, intraoperative fluorescence imaging of bone and soft tissue sarcoma has been explored in clinical studies, animal experimentation, and these studies have led to the development of new probes.
Based on the principle of fluorescent probes, we divide the current fluorescent probes into four types and summarize them in Figure 1.
Figure 1 (A) Non-Specific Fluorescent Probes (ICG): the fluorescent dye remains in areas where the vasculature is highly disordered. (B) Activatable Fluorescence Probes: the dye fluorescence only when the group breaks down under tumor circumstances. (C) Specific fluorescent Probes: the fluorescent dye combines antibodies bind to biomarkers. (D) Multimodality Fluorescence Imaging Probes: the fluorescent dye used for both NIR imaging and SPECT/CT, MRI.
Most non-specific probes rely on a passive targeting strategy, which preferentially accumulates molecules in tumors. The passive targeting strategy attributes to the tumor microenvironment such as accumulation of acidity, anoxic habitat, and necrotic tissue (8). It is not specific so that burns, wounded, and other tissues can also retain more fluorescent dye than surrounding tissues.
The principle fluorescent component of tetracycline is achelate formed upon combination with calcium ions ontrabecular bone (9). OWEN et al. (10) studied the fluorescence of tetracycline medicines in bone cancers and normal bone in 1961. Normal bone tissue has strong fluorescence, while necrotic bone has no or low fluorescence. Tetracycline is nowadays used only infrequently for intraoperative imaging of bone and soft tissue sarcoma, not only because tetracycline drugs are toxic and have a high rate of adverse reactions, but also because the wavelength of tetracycline excitation light is 450-490nm, which is in the visible light range. This wavelength overlaps with normal tissues and is heavily absorbed in tissues such as hemoglobin and myoglobin (11).
Compared with fluorescent probes for fluorescence imaging in the visible region, the research direction in recent years has focused more on fluorescent probes with excitation wavelength in the near-infrared region NIR-1 (700-900nm). Near-infrared fluorescence with a wavelength of 700-900nm is rarely absorbed in tissues (12).
For example, the most commonly used and clinically approved fluorescent probe is indocyanine green (ICG). ICG has absorption and fluorescence spectra in the near-infrared (NIR) region. The excitation wavelength is 780nm, and it emits fluorescence in the range of 700-850nm. The red light is visible to the naked eye, but most of the light is not (13). Most researchers believe that the accumulation of ICG within solid tumors attributes to the EPR (enhanced permeability and retention) effect (14). Due to the presence of defective endothelial cells and wide fenestrations (600 to 800 nm) in nascent blood vessels, small molecules such as ICG are injected systemically and passively accumulate in tumors (15). However, Pandit et al. (16) pointed out that in addition to the EPR effect, transcytosis is the principle of molecular accumulation in tumors. It is the same as the research on ICG in Colorectal Cancer. Cancer cells have a high endocytic rate (17). ICG was preferentially taken up by cancer cells via clathrin-mediated endocytosis (CME) (18). Indocyanine green is a safe, basically non-toxic drug, which rarely reacts with other drugs (19). However, Indocyanine green accumulates in bone tumors, inflammation, and bone deformities.
Many factors can influence the EPR effect, including tumor type, size, and vascular mediators. As a result, the intensity of the ICG signal is unpredictable (20). If the patient has a fracture or ischemia at the surgical site during surgery, it will cause false-positive results and affect the judgment. According to research, encapsulation of ICG improves its targeting abilities and circulation time (21, 22).
Some scientists have also designed activatable fluorescent probes that emit fluorescence only in tumor tissues. This probe contains a chemical group, which can be broken down via some enzymes in the tumor and microenvironment, therefore this probe is activated.
Many activatable fluorescent probes have applications in other types of tumors, and could theoretically be used for intraoperative imaging of bone and soft tissue tumors. The activatable fluorescent probes for the tumor microenvironment are mainly activated by extracellular enzymes specifically emitted in the tumor microenvironment. In addition to cathepsin-activated fluorescent probes that have been used in soft tissue sarcoma animal experimentation, there are also fluorescent probes activated by matrix metalloproteinases (23). The activatable fluorescent probes for tumor cells consist of two parts. One is the activation of intracellular enzymes, such as β-galactosidase bioactivation (24) and glutathione (GSH) bioactivation (25). And the other one is the activation of fluorescence by the tumor cell hypoxia environment (26). Besides, the pH of the tumor microenvironment is generally between 6.7-7.1, the pH of tumor cells is between 5.9-6.2, and the pH of advanced tumor cells can even reach 5.0-5.5, which is an acidic environment compared with normal tissues. Some probes are sensitive to pH, and their fluorescence is activated in an acidic environment allowing fluorescence imaging of tumors and tumor microenvironments (27).
Activatable fluorescence probes reduce the fluorescence intensity of normal tissues and further increase the tumor-to-background ratio (TBR). But at the same time, the chemical synthesis of probes is complicated, and there is still a lack of further research on the adverse reactions of these probes.
Unlike ICG with the EPR effect, specific probes do not rely on the tumor microenvironment but instead rely on a targeting moiety conjugated to a contrast agent with a high binding affinity. These probes have higher targeting properties than indocyanine green (28).
The original design method is to combine monoclonal antibodies with fluorescent dyes to create fluorescent probes. Previously, monoclonal antibodies were used as targeted drugs to treat tumors. For example, Bevacizumab is a monoclonal antibody that binds to vascular endothelial growth factor-A(VEGF-A)which is highly expressed in tumor cells (29) and plays a direct role in vascular endothelial production (30). Combine bevacizumab with the fluorescent dye IRDye800CW to synthesize a fluorescent probe that can specifically bind to tumors. Scientists designed Panitumumab-800CW (31) and Cetuximab-800CW (32) based on the principle of similars. Panitumumab is a monoclonal IgG2 antibody that binds to the Epidermal Growth Factor Receptor (EGFR) with high specificity (33). EGFR is highly expressed in bone and soft tissue sarcoma and is involved in osteolytic metastases of bone tumors. Cetuximab is also an anti-EGFR monoclonal antibody.
In recent years, with the development of chemical synthesis technology, moieties for active targeting have become available, such as nanoparticle scaffolds, peptides, ligands, and aptamers. Compared with antibodies, the moieties have similar binding characteristics but show better tumor penetration and more rapid clearance from non-targeted tissues (34). For example, ABY-029 is an EGFR-targeted affibody molecule labeled with IRDye 800CW (35). While performing intraoperative tumor fluorescence imaging, ABY-029 can be injected on the same day. Besides, compared with bevacizumab, panitumumab, and cetuximab, ABY-029 retains high EGFR specificity (36) with low immunogenicity and low toxicity (37).
Specific fluorescent probes are based on active targeting, their synthesis is complicated. Tumors are heterogeneous, so we can’t find a tumor marker expressed in each tumor tissue. The majority of specific probes are still in the pre-clinical stage. It requires more feasibility and toxicity studies, particularly for small molecule probes before clinical trials.
SPECT/CT, MRI, and NIR combined multimodal imaging technology have gained significant popularity. Scientists have designed fluorescent probes with SPECT/CT, MRI sensitive groups, and fluorescent dyes (38) (Figure 2). The contrast of preoperative SPECT/CT, MRI tumor imaging is improved by preoperative injection of multimodality fluorescent probes. The fluorescent sign of the tumor can also be collected during the operation. This combination of imaging and fluorescence imaging can significantly increase the detection rate of tumors and obtain more accurate tumor boundaries. This probe is used for preoperative tumor imaging, surgical planning, and intraoperative tumor fluorescence imaging.
Figure 2 The chemical structure of fluorescence, magnetic, and SPECT nanoparticles that can compose multimodality probes. (A) A fluorescent dye: Cy5.5 carboxylic acid. (B) A magnetic nanoparticle as molecular imaging agent: gadodiamide. (C) A SPECT-CT tumor imaging agent: technetium Methylenediphosphonate(99mTc-MDT).
Schematically, this kind of probe has much potential. For example, if we expand our scope to treatment, scientists have designed probes that combine Photodynamic therapy with fluorescence imaging (39, 40).
All imaging techniques have their limitations, e.g., MRI has problems with relatively low sensibility, and optical imaging has issues with low spatial resolution and small penetration depth (41). Multiple imaging techniques aid in early diagnosis and treatment planning. However, it is worth exploring whether it is necessary to enhance preoperative MRI and SPECT/CT tumor signal intensity in clinical practice (42).
In recent years, some researchers have focused on fluorescent probes in the NIR-2 range (1000-1700nm) and have produced several fluorescent probes for tumor imaging in the NIR-2 range (43). According to some researchers, fluorescence with a wavelength of 1000-1700nm, can reduce scattering when passing through the skin and is less affected by normal tissue autofluorescence. Compared with NIR-1 imaging, it can penetrate deeper tissues (44).
Presently the widely used non-specific fluorescent probe in tumor surgery is indocyanine green. Overall, these cases in the past five years support that bone and tissue sarcoma can fluoresce after injection, and the tumor boundary is consistent with the pathological section control.
For example, Fourman (2018) (45) injected osteosarcoma cells into the left hind limb of BALB/c Mice. Researchers used pathological sections to confirm that the fluorescent part of the hind limb was a bone sarcoma. Mice with fluorescent lung tissue developed lung metastases from osteosarcoma. Interestingly, the researchers discovered that the higher the fluorescence intensity of the primary bone tumor, the greater the possibility of lung metastases. This finding suggests that we can early predict the probability of lung metastases in osteosarcoma patients through intraoperative bone tumor fluorescence intensity.
Another example of what is meant by Mahjoub (46), is that they injected ICG into 11 osteosarcoma mice 12 hours before surgery for fluorescence-guided osteosarcoma surgery. The recurrence rate of mice with osteosarcoma resection guided by ICG was much lower than that of mice with conventional resection.
In addition to indocyanine green, non-specific fluorescent probes such as Alizarin Red and Tetracycline had been expected to have great potential for intraoperative imaging of bone and soft tissue sarcoma. However, the fluorescence excitation wavelengths are 465nm and 490nm, which are both in the visible light range, and the fluorescence area overlaps with normal tissues. There have been few related studies in recent years.
According to the different characteristics of tumor cells and tumor microenvironments from normal tissues, scientists have designed activatable optical probes. In 2016, Bartholf Dewitt S (47) used the cathepsin-activated fluorescent probe LUM015 in dogs with soft tissue sarcoma for intraoperative fluorescence imaging. According to previous studies, cathepsin is overexpressed in soft tissue sarcoma and other tumors while rarely expressed in normal tissues. All the dogs’ soft tissue sarcoma fluorescence when imaging. The pathologist took 33 parts of the excised tissue for biopsy, all of which were tumor tissues. The cathepsin-activated fluorescent probe is further exemplified in studies by Prince et al. (48). The researchers compare the TBR and effect of prosense750EX (another cathepsin-activated fluorescent probe) with multiple fluorescent probes for fluorescence imaging of soft tissue sarcoma. Prosense750EX, like the other probes in the study, can identify tumor beds with a diameter of less than 1mm intraoperatively. Therefore, the Prosense750EX can be used as a probe for fluorescence imaging.
Some specific probes have been designed, and animal experimentation has proved their specificity and sensitivity. Most specific probes are created by combining fluorescent dyes with antibodies or ligands that precisely bind to tumor cells (Table 1).
Based on this probe design idea, our team designed a specific fluorescent probe CS2-N-E9R for Ewing’s sarcoma-specific fusion protein EWS-FLI1 (E/F) in 2021 (49). Our specific probe can make Ewing’s sarcoma fluorescence imaging in cell experimentation and animal experimentation. Besides, it does not show fluorescence for E/F-negative osteosarcoma cells.
For example, Li et al. (50) combined the non-antibody binding protein of CD105 with fluorescein isothiocyanate(FITC) to obtain a fluorescent probe targeting osteosarcoma. This fluorescent probe causes the osteosarcoma cells, dissected osteosarcoma tissues, and osteosarcoma in mice to emit fluorescence, proving that it can label osteosarcoma.
Another example of what is designed by Zhou (51) is CH1055-PEG-PT and CH1055-PEG-Affibody. These probes combined Small molecule protein binding to 143b osteosarcoma cells with Fluorescent dyes in the NIR-2 region. Both of these probes can image fluorescence in osteosarcoma. The surgeons used fluorescence guidance for tumor resection. Pathology specialists sectioned the tumor and adjacent tissues and stained them for microscopic examination after the surgery. The results revealed that the fluorescence intensity of the tumor was higher than that of adjacent tissues. The researcher suggests that, compared with CT, the fluorescent probe can image tumors smaller than 1 cm in diameter and has a clear fluorescence boundary.
This technology is further exemplified in animal experimentation using indocyanine green and ABY-029 combined fluorescence imaging in soft tissue sarcoma surgery. Sardar et al. (52) discovered that fluorescence imaging with ICG and ABY-029 is superior to ICG or ABY-029 alone. Among them, ABY-029 is more concentrated in the high-cell living tissue area, while ICG is more concentrated in the low-cell area. The article did not explore the reasons further. A possible explanation for these results may be related to the difference in imaging principles between the two probes. ICG accumulates more in new blood vessels, whereas ABY-029 binds to cancer cells specifically. It suggests that combining two fluorescent probes with different localization areas and fluorescence imaging principles could improve the specificity and sensitivity of fluorescence imaging in bone and soft tissue tumor surgery.
Xu’s experimentation study explored the feasibility of specific fluorescent probes used for intraoperative imaging after radiotherapy and chemotherapy (36). Xu designed a mouse model of soft tissue sarcoma after chemotherapy and radiotherapy and injected ABY-029 intraoperative fluorescence imaging into the mice 4-8 hours before surgery. It might be possible to estimate whether most patients with soft tissue sarcoma undergoing preoperative radiotherapy and chemotherapy can use ABY-029 Intraoperative fluorescence imaging. The results confirmed the feasibility of fluorescence imaging of soft tissue sarcoma in mice after radiotherapy and chemotherapy. This outcome is contrary to that of Nicoli et al. (53) who found indocyanine green could not fluorescently label osteosarcoma after radiotherapy. This result demonstrates the superiority of specific fluorescent probes compared to fluorescence imaging in indocyanine green.
Another research compared several fluorescent probes on soft tissue sarcoma mice (48). The researchers compare the intraoperative tissue fluorescence range with HE stained sections, and immunohistochemistry(IHC) to quantitatively compare TBR. Compared to DC101(binding to VEGFR-2) TBR 3.7, IntegriSense750(A small-molecule probe binding to integrin αvβ3) TBR 7.0, and ProSense750EX (activated by locally expressed cathepsin)TBR 5.8, the TBR of cetuximab-IRDye800CW was 16.8, which was significantly higher than other fluorescent probes.
In addition to the fluorescent probes that have been assessed on bone and soft tissue sarcoma, many newly designed fluorescent probes may have the potential to be used in intraoperative imaging of bone and soft tissue sarcoma. Mahalingam et al. (54) designed the Centyrin-Based Near-Infrared Probe, a fluorescent probe that images EGFR-positive tumors. Reviews show that osteosarcoma and soft tissue sarcoma can overexpress EGFR (55). In the future, we can build mouse models and conduct further animal experimentation to explore whether this probe is used for bone and soft tissue tumor imaging.
For a ligand or antibody that specifically binds to bone and soft tissue sarcoma, the ideal is to find a target not expressed in other tissues and expressed in all bone and soft tissue sarcoma, especially tissue cells surrounding the tumor. There are many studies on tumor-specific markers of bone and soft tissue tumor cells. CxCR4 (Cys-X-Cys receptor 4), PDGFR-β(Platelet-derived growth factor receptor-β), TEM1 (Tumor Endothelial Marker 1), VEGFR-1, EGFR, VEGFR-2, IGF-1R, IGF-2R, CD40, et al. are high specific tumor markers (56–58). Scientists use these tumor-specific markers to create antibodies or ligands and combine antibodies and ligands with fluorescent dyes to make specific fluorescent probes. According to animal experiments in the past five years, ligands and small-molecule peptides spread faster than antibodies and are more likely to accumulate in tumor tissue. There are numerous fluorescent dyes on the market currently, most of them are classified as rhodamines, oxazines, fluoresceins, cyanines, and carbopyronines in structure (59). The commonly used near-infrared fluorescent dyes such as IRDye800CW still have high development prospects.
In the case of specific fluorescent probes, future research could focus on developing new probes specifically binding to bone and soft tissue sarcoma, determining whether existing fluorescent probes can be used for bone and soft tissue sarcoma, and evaluating the advantages, disadvantages, and effectiveness of the probes.
Probes for multimodal visualization in MRI, SPECT/CT, and Near-Infrared Optical Imaging have gotten attention in the past five years. These probes have the potential for preoperative tumor imaging, surgical planning, and intraoperative tumor fluorescence imaging.
It is exemplified in the animal experimentation undertaken by Xu with 99mTc-Gd@OVA-Cy nanoprobe (60). Researchers performed preoperative NIR fluorescence imaging, MRI, and SPECT/CT of osteosarcoma with nanoprobe. After 15 minutes of intravenous injection of the fluorescent probe, the images of all three modes showed enhanced signals of osteosarcoma. In MRI, SPECT/CT, and NIR imaging, researchers can observe a clear boundary of osteosarcoma, and the tumor boundary is consistent with the results of HE staining sections. Surprisingly, the researchers also found that the fluorescent probe can show lymph drainage and sentinel lymph nodes. Therefore Xu considered that this probe might be used for osteosarcoma to improve lymph node resection and preoperative planning.
Scientists designed many fluorescent probes for multimodal imaging in the past five years. But there are few animal experiments on whether these fluorescent probes can be applied to bone and soft tissue sarcoma. Lee et al. (61) designed an Nd3+-UCNPs nanoprobe specifically binding to CD44. The nanoprobe is injected into the hepatocellular carcinoma of patients, used for preoperative MRI detection and intraoperative NIR tumor imaging. Related literature shows that bone and soft tissue sarcoma can express CD44 (62). Therefore, this multimodal probe may be significant in intraoperative and preoperative tumor imaging for CD44-positive bone and soft tissue sarcoma.
Researchers also focus on probes for multimodal visualization in SPECT/CT and intraoperative near-infrared optical imaging. A notable example is the folate-ECG-ROX targeted folate receptor in the tumor (63). Another example designed by Manca is the ICG-99mTc probe, which facilitates visualization of lymph drainage and assesses the sentinel lymph node (64).
Reports about intraoperative fluorescence imaging of bone and soft tissue sarcoma are limited (Table 2). In 2019, Samkoe et al. (65) reported a case of using ABY-029 intraoperative fluorescence imaging for soft tissue sarcoma. The intraoperative fluorescence intensity ratio of soft tissue sarcoma to normal tissue/background is 2.0/3.4, which is sufficient to distinguish tumor from normal tissue by fluorescence during operation. The tumor was stained with hematoxylin-eosin staining and IHC postoperatively, and the fluorescent tissue was confirmed to be soft tissue sarcoma, and the fluorescence signal was highly associated with the expression of EGFR.
In a similar case in the UK, 11 patients with bone and soft tissue sarcoma were admitted for ICG intraoperative fluorescence imaging (53). ICG was injected intravenously 16-24 hours before the operation, and the Stryker Spy Phi near-infrared device collected the fluorescence signal during the operation. Surgeons believe that in three of the 11 cases, they removed more tissue during the operation due to fluorescence. Nine of the 11 instances revealed tumor fluorescence during surgery. Two instances exhibited no fluorescence during surgery, one was grade 1 myxofibrosarcoma, and the other was osteosarcoma with more than 90% necrosis after chemotherapy. The failure could be because ICG fluorescence imaging is better suited to tumors with a higher degree of malignancy, no treatment, and fewer necrotic areas.
This technology is further demonstrated in studies using Bevacizumab-IRDye800cw fluorescence imaging in 15 patients with soft tissue sarcoma during surgery (66). Researchers found fluorescence in soft tissue sarcoma during and after the operation in all 15 cases and no adverse reactions. Furthermore, the researcher discovered that the necrotic area of soft tissue sarcoma treated by neoadjuvant chemotherapy had no fluorescence. Auspiciously, we noticed in clinical practice that the necrotic area is more inside the tumor and has few effects on the fluorescence of the tumor border.
Furthermore, bone and soft tissue tumor metastasis are frequent. Fluorescence imaging can detect tumor metastasis in bone and soft tissue. These clinical trials reveal the need for fluorescence imaging among metastases. Patients subjected toI CG injection were assessed after 24 hours (not overlapping with the optimal time for ICG to show bone and soft tissue sarcoma). Among 44 patients with soft tissue sarcoma lung metastases, 40 lung metastases showed fluorescence during Video-assisted thoracoscopic surgery(VATS). Among 40 cases of osteosarcoma lung metastases, 36 cases had fluorescence. The depth of all lung metastases without fluorescence imaging was more than 2 cm. According to Predina, fluorescence imaging during ICG surgery is better for detecting tumor metastasis with a depth smaller than 2 cm and a diameter greater than 5 mm (67).
Scheichel (68) performed a clinical trial using 5-aminolevulinic acid (5-ALA) intraoperative fluorescence imaging in fifty patients with bone and soft tissue infiltrating meningiomas. All bone fluorescence shows tumor invasion into bone tissue. Three patients showed additional fluorescence in the periosteum and temporal muscles, and histopathological examination confirmed tumor infiltration (68).
Predina and colleagues studied a patient with osteosarcoma lung metastases undergoing surgery and showed that fluorescence imaging with OTL38 enabled the detection of Lung metastases. According to previous studies, FR-α is overexpressed in 80% of primary osteosarcoma. The lung metastases had strong fluorescence after intravenous injection of 0.025mg/kg OTL38. However, the researchers did not specify whether fluorescence was observed in the primary osteosarcoma (69).
At present, intraoperative fluorescence imaging does not use quantitative norms to determine whether it is tumor tissue. There is no standard for how high the fluorescence contrast should be to indicate a tumor in intraoperative fluorescence imaging technology. To determine the standard, it is important to conduct clinical trials including large sample size and compare with pathological results. Futhermore, a technique combining biophysics-inspired modeling and artificial intelligence (AI) was envisioned to monitor intraoperative changes in NIR intensities over time in different tissue and provide clinically significant lesion identification (70). In addition, mixed reality(MR) techniques that combine fluorescence imaging with CT have been used in liver resection (71). We can embed an augmented reality (AR)-based navigation system in the fluorescence imaging devices (72), and evaluate the usefulness of the system in the experimental study.
The manufacture and use of fluorescent probes for intraoperative fluorescence imaging of bone and soft tissue sarcoma have a potential future. Non-specific probes may additionally fluoresce in non-tumor areas, which can cause surgeons to misjudge. The main direction of new fluorescent probes will be specific fluorescent probes with high specificity to label tumors. With the further investigation of the mechanism of bone and soft tissue sarcoma, scientists will discover more specific tumor-expressed molecules. We can accordingly design specific fluorescent probes with high specificity and sensitivity.
Simultaneously, we noticed that tumors are heterogeneous, and it is difficult for a probe to image all tumors of the same type. Experiments are currently underway to combine two fluorescent probes with different principles to increase accuracy and lower the negative rate. In the future, we can design fluorescent probes with multiple responses to tumors and the microenvironment to further reduce the false-negative rate of fluorescence during tumor surgery.
ZC: writing-original draft. HH: visualization, formal analysis. SH: investigation. YW: supervision. LC: conceptualization. YX: writing—review and editing. All authors contributed to the article and approved the submitted version.
This work was supported by National Natural Science Foundation of China (NO. 82103285), the Improvement Project for Theranostic ability on Difficulty miscellaneous disease(Tumor) (ZLYNXM202005), the Research Fund from Medical Sci-Tech Innovation Platform of Zhongnan Hospital, Wuhan University (PTXM2021003).
The authors declare that the research was conducted in the absence of any commercial or financial relationships that could be construed as a potential conflict of interest.
All claims expressed in this article are solely those of the authors and do not necessarily represent those of their affiliated organizations, or those of the publisher, the editors and the reviewers. Any product that may be evaluated in this article, or claim that may be made by its manufacturer, is not guaranteed or endorsed by the publisher.
CT, computed tomography; MRI, magnetic resonance imaging; SPECT, single photon emission computed tomography; TBR, tumor-to-background ratio; ICG, indocyanine green; NIR, near-infrared; IHC, immunohistochemistry; VEGF, vascular endothelial growth factor; EGFR, epidermal growth factor receptor; VEGFR, vascular endothelial growth factor receptor; 5-ALA, 5-aminolevulinic acid; FR-α, folate receptor-α; insulin-like growth factor, IGF; EPR, enhanced permeability and retention; CME, clathrin-mediated endocytosis; glutathione, GSH.
1. Reijnders K, Coppes MH, van Hulzen ALJ, Gravendeel JP, van Ginkel RJ, Hoekstra HJ. Image Guided Surgery: New Technology for Surgery of Soft Tissue and Bone Sarcomas. Eur J Surg Oncol (EJSO) (2007) 33:390–8. doi: 10.1016/j.ejso.2006.10.030
2. Israel O, Pellet O, Biassoni L, De Palma D, Estrada-Lobato E, Gnanasegaran G, et al. Two Decades of SPECT/CT - the Coming of Age of a Technology: An Updated Review of Literature Evidence. Eur J Nucl Med Mol Imaging (2019) 46:1990–2012. doi: 10.1007/s00259-019-04404-6
3. Yu Y, Tan Y, Xie C, Hu Q, Ouyang J, Chen Y, et al. Development and Validation of a Preoperative Magnetic Resonance Imaging Radiomics-Based Signature to Predict Axillary Lymph Node Metastasis and Disease-Free Survival in Patients With Early-Stage Breast Cancer. JAMA Netw Open (2020) 3:e2028086. doi: 10.1001/jamanetworkopen.2020.28086
4. Hernot S, van Manen L, Debie P, Mieog J, Vahrmeijer AL. Latest Developments in Molecular Tracers for Fluorescence Image-Guided Cancer Surgery. Lancet Oncol (2019) 20:e354–67. doi: 10.1016/S1470-2045(19)30317-1
5. Zhang DY, Singhal S, Lee J. Optical Principles of Fluorescence-Guided Brain Tumor Surgery: A Practical Primer for the Neurosurgeon. Neurosurgery (2019) 85:312–24. doi: 10.1093/neuros/nyy315
6. Lee J, Kim B, Park B, Won Y, Kim SY, Lee S. Real-Time Cancer Diagnosis of Breast Cancer Using Fluorescence Lifetime Endoscopy Based on the Ph. Sci Rep (2021) 11:16864. doi: 10.1038/s41598-021-96531-0
7. Loshchenov M, Levkin V, Kalyagina N, Linkov K, Kharnas S, Efendiev K, et al. Laser-Induced Fluorescence Diagnosis of Stomach Tumor. Lasers Med Sci (2020) 35:1721–8. doi: 10.1007/s10103-020-02963-x
8. Ali I, Alfarouk KO, Reshkin SJ, Ibrahim ME. Doxycycline as Potential Anti-Cancer Agent. Anticancer Agents Med Chem (2017) 17:1617–23. doi: 10.2174/1871520617666170213111951
9. Frost HM, Villanueva AR, Roth H, Stanisavljevic S. Tetracycline Bone Labeling. J New Drugs (1961) 1:206–16. doi: 10.1177/009127006100100503
10. Owen LN. Fluorescence of Tetracyclines in Bone Tumours, Normal Bone and Teeth. Nature (1961) 190:500–2. doi: 10.1038/190500a0
11. Ristow O, Pautke C. Auto-Fluorescence of the Bone and its Use for Delineation of Bone Necrosis. Int J Oral Max Surg (2014) 43:1391–3. doi: 10.1016/j.ijom.2014.07.017
12. Nagaya T, Nakamura YA, Choyke PL, Kobayashi H. Fluorescence-Guided Surgery. Front Oncol (2017) 7:314. doi: 10.3389/fonc.2017.00314
13. Matsuura Y, Ichinose J, Nakao M, Okumura S, Mun M. Recent Fluorescence Imaging Technology Applications of Indocyanine Green in General Thoracic Surgery. Surg Today (2020) 50:1332–42. doi: 10.1007/s00595-019-01906-6
14. Maeda H, Nakamura H, Fang J. The EPR Effect for Macromolecular Drug Delivery to Solid Tumors: Improvement of Tumor Uptake, Lowering of Systemic Toxicity, and Distinct Tumor Imaging In Vivo. Adv Drug Delivery Rev (2013) 65:71–9. doi: 10.1016/j.addr.2012.10.002
15. Matsumura Y, Maeda H. A New Concept for Macromolecular Therapeutics in Cancer Chemotherapy: Mechanism of Tumoritropic Accumulation of Proteins and the Antitumor Agent Smancs. Cancer Res (1986) 46:6387–92.
16. Pandit S, Dutta D, Nie S. Active Transcytosis and New Opportunities for Cancer Nanomedicine. Nat Mater (2020) 19:478–80. doi: 10.1038/s41563-020-0672-1
17. Onda N, Mizutani-Morita R, Yamashita S, Nagahara R, Matsumoto S, Yoshida T, et al. Fluorescence Contrast-Enhanced Proliferative Lesion Imaging by Enema Administration of Indocyanine Green in a Rat Model of Colon Carcinogenesis. Oncotarget (2017) 8:90278–90. doi: 10.18632/oncotarget.21744
18. Onda N, Kimura M, Yoshida T, Shibutani M. Preferential Tumor Cellular Uptake and Retention of Indocyanine Green for In Vivo Tumor Imaging. Int J Cancer (2016) 139:673–82. doi: 10.1002/ijc.30102
19. Reinhart MB, Huntington CR, Blair LJ, Heniford BT, Augenstein VA. Indocyanine Green. Surg Innov (2016) 23:166–75. doi: 10.1177/1553350615604053
20. Rijs Z, Jeremiasse B, Shifai N, Gelderblom H, Sier C, Vahrmeijer AL, et al. Introducing Fluorescence-Guided Surgery for Pediatric Ewing, Osteo-, and Rhabdomyosarcomas: A Literature Review. Biomedicines (2021) 9. doi: 10.3390/biomedicines9101388
21. Hill TK, Abdulahad A, Kelkar SS, Marini FC, Long TE, Provenzale JM, et al. Indocyanine Green-Loaded Nanoparticles for Image-Guided Tumor Surgery. Bioconjug Chem (2015) 26:294–303. doi: 10.1021/bc5005679
22. Chen Q, Xu L, Liang C, Wang C, Peng R, Liu Z. Photothermal Therapy With Immune-Adjuvant Nanoparticles Together With Checkpoint Blockade for Effective Cancer Immunotherapy. Nat Commun (2016) 7:13193. doi: 10.1038/ncomms13193
23. Ren L, Wang Y, Zhu L, Shen L, Zhang J, Wang J, et al. Optimization of a MT1-MMP-Targeting Peptide and its Application in Near-Infrared Fluorescence Tumor Imaging. Sci Rep-Uk (2018) 8:10334. doi: 10.1038/s41598-018-28493-9
24. Huang J, Jiang Y, Li J, Huang J, Pu K. Molecular Chemiluminescent Probes With a Very Long Near-Infrared Emission Wavelength for In Vivo Imaging. Angew Chemie Int Edition (2021) 60:3999–4003. doi: 10.1002/anie.202013531
25. Guo R, Huang F, Zhang B, Yan Y, Che J, Jin Y, et al. GSH Activated Biotin-Tagged Near-Infrared Probe for Efficient Cancer Imaging. Theranostics (2019) 9:3515–25. doi: 10.7150/thno.32742
26. Zhang Z, Wang R, Huang X, Zhu W, He Y, Liu W, et al. A Simple Aggregation-Induced Emission Nanoprobe With Deep Tumor Penetration for Hypoxia Detection and Imaging-Guided SurgeryIn Vivo. Anal Chem (2021) 93:1627–35. doi: 10.1021/acs.analchem.0c04101
27. Voskuil FJ, Steinkamp PJ, Zhao T, van der Vegt B, Koller M, Doff JJ, et al. Exploiting Metabolic Acidosis in Solid Cancers Using a Tumor-Agnostic pH-Activatable Nanoprobe for Fluorescence-Guided Surgery. Nat Commun (2020) 11:3257. doi: 10.1038/s41467-020-16814-4
28. Srinivasarao M, Galliford CV, Low PS. Principles in the Design of Ligand-Targeted Cancer Therapeutics and Imaging Agents. Nat Rev Drug Discovery (2015) 14:203–19. doi: 10.1038/nrd4519
29. Zhao H, Wu Y, Chen Y, Liu H. Clinical Significance of Hypoxia-Inducible Factor 1 and VEGF-A in Osteosarcoma. Int J Clin Oncol (2015) 20:1233–43. doi: 10.1007/s10147-015-0848-x
30. Papadopoulos N, Martin J, Ruan Q, Rafique A, Rosconi MP, Shi E, et al. Binding and Neutralization of Vascular Endothelial Growth Factor (VEGF) and Related Ligands by VEGF Trap, Ranibizumab and Bevacizumab. Angiogenesis (2012) 15:171–85. doi: 10.1007/s10456-011-9249-6
31. Nishio N, van den Berg NS, van Keulen S, Martin BA, Fakurnejad S, Zhou Q, et al. Optimal Dosing Strategy for Fluorescence-Guided Surgery With Panitumumab-IRDye800CW in Head and Neck Cancer. Mol Imaging Biol (2020) 22:156–64. doi: 10.1007/s11307-019-01358-x
32. Gao RW, Teraphongphom N, de Boer E, Berg NSVD, Divi V, Kaplan MJ, et al. Safety of Panitumumab-IRDye800CW and Cetuximab-IRDye800CW for Fluorescence-Guided Surgical Navigation in Head and Neck Cancers. Theranostics (2018) 8:2488–95. doi: 10.7150/thno.24487
33. Napier TS, Udayakumar N, Jani AH, Hartman YE, Houson HA, Moore L, et al. Comparison of Panitumumab-IRDye800CW and 5-Aminolevulinic Acid to Provide Optical Contrast in a Model of Glioblastoma Multiforme. Mol Cancer Ther (2020) 19:1922–9. doi: 10.1158/1535-7163.MCT-19-0819
34. Olson MT, Ly QP, Mohs AM. Fluorescence Guidance in Surgical Oncology: Challenges, Opportunities, and Translation. Mol Imaging Biol (2019) 21:200–18. doi: 10.1007/s11307-018-1239-2
35. Samkoe KS, Bates BD, Elliott JT, LaRochelle E, Gunn JR, Marra K, et al. Application of Fluorescence-Guided Surgery to Subsurface Cancers Requiring Wide Local Excision. Cancer Control (2018) 25:544015489. doi: 10.1177/1073274817752332
36. Xu X, Samkoe KS, Henderson ER. Effect of Preoperative Cancer Treatment on Epidermal Growth Factor Receptor (EGFR) Receptor Expression Level in ABY-029 Guided Sarcoma Surgery. Proc SPIE Int Soc Opt Eng (2020) 11222. doi: 10.1117/12.2546963
37. Samkoe KS, Gunn JR, Marra K, Hull SM, Moodie KL, Feldwisch J, et al. Toxicity and Pharmacokinetic Profile for Single-Dose Injection of ABY-029: A Fluorescent Anti-EGFR Synthetic Affibody Molecule for Human Use. Mol Imaging Biol (2017) 19:512–21. doi: 10.1007/s11307-016-1033-y
38. Zhao M, Ding J, Mao Q, Zhang Y, Gao Y, Ye S, et al. A Novel Alphavbeta3 Integrin-Targeted NIR-II Nanoprobe for Multimodal Imaging-Guided Photothermal Therapy of Tumors In Vivo. Nanoscale (2020) 12:6953–8. doi: 10.1039/c9nr10720g
39. Xu Y, Tuo W, Yang L, Sun Y, Li C, Chen X, et al. Design of a Metallacycle-Based Supramolecular Photosensitizer for In Vivo Image-Guided Photodynamic Inactivation of Bacteria. Angew Chem Int Ed Engl (2022) 61:e202110048. doi: 10.1002/anie.202110048
40. Jiang Y, Pang X, Liu R, Xiao Q, Wang P, Leung AW, et al. Design of an Amphiphilic iRGD Peptide and Self-Assembling Nanovesicles for Improving Tumor Accumulation and Penetration and the Photodynamic Efficacy of the Photosensitizer. ACS Appl Mater Interfaces (2018) 10:31674–85. doi: 10.1021/acsami.8b11699
41. Luengo MY, Ovejero PK, Lozano CL, Marciello M, Filice M. Recent Advances in Multimodal Molecular Imaging of Cancer Mediated by Hybrid Magnetic Nanoparticles. Polymers (Basel) (2021) 13. doi: 10.3390/polym13172989
42. Liu M, Anderson RC, Lan X, Conti PS, Chen K. Recent Advances in the Development of Nanoparticles for Multimodality Imaging and Therapy of Cancer. Med Res Rev (2020) 40:909–30. doi: 10.1002/med.21642
43. Shou K, Qu C, Sun Y, Chen H, Chen S, Zhang L, et al. Multifunctional Biomedical Imaging in Physiological and Pathological Conditions Using a NIR-II Probe. Adv Funct Mater (2017) 27:1700995. doi: 10.1002/adfm.201700995
44. Zhang X, Wang H, Antaris AL, Li L, Diao S, Ma R, et al. Traumatic Brain Injury Imaging in the Second Near-Infrared Window With a Molecular Fluorophore. Adv Mater (2016) 28:6872–9. doi: 10.1002/adma.201600706
45. Fourman MS, Mahjoub A, Mandell JB, Yu S, Tebbets JC, Crasto JA, et al. Quantitative Primary Tumor Indocyanine Green Measurements Predict Osteosarcoma Metastatic Lung Burden in a Mouse Model. Clin Orthop Relat Res (2018) 476:479–87. doi: 10.1007/s11999.0000000000000003
46. Mahjoub A, Morales-Restrepo A, Fourman MS, Mandell JB, Feiqi L, Hankins ML, et al. Tumor Resection Guided by Intraoperative Indocyanine Green Dye Fluorescence Angiography Results in Negative Surgical Margins and Decreased Local Recurrence in an Orthotopic Mouse Model of Osteosarcoma. Ann Surg Oncol (2019) 26:894–8. doi: 10.1245/s10434-018-07114-9
47. Bartholf DeWitt S, Eward WC, Eward CA, Lazarides AL, Whitley MJ, Ferrer JM, et al. A Novel Imaging System Distinguishes Neoplastic From Normal Tissue During Resection of Soft Tissue Sarcomas and Mast Cell Tumors in Dogs. Vet Surg (2016) 45:715–22. doi: 10.1111/vsu.12487
48. Prince AC, McGee AS, Siegel H, Rosenthal EL, Behnke NK, Warram JM. Evaluation of Fluorescence-Guided Surgery Agents in a Murine Model of Soft Tissue Fibrosarcoma. J Surg Oncol (2018) 117:1179–87. doi: 10.1002/jso.24950
49. Wang Y, Mai H, Yuan Y, Chen H, Wu S, Hu X, et al. EWS–FLI1-Targeting Peptide Identifies Ewing Sarcoma Tumor Boundaries and Lymph Node Metastasis via Near-Infrared Imaging. Mol Oncol (2021) 15:3706–20. doi: 10.1002/1878-0261.13081
50. Li X, Huang X, Zhang J, Huang H, Zhao L, Yu M, et al. A Novel Peptide Targets CD105 for Tumour Imaging In Vivo. Oncol Rep (2018) 40:2935–43. doi: 10.3892/or.2018.6643
51. Zhou H, Yi W, Li A, Wang B, Ding Q, Xue L, et al. Specific Small-Molecule NIR-II Fluorescence Imaging of Osteosarcoma and Lung Metastasis. Adv Healthc Mater (2019) 9:1901224. doi: 10.1002/adhm.201901224
52. Sardar HS, Zai Q, Xu X, Gunn JR, Pogue BW, Paulsen KD, et al. Dual-Agent Fluorescent Labeling of Soft-Tissue Sarcomas Improves the Contrast Based Upon Targeting Both Interstitial and Cellular Components of the Tumor Milieu. J Surg Oncol (2020) 122:1711–20. doi: 10.1002/jso.26190
53. Nicoli F, Saleh DB, Baljer B, Chan CD, Beckingsale T, Ghosh KM, et al. Intraoperative Near-Infrared Fluorescence (NIR) Imaging With Indocyanine Green (ICG) can Identify Bone and Soft Tissue Sarcomas Which may Provide Guidance for Oncological Resection. Ann Surg (2021) 273:e63–8. doi: 10.1097/SLA.0000000000003857
54. Mahalingam SM, Dudkin VY, Goldberg S, Klein D, Yi F, Singhal S, et al. Evaluation of a Centyrin-Based Near-Infrared Probe for Fluorescence-Guided Surgery of Epidermal Growth Factor Receptor Positive Tumors. Bioconjugate Chem (2017) 28:2865–73. doi: 10.1021/acs.bioconjchem.7b00566
55. Dobashi Y, Suzuki S, Sato E, Hamada Y, Yanagawa T, Ooi A. EGFR-Dependent and Independent Activation of Akt/mTOR Cascade in Bone and Soft Tissue Tumors. Modern Pathol (2009) 22:1328–40. doi: 10.1038/modpathol.2009.104
56. Bosma SE, van Driel PB, Hogendoorn PC, Dijkstra PS, Sier CF. Introducing Fluorescence Guided Surgery Into Orthopedic Oncology: A Systematic Review of Candidate Protein Targets for Ewing Sarcoma. J Surg Oncol (2018) 118:906–14. doi: 10.1002/jso.25224
57. Hassan SE, Bekarev M, Kim MY, Lin J, Piperdi S, Gorlick R, et al. Cell Surface Receptor Expression Patterns in Osteosarcoma. Cancer-Am Cancer Soc (2012) 118:740–9. doi: 10.1002/cncr.26339
58. Yang J, Zhang W. New Molecular Insights Into Osteosarcoma Targeted Therapy. Curr Opin Oncol (2013) 25:398–406. doi: 10.1097/CCO.0b013e3283622c1b
59. Dempsey GT, Vaughan JC, Chen KH, Bates M, Zhuang X. Evaluation of Fluorophores for Optimal Performance in Localization-Based Super-Resolution Imaging. Nat Methods (2011) 8:1027–36. doi: 10.1038/nmeth.1768
60. Xu Z, Wang Y, Han J, Xu Q, Ren J, Xu J, et al. Noninvasive Multimodal Imaging of Osteosarcoma and Lymph Nodes Using A99m Tc-Labeled Biomineralization Nanoprobe. Anal Chem (2018) 90:4529–34. doi: 10.1021/acs.analchem.7b04925
61. Lee J, Gordon AC, Kim H, Park W, Cho S, Lee B, et al. Targeted Multimodal Nano-Reporters for Pre-Procedural MRI and Intra-Operative Image-Guidance. Biomaterials (2016) 109:69–77. doi: 10.1016/j.biomaterials.2016.09.013
62. Kim C, Oh S, Kim S, Leem S, Heo J, Chung S. Correlation of IGF1R Expression With ABCG2 and CD44 Expressions in Human Osteosarcoma. Genes Genom (2018) 40:381–8. doi: 10.1007/s13258-017-0639-z
63. Kim MH, Kim S, Kim D. A Novel Dual-Modality Imaging Agent Targeting Folate Receptor of Tumor for Molecular Imaging and Fluorescence-Guided Surgery. Ann Nucl Med (2019) 33:606–16. doi: 10.1007/s12149-019-01369-2
64. Manca G, Garau LM, Mazzarri S, Mazzuca L, Muccioli S, Ghilli M, et al. Novel Experience in Hybrid Tracers. Clin Nucl Med (2021) 46:e181–7. doi: 10.1097/RLU.0000000000003478
65. Samkoe KS, Sardar HS, Gunn J, Feldwisch J, Linos K, Henderson E, et al. Measuring Microdose ABY-029 Fluorescence Signal in a Primary Human Soft-Tissue Sarcoma Resection. Proc SPIE Int Soc Opt Eng (2019) 10862. doi: 10.1117/12.2510935
66. Steinkamp PJ, Pranger BK, Li MF, Linssen MD, Voskuil FJ, Been LB, et al. Fluorescence-Guided Visualization of Soft-Tissue Sarcomas by Targeting Vascular Endothelial Growth Factor a: A Phase 1 Single-Center Clinical Trial. J Nucl Med (2021) 62:342–7. doi: 10.2967/jnumed.120.245696
67. Predina JD, Newton AD, Corbett C, Shin M, Sulfyok LF, Okusanya OT, et al. Near-Infrared Intraoperative Imaging for Minimally Invasive Pulmonary Metastasectomy for Sarcomas. J Thorac Cardiovasc Surg (2019) 157:2061–9. doi: 10.1016/j.jtcvs.2018.10.169
68. Scheichel F, Popadic B, Kitzwoegerer M, Ungersboeck K, Marhold F. Fluorescence-Guided Resection in Bone and Soft Tissue Infiltrating Meningiomas. Acta Neurochir (Wien) (2020) 162:605–11. doi: 10.1007/s00701-019-04179-7
69. Predina JD, Newton A, Deshpande C, Low P, Singhal S. Utilization of Targeted Near-Infrared Molecular Imaging to Improve Pulmonary Metastasectomy of Osteosarcomas. J BioMed Opt (2018) 23:1–4. doi: 10.1117/1.JBO.23.1.016005
70. Cahill RA. Ways of Seeing - It's All in the Image. Colorectal Dis (2018) 20:467–8. doi: 10.1111/codi.14265
71. Aoki T, Koizumi T, Sugimoto M, Murakami M. Holography-Guided Percutaneous Puncture Technique for Selective Near-Infrared Fluorescence-Guided Laparoscopic Liver Resection Using Mixed-Reality Wearable Spatial Computer. Surg Oncol (2020) 35:476–7. doi: 10.1016/j.suronc.2020.10.013
72. Cho HS, Park MS, Gupta S, Han I, Kim HS, Choi H, et al. Can Augmented Reality be Helpful in Pelvic Bone Cancer Surgery? An In Vitro Study. Clin Orthop Relat Res (2018) 476:1719–25. doi: 10.1007/s11999.0000000000000233
Keywords: fluorescence imaging, bone sarcoma, soft tissue sarcoma, fluorescent probes, cancer imaging
Citation: Chen Z, Huang H, He S, Wang Y, Cai L and Xie Y (2022) Progresses in Fluorescence Imaging Guidance for Bone and Soft Tissue Sarcoma Surgery. Front. Oncol. 12:879697. doi: 10.3389/fonc.2022.879697
Received: 20 February 2022; Accepted: 14 June 2022;
Published: 04 July 2022.
Edited by:
Haibin Shi, Soochow University, ChinaCopyright © 2022 Chen, Huang, He, Wang, Cai and Xie. This is an open-access article distributed under the terms of the Creative Commons Attribution License (CC BY). The use, distribution or reproduction in other forums is permitted, provided the original author(s) and the copyright owner(s) are credited and that the original publication in this journal is cited, in accordance with accepted academic practice. No use, distribution or reproduction is permitted which does not comply with these terms.
*Correspondence: Yuanlong Xie, eXVhbmxvbmd4aWVAd2h1LmVkdS5jbg==
Disclaimer: All claims expressed in this article are solely those of the authors and do not necessarily represent those of their affiliated organizations, or those of the publisher, the editors and the reviewers. Any product that may be evaluated in this article or claim that may be made by its manufacturer is not guaranteed or endorsed by the publisher.
Research integrity at Frontiers
Learn more about the work of our research integrity team to safeguard the quality of each article we publish.