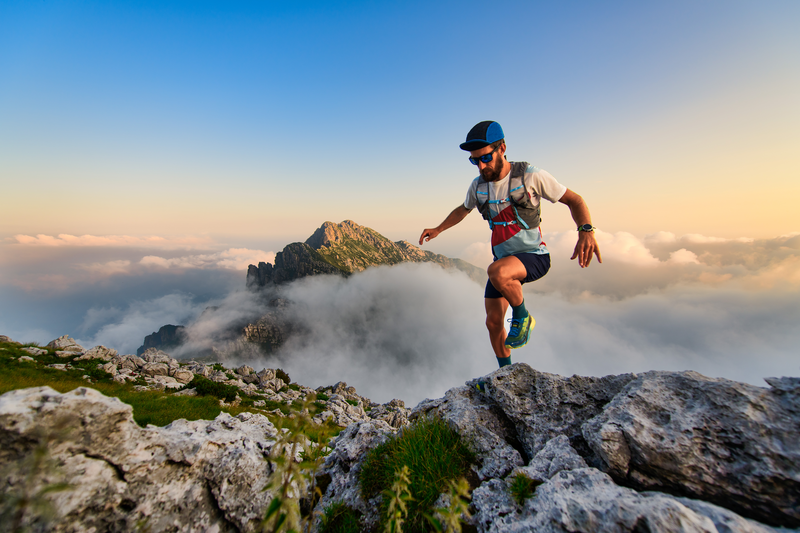
95% of researchers rate our articles as excellent or good
Learn more about the work of our research integrity team to safeguard the quality of each article we publish.
Find out more
REVIEW article
Front. Oncol. , 26 May 2022
Sec. Cancer Molecular Targets and Therapeutics
Volume 12 - 2022 | https://doi.org/10.3389/fonc.2022.874823
Non-small cell lung cancer (NSCLC) is the most common type of lung cancer and a common cause of cancer-related death. Better understanding of the molecular mechanisms, pathogenesis, and treatment of NSCLC can help improve patient outcomes. Significant progress has been made in the treatment of NSCLC, and immunotherapy can prolong patient survival. However, the overall cure and survival rates are low, especially in patients with advanced metastases. Interleukin-35 (IL-35), an immunosuppressive factor, is associated with the onset and prognosis of various cancers. Studies have shown that IL-35 expression is elevated in NSCLC, and it is closely related to the progression and prognosis of NSCLC. However, there are few studies on the mechanism of IL-35 in NSCLC. This study discusses the role of IL-35 and its downstream signaling pathways in the pathogenesis of NSCLC and provides new insights into its therapeutic potential.
Lung cancer (LC) is one of the deadliest cancers worldwide. In 2018, a global report suggested high incidence and mortality associated with LC, and important impact of LC on global health problems (1). It is classified into small-cell LC (SCLC, approximately 15% cases) and non-small-cell LC (NSCLC, approximately 85% cases) (2). Its etiology is multifactorial, and pathogenesis is incompletely understood. Available literature reveals that dysregulated inflammatory responses increase the risk of chronic diseases and cancers, including LC (3). Anti-inflammatory cytokines play an active role in reducing tumor growth, metastasis, apoptosis, and angiogenesis (4). Thus, studies evaluating LC pathogenesis and molecular mechanisms can provide a basis for identifying new biomarkers and developing targeted therapies.
LC and anticancer treatment result in airway obstruction and opportunistic infections, thereby increasing the morbidity and mortality (5, 6). Additionally, presence of febrile neutropenia in LC patients receiving myelosuppressive chemotherapy has the risk of bacterial infections. Thus, early diagnosis and treatment of these infections is crucial to improve the prognosis (7).
Interleukin 35 (IL-35) is a newly discovered member of the interleukin family and has been reported to have anti-inflammatory and immunoregulatory properties (8–10). Structurally, it is a heterodimer comprising of two subunits, EBI3 and IL-12p35 (11–14). Additionally, IL-35 receptor includes two subunits, IL-12Rβ2 and glycoprotein 130 (gp130). IL-35 mediates signaling through signal transducer and activator of transcription (STAT) 4, STAT1, and STAT4/STAT1 in the presence of subunits IL-12 Rβ2, gp130, and IL-12Rβ2/gp130, respectively (15).
IL-35 is overexpressed in prostate cancer (16), LC (17), gastric cancer (18), and hepatocellular carcinoma (19), and its overexpression is directly implicated in tumor progression and poor prognosis of LC (20), pancreatic cancer (21), hepatocellular carcinoma (22), breast cancer (23), renal cell carcinoma (24), laryngeal squamous cell carcinoma (25), osteosarcoma (26), and colorectal cancer (27). IL-35 levels are positively correlated with tumor stage (tumor size, metastasis to adjacent lymph nodes, and distant metastases) in pancreatic ductal adenocarcinoma (28), breast cancer (29, 30), acute myeloid leukemia (31), prostate cancer (32, 33), and colorectal cancer (27). Additionally, it promotes tumor growth as well as immune escape, and can be used as a prognostic indicator (10, 27, 28).
IL-35 limits anti-tumor immunity in the tumor microenvironment by regulating T cell responses (16, 34, 35). In breast cancer, it promotes tumor progression by inducing the conversion of conventional T cells to inducible Tregs (iTr35) (30). Additionally, it can recruit Treg cells in colorectal cancer (27), induce the accumulation of CD11b+ Gr1+ myeloid cells in the tumor microenvironment (36), stimulate angiogenesis, and reduce the infiltration of activated CD8+ T cells into the tumor (10).
In the tumor microenvironment, the relationship between inflammation and the immune system is very complex. The role of IL-35 in vivo (Table 1) and in vitro cellular level (Table 2) with autoimmune diseases, allergic diseases, and tumors has been extensively studied. It is an anti-inflammatory factor that inhibits Th2-type cytokine production in allergic rhinitis and asthma and reduces eosinophilic airway inflammation (37, 50). In systemic lupus erythematosus (SLE) (42), inflammatory bowel disease (45), collagen-induced arthritis (CIA), psoriasis (48), autoimmune uveitis (39), and other autoimmune diseases, IL-35 is involved in the development of disease by regulating the expression of inflammatory factors and immune response. In sepsis, it exerts anti-apoptotic and inflammatory effects (46). In sarcoidosis, it is associated with the inflammation of loose nodular granulomas, as well as increased Breg and decreased Treg in peripheral blood (38). In acute kidney injury, it exerts anti-inflammatory effects by decreasing the secretion of pro-inflammatory factors (47). Contrarily, in acute respiratory distress syndrome (ARDS), IL-35 appears to be protective, and lung injury is a result of its neutralization (44).
In liver cancer, IL-35 induces epithelial-mesenchymal transition (EMT) and mesenchymal-epithelial transition factor (MET) in macrophages with different polarization states, and promotes tumor progression (52). In pancreatic cancer, it promotes cell proliferation and inhibits apoptosis (21). In prostate cancer, it increases Treg expression, promotes proliferation of myeloid-derived inhibitory cells (MDSCs), angiogenesis, and tumor progression and inhibits CD4+ and CD8+ T lymphocyte levels (16).
IL-35 expression is increased in the serum and tumor tissue of NSCLC patients (17) and in bronchoalveolar lavage fluid (BALF) and serum of NSCLC patients undergoing immunotherapy (54), demonstrating that this cytokine can serve as a therapeutic target for NSCLC. The study by Zhang et al. showed that plasma IL-35 levels in NSCLC patients were significantly higher than those in healthy controls (55). Additionally, the overexpression of IL-35 was significantly correlated with prognostic factors such as T stage, lymph node metastasis, micro-vessel density, and tumor differentiation, and total survival time increased in patients with low expression of IL-35 (55). A recent study by Li et al. showed that plasma IL-35 in the stage IV NSCLC patients was higher than that of the healthy group, and its expression levels were higher in the cachexia group than that of the non-cachexia group. Another study demonstrated that IL-35 was significantly associated with skeletal muscle atrophy (56). This was further confirmed in a mouse model that elevated IL-35 levels can induce skeletal muscle atrophy and cachexia (56). Overall, IL-35 is a key regulator of the development and prognosis of NSCLC. Additionally, a study involving surgically managed NSCLC patients demonstrated that compared with healthy controls, serum IL-35 levels were increased in patients with lung adenocarcinoma (ADC) and decreased in patients with lung squamous cell carcinoma (SCC). ADC patients had increased IL-35-expressing cells in tumor areas compared to corresponding tumor-free control areas. In SCC patients, there was also a trend of increased IL-35 in the tumor region, but this did not reach statistically significant level (17). The CD4 mRNA in the tumor region of ADC and SCC patients is reported to be lower than that of the control group and peritumoral region, respectively (17). It is suggested that IL-35 exerts an immunosuppressive effect by inhibiting CD4+ T cell-mediated immune responses, and ADC is more immunosuppressive than SCC (17). Furthermore, toll-like receptor 4 promotes the expression of histone lysine demethylase 3A (KDM3A) in lung ADC cell line, KDM3A interacts with forkhead box protein 3 (Foxp3) and promotes the secretion of immunosuppressive factors such as IL-35, which promotes immune escape of lung ADC (57). While IL-35 may not affect the survival and death of lung cancer cells (17), it may be involved in the pathological process of the NSCLC by regulating the microenvironment and immune response. Contrarily, an in vitro study demonstrated that IL-35 overexpression inhibits cancer growth by promoting apoptosis and inducing cell cycle arrest (58). The discrepancy in the results between tumor types may be because IL-35 expression depends on tumor type, stage, and microenvironment. Further studies are required to elucidate the underlying mechanism of IL-35 in NSCLC. This study discusses the molecular role of IL-35 in NSCLC.
CD4+ T cells participate in anti-tumor immunity and prevent immune escape by regulating the immune response. According to the pattern of cytokine secretion, these cells are classified into Tregs and T helper types 1 (Th1), 2 (Th2), and 17 (Th17) (59–63). IL-35 is secreted by Treg cells and inhibits T cell proliferation and function (8, 11, 35). Tregs promote tumor growth by suppressing host immune responses and promoting immune escape via the expression of transforming growth factor β, IL-10, and IL-35 (64–67). The increase in Tregs promotes tumor recurrence and reduces survival, thereby worsening prognosis (22, 68, 69). In NSCLC patients with checkpoint inhibitor pneumonitis (CIP), IL-35 expression increases the number of Treg and Th1 cells and the Th1/Th2 ratio (54). The number of Treg cells is increased in NSCLC (70, 71) and is closely linked with clinical stage and prognosis (72). IL-35 improves the ability of Tregs to induce immunosuppression and help prevent diseases (8, 73, 74). It participates in the pathogenesis of colorectal cancer by increasing Tregs and recruiting these cells to the tumor site (27). Currently, the complex mechanism of immune escape mediated by Treg cells is incompletely understood. Thus, further understanding of the role of IL-35 and Tregs in NSCLC may provide new insights into NSCLC treatment.
Some immunological studies in NSCLC have focused on Th1 and Th2 cells, and related factors (75–78). Th2 cytokines are increased, while Th1 cytokines are decreased in the peripheral blood of NSCLC patients compared with healthy controls (75), and the Th1/Th2 ratio is negatively correlated with LC prognosis (79).
Th17 regulates the expression and secretion of IL-17 and other cytokines and participates in tumor pathogenesis (80, 81). Additionally, both Th17 and IL-17 play a fundamental role in LC immunity (82–84), and have an anti-tumor or pro-tumor effect depending on the type of cancer (85, 86). The reasons for this paradox are unclear and require further investigation. IL-17 promotes the growth of NSCLC by inducing tumor cell proliferation (87), blood vessel formation (88, 89), lymphangiogenesis (90), and tumor invasiveness. Serum IL-17 levels are increased in NSCLC patients and are an independent prognostic factor (91). Th17 cells exert anti-tumor effects indirectly by recruiting CD8+ T cells and other immune cells (92).
Imbalances between Treg and Th17 cells occur in NSCLC. The number of these cells, and Foxp3 and RORγt expression are reported to be higher in the peripheral blood of NSCLC patients than in healthy controls. The number of Th17 cells is inversely correlated with the number of Tregs (93). The TregFoxp3+/Th17 ratio is valuable for diagnosing NSCLC and increases with increase in tumor stage (84).
IL-35 promotes tumor immune escape by increasing the number of IL-35producing iTr35 cells (30, 34, 73, 94). It also plays an immunosuppressive effect by promoting Treg cell proliferation and inhibiting Th17 cell differentiation (11, 95). These findings suggest that IL-35 is closely linked to Treg and Th17 cells in NSCLC; however, the mechanisms underlying this correlation remain unclear.
Therapeutic monoclonal antibodies targeting programmed cell death protein 1 (PD-1) or programmed cell death protein ligand 1 (PD-L1) can effectively treat NSCLC (96–98). PD-1 is expressed in B cells, T cells, and natural killer T cells (NK) (99). The role of PD-1/PD-L1 in CD8+ T cell failure has been elucidated (100–103). Anti-PD-1 therapy induces the expansion of specific subsets of exhausted CD8+ T cells that infiltrate the tumor (104), and inhibits CD8+ T cell-mediated tumor growth (105). The interaction between PD-1 and PD-L1 reduces the susceptibility of tumor cells to T cell cytotoxicity (106–108). The number of IL-35+ Foxp3+ T cells is positively associated with the number of thyroid transcription factor 1+ PD-L1+ cells in NSCLC (17). Treg-derived IL-35 induces the expression of PD-1 and other inhibitory molecules, and impairs T cell function in the tumor environment, thereby leading to tumor growth (35). Thus, blocking the PD-1/PD-L1 interaction can enhance the anti-tumor response by reducing the inhibitory activity of Tregs (109). Additionally, the IL-35 inhibitors can reduce the expression of PD-1 and other inhibitory cytokines and restore the anti-tumor immune activity of T cells. IL-35 participates in immunosuppression, and its expression is increased in PD-L1+ cells in NSCLC patients (17). Th1/Th2 cell and Th17/Treg cell ratios are unbalanced in NSCLC patients with CIP undergoing anti-PD-1/PD-L1 immunotherapy, leading to the increased secretion of IL-17A and IL-35 in BALF and serum (54). Thus, IL-35 inhibitors and PD-1/PD-L1 combination therapy may have a synergistic effect on NSCLC (17). Additionally, IL-35 can be used to assess the severity and improvement of CIP in NSCLC patients during immunotherapy (54). The roles of IL-35 in NSCLC are illustrated in Figure 1.
Figure 1 Potential role of IL-35 in non-small cell lung cancer. 1. Foxp3 targets the EBI3 subunit of IL-35 and induces tumor growth and metastasis by activating the Wnt/β-catenin signaling pathway. 2. IL-35 induces the conversion of conventional T cells to iTr35 through the STAT1/STAT3 pathway. 3. EBI3 promotes tumor growth through the gp130-STAT3 signaling. 4. Tumor-associated macrophages secrete IL-35 and promote metastatic colonization by regulating epithelial-mesenchymal transition through the JAK2-STAT6-GATA3 signaling pathway. 5. IL-35 is secreted by Treg cells, and IL-35 produced by cancer cells recruits Treg cells and induces tumor growth. 6. Imbalances between Treg and Th17 cells. IL-35 plays an immunosuppressive effect by promoting Treg proliferation and inhibiting Th17 cell differentiation. 7. IL-35 produced by Treg cells induces the expression of PD-1 and other inhibitory cytokines, impairing T cell function in the tumor environment and promoting tumor growth.
EMT is a complex process of phenotypic transition from epithelial cells to mesenchymal cells, while MET is the reverse transformation of the above phenotype (110). EMT plays a critical role in the occurrence, development, and treatment of resistant NSCLC (111, 112). Inflammatory cytokines promote the occurrence of EMT and advanced tumor progression. IL-35 has been shown to promote EMT and MET in different polarization states. In liver cancer, M1 macrophages secrete IL-35 to promote EMT through STAT3, and IL-35 leads to MET through M2 macrophage polarization, creating conditions for liver cancer progression (52). Interestingly, IL-35 secreted by tumor-associated macrophages can reverse EMT-promoted metastatic tumor colonization (113).
IL-35 inhibits PD-L1 expression in serum-starved ADC tumor cells (17). It has been shown to promote or reverse EMT in specific contexts, and EMT induces elevated PD-L1 expression in LC A549 cells (114). PD-1/PD-L1 blockade immunotherapy may be more effective in lung ADC patients with EMT phenotype (115). Interestingly, studies have shown that reversing EMT to a more epithelial phenotype contributes to increased responsiveness to immune checkpoint inhibitor therapy (116). Uncovering the complex mechanism of action of IL-35 on EMT and PD-L1 in NSCLC may help guide the treatment of LC.
Targeted drug therapy is a promising area for NSCLC, but drug resistance presents challenges for targeted therapy. EMT is associated with multiple targeted drug resistance mechanisms. Epidermal growth factor receptor (EGFR) tyrosine kinase inhibitor (TKI) is mainly aimed at the target of EGFR mutation gene in LC, but drug resistance is more common. EMT is a common mechanism of resistance to EGFR-TKI targeted therapy in LC (117). Reversal of EMT helps restore sensitivity to EGFR-TKI therapy in NSCLC patients (118). For anaplastic lymphoma kinase (ALK) rearranged NSCLC, ALK inhibitors are effective drugs. EMT is one of the mechanisms of drug resistance in ALK-TKI treatment of NSCLC patients (119). Additionally, hypoxia induced EMT resistance to ALK inhibitors with echinoderm microtubule-associated protein-like 4-ALK rearrangement (120). IL-35 can promote or reverse EMT in specific tumor microenvironments. Based on the complexity of the mechanism of NSCLC, IL-35 may regulate the molecular mechanism of NSCLC and the effect of targeted therapy drugs through various mechanisms, which is worth further exploration.
Immunotherapy including ICI against PD-1/PD-L1 is a promising treatment for LC. Available literature suggests that baseline systemic inflammatory markers and cytokines prior to treatment can predict ICI treatment effect and patient prognosis (121). Higher baseline inflammation is associated with poor prognosis (122, 123). Pre-treatment high inflammatory state and high levels of IL-6, and IL-8 cytokines are poor prognostic indicators of PD-1 inhibitor therapy, and high levels of IFN-γ are markers of good ICI treatment effect and prognosis (121). Additionally, high baseline levels of C-reactive protein, erythrocyte sedimentation rate, and procalcitonin during ICI treatment of NSCLC indicate poor prognosis (124). A series of adverse events, including CIP, can occur with ICI treatment. Pretreatment with COPD, high expression of PD-L1, and high baseline IL-8 levels are reported to be the risk factors for CIP (125). A study by Wang et al. demonstrated that serum IL-17A and IL-35 levels are significantly raised at the time of CIP diagnosis compared with those prior to treatment, and significantly decreased after clinical recovery. IL-17A and IL-35 were also increased during CIP in BALF. IL-35 was associated with changes in T lymphocyte subsets during the development of CIP. Thus, it is suggested that IL-35 may play a key role in the regulation of T-cell immune responses in CIP (54). However, at present, there are limited studies evaluating the effect of baseline systemic inflammatory markers combined with cytokine IL-35 on the treatment response and prognosis in NSCLC patients receiving ICI therapy. Further prospective studies are required to assess the molecular mechanism and clarify the role of IL-35 in ICI therapy. Relationship of IL-35 with baseline inflammation and immune modulation will help identify immunotherapy response effects and impact on patient outcomes.
The function of IL-35 is enigmatic, and its mechanistic studies in NSCLC are currently in the initial stage and have not yet reached the clinical trial stage. IL-35 has the potential to promote tumor development in NSCLC, and it has a central role in EMT, tumor resistance, and PD-1/PD-L1. At present, little is known about the kinetics of IL-35 during NSCLC chemotherapy or ICI treatment. Turnis et al. blocked anti-IL-35 and anti-PD1 in a tumor model, but showed no increase in tumor clearance, suggesting that they may in part be acting on the same pathway (35). Liao et al. established an extended model to explore the mode of action of anti-IL-35 therapy in a cancer model, and demonstrated that continuous injection was more effective than intermittent injection (10). Anti-IL-35 combined with ICI therapy provides a good prospect for the treatment of NSCLC.
The Wnt/β-catenin signaling pathway is conserved and closely related to embryonic development, homeostasis, and cancer (126), including NSCLC (127–129). The activity of this pathway depends on the cellular localization of β-catenin (127). Wnt-1 expression is positively correlated with the expression of c-Myc, cyclin D1, VEGF-A, MMP-7, and Ki-67 index, and with a poor prognosis of NSCLC (130, 131).
The expression of Foxp3 is upregulated in NSCLC and induces tumor growth and metastasis by stimulating the Wnt/β-catenin signaling pathway (132). Foxp3 induces EMT, tumor metastasis and growth, and reduces overall and recurrence-free survival, thereby worsening the prognosis (132). IL-35, produced by Foxp3-expressing Tregs, and the EBI3 subunit of IL-35, is a downstream target of Foxp3 (8). The serum levels of EBI3 are increased in LC patients, resulting in poor prognosis (20). Thus, IL-35 and Wnt/β-catenin may be useful diagnostic biomarkers for NSCLC. We hypothesize that IL-35 and the Wnt/β-catenin signaling pathway are closely related to the occurrence and development of NSCLC. However, additional studies are necessary to elucidate the underlying mechanisms.
The IL-35 subunit EBI3 regulates the differentiation of T and B cells through the gp130-STAT3 pathway (133). STAT3 is activated in LC (134). It plays a dual role by inhibiting tumor cell growth and promoting metastasis in LC patients (135). Its increased expression promotes tumor progression, invasion, and metastasis (136), leading to poor prognosis (134, 137, 138). In coronary artery disease, IL-35 improves the function of B cells by suppressing the expression of IFN-γ and TNF by T cells, and the STAT3 signaling pathway may be involved in the suppression of T cell-mediated inflammation (139). IL-35 inhibits the differentiation and maturation of dendritic cells derived from monocytes via the STAT1/STAT3 and MAPK/NF-KB signaling pathways (140). In colorectal cancer, the expression of EBI3, gp130, and pSTAT3 is upregulated, and EBI3 promotes tumor growth through the gp130-STAT3 signaling pathway (141). In breast cancer, IL-35 induces the conversion of conventional T cells to iTr35 via the STAT1/STAT3 pathway (30). In rheumatoid arthritis, IL-35 inhibits angiogenesis through the Janus-related kinase (JAK)-STAT1 signaling pathway (142). However, whether IL-35 promotes angiogenesis in LC and other cancers is unknown. IL-35 is expressed in the trophoblasts of pregnant women and maintains maternal-fetal tolerance, probably via STAT1 and STAT3 (143). IL-35 protects against cardiac ischemia-reperfusion injury by reducing cardiomyocyte damage through the gp130-STAT3 signaling axis (144). These findings suggest that IL-35 and other factors in the STAT signaling pathway may serve as therapeutic targets for LC.
EMT stimulates the metastasis of a variety of cancers (145, 146), such as colorectal cancer (147), bladder cancer (148), squamous cell carcinoma of the head and neck (149), and NSCLC (150, 151). Additionally, tumor-associated macrophages secrete IL-35 and promote metastatic colonization by regulating EMT through the activation of JAK2-STAT6-GATA3 signaling (113). However, the role of IL-35 and EMT in NSCLC is incompletely understood and warrants further research.
IL-35 is an immunosuppressive factor strongly implicated in the development, treatment, and prognosis of cancers, including NSCLC. Based on the complexity of the mechanism of NSCLC, IL-35 may regulate the microenvironment and participate in immune escape and immunosuppression through various mechanisms, and more research is needed in the future. Thus, elucidating the role of IL-35 and its downstream signaling pathways in NSCLC may help guide individualized treatment and improve patient outcomes.
HD and YH drafted and revised the manuscript. WL, XL, and BS collected information and prepared the figures. PG conceived and designed the study. All authors contributed to the article and approved the submitted version.
The authors declare that the research was conducted in the absence of any commercial or financial relationships that could be construed as a potential conflict of interest.
All claims expressed in this article are solely those of the authors and do not necessarily represent those of their affiliated organizations, or those of the publisher, the editors and the reviewers. Any product that may be evaluated in this article, or claim that may be made by its manufacturer, is not guaranteed or endorsed by the publisher.
ADC, adenocarcinoma; AKI, acute kidney injury; ALK, anaplastic lymphoma kinase; ARDS, acute respiratory distress syndrome; CIA, collagen-induced arthritis; CIP, checkpoint inhibitor pneumonitis; COX-2, cyclooxygenase-2; DNP, diabetic neuropathic pain; EBI3, Epstein-Barr virus (EBV)-induced gene 3; EGFR, Epidermal growth factor receptor; EMT, epithelial-mesenchymal transition; FLS, fibroblast-like synoviocyte; Foxp3, forkhead box protein 3; gp130, glycoprotein 130; GATA 3, GATA binding protein 3; HNECs, human nasal epithelial cells; HUVECs, human umbilical vein endothelial cells; ICAM-1, intercellular adhesion molecule-1; ICI, immune checkpoint inhibition; IFN-γ, Interferon-gamma; ILC2, II innate lymphoid cells; IL-10, interleukin 10; IL-12, interleukin-12; IL-12Rβ2, interleukin 12 receptor β2 subunit; IL-35, interleukin-35; iNOS, Inducible nitric oxide synthase; JAK, Janus-related kinase; JNK, Jun N-terminal kinase; KDM3A, histone lysine demethylase 3A; LPS, Lipopolysaccharide; MAPK, mitogen-activated protein kinases; MDSCs, myeloid-derived inhibitory cells; MET, mesenchymal-epithelial transition factor; NK, natural killer T cells; NSCLC, non-small cell lung cancer; PBMCs, peripheral blood mononuclear cells; PD-1, programmed cell death protein; PD-L1, pIL-35, plasmid-IL-35; programmed cell death protein ligand 1; rIL-35, recombinant IL-35; rhIL-35, recombinant human IL-35; SCC, squamous cell carcinoma; SLE, systemic lupus erythematosus; STAT, signal transducer and activator of transcription; Tregs, regulatory T cells; TSLP, thymic stromal lymphopoietin; Th1, T helper type 1; Th2, T helper type 2; Th17, T helper type 17; TGF-β, tumor necrosis factor beta; TKI, tyrosine kinase inhibitor; TNF-α, tumor Necrosis Factor alpha; TTF, thyroid transcription factor; VCAM-1, vascular cellular adhesion molecule-1.
1. Bray F, Ferlay J, Soerjomataram I, Siegel RL, Torre LA, Jemal A. Global Cancer Statistics 2018: GLOBOCAN Estimates of Incidence and Mortality Worldwide for 36 Cancers in 185 Countries. CA: Cancer J Clin (2018) 68(6):394–424. doi: 10.3322/caac.21492
2. Blandin Knight S, Crosbie PA, Balata H, Chudziak J, Hussell T, Dive C. Progress and Prospects of Early Detection in Lung Cancer. Open Biol (2017) 7(9):170070. doi: 10.1098/rsob.170070
3. Dougan M, Li D, Neuberg D, Mihm M, Googe P, Wong K-K, et al. A Dual Role for the Immune Response in a Mouse Model of Inflammation-Associated Lung Cancer. J Clin Invest (2011) 121(6):2436–46. doi: 10.1172/JCI44796
4. Zhong Z, Sanchez-Lopez E, Karin M. Autophagy, Inflammation, and Immunity: A Troika Governing Cancer and Its Treatment. Cell (2016) 166(2):288–98. doi: 10.1016/j.cell.2016.05.051
5. Akinosoglou KS, Karkoulias K, Marangos M. Infectious Complications in Patients With Lung Cancer. Eur Rev Med Pharmacol Sci (2013) 17(1):8–18.
7. Lanoix JP, Pluquet E, Lescure FX, Bentayeb H, Lecuyer E, Boutemy M, et al. Bacterial Infection Profiles in Lung Cancer Patients With Febrile Neutropenia. BMC Infect Dis (2011) 11:183. doi: 10.1186/1471-2334-11-183
8. Collison LW, Workman CJ, Kuo TT, Boyd K, Wang Y, Vignali KM, et al. The Inhibitory Cytokine IL-35 Contributes to Regulatory T-Cell Function. Nature (2007) 450(7169):566–9. doi: 10.1038/nature06306
9. Chaturvedi V, Collison LW, Guy CS, Workman CJ, Vignali DAA. Cutting Edge: Human Regulatory T Cells Require IL-35 to Mediate Suppression and Infectious Tolerance. J Immunol (Baltimore Md 1950) (2011) 186(12):6661–6. doi: 10.4049/jimmunol.1100315
10. Liao K-L, Bai X-F, Friedman A. Mathematical Modeling of Interleukin-35 Promoting Tumor Growth and Angiogenesis. PloS One (2014) 9(10):e110126. doi: 10.1371/journal.pone.0110126
11. Niedbala W, Wei X-Q, Cai B, Hueber AJ, Leung BP, McInnes IB, et al. IL-35 is a Novel Cytokine With Therapeutic Effects Against Collagen-Induced Arthritis Through the Expansion of Regulatory T Cells and Suppression of Th17 Cells. Eur J Immunol (2007) 37(11):3021–9. doi: 10.1002/eji.200737810
12. Devergne O, Birkenbach M, Kieff E. Epstein-Barr Virus-Induced Gene 3 and the P35 Subunit of Interleukin 12 Form a Novel Heterodimeric Hematopoietin. Proc Natl Acad Sci USA (1997) 94(22):12041–6. doi: 10.1073/pnas.94.22.12041
13. Su L-C, Liu X-Y, Huang A-F, Xu W-D. Emerging Role of IL-35 in Inflammatory Autoimmune Diseases. Autoimmun Rev (2018) 17(7):665–73. doi: 10.1016/j.autrev.2018.01.017
14. Dambuza IM, He C, Choi JK, Yu C-R, Wang R, Mattapallil MJ, et al. IL-12p35 Induces Expansion of IL-10 and IL-35-Expressing Regulatory B Cells and Ameliorates Autoimmune Disease. Nat Commun (2017) 8(1):719. doi: 10.1038/s41467-017-00838-4
15. Collison LW, Delgoffe GM, Guy CS, Vignali KM, Chaturvedi V, Fairweather D, et al. The Composition and Signaling of the IL-35 Receptor are Unconventional. Nat Immunol (2012) 13(3):290–9. doi: 10.1038/ni.2227
16. Zhu J, Wang Y, Li D, Zhang H, Guo Z, Yang X. Interleukin-35 Promotes Progression of Prostate Cancer and Inhibits Anti-Tumour Immunity. Cancer Cell Int (2020) 20:487. doi: 10.1186/s12935-020-01583-3
17. Heim L, Kachler K, Siegmund R, Trufa DI, Mittler S, Geppert CI, et al. Increased Expression of the Immunosuppressive Interleukin-35 in Patients With Non-Small Cell Lung Cancer. Br J Cancer (2019) 120(9):903–12. doi: 10.1038/s41416-019-0444-3
18. Gu JH, Wang XG, Wang LQ, Zhou LN, Tang M, Li P, et al. Serum Level of Interleukin-35 as a Potential Prognostic Factor for Gastric Cancer. Asia-Pacific J Clin Oncol (2020) 17(1):52–9. doi: 10.1111/ajco.13403
19. Liu X, Ren H, Guo H, Wang W, Zhao N. Interleukin-35 has a Tumor-Promoting Role in Hepatocellular Carcinoma. Clin Exp Immunol (2020) 203(2):219–29. doi: 10.1111/cei.13535
20. Nishino R, Takano A, Oshita H, Ishikawa N, Akiyama H, Ito H, et al. Identification of Epstein-Barr Virus-Induced Gene 3 as a Novel Serum and Tissue Biomarker and a Therapeutic Target for Lung Cancer. Clin Cancer Res (2011) 17(19):6272–86. doi: 10.1158/1078-0432.CCR-11-0060
21. Nicholl MB, Ledgewood CL, Chen X, Bai Q, Qin C, Cook KM, et al. IL-35 Promotes Pancreas Cancer Growth Through Enhancement of Proliferation and Inhibition of Apoptosis: Evidence for a Role as an Autocrine Growth Factor. Cytokine (2014) 70(2):126–33. doi: 10.1016/j.cyto.2014.06.020
22. Fu Y-P, Yi Y, Cai X-Y, Sun J, Ni X-C, He H-W, et al. Overexpression of Interleukin-35 Associates With Hepatocellular Carcinoma Aggressiveness and Recurrence After Curative Resection. Br J Cancer (2016) 114(7):767–76. doi: 10.1038/bjc.2016.47
23. Chen G, Liang Y, Guan X, Chen H, Liu Q, Lin B, et al. Circulating Low IL-23: IL-35 Cytokine Ratio Promotes Progression Associated With Poor Prognosisin Breast Cancer. Am J Transl Res (2016) 8(5):2255–64.
24. Jin L, Xu X, Ye B, Pan M, Shi Z, Hu Y. Elevated Serum Interleukin-35 Levels Correlate With Poor Prognosis in Patients With Clear Cell Renal Cell Carcinoma. Int J Clin Exp Med (2015) 8(10):18861–6.
25. Wu W, Jiang H, Li Y, Yan M-X. IL-35 Expression is Increased in Laryngeal Squamous Cell Carcinoma and in the Peripheral Blood of Patients. Oncol Lett (2017) 13(5):3303–8. doi: 10.3892/ol.2017.5858
26. Liu M-X, Liu Q-Y, Liu Y, Cheng Z-M, Liu L, Zhang L, et al. Interleukin-35 Suppresses Antitumor Activity of Circulating CD8 T Cells in Osteosarcoma Patients. Connect Tissue Res (2019) 60(4):367–75. doi: 10.1080/03008207.2018.1552267
27. Zeng J-C, Zhang Z, Li T-Y, Liang Y-F, Wang H-M, Bao J-J, et al. Assessing the Role of IL-35 in Colorectal Cancer Progression and Prognosis. Int J Clin Exp Pathol (2013) 6(9):1806–16.
28. Jin P, Ren H, Sun W, Xin W, Zhang H, Hao J. Circulating IL-35 in Pancreatic Ductal Adenocarcinoma Patients. Hum Immunol (2014) 75(1):29–33. doi: 10.1016/j.humimm.2013.09.018
29. Zhao Z, Chen X, Hao S, Jia R, Wang N, Chen S, et al. Increased Interleukin-35 Expression in Tumor-Infiltrating Lymphocytes Correlates With Poor Prognosis in Patients With Breast Cancer. Cytokine (2017) 89:76–81. doi: 10.1016/j.cyto.2016.09.012
30. Hao S, Chen X, Wang F, Shao Q, Liu J, Zhao H, et al. Breast Cancer Cell-Derived IL-35 Promotes Tumor Progression via Induction of IL-35-Producing Induced Regulatory T Cells. Carcinogenesis (2018) 39(12):1488–96. doi: 10.1093/carcin/bgy136
31. Tao Q, Pan Y, Wang Y, Wang H, Xiong S, Li Q, et al. Regulatory T Cells-Derived IL-35 Promotes the Growth of Adult Acute Myeloid Leukemia Blasts. Int J Cancer (2015) 137(10):2384–93. doi: 10.1002/ijc.29563
32. Zhu J, Yang X, Wang Y, Zhang H, Guo Z. Interleukin-35 is Associated With the Tumorigenesis and Progression of Prostate Cancer. Oncol Lett (2019) 17(6):5094–102. doi: 10.3892/ol.2019.10208
33. Chatrabnous N, Ghaderi A, Ariafar A, Razeghinia MS, Nemati M, Jafarzadeh A. Serum Concentration of Interleukin-35 and its Association With Tumor Stages and FOXP3 Gene Polymorphism in Patients With Prostate Cancer. Cytokine (2019) 113:221–7. doi: 10.1016/j.cyto.2018.07.006
34. Xue W, Yan D, Kan Q. Interleukin-35 as an Emerging Player in Tumor Microenvironment. J Cancer (2019) 10(9):2074–82. doi: 10.7150/jca.29170
35. Turnis ME, Sawant DV, Szymczak-Workman AL, Andrews LP, Delgoffe GM, Yano H, et al. Interleukin-35 Limits Anti-Tumor Immunity. Immunity (2016) 44(2):316–29. doi: 10.1016/j.immuni.2016.01.013
36. Wang Z, Liu JQ, Liu Z, Shen R, Zhang G, Xu J, et al. Tumor-Derived IL-35 Promotes Tumor Growth by Enhancing Myeloid Cell Accumulation and Angiogenesis. J Immunol (Baltimore Md 1950) (2013) 190(5):2415–23. doi: 10.4049/jimmunol.1202535
37. Nie M, Zeng Q, Xi L, Tang Y, Luo R, Liu W. The Effect of IL-35 on the Expression of Nasal Epithelial-Derived Proinflammatory Cytokines. Mediators Inflammation (2021) 2021:1110671. doi: 10.1155/2021/1110671
38. Mengmeng Z, Jiacui S, Shanshan D, Yuan Z, Ying Z, Qiuhong L, et al. Serum IL-35 Levels Are Associated With Activity and Progression of Sarcoidosis. Front Immunol (2020) 11:977. doi: 10.3389/fimmu.2020.00977
39. Kang M, Choi JK, Jittayasothorn Y, Egwuagu CE. Interleukin 35-Producing Exosomes Suppress Neuroinflammation and Autoimmune Uveitis. Front Immunol (2020) 11:1051. doi: 10.3389/fimmu.2020.01051
40. Jiang Y, Wang J, Li H, Xia L. IL-35 Promotes Microglial M2 Polarization in a Rat Model of Diabetic Neuropathic Pain. Arch Biochem Biophysics (2020) 685:108330. doi: 10.1016/j.abb.2020.108330
41. Jiang Y, Wang J, Li H, Xia L. IL-35 Alleviates Inflammation Progression in a Rat Model of Diabetic Neuropathic Pain via Inhibition of JNK Signaling. J Inflamm (London England) (2019) 16:19. doi: 10.1186/s12950-019-0217-z
42. Cai Z, Zhang S, Wu P, Ren Q, Wei P, Hong M, et al. A Novel Potential Target of IL-35-Regulated JAK/STAT Signaling Pathway in Lupus Nephritis. Clin Trans Med (2021) 11(2):e309. doi: 10.1002/ctm2.309
43. Peng M, Qiang L, Xu Y, Li C, Li T, Wang J. IL-35 Ameliorates Collagen-Induced Arthritis by Promoting TNF-α-Induced Apoptosis of Synovial Fibroblasts and Stimulating M2 Macrophages Polarization. FEBS J (2019) 286(10):1972–85. doi: 10.1111/febs.14801
44. Wang CJ, Zhang M, Wu H, Lin SH, Xu F. IL-35 Interferes With Splenic T Cells in a Clinical and Experimental Model of Acute Respiratory Distress Syndrome. Int Immunopharmacol (2019) 67:386–95. doi: 10.1016/j.intimp.2018.12.024
45. Wang Y, Mao Y, Zhang J, Shi G, Cheng L, Lin Y, et al. IL-35 Recombinant Protein Reverses Inflammatory Bowel Disease and Psoriasis Through Regulation of Inflammatory Cytokines and Immune Cells. J Cell Mol Med (2018) 22(2):1014–25. doi: 10.1111/jcmm.13428
46. Li M, Liu Y, Fu Y, Gong R, Xia H, Huang X, et al. Interleukin-35 Inhibits Lipopolysaccharide-Induced Endothelial Cell Activation by Downregulating Inflammation and Apoptosis. Exp Cell Res (2021) 407(2):112784. doi: 10.1016/j.yexcr.2021.112784
47. Hu L, Chen C, Zhang J, Wu K, Zhang X, Liu H, et al. IL-35 Pretreatment Alleviates Lipopolysaccharide-Induced Acute Kidney Injury in Mice by Inhibiting NF-κb Activation. Inflammation (2017) 40(4):1393–400. doi: 10.1007/s10753-017-0582-9
48. Zhang J, Lin Y, Li C, Zhang X, Cheng L, Dai L, et al. IL-35 Decelerates the Inflammatory Process by Regulating Inflammatory Cytokine Secretion and M1/M2 Macrophage Ratio in Psoriasis. J Immunol (Baltimore Md 1950) (2016) 197(6):2131–44. doi: 10.4049/jimmunol.1600446
49. Wang W, Zhao N, Li B, Gao H, Yan Y, Guo H. Inhibition of Cardiac Allograft Rejection in Mice Using Interleukin-35-Modified Mesenchymal Stem Cells. Scandinavian J Immunol (2019) 89(4):e12750. doi: 10.1111/sji.12750
50. Dong J, Wong CK, Cai Z, Jiao D, Chu M, Lam CW. Amelioration of Allergic Airway Inflammation in Mice by Regulatory IL-35 Through Dampening Inflammatory Dendritic Cells. Allergy (2015) 70(8):921–32. doi: 10.1111/all.12631
51. Xie F, Hu Q, Cai Q, Yao R, Ouyang S. IL-35 Inhibited Th17 Response in Children With Allergic Rhinitis. ORL J Oto-rhino-laryngol Related Specialties (2020) 82(1):47–52. doi: 10.1159/000504197
52. He Y, Pei JH, Li XQ, Chi G. IL-35 Promotes EMT Through STAT3 Activation and Induces MET by Promoting M2 Macrophage Polarization in HCC. Biochem Biophys Res Commun (2021) 559:35–41. doi: 10.1016/j.bbrc.2021.04.050
53. Wang Y, Yu Y, Yu W, Bian X, Gong L. IL-35 Inhibits Cell Pyroptosis and Attenuates Cell Injury in TNF-α-Induced Bronchial Epithelial Cells via P38 MAPK Signaling Pathway. Bioengineered (2022) 13(1):1758–66. doi: 10.1080/21655979.2021.2022266
54. Wang YN, Lou DF, Li DY, Jiang W, Dong JY, Gao W, et al. Elevated Levels of IL-17A and IL-35 in Plasma and Bronchoalveolar Lavage Fluid are Associated With Checkpoint Inhibitor Pneumonitis in Patients With non-Small Cell Lung Cancer. Oncol Lett (2020) 20(1):611–22. doi: 10.3892/ol.2020.11618
55. Zhang T, Nie J, Liu X, Han Z, Ding N, Gai K, et al. Correlation Analysis Among the Level of IL-35, Microvessel Density, Lymphatic Vessel Density, and Prognosis in Non-Small Cell Lung Cancer. Clin Trans Sci (2021) 14(1):389–94. doi: 10.1111/cts.12891
56. Li Z, Zhu L, Zheng H, Jiang W, Wang Y, Jiang Z, et al. Serum IL-35 Levels is a New Candidate Biomarker of Cancer-Related Cachexia in Stage IV non-Small Cell Lung Cancer. Thorac Cancer (2022) 13(5):716–23. doi: 10.1111/1759-7714.14307
57. Li Y, Yang W, Wu B, Liu Y, Li D, Guo Y, et al. KDM3A Promotes Inhibitory Cytokines Secretion by Participating in TLR4 Regulation of Foxp3 Transcription in Lung Adenocarcinoma Cells. Oncol Lett (2017) 13(5):3529–37. doi: 10.3892/ol.2017.5949
58. Long J, Zhang X, Wen M, Kong Q, Lv Z, An Y, et al. IL-35 Over-Expression Increases Apoptosis Sensitivity and Suppresses Cell Growth in Human Cancer Cells. Biochem Biophys Res Commun (2013) 430(1):364–9. doi: 10.1016/j.bbrc.2012.11.004
59. Abbas AK, Murphy KM, Sher A. Functional Diversity of Helper T Lymphocytes. Nature (1996) 383(6603):787–93. doi: 10.1038/383787a0
60. Zhu J, Yamane H, Paul WE. Differentiation of Effector CD4 T Cell Populations (*). Annu Rev Immunol (2010) 28:445–89. doi: 10.1146/annurev-immunol-030409-101212
61. Tanaka A, Sakaguchi S. Targeting Treg Cells in Cancer Immunotherapy. Eur J Immunol (2019) 49(8):1140–6. doi: 10.1002/eji.201847659
62. Wei WZ, Morris GP, Kong YC. Anti-Tumor Immunity and Autoimmunity: A Balancing Act of Regulatory T Cells. Cancer Immunol Immunother CII (2004) 53(2):73–8. doi: 10.1007/s00262-003-0444-1
63. Vinay DS, Ryan EP, Pawelec G, Talib WH, Stagg J, Elkord E, et al. Immune Evasion in Cancer: Mechanistic Basis and Therapeutic Strategies. Semin Cancer Biol (2015) 35 Suppl:S185–s98. doi: 10.1016/j.semcancer.2015.03.004
64. Whiteside TL. What are Regulatory T Cells (Treg) Regulating in Cancer and Why? Semin Cancer Biol (2012) 22(4):327–34. doi: 10.1016/j.semcancer.2012.03.004
65. Sakaguchi S, Vignali DAA, Rudensky AY, Niec RE, Waldmann H. The Plasticity and Stability of Regulatory T Cells. Nat Rev Immunol (2013) 13(6):461–7. doi: 10.1038/nri3464
66. Sasidharan Nair V, Elkord E. Immune Checkpoint Inhibitors in Cancer Therapy: A Focus on T-Regulatory Cells. Immunol Cell Biol (2018) 96(1):21–33. doi: 10.1111/imcb.1003
67. Vignali DAA, Collison LW, Workman CJ. How Regulatory T Cells Work. Nat Rev Immunol (2008) 8(7):523–32. doi: 10.1038/nri2343
68. Liao J, Xiao J, Zhou Y, Liu Z, Wang C. Effect of Transcatheter Arterial Chemoembolization on Cellular Immune Function and Regulatory T Cells in Patients With Hepatocellular Carcinoma. Mol Med Rep (2015) 12(4):6065–71. doi: 10.3892/mmr.2015.4171
69. Qiu X, Wang X, Song Y, Chen L. Plasma Level of Interleukin-35 as an Independent Prognostic Indicator in Hepatocellular Carcinoma. Dig Dis Sci (2016) 61(12):3513–21. doi: 10.1007/s10620-016-4270-7
70. Wang Y-Y, He X-Y, Cai Y-Y, Wang Z-J, Lu S-H. The Variation of CD4+CD25+ Regulatory T Cells in the Periphery Blood and Tumor Microenvironment of Non-Small Cell Lung Cancer Patients and the Downregulation Effects Induced by CpG ODN. Target Oncol (2011) 6(3):147–54. doi: 10.1007/s11523-011-0182-9
71. Wei T, Zhang J, Qin Y, Wu Y, Zhu L, Lu L, et al. Increased Expression of Immunosuppressive Molecules on Intratumoral and Circulating Regulatory T Cells in non-Small-Cell Lung Cancer Patients. Am J Cancer Res (2015) 5(7):2190–201.
72. He LY, Li L, Guo ML, Zhang Y, Zhang HZ. Relationship Between CD4+CD25+ Treg and Expression of HIF-1α and Ki-67 in NSCLC Patients. Eur Rev Med Pharmacol Sci (2015) 19(8):1351–5.
73. Collison LW, Chaturvedi V, Henderson AL, Giacomin PR, Guy C, Bankoti J, et al. IL-35-Mediated Induction of a Potent Regulatory T Cell Population. Nat Immunol (2010) 11(12):1093–101. doi: 10.1038/ni.1952
74. Bettini M, Castellaw AH, Lennon GP, Burton AR, Vignali DAA. Prevention of Autoimmune Diabetes by Ectopic Pancreatic β-Cell Expression of Interleukin-35. Diabetes (2012) 61(6):1519–26. doi: 10.2337/db11-0784
75. Li J, Wang Z, Mao K, Guo X. Clinical Significance of Serum T Helper 1/T Helper 2 Cytokine Shift in Patients With Non-Small Cell Lung Cancer. Oncol Lett (2014) 8(4):1682–6. doi: 10.3892/ol.2014.2391
76. Ito N, Nakamura H, Metsugi H, Ohgi S. Dissociation Between T Helper Type 1 and Type 2 Differentiation and Cytokine Production in Tumor-Infiltrating Lymphocytes in Patients With Lung Cancer. Surg Today (2001) 31(5):390–4. doi: 10.1007/s005950170127
77. Asselin-Paturel C, Echchakir H, Carayol G, Gay F, Opolon P, Grunenwald D, et al. Quantitative Analysis of Th1, Th2 and TGF-Beta1 Cytokine Expression in Tumor, TIL and PBL of Non-Small Cell Lung Cancer Patients. Int J Cancer (1998) 77(1):7–12. doi: 10.1002/(SICI)1097-0215(19980703)77:1<7::AID-IJC2>3.0.CO;2-Y
78. Almatroodi SA, McDonald CF, Darby IA, Pouniotis DS. Characterization of M1/M2 Tumour-Associated Macrophages (TAMs) and Th1/Th2 Cytokine Profiles in Patients With NSCLC. Cancer Microenviron Off J Int Cancer Microenviron Soc (2016) 9(1):1–11. doi: 10.1007/s12307-015-0174-x
79. Ito N, Suzuki Y, Taniguchi Y, Ishiguro K, Nakamura H, Ohgi S. Prognostic Significance of T Helper 1 and 2 and T Cytotoxic 1 and 2 Cells in Patients With Non-Small Cell Lung Cancer. Anticancer Res (2005) 25(3b):2027–31.
80. Kesselring R, Thiel A, Pries R, Trenkle T, Wollenberg B. Human Th17 Cells can be Induced Through Head and Neck Cancer and Have a Functional Impact on HNSCC Development. Br J Cancer (2010) 103(8):1245–54. doi: 10.1038/sj.bjc.6605891
81. Guéry L, Hugues S. Th17 Cell Plasticity and Functions in Cancer Immunity. BioMed Res Int (2015) 2015:314620. doi: 10.1155/2015/314620
82. Li Y, Cao ZY, Sun B, Wang GY, Fu Z, Liu YM, et al. Effects of IL-17A on the Occurrence of Lung Adenocarcinoma. Cancer Biol Ther (2011) 12(7):610–6. doi: 10.4161/cbt.12.7.16302
83. Liao T, Fan J, Lv Z, Xu J, Wu F, Yang G, et al. Comprehensive Genomic and Prognostic Analysis of the IL−17 Family Genes in Lung Cancer. Mol Med Rep (2019) 19(6):4906–18. doi: 10.3892/mmr.2019.10164
84. Li S, Li Y, Qu X, Liu X, Liang J. Detection and Significance of TregFoxP3(+) and Th17 Cells in Peripheral Blood of non-Small Cell Lung Cancer Patients. Arch Med Sci AMS (2014) 10(2):232–9. doi: 10.5114/aoms.2014.42573
85. Duan MC, Zhong XN, Liu GN, Wei JR. The Treg/Th17 Paradigm in Lung Cancer. J Immunol Res (2014) 2014:730380. doi: 10.1155/2014/730380
86. Asadzadeh Z, Mohammadi H, Safarzadeh E, Hemmatzadeh M, Mahdian-Shakib A, Jadidi-Niaragh F, et al. The Paradox of Th17 Cell Functions in Tumor Immunity. Cell Immunol (2017) 322:15–25. doi: 10.1016/j.cellimm.2017.10.015
87. Wei L, Wang H, Yang F, Ding Q, Zhao J. Interleukin-17 Potently Increases non-Small Cell Lung Cancer Growth. Mol Med Rep (2016) 13(2):1673–80. doi: 10.3892/mmr.2015.4694
88. Pan B, Che D, Cao J, Shen J, Jin S, Zhou Y, et al. Interleukin-17 Levels Correlate With Poor Prognosis and Vascular Endothelial Growth Factor Concentration in the Serum of Patients With Non-Small Cell Lung Cancer. Biomarkers Biochem Indic Exposure Response Susceptibility Chem (2015) 20(4):232–9. doi: 10.3109/1354750X.2015.1068853
89. Numasaki M, Watanabe M, Suzuki T, Takahashi H, Nakamura A, McAllister F, et al. IL-17 Enhances the Net Angiogenic Activity and In Vivo Growth of Human Non-Small Cell Lung Cancer in SCID Mice Through Promoting CXCR-2-Dependent Angiogenesis. J Immunol (Baltimore Md 1950) (2005) 175(9):6177–89. doi: 10.4049/jimmunol.175.9.6177
90. Chen X, Xie Q, Cheng X, Diao X, Cheng Y, Liu J, et al. Role of Interleukin-17 in Lymphangiogenesis in non-Small-Cell Lung Cancer: Enhanced Production of Vascular Endothelial Growth Factor C in Non-Small-Cell Lung Carcinoma Cells. Cancer Sci (2010) 101(11):2384–90. doi: 10.1111/j.1349-7006.2010.01684.x
91. Xu C, Hao K, Yu L, Zhang X. Serum Interleukin-17 as a Diagnostic and Prognostic Marker for non-Small Cell Lung Cancer. Biomarkers Biochem Indic Exposure Response Susceptibility Chem (2014) 19(4):287–90. doi: 10.3109/1354750X.2014.908954
92. Martin-Orozco N, Muranski P, Chung Y, Yang XO, Yamazaki T, Lu S, et al. T Helper 17 Cells Promote Cytotoxic T Cell Activation in Tumor Immunity. Immunity (2009) 31(5):787–98. doi: 10.1016/j.immuni.2009.09.014
93. Duan MC, Han W, Jin PW, Wei YP, Wei Q, Zhang LM, et al. Disturbed Th17/Treg Balance in Patients With Non-Small Cell Lung Cancer. Inflammation (2015) 38(6):2156–65. doi: 10.1007/s10753-015-0198-x
94. Collison LW, Pillai MR, Chaturvedi V, Vignali DA. Regulatory T Cell Suppression is Potentiated by Target T Cells in a Cell Contact, IL-35- and IL-10-Dependent Manner. J Immunol (Baltimore Md 1950) (2009) 182(10):6121–8. doi: 10.4049/jimmunol.0803646
95. Song M, Ma X. The Immunobiology of Interleukin-35 and Its Regulation and Gene Expression. Adv Exp Med Biol (2016) 941:213–25. doi: 10.1007/978-94-024-0921-5_10
96. Xia L, Liu Y, Wang Y. PD-1/PD-L1 Blockade Therapy in Advanced Non-Small-Cell Lung Cancer: Current Status and Future Directions. Oncologist (2019) 24(Suppl 1):S31–41. doi: 10.1634/theoncologist.2019-IO-S1-s05
97. Xia B, Herbst RS. Immune Checkpoint Therapy for Non-Small-Cell Lung Cancer: An Update. Immunotherapy (2016) 8(3):279–98. doi: 10.2217/imt.15.123
98. Liu B, Song Y, Liu D. Recent Development in Clinical Applications of PD-1 and PD-L1 Antibodies for Cancer Immunotherapy. J Hematol Oncol (2017) 10(1):174. doi: 10.1186/s13045-017-0541-9
99. Keir ME, Butte MJ, Freeman GJ, Sharpe AH. PD-1 and its Ligands in Tolerance and Immunity. Annu Rev Immunol (2008) 26:677–704. doi: 10.1146/annurev.immunol.26.021607.090331
100. Chang K, Svabek C, Vazquez-Guillamet C, Sato B, Rasche D, Wilson S, et al. Targeting the Programmed Cell Death 1: Programmed Cell Death Ligand 1 Pathway Reverses T Cell Exhaustion in Patients With Sepsis. Crit Care (London England) (2014) 18(1):R3. doi: 10.1186/cc13176
101. Barber DL, Wherry EJ, Masopust D, Zhu B, Allison JP, Sharpe AH, et al. Restoring Function in Exhausted CD8 T Cells During Chronic Viral Infection. Nature (2006) 439(7077):682–7. doi: 10.1038/nature04444
102. Liu J, Zhang S, Hu Y, Yang Z, Li J, Liu X, et al. Targeting PD-1 and Tim-3 Pathways to Reverse CD8 T-Cell Exhaustion and Enhance Ex Vivo T-Cell Responses to Autologous Dendritic/Tumor Vaccines. J Immunother (2016) 39(4):171–80. doi: 10.1097/CJI.0000000000000122
103. Blackburn SD, Shin H, Freeman GJ, Wherry EJ. Selective Expansion of a Subset of Exhausted CD8 T Cells by alphaPD-L1 Blockade. Proc Natl Acad Sci USA (2008) 105(39):15016–21. doi: 10.1073/pnas.0801497105
104. Wei SC, Levine JH, Cogdill AP, Zhao Y, Anang NAS, Andrews MC, et al. Distinct Cellular Mechanisms Underlie Anti-CTLA-4 and Anti-PD-1 Checkpoint Blockade. Cell (2017) 170(6):1120–33.e17. doi: 10.1016/j.cell.2017.07.024
105. Wang M, Yao LC, Cheng M, Cai D, Martinek J, Pan CX, et al. Humanized Mice in Studying Efficacy and Mechanisms of PD-1-Targeted Cancer Immunotherapy. FASEB J Off Publ Fed Am Societies Exp Biol (2018) 32(3):1537–49. doi: 10.1096/fj.201700740R
106. Iwai Y, Ishida M, Tanaka Y, Okazaki T, Honjo T, Minato N. Involvement of PD-L1 on Tumor Cells in the Escape From Host Immune System and Tumor Immunotherapy by PD-L1 Blockade. Proc Natl Acad Sci USA (2002) 99(19):12293–7. doi: 10.1073/pnas.192461099
107. Low JL, Walsh RJ, Ang Y, Chan G, Soo RA. The Evolving Immuno-Oncology Landscape in Advanced Lung Cancer: First-Line Treatment of non-Small Cell Lung Cancer. Ther Adv Med Oncol (2019) 11:1758835919870360. doi: 10.1177/1758835919870360
108. Ohaegbulam KC, Assal A, Lazar-Molnar E, Yao Y, Zang X. Human Cancer Immunotherapy With Antibodies to the PD-1 and PD-L1 Pathway. Trends Mol Med (2015) 21(1):24–33. doi: 10.1016/j.molmed.2014.10.009
109. Overacre-Delgoffe AE, Chikina M, Dadey RE, Yano H, Brunazzi EA, Shayan G, et al. Interferon-γ Drives T Fragility to Promote Anti-Tumor Immunity. Cell (2017) 169(6):1130–41.e11. doi: 10.1016/j.cell.2017.05.005
110. Bakir B, Chiarella AM, Pitarresi JR, Rustgi AK. EMT. MET, Plasticity, and Tumor Metastasis. Trends Cell Biol (2020) 30(10):764–76. doi: 10.1016/j.tcb.2020.07.003
111. Tang Z, Ding Y, Shen Q, Zhang C, Li J, Nazar M, et al. KIAA1199 Promotes Invasion and Migration in Non-Small-Cell Lung Cancer (NSCLC) via PI3K-Akt Mediated EMT. J Mol Med (Berlin Germany) (2019) 97(1):127–40. doi: 10.1007/s00109-018-1721-y
112. Otsuki Y, Saya H, Arima Y. Prospects for New Lung Cancer Treatments That Target EMT Signaling. Dev Dynamics Off Publ Am Assoc Anatomists (2018) 247(3):462–72. doi: 10.1002/dvdy.24596
113. Lee CC, Lin JC, Hwang WL, Kuo YJ, Chen HK, Tai SK, et al. Macrophage-Secreted Interleukin-35 Regulates Cancer Cell Plasticity to Facilitate Metastatic Colonization. Nat Commun (2018) 9(1):3763. doi: 10.1038/s41467-018-06268-0
114. Li F, Zhu T, Yue Y, Zhu X, Wang J, Liang L. Preliminary Mechanisms of Regulating PD−L1 Expression in non−Small Cell Lung Cancer During the EMT Process. Oncol Rep (2018) 40(2):775–82. doi: 10.3892/or.2018.6474
115. Kim S, Koh J, Kim MY, Kwon D, Go H, Kim YA, et al. PD-L1 Expression is Associated With Epithelial-to-Mesenchymal Transition in Adenocarcinoma of the Lung. Hum Pathol (2016) 58:7–14. doi: 10.1016/j.humpath.2016.07.007
116. Thompson JC, Hwang WT, Davis C, Deshpande C, Jeffries S, Rajpurohit Y, et al. Gene Signatures of Tumor Inflammation and Epithelial-to-Mesenchymal Transition (EMT) Predict Responses to Immune Checkpoint Blockade in Lung Cancer With High Accuracy. Lung Cancer (Amsterdam Netherlands) (2020) 139:1–8. doi: 10.1016/j.lungcan.2019.10.012
117. Weng CH, Chen LY, Lin YC, Shih JY, Lin YC, Tseng RY, et al. Epithelial-Mesenchymal Transition (EMT) Beyond EGFR Mutations Per Se is a Common Mechanism for Acquired Resistance to EGFR TKI. Oncogene (2019) 38(4):455–68. doi: 10.1038/s41388-018-0454-2
118. Lee AF, Chen MC, Chen CJ, Yang CJ, Huang MS, Liu YP. Reverse Epithelial-Mesenchymal Transition Contributes to the Regain of Drug Sensitivity in Tyrosine Kinase Inhibitor-Resistant Non-Small Cell Lung Cancer Cells. PloS One (2017) 12(7):e0180383. doi: 10.1371/journal.pone.0180383
119. Fukuda K, Takeuchi S, Arai S, Katayama R, Nanjo S, Tanimoto A, et al. Epithelial-To-Mesenchymal Transition Is a Mechanism of ALK Inhibitor Resistance in Lung Cancer Independent of ALK Mutation Status. Cancer Res (2019) 79(7):1658–70. doi: 10.1158/0008-5472.CAN-18-2052
120. Kogita A, Togashi Y, Hayashi H, Sogabe S, Terashima M, De Velasco MA, et al. Hypoxia Induces Resistance to ALK Inhibitors in the H3122 Non-Small Cell Lung Cancer Cell Line With an ALK Rearrangement via Epithelial-Mesenchymal Transition. Int J Oncol (2014) 45(4):1430–6. doi: 10.3892/ijo.2014.2574
121. Kauffmann-Guerrero D, Kahnert K, Kiefl R, Sellmer L, Walter J, Behr J, et al. Systemic Inflammation and Pro-Inflammatory Cytokine Profile Predict Response to Checkpoint Inhibitor Treatment in NSCLC: A Prospective Study. Sci Rep (2021) 11(1):10919. doi: 10.1038/s41598-021-90397-y
122. Martino EC, Misso G, Pastina P, Costantini S, Vanni F, Gandolfo C, et al. Immune-Modulating Effects of Bevacizumab in Metastatic non-Small-Cell Lung Cancer Patients. Cell Death Discov (2016) 2:16025. doi: 10.1038/cddiscovery.2016.25
123. Botta C, Misso G, Martino EC, Pirtoli L, Cusi MG, Tassone P, et al. The Route to Solve the Interplay Between Inflammation, Angiogenesis and Anti-Cancer Immune Response. Cell Death Dis (2016) 7(7):e2299. doi: 10.1038/cddis.2016.211
124. Nardone V, Giannicola R, Bianco G, Giannarelli D, Tini P, Pastina P, et al. Inflammatory Markers and Procalcitonin Predict the Outcome of Metastatic Non-Small-Cell-Lung-Cancer Patients Receiving PD-1/PD-L1 Immune-Checkpoint Blockade. Front Oncol (2021) 11:684110. doi: 10.3389/fonc.2021.684110
125. Chao Y, Zhou J, Hsu S, Ding N, Li J, Zhang Y, et al. Risk Factors for Immune Checkpoint Inhibitor-Related Pneumonitis in Non-Small Cell Lung Cancer. Trans Lung Cancer Res (2022) 11(2):295–306. doi: 10.21037/tlcr-22-72
126. Lie D-C, Colamarino SA, Song H-J, Désiré L, Mira H, Consiglio A, et al. Wnt Signalling Regulates Adult Hippocampal Neurogenesis. Nature (2005) 437(7063):1370–5. doi: 10.1038/nature04108
127. Clevers H. Wnt/beta-Catenin Signaling in Development and Disease. Cell (2006) 127(3):469–80. doi: 10.1016/j.cell.2006.10.018
128. Rapp J, Jaromi L, Kvell K, Miskei G, Pongracz JE. WNT Signaling - Lung Cancer is No Exception. Respir Res (2017) 18(1):167. doi: 10.1186/s12931-017-0650-6
129. Lv X-J, Zhao L-J, Hao Y-Q, Su Z-Z, Li J-Y, Du Y-W, et al. Schisandrin B Inhibits the Proliferation of Human Lung Adenocarcinoma A549 Cells by Inducing Cycle Arrest and Apoptosis. Int J Clin Exp Med (2015) 8(5):6926–36.
130. Huang CL, Liu D, Ishikawa S, Nakashima T, Nakashima N, Yokomise H, et al. Wnt1 Overexpression Promotes Tumour Progression in Non-Small Cell Lung Cancer. Eur J Cancer (Oxford Engl 1990) (2008) 44(17):2680–8. doi: 10.1016/j.ejca.2008.08.004
131. Stewart DJ. Wnt Signaling Pathway in Non-Small Cell Lung Cancer. J Natl Cancer Institute (2014) 106(1):djt356. doi: 10.1093/jnci/djt356
132. Yang S, Liu Y, Li M-Y, Ng CSH, Yang S-L, Wang S, et al. FOXP3 Promotes Tumor Growth and Metastasis by Activating Wnt/β-Catenin Signaling Pathway and EMT in Non-Small Cell Lung Cancer. Mol Cancer (2017) 16(1):124. doi: 10.1186/s12943-017-0700-1
133. Ma N, Fang Y, Xu R, Zhai B, Hou C, Wang X, et al. Ebi3 Promotes T- and B-Cell Division and Differentiation via STAT3. Mol Immunol (2019) 107:61–70. doi: 10.1016/j.molimm.2019.01.009
134. Jiang R, Jin Z, Liu Z, Sun L, Wang L, Li K. Correlation of Activated STAT3 Expression With Clinicopathologic Features in Lung Adenocarcinoma and Squamous Cell Carcinoma. Mol Diagn Ther (2011) 15(6):347–52. doi: 10.1007/BF03256470
135. Zhou J, Qu Z, Yan S, Sun F, Whitsett JA, Shapiro SD, et al. Differential Roles of STAT3 in the Initiation and Growth of Lung Cancer. Oncogene (2015) 34(29):3804–14. doi: 10.1038/onc.2014.318
136. Achcar Rde O, Cagle PT, Jagirdar J. Expression of Activated and Latent Signal Transducer and Activator of Transcription 3 in 303 Non-Small Cell Lung Carcinomas and 44 Malignant Mesotheliomas: Possible Role for Chemotherapeutic Intervention. Arch Pathol Lab Med (2007) 131(9):1350–60. doi: 10.5858/2007-131-1350-EOAALS
137. Ai T, Wang Z, Zhang M, Zhang L, Wang N, Li W, et al. Expression and Prognostic Relevance of STAT3 and Cyclin D1 in non-Small Cell Lung Cancer. Int J Biol Markers (2012) 27(2):e132–8. doi: 10.5301/JBM.2012.9146
138. Yu Y, Zhao Q, Wang Z, Liu XY. Activated STAT3 Correlates With Prognosis of Non-Small Cell Lung Cancer and Indicates New Anticancer Strategies. Cancer Chemother Pharmacol (2015) 75(5):917–22. doi: 10.1007/s00280-015-2710-2
139. Zhu Z, Zhang Y, Ye J, Wang X, Fu X, Yin Y, et al. IL-35 Promoted STAT3 Phosphorylation and IL-10 Production in B Cells, But its Production was Reduced in Patients With Coronary Artery Diseases. Hum Immunol (2018) 79(12):869–75. doi: 10.1016/j.humimm.2018.10.009
140. Chen X, Hao S, Zhao Z, Liu J, Shao Q, Wang F, et al. Interleukin 35: Inhibitory Regulator in Monocyte-Derived Dendritic Cell Maturation and Activation. Cytokine (2018) 108:43–52. doi: 10.1016/j.cyto.2018.03.008
141. Liang Y, Chen Q, Du W, Chen C, Li F, Yang J, et al. Epstein-Barr Virus-Induced Gene 3 (EBI3) Blocking Leads to Induce Antitumor Cytotoxic T Lymphocyte Response and Suppress Tumor Growth in Colorectal Cancer by Bidirectional Reciprocal-Regulation STAT3 Signaling Pathway. Mediators Inflammation (2016) 2016:3214105. doi: 10.1155/2016/3214105
142. Wu S, Li Y, Yao L, Li Y, Jiang S, Gu W, et al. Interleukin-35 Inhibits Angiogenesis Through STAT1 Signalling in Rheumatoid Synoviocytes. Clin Exp Rheumatol (2018) 36(2):223–7.
143. Liu J, Hao S, Chen X, Zhao H, Du L, Ren H, et al. Human Placental Trophoblast Cells Contribute to Maternal-Fetal Tolerance Through Expressing IL-35 and Mediating it(R)35 Conversion. Nat Commun (2019) 10(1):4601. doi: 10.1038/s41467-019-12484-z
144. Zhou X, Xia N, Lv B, Tang T, Nie S, Zhang M, et al. Interleukin 35 Ameliorates Myocardial Ischemia-Reperfusion Injury by Activating the Gp130-STAT3 Axis. FASEB J Off Publ Fed Am Societies Exp Biol (2020) 34(2):3224–38. doi: 10.1096/fj.201901718RR
145. Lee JM, Dedhar S, Kalluri R, Thompson EW. The Epithelial-Mesenchymal Transition: New Insights in Signaling, Development, and Disease. J Cell Biol (2006) 172(7):973–81. doi: 10.1083/jcb.200601018
146. Guarino M, Rubino B, Ballabio G. The Role of Epithelial-Mesenchymal Transition in Cancer Pathology. Pathology (2007) 39(3):305–18. doi: 10.1080/00313020701329914
147. Ngan CY, Yamamoto H, Seshimo I, Tsujino T, Man-i M, Ikeda JI, et al. Quantitative Evaluation of Vimentin Expression in Tumour Stroma of Colorectal Cancer. Br J Cancer (2007) 96(6):986–92. doi: 10.1038/sj.bjc.6603651
148. Baumgart E, Cohen MS, Silva Neto B, Jacobs MA, Wotkowicz C, Rieger-Christ KM, et al. Identification and Prognostic Significance of an Epithelial-Mesenchymal Transition Expression Profile in Human Bladder Tumors. Clin Cancer Res (2007) 13(6):1685–94. doi: 10.1158/1078-0432.CCR-06-2330
149. Mandal M, Myers JN, Lippman SM, Johnson FM, Williams MD, Rayala S, et al. Epithelial to Mesenchymal Transition in Head and Neck Squamous Carcinoma: Association of Src Activation With E-Cadherin Down-Regulation, Vimentin Expression, and Aggressive Tumor Features. Cancer (2008) 112(9):2088–100. doi: 10.1002/cncr.23410
150. Tsoukalas N, Aravantinou-Fatorou E, Tolia M, Giaginis C, Galanopoulos M, Kiakou M, et al. Epithelial-Mesenchymal Transition in Non Small-Cell Lung Cancer. Anticancer Res (2017) 37(4):1773–8. doi: 10.21873/anticanres.11510
Keywords: NSCLC, IL-35, Treg, PD-1/PD-L1, Th17
Citation: Hao Y, Dong H, Li W, Lv X, Shi B and Gao P (2022) The Molecular Role of IL-35 in Non-Small Cell Lung Cancer. Front. Oncol. 12:874823. doi: 10.3389/fonc.2022.874823
Received: 13 February 2022; Accepted: 25 April 2022;
Published: 26 May 2022.
Edited by:
Kwong Tsang, Precision Biologics, Inc., United StatesReviewed by:
Massimo Fantini, Precision Biologics, Inc., United StatesCopyright © 2022 Hao, Dong, Li, Lv, Shi and Gao. This is an open-access article distributed under the terms of the Creative Commons Attribution License (CC BY). The use, distribution or reproduction in other forums is permitted, provided the original author(s) and the copyright owner(s) are credited and that the original publication in this journal is cited, in accordance with accepted academic practice. No use, distribution or reproduction is permitted which does not comply with these terms.
*Correspondence: Peng Gao, Z2FvcGVuZzEyMzRAamx1LmVkdS5jbg==
†These authors share first authorship
Disclaimer: All claims expressed in this article are solely those of the authors and do not necessarily represent those of their affiliated organizations, or those of the publisher, the editors and the reviewers. Any product that may be evaluated in this article or claim that may be made by its manufacturer is not guaranteed or endorsed by the publisher.
Research integrity at Frontiers
Learn more about the work of our research integrity team to safeguard the quality of each article we publish.