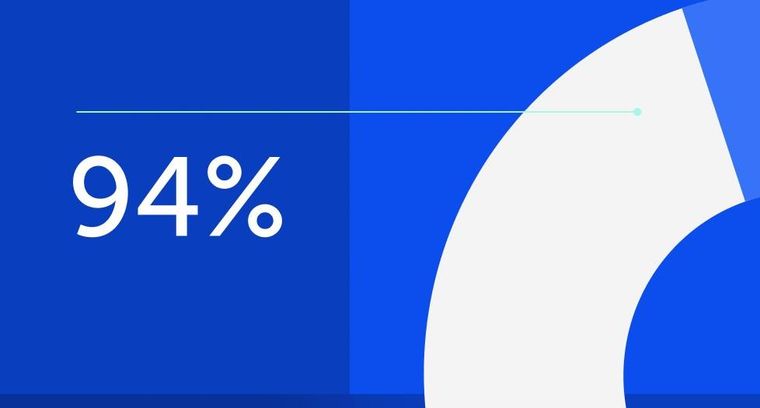
94% of researchers rate our articles as excellent or good
Learn more about the work of our research integrity team to safeguard the quality of each article we publish.
Find out more
ORIGINAL RESEARCH article
Front. Oncol., 23 June 2022
Sec. Pediatric Oncology
Volume 12 - 2022 | https://doi.org/10.3389/fonc.2022.874317
This article is part of the Research TopicWomen in Pediatric Oncology: 2021View all 13 articles
Background: Neurocognitive deficits in pediatric cancer survivors occur frequently; however, individual outcomes are unpredictable. We investigate clinical, genetic, and imaging predictors of neurocognition in pediatric cancer survivors, with a focus on survivors of central nervous system (CNS) tumors exposed to radiation.
Methods: One hundred eighteen patients with benign or malignant cancers (median diagnosis age: 7; 32% embryonal CNS tumors) were selected from an existing multi-institutional cohort (RadART Pro) if they had: 1) neurocognitive evaluation; 2) available DNA; 3) standard imaging. Utilizing RadART Pro, we collected clinical history, genomic sequencing, CNS imaging, and neurocognitive outcomes. We performed single nucleotide polymorphism (SNP) genotyping for candidate genes associated with neurocognition: COMT, BDNF, KIBRA, APOE, KLOTHO. Longitudinal neurocognitive testing were performed using validated computer-based CogState batteries. The imaging cohort was made of patients with available iron-sensitive (n = 28) and/or T2 FLAIR (n = 41) sequences. Cerebral microbleeds (CMB) were identified using a semi-automated algorithm. Volume of T2 FLAIR white matter lesions (WML) was measured using an automated method based on a convolutional neural network. Summary statistics were performed for patient characteristics, neurocognitive assessments, and imaging. Linear mixed effects and hierarchical models assessed patient characteristics and SNP relationship with neurocognition over time. Nested case-control analysis was performed to compare candidate gene carriers to non-carriers.
Results: CMB presence at baseline correlated with worse performance in 3 of 7 domains, including executive function. Higher baseline WML volumes correlated with worse performance in executive function and verbal learning. No candidate gene reliably predicted neurocognitive outcomes; however, APOE ϵ4 carriers trended toward worse neurocognitive function over time compared to other candidate genes and carried the highest odds of low neurocognitive performance across all domains (odds ratio 2.85, P=0.002). Hydrocephalus and seizures at diagnosis were the clinical characteristics most frequently associated with worse performance in neurocognitive domains (5 of 7 domains). Overall, executive function and verbal learning were the most frequently negatively impacted neurocognitive domains.
Conclusion: Presence of CMB, APOE ϵ4 carrier status, hydrocephalus, and seizures correlate with worse neurocognitive outcomes in pediatric cancer survivors, enriched with CNS tumors exposed to radiation. Ongoing research is underway to verify trends in larger cohorts.
Pediatric cancer survivors, particularly survivors of central nervous system (CNS) tumors, suffer from a range of late effects related to their tumor diagnosis and therapies, often leading to long-term negative impacts on quality of life (1, 2). Arguably, one of the most challenging late effects seen in this population is neurocognitive impairment (3–5). This is especially true after exposure to CNS radiation. Adults that survive childhood CNS tumors have lower intelligence quotients (IQs) and neurocognitive deficits specific to a variety of domains such as attention, processing speed, and executive function that worsen over time (6–10). Although neurocognitive outcomes for pediatric CNS tumor survivors show poorer neurocognitive functioning when compared to population means and normal matched controls (4, 11, 12), there remains great variability among individuals (7, 13). It is well supported that certain interventions such as cranial radiation therapy (14), particularly in the youngest patients, negatively impact neurocognition. Other clinical characteristics such as young age, hydrocephalus, and seizure disorder at diagnosis have also shown inverse relationships with later neurocognitive aptitude (15, 16).
Across adult literature, limited pediatric literature, and in preclinical models for aging and dementia, there are several candidate genes linked to neurocognitive outcomes. Within these genes, there are single nucleotide polymorphisms (SNPs) that correlate with neurocognitive performance. In aging adult populations, catechol-O-methyltransferase (COMT, rs4680); brain-derived neurotrophic factor (BDNF, rs6265); kidney and brain expressed protein (KIBRA, rs17070145); apolipoprotein E (APOE, rs429358, rs7412); and klotho (KL, rs9536314, rs95270025) each carry allelic variants that can be beneficial or detrimental to neurocognition (17–19). APOE, BDNF, and COMT candidate genes have demonstrated influence on neurocognition in oncology populations, though limited data exists specific to pediatric cancer and CNS tumor populations (20, 21). Confirmation of the role of such genetic predictors on neurocognitive outcomes in pediatric cancer survivors, particularly those with CNS tumors, could help personalize cancer therapy with the potential to limit neurocognitive injury and refine follow-up care. Further, at diagnosis, identification of predictors would help families make treatment-related decisions; prepare families for potentially significant, long-term impacts on their child’s life; and identify children at greatest risk.
In addition to genetic correlates, radiographic and radiogenomic signatures of neurocognitive outcomes would augment our understanding of which patients are at greatest risk of neurocognitive injury and who may benefit from early educational or cognitive interventions. Patients who undergo cranial radiation are at risk of developing cerebral microbleeds (CMB), which associate with higher doses of radiation, volume of radiation field, longer follow up, and age (22–26). High resolution 7T MRI studies have reported as high as 100% prevalence in CMB detection after 1 year following radiation therapy (27, 28) CMB presence in the frontal lobe associates with worse performance in executive functioning in the RadART cohort (29). Similarly, white matter lesions (WML), as measured by T2 FLAIR sequences on MR imaging, are an established neuroimaging marker of chronic effects of pediatric cancer therapy, such as radiation. The risk of accumulating WMLs is increased by younger age at diagnosis, hydrocephalus, methotrexate exposure, and treatment with radiation. Further, higher dose and volume of irradiated tissue impact the accumulation of WMLs (22, 23, 25, 30), which ultimately correlate with negative effects on intelligent quotient (IQ) and cognitive domains such as processing speed (31, 32).
In the current study, we assessed the impact of clinical characteristics, CMB and WML, and cognition-related genes (COMT, BDNF, KIBRA, APOE, and KLOTHO) on neurocognitive outcomes in a cohort of pediatric cancer survivors, enriched with CNS tumors, using an established multi-institutional cohort (Rad ART Pro) (29, 33). We hypothesize certain clinical characteristics, extent of CMB and WML, and genetic variants related to cognition will augment prediction of neurocognitive outcomes in survivors of CNS tumors. Our long-term aim is to improve anticipatory guidance, contribute to treatment stratification, and improve protective interventions for this high-risk population.
The patient population included in this study was selected from a cohort of patients who were previously enrolled in a multicenter, longitudinal cohort investigating radiation-induced arteriopathy, RadART Pro. The study collects clinical characteristics, DNA samples from peripheral blood collections, imaging, and neurocognitive performance outcomes in pediatric cancer survivors (29, 33). The cohort is enriched with patients with CNS tumors previously exposed to radiation therapy. Initial inclusion criteria for enrollment into RadART Pro were: 1) prior diagnosis of cancer, 2) previous exposure to radiation of the brain and/or neck, 3) age ≤ 21 years at time of radiation exposure, 4) anticipated survival > 1 year post-radiation. In 2015, the study expanded to include a comparison group of pediatric brain tumor patients that did not receive radiation therapy. For this group, diagnosis of a brain tumor must have occurred at age ≤ 21 years. Patients were recruited from four sites, including UCSF Benioff Children’s Hospital – San Francisco and Oakland sites (San Francisco, CA; Oakland, CA); Valley Children’s Hospital (Madera, CA); and St, Louis Children’s Hospital (St. Louis, MO). The institutional review boards of all participating sites of the RadART Pro study approved the protocol and procedures for that study. Informed consent was obtained from all patients prior to participation.
To be included in the integrated analyses in the current work, patients must have had at least candidate gene sequencing and one timepoint of neurocognitive testing.
SNP genotyping for COMT, BDNF, KIBRA, APOE, and KLOTHO was performed for each patient (Supplemental Table 1). Amplified product was sequenced in both directions with PCR primers using the Sanger method (Quintara Biosciences, Berkeley, CA). The complete sequencing protocol is included in the Supplemental Methods section.
Neurocognitive assessments were completed for all patients at an initial visit and regular follow-up intervals (about yearly) using computer-based CogState testing. CogState has been validated for patients 5 years and older, across a variety of populations, including the CNS tumor population (34–36). The CogState battery used in our study included the following tests: Identification test (IDN; attention), Continuous Paired Associate Learning test (CPAL; paired associate learning), Detection test (DET; psychomotor function), Groton Maze Learning test (GML; executive function), International Shopping List test (ISL and ISRL; verbal learning and verbal memory), and One Back test (ONB; working memory). All tests were administered by trained clinical research associates during standard of care clinic visits and under appropriate test-taking environments. Scores were collected for each test and converted to z-scores based on age-normed population means. For younger ages, some tests lacked sufficient population norms (e.g. age 5 to 9 years for the Groton Maze Learning, International Shopping List, and Continuous Paired Associate Learning tests). In these instances, z-scores were derived from age-matched comparisons within the patient cohort itself, as per vendor guidance.
Initial neurocognitive screens were typically conducted following completion of tumor-directed therapy for the primary diagnosis. Subsequent screens were completed at standard of care clinic visits for regular tumor surveillance. Due to the nature of this study opening several years after some patients completed therapy, initial testing occurred at variable post-therapy time points for individual patients. A continuous variable, “time from radiation” was used in all models to address the heterogeneity in timing of initial testing and follow-up. This variable reflected the time in years from end of cranial radiation therapy to the follow-up time point being tested.
Participants enrolled in RadART Pro were followed prospectively with structural and cerebrovascular brain imaging, as available. Imaging interval and acquisition parameters were based on institutional standards for routine clinical care and tumor surveillance. All imaging was performed on 1.5 and 3T scanners. Iron-sensitive imaging and T2 FLAIR sequences were acquired following primary therapy completion and within 180 days of the date of neurocognitive assessment.
Iron-sensitive imaging including T2*-weighted gradient echo sequences or susceptibility-weighted imaging (SWI; a technique that combines T2* magnitude and phase images to further enhance susceptibility contrast) were collected to detect, segment, and quantify CMBs using MATLAB-based semi-automated CMB detection and segmentation (37). CMBs were defined as hypointense foci that were present on consecutive, axial slices exceeding a threshold degree of radial symmetry (38). CMB candidates were excluded if in close proximity to perpendicular vessels or the tumor cavity. A single reader (LB) reviewed CMB candidates to determine if the segmented lesions were true CMBs or false positives. Segmented CMBs were counted and the cumulative CMB aggregate volume (mm3) was calculated.
T2-weighted fluid-attenuated inversion-recovery (FLAIR) sequences were collected across study sites, with inter-site variability in two-dimensional and three-dimensional acquisitions. A previously described convolutional neural network with 3D U-net architecture (39, 40) was trained to identify abnormal FLAIR signal attributable to prior radiation, excluding abnormal FLAIR signal attributable to post-surgical changes or treated tumor tissue. Training data consisted of 246 expert manual segmentations of target FLAIR signal, which were initially segmented by a research specialist with several years of brain MRI segmentation experience and modified or verified by a board-certified neuroradiologist with 4 years of post-residency experience. Training data were independent of test data, noting that 9 of the MRIs used for training were from patients that were also included in the test set, but from MRIs obtained in different years from those in the test set. Training hyperparameters included a kernel size of 3 x 3 x 3, cross-entropy loss function, and an Adam optimizer with learning rate of 1 x 10−4, implemented in TensorFlow 2 (https://www.tensorflow.org) using the Python programming language. The network was trained for 110 epochs, with a batch size of 37 3D patches (96 x 96 x 96 mm each). The implementation was on a DGX-2 AI server (version 4.5.0; NVIDIA). The fully trained U-net was then applied to the patients in our cohort with available FLAIR sequences and neurocognitive assessments to detect and segment areas of abnormal FLAIR signal attributable to radiation treatment, and the volume of this abnormality was quantified.
Clinical, genomic, and imaging variables were defined as follows: time from radiation (continuous variable), age at diagnosis (continuous variable), presence of hydrocephalus at diagnosis (binary variable), presence of seizures at diagnosis (binary variable), chemotherapy exposure (binary variable), radiation exposure (binary variable), gender (binary variable), tumor type (categorical variable), tumor location (categorical variable), presence of CMB at baseline (binary variable), and WML volume at baseline (continuous variable). Radiation was included as a binary variable to accommodate patients for which we did not have details on radiation dose. Across each model and statistical comparison, neurocognitive outcomes were evaluated per neurocognitive domain tested.
Summary statistics for patient characteristics, neurocognitive assessments, and imaging variables are presented as frequencies and percentages for categorical measures and median and interquartile range (IQR) for continuous variables. Neurocognitive outcomes are plotted over time with trajectories stratified by SNP carrier status (heterozygous or homozygous [carrier] vs non-carrier). Linear mixed effects models with time from radiation were used to assess the significance of SNP carriers on neurocognitive outcomes over time. The association of patient characteristics on neurocognitive outcomes were evaluated similarly. Characteristics significantly associated with most neurocognitive assessments were included in adjusted models considering SNP carrier status effect on outcome measures. Baseline association of imaging variables with patient characteristics, neurocognitive assessments, and SNP carrier status were evaluated by Chi-Square or Fisher’s exact tests, Wilcoxon rank-sum or Kruskal-Wallis tests, or Spearman correlation as appropriate. Hierarchical modeling with the addition of baseline CMB presence and WML volume to our adjusted models is used to assess longitudinal effect. All inference was conducted with significance level 0.05. All analyses were figures are generated in R 4.1.2.
Nested case-control analysis was done to compare candidate gene carriers (cases) and non-carriers (controls). Odds ratios were calculated to compare the prevalence of carriers and non-carriers in the lowest and highest performers on neurocognitive testing. Scores that were at least one standard deviation above or below the mean were considered high and low performers, respectively.
Within the full RadART Pro cohort (n=447), 118 patients met criteria for completion of both candidate sequencing and at least one timepoint of neurocognitive testing (n=57 males; median age at diagnosis 7 years [IQR 4, 11]; Table 1 and Figure 1). A total of 28 patients in this cohort had available iron-sensitive imaging sequences for assessment of CMBs and 41 patients had T2 FLAIR imaging for assessment of WMLs. These subcohorts are described in detail below.
Table 1 Summary of patient demographics, tumor characteristics, and baseline clinical symptoms across each subcohort by column.
Figure 1 Diagram of modalities investigated and bivariate and multivariate analyses with individual subcohort size and characteristics. Diagram details delineate data type at each level of analysis: neurocognitive assessments (computer screen), candidate gene sequencing (double helix), and imaging (CMBs as axial view, FLAIR WML as coronal view). RT, radiation therapy. Created with BioRender.com.
Embryonal tumors were the most frequent tumor diagnosis (n=38, 32%) with cerebellum/posterior fossa being the most common primary tumor location (n=35, 30%). Most patients (n=100, 85%) were treated with radiation therapy. Median time from cranial radiation therapy to time of initial neurocognitive testing was 3.9 years (IQR 2.1, 6.5) and median age at time of initial neurocognitive testing was 13 years (IQR 9.0, 18). Median time from diagnosis to initial neurocognitive testing was 5.0 years (IQR 3.0, 8.0; Table 2).
Table 2 Time of initial neurocognitive assessments in relationship to patient diagnosis and radiation by subcohort.
Of the 28 patients with available iron-sensitive imaging, 17 were male (61%; median age of diagnosis was 5 years [IQR 3, 8]; Table 1). Embryonal tumors were the most frequent tumor diagnosis (n = 11, 39%) with cerebellum/posterior fossa as the most common primary tumor location (n=9, 32%). Most patients (n=26, 93%) were treated with radiation therapy. Median age at initial neurocognitive assessment was 13 years (IQR 9, 15) and median time from diagnosis to initial neurocognitive testing was 6.5 (IQR 4.0, 9.0; Table 2). In patients previously treated with radiation therapy, median time from radiation therapy to initial neurocognitive testing was 4.5 years (IQR 2.5, 6.5). At least one CMB was detected in 10 patients (36%) at the time of initial neurocognitive assessment. Among those with at least one CMB, the median number of CMBs was 5.0 (IQR 4.0, 5.0) and the median total volume of CMBs was 120 mm3 (Figure 2). Neither the age at diagnosis nor the time from radiation significantly differed between the CMB-present and CMB-absent groups.
Figure 2 Visual representation of CMB analysis. Imaging inclusive of semi-automated lesion segmentation iron-sensitive sequence analysis. Left panel shows sequence without segmentation label with manually insertion of red circle outlining area of known cerebral microbleed. Right panel displays with semi-automated insertion of white circle overlying area of cerebral microbleed identified on segmentation.
Of the 41 patients with available T2 FLAIR imaging, 21 were male (51%; median age of diagnosis was 7 years [IQR 3. 10]; Table 1). Embryonal tumors were the most frequent tumor diagnosis (n = 19, 46%) with cerebellum/posterior fossa as the most common primary tumor location (n=17, 41%). Most patients (n=36, 88%) were treated with radiation therapy. Median age at initial neurocognitive assessment was 12 years (IQR 9, 17 and median time from diagnosis to initial neurocognitive testing was 6.0 years (IQR 3.0, 8.0; Table 2). In patients previously treated with radiation therapy, median time from radiation therapy to initial neurocognitive testing was 4.3 years (IQR 1.5, 6.4). Most patients (n=39; 95%) had measurable WML volumes identified by the convolutional neural network, of which the median volume was 1400 mm3 (IQR 349, 4590; Figure 3).
Figure 3 Visual representation of WML analysis. Imaging inclusive of manual T2-FLAIR white matter lesion segmentations with RT-induced (red) and non-RT-induced (green) labeling. RT, radiation therapy.
Each genetic variant was present at varying prevalence across the cohorts, with the largest difference in candidate gene carrier proportion being 32% and 46% for BDNF rs6265 in the CMB and WML cohorts, respectively (Supplemental Table 2). For the subset of 118 total patients, sequencing was unsuccessful for individual two alleles of interest (APOE: n=9; BDNF: n=1).
We initially performed bivariate analyses to identify isolated associations between clinical, genomic, and imaging characteristics with neurocognitive outcomes and inclusive of time to radiation as a variable. Based on statistical significance in bivariate analyses, we then determined which variables would be used in a hierarchical analysis to identify contributions of multiple variables on each neurocognitive domain tested.
Hydrocephalus and seizures were the most common clinical characteristics associated with worse neurocognitive outcome. These were each associated with 5 of 7 domains tested, including verbal learning, verbal memory, working memory, attention, and executive function (Table 3). Of tumor diagnoses, germ cell tumors associated with the highest number of affected domains (3 of 7), including verbal learning, working memory, and executive function. Age at diagnosis associated with worse performance in attention (P=0.03) and time from radiation associated with worse performance in verbal learning (P=0.03).
No candidate gene reliably predicted neurocognitive outcomes at a statistically significant level. However, compared to non-carriers, APOE ϵ4 carriers demonstrated worsening neurocognitive performance over time across all domains, albeit in a small cohort in later years of analysis (Figure 4). No other genetic variants demonstrated obvious trends on neurocognitive outcomes over time (Supplemental Figures 1–4). In the nested case-control candidate gene analyses comparing proportion of APOE ϵ4 carrier and non-carriers within the highest and lowest performers in neurocognitive testing, APOE ϵ4 carriers had the greatest odds of being among the poorest performers across all neurocognitive domains at all time points tested (Odds ratio [OR] 2.85, P=0.002), BDNF carriers showed the lowest odds, (OR 0.52, P=0.001), and COMT did not reach statistical significance (Table 4).
Figure 4 Longitudinal impact of APOE ϵ4 carrier status across each neurocognitive domain tested at baseline, Year 1, and Year 2 of enrollment. Trajectory of APOE ϵ4 carrier versus non-carrier mean performance across each neurocognitive domain from initial neurocognitive testing (baseline) to timepoint 3 of neurocognitive testing (Year 2). Blue line=non-carrier, red line=carrier.
Table 4 Prevalence of candidate gene carriers among high and low performers of neurocognitive assessments for timepoints 1 to 3 of testing.
No candidate gene was found to correlate with CMBs or WML volume at baseline assessment.
The impact of baseline CMBs and WML volume on neurocognition was evaluated independently and in combination. The presence of CMBs at time of initial neurocognitive assessment associated with worse performance in psychomotor function, executive function, verbal learning with median z-scores across each domain of -1.84 (P=0.01), -1.75 (P=0.02), and -0.77 (P=0.03), respectively. The impact of volume of WMLs at time of initial neurocognitive assessment was evaluated using Spearman correlation coefficients. Higher baseline WML volumes trended with worse performance in executive function (P=0.05) and verbal learning (P=0.06), but these did not reach statistical significance.
While we recognize limitations of our cohort size, we sought to preliminarily explore interactions of possible neurocognitive predictors. Based on statistical significance from bivariate analyses combined with prior work to suspect association with neurocognitive outcome (i.e. time from radiation), we performed hierarchical analyses across each candidate gene and neurocognitive outcome. The first model incorporated candidate gene carrier status, hydrocephalus, seizures, tumor type and time from radiation, and the second model added CMB and WML volume at baseline. Across the models without imaging findings, hydrocephalus or seizures at baseline continued to be the most prevalent characteristics associated with negative neurocognitive outcomes. No candidate gene demonstrated significant association once other characteristics were considered. Across these models, verbal learning, memory, working memory, executive function were consistently significantly impacted, most frequently in association with baseline hydrocephalus or seizures (P<0.05). In contrast, paired associate learning, psychomotor function, and attention were not significantly impacted across the variables tested.
As children complete therapy and enter long-term surveillance, identification of those at high-risk of neurocognitive injury based on genomics and/or radiographic imaging could lead to more aggressive and earlier neurocognitive and educational intervention. Our study sought to broaden understanding of predictors of neurocognitive outcomes in pediatric cancer survivors. Compared to adult populations, few studies exist that investigate genetic predictors for neurocognition in long-term survivors (17, 18, 41–43). COMT has been investigated in childhood brain tumor survivors, where Met/Val heterozygotes outperform Met/Met and Val/Val homozygotes (rs4680; Val158Met) (44). And, a common polymorphism in BDNF, Val66Met, shows valine homozygosity associates with higher IQs, processing speed, and memory in adults (17), but lacks demonstrated impact on neurocognitive function in adult CNS tumor survivors (20). In other adult studies, heterozygosity for the KL haplotype, KL-VS (Phe352Val and Cys370Ser), leads to improved cognition, executive function, and larger brain volumes in aging adults and transgenic mouse models (18, 43, 45). Meanwhile, in healthy children, APOE ϵ4 homozygotes have shown poorer executive function, memory, and attention, and a potential relationship to smaller hippocampal volumes (46). Further, APOE ϵ4 has been linked to poorer neurocognition, memory, and executive function in adult CNS tumor patients (19, 47, 48) and was linked to tau-mediated neuroinflammation and neurodegeneration, independent of amyloid-ß deposition (19, 49).
Although no single gene was a reliable predictor across all tested neurocognitive domains in our cohort, APOE ϵ4 carrier status most robustly associated with neurocognitive worsening over time. Other candidate genes, COMT, BDNF, KIBRA and KLOTHO, demonstrated mixed impact on neurocognitive outcomes and sometimes unexpected impact, such as the apparent protective effect of BDNF Val66Met. We recognize that we did not explore all possible SNP possibilities that may play a role across these genes and explored only SNPs of interest, which may inform future analyses of this cohort. Further, the cohort of patients with the SNPs of interest remained small overall and warrants study in a larger population. Additionally, we did not fully explore circulating peripheral protein levels, which may inform genotype: phenotype relationships. For example, high circulating levels of BDNF negatively correlate with neurocognitive function (50), while elevated klotho levels correlate with improved cognitive (43, 45). A next iteration of our work will be to correlate protein levels with genotype, which is currently under way for cerebrospinal and blood collections from our cohort.
We previously demonstrated that the presence of CMBs is associated with poor neurocognitive outcomes in pediatric CNS tumor survivors (28, 29). Of particular interest to the current work is that CMBs also associate with Alzheimer’s disease (29, 51) and APOE ϵ4 has been linked directly to CMBs in non-demented elderly patients, as well as neurovascular disease, decreased neuronal repair, and increased brain atrophy (52, 53). Given the role of APOE ϵ4 across broad pathophysiologic processes, it is possible that radiation injury in the setting of APOE ϵ4 exacerbates underlying predisposition to multifactorial neurocognitive injury. This would support our preliminary APOE ϵ4 signal as a biomarker of neurocognitive outcomes in our patient cohort, which again was enriched with patients that previously received CNS radiation. Although our findings include a limited sample size, we feel they confirm that future exploration should explore volumetric analyses, white matter changes based on DTI sequences, and in larger patient numbers.
From a clinical standpoint, our work supported previous reports identifying hydrocephalus and seizures at baseline as predictors of worse cognitive function (2, 6, 8, 54–58). In our cohort, across both bivariate and multivariate analyses, hydrocephalus and seizures reliably correlated with worse performance across several domains, including executive function. Of interest was that age at diagnosis and time from radiation did not reliably correlate with worse outcomes as in Morrison et al. (28), while germ cell tumors seem to most commonly correlated with worse outcomes albeit in overall small numbers. The lack of impact from age and time could be in part due distinctions in analyses, including our use of age as a continuous variable compared to other analyses using age cut-offs as binary or categorical variables (56, 59–61). Additionally, from a longitudinal perspective, it is possible that because our patients were commonly already five or more years from diagnosis, we see a decreased impact on rate of change (i.e. patients entered the study at an already lower baseline performance). In contrast, the contribution of the germ cell diagnosis could be reflective of the radiation field in these tumors and overlap with critical structures like the hippocampus (12, 57–59), but the validity of finding related to germ cell tumors require verification in larger patient numbers.
The strengths of our study arise from the diversity of the patient population and long-term follow-up, typically spanning over five years. Our cohort included several different tumor types and tumor locations, as well as patients across a range of ages and demographics. By pooling of patients from four separate sites across California and Missouri, we capture a broad and diverse patient population from multiple socioeconomic backgrounds, races, and ethnicities. The longitudinal time points of follow-up also strengthen our findings, as neurocognitive injury in pediatric brain tumor survivors worsens over time and becomes more impacting as patients age (2, 62). Lastly, the consistency of the neurocognitive measurements and analyses among each gene solidified the comparison between genes, more so than if the genes had been evaluated in separate studies. In contrast, the main shortfalls of our study arise from non-standardized follow-up periods, late timepoints to initial neurocognitive testing, and overall small cohort size for multivariate analyses. First, there was a limited number of patients that had reliable follow-up and a cohort of patients with missing treatment details. This was both a result of not yet reaching the assigned time point of follow up, but also due to patients being lost to follow-up and in recent years and clinical research restrictions imposed by the COVID-19 pandemic. Unfortunately, this contributed to missingness within the hierarchical analysis, inclusive of imaging variables, and thus limited these models. Future studies will need to expand the cohort size to accommodate the number of variables. Second, the follow-up intervals are not uniform throughout the cohort. This was driven by the neurocognitive tests being given as part of standard of care clinic visits and follow-up appointments being patient-dependent. We attempted to address this in our statistical analysis by including time from radiation as a continuous variable. Additionally, the patient population, although diverse, predominantly included patients with embryonal tumors reflecting the fact that medulloblastoma is the most common malignant pediatric CNS tumor. Further, we did not delineate dose of radiation or type of chemotherapy and only included these as binary variables in this initial review. Lastly, we recognize the nature of our investigation does not utilize reliable change indices as has been previously proposed for longitudinal neuropsychological testing (63) and the derivation of some normative values for the youngest patients within our cohort can be considered a shortcoming of this study, as this normalization may skew z-scores to higher performance than if compared to healthy populations alone. On the other hand, given that all testing was analyzed in the same manner across all genetic variants, we feel the reliability of the comparison across the genes remains intact and we would not expect the trend differences between the genes to be impacted by practiced learning or developmental changes within age groups over time.
In summary, our work shows that CMBs, WMLs, APOE ϵ4 carrier status, hydrocephalus and/or seizures at baseline may serve as markers for long-term cognitive dysfunction in pediatric cancer survivors, especially in patients with CNS tumors previously treated with radiation. Work is actively underway to expand the preliminary findings in this report and to include additional retrospective and prospective genetic and imaging studies for this target population.
The raw data supporting the conclusions of this article will be made available by the authors, without undue reservation.
The studies involving human participants were reviewed and approved by UCSF Benioff Children’s Hospital – San Francisco and Oakland sites (San Francisco, CA; Oakland, CA); Valley Children’s Hospital (Madera, CA); and Washington University School of Medicine, St, Louis (St. Louis, MO).Written informed consent to participate in this study was provided by all participants and/or participants' legal guardian.
CK, SM, HF contributed to conception and design of the study. DS recruited and enrolled participants on to the study. WF, SS performed the statistical analysis with review done by CK. LB, JL, MM, AR, PN performed imaging data analysis. CK, SS wrote first draft of the manuscript. JL, MM, AR, PN, WF contributed to specific sections of the manuscript. CK, SS, WF created all figures and tables. All authors reviewed and provided feedback to manuscript first draft. CK, SS completed the manuscript final revision. All authors contributed to the article and approved the submitted version.
This work was supported by a donation from the LaRoche family (HF, SM).
The authors declare that the research was conducted in the absence of any commercial or financial relationships that could be construed as a potential conflict of interest.
All claims expressed in this article are solely those of the authors and do not necessarily represent those of their affiliated organizations, or those of the publisher, the editors and the reviewers. Any product that may be evaluated in this article, or claim that may be made by its manufacturer, is not guaranteed or endorsed by the publisher.
The authors would like to thank all participants for their time to contribute to the study. We would like to thank Quintara Biosciences for completing the SNP genotyping and assisting with the supplementary methods section as well as Adrian Schembri and Paul Maruff at CogState, Ltd. for assistance in organizing and interpreting scores from neurocognitive testing.
The Supplementary Material for this article can be found online at: https://www.frontiersin.org/articles/10.3389/fonc.2022.874317/full#supplementary-material
Supplementary Figure 1 | Longitudinal impact of BDNF carrier status across each neurocognitive domain tested at baseline, Year 1, and Year 2 of enrollment. Trajectory of BDNF carrier versus non-carrier performance across each neurocognitive domain from initial neurocognitive testing (baseline) to timepoint 3 of neurocognitive testing (Year 2). Blue line=non-carrier, red line=carrier.
Supplementary Figure 2 | Longitudinal impact of COMT carrier status across each neurocognitive domain tested at baseline, Year 1, and Year 2 of enrollment. Trajectory of COMT carrier versus non-carrier performance across each neurocognitive domain from initial neurocognitive testing (baseline) to timepoint 3 of neurocognitive testing (Year 2). Blue line=non-carrier, red line=carrier.
Supplementary Figure 3 | Longitudinal impact of KIBRA carrier status across each neurocognitive domain tested at baseline, Year 1, and Year 2 of enrollment. Trajectory of KIBRA carrier versus non-carrier performance across each neurocognitive domain from initial neurocognitive testing (baseline) to timepoint 3 of neurocognitive testing (Year 2). Blue line=non-carrier, red line=carrier.
Supplementary Figure 4 | Longitudinal impact of KL-VS carrier status across each neurocognitive domain tested at baseline, Year 1, and Year 2 of enrollment. Trajectory of KL-VS carrier versus non-carrier performance across each neurocognitive domain from initial neurocognitive testing (baseline) to timepoint 3 of neurocognitive testing (Year 2). Blue line=non-carrier, red line=carrier.
Supplementary Table 1 | Description of candidate gene SNPs of interest and anticipated impact on neurocognitive outcome. Candidate gene alleles sequenced and expected effects on neurocognitive outcomes
Supplementary Table 2 | Prevalence of candidate gene SNPs of interest across each investigated subcohort. Candidate gene allele frequencies by cohort across each row.
APOE4, apolipoprotein E (APOE, rs429358, rs7412); BDNF, brain-derived neurotrophic factor (BDNF, rs6265); COMT, catechol-O-methyltransferase (COMT, rs4680); CMBs, cerebral microbleeds; KIBRA, kidney and brain expressed protein (KIBRA, rs17070145); WML, white matter lesions.
1. Macartney G, Harrison MB, Vandenkerkhof E, Stacey D, Mccarthy P. Quality of Life and Symptoms in Pediatric Brain Tumor Survivors: A Systematic Review. J Pediatr Oncol Nurs (2014) 31:65–77. doi: 10.1177/1043454213520191
2. Roddy E, Mueller S. Late Effects of Treatment of Pediatric Central Nervous System Tumors. J Child Neurol (2016) 31:237–54. doi: 10.1177/0883073815587944
3. Kiehna EN, Mulhern RK, Li C, Xiong X, Merchant TE. Changes in Attentional Performance of Children and Young Adults With Localized Primary Brain Tumors After Conformal Radiation Therapy. J Clin Oncol (2006) 24:5283–90. doi: 10.1200/JCO.2005.03.8547
4. Pietila S, Korpela R, Lenko HL, Haapasalo H, Alalantela R, Nieminen P, et al. Neurological Outcome of Childhood Brain Tumor Survivors. J Neurooncol (2012) 108:153–61. doi: 10.1007/s11060-012-0816-5
5. Sato I, Higuchi A, Yanagisawa T, Murayama S, Kumabe T, Sugiyama K, et al. Impact of Late Effects on Health-Related Quality of Life in Survivors of Pediatric Brain Tumors: Motility Disturbance of Limb(s), Seizure, Ocular/Visual Impairment, Endocrine Abnormality, and Higher Brain Dysfunction. Cancer Nurs (2014) 37:E1–E14. doi: 10.1097/NCC.0000000000000110
6. Robinson KE, Kuttesch JF, Champion JE, Andreotti CF, Hipp DW, Bettis A, et al. A Quantitative Meta-Analysis of Neurocognitive Sequelae in Survivors of Pediatric Brain Tumors. Pediatr Blood Cancer (2010) 55:525–31. doi: 10.1002/pbc.22568
7. Lafay-Cousin L, Fay-Mcclymont T, Johnston D, Fryer C, Scheinemann K, Fleming A, et al. Neurocognitive Evaluation of Long Term Survivors of Atypical Teratoid Rhabdoid Tumors (ATRT): The Canadian Registry Experience. Pediatr Blood Cancer (2015) 62:1265–9. doi: 10.1002/pbc.25441
8. Lassaletta A, Bouffet E, Mabbott D, Kulkarni AV. Functional and Neuropsychological Late Outcomes in Posterior Fossa Tumors in Children. Childs Nerv Syst (2015) 31:1877–90. doi: 10.1007/s00381-015-2829-9
9. Liu F, Scantlebury N, Tabori U, Bouffet E, Laughlin S, Strother D, et al. White Matter Compromise Predicts Poor Intellectual Outcome in Survivors of Pediatric Low-Grade Glioma. Neuro Oncol (2015) 17:604–13. doi: 10.1093/neuonc/nou306
10. Fay-Mcclymont TB, Ploetz DM, Mabbott D, Walsh K, Smith A, Chi SN, et al. Long-Term Neuropsychological Follow-Up of Young Children With Medulloblastoma Treated With Sequential High-Dose Chemotherapy and Irradiation Sparing Approach. J Neurooncol (2017) 133:119–28. doi: 10.1007/s11060-017-2409-9
11. Mulhern RK, White HA, Glass JO, Kun LE, Leigh L, Thompson SJ, et al. Attentional Functioning and White Matter Integrity Among Survivors of Malignant Brain Tumors of Childhood. J Int Neuropsychol Soc (2004) 10:180–9. doi: 10.1017/S135561770410204X
12. Nagel BJ, Delis DC, Palmer SL, Reeves C, Gajjar A, Mulhern RK. Early Patterns of Verbal Memory Impairment in Children Treated for Medulloblastoma. Neuropsychology (2006) 20:105–12. doi: 10.1037/0894-4105.20.1.105
13. Beebe DW, Ris MD, Armstrong FD, Fontanesi J, Mulhern R, Holmes E, et al. Cognitive and Adaptive Outcome in Low-Grade Pediatric Cerebellar Astrocytomas: Evidence of Diminished Cognitive and Adaptive Functioning in National Collaborative Research Studies (CCG 9891/POG 9130). J Clin Oncol (2005) 23:5198–204. doi: 10.1200/JCO.2005.06.117
14. Silber JH, Radcliffe J, Peckham V, Perilongo G, Kishnani P, Fridman M, et al. Whole-Brain Irradiation and Decline in Intelligence: The Influence of Dose and Age on IQ Score. J Clin Oncol (1992) 10:1390–6. doi: 10.1200/JCO.1992.10.9.1390
15. Reimers TS, Ehrenfels S, Mortensen EL, Schmiegelow M, Sonderkaer S, Carstensen H, et al. Cognitive Deficits in Long-Term Survivors of Childhood Brain Tumors: Identification of Predictive Factors. Med Pediatr Oncol (2003) 40:26–34. doi: 10.1002/mpo.10211
16. Chow EJ, Chen Y, Hudson MM, Feijen E, Kremer LC, Border WL, et al. Prediction of Ischemic Heart Disease and Stroke in Survivors of Childhood Cancer. J Clin Oncol (2018) 36:44–52. doi: 10.1200/JCO.2017.74.8673
17. Papenberg G, Salami A, Persson J, Lindenberger U, Backman L. Genetics and Functional Imaging: Effects of APOE, BDNF, COMT, and KIBRA in Aging. Neuropsychol Rev (2015) 25:47–62. doi: 10.1007/s11065-015-9279-8
18. Yokoyama JS, Sturm VE, Bonham LW, Klein E, Arfanakis K, Yu L, et al. Variation in Longevity Gene KLOTHO is Associated With Greater Cortical Volumes. Ann Clin Transl Neurol (2015) 2:215–30. doi: 10.1002/acn3.161
19. Correa DD, Kryza-Lacombe M, Zhou X, Baser RE, Beattie BJ, Beiene Z, et al. A Pilot Study of Neuropsychological Functions, APOE and Amyloid Imaging in Patients With Gliomas. J Neurooncol (2018) 136:613–22. doi: 10.1007/s11060-017-2692-5
20. Correa DD, Satagopan J, Cheung K, Arora AK, Kryza-Lacombe M, Xu Y, et al. COMT, BDNF, and DTNBP1 Polymorphisms and Cognitive Functions in Patients With Brain Tumors. Neuro Oncol (2016) 18:1425–33. doi: 10.1093/neuonc/now057
21. Correa DD, Satagopan J, Martin A, Braun E, Kryza-Lacombe M, Cheung K, et al. Genetic Variants and Cognitive Functions in Patients With Brain Tumors. Neuro Oncol (2019) 21:1297–309. doi: 10.1093/neuonc/noz094
22. Lupo JM, Chuang CF, Chang SM, Barani IJ, Jimenez B, Hess CP, et al. 7-Tesla Susceptibility-Weighted Imaging to Assess the Effects of Radiotherapy on Normal-Appearing Brain in Patients With Glioma. Int J Radiat Oncol Biol Phys (2012) 82:e493–500. doi: 10.1016/j.ijrobp.2011.05.046
23. Lupo JM, Molinaro AM, Essock-Burns E, Butowski N, Chang SM, Cha S, et al. The Effects of Anti-Angiogenic Therapy on the Formation of Radiation-Induced Microbleeds in Normal Brain Tissue of Patients With Glioma. Neuro Oncol (2016) 18:87–95. doi: 10.1093/neuonc/nov128
24. Passos J, Nzwalo H, Valente M, Marques J, Azevedo A, Netto E, et al. Microbleeds and Cavernomas After Radiotherapy for Paediatric Primary Brain Tumours. J Neurol Sci (2017) 372:413–6. doi: 10.1016/j.jns.2016.11.005
25. Wahl M, Anwar M, Hess CP, Chang SM, Lupo JM. Relationship Between Radiation Dose and Microbleed Formation in Patients With Malignant Glioma. Radiat Oncol (2017) 12:126. doi: 10.1186/s13014-017-0861-5
26. Klos J, Van Laar PJ, Sinnige PF, Enting RH, Kramer MCA, van der Weide HL, et al. Quantifying Effects of Radiotherapy-Induced Microvascular Injury; Review of Established and Emerging Brain MRI Techniques. Radiother Oncol (2019) 140:41–53. doi: 10.1016/j.radonc.2019.05.020
27. Morrison MA, Hess CP, Clarke JL, Butowski N, Chang SM, Molinaro AM, et al. Risk Factors of Radiotherapy-Induced Cerebral Microbleeds and Serial Analysis of Their Size Compared With White Matter Changes: A 7t MRI Study in 113 Adult Patients With Brain Tumors. J Magn Reson Imaging (2019) 50:868–77. doi: 10.1002/jmri.26651
28. Morrison MA, Mueller S, Felton E, Jakary A, Stoller S, Avadiappan S, et al. Rate of Radiation-Induced Microbleed Formation on 7T MRI Relates to Cognitive Impairment in Young Patients Treated With Radiation Therapy for a Brain Tumor. Radiother Oncol (2021) 154:145–53. doi: 10.1016/j.radonc.2020.09.028
29. Roddy E, Sear K, Felton E, Tamrazi B, Gauvain K, Torkildson J, et al. Presence of Cerebral Microbleeds is Associated With Worse Executive Function in Pediatric Brain Tumor Survivors. Neuro Oncol (2016) 18:1548–58. doi: 10.1093/neuonc/now082.02
30. Partap S, Russo S, Esfahani B, Yeom K, Mazewski C, Embry L, et al. A Review of Chronic Leukoencephalopathy Among Survivors of Childhood Cancer. Pediatr Neurol (2019) 101:2–10. doi: 10.1016/j.pediatrneurol.2019.03.006
31. Fouladi M, Chintagumpala M, Laningham FH, Ashley D, Kellie SJ, Langston JW, et al. White Matter Lesions Detected by Magnetic Resonance Imaging After Radiotherapy and High-Dose Chemotherapy in Children With Medulloblastoma or Primitive Neuroectodermal Tumor. J Clin Oncol (2004) 22:4551–60. doi: 10.1200/JCO.2004.03.058
32. Aleksonis HA, Krishnamurthy LC, King TZ. White Matter Hyperintensity Volumes are Related to Processing Speed in Long-Term Survivors of Childhood Cerebellar Tumors. J Neurooncol (2021) 154:63–72. doi: 10.1007/s11060-021-03799-3
33. Nordstrom M, Felton E, Sear K, Tamrazi B, Torkildson J, Gauvain K, et al. Large Vessel Arteriopathy After Cranial Radiation Therapy in Pediatric Brain Tumor Survivors. J Child Neurol (2018) 33:359–66. doi: 10.1177/0883073818756729
34. Bangirana P, Sikorskii A, Giordani B, Nakasujja N, Boivin MJ. Validation of the CogState Battery for Rapid Neurocognitive Assessment in Ugandan School Age Children. Child Adolesc Psychiatry Ment Health (2015) 9:38. doi: 10.1186/s13034-015-0063-6
35. Cromer JA, Harel BT, Yu K, Valadka JS, Brunwin JW, Crawford CD, et al. Comparison of Cognitive Performance on the Cogstate Brief Battery When Taken In-Clinic, In-Group, and Unsupervised. Clin Neuropsychol (2015) 29:542–58. doi: 10.1080/13854046.2015.1054437
36. Heitzer AM, Ashford JM, Harel BT, Schembri A, Swain MA, Wallace J, et al. Computerized Assessment of Cognitive Impairment Among Children Undergoing Radiation Therapy for Medulloblastoma. J Neurooncol (2019) 141:403–11. doi: 10.1007/s11060-018-03046-2
37. Morrison MA, Payabvash S, Chen Y, Avadiappan S, Shah M, Zou X, et al. A User-Guided Tool for Semi-Automated Cerebral Microbleed Detection and Volume Segmentation: Evaluating Vascular Injury and Data Labelling for Machine Learning. NeuroImage: Clin (2018) 20:498–505. doi: 10.1016/j.nicl.2018.08.002
38. Bian W, Hess CP, Chang SM, Nelson SJ, Lupo JM. Computer-Aided Detection of Radiation-Induced Cerebral Microbleeds on Susceptibility-Weighted MR Images. NeuroImage Clin (2013) 2:282–90. doi: 10.1016/j.nicl.2013.01.012
39. Duong MT, Rudie JD, Wang J, Xie L, Mohan S, Gee JC, et al. Convolutional Neural Network for Automated FLAIR Lesion Segmentation on Clinical Brain MR Imaging. AJNR Am J Neuroradiol (2019) 40:1282–90. doi: 10.3174/ajnr.A6138
40. Rauschecker AM, Gleason TJ, Nedelec P, Duong MT, Weiss DA, Calabrese E, et al. Interinstitutional Portability of a Deep Learning Brain MRI Lesion Segmentation Algorithm. Radiol Artif Intell (2021) 4. doi: 10.1148/ryai.2021200152
41. Arking DE, Krebsova A, Macek MSR, Macek M Jr., Arking A, Mian IS, et al. Association of Human Aging With a Functional Variant of Klotho. Proc Natl Acad Sci USA (2002) 99:856–61. doi: 10.1073/pnas.022484299
42. Duce JA, Podvin S, Hollander W, Kipling D, Rosene DL, Abraham CR. Gene Profile Analysis Implicates Klotho as an Important Contributor to Aging Changes in Brain White Matter of the Rhesus Monkey. Glia (2008) 56:106–17. doi: 10.1002/glia.20593
43. Dubal DB, Yokoyama JS, Zhu L, Broestl L, Worden K, Wang D, et al. Life Extension Factor Klotho Enhances Cognition. Cell Rep (2014) 7:1065–76. doi: 10.1016/j.celrep.2014.03.076
44. Howarth RA, Adamson AM, Ashford JM, Merchant TE, Ogg RJ, Schulenberg SE, et al. Investigating the Relationship Between COMT Polymorphisms and Working Memory Performance Among Childhood Brain Tumor Survivors. Pediatr Blood Cancer (2014) 61:40–5. doi: 10.1002/pbc.24649
45. Dubal DB, Zhu L, Sanchez PE, Worden K, Broestl L, Johnson E, et al. Life Extension Factor Klotho Prevents Mortality and Enhances Cognition in hAPP Transgenic Mice. J Neurosci (2015) 35:2358–71. doi: 10.1523/JNEUROSCI.5791-12.2015
46. Chang L, Douet V, Bloss C, Lee K, Pritchett A, Jernigan TL, et al. Gray Matter Maturation and Cognition in Children With Different APOE Epsilon Genotypes. Neurology (2016) 87:585–94. doi: 10.1212/WNL.0000000000002939
47. Correa DD, Deangelis LM, Shi W, Thaler HT, Lin M, Abrey LE. Cognitive Functions in Low-Grade Gliomas: Disease and Treatment Effects. J Neurooncol (2007) 81:175–84. doi: 10.1007/s11060-006-9212-3
48. Correa DD, Satagopan J, Baser RE, Cheung K, Richards E, Lin M, et al. APOE Polymorphisms and Cognitive Functions in Patients With Brain Tumors. Neurology (2014) 83:320–7. doi: 10.1212/WNL.0000000000000617
49. Arking DE, Becker DM, Yanek LR, Fallin D, Judge DP, Moy TF, et al. KLOTHO Allele Status and the Risk of Early-Onset Occult Coronary Artery Disease. Am J Hum Genet (2003) 72:1154–61. doi: 10.1086/375035
50. Yeom CW, Park YJ, Choi SW, Bhang SY. Association of Peripheral BDNF Level With Cognition, Attention and Behavior in Preschool Children. Child Adolesc Psychiatry Ment Health (2016) 10:10. doi: 10.1186/s13034-016-0097-4
51. De Reuck J, Deramecourt V, Cordonnier C, Leys D, Pasquier F, Maurage CA. Prevalence of Small Cerebral Bleeds in Patients With a Neurodegenerative Dementia: A Neuropathological Study. J Neurol Sci (2011) 300:63–6. doi: 10.1016/j.jns.2010.09.031
52. Liu CC, Liu CC, Kanekiyo T, Xu H, Bu G. Apolipoprotein E and Alzheimer Disease: Risk, Mechanisms and Therapy. Nat Rev Neurol (2013) 9:106–18. doi: 10.1038/nrneurol.2012.263
53. Graff-Radford J, Simino J, Kantarci K, Mosley TH Jr, Griswold ME, Windham BG, et al. Neuroimaging Correlates of Cerebral Microbleeds: The ARIC Study (Atherosclerosis Risk in Communities). Stroke (2017) 48:2964–72. doi: 10.1161/STROKEAHA.117.018336
54. Nathan PC, Patel SK, Dilley K, Goldsby R, Harvey J, Jacobsen C, et al. Guidelines for Identification of, Advocacy for, and Intervention in Neurocognitive Problems in Survivors of Childhood Cancer: A Report From the Children's Oncology Group. Arch Pediatr Adolesc Med (2007) 161:798–806. doi: 10.1001/archpedi.161.8.798
55. Ellenberg L, Liu Q, Gioia G, Yasui Y, Packer RJ, Mertens A, et al. Neurocognitive Status in Long-Term Survivors of Childhood CNS Malignancies: A Report From the Childhood Cancer Survivor Study. Neuropsychology (2009) 23:705–17. doi: 10.1037/a0016674
56. Duffner PK. Risk Factors for Cognitive Decline in Children Treated for Brain Tumors. Eur J Paediatr Neurol (2010) 14:106–15. doi: 10.1016/j.ejpn.2009.10.005
57. Kline CN, Mueller S. Neurocognitive Outcomes in Children With Brain Tumors. Semin Neurol (2020) 40:315–21. doi: 10.1055/s-0040-1708867
58. Tsang DS, Kim L, Liu ZA, Janzen L, Khandwala M, Bouffet E, et al. Intellectual Changes After Radiation for Children With Brain Tumors: Which Brain Structures are Most Important? Neuro Oncol (2021) 23:487–97. doi: 10.1093/neuonc/noaa217
59. Greenberger BA, Pulsifer MB, Ebb DH, Macdonald SM, Jones RM, Butler WE, et al. Clinical Outcomes and Late Endocrine, Neurocognitive, and Visual Profiles of Proton Radiation for Pediatric Low-Grade Gliomas. Int J Radiat Oncol Biol Phys (2014) 89:1060–8. doi: 10.1016/j.ijrobp.2014.04.053
60. Pulsifer MB, Sethi RV, Kuhlthau KA, Macdonald SM, Tarbell NJ, Yock TI. Early Cognitive Outcomes Following Proton Radiation in Pediatric Patients With Brain and Central Nervous System Tumors. Int J Radiat Oncol Biol Phys (2015) 93:400–7. doi: 10.1016/j.ijrobp.2015.06.012
61. Michalski JM, Janss AJ, Vezina LG, Smith KS, Billups CA, Burger PC, et al. Children's Oncology Group Phase III Trial of Reduced-Dose and Reduced-Volume Radiotherapy With Chemotherapy for Newly Diagnosed Average-Risk Medulloblastoma. J Clin Oncol (2021) 39:2685–97. doi: 10.1200/JCO.20.02730
62. Mulhern RK, Merchant TE, Gajjar A, Reddick WE, Kun LE. Late Neurocognitive Sequelae in Survivors of Brain Tumours in Childhood. Lancet Oncol (2004) 5:399–408. doi: 10.1016/S1470-2045(04)01507-4
Keywords: pediatric cancer survivors, Apo E4, neurocognition, late effects, radiation
Citation: Kline C, Stoller S, Byer L, Samuel D, Lupo JM, Morrison MA, Rauschecker AM, Nedelec P, Faig W, Dubal DB, Fullerton HJ and Mueller S (2022) An Integrated Analysis of Clinical, Genomic, and Imaging Features Reveals Predictors of Neurocognitive Outcomes in a Longitudinal Cohort of Pediatric Cancer Survivors, Enriched with CNS Tumors (Rad ART Pro). Front. Oncol. 12:874317. doi: 10.3389/fonc.2022.874317
Received: 11 February 2022; Accepted: 16 May 2022;
Published: 23 June 2022.
Edited by:
Paraskevi Panagopoulou, Aristotle University of Thessaloniki, GreeceReviewed by:
Maura Massimino, National Cancer Institute Foundation (IRCCS), ItalyCopyright © 2022 Kline, Stoller, Byer, Samuel, Lupo, Morrison, Rauschecker, Nedelec, Faig, Dubal, Fullerton and Mueller. This is an open-access article distributed under the terms of the Creative Commons Attribution License (CC BY). The use, distribution or reproduction in other forums is permitted, provided the original author(s) and the copyright owner(s) are credited and that the original publication in this journal is cited, in accordance with accepted academic practice. No use, distribution or reproduction is permitted which does not comply with these terms.
*Correspondence: Sabine Mueller, c2FiaW5lLm11ZWxsZXJAdWNzZi5lZHU=
†These authors have contributed equally to this work
Disclaimer: All claims expressed in this article are solely those of the authors and do not necessarily represent those of their affiliated organizations, or those of the publisher, the editors and the reviewers. Any product that may be evaluated in this article or claim that may be made by its manufacturer is not guaranteed or endorsed by the publisher.
Research integrity at Frontiers
Learn more about the work of our research integrity team to safeguard the quality of each article we publish.