- 1Department of Cardiovascular Surgery, The First People's Hospital of Yunnan Province, Kunming, Yunnan, China
- 2Department of Thoracic Surgery, The Second Affiliated Hospital of Kunming Medical University, Kunming, Yunnan, China
- 3Key Laboratory of Molecular Oncology and Epigenetics, The First Affiliated Hospital of Chongqing Medical University, Chongqing, China
- 4Department of Thoracic Surgery, The First People's Hospital of Yunnan Province, Kunming, Yunnan, China
- 5The Sbarro Institute for Cancer Research and Molecular Medicine, Center for Biotechnology, College of Science and Technology, Temple University, Philadelphia, PA, United States
- 6Kunming College of Life Science, University of Chinese Academy of Sciences, Beijing, China
Lung cancer is the leading cause of cancer-related deaths worldwide. Despite the recent advent of promising new targeted therapies, lung cancer diagnostic strategies still have difficulty in identifying the disease at an early stage. Therefore, the characterizations of more sensible and specific cancer biomarkers have become an important goal for clinicians. Circular RNAs are covalently close, endogenous RNAs without 5′ end caps or 3′poly (A) tails and have been characterized by high stability, abundance, and conservation as well as display cell/tissue/developmental stage-specific expressions. Numerous studies have confirmed that circRNAs act as microRNA (miRNA) sponges, RNA-binding protein, and transcriptional regulators; some circRNAs even act as translation templates that participate in multiple pathophysiological processes. Growing evidence have confirmed that circRNAs are involved in the pathogenesis of lung cancers through the regulation of proliferation and invasion, cell cycle, autophagy, apoptosis, stemness, tumor microenvironment, and chemotherapy resistance. Moreover, circRNAs have emerged as potential biomarkers for lung cancer diagnosis and prognosis and targets for developing new treatments. In this review, we will summarize recent progresses in identifying the biogenesis, biological functions, potential mechanisms, and clinical applications of these molecules for lung cancer diagnosis, prognosis, and targeted therapy.
Introduction
According to the cancer statistics of 2020, lung cancer is the second most frequent human tumor and the leading cause of cancer-related deaths worldwide (1). Lung cancer includes small-cell lung carcinoma (SCLC) and non-small-cell lung carcinoma (NSCLC). NSCLC accounts for approximately 85% of all cases (2) and is mostly represented by lung adenocarcinoma (ADC), lung squamous cell carcinoma (SCC), and large-cell lung carcinoma.
Despite the recent rapid advances in diagnostic tumor biomarkers and therapeutic approaches such as surgery, chemotherapy, radiotherapy, targeted therapy, or immunotherapy, the 5-year overall survival for lung cancer still remains poor (3). Therefore, finding new biomarkers and therapeutic targets for this cancer is paramount.
The initiation and progression of lung cancer is an extremely complex process that involves genetic mutations, the role of the tumor microenvironment, and the dysregulation of epigenetic pathways (4–6). Epigenetic changes in lung cancer such as histone modifications (7), DNA methylation (8), and noncoding RNAs (ncRNAs) (9) have been extensively studied. Cellular ribonucleic acids (RNAs) can be divided into coding and non-coding RNAs; these latter kind include small interfering RNAs (siRNAs), small nuclear RNAs (snRNAs), small nucleolar RNAs (snoRNAs), PIWI-interacting RNAs (piRNAs), transfer RNAs (tRNAs), ribosomal RNAs (rRNAs), microRNAs (miRNAs), long non-coding RNAs (lncRNAs), and circular RNAs (circRNAs) (10–12).
Several evidence have revealed that numerous ncRNAs are dysregulated and involved in lung cancer initiation and progression (13–15). In particular, miRNAs and lncRNAs have been shown to be involved in lung cancer progression—for example, microRNA-485-5p suppresses growth and metastasis in non-small-cell lung cancer cells by targeting IGF2BP2 (16), whereas miRNA-124 suppresses cell proliferation in NSCLC by the down-regulation of SOX8 expression (17), and lncRNA H19 promotes lung cancer proliferation and metastasis by inhibiting the miR-200a function (18). lncRNA TUC338 promotes the invasion of lung cancer by activating the MAPK pathway (19). While the role of miRNAs and lncRNAs in cancer development is currently being progressively unraveled, the functions of circRNA in the initiation and progression of lung cancers need further investigation.
As a new type of ncRNAs with distinct properties and diverse functions, unlike lncRNA and miRNA, circRNA is an endogenous RNA molecule with covalently closed loop structures without termination at 5′ caps and 3′ poly(A) tails. The first circRNA was observed in viroid (20). Next, circRNAs were discovered in the cytoplasm of eukaryotic cells by electron microscopy (21) and were earlier mainly considered to be “junk RNAs” produced by aberrant splicing events (22), until it was hypothesized as a possible function of the testis-specific circRNA, expressed by Mus spretus Sry genes (23). Recently, with the development of high-throughput RNA sequencing and bioinformatics algorithms, thousands of circRNAs in eukaryotes have been identified, including plants, fungi, protists, fish, worms, and mammals (24–26). circRNAs are composed of exonic and/or intronic sequences and are primarily generated by back-splicing, a non-canonical alternative RNA splicing event mediated by the spliceosome and regulated by a combination of cis-elements and trans-factors (27, 28). According to biogenesis from a different mechanism, circRNA can be divided into three types: exonic circRNA (ecircRNNAs) (29), intronic RNAs (ciRNAs) (30), and exon–intron circRNAs (EIcircRNA) characterized by the co-presence of both exons and introns (30).
Despite the lack of polyadenylation [poly(A)] and capping, circRNAs generally localize to the cytoplasm (29), while the exon–intron circRNAs and ciRNAs generally localize in the nucleus and promote the transcription of their parental genes via interaction with the U1 small nuclear ribonucleoprotein (31). circRNAs are characterized by abundance (32), stability (33), translational capacity (34), and cell specificity/tissue specificity/developmental stage specificity (29, 35). Numerous studies have demonstrated that circRNAs play a critical role in gene expression (36), act as miRNA sponge (37), interact with RNA binding protein (38), and translate to small peptides (39). Moreover, circRNAs could play paramount roles in physiological processes, including aging (40), myogenesis (41), male reproductive function (42), adipogenesis (43), innate immune response (28), synaptic function (44), insulin secretion (45), and mitochondrial ROS (mROS) output (46). On the other hand, dysregulation circRNAs are also involved in various human diseases, including neurological disorders (47), cardiovascular diseases (32), chronic inflammatory diseases (48), diabetes mellitus (49), and especially human cancers (50, 51).
Liquid biopsy is a biopsy that uses body liquids as the sample source to diagnose, predict the outcome of, or monitor the development of human diseases (52), and because of their high stability, abundant expression, and high specificity, circRNAs detected with liquid biopsy could become promising biomarkers for human diseases (53). circRNAs are involved in the pathogenesis of lung cancer through the regulation of proliferation and invasion (54), cell cycle (55), stemness of lung cancer stem cell (56), chemotherapy resistance (57), and tumor microenvironment (58, 59). Therefore, we will summarize recent progresses in identifying the biological and prognostic role of circRNAs and their potential exploitation as actionable targets in lung cancer.
Biogenesis and properties of circRNAs
The classification and biogenesis of circRNA
circRNAs are generated by RNA polymerase II from pre-mRNA (60). These circRNAs are distinct from their linear RNA counterparts because they lack the 5′–3′ ends and poly-adenylated tail due to their closed covalent structure, which usually decides the fate of many RNA transcripts (29). According to the generation mechanism, the circRNAs can be divided into exonic circRNAs (ecircRNAs) originated from one or more exonic sequences (61) and intronic circRNAs (ciRNAs) originated from intronic sequences (30), while the exon–intron circRNAs (EIciRNAs) can be produced from intron-containing exons (62). Exonic circRNAs are cytoplasmic and result from pre-mRNA splicing where the 3′ splice donor attaches to the 5′ splice acceptor forming an exonic circRNA (61). Exon–intron circRNAs are predominantly nuclear and composed of introns and exons that interact with U1 snRNP and promote the transcription of their parental genes (31). Intronic RNA (ciRNA) formation depends on the 7-nt GU-rich element near the 5′ splice site and an 11-nt C-rich element close to the branchpoint site (30).
At the moment, there are several hypotheses to explain circRNA biogenesis, including (1) intron-pairing-driven circularization (2), RNA-binding protein (RBP)-driven circularization (3), exon skipping, and (4) intron lariat circularization (63).
The intron-pairing-driven circularization model suggests that introns flanking the exon/exons of a pre-mRNA have a structure capable of joining each other. The flanking introns approach each other, creating a secondary conformation that makes the splice sites possibly carry on back-splicing and generate exonic circRNA. Adenosine deaminase 1 acting on RNA (ADAR1) is involved in the intron pairing process of circRNA formation (64).
In the RBP-driven circularization model, RBPs bind to pre-mRNAs to connect the flanking introns. This process is induced by protein dimerization, which forms an RNA loop. Muscle blind-like splicing regulator 1 (MBNL1) protein is the most frequent RBP responsible for circRNA biogenesis (65). In the exon skipping model, one or multiple exons of the mature mRNA will be missing, whereas the lariat-driven circularization hypothesis is based on the binding of adjacent exons, leading to the formation of linear mRNA and exon–intron or multiple-exon circRNA transcript with lariat structure. Finally, the fourth proposed mechanism is the intron lariats, which can form ciRNAs (66).
Role of cis-elements and trans-factors in circular RNA formation
Mounting evidence have shown that back-splicing requires spliceosomal machinery and that the regulation of circRNA formation depends on both cis-regulatory elements and trans-acting factors. In this review, we shall focus on how cis-regulatory elements and trans-acting factors influence the biogenesis of circRNA (Figure 1).
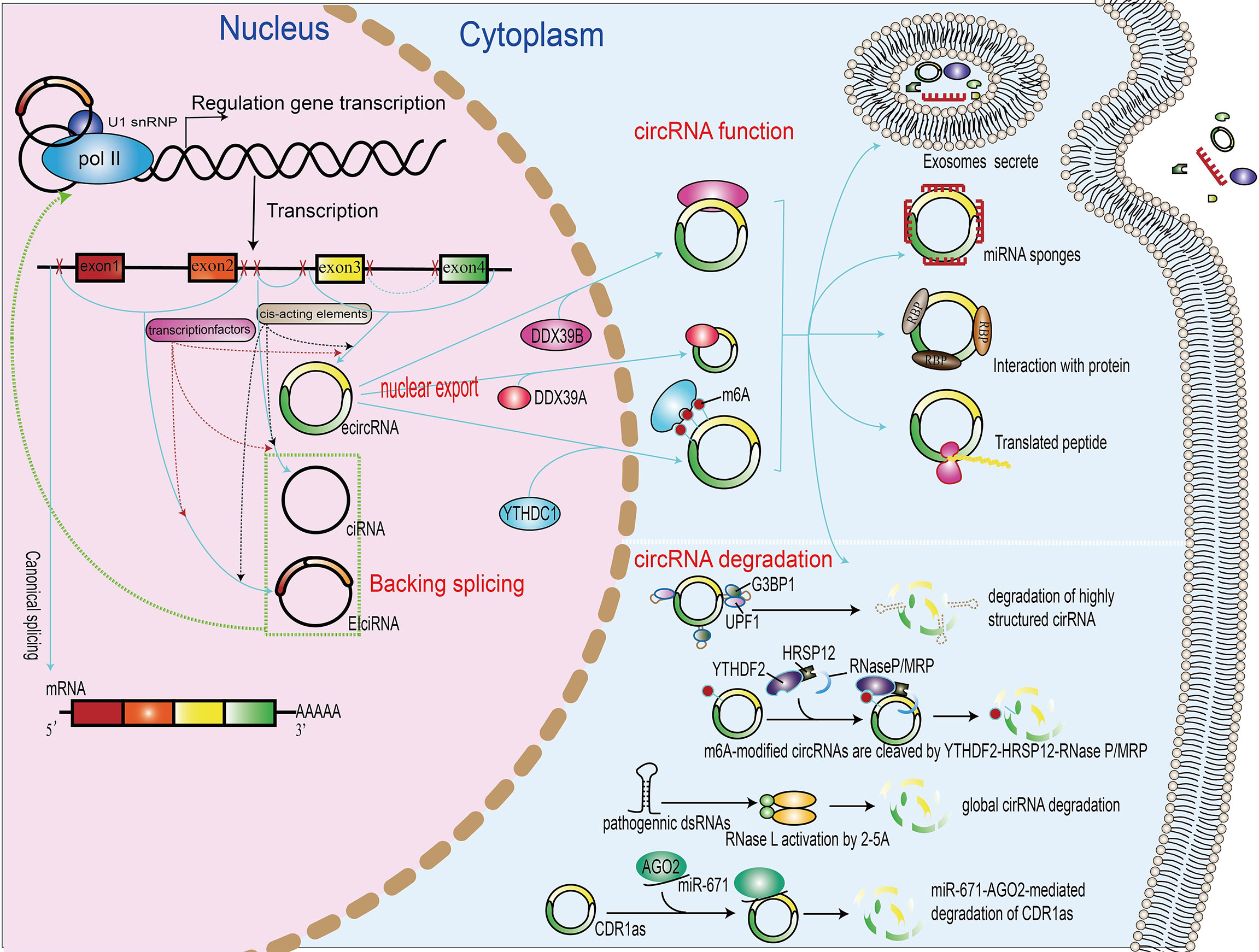
Figure 1 Biogenesis, nuclear export, functions, and degradation of circRNAs. In the nucleus of eukaryotic cells, DNA is transcribed to form precursor mRNA (pre-mRNA), which contains coding exons and introns. Differently from linear mRNAs, which are formed regulated by cis-acting elements and transcription factors, according to the generation mechanism, the circRNAs can be divided into exonic–intronic circRNAs (ecircRNAs), ciRNAs, and circRNAs (EIciRNAs). The proteins UAP56, URH49, and YTHDC1 can promote the nuclear export of circRNAs. The functions of circRNAs include the regulation of gene transcription or splicing, acting as miRNA sponge, binding with RNA-binding protein, translation into peptide or protein, and packing in exosomes. The degradation of circRNA includes miRNA-directed circRNA decay, m6A-mediated circRNA decay, RNase L-mediated circRNA decay, and overall structure-mediated circRNA decay.
Cis elements regulate the formation of circRNAs
Back-splicing often requires regulatory elements residing in introns flanking circularized exons. Flanking intronic complementary sequences (ICSs) can promote exon circularization and then give rise to the formation of circRNAs (27). Most circRNAs in mammals are produced from internal exons with long flanking introns usually containing ICSs. RNA pairing formed across introns that flank back-spliced exons is expected to bring the distal single strand into close proximity to facilitate circRNA biogenesis (61). Consistently, the elimination of RNA pairs significantly reduces and, sometimes, even removes circRNA production, as revealed by mutagenesis analysis in circRNA expression vectors (67, 68)—for example, in cultured mouse cells, a previous study showed that circSry, derived from Sry gene, is flanked by many intronic complementary sequences, and the presence of long inverted repeats (IR) flanking of Sry gene results in the formation of the Sry circular transcript (69). Furthermore, the fusion-circRNAs (f-circRNAs) that originated from aberrant chromosomal translocations in cancers also supported the view that intronic RNA pairing across circle-forming exons is critical for enhanced circRNA formation (70). F-circM9 was identified to be produced by the MLL/AF9 fusion gene that contains the MLL gene exon 1–8 and AF9 gene exon 6–11. After translocation, the trans intronic sequences of the MLL and AF9 genes are juxtaposed in cis, which subsequently facilitates circRNA generation by forming newly paired ICSs flanking the translocation breakpoint (70). ICSs even have 23 nucleotides flanking intronic regions and also promote the formation of natural circRNA in platelets (27). It has been shown that Alu elements are abundant and constitute 11% of the reference human genome, and nearly half of them is located in human introns (71). A recent study demonstrated that complementary inverted-repeat Alu elements drive the RNA pairs, which enhances the exon circularization efficiency and leads to multiple circular RNA transcripts produced from a single gene (27). RNA pairing formed across flanking introns generally promotes circRNA formation (72). Furthermore, RNA pairing within individual introns generally facilitates canonical splicing in linear RNA, competing with RNA pairing across flanking introns and leading to reduced circRNA formation from the same gene locus (36). These recent findings together reveal that cis elements play a key role in circRNA biogenesis.
Trans-acting factor regulate the formation of circRNAs
Previous studies have demonstrated that RNA-binding proteins are also involved in spliceosome function via the modulation of alternative splicing which control the information of circRNAs. In Drosophila cells, using RNAi depletion or the pharmacological inhibition of spliceosome-related gene expression inhibits canonical pre-mRNA processing and increases the output of circular RNAs (73). Another study likewise shows that the pharmacological inhibition of the spliceosome promotes the biogenesis of circRNAs in mouse brain (74). Therefore, one can assume that spliceosome regulates the activity of back-splicing and canonical splicing and then affects the biogenesis of circRNAs and mRNA. The abundance of core spliceosomal factors promotes the biogenesis of circRNAs and reduces the product of linear mRNA. The RNA-binding proteins regulate the back-splicing process through an interaction with ICSs, whereas the double-stranded RNA-binding domains in RBPs bind to ICSs and stabilize the transiently formed intronic RNA pair flanking—for example, the NF90/NF110 encoded by interleukin enhancer binding factor 3 gene, which includes a double-stranded RNA-binding domain, via binding to intronic inverted-repeat Alu elements, promotes circRNA production (28). The immune factors NF90 and/or NF110, each containing two dsRNA-binding domains (dsRBDs), promote circRNA formation by direct binding to IRAlus formed in nascent pre-mRNA. In the endogenous NF90 knockdown condition, the re-introduction of wild-type NF90, but not NF90 mutants with dsRBD truncations, rescued circRNA expression, further supporting that both dsRBDs of NF90 are required for circRNA production (28). However, dsRBPs can also inhibit circRNA formation by destabilizing the RNA pairing—for example, it was reported that the depletion of DHX9 from nuclear RNA helicase, known to bind to inverted-repeat Alu elements, thus regulating the biogenesis of circRNAs, leads to the double-stranded RNA accumulation defects and increased the circular RNA production (75). ADAR1, a double-strand RNA-editing enzyme, edits A-to-I of inverted-repeat Alu elements flanking circRNA-forming exons and significantly increases circRNA expression (24). RNA-binding proteins without a dsRBD domain also participate in the regulation of circRNA levels by direct binding to specific RNA motifs—for instance, Quaking (QKI), which is an alternative splicing factor, promotes the interaction between QKI motifs and introns flanking circRNA-forming exons, thus leading to the biogenesis of hundreds of circRNAs with a paramount role in human epithelial–mesenchymal transition (EMT) (76). Other splicing factors have been found to regulate back-splicing in different biological settings: RBM20 promotes the biogenesis of circRNAs by regulating the exclusion of specific exons (77) and heterogeneous nuclear ribonucleoprotein L (HNRNPL) regulates back-splicing and promotes the biogenesis of circRNAs and tumor growth in prostate cancer (76). Splicing factor-regulated back-splicing events also occur in other species. In Drosophila melanogaster, the splicing factor Muscle blind (Mbl) regulates circRNA production from its own pre-mRNA by binding to multiple Mbl-binding sites in introns flanking the circularized exon in Mbl pre-mRNA (36). These recent findings together revealed that circRNA production is highly dependent on biological background and tightly regulated in cells using different cis elements and trans factors that are back-splicing specific.
The properties of circRNAs
circRNAs have the characteristics of abundance (32), specificity (25), stability (33), and translational capacity (34). It has also been shown that circRNAs usually have higher expression levels in low-proliferating cells, and developing tissues such as the heart, lung, and brain usually show higher levels of circRNA (78). These evidence show that the expression patterns of circRNAs are tissue and development specific. While some studies found that the expression of circRNAs was not correlated with their host mRNAs, only in some special circumstances were the expression levels of circRNAs higher than those of their host mRNAs (79). Most of the circular RNAs are cytoplasmic due to the lack of 5′ caps and 3′ poly-A tails that confer resistance to ribonuclease R (RNase R)-dependent degradation (33). The half-life of circRNAs far exceeds that of their linear RNA counterparts (48 and 10 h, respectively (61)). These characteristics make circRNAs ideal biomarkers of cancer.
Numerous results have shown that the expression of circRNAs exhibit specificity to certain tissues and developmental stage—for example, one study found that circRNAs were extraordinarily enriched in the mammalian brain compared to other tissues (80).
Although circRNAs belong to non-coding RNAs, recent studies unraveled that circRNAs can be translated into proteins or peptides—for example, circ-FBXW7 encodes a novel 21-kDa protein, called FBXW7-185aa, and the depletion of FBXW7-185aa promotes malignant phenotypes in glioma (81).
The nuclear export of circular RNAs
In spite of the fact that most circRNAs are primarily cytoplasmic while circRNAs containing retained introns are nuclear (31), some circRNAs are formed in the nucleus and then transported to the cytoplasm.
Their elaborated control of transport is essential for circular RNAs to exert their functions properly in eukaryotic cells. A recent study has demonstrated that m6A modification and other proteins modulate the circRNA export and that knock-down of the DExH/D-box helicase Hel25E leads to the nuclear accumulation of circRNAs longer than 800 nucleotides. Moreover, the depletion of UAP56 or URH49 results in long and short circRNA enrichment in the nucleus, respectively (82). These evidence indicate that the lengths of mature circRNAs may be a decisive factor for their nuclear export. In addition, a more recent study suggest that N6-methyladenosine RNA modification plays a significant role in the regulation of the nuclear export YTHDC1, a circRNA m6A reader that mediates the export of methylated mRNA from the nucleus to the cytoplasm as demonstrated by the depletion of YTHDC1 that leads to the accumulation of m6A-containing transcripts in the nucleus. Another study demonstrated the interaction of YTHDC1 with the splicing factor and nuclear export adaptor protein SRSF3, leading to the increased nuclear export of m6A-containing transcripts (83).
Very similarly, in colorectal carcinoma patients, Chen et al. recently identified the pro-metastatic effect of the upregulation of the m6A-modified circRNA circNSUN2 that enhances the stability of high-mobility group AT-hook protein 2 mRNA. Furthermore, the m6A modification increases the export of circNSUN2 to the cytoplasm (84) (Figure 1).
To conclude, it can be assumed that the mechanisms for extracellular transportation are not yet fully understood, and further studies on this function are certainly warranted.
The degradation of circular RNAs
The circular structure confers resistance of circRNAs to degradation by RNA decay nuclease and other proteins. Therefore, circRNAs have much higher stability than their cognate linear transcripts (85). Nevertheless, under stress conditions, circRNAs can also be degraded through different mechanisms. Thomas Hansen et al. revealed that miRNAs directly bind circRNAs and promote their degradation process. miR-671 directly cleaves a circular antisense transcript of the CDR1 locus in an Ago2-slicer-dependent manner, whereas the miR-671 target site of the circRNA CDR1as/ciRS-7 recruits miR-671-loading Ago2 to CDR1as/ciRS-7, causing endonucleolytic cleavage by Ago2 and subsequent exonucleolytic RNA degradation (86). In addition, many endonucleases participate in the circRNA degradation process. Under viral infection, double-stranded RNA (dsRNA) activates the RNase L that, in turn, promotes the degradation of circRNAs (87). Fischer et al. have revealed that, during this novel structure-mediated circRNA decay, UPF1 interacts with G3BP1 and accelerates the structure-mediated circRNA decay degradation (88). Interestingly, N6-methyladenosine RNA modifications also affect the degradation of circRNAs; m6A-containing circRNAs use m6A as a recruiter of the m6A reader protein YTHDF2 and adaptor protein HRSP12. Eventually, HRSP12 directly binds to a GGUUC motif on circRNAs and serves as a bridge to bring YTHDF2 and endoribonuclease RNase P/MRP together, thus enabling RNase P/MRP to initiate circRNA degradation (89) (Figure 1).
The roles of circRNAs in physiological conditions
Previous studies have demonstrated that circRNAs contribute to gene regulation through a variety of actions, including regulating transcription, sponging microRNAs, interacting with RNA-binding proteins, and protein translation. The potential functions of circRNAs have been extensively studied (Figure 1). A number of studies have begun to reveal that at least some circRNAs could play crucial roles in physiological conditions by distinct modes of action at the molecular level.
circRNAs regulate transcription and splicing
ciRNAs and EIciRNAs are the products of processed intron lariats and back-splicing with retained introns, respectively. Both of them are mostly nuclear and regulate gene expression at the transcriptional and post-transcriptional levels (31). EIciRNAs interact with U1 small nuclear ribonucleoproteins (snRNP) and RNA PoII in the promoter region of the parental gene to enhance gene expression in cis—for instance, the blockage of circEIF3J and circPAIP2 reduces the transcriptional level of host genes (31). As expected, the processing of circRNAs affects the alternative splicing of their linear cognates and competes with pre-mRNA splicing, suggesting a negative relationship between circRNA and their linear isoforms (36)—for example, circMbl derived from the circularization of the second exon of the MBL competes with linear MBL mRNA to maintain the balance between canonical splicing and circRNA production (36). However, in addition to exon skipping, whether there are additional regulators that affect exon circularization remains to be explored. Recently, a study showed that circSEP3, derived from exon 6 of the SEPALLATA3 gene, concurs to form a RNA–DNA complex that participates in the transcription pausing and alternatively splicing process of the SEP3 gene (31). The above-mentioned research shows that some localized nuclear circRNAs can modulate gene transcription and splicing via different mechanisms
circRNAs act as miRNA sponges
A scenario in which competitive endogenous RNA (ceRNA) transcripts with shared microRNA (miRNA) binding sites compete for the post-transcriptional control expression of mRNA is hypothesized (90). In the case of cytoplasmic circRNAs, it acts as miRNA sponge and stabilizes mRNA stability, preventing miRNA-mediated degradation. There are many evidences showing that circRNAs act as miRNA sponges: as a sponge for miR-7, ciRS-7 contains more than 70 selectively conserved miR-7 target sites, is highly and widely connected with AGO proteins, strongly suppresses miR-7 activity, and increases the expression of miR-7 targets (37). circRNA Sry, the testis-specific circRNA in sex-determining region Y (Sry), is a potent miRNA 138 sponge that contains 16 putative target sites for miR-138 and directly binds miRNA 138 to participate in the development of mouse testis (37). Similarly, a recent study indicated that circHIPK3, derived from Exon2 of the HIPK3 gene, contains many potential binding sites of miR-124 and acts as a sponge-inhibiting miR-124, an activity with subsequent pivotal biological effects in human cells (91). It has been shown that circZNF91, sponge for miR-23b-3p, modulates human epidermal stem cell differentiation (92), whereas circBIRC6 directly binds miR-34a and miR-145 and modulates the pluripotency and differentiation of human embryonic stem cells (93). These evidence suggest that circRNAs play a crucial role in controlling the stability and quantity of miRNAs.
circRNAs interact with RNA-binding proteins
Although circRNAs mostly act as miRNA sponges, many research studies have been carried out to explore the functional relevance of circRNA–protein interactions. The most well-known proteins interacting with RNA molecules are the RNA-binding proteins (RBPs). RBPs contain a large class of over 2,000 proteins that interact with transcripts and play an important role in RNA metabolic processes (94). Previous evidence have shown that circRNAs interact with RBPs to form specific circRNA–protein complexes and affect protein localization and function. Exon–intron circRNAs interact with U1 snRNP and regulate the expression of their related parental genes (31). Circular RNA circ-Foxo3 is mostly cytoplasmic, where it interacts with the anti-senescent protein ID-1, the transcription factor E2F1, FAK, and HIF1α, resulting in increased cellular senescence (95). circ-Foxo3 interacts with cell cycle protein cyclin-dependent kinase 2 and cyclin-dependent kinase inhibitor 1 and leads to the formation of a ternary complex, thus promoting the cell cycle progression of non-cancer cells (96). Although circ-Foxo3 is minimally expressed in tumor specimens, the expression of circ-Foxo3 gradually increases during cancer cell apoptosis. Other studies demonstrate that circ-Foxo3 and MDM2/p53 form the complex circ-Foxo3/MDM2/p53 that promotes MDM2-induced p53 ubiquitination and subsequent degradation. circ-Foxo3 plays an important regulatory role in cell apoptosis because it prevents MDM2 from inducing Foxo3 ubiquitination and degradation, resulting in elevated levels of Foxo3 protein (97). It has also been reported that circACC1 interacts with AMPK and elevates the stability of AMPK holoenzyme, promotes the enzymatic activity of the AMPK holoenzyme by forming a ternary complex with the regulatory β and γ subunits of AMPK holoenzyme, and plays a critical role in both fatty acid β-oxidation and glycolysis (98). Abdelmohsen K and colleagues provided evidence that CircPABPN1 prevents HuR binding to PABPN1 mRNA that results in the suppression of PABPN1 translation (99). Another typical example is circ-AMOTL1, which directly binds to PDK1 and AKT1, leading to AKT1 phosphorylation and nuclear translocation, and plays a cardio-protective role in cardiovascular disease (100). Furthermore, in GBM, circSMARCA5 acts as sponge for SRSF1 as well as participates in the process of VEGFA mRNA splicing regulation and angiogenesis (101). In summary, these evidence indicated that circRNAs interact with RNA-binding proteins and play a significant role in protein function regulation.
CircANRI binds pescadillo homologue 1 (PES1) and is an essential 60S-preribosomal assembly factor that impairs exonuclease-mediated pre-rRNA processing and ribosome biogenesis in vascular smooth muscle cells and macrophages (48). CircHECTD1 promotes fibroblast proliferation and migration via interaction with HECTD1/ZC3H12A (102). CircZKSCAN1 competes with the binding between FMRP and β-catenin-binding protein cell cycle and apoptosis regulator 1 (CCAR1) mRNA, with subsequent inhibition of WNT signaling and HCC stemness (103). CircARSP9 increases the susceptibility of HCC cells to NK cell cytotoxicity in HCC cells by the upregulation of UL16-binding protein (ULBP1) expression (104). AR suppresses CircARSP91 expression by upregulating ADAR1, while CircARSP91 plays an inhibitory role in HCC tumor growth (105). Circular RNA FECR1 controls breast cancer tumor growth by the recruitment of TET1 to FLI1 promoter, determining the over-expression of FLI1 (106). circRNA−MTO1 modulates the Eg5 protein expression, suppresses cell viability, promotes monastrol-induced cell cytotoxicity, and reverses monastrol resistance (107) via tumor necrosis factor receptor-associated factor 4 (TRAF4). Circ-Dnmt1 interacts with both p53 and AUF1, promotes the nuclear translocation of both proteins, and plays an oncogenic role in breast cancer cell autophagy (108). CircECE1 was reported to interact with c-Myc to prevent speckle-type POZ-mediated c-Myc ubiquitination and degradation and exert the proliferation and metastatic capability of osteosarcoma cells (109). circRNAs interact with proteins to influence their cellular functions, thereby regulating gene transcription and inhibiting cell cycle progression but promoting cardiac senescence, apoptosis, and cell proliferation.
circRNAs can be translated
Due to lack of 5–3′ polarity, polyadenylated tails, and internal ribosome entry sites (IRES), circRNAs were initially defined as a noncoding RNA. Notwithstanding, a recent study found that, under certain conditions, circRNAs possess translational ability and can code functional peptides—for example, Circ-ZNF609 contains an open reading frame span that can be translated into a protein that controls myoblast proliferation (39). Moreover, m6A modification regulates circRNA translation and METTL3 and YTHDC1 regulate back-splicing, while the knockdown of METTL3 downregulates Circ-ZNF609.
Another study shows that YTHDF3 and eIF4G2 recognize the m6A modifications in Circ-ZNF609 and modulate its translation (110). It was suggested that circular RNA derived from the long intergenic non-protein-coding RNA p53-induced transcript (LINC-PINT) can code an 87-amino-acid peptide. This peptide directly interacts with PAF1c, inhibits the transcriptional elongation of multiple oncogenes, and plays a suppressive role in glioblastoma cells (111). Circ-FBXW7, highly expressed in normal human brain, has been reported to encode a novel 21-kDa protein, named FBXW7-185aa, that reduces the half-life of c-Myc by antagonizing USP28-induced c-Myc stabilization, thus acting as tumor suppressor in glioblastoma cells (34). In addition, circ-SHPRH contains an open reading frame.
The IRES translate a functional protein named SHPRH-146aa and play an additional inhibitory effect in GBM, protecting the full-length SHPRH from ubiquitination (112). Similarly, circβ-catenin is highly expressed in liver cancer tissues where it promotes liver cancer cell growth. Another study shows that Circβ-catenin is predominantly localized in the cytoplasm and encodes a novel 370-amino-acid β-catenin isoform via a linear β-catenin mRNA. This β-catenin isoform increases the stability of full-length β-catenin by antagonizing GSK3β-induced β-catenin phosphorylation and degradation and eventually results in the activation of the Wnt pathway (113). Circ PPP1R12A contains an open reading frame encoding the functional protein circPPP1R12A-73aa that promotes the growth and metastasis of colon cancer via activating the Hippo-YAP signaling pathway (114).
We named AKT3-174aa as circ AKT3 encoding a 174-amino-acid novel protein that competes with phosphorylated PDK1, reduces AKT-thr308 phosphorylation, and plays an inhibitory role in the tumorigenicity of GBM cells (115).
CircE7 is derived from human papillomaviruses and, via its interactionwith polyribosomes, is translated into the E7 oncoprotein. E7 oncoprotein depletion significantly suppresses cancer cell growth and tumor xenografts (116). CircLgr4 is highly expressed in colorectal tumors and colorectal cancer stem cells. The knockdown of circLgr4 inhibits colorectal cancer stem cell (CSC) self-renewal, colorectal tumorigenesis, and invasion. CircLgr4 encodes the circLgr4-peptide, which interacts with LGR4 to activate the LGR4-Wnt signaling pathway that, in turn, drives the self-renewal of colorectal CSCs (117). In colon cancer cell lines and tissue, circ FNDC3B is mostly localized in the cytoplasm, and its over-expression inhibits the proliferation, invasion, and migration of colon cancer cells. CircFNDC3B also encodes a novel protein circFNDC3B-218aa that suppresses colon cancer progression (118). These evidence pave the avenue to future studies on the translational function of circRNA.
Other physiological functions
A growing body of evidence show that circRNAs play a critical role in the physiological processes, including exosomes secretion (119), aging (40), myogenesis (41), male reproductive function (42), adipogenesis (43), innate immune response (28), synaptic function (44), insulin secretion (45), and mROS output (46). Exosomes are extracellular membranous micro-vesicles with a diameter of 40–160 nm and secreted by various cell types (120). Exosomes derived from host cells can be taken up and exert biological effects both on adjacent and distant cells (121). Exosomes have been implicated in the occurrence and development of many diseases, including cancer (122). Exosomes may include biological molecules such as lipids, proteins, DNA, and non-coding RNAs (ncRNAs), and their content and biological effects depend on the host cells.
ncRNA-containing exosomes play a role in disease progression, including cancer (123). A recent study by Zhang N et al. reported that circSATB2 is highly expressed in NSCLC cancer exosomes and promotes the proliferation, migration, and invasion of NLCSC cells. SATB2-containing exosome can be taken up by NLCSC cells to promote cell–cell communication, progression of NSCLC cells, proliferation of normal bronchial epithelial cells, and lymphatic spreading. The receiver operating characteristic (ROC) analysis curve of exosomial circSATB2 shows an area under the ROC curve (AUC) value of 0.660 and 0.797 in serum from patients with lung cancer and metastatic lung cancer patients, respectively. These results indicate that exosomial circSATB2 could act as a blood detection index for the diagnosis of lung cancer and lung cancer metastasis with high sensitivity and specificity (124). Similarly, it has also been reported that circRNA 002178 exosomes from the plasma of LUAC patients were highly expressed in LUAC tissues and LUAC cancer cells. Functional studies have demonstrated that circRNA-002178 enhances PDL1 and induces T cell exhaustion via sponging miR-34 in cancer cells. Interestingly, circRNA-002178 could be exploited as a novel diagnosis biomarker for lung cancer because the AUCs have been demonstrated to be higher (0.9956) in the exosomes derived from cancer cells than in those derived from normal human bronchial epithelial cells (125).
Other evidence indicate that circRNAs play a crucial role in the mitochondria, including non-alcoholic steatohepatitis pathogenesis. The steatohepatitis-associated circRNA ATP5B Regulator (SCAR) is primarily localized in the mitochondria and alleviates meta-inflammation by reducing the mROS output. Mechanistic analyses have demonstrated that circRNA SCAR binds to ATP5B and inhibits mPTP by blocking the CypD–mPTP interaction with subsequent reduction of both mROS generation and fibroblast activation (46). Therefore, circRNA SCAR is a potential therapeutic target for nonalcoholic steatohepatitis. CircSamd4 is upregulated during the differentiation of mouse C2C12 myoblasts into myotube, whereas the overexpression of circSamd4 interferes with the binding of PUR proteins to the Mhc promoter and promotes myogenesis (41). CircArhgap5-2 promotes adipogenesis through maintaining the global adipocyte transcriptional program involved in lipid biosynthesis and metabolism (43). Flies missing circular Boule (circBoule) RNAs have decreased male fertility, under heat stress conditions, and the knockdown of circBoule decreases the fertilization capacity. Moreover, circBoule RNAs inhibits\ the spermatogenesis process by interacting with heat shock proteins (HSPs) and promoting their ubiquitination (42). CircSfl is upregulated in the brain and muscle, and its overexpression significantly extends the lifespan of fruit flies, whereas mechanistic analyses have demonstrated that circSfl is translated into a protein sharing some functions with the full-length Sfl protein encoded by the host gene and extending the lifespan of cells (39). On the whole, these studies demonstrate that circRNAs play an important role in physiological processes.
The roles of circRNAs in lung cancer pathological conditions
Numerous circRNAs have been found to be dysregulated in lung cancer tissues, playing oncogenic or tumor-suppressor roles. Extensive evidence has demonstrated that there are many differentially expressed circRNAs in the tissues and plasma of lung cancer patients. Chen et al. analyzed the circular RNA expression by high-throughput sequencing of plasmatic exosomes from patients with early-stage lung adenocarcinoma and detected 182 differentially expressed exosomal circRNAs, which included 105 upregulated and 78 downregulated compared with the controls (126). Using next-generation sequencing, Zhang et al. found that 35 circRNAs were aberrantly expressed in small-cell lung cancer tissue. Among these, five circRNAs were significantly upregulated, and 30 circRNAs were significantly downregulated (127). The roles of dysregulated circRNAs in lung cancer include proliferation, migration, invasion, apoptosis, cell cycle, stemness of lung cancer stem cell, chemotherapy resistance, tumor metabolism, tumor microenvironment (TME), and immune evasion of lung cancer cells. Here we discuss biological activities and pathogenic mechanisms of cirRNAs in lung cancer (Figure 2).
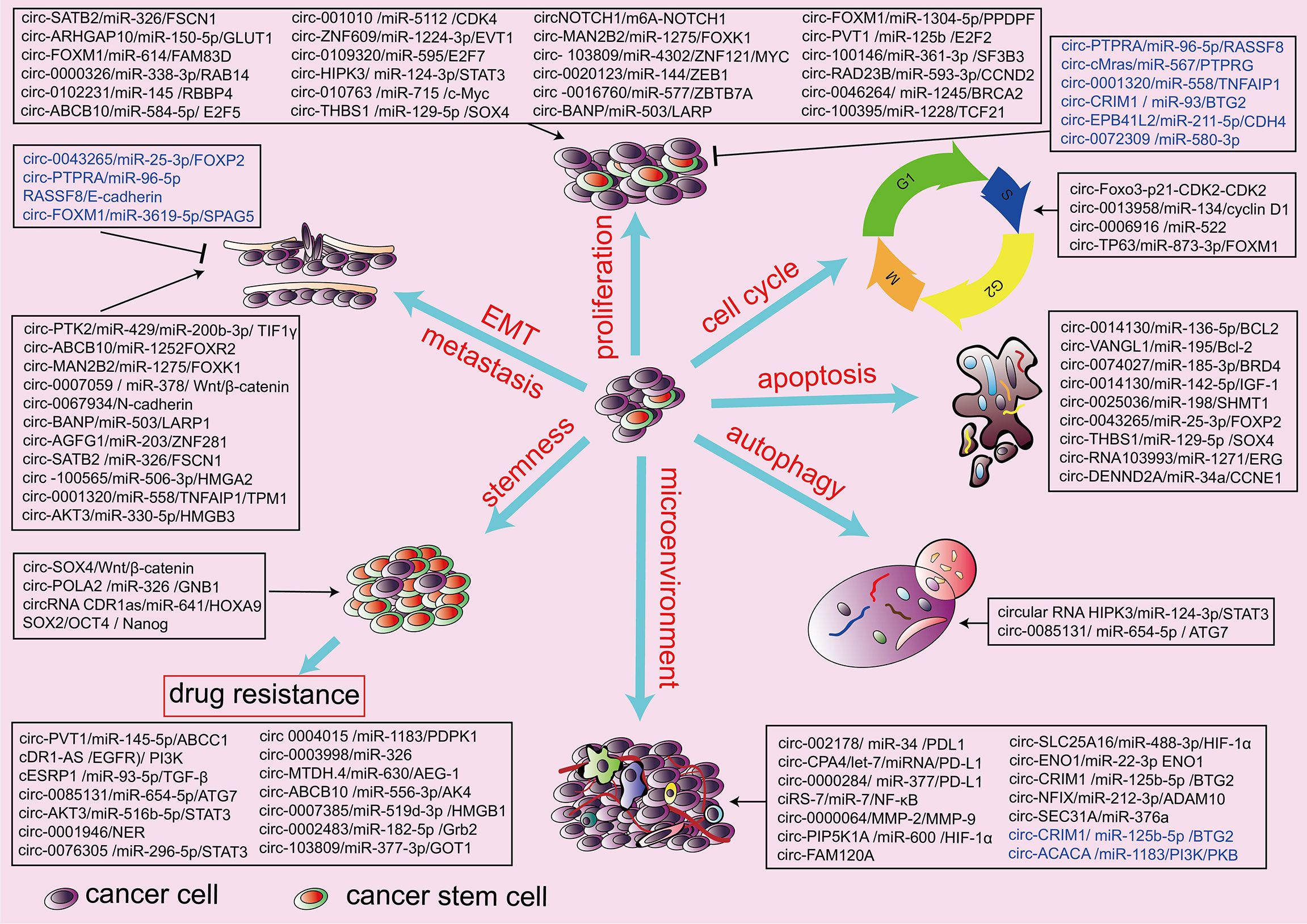
Figure 2 Biological function of circRNAs in the hallmarks of lung cancers. circRNAs are involved in the pathogenesis of lung cancers through the regulation of proliferation and invasion, cell cycle, cell autophagy, cell apoptosis, stemness, chemotherapy resistance, and tumor microenvironment. Black indicates an oncogenic role, and blue indicates a tumor-suppressive role.
circRNAs regulate the proliferation and cell cycle of lung cancer cells
As the most important hallmarks of lung cancer, the proliferation of tumor cells accounts for over 50% of the cases (128). There are many evidence that circRNAs promote carcinogenesis and the progression of lung cancer, inducing cell cycle progression and proliferation through different mechanisms. Previous studies have demonstrated that endogenous F-circEA, which is derived from the EML4-ALK fusion gene, elevates the expression of F-circEA and promotes the migration and invasion ability of lung cells. F-circEA could be a novel liquid biopsy biomarker for the diagnosis of lung cancer because it is detectable in the plasma of patients with EML4-ALK translocation (129). In addition, circSATB2 is highly expressed in tumor cells and tissues and detectable in serum exosomes from patients with NSCLC. It acts as a sponge for miR-326, resulting in the upregulation of the actin-bundling protein 1 (FSCN1) expression and promoting the proliferation, migration, and invasion of NSCLC cells (124). It has been shown that circBIRC6 is upregulated in primary human NSCLC tissues and cells and, when knocked down, inhibits growth, proliferation, migration, and invasion of these tumor cells by sponging the tumor suppressor miR-145 (130). Circ NT5E promotes human NSCLC cell progression by sponging miR-134 (131), whereas circ-ARHGAP10 promotes human NSCLC cell progression via the miR-150-5p/GLUT1 axis (132). Circ-FOXM1 promotes the proliferation of NSCLC cells acting as sponge for miR-614, thus upregulating the expression of FAM83D (133). The high expression of Circ-0000326 in LUAC is correlated with tumor size, regional lymph node status, and differentiation. Mechanistic studies show that circ-0000326 acts as a miR-338-3p sponge and upregulates the expression of the downstream target RAB14, promoting the proliferation, migration, and apoptosis of LUAC cells (134). Circ PTPRA suppresses EMT and the metastasis of NSCLC cell lines by sponging miR-96-5p and upregulation of the downstream tumor suppressor RASSF8 (135). It has been shown that circ-0102231 is mainly localized to the cytoplasm, significantly upregulating the expression of RBBP4 (136) and promoting NSCLC cell proliferation and invasion by sponging miR-145. Circular RNA cMras inhibits LUAC progression via modulation HTTof the miR-567/PTPRG regulatory pathway (137). Circ-100565 modulates the expression of HMGA2 by sponging its regulatory Mir (miR-506-3p) (138). Reduction of circ-100565 significantly suppresses in vitro proliferation, migration, and invasion and the in vivo tumor growth of NSCLC cells, whereas in patients with NSCLC high levels of circ-100565 are associated with poor overall survival.
Circ-ABCB10 promotes NSCLC progression via modulating the miR-584-5p/E2F5 regulatory pathway (139). Cytoplasmic circ-0001320 is downregulated in lung cancer cells and inhibits the growth and invasion of lung cancer cells through the miR-558/TNFAIP1 and TPM1 pathways (126). circRNA-001010 is highly expressed in NSCLC patients and acts as a molecular sponge of miR-5112, leading to the increased expression of the oncogene CDK4 (127) with subsequent proliferation, migration, and invasion of NSCLC cells.
Knockdown of circ-0087862 significantly reduces NSCLC cell viability, migration, and invasion and enhances apoptosis, and a high expression of circ-0087862 is related with poor clinical outcome in NSCLC patients. It is hypothesized that circ-0087862 increases the expression of RAB3D by sponging miR-1253 (140). A decreased expression of Circ-CRIM1 in LUAC cancer is significantly correlated with lymphatic metastasis and more advanced TNM stage and is an independent negative biomarker for the overall survival of patients with LUAC. CircCRIM1 suppresses the invasion and metastasis of LUAC through sponging miR-93 and miR-182 and increases the expression of leukemia inhibitory factor receptor, a well-known tumor suppressor (141). circ-RNA EPB41L2 plays an inhibitory role in LUAC through regulating the miR-211-5p/CDH4 axis (142). Circ-ZNF609 is significantly upregulated in LUAC tissues and cell lines and promotes cell proliferation of LUAC cells through sponge miR-1224-3p to promote EVT1 expression (143). Circ-0072309 is lowly expressed in NSCLC tissues and cell lines and plays an inhibitory role in LUAC through blocking the expression of miR-580-3p (144). Overexpression of circ-11780 inhibits the proliferation, migration, and invasion of NSCLC cells in vitro and tumor growth in vivo via the miR-544a overexpression and reduced the protein concentration of F-Box and WD repeat domain containing 7 (FBXW7) (145).
Circ-0072088 acts as sponge for miR-377-5p, leading to the upregulation of NOVA2, and promotes the proliferation and metastasis of NSCLC cells (146). Circ-0109320 is significantly more expressed in NSCLC and associated with more advanced staging and lymph node metastasis of NSCLC via sponging of miR-595 and subsequently upregulates E2F7 expression (147). A novel circRNA (circHIPK3) increases STAT3 expression by inhibiting miR-124-3p in STK11 mutant lung cancer cells (148). Moreover, silencing circHIPK3 results in the reduction of cell proliferation, migration invasion, and promotion of macroautophagy/autophagy via MIR124-3p-STAT3-PRKAA/AMPKα signaling in STK11 mutant lung cancer (148). Overexpression of circAKT3 significantly promotes proliferation, migration, and invasion by sponging miR-330-5p, resulting in increased HMGB3 expression in non-small-cell lung cancer cells (149). circRNA-010763 promotes the growth and invasion of lung cancer through the regulation of the circRNA-010763/miR-715/c-Myc axis (150). Circ-THBS1 is highly expressed in patients with metastatic NSCLC and promotes the cell proliferation of LUAC cells by sponging miR-129-5p and SOX4 overexpression (151). There is also evidence that circ- 0012673 facilitates lung cancer cell proliferation and invasion via the miR-320a/LIMK18521axis (152). Several other regulatory cascades comprising circRNA, miRNA, and mRNA have been reported in lung cancer, including circ-MAN2B2/miR-1275/FOXK1 (153), circ- 103809/miR-4302/ZNF121/MYC (154), circ-0020123/miR-144/ZEB1 and EZH2 (155), circ-FADS2/miR-498 (156), circ-0026134/miR-1256/miR-1287 (157), circ -0016760/miR-577/ZBTB7A (158), circ-0016760/miR-1287/GAGE1 (159), circ-BANP/miR-503/LARP (160), circ-001569/Wnt/β-catenin (161), circ -FOXM1/miR-1304-5p/PPDPF/MACC1 (162), Circ-FGFR3/miR-22-3p/Gal-1,p-AKT, p-ERK1/2 (163), circ-PVT1/miR-125b/E2F2 (164), circ-PVT1/miR-497/Bcl-2 (165), circ-0000735/miR-1179/miR-1182 (166), circ-100146/miR-361-3p/SF3B3 (167), circ-0043278/miR-520f/ROCK1, CDKN1B and AKT3 (168), circ-RAD23B/miR-593-3p/CCND2 and circ -RAD23B/miR-653-5p/TIAM1 (169), circ-0003645/miR-1179/TMEM14A (170), circ-P4HB/miR-133a-5p/vimentin (171), circ-0046264/miR-1245/BRCA2 (172), and circ- 100395/miR-1228/TCF21 (54).
Extensive evidence has demonstrated that circRNAs play a significant regulatory role in cell cycle transition. The dysregulation expression of circRNAs leads to tumor cell cycle progression.
circRNAs are involved in the regulation of G1/S checkpoint and participate in the development and progression of lung cancer. p21 is a cyclin-dependent kinase inhibitor that binds and inhibits the catalytic activity of Cdk2, leading to G1-phase cell cycle arrest (173). circ-Foxo3 binds to CDK2 and p21, giving origin to a circ-Foxo3-p21-CDK2 ternary complex that inhibits CDK2-dependent G1/S cell cycle progression (96). In addition, circ-0013958 acts as a sponge of miR-134, with subsequent upregulation of oncogenic cyclin D1 that plays a pivotal in the development of non-small-cell lung cancer (174). Circ-0006916 inhibits miR-522, promoting the G0/G1 progression of NSCLC (175). Circ-TP63 is highly expressed in LUSC tissues and correlates with a more advanced TNM stage. circ-TP63 competes for binding with miR-873-3p and then upregulates FOXM that, in turn, upregulates CENPA and CENP, thus promoting cell cycle progression (176).
circRNAs regulate the invasion and metastasis of lung cancer cells
Mounting evidence revealed that the aberrant expression of circRNAs is implicated in the invasion and metastasis of lung cancer —for example, circPTK2 acts a sponge for miR-429/miR-200b-3p, thus promoting TGF-β-induced EMT via TIF1γ and the invasiveness of NSCLC cell (15).
Circ-ABCB10 is also increased in NSCLC cell lines, and the knockdown of circ-ABCB10 suppresses NSCLC cell migration by promoting microRNA miR-1252 expression and suppressing Forkhead box 2 (FOXR2) (177). Circ-MAN2B2 promotes lung cancer cell invasion via the miR-1275/FOXK1 axis (153). The upregulated expression of circ-0067934 is associated with the invasiveness and migration of tumor cells and the overexpression of some EMT-associated markers such as N-cadherin (178). Circular RNA cESRP1 acts as a sponge for miR-93-5p and targets Smad 7/p21(CDKN1A), causing the inhibition of TGF-β-mediated EMT progress in lung cancer (179).
Circ-BANP promotes the migration, invasiveness, and increased expression of LARP1 in lung cancer cells via miR-93-5p and the inhibition of miR-503, respectively (160).
CircAGFG1 is elevated in NSCLC tissues and promotes invasion, migration, and epithelial–mesenchymal transition via the circ-AGFG1/miR-203/ZNF281 axis (180).
Circ-PTPRA suppresses EMT in NSCLC cell lines through the circ-PTPRA/miR-96-5p/RASSF8/E-cadherin axis and is downregulated in NSCLC tumor (135). Naringenin inhibits cell migration and invasion by regulating the circ-FOXM1/miR-3619-5p/SPAG5 axis in lung cancer (181). Several other regulatory cascades including circRNA, miRNA, and mRNA have been reported in lung cancer: circ-SATB2/miR-326/FSCN1 (124), circ-BIRC6/miR-145 (130), circ-NT5E/miR-134 (131), circ-ARHGAP10/miR-150-5p/GLUT1 (132), circ-FOXM1/miR-338-3p/RAB14 (134), circ-PTPRA/miR-96-5p/RASSF8 (135), circ-0102231/miR-145/RBBP4 (136), circ -100565/miR-506-3p/HMGA2 (138), circ-0001320/miR-558/TNFAIP1/TPM1 (126), circ-001010/miR-5112/CDK4 (126), circ-0087862/miR-1253/RAB3D (140), circ-CRIM1/miR-93 and miR-182 (141), circ-11780/miR-544a/FBXW7 (145), circ -0072088/miR-377-5p/NOVA2 (146), circ-0109320/miR-595/E2F7 (147), circ-HIPK3/miR124-3p-STAT3 (148), circ-AKT3/miR-330-5p/HMGB3 (149), circ- 010763miR-715/c-Myc (150), and circ-0012673/miR-320a/LIMK18521 axis (152).
circRNAs regulate the stemness and resistance of lung cancer stem cells
NSCLC is often a stubborn disease characterized by chemoresistance that represents a significant clinical challenge and contributes largely to disease progression, recurrence, and mortality (182). Despite the recent therapeutic advances (i.e., targeted therapy and immunotherapy), chemotherapy is still the backbone treatment for patients with advanced NSCLC cancer. Either cisplatin or carboplatin is used in combination with gemcitabine, vinorelbine, pemetrexed, or taxanes (docetaxel or paclitaxel) (183), while most patients with NSCLC are sensitive to chemotherapy at the early and the late stage. They show drug resistance that requires the identification of novel targets and development of more personalized medicine in the future (184). Despite intense efforts to overcome such resistance in lung cancer and other cancer types using novel agents (alone or in combination with chemo- and radiotherapy), the underlying mechanisms conferring this resistant phenotype in lung cancer remain largely unknown (185). It is now well established that CSCs constitute a unique subset of cells which are distinct from the bulk of tumor cells by their exclusive ability to perpetuate the growth of a malignant population of cells. This may explain the ineffectiveness of many conventional therapies and patient relapse (186). The ability of CSC to self-renew and differentiate results in tumor growth, progression, metastasis, and drug cancer treatment failure (187). This multi-drug resistance is mostly due to channel proteins that expel anticancer drugs, leading to decreased drug cell concentration (187). The stemness markers of lung cancer stem cells mainly include ALDH1, ABCG2, CD44, CD133, NANOG, and SRY-box transcription factor 2 (SOX2) (188). However, a recent study showed that circRNAs underpin cancer cell stemness by the upregulation of these stemness markers (189).On the contrary, circ-SOX4 suppresses cell proliferation, self-renewal, migration, and the invasiveness of lung tumor-initiating cells. Additionally, circ-SOX4 activates the Wnt/β-catenin pathway to maintain the stemness of lung cancer stem cells (190). Depletion of circPOLA that acts as a sponge for miR-326 with the subsequent upregulation of the G protein subunit beta 1 (GNB1) expression (56) leads to reduction of sphere formation ability, ALDH1 activity, and stemness marker expression of lung cancer cells. Consistently, the high expression of circPOLA in lung cancer tissues is associated with poor prognosis. CDR1as is upregulated in CDDP-resistant NSCLC cells, whereas its overexpression enhances the stemness signatures (SOX2, OCT4, and Nanog) via the miR-641/HOXA9 axis and confers resistance to CDDP-sensitive NSCLC cells (191).
Circ-PVT1 is highly expressed in LUAC cell lines and tissues and related to CDDP and MTA. Circ PVT1 mediates CDDP and MTA resistance via the miR-145-5p/ABCC1 axis, and Circ PVT1 knockout sensitizes tumor cells to CDDP and MTA (192). Mao et al. reported that CDR1-AS is also upregulated in cell lines and LUAC tissues and cell lines and confers resistance of patients to paclitaxel (PTX) and CDDP via the epidermal growth factor receptor (EGFR)/phosphatidylinositol 3-kinase (PI3K) signaling pathway (193).
cESRP1 is significantly downregulated in the chemoresistant cells and augments drug sensitivity by sponging miR-93-5p in SCLC, thereby upregulating CDKN1A, and subsequently inhibits transforming growth factor-β-mediated epithelial–mesenchymal transition via miR-93-5p (179). Circ-0085131 acts as a ceRNA of miR-654-5p and upregulates the expression of autophagy-associated factor ATG7, thereby modulating cell chemoresistance. Circ-0085131 is more expressed in NSCLC tumor tissue than in the adjacent normal tissue, and the higher expression is associated with the recurrence and poorer survival of NSCLC (194). He and colleagues have shown that circ-0000567 and circ-0006867 are upregulated and downregulated, respectively, in two gefitinib-resistant cell lines. The Gene Ontology and Kyoto Encyclopedia of Genes and Genomes pathway analysis indicates that dysregulation of circRNAs might play an important role in the development of acquired resistance to gefitinib in NSCLC (195). Circ AKT3 inhibits the cisplatin sensitivity of lung cancer cells via the miR-516b-5p/STAT3 axis-mediated glycolysis balance (196). Circ-AKT3 is upregulated in lung cancer tissues and cells, and knockdown of circAKT3 improves the cell sensitivity to CDDP and reduces glycolysis. Consistently, the inhibition of HIF-1α-dependent glycolysis attenuates the circAKT3-induced increase of chemo-resistance in A549 cells. Circ-0001946 promotes the viability, proliferation, migration, and invasion of NSCLC cells and inhibits cell apoptosis. Circ-0001946 has also been proven to reduce the sensitivity of NSCLC cells to CDDP by regulating the nucleotide excision repair (NER) signaling pathway (197). Circ-0076305 is elevated in CDDP-resistant NSCLC tissues and cells and exerts this effect via targeting miR-296-5p and enhancing the expression of STAT3 (198). Circ 0004015 enhances the resistance of HCC827 to gefitinib by sponging miR-1183 and upregulating the expression of PDPK1 (199). Circ-0003998 targets miR-326 and inhibits the miR-326-mediated effect on chemosensitivity (200). It is highly expressed in LUAC tissues and docetaxel-resistant cell lines, and its depletion decreases chemoresistance, inhibits proliferation, and enhances apoptosis in docetaxel-resistant LUAC cells.
Circ MTDH.4 regulates AEG-1 expression via miR-630 and promotes chemoresistance and radio-resistance in NSCLC cells (201). Circ-ABCB10 increases the expression of AK4, promotes lung cancer progression, and sensitizes lung cancer cells to cisplatin via sponging miR-556-3p (202). Knockdown of circ-103762 promotes CHOP expression and inhibits multidrug resistance in NSCLC (203). Wang et al. have shown that circRNA 002178 enhances PDL1 expression and induces T cell exhaustion via targeting miR-34. Furthermore, circ-002178 is detectable in the exosomes of plasma from LUAC patients and is a potential biomarker for the early diagnosis of LUAC (125). Circ-FGFR1 enhances the expression of the motif chemokine receptor 4 (CXCR4) via miR-381-3p, thus promoting NSCLC progression and resistance to anti-programmed cell death 1 (PD-1)-based therapy (204).
Depletion of circ-0007385 suppresses cell proliferation, migration, and invasion in NSCLC cells and cisplatin (DDP) resistance. Circ-0007385 is highly expressed in NSCLC tissues and cell lines and associated with poor overall survival.
Circ-0007385 regulates HMGB1 expression and promotes the chemoresistance in NSCLC cells via sponging miR-519d-3p (205).
Circ-0002483 is downregulated in NSCLC cells, enhances the sensitivity of NSCLC cells by sponging miR-182-5p, and regulates its target gene growth factor receptor-bound protein2 (Grb2), forkhead box protein O1 (Foxo1), and forkhead box protein O3 (Foxo3) (57). One group investigated the expression profile of circRNAs involved in the development of early-stage lung adenocarcinoma and found that circRNA 404833 and circ 406483 might be exceptional potential candidates as early diagnostic biomarkers for lung cancer. Among them, circ-404833, through targeting miR-149-5p, regulates the cell motility and gefitinib resistance in lung adenocarcinoma (172). It has been shown that Circ-SMARCA5 is downregulated in non-small-cell lung cancer. Its low expression was negatively correlated with tumor size, lymph node metastasis, and TNM stage but positively correlated with disease-free survival and overall survival (OS). Over-expression inhibits the cell proliferation of NSCLC and enhances the chemosensitivity to cisplatin and gemcitabine (206). Overall, these studies illustrate that circRNAs have significant regulatory functions in the chemotherapy resistance of lung cancer.
circRNAs regulate the tumor metabolism and tumor microenvironment of lung cancer
Tumor metabolic reprogramming is a hallmark of cancer. In terms of energy metabolism, the glycolysis pathway often has abnormal activation in cancer cells (207). There is an increasing number of evidence that have been uncovered which show that circRNAs, through a different mechanism, can activate the glycolysis pathway and participate in the progression of cancer. One such study has shown that circ SLC25A16 can accelerate the glycolysis and proliferation of NSCLC cells. Mechanistic research demonstrated that circ-SLC25A16 can act as a sponge for miR-488-3p and elevate the expression of HIF-1α that is a target gene of miR-488-3p. In turn, HIF-1α activates lactate dehydrogenase A (LDHA) by facilitating its transcription (208). It was suggested that the overexpression of Circ-CRIM1 inhibited LUAC cell migration, invasion, EMT, glycolysis, and tumor growth through reducing the expression of miR-125b-5p and resulted in the enhance expression of BTG2 (142). The depletion of circ-ACACA inhibited the proliferation and migration of NSCLC cells and also reduced the glycolysis rate. The details of this molecular mechanism include circ-ACACA by its sponging of miR-1183 and inactivating the PI3K/PKB signaling pathway (209). Circ-ENO1, which is derived from its host gene ENO1, was upregulated in LUAC. The overexpression of circ-ENO1 can promote glycolysis, proliferation, migration, and EMT and induce apoptosis in LUAC cells. circ-ENO1 acted as a ceRNA to interact with miR-22-3p, and an upregulated ENO1 expression promoted glycolysis and tumor progression in LUAC (58). Moreover, it was reported that Circ-CRIM1, through targeting miR-125b-5p, results in an increase of the expression of BTG2, leading to the promotion of glycolysis in lung adenocarcinoma cells (142). Circ-NFIX was highly expressed in NSCLC. The overexpression of circNFIX can promote NSCLC cell viability, migration, invasion, and glycolysis in vitro and hampered tumor growth in vivo. Mechanistic analyses have demonstrated that circ-NFIX acted as a molecular sponge of miR-212-3p and upregulated the expression of ADAM10 (210).
TME is the product of the crosstalk between different cell types and plays a crucial role in the progression, metastasis, and therapeutic treatment of cancer (211). Tumor immune escape refers to the phenomenon of tumor cells growing and metastasizing via various mechanisms to avoid recognition and attack by the immune system. The mechanism of tumor immune escape includes immunosuppression. Programmed death 1/programmed death-ligand 1 (PD-1/PD-L1), known as an immune checkpoint, is an important component of tumor immunosuppression (212). It has also been reported that circ-002178, from the exosomes of plasma from LUAC patients, was highly expressed in LUAC tissues and LUAC cancer cells. Functional studies have demonstrated that circ-002178 could enhance PDL1 expression via sponging miR-34 in cancer cells to induce T cell exhaustion (125). Circ-CPA4 was recently identified to be upregulated in NSCLC cells and cancer tissues. Compared to human bronchial epithelial cells and their paired clinical normal adjacent tissues, circ-CPA4 regulated cell growth, mobility, stemness, and drug resistance in NSCLC cells and inactivated CD8+ T cells in the tumor immune microenvironment through the let-7 miRNA/PD-L1 axis (59). Interestingly, a recent study showed that circ0000284 facilitated the progression of NSCLC by upregulating the PD-L1 expression as a competing endogenous RNA (ceRNA) of miR-377 (213). Therefore, regulating the PD-1/PD-L1 expression by targeting related circRNAs may be a direction of future immune checkpoint therapeutic research. Furthermore, some circRNAs regulate the cytokine expression to influence the function of immune cells—for example, ciRS-7 interacts with miR-7 to modulate the expression of NF-κB, modulating the activities of immune cells and affecting the development of lung cancer (214, 215). The non-cellular components of TME mainly include the extracellular matrix (ECM) and hypoxia environment. ECM, composed of matricellular proteins, elastin, collagen, and cytokines, provides the structural and functional bases for tumor cellular function (216). Circ-0000064 was reported to promote cell proliferation and inhibit apoptosis by the regulation of MMP-2 and MMP-9, participating in the destruction of the histological barrier as well as the invasion of cancer cells (217). The metabolism of cellular components in TME may result in a hypoxia environment, causing the generation of hypoxia-inducible factors (HIFs) to modulate the progression of tumor (218). A previous study has shown that upregulated circPIP5K1A inhibits miR-600 to enhance the HIF-1α expression to promote the migration and proliferation of NSCLC cells (219). Furthermore, circ-FAM120A expression was found to be downregulated in hypoxic LUAC, while HIF-1α expression increased at the same time, both promoting tumor growth (220). Therefore, circRNAs are identified to influence the hypoxia environment of TME in lung cancer.
The diagnostic and prognostic value of circRNAs
The high stability, abundance, and spatiotemporal-specific expression of circRNAs make them ideal biomarkers for liquid biopsy. The important functions of circRNAs in blood cells suggest that the dysregulation of circRNA expression in blood cells is likely to contribute to the occurrence and progression of lung cancers—for instance, in NSCLC patients, Tan et al. found that F-circEA, an f-circRNA originating from the EML4-ALK fusion gene, was exclusively expressed in the plasma of patients with the EML4-ALK fusion. Therefore, plasma F-circEA may serve as a liquid biopsy biomarker to diagnose NSCLC patients with EML4-ALK translocation and guide the targeted therapy for NSCLC patients in this subgroup (129). In a recent study, Luo et al. measured the expression levels of two circRNAs in the plasma samples of 231 lung cancer patients and 41 healthy controls using reverse transcription droplet digital PCR (221). They identified has circ-0000190 as a circRNA biomarker in human blood plasma that can predict the survival outcomes of lung cancer patients (221). Furthermore, the increased expression of circ-0000190 in plasma was also correlated with poor response to systemic therapy and immunotherapy in lung cancer patients (221). Similarly, Li et al. observed that SCLC patients with lower exosomal circFLI1 expression levels experienced longer disease remissions, indicating its prognostic power in SCLC. The authors also suggested that serum exosomal circFLI1 may be used as a biomarker that can monitor the clinical 191response to chemotherapy in SCLC patients (222). Notably, they observed that SCLC patients with lower exosomal circ-FLI1 expression levels experienced longer disease remissions, indicating its prognostic power in SCLC. The authors also suggested that serum exosomal circFLI1 may be used as a biomarker that can monitor the clinical response to chemotherapy in SCLC patients (222).
The main evaluative criterion of the diagnostic value of circRNAs is AUC in the ROC analysis. Previous studies have shown that many circRNAs may serve as potential diagnostic biomarkers. Circ 0000729 had an area under the receiver operating characteristic curve (AUC-ROC) of 0.815 for discriminating LUAC from normal controls (223). Circ-0000064, being located in the cytoplasm circRNA, was reported to be upregulated in lung cancer tissues and lung cancer cell lines. Its aberrant expression was correlated with several clinical characteristics, including T stage, lymphatic metastasis, and TNM stage, which represents a novel potential biomarker for lung cancer diagnosis (217). It was suggested that circ-102231 was highly expressed in lung adenocarcinoma tissues and associated with advanced TNM stage, lymph node metastasis, and poor overall survival of lung cancer patients. The depletion of circ-102231 significantly restrains lung cancer cell proliferation and its invasion ability. It counts as a potential diagnostic biomarker for lung cancer patients. Its area under the ROC curve was 0.897, and circ 102231 showed good sensitivity and specificity of 81.2 and 88.7%, respectively (224). Circ-0014130 was elevated in NSCLC tissues. Its high expression correlated with TNM stage and lymphatic metastasis. The area under the receiver operating characteristic curve of circ-0014130 in NSCLC was 0.878, which showed good diagnostic potential. Furthermore, via gene oncology analysis and pathway analysis, it was indicated that circ-0014130 could participate in NSCLC development and could be used as a potential NSCLC diagnostic biomarker (225). Li et al. reported that the circ-0075930 expression levels were significantly higher in NSCLC cell lines and tissues. Its high expression was correlated with tumor size and lymph node metastasis. circ-0075930 had an AUC-ROC of 0.756 for discriminating NSCLC from normal controls, with sensitivity and specificity of 76.2 and 72.1%, respectively (226). Lu et al. reported that the circ0001715 levels were significantly higher in lung adenocarcinoma patients versus healthy controls. Its high expression significantly correlated with TNM stage and distant metastasis. Furthermore, the ROC curve analysis showed that the AUC of circ-0001715 was 0.871. The univariate and multivariate survival analyses showed that the plasma circ 0001715 level was an independent prognostic factor for the OS (227). The ROC curve indicated that the AUC of circ FOXO3 was 0.782, and the sensitivity and specificity of diagnosing NSLCL with circRNA-FOXO3 reached 80.0 and 73.3%, respectively (228).
circRNAs have stable circular structures which make them more stable and particularly attractive as liquid biopsy biomarkers. Circ-0013958 was elevated in lung adenocarcinoma tissues, cells, and plasma. Its high expression was associated with TNM stage and lymphatic metastasis. The area under the receiver operating characteristic curve was 0.815. In addition, circ-0013958 could be a sponge of miR-134 and thus upregulated oncogenic cyclin D1. It may be a potential non-invasive biomarker for the early detection and screening of lung adenocarcinoma (174). Circ-FARSA, derived from exon 5–7 of the FARSA gene, was elevated in cancerous tissues and patients’ plasma. The elevated expression of circ-FARSA significantly promoted cell migration and invasion in NSCLC cells. Circ-FARSA could inhibit the activity of miR-330-5p and miR-326, thereby relieving their inhibitory effects on oncogene fatty acid synthase. A further analysis of the diagnostic value of plasma circ-FARSA in distinguishing NSCLC patients from non-cancer patients indicated the area under the ROC curve to be 0.71. This evidence indicated that plasma circFARSA could be a noninvasive biomarker for this NSCLC malignancy (229).
Circ-0067934 was shown to be markedly overexpressed in NSCLC tissues and cell lines. Its high expression was correlated with TNM stage, lymph node status, and distant metastasis in NSCLC patients. A higher expression of circ-0067934 results in significantly poorer survival, and the results of a multivariate Cox proportional hazard analysis indicated that circ-0067934 was an independent poor prognostic factor for patients with NSCLC. The depletion of circ-0067934 significantly suppressed NSCLC cell proliferation, migration, and invasion (178). Circ 100876 has been reported to be significantly elevated in NSCLC tissues than their adjacent nontumorous tissues. Its high expression correlated with lymph node metastasis, tumor stage, and poor overall survival in NSCLC. It might serve as a potential prognostic biomarker for NSCLC (230). It was suggested that circ-FOXM1 is highly expressed in NSCLC tissues and closely correlated with lymph node invasion, higher TNM stage, and unfavorable prognosis (162). The depletion of circ FOXM1 significantly restrains the growth, migration, and invasion of NSCLC cells. A further study has shown that Circ FOXM1 upregulated the expression of PPDPF and MACC1 via inhibiting the activity of miR-1304-5p (162). The above-mentioned examples demonstrated that some circRNAs could be promising biomarkers for the diagnosis and prognosis of lung cancer.
Lin et al. have shown that circ PRKCI was overexpressed in lung adenocarcinoma tissues and promoted the proliferation and tumorigenesis of lung adenocarcinoma. This group established a nude mouse xenograft model. Compared with the siRNA-transfected cell-derived tumors, the si-circPRKCI-transfected cell-derived tumors were smaller and lighter in weight (231). The therapeutic potential of circPRKCI was evaluated via an intratumoral injection of cholesterol-conjugated si-circPRKCI and control siRNA in patient-derived tumor xenografts (PDTX). The results indicated that si-circPRKCI significantly inhibited the growth of PDTX in vivo, suggesting that circPRKCI may be a promising therapeutic target for LUAC (231).
Conclusion and future direction
With the rapid development of high-throughput sequencing technologies and bioinformatics approaches, increasing evidence have uncovered that circRNAs play an important role in several human physiological and pathological processes, such as adipogenesis (43), lung inflammation (232), brain development (233), repair of ischemic muscle injury (234), mitochondrial metabolism (46), and cancer progression (235). Lung cancer is the leading form of cancer in terms of both morbidity and mortality. Due to the lack of effective diagnostic markers, many lung cancer patients are approaching the advanced stage. Recently, with the widespread use of computed tomography and magnetic resonance imaging, incidental renal masses are increasingly being detected. However, there is an urgent need for specific biomarkers to allow for the early identification of postoperative lung cancer recurrence and metastasis. Screening and early diagnosis of lung cancer are critical for improving the treatment efficacy and reducing the mortality of patients with lung cancer and have been identified as a research priority. As a novel noncoding RNA, circRNA influences the initiation and progression of lung cancer through participation in diverse processes of lung cancer pathogenesis, including proliferation, migration, invasion, apoptosis, cell cycle, stemness of lung cancer stem cell, tumor metabolism of lung cancer, radiotherapy, and chemotherapy resistance. All of these findings suggest that circRNAs play pivotal roles in the pathological progression of cancer and may be useful as cancer biomarkers. circRNAs can be easily detected from a wide range of biological samples due to their high stability and specificity, representing as ideal diagnostic and prognostic biomarkers in lung cancer.
Previously identified lung CSC subsets have been shown to confer resistance to conventional chemotherapeutics, biological molecules, targeted therapies, and radiotherapy used in the current management of lung cancer. Specific targeting of cancer stem cells in combination with first-line chemotherapeutic agents holds great promise as a strategy to overcome chemoresistance, tumor relapse, and metastasis. A number of CSC markers have been identified and studied. These include ALDH1, ABCG2, CD44, CD133, NANOG, and SRY-box transcription factor 2 (SOX2), all of which have been linked to chemoresistance in a number of first line anti-cancer therapies—for example, axitinib is an oral, potent, small molecule ATP-competitive multitarget tyrosine kinase inhibitor. It inhibits the CSC marker ABCG2. Axitinib has also been shown to reverse multidrug resistance via ABCG2 inhibition both in vitro and in vivo. The axitinib–doxorubicin combination treatment promoted the intracellular accumulation of doxorubicin within the side population of CSC cells and significantly enhanced the cytotoxic effects of doxorubicin (236). In addition, a recent finding by our group was that, under normoxic conditions, YTHDF1 is highly expressed in non-small-cell lung cancer cancerous tissues and cell lines to promote cell proliferation via increasing cell-cycle-related factor expression. However, under hypoxic conditions or stressful chemotherapy conditions, YTHDF1 is downregulated, which leads to reduced Keap1 mRNA translational efficiency and Nrf2 protein stabilization (5).
With the deepening research on circRNAs, it is very important to choose appropriate and efficient database and analysis software for the precise analysis of circRNAs. We will also summarize recently developed database that have been developed to provide tremendous valuable information. CircBase mainly collect circRNA information of multiple species, including human, mouse, Caenorhabditis elegans, D. melanogaster, Latimeria chalumnae, and coelacanth (237). DeepBase, a database that integrate all public deep-sequencing data, provides an integrative evaluation of miRBase-annotated miRNA genes and other known ncRNAs and explores the expression patterns of miRNAs and other ncRNAs (238). CircNet database provides the following information related to circRNAs: novel circRNAs, integrated miRNA–target networks, expression profiles of circRNA isoforms, genomic annotations of circRNA isoforms, and sequences of circRNA isoforms. In addition, the CircNet database that provides tissue-specific circRNA expression profiles and circRNA–miRNAgene regulatory networks illustrates the regulation between circRNAs, miRNAs, and genes (239). circRNADb contains human circular RNAs with protein-coding annotations. The detailed information of the circRNA included genomic information, exon splicing, genome sequence, IRES, open reading frame (240). CircInteractome database is used for mapping RBP- and miRNA-binding sites on human circRNAs. circInteractome also carried out the following functions: identify potential circRNAs which can act as RBP sponges, design junction-spanning primers for the specific detection of circRNAs of interest, design siRNAs for circRNA silencing, and identify potential IRES (241). CSCD is a database for cancer-specific circular RNAs. It includes the following information: the microRNA response element sites and RNA-binding protein sites for each circRNA, the predicted potential open reading frames of circRNAs, and the splicing events in the linear transcripts of each circRNA (242). CIRCpedia v2 is a database for comprehensive circRNAs with expression features in various cell types/tissues, including disease samples. It also can perform a conservation analysis of circRNAs between humans and mice (243). TSCD is for Tissue-Specific CircRNA Database, containing information about tissue-specific circRNAs in human and mouse (244). ExoRBase is a database of circRNA, lncRNA, and mRNA derived from RNA-seq data analyses of human blood exosomes (245). circRNA Disease is a database that provides a user-friendly interface for searching disease-associated circRNAs (246). CircVAR is a database used to collect SNPs and small insertions and deletions in putative circRNA regions and to identify their potential phenotypic information (247). TransCirc is a specialized database that provides comprehensive evidences supporting the translation potential of circular RNAs (248). Circ2GO is a database that contains information on circRNAs and their function, processes, and miRNA targets (249). Although the listed databases have different advantages, there are still many problems, such as prediction overlap, lack of a unified identification standard, the results predicted by different databases that vary greatly, and the lack of a uniform identification standard. Therefore, when using these informatics methods, it is necessary to set specific conditions and improve the certainty of the data.
Exosomes can mediate cell-to-cell communication in both physiologic and pathologic processes. Exosomes contain RNAs, proteins, and lipids, all of which affect exosome functions and reflect cell characteristics. The major nucleic acids of exosomes are RNAs that contain microRNA (miRNA), tRNA, and long noncoding RNA (lncRNA) as well as circRNAs (250). Exosomes can serve as a novel cellular communication bond by transferring their contents to target cells in a lung cancer microenvironment, thereby regulating lung cancer cell progression (251)—for example, under hypoxic conditions, miR-23a was significantly increased in exosomes from lung cancer cells, and exosomes transferred miR-23a to endothelial cells. miR-23a directly inhibited its target prolyl hydroxylase 1 and 2 (PHD1 and 2) expression, resulting in the accumulation of hypoxia-inducible factor-1α in endothelial cells. Consequently, exosomes derived from hypoxic lung cancer cells increased endothelial permeability and cancer cell transendothelial migration in vitro and enhanced neovascularization and tumor growth in vivo (252). In addition, tumor-derived exosomes carry immunosuppressive molecules, transfer these cargos to immune cells, and directly or indirectly suppress the functions of immune cells, thereby promoting tumor progression (253). circRNAs can be packaged into exosomes and transferred to receptor cells, further impacting the development of diseases. A recent study by Zhang N et al. reported that circSATB2 was highly expressed in NSCLC cancer exosomes, which can promote the proliferation, migration, and invasion of NLCSC cells. Exosomes which contained circ SATB2 can be taken up by NLCSC cells to participate in cell–cell communication, further affecting the progression of NSCLC cells and the proliferation of normal bronchial epithelial cells. Moreover, exosomal circSATB2 expression was related to lung cancer lymphatic metastasis. These results indicate that exosomal circSATB2 has the potential to act as a blood detection index for the diagnosis of lung cancer and lung cancer metastasis with high sensitivity and specificity (124). Similarly, it has also been reported that circRNA 002178 from the exosomes of plasma from LUAC patients was highly expressed in LUAC tissues and LUAC cancer cells. Functional studies have demonstrated that circRNA-002178 could enhance PDL1 expression via sponging miR-34 in cancer cells to induce T cell exhaustion. Interestingly, the area under the curve (AUCs) of circRNA-002178 was 0.9956, which was higher in the exosomes derived from cancer cells than the exosomes derived from normal human bronchial epithelial cells, and could serve as a novel diagnosis biomarker for lung cancer (125).
TME is the product of the crosstalk between different cell types and plays a crucial role in the progression, metastasis, and therapeutic treatment of cancer (211). The TME in both primary and secondary lung tumors is recognized as a target-rich environment for the development of novel anticancer agents. It has been reported that circRNA could modulate immune responses in the tumor microenvironment of lung cancer and be involved in lung cancer progression—for instance, ciRS-7 interacts with miR-7 to modulate the expression of NF-κB, modulating the activities of immune cells and affecting the development of lung cancer (214, 215). Mechanistic insights into the tumor-reprogrammed microenvironmental landscape in lung cancer together with the development of drugs that specifically inhibit the components of the landscape have ushered in a new era of cancer medicine.
Although there are many studies that have reported the biological function of RNA in lung cancer, there are still many problems surrounding circRNAs that remain to be clarified. First, the mechanism of expression and localization of circRNA is still unclear. Second, many new technologies, such as antisense oligonucleotide and CRISPR/Cas9-mediated knockout could be used to interfere the endogenous function of circRNA that promote cancer progression. Exosomes can transfer biologically active molecules between lung cancer cells and their microenvironment, but the mechanisms of exosome-related circRNA involved in lung cancer progression need to be illuminated. Finally, as circRNAs are stable and have unique structural conformations, additional investigations are still required to decipher the complex molecular mechanism so that circRNA could be implemented clinically through translational medicine, especially in the case of a disease such as lung cancer.
Author contributions
WR and YY contributed to the direction and guidance of this review and prepared the figure. JP, LM and XJ drafted the manuscript and revised the manuscript; All authors read and approved the final manuscript.
Funding
This work was supported by Yunnan Fundamental Research Projects (grant no. 202101AS070043), Kunming Science and Technology Plan Project (grant no. 2020-1-H-003), Kunming Medical University Graduate Student Innovation Fund (grant no. 2022B24), and The First People’s Hospital of Yunnan Province Clinical Medicine Center Opening Project (grant no. 2022LCZXKF-XZ02).
Conflict of interest
The authors declare that the research was conducted in the absence of any commercial or financial relationships that could be construed as a potential conflict of interest.
Publisher’s note
All claims expressed in this article are solely those of the authors and do not necessarily represent those of their affiliated organizations, or those of the publisher, the editors and the reviewers. Any product that may be evaluated in this article, or claim that may be made by its manufacturer, is not guaranteed or endorsed by the publisher.
Abbreviations
LUAC, lung adenocarcinoma; NSCLC, non-small-cell lung cancer; LUSC, lung squamous cell carcinoma; LC, lung cancer; SCLC, small-cell lung cancer; pre-mRNA, premessenger RNA; m6A, N6-methyladinosinek; ORF, open reading frame; hnRNPs, heterogeneous nuclear ribonucleoproteins; DHX9, ATP-dependent RNA helicase A; AGO2, argonaute 2; RNAi, RNA interference; circRNAs, circular RNAs; ncRNAs, non-coding RNAs; miRNAs, microRNAs; ecircRNAs, exonic circRNAs; ElciRNAs, exon–intron circRNAs; ciRNAs, intronic circRNAs; Pol II, RNA polymerase II; snRNP, small nuclear ribonucleoprotein; RBPs, RNA-binding proteins; ceRNA, competing endogenous RNA; EMT, epithelial–mesenchymal transition; TNM, tumor–node–metastasis; OS, overall survival; ROC, receiver operating characteristic; ASOs, anti-sense oligonucleotides; CRISPR/Cas9, clustered regularly interspaced short palindromic; repeats-associated nuclease Cas9; circ-AS, circRNA alternative splicing; ICSs, flanking intronic complementary sequences; CSC, cancer stem cell.
References
2. Molina JR, Yang P, Cassivi SD, Schild SE, Adjei AA. Non-small cell lung cancer: epidemiology, risk factors, treatment, and survivorship. Mayo Clinic Proc (2008) 83(5):584–94. doi: 10.4065/83.5.584
3. Reck M, Rabe KF. Precision diagnosis and treatment for advanced non-Small-Cell lung cancer. N Engl J Med (2017) 377(9):849–61.
4. Govindan R, Ding L, Griffith M, Subramanian J, Dees ND, Kanchi KL, et al. Genomic landscape of non-small cell lung cancer in smokers and never-smokers. Cell (2012) 150(6):1121–34.
5. Shi Y, Fan S, Wu M, Zuo Z, Li X, Jiang L, et al. YTHDF1 links hypoxia adaptation and non-small cell lung cancer progression. Nat Commun (2019) 10(1):4892.
6. Duruisseaux M, Esteller M. Lung cancer epigenetics: From knowledge to applications. Semin Cancer Biol (2018) 51:116–28.
7. Chen Y, Liu X, Li Y, Quan C, Zheng L, Huang K. Lung cancer therapy targeting histone methylation: Opportunities and challenges. Comput Struct Biotechnol J (2018) 16:211–23.
8. Hulbert A, Jusue-Torres I, Stark A, Chen C, Rodgers K, Lee B, et al. Early detection of lung cancer using DNA promoter hypermethylation in plasma and sputum. Clin Cancer Res (2017) 23(8):1998–2005.
9. Hua Q, Jin M, Mi B, Xu F, Li T, Zhao L, et al. LINC01123, a c-myc-activated long non-coding RNA, promotes proliferation and aerobic glycolysis of non-small cell lung cancer through miR-199a-5p/c-Myc axis. J Hematol Oncol (2019) 12(1):91.
11. Hombach S, Kretz M. Non-coding RNAs: Classification, biology and functioning. Adv Exp Med Biol (2016) 937:3–17.
12. Li X, Yang L, Chen LL. The biogenesis, functions, and challenges of circular RNAs. Mol Cell (2018) 71(3):428–42.
13. Chen Z, Li JL, Lin S, Cao C, Gimbrone NT, Yang R, et al. cAMP/CREB-regulated LINC00473 marks LKB1-inactivated lung cancer and mediates tumor growth. J Clin Invest (2016) 126(6):2267–79.
14. Iqbal MA, Arora S, Prakasam G, Calin GA, Syed MA. MicroRNA in lung cancer: role, mechanisms, pathways and therapeutic relevance. Mol Aspects Med (2019) 70:3–20.
15. Wang L, Tong X, Zhou Z, Wang S, Lei Z, Zhang T, et al. Circular RNA hsa_circ_0008305 (circPTK2) inhibits TGF-β-induced epithelial-mesenchymal transition and metastasis by controlling TIF1γ in non-small cell lung cancer. Mol Cancer (2018) 17(1):140. doi: 10.1186/s12943-018-0889-7
16. Huang RS, Zheng YL, Li C, Ding C, Xu C, Zhao J. MicroRNA-485-5p suppresses growth and metastasis in non-small cell lung cancer cells by targeting IGF2BP2. Life Sci (2018) 199:104–11.
17. Xie C, Han Y, Liu Y, Han L, Liu J. miRNA-124 down-regulates SOX8 expression and suppresses cell proliferation in non-small cell lung cancer. Int J Clin Exp Pathol (2014) 7(11):7518–26.
18. Zhao Y, Feng C, Li Y, Ma Y, Cai R. LncRNA H19 promotes lung cancer proliferation and metastasis by inhibiting miR-200a function. Mol Cell Biochem (2019) 460(1-2):1–8.
19. Zhang YX, Yuan J, Gao ZM, Zhang ZG. LncRNA TUC338 promotes invasion of lung cancer by activating MAPK pathway. Eur Rev Med Pharmacol Sci (2018) 22(2):443–9. doi: 10.26355/eurrev_201801_14193
20. Sanger HL, Klotz G, Riesner D, Gross HJ, Kleinschmidt AK. Viroids are single-stranded covalently closed circular RNA molecules existing as highly base-paired rod-like structures. Proc Natl Acad Sci U.S.A. (1976) 73(11):3852–6.
21. Hsu MT, Coca-Prados M. Electron microscopic evidence for the circular form of RNA in the cytoplasm of eukaryotic cells. Nature (1979) 280(5720):339–40.
22. Cocquerelle C, Mascrez B, Hétuin D, Bailleul B. Mis-splicing yields circular RNA molecules. FASEB J (1993) 7(1):155–60.
23. Capel B, Swain A, Nicolis S, Hacker A, Walter M, Koopman P, et al. Circular transcripts of the testis-determining gene sry in adult mouse testis. Cell (1993) 73(5):1019–30.
24. Ivanov A, Memczak S, Wyler E, Torti F, Porath HT, Orejuela MR, et al. Analysis of intron sequences reveals hallmarks of circular RNA biogenesis in animals. Cell Rep (2015) 10(2):170–7.
25. Salzman J, Chen RE, Olsen MN, Wang PL, Brown PO. Cell-type specific features of circular RNA expression. PloS Genet (2013) 9(9):e1003777.
26. Wang PL, Bao Y, Yee MC, Barrett SP, Hogan GJ, Olsen MN, et al. Circular RNA is expressed across the eukaryotic tree of life. PloS One (2014) 9(6):e90859.
27. Zhang XO, Wang HB, Zhang Y, Lu X, Chen LL, Yang L. Complementary sequence-mediated exon circularization. Cell (2014) 159(1):134–47.
28. Li X, Liu CX, Xue W, Zhang Y, Jiang S, Yin QF, et al. Coordinated circRNA biogenesis and function with NF90/NF110 in viral infection. Mol Cell (2017) 67(2):214–227.e7. doi: 10.1016/j.molcel.2017.05.023
29. Zhang Y, Zhang XO, Chen T, Xiang JF, Yin QF, Xing YH, et al. Circular intronic long noncoding RNAs. Mol Cell (2013) 51(6):792–806.
30. Memczak S, Jens M, Elefsinioti A, Torti F, Krueger J, Rybak A, et al. Circular RNAs are a large class of animal RNAs with regulatory potency. Nature (2013) 495(7441):333–8.
31. Li Z, Huang C, Bao C, Chen L, Lin M, Wang X, et al. Exon-intron circular RNAs regulate transcription in the nucleus. Nat Struct Mol Biol (2015) 22(3):256–64.
32. Aufiero S, Reckman YJ, Pinto YM, Creemers EE. Circular RNAs open a new chapter in cardiovascular biology. Nat Rev Cardiol (2019) 16(8):503–14.
33. Suzuki H, Zuo Y, Wang J, Zhang MQ, Malhotra A, Mayeda A. Characterization of RNase r-digested cellular RNA source that consists of lariat and circular RNAs from pre-mRNA splicing. Nucleic Acids Res (2006) 34(8):e63. doi: 10.1093/nar/gkl151
34. Yang Y, Gao X, Zhang M, Yan S, Sun C, Xiao F, et al. Novel role of FBXW7 circular RNA in repressing glioma tumorigenesis. J Natl Cancer Inst (2018) 110(3):304–15.
35. Xu T, Wu J, Han P, Zhao Z, Song X. Circular RNA expression profiles and features in human tissues: a study using RNA-seq data. BMC Genomics (2017) 18(Suppl 6):680.
36. Ashwal-Fluss R, Meyer M, Pamudurti NR, Ivanov A, Bartok O, Hanan M, et al. circRNA biogenesis competes with pre-mRNA splicing. Mol Cell (2014) 56(1):55–66.
37. Hansen TB, Jensen TI, Clausen BH, Bramsen JB, Finsen B, Damgaard CK, et al. Natural RNA circles function as efficient microRNA sponges. Nature (2013) 495(7441):384–8. doi: 10.1038/nature11993
38. Ansell SM, Lesokhin AM, Borrello I, Halwani A, Scott EC, Gutierrez M, et al. PD-1 blockade with nivolumab in relapsed or refractory hodgkin's lymphoma. N Engl J Med (2015) 372(4):311–9.
39. Legnini I, Di Timoteo G, Rossi F, Morlando M, Briganti F, Sthandier O, et al. Circ-ZNF609 is a circular RNA that can be translated and functions in myogenesis. Mol Cell (2017) 66(1):22–37.e9.
40. Weigelt CM, Sehgal R, Tain LS, Cheng J, Eßer J, Pahl A, et al. An insulin-sensitive circular RNA that regulates lifespan in drosophila. Mol Cell (2020) 79(2):268–279.e5.
41. Pandey PR, Yang JH, Tsitsipatis D, Panda AC, Noh JH, Kim KM, et al. circSamd4 represses myogenic transcriptional activity of PUR proteins. Nucleic Acids Res (2020) 48(7):3789–805.
42. Gao L, Chang S, Xia W, Wang X, Zhang C, Cheng L, et al. Circular RNAs from BOULE play conserved roles in protection against stress-induced fertility decline. Sci Adv (2020) 6(46).
43. Arcinas C, Tan W, Fang W, Desai TP, Teh DCS, Degirmenci U, et al. Adipose circular RNAs exhibit dynamic regulation in obesity and functional role in adipogenesis. Nat Metab (2019) 1(7):688–703.
44. You X, Vlatkovic I, Babic A, Will T, Epstein I, Tushev G, et al. Neural circular RNAs are derived from synaptic genes and regulated by development and plasticity. Nat Neurosci (2015) 18(4):603–10.
45. Stoll L, Rodríguez-Trejo A, Guay C, Brozzi F, Bayazit MB, Gattesco S, et al. A circular RNA generated from an intron of the insulin gene controls insulin secretion. Nat Commun (2020) 11(1):5611.
46. Zhao Q, Liu J, Deng H, Ma R, Liao JY, Liang H, et al. Targeting mitochondria-located circRNA SCAR alleviates NASH via reducing mROS output. Cell (2020) 183(1):76–93.e22.
48. Holdt LM, Stahringer A, Sass K, Pichler G, Kulak NA, Wilfert W, et al. Circular non-coding RNA ANRIL modulates ribosomal RNA maturation and atherosclerosis in humans. Nat Commun (2016) 7:12429. doi: 10.1038/ncomms12429
49. Fang Y, Wang X, Li W, Han J, Jin J, Su F, et al. Screening of circular RNAs and validation of circANKRD36 associated with inflammation in patients with type 2 diabetes mellitus. Int J Mol Med (2018) 42(4):1865–74.
50. Hansen TB, Kjems J, Damgaard CK. Circular RNA and miR-7 in cancer. Cancer Res (2013) 73(18):5609–12.
51. Vo JN, Cieslik M, Zhang Y, Shukla S, Xiao L, Zhang Y, et al. The landscape of circular RNA in cancer. Cell (2019) 176(4):869–881.e13.
52. De Rubis G, Rajeev Krishnan S, Bebawy M. Liquid biopsies in cancer diagnosis, monitoring, and prognosis. Trends Pharmacol Sci (2019) 40(3):172–86.
53. Zhang Z, Yang T, Xiao J. Circular RNAs: Promising biomarkers for human diseases. EBioMedicine (2018) 34:267–74.
54. Chen D, Ma W, Ke Z, Xie F. CircRNA hsa_circ_100395 regulates miR-1228/TCF21 pathway to inhibit lung cancer progression. Cell Cycle (2018) 17(16):2080–90.
55. Chen T, Yang Z, Liu C, Wang L, Yang J, Chen L, et al. Circ_0078767 suppresses non-small-cell lung cancer by protecting RASSF1A expression via sponging miR-330-3p. Cell Prolif (2019) 52(2):e12548.
56. Fan Z, Bai Y, Zhang Q, Qian P. CircRNA circ_POLA2 promotes lung cancer cell stemness via regulating the miR-326/GNB1 axis. Environ Toxicol (2020) 35(10):1146–56.
57. Li X, Yang B, Ren H, Xiao T, Zhang L, Li L, et al. Hsa_circ_0002483 inhibited the progression and enhanced the taxol sensitivity of non-small cell lung cancer by targeting miR-182-5p. Cell Death Dis (2019) 10(12):953.
58. Zhou J, Zhang S, Chen Z, He Z, Xu Y, Li Z. CircRNA-ENO1 promoted glycolysis and tumor progression in lung adenocarcinoma through upregulating its host gene ENO1. Cell Death Dis (2019) 10(12):885.
59. Hong W, Xue M, Jiang J, Zhang Y, Gao X. Circular RNA circ-CPA4/ let-7 miRNA/PD-L1 axis regulates cell growth, stemness, drug resistance and immune evasion in non-small cell lung cancer (NSCLC). J Exp Clin Cancer Res (2020) 39(1):149.
60. Chen LL. The biogenesis and emerging roles of circular RNAs. Nat Rev Mol Cell Biol (2016) 17(4):205–11.
61. Jeck WR, Sorrentino JA, Wang K, Slevin MK, Burd CE, Liu J, et al. Circular RNAs are abundant, conserved, and associated with ALU repeats. Rna (2013) 19(2):141–57. doi: 10.1261/rna.035667.112
62. Jeck WR, Sharpless NE. Detecting and characterizing circular RNAs. Nat Biotechnol (2014) 32(5):453–61.
63. Barrett SP, Wang PL, Salzman J. Circular RNA biogenesis can proceed through an exon-containing lariat precursor. Elife (2015) 4:e07540.
64. Athanasiadis A, Rich A, Maas S. Widespread a-to-I RNA editing of alu-containing mRNAs in the human transcriptome. PloS Biol (2004) 2(12):e391.
66. Kristensen LS, Andersen MS, Stagsted LVW, Ebbesen KK, Hansen TB, Kjems J. The biogenesis, biology and characterization of circular RNAs. Nat Rev Genet (2019) 20(11):675–91.
67. Zhang XO, Dong R, Zhang Y, Zhang JL, Luo Z, Zhang J, et al. Diverse alternative back-splicing and alternative splicing landscape of circular RNAs. Genome Res (2016) 26(9):1277–87.
68. Liang D, Wilusz JE. Short intronic repeat sequences facilitate circular RNA production. Genes Dev (2014) 28(20):2233–47. doi: 10.1101/gad.251926.114
69. Dubin RA, Kazmi MA, Ostrer H. Inverted repeats are necessary for circularization of the mouse testis sry transcript. Gene (1995) 167:245–8.
70. Guarnerio J, Bezzi M, Jeong JC, Paffenholz SV, Berry K, Naldini MM, et al. Oncogenic role of fusion-circRNAs derived from cancer-associated chromosomal translocations. Cell (2016) 166(4):1055–6.
71. Lander ES, Linton LM, Birren B, Nusbaum C, Zody MC, Baldwin J, et al. Initial sequencing and analysis of the human genome. Nature (2001) 409(6822):860–921.
72. Dong R, Zhang XO, Zhang Y, Ma XK, Chen LL, Yang L. CircRNA-derived pseudogenes. Cell Res (2016) 26(6):747–50. doi: 10.1038/cr.2016.42
73. Liang D, Tatomer DC, Luo Z, Wu H, Yang L, Chen LL, et al. The output of protein-coding genes shifts to circular RNAs when the pre-mRNA processing machinery is limiting. Mol Cell (2017) 68(5):940–954.e3.
74. Wang M, Hou J, Müller-McNicoll M, Chen W, Schuman EM. Long and repeat-rich intronic sequences favor circular RNA formation under conditions of reduced spliceosome activity. iScience (2019) 20:237–47. doi: 10.1016/j.isci.2019.08.058
75. Aktaş T, Avşar Ilık İ, Maticzka D, Bhardwaj V, Pessoa Rodrigues C, Mittler G, et al. DHX9 suppresses RNA processing defects originating from the alu invasion of the human genome. Nature (2017) 544(7648):115–9. doi: 10.1038/nature21715
76. Fei T, Chen Y, Xiao T, Li W, Cato L, Zhang P, et al. Genome-wide CRISPR screen identifies HNRNPL as a prostate cancer dependency regulating RNA splicing. Proc Natl Acad Sci United States America (2017) 114(26):E5207–15.
77. Khan MA, Reckman YJ, Aufiero S, van den Hoogenhof MM, van der Made I, Beqqali A, et al. RBM20 regulates circular RNA production from the titin gene. Circ Res (2016) 119(9):996–1003. doi: 10.1161/CIRCRESAHA.116.309568
78. Bachmayr-Heyda A, Reiner AT, Auer K, Sukhbaatar N, Aust S, Bachleitner-Hofmann T, et al. Correlation of circular RNA abundance with proliferation–exemplified with colorectal and ovarian cancer, idiopathic lung fibrosis, and normal human tissues. Sci Rep (2015) 5:8057.
79. Guo JU, Agarwal V, Guo H, Bartel DP. Expanded identification and characterization of mammalian circular RNAs. Genome Biol (2014) 15(7):409. doi: 10.1186/s13059-014-0409-z
80. Circular RNAs in the mammalian brain are highly abundant, conserved, and dynamically expressed. Mol Cell (2015) 58(5):870–85. doi: 10.1016/j.molcel.2015.03.027
81. Jiang N, Meng X, Mi H, Chi Y, Li S, Jin Z, et al. Circulating lncRNA XLOC_009167 serves as a diagnostic biomarker to predict lung cancer. Clin Chim Acta (2018) 486:26–33. doi: 10.1038/s41467-019-12651-2
82. Huang C, Liang D, Tatomer DC, Wilusz JE. A length-dependent evolutionarily conserved pathway controls nuclear export of circular RNAs. Genes Dev (2018) 32:639–44.
83. Roundtree IA, Luo GZ, Zhang Z, Wang X, Zhou T, Cui Y, et al. YTHDC1 mediates nuclear export of n-methyladenosine methylated mRNAs. eLife (2017) 6.
84. Chen RX, Chen X, Xia LP, Zhang JX, Pan ZZ, Ma XD, et al. N-methyladenosine modification of circNSUN2 facilitates cytoplasmic export and stabilizes HMGA2 to promote colorectal liver metastasis. Nat Commun (2019) 10(1):4695.
85. Enuka Y, Lauriola M, Feldman ME, Sas-Chen A, Ulitsky I, Yarden Y, et al. Circular RNAs are long-lived and display only minimal early alterations in response to a growth factor. Nucleic Acids Res (2016) 44(3):1370–83. doi: 10.1093/nar/gkv1367
86. Hansen TB, Wiklund ED, Bramsen JB, Villadsen SB, Statham AL, Clark SJ, et al. miRNA-dependent gene silencing involving Ago2-mediated cleavage of a circular antisense RNA. EMBO J (2011) 30(21):4414–22. doi: 10.1038/emboj.2011.359
87. Liu CX, Li X, Nan F, Jiang S, Gao X, Guo SK, et al. Structure and degradation of circular RNAs regulate PKR activation in innate immunity. Cell (2019) 177(4):865–880.e21.
88. Guo Y, Wei X, Peng Y. Structure-mediated degradation of CircRNAs. Trends Cell Biol (2020) 30(7):501–3. doi: 10.1016/j.tcb.2020.04.001
89. Park OH, Ha H, Lee Y, Boo SH, Kwon DH, Song HK, et al. Endoribonucleolytic cleavage of mA-containing RNAs by RNase P/MRP complex. Mol Cell (2019) 74(3):494–507.e8. doi: 10.1016/j.molcel.2019.02.034
90. Thomson DW, Dinger ME. Endogenous microRNA sponges: evidence and controversy. Nat Rev Genet (2016) 17(5):272–83.
91. Zheng Q, Bao C, Guo W, Li S, Chen J, Chen B, et al. Circular RNA profiling reveals an abundant circHIPK3 that regulates cell growth by sponging multiple miRNAs. Nat Commun (2016) 7:11215. doi: 10.1038/ncomms11215
92. Kristensen LS, Okholm TLH, Venø MT, Kjems J. Circular RNAs are abundantly expressed and upregulated during human epidermal stem cell differentiation. RNA Biol (2018) 15(2):280–91.
93. Yu CY, Li TC, Wu YY, Yeh CH, Chiang W, Chuang CY, et al. The circular RNA circBIRC6 participates in the molecular circuitry controlling human pluripotency. Nat Commun (2017) 8(1):1149. doi: 1038/s41467-017-01216-w
94. Corley M, Burns MC, Yeo GW. How RNA-binding proteins interact with RNA: Molecules and mechanisms. Mol Cell (2020) 78(1):9–29. doi: 10.1016/j.molcel.2020.03.011
95. Du WW, Yang W, Chen Y, Wu ZK, Foster FS, Yang Z, et al. Foxo3 circular RNA promotes cardiac senescence by modulating multiple factors associated with stress and senescence responses. Eur Heart J (2017) 38(18):1402–12. doi: 10.1093/eurheartj/ehw001
96. Du WW, Yang W, Liu E, Yang Z, Dhaliwal P, Yang BB, et al. Foxo3 circular RNA retards cell cycle progression via forming ternary complexes with p21 and CDK2. Nucleic Acids Res (2016) 44(6):2846–58. doi: 10.1093/nar/gkw027
97. Du WW, Fang L, Yang W, Wu N, Awan FM, Yang Z, et al. Induction of tumor apoptosis through a circular RNA enhancing Foxo3 activity. Cell Death differentiation (2017) 24(2):357–70.
98. Li Q, Wang Y, Wu S, Zhou Z, Ding X, Shi R, et al. CircACC1 regulates assembly and activation of AMPK complex under metabolic stress. Cell Metab (2019) 30(1):157–173.e7. doi: 10.1016/j.cmet.2019.05.009
99. Abdelmohsen K, Panda AC, Munk R, Grammatikakis I, Dudekula DB, De S, et al. Identification of HuR target circular RNAs uncovers suppression of PABPN1 translation by CircPABPN1. RNA Biol (2017) 14(3):361–9. doi: 10.1080/15476286.2017.1279788
100. Zeng Y, Du WW, Wu Y, Yang Z, Awan FM, Li X, et al. A circular RNA binds to and activates AKT phosphorylation and nuclear localization reducing apoptosis and enhancing cardiac repair. Theranostics (2017) 7(16):3842–55.
101. Barbagallo D, Caponnetto A, Brex D, Mirabella F, Barbagallo C, Lauretta G, et al. CircSMARCA5 regulates VEGFA mRNA splicing and angiogenesis in glioblastoma multiforme through the binding of SRSF1. Cancers (2019) 11(2). doi: 10.3390/cancers11020194
102. Zhou Z, Jiang R, Yang X, Guo H, Fang S, Zhang Y, et al, et al. circRNA mediates silica-induced macrophage activation via HECTD1/ZC3H12A-dependent ubiquitination. Theranostics (2018) 8(2):575–92. doi: 10.7150/thno.21648
103. Zhu YJ, Zheng B, Luo GJ, Ma XK, Lu XY, Lin XM, et al. Circular RNAs negatively regulate cancer stem cells by physically binding FMRP against CCAR1 complex in hepatocellular carcinoma. Theranostics (2019) 9(12):3526–40. doi: 10.7150/thno.32796
104. Ma Y, Zhang C, Zhang B, Yu H, Yu Q. circRNA of AR-suppressed PABPC1 91 bp enhances the cytotoxicity of natural killer cells against hepatocellular carcinoma via upregulating UL16 binding protein 1. Oncol Lett (2019) 17(1):388–97. doi: 10.3892/ol.2018.9606
105. Shi L, Yan P, Liang Y, Sun Y, Shen J, Zhou S, et al. Circular RNA expression is suppressed by androgen receptor (AR)-regulated adenosine deaminase that acts on RNA (ADAR1) in human hepatocellular carcinoma. Cell Death Dis (2017) 8(11):e3171. doi: 10.1038/cddis.2017.556
106. Chen N, Zhao G, Yan X, Lv Z, Yin H, Zhang S, et al. A novel FLI1 exonic circular RNA promotes metastasis in breast cancer by coordinately regulating TET1 and DNMT1. Genome Biol (2018) 19(1):218. doi: 10.1186/s13059-018-1594-y
107. Liu Y, Dong Y, Zhao L, Su L, Luo J. Circular RNA−MTO1 suppresses breast cancer cell viability and reverses monastrol resistance through regulating the TRAF4/Eg5 axis. Int J Oncol (2018) 53(4):1752–62.
108. Du WW, Yang W, Li X, Awan FM, Yang Z, Fang L, et al. A circular RNA circ-DNMT1 enhances breast cancer progression by activating autophagy. Oncogene (2018) 37(44):5829–42. doi: 10.1038/s41388-018-0369-y
109. Shen S, Yao T, Xu Y, Zhang D, Fan S, Ma J. CircECE1 activates energy metabolism in osteosarcoma by stabilizing c-myc. Mol Cancer (2020) 19(1):151.
110. Di Timoteo G, Dattilo D, Centrón-Broco A, Colantoni A, Guarnacci M, Rossi F, et al. Modulation of circRNA metabolism by m(6)A modification. Cell Rep (2020) 31(6):107641.
111. Zhang M, Zhao K, Xu X, Yang Y, Yan S, Wei P, et al. A peptide encoded by circular form of LINC-PINT suppresses oncogenic transcriptional elongation in glioblastoma. Nat Commun (2018) 9(1):4475.
112. Zhang M, Huang N, Yang X, Luo J, Yan S, Xiao F, et al. A novel protein encoded by the circular form of the SHPRH gene suppresses glioma tumorigenesis. Oncogene (2018) 37(13):1805–14.
113. Liang WC, Wong CW, Liang PP, Shi M, Cao Y, Rao ST, et al. Translation of the circular RNA circβ-catenin promotes liver cancer cell growth through activation of the wnt pathway. Genome Biol (2019) 20(1):84.
114. Zheng X, Chen L, Zhou Y, Wang Q, Zheng Z, Xu B, et al. A novel protein encoded by a circular RNA circPPP1R12A promotes tumor pathogenesis and metastasis of colon cancer via hippo-YAP signaling. Mol Cancer (2019) 18(1):47.
115. Xia X, Li X, Li F, Wu X, Zhang M, Zhou H, et al. A novel tumor suppressor protein encoded by circular AKT3 RNA inhibits glioblastoma tumorigenicity by competing with active phosphoinositide-dependent kinase-1. Mol Cancer (2019) 18(1):131. doi: 10.1186/s12943-019-1056-5
116. Zhao J, Lee EE, Kim J, Yang R, Chamseddin B, Ni C, et al. Transforming activity of an oncoprotein-encoding circular RNA from human papillomavirus. Nat Commun (2019) 10(1):2300.
117. Zhi X, Zhang J, Cheng Z, Bian L, Qin J. circLgr4 drives colorectal tumorigenesis and invasion through Lgr4-targeting peptide. Int J Cancer (2019).
118. Pan Z, Cai J, Lin J, Zhou H, Peng J, Liang J, et al. A novel protein encoded by circFNDC3B inhibits tumor progression and EMT through regulating snail in colon cancer. Mol Cancer (2020) 19(1):71. doi: 10.1186/s12943-020-01179-5
119. Wang Y, Liu J, Ma J, Sun T, Zhou Q, Wang W, et al. Exosomal circRNAs: biogenesis, effect and application in human diseases. Mol Cancer (2019) 18(1):116. doi: 10.1186/s12943-019-1041-z
121. Qu L, Ding J, Chen C, Wu ZJ, Liu B, Gao Y, et al. Exosome-transmitted lncARSR promotes sunitinib resistance in renal cancer by acting as a competing endogenous RNA. Cancer Cell (2016) 29(5):653–68. doi: 10.1016/j.ccell.2016.03.004
122. Wang X, Luo G, Zhang K, Cao J, Huang C, Jiang T, et al. Hypoxic tumor-derived exosomal miR-301a mediates M2 macrophage polarization via PTEN/PI3Kγ to promote pancreatic cancer metastasis. Cancer Res (2018) 78(16):4586–98.
123. Chen G, Huang AC, Zhang W, Zhang G, Wu M, Xu W, et al. Exosomal PD-L1 contributes to immunosuppression and is associated with anti-PD-1 response. Nature (2018) 560(7718):382–6. doi: 10.1158/0008-5472.CAN-17-3841
124. Zhang N, Nan A, Chen L, Li X, Jia Y, Qiu M, et al. Circular RNA circSATB2 promotes progression of non-small cell lung cancer cells. Mol Cancer (2020) 19(1):101. doi: 10.1038/s41419-020-2230-9
125. Wang J, Zhao X, Wang Y, Ren F, Sun D, Yan Y, et al. circRNA-002178 act as a ceRNA to promote PDL1/PD1 expression in lung adenocarcinoma. Cell Death Dis (2020) 11(1):32.
126. Hirsch FR, Scagliotti GV, Mulshine JL, Kwon R, Curran WJ Jr., Wu YL, et al. Lung cancer: current therapies and new targeted treatments. Lancet (2017) 389(10066):299–311.
127. Tan S, Gou Q, Pu W, Guo C, Yang Y, Wu K, et al. Circular RNA f-circEA produced from EML4-ALK fusion gene as a novel liquid biopsy biomarker for non-small cell lung cancer. Cell Res (2018) 28(6):693–5. doi: 10.1038/s41422-018-0033-7
128. Thompson JC, Hwang WT, Davis C, Deshpande C, Jeffries S, Rajpurohit Y, et al. Gene signatures of tumor inflammation and epithelial-to-mesenchymal transition (EMT) predict responses to immune checkpoint blockade in lung cancer with high accuracy. Lung Cancer (2020) 139:1–8. doi: 10.1016/j.lungcan.2019.10.012
129. Yuan Z, Yuan Z, Hasnat M, Zhang H, Liang P, Sun L, et al. A new perspective of triptolide-associated hepatotoxicity: the relevance of NF- κ b and NF- κ b-mediated cellular FLICE-inhibitory protein. Acta Pharm Sin B (2020) 10(5):861–77. doi: 10.1016/j.apsb.2020.02.009
130. Jin M, Shi C, Yang C, Liu J, Huang G. Upregulated circRNA ARHGAP10 predicts an unfavorable prognosis in NSCLC through regulation of the miR-150-5p/GLUT-1 axis. Mol Ther Nucleic Acids (2019) 18:219–31.
131. Yu C, Cheng Z, Cui S, Mao X, Li B, Fu Y, et al. circFOXM1 promotes proliferation of non-small cell lung carcinoma cells by acting as a ceRNA to upregulate FAM83D. J Exp Clin Cancer Res CR (2020) 39(1):55.
132. Xiong Q, Liu B, Ding M, Zhou J, Yang C, Chen Y. Hypoxia and cancer related pathology. Cancer Lett (2020) 486:1–7.
133. Cao X, Li F, Shao J, Lv J, Chang A, Dong W, et al. Circular RNA hsa_circ_0102231 sponges miR-145 to promote non-small cell lung cancer cell proliferation by up-regulating the expression of RBBP4. J Biochem (2020). doi: 10.1093/jb/mvaa093
134. Yu C, Tian F, Liu J, Su M, Wu M, Zhu X, et al. Circular RNA cMras inhibits lung adenocarcinoma progression via modulating miR-567/PTPRG regulatory pathway. Cell proliferation (2019) 52(3):e12610.
135. Li L, Wei H, Zhang H, Xu F, Che G. Circ_100565 promotes proliferation, migration and invasion in non-small cell lung cancer through upregulating HMGA2 via sponging miR-506-3p. Cancer Cell Int (2020) 20:160.
136. Ma D, Qin Y, Huang C, Chen Y, Han Z, Zhou X, et al. Circular RNA ABCB10 promotes non-small cell lung cancer progression by increasing E2F5 expression through sponging miR-584-5p. Cell Cycle (2020), 1–10. doi: 10.1080/15384101.2020.1761617
137. Mao Y, He JX, Zhu M, Dong YQ, He JX. Circ0001320 inhibits lung cancer cell growth and invasion by regulating TNFAIP1 and TPM1 expression through sponging miR-558. Hum Cell (2020). doi: 10.1007/s13577-020-00453-4
138. Wang Q, Kang PM. CircRNA_001010 adsorbs miR-5112 in a sponge form to promote proliferation and metastasis of non-small cell lung cancer (NSCLC). Eur Rev Med Pharmacol Sci (2020) 24(8):4271–80. doi: 10.26355/eurrev_202004_21007
139. Li L, Wan K, Xiong L, Liang S, Tou F, Guo S, et al. CircRNA hsa_circ_0087862 acts as an oncogene in non-small cell lung cancer by targeting miR-1253/RAB3D axis. OncoTargets and therapy. (2020) 13:2873–86.
140. Wang Liang L, Mao Y, Xia Q, Chen W, Shen B, H, et al. Circular RNA circCRIM1 inhibits invasion and metastasis in lung adenocarcinoma through the microRNA (miR)-182/miR-93-leukemia inhibitory factor receptor pathway. Cancer Sci (2019) 110(9):2960–72.
141. Zuo Y, Shen W, Wang C, Niu N, Pu J. Circular RNA circ-ZNF609 promotes lung adenocarcinoma proliferation by modulating miR-1224-3p/ETV1 signaling. Cancer Manage Res (2020) 12:2471–9.
142. Ruiz-Cordero R, Devine WP. Targeted therapy and checkpoint immunotherapy in lung cancer. Surg Pathol Clin (2020) 13(1):17–33.
143. Liu Y, Yang C, Cao C, Li Q, Jin X, Shi H, et al. Hsa_circ_RNA_0011780 represses the proliferation and metastasis of non-small cell lung cancer by decreasing FBXW7 via targeting miR-544a. OncoTargets Ther (2020) 13:745–55.
144. Tan Z, Cao F, Jia B, Xia L. Circ_0072088 promotes the development of non-small cell lung cancer via the miR-377-5p/NOVA2 axis. Thorac Cancer (2020).
145. Bai Q, Li L, Chen F, Zhu J, Cao L, Yang Y, et al. Suppression of circular RNA Hsa_circ_0109320 attenuates non-small cell lung cancer progression via MiR-595/E2F7 axis. Med Sci monitor Int Med J Exp Clin Res (2020) 26:e921200.
146. Chen X, Mao R, Su W, Yang X, Geng Q, Guo C, et al. Circular RNA circHIPK3 modulates autophagy via MIR124-3p-STAT3-PRKAA/AMPKα signaling in STK11 mutant lung cancer. Autophagy (2020) 16(4):659–71.
147. Zhou ZF, Wei Z, Yao JC, Liu SY, Wang F, Wang Z, et al. CircRNA_102179 promotes the proliferation, migration and invasion in non-small cell lung cancer cells by regulating miR-330-5p/HMGB3 axis. Pathol Res Pract (2020) 216(11):153144.
148. Zhang P, Xue XF, Ling XY, Yang Q, Yu Y, Xiao J, et al. CircRNA_010763 promotes growth and invasion of lung cancer through serving as a molecular sponge of miR-715 to induce c-myc expression. Eur Rev Med Pharmacol Sci (2020) 24(13):7310–9.
149. Chen H, Wu G, Chen G, Wang W, Ruan F. Circular RNA THBS1 promotes proliferation and apoptosis of non-small cell lung cancer cells by sponging miR-129-5p and regulating SOX4 expression. J buon (2020) 25(4):1721–7.
150. Qin H, Liu J, Du ZH, Hu R, Yu YK, Wang QA. Circular RNA hsa_circ_0012673 facilitates lung cancer cell proliferation and invasion via miR-320a/LIMK18521 axis. Eur Rev Med Pharmacol Sci (2020) 24(4):1841–52.
151. Ma X, Yang X, Bao W, Li S, Liang S, Sun Y, et al. Circular RNA circMAN2B2 facilitates lung cancer cell proliferation and invasion via miR-1275/FOXK1 axis. Biochem Biophys Res Commun (2018) 498(4):1009–15.
152. Liu W, Ma W, Yuan Y, Zhang Y, Sun S. Circular RNA hsa_circRNA_103809 promotes lung cancer progression via facilitating ZNF121-dependent MYC expression by sequestering miR-4302. Biochem Biophys Res Commun (2018) 500(4):846–51.
153. Qu D, Yan B, Xin R, Ma T. A novel circular RNA hsa_circ_0020123 exerts oncogenic properties through suppression of miR-144 in non-small cell lung cancer. Am J Cancer Res (2018) 8(8):1387–402.
154. Zhao F, Han Y, Liu Z, Zhao Z, Li Z, Jia K. circFADS2 regulates lung cancer cells proliferation and invasion via acting as a sponge of miR-498. Biosci Rep (2018) 38(4).
155. Chang H, Qu J, Wang J, Liang X, Sun W. Circular RNA circ_0026134 regulates non-small cell lung cancer cell proliferation and invasion via sponging miR-1256 and miR-1287. BioMed Pharmacother (2019) 112:108743.
156. Hao Y, Xi J, Peng Y, Bian B, Hao G, Xi Y, et al. Circular RNA Circ_0016760 modulates non-Small-Cell lung cancer growth through the miR-577/ZBTB7A axis. Cancer Manag Res (2020) 12:5561–74.
157. Li Y, Hu J, Li L, Cai S, Zhang H, Zhu X, et al. Upregulated circular RNA circ_0016760 indicates unfavorable prognosis in NSCLC and promotes cell progression through miR-1287/GAGE1 axis. Biochem Biophys Res Commun (2018) 503(3):2089–94.
158. Han J, Zhao G, Ma X, Dong Q, Zhang H, Wang Y, et al. CircRNA circ-BANP-mediated miR-503/LARP1 signaling contributes to lung cancer progression. Biochem Biophys Res Commun (2018) 503(4):2429–35. doi: 10.1016/j.bbrc.2018.06.172
159. Ding L, Yao W, Lu J, Gong J, Zhang X. Upregulation of circ_001569 predicts poor prognosis and promotes cell proliferation in non-small cell lung cancer by regulating the wnt/β-catenin pathway. Oncol Lett (2018) 16(1):453–8. doi: 10.3892/ol.2018.8673
160. Liu G, Shi H, Deng L, Zheng H, Kong W, Wen X, et al. Circular RNA circ-FOXM1 facilitates cell progression as ceRNA to target PPDPF and MACC1 by sponging miR-1304-5p in non-small cell lung cancer. Biochem Biophys Res Commun (2019) 513(1):207–12.
161. Qiu BQ, Zhang PF, Xiong D, Xu JJ, Long X, Zhu SQ, et al. CircRNA fibroblast growth factor receptor 3 promotes tumor progression in non-small cell lung cancer by regulating galectin-1-AKT/ERK1/2 signaling. J Cell Physiol (2019) 234(7):11256–64.
162. Li X, Zhang Z, Jiang H, Li Q, Wang R, Pan H, et al. Circular RNA circPVT1 promotes proliferation and invasion through sponging miR-125b and activating E2F2 signaling in non-small cell lung cancer. Cell Physiol Biochem (2018) 51(5):2324–40.
163. Qin S, Zhao Y, Lim G, Lin H, Zhang X, Zhang X. Circular RNA PVT1 acts as a competing endogenous RNA for miR-497 in promoting non-small cell lung cancer progression. BioMed Pharmacother (2019) 111:244–50. doi: 10.1016/j.biopha.2018.12.007
164. Li W, Jiang W, Liu T, Lv J, Guan J. Enhanced expression of circ_0000735 forecasts clinical severity in NSCLC and promotes cell progression via sponging miR-1179 and miR-1182. Biochem Biophys Res Commun (2019) 510(3):467–71.
165. Chen L, Nan A, Zhang N, Jia Y, Li X, Ling Y, et al. Circular RNA 100146 functions as an oncogene through direct binding to miR-361-3p and miR-615-5p in non-small cell lung cancer. Mol Cancer (2019) 18(1):13.
166. Cui J, Li W, Liu G, Chen X, Gao X, Lu H, et al. A novel circular RNA, hsa_circ_0043278, acts as a potential biomarker and promotes non-small cell lung cancer cell proliferation and migration by regulating miR-520f. Artif Cells Nanomed Biotechnol (2019) 47(1):810–21.
167. Han W, Wang L, Zhang L, Wang Y, Li Y. Circular RNA circ-RAD23B promotes cell growth and invasion by miR-593-3p/CCND2 and miR-653-5p/TIAM1 pathways in non-small cell lung cancer. Biochem Biophys Res Commun (2019) 510(3):462–6.
168. An J, Shi H, Zhang N, Song S. Elevation of circular RNA circ_0003645 forecasts unfavorable prognosis and facilitates cell progression via miR-1179/TMEM14A pathway in non-small cell lung cancer. Biochem Biophys Res Commun (2019) 511(4):921–5.
169. Wang T, Wang X, Du Q, Wu N, Liu X, Chen Y, et al. The circRNA circP4HB promotes NSCLC aggressiveness and metastasis by sponging miR-133a-5p. Biochem Biophys Res Commun (2019) 513(4):904–11.
170. Yang L, Wang J, Fan Y, Yu K, Jiao B, Su X. Hsa_circ_0046264 up-regulated BRCA2 to suppress lung cancer through targeting hsa-miR-1245. Respir Res (2018) 19(1):115.
171. Abbas T, Dutta A. p21 in cancer: intricate networks and multiple activities. Nat Rev Cancer (2009) 9(6):400–14.
172. Zhu X, Wang X, Wei S, Chen Y, C. Y, Fan X, et al. hsa_circ_0013958: a circular RNA and potential novel biomarker for lung adenocarcinoma. FEBS J (2017) 284(14):2170–82. doi: 10.1111/febs.14132
173. Dai X, Zhang N, Cheng Y, Yang T, Chen Y, Liu Z, et al. RNA-Binding protein trinucleotide repeat-containing 6A regulates the formation of circular RNA circ0006916, with important functions in lung cancer cells. Carcinogenesis (2018) 39(8):981–92. doi: 10.1093/carcin/bgy061
174. Cheng Z, Yu C, Cui S, Wang H, Jin H, Wang C, et al. circTP63 functions as a ceRNA to promote lung squamous cell carcinoma progression by upregulating FOXM1. Nat Commun (2019) 10(1):3200.
175. Tian X, Zhang L, Jiao Y, Chen J, Shan Y, Yang W. CircABCB10 promotes nonsmall cell lung cancer cell proliferation and migration by regulating the miR-1252/FOXR2 axis. J Cell Biochem (2019) 120(3):3765–72. doi: 10.1002/jcb.27657
176. Wang J, Li H. CircRNA circ_0067934 silencing inhibits the proliferation, migration and invasion of NSCLC cells and correlates with unfavorable prognosis in NSCLC. Eur Rev Med Pharmacol Sci (2018) 22(10):3053–60.
177. Huang W, Yang Y, Wu J, Niu Y, Yao Y, Zhang J, et al. Circular RNA cESRP1 sensitises small cell lung cancer cells to chemotherapy by sponging miR-93-5p to inhibit TGF-β signalling. Cell Death Differ (2020) 27(5):1709–27. doi: 10.1038/s41418-019-0455-x
178. Xue YB, Ding MQ, Xue L, Luo JH. CircAGFG1 sponges miR-203 to promote EMT and metastasis of non-small-cell lung cancer by upregulating ZNF281 expression. Thorac Cancer (2019) 10(8):1692–701.
179. Wei S, Zheng Y, Jiang Y, Li X, Geng J, Shen Y, et al. The circRNA circPTPRA suppresses epithelial-mesenchymal transitioning and metastasis of NSCLC cells by sponging miR-96-5p. EBioMedicine (2019) 44:182–93. doi: 10.1016/j.ebiom.2019.05.032
180. Tan Z, Sun Y, Liu M, Xia L, Cao F, Qi Y, et al. Naringenin inhibits cell migration, invasion, and tumor growth by regulating circFOXM1/miR-3619-5p/SPAG5 axis in lung cancer. Cancer Biother Radiopharm (2020). doi: 10.1089/cbr.2019.3520
181. Sarkar FH, Li Y, Wang Z, Kong D, Ali S. Implication of microRNAs in drug resistance for designing novel cancer therapy. Drug Resist Update (2010) 13(3):57–66. doi: 10.1016/j.drup.2010.02.001
182. Suresh R, Ali S, Ahmad A, Philip PA, Sarkar FH. The role of cancer stem cells in recurrent and drug-resistant lung cancer. Adv Exp Med Biol (2016) 890:57–74.
183. Gower A, Wang Y, Giaccone G. Oncogenic drivers, targeted therapies, and acquired resistance in non-small-cell lung cancer. J Mol Med (Berl) (2014) 92(7):697–707.
184. Szakács G, Paterson JK, Ludwig JA, Booth-Genthe C, Gottesman MM. Targeting multidrug resistance in cancer. Nat Rev Drug Discovery (2006) 5(3):219–34.
185. Nguyen LV, Vanner R, Dirks P, Eaves CJ. Cancer stem cells: an evolving concept. Nat Rev Cancer (2012) 12(2):133–43.
186. Yadav AK, Desai NS. Cancer stem cells: Acquisition, characteristics, therapeutic implications, targeting strategies and future prospects. Stem Cell Rev Rep (2019) 15(3):331–55. doi: :10.1007/s12015-019-09887-2
187. Moro M, Bertolini G, Pastorino U, Roz L, Sozzi G. Combination treatment with all-trans retinoic acid prevents cisplatin-induced enrichment of CD133+ tumor-initiating cells and reveals heterogeneity of cancer stem cell compartment in lung cancer. J Thorac Oncol (2015) 10(7):1027–36.
188. Szabo L, Morey R, Palpant NJ, Wang PL, Afari N, Jiang C, et al. Statistically based splicing detection reveals neural enrichment and tissue-specific induction of circular RNA during human fetal development. Genome Biol (2015) 16:126.
189. Wang L, Zheng C, Wu X, Zhang Y, Yan S, Ruan L, et al. Circ-SOX4 promotes non-small cell lung cancer progression by activating the wnt/β-catenin pathway. Mol Oncol (2020). doi: 10.1002/1878-0261.12656
190. Zhao Y, Zheng R, Chen J, Ning D. CircRNA CDR1as/miR-641/HOXA9 pathway regulated stemness contributes to cisplatin resistance in non-small cell lung cancer (NSCLC). Cancer Cell Int (2020) 20:289.
191. Zheng F, Xu R. CircPVT1 contributes to chemotherapy resistance of lung adenocarcinoma through miR-145-5p/ABCC1 axis. BioMed Pharmacother (2020) 124:109828.
192. Mao Y, Xu R. Circular RNA CDR1-AS contributes to pemetrexed and cisplatin chemoresistance through EGFR/PI3K signaling pathway in lung adenocarcinoma. BioMed Pharmacother (2020) 123:109771. doi: 10.1016/j.biopha.2019.109771
193. R K. Circular RNA hsa_circ_0085131 is involved in cisplatin-resistance of non-small cell lung cancer cells by regulating autophagy. Cell Biol Int (2020).
194. Wen C, Xu G, He S, Huang Y, Shi J, Wu L, et al. Screening circular RNAs related to acquired gefitinib resistance in non-small cell lung cancer cell lines. J Cancer (2020) 11(13):3816–26.
195. Xu Y, Jiang T, Wu C, Zhang Y. CircAKT3 inhibits glycolysis balance in lung cancer cells by regulating miR-516b-5p/STAT3 to inhibit cisplatin sensitivity. Biotechnol Lett (2020) 42(7):1123–35.
196. Huang MS, Liu JY, Xia XB, Liu YZ, Li X, Yin JY, et al. Hsa_circ_0001946 inhibits lung cancer progression and mediates cisplatin sensitivity in non-small cell lung cancer via the nucleotide excision repair signaling pathway. Front Oncol (2019) 9:508.
197. Dong Y, Xu T, Zhong S, Wang B, Zhang H, Wang X, et al. Circ_0076305 regulates cisplatin resistance of non-small cell lung cancer via positively modulating STAT3 by sponging miR-296-5p. Life Sci (2019) 239:116984.
198. Zhou Y, Zheng X, Xu B, Chen L, Wang Q, Deng H, et al. Circular RNA hsa_circ_0004015 regulates the proliferation, invasion, and TKI drug resistance of non-small cell lung cancer by miR-1183/PDPK1 signaling pathway. Biochem Biophys Res Commun (2019) 508(2):527–35.
199. Yu W, Peng W, Sha H, Li J. Hsa_circ_0003998 promotes chemoresistance via modulation of miR-326 in lung adenocarcinoma cells. Oncol Res (2019) 27(5):623–8.
200. Li YH, Xu CL, He CJ, Pu HH, Liu JL, Wang Y. circMTDH.4/miR-630/AEG-1 axis participates in the regulation of proliferation, migration, invasion, chemoresistance, and radioresistance of NSCLC. Mol Carcinog (2020) 59(2):141–53.
201. Wu Z, Gong Q, Yu Y, Zhu J, Li W. Knockdown of circ-ABCB10 promotes sensitivity of lung cancer cells to cisplatin via miR-556-3p/AK4 axis. BMC Pulm Med (2020) 20(1):10.
202. Xiao G, Huang W, Zhan Y, Li J, Tong W. CircRNA_103762 promotes multidrug resistance in NSCLC by targeting DNA damage inducible transcript 3 (CHOP). J Clin Lab Anal (2020):e23252.
203. Zhang PF, Pei X, Li KS, Jin LN, Wang F, Wu J, et al. Circular RNA circFGFR1 promotes progression and anti-PD-1 resistance by sponging miR-381-3p in non-small cell lung cancer cells. Mol Cancer (2019) 18(1):179.
204. Ye Y, Zhao L, Li Q, Xi C, Li Y, Li Z, et al. circ_0007385 served as competing endogenous RNA for miR-519d-3p to suppress malignant behaviors and cisplatin resistance of non-small cell lung cancer cells. Thorac Cancer (2020).
205. Tong S. Circular RNA SMARCA5 may serve as a tumor suppressor in non-small cell lung cancer. J Clin Lab Anal (2020) 34(5):e23195.
207. Shangguan H, Feng H, Lv D, Wang J, Tian T, Wang X, et al. Circular RNA circSLC25A16 contributes to the glycolysis of non-small-cell lung cancer through epigenetic modification. Cell Death Dis (2020) 11(6):437. doi: 10.1038/s41419-020-2635-5
208. Wu W, Xi W, Li H, Yang M, Yao X. Circular RNA circ−ACACA regulates proliferation, migration and glycolysis in non−small−cell lung carcinoma via miR−1183 and PI3K/PKB pathway. Int J Mol Med (2020) 45(6):1814–24.
209. Zhang SJ, Ma J, Wu JC, Hao ZZ, Zhang YA, Zhang YJ. Circular RNA circCRIM1 suppresses lung adenocarcinoma cell migration, invasion, EMT, and glycolysis through regulating miR-125b-5p/BTG2 axis. Eur Rev Med Pharmacol Sci (2020) 24(7):3761–74. doi: 10.26355/eurrev_202004_20841
210. Lu J, Zhu Y, Qin Y, Chen Y. CircNFIX acts as a miR-212-3p sponge to enhance the malignant progression of non-small cell lung cancer by up-regulating ADAM10. Cancer Manag Res (2020) 12:9577–87. doi: 10.2147/CMAR.S272309
211. Quail DF, Joyce JA. Microenvironmental regulation of tumor progression and metastasis. Nat Med (2013) 19(11):1423–37. doi: 10.1038/nm.3394
212. Keir ME, Butte MJ, Freeman GJ, Sharpe AH. PD-1 and its ligands in tolerance and immunity. Annu Rev Immunol (2008) 26:677–704. doi: 10.1146/annurev.immunol.26.021607.090331
213. Li L, Zhang Q, Lian K. Circular RNA circ_0000284 plays an oncogenic role in the progression of non-small cell lung cancer through the miR-377-3p-mediated PD-L1 promotion. Cancer Cell Int (2020) 20:247.
214. Su C, Han Y, Zhang H, Li Y, Yi L, Wang X, et al. CiRS-7 targeting miR-7 modulates the progression of non-small cell lung cancer in a manner dependent on NF-κB signalling. J Cell Mol Med (2018) 22(6):3097–107.
215. Kumar M, Sahu SK, Kumar R, Subuddhi A, Maji RK, Jana K, et al. MicroRNA let-7 modulates the immune response to mycobacterium tuberculosis infection via control of A20, an inhibitor of the NF-κB pathway. Cell Host Microbe (2015) 17(3):345–56.
216. Schaefer L, Reinhardt DP. Special issue: Extracellular matrix: Therapeutic tools and targets in cancer treatment. Adv Drug Delivery Rev (2016) 97:1–3.
217. Luo YH, Zhu XZ, Huang KW, Zhang Q, Fan YX, Yan PW, et al. Emerging roles of circular RNA hsa_circ_0000064 in the proliferation and metastasis of lung cancer. BioMed Pharmacother (2017) 96:892–8.
218. Velasco-Hernandez T, Hyrenius-Wittsten A, Rehn M, Bryder D, Cammenga J. HIF-1α can act as a tumor suppressor gene in murine acute myeloid leukemia. Blood (2014) 124(24):3597–607.
219. Chi Y, Luo Q, Song Y, Yang F, Wang Y, Jin M, et al. Circular RNA circPIP5K1A promotes non-small cell lung cancer proliferation and metastasis through miR-600/HIF-1α regulation. J Cell Biochem (2019) 120(11):19019–30.
220. Cheng X, Qiu J, Wang S, Yang Y, Guo M, Wang D, et al. Comprehensive circular RNA profiling identifies CircFAM120A as a new biomarker of hypoxic lung adenocarcinoma. Ann Transl Med (2019) 7(18):442.
221. Luo YH, Yang YP, Chien CS, Yarmishyn AA, Ishola AA, Chien Y, et al. Plasma level of circular RNA hsa_circ_0000190 correlates with tumor progression and poor treatment response in advanced lung cancers. Cancers (Basel) (2020) 12(7).
222. Chen X, Xie R, Gu P, Huang M, Han J, Dong W, et al. Long noncoding RNA LBCS inhibits self-renewal and chemoresistance of bladder cancer stem cells through epigenetic silencing of SOX2. Clin Cancer Res (2019) 25(4):1389–403.
223. Huang H, Weng H, Sun W, Qin X, Shi H, Wu H, et al. Recognition of RNA N(6)-methyladenosine by IGF2BP proteins enhances mRNA stability and translation. Nat Cell Biol (2018) 20(3):285–95.
224. Zong L, Sun Q, Zhang H, Chen Z, Deng Y, Li D, et al. Increased expression of circRNA_102231 in lung cancer and its clinical significance. Biomedicine pharmacotherapy (2018) 102:639–44. doi: 10.1016/j.biopha.2018.03.084
225. Zhang S, Zeng X, Ding T, Guo L, Li Y, Ou S, et al. Microarray profile of circular RNAs identifies hsa_circ_0014130 as a new circular RNA biomarker in non-small cell lung cancer. Sci Rep (2018) 8(1):2878.
226. Li J, Wang J, Chen Z, Chen Y, Jin M. Hsa_circ_0079530 promotes cell proliferation and invasion in non-small cell lung cancer. Gene (2018) 665:1–5.
227. Lu GJ, Cui J, Qian Q, Hou ZB, Xie HY, Hu W, et al. Overexpression of hsa_circ_0001715 is a potential diagnostic and prognostic biomarker in lung adenocarcinoma. Onco Targets Ther (2020) 13:10775–83.
228. Zhang Y, Zhao H, Zhang L. Identification of the tumor−suppressive function of circular RNA FOXO3 in non−small cell lung cancer through sponging miR−155. Mol Med Rep (2018) 17(6):7692–700.
229. Hang Zhou D, Qin J, Zhou N, Ma W, Jin H, G, et al. A novel plasma circular RNA circFARSA is a potential biomarker for non-small cell lung cancer. Cancer Med (2018) 7(6):2783–91.
230. Yao JT, Zhao SH, Liu QP, Lv MQ, Zhou DX, Liao ZJ, et al. Over-expression of CircRNA_100876 in non-small cell lung cancer and its prognostic value. Pathology Res Pract (2017) 213(5):453–6.
231. Qiu M, Xia W, Chen R, Wang S, Xu Y, Ma Z, et al. The circular RNA circPRKCI promotes tumor growth in lung adenocarcinoma. Cancer Res (2018) 78(11):2839–51.
232. Li M, Hua Q, Shao Y, Zeng H, Liu Y, Diao Q, et al. Circular RNA circBbs9 promotes PM(2.5)-induced lung inflammation in mice via NLRP3 inflammasome activation. Environ Int (2020) 143:105976.
233. Xu K, Zhang Y, Xiong W, Zhang Z, Wang Z, Lv L, et al. CircGRIA1 shows an age-related increase in male macaque brain and regulates synaptic plasticity and synaptogenesis. Nat Commun (2020) 11(1):3594.
234. Ke L, Yang DC, Wang Y, Ding Y, Gao G. AnnoLnc2: the one-stop portal to systematically annotate novel lncRNAs for human and mouse. Nucleic Acids Res (2020) 48(W1):W230–w238.
235. Zhang HD, Jiang LH, Sun DW, Hou JC, Ji ZL. CircRNA: a novel type of biomarker for cancer. Breast Cancer (2018) 25(1):1–7.
236. Wang F, Mi YJ, Chen XG, Wu XP, Liu Z, Chen SP, et al. Axitinib targeted cancer stemlike cells to enhance efficacy of chemotherapeutic drugs via inhibiting the drug transport function of ABCG2. Mol Med (2012) 18(1):887–98.
237. Glažar P, Papavasileiou P, Rajewsky N. circBase: a database for circular RNAs. Rna (2014) 20(11):1666–70.
238. Yang JH, Qu LH. DeepBase: annotation and discovery of microRNAs and other noncoding RNAs from deep-sequencing data. Methods Mol Biol (2012) 822:233–48.
239. Liu YC, Li JR, Sun CH, Andrews E, Chao RF, Lin FM, et al. CircNet: a database of circular RNAs derived from transcriptome sequencing data. Nucleic Acids Res (2016) 44(D1):D209–15.
240. Chen X, Han P, Zhou T, Guo X, Song X, Li Y. circRNADb: A comprehensive database for human circular RNAs with protein-coding annotations. Sci Rep (2016) 6:34985.
241. Dudekula DB, Panda AC, Grammatikakis I, De S, Abdelmohsen K, Gorospe M. CircInteractome: A web tool for exploring circular RNAs and their interacting proteins and microRNAs. RNA Biol (2016) 13(1):34–42.
242. Xia S, Feng J, Chen K, Ma Y, Gong J, Cai F, et al. CSCD: a database for cancer-specific circular RNAs. Nucleic Acids Res (2018) 46(D1):D925–d929.
243. Dong R, Ma XK, Li GW, Yang L. CIRCpedia v2: An updated database for comprehensive circular RNA annotation and expression comparison. Genomics Proteomics Bioinf (2018) 16(4):226–33.
244. Xia S, Feng J, Lei L, Hu J, Xia L, Wang J, et al. Comprehensive characterization of tissue-specific circular RNAs in the human and mouse genomes. Brief Bioinform (2017) 18(6):984–92.
245. Li S, Li Y, Chen B, Zhao J, Yu S, Tang Y, et al. exoRBase: a database of circRNA, lncRNA and mRNA in human blood exosomes. Nucleic Acids Res (2018) 46(D1):D106–d112.
246. Zhao Z, Wang K, Wu F, Wang W, Zhang K, Hu H, et al. circRNA disease: a manually curated database of experimentally supported circRNA-disease associations. Cell Death Dis (2018) 9(5):475.
247. Zhao M, Qu H. circVAR database: genome-wide archive of genetic variants for human circular RNAs. BMC Genomics (2020) 21(1):750.
248. Huang W, Ling Y, Zhang S, Xia Q, Cao R, Fan X, et al. TransCirc: an interactive database for translatable circular RNAs based on multi-omics evidence. Nucleic Acids Res (2020).
249. Lyu Y, Caudron-Herger M, Diederichs S. circ2GO: A database linking circular RNAs to gene function. Cancers (Basel) (2020) 12(10).
250. Chaput N, Flament C, Viaud S, Taieb J, Roux S, Spatz A, et al. Dendritic cell derived-exosomes: biology and clinical implementations. J Leukoc Biol (2006) 80(3):471–8.
251. Chen R, Xu X, Qian Z, Zhang C, Niu Y, Wang Z, et al. The biological functions and clinical applications of exosomes in lung cancer. Cell Mol Life Sci (2019) 76(23):4613–33.
252. Hsu YL, Hung JY, Chang WA, Lin YS, Pan YC, Tsai PH, et al. Hypoxic lung cancer-secreted exosomal miR-23a increased angiogenesis and vascular permeability by targeting prolyl hydroxylase and tight junction protein ZO-1. Oncogene (2017) 36(34):4929–42.
Keywords: circRNA, lung cancer, clinical significance, diagnostic, mechanism
Citation: Ren W, Yuan Y, Peng J, Mutti L and Jiang X (2022) The function and clinical implication of circular RNAs in lung cancer. Front. Oncol. 12:862602. doi: 10.3389/fonc.2022.862602
Received: 26 January 2022; Accepted: 26 August 2022;
Published: 19 October 2022.
Edited by:
Ying Liu, Qingdao University, ChinaReviewed by:
Huaichao Luo, Sichuan Cancer Hospital, ChinaJie Song, Chinese Academy of Medical Sciences and Peking Union Medical College, China
Copyright © 2022 Ren, Yuan, Peng, Mutti and Jiang. This is an open-access article distributed under the terms of the Creative Commons Attribution License (CC BY). The use, distribution or reproduction in other forums is permitted, provided the original author(s) and the copyright owner(s) are credited and that the original publication in this journal is cited, in accordance with accepted academic practice. No use, distribution or reproduction is permitted which does not comply with these terms.
*Correspondence: Xiulin Jiang, jiangxiulin@mail.kiz.ac.cn; Luciano Mutti, luciano.mutti@temple.edu; Jun Peng, pengjun_fphyp@126.com
†These authors have contributed equally to this work