- 1College of Agronomy, Liaocheng University, Liaocheng, China
- 2Veterinary Medicine, Xinjiang Agricultural University, Urumqi, China
The makorin RING finger protein (MKRN) gene family encodes proteins (makorins) with a characteristic array of zinc-finger motifs present in a wide array from invertebrates to vertebrates. MKRNs (MKRN1, MKRN2, MKRN3, MKRN4) as RING finger E3 ligases that mediate substrate degradation are related with conserved RING finger domains that control multiple cellular components via the ubiquitin-proteasome system (UPS), including p53, p21, FADD, PTEN, p65, Nptx1, GLK, and some viral or bacterial proteins. MKRNs also served as diverse roles in disease, like MKRN1 in transcription regulation, metabolic disorders, and tumors; MKRN2 in testis physiology, neurogenesis, apoptosis, and mutation of MKRN2 regulation signals transduction, inflammatory responses, melanoma, and neuroblastoma; MKRN3 in central precocious puberty (CPP) therapy; and MKRN4 firstly reported as a novel E3 ligase instead of a pseudogene to contribute to systemic lupus erythematosus (SLE). Here, we systematically review advances in the gene’s expression, function, and role of MKRNs orthologs in disease and pathogens infection. Further, MKRNs can be considered targets for the host’s innate intracellular antiviral defenses and disease therapy.
Introduction
Orthologs of the Makorin RING finger protein (MKRN) have been found in fungi, plants, and mammals (1). The mkrn gene family encodes proteins with unique zinc finger arrays, including C3H motifs, a new Cys-His motif, and a RING finger. The ancestral founder of this gene family is mkrn1. Another component, mkrn2, may have evolved 450 million years ago due to mkrn1 gene duplication (2). mkrn3 is an intronless gene found on chromosome 15’s long arm in the Prader-Willi syndrome (PWS) crucial area (3). mkrn4 has been previously known as MKRNB (4).
Ubiquitination is a post-translational modification mechanism involved in several biological processes, including cell survival, differentiation, innate and adaptive immunity. Ub is covalently coupled to the target protein with single or multiple 76 amino acid globular protein by activating (E1), conjugating (E2), and ligating (E3) enzymes (5). These three types of enzymes work in a certain order. E1 activates Ub before it is passed to an E2 conjugating enzyme. Following that, E3 ubiquitin ligases attach to E2 and the substrate, principally supplying the Ub chain to the substrate and promoting isopeptide synthesis. Finally, the 26S proteasome degrades the target protein into tiny peptide fragments (6, 7). Indeed, the presence of the RING finger domain is the most noticeable structural feature of MKRNs, not only because this region is found in proteins that form repressive complexes to reduce target gene activity (8), but also because it is a signature domain of E3 ubiquitin ligases (9). This review will concentrate on the function of E3 ubiquitin ligases to mediate substrate degradation by UPS of MKRN1, MKRN2, MKRN3, and MKRN4. Special attention is also focused on the potential role of MKRNs in physiological functions and disease regulation.
mkrn Gene and Expression
Makorin RING finger protein (MKRN) is produced by the mkrn gene family, distinguished by the intron-containing founder of the intronless and a high level of sequence conservation in taxa spanning from invertebrates to vertebrates. Nine mkrn family loci spread throughout the human genome (2). So far, four functional mkrn genes mkrn1, mkrn2, mkrn3 and mkrn4 have been elaborated in literature. mkrn1 is ancestral gene of this family and characterized in humans, mice, wallabies, chickens, pigs drosophila, nematode and plants (10, 11). MKRN1 is substantially and ubiquitously expressed in human organs, including the hypothalamus and the amygdala, according to expression assessments. When transfected into different cell types, MKRN1 is expressed in the nucleus and cytoplasm.
The identification and characterization of the mkrn2 locus in yellowtail fish aided research into the makorin gene family. mkrn2 orthologs are found in humans, mice, and zebrafish. mkrn2 is assumed to have evolved from an ancestral mkrn1 by gene duplication 450 million years ago, and it (mkrn2) partially overlaps with the raf1 protooncogene in an antisense transcriptional direction. MKRN2 is found in all human tissues and cell lines, according to expression studies (12). MKRN2 expression was greater in primary leukemia samples than in age-matched normal BM cells. However, no significant association was found between MKRN2 expression levels in any leukemia subtypes (13).
mkrn3 is specific to therian mammals, and it is an intronless retrocopy of mkrn1 produced by reverse transcription of an mkrn1 mRNA molecule. The reverse transcriptase encoded by autonomous retrotransposable elements catalyzes the production of such retrogenes. Several more mkrn1 retrocopies have been found in mammalian genomes, with the majority of these most likely belonging to pseudogenes (14). The presence of mkrn3 in the dog, mouse, and human genomes, together with its lack in the chicken, fish, and platypus genomes, suggests that Prader-Willi syndrome (PWS) acquired mkrn3 critical area about 80-90 million years before (15). MKRN3 is ubiquitously expressed in adult tissues in both mice and humans, with the greatest level in the testis. MKRN3 was found in high concentrations in the brain and lung of human embryonic tissues. mkrn3 gene expression has been identified in mice from the blastocyst stage and embryonic days 8 to 17 and in ESCs (3).
However, mkrn4, a novel member of the makorin gene family, was discovered in poeciliidae fish. MKRN4 has been discovered as having gonad-specific expression in vertebrates (4). MKRN4 shares 81% of its amino acid identity with MKRN1, 46% with MKRN2, and 52% with MKRN3 (16). mkrn4 gene has been previously known as MKRNB, although it is also present in the human genome, labelled as a pseudogene (4). MKRN4 expression investigation in medaka, zebrafish (ray-finned fishes), and amphibians revealed a substantially gonad-biased expression pattern, as did MKRN1 and MKRN2, with particularly strong expression in the ovaries (2, 17).
The gene structures of the members of the makorin protein family (human mkrn1, mkrn2, mkrn3, mkrn4) were analyzed in Figure 1 to show their similarities and differences. mkrn1 and mkrn2 have the same exons, but mkrn3 has only one exon, mkrn4 has five exons. All of them possessed different exons about location and sizes.
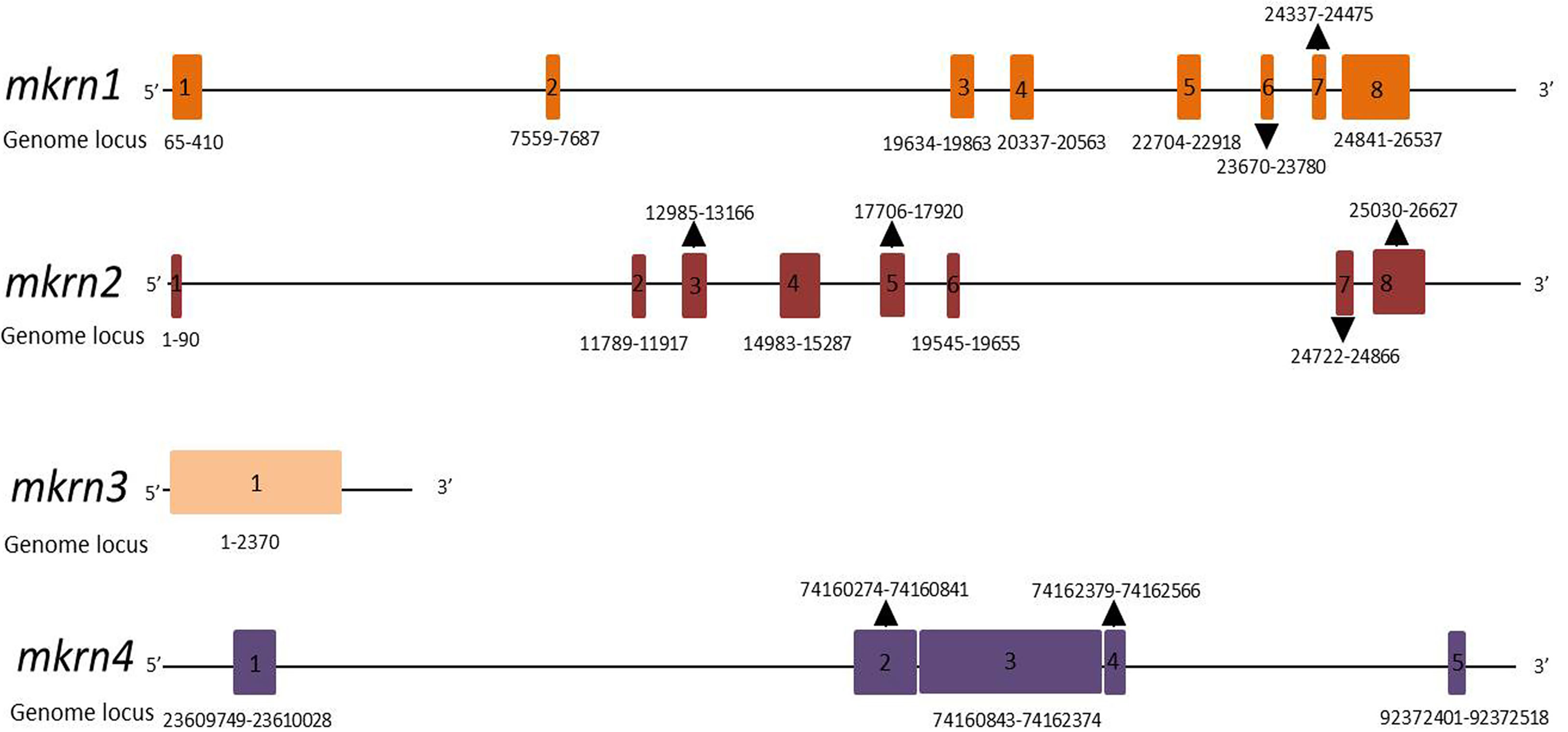
Figure 1 Schematic gene structures of human mkrn1, mkrn2, mkrn3 and mkrn4 loci. Exons are represented as boxes. Number and locus of exons in genome are indicated.
MKRN Functional Domains
The mkrn gene family encodes different proteins with diverse zinc-finger motif composition and structure, including numerous C3H motifs, a RING finger motif, and a Cys-His motif (2, 3). C3H zinc fingers, which are present in various ribonucleoproteins and may serve as RNA-binding proteins, affect post-transcriptional RNA processing at numerous levels, including alternative splicing, mRNA stability, mRNA localization, and translation efficiency (18, 19). Most E3 ubiquitin ligases have the RING finger domain, which mediates the transfer of ubiquitin from an E2 ubiquitin-conjugating enzyme to target protein substrates (20). Figure 2 demonstrated the functional domains of human MKRN1, MKRN2, MKRN3, and MKRN4. It showed that MKRN1, MKRN2, and MKRN4 have the same number of C3H-type zinc fingers, but MKRN3 has one less. They all share the identical Cys-His (CH) and C3HC4-type RING finger domains as makorins (Figure 2). Human MKRN1 has four isoforms that encoded by a single mkrn1 gene and emerge via alternative splicing and variable polyadenylation. MKRN1-long has four C3H-type zinc fingers, a Cys-His-type motif, and a highly conserved C3HC4-type RING finger domain. MKRN1-short1/MKRN1-short3 human MKRN1 transcript variants are missing the C-terminal ZF and the final 6 amino acids (aa) of the RING finger domain (RFCC), which are required for binding the second zinc ion, or the N-terminal segment (64 aa). Previous research found that the pattern of pig MKRN1 expression is similar to that of human MKRN1-short 2, which lacks the N-terminal 64 aa seen in MKNR1-long (11) (Figure 2). Three C3H zinc fingers flank the RING finger domain on its N-terminal side and one C3H zinc finger on its C-terminal side in most plants and invertebrates (but not in drosophila). The cysteine and histidine residues motif (the Cys-His motif) is located between the third C3H zinc finger and the RING domain. These domains are associated with their function, as discussed below.
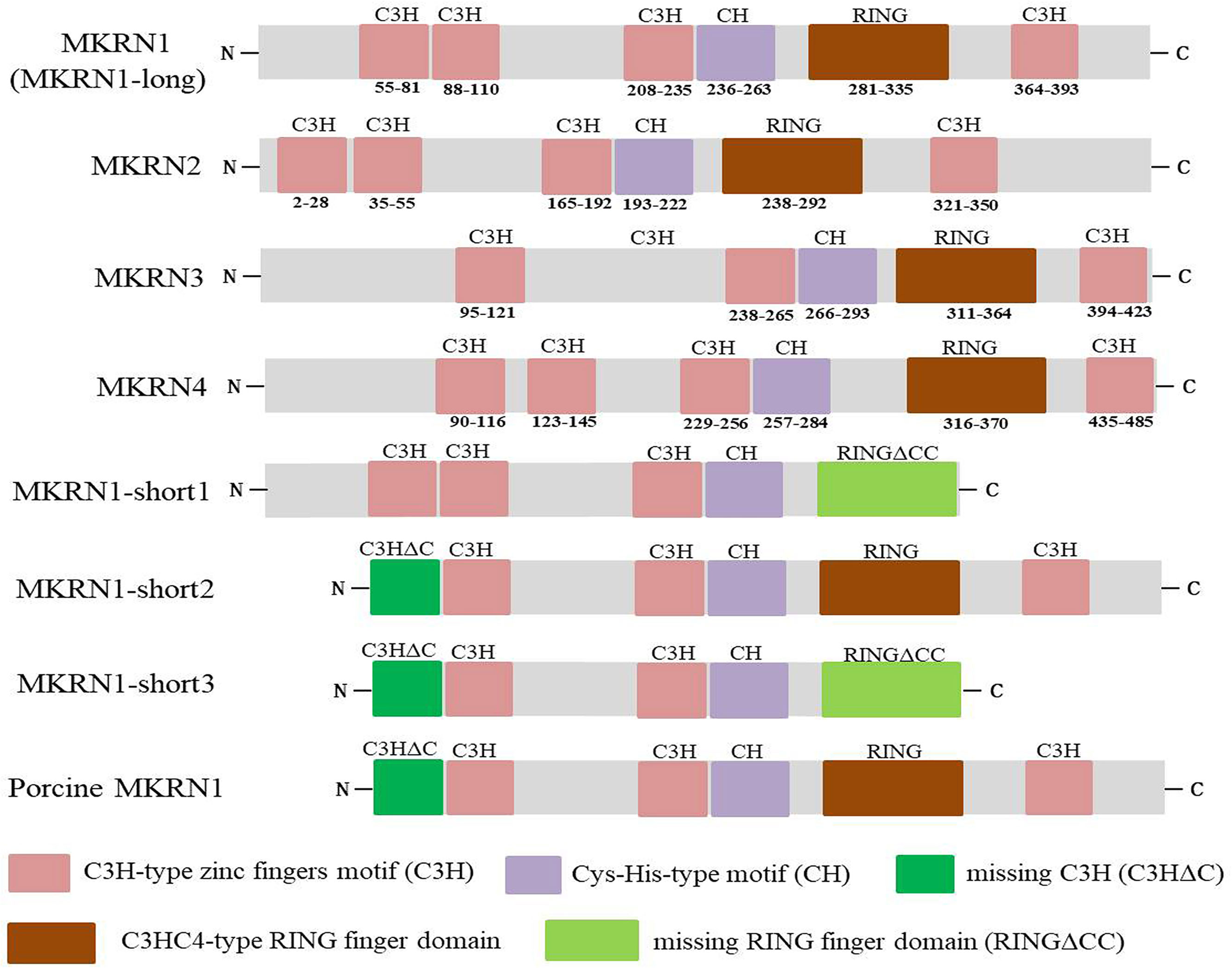
Figure 2 Functional domains of human MKRN1 (MKRN1-long), MKRN2, MKRN3 and MKRN4, the transcript variants of human MKRN1 (MKRN1-short1, MKRN1-short2, MKRN1-short3) and porcine MKRN1. The makorins contain several C3H zinc finger domains (pink), a Cys-His (CH) motif (purple), a C3HC4 RING domain (red), missing C3H motif (C3HΔC) (green) and RING finger domain (RINGΔCC) (aqua).
MKRN1 Function
Transcription Regulation
MKRN1 can regulate RNA polymerase II-dependent transcription. As a transcriptional factor, it inhibits not just c-Jun/AP-1 transcriptional activity but also numerous other RNA polymerases II-dependent transcriptional activators, including p53, NF-κB (p65), and the human androgen receptor (hAR) (21), its transrepression action is unrelated to its ubiquitin ligase activity (22). MKRN1 has been reported to act downstream of OCT 4, a transcriptional factor, suggested playing a role in establishing and maintaining totipotency or pluripotency of embryonic and undifferentiated stem cells, embryonal carcinoma cells, and embryonic germ cells in vitro (23). The pseudogene trans-regulation model is predicated on active, imprinted transcription of mkrn1-p1 to stabilize mkrn1 mRNAs in trans and down-regulate mkrn1 transcripts to exhibit the reported kidney and bone phenotypes (24). According to this scenario, the mechanism and the pseudogene should be maintained between species since their absence would destabilize MRKN1 and significantly lower reproductive fitness due to severe newborn mortality associated with its disruption. However, mkrn1-p1 is solely found in mice and not in any other species, including rats. To address this discrepancy, it was postulated that other mammalian species employ distinct mkrn1-derived pseudogenes to conduct the identical trans-stabilization of mRNA generated by the mkrn1 source gene (25).
MKRN1 was also shown to be connected with several RNA-binding proteins, indicating that it is a component of the ribonucleoprotein complex. Because MKRN1 has four C3H zinc finger domains associated with RNA-binding capability, UV crosslinking and immunoprecipitation can be used to examine MKRN1’s ability to interact directly with RNA (CLIP) (20, 26). MKRN1 has a hitherto unknown RNA-binding function, according to this study.
MKRN1 as an E3 Ubiquitin Ligase
Ubiquitylation is well recognized for directing proteins for destruction by the 26S proteasome, as well as internalization and lysosomal targeting, transcriptional control, protein interaction modulation, subcellular distribution change, DNA repair, and transmembrane signaling propagation (27–30). Ubiquitylation has been connected to almost every biological activity, which is not unexpected. The great majority of E3 ligases are RING-domain E3 ligases (RING) and RING-related E3s, which include plant homeodomain (PHD), leukemia-associated protein (LAP) finger proteins, and U-box family members (9, 31–33). More than 600 possible RING finger domain E3s are encoded in the mammalian genome (34, 35). A classical RING finger is a Zn2+-coordinating domain made up of a succession of precisely spaced cysteine and histidine residues that facilitate E2-dependent ubiquitylation (36, 37).
In the search for regulators of the ubiquitination and proteasome-dependent degradation of human telomerase reverse transcriptase (hTERT), mkrn1 was discovered as a new RING finger gene expressing E3 ligase (38). MKRN1’s E3 ligase activity, on the other hand, is linked to its gene structure in distinct orthologs. MKRN1 has recently been demonstrated to promote the degradation of several substrates via the ubiquitin-proteasome system (UPS), including host proteins p53, p21, FADD, PTEN, AMPK1 and 2, as well as viral proteins or bacteria associated with intact RING finger domains (11, 39–43).
MKRN1 interacts with viral proteins and polyubiquitinates these proteins. MKRN1 preferentially targets PCV2 Cap lysine residues and promotes polyubiquitination mediated destruction. Mutation of either of the three lysine residues in the Cap protein or histidine at residue 243 within MKRN1’s RING finger domain abolished MKRN1’s E3 ligase function, making cells incapable of triggering Cap ubiquitination and destruction (11). In a proteasome-dependent manner, MKRN1 may also cause WNVCp ubiquitination and destruction. Interestingly, MKRN1 degraded the WNV Cp mutant with amino acids 1 to 105 deleted, but not the mutant with amino acids 1 to 90 deleted. When three lysine sites at positions 101, 103, and 104 of WNV Cp were replaced with alanine, MKRN1-mediated ubiquitination and mutant degradation were significantly inhibited, indicating that these sites are required for ubiquitination (40).
Recombinant MKRN1 ubiquitinates entire M. tuberculosis in vitro, indicating a new potential role for MKRN1 against mycobacteria (44), suggesting MKRN1 E3 ligase acts as a defensive effect during pathogens infection.
In HAdV-C5-infected cells, however, the cellular E3 ubiquitin ligase MKRN1 is a unique precursor pVII interacting protein. Surprisingly, the endogenous MKRN1 protein was degraded by proteasomes during the late phase of HAdV-C5 infection in various human cell lines (45), implying that HAdV may have evolved a mechanism to avoid MKRN1-mediated host defensive strategies to benefit their replication. MKRN1 was identified as a possible common target throughout several viral infections.
Regulation of MKRN1 in Disease
MKRN1 is an E3 ubiquitin ligase that regulates metabolic diseases and malignancies through the ubiquitination of substrate proteins (39, 46). AMPK is implicated in a variety of metabolic disorders, including obesity, type 2 diabetes, fatty liver syndrome, cardiovascular disease, and cancer. AMPK is an appealing target for controlling or curing metabolic illnesses due to its impact on creating brown and beige adipose tissues and mitochondrial regeneration. MKRN1 regulates AMPK/AMPK ubiquitination and proteasome-dependent degradation to maintain its protein homeostasis might have major systemic metabolic consequences, allowing researchers to create innovative treatment techniques that target not just AMPK but also its regulators. An oncogene initiates the senescence process by activating p14ARF and then p53, preventing cells from becoming tumorigenic in the tumor suppression process (39). The endogenic p53 protein is tightly regulated by ubiquitin proteasome degradation pathway induced by negative regulator murine double minute 2 (MDM2), which inhibits the oncogenic action of MDM2 and enhances p53-dependent transactivation and apoptosis (47). However, the tumor suppressor protein p53 is also a transcriptional activator of PTEN, and this inhibits the downstream signaling of phosphatidylinositide 3 (PI3)-kinase, leading to the inactivation of AKT and, eventually, mTOR. In this mechanism, MKRN1 may cause the degradation of p14ARF, p53, and PTEN (39).
The adenomatous polyposis coli (APC) protein, which acts as a negative regulator of the Wnt signaling pathway, is also a tumor suppressor. MKRN1, an E3 ligase, has been shown to bind with and ubiquitylate APC, increasing its proteasome degradation and favorably regulating Wnt/-catenin-mediated biological activities (46). MKRN1 promotes Fas-associated protein with death domain (FADD) substrate ubiquitination and proteasome pathway degradation to delay the cell death-receptor apoptosis cascade activation by caspase 8, lower cell sensitivity to death ligands, and eventually protect against cell death. FADD content rises in MKRN1-depleted cervical cancer cells, indicating increased susceptibility to exogenous apoptotic ligands (41). Surprisingly, MKRN1 expression is reduced in cardiac tissues during intermittent hypoxia (IH). Furthermore, MKRN1 stimulates p21 ubiquitination and proteasome pathway degradation to down-regulate p21 expression, decreasing IH-induced ROS generation and myocardial apoptosis, providing a novel target for lowering cardiovascular risk in obstructive sleep apnea (OSA) patients (48).
In promoting atherosclerosis, MKRN1 expression was uniquely inhibited and contributed to endothelial cell (EC) activation and senescence, in which process TERF2IP S205 was phosphorylated and induced a downstream event of p90RSK activation (49). mkrn1 gene functions in the metabolic regulation of oogenesis through up-regulating the MKRN1 protein. It functions as a tissue-specific regulator of the insulin/Tor signaling pathway (upstream of Akt/S6K) to stimulate oogenesis in the ovaries in a nutrient-dependent manner (50). In pancreatic malignancies, lncRNA-CF129145.1 (CF129) stimulates the interaction of p53 with the E3 ligase MKRN1, resulting in the ubiquitination and degradation of the p53 protein, which inhibits the proliferation and metastasis of PC cells.
MKRN2 Function
MKRN2’s possible physiological activities have recently been identified. MKRN2 knockout mice, for example, have been shown to have problems in male fertility as well as abnormalities in testis function (51). Furthermore, MKRN2 has been implicated as a negative regulator of a variety of cellular and physiological pathways, including neurogenesis in Xenopus laevis (52, 53), NF-kB signaling in human cells (54), and non-small-cell lung cancer cell metastasis via the PI3K/Akt signaling pathway (55). MKRN2 inhibits the p53 apoptosis effector related to PMP22 (PERP) expression, and levels of the protein in sperm samples have an inverse correlation with infertility levels, implying that MKRN2 is important for protecting germ cells from excessive apoptosis and implicating MKRN2-based suppression of the p53/PERP signaling pathway in spermatogenesis and male fertility (56). In addition, specific gene missense mutation of MKRN2 is associated with degenerative lumbar spinal stenosis (DLSS), the major variant type was single nucleotide polymorphism (SNP), and C > T was the most common single nucleotide change (57), and genetic differences in microRNA 154-binding sites, as well as MKRN2, reduce or abolish microRNA-mediated regulation of genes related with cardiometabolic abnormalities (58).
MKRN2 also has E3 ligase activity associated with the RING finger domain, which is bound to p65 and promotes polyubiquitination, proteasome-dependent degradation of p65 via the MKRN2 RING finger domain, which inhibits p65-mediated NF-κB transactivation and inflammatory responses (54). Furthermore, MKRN2 controlled melanoma cell proliferation via interacting with and ubiquitylating p53, implying that MKRN2 might be a therapeutic target for melanoma (59). Previous research found that IGF2BP3 is a novel ubiquitylating substrate for MKRN2 and that MKRN2 reduces central nervous system tumors by regulating CD44 and PDPN in an IGF2BP3-dependent way. As a result, MKRN2 may be a promising therapeutic target for neuroblastoma (60).
MKRN3 Function
The RING finger domain of MKRN3 is thought to be a potential E3 ubiquitin ligase that suppresses Nptx1 expression by polyubiquitination before puberty. Genetic variations in and near the MKRN3 gene have been linked to instances of familial and non-familial central precocious puberty (61–64). As a result, MKRN3 has been found to inhibit trigger protein maturation in juvenile puberty delay. The genetic treatment for accurate MKRN3 compensation may be a promising strategy for central precocious puberty (CPP) therapy.
MKRN4 Function
Because of the lack of functional promoters, integrated, processed pseudogenes have been assumed to be untranscribed and utterly non-functional since their discovery (65). Processed pseudogenes may occasionally gain promoter activity and be transcribed (66, 67). Interestingly, a recent study reported that the novel E3 ligase MKRN4 was identified that induces GLK protein degradation, which suppresses GLK protein overexpression and has a positive SLE function. For the first time, MKRN4 is shown as an E3 ubiquitin ligase instead of a pseudogene (16).
Conclusion and Future Perspectives
mkrn gene family has similar the RING finger domain required for E3 ubiquitin ligases of the RING finger class, despite a different array of zinc-finger motifs. However, as multiply function proteins, MKRNs served as E3 ubiquitin ligase play a leading role in degrading many substrates and regulating several diseases (Figure 3). Thus, in the future, novel therapeutic techniques that target protein-protein interactions and interfere with the binding of MKRNs and their substrates may be developed, and these tactics may create fresh therapeutic options for the treatment of illnesses or pathogens infection.
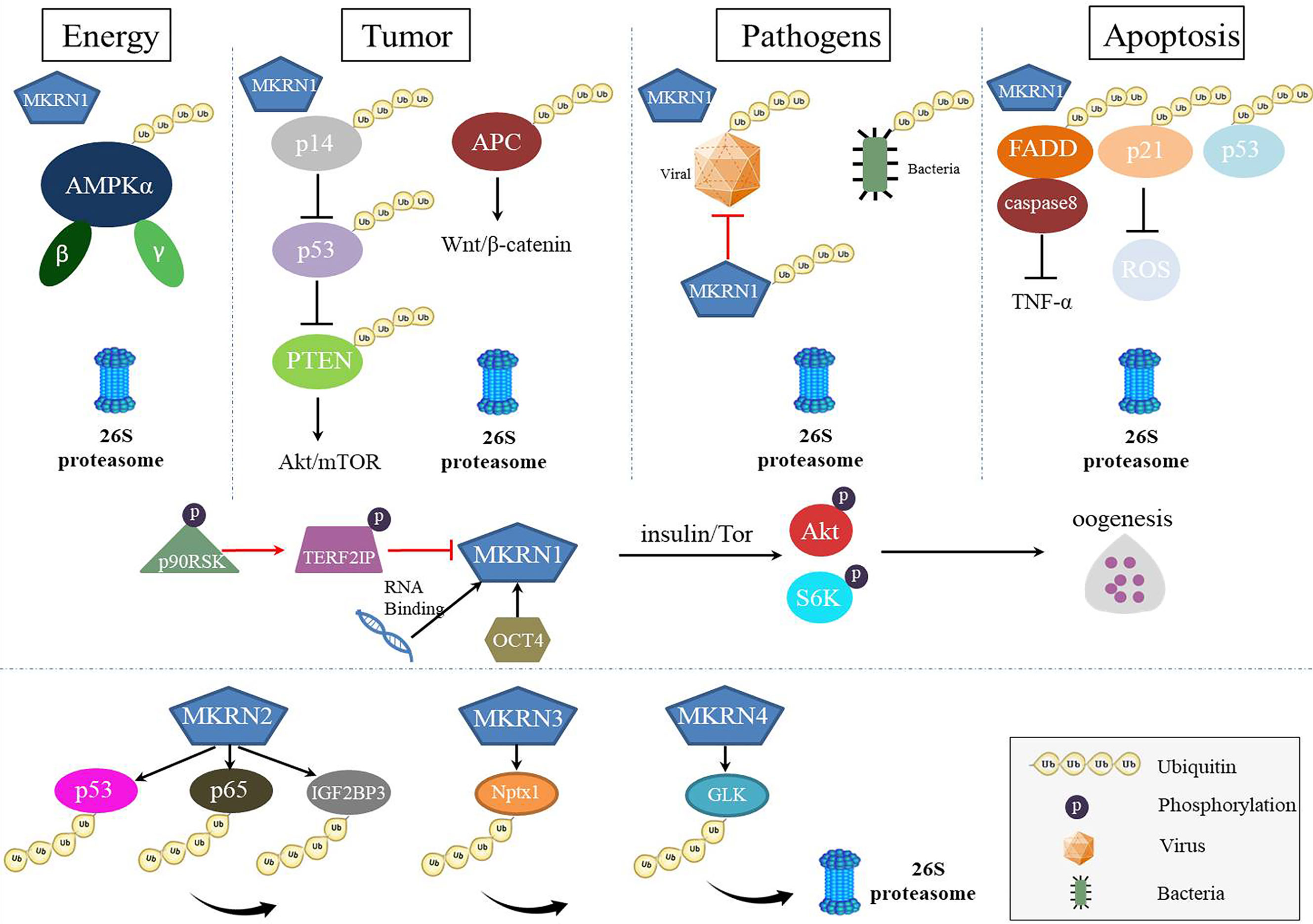
Figure 3 The roles of MKRNs in cells. MKRNs (MKRN1, MKRN2, MKRN3, MKRN4) served as E3 ubiquitin ligase play a leading role in degrading many substrates and regulating several diseases. MKRN1 regulates energy metabolism, tumor suppression, viral protein or bacteria ubiquitination, and apoptosis by degrading their substrates. MKRN1 has RNA-binding functions, up-expressed to regulate the insulin signaling pathway during oogenesis and down-expressed to regulate the senescence-associated secretory phenotype (SASP). MKRN2, MKRN3, and MKRN4 also mediated substrates ubiquitination and degradation.
Author Contributions
TW and LL conceived and wrote the manuscript. WL, CW, MA, and YL revised the manuscript. All authors contributed to the article and approved the submitted version.
Funding
This work was supported by grants from the National Natural Science Foundation of China (32002248) and the Natural Science Foundation of Shandong Province (ZR2020QC016, ZR2020QC017).
Conflict of Interest
The authors declare that the research was conducted in the absence of any commercial or financial relationships that could be construed as a potential conflict of interest.
Publisher’s Note
All claims expressed in this article are solely those of the authors and do not necessarily represent those of their affiliated organizations, or those of the publisher, the editors and the reviewers. Any product that may be evaluated in this article, or claim that may be made by its manufacturer, is not guaranteed or endorsed by the publisher.
References
1. Wadekar HB, Sahi VP, Morita EH, Abe S. MKRN Expression Pattern During Embryonic and Post-Embryonic Organogenesis in Rice (Oryza Sativa L. Var. Nipponbare). Planta (2013) 237:1083–95. doi: 10.1007/s00425-012-1828-2
2. Gray TA, Hernandez L, Carey AH, Schaldach MA, Smithwick MJ, Rus K, et al. The Ancient Source of a Distinct Gene Family Encoding Proteins Featuring RING and C(3)H Zinc-Finger Motifs With Abundant Expression in Developing Brain and Nervous System. Genomics (2000) 66:76–86. doi: 10.1006/geno.2000.6199
3. Jong MT, Gray TA, Ji Y, Glenn CC, Saitoh S, Driscoll DJ, et al. A Novel Imprinted Gene, Encoding a RING Zinc-Finger Protein, and Overlapping Antisense Transcript in the Prader-Willi Syndrome Critical Region. Hum Mol Genet (1999) 8:783–93. doi: 10.1093/hmg/8.5.783
4. Bohne A, Darras A, D’Cotta H, Baroiller JF, Galiana-Arnoux D, Volff JN. The Vertebrate Makorin Ubiquitin Ligase Gene Family has Been Shaped by Large-Scale Duplication and Retroposition From an Ancestral Gonad-Specific, Maternal-Effect Gene. BMC Genomics (2010) 11:721. doi: 10.1186/1471-2164-11-721
5. Dikic I. Proteasomal and Autophagic Degradation Systems. Annu Rev Biochem (2017) 86:193–224. doi: 10.1146/annurev-biochem-061516-044908
6. Pickart CM. Mechanisms Underlying Ubiquitination. Annu Rev Biochem (2001) 70:503–33. doi: 10.1146/annurev.biochem.70.1.503
7. Sommer T, Wolf DH. The Ubiquitin-Proteasome-System. Biochim Biophys Acta (2014) 1843:1. doi: 10.1016/j.bbamcr.2013.09.009
8. Childs KS, Goodbourn S. Identification of Novel Co-Repressor Molecules for Interferon Regulatory Factor-2. Nucleic Acids Res (2003) 31:3016–26. doi: 10.1093/nar/gkg431
9. Deshaies RJ, Joazeiro CA. RING Domain E3 Ubiquitin Ligases. Annu Rev Biochem (2009) 78:399–434. doi: 10.1146/annurev.biochem.78.101807.093809
10. Sahi VP, Wadekar HB, Ravi NS, Arumugam TU, Morita EH, Abe S. A Molecular Insight Into Darwin’s “Plant Brain Hypothesis” Through Expression Pattern Study of the MKRN Gene in Plant Embryo Compared With Mouse Embryo. Plant Signal Behav (2012) 7:375–81. doi: 10.4161/psb.19094
11. Wang T, Du Q, Wu X, Niu Y, Guan L, Wang Z, et al. Porcine MKRN1 Modulates the Replication and Pathogenesis of Porcine Circovirus Type 2 by Inducing Capsid Protein Ubiquitination and Degradation. J Virol (2018) 92:e00100-18. doi: 10.1128/JVI.00100-18
12. Gray TA, Azama K, Whitmore K, Min A, Abe S, Nicholls RD. Phylogenetic Conservation of the Makorin-2 Gene, Encoding a Multiple Zinc-Finger Protein, Antisense to the RAF1 Proto-Oncogene. Genomics (2001) 77:119–26. doi: 10.1006/geno.2001.6627
13. Lee KY, Chan KY, Tsang KS, Chen YC, Kung HF, Ng PC, et al. Ubiquitous Expression of MAKORIN-2 in Normal and Malignant Hematopoietic Cells and Its Growth Promoting Activity. PloS One (2014) 9:e92706. doi: 10.1371/journal.pone.0092706
14. Gray TA, Wilson A, Fortin PJ, Nicholls RD. The Putatively Functional Mkrn1-P1 Pseudogene Is Neither Expressed Nor Imprinted, Nor Does It Regulate Its Source Gene in Trans. Proc Natl Acad Sci U S A (2006) 103:12039–44. doi: 10.1073/pnas.0602216103
15. Rapkins RW, Hore T, Smithwick M, Ager E, Pask AJ, Renfree MB, et al. Recent Assembly of an Imprinted Domain From Non-Imprinted Components. PloS Genet (2006) 2:e182. doi: 10.1371/journal.pgen.0020182
16. Chuang HC, Hung WT, Chen YM, Hsu PM, Yen JH, Lan JL, et al. Genomic Sequencing and Functional Analyses Identify MAP4K3/GLK Germline and Somatic Variants Associated With Systemic Lupus Erythematosus. Ann Rheum Dis (2021) 81:243–54. doi: 10.1136/annrheumdis-2021-221010
17. Tingaud-Sequeira A, Chauvigne F, Lozano J, Agulleiro MJ, Asensio E, Cerda J. New Insights Into Molecular Pathways Associated With Flatfish Ovarian Development and Atresia Revealed by Transcriptional Analysis. BMC Genomics (2009) 10:434. doi: 10.1186/1471-2164-10-434
18. Cassar PA, Carpenedo RL, Samavarchi-Tehrani P, Olsen JB, Park CJ, Chang WY, et al. Integrative Genomics Positions MKRN1 as a Novel Ribonucleoprotein Within the Embryonic Stem Cell Gene Regulatory Network. EMBO Rep (2015) 16:1334–57. doi: 10.15252/embr.201540974
19. Hall TM. Multiple Modes of RNA Recognition by Zinc Finger Proteins. Curr Opin Struct Biol (2005) 15:367–73. doi: 10.1016/j.sbi.2005.04.004
20. Miroci H, Schob C, Kindler S, Olschlager-Schutt J, Fehr S, Jungenitz T, et al. Makorin Ring Zinc Finger Protein 1 (MKRN1), a Novel Poly(A)-Binding Protein-Interacting Protein, Stimulates Translation in Nerve Cells. J Biol Chem (2012) 287:1322–34. doi: 10.1074/jbc.M111.315291
21. Omwancha J, Zhou XF, Chen SY, Baslan T, Fisher CJ, Zheng Z, et al. Makorin RING Finger Protein 1 (MKRN1) Has Negative and Positive Effects on RNA Polymerase II-Dependent Transcription. Endocrine (2006) 29:363–73. doi: 10.1385/ENDO:29:2:363
22. Naule L, Kaiser UB. Evolutionary Conservation of MKRN3 and Other Makorins and Their Roles in Puberty Initiation and Endocrine Functions. Semin Reprod Med (2019) 37:166–73. doi: 10.1055/s-0039-3400965
23. Du Z, Cong H, Yao Z. Identification of Putative Downstream Genes of Oct-4 by Suppression-Subtractive Hybridization. Biochem Biophys Res Commun (2001) 282:701–6. doi: 10.1006/bbrc.2001.4636
24. Hirotsune S, Yoshida N, Chen A, Garrett L, Sugiyama F, Takahashi S, et al. An Expressed Pseudogene Regulates the Messenger-RNA Stability of Its Homologous Coding Gene. Nature (2003) 423:91–6. doi: 10.1038/nature01535
25. Podlaha O, Zhang J. Nonneutral Evolution of the Transcribed Pseudogene Makorin1-P1 in Mice. Mol Biol Evol (2004) 21:2202–9. doi: 10.1093/molbev/msh230
26. Carpenedo RL, Cassar PA, Stanford WL. MKRN1: Uncovering Function by an Unbiased Systems Approach. Cell Cycle (2016) 15:303–4. doi: 10.1080/15384101.2015.1124698
27. Baravalle G, Park H, McSweeney M, Ohmura-Hoshino M, Matsuki Y, Ishido S, et al. Ubiquitination of CD86 Is a Key Mechanism in Regulating Antigen Presentation by Dendritic Cells. J Immunol (2011) 187:2966–73. doi: 10.4049/jimmunol.1101643
28. Geng R, Tan X, Wu J, Pan Z, Yi M, Shi W, et al. RNF183 Promotes Proliferation and Metastasis of Colorectal Cancer Cells via Activation of NF-kappaB-IL-8 Axis. Cell Death Dis (2017) 8:e2994. doi: 10.1038/cddis.2017.400
29. Metzger MB, Hristova VA, Weissman AM. HECT and RING Finger Families of E3 Ubiquitin Ligases at a Glance. J Cell Sci (2012) 125:531–7. doi: 10.1242/jcs.091777
30. Zhang Q, Meng Y, Zhang L, Chen J, Zhu D. RNF13: A Novel RING-Type Ubiquitin Ligase Over-Expressed in Pancreatic Cancer. Cell Res (2009) 19:348–57. doi: 10.1038/cr.2008.285
31. Clague MJ, Urbe S. Ubiquitin: Same Molecule, Different Degradation Pathways. Cell (2010) 143:682–5. doi: 10.1016/j.cell.2010.11.012
32. Hatakeyama S, Nakayama KI. U-Box Proteins as a New Family of Ubiquitin Ligases. Biochem Biophys Res Commun (2003) 302:635–45. doi: 10.1016/S0006-291X(03)00245-6
33. Park YC, Chapagain S, Jang CS. A Negative Regulator in Response to Salinity in Rice: Oryza Sativa Salt-, ABA- and Drought-Induced RING Finger Protein 1 (OsSADR1). Plant Cell Physiol (2018) 59:575–89. doi: 10.1093/pcp/pcy009
34. Cao Y, Zhou H, Chen X, Li Y, Hu J, Zhou G, et al. Recent Insight Into the Role of RING-Finger E3 Ligases in Glioma. Biochem Soc Trans (2021) 49:519–29. doi: 10.1042/BST20201060
35. Li W, Bengtson MH, Ulbrich A, Matsuda A, Reddy VA, Orth A, et al. Genome-Wide and Functional Annotation of Human E3 Ubiquitin Ligases Identifies MULAN, A Mitochondrial E3 That Regulates the Organelle’s Dynamics and Signaling. PloS One (2008) 3:e1487. doi: 10.1371/journal.pone.0001487
36. Lorick KL, Jensen JP, Fang S, Ong AM, Hatakeyama S, Weissman AM. RING Fingers Mediate Ubiquitin-Conjugating Enzyme (E2)-Dependent Ubiquitination. Proc Natl Acad Sci U S A (1999) 96:11364–9. doi: 10.1073/pnas.96.20.11364
37. Zhang Y, Li LF, Munir M, Qiu HJ. RING-Domain E3 Ligase-Mediated Host-Virus Interactions: Orchestrating Immune Responses by the Host and Antagonizing Immune Defense by Viruses. Front Immunol (2018) 9:1083. doi: 10.3389/fimmu.2018.01083
38. Kim JH, Park SM, Kang MR, Oh SY, Lee TH, Muller MT, et al. Ubiquitin Ligase MKRN1 Modulates Telomere Length Homeostasis Through a Proteolysis of hTERT. Genes Dev (2005) 19:776–81. doi: 10.1101/gad.1289405
39. Han H, Chae S, Hwang D, Song J. Attenuating MKRN1 E3 Ligase-Mediated AMPKalpha Suppression Increases Tolerance Against Metabolic Stresses in Mice. Cell Stress (2018) 2:325–8. doi: 10.15698/cst2018.11.164
40. Ko A, Lee EW, Yeh JY, Yang MR, Oh W, Moon JS, et al. MKRN1 Induces Degradation of West Nile Virus Capsid Protein by Functioning as an E3 Ligase. J Virol (2010) 84:426–36. doi: 10.1128/JVI.00725-09
41. Lee EW, Kim JH, Ahn YH, Seo J, Ko A, Jeong M, et al. Ubiquitination and Degradation of the FADD Adaptor Protein Regulate Death Receptor-Mediated Apoptosis and Necroptosis. Nat Commun (2012) 3:978. doi: 10.1038/ncomms1981
42. Lee EW, Lee MS, Camus S, Ghim J, Yang MR, Oh W, et al. Differential Regulation of P53 and P21 by MKRN1 E3 Ligase Controls Cell Cycle Arrest and Apoptosis. EMBO J (2009) 28:2100–13. doi: 10.1038/emboj.2009.164
43. Lee MS, Jeong MH, Lee HW, Han HJ, Ko A, Hewitt SM, et al. PI3K/AKT Activation Induces PTEN Ubiquitination and Destabilization Accelerating Tumourigenesis. Nat Commun (2015) 6:7769. doi: 10.1038/ncomms8769
44. Subrahmanian M, Marimuthu J, Sairam T, Sankaran R. In Vitro Ubiquitination of Mycobacterium Tuberculosis by E3 Ubiquitin Ligase, MKRN1. Biotechnol Lett (2020) 42:1527–34. doi: 10.1007/s10529-020-02873-6
45. Inturi R, Mun K, Singethan K, Schreiner S, Punga T. Human Adenovirus Infection Causes Cellular E3 Ubiquitin Ligase MKRN1 Degradation Involving the Viral Core Protein pVII. J Virol (2018) 92:e01154–17. doi: 10.1128/JVI.01154-17
46. Lee HK, Lee EW, Seo J, Jeong M, Lee SH, Kim SY, et al. Ubiquitylation and Degradation of Adenomatous Polyposis Coli by MKRN1 Enhances Wnt/beta-Catenin Signaling. Oncogene (2018) 37:4273–86. doi: 10.1038/s41388-018-0267-3
47. Chasov V, Mirgayazova R, Zmievskaya E, Khadiullina R, Valiullina A, Stephenson Clarke J, et al. Key Players in the Mutant P53 Team: Small Molecules, Gene Editing, Immunotherapy. Front Oncol (2020) 10:1460. doi: 10.3389/fonc.2020.01460
48. Bai X, Yang H, Pu J, Zhao Y, Jin Y, Yu Q. MKRN1 Ubiquitylates P21 to Protect Against Intermittent Hypoxia-Induced Myocardial Apoptosis. Oxid Med Cell Longev (2021) 2021:9360339. doi: 10.1155/2021/9360339
49. Kotla S, Le NT, Vu HT, Ko KA, Gi YJ, Thomas TN, et al. Endothelial Senescence-Associated Secretory Phenotype (SASP) Is Regulated by Makorin-1 Ubiquitin E3 Ligase. Metabolism (2019) 100:153962. doi: 10.1016/j.metabol.2019.153962
50. Jeong EB, Jeong SS, Cho E, Kim EY. Makorin 1 Is Required for Drosophila Oogenesis by Regulating Insulin/Tor Signaling. PloS One (2019) 14:e0215688. doi: 10.1371/journal.pone.0215688
51. Qian X, Wang L, Zheng B, Shi ZM, Ge X, Jiang CF, et al. Deficiency of Mkrn2 Causes Abnormal Spermiogenesis and Spermiation, and Impairs Male Fertility. Sci Rep (2016) 6:39318. doi: 10.1038/srep39318
52. Cheung WK, Yang PH, Huang QH, Chen Z, Chen SJ, Lin MC, et al. Identification of Protein Domains Required for Makorin-2-Mediated Neurogenesis Inhibition in Xenopus Embryos. Biochem Biophys Res Commun (2010) 394:18–23. doi: 10.1016/j.bbrc.2010.02.041
53. Yang PH, Cheung WK, Peng Y, He ML, Wu GQ, Xie D, et al. Makorin-2 Is a Neurogenesis Inhibitor Downstream of Phosphatidylinositol 3-Kinase/Akt (PI3K/Akt) Signal. J Biol Chem (2008) 283:8486–95. doi: 10.1074/jbc.M704768200
54. Shin C, Ito Y, Ichikawa S, Tokunaga M, Sakata-Sogawa K, Tanaka T. MKRN2 Is a Novel Ubiquitin E3 Ligase for the P65 Subunit of NF-kappaB and Negatively Regulates Inflammatory Responses. Sci Rep (2017) 7:46097. doi: 10.1038/srep46097
55. Jiang J, Xu Y, Ren H, Wudu M, Wang Q, Song X, et al. MKRN2 Inhibits Migration and Invasion of Non-Small-Cell Lung Cancer by Negatively Regulating the PI3K/Akt Pathway. J Exp Clin Cancer Res (2018) 37:189. doi: 10.1186/s13046-018-0855-7
56. Qian YC, Xie YX, Wang CS, Shi ZM, Jiang CF, Tang YY, et al. Mkrn2 Deficiency Induces Teratozoospermia and Male Infertility Through P53/PERP-Mediated Apoptosis in Testis. Asian J Androl (2020) 22:414–21. doi: 10.4103/aja.aja_76_19
57. Jiang X, Chen D. The Identification of Novel Gene Mutations for Degenerative Lumbar Spinal Stenosis Using Whole-Exome Sequencing in a Chinese Cohort. BMC Med Genomics (2021) 14:134. doi: 10.1186/s12920-021-00981-4
58. Ghanbari M, Franco OH, de Looper HW, Hofman A, Erkeland SJ, Dehghan A. Genetic Variations in MicroRNA-Binding Sites Affect MicroRNA-Mediated Regulation of Several Genes Associated With Cardio-Metabolic Phenotypes. Circ Cardiovasc Genet (2015) 8:473–86. doi: 10.1161/CIRCGENETICS.114.000968
59. Zhang Y, Cui N, Zheng G. Ubiquitination of P53 by E3 Ligase MKRN2 Promotes Melanoma Cell Proliferation. Oncol Lett (2020) 19:1975–84. doi: 10.3892/ol.2020.11261
60. Jia C, Tang H, Yang Y, Yuan S, Han T, Fang M, et al. Ubiquitination of IGF2BP3 by E3 Ligase MKRN2 Regulates the Proliferation and Migration of Human Neuroblastoma SHSY5Y Cells. Biochem Biophys Res Commun (2020) 529:43–50s. doi: 10.1016/j.bbrc.2020.05.112
61. Latronico AC, Brito VN, Carel JC. Causes, Diagnosis, and Treatment of Central Precocious Puberty. Lancet Diabetes Endocrinol (2016) 4:265–74. doi: 10.1016/S2213-8587(15)00380-0
62. Liu H, Kong X, Chen F. Mkrn3 Functions as a Novel Ubiquitin E3 Ligase to Inhibit Nptx1 During Puberty Initiation. Oncotarget (2017) 8:85102–9. doi: 10.18632/oncotarget.19347
63. Ortiz-Cabrera NV, Riveiro-Alvarez R, Lopez-Martinez MA, Perez-Segura P, Aragon-Gomez I, Trujillo-Tiebas MJ, et al. Clinical Exome Sequencing Reveals MKRN3 Pathogenic Variants in Familial and Nonfamilial Idiopathic Central Precocious Puberty. Horm Res Paediatr (2017) 87:88–94. doi: 10.1159/000453262
64. Simon D, Ba I, Mekhail N, Ecosse E, Paulsen A, Zenaty D, et al. Mutations in the Maternally Imprinted Gene MKRN3 are Common in Familial Central Precocious Puberty. Eur J Endocrinol (2016) 174:1–8. doi: 10.1530/EJE-15-0488
65. Kaneko S, Aki I, Tsuda K, Mekada K, Moriwaki K, Takahata N, et al. Origin and Evolution of Processed Pseudogenes That Stabilize Functional Makorin1 mRNAs in Mice, Primates and Other Mammals. Genetics (2006) 172:2421–9. doi: 10.1534/genetics.105.052910
66. Harrison PM, Zheng D, Zhang Z, Carriero N, Gerstein M. Transcribed Processed Pseudogenes in the Human Genome: An Intermediate Form of Expressed Retrosequence Lacking Protein-Coding Ability. Nucleic Acids Res (2005) 33:2374–83. doi: 10.1093/nar/gki531
Keywords: makorin RING finger protein (MKRN), disease, infection, regulation, role
Citation: Wang T, Liu W, Wang C, Ma X, Akhtar MF, Li Y and Li L (2022) MRKNs: Gene, Functions, and Role in Disease and Infection. Front. Oncol. 12:862206. doi: 10.3389/fonc.2022.862206
Received: 14 February 2022; Accepted: 17 March 2022;
Published: 08 April 2022.
Edited by:
Jernej Kovac, University Medical Center Ljubljanaju, SloveniaReviewed by:
Emil Bulatov, Kazan Federal University, RussiaRibhav Mishra, Northwestern University, United States
Copyright © 2022 Wang, Liu, Wang, Ma, Akhtar, Li and Li. This is an open-access article distributed under the terms of the Creative Commons Attribution License (CC BY). The use, distribution or reproduction in other forums is permitted, provided the original author(s) and the copyright owner(s) are credited and that the original publication in this journal is cited, in accordance with accepted academic practice. No use, distribution or reproduction is permitted which does not comply with these terms.
*Correspondence: Yubao Li, liyubao@lcu.edu.cn; Liangliang Li, lifeiyang2017@163.com