- 1Radiation Immuno-Oncology Group, Center for Translational Cancer Research (TranslaTUM), School of Medicine, Klinikum Rechts der Isar, Technical University of Munich (TUM), Munich, Germany
- 2Department of Radiation Oncology, School of Medicine, Klinikum Rechts der Isar, Technical University of Munich (TUM), Munich, Germany
As overexpression and membrane localization of stress proteins together with high lactate levels promote radioresistance in tumor cells, we studied the effect of the Hsp90 inhibitor NVP-AUY922 on the cytosolic and membrane expression of heat shock proteins (HSPs) and radiosensitivity in murine melanoma (B16F10) and human colorectal (LS174T) wildtype (WT) and lactate dehydrogenases A/B double knockout (LDH−/−) tumor cells. Double knockout for LDHA/B has been found to reduce cytosolic as well as membrane HSP levels, whereas treatment with NVP-AUY922 stimulates the synthesis of Hsp27 and Hsp70, but does not affect membrane Hsp70 expression. Despite NVP-AUY922-inducing elevated levels of cytosolic HSP, radiosensitivity was significantly increased in WT cells and even more pronounced in LDH−/− cells. An impaired lipid metabolism in LDH−/− cells reduces the Hsp70 membrane-anchoring sphingolipid globotriaosylceramide (Gb3) and thereby results in a decreased Hsp70 cell surface density on tumor cells. Our results demonstrate that the membrane Hsp70 density, but not cytosolic HSP levels determines the radiosensitizing effect of the Hsp90 inhibitor NVP-AUY922 in LDH−/− cells.
Introduction
Many tumor cell types including colorectal carcinoma and melanoma, exhibit an increased synthesis of heat shock proteins (HSPs) such as Hsp90, Hsp70 and Hsp27 which in turn promotes tumor progression, malignant transformation and therapy resistance (1). In recent years, the therapeutic potential of several different HSP-targeting drugs has been tested in preclinical and clinical trials (2). Although, the Hsp90 inhibitor AUY-NVP922 exhibited promising radiosensitizing potential by impairing the DNA damage repair and the cell cycle, not only in different tumor cell entities including lung cancer cells, uterine cervical carcinoma, head and neck squamous cell carcinoma and colorectal carcinoma cells but also in a human head and neck squamous cell carcinoma xenograft model (3–5), its efficacy is limited due to its hepatotoxicity and a compensatory upregulation of the transcription of other HSPs, especially the major stress-inducible, anti-apoptotic Hsp70. As a consequence, combined treatment strategies with inhibitors targeting different HSP families concomitantly are currently under investigation, although clinical data are not yet available (2).
Our laboratory has previously demonstrated that a pharmacological inhibition of the lactate dehydrogenase (LDH) as well as a lactate dehydrogenase A/B (LDHA/B) double knockout (LDH−/−) has the capacity to decrease the expression of Hsp90, Hsp70 and Hsp27 and thereby can increase the radiosensitivity in cancer cells (6). An increased LDH activity causes high lactate concentrations and an acidic tumor microenvironment which further enhances tumor growth (7), suppresses immune cell functions including effector T and NK cells (8–10), correlates with an aggressive tumor phenotype and increases the risk of metastatic spread and tumor recurrence (11).
Compared to normal cells, tumor cells frequently overexpress Hsp70 in the cytosol and present it on their plasma membrane in a tumor-specific manner. A high cell surface density of Hsp70 stabilizes plasma membranes of tumor cells and thereby contributes to cell survival and radioresistance (12–14). Herein, we assessed the mechanism(s) via which an impaired lactate metabolism in combination with an Hsp90 inhibition impacts the stress protein expression and membrane localization of tumor cells in context with their radiosensitivity.
Materials and Methods
Cells and Cell Culture
The wildtype (WT) B16F10 murine melanoma (ATCC® CRL-6475TM; ATCC, Manassas, VA, USA) and LS174T human colorectal adenocarcinoma (ATCC® CL-188™; ATCC, Manassas, VA, USA) cell lines and their LDHA/B double knockout (LDH−/−) counterparts (kindly provided by Marina Kreutz and Jacques Pouyssegur (15) were grown in complete growth medium, consisting of Rosewell Park Memorial Institute (RPMI)-1640 medium (Sigma-Aldrich, St. Louis, MO, USA) or high glucose Dulbecco`s Eagle`s Minimum Essential Medium (DMEM) (Sigma-Aldrich) respectively, supplemented with 10% v/v heat inactivated fetal bovine serum (FBS, Sigma-Aldrich), 1% antibiotics (10,000 IU/mL penicillin, 10 mg/mL streptomycin, Sigma-Aldrich), 2 mM L-glutamine (Sigma-Aldrich) and 1 mM sodium pyruvate (Sigma-Aldrich). Cells were routinely checked and confirmed negative for mycoplasma contamination.
Reagents and Treatment
A stock solution (10 mM) of the Hsp90 inhibitor NVP-AUY922 (Santa Cruz Biotechnology, Dallas, TX, USA) was prepared in dimethyl sulfoxide (DMSO) and further diluted in phosphate buffered saline (PBS). Control cells were incubated with the respective amounts of DMSO. Cells were treated with NVP-AUY922 for 24 h.
Western Blot Analysis
Cells were lysed in Radioimmunoprecipitation Assay (RIPA) buffer containing 50 mM Tris-HCl (pH 8.0), 150 mM NaCl, 1 mM EDTA, 1% v/v Triton-X-100, 0.1% w/v sodium dodecyl sulphate (SDS), 0.5% w/v sodium deoxycholate, protease inhibitor cocktail (Roche, Basel, Switzerland). The protein amount was measured using the Pierce™ BCA Protein Assay Kit (Thermo Fisher Scientific, Waltham, MA, USA). Proteins were separated by SDS-PAGE, transferred on nitrocellulose membranes and detected by immunoblotting with the following primary and secondary antibodies: Hsp27 (NBP2-32972, clone G3.1, Novus Biologicals, Centennial, CO, USA), Hsp70 (clone cmHsp70.1, murine IgG1, multimmune GmbH, Munich, Germany), LDHA (NBP1-48336, rabbit polyclonal, Novus Biologicals), LDHB (NBP2-53421, rabbit polyclonal, Novus Biologicals), AKT (9272S, rabbit, Cell Signaling Technology, Danvers, MA, USA), ß-Actin (A2228, clone AC-74, Sigma-Aldrich), horseradish peroxidase (HRP)-conjugated rabbit anti-mouse immunoglobulins (P0260, Dako-Agilent, Santa Clara, CA, USA) and HRP-conjugated swine anti-rabbit immunoglobulins (P0217, Dako-Agilent). The Pierce™ ECL Western Kit (Thermo Fisher Scientific) was used to detect immune complexes which were then imaged digitally (ChemiDoc™ Touch Imaging System, Bio-Rad, Hercules, CA, USA). The Fiji software (16) was used for quantifying Western Blot signals.
Lactate Dehydrogenase (LDH) Activity Measurement
LDH activity was measured using the Lactate Dehydrogenase Activity kit (Sigma-Aldrich) according to the manufacturer’s protocol.
Cell Counting
Cell count and viability were determined using a Sigma-Aldrich Cell Counting Kit-8 (CCK-8), following the manufacturer’s protocol.
Irradiation
Tumor cells were irradiated with a single dose of 0 Gy (sham), 0.5 Gy, 1 Gy and 2 Gy using the Gulmay RS225A device (Gulmay Medical Ltd., Camberley, UK) at a dose rate of 1.1 Gy/min (15 mA, 200 kV).
Clonogenic Assay
Tumor cells were seeded into 12-well plates and one day later they were treated with 5 nM NVP-AUY922 for 24 h and then irradiated with the indicated doses. After irradiation cells were cultured in fresh, drug-free medium. After 9-10 days colonies were washed with PBS, fixed with ice-cold methanol and stained with 0.1% w/v crystal violet. The number of colonies consisting of ≥ 50 cells were counted automatically by a Bioreader® 3000 (Bio-Sys GmbH, Karben, Deutschland). Survival curves were fitted to the linear quadratic model using SigmaPlot (Systat Software Inc, San Jose, CA, USA).
Analysis of Membrane Hsp70 (mHsp70) Expression by Flow Cytometry
The membrane Hsp70 (mHsp70) phenotype was analyzed by flow cytometry using the FITC-conjugated cmHsp70.1 monoclonal antibody (mAb, IgG1, multimmune GmbH, Munich, Germany) on a FACSCalibur flow cytometer (BD Biosciences, Heidelberg, Germany). Tumor cells (0.2 x 106 cells) were washed with flow cytometry buffer (PBS/10% v/v fetal bovine serum, FBS) and incubated either with the cmHsp70.1 mAb or with an isotype matched FITC-labeled control immunoglobulin (mouse IgG1 FITC, 345815, BD Biosciences) on ice in the dark for 30 min. After a second washing step, viable cells (propidium iodide negative cells) were gated upon, and the proportion of positively stained cells were analyzed.
Statistics
Each experiment was performed independently at least 3 times (biological replicates). Comparative analysis of two or multiple groups was carried out using the Student’s t-test or the Tukey Test respectively (*p ≤ 0.05, **p ≤ 0.01, ***p ≤ 0.001). Data are presented as mean values with standard deviation (SD).
Results
Hsp90 Inhibition by NVP-AUY922 Increases Cytosolic Hsp70 and Hsp27 Expression in B16F10 and LS174T WT and LDH−/− Cells
The radiosensitizing effects of the Hsp90 inhibitor NVP-AUY922 was studied using murine (B16F10) and human (LS174T) wildtype (WT) and CRISPR/Cas9 lactate dehydrogenases A/B (LDH−/−) double knockout tumor cells with an impaired lactate metabolism (6, 15). The Hsp90 inhibitor NVP-AUY922 induced a comparable and concentration-dependent reduction in the viability of WT and LDH−/− tumor cells (B16F10, LS174T; Figures 1A, B). In line with our previous data, a LDHA/B double knockout significantly reduced the cytosolic Hsp70 and Hsp27 expression (Figures 1C, D) (6). Despite significant differences in their basal levels of HSP expression, NVP-AUY922 caused a comparable upregulation of intracellular Hsp70 and Hsp27 in WT and LDH−/− cells above the initial levels of WT cells (Figures 1C, D). Due to a very low Hsp27 expression, Hsp27 levels could not be quantified in B16F10 cells.
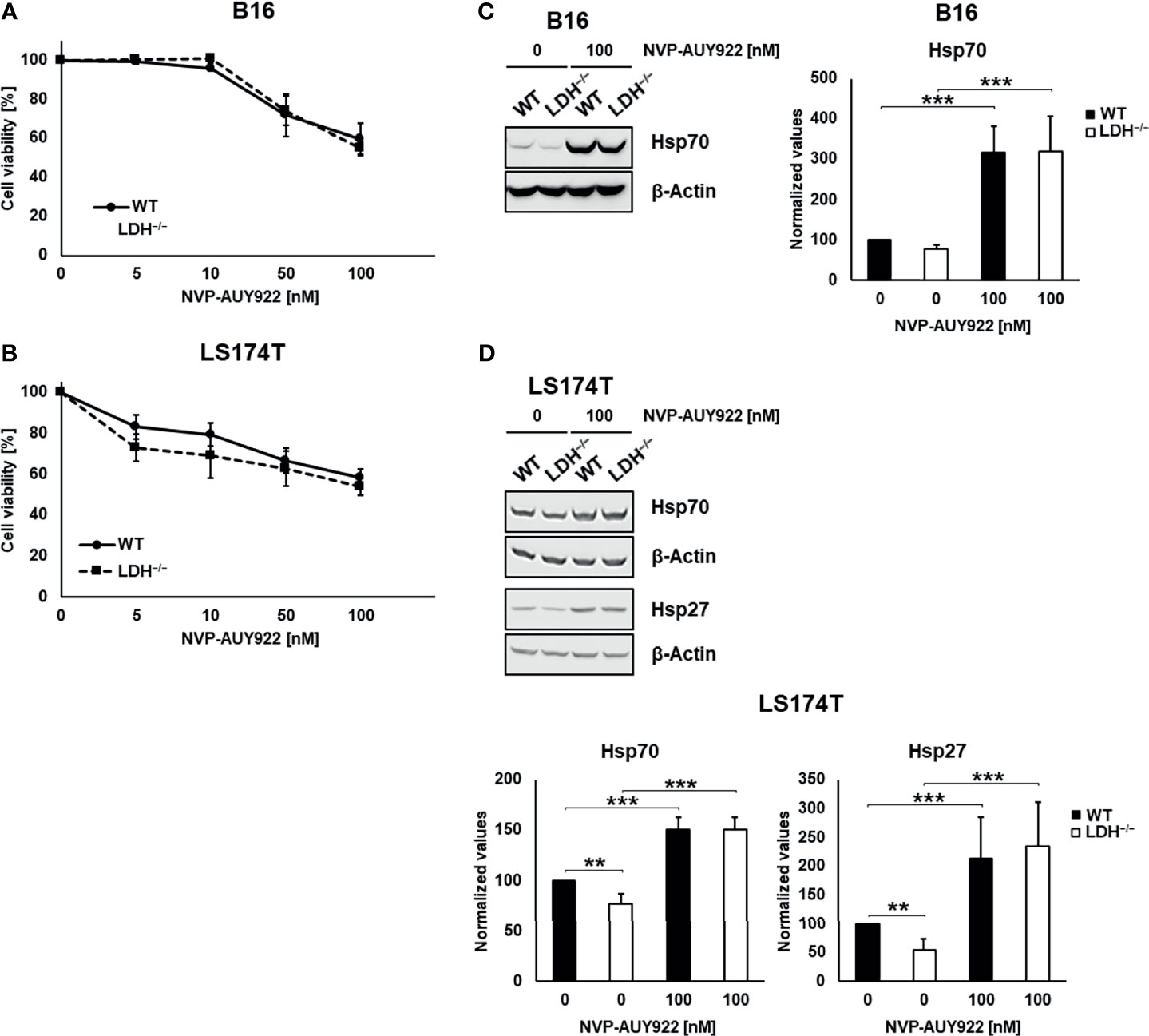
Figure 1 Hsp90 inhibition reduces cell viability in a concentration-dependent manner and increases cytosolic Hsp70 and Hsp27 levels. (A, B) Toxicity assay of B16F10 (A) and LS174T (B) WT and LDH−/− cells treated with NVP-AUY922 (0, 5, 10, 50, 100 nM) for 24 h. (C, D) Representative immunoblot showing intracellular Hsp70, Hsp27 and β-Actin levels in B16F10 (C) and LS174T (D) cells upon treatment with NVP-AUY922 (100 nM) for 24 h. Quantification of the heat shock protein (HSP) levels are shown in the adjacent bar chart (**p ≤ 0.01, ***p ≤ 0.001).
Low Hsp90 Inhibitor Concentration Potentiates Radiosensitivity Especially in LDH−/− Cells
In line with previous data, clonogenic cell survival assays revealed that LS174T WT cells are significantly more radioresistant than LDH−/− cells (Figure 2A) (6). Despite elevated cytosolic HSP levels a low concentration of the Hsp90 inhibitor NVP-AUY922 (5 nM) increased radiosensitivity in WT and LDH−/− cells. This radiosensitizing effect was significantly more pronounced in LDH−/− cells (Figures 2B–D). Since a low concentration of 5 nM NVP-AUY922 completely inhibited clonogenic cell survival of B16F10 cells (Supplementary Figure 1) an additive radiosensitizing effect could not be shown in this cell line.
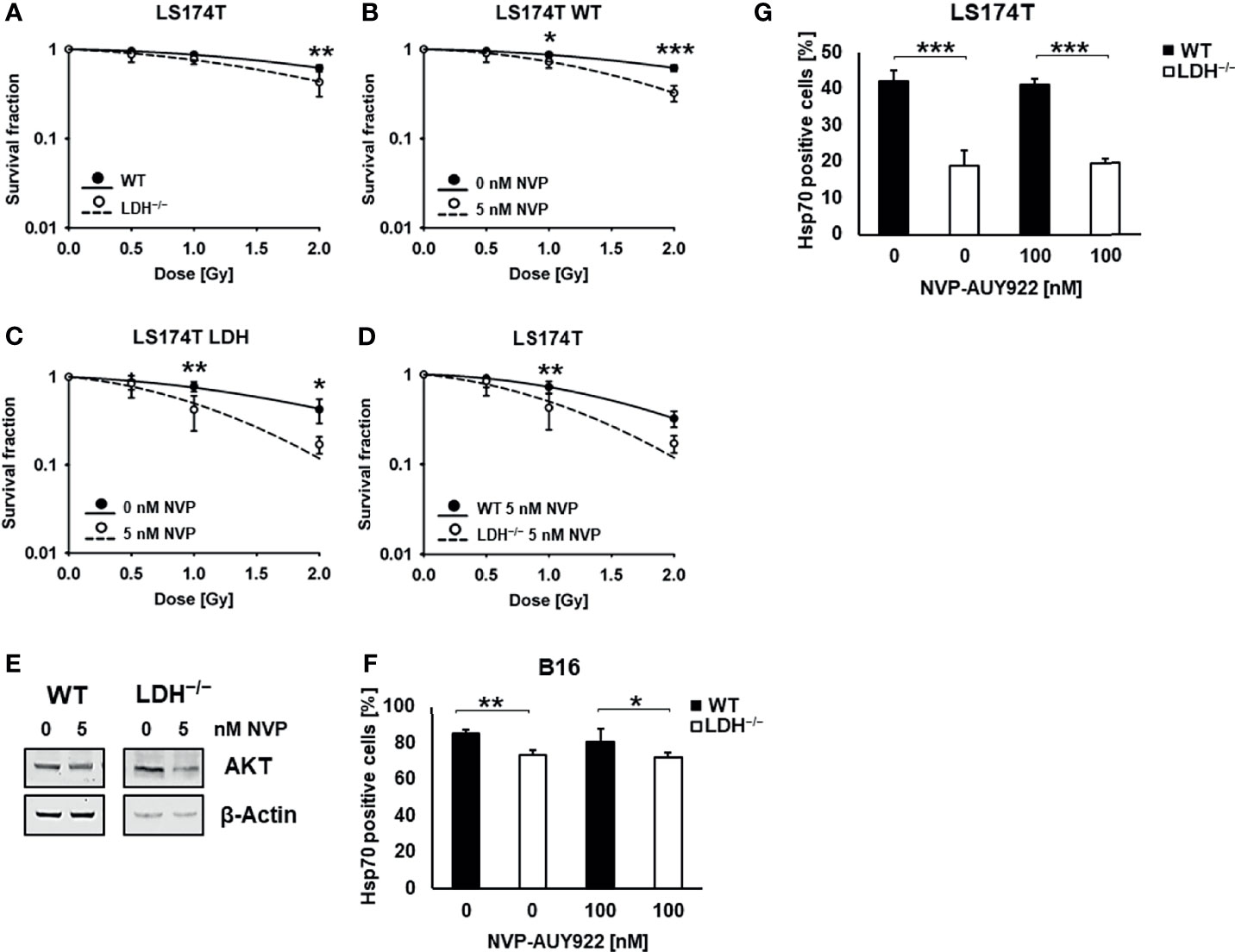
Figure 2 Hsp90 inhibition potentiates radiosensitivity in LS174T cells. (A) Colony forming assay of LS174T WT and LDH−/− cells after irradiation with 0, 0.5, 1 and 2 Gy (**p ≤ 0.01). Colony forming assay of LS174T WT (B) and LDH−/− (C) cells after treatment with a low concentration of NVP-AUY922 (5 nM) for 24 h and irradiation with 0, 0.5, 1 and 2 Gy (*p ≤ 0.05, **p ≤ 0.01, ***p ≤ 0.001). (D) Comparison of WT and LDH−/− cells treated with a low dose of NVP-AUY922 (5 nM) (**p ≤ 0.01). (E) Representative immunoblot showing the expression of AKT and β-Actin in LS174T cells upon treatment with NVP-AUY922 (5 nM) for 24 h. (F, G) Membrane Hsp70 expression on B16F10 (F) and LS174T (G) cells treated with 100 nM NVP-AUY922 for 24 h, as determined by flow cytometry using the cmHsp70.1 mAb. The proportion of positively stained cells is shown (*p ≤ 0.05, **p ≤ 0.01, ***p ≤ 0.001).
As demonstrated in Table 1, the D50 value of NVP-AUY922-treated WT versus LDH−/− cells was 1.54 Gy and 1.0 Gy, respectively, and the sensitizing enhancement ratio (SER) was greater 1.20 (1.58 and 1.79, respectively) in both cell types. A downregulation of the Hsp90 client protein AKT (Figure 2E) confirmed the activity of the Hsp90 inhibitor NVP-AUY922 at a low concentration of 5 nM.
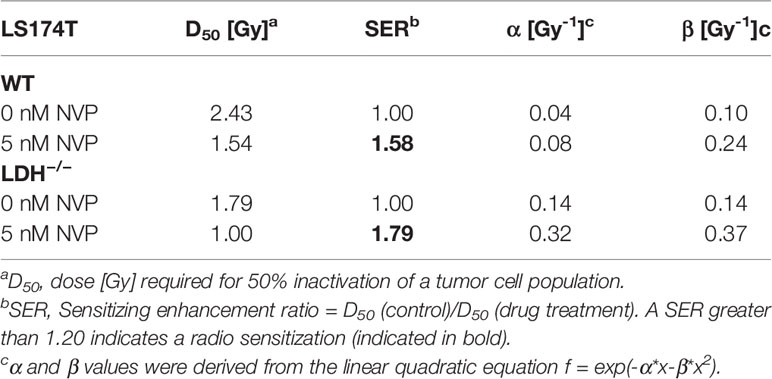
Table 1 Summary of radiobiological parameters depicted in Figures 2A–D.
In contrast to the cytosolic Hsp70 levels, the low membrane Hsp70 expression (6) remained unaltered low by Hsp90 inhibition in LDH−/− B16F10 and LS174T tumor cells (Figures 2F, G).
Discussion
Since many cells of different tumor entities including lung, breast, pancreatic and colorectal overexpress HSPs and are thus more resistant to therapy including radiotherapy, efforts are being made to develop HSP inhibitors (1, 17, 18). Although the synthetic, isoxazole/resorcinol-based Hsp90 inhibitor NVP-AUY922 has shown promising results in tumor cell lines and a human head and neck squamous cell carcinoma xenograft model (3, 5), its hepatotoxicity and a compensatory upregulated expression of anti-apoptotic HSPs limits its broader clinical application. The effectiveness of combination therapies using inhibitors targeting different HSP have therefore recently been investigated, although clinical data are still missing (2, 19). Our previous data demonstrated that combining the Heat Shock Factor 1 (HSF1) knockdown with Hsp90 inhibition using NVP-AUY922 radiosensitizes H1339 human lung cancer cells by impairing the DNA double-strand break repair (20). Furthermore, we have shown recently that targeting the lactate/pyruvate metabolism in cancer cells by a pharmacological or genetic inhibition of LDHA/B results in decreased cytosolic Hsp90, Hsp70 and Hsp27 levels and a reduced membrane Hsp70 expression (6). Therefore, we studied the radiosensitization effect of NVP-AUY922 in B16F10 and LS174T cells having an impaired lactate metabolism, and correlated radiosensitization with the cytosolic expression of different HSPs including Hsp27 and Hsp70 and the membrane Hsp70 positivity. A LDHA/B double knockout diminishes the HSP transcription and thereby reduces the cytosolic amounts of HSF1-regulated Hsp27 and Hsp70 (Figures 1C, D). However, the addition of NVP-AUY922 reversed this beneficial effect and resulted in a significant upregulation of cytosolic Hsp70 and Hsp27 in both, WT and LDH−/− cells, even highly above initial levels (Figures 1C, D).
In contrast to the elevated cytosolic HSP levels, membrane Hsp70 expression remained unaffected by Hsp90 inhibition in both tumor cell types (Figures 2F, G). Tumor cells with an impaired lactate metabolism had a significantly lower membrane Hsp70 expression than WT cells after Hsp90 inhibition. Since the radiosensitizing effect of NVP-AUY922, even at low concentrations, was significantly more pronounced in LDH−/− compared to WT cells (Figures 2B–D), despite the fact that both cell types exhibited comparably high cytosolic HSP levels, we propose that the increased radiosensitivity of LDH−/− cells is associated with a reduced membrane Hsp70 positivity (Figures 2F, G) (6). The localization and anchorage of Hsp70 on the plasma membrane of tumor cells is enabled by a spontaneous interaction of Hsp70 with negatively charged sphingolipids including sulfogalactosyl ceramide (21) or globotriaosylceramide Gb3 (22) which are elevated in tumor cells and reside in cholesterol rich domains also termed lipid rafts (23). Atomic force microscopy studies (24) as well as the formation of ion conductance channels (25, 26) revealed a dimerization/clustering of Hsp70 in artificial lipid membranes which may affect the stability/fluidity of lipid membranes (27–29). Interference with the lactate/pyruvate metabolism results in an altered lipid metabolism (6) which also affects the production of Gb3. A reduction in the amount of the Hsp70-anchoring glycolipid Gb3 causes a significant decrease in the amount of plasma membrane-bound Hsp70 in LDH−/− cells compared to WT cells. It remains to be determined whether an interference of the lactate/pyruvate metabolism also affects the trafficking of cytosolic Hsp70 to the plasma membrane and the release of Hsp70 in exosomes (30) into the extracellular milieu. Transport inhibitor studies revealed that membrane transport and exosomal export of Hsp70 are mediated via a non-classical liposomal but not a classical ER/Golgi pathway (31). Live cell STED nanoscopy has demonstrated that tumor cell-to-tumor cell connections are enabled by tunneling nanotubes that originate form membrane Hsp70 residing in cholesterol rich microdomains (32). It is conceivable that these nanotubes and cell interactions might also be impaired by an interference with the lactate metabolism.
A plasma membrane expression of Hsp70 on tumor cells correlates with the localization of Hsp70 in lysosomal membranes (33). Functionally, Hsp70 not only stabilizes plasma but also lysosomal membranes and thereby mediates resistance to chemical and/or physical-induced membrane permeabilization, such as anticancer drugs or radiation (13, 33, 34). Murakami et al. have demonstrated that not only cytosolic, but also plasma membrane-bound Hsp70 affects radiosensitivity (14). In this study, we demonstrate that the membrane Hsp70 status, not cytosolic Hsp70 levels, regulated by the lactate/pyruvate metabolism, determines the radiosensitizing effect of the Hsp90 inhibitor NVP-AUY922 in tumor cells.
Based on these findings, combining LDH and Hsp90 inhibition might provide a promising strategy to combat radioresistance, however further studies are necessary to identify more potent LDH inhibitors for clinical use with an improved efficacy, higher stability and lower off-target effects (35). The clinically approved, nonsteroidal anti-inflammatory drug (NSAID) diclofenac could be a potential candidate for efficiently inhibiting LDH activity (35–38).
Data Availability Statement
The original contributions presented in the study are included in the article/Supplementary Material. Further inquiries can be directed to the corresponding author.
Author Contributions
Conceptualization, MS and GM. Methodology, MS. Investigation, MS. Writing—original draft preparation, MS. Writing—review and editing, GM. Supervision, GM. Project administration, GM. Funding acquisition, GM. All authors have read and agreed to the published version of the manuscript.
Funding
This research was supported by grants of the DFG (KU3500/2-1, SFB824, STA1520/1-1) and by BMWi (ZF4320104AJ8, ZF4320102CS7).
Conflict of Interest
The authors declare that the research was conducted in the absence of any commercial or financial relationships that could be construed as a potential conflict of interest.
Publisher’s Note
All claims expressed in this article are solely those of the authors and do not necessarily represent those of their affiliated organizations, or those of the publisher, the editors and the reviewers. Any product that may be evaluated in this article, or claim that may be made by its manufacturer, is not guaranteed or endorsed by the publisher.
Acknowledgments
The authors would like to thank Marina Kreutz and Jacques Pouyssegur for providing the B16F10 and LS174T WT and LDH−/− cell lines. The authors also acknowledge that antibody cmHsp70.1 provided by CSO of multimmune GmbH.
Supplementary Material
The Supplementary Material for this article can be found online at: https://www.frontiersin.org/articles/10.3389/fonc.2022.861266/full#supplementary-material
References
1. Ciocca DR, Calderwood SK. Heat Shock Proteins in Cancer: Diagnostic, Prognostic, Predictive, and Treatment Implications. Cell Stress Chaperones (2005) 10:86–103. doi: 10.1379/CSC-99r.1
2. Jego G, Hazoume A, Seigneuric R, Garrido C. Targeting Heat Shock Proteins in Cancer. Cancer Lett (2013) 332:275–85. doi: 10.1016/j.canlet.2010.10.014
3. Schilling D, Bayer C, Li W, Molls M, Vaupel P, Multhoff G. Radiosensitization of Normoxic and Hypoxic H1339 Lung Tumor Cells by Heat Shock Protein 90 Inhibition is Independent of Hypoxia Inducible Factor-1alpha. PloS One (2012) 7:e31110. doi: 10.1371/journal.pone.0031110
4. Stingl L, Stuhmer T, Chatterjee M, Jensen MR, Flentje M, Djuzenova CS. Novel HSP90 Inhibitors, NVP-AUY922 and NVP-BEP800, Radiosensitise Tumour Cells Through Cell-Cycle Impairment, Increased DNA Damage and Repair Protraction. Br J Cancer (2010) 102:1578–91. doi: 10.1038/sj.bjc.6605683
5. Zaidi S, McLaughlin M, Bhide SA, Eccles SA, Workman P, Nutting CM, et al. The HSP90 Inhibitor NVP-AUY922 Radiosensitizes by Abrogation of Homologous Recombination Resulting in Mitotic Entry With Unresolved DNA Damage. PloS One (2012) 7:e35436. doi: 10.1371/journal.pone.0035436
6. Schwab M, Thunborg K, Azimzadeh O, von Toerne C, Werner C, Shevtsov M, et al. Targeting Cancer Metabolism Breaks Radioresistance by Impairing the Stress Response. Cancers (Basel) (2021) 13. doi: 10.3390/cancers13153762
7. Hirschhaeuser F, Sattler UG, Mueller-Klieser W. Lactate: A Metabolic Key Player in Cancer. Cancer Res (2011) 71:6921–5. doi: 10.1158/0008-5472.CAN-11-1457
8. Bohn T, Rapp S, Luther N, Klein M, Bruehl TJ, Kojima N, et al. Tumor Immunoevasion via Acidosis-Dependent Induction of Regulatory Tumor-Associated Macrophages. Nat Immunol (2018) 19:1319–29. doi: 10.1038/s41590-018-0226-8
9. Brand A, Singer K, Koehl GE, Kolitzus M, Schoenhammer G, Thiel A, et al. LDHA-Associated Lactic Acid Production Blunts Tumor Immunosurveillance by T and NK Cells. Cell Metab (2016) 24:657–71. doi: 10.1016/j.cmet.2016.08.011
10. Siska PJ, Singer K, Evert K, Renner K, Kreutz M. The Immunological Warburg Effect: Can a Metabolic-Tumor-Stroma Score (MeTS) Guide Cancer Immunotherapy? Immunol Rev (2020) 295:187–202. doi: 10.1111/imr.12846
11. Walenta S, Wetterling M, Lehrke M, Schwickert G, Sundfor K, Rofstad EK, et al. High Lactate Levels Predict Likelihood of Metastases, Tumor Recurrence, and Restricted Patient Survival in Human Cervical Cancers. Cancer Res (2000) 60:916–21.
12. Multhoff G, Botzler C, Wiesnet M, Muller E, Meier T, Wilmanns W, et al. A Stress-Inducible 72-kDa Heat-Shock Protein (HSP72) is Expressed on the Surface of Human Tumor Cells, But Not on Normal Cells. Int J Cancer (1995) 61:272–9. doi: 10.1002/ijc.2910610222
13. Multhoff G, Pockley AG, Schmid TE, Schilling D. The Role of Heat Shock Protein 70 (Hsp70) in Radiation-Induced Immunomodulation. Cancer Lett (2015) 368:179–84. doi: 10.1016/j.canlet.2015.02.013
14. Murakami N, Kuhnel A, Schmid TE, Ilicic K, Stangl S, Braun IS, et al. Role of Membrane Hsp70 in Radiation Sensitivity of Tumor Cells. Radiat Oncol (2015) 10:149. doi: 10.1186/s13014-015-0461-1
15. Zdralevic M, Brand A, Di Ianni L, Dettmer K, Reinders J, Singer K, et al. Double Genetic Disruption of Lactate Dehydrogenases A and B Is Required to Ablate the "Warburg Effect" Restricting Tumor Growth to Oxidative Metabolism. J Biol Chem (2018) 293:15947–61. doi: 10.1074/jbc.RA118.004180
16. Schindelin J, Arganda-Carreras I, Frise E, Kaynig V, Longair M, Pietzsch T, et al. Fiji: An Open-Source Platform for Biological-Image Analysis. Nat Methods (2012) 9:676–82. doi: 10.1038/nmeth.2019
17. Soo ET, Yip GW, Lwin ZM, Kumar SD, Bay BH. Heat Shock Proteins as Novel Therapeutic Targets in Cancer. In Vivo (2008) 22:311–5.
18. Wu J, Liu T, Rios Z, Mei Q, Lin X, Cao S. Heat Shock Proteins and Cancer. Trends Pharmacol Sci (2017) 38:226–56. doi: 10.1016/j.tips.2016.11.009
19. Schilling D, Kuhnel A, Konrad S, Tetzlaff F, Bayer C, Yaglom J, et al. Sensitizing Tumor Cells to Radiation by Targeting the Heat Shock Response. Cancer Lett (2015) 360:294–301. doi: 10.1016/j.canlet.2015.02.033
20. Kuhnel A, Schilling D, Combs SE, Haller B, Schwab M, Multhoff G. Radiosensitization of HSF-1 Knockdown Lung Cancer Cells by Low Concentrations of Hsp90 Inhibitor NVP-Auy922. Cells (2019) 8. doi: 10.3390/cells8101166
21. Mamelak D, Mylvaganam M, Whetstone H, Hartmann E, Lennarz W, Wyrick PB, et al. Hsp70s Contain a Specific Sulfogalactolipid Binding Site. Differential Aglycone Influence on Sulfogalactosyl Ceramide Binding by Recombinant Prokaryotic and Eukaryotic Hsp70 Family Members. Biochemistry (2001) 40:3572–82. doi: 10.1021/bi001643u
22. Gehrmann M, Liebisch G, Schmitz G, Anderson R, Steinem C, De Maio A, et al. Tumor-Specific Hsp70 Plasma Membrane Localization is Enabled by the Glycosphingolipid Gb3. PloS One (2008) 3:e1925. doi: 10.1371/journal.pone.0001925
23. Horvath I, Multhoff G, Sonnleitner A, Vigh L. Membrane-Associated Stress Proteins: More Than Simply Chaperones. Biochim Biophys Acta (2008) 1778:1653–64. doi: 10.1016/j.bbamem.2008.02.012
24. Lamprecht C, Gehrmann M, Madl J, Romer W, Multhoff G, Ebner A. Molecular AFM Imaging of Hsp70-1A Association With Dipalmitoyl Phosphatidylserine Reveals Membrane Blebbing in the Presence of Cholesterol. Cell Stress Chaperones (2018) 23:673–83. doi: 10.1007/s12192-018-0879-0
25. Arispe N, De Maio A. ATP and ADP Modulate a Cation Channel Formed by Hsc70 in Acidic Phospholipid Membranes. J Biol Chem (2000) 275:30839–43. doi: 10.1074/jbc.M005226200
26. Macazo FC, White RJ. Monitoring Charge Flux to Quantify Unusual Ligand-Induced Ion Channel Activity for Use in Biological Nanopore-Based Sensors. Anal Chem (2014) 86:5519–25. doi: 10.1021/ac500832a
27. Balogi Z, Multhoff G, Jensen TK, Lloyd-Evans E, Yamashima T, Jaattela M, et al. Hsp70 Interactions With Membrane Lipids Regulate Cellular Functions in Health and Disease. Prog Lipid Res (2019) 74:18–30. doi: 10.1016/j.plipres.2019.01.004
28. Csoboz B, Balogh GE, Kusz E, Gombos I, Peter M, Crul T, et al. Membrane Fluidity Matters: Hyperthermia From the Aspects of Lipids and Membranes. Int J Hyperthermia (2013) 29:491–9. doi: 10.3109/02656736.2013.808765
29. Torok Z, Horvath I, Goloubinoff P, Kovacs E, Glatz A, Balogh G, et al. Evidence for a Lipochaperonin: Association of Active Protein-Folding GroESL Oligomers With Lipids can Stabilize Membranes Under Heat Shock Conditions. Proc Natl Acad Sci USA (1997) 94:2192–7. doi: 10.1073/pnas.94.6.2192
30. Gastpar R, Gehrmann M, Bausero MA, Asea A, Gross C, Schroeder JA, et al. Heat Shock Protein 70 Surface-Positive Tumor Exosomes Stimulate Migratory and Cytolytic Activity of Natural Killer Cells. Cancer Res (2005) 65:5238–47. doi: 10.1158/0008-5472.CAN-04-3804
31. Bausero MA, Gastpar R, Multhoff G, Asea A. Alternative Mechanism by Which IFN-Gamma Enhances Tumor Recognition: Active Release of Heat Shock Protein 72. J Immunol (2005) 175:2900–12. doi: 10.4049/jimmunol.175.5.2900
32. Reindl J, Shevtsov M, Dollinger G, Stangl S, Multhoff G. Membrane Hsp70-Supported Cell-to-Cell Connections via Tunneling Nanotubes Revealed by Live-Cell STED Nanoscopy. Cell Stress Chaperones (2019) 24:213–21. doi: 10.1007/s12192-018-00958-w
33. Nylandsted J, Gyrd-Hansen M, Danielewicz A, Fehrenbacher N, Lademann U, Hoyer-Hansen M, et al. Heat Shock Protein 70 Promotes Cell Survival by Inhibiting Lysosomal Membrane Permeabilization. J Exp Med (2004) 200:425–35. doi: 10.1084/jem.20040531
34. Kirkegaard T, Roth AG, Petersen NH, Mahalka AK, Olsen OD, Moilanen I, et al. Hsp70 Stabilizes Lysosomes and Reverts Niemann-Pick Disease-Associated Lysosomal Pathology. Nature (2010) 463:549–53. doi: 10.1038/nature08710
35. Feichtinger RG, Lang R. Targeting L-Lactate Metabolism to Overcome Resistance to Immune Therapy of Melanoma and Other Tumor Entities. J Oncol (2019) 2019:2084195. doi: 10.1155/2019/2084195
36. Chirasani SR, Leukel P, Gottfried E, Hochrein J, Stadler K, Neumann B, et al. Diclofenac Inhibits Lactate Formation and Efficiently Counteracts Local Immune Suppression in a Murine Glioma Model. Int J Cancer (2013) 132:843–53. doi: 10.1002/ijc.27712
37. Gottfried E, Lang SA, Renner K, Bosserhoff A, Gronwald W, Rehli M, et al. New Aspects of an Old Drug–Diclofenac Targets MYC and Glucose Metabolism in Tumor Cells. PloS One (2013) 8:e66987. doi: 10.1371/journal.pone.0066987
Keywords: LDHA/B double knockout, stress response, membrane Hsp70, radiosensitization, Hsp90 inhibitor NVP-AUY922
Citation: Schwab M and Multhoff G (2022) A Low Membrane Hsp70 Expression in Tumor Cells With Impaired Lactate Metabolism Mediates Radiosensitization by NVP-AUY922. Front. Oncol. 12:861266. doi: 10.3389/fonc.2022.861266
Received: 24 January 2022; Accepted: 15 March 2022;
Published: 07 April 2022.
Edited by:
Ira Ida Skvortsova, Innsbruck Medical University, AustriaReviewed by:
Yanmei Zou, Huazhong University of Science and Technology, ChinaKang-Seo Park, University of Ulsan, South Korea
Copyright © 2022 Schwab and Multhoff. This is an open-access article distributed under the terms of the Creative Commons Attribution License (CC BY). The use, distribution or reproduction in other forums is permitted, provided the original author(s) and the copyright owner(s) are credited and that the original publication in this journal is cited, in accordance with accepted academic practice. No use, distribution or reproduction is permitted which does not comply with these terms.
*Correspondence: Melissa Schwab, bWVsaXNzYS5zY2h3YWJAdHVtLmRl