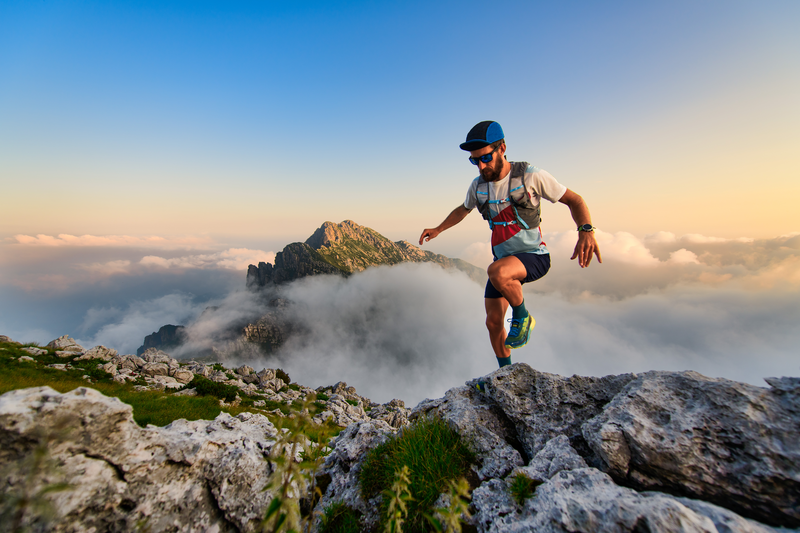
95% of researchers rate our articles as excellent or good
Learn more about the work of our research integrity team to safeguard the quality of each article we publish.
Find out more
MINI REVIEW article
Front. Oncol. , 02 March 2022
Sec. Neuro-Oncology and Neurosurgical Oncology
Volume 12 - 2022 | https://doi.org/10.3389/fonc.2022.855587
This article is part of the Research Topic Investigating the Gliomas/White Matter Interplay and its Implications for Multidisciplinary Treatment: State of Art and Future Perspectives View all 6 articles
For many decades, interactions between diffuse lower-grade glioma (LGG) and brain connectome were neglected. However, the neoplasm progression is intimately linked to its environment, especially the white matter (WM) tracts and their myelin status. First, while the etiopathogenesis of LGG is unclear, this tumor seems to appear during the adolescence, and it is mostly located within anterior and associative cerebral areas. Because these structures correspond to those which were myelinated later in the brain maturation process, WM myelination could play a role in the development of LGG. Second, WM fibers and the myelin characteristics also participate in LGG diffusion, since glioma cells migrate along the subcortical pathways, especially when exhibiting a demyelinated phenotype, which may result in a large invasion of the parenchyma. Third, such a migratory pattern can induce functional (neurological, cognitive and behavioral) disturbances, because myelinated WM tracts represent the main limitation of neuroplastic potential. These parameters are critical for tailoring an individualized therapeutic strategy, both (i) regarding the timing of active treatment(s) which must be proposed earlier, before a too wide glioma infiltration along the WM bundles, (ii) and regarding the anatomic extent of surgical resection and irradiation, which should take account of the subcortical connectivity. Therefore, the new science of connectomics must be integrated in LGG management, based upon an improved understanding of the interplay across glioma dissemination within WM and reactional neural networks reconfiguration, in order to optimize long-term oncological and functional outcomes. To this end, mechanisms of activity-dependent myelin plasticity should be better investigated.
For many decades, oncological considerations prevailed in the investigation and treatment of brain tumors, whereas the central nervous system per se received less attention. However, cerebral neoplasms and their environment, i.e., neural networks, are intimately linked, especially in diffuse tumors such as lower-grade gliomas (LGG) (1–3). Understanding these interdependencies is critical. In neurosciences, recent advances in the field of connectomics have emphasized the pivotal role of the white matter (WM) tracts in cognition and behavior (4, 5). Therefore, the dynamic interplay between WM fibers and LGG should be more systematically explored in order to better predict both the tumor progression and the reactional brain circuitry reconfiguration, with the ultimate aim of tailoring an optimal multistep treatment strategy for each patient.
Here, the goal is to review the implications of constant interactions between WM tracts, with special attention to their myelin status, and LGG concerning (i) the origins of this tumor (ii) the patterns of dissemination of LGG within the cerebral parenchyma (iii) the functional consequences of glioma infiltration (iv) the personalized management to be continuously adapted accordingly. To this end, only diffuse LGG have been studied, by excluding circumscribed gliomas such as pilocytic astrocytoma, pleomorphic xanthoastrocytoma or ganglioglioma.
Although the causative factors of LGG are still poorly known, some data about their temporal and spatial origins have been reported (6). Regarding its temporal origin, this tumor seems to appear during the adolescence (7). Since the LGG velocity of growth is linear during the initial stage of the disease (8, 9), it was possible to extrapolate backward in time using computational models (especially in incidental LGG) and to estimate glioma date of birth in teenage-hood/early adulthood (7, 10). Concerning its brain spatial distribution, LGG is mostly localized within anterior cerebral regions (11). Thanks to a method of graph-based spatial position mapping (12), a probabilistic atlas of LGG locations revealed a preferential distribution within frontal (33%), insular (37%) and temporal (18%) areas, with very few LGG involving posterior structures (13) – less than 2% of occipital LGG (14–16).
Interestingly, a parallel can be made with the spatiotemporal pattern of myelination, which is a dynamic process in the developing brain and which represents an excellent marker of cerebral maturation (17). Advances in MRI, particularly in diffusion tensor imaging (DTI), showed that WM myelination occurs during ontogeny in highly orderly and predictable patterns (18–20). Regarding its time course, whereas this process is faster during the first decade of life, WM continues to mature during adolescence (21). Concerning its spatial distribution, myelination varies across cerebral regions, with a progression from the posterior to the anterior parts of the brain (22, 23). Especially, myelination occurs earlier in sensory pathways (somatosensory, vision) (24), whereas an increase in the degree of myelination is detectable in the frontal subcortical WM in the late phase of development (25). In addition to this caudo-rostral gradient, myelination occurs earlier in projection tracts than in associative fibers, showing that more complex cerebral structures required for the highest level integrative and executive functions are myelinated later than less complex areas underlying basic neurological functions (26). However, although biologically expensive hubs of the brain connectome were less myelinated than primary cortical areas at 14 years, association areas had faster rates of myelination over the course of adolescence (27). Remarkably, incompletely myelinated axons during teenage-hood and even during young adulthood (28), resulting in variations in conduction velocities within neural circuits, might participate in network-level neuroplasticity through activity-dependent myelination in response to environmental stimuli (29, 30).
Therefore, one could hypothesize that WM maturation process might play a role in the genesis of LGG. Indeed, myelination pattern shows spatiotemporal similarities with the natural history of LGG, i.e., occurring during adolescence, with a predominance of tumor location in regions which have been myelinated later - while LGG rarely involve the sensory areas myelinated earlier. This is in agreement with the retrogenesis hypothesis, based upon changes in WM properties in developing and aging brain, which postulates that late maturating tissue, especially late myelinated axons constituting the “top of the pyramid”, are more vulnerable to decline over the lifespan (31). Furthermore, myelin structural and functional adaptive changes induced by neuronal activity (32), especially in regions involved in higher brain functions such as the prefrontal cortex (33), are underpinned by molecular mechanisms which include modifications in oligodendrocyte precursor cells (OPC) proliferation (34). Importantly, neural regulation of brain development and cancer seem to share similar mechanisms (35). Taken into account the robust mitogenic effect of this neuronal activity on OPC lineage, dysregulation of activity-dependent proliferation signals might contribute to the initiation or growth of brain tumors that molecularly resemble OPCs (36). In reciprocity to this neuronal-activity induced proliferation of tumoral cells (37), gliomas themselves can increase the excitability of the surrounding neural circuits (38). These bidirectional mechanisms of neuron-glial interactions could participate in activity-regulated myelin plasticity (39). This might explain why LGG incidence is elevated in association regions with a high functional connectivity (40). These neural hubs which seem more vulnerable to LGG correspond to brain areas populated with presumed cells of origin for gliomas, especially OPCs, as evidenced by a recent probabilistic map (41). This atlas also showed that gliomas predominantly involved cerebral regions enriched with expression of genes associated with chromatin organization and synaptic signalling, making a link between genetic, cellular and connectomic levels (41). Correlations between molecular profile and glioma location have also been evidenced in oligodendroglial tumors, suggesting that subtypes of oligodendrogliomas may derive from site-specific precursors (42). Furthermore, in a genome-wide association study of diffusion MRI data aiming of exploring genetic variation influencing WM microstructure, among the 25 reported genetic risk regions of glioma, 11 were also correlated with WM microstructure: these findings support the close genetic relationship between glioma and WM integrity (43).
To conclude, parallel mechanisms in both normal myelin plasticity and in glioma have been evidenced, such as the parallel importance of the PI3K/AKT/mTOR pathway [for a review, see (39)]. The implication of these ultrastructural mechanisms in neural networks compensation before and after surgery of LGG have to be explored more, especially by investigating their actual role in the redistribution of the functional connectivity already demonstrated at a macroscopical level (3).
Since the seminal works by Scherer in 1938 (44), glioma cells are known to migrate along the WM fibers, even at early stages of disease (45, 46). DTI studies evidenced an anisotropic dissemination of LGG with a tropism for main subcortical bundles, such as the pyramidal tract (47), uncinate fasciculus (UF), inferior fronto-occipital fasciculus (IFOF), arcuate fasciculus (AF) (48–50), or the corpus callosum, leading to bilateral invasion (51). Such patterns of tumor diffusion within the fibers tracts resulted on the proposal of new classification systems to distinguish various LGG according to their WM invasiveness, for example for insular/paralimbic gliomas (52). Determination of tumor migration fingerprint within the connectome is essential for adapting a personalized therapeutic strategy, especially for surgical planning [(53), see below].
Nevertheless, mechanisms underpinning glioma invasion are still unclear, even though the need to better understand intercommunications across tumoral and neuronal cells is now emphasized to explain the spatial anisotropy of diffusion (54, 55). Upregulation of genes involved in cell motility might facilitate the spread of both LGG and high-grade gliomas along WM tracts and might contribute to their invasive phenotype (56). Interestingly, the myelin status seems to play a pivotal role in this tumoral dissemination. Indeed, glioma cells migrated along the outer surface of myelin sheaths and/or along neuronal axons inside myelin sheaths (56). Molecules at the level of this myelin sheath may inhibit glioma cell migration and proliferation (46, 57). WM is a pro-differentiative niche for glioblastomas, since glioma cells in contact with WM can acquire pre-oligodendrocyte fate, leading to a decreased proliferation and invasion (58). However, the neoplasm itself may damage WM, especially by secreting mettaloproteinases able to overcome the inhibitory effect of myelin and to create suitable conditions for tumor cell invasion (59). Moreover, Notch pathway activation could represent an important driving force by which glioma cells migrate within WM tracts (46). These mechanisms may explain why glioma cells are mainly distributed along WM fibers, particularly which exhibit a demyelineated phenotype: glioma cells could be more likely to migrate along the surface of unmyelinated axons or to enter axons for invasion via unmyelinated regions, i.e., when the myelin sheath was damaged by the neoplasm (46). Indeed, extensive demyelination changes are frequent in WM tracts invaded by glioma, as confirmed by DTI (49, 50).
In summary, it seems that myelin constitutes a protection against glioma cells migration, but that its destruction results in fragility sites facilitating tumor invasiveness. This hypothesis is in accordance with the preferential spatial distribution of LGG previously discussed, namely, in brain locations which were myelinated later.
Even though LGG frequently involve highly connected functional hubs, patients usually exhibit no or only mild neurological disturbances at diagnosis, due to mechanisms of neuroplasticity progressively induced by this slow-growing neoplasm (2, 60). A recent meta-networking theory of brain functioning revealed a dynamic organization of the central nervous system, with perpetual succession of new equilibrium states relying on constant changes within and between neural networks, and allowing behavioral adaption to the environment as well as reactional reshaping after brain lesion (61). This flexible model breaking with the rigid localizationist dogma explains how functional compensation is possible despite large tumoral infiltration of cerebral areas traditionally conceived as “eloquent” (3). Nonetheless, the neuroplastic potential is not infinite: the WM connectivity represents a major limitation of network reconfiguration, as evidenced by atlases of cortico-subcortical circuits critical for brain functions identified by intraoperative electrostimulation in LGG patients who underwent awake surgery (5, 62, 63). While anatomo-functional variability and plasticity are high at the cortical level, they are very low at the level of the WM tracts (64).
For many years, WM was conceived as electrical wires allowing a simple conduction of information: in fact, a complex transport system with active computational properties has recently been acknowledged (65, 66). Such a neural computing dynamically performed by the WM fibers themselves is highly depending on the myelination status, since myelin around axons facilitates saltatory neurotransmission and affects velocity of action potentials (67). Importantly, myelin remodeling is a continuous process throughout the lifespan (68), which depends on experience (69), i.e., on the acquisition of complex behaviors (70). For instance, learning piano playing (71) or juggling (72) is correlated to the enhancement of WM microstructure in networks underlying the new skill. Activity-dependent myelin regulation can be considered as an additional form of neural plasticity, able to modulate spike time arrival and coordinate neural circuit oscillations (29, 30, 33, 73). Thus, dysregulation of myelin plasticity can have a negative influence on neural processing by disrupting signal integration, propagation and synchronization within and across networks (39). This alteration in neural coherence may have direct clinical impact in brain-damaged patients, e.g., changes in myelin status in patients with multiple sclerosis were correlated with walking difficulty (74).
Therefore, LGG migration within WM fibers can generate functional consequences, partly due to a loss of activity-regulated myelination. When the plastic compensatory capacity is overwhelmed because of glioma-induced WM damage, epileptic activity may occur, which usually leads to the diagnosis of LGG (75). Even though these patients are frequently enjoying an active life, if an extensive neuropsychological examination is performed before any treatment, over 55% of them already experience cognitive impairments (76). By calculating the degree of disconnection of each associative pathway (based upon the degree of LGG infiltration) using voxel-based and tractwise lesion-symptom analyses (77), significant relationships were found between WM tracts invasion and performance decrease in specific domains related to the function subserved by the network invaded. Typically, LGG patients with involvement of the left IFOF may exhibit a decline of semantic fluency at diagnosis (78) (Figure 1A). Using each patients’ DTI, reduced fractional anisotropy values in the right superior longitudinal fasciculus (SLF) affected by the glioma were associated to visuospatial impairments (82).
Figure 1 Correlations between the degree of infiltration of WM tracts by the LGG and cognitive deteriorations: (A) Preoperative voxel-based lesion symptom map for semantic fluency, evidencing significant relationship between the inferior fronto-occipital fasciculus infiltrated voxels by LGG and deficit of language semantics [from (78) with permission]; (B) Voxel-based lesion symptom of postsurgical lasting anomia performed on residual infiltration map, showing correlations between postoperative lexical retrieval troubles and LGG invasion of the left inferior longitudinal fasciculus [from (79) with permission]; (C) Significant relationship between postoperative residual tumor volume in the arcuate fasciculus and decreased low-level mentalizing accuracy [from (80) with permission]; (D) Disconnectome analysis demonstrating a significant link between postoperative persistent deficit of executive functions and the residual LGG infiltration volume in the superior longitudinal fasciculus [from (81) with permission]. ***Statistically significant.
Tractwise and disconnectome-behavior analyses were also performed after LGG surgery in order to correlate postoperative neurocognitive scores to the residual tumor infiltration within the WM fibers - voluntary left for preventing severe long-lasting deficit thanks to a connectome-based resection in awake patients (83). Lexical retrieval impairments were predicted by postsurgical residual lesion volume in the left inferior longitudinal fasciculus (ILF) (79). A deterioration of theory of mind (i.e., low-level face-based mentalizing or empathy) was linked to the degree of disconnection by the residual tumor in the right AF (80, 84), whereas high-level mentalizing capacity (i.e., the ability to infer the intention of other’s) was linked to the residual infiltration in the cingulate fasciculus (80). Some degree of postoperative anosognosia was associated with remaining tumor infiltration in the right cingulate bundle (85). Postsurgical worsening of executive functions was linked to residual glioma invasion within the frontoparietal connectivity, especially with significant correlations between decline of mental flexibility and involvement of the layer II of the left SLF (81) (Figures 1B–D).
To sum up, despite some potential of WM plasticity, axonal and myelin-induced injury due to glioma migration may result in seizure and performance decline in LGG patients, which should be objectively assessed by a neuropsychological evaluation before to treat, and which represents a valuable parameter in order to predict the risk of persistent cognitive worsening, particularly following surgical resection. It is worth noting that the brain parenchyma infiltrated by LGG is thought to be more extensive than the FLAIR hypersignal (86). Thus, the real invasion into WM may be underestimated. To improve the sensitivity of neuroimaging, quantitative analysis of DTI indices may provide useful information for assessing tumor microstructures and glioma cell invasion within the WM (86, 87). Indeed, DTI values such as fractional anisotropy and perpendicular diffusivity seem to be sensitive and specific biomarkers, reflecting the integrity of the myelin in various pathological or physiological processes, e.g., WM maturation, demyelination, or dysmyelination (87–89). However, even though these data are promising, it should be acknowledged that they have been acquired in animal models. Therefore, further studies using each patients’ DTI are needed in order to examine the relationship between WM invasion by the glioma, the consequence on myelin, and brain functions.
WM infiltration is critical for elaborating an individualized management, both regarding the timing of active treatment(s) which should be proposed earlier (including in incidental LGG), namely, before a too wide involvement of the WM bundles, as well as regarding the anatomic extent of surgical resection and/or irradiation which should take account of the subcortical connectivity (53).
Although the risk of severe persistent neurological deficit is almost nil in recent surgical series using awake mapping for LGG resections, with a high rate (94% to 97%) of return to work (76, 90), extensive postsurgical neuropsychological assessments have nonetheless revealed a subset of patients who kept some degrees of impairment regarding higher-order cognitive functions as well as behavior and personality (76, 85). By using tractwise and disconnectome-behavior analyses, these subtle but objective deficits, which may have a negative impact on quality of life, have mostly been linked to a surgical disconnection of WM fibers. For example, correlations were demonstrated between damage of the left SLF as well as the left frontal aslant tract and lasting executive decline (81); injury of the left UF and heightened schizotypal traits (91); disruption of the left IFOF and behavior changes such as hyperactivity (85); disconnection of the right UF as well as the right IFOF and subjective empathy impairment (92); damage of the right AF and social cognition (mentalizing) deterioration (84); lesion of the left ILF and lexical access disturbances (79); or surgical disruption of the SLF/cingulate bundle and diminished performance in the voluntary deployment of visuospatial attention (93).
These findings confirming the low plastic capacity of the WM fibers play a critical role in the surgical strategy, not only regarding the principle of connectome-based resection relying on the mapping of cortico-subcortical networks critical for brain functions (with special emphasis on the preservation of WM connections) (94), but also concerning the indications of potential reoperation(s) (95). Indeed, the degree of additional functional reorganization occurring after the first surgery and making (or not) possible subsequent resection(s) is constrained by the prominent LGG relapse within the subcortical connectivity (96). Because glioma stem cells are preferentially located along WM fibers exhibiting a demyelinated phenotype at the invasive frontier of tumor tissues (46), the more the neoplasm will exhibit a migratory pattern (rather than a proliferative, bulky one), the less other(s) radical resection(s) will be conceivable for functional issues (53).
Similarly, the neural connectivity should be taken into consideration for adjuvant medical treatments, especially by incorporating WM tracts as structures at risk for planning radiotherapy (97). Delayed radiation-induced cognitive deteriorations are frequent in long-term survivors with LGG (98), mostly due to injury of the WM bundles, as evidenced by correlations between behavior outcomes and DTI following radiotherapy (99–101). For instance, attention and processing speed decline were observed after radiotherapy of the corpus callosum and intrahemispheric WM fibers (102); language deterioration after radiotherapy of left-sided perisylvian WM (103); memory decline after radiotherapy of medial temporal WM (104); or executive function impairment following radiotherapy of the anterior cingulate bundle (105). This progressive disruption of the WM integrity, which occurs even after focal radiation (106), is mainly elicited by axonal degeneration and demyelination (107–109). This was confirmed by DTI studies which showed increased radial diffusion (100, 108), a radiological marker associated with histologic evidence of demyelination (110).
Furthermore, regarding mechanisms underlying the “chemo-brain” phenomenon, namely, chemotherapy-related cognitive impairment (111, 112), an experimental mouse model showed that these neuropsychological effects may be due to depletion of white matter OPC (113), with a block of activity-regulated myelination induced by methotrexate (114).
While neglected for a long time, WM tracts are of utmost importance in glioma patients, since their infiltration is one of the main causes of poor outcome. From an oncological perspective, a more extensive glioma involvement of WM fibers was correlated to tumor relapse (115), decreased progression-free survival and shorter overall survival (116). From a functional perspective, glioma diffusion along WM pathways which represent the skeleton of the “minimal common brain” (with a low potential of neuroplasticity) (5, 62), is linked to a higher risk of cognitive decline, partly due to a deficit in activity-dependent myelination (39). Such a migratory pattern within the subcortical connectivity should lead to adapt the therapeutic strategy, by tailoring “à la carte” both the surgical resection according to functional boundaries mapped in awake patients as well as the irradiation planning (79, 97). Therefore, incorporation of these connectomal constraints is critical in the quest for optimization of the onco-functional balance via individualized multistage management of LGG patients, especially by proposing earlier treatment(s) before a too large diffusion of tumoral cells (53). In this sprit, neurooncologists must refine their understanding of activity-dependent myelin plasticity regulated by oligodendrocyte/OPC dynamics (69, 117, 118), which seems to be a cornerstone in LGG origin and dissemination. To this end, recent models of 3D anisotropic migration have been elaborated (119, 120), which could be helpful to identify new therapeutic targets in order to inhibit glioma invasion along WM (46, 48, 120, 121). Another promising treatment avenue would be to promote remyelination (122), which might result in possible cognitive improvement, as shown in animal model of chemo-brain (114).
The author confirms being the sole contributor of this work and has approved it for publication.
The author declares that the research was conducted in the absence of any commercial or financial relationships that could be construed as a potential conflict of interest.
All claims expressed in this article are solely those of the authors and do not necessarily represent those of their affiliated organizations, or those of the publisher, the editors and the reviewers. Any product that may be evaluated in this article, or claim that may be made by its manufacturer, is not guaranteed or endorsed by the publisher.
1. Monje M. Synaptic Communication in Brain Cancer. Cancer Res (2020) 80:2979–82. doi: 10.1158/0008-5472.CAN-20-0646
2. Duffau H. Lessons From Brain Mapping in Surgery for Low-Grade Glioma: Insights Into Associations Between Tumour and Brain Plasticity. Lancet Neurol (2005) 4(8):476–86. doi: 10.1016/S1474-4422(05)70140-X
3. Duffau H. Functional Mapping Before and After Low-Grade Glioma Surgery: A New Way to Decipher Various Spatiotemporal Patterns of Individual Neuroplastic Potential in Brain Tumor Patients. Cancers (Basel) (2020) 12(9):2611. doi: 10.3390/cancers12092611
4. Duffau H. Stimulation Mapping of White Matter Tracts to Study Brain Functional Connectivity. Nat Rev Neurol (2015) 11:255–65. doi: 10.1038/nrneurol.2015.51
5. Herbet G, Maheu M, Costi E, Lafargue G, Duffau H. Mapping Neuroplastic Potential in Brain-Damaged Patients. Brain (2016) 139:829–44. doi: 10.1093/brain/awv394
6. Darlix A, Gozé C, Rigau V, Bauchet L, Taillandier L, Duffau H. The Etiopathogenesis of Diffuse Low-Grade Gliomas. Crit Rev Oncol Hematol (2017) 109:51–62. doi: 10.1016/j.critrevonc.2016.11.014
7. Gerin C, Pallud J, Grammaticos B, Mandonnet E, Deroulers C, Varlet P, et al. Improving the Time-Machine: Estimating Date of Birth of Grade II Gliomas. Cell Prolif (2012) 45(1):76–90. doi: 10.1111/j.1365-2184.2011.00790.x
8. Mandonnet E, Delattre JY, Tanguy ML, Swanson KR, Carpentier AF, Duffau H, et al. Continuous Growth of Mean Tumor Diameter in a Subset of Grade II Gliomas. Ann Neurol (2003) 53(4):524–8. doi: 10.1002/ana.10528
9. Pallud J, Fontaine D, Duffau H, Mandonnet E, Sanai N, Taillandier L, et al. Natural History of Incidental World Health Organization Grade II Gliomas. Ann Neurol (2010) 68(5):727–33. doi: 10.1002/ana.22106
10. Duffau H, Pallud J, Mandonnet E. Evidence for the Genesis of WHO Grade II Glioma in an Asymptomatic Young Adult Using Repeated MRIs. Acta Neurochir (Wien) (2011) 153(3):473–7. doi: 10.1007/s00701-010-0917-x
11. Duffau H, Capelle L. Preferential Brain Locations of Low-Grade Gliomas. Cancer (2004) 100(12):2622–6. doi: 10.1002/cncr.20297
12. Parisot S, Duffau H, Chemouny S, Paragios N. Graph Based Spatial Position Mapping of Low-Grade Gliomas. Med Image Comput Comput Assist Interv (2011) 14(Pt 2):508–15. doi: 10.1007/978-3-642-23629-7_62
13. Parisot S, Darlix A, Baumann C, Zouaoui S, Yordanova Y, Blonski M, et al. A Probabilistic Atlas of Diffuse WHO Grade II Glioma Locations in the Brain. PloS One (2016) 11(1):e0144200. doi: 10.1371/journal.pone.0144200
14. Viegas C, Moritz-Gasser S, Rigau V, Duffau H. Occipital WHO Grade II Gliomas: Oncological, Surgical and Functional Considerations. Acta Neurochir (Wien) (2011) 153(10):1907–17. doi: 10.1007/s00701-011-1125-z
15. Chang EF, Clark A, Smith JS, Polley MY, Chang SM, Barbaro NM, et al. Functional Mapping-Guided Resection of Low-Grade Gliomas in Eloquent Areas of the Brain: Improvement of Long-Term Survival. J Neurosurg (2011) 114(3):566–73. doi: 10.3171/2010.6.JNS091246
16. Capelle L, Fontaine D, Mandonnet E, Taillandier L, Golmard JL, Bauchet L, et al. Spontaneous and Therapeutic Prognostic Factors in Adult Hemispheric World Health Organization Grade II Gliomas: A Series of 1097 Cases. J Neurosurg (2013) 118:1157–68. doi: 10.3171/2013.1.JNS121
17. Ashikaga R, Araki Y, Ono Y, Nishimura Y, Ishida O. Appearance of Normal Brain Maturation on Fluid-Attenuated Inversion-Recovery (FLAIR) MR Images. AJNR Am J Neuroradiol (1999) 20(3):427–31.
18. Paus T, Collins DL, Evans AC, Leonard G, Pike B, Zijdenbos A. Maturation of White Matter in the Human Brain: A Review of Magnetic Resonance Studies. Brain Res Bull (2001) 54(3):255–66. doi: 10.1016/S0361-9230(00)00434-2
19. Dubois J, Dehaene-Lambertz G, Kulikova S, Poupon C, Hüppi PS, Hertz-Pannier L. The Early Development of Brain White Matter: A Review of Imaging Studies in Fetuses, Newborns and Infants. Neuroscience (2014) 276(C):48–71. doi: 10.1016/j.neuroscience.2013.12.044
20. Lebel C, Treit S, Beaulieu C. A Review of Diffusion MRI of Typical White Matter Development From Early Childhood to Young Adulthood. NMR BioMed (2019) 32(4):e3778. doi: 10.1002/nbm.3778
21. Paus T. Mapping Brain Maturation and Cognitive Development During Adolescence. Trends Cognit Sci (2005) 9(2):60–8. doi: 10.1016/j.tics.2004.12.008
22. Barkovich AJ, Kjos BO, Jackson DE, Norman D. Normal Maturation of the Neonatal and Infant Brain: MR Imaging at 1.5T. Radiology (1988) 166:173–80. doi: 10.1148/radiology.166.1.3336675
23. Brody BA, Kinney HC, Kloman AS, Gilles FH. Sequence of Central Nervous System Myelination in Human Infancy. I. An Autopsy Study of Myelination. J Neuropathol Exp Neurol (1987) 46(3):283–301. doi: 10.1097/00005072-198705000-00005
24. Kinney HC, Brody BA, Kloman AS, Gilles FH. Sequence of Central Nervous System Myelination in Human Infancy. II. Patterns of Myelination in Autopsied Infants. J Neuropathol Exp Neurol (1988) 47(3):217–34. doi: 10.1097/00005072-198805000-00003
25. Hassink RI, Hiltbrunner B, Müller S, Lütschg J. Assessment of Brain Maturation by T2-Weighted MRI. Neuropediatrics (1992) 23(2):72–4. doi: 10.1055/s-2008-1071316
26. Dubois J, Adibpour P, Poupon C, Hertz-Pannier L, Dehaene-Lambertz G. MRI and M/EEG Studies of the White Matter Development in Human Fetuses and Infants: Review and Opinion. Brain Plast (2016) 2(1):49–69. doi: 10.3233/BPL-160031
27. Whitaker KJ, Vértes PE, Romero-Garcia R, Váša F, Moutoussis M, Prabhu G, et al. Adolescence Is Associated With Genomically Patterned Consolidation of the Hubs of the Human Brain Connectome. Proc Natl Acad Sci USA (2016) 113(32):9105–10. doi: 10.1073/pnas.1601745113
28. Olivares R, Montiel J, Aboitiz F. Species Differences and Similarities in the Fine Structure of the Mammalian Corpus Callosum. Brain Behav Evol (2001) 57:98–105. doi: 10.1159/000047229
29. Chang KJ, Redmond SA, Chan JR. Remodeling Myelination: Implications for Mechanisms of Neural Plasticity. Nat Neurosci (2016) 19(2):190–7. doi: 10.1038/nn.4200
30. Fields RD. A New Mechanism of Nervous System Plasticity: Activity-Dependent Myelination. Nat Rev Neurosci (2015) 16(12):756–67. doi: 10.1038/nrn4023
31. Yeatman JD, Wandell BA, Mezer AA. Lifespan Maturation and Degeneration of Human Brain White Matter. Nat Commun (2014) 5:4932. doi: 10.1038/ncomms5932
32. Gibson EM, Purger D, Mount CW, Goldstein AK, Lin GL, Wood LS, et al. Neuronal Activity Promotes Oligodendrogenesis and Adaptive Myelination in the Mammalian Brain. Science (2014) 344(6183):1252304. doi: 10.1126/science.1252304
33. Nickel M, Gu C. Regulation of Central Nervous System Myelination in Higher Brain Functions. Neural Plast (2018) 2018:6436453. doi: 10.1155/2018/6436453
34. Purger D, Gibson EM, Monje M. Myelin Plasticity in the Central Nervous System. Neuropharmacology (2016) 110(Pt B):563–73. doi: 10.1016/j.neuropharm.2015.08.001
35. Venkatesh H, Monje M. Neuronal Activity in Ontogeny and Oncology. Trends Cancer (2017) 3(2):89–112. doi: 10.1016/j.trecan.2016.12.008
36. Venkatesh HS, Johung TB, Caretti V, Noll A, Tang Y, Nagaraja S, et al. Neuronal Activity Promotes Glioma Growth Through Neuroligin-3 Secretion. Cell (2015) 161(4):803–16. doi: 10.1016/j.cell.2015.04.012
37. Gillespie S, Monje M. An Active Role for Neurons in Glioma Progression: Making Sense of Scherer's Structures. Neuro Oncol (2018) 20(10):1292–9. doi: 10.1093/neuonc/noy083
38. Venkatesh HS, Morishita W, Geraghty AC, Silverbush D, Gillespie SM, Arzt M, et al. Electrical and Synaptic Integration of Glioma Into Neural Circuits. Nature (2019) 573(7775):539–45. doi: 10.1038/s41586-019-1563-y
39. Gibson EM, Geraghty AC, Monje M. Bad Wrap: Myelin and Myelin Plasticity in Health and Disease. Dev Neurobiol (2018) 78(2):123–35. doi: 10.1002/dneu.22541
40. Romero-Garcia R, Hart MG, Bethlehem RAI, Mandal A, Assem M, Crespo-Facorro B, et al. BOLD Coupling Between Lesioned and Healthy Brain Is Associated With Glioma Patients' Recovery. Cancers (Basel) (2021) 13(19):5008. doi: 10.3390/cancers13195008
41. Mandal AS, Romero-Garcia R, Hart MG, Suckling J. Genetic, Cellular, and Connectomic Characterization of the Brain Regions Commonly Plagued by Glioma. Brain (2020) 143(11):3294–307. doi: 10.1093/brain/awaa277
42. Laigle-Donadey F, Martin-Duverneuil N, Lejeune J, Crinière E, Capelle L, Duffau H, et al. Correlations Between Molecular Profile and Radiologic Pattern in Oligodendroglial Tumors. Neurology (2004) 63(12):2360–2. doi: 10.1212/01.WNL.0000148642.26985.68
43. Zhao B, Li T, Yang Y, Wang X, Luo T, Shan Y, et al. Common Genetic Variation Influencing Human White Matter Microstructure. Science (2021) 372(6548):eabf3736. doi: 10.1126/science.abf3736
45. Giese A, Westphal M. Glioma Invasion in the Central Nervous System. Neurosurgery (1996) 39(2):235–50. doi: 10.1097/00006123-199608000-00001
46. Wang J, Xu SL, Duan JJ, Yi L, Guo YF, Shi Y, et al. Invasion of White Matter Tracts by Glioma Stem Cells is Regulated by a NOTCH1-SOX2 Positive-Feedback Loop. Nat Neurosci (2019) 22(1):91–105. doi: 10.1038/s41593-018-0285-z
47. Stadlbauer A, Pölking E, Prante O, Nimsky C, Buchfelder M, Kuwert T, et al. Detection of Tumour Invasion Into the Pyramidal Tract in Glioma Patients With Sensorimotor Deficits by Correlation of (18)F-Fluoroethyl-L: -Tyrosine PET and Magnetic Resonance Diffusion Tensor Imaging. Acta Neurochir (Wien) (2009) 151(9):1061–9. doi: 10.1007/s00701-009-0378-2
48. Jbabdi S, Mandonnet E, Duffau H, Capelle L, Swanson KR, Pélégrini-Issac M, et al. Simulation of Anisotropic Growth of Low-Grade Gliomas Using Diffusion Tensor Imaging. Magn Reson Med (2005) 54(3):616–24. doi: 10.1002/mrm.20625
49. Yen PS, Teo BT, Chiu CH, Chen SC, Chiu TL, Su CF, et al. White Matter Tract Involvement in Brain Tumors: A Diffusion Tensor Imaging Analysis. Surg Neurol (2009) 72:464–9. doi: 10.1016/j.surneu.2009.05.008
50. Voets NL, Bartsch A, Plaha P. Brain White Matter Fibre Tracts: A Review of Functional Neuro-Oncological Relevance. J Neurol Neurosurg Psychiatry (2017) 88(12):1017–25. doi: 10.1136/jnnp-2017-316170
51. Shen S, Feng S, Liu H, Jiang J, Yu X. Associations of Histological and Molecular Alterations With Invasion of the Corpus Callosum in Gliomas. Acta Neurochir (Wien) (2020) 162(7):1691–9. doi: 10.1007/s00701-020-04376-9
52. Mandonnet E, Capelle L, Duffau H. Extension of Paralimbic Low Grade Gliomas: Toward an Anatomical Classification Based on White Matter Invasion Patterns. J Neurooncol (2006) 78(2):179–85. doi: 10.1007/s11060-005-9084-y
53. Duffau H. Dynamic Interplay Between Lower-Grade Glioma Instability and Brain Metaplasticity: Proposal of an Original Model to Guide the Therapeutic Strategy. Cancers (Basel) (2021) 13(19):4759. doi: 10.3390/cancers13194759
54. Cuddapah VA, Robel S, Watkins S, Sontheimer H. A Neurocentric Perspective on Glioma Invasion. Nat Rev Neurosci (2014) 15(7):455–65. doi: 10.1038/nrn3765
55. Parmigiani E, Scalera M, Mori E, Tantillo E, Vannini E. Old Stars and New Players in the Brain Tumor Microenvironment. Front Cell Neurosci (2021) 15:709917. doi: 10.3389/fncel.2021.709917
56. Giese A, Bjerkvig R, Berens ME, Westphal M. Cost of Migration: Invasion of Malignant Gliomas and Implications for Treatment. J Clin Oncol (2003) 21(8):1624–36. doi: 10.1200/JCO.2003.05.063
57. Iwadate Y, Fukuda K, Matsutani T, Saeki N. Intrinsic Protective Mechanisms of the Neuron-Glia Network Against Glioma Invasion. J Clin Neurosci (2016) 26:19–25. doi: 10.1016/j.jocn.2015.07.024
58. Brooks LJ, Clements MP, Burden JJ, Kocher D, Richards L, Devesa SC, et al. The White Matter Is a Pro-Differentiative Niche for Glioblastoma. Nat Commun (2021) 12(1):2184. doi: 10.1038/s41467-021-22225-w
59. Beliën AT, Paganetti PA, Schwab ME. Membrane-Type 1 Matrix Metalloprotease (MT1-MMP) Enables Invasive Migration of Glioma Cells in Central Nervous System White Matter. J Cell Biol (1999) 144(2):373–84. doi: 10.1083/jcb.144.2.373
60. Duffau H. The Huge Plastic Potential of Adult Brain and the Role of Connectomics: New Insights Provided by Serial Mappings in Glioma Surgery. Cortex (2014) 58:325–37. doi: 10.1016/j.cortex.2013.08.005
61. Herbet G, Duffau H. Revisiting the Functional Anatomy of the Human Brain: Toward a Meta-Networking Theory of Cerebral Functions. Physiol Rev (2020) 100(3):1181–228. doi: 10.1152/physrev.00033.2019
62. Ius T, Angelini E, Thiebaut de Schotten M, Mandonnet E, Duffau H. Evidence for Potentials and Limitations of Brain Plasticity Using an Atlas of Functional Resectability of WHO Grade II Gliomas: Towards a "Minimal Common Brain". Neuroimage (2011) 56(3):992–1000. doi: 10.1016/j.neuroimage.2011.03.022
63. Sarubbo S, Tate M, De Benedictis A, Merler S, Moritz-Gasser S, Herbet G, et al. Mapping Critical Cortical Hubs and White Matter Pathways by Direct Electrical Stimulation: An Original Functional Atlas of the Human Brain. Neuroimage (2020) 205:116237. doi: 10.1016/j.neuroimage.2019.116237
64. Duffau H. A Two-Level Model of Interindividual Anatomo-Functional Variability of the Brain and its Implications for Neurosurgery. Cortex (2017) 86:303–13. doi: 10.1016/j.cortex.2015.12.009
65. Paus T, Pesaresi M, French L. White Matter as a Transport System. Neuroscience (2014) 276:117–25. doi: 10.1016/j.neuroscience.2014.01.055
66. Innocenti GM. Network Causality, Axonal Computations, and Poffenberger. Exp Brain Res (2017) 235(8):2349–57. doi: 10.1007/s00221-017-4948-x
67. Hartline DK, Colman DR. Rapid Conduction and the Evolution of Giant Axons and Myelinated Fibers. Curr Biol (2007) 17:R29–35. doi: 10.1016/j.cub.2006.11.042
68. Hill RA, Li AM, Grutzendler J. Lifelong Cortical Myelin Plasticity and Agerelated Degeneration in the Live Mammalian Brain. Nat Neurosci (2018) 21:683–95. doi: 10.1038/s41593-018-0120-6
69. Hughes EG, Orthmann-Murphy JL, Langseth AJ, Bergles DE. Myelin Remodeling Through Experience-Dependent Oligodendrogenesis in the Adult Somatosensory Cortex. Nat Neurosci (2018) 21(5):696–706. doi: 10.1038/s41593-018-0121-5
70. McKenzie IA, Ohayon D, Li H, de Faria JP, Emery B, Tohyama K, et al. Motor Skill Learning Requires Active Central Myelination. Science (2014) 346:318–22. doi: 10.1126/science.1254960
71. Bengtsson SL, Nagy Z, Skare S, Forsman L, Forssberg H, Ullen F. Extensive Piano Practicing has Regionally Specific Effects on White Matter Development. Nat Neurosci (2005) 8:1148–50. doi: 10.1038/nn1516
72. Scholz J, Klein MC, Behrens TE, Johansen-Berg H. Training Induces Changes in White-Matter Architecture. Nat Neurosci (2009) 12:1370–1. doi: 10.1038/nn.2412
73. Pajevic S, Basser PJ, Fields RD. Role of Myelin Plasticity in Oscillations and Synchrony of Neuronal Activity. Neuroscience (2014) 276:135–47. doi: 10.1016/j.neuroscience.2013.11.007
74. King EM, Sabatier MJ, Hoque M, Kesar TM, Backus D, Borich MR. Myelin Status is Associated With Change in Functional Mobility Following Slope Walking in People With Multiple Sclerosis. Mult Scler J Exp Transl Clin (2018) 4(2):2055217318773540. doi: 10.1177/2055217318773540
75. Szalisznyo K, Silverstein DN, Duffau H, Smits A. Pathological Neural Attractor Dynamics in Slowly Growing Gliomas Supports an Optimal Time Frame for White Matter Plasticity. PloS One (2013) 8(7):e69798. doi: 10.1371/journal.pone.0069798
76. Lemaitre AL, Herbet G, Ng S, Moritz-Gasser S, Duffau H. Cognitive Preservation Following Awake Mapping-Based Neurosurgery for Low-Grade Gliomas: A Longitudinal, Within-Patient Design Study. Neuro-Oncol (2021), noab275. doi: 10.1093/neuonc/noab275
77. Herbet G, Lafargue G, Duffau H. Rethinking Voxel-Wise Lesion-Deficit Analysis: A New Challenge for Computational Neuropsychology. Cortex (2015) 64:413–6. doi: 10.1016/j.cortex.2014.10.021
78. Almairac F, Herbet G, Moritz-Gasser S, de Champfleur NM, Duffau H. The Left Inferior Fronto-Occipital Fasciculus Subserves Language Semantics: A Multilevel Lesion Study. Brain Struct Funct (2015) 220(4):1983–95. doi: 10.1007/s00429-014-0773-1
79. Herbet G, Moritz-Gasser S, Boiseau M, Duvaux S, Cochereau J, Duffau H. Converging Evidence for a Cortico-Subcortical Network Mediating Lexical Retrieval. Brain (2016) 139(11):3007–21. doi: 10.1093/brain/aww220
80. Herbet G, Lafargue G, Bonnetblanc F, Moritz-Gasser S, Menjot de Champfleur N, Duffau H. Inferring a Dual-Stream Model of Mentalizing From Associative White Matter Fibres Disconnection. Brain (2014) 137(Pt 3):944–59. doi: 10.1093/brain/awt370
81. Cochereau J, Lemaitre AL, Wager M, Moritz-Gasser S, Duffau H, Herbet G. Network-Behavior Mapping of Lasting Executive Impairments After Low-Grade Glioma Surgery. Brain Struct Funct (2020) 225(8):2415–29. doi: 10.1007/s00429-020-02131-5
82. Liu D, Liu Y, Hu X, Hu G, Yang K, Xiao C, et al. Alterations of White Matter Integrity Associated With Cognitive Deficits in Patients With Glioma. Brain Behav (2020) 10(7):e01639. doi: 10.1002/brb3.1639
83. Duffau H. New Philosophy, Clinical Pearls, and Methods for Intraoperative Cognition Mapping and Monitoring "a La Carte" in Brain Tumor Patients. Neurosurgery (2021) 88(5):919–30. doi: 10.1093/neuros/nyaa363
84. Nakajima R, Yordanova YN, Duffau H, Herbet G. Neuropsychological Evidence for the Crucial Role of the Right Arcuate Fasciculus in the Face-Based Mentalizing Network: A Disconnection Analysis. Neuropsychologia (2018) 115:179–87. doi: 10.1016/j.neuropsychologia.2018.01.024
85. Lemaitre AL, Herbet G, Duffau H, Lafargue G. Personality and Behavioral Changes After Brain Tumor Resection: A Lesion Mapping Study. Acta Neurochir (Wien) (2021) 163(5):1257–7. doi: 10.1007/s00701-021-04756-9
86. Castellano A, Donativi M, Rudà R, De Nunzio G, Riva M, Iadanza A, et al. Evaluation of Low-Grade Glioma Structural Changes After Chemotherapy Using DTI-Based Histogram Analysis and Functional Diffusion Maps. Eur Radiol (2016) 26(5):1263–73. doi: 10.1007/s00330-015-3934-6
87. Wang S, Zhou J. Diffusion Tensor Magnetic Resonance Imaging of Rat Glioma Models: A Correlation Study of MR Imaging and Histology. J Comput Assist Tomogr (2012) 36(6):739–44. doi: 10.1097/RCT.0b013e3182685436
88. Song SK, Sun SW, Ramsbottom MJ, Chang C, Russell J, Cross AH. Dysmyelination Revealed Through MRI as Increased Radial (But Unchanged Axial) Diffusion of Water. NeuroImage (2002) 17(3):1429–36. doi: 10.1006/nimg.2002.1267
89. Lope-Piedrafita S, Garcia-Martin ML, Galons JP, Gillies RJ, Trouard TP. Longitudinal Diffusion Tensor Imaging in a Rat Brain Glioma Model. NMR BioMed (2008) 21(8):799–808. doi: 10.1002/nbm.1256
90. Ng S, Herbet G, Moritz-Gasser S, Duffau H. Return to Work Following Surgery for Incidental Diffuse Low-Grade Glioma: A Prospective Series With 74 Patients. Neurosurgery (2020) 87(4):720–9. doi: 10.1093/neuros/nyz513
91. Lemaitre AL, Lafargue G, Duffau H, Herbet G. Damage to the Left Uncinate Fasciculus is Associated With Heightened Schizotypal Traits: A Multimodal Lesion-Mapping Study. Schizophr Res (2018) 97:240–8. doi: 10.1016/j.schres.2018.02.027
92. Herbet G, Lafargue G, Moritz-Gasser S, Menjot de Champfleur N, Costi E, Bonnetblanc F, et al. A Disconnection Account of Subjective Empathy Impairments in Diffuse Low-Grade Glioma Patients. Neuropsychologia (2015) 70:165–76. doi: 10.1016/j.neuropsychologia.2015.02.015
93. Herbet G, Duffau H. Critical Contribution of the Medial Eye Field Network to the Voluntary Deployment of Visuospatial Attention. Nat Commun (2022) 13(1):328. doi: 10.1038/s41467-022-28030-3
94. Duffau H. Brain Connectomics Applied to Oncological Neuroscience: From a Traditional Surgical Strategy Focusing on Glioma Topography to a Meta-Network Approach. Acta Neurochir (Wien) (2021) 163(4):905–17. doi: 10.1007/s00701-021-04752-z
95. Hamdan N, Duffau H. Extending the Multistage Surgical Strategy for Recurrent Initially Low-Grade Gliomas: Functional and Oncological Outcomes in 31 Consecutive Patients Who Underwent a Third Resection Under Awake Mapping. J Neurosurg (2021), 1–10. doi: 10.3171/2021.3.JNS21264
96. Picart T, Herbet G, Moritz-Gasser S, Duffau H. Iterative Surgical Resections of Diffuse Glioma With Awake Mapping: How to Deal With Cortical Plasticity and Connectomal Constraints? Neurosurgery (2019) 85(1):105–16. doi: 10.1093/neuros/nyy218
97. Duffau H. Why Brain Radiation Therapy Should Take Account of the Individual Structural and Functional Connectivity: Toward an Irradiation "a La Carte". Crit Rev Oncol Hematol (2020) 154:103073. doi: 10.1016/j.critrevonc.2020.103073
98. Douw L, Klein M, Fagel SS, van den Heuvel J, Taphoorn MJ, Aaronson NK, et al. Cognitive and Radiological Effects of Radiotherapy in Patients With Low-Grade Glioma: Long-Term Follow-Up. Lancet Neurol (2009) 8(9):810–8. doi: 10.1016/S1474-4422(09)70204-2
99. Chapman CH, Nagesh V, Sundgren PC, Buchtel H, Chenevert TL, Junck L, et al. Diffusion Tensor Imaging of Normal-Appearing White Matter as Biomarker for Radiation-Induced Late Delayed Cognitive Decline. Int J Radiat Oncol Biol Phys (2012) 82(5):2033–40. doi: 10.1016/j.ijrobp.2011.01.068
100. Chapman CH, Zhu T, Nazem-Zadeh M, Tao Y, Buchtel HA, Tsien CI, et al. Diffusion Tensor Imaging Predicts Cognitive Function Change Following Partial Brain Radiotherapy for Low-Grade and Benign Tumors. Radiother Oncol (2016) 120(2):234–40. doi: 10.1016/j.radonc.2016.06.021
101. Connor M, Karunamuni R, McDonald C, Seibert T, White N, Moiseenko V, et al. Regional Susceptibility to Dose-Dependent White Matter Damage After Brain Radiotherapy. Radiother Oncol (2017) 123(2):209–17. doi: 10.1016/j.radonc.2017.04.006
102. Huynh-Le MP, Tibbs MD, Karunamuni R, Salans M, Tringale KR, Yip A, et al. Microstructural Injury to Corpus Callosum and Intrahemispheric White Matter Tracts Correlate With Attention and Processing Speed Decline After Brain Radiation. Int J Radiat Oncol Biol Phys (2021) 110(2):337–47. doi: 10.1016/j.ijrobp.2020.12.046
103. Tibbs MD, Huynh-Le MP, Karunamuni R, Reyes A, Macari AC, Tringale KR, et al. Microstructural Injury to Left-Sided Perisylvian White Matter Predicts Language Decline After Brain Radiation Therapy. Int J Radiat Oncol Biol Phys (2020) 108(5):1218–28. doi: 10.1016/j.ijrobp.2020.07.032
104. Tringale KR, Nguyen TT, Karunamuni R, Seibert T, Huynh-Le MP, Connor M, et al. Quantitative Imaging Biomarkers of Damage to Critical Memory Regions Are Associated With Post-Radiation Therapy Memory Performance in Brain Tumor Patients. Int J Radiat Oncol Biol Phys (2019) 105(4):773–83. doi: 10.1016/j.ijrobp.2019.08.003
105. Tringale KR, Nguyen T, Bahrami N, Marshall DC, Leyden KM, Karunamuni R, et al. Identifying Early Diffusion Imaging Biomarkers of Regional White Matter Injury as Indicators of Executive Function Decline Following Brain Radiotherapy: A Prospective Clinical Trial in Primary Brain Tumor Patients. Radiother Oncol (2019) 132:27–33. doi: 10.1016/j.radonc.2018.11.018
106. Ding Z, Zhang H, Lv XF, Xie F, Liu L, Qiu S, et al. Radiation-Induced Brain Structural and Functional Abnormalities in Presymptomatic Phase and Outcome Prediction. Hum Brain Mapp (2018) 39(1):407–27. doi: 10.1002/hbm.23852
107. Hope TR, Vardal J, Bjørnerud A, Larsson C, Arnesen MR, Salo RA, et al. Serial Diffusion Tensor Imaging for Early Detection of Radiation-Induced Injuries to Normal-Appearing White Matter in High-Grade Glioma Patients. J Magn Reson Imaging (2015) 41(2):414–23. doi: 10.1002/jmri.24533
108. Zhu T, Chapman CH, Tsien C, Kim M, Spratt DE, Lawrence TS, et al. Effect of the Maximum Dose on White Matter Fiber Bundles Using Longitudinal Diffusion Tensor Imaging. Int J Radiat Oncol Biol Phys (2016) 96(3):696–705. doi: 10.1016/j.ijrobp.2016.07.010
109. Milic M, Rees JH. Acute Demyelination Following Radiotherapy for Glioma: A Cautionary Tale. Pract Neurol (2017) 17:35–8. doi: 10.1136/practneurol-2016-001432
110. Wang S, Wu EX, Qiu D, Leung LHT, Lau HF, Khong PL. Longitudinal Diffusion Tensor Magnetic Resonance Imaging Study of Radiation-Induced White Matter Damage in a Rat Model. Cancer Res (2009) 69:1190–8. doi: 10.1158/0008-5472.CAN-08-2661
111. Kaiser J, Bledowski C, Dietrich J. Neural Correlates of Chemotherapy-Related Cognitive Impairment. Cortex (2014) 54:33–50. doi: 10.1016/j.cortex.2014.01.010
112. Pierson C, Waite E, Pyykkonen B. A Meta-Analysis of the Neuropsychological Effects of Chemotherapy in the Treatment of Childhood Cancer. Pediatr Blood Cancer (2016) 63:1998–2003. doi: 10.1002/pbc.26117
113. Gibson EM, Nagaraja S, Ocampo A, Tam LT, Wood LS, Pallegar PN, et al. Methotrexate Chemotherapy Induces Persistent Tri-Glial Dysregulation That Underlies Chemotherapy-Related Cognitive Impairment. Cell (2019) 176(1-2):43–55.e13. doi: 10.1016/j.cell.2018.10.049
114. Geraghty AC, Gibson EM, Ghanem RA, Greene JJ, Ocampo A, Goldstein AK, et al. Loss of Adaptive Myelination Contributes to Methotrexate Chemotherapy-Related Cognitive Impairment. Neuron (2019) 103(2):250–65. doi: 10.1016/j.neuron.2019.04.032
115. Bette S, Huber T, Gempt J, Boeckh-Behrens T, Wiestler B, Kehl V, et al. Local Fractional Anisotropy is Reduced in Areas With Tumor Recurrence in Glioblastoma. Radiology (2017) 283:499–507. doi: 10.1148/radiol.2016152832
116. Mickevicius NJ, Carle AB, Bluemel T, Santarriaga S, Schloemer F, Shumate D, et al. Location of Brain Tumor Intersecting White Matter Tracts Predicts Patient Prognosis. J Neurooncol (2015) 125(2):393–400. doi: 10.1007/s11060-015-1928-5
117. Sampaio-Baptista C, Johansen-Berg H. White Matter Plasticity in the Adult Brain. Neuron (2017) 96(6):1239–51. doi: 10.1016/j.neuron.2017.11.026
118. Foster AY, Bujalka H, Emery B. Axoglial Interactions in Myelin Plasticity: Evaluating the Relationship Between Neuronal Activity and Oligodendrocyte Dynamics. Wiley New York (2019) 67(11):2038–49. doi: 10.1002/glia.23629
119. Saleh A, Marhuenda E, Fabre C, Hassani Z, Weille J, Boukhaddaoui H, et al. A Novel 3D Nanofibre Scaffold Conserves the Plasticity of Glioblastoma Stem Cell Invasion by Regulating Galectin-3 and Integrin-β1 Expression. Sci Rep (2019) 9(1):14612. doi: 10.1038/s41598-019-51108-w
120. Marhuenda E, Fabre C, Zhang C, Martin-Fernandez M, Iskratsch T, Saleh A, et al. Glioma Stem Cells Invasive Phenotype at Optimal Stiffness Is Driven by MGAT5 Dependent Mechanosensing. J Exp Clin Cancer Res (2021) 40(1):139. doi: 10.1186/s13046-021-01925-7
121. Hassani Z, Saleh A, Turpault S, Khiati S, Morelle W, Vignon J, et al. Phostine PST3.1a Targets MGAT5 and Inhibits Glioblastoma-Initiating Cell Invasiveness and Proliferation. Mol Cancer Res (2017) 15(10):1376–87. doi: 10.1158/1541-7786
Keywords: brain mapping, cognition, lower-grade glioma, myelin, neural networks, neuroplasticity, white matter tracts, brain connectome
Citation: Duffau H (2022) White Matter Tracts and Diffuse Lower-Grade Gliomas: The Pivotal Role of Myelin Plasticity in the Tumor Pathogenesis, Infiltration Patterns, Functional Consequences and Therapeutic Management. Front. Oncol. 12:855587. doi: 10.3389/fonc.2022.855587
Received: 15 January 2022; Accepted: 14 February 2022;
Published: 02 March 2022.
Edited by:
Francesco Latini, Uppsala University, SwedenReviewed by:
Rajiv Magge, Cornell University, United StatesCopyright © 2022 Duffau. This is an open-access article distributed under the terms of the Creative Commons Attribution License (CC BY). The use, distribution or reproduction in other forums is permitted, provided the original author(s) and the copyright owner(s) are credited and that the original publication in this journal is cited, in accordance with accepted academic practice. No use, distribution or reproduction is permitted which does not comply with these terms.
*Correspondence: Hugues Duffau, aC1kdWZmYXVAY2h1LW1vbnRwZWxsaWVyLmZy
Disclaimer: All claims expressed in this article are solely those of the authors and do not necessarily represent those of their affiliated organizations, or those of the publisher, the editors and the reviewers. Any product that may be evaluated in this article or claim that may be made by its manufacturer is not guaranteed or endorsed by the publisher.
Research integrity at Frontiers
Learn more about the work of our research integrity team to safeguard the quality of each article we publish.