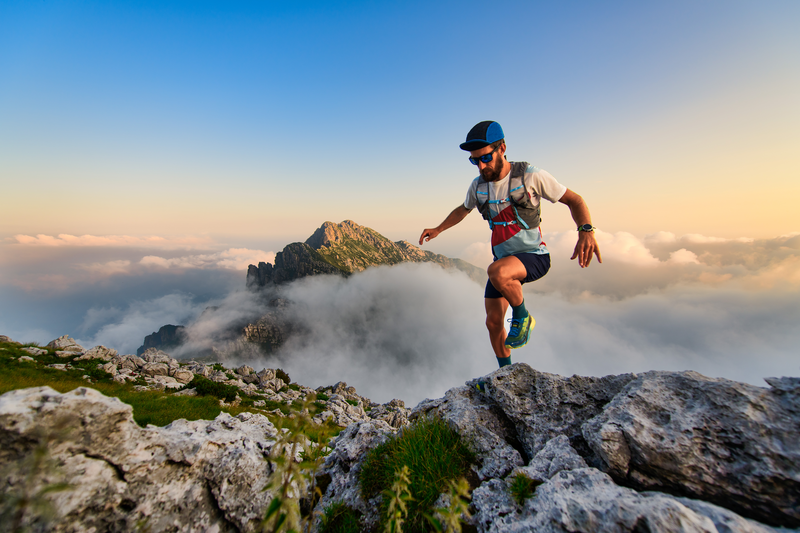
95% of researchers rate our articles as excellent or good
Learn more about the work of our research integrity team to safeguard the quality of each article we publish.
Find out more
ORIGINAL RESEARCH article
Front. Oncol. , 28 July 2022
Sec. Molecular and Cellular Oncology
Volume 12 - 2022 | https://doi.org/10.3389/fonc.2022.854702
This article is part of the Research Topic Targeting the Wnt/β-catenin Signaling Pathway in Cancer View all 10 articles
Background: A-kinase interacting protein 1 (AKIP1) promotes tumor progression and chemoresistance in several malignancies; meanwhile, it is related to higher tumor size and recurrence risk of papillary thyroid carcinoma, while the role of AKIP1 in anaplastic thyroid carcinoma (ATC) is unclear. The aim of this study is to explore the effect of AKIP1 knockdown on cell malignant behaviors and doxorubicin resistance in ATC.
Methods: AKIP1 knockdown was conducted in ATC cell lines (8505C and CAL-62 cells) by siRNA; then, cell viability, apoptosis, invasion, PI3K/AKT and β-catenin pathways, and doxorubicin sensitivity were detected. Subsequently, doxorubicin-resistant 8505C cells (8505C/Dox) were established. Additionally, AKIP1 was modified in 8505C and 8505C/Dox cells that underwent doxorubicin treatment by siRNA or overexpression plasmid, followed by cellular function and pathway detection.
Results: AKIP1 was elevated in FRO, 8505C, CAL-62, and KHM-5M cells compared to control cells (all p < 0.05). Subsequently, AKIP1 knockdown elevated apoptosis, inhibited viability and invasion, and inactivated PI3K/AKT and β-catenin pathways in 8505C and CAL-62 cells (all p < 0.05). AKIP1 knockdown decreased relative cell viability in doxorubicin-treated 8505C and CAL-62 cells; then, AKIP1 was elevated in 8505C/Dox cells compared to 8505C cells (all p < 0.05). Furthermore, AKIP1 knockdown restored doxorubicin sensitivity (reflected by decreased cell viability and invasion, and increased apoptosis), but inactivated PI3K/AKT and β-catenin pathways in doxorubicin-treated 8505C/Dox cells. However, AKIP1 overexpression presented an opposite effect on these functions and pathways in doxorubicin-treated 8505C cells.
Conclusion: AKIP1 knockdown decreases cell survival and invasion while promoting sensitivity to doxorubicin via inactivating PI3K/AKT and β-catenin pathways in ATC.
Anaplastic thyroid carcinoma (ATC), a rare but the most malignant type of thyroid carcinomas, is characterized by poor differentiation, a highly aggressive nature, and quick metastasis (1–4). Currently, treatments of ATC typically consist of surgery, chemotherapy, radiotherapy, molecular targeted therapy, etc. (1, 5, 6). However, these comprehensive treatments of ATC still fail to demonstrate an obvious survival benefit for ATC patients due to its intrinsic characteristics; thus, ATC is viewed as a devastating disease with a high mortality rate (5). Hence, exploring the potential molecular mechanism and possible target to improve treatment of ATC is crucial and urgent.
A-kinase interacting protein 1 (AKIP1), originally named breast cancer-associated protein 3, facilitates the nuclear translocation of catalytic subunit of protein kinase A (7, 8). Recently, it has been reported that AKIP1 is viewed as a tumor promoter (7–11). For instance, it is proposed that AKIP1 induces the nuclear factor kappa-B (NF-κB)-dependent chemokines to promote angiogenesis and tumor growth in cervical cancer (8). Moreover, AKIP1 elevates vascular endothelial growth factor-C (VEGF-C) to accelerate angiogenesis and lymphangiogenesis in human esophageal squamous cell carcinoma (9). Another interesting study discloses that AKIP1 activates Zinc Finger E-Box Binding Homeobox 1 (ZEB1) to facilitate tumor metastasis in non-small cell lung cancer (10). In addition, AKIP1 is also considered as a regulator of treatment resistance in malignancies (11, 12). For example, AKIP1 upregulates C-X-C motif chemokine ligand (CXCL)1 and CXCL8 to decrease chemoradiation sensitivity in glioblastoma (12). Additionally, AKIP1 interacts with Tap73 to modulate the radiotherapy sensitivity of cervical cancer cells (11). Importantly, recent research has presented that AKIP1 is correlated with advanced tumor features and higher recurrence risk in papillary thyroid carcinoma (13). According to the above-mentioned information, we deduce that AKIP1 may play an important role in tumor progression and treatment resistance in ATC, while related data are scarce.
In the current study, AKIP1 modification was conducted in ATC cell lines, followed by detection of cellular functions, chemotherapy resistance, and downstream pathways, aiming to explore the potential of AKIP1 as a treatment target for ATC. The current study discovered that AKIP1 knockdown inhibited cell viability and invasion but promoted cell apoptosis, as well as restored doxorubicin sensitivity via inactivating PI3K/AKT and β-catenin pathways in ATC, indicating that targeting AKIP1 might provide a new treatment choice for ATC.
Human normal thyroid cell line (Nthy-ori 3-1) was purchased from the European Collection of Authenticated Cell Cultures (ECACC). Human ATC cell lines, including FRO, 8505C, C643, CAL-62, and KHM-5M, were purchased from the National Collection of Authenticated Cell Cultures (Shanghai, China). Cells were maintained in DMEM (Lonza, Swiss) (8505C and CAL-62) or RPMI-1640 medium (Lonza, Swiss) (Nthy-ori 3-1, FRO, C643, and KHM-5M) containing 10% fetal bovine serum (FBS) (Sigma, USA) and 1% penicillin/streptomycin (Beyotime, China) at 37°C in an incubator with 5% CO2. The doxorubicin-resistant 8505C cells (8505C/Dox) were established from the parental 8505C cells by exposing cells to gradually increasing concentrations of doxorubicin (Sigma, USA) from 0.1 μM to 10 μM over 8 months (14). 8505C/Dox cells were cultured in culture medium containing 2 μM doxorubicin to maintain drug resistance phenotype.
The AKIP1 siRNA (si-AKIP1) and negative control (si-NC) were commercially designed and synthesized by Shanghai GenePharma Co., Ltd. (Shanghai, China). The AKIP1 overexpression plasmids (pcDNA-AKIP1) and negative control (pcDNA-NC) were obtained from Guangzhou Ribobio Co., Ltd. (Guangzhou, China). ATC cells were cultured and transfected with 50 nM siRNA (si-AKIP1 or si-NC) or 0.8 μg of plasmids (pcDNA-AKIP1 or pcDNA-NC) with Lipofectamine® 3000 (Invitrogen, USA) for 6 h. The AKIP1 siRNA sequence was as follows: sense, 5’ GTGGGCTCAAATGACTTAATT 3’; antisense, 5’ TTAAGTCATTTGAGCCCACTT 3’. The non-transfected cells served as normal controls.
The 8505C cells were incubated with 0, 0.2, 0.4, 0.8, 1.6, 3.2, and 6.4 μM doxorubicin; CAL-62 cells were incubated with 0, 0.02, 0.04, 0.08, 0.16, 0.32, and 0.64 μM doxorubicin; and 8505C/Dox cells were incubated with 0, 2, 4, 8, 16, 32, and 64 μM doxorubicin (15, 16). After 48 h of treatment, CCK-8 assay was performed by the methods mentioned in the Cell Viability Assay subsection and half maximal inhibitory concentration (IC50) was calculated using the sigmoidal dose–response function of the GraphPad Prism software (Version 7.0) (17).
To assess whether AKIP1 participated in the drug resistance of ATC cells, the 8505C cells were transfected with pcDNA-AKIP1 and cultured with 1.6 μM doxorubicin (selected by IC50); meanwhile, 8505C/Dox cells were transfected with si-AKIP1 and cultured with 16 μM doxorubicin. Briefly, 8505C cells were divided into five groups, including the Normal group (non-treated), the pcDNA-NC group (transfected with NC plasmids), the pcDNA-AKIP1 group (transfected with AKIP1 overexpression plasmids), the pcDNA-NC and Dox group (transfected with NC plasmids and treated with 1.6 μM doxorubicin), and the pcDNA-AKIP1 and Dox group (transfected with AKIP1 overexpression plasmids and treated with 1.6 μM doxorubicin). Analogously, 8505C/Dox cells were divided into five groups, including the Normal group (non-treated), the si-NC group (transfected with NC siRNA), the si-AKIP1 group (transfected with AKIP1 siRNA), the si-NC and Dox group (transfected with NC siRNA and treated with 16 μM doxorubicin), and the si-AKIP1 and Dox group (transfected with AKIP1 siRNA and treated with 16 μM doxorubicin). After 48 h of treatment, further assays were carried out.
In brief, total RNA from ATC cells was extracted via Beyozol (Beyotime, China). Reverse transcription of RNA was completed by the GeneAce Reverse Transcriptase Kit (Nippon, Japan). The PCR program was implemented using the SYBR® Premix DimmerEraser™ kit (Takara, Japan). The thermal cycle of qPCR was as follows: 1 cycle, 95°C for 30 s; 40 cycles, 95°C for 5 s and 61°C for 30 s. The AKIP1 mRNA expression was evaluated based on the 2−ΔΔCt method with GAPDH as an endogenous control. The primer sequences were listed as follows (5’→3’): AKIP1 forward, CATGGACAACTGTTTGGCGG, AKIP1 reverse: TAGAGCCAGCCTTGCTGAAC; GAPDH forward, GAGTCCACTGGCGTCTTCAC, GAPDH reverse, ATCTTGAGGCTGTTGTCATACTTCT.
Western blotting assays of ATC cells were implemented at 48 h after treatment. First of all, ATC cells were lysed by RIPA comprising 1 mM PMSF (Selleck, USA) and the concentration of total proteins was quantified using the bicinchoninic acid kit (Beyotime, China). Secondly, 40 μg of proteins was separated by SDS-PAGE and transferred onto a nitrocellulose membrane (Pall, USA). Then, membranes were blocked using 5% nonfat milk (Beyotime, China), hatched with primary antibodies overnight at 4°C, and subsequently incubated with secondary antibody (1:10,000) (Affinity, China) for 1 h at 37°C. Finally, blots were detected via ECL-PLUS kit (Beyotime, China) and analyzed using ImageJ software (version 1.8.0, NIH). The primary antibodies used in this study were bought from Abcam (Cambridge, USA) and listed as follows: anti-AKIP1 (1:500, ab135996), anti-p-PI3K (1:1,000, ab182651), anti-PI3K (1:1,500, ab86714), anti-p-AKTSer473 (1:1,000, ab81283), anti-AKT (1:1,500, ab8805), anti-β-catenin (1:1,000, ab68813), anti-GAPDH (1:5,000, ab181602), and anti-Histone H3 (1:5,000, ab1791).
Cell viability was evaluated by the Cell Counting Kit-8 (CCK-8) (Sigma, USA) assay. In brief, ATC cells were plated in 96‐well plates (2 × 103 cells/well) and cultured for 48 h and 72 h. Then, 10 μl of CCK-8 reagent was added and further cultured for another 2 h at 37°C. Finally, the optical density (OD) values at 450 nm were assessed with a microplate reader (Molecular Devices, USA).
Cell apoptosis rate was detected using the TUNEL apoptosis kit (Sangon, China). Briefly, at 48 h and 72 h after treatment, ATC cells (1 × 104 cells/well) were fixed with 4% paraformaldehyde (Sangon, China) and permeabilized with 0.1% Triton X-100 (Sangon, China). Then, cells were incubated with TUNEL reagent for 20 min and DAPI solution (Sigma, USA) for 10 min at room temperature (RT), successively. The apoptotic cells were viewed and imaged with a microscope (Olympus, Japan).
At 48 h after treatment, the invasion ability of ATC cells was assessed by the transwell assay. In brief, cells were adjusted to 5 × 104 cells/well with serum-free medium and plated into the upper chamber of Matrigel-coated transwell chamber plates (Life, USA). The lower chambers were covered with 600 μl of DMEM containing 10% FBS. At 24 h after culture, the invasive cells were counted under a microscope after being stained with crystal violet (Beyotime, China) for 10 min at RT (16, 18).
The experiment was performed in triplicate. One-way ANOVA with Tukey’s or Dunnett’s post-hoc test and Student’s t-test were used for comparisons. All statistical analyses were calculated by GraphPad Prism. p < 0.05 was considered to be statistically significant.
No difference was found in AKIP1 between Nthy-ori3-1 cells and C643 cells (p > 0.05), while AKIP1 was increased in FRO, 8505C, CAL-62, and KHM-5M cells compared to Nthy-ori 3-1 cells (all p < 0.05); since AKIP1 was dramatically elevated in 8505C and CAL-62 cells, these two cell lines were selected for further experiments (Figures 1A–C). Moreover, 8505C and CAL-62 cells were transfected with AKIP1 siRNA or NC siRNA; data showed that AKIP1 was decreased in 8505C and CAL-62 cells transfected with AKIP1 siRNA compared to those transfected with NC siRNA, suggesting successful transfection (Figures 1D–H).
Figure 1 Detection of AKIP1 expression. Comparison of AKIP1 mRNA expression between human normal thyroid cell line and ATC cell lines (A); example image of AKIP1 protein in human normal thyroid cell line and ATC cell lines through Western blot (B); comparison of AKIP1 protein expression between human normal thyroid cell line and ATC cell lines (C); comparison of AKIP1 mRNA expression among groups in 8505C cells (D) and CAL-62 cells (E) after transfection; detection of AKIP1 protein expression by Western blot in 8505C cells and CAL-62 cells after transfection (F); comparison of AKIP1 protein expression among groups in 8505C cells (G) and CAL-62 cells (H) after transfection by one-way ANOVA followed by Dunnett’s post-hoc test. ATC, anaplastic thyroid carcinoma; AKIP1, A-kinase interacting protein 1; NS, not significant; *p < 0.05; **p < 0.01; ***p < 0.001.
In order to explore the regulation of AKIP1 on cellular functions, cell viability, apoptosis, and invasion assays were conducted. The data showed that AKIP1 knockdown elevated apoptosis but declined cell viability and invasive cell count in both 8505C and CAL-62 cells at 48 h after transfection (all p < 0.05); moreover, the rates of inhibition of survival of 8505C and CAL-62 cells transfected with si-AKIP1 were 37.14% and 25.82%, respectively, at 48 h after transfection; meanwhile, the apoptosis rate of normal 8505C cells, and 8505C cells transfected with si-NC and si-AKIP1 was 5.52%, 5.54%, and 14.93%, respectively; moreover, the apoptosis rate of normal CAL-62 cells, and CAL-62 cells transfected with si-NC and si-AKIP1 was 4.92%, 5.26%, and 12.52%, respectively (Figures 2A–H). Furthermore, cell viability and apoptosis presented similar trends at 72 h after AKIP1 knockdown (Supplementary Figures 1A–E).
Figure 2 Cellular functions in ATC cell lines at 48 h after siRNA transfection. Comparison of relative cell viability among groups in 8505C cells (A) and CAL-62 cells (B) after transfection; comparison of apoptosis among groups in 8505C cells (C) and CAL-62 cells (D) after transfection; comparison of invasive cell count among groups in 8505C cells (E) and CAL-62 cells (F) after transfection by one-way ANOVA followed by Dunnett’s post-hoc test; example image of cell apoptosis through TUNEL Apoptosis Assay Kit (G) and example image of cell invasion through transwell assay (H) in 8505C cells and CAL-62 cells after transfection. ATC, anaplastic thyroid carcinoma; siRNA, small interfering RNA; AKIP1, A-kinase interacting protein 1; *p < 0.05; **p < 0.01.
Previous studies have presented that AKIP1 modulates PI3K/AKT and β-catenin pathways to regulate tumor progression (12, 19, 20); thus, the effect of AKIP1 knockdown on these pathways in ATC cell lines was explored by Western blot, which revealed that AKIP1 knockdown inhibited the phosphorylation of PI3K and AKT in 8505C and CAL-62 cells (both p < 0.05); meanwhile, AKIP1 knockdown inhibited expression of β-catenin in CAL-62 cells (p < 0.05) but not in 8505C cells (p > 0.05) (Figures 3A–C). Furthermore, AKIP1 knockdown inhibited the nuclear translocation of β-catenin in 8505C and CAL-62 cells (both p < 0.01) (Supplementary Figures 2A–C).
Figure 3 PI3K/AKT and β-catenin pathways in ATC cell lines after siRNA transfection. Detection of p-PI3K, PI3K, p-AKT, AKT, and β-catenin protein expressions by Western blot in 8505C cells and CAL-62 cells after transfection (A); comparison of p-PI3K/PI3K, p-AKT/AKT, and β-catenin expressions among groups in 8505C cells (B) and CAL-62 cells (C) after transfection by one-way ANOVA followed by Dunnett’s post-hoc test. ATC, anaplastic thyroid carcinoma; siRNA, small interfering RNA; AKIP1, A-kinase interacting protein 1; NS, not significant; PI3K, phosphatidylinositol-3-kinase; AKT, protein kinase B; GAPDH, glyceraldehyde-3-phosphate dehydrogenase; *p < 0.05; **p < 0.01.
Relative cell viability was declined along with the increasing concentration of doxorubicin in 8505C and CAL-62 cells; meanwhile, the IC50 concentration of doxorubicin was identified as 1.77 μM in 8505C cells and 0.16 μM in CAL-62 cells (Figures 4A, B). Furthermore, 1.6 μM doxorubicin was used to treat 8505C cells and 0.16 μM doxorubicin was used to treat CAL-62 cells after transfection; data showed that AKIP1 knockdown decreased relative cell viability in 8505C cells and CAL-62 cells under doxorubicin treatment (both p < 0.05) (Figures 4C, D). In addition, AKIP1 knockdown declined relative cell viability in 0.8 μM, 1.6 μM, and 3.2 μM doxorubicin-treated 8505C cells; meanwhile, AKIP1 knockdown decreased relative cell viability in 0.04 μM, 0.08 μM, 0.16 μM, and 0.32 μM doxorubicin-treated CAL-62 cells (all p < 0.05) (Supplementary Figures 3A, B). To explore whether AKIP1 participated in drug resistance of ATC, 8505C/Dox cells with an IC50 concentration of 18.2 μM were established (Figure 4E). Furthermore, AKIP1 was elevated in 8505C/Dox cells compared to 8505C cells (both p < 0.05) (Figures 4F–H).
Figure 4 AKIP1 involved in doxorubicin sensitivity in ATC cell lines. Relative cell viability of 8505C cells (A) and CAL-62 cells (B) under different concentrations of doxorubicin treatment without siRNA transfection; comparison of relative cell viability between groups in 8505C cells after siRNA transfection under 1.6 μM doxorubicin treatment (C) and CAL-62 cells after siRNA transfection under 0.16 μM doxorubicin treatment (D); relative cell viability under different concentrations of doxorubicin treatment in 8505C/Dox cells without siRNA transfection (E); comparison of AKIP1 mRNA expression between 8505C cells and 8505C/Dox cells without siRNA transfection or doxorubicin treatment (F); detection of AKIP1 protein expression by Western blot in 8505C cells and 8505C/Dox cells without siRNA transfection or doxorubicin treatment (G); comparison of AKIP1 protein expression between 8505C cells and 8505C/Dox cells without siRNA transfection or doxorubicin treatment (H) by one-way ANOVA followed by Dunnett’s post-hoc test and Student’s t-test. ATC, anaplastic thyroid carcinoma; AKIP1, A-kinase interacting protein 1; Dox, doxorubicin; IC50, half maximal inhibitory concentration; *p < 0.05; **p < 0.01.
To further explore the effect of AKIP1 on drug resistance in ATC cell lines, 8505C cells were transfected with AKIP1 or NC overexpression plasmids; meanwhile, 8505C/Dox cells were transfected with AKIP1 siRNA or NC siRNA; data showed that AKIP1 was elevated in 8505C cells transfected with the AKIP1 overexpression plasmid compared to those transfected with the NC overexpression plasmid (both p < 0.001), while AKIP1 was declined in 8505C/Dox cells transfected with AKIP1 siRNA compared to NC siRNA (both p < 0.01) (Figures 5A–E). Moreover, AKIP1 overexpression elevated relative cell viability in 0.8 μM, 1.6 μM, 3.2 μM, and 6.4 μM doxorubicin-treated 8505C cells; moreover, AKIP1 knockdown decreased relative cell viability in 8 μM, 16 μM, 32 μM, and 64 μM doxorubicin-treated 8505C/Dox cells, as well as promoted the doxorubicin sensitivity in 8505C/Dox cells (IC50 in normal cells: 18.50 μm, IC50 in siRNA-AKIP1 cells: 10.05 μm) (Figures 5F, G).
Figure 5 AKIP1 modification regulated ATC cell viability under different concentrations of doxorubicin. Comparison of AKIP1 mRNA expression among groups in 8505C cells (A) and 8505C/Dox (B) after AKIP1 modification; detection of AKIP1 protein expression by Western blot in 8505C cells and 8505C/Dox after AKIP1 modification (C); comparison of AKIP1 protein expression among groups in 8505C cells (D) and 8505C/Dox (E) after AKIP1 modification; comparison of relative cell viability among groups in 8505C cells after AKIP1 modification under 0–6.4 μM doxorubicin treatment (F) and 8505C/Dox after AKIP1 modification under 0–64 μM doxorubicin treatment (G) by one-way ANOVA followed by Dunnett’s post-hoc test. ATC, anaplastic thyroid carcinoma; AKIP1, A-kinase interacting protein 1; Dox, doxorubicin; IC50, half maximal inhibitory concentration; siRNA, small interfering RNA; NC, negative control *p < 0.05; **p < 0.01; ***p < 0.001.
To further verify the effect of AKIP1 on drug resistance in ATC cell lines, 8505C cells after transfection were treated by 1.6 μM doxorubicin and 8505C/Dox cells after transfection were treated by 16 μM doxorubicin. Data presented that AKIP1 overexpression elevated relative cell viability and invasive cell count, but decreased apoptosis rate in doxorubicin-treated 8505C cells (all p < 0.05); furthermore, AKIP1 knockdown decreased relative cell viability and invasive cell count, but elevated apoptosis rate in doxorubicin-treated 8505C/Dox cells (all p < 0.05) (Figures 6A–J). Moreover, AKIP1 overexpression increased p-AKT and β-catenin in doxorubicin-treated 8505C cells, while AKIP1 knockdown decreased p-PI3K, p-AKT, and β-catenin in doxorubicin-treated 8505C/Dox cells (all p < 0.05) (Figures 7A–D). In addition, AKIP1 overexpression increased the nuclear translocation of β-catenin in doxorubicin-treated 8505C cells, while AKIP1 knockdown declined the nuclear translocation of β-catenin in doxorubicin-treated 8505C/Dox cells (both p < 0.05) (Supplementary Figures 4A–C).
Figure 6 ATC cellular functions after AKIP1 modification and doxorubicin treatment. Comparison of relative cell viability among groups in 8505C cells (A) and 8505C/Dox cells (B) after AKIP1 modification and doxorubicin treatment; comparison of apoptosis rate among groups in 8505C cells (C) and 8505C/Dox cells (D) after AKIP1 modification and doxorubicin treatment; comparison of invasive cell count among groups in 8505C cells (E) and 8505C/Dox cells (F) after AKIP1 modification and doxorubicin treatment by one-way ANOVA followed by Tukey’s post-hoc test; example image of cell apoptosis through TUNEL Apoptosis Assay Kit in 8505C cells (G) and 8505C/Dox cells (H) after AKIP1 modification and doxorubicin treatment; example image of cell invasion through transwell assay in 8505C cells (I) and 8505C/Dox cells (J) after AKIP1 modification and doxorubicin treatment. ATC, anaplastic thyroid carcinoma; AKIP1, A-kinase interacting protein 1; Dox, doxorubicin; NC, negative control; siRNA, small interfering RNA; TUNEL, terminal deoxynucleotidyl transferase-mediated dUTP-biotin nick end labeling; NS, not significant; *p < 0.05; **p < 0.01; ***p < 0.001.
Figure 7 PI3K/AKT and β-catenin pathways after AKIP1 modification and doxorubicin treatment in ATC cell lines. Detection of p-PI3K, PI3K, p-AKT, AKT, and β-catenin protein expressions by Western blot in 8505C cells (A) and 8505C/Dox cells (B) after AKIP1 modification and doxorubicin treatment; comparison of p-PI3K/PI3K, p-AKT/AKT, and β-catenin expressions among groups in 8505C cells (C) and 8505C/Dox cells (D) after AKIP1 modification and doxorubicin treatment by one-way ANOVA followed by Tukey’s post-hoc test. ATC, anaplastic thyroid carcinoma; AKIP1, A-kinase interacting protein 1; Dox, doxorubicin; NS, not significant; NC, negative control; siRNA, small interfering RNA; PI3K, phosphatidylinositol-3-kinase; AKT, protein kinase B; GAPDH, glyceraldehyde-3-phosphate dehydrogenase; *, p < 0.05; **, p < 0.01.
ATC accounts for only 2% of all thyroid carcinomas; although it is rare, ATC is viewed as one of the invariably lethal diseases worldwide (1–3). Until now, the prognosis of ATC is relatively poor due to the characteristics of ATC, such as aggressive nature and metastasis at an early stage (4–6). Moreover, partly because ATC lacks thyroid-like phenotype and function, the treatment option of ATC is very limited; meanwhile, ATC usually presents an unfavorable response rate to chemotherapy and other available therapies (21). Given that ATC is a worldwide threat and extremely hard to manage, exploring the potential target to improve treatment is crucial. In the current study, we explored the interaction of AKIP1 with chemosensitivity and pathways in ATC. Interestingly, we discovered that AKIP1 knockdown could inhibit ATC cell survival and invasion while enhancing doxorubicin sensitivity via inhibiting PI3K/AKT and β-catenin pathways.
Over the past decades, AKIP1 has drawn a lot of attention in oncology research, due to its important biological role in multiple malignancies (8, 19, 22, 23). For instance, AKIP1 promotes breast cancer cell motility via suppressing Akt/glycogen synthase kinase3β/Snail pathways (22); moreover, AKIP1 elevates hepatocellular carcinoma (HCC) metastasis through Wnt/β-catenin/cyclic AMP response element-binding protein pathways (19); furthermore, AKIP1 facilitates gastric cancer metastasis and cell growth and through activating epithelial–mesenchymal transition (23); additionally, AKIP1 accelerates glioblastoma viability, mobility, and chemoradiation resistance via the NF-κB pathway (12); meanwhile, AKIP1 is also considered as a molecular determinant of protein kinase in the NF-κB pathway (24); furthermore, AKIP1 is able to bind the p65 subunit of NF-κB and modulate its transcriptional activity (25). Thus, combined with previous studies, we hypothesize that AKIP1 also takes part in the pathophysiology of ATC, while the information about this issue is scarce. In the current study, we disclosed that AKIP1 was elevated in ATC cell lines compared to the human normal thyroid cell line; meanwhile, we also found that AKIP1 knockdown elevated apoptosis but inhibited relative cell viability and cell invasion in ATC cell lines, which was consistent with the results of previous research about other malignancies (8, 19, 22, 23). The possible explanations might be that (1) AKIP1 knockdown could inhibit ATC cell survival and invasion through several approaches (such as inactivating slug-induced epithelial–mesenchymal transition and downregulating CXC-chemokines) (8, 23) and (2) AKIP1 knockdown might decrease ATC cell survival and invasion through modulating PI3K/AKT and β-catenin pathways (results of our subsequent research).
The PI3K/AKT pathway is viewed as a vital modulator among numerous cancers, such as breast cancer and lung adenocarcinoma (26–30). Importantly, a variety of previous studies suggest that the PI3K/AKT pathway also plays an important role in tumor progression and drug resistance in ATC (31–33). For instance, inhibition of the PI3K/AKT pathway suppresses ATC aggressiveness, such as decreasing cell invasion and survival (31–34); meanwhile, inactivating the PI3K/AKT pathway elevates chemosensitivity in ATC (35). In addition, β-catenin dysregulation is usually found in different malignancies, including ATC (32, 36, 37). For instance, β-catenin inactivation declined cell survival and invasiveness in ATC (31, 36, 38); moreover, β-catenin inactivation is able to elevate iodine uptake in ATC (36). In addition, AKIP1 is reported to regulate PI3K/AKT and β-catenin pathways in several malignancies (12, 19, 20). Inspired by previous data, in the current study, we explored the interaction of AKIP1 with PI3K/AKT and β-catenin pathways in ATC, and data showed that AKIP1 knockdown inactivated PI3K/AKT and β-catenin pathways in ATC cell lines.
It is a big challenge for chemoresistance to elevate outcome among ATC patients, which is regulated by several pathways, including PI3K/AKT and β-catenin pathways (6, 21, 26, 36). Moreover, AKIP1 is proposed to modulate chemoresistance in several cancers (11, 12). Thus, we hypothesized that AKIP1 could regulate chemosensitivity through PI3K/AKT and β-catenin pathways in ATC. In the current research, we chose doxorubicin in the chemosensitivity assay, because doxorubicin is the main cornerstone recommended by the National Comprehensive Cancer Network guideline for the treatment of ATC (39, 40). Surprisingly, we discovered that AKIP1 knockdown elevated apoptosis rate, decreased relative cell viability and invasive cell count, and inactivated PI3K/AKT and β-catenin pathways in chemo-resistant ATC cells under doxorubicin treatment, indicating that AKIP1 knockdown restored doxorubicin sensitivity via inhibiting PI3K/AKT and β-catenin pathways in ATC. The possible reasons might be that (1) AKIP1 knockdown could decrease excretion of C-X-C motif chemokine ligand families, which consequently elevated chemosensitivity (19, 20, 31) and (2) AKIP1 knockdown could inactivate PI3K/AKT and β-catenin pathways to decline stemness, which indirectly elevated chemosensitivity (41–43).
To be conclusive, AKIP1 knockdown decreases cell survival and invasion, while restoring doxorubicin sensitivity through inactivating PI3K/AKT and β-catenin pathways in ATC, indicating that AKIP1 may be a potential target to improve the treatment of ATC.
The datasets presented in this study can be found in online repositories. The names of the repository/repositories and accession number(s) can be found in the article/Supplementary Material.
HZ and QL designed the research study. HZ, QL, and YR performed the research. YR provided help and advice on administration. HZ and QL analyzed the data. HZ, QL, and YR wrote the manuscript. All authors contributed to editorial changes in the manuscript. All authors read and approved the final manuscript.
This work was supported by the Project of Biobank (No.YBKB201911) from Shanghai Ninth People’s Hospital, Shanghai Jiao Tong University School of Medicine and the Cross Disciplinary Research Projects of Ninth People's Hospital, Shanghai Jiao Tong University School of Medicine (JYJC202106).
The authors declare that the research was conducted in the absence of any commercial or financial relationships that could be construed as a potential conflict of interest.
All claims expressed in this article are solely those of the authors and do not necessarily represent those of their affiliated organizations, or those of the publisher, the editors and the reviewers. Any product that may be evaluated in this article, or claim that may be made by its manufacturer, is not guaranteed or endorsed by the publisher.
The Supplementary Material for this article can be found online at: https://www.frontiersin.org/articles/10.3389/fonc.2022.854702/full#supplementary-material
1. Yang J, Barletta JA. Anaplastic Thyroid Carcinoma. Semin Diagn Pathol (2020) 37(5):248–56. doi: 10.1053/j.semdp.2020.06.005
2. Lim AM, Solomon BJ. Immunotherapy for Anaplastic Thyroid Carcinoma. J Clin Oncol (2020) 38(23):2603–4. doi: 10.1200/JCO.20.01437
3. Molinaro E, Romei C, Biagini A, Sabini E, Agate L, Mazzeo S, et al. Anaplastic Thyroid Carcinoma: From Clinicopathology to Genetics and Advanced Therapies. Nat Rev Endocrinol (2017) 13(11):644–60. doi: 10.1038/nrendo.2017.76
4. Saini S, Tulla K, Maker AV, Burman KD, Prabhakar BS. Therapeutic Advances in Anaplastic Thyroid Cancer: A Current Perspective. Mol Cancer (2018) 17(1):154. doi: 10.1186/s12943-018-0903-0
5. Rao SN, Zafereo M, Dadu R, Busaidy NL, Hess K, Cote GJ, et al. Patterns of Treatment Failure in Anaplastic Thyroid Carcinoma. Thyroid (2017) 27(5):672–81. doi: 10.1089/thy.2016.0395
6. Perrier ND, Brierley JD, Tuttle RM. Differentiated and Anaplastic Thyroid Carcinoma: Major Changes in the American Joint Committee on Cancer Eighth Edition Cancer Staging Manual. CA Cancer J Clin (2018) 68(1):55–63. doi: 10.3322/caac.21439
7. Keprova A, Korinkova L, Krizova I, Hadravova R, Kaufman F, Pichova I, et al. Various AKIP1 Expression Levels Affect its Subcellular Localization But Have No Effect on NF-kappaB Activation. Physiol Res (2019) 68(3):431–43. doi: 10.33549/physiolres.933961
8. Zhang W, Wu Q, Wang C, Yang L, Liu P, Ma C. AKIP1 Promotes Angiogenesis and Tumor Growth by Upregulating CXC-Chemokines in Cervical Cancer Cells. Mol Cell Biochem (2018) 448(1-2):311–20. doi: 10.1007/s11010-018-3335-7
9. Lin C, Song L, Liu A, Gong H, Lin X, Wu J, et al. Overexpression of AKIP1 Promotes Angiogenesis and Lymphangiogenesis in Human Esophageal Squamous Cell Carcinoma. Oncogene (2015) 34(3):384–93. doi: 10.1038/onc.2013.559
10. Guo X, Zhao L, Cheng D, Mu Q, Kuang H, Feng K. AKIP1 Promoted Epithelial-Mesenchymal Transition of non-Small-Cell Lung Cancer via Transactivating ZEB1. Am J Cancer Res (2017) 7(11):2234–44.
11. Leung TH, Ngan HY. Interaction of TAp73 and Breast Cancer-Associated Gene 3 Enhances the Sensitivity of Cervical Cancer Cells in Response to Irradiation-Induced Apoptosis. Cancer Res (2010) 70(16):6486–96. doi: 10.1158/0008-5472.CAN-10-0688
12. Han D, Zhang N, Zhao S, Liu H, Wang X, Yang M, et al. AKIP1 Promotes Glioblastoma Viability, Mobility and Chemoradiation Resistance via Regulating CXCL1 and CXCL8 Mediated NF-kappaB and AKT Pathways. Am J Cancer Res (2021) 11(4):1185–205.
13. Zhang L, Tao H, Ke K, Ma C. A-Kinase Interacting Protein 1 as a Potential Biomarker of Advanced Tumor Features and Increased Recurrence Risk in Papillary Thyroid Carcinoma Patients. J Clin Lab Anal (2020) 34(10):e23452. doi: 10.1002/jcla.23452
14. Liang Y, Song X, Li Y, Su P, Han D, Ma T, et al. Circkdm4c Suppresses Tumor Progression and Attenuates Doxorubicin Resistance by Regulating miR-548p/PBLD Axis in Breast Cancer. Oncogene (2019) 38(42):6850–66. doi: 10.1038/s41388-019-0926-z
15. Garg M, Kanojia D, Mayakonda A, Ganesan TS, Sadhanandhan B, Suresh S, et al. Selinexor (KPT-330) has Antitumor Activity Against Anaplastic Thyroid Carcinoma In Vitro and In Vivo and Enhances Sensitivity to Doxorubicin. Sci Rep (2017) 7(1):9749. doi: 10.1038/s41598-017-10325-x
16. Lin B, Lu B, Hsieh IY, Liang Z, Sun Z, Yi Y, et al. Synergy of GSK-J4 With Doxorubicin in KRAS-Mutant Anaplastic Thyroid Cancer. Front Pharmacol (2020) 11:632. doi: 10.3389/fphar.2020.00632
17. Tian S, Lou L, Tian M, Lu G, Tian J, Chen X. MAPK4 Deletion Enhances Radiation Effects and Triggers Synergistic Lethality With Simultaneous PARP1 Inhibition in Cervical Cancer. J Exp Clin Cancer Res (2020) 39(1):143. doi: 10.1186/s13046-020-01644-5
18. Li R, Wan T, Qu J, Yu Y, Zheng R. Long non-Coding RNA DLEUI Promotes Papillary Thyroid Carcinoma Progression by Sponging miR-421 and Increasing ROCK1 Expression. Aging (Albany NY) (2020) 12(20):20127–38. doi: 10.18632/aging.103642
19. Cui Y, Wu X, Lin C, Zhang X, Ye L, Ren L, et al. AKIP1 Promotes Early Recurrence of Hepatocellular Carcinoma Through Activating the Wnt/beta-Catenin/CBP Signaling Pathway. Oncogene (2019) 38(27):5516–29. doi: 10.1038/s41388-019-0807-5
20. Zhang X, Liu S, Zhu Y. A-Kinase-Interacting Protein 1 Promotes EMT and Metastasis via PI3K/Akt/IKKbeta Pathway in Cervical Cancer. Cell Biochem Funct (2020) 38(6):782–91. doi: 10.1002/cbf.3547
21. Amaral M, Afonso RA, Gaspar MM, Reis CP. Anaplastic Thyroid Cancer: How Far can We Go? EXCLI J (2020) 19:800–12. doi: 10.17179/excli2020-1302
22. Mo D, Li X, Li C, Liang J, Zeng T, Su N, et al. Overexpression of AKIP1 Predicts Poor Prognosis of Patients With Breast Carcinoma and Promotes Cancer Metastasis Through Akt/GSK-3beta/Snail Pathway. Am J Transl Res (2016) 8(11):4951–9.
23. Chen D, Cao G, Liu Q. A-Kinase-Interacting Protein 1 Facilitates Growth and Metastasis of Gastric Cancer Cells via Slug-Induced Epithelial-Mesenchymal Transition. J Cell Mol Med (2019) 23(6):4434–42. doi: 10.1111/jcmm.14339
24. Gao N, Hibi Y, Cueno M, Asamitsu K, Okamoto T. A-Kinase-Interacting Protein 1 (AKIP1) Acts as a Molecular Determinant of PKA in NF-kappaB Signaling. J Biol Chem (2010) 285(36):28097–104. doi: 10.1074/jbc.M110.116566
25. King CC, Sastri M, Chang P, Pennypacker J, Taylor SS. The Rate of NF-kappaB Nuclear Translocation is Regulated by PKA and A Kinase Interacting Protein 1. PLoS One (2011) 6(4):e18713. doi: 10.1371/journal.pone.0018713
26. Milosevic Z, Pesic M, Stankovic T, Dinic J, Milovanovic Z, Stojsic J, et al. Targeting RAS-MAPK-ERK and PI3K-AKT-mTOR Signal Transduction Pathways to Chemosensitize Anaplastic Thyroid Carcinoma. Transl Res (2014) 164(5):411–23. doi: 10.1016/j.trsl.2014.06.005
27. Yang J, Nie J, Ma X, Wei Y, Peng Y, Wei X. Targeting PI3K in Cancer: Mechanisms and Advances in Clinical Trials. Mol Cancer (2019) 18(1):26. doi: 10.1186/s12943-019-0954-x
28. Aoki M, Fujishita T. Oncogenic Roles of the PI3K/AKT/mTOR Axis. Curr Top Microbiol Immunol (2017) 407:153–89. doi: 10.1007/82_2017_6
29. Yan W, Ma X, Zhao X, Zhang S. Baicalein Induces Apoptosis and Autophagy of Breast Cancer Cells via Inhibiting PI3K/AKT Pathway In Vivo and Vitro. Drug Des Devel Ther (2018) 12:3961–72. doi: 10.2147/DDDT.S181939
30. Yu M, Qi B, Xiaoxiang W, Xu J, Liu X. Baicalein Increases Cisplatin Sensitivity of A549 Lung Adenocarcinoma Cells via PI3K/Akt/NF-kappaB Pathway. BioMed Pharmacother (2017) 90:677–85. doi: 10.1016/j.biopha.2017.04.001
31. Kim SH, Kang JG, Kim CS, Ihm SH, Choi MG, Yoo HJ, et al. 17-Allylamino-17-Demethoxygeldanamycin and Herbimycin A Induce Cell Death by Modulating Beta-Catenin and PI3K/AKT Signaling in FRO Anaplastic Thyroid Carcinoma Cells. Anticancer Res (2015) 35(10):5453–60.
32. Kim SH, Kang JG, Kim CS, Ihm SH, Choi MG, Yoo HJ, et al. CCAAT/Enhancer-Binding Protein-Homologous Protein Sensitizes to SU5416 by Modulating P21 and PI3K/Akt Signal Pathway in FRO Anaplastic Thyroid Carcinoma Cells. Horm Metab Res (2013) 45(1):9–14. doi: 10.1055/s-0032-1323680
33. Xing M. Genetic Alterations in the Phosphatidylinositol-3 Kinase/Akt Pathway in Thyroid Cancer. Thyroid (2010) 20(7):697–706. doi: 10.1089/thy.2010.1646
34. Nozhat Z, Mohammadi-Yeganeh S, Azizi F, Zarkesh M, Hedayati M. Effects of Metformin on the PI3K/AKT/FOXO1 Pathway in Anaplastic Thyroid Cancer Cell Lines. Daru (2018) 26(2):93–103. doi: 10.1007/s40199-018-0208-2
35. Francipane MG, Eterno V, Spina V, Bini M, Scerrino G, Buscemi G, et al. Suppressor of Cytokine Signaling 3 Sensitizes Anaplastic Thyroid Cancer to Standard Chemotherapy. Cancer Res (2009) 69(15):6141–8. doi: 10.1158/0008-5472.CAN-09-0994
36. Lan L, Basourakos S, Cui D, Zuo X, Deng W, Huo L, et al. ATRA Increases Iodine Uptake and Inhibits the Proliferation and Invasiveness of Human Anaplastic Thyroid Carcinoma SW1736 Cells: Involvement of Beta-Catenin Phosphorylation Inhibition. Oncol Lett (2017) 14(6):7733–8. doi: 10.3892/ol.2017.7225
37. Valenta T, Hausmann G, Basler K. The Many Faces and Functions of Beta-Catenin. EMBO J (2012) 31(12):2714–36. doi: 10.1038/emboj.2012.150
38. Sastre-Perona A, Santisteban P. Wnt-Independent Role of Beta-Catenin in Thyroid Cell Proliferation and Differentiation. Mol Endocrinol (2014) 28(5):681–95. doi: 10.1210/me.2013-1377
39. Smallridge RC, Ain KB, Asa SL, Bible KC, Brierley JD, Burman KD, et al. American Thyroid Association Guidelines for Management of Patients With Anaplastic Thyroid Cancer. Thyroid (2012) 22(11):1104–39. doi: 10.1089/thy.2012.0302
40. Haddad RI, Lydiatt WM, Ball DW, Busaidy NL, Byrd D, Callender G, et al. Anaplastic Thyroid Carcinoma, Version 2.2015. J Natl Compr Canc Netw (2015) 13(9):1140–50. doi: 10.6004/jnccn.2015.0139
41. Madsen RR. PI3K in Stemness Regulation: From Development to Cancer. Biochem Soc Trans (2020) 48(1):301–15. doi: 10.1042/BST20190778
42. Basu S, Cheriyamundath S, Ben-Ze'ev A. Cell-Cell Adhesion: Linking Wnt/beta-Catenin Signaling With Partial EMT and Stemness Traits in Tumorigenesis. F1000Res (2018) 7:F1000 Faculty Rev-1488. doi: 10.12688/f1000research.15782.1
Keywords: AKIP1, anaplastic thyroid carcinoma, doxorubicin sensitivity, cell malignant behaviors, PI3K/AKT and β-catenin pathways
Citation: Zheng H, Lin Q and Rao Y (2022) A-Kinase Interacting Protein 1 Knockdown Restores Chemosensitivity via Inactivating PI3K/AKT and β-Catenin Pathways in Anaplastic Thyroid Carcinoma. Front. Oncol. 12:854702. doi: 10.3389/fonc.2022.854702
Received: 14 January 2022; Accepted: 19 April 2022;
Published: 28 July 2022.
Edited by:
Gary Piazza, Auburn University, United StatesReviewed by:
Rozita Bagheri-Yarmand, University of Texas MD Anderson Cancer Center, United StatesCopyright © 2022 Zheng, Lin and Rao. This is an open-access article distributed under the terms of the Creative Commons Attribution License (CC BY). The use, distribution or reproduction in other forums is permitted, provided the original author(s) and the copyright owner(s) are credited and that the original publication in this journal is cited, in accordance with accepted academic practice. No use, distribution or reproduction is permitted which does not comply with these terms.
*Correspondence: Yamin Rao, cmFveWF5YTIwMDZAMTI2LmNvbQ==
†These authors have contributed equally to this work
Disclaimer: All claims expressed in this article are solely those of the authors and do not necessarily represent those of their affiliated organizations, or those of the publisher, the editors and the reviewers. Any product that may be evaluated in this article or claim that may be made by its manufacturer is not guaranteed or endorsed by the publisher.
Research integrity at Frontiers
Learn more about the work of our research integrity team to safeguard the quality of each article we publish.