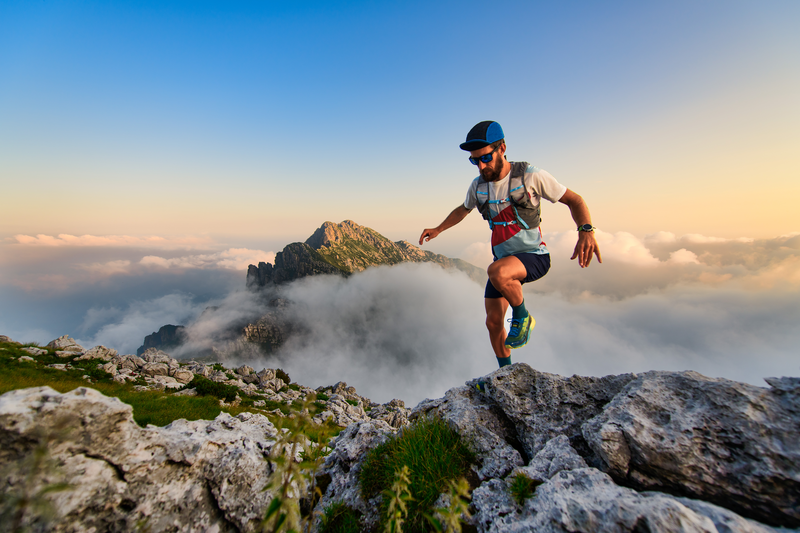
95% of researchers rate our articles as excellent or good
Learn more about the work of our research integrity team to safeguard the quality of each article we publish.
Find out more
REVIEW article
Front. Oncol. , 25 May 2022
Sec. Cancer Imaging and Image-directed Interventions
Volume 12 - 2022 | https://doi.org/10.3389/fonc.2022.854658
This article is part of the Research Topic Cellular Metabolism and Molecular Imaging in Cancer View all 7 articles
Fibroblast activation protein (FAP), a type II transmembrane serine protease, is highly expressed in more than 90% of epithelial tumors and is closely associated with various tumor invasion, metastasis, and prognosis. Using FAP as a target, various FAP inhibitors (FAPIs) have been developed, most of which have nanomolar levels of FAP affinity and high selectivity and are used for positron emission tomography (PET) imaging of different tumors. We have conducted a systematic review of the available data; summarized the biological principles of FAPIs for PET imaging, the synthesis model, and metabolic characteristics of the radiotracer; and compared the respective values of FAPIs and the current mainstream tracer 18F-Fludeoxyglucose (18F-FDG) in the clinical management of tumor and non-tumor lesions. Available research evidence indicates that FAPIs are a molecular imaging tool complementary to 18F-FDG and are expected to be the new molecule of the century with better imaging effects than 18F-FDG in a variety of cancers, including gastrointestinal tumors, liver tumors, breast tumors, and nasopharyngeal carcinoma.
Malignant tumors are the second leading cause of patient death worldwide (1). The tumor stroma is an important component of the tumor microenvironment (TME), where cancer cell-autonomous mutations (and other alterations) combine with changes in the tumor stroma to drive tumorigenesis, progression, metastasis, and treatment resistance formation. The tumor stroma consists of specialized connective tissue cells, including fibroblasts, mesenchymal stromal cells (MSCs), osteoblasts, and chondrocytes, as well as the extracellular matrix (ECM). Cells in the non-malignant stroma are usually quiescent and maintain a balance between the ECM and the epithelial cell zone. When cancer develops, when cancer develops, the stroma undergoes vast changes to become fibrotic and activated, and fibroblasts and MSCs become more proliferative, secreting higher levels of growth factors, cytokines, and chemokines, with fibroblasts acting as sentinel cells that often begin to activate and accumulate early in the lesion; fibroblasts are called cancer-associated fibroblasts (CAFs), myofibroblasts are further differentiated fibroblasts that are present during wound healing or other processes that cause matrix remodeling, and in cancer, and CAFs often resemble myofibroblasts due to the presence of activated stroma (2). Fibroblast activation protein (FAP), which is highly expressed in CAFs but largely absent in normal tissues, is one of the specific markers of CAFs in TME and is closely related to tumor invasion, metastasis, angiogenesis, and prognosis and is considered an essential target for the diagnosis and treatment of malignant tumors (3–5). We searched PubMed, MEDLINE, and Scopus databases for one or more combinations of the following terms: “cancer-associated fibroblasts”, “fibroblast activating protein”, “FAPI”, “FAPI-PET”, “fibroblast activation protein inhibitor”, “FAPI”, and “FAPI-PET”. All English-language papers in the search return results were evaluated and included if they fell within the scope of this review.
Originally known as the F19 antigen, FAP is a type II transmembrane serine protease consisting of 761 amino acids in a dimer consisting of an α subunit of 95 kDa and a β subunit of 105 kDa with collagenase activity that breaks down gelatin and type I collagen as well as a dipeptidyl peptidase (DPP)–like activity (6). FAP belongs to the DPP subfamily, which includes DPP4, quiescent cell proline dipeptidase (QPP), FAP, prolyl oligopeptidase (POP), DPP8, DPP, DPP6, and DPP10 (7). Among them, FAP has 50% amino acid sequence homology and 70% homology of catalytic structural domain with DPP4, and they can form heterodimerization complexes to synergistically regulate the growth, differentiation, adhesion, and metastasis of tumor cells (8). FAP is highly expressed in the stroma surrounding more than 90% of epithelial-derived tumors and their metastases and is consistently expressed in bone and soft tissue tumor cells. It may also be transiently expressed in fetal mesenchymal tissues, healing wounds after endometrial detachment in adults, and organ reconstruction sites; however, it is usually not expressed in normal tissues, benign lesions, and precancerous tissues (9, 10). Studies have shown that a large amount of tumor mesenchyme (50%–90%) is present in most malignant lesions, and high expression of FAP is closely associated with invasion, metastasis, and poor prognosis of these malignant tumors, suggesting that FAP is promising as an essential target for malignant tumor imaging and treatment (11, 12).
Imaging studies of malignancies targeting FAP are performed by linking FAP inhibitors (FAPIs) or FAP antibodies to radionuclides using chelators followed by positron emission tomography/computed tomography (PET/CT) imaging, PET/magnetic resonance imaging, or single-photon emission computed tomography (SPECT) imaging. Much of the early work on FAPIs focused on pyrrolidine-2-boronic acid derivatives, of which the first pyrrolidine-2-boronic acid derivative to be clinically tested was Val-boroPro (Talabostat, PT-100), which exhibited a significant affinity for one or more members of the DPP subfamily members but are not very selective for FAP (13). In addition, a small-molecule antibody B12 IgG targeting the extracellular structural domain of FAP is being used in preclinical studies for prostate imaging, showing that the considerable molecular weight of the FAP antibody results in slow clearance in vivo, resulting in high background signal and limited sensitivity for focal detection (14). Small-molecule FAPIs (UAMC-1110) based on the 4-quinolinyl-glycyl-2-cyanopyrrolidine framework overcome the disadvantages of pyrrolidine-2-boronic acid derivatives FAP antibody B12 IgG due to their simultaneous nanomolar affinity for FAP and high selectivity (15). Much of the current work in FAPI research has focused on the chemical modification of quinoline-based small-molecule inhibitors to obtain derivatives with higher affinity for FAP and superior pharmacokinetics. Several derivatives have shown promising clinical results, including FAPI-02 (16), FAPI-04 (17), FAPI-46 (18), FAPI-34 (19), FAPI-74 (20), DOTA.SA.FAPI and DATA5m.SA.FAPI (21), DOTA-2P(FAPI)2 (22), and FAPI-42 (23). Many derivatives are being developed, and some promising preclinical data have been obtained, such as 18F-fluoroglycosylation-FAPI (18F-FGlc-FAPI) (24), QCP01 and QCP02 (25), and AAZTA5.SA.FAPI (26) (Table 1).
Jiang et al. attempted to label NOTA-FAPI-04 with [18F]AlF, the radioactivity per batch was 9.095 ± 0.587 GBq and the radiochemical yield (RCY) was 26.4 ± 1.5%, and the radiotracer obtained by the automated synthesis equipment was used for PET/CT imaging of a patient with liver metastases from invasive cholangiocarcinoma (IDC). The images showed high tumor-to-background uptake ratios (TBRs) of 8.44 (28). Dahl et al. successfully produced good manufacturing practice (GMP)–compliant 18F-FAPI-74 in the GE TRACERlab FX2N module and the cassette-based module Trasis AllInOne (AIO) in radioactivity per batch of 6410 MBq and 6900 MBq, respectively, with the RCY of about 20% (29). Naka et al. performed a proof-of-concept automated synthesis of [18F] AlF-FAPI-74 with one-pot and one-step by a multi-purpose synthesizer CFN-MPS200; the radioactivity per batch was 11.3 ± 1.1 GBq, and the RCY was 37.0% ± 4.3% (30).
FAPIs coupled to radionuclide 68Ga via DOTA chelator are mainly FAPI-04, FAPI-46, FAPI-74, DOTA-2P(FAPI)2, DOTA.SA.FAPI, and DATA5m.SA.FAPI.
FAPI-04 is a small-molecule FAPI derivative that has been chemically modified to provide better imaging results, with effective doses of 1.64E-02mSv/MBq. Kratochwil et al. performed 68Ga-FAPI-02 and 68Ga-FAPI-04 PET/CT imaging on 80 patients and obtained uptake data for tracers of 28 types of tumors (31). Among them, sarcoma, esophageal, breast, cholangiocarcinoma, and lung cancer showed high uptake rates with Maximum Standardized Uptake Value (SUVmax) >12; liver tumors and colorectal tumors, head and neck tumors, pancreatic tumors, ovarian tumors, and prostate tumors had moderate uptake rates (6 < SUVmax < 12). Pheochromocytoma, renal cell carcinoma, differentiated thyroid cancer, adenoid cyst, and gastric cancer had the lowest uptake rates (SUVmax < 6). The images from this study were named “Image of the Year” at the 2019 North American Nuclear Medicine Annual Meeting (Figure 1). Giesel et al. analyzed the biodistribution and preliminary dosimetric data of 68Ga-FAPI-02 and 68Ga-FAPI-04 in 50 patients containing 14 different types of tumors (32). Compared with 18F-Fludeoxyglucose (18F-FDG), 68Ga-FAPI showed lower uptake in the brain, liver, and oral/pharyngeal mucosa and no significant difference in tumor and other normal tissues, showing higher TBRs. Wang et al. performed the first biodistribution and internal radiation dosimetry after 68Ga-FAPI-04 injection in six Chinese adults, and the results were in general agreement with the findings of Giesel et al. (32, 33).
Figure 1 Six patients underwent intra-individual comparison of 18F-FDG-PET and 68Ga-FAPI-PET imaging over a 9-day period. Five of the six patients showed similar high tumor uptake in 18F-FDG-PET and 68Ga-FAPI-PET, and three of the six patients had more lesions detected due to low background uptake in the visceral or pharyngeal mucosa. Patients with iodine uptake negative thyroid cancer exhibited low 68Ga-FAPI tracer uptake compared with 18F-FDG-PET; Ca, cancer; NSCLC, non–small cell lung cancer (31).
FAPI-46 is another FAPI derivative with highly desirable imaging results, with effective doses of 7.80E-03mSv/MBq. The initial clinical imaging study work with FAPI-46 included eight patients, and compared with FAPI-04, 68Ga-FAPI-46 had a higher TBR and has a longer tumor retention time, making it ideal for tumor imaging and targeted therapy (18). After that, a study by Meyer et al. showed that 68Ga-FAPI-46 PET/CT has good dosimetric properties with high TBR increasing over time (34).
Zhao Let al. synthesized DOTA-2P(FAPI)2 with two FAPI-46 monomers (22). 68Ga-DOTA-2P(FAPI)2 has an effective dose of 1.19E-02mSv/MBq, higher than that of 68Ga-FAPI-46 and comparable to 68Ga-FAPI-02 (1.80E-02mSv/MBq) and 68Ga-FAPI-04. Intra-tumoral uptake of 68Ga-DOTA-2P(FAPI)2 is higher than that of 68Ga-FAPI-46 (SUVmax of 8.1–39.0 vs. 1.7–24.0) in most tumor lesions in nasopharyngeal carcinoma, papillary thyroid carcinoma, and hepatocellular liver cancer. The above studies suggest that FAPI dimers exhibit better in vivo pharmacokinetics and higher tumor uptake than FAPI monomers, leading to a more apparent contrast of primary lesions and metastases with normal tissue, thus potentially improving the sensitivity of lesion detection. However, the tracer showed higher physiological uptake in the thyroid and pancreas and prolonged retention time in the blood pool. The data from this study need to be validated with a larger sample size.
Moon et al. reported FAPI derivatives DOTA.SA.FAPI and DATA5m.SA.FAPI with IC50 of 0.7–1.4 nM; SA replaces the heterocyclic nitrogen portion of FAPI-04 to form a squaramide unit. The chelators used were macrocyclic DOTA and the hybrid chelator DATA5m (21). In animal studies, 68GaDOTA.SA.FAPI had good TBR, comparable to the previously reported animal data for FAPI-04 (21). In clinical studies, 68Ga-DATA5m.SA.FAPI PET/CT showed specific uptake in focal nodular hyperplasia in the liver (35), and there was no significant difference in tumor uptake between 68Ga-DOTA.SA.FAPI and 18F-FDG compared with 18F-FDG in 14 types of tumor imaging in a total of 54 patients, with the exception of brain metastatic lesions (27). Next, the team found that radiolabeling SA.FAPI with AAZTA5 as a chelator (i.e., AAZTA5.SA.FAPI) was superior to both DOTA and DATA5m in terms of labeling process and drug yield, and the IC50 of the product was in the low nanomolar range (0.55–0.57 nM), but the drug is still in preclinical studies (26).
With the development of 18F production equipment and labeling technology, the advantages of 18F clinical application will gradually increase: first, the maximum β+ energy of 18F is significantly lower than that of 68Ga, so the sensitivity and spatial resolution of 18F-PET imaging is better than 68Ga-PET imaging, which can improve the image contrast to a certain extent; second, 68Ga has become expensive due to a sharp increase in global demand and thus a shortage of supply (36); in addition, the 68Ge/68Ga generator is not yet capable for mass production of 68Ga due to technical limitations, which means that the automated production of large quantities of FAPIs radiotracers is limited. In an automated synthesis study of 68Ga-FAPI-46 by Spreckelmeyer et al., it was found that the radioactivity at the end of the synthesis was only 700–1,700 MBq, which was only sufficient for the examination of four to six patients (37). In comparison, the long half-life of 18F (1.13 h) supports centralized commercial production and distribution to customers with no 18F production facilities, resulting in a significant reduction in production costs (38). The development of low-power benchtop cyclotrons has made 18F dispersion production easier (automated, low cost, and on-demand production); the 18F labeling of peptides has become more efficient due to the development of chelator and labeling technologies. Currently, in FAPI radiotracer research, an increasing number of researchers are experimenting with 18F labeling of FAPIs to develop radiopharmaceuticals that can meet the global demand for large-scale assays, especially in developing countries. For example, 18F-FAPI-74 and 18F-NOTA-FAPI-04 showed good visualization in clinical studies, and 18F-FGlc-FAPI-04 showed superior performance in in vitro experiments (20, 23, 24).
Giesel et al. applied 18F-FAPI-74 and 68Ga-FAPI-74 for the first time in clinical practice and successfully attached [18F]AlF to FAPI via 1,4,7-triazacyclonane-N,N′,N′-triacetin acid (NOTA) for the first time and showed satisfactory imaging results. A total of 10 patients with non–small cell lung cancer in this study received 68Ga-FAPI-74 and 18F-FAPI-74 imaging within 1 week. The data showed that the best tumor-to-background contrast presents 1 h after injection, and the estimated radiation dose of 18F-FAPI-74 was lower than that of other 18F-labeled PET scanner tracers, and there was no difference in tumor uptake between lung adenocarcinoma and lung squamous cell carcinoma. This study shows the value of 18F-FAPI-74 for lung cancer diagnosis and detection of small lung cancer lesions, but the number of cases is too small to analyze whether it is useful for staging and radiotherapy planning guidance (20) (Figure 2). Others have attempted to label multiple FAPIs with [18F] AlF and found that 18F-FAPI-74 gave the most favorable results in an in vitro study, and they performed a visualization study in a patient with metastatic non–small cell lung cancer and obtained conclusions similar to those of Giesel et al. (20, 39).
Figure 2 One patient with non–small cell lung cancer who developed systemic metastasis. (A) Maximum intensity projections at 10 min, 1 h, and 3 h after injection of 18F-FAPI-74 PET. (B) The uptake of 18F-FAPI-74 was significantly different between tumor and normal myocardium. (C) FAPI-positive lesions were confirmed on CT examination. (D, E) Some bone metastases were detected only by FAPI PET. All highlighted arrows represent FAPI uptake in relation to morphology (20).
18F-NOTA-FAPI-04 (18F-FAPI-42) was obtained by replacing the DOTA with chelator NOTA in FAPI-04 and Aqueous18F-Labeling by the Al18F chelation technique (21, 40). 18F-FAPI-42 was initially used to diagnose lung, pancreatic, colorectal, prostate, and lymphoma and was compared with 18F-FDG (21). The results showed that the excretion route of 18F-FAPI-42 was mainly in the urinary and biliary systems, similar to the previously reported tracers 18F-FAPI-74, 68Ga-FAPI-04, and 68Ga-FAPI-46; compared with 18F-FDG imaging, 18F-FAPI-42 had lower SUVmean in the liver, brain, spleen, and bone marrow; in addition, 18F-FAPI-42 bone uptake was low, indicating that this tracer was not defluorinated at the time of detection. Another study including a total of 22 patients with hepatocellular carcinoma, lung cancer, colorectal cancer, gastric cancer, esophageal cancer, cheek carcinosarcoma, abdominal neuroendocrine carcinoma, and clear cell carcinoma of the bladder conducted a human biodistribution study of 18F-FAPI-42 and compared it with 68Ga-FAPI-04 (40). Data from this study showed that the optimal image acquisition time for 18F-FAPI-42 was 1 h after injection; 68Ga-FAPI-42 showed higher physiological uptake in the parotid, salivary gland, thyroid, and pancreas compared with 68Ga-FAPI-04; both had equal detection rates for lesions; in addition, 18F-FAPI-42 showed higher TBRs in the liver, bone, lymph nodes, pleura, and peritoneal metastases.
18F-FGlc-FAPI-04 is an [18F]-6-fluoro-6-deoxyglycosylated FAPI radiotracer with an IC50 of 167 nM, much higher than 68Ga-FAPI-04 (32 nM), showing higher tumor uptake and longer tracer clearance times were shown in HT1080-xenografts and U87MG-xenografts. However, because the tracer is mainly eliminated via the hepatobiliary pathway and sees intraosseous and intra-articular uptake, this has hindered the entry of the tracer into clinical studies (24).
FAPI-34, QCP01[1-(6-((3-((4-((2-((S)-2-cyanopyrrolidin-1-yl)-2-oxoethyl)-carbamoyl)quinolin-6-yl)oxy)propyl)amino)-6-oxohexyl)-2-((E)-2-((E)-3-(2-((E)-3,3-dimethyl-5-(trioxidaneylthio)-1-(4-(trioxidaneylthio)butyl)indolin-2-ylidene)ethylidene)-2-(4-(trioxidaneylthio)phenoxy)cyclohex-1-en-1-yl)vinyl)-3,3-dimethyl-3H-indol-1-ium-5-sulfonate)] and QCP02(2,2′,2″-(10-(1-carboxy-4-((3-((4-((2-((S)-2-cyanopyrrolidin-1-yl)-2-oxoethyl)carbamoyl)quinolin-6-yl)oxy)propyl)amino)-4-oxobutyl)-1,4,7,10-tetraazacyclododecane-1,4,7-triyl)triacetic acid) both demonstrated low nanomolar inhibition (19, 25). FAPI-34, with an IC50 of 6.4–12.7 nM, has been used for SPECT imaging of patients with ovarian and pancreatic cancer metastases, showing good imaging results. When PET imaging is not possible due to equipment, 99mTc-FAPI-34 can be used as the imaging agent for SPECT imaging, which can also be used for diagnosis and efficacy evaluation (19). QCP01 and QCP02 were applied in tumor transplantation models with higher tumor and normal organ uptake than FAPI-02 and FAPI-04, indicating that QCP01 and QCP02 have high affinity for FAP. In addition, 111In-QCP02 SPECT imaging showed higher tumor uptake and lower kidney uptake, which is still in the preclinical study stage (25).
With FAPI-specific PET imaging, patients do not require dietary preparation, and high-quality images can be obtained early (10 min to 1 h) after tracer injection (20, 41) (Figure 3).
Figure 3 This is a case of inconsistent early and late imaging in a 54-year-old patient with metastatic pancreatic cancer. Re-staging was performed with FAPI-46 PET after resection of the primary focus. FAPI-46 PET performed 11 min after injection showed two intrahepatic metastases, one of which was not detected at 60 min of imaging (41).
In head and neck tumors, compared with 18F-FDG, it was able to improve the staging, target area contour, and prognostic assessment of oral squamous carcinoma, nasopharyngeal carcinoma, and adenoid cystic carcinoma. For example, in 45 patients with nasopharyngeal carcinoma, the detection rates of primary and metastatic foci by FDG were 97.0% and 75.2%, respectively, and the detection rates of primary and metastatic foci by FAPI-04 were 100% and 95.0%, respectively (42); FAPI-PET/CT detected more details of primary foci and lymph node metastases, resulting in a change in staging in 5 of 12 cases of adenoid cystic carcinoma (43). Among 18 patients with head and neck tumors in whom primary lesions were not detected by FDG PET/CT imaging, FAPI PET/CT detected primary lesions in 5 of them (44). However, FAPI may not be advantageous in detecting lymph nodes and distant metastases (41, 44) (Figure 4).
Figure 4 PET/CT scans of 18F-FDG (A) and 68Ga-FAPI (B) in a 41-year-old patient with lymph node metastasis from salivary gland ductal carcinoma. 18F-FDG-PET/CT was negative for the primary tumor. On 68Ga-FAPI-PET/CT, the right submandibular gland shows strong uptake (B, red arrow; SUVmax = 15.80), whereas the left submandibular gland has low background uptake (SUVmax = 6.87) (black and white arrows indicate lymph node metastases) (44).
In malignant brain lesions, cranial MRI is currently the primary imaging method for diagnosis, staging, and outlining radiotherapy target areas for high-grade gliomas in the brain. However, PET/CT and PET/MRI often provide more tumor progression and prognostic assessment (45). Conventional 18F-FDG tracers have high physiological uptake in the brain, resulting in low TBRs and obscuring accurate information about the tumor. FAPI has low physiological uptake in the brain and is highly expressed on the cell surface of high-grade gliomas (excluding diffuse astrocytomas). Clinical studies have shown high uptake of FAPI imaging agents in high-grade gliomas (SUVmax = 2.8 ± 0.6), whereas low-grade gliomas showed only mild uptake (SUVmax = 0.35 ± 0.10) (46, 47). Therefore, FAPI imaging agents may be helpful in the detection and target area sketching of high-grade gliomas in the brain, non-invasive grading of gliomas, and assessment of prognosis after radiotherapy. In addition, it showed higher TBR for brain metastases from gastric, breast, lung, and liver cancers, with a higher detection rate than18F-FDG (47–50).
FAPI PET/CT shows high uptake and high TBRs in primary and lymph node metastases of non–small cell lung cancer, and the detection rate of lymph node metastases is higher than 18F-FDG and thus may improve the staging of lung cancer and contribute to the outline of radiotherapy target areas (20, 50).
18F-FDG PET and PET/CT are less sensitive for detecting regional lymph node metastases in esophageal squamous cell carcinoma (about 66%) (51). Because of the high expression of FAP in esophageal cancer, FAPI PET shows a high uptake rate (SUVmax > 12) and high TBR in esophageal cancer and its metastases, and lymph node metastases of esophageal cancer with a false-negative 18F-FDG test are strongly positive by FAPI test, which improves the detection rate of lymph node lesions (31). The high TBR and high sensitivity of FAPI for primary foci and lymph node metastases provide more information for clinical purposes, both to improve the staging of esophageal cancer and to help outline the target volume (GTV) in precision radiotherapy. 18F-FDG has limitations in detecting certain pathological types of gastric malignancies, such as non-mesenchymal diffuse carcinoma, mucinous carcinoma, and indolent cell carcinoma (52, 53). In contrast, in some small sample studies, although FAPI showed mostly low uptake in gastric cancer, it also showed high TBR, which may still bring a turnaround for PET/CT detection of the abovementioned tumors (31). For example, in a study that included 10 patients with primary gastric tumors, the detection rates of FDG and FAPI were 50% (5/10) and 100% (10/10), respectively (47). In addition, it has been suggested that FAPI appears to be the best performing imaging agent for PET imaging of liver malignancies at present. Because of the high non-specific uptake of 18F-FDG in the liver, the false-negative rate of 18F-FDG PET in detecting HCCs is high, about 40%–50% (54). In a study of 25 patients with suspected primary liver malignancies (hepatocellular carcinoma/intrahepatic cholangiocarcinoma) on 68Ga-FAPI-04 imaging, the detection rate of primary liver malignancies was higher with FAPI than with 18F-FDG (96% vs. 65%) (55). Another study that included 20 patients with suspected primary malignancy of the liver also showed a higher sensitivity of FAPI for the diagnosis of malignancy (100% vs. 58.8%) (56).
Breast carcinoma (15%), ovarian carcinoma (5%), and uterine carcinoma (4%) are the most common gynecologic malignancies, and accurate staging is essential for treatment guidance (1). The most commonly used radiotracer for PET/CT diagnosis of gynecologic malignancies is 18F-FDG. A multicenter prospective study including 31 gynecologic malignancies showed higher TBRs in breast, ovarian, cervical, endometrial, and smooth muscle metastases; uterine smooth muscle sarcoma; and fallopian tube cancer. FAPI PET had higher TBRs in metastatic lesions than 18F-FDG [regional lymph node metastases (31.9 vs. 27.4) and distant metastases (13.0 vs. 5.7)]. However, there were significant differences in FAPI uptake between premenopausal and postmenopausal patients in the endometrium (mean SUVmax of 11.7 vs. 3.0) and breast (mean SUVmax of 1.8 vs. 1.0), which may affect the detection of lesions at these sites (57) (Figure 5).
Figure 5 A 58-year-old patient with cervical cancer underwent 68Ga-FAPI-PET/CT staging prior to radiotherapy. recurrence of cervical cancer (SUVmax = 14.4) (A). In addition, intracranial metastases showed strong FAPI uptake (SUVmax = 32.3) (B), which allowed for precise delineation of radiotherapy target areas (57).
In a case report of liver metastasis from malignant melanoma, the lesion showed moderate 68Ga-FAPI-04 uptake (SUVmax of 6.5) but was not compared with the current PET standard tracer 18F-FDG (58). In another retrospective analysis of FAPI PET/CT imaging of rare malignancies, patients with melanoma (one case) also showed more favorable imaging results (59).
In conclusion, FAPI-specific tracers simplify the clinical workflow and have significant advantages in detecting malignancies in the brain, liver, and other sites.
IgG4-related disease (IgG4-RD) is an autoimmune inflammatory disease that occurs mainly in the pancreas, biliary tract, salivary glands, kidneys, aorta, and other organs, and the disease is often accompanied by infiltration of IgG4+ cells or (and) activated fibroblasts (60). A study that included 26 patients with IgG4-RD showed that 68Ga-FAPI PET/CT detected an additional 18 (13.2%) involved organs out of 136 involved organs in 26 patients compared with 18F-FDG PET/CT and had a higher positive rate than 18F-FDG PET/CT in detecting pancreatic, bile duct/liver, and lacrimal gland involvement, but the former was at a disadvantage in detecting lymph node involvement (60). Co-imaging with 68Ga-FAPI-04 and 18F-FDG-PET may enable non-invasive tracking of the evolution of IgG4-RD from inflammation to fibrosis (60, 61). In a FAPI PET/CT imaging of patients with early-stage immune checkpoint inhibitor (ICI)–associated myocarditis, the heart showed specific uptake (62). High tracer accumulation was seen in the left ventricular myocardium on FAPI PET/CT imaging in a patient undergoing chemotherapy for pancreatic cancer. These study data may provide new ideas for early diagnosis of myocardial injury and intervention (63). In a case of thyroiditis with 68Ga-FAPI PET/CT, the uptake rate of inflammatory lesions (SUVmax of 4.2) was similar to that of hypofractionated thyroid cancer (SUVmax < 6), suggesting that FAPI PET/CT has difficulty in differentiating hypofractionated thyroid cancer from benign lesions (31, 64). Monitoring the activity of aortitis often requires a joint assessment of imaging data, clinical data, and biological data, which are not always correlated, making the work challenging. A patient with aortitis underwent 68Ga-FAPI-PET/CT, showing mild thickening of the walls of the bilateral carotid, subclavian, and thoracoabdominal aorta, where there is no specific uptake of 18F-FDG. Therefore, 68Ga-FAPI-PET/CT may be superior to 18F-FDG in monitoring the activity of aortitis (65). In two case reports, the TB lesions showed high uptake of the FAPI tracer with higher intensity than 18F-FDG; this helps in assessing extra-pulmonary tuberculosis lesions (66, 67).
The intensity of FAPI imaging agent uptake by various tumors is closely related to the degree of FAP expression, e.g., breast cancer and esophageal cancer, where FAP expression levels are high and tumor uptake of FAPI imaging agent is very high (SUVmax >12) (9, 31). The non-specific uptake of FAPI visualizers also correlates with the FAP expression profile, with FAP expression levels often upregulated during stromal remodeling, as in wound healing, and these sites tend to exhibit higher FAPI visualizer uptake (68, 69). Interestingly, cancers such as colon and pancreatic cancers, which have the highest desmoplastic reaction by histopathology, exhibit only moderate 68Ga-FAPI uptake (SUVmax of 6–12), this may be due to the variability of cancer, i.e., cancer development is complex and FAP expression varies at different stages of stromal remodeling, a conjecture that requires large sample studies to verify (31, 70).
Stromal remodeling occurs not only in malignancies but also in development, wound healing, chronic inflammation (e.g., arthritis, atherosclerotic plaques, and fibrosis), and certain physiologic processes where FAP is often highly expressed, posing challenges for differentiating benign from malignant, tumor and peritumor chronic inflammation in FAPI imaging (71). For example, a clinical study including 92 patients found high nonspecific uptake of FAPI-04 and FAPI-46 at sites where scarring (SUVmax of 7.7 ± 3.3) or degenerative lesions (SUVmax of 7.7 ± 2.9) occurred in patients, i.e., these sites may show false-positive results on tumor detection (69); one patient with pancreatic cancer who developed chronic peritumor inflammation due to radiotherapy was unable to identify the target volume for radiotherapy with FAPI tracer (72). In addition, some sites with low or moderate FAP expression, such as the uterus and breast, also showed higher FAPI tracer uptake in a recent study with SUVmax of 12.2 ± 7.3 and 4.5 ± 1.5, respectively, and this difference may be related to the biological age of women, and large sample studies are needed to confirm this finding (69, 73). Furthermore, FAPI-specific PET is still controversial in diagnosing bone metastases and lymph node metastases in different tumors (44, 74, 75), whether it is superior to 18F-FDG in diagnosing primary colorectal cancer and pancreatic cancer combined with chronic inflammation of the pancreas also needs further study (76). In another way, changes in the stroma during tumor development may lead to changes in the expression of FAP, so whether different aspects of tumor variability may have an impact on FAPI imaging results needs to be further explored. In brief, FAPIs cannot yet replace the work of FDG, but they can be used as a complement to it.
A total of 948 patients were included in the review, of which 891 patients were included in the malignancy imaging portion, including 598 Europeans, 287 Asians, and 1 North American, with an age range of 18–86 years. Existing studies did not find differences in the biodistribution of FAPI imaging agents between individuals, but age differences may have an impact on FAPI imaging results, e.g., the intensity of FAPI uptake in the uterus and breast is significantly higher in women of childbearing age than in women of non-childbearing age, which may pose a clinical challenge (57). The study is at a preliminary stage, and age, epidemiological factors and other factors that may contribute to differences in imaging results require further studies with large sample acquisition.
Although clinical studies of FAPI PET/CT are still at an early stage, mostly small sample size studies and lack of long-term follow-up data, and some clinical issues (e.g., tumor combined with inflammatory lesions and physiological uptake of organs that may be mixed with malignant lesions) need further clarification in subsequent studies. However, the light has just dawned, the available studies have shown that FAPIs are a promising class of tracers, and most of the FAPIs currently applied for imaging present relatively favorable pharmacokinetics and FAP affinity. In a variety of cancers such as glioma, gastrointestinal tumors, liver tumors, oral squamous carcinoma, and nasopharyngeal carcinoma, FAPIs are better than FDG for imaging, providing important molecular information and imaging basis for early diagnosis, precise staging, and guiding treatment. They also perform superiorly in non-malignant diseases (e.g., myocarditis and IgG4-RDs) and are expected to become the new molecule of the century.
Nineteen prospective studies designed to further explore the imaging characteristics of 68Ga-FAPIs are enrolling subjects in trials that will compare head-to-head with conventional imaging agents such as FDG, PSMA, FDOPA, and DOTATATE. These upcoming studies not only will include more types of tumors and expand the sample size of existing studies but will also perform immunohistochemical staining to correlate tracer uptake in tumors with FAP expression. While 68Ga-FAPI is the initial FAPI tracer used for PET imaging, both 68Ga and 18F-labeled FAPIs show more favorable imaging results, and the long half-life advantage demonstrated by 18F-labeled FAPIs is more favorable for its promotion in clinical applications.
We believe that FAPI imaging will play an important role in the identification of benign and malignant diseases, the accurate staging of malignant tumors, finding the primary foci of malignant tumors, monitoring the efficacy, identifying postoperative residual and fibrous tissue proliferation, assessing recurrence, finding biopsy sites where positive results can be more easily obtained, and guiding radiotherapy.
YH, LC, and RH conceived and designed the study. RH, YP, SH, CY, FY, YZP and JL retrieved and analyzed the documents. YP and SH collected the Figures. RH wrote the paper. YH and LC supervised the study, reviewed, and edited the manuscript. All authors contributed to the article and approved the submitted version.
This study was Supported by the opening project of medical imaging key laboratory of Sichuan Province (MIKLSP202001), the National Natural Science Foundation of China (No. 81960496), Yunnan Fundamental Research Projects (No. 202101AT070050), National Natural Science Foundation of China (81901781), and Science and Technology Planning Project of Guangzhou, China (202102011130280027).
The authors declare that the research was conducted in the absence of any commercial or financial relationships that could be construed as a potential conflict of interest.
All claims expressed in this article are solely those of the authors and do not necessarily represent those of their affiliated organizations, or those of the publisher, the editors and the reviewers. Any product that may be evaluated in this article, or claim that may be made by its manufacturer, is not guaranteed or endorsed by the publisher.
1. Siegel RL, Miller KD, Fuchs HE, Jemal A. Cancer Statistics, 2021. CA: Cancer J Clin (2021) 71(1):7–33. doi: 10.3322/caac.21654
2. Valkenburg KC, de Groot AE, Pienta KJ. Targeting the Tumour Stroma to Improve Cancer Therapy. Nat Rev Clin Oncol (2018) 15(6):366–81. doi: 10.1038/s41571-018-0007-1
3. Koustoulidou S, Hoorens MWH, Dalm SU, Mahajan S, Debets R, Seimbille Y, et al. Cancer-Associated Fibroblasts as Players in Cancer Development and Progression and Their Role in Targeted Radionuclide Imaging and Therapy. Cancers (2021) 13(5):1100. doi: 10.3390/cancers13051100
4. Kalluri R. The Biology and Function of Fibroblasts in Cancer. Nat Rev Cancer (2016) 16(9):582–98. doi: 10.1038/nrc.2016.73
5. Micallef L, Vedrenne N, Billet F, Coulomb B, Darby IA, Desmoulière A. The Myofibroblast, Multiple Origins for Major Roles in Normal and Pathological Tissue Repair. Fibrogen Tissue Repair (2012) 5(Suppl 1):S5. doi: 10.1186/1755-1536-5-s1-s5
6. Rettig WJ, Garin-Chesa P, Healey JH, Su SL, Jaffe EA, Old LJ. Identification of Endosialin, a Cell Surface Glycoprotein of Vascular Endothelial Cells in Human Cancer. Proc Natl Acad Sci USA (1992) 89(22):10832–6. doi: 10.1073/pnas.89.22.10832
7. Juillerat-Jeanneret L, Tafelmeyer P, Golshayan D. Fibroblast Activation Protein-α in Fibrogenic Disorders and Cancer: More Than a Prolyl-Specific Peptidase? Expert Opin Ther Targets (2017) 21(10):977–91. doi: 10.1080/14728222.2017.1370455
8. Jiang GM, Xu W, Du J, Zhang KS, Zhang QG, Wang XW, et al. The Application of the Fibroblast Activation Protein α-Targeted Immunotherapy Strategy. Oncotarget (2016) 7(22):33472–82. doi: 10.18632/oncotarget.8098
9. Scanlan MJ, Raj BK, Calvo B, Garin-Chesa P, Sanz-Moncasi MP, Healey JH, et al. Molecular Cloning of Fibroblast Activation Protein Alpha, a Member of the Serine Protease Family Selectively Expressed in Stromal Fibroblasts of Epithelial Cancers. Proc Natl Acad Sci USA (1994) 91(12):5657–61. doi: 10.1073/pnas.91.12.5657
10. Dohi O, Ohtani H, Hatori M, Sato E, Hosaka M, Nagura H, et al. Histogenesis-Specific Expression of Fibroblast Activation Protein and Dipeptidylpeptidase-IV in Human Bone and Soft Tissue Tumours. Histopathology (2009) 55(4):432–40. doi: 10.1111/j.1365-2559.2009.03399.x
11. Keane FM, Nadvi NA, Yao TW, Gorrell MD. Neuropeptide Y, B-Type Natriuretic Peptide, Substance P and Peptide YY are Novel Substrates of Fibroblast Activation Protein-α. FEBS J (2011) 278(8):1316–32. doi: 10.1111/j.1742-4658.2011.08051.x
12. Cohen SJ, Alpaugh RK, Palazzo I, Meropol NJ, Rogatko A, Xu Z, et al. Fibroblast Activation Protein and its Relationship to Clinical Outcome in Pancreatic Adenocarcinoma. Pancreas (2008) 37(2):154–8. doi: 10.1097/MPA.0b013e31816618ce
13. Eager RM, Cunningham CC, Senzer N, Richards DA, Raju RN, Jones B, et al. Phase II Trial of Talabostat and Docetaxel in Advanced non-Small Cell Lung Cancer. Clin Oncol (Royal Coll Radiologists (Great Britain)) (2009) 21(6):464–72. doi: 10.1016/j.clon.2009.04.007
14. Hintz HM, Gallant JP, Vander Griend DJ, Coleman IM, Nelson PS, LeBeau AM. Imaging Fibroblast Activation Protein Alpha Improves Diagnosis of Metastatic Prostate Cancer With Positron Emission Tomography. Clin Cancer Res an Off J Am Assoc Cancer Res (2020) 26(18):4882–91. doi: 10.1158/1078-0432.Ccr-20-1358
15. Jansen K, Heirbaut L, Cheng JD, Joossens J, Ryabtsova O, Cos P, et al. Selective Inhibitors of Fibroblast Activation Protein (FAP) With a (4-Quinolinoyl)-Glycyl-2-Cyanopyrrolidine Scaffold. ACS Medicinal Chem Lett (2013) 4(5):491–6. doi: 10.1021/ml300410d
16. Loktev A, Lindner T, Mier W, Debus J, Altmann A, Jäger D, et al. A Tumour-Imaging Method Targeting Cancer-Associated Fibroblasts. J Nucl Med Off Publication Soc Nucl Med (2018) 59(9):1423–9. doi: 10.2967/jnumed.118.210435
17. Lindner T, Loktev A, Altmann A, Giesel F, Kratochwil C, Debus J, et al. Development of Quinoline-Based Theranostic Ligands for the Targeting of Fibroblast Activation Protein. J Nucl Med Off Publication Soc Nucl Med (2018) 59(9):1415–22. doi: 10.2967/jnumed.118.210443
18. Loktev A, Lindner T, Burger EM, Altmann A, Giesel F, Kratochwil C, et al. Development of Fibroblast Activation Protein-Targeted Radiotracers With Improved Tumour Retention. J Nucl Med Off Publication Soc Nucl Med (2019) 60(10):1421–9. doi: 10.2967/jnumed.118.224469
19. Lindner T, Altmann A, Krämer S, Kleist C, Loktev A, Kratochwil C, et al. Design and Development of (99m)Tc-Labeled FAPI Tracers for SPECT Imaging and (188)Re Therapy. J Nucl Med Off Publication Soc Nucl Med (2020) 61(10):1507–13. doi: 10.2967/jnumed.119.239731
20. Giesel FL, Adeberg S, Syed M, Lindner T, Jiménez-Franco LD, Mavriopoulou E, et al. FAPI-74 PET/CT Using Either (18)F-AlF or Cold-Kit (68)Ga Labeling: Biodistribution, Radiation Dosimetry, and Tumour Delineation in Lung Cancer Patients. J Nucl Med Off Publication Soc Nucl Med (2021) 62(2):201–7. doi: 10.2967/jnumed.120.245084
21. Moon ES, Elvas F, Vliegen G, De Lombaerde S, Vangestel C, De Bruycker S, et al. Targeting Fibroblast Activation Protein (FAP): Next Generation PET Radiotracers Using Squaramide Coupled Bifunctional DOTA and DATA5m Chelators. EJNMMI Radiopharm Chem (2020) 5(1):19. doi: 10.1186/s41181-020-00102-z
22. Zhao L, Niu B, Fang J, Pang Y, Li S, Xie C, et al. Synthesis, Preclinical Evaluation, and a Pilot Clinical PET Imaging Study of (68)Ga-Labeled FAPI Dimer. J Nucl Med Off Publication Soc Nucl Med (2021) 8. doi: 10.2967/jnumed.121.263016
23. Wang S, Zhou X, Xu X, Ding J, Liu S, Hou X, et al. Clinical Translational Evaluation of Al(18)F-NOTA-FAPI for Fibroblast Activation Protein-Targeted Tumour Imaging. Eur J Nucl Med Mol Imaging (2021) 48(13):4259–71. doi: 10.1007/s00259-021-05470-5
24. Toms J, Kogler J, Maschauer S, Daniel C, Schmidkonz C, Kuwert T, et al. Targeting Fibroblast Activation Protein: Radiosynthesis and Preclinical Evaluation of an (18)F-Labeled FAP Inhibitor. J Nucl Med Off Publication Soc Nucl Med (2020) 61(12):1806–13. doi: 10.2967/jnumed.120.242958
25. Slania SL, Das D, Lisok A, Du Y, Jiang Z, Mease RC, et al. Imaging of Fibroblast Activation Protein in Cancer Xenografts Using Novel (4-Quinolinoyl)-Glycyl-2-Cyanopyrrolidine-Based Small Molecules. J Medicinal Chem (2021) 64(7):4059–70. doi: 10.1021/acs.jmedchem.0c02171
26. Moon ES, Van Rymenant Y, Battan S, De Loose J, Bracke A, van der Veken P, et al. In Vitro Evaluation of the Squaramide-Conjugated Fibroblast Activation Protein Inhibitor-Based Agents AAZTA(5).SA.FAPi and DOTA.SA.FAPi. Molecules (2021) 26(12):3482. doi: 10.3390/molecules26123482
27. Ballal S, Yadav MP, Moon ES, Kramer VS, Roesch F, Kumari S, et al. Biodistribution, Pharmacokinetics, Dosimetry of [(68)Ga]Ga-DOTA.SA.FAPi, and the Head-To-Head Comparison With [(18)F]F-FDG PET/CT in Patients With Various Cancers. Eur J Nucl Med Mol Imaging (2021) 48(6):1915–31. doi: 10.1007/s00259-020-05132-y
28. Jiang X, Wang X, Shen T, Yao Y, Chen M, Li Z, et al. FAPI-04 PET/CT Using [18f]AlF Labeling Strategy: Automatic Synthesis, Quality Control, and In Vivo Assessment in Patient. Front Oncol (2021) 11:649148. doi: 10.3389/fonc.2021.649148
29. Dahl K, Jussing E, Bylund L, Moein MM, Samén E, Tran T. Fully Automated Production of the Fibroblast Activation Protein Radiotracer [(18) F]FAPI-74. J Labelled Compounds Radiopharm (2021) 64(8):346–52. doi: 10.1002/jlcr.3926
30. Naka S, Watabe T, Lindner T, Cardinale J, Kurimoto K, Moore M, et al. One-Pot and One-Step Automated Radio-Synthesis of [18F]AlF-FAPI-74 Using a Multi Purpose Synthesizer: A Proof-Of-Concept Experiment. EJNMMI Radiopharm Chem (2021) 6(1):28. doi: 10.1186/s41181-021-00142-z
31. Kratochwil C, Flechsig P, Lindner T, Abderrahim L, Altmann A, Mier W, et al. 68Ga-FAPI PET/CT: Tracer Uptake in 28 Different Kinds of Cancer. J Nucl Med Off Publication Soc Nucl Med (2019) 60(6):801–5. doi: 10.2967/jnumed.119.227967
32. Giesel FL, Kratochwil C, Lindner T, Marschalek MM, Loktev A, Lehnert W, et al. 68Ga-FAPI PET/CT: Biodistribution and Preliminary Dosimetry Estimate of 2 DOTA-Containing FAP-Targeting Agents in Patients With Various Cancers. J Nucl Med Off Publication Soc Nucl Med (2019) 60(3):386–92. doi: 10.2967/jnumed.118.215913
33. Wang S, Zhou X, Xu X, Ding J, Liu T, Jiang J, et al. Dynamic PET/CT Imaging of 68Ga-FAPI-04 in Chinese Subjects. Front Oncol (2021) 11:651005. doi: 10.3389/fonc.2021.651005
34. Meyer C, Dahlbom M, Lindner T, Vauclin S, Mona C, Slavik R, et al. Radiation Dosimetry and Biodistribution of 68Ga-FAPI-46 PET Imaging in Cancer Patients. J Nucl Med Off Publication Soc Nucl Med (2020) 61(8):1171–7. doi: 10.2967/jnumed.119.236786
35. Kreppel B, Gärtner FC, Marinova M, Attenberger U, Meisenheimer M, Toma M, et al. [68ga]Ga-DATA5m.SA.FAPi PET/CT: Specific Tracer-Uptake in Focal Nodular Hyperplasia and Potential Role in Liver Tumour Imaging. Nuklearmedizin Nucl Med (2020) 59(5):387–9. doi: 10.1055/a-1164-5667
36. Fersing C, Bouhlel A, Cantelli C, Garrigue P, Lisowski V, Guillet BA. Comprehensive Review of Non-Covalent Radiofluorination Approaches Using Aluminum [(18)F]fluoride: Will [(18)F]AlF Replace (68)Ga for Metal Chelate Labeling? Molecules (2019) 24(16):2866. doi: 10.3390/molecules24162866
37. Spreckelmeyer S, Balzer M, Poetzsch S, Brenner W. Fully-Automated Production of [68Ga]Ga-FAPI-46 for Clinical Application. EJNMMI Radiopharm Chem (2020) 5(1):31. doi: 10.1186/s41181-020-00112-x
38. Uz Zaman M, Fatima N, Sajjad Z, Zaman U, Tahseen R, Zaman A. 18fdg Synthesis and Supply: A Journey From Existing Centralized to Future Decentralized Models. Asian Pacif J Cancer Prev APJCP (2014) 15(23):10057–9. doi: 10.7314/apjcp.2014.15.23.10057
39. Lindner T, Altmann A, Giesel F, Kratochwil C, Kleist C, Krämer S, et al. 18f-Labeled Tracers Targeting Fibroblast Activation Protein. EJNMMI Radiopharm Chem (2021) 6(1):26. doi: 10.1186/s41181-021-00144-x
40. Hu K, Wang L, Wu H, Huang S, Tian Y, Wang Q, et al. [(18)F]FAPI-42 PET Imaging in Cancer Patients: Optimal Acquisition Time, Biodistribution, and Comparison With [(68)Ga]Ga-FAPI-04. Eur J Nucl Med Mol Imaging (2021). doi: 10.1007/s00259-021-05646-z
41. Ferdinandus J, Kessler L, Hirmas N, Trajkovic-Arsic M, Hamacher R, Umutlu L, et al. Equivalent Tumour Detection for Early and Late FAPI-46 PET Acquisition. Eur J Nucl Med Mol Imaging (2021) 48(10):3221–7. doi: 10.1007/s00259-021-05266-7
42. Zhao L, Pang Y, Zheng H, Han C, Gu J, Sun L, et al. Clinical Utility of [(68)Ga]Ga-Labeled Fibroblast Activation Protein Inhibitor (FAPI) Positron Emission Tomography/Computed Tomography for Primary Staging and Recurrence Detection in Nasopharyngeal Carcinoma. Eur J Nucl Med Mol Imaging (2021) 48(11):3606–17. doi: 10.1007/s00259-021-05336-w
43. Syed M, Roehrich M, Giesel F, Liermann J, Choyke P, Kauczor HU, et al. FAPI-PET/CT Improves Diagnostic Staging and Radiotherapy Planning of Adenoid Cystic Carcinomas - Imaging Analysis and Histological Validation. Int J Radiat Oncol Biol Phys (2021) 111(3s):e415. doi: 10.1016/j.ijrobp.2021.07.1191
44. Gu B, Xu X, Zhang J, Ou X, Xia Z, Guan Q, et al. The Added Value of (68)Ga-FAPI-04 PET/CT in Patients With Head and Neck Cancer of Unknown Primary With (18)F-FDG Negative Findings. J Nucl Med Off Publication Soc Nucl Med (2021) Jnumed.121.262790. doi: 10.2967/jnumed.121.262790
45. Marner L, Henriksen OM, Lundemann M, Larsen VA, Law I. Clinical PET/MRI in Neurooncology: Opportunities and Challenges From a Single-Institution Perspective. Clin Trans Imaging (2017) 5(2):135–49. doi: 10.1007/s40336-016-0213-8
46. Röhrich M, Loktev A, Wefers AK, Altmann A, Paech D, Adeberg S, et al. IDH-Wildtype Glioblastomas and Grade III/IV IDH-Mutant Gliomas Show Elevated Tracer Uptake in Fibroblast Activation Protein-Specific PET/Ct. Eur J Nucl Med Mol Imaging (2019) 46(12):2569–80. doi: 10.1007/s00259-019-04444-y
47. Kuten J, Levine C, Shamni O, Pelles S, Wolf I, Lahat G, et al. Head-To-Head Comparison of [(68)Ga]Ga-FAPI-04 and [(18)F]-FDG PET/CT in Evaluating the Extent of Disease in Gastric Adenocarcinoma. Eur J Nucl Med Mol Imaging (2021) 49(2):743–50. doi: 10.1007/s00259-021-05494-x
48. Giesel FL, Heussel CP, Lindner T, Röhrich M, Rathke H, Kauczor HU, et al. FAPI-PET/CT Improves Staging in a Lung Cancer Patient With Cerebral Metastasis. Eur J Nucl Med Mol Imaging (2019) 46(8):1754–5. doi: 10.1007/s00259-019-04346-z
49. Kömek H, Can C, Güzel Y, Oruç Z, Gündoğan C, Yildirim ÖA, et al. (68)Ga-FAPI-04 PET/CT, a New Step in Breast Cancer Imaging: A Comparative Pilot Study With the (18)F-FDG PET/Ct. Ann Nucl Med (2021) 35(6):744–52. doi: 10.1007/s12149-021-01616-5
50. Chen H, Pang Y, Wu J, Zhao L, Hao B, Wu J, et al. Comparison of [(68)Ga]Ga-DOTA-FAPI-04 and [(18)F] FDG PET/CT for the Diagnosis of Primary and Metastatic Lesions in Patients With Various Types of Cancer. Eur J Nucl Med Mol Imaging (2020) 47(8):1820–32. doi: 10.1007/s00259-020-04769-z
51. Jiang C, Chen Y, Zhu Y, Xu Y. Systematic Review and Meta-Analysis of the Accuracy of 18F-FDG PET/CT for Detection of Regional Lymph Node Metastasis in Esophageal Squamous Cell Carcinoma. J Thorac Dis (2018) 10(11):6066–76. doi: 10.21037/jtd.2018.10.57
52. Jiang D, Chen X, You Z, Wang H, Zhang X, Li X, et al. Comparison of [(68) Ga]Ga-FAPI-04 and [(18)F]-FDG for the Detection of Primary and Metastatic Lesions in Patients With Gastric Cancer: A Bicentric Retrospective Study. Eur J Nucl Med Mol Imaging (2021) 49(2):732–42. doi: 10.1007/s00259-021-05441-w
53. De Raffele E, Mirarchi M, Cuicchi D, Lecce F, Cola B. Evolving Role of FDG-PET/CT in Prognostic Evaluation of Resectable Gastric Cancer. World J Gastroenterol (2017) 23(38):6923–6. doi: 10.3748/wjg.v23.i38.6923
54. Ho CL, Yu SC, Yeung DW. 11c-Acetate PET Imaging in Hepatocellular Carcinoma and Other Liver Masses. J Nucl Med Off Publication Soc Nucl Med (2003) 44(2):213–21.
55. Guo W, Pang Y, Yao L, Zhao L, Fan C, Ke J, et al. Imaging Fibroblast Activation Protein in Liver Cancer: A Single-Center Post Hoc Retrospective Analysis to Compare [(68)Ga]Ga-FAPI-04 PET/CT Versus MRI and [(18)F]-FDG PET/Ct. Eur J Nucl Med Mol Imaging (2021) 48(5):1604–17. doi: 10.1007/s00259-020-05095-0
56. Shi X, Xing H, Yang X, Li F, Yao S, Congwei J, et al. Comparison of PET Imaging of Activated Fibroblasts and (18)F-FDG for Diagnosis of Primary Hepatic Tumours: A Prospective Pilot Study. Eur J Nucl Med Mol Imaging (2021) 48(5):1593–603. doi: 10.1007/s00259-020-05070-9
57. Dendl K, Koerber SA, Finck R, Mokoala KMG, Staudinger F, Schillings L, et al. (68)Ga-FAPI-PET/CT in Patients With Various Gynecological Malignancies. Eur J Nucl Med Mol Imaging (2021) 48(12):4089–100. doi: 10.1007/s00259-021-05378-0
58. Erol Fenercioğlu Ö, Beyhan E, Ergül N, Arslan E, Çermik TF. 18f-FDG PET/CT and 68Ga-FAPI-4 PET/CT Findings of Bilateral Knee Osteoarthritis in a Patient With Uveal Malignant Melanoma. Clin Nucl Med (2021) 47(2):e144–6. doi: 10.1097/rlu.0000000000003854
59. Dendl K, Finck R, Giesel FL, Kratochwil C, Lindner T, Mier W, et al. FAP Imaging in Rare Cancer Entities-First Clinical Experience in a Broad Spectrum of Malignancies. Eur J Nucl Med Mol Imaging (2021) 49(2):721–31. doi: 10.1007/s00259-021-05488-9
60. Luo Y, Pan Q, Yang H, Peng L, Zhang W, Li F. Fibroblast Activation Protein-Targeted PET/CT With (68)Ga-FAPI for Imaging IgG4-Related Disease: Comparison to (18)F-FDG PET/Ct. J Nucl Med Off Publication Soc Nucl Med (2021) 62(2):266–71. doi: 10.2967/jnumed.120.244723
61. Schmidkonz C, Rauber S, Atzinger A, Agarwal R, Götz TI, Soare A, et al. Disentangling Inflammatory From Fibrotic Disease Activity by Fibroblast Activation Protein Imaging. Ann Rheum Dis (2020) 79(11):1485–91. doi: 10.1136/annrheumdis-2020-217408
62. Finke D, Heckmann MB, Herpel E, Katus HA, Haberkorn U, Leuschner F, et al. Early Detection of Checkpoint Inhibitor-Associated Myocarditis Using (68)Ga-FAPI PET/Ct. Front Cardiovasc Med (2021) 8:614997. doi: 10.3389/fcvm.2021.614997
63. Totzeck M, Siebermair J, Rassaf T, Rischpler C. Cardiac Fibroblast Activation Detected by Positron Emission Tomography/Computed Tomography as a Possible Sign of Cardiotoxicity. Eur Heart J (2020) 41(9):1060. doi: 10.1093/eurheartj/ehz736
64. Zhou Y, He J, Chen Y. (68)Ga-FAPI PET/CT Imaging in a Patient With Thyroiditis. Endocrine (2021) 73(2):485–6. doi: 10.1007/s12020-021-02605-4
65. Wu S, Pang Y, Zhao L, Zhao L, Chen H. 68Ga-FAPI PET/CT Versus 18f-FDG PET/CT for the Evaluation of Disease Activity in Takayasu Arteritis. Clin Nucl Med (2021) 46(10):847–9. doi: 10.1097/rlu.0000000000003692
66. Gu B, Luo Z, He X, Wang J, Song S. 68Ga-FAPI and 18F-FDG PET/CT Images in a Patient With Extrapulmonary Tuberculosis Mimicking Malignant Tumour. Clin Nucl Med (2020) 45(11):865–7. doi: 10.1097/rlu.0000000000003279
67. Zheng J, Lin K, Zheng S, Yao S, Miao W. 68Ga-FAPI and 18F-PET/CT Images in Intestinal Tuberculosis. Clin Nucl Med (2021) 47(3):239–40. doi: 10.1097/rlu.0000000000003917
68. Mathew S, Scanlan MJ, Mohan Raj BK, Murty VV, Garin-Chesa P, Old LJ, et al. The Gene for Fibroblast Activation Protein Alpha (FAP), A Putative Cell Surface-Bound Serine Protease Expressed in Cancer Stroma and Wound Healing, Maps to Chromosome Band 2q23. Genomics (1995) 25(1):335–7. doi: 10.1016/0888-7543(95)80157-h
69. Kessler L, Ferdinandus J, Hirmas N, Zarrad F, Nader M, Kersting D, et al. Pitfalls and Common Findings in (68)Ga-FAPI-PET - A Pictorial Analysis. J Nucl Med Off Publication Soc Nucl Med (2021) Jnumed.121.262808. doi: 10.2967/jnumed.121.262808
70. Garin-Chesa P, Old LJ, Rettig WJ. Cell Surface Glycoprotein of Reactive Stromal Fibroblasts as a Potential Antibody Target in Human Epithelial Cancers. Proc Natl Acad Sci USA (1990) 87(18):7235–9. doi: 10.1073/pnas.87.18.7235
71. Egger C, Cannet C, Gérard C, Suply T, Ksiazek I, Jarman E, et al. Effects of the Fibroblast Activation Protein Inhibitor, PT100, in a Murine Model of Pulmonary Fibrosis. Eur J Pharmacol (2017) 809:64–72. doi: 10.1016/j.ejphar.2017.05.022
72. Röhrich M, Naumann P, Giesel FL, Choyke PL, Staudinger F, Wefers A, et al. Impact of (68)Ga-FAPI PET/CT Imaging on the Therapeutic Management of Primary and Recurrent Pancreatic Ductal Adenocarcinomas. J Nucl Med Off Publication Soc Nucl Med (2021) 62(6):779–86. doi: 10.2967/jnumed.120.253062
73. Rettig WJ, Su SL, Fortunato SR, Scanlan MJ, Raj BK, Garin-Chesa P, et al. Fibroblast Activation Protein: Purification, Epitope Mapping and Induction by Growth Factors. Int J Cancer (1994) 58(3):385–92. doi: 10.1002/ijc.2910580314
74. Wu J, Wang Y, Liao T, Rao Z, Gong W, Ou L, et al. Comparison of the Relative Diagnostic Performance of [(68)Ga]Ga-DOTA-FAPI-04 and [(18)F]FDG PET/CT for the Detection of Bone Metastasis in Patients With Different Cancers. Front Oncol (2021) 11:737827. doi: 10.3389/fonc.2021.737827
75. Qin C, Liu F, Huang J, Ruan W, Liu Q, Gai Y, et al. A Head-To-Head Comparison of (68)Ga-DOTA-FAPI-04 and (18)F-FDG PET/MR in Patients With Nasopharyngeal Carcinoma: A Prospective Study. Eur J Nucl Med Mol Imaging (2021) 48(10):3228–37. doi: 10.1007/s00259-021-05255-w
Keywords: fibroblast-activating protein, FAPI, PET, Neoplasms, imaging, 18 F-FDG
Citation: Huang R, Pu Y, Huang S, Yang C, Yang F, Pu Y, Li J, Chen L and Huang Y (2022) FAPI-PET/CT in Cancer Imaging: A Potential Novel Molecule of the Century. Front. Oncol. 12:854658. doi: 10.3389/fonc.2022.854658
Received: 14 January 2022; Accepted: 07 April 2022;
Published: 25 May 2022.
Edited by:
Bradley T Scroggins, National Cancer Institute (NIH), United StatesReviewed by:
Sikandar Shaikh, Shadan Hospital and Institute of Medical Sciences, IndiaCopyright © 2022 Huang, Pu, Huang, Yang, Yang, Pu, Li, Chen and Huang. This is an open-access article distributed under the terms of the Creative Commons Attribution License (CC BY). The use, distribution or reproduction in other forums is permitted, provided the original author(s) and the copyright owner(s) are credited and that the original publication in this journal is cited, in accordance with accepted academic practice. No use, distribution or reproduction is permitted which does not comply with these terms.
*Correspondence: Long Chen, bG9uZWNoZW4xOTgzQGhvdG1haWwuY29t; Yunchao Huang, aHVhbmd5dW5jaDIwMTdAMTI2LmNvbQ==
†These authors have contributed equally to this work
Disclaimer: All claims expressed in this article are solely those of the authors and do not necessarily represent those of their affiliated organizations, or those of the publisher, the editors and the reviewers. Any product that may be evaluated in this article or claim that may be made by its manufacturer is not guaranteed or endorsed by the publisher.
Research integrity at Frontiers
Learn more about the work of our research integrity team to safeguard the quality of each article we publish.