- 1Department of General Surgery, Affiliated Minzu Hospital of Guangxi Medical University, Nanning, China
- 2Oncology Medical College, Guangxi Medical University, Nanning, China
With completing the whole genome sequencing project, awareness of lncRNA further deepened. The growth arrest-specific transcript 5 (GAS5) was initially identified in growth-inhibiting cells. GAS5 is a lncRNA (long non-coding RNA), and it plays a crucial role in various human cancers. There are small ORFs (open reading frames) in the exons of the GAS5 gene sequence, but they do not encode functional proteins. In addition, GAS5 is also the host gene of several small nucleolar RNAs (snoRNA). These snoRNAs are believed to play a suppressive role during tumor progression by methylating ribosomal RNA (rRNA). As a result, GAS5 expression levels in tumor tissues are significantly reduced, leading to increased malignancy, poor prognosis, and drug resistance. Recent studies have demonstrated that GAS5 can interact with miRNAs by base-pairing and other functional proteins to inhibit their biological functions, impacting signaling pathways and changing the level of intracellular autophagy, oxidative stress, and immune cell function in vivo. In addition, GAS5 participates in regulating proliferation, invasion, and apoptosis through the above molecular mechanisms. This article reviews the recent discoveries on GAS5, including its expression levels in different tumors, its biological behavior, and its molecular regulation mechanism in human cancers. The value of GAS5 as a molecular marker in the prevention and treatment of cancers is also discussed.
Introduction
The human genome is composed of coding genes and non-coding genes. There are about 20,000 protein-coding genes, but these genes only represent less than 2% of the human genome. Non-coding RNAs (ncRNAs) account for the other 98% and have been called “dark matter” by researchers, contrary to the dogma, they cannot translate proteins (1). However, ncRNAs are not a “noise” of genetic transcription; on the contrary, increasing studies have shown that ncRNAs are widely involved in the process of cell physiology, for example, in regulating gene expression, maintaining genome stability, and contributing to the formation of the complexity of the organism (2).
Based on their length, RNAs can be classified into short RNAs (sRNAs, < 200 nt) or long ncRNAs (lncRNAs, > 200 nt). Short RNAs include micro RNAs (miRNAs, 22 nt), small interfering RNAs (siRNAs, 22 nt), small nuclear RNAs (snRNAs, 100–300 nt), PIWI-associated RNAs (piRNAs, 27 nt), and small nucleolar RNAs (snoRNAs, 70 nt) and other types of sRNAs (2, 3). Brannan et al. (4) isolated and sequenced the first lncRNA, H19, from the human genome in 1990. One of the first observations was that H19 does not encode a protein, unlike traditional mRNA. Numerous lncRNAs are transcribed by RNA polymerase II (RNA Pol II) and can be classified as exonic, intronic, overlapping, or intergenic lncRNAs according to the location of the gene (5). Depending on the function of the lncRNA, they can be further classified as cis-acting lncRNAs that affect local gene expression or trans-acting lncRNAs that operate far away from the transcription site (6). Furthermore, lncRNA can accelerate targeted protein binding to specific genomic loci, influence the bioactivity of protein-binding partners, and regulate gene expression as a small nuclear RNA precursor (7).
GAS5
Schneider et al. (8) initially found GAS5 in a mouse cell model of negative growth regulation. GAS5 is a lncRNA with 651 nucleotides encoded by a sequence located at 1q25.1. The GAS5 sequence includes 12 exons, 11 introns, and 10 identical small ORFs on the corresponding introns snoRNA, but this sequence does not encode a protein (9, 10). GAS5 can be transcribed into two mature RNAs, GAS5a and GAS5b, using the selective 5’-splice donor site on the 7th exon (Figure 1) (11). GAS5 transcripts contain a 7-nucleotide 5’oligopyrimidine tract, a translation initiation point, and a short 5 ‘UTR. Hence, it is considered a member of the 5’TOP gene family (12).
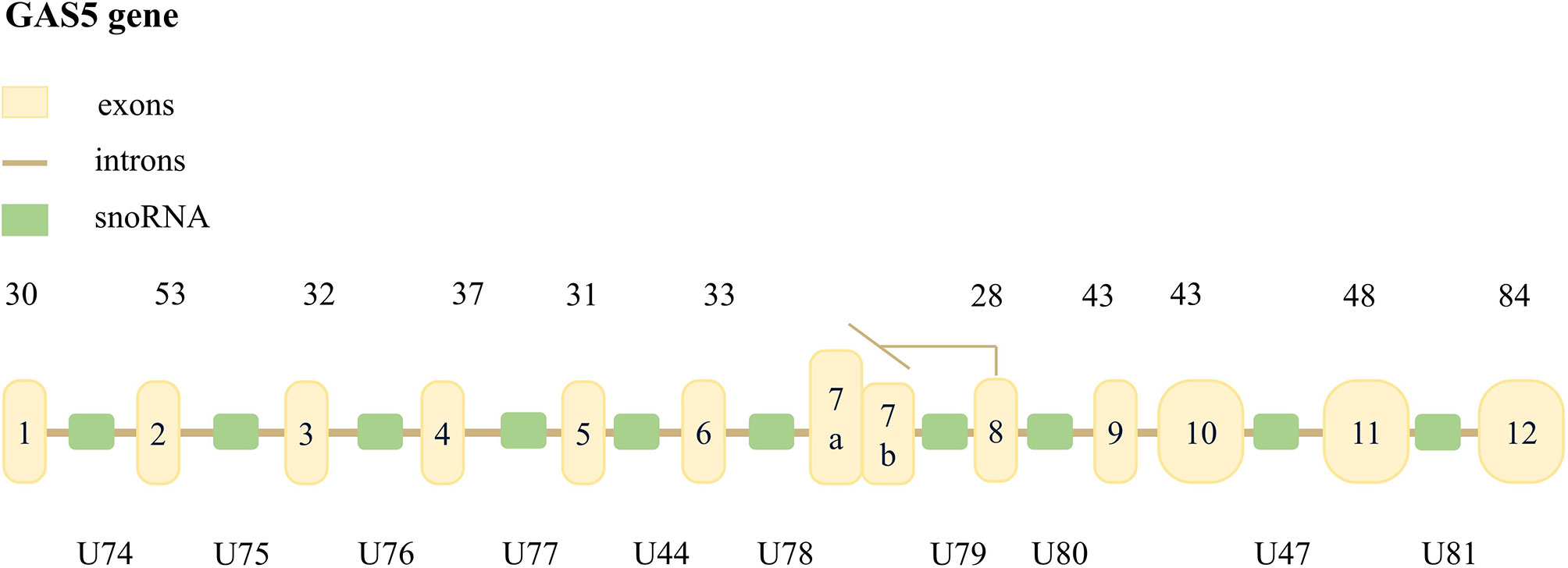
Figure 1 The structure of GAS5 in the human genome. The yellow boxes represent the 12 exons of human GAS5, the brown line represents 11 introns, and the green boxes represent 10 snoRNAs. The number above the gene indicates the length of the exon, and the bottom of the gene is the symbol of snoRNA. It is pointed out that alternative splicing of exon 7 may transcribe two mature lncRNAs, named GAS5a and GAS5b, respectively. The former is 39 bases longer than the latter.
More than 20 splice variants of GAS5 have been detected, with two variants, “Full-Length” (FL) and “Clone 2” (C2), having been shown to have different effects on the cell proliferation phenotype of neuroblastoma. Research on molecule mechanism shows that silencing GAS5 increased the number of p53 phosphorylation. At the same time, another candidate gene, BRCA1(Breast Cancer 1), was identified as co-activating with p53, and their downstream co-acting target GADD45A (Growth Arrest and DNA Damage Inducible Alpha) was further detected. GADD45A was shown to induce cell cycle arrest and promote apoptosis. Although the supplementation of the FL splice variant of GAS5 reduces p53 levels, the supplementation of the C2 variant does no effect on p53 and drives apoptosis. Therefore, the above studies suggest that GAS5 splicing variants may cause tumor suppressor or tumor-promoting effect of GAS5 in different cancers (13).
Recent studies supported that GAS5 regulates cell cycle progression and exerts biological functions. Active cyclin dependent kinases 4/6 (CDK4/6) release part of the restriction of E2F1 by phosphorylation of the retinoblastoma protein (Rb), which inhibits cell proliferation as a tumor suppressor (14). c-MYC is considered to be a proto-oncogene. After translation, c-MYC is suppressed by GAS5, which can stop the G1-S transition by upregulating the expression levels of CDK inhibitors and p21 (15). GAS5 has a hormone response element mimic (HREM) sequence, which can bind to nuclear steroid receptors to accelerate cell apoptosis, improve the effect of chemotherapy drugs and DNA damage from radiation, and other in vitro apoptosis-stimulating factors (16).
The Tumor Suppressor Mechanism of GAS5 in Various Types of Cancer
The lncRNA GAS5 evolved various strategies to regulate gene expression as vital tumor proliferation suppressor. GAS5 acts as a “sponge-like” to interact with miRNA molecules (Figure 2), binds to one or more functional proteins, and limits tumor angiogenesis. Other related mechanisms are the tumor microenvironment affected by intracellular oxidative stress and immune escape (Figure 3). In this part, we will focus on the latest discoveries and various features of GAS5 that regulate malignant tumors.
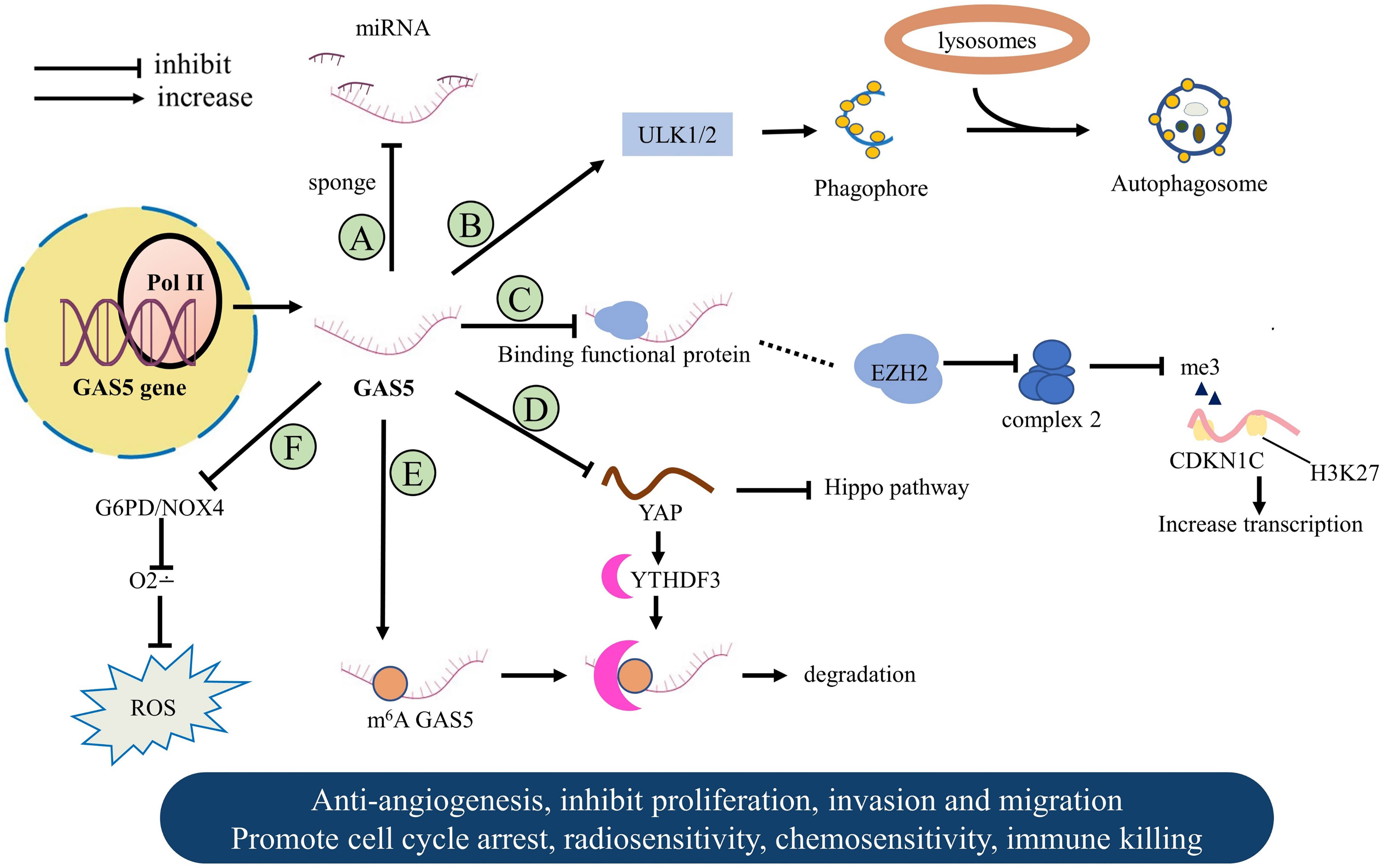
Figure 3 The molecular mechanism of GAS5 in a variety of human tumors. (A) GAS5 acts as a sponge to bond miRNA to suppress cancer. (B) GAS5 promotes the formation of autophagy in breast cancer. (C) GAS5 combines functional proteins to promote tumor suppressor gene expression. (D) GAS5 bind directly to WW domain of YAP in Colorectal cancer. (E) Methylated GAS5 is regulated downstream to form a negative feedback loop. (F) GAS5 changes the level of intracellular oxidative stress to maintain cell growth arrest.
Breast Cancer
Asghar et al. (17) found that the expression of GAS5 in breast cancer (BC) tissues was less compared to the control group, and the expression of the younger group (< 45 years) was less than that found in the older group (> 45 years). The action mechanism indicated that GAS5 combined with miR-216b regulates the epithelial-mesenchymal transition of breast cancer tissues, promotes apoptosis, and weakens the invasion ability (18).
Autophagy refers to the process in which double-membrane vesicles wrap intracellular proteins or damaged organelles and integrate into lysosomes to decompose (19). The autophagic progress may play a dual role in the complex tumor microenvironment (20). Unc-51-like kinase 1 (ULK1) plays an active role in the initiation of autophagy. ULK1 and its homolog ULK2 both have been reported to induce autophagy and inhibit tumor progression. GAS5 is positively related to ULK1/2, and autophagy induction promotes autophagosome formation in BC. This can limit malignant behaviors such as proliferation, invasion, and formation of tumors (21).
Increasing evidence has shown that GAS5 is also involved in regulating thesensitivity of BC to chemotherapy drugs. As a member of the dickkopf family, dickkopf 2 (DKK2) regulates the Wnt/β-catenin signaling pathway by binding to the lipoprotein receptor related protein 5/6, a component of the Wnt receptor complex. GAS5 binds miR-221-3p to reduce the latter’s expression and increases DKK2 expression, further repressing the Wnt/β-catenin signaling pathway, thus reversing resistance to ADR (22). Similar studies by Gu et al. (23) also showed that GAS5 overexpression can act as a molecular sponge binding with miR-222, upregulating its endogenous target phosphatase and tensin homologs (PTEN), silencing the AKT/mTOR signaling pathway, and increasing the sensitivity of BC to tamoxifen.
Triple-negative BC (TNBC) is a unique subtype of breast cancer identified after the lack expression of the estrogen receptor, progesterone receptor, and epidermal growth factor receptor 2. TNBC is highly invasive and resistant to chemotherapy, and therefore it is considered the most aggressive and fatal type of BC. There is a study shows that GAS5 inhibits miR-196a-5p expression and its downstream target FOXO1 expression increases, which in turn inhibits PI3K/AKT phosphorylation, thus limiting TNBC progression (24). Another study discovered that overexpressed GAS5 could increase the expression level of SUFU by sponging miR-378a -5p in TNBC cells, resulting in enhanced apoptosis of cells resistant to paclitaxel (PTX) and cisplatin (CIS) (25).
Lung Cancer
Non-small cell lung cancer (NSCLC) is the most common type of lung cancer. The intrinsic level of GAS5 is downregulated in NSCLC. This downregulation is inversely related to poor clinicopathological factors. GAS5 significantly inhibits the expression level of miR-205 in NSCLC, and miR-205 has been shown to interact with PTEN (26). Furthermore, Xue et al. (27) revealed that GAS5 remarkably inhibited miR-135b expression, increasing the sensitivity of NSCLC to radiotherapy.
Due to their different contents, exosomes are currently extensive studied as emerging players in intercellular information transmission. Poulet et al. (28) found that the low expression of exosomal GAS5 in serum from NSCLC patients was positively associated with advanced tumor–node–metastasis (TNM) stages and larger tumor diameter than healthy controls. In human umbilical vein endothelial cells, GAS5 in exosomes has been shown to bind competitively with miR-29-3p, changing PTEN and blocking the PI3K/AKT pathway (HUVEC). As a result, exosomal GAS5 might affect the control of NSCLC angiogenesis (29).
Prostate Cancer
Recently, programmed cell death 4 (PDCD4) affects the expression of various oncogenes and may be positively correlated with prostate cell differentiation. Single-nucleotide polymorphisms (SNPs) were shown to affect the expression level of the lncRNA in which it is located. The two SNPs (rs55829688 T > C and rs145204276 INS > DEL) in GAS5 have been shown to upregulate GAS5 expression by methylating its promoter, and then GAS5 binds miR-21 and miR‐2184 directly as a sponge, targeting PTEN, PDCD4, respectively, further inducing cell apoptosis in Prostate Cancer (PCa). The aforementioned findings mentioned above indicate that GAS5 may be a novel prognostic marker of PCa (30).
Colorectal Cancer
GAS5 expression was associated with the clinicopathological characteristics of CRC, and patients with high GAS5 expression had a smaller tumor diameter and early TNM staging. GAS5 levels are significantly reduced compared to normal controls, causing the proliferation, migration, and invasion of cancer tissues (31). Cheng et al. (32) reported that FOXO3a inhibits the phosphorylation of the PI3K/AKT signaling pathway as a pro-apoptotic transcription factor, GAS5 directly adsorbs miR-182-5p, increases the expression of the downstream target FOXO3a, which causes the proliferation of CRC and promotes apoptosis.
Sirtuin 1 (SIRT1) is a type III nuclear deacetylase, which is upregulated in CRC and negatively regulates the expression level of mTOR, which is negatively correlated with the level of intracellular macroautophagy activity. Separate studies have demonstrated that GAS5 directly adsorbs miR-34a like a sponge, releasing its inhibition of SIRT1 expression, further inhibiting the level of macroautophagy activation and inducing CRC apoptosis. Furthermore, the 5’-TOP sequence located in the GAS5 exon controls its transcription. Overexpression of miR-34a forms a negative feedback regulation loop through the SIRT1/mTOR/GAS5 axis, which may explain why GAS5 - mediated autophagy in CRC is in a relatively balanced state. Thus, it plays an anti-apoptotic role in cancer progression and provides a new perspective on the molecular mechanism of CRC (33).
A central downstream sensor of the Hippo pathway is phosphorylated YAP, which can promote the progression of CRC. GAS5 binds the 171-263 amino acid region (WW domain) of YAP directly to promote ubiquitination and degradation of the latter, thus inhibiting YAP signaling. Further research indicated that YTH-domain family member 3 (YTHDF3) acts as an N6-methyladenosine (m6A) reader, considered as a new YAP target. YTHDF3 selectively binds to this structure and promotes the degradation of GAS5 through methylation dependence, forming a negative feedback loop. Therefore, YAP activates ectopically and promotes the malignant behavior of CRC in the absence of GAS5. The stable expression level of GAS5 is of great significance in limiting the progression of CRC (34).
Malignant Melanoma
Previous reports have indicated that malignant melanoma (MM) is characterized by aggressiveness and early metastasis, and its pathogenesis and development are related to intracellular oxidative stress (35). Reactive oxygen species are oxygen-containing derivatives composed of highly unstable oxygen free radicals and are elevated in tumor cells. ROS has been indicated act as a cancer promoters due to the abnormal process of proliferation, apoptosis, and angiogenesis effect (36). Chen et al. reported that GAS5 diminished remarkably in advanced melanoma. GAS5 overexpression induces cancer cells to stay in the G0/G1 phase and inhibits the expression of G6PD and NOX4, further leading to a decrease in O2∸. The above changes reduce the level of reactive oxygen species and destabilize the redox balance in MM cells (37). The work of Xu et al. (35) showed that GAS5 participates in facilitating tumor repression by directly binding EZH2, a member of the Polycomb inhibitory complex 2, further reducing lysine 27 trimethylation (H3K27me3) of histone H3, enhancing the expression of the target gene CDKN1C. Taken together, these processes mentioned above accelerate intracellular oxidative stress and inhibit the vitality of MM.
Bladder Cancer
Studies by Avgeris et al. (38) encouraged the notion that bladder cancer (BlCa) expressing lower levels of GAS5 than that in adjacent normal tissues, and GAS5 expression in invasive (T1-T4) tumors was significantly attenuated than in superficial Ta tumors. One mechanism shows that overexpressed GAS5 acts as a sponge via binding with and downregulation of miR-21, amplify-function mutations in PTEN, and promote tumor apoptosis; knocking out GAS5, in contrast, increases the proportion of S and G2 cancer cells, thereby promoting proliferation (39). In bladder transitional cell carcinomas (BTCC), GAS5 expression is reduced and the patient’s prognosis worsens. Whereas overexpression of GAS5 inhibits the anti-apoptotic gene Bcl-2, upregulation of Bcl-2 reversed the inhibitory effect of GAS5 on doxorubicin-resistant BTCC. Accordingly, GAS5 contributes to the development of drug resistance (40).
Hepatic Carcinoma
The low expression of GAS5 may reflect the poor survival rate of patients with hepatocellular carcinoma (HCC). The cytochrome P450 family 2 subfamily C member 8 (CYP2C8) is well recognized for inhibiting cell proliferation growth in HCC. GAS5 induces apoptosis by sponging miR-382-3p and upregulating the expression of CYP2C8 proteins (41). Reversion-inducing cysteine-rich protein with Kazal motifs (RECK) is an anti-metastatic gene cloned from NIH3T3 cells, which encodes extracellular proteins and restricts the metastasis of HCC by blunting the effects of matrix metalloproteinases (MMPs). Notably, the invasive ability of the tumor may be eliminated through a combination of upregulated GAS5 and miR-135b, exhibiting increased RECK, and the expression and activity of MMP-2 are insufficient (42). The tumor protein p53-inducible nuclear protein 1 (TP53INP1) can induce apoptosis as a stress-induced gene by regulating the p53 and p73 pathways and is attenuated by miR-1323. The apoptotic machinery is composed of GAS5 that binds to miR-1323, directly stimulating the function of TP53INP1 as a barrier to the pathogenesis of HCC (43).
Evidence began to accumulate in GAS5-mediated regulation of the immune system. For example, the activating receptor NKp46 encoded by the natural cytotoxicity receptor 1 (NCR1) can be expressed in NK cells; this work limits tumor growth and metastasis by improving IFN-c release, while Runt-related transcription factor 3 (RUNX3) acts on the NCR1 promoter in NK cells and has demonstrable increases in function. Studies on controlling mechanisms suggest that GAS5 negatively regulates miR-544, activating RUNX3 expression, and increasing the immune killing of HCC through the RUNX3/NCR1/NKp46 axis. As such, these may represent a potential hallmark for the treatment of liver cancer (44).
Gastric Cancer
Li et al. (45) found that GAS5 binds miR-222 through an endogenous sponge mechanism that acts on PTEN, which modulates the classical signaling pathway PI3K/Akt/mTOR and subsequently inhibits GC proliferation. In addition, Liu et al. (46) reported that tumor size, lymph node metastasis, and TNM staging in patients were negatively associated with GAS5 gene amplification. Higher GAS5 expression increases protein expression of p53 and its target gene KAI1/CD82, and helps extend the half-life of p53, which correlates with poor metastasis capacity with GC.
Moreover, a study demonstrated that the progression of GC is driven by rs145204276 of GAS5, patients with allele del of rs145204276 located in the promoter region of GAS5, would result in a smaller tumor size and a lower risk of GC progression (47).
Cervical Cancer
Cancer cells with low express-mutated GAS5 in cervical tissue represent a survival advantage; on the contrary, its overexpression weakens the malignant biological behavior of cervical cancer (CC). The underlying mechanism suggests that GAS5 has shown a downside function in regulating miR-135a, silencing the JAK/STAT signaling pathway (48). Besides, Yang et al. uncovered that elevated GAS5 expression limits the growth and metastasis of CC by interacting with the epithelial-mesenchymal transition (49). In drug resistance research, GAS5 overexpression was remarkably associated with inhibition of miR-21 expression and significantly increased the expression level of PCDC4 and TIMP3, which activate sensitivity to cisplatin in CC cells (50). Similarly, upregulation of IER3 shortens the cycle of keratinocytes and enhances the sensitivity of CC cells to radiation. Mechanically, GAS5 can decrease miR-106b expression and raise the expression level of IER3 (51).
Thyroid Cancer
In thyroid cancer, GAS5 expression is decreased in poor phenotypes, suggesting that patients are at increased risk of recurrence (52). A recent study suggests that the suppressor of morphogenesis in genitalia 1 (SMG1) inactivates the AKT/mTOR signaling pathway in various cancers. Remarkably, GAS5 overexpression competitively binds miR362-5p and therefore supports an active effect of SMG1, facilitating apoptosis of 131I-resistant TC via the dephosphorylated Akt/mTOR signal. This mechanism almost indicates that GAS5 is involved in the regulation of radiotherapy sensitivity with TC cells (53).
Ovarian Carcinoma
Both GAS5 and ARID1A (AT-rich interactive domain 1A) are underexpressed in ovarian clear cell carcinoma (OCCC). Aberrant loss of ARID1A expression in most patients with OCCC, resulting in loss of the expression of BRG-associated factor 250a (BAF250a) protein, is associated with a poor prognosis in OCCC. Further research indicated that GAS5 could binds with miR-31-5p and significantly upregulate ARID1A to suppress the viability and invasion of OCCC cells (54). Recently, GAS5 overexpression has been shown to inhibit the proliferation and migration ability of ovarian carcinoma (OC). Mechanically, GAS5 binds miR-96-5p as a ceRNA. Further research confirmed that miR-96-5p specifically decreased the expression of PTEN (55). Similarly, Zhao et al. demonstrated that GAS5 could act as a key target of miR-196-5p to downregulating the expression of HOXA5. Abnormal deletion of HOXA5 is implicated in proliferation and apoptosis in OC. Thus, the GAS5/miR-196a-5p/HOXA5 signaling pathway may be a novel lncRNA-based strategy to improve the prognosis of OC (56).
Mesothelioma
Malignant pleural mesothelioma (MPM) is a rare tumor caused primarily by exposure to asbestos. A previous study indicated that the expression level of GAS5 is lower in MPM compared to normal mesothelial cells. Interestingly, decreased GAS5 increases the expression of genes that respond to glucocorticoid receptor in MPM. Studies have shown that glucocorticoids play a vital role in the progression of MPM, GAS5 may inhibit the transcription of glucocorticoid-responsive genes through its structural glucocorticoid receptor response element (57).
Conclusions and Future Perspectives
We have sought to find an overall understanding of the mechanism in the GAS5-mediated cancer process (Table 1), which can offer a perspective on revealing the complex biology of cancer. As illustrated in this review, the lncRNA GAS5 has been dysregulated in several types of cancer and is reported to significantly decrease in most types of cancers. These works are closely paving the way for a GAS5-related clinical phenotype and a poor prognosis in cancer patients. The molecular mechanism clarified that GAS5 interacted with several miRNAs to deliver suppressive information, resulting in uncontrollable interactions of many signaling molecules and pathways, including the SUFU signaling, Wnt/β-catenin signaling, and PI3K/AKT signaling pathways. Other GAS5 mechanistic models have identified that cancer cells may further deteriorate due to the dysregulation of autophagy, oxidative stress, and immunity. Furthermore, GAS5 can also regulate sensitivity to chemoradiation, demonstrating that GAS5 has potential clinical application value for tumor therapy.
Many studies highlight that GAS5 acts as a sponge to regulate various miRNAs. However, the specific mechanisms are rarely discussed. Previous research predicted that GAS5 has a miR-1323 binding site and demonstrated it by luciferase reporter assays (43),which indicated that GAS5 base pair has a particular complementary sequence with miRNA. Additionally, we predict that RNA-induced silencing complex (RISC) may play an important role in binding GAS5 and miRNA, a primary miRNA transcript (pri-miRNA) that undergoes cleavage, transport, and cleavage into mature miRNAs, which are finally incorporated into the RISC. Zhang et al. detected that over expression of GAS5 induces the reduction of mature miR-21. However, pri-miR-21 and pre-miR-21 are not affected by GAS5. Therefore, GAS5 may regulate miRNAs in the RISC complex via a post-transcriptional pathway. Furthermore, they detected that both GAS5 and miR-21 are enriched in AGO2 - an essential part of RISC (58). The combination of GAS5 and miR-21 in RISC may suggest why GAS5 easily binds miRNA, but the related mechanism needs further research to verify.
In the present review, there is an essential general trend to explore the interaction between GAS5 and intracellular oncogenes. Such observations suggest that GAS5 was portrayed as a tumor suppressor in the cancer cell cycle. It is also observed that some activators partly regulate the level of GAS5, and this may provide a foundational theoretical basis for explaining the occurrence and development of malignant tumors. However, the interaction between GAS5 and the molecules presents diversity and complexity in the tumor microenvironment, and a complete understanding of the mechanism network is still far beyond our reach. Therefore, further searching for highly sensitive and specific biomolecular markers that interact with GAS5 exists is of great clinical research value.
Author Contributions
GL and TW contributed to conceptualization and full-text writing. XG, ZH and WN contributed to critical reading of the manuscript. All authors read and approved the final manuscript.
Conflict of Interest
The authors declare that the research was conducted in the absence of any commercial or financial relationships that could be construed as a potential conflict of interest.
Publisher’s Note
All claims expressed in this article are solely those of the authors and do not necessarily represent those of their affiliated organizations, or those of the publisher, the editors and the reviewers. Any product that may be evaluated in this article, or claim that may be made by its manufacturer, is not guaranteed or endorsed by the publisher.
References
1. Cheng J. Transcriptional Maps of 10 Human Chromosomes at 5-Nucleotide Resolution. Science (2005) 308(5725):1149–54. doi: 10.1126/science.1108625
2. Cech TR, Steitz JA. The Noncoding RNA Revolution-Trashing Old Rules to Forge New Ones. Cell (2014) 157(1):77–94. doi: 10.1016/j.cell.2014.03.008
3. Kapranov P, Cheng J, Dike S, Nix DA, Duttagupta R, Willingham AT, et al. RNA Maps Reveal New RNA Classes and a Possible Function for Pervasive Transcription. Science (2007) 316(5830):1484–8. doi: 10.1126/science.1138341
4. Brannan CI, Dees EC, Ingram RS, Tilghman SM. The Product of the H19 Gene may Function as an RNA. Mol Cell Biol (1990) 10(1):28–36. doi: 10.1128/mcb.10.1.28-36.1990
5. Shi X, Sun M, Liu H, Yao Y, Song Y. Long non-Coding RNAs: A New Frontier in the Study of Human Diseases. Cancer Letters (2013) 3 39(2):159–66. doi: 10.1016/j.canlet.2013.06.013
6. Kopp F, Mendell JT. Functional Classification and Experimental Dissection of Long Noncoding RNAs. Cell (Cambridge) (2018) 172(3):393–407. doi: 10.1016/j.cell.2018.01.011
7. Wilusz JE, Sunwoo H, Spector DL. Long Noncoding RNAs: Functional Surprises From the RNA World. Genes Dev (2009) 23(13):1494–504. doi: 10.1101/gad.1800909
8. Schneider C, King RM, Philipson L. Genes Specifically Expressed at Growth Arrest of Mammalian Cells. Cell (Cambridge) (1988) 54(6):787–93. doi: 10.1016/S0092-8674(88)91065-3
9. Yu Y, Hann SS. Novel Tumor Suppressor lncRNA Growth Arrest-Specific 5 (GAS5) In Human Cancer. OncoTarg Ther (2019) 12:8421–36. doi: 10.2147/OTT.S221305
10. Tornesello ML, Faraonio R, Buonaguro L, Annunziata C, Starita N, Cerasuolo A, et al. The Role of microRNAs, Long Non-Coding RNAs, and Circular RNAs in Cervical Cancer. Front Oncol (2020) 10. doi: 10.3389/fonc.2020.00150
11. Pickard MR, Williams GT. Molecular and Cellular Mechanisms of Action of Tumour Suppressor GAS5 LncRNA. Genes (Basel) (2015) 6(3):484–99. doi: 10.3390/genes6030484
12. Smith CM, Steitz JA. Classification of Gas5 as a Multi-Small-Nucleolar-RNA (snoRNA) Host Gene and a Member of the 5'-Terminal Oligopyrimidine Gene Family Reveals Common Features of snoRNA Host Genes. Mol Cell Biol (1998) 18(12):6897–909. doi: 10.1128/MCB.18.12.6897
13. Mazar J, Rosado A, Shelley J, Marchica J, Westmoreland TJ. The Long Non-Coding RNA GAS5 Differentially Regulates Cell Cycle Arrest and Apoptosis Through Activation of BRCA1 and P53 in Human Neuroblastoma. Oncotarget (2017) 8(4):6589–607. doi: 10.18632/oncotarget.14244
14. Gelbert LM, Cai S, Lin X, Sanchez-Martinez C, Del PM, Lallena MJ, et al. Preclinical Characterization of the CDK4/6 Inhibitor LY2835219: in-Vivo Cell Cycle-Dependent/Independent Anti-Tumor Activities Alone/in Combination With Gemcitabine. Invest New Drugs (2014) 32(5):825–37. doi: 10.1007/s10637-014-0120-7
15. Tokgun PE, Tokgun O, Kurt S, Tomatir AG, Akca H. MYC-Driven Regulation of Long non-Coding RNA Profiles in Breast Cancer Cells. Gene (2019) 714:143955. doi: 10.1016/j.gene.2019.143955
16. Pickard MR, Williams GT. The Hormone Response Element Mimic Sequence of GAS5 lncRNA is Sufficient to Induce Apoptosis in Breast Cancer Cells. Oncotarget (2016) 7(9):10104–16. doi: 10.18632/oncotarget.7173
17. Arshi A, Sharifi FS, Khorramian GM, Faghih Z, Doosti A, Ostovari S, et al. Expression Analysis of MALAT1, GAS5, SRA, and NEAT1 lncRNAs in Breast Cancer Tissues From Young Women and Women Over 45 Years of Age. Mol Ther Nucleic Acids (2018) 12:751–7. doi: 10.1016/j.omtn.2018.07.014
18. Li Y, Guo XB, Wei YH. LncRNA GAS5 Affects Epithelial-Mesenchymal Transition and Invasion of Breast Cancer Cells by Regulating miR-216b. Eur Rev Med Pharmacol Sci (2020) 24(9):4873–81. doi: 10.26355/eurrev_202005_21176
19. Onorati AV, Dyczynski M, Ojha R, Amaravadi RK. Targeting Autophagy in Cancer. Cancer (2018) 124(16):3307–18. doi: 10.1002/cncr.31335
20. Folkerts H, Hilgendorf S, Vellenga E, Bremer E, Wiersma VR. The Multifaceted Role of Autophagy in Cancer and the Microenvironment. Med Res Rev (2019) 39(2):517–60. doi: 10.1002/med.21531
21. Li G, Qian L, Tang X, Chen Y, Zhao Z, Zhang C. Long Noncoding RNA Growth Arrestspecific 5 (GAS5) Acts as a Tumor Suppressor by Promoting Autophagy in Breast Cancer. Mol Med Rep (2020) 22(3):2460–8. doi: 10.3892/mmr.2020.11334
22. Chen Z, Pan T, Jiang D, Jin L, Geng Y, Feng X, et al. The lncRNA-GAS5/miR-221-3p/DKK2 Axis Modulates ABCB1-Mediated Adriamycin Resistance of Breast Cancer via the Wnt/beta-Catenin Signaling Pathway. Mol Ther Nucleic Acids (2020) 19:1434–48. doi: 10.1016/j.omtn.2020.01.030
23. Gu J, Wang Y, Wang X, Zhou D, Shao C, Zhou M, et al. Downregulation of lncRNA GAS5 Confers Tamoxifen Resistance by Activating miR-222 in Breast Cancer. Cancer Lett (2018) 434:1–10. doi: 10.1016/j.canlet.2018.06.039
24. Li S, Zhou J, Wang Z, Wang P, Gao X, Wang Y. Long Noncoding RNA GAS5 Suppresses Triple Negative Breast Cancer Progression Through Inhibition of Proliferation and Invasion by Competitively Binding miR-196a-5p. Biomed Pharmacother (2018) 104:451–7. doi: 10.1016/j.biopha.2018.05.056
25. Zheng S, Li M, Miao K, Xu H. lncRNA GAS5-Promoted Apoptosis in Triple-Negative Breast Cancer by Targeting miR-378a-5p/SUFU Signaling. J Cell Biochem (2020) 121(3):2225–35. doi: 10.1002/jcb.29445
26. Dong L, Li G, Li Y, Zhu Z. Upregulation of Long Noncoding RNA GAS5 Inhibits Lung Cancer Cell Proliferation and Metastasis via miR-205/PTEN Axis. Med Sci Monit (2019) 25:2311–9. doi: 10.12659/MSM.912581
27. Xue Y, Ni T, Jiang Y, Li Y. Long Noncoding RNA GAS5 Inhibits Tumorigenesis and Enhances Radiosensitivity by Suppressing miR-135b Expression in Non-Small Cell Lung Cancer. Oncol Res (2017) 25(8):1305–16. doi: 10.3727/096504017X14850182723737
28. Poulet C, Njock MS, Moermans C, Louis E, Louis R, Malaise M, et al. Exosomal Long Non-Coding RNAs in Lung Diseases. Int J Mol Sci (2020) 21(10):3580. doi: 10.3390/ijms21103580
29. Cheng Y, Dai X, Yang T, Zhang N, Liu Z, Jiang Y. Low Long Noncoding RNA Growth Arrest-Specific Transcript 5 Expression in the Exosomes of Lung Cancer Cells Promotes Tumor Angiogenesis. J Oncol (2019) 2019:2476175. doi: 10.1155/2019/2476175
30. Zhu L, Zhu Q, Wen H, Huang X, Zheng G. Mutations in GAS5 Affect the Transformation From Benign Prostate Proliferation to Aggressive Prostate Cancer by Affecting the Transcription Efficiency of GAS5. J Cell Physiol (2019) 234(6):8928–40. doi: 10.1002/jcp.27561
31. Li J, Wang Y, Zhang CG, Xiao HJ, Xiao HJ, Hu JM, et al. Effect of Long non-Coding RNA Gas5 on Proliferation, Migration, Invasion and Apoptosis of Colorectal Cancer HT-29 Cell Line. Cancer Cell Int (2018) 18:4. doi: 10.1186/s12935-017-0478-7
32. Cheng K, Zhao Z, Wang G, Wang J, Zhu W. lncRNA GAS5 Inhibits Colorectal Cancer Cell Proliferation via the Mir1825p/FOXO3a Axis. Oncol Rep (2018) 40(4):2371–80. doi: 10.3892/or.2018.6584
33. Zhang HG, Wang FJ, Wang Y, Zhao ZX, Qiao PF. lncRNA GAS5 Inhibits Malignant Progression by Regulating Macroautophagy and Forms a Negative Feedback Regulatory Loop With the Mir34a/mTOR/SIRT1 Pathway in Colorectal Cancer. Oncol Rep (2021) 45(1):202–16. doi: 10.3892/or.2021.8153
34. Ni W, Yao S, Zhou Y, Liu Y, Huang P, Zhou A, et al. Long Noncoding RNA GAS5 Inhibits Progression of Colorectal Cancer by Interacting With and Triggering YAP Phosphorylation and Degradation and is Negatively Regulated by the M(6)A Reader YTHDF3. Mol Cancer (2019) 18(1):143. doi: 10.1186/s12943-019-1079-y
35. Xu W, Yan Z, Hu F, Wei W, Yang C, Sun Z. Long non-Coding RNA GAS5 Accelerates Oxidative Stress in Melanoma Cells by Rescuing EZH2-Mediated CDKN1C Downregulation. Cancer Cell Int (2020) 20:116. doi: 10.1186/s12935-020-01167-1
36. Moloney JN, Cotter TG. ROS Signalling in the Biology of Cancer. Semin Cell Dev Biol (2018) 80:50–64. doi: 10.1016/j.semcdb.2017.05.023
37. Chen L, Yang H, Yi Z, Jiang L, Li Y, Han Q, et al. LncRNA GAS5 Regulates Redox Balance and Dysregulates the Cell Cycle and Apoptosis in Malignant Melanoma Cells. J Cancer Res Clin Oncol (2019) 145(3):637–52. doi: 10.1007/s00432-018-2820-4
38. Avgeris M, Tsilimantou A, Levis PK, Tokas T, Sideris DC, Stravodimos K, et al. Loss of GAS5 Tumour Suppressor lncRNA: An Independent Molecular Cancer Biomarker for Short-Term Relapse and Progression in Bladder Cancer Patients. Br J Cancer (2018) 119(12):1477–86. doi: 10.1038/s41416-018-0320-6
39. Chen D, Guo Y, Chen Y, Guo Q, Chen J, Li Y, et al. LncRNA Growth Arrest-Specific Transcript 5 Targets miR-21 Gene and Regulates Bladder Cancer Cell Proliferation and Apoptosis Through PTEN. Cancer Med (2020) 9(8):2846–58. doi: 10.1002/cam4.2664
40. Zhang H, Guo Y, Song Y, Shang C. Long Noncoding RNA GAS5 Inhibits Malignant Proliferation and Chemotherapy Resistance to Doxorubicin in Bladder Transitional Cell Carcinoma. Cancer Chemother Pharmacol (2017) 79(1):49–55. doi: 10.1007/s00280-016-3194-4
41. Li K, Chen Y. CYP2C8 Regulated by GAS5/miR-382-3p Exerts Anti-Cancerous Properties in Liver Cancer. Cancer Biol Ther (2020) 21(12):1145–53. doi: 10.1080/15384047.2020.1840886
42. Yang L, Jiang J. GAS5 Regulates RECK Expression and Inhibits Invasion Potential of HCC Cells by Sponging miR-135b. BioMed Res Int (2019) 2019:2973289. doi: 10.1155/2019/2973289
43. Zhang F, Yang C, Xing Z, Liu P, Zhang B, Ma X, et al. LncRNA GAS5-Mediated miR-1323 Promotes Tumor Progression by Targeting TP53INP1 in Hepatocellular Carcinoma. Onco Targets Ther (2019) 12:4013–23. doi: 10.2147/OTT.S209439
44. Fang P, Xiang L, Chen W, Li S, Huang S, Li J, et al. LncRNA GAS5 Enhanced the Killing Effect of NK Cell on Liver Cancer Through Regulating miR-544/Runx3. Innate Immun (2019) 25(2):99–109. doi: 10.1177/1753425919827632
45. Li Y, Gu J, Lu H. The GAS5/miR-222 Axis Regulates Proliferation of Gastric Cancer Cells Through the PTEN/Akt/mTOR Pathway. Dig Dis Sci (2017) 62(12):3426–37. doi: 10.1007/s10620-017-4831-4
46. Liu Y, Yin L, Chen C, Zhang X, Wang S. Long non-Coding RNA GAS5 Inhibits Migration and Invasion in Gastric Cancer via Interacting With P53 Protein. Dig Liver Dis (2020) 52(3):331–8. doi: 10.1016/j.dld.2019.08.012
47. Li Q, Ma G, Sun S, Xu Y, Wang B. Polymorphism in the Promoter Region of lncRNA GAS5 is Functionally Associated With the Risk of Gastric Cancer. Clin Res Hepatol Gastroenterol (2018) 42(5):478–82. doi: 10.1016/j.clinre.2018.01.006
48. Yan Z, Ruoyu L, Xing L, Hua L, Jun Z, Yaqin P, et al. Long non-Coding RNA GAS5 Regulates the Growth and Metastasis of Human Cervical Cancer Cells via Induction of Apoptosis and Cell Cycle Arrest. Arch Biochem Biophys (2020) 684:108320. doi: 10.1016/j.abb.2020.108320
49. Yang W, Xu X, Hong L, Wang Q, Huang J, Jiang L. Upregulation of lncRNA GAS5 Inhibits the Growth and Metastasis of Cervical Cancer Cells. J Cell Physiol (2019) 234(12):23571–80. doi: 10.1002/jcp.28926
50. Yao T, Lu R, Zhang J, Fang X, Fan L, Huang C, et al. Growth Arrest-Specific 5 Attenuates Cisplatin-Induced Apoptosis in Cervical Cancer by Regulating STAT3 Signaling via miR-21. J Cell Physiol (2019) 234(6):9605–15. doi: 10.1002/jcp.27647
51. Gao J, Liu L, Li G, Cai M, Tan C, Han X, et al. LncRNA GAS5 Confers the Radio Sensitivity of Cervical Cancer Cells via Regulating miR-106b/IER3 Axis. Int J Biol Macromol (2019) 126:994–1001. doi: 10.1016/j.ijbiomac.2018.12.176
52. Guo LJ, Zhang S, Gao B, Jiang Y, Zhang XH, Tian WG, et al. Low Expression of Long non-Coding RNA GAS5 is Associated With Poor Prognosis of Patients With Thyroid Cancer. Exp Mol Pathol (2017) 102(3):500–4. doi: 10.1016/j.yexmp.2017.05.008
53. Li L, Lin X, Xu P, Jiao Y, Fu P. LncRNA GAS5 Sponges miR-362-5p to Promote Sensitivity of Thyroid Cancer Cells to (131) I by Upregulating SMG1. IUBMB Life (2020) 72(11):2420–31. doi: 10.1002/iub.2365
54. Zhang J, Yang ZM, Huang Y, Wang KN, Xie Y, Yang N. LncRNA GAS5 Inhibits the Proliferation and Invasion of Ovarian Clear Cell Carcinoma via the miR-31-5p/ARID1A Axis. Kaohsiung J Med Sci (2021) 37(11):940–50. doi: 10.1002/kjm2.12420
55. Dong Q, Long X, Cheng J, Wang W, Tian Q, Di W. LncRNA GAS5 Suppresses Ovarian Cancer Progression by Targeting the miR-96-5p/PTEN Axis. Ann Transl Med (2021) 9(24):1770. doi: 10.21037/atm-21-6134
56. Zhao H, Yu H, Zheng J, Ning N, Tang F, Yang Y, et al. Lowly-Expressed lncRNA GAS5 Facilitates Progression of Ovarian Cancer Through Targeting miR-196-5p and Thereby Regulating HOXA5. Gynecol Oncol (2018) 151(2):345–55. doi: 10.1016/j.ygyno.2018.08.032
57. Renganathan A, Kresoja-Rakic J, Echeverry N, Ziltener G, Vrugt B, Opitz I, et al. GAS5 Long non-Coding RNA in Malignant Pleural Mesothelioma. Mol Cancer (2014) 13:119. doi: 10.1186/1476-4598-13-119
Keywords: growth arrest specific transcript 5, long non-coding RNA, neoplasms, neoplasm invasiveness, prognosis
Citation: Lin G, Wu T, Gao X, He Z and Nong W (2022) Research Progress of Long Non-Coding RNA GAS5 in Malignant Tumors. Front. Oncol. 12:846497. doi: 10.3389/fonc.2022.846497
Received: 31 December 2021; Accepted: 30 May 2022;
Published: 28 June 2022.
Edited by:
Jing Zhang, Shanghai Jiao Tong University, ChinaReviewed by:
Petar Ozretić, Rudjer Boskovic Institute, CroatiaArun Renganathan, Washington University in St. Louis, United States
Copyright © 2022 Lin, Wu, Gao, He and Nong. This is an open-access article distributed under the terms of the Creative Commons Attribution License (CC BY). The use, distribution or reproduction in other forums is permitted, provided the original author(s) and the copyright owner(s) are credited and that the original publication in this journal is cited, in accordance with accepted academic practice. No use, distribution or reproduction is permitted which does not comply with these terms.
*Correspondence: Wenwei Nong, bnd3b25lQDE2My5jb20=
†These authors have contributed equally to this work