- 1The Shraga Segal Department of Microbiology, Immunology and Genetics, Faculty of Health Sciences, Ben-Gurion University of the Negev, Beer-Sheva, Israel
- 2Joyce & Irving Goldman Medical School, Faculty of Health Sciences, Ben-Gurion University of the Negev, Beer-Sheva, Israel
- 3Department of Public Health, Faculty of Health Sciences, Ben-Gurion University of the Negev, Beer-Sheva, Israel
In light of the development of RAS inhibitors, a reliable assessment of the prevalence of RAS mutations and their correlation with the clinical features of patients with HNC is crucially needed. This meta-analysis compiles the findings of 149 studies with over 8500 HNC patients and assesses the global prevalence of mutations in the HRAS, KRAS and NRAS genes. The available data were stratified according to geographical region, clinical features, and tumor characteristics, including human papillomavirus (HPV) infection status and tumor stage. In addition, the distribution of codon substitutions in each RAS gene was assessed. The estimated mutation rate is highest for HRAS (7%), followed by KRAS (2.89%) and NRAS (2.20%). HRAS prevalence in South Asia (15.28%) is twice as high as the global estimate. HRAS mutations are more prevalent in oral cavity and salivary gland tumors. In contrast, KRAS mutations are found more frequently in sinonasal tumors, and NRAS mutations are found chiefly in tumors of the nasopharynx. OR analyses show a significant association between HRAS mutations and a high tumor stage (OR=3.63). In addition, there is a significant association between HPV-positive status and KRAS mutations (OR=2.09). This study highlights RAS as a potential therapeutic target in certain subsets of HNC patients.
1 Introduction
Head and neck cancer (HNC) includes neoplasms that arise in the oral cavity, pharynx, larynx, sinuses, nasal cavity, and salivary glands (1). The main risk factors associated with HNC include tobacco smoking, alcohol abuse, and human papillomavirus (HPV) infection. Other risk factors include exposure to wood and leather dust, Epstein Barr Virus (EBV) infection, and betel nut chewing (2). In recent decades intensive research has confirmed that HNC is exceptionally heterogeneous at the molecular level, and there is no single genetic alteration or a unique dysregulated molecular pathway responsible for its development and progression (3–5). This heterogeneity may explain the limited efficiency of current systemic therapies for HNC, which emphasizes the need to study specific and less common genetic alterations that may affect disease characteristics and clinical outcomes in HNC patients.
RAS GTPase family proteins are crucial players in many signaling networks, controlling cell proliferation, differentiation, and survival (6). The RAS family members, HRAS, KRAS, and NRAS, share significant sequence homology and largely overlapping functions (7). Mutations in RAS family members are well-established drivers of cancer. Gain-of-function mutations in RAS genes are found in ∼19% of human cancers, most clustering in three hotspots at codons 12, 13, and 61 (8). The immense effort invested in the development of RAS inhibitors has led to several breakthroughs in recent years, allowing for the targeted treatment of patients with alterations in these RAS genes (9, 10), including HNC patients (11–13).
Many studies reported on the frequency of HRAS, KRAS, and NRAS mutational status in HPV-positive and HPV-negative HNC patients. Even though mutations in the members of the RAS gene family are seemingly rare in the general HNC patient population, these studies vary in their assessments on the prevalence of mutations in RAS genes. Therefore, the purpose of the current study was to conduct the first systematic review and meta-analysis evaluating the prevalence of mutations in RAS genes in HNC. By collecting data on over 8500 patients from 149 studies, we were able to reveal differences in the prevalence of RAS mutations between geographical regions, anatomical sites, stage of disease, and HPV status. Moreover, in light of the clinical development of codon-specific RAS inhibitors (namely, G12C and G12D), we have included a comprehensive analysis of codon substitution in RAS mutations.
2 Methods
This systematic review adhered to the Preferred Reporting Items for Systematic Reviews and Meta-Analyses (PRISMA) Checklist (14).
2.1 Study Design
We evaluated the prevalence of mutations in HRAS, KRAS, and NRAS genes in HNC patients.
2.2 Search Strategy
A systematic review of the literature was conducted by searching the PubMed, Embase, Web of Science, and Cochrane Central Register of Controlled Trials databases in June 2021 for studies published since 1 January 2000. The strings used in the systemic search of databases are detailed in the Supplementary Methods section. The bibliographies of retrieved studies and systematic reviews identified in the search were screened for relevant references. Publicly available databases were screened for unpublished data.
2.3 Selection Criteria
The inclusion criteria for the meta-analysis were that the study had to include a mutational analysis of at least one of the target genes (HRAS, KRAS, or NRAS) and a report the prevalence and frequency of mutations as an outcome measure. Exclusion criteria were defined as: 1) Studies displaying results from patients with tumors other than HNCs or mutations other than those in the target genes; 2) studies that did not report data related to the prevalence or frequency of mutations in the target genes; 3) studies that did not evaluate target genes for somatic mutations; 4) studies published before 1 January 2000; 5) studies that were conducted using cell lines or animal models; 6) studies of pediatric populations; 7) review articles, letters, personal opinions, book chapters, or conference abstracts; 8) studies containing data included in other studies or studies in which it was not possible to determine whether duplicate data were included; and 9) studies enrolling fewer than ten patients.
2.4 Data Extraction
Two researchers (SJ, ON) screened the studies at the title and abstract level, followed by a full-text review. Disagreements over inclusion were resolved by consensus adjudication, and studies were extracted into a standardized extraction database. Extracted variables included study cohort size, number of mutated cases for each RAS family gene, primary tumor location, tumor grade or stage, geographical origin of studied patients, mutation assessment method, mutated codon, HPV status, and biopsy type, if reported.
2.5 Evaluation of Quality and Risk of Bias
Our study selection process excluded individual case reports and cohorts of less than ten patients due to the risk of bias. All papers considered after initial screening were reviewed and scored for risk of bias according to the Joanna Briggs Institute Critical Appraisal Checklist for Studies Reporting Prevalence Data (15). Studies that did not evaluate all three RAS family members were considered more prone to risk of bias and were not included in the general prevalence analysis. In addition, publication bias and heterogeneity were assessed by visual inspection of funnel plots and via Egger’s regression test (16) (Figure S1 in the Supplementary Material).
2.6 Statistical Analysis
Pooled prevalence rates, pooled odds ratios (ORs), and forest plots were generated using the R Meta and MetaFor Packages (17, 18). The Cochrane Q chi-squared test and the inconsistency index statistic (I2) were used to examine the heterogeneity across studies. Fixed-effects models were used to assess the pooled prevalence of genes for results with low heterogeneity (I2 ≤ 50%). Otherwise, random-effects models were used for the analyses. A sensitivity analysis using a “leave-one-out” paradigm from the built-in function in MetaFor, as proposed by Wang et al. (19), was used to assess each study’s effect on the overall pooled prevalence and detected outliers (19). First, the pooled overall prevalence of mutations in the three different target genes (KRAS, HRAS, NRAS) was calculated with a corresponding 95% confidence interval (95% CI). Next, subgroup analyses were performed according to geographical region, mutated codon position and anatomical site. Finally, we assessed the association between the RAS gene mutational status and HPV status or tumor grade using the R MetaBin function.
3 Results
3.1 Study Selection
The flow diagram shown in Figure 1 depicts the search strategy and study selection process. A total of 867 studies were retrieved from four electronic databases and a bibliography screen. After the removal of duplicates, 375 studies were considered potentially eligible for evaluation, but 217 did not meet all the inclusion criteria, leaving a final sample of 158 studies. Nine additional studies were excluded due to the high risk of bias. To reduce the risk of bias, only papers with the highest grade (n = 85) were included for pooled analyses of the overall mutation prevalence. The literature references for the studies included in the meta-analysis are listed in Table S1 in the Supplementary Material.
3.2 Study Characteristics
Detailed characteristics of the studies are provided in Table S2 in the Supplementary Material. Of the 149 studies included in the analysis, 112, 130, and 93 contained data pertaining to gene alterations in HRAS, KRAS, and NRAS, respectively, and 85 presented analyses of all three RAS family genes. In total, 148 of the included studies were cohort studies, while one was a phase 1 clinical trial. Forty-seven studies used targeted next-generation sequencing, 46 utilized Sanger sequencing, 23 employed whole-exome sequencing, 9 conducted Mass Array analysis, 4 used whole-genome analyses, and 20 employed other or mixed analysis methods. The anatomical location of the tumors in the included study cohorts are detailed in Table S3 in the Supplementary Material. The studies were conducted in 29 different countries. Four studies included mixed populations from various geographical regions.
3.3 Risk of Bias Within Studies
Nine studies were classified as having a high risk of bias and were therefore excluded from this meta-analysis. Eleven studies were classified as having a moderate risk of bias due to a small cohort size, while 57 studies were classified as having a moderate risk of bias, since they analyzed only one of the three RAS family target genes. The remaining 85 studies were classified as having a low risk of bias and were used in the general prevalence analysis. All low and moderate risk studies were used in prevalence analyses pertaining to tumor anatomical sites, mutated codons, and the association between RAS mutations and patient clinical features.
3.4 Prevalence of RAS Mutations
3.4.1 HRAS Mutations
Mutations in HRAS were identified in 564 tumors from 8501 patients. The mean prevalence of HRAS mutations was 7% (95% CI, 5.38-9.06, p <0.01, I2 = 87%) (Figure 2A). Geographical region-specific analyses revealed significant differences in these rates in different regions of the world (Q = 22.51, Pv <0.0001). The mean frequency of HRAS mutations in South Asia was 15.28%, with this rate being higher than the rates in other geographical regions, including East Asia (5.07%), Europe (4.65%), and North America (6.87) (Figure 3A, Figure S2 in the Supplementary Material).
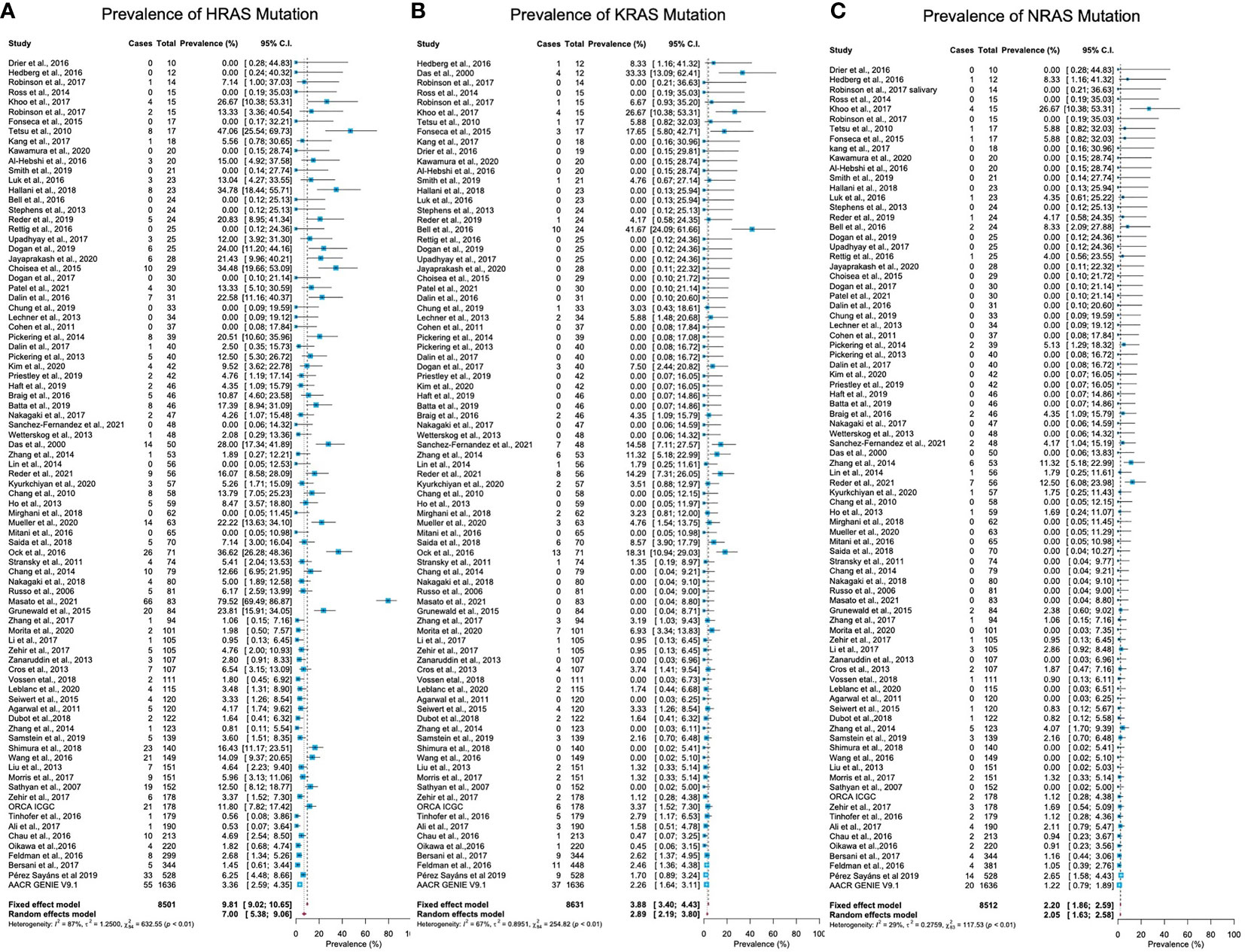
Figure 2 Prevalence of RAS mutations in head and neck cancer. (A) The prevalence of HRAS mutations is 7% (95% CI, 5.38-9.06, p <0.01, I2 = 87%). (B) The prevalence of KRAS mutations is 2.89% (95% CI, 2.19-3.80, p <0.0.1, I2 = 67%). (C) The prevalence of NRAS mutations is 2.20% (95% CI, 1.86-2.59, p <0.01, I2 = 29%). CI: Confidence interval. I2: Inconsistency index.
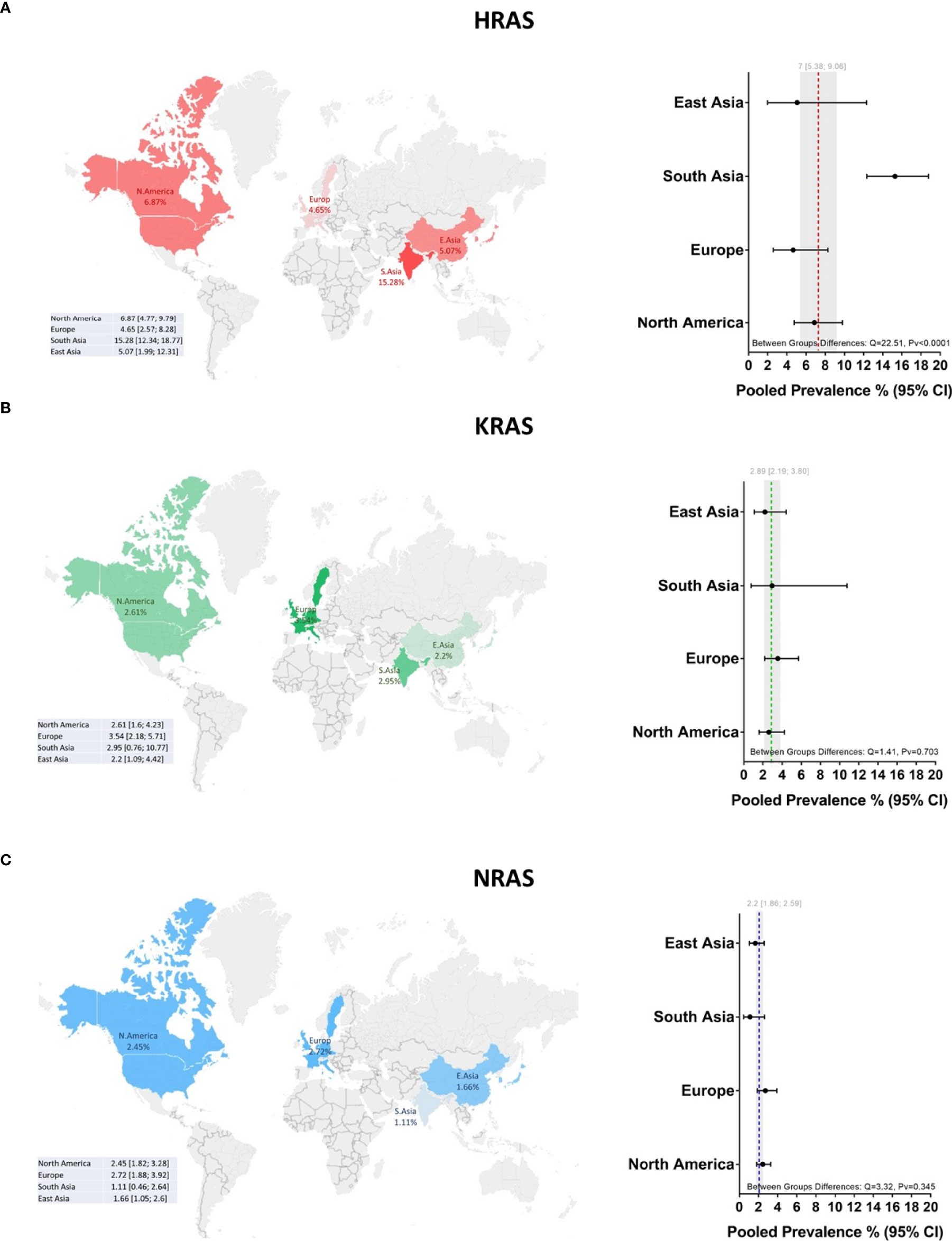
Figure 3 Global prevalence of RAS mutations in head and neck cancer. Cohort studies were grouped according to the geographical origins of the patients. On the map of the world are shown the frequencies [%] of (A) HRAS, (B) KRAS, and (C) NRAS mutations in East Asia, South Asia, Europe, and North America. Dotted lines and gray shading correspond to the overall prevalence and the 95% CI. CI, Confidence interval; I2, Inconsistency index.
3.4.2 KRAS mutations
Mutations in KRAS were identified in 188 tumors from 8631 patients. The mean prevalence of KRAS mutations was 2.89% (95% CI, 2.19-3.80, p <0.0.1, I2 = 67%) (Figure 2B), with no significant differences in prevalence between analyzed geographical regions (Q = 1.41, Pv = 0.7) (Figure 3B, Figure S2 in the Supplementary Material).
3.4.3 NRAS Mutations
Mutations in NRAS were identified in 113 tumors from 8512 patients. The mean prevalence of NRAS mutations was 2.20% (95% CI, 1.86-2.59, p <0.01, I2 = 29%) (Figure 2C). No significant differences in these rates were observed between the different parts of the world (Q = 3.32, Pv = 0.34) (Figure S2 in the Supplementary Material).
3.5 Hot Spot Mutations and Amino Acid Substitutions
3.5.1 HRAS Mutations
In an analysis of all cases with HRAS mutations, 27%, 18%, and 36% were situated in codons 12, 13, and 61, respectively (Figure 4A). Mutations in codon 12 were mostly G12S point mutations (56.3%), while those in codon 13 were primarily G13R point mutations (46.8%). Lastly, mutations found in Q61 were primarily Q61R (49.2%), Q61K (26.4%), and Q61L (22.2%) point mutations (Figure 4B).
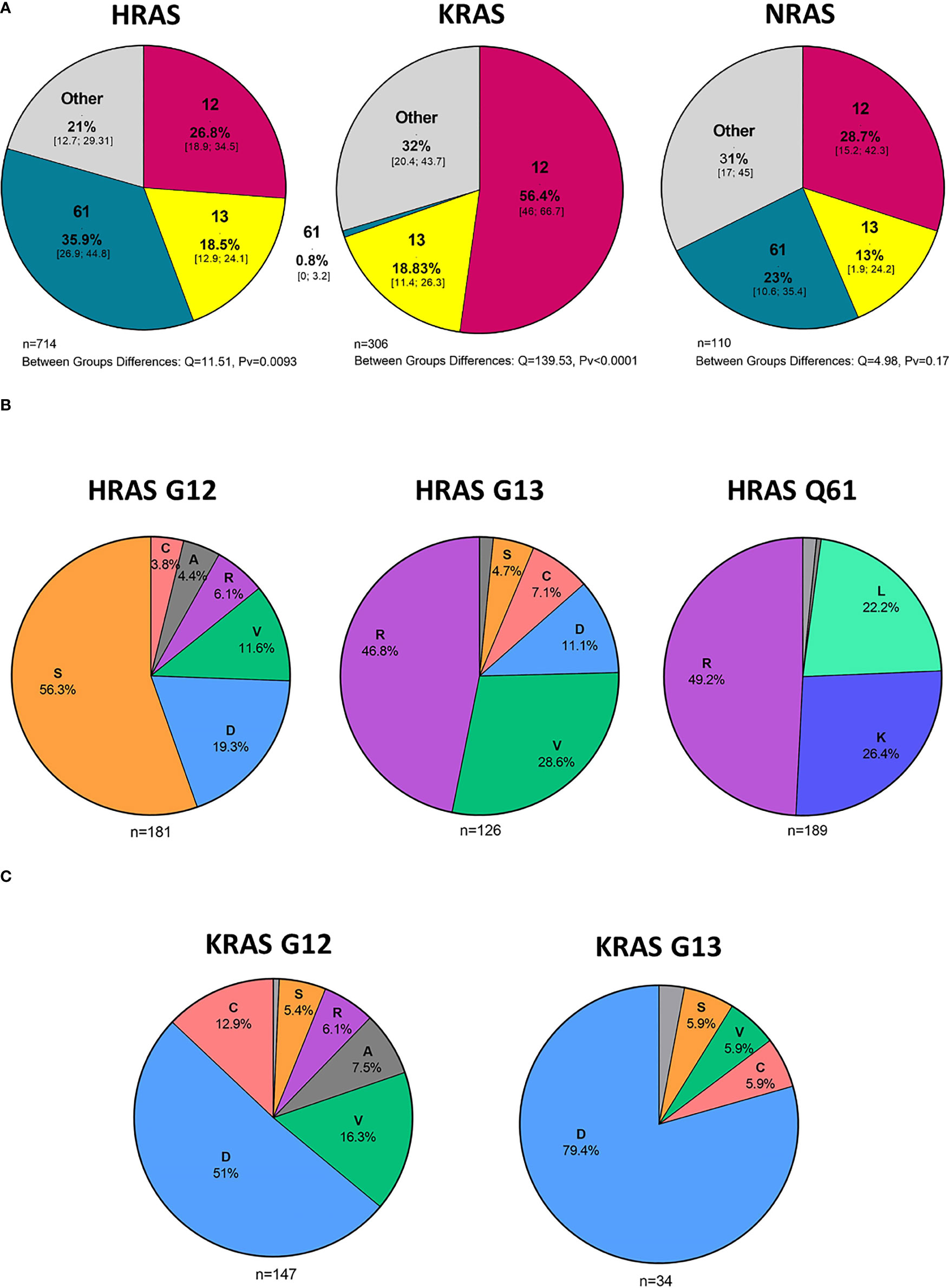
Figure 4 Hot spot mutations and amino acid substitutions. (A) Mutated codons [%] in cases with HRAS, KRAS, and NRAS mutations and 95% CI. (B) Amino acid substitutions [%] in cases with KRAS G12 and G13 mutations. (C) Amino acid substitutions [%] in cases with HRAS G12, G13, and Q61 mutations. D - aspartic acid, C, cysteine; V, valine; S, serine; R, arginine; A, alanine; K, lysine; L, leucine; CI, Confidence interval.
3.5.2 KRAS Mutations
In an analysis of all cases with KRAS mutations, 56%, 19%, and 0.8% were situated in codons 12, 13, and 61, respectively (Figure 4A). Among the codon 12 mutations, the most common amino acid substitution was G12D (51%), followed by G12V (16.3%) and G12C (12.9%) (Figure 4C).
3.5.3 NRAS Mutations
NRAS mutations were more evenly distributed among the different codons, with 29%, 13%, and 23% being situated in codons 12, 13, and 61, respectively. (Figure 4A). Analysis of amino acid substitutions was not feasible for NRAS mutations due to the low number of cases.
3.6 Difference in Prevalence of RAS Mutations Between Anatomical Sites
As HNC includes tumors that arise from a wide range of anatomical sites and sub-sites, an analysis of the frequency of mutations in the three RAS genes was performed for seven major anatomical areas. A summary of these analyses is presented in Figure 5A and Figure S3 in the Supplementary Material.
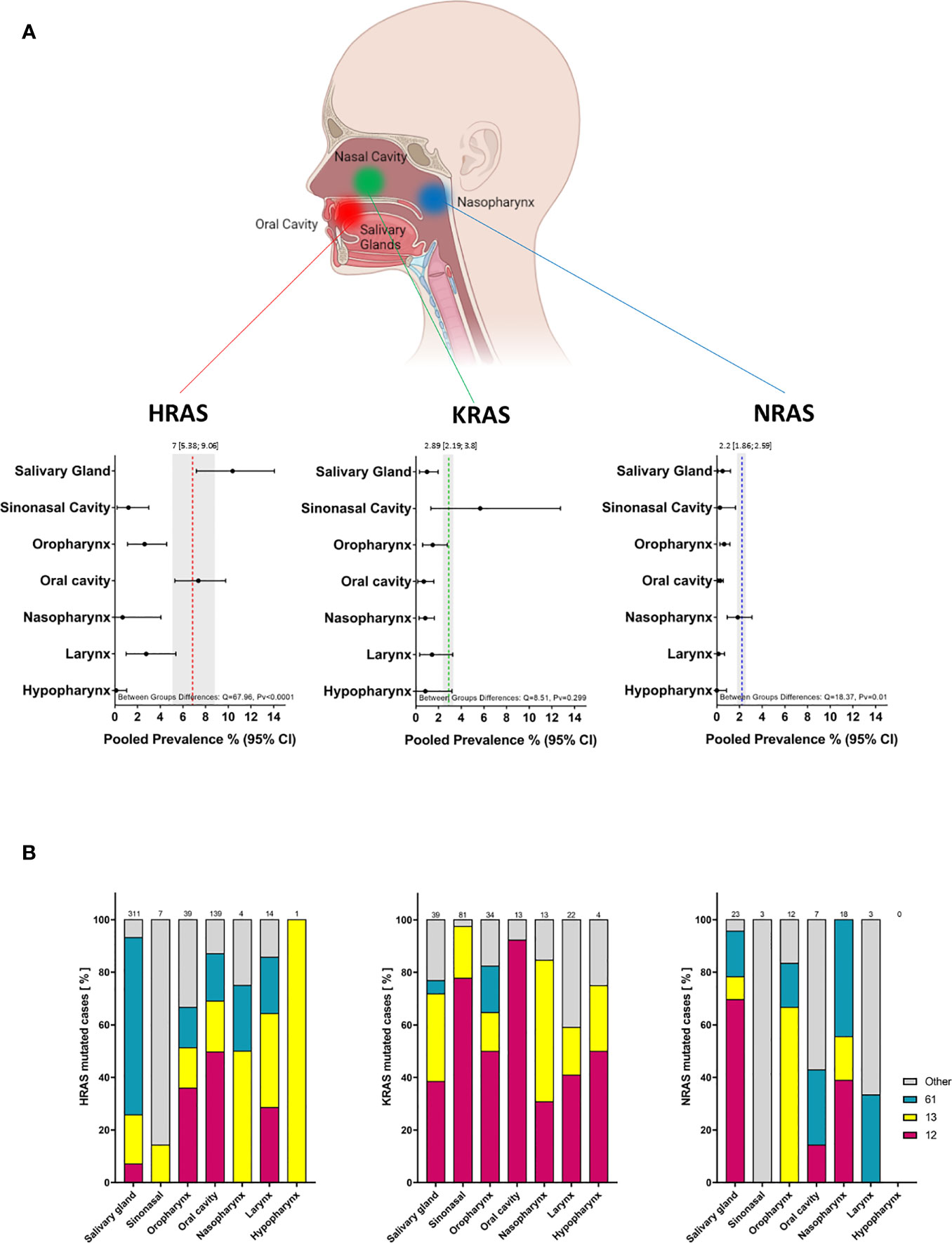
Figure 5 Prevalence of RAS mutations and locations of mutated codons according to tumor anatomical site. (A) Prevalence of RAS mutations according to tumor anatomical site. Dotted lines and gray shading correspond to the overall prevalence and the 95% CI. (B) Mutated codon locations [%] according to tumor anatomical site. CI, Confidence interval; I2, Inconsistency index.
3.6.1 HRAS mutations
A significant difference in the prevalence of HRAS mutations was detected between anatomical sites (Q = 67.96, Pv <0.0001): HRAS mutations were found more frequently in tumors of the salivary glands (10.37%; 95% CI, 7.18-14.06) and oral cavity (7.36%; 95% CI, 5.39-9.76) than in tumors of the sinonasal cavity (1.2%; 95% CI, 0.2-3), oropharynx (2.6%; 95% CI, 1.12-4.56), nasopharynx (0.68%; 95% CI, 0-4.06), larynx (2.76%; 95% CI, 0.99-5.38), or hypopharynx (0.12%; 95% CI, 0-0.04). Salivary gland tumors exhibited a higher frequency of mutations in codon 61 (67%), while in tumors of the oral cavity, mutations in codon 12 were the most frequent (50%) (Figure 5B, left side).
3.6.2 KRAS mutations
A trend towards more frequent KRAS mutations was observed for tumors of the sinonasal cavity (5.67%; 95% CI, 1.33-12.74) as compared to tumors of the salivary glands (0.98%; 95% CI, 0.33-1.96), oral cavity (0.7%; 95% CI, 0.17-1.59), oropharynx (1.49%; 95% CI, 0.6-2.77), nasopharynx (0.83%; 95% CI, 0.29-1.63), larynx (1.43%; 95% CI, 0.34-3.25), or hypopharynx (0.84%; 95% CI, 0-3.18). However, these differences were not robust (Q = 8.5, Pv = 0.29). Mutations in codon 12 were the most frequent across all anatomic sites, followed by those in codon 13. Mutations in codon 61 were primarily detected in tumors of the oropharynx (17%) (Figure 5B, middle).
3.6.3 NRAS mutations
A significant difference between anatomical sites was also seen for NRAS mutations (Q = 18.37, Pv = 0.01), with a rate of 1.85% (95% CI, 0.92-3.1) in the nasopharynx compared to lower rates in tumors of the salivary glands (0.51%; 95% CI, 0.11-1.22), oral cavity (0.3%; 95% CI, 0.11-0.58), sinonasal cavity (0.28%; 95% CI, 0-1.65), oropharynx (0.65%; 95% CI, 0.28-1.16), larynx (0.16%; 95% CI, 0-0.68), or hypopharynx (0%; 95% CI, 0-0.85).
We note that the analyses of the mutated position in specific anatomical sites, and of the specific amino acid substitution, should be interpreted with caution owing to the limited number of mutated cases.
3.7 Association Between RAS Mutations and Disease Stage/Grade
Tumor grade and stage are well-studied prognostic factors for HNC (20). In total, 44 studies reported details of the tumor stage or grade of patients along with the mutation status. Tumors with a stage or grade of 1 and 2 were defined as low-grade tumors, while those with a stage or grade of 3 and 4 were categorized as high-grade tumors. An OR analysis revealed a significant association between HRAS mutation and advanced stage (OR = 3.63; 95% CI, 1.53-8.64) (Figure 6A). KRAS (OR = 2.41; 95% CI, 0.85-6.86) and NRAS (OR = 1.52; 95% CI, 0.68-3.41) mutations were both associated with an OR>1, but the association did not reach statistical significance (Figure S4 in the Supplementary Material).
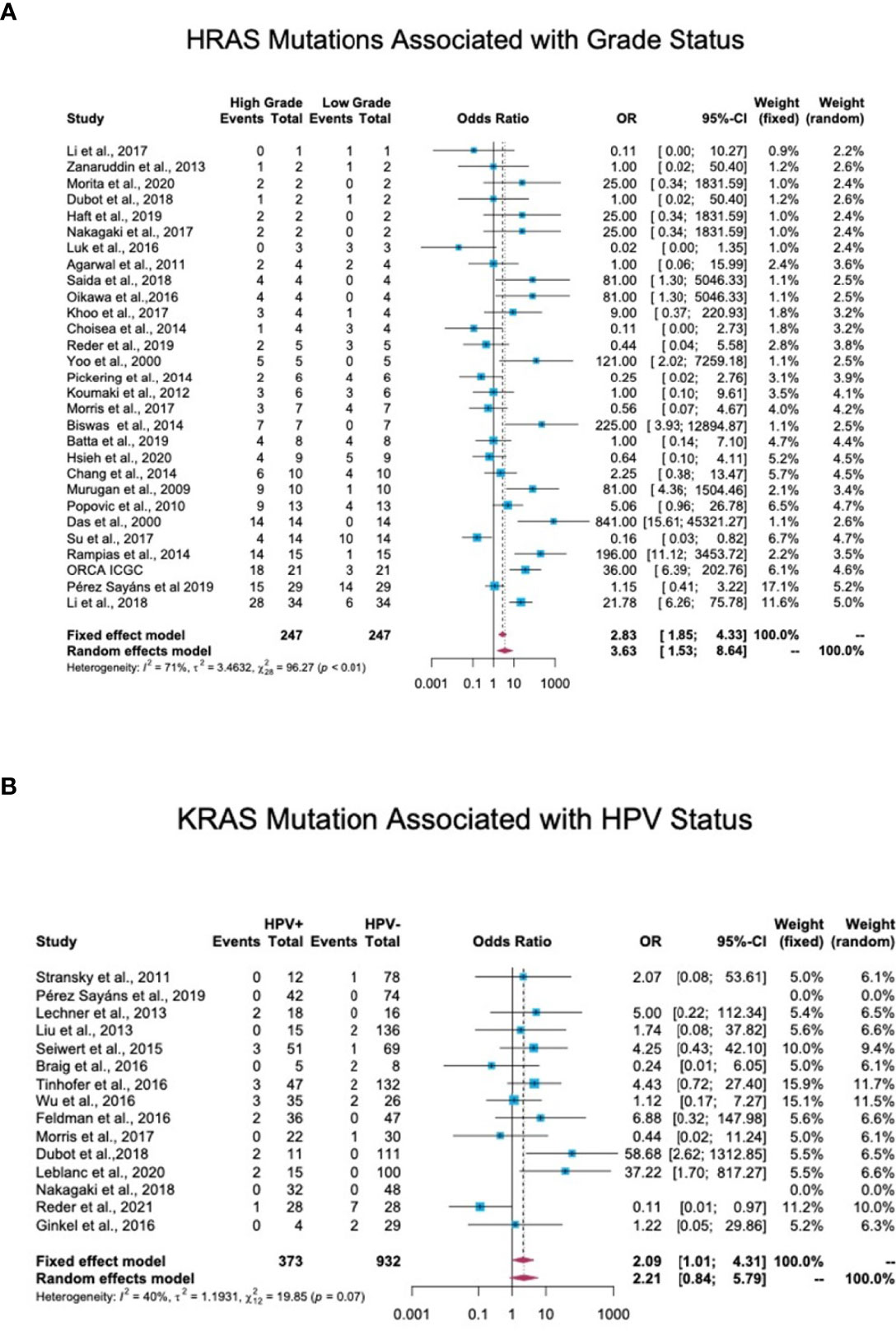
Figure 6 Association between RAS mutations and patients’ clinical features. (A) Association between HRAS mutations and tumor grade. In total, 44 studies reported details regarding tumor stage or grade and mutation status. Stage/grade 1 and 2 tumors were categorized as low-grade, while stage/grade 3 and 4 tumors were categorized as high-grade. An OR analysis exhibited a significant association between HRAS mutation status and advanced stage (OR = 3.63; 95% CI, 1.53-8.64). (B) Association between KRAS mutations and human papillomavirus (HPV) infection status. In total, 17 studies reported the detection of RAS mutations in both HPV-positive and HPV-negative patients. An OR analysis revealed a significant association between KRAS mutation status and HPV infection (OR = 2.09; 95% CI, 1.01-4.31). CI, Confidence interval; I2, Inconsistency index; OR, Odds ratio.
3.8 Association Between RAS Mutations and HPV Status
Of the 38 cohort studies that reported the HPV status of HNC patients, only 25 provided specific patient data, and of these, 17 included both HPV-negative and HPV-positive patients, thus allowing an OR analysis. This analysis revealed a significant association between HPV-positive status and KRAS mutations, with an OR of 2.09 (95% CI, 1.01-4.31) (Figure 6B), but no significant correlation between HPV-positive status and HRAS or NRAS mutations (Figure S5 in the Supplementary Material).
4 Discussion
After years of extensive research, new strategies that target the RAS-MAPK pathway are now opening new therapeutic options for affected patients (9). The meta-analysis presented here compiles findings from the past two decades and provides updated insight into the global prevalence of mutations in RAS family genes, underscoring their potential as therapeutic targets in HNC patients.
The prevalence of mutations was highest for the HRAS gene, followed by KRAS and NRAS. This finding aligns with previous reports of the higher frequency of HRAS mutations in HNC as compared to its frequency in other cancer types in which KRAS mutations are most prevalent, followed by NRAS mutations (8). The results of our prevalence analysis diverge slightly from the results of The Cancer Genome Atlas project (21, 22), which has carried out one of the most significant studies on an HNC patient population. These slight differences may be due to the more heterogeneous population of patients from diverse geographical regions, disease stages, and detection methods included in our analysis.
Our analyses revealed differences in the prevalences of RAS mutations according to the anatomical site, which may account for some of the heterogeneity between cohorts in the overall prevalence analysis. HRAS mutations were more prevalent in oral cavity and salivary gland tumors. In contrast, KRAS mutations were more frequent in sinonasal tumors, and NRAS mutations were found chiefly in tumors of the nasopharynx. These findings emphasize the importance of taking the anatomical site of the tumor into consideration so as to achieve a more accurate assessment of RAS mutation frequencies. The variation in frequencies between tissue types may be due to differences in baseline expression and activity of RAS in different anatomical sites, which may, in turn, affect cellular reprogramming and tumor formation (8). Another explanation might be differences in the quality and quantity of exposure to risk factors (23).
Our data reveal a significantly higher prevalence of HRAS mutations in South Asia, corroborating previous studies on oral cancer in India (24–27). Those studies identified region-specific risk factors, such as smoking bidis (cigarettes wrapped in a tendu or temburni leaf) (28, 29), chewing betel nuts (30), and oral hygiene (31), that contribute, separately or synergistically, to the development of tumors, specifically within the oral cavity (32–35). Indeed, in our database, 86% of the patients from South Asia suffered from oral cancer, as opposed to primary tumors in other sites. As noted above, the HRAS mutation frequency is higher in oral cancer worldwide. Thus, further studies are needed to determine whether exposure to such risk factors directly causes mutations in HRAS or whether these factors increase the odds of tumors developing in the oral cavity, in which the prevalence of HRAS mutations is high.
We found that the most frequent amino acid substitution in codon 12 of KRAS was G12D (51%), followed by G12V (16.3%) and G12C (12.9%). This finding provides an indication of the size of the population that could benefit from treatment with mutant-specific inhibitors, i.e., G12C and G12D KRAS inhibitors, that are in various stages of development.
A considerable percentage of HRAS mutations were present in codon 61, particularly in salivary gland tumors. To date, only limited studies have been performed to elucidate the etiology of these specific alterations, but recent analyses of patients with salivary gland cancer have indicated the diagnostic significance of these mutations (36). These findings may help evaluate the size of the subpopulations that could benefit from a particular treatment.
Data regarding the association between RAS gene mutations and prognosis in HNC are contradictory. Some studies link RAS mutations with stage and disease recurrence (37–40), while others predict better prognosis and overall survival (41–43). Our meta-analysis found that mutations in HRAS are significantly associated with high stage/grade scores, emphasizing the importance of considering RAS mutational status when assessing patient prognosis. KRAS and NRAS mutations also exhibited a trend towards being associated with high stage/grade scores. The lower number of cases with KRAS or particularly of NRAS mutations that were available for OR analysis may account for the observed lack of statistical significance.
An association between RAS mutations and HPV status in HNC has been suggested (38, 44). In keeping with these studies, our data reveal a significant association between HPV-positive status and KRAS mutations. Studies on HPV-related cancers, mainly cervical cancer, demonstrate a similar association (45–47). Notably, KRAS mutations, HRAS mutations, and HPV infection were mutually exclusive in benign neoplasms of the head and neck (48). These findings suggest that RAS mutations in the context of HPV infection contribute to carcinogenesis.
Several inhibitors of the RAS-MAPK pathway are currently under evaluation as therapeutics for various cancers [(reviewed in (9)]. Therefore, knowledge regarding the prevalence of RAS family mutations and associated characteristics in HNC may enable researchers to better assess the need for and the potential of trials with molecularly relevant targeted therapeutics.
4.1 Limitations
Certain methodological limitations of this review should be considered. First, even after selecting only those studies with a low ‘risk of bias score,’ the heterogeneity between studies remained high. We believe that this is due to the heterogeneous nature of HNC, which includes a wide range of anatomical sites and etiologies. We attempted to address this issue by conducting additional sub-group analyses, which consistently revealed significant differences between groups. Due to differences in the categorization of sub-anatomic sites between reports and the lack of a minimal number of cases needed for sufficient statistical power, we could not perform analysis on sub-anatomical sites within the seven major anatomical sites. A second limitation of this analysis derives from the differences in the sequencing methods used in the various studies, which may have influenced overall pooled results by interfering with the accuracy and precision of pooled estimates. Third, we could not provide an analysis on RAS mutations association with exposure to risk factors due to insufficient patient-specific data and a lack of standardized categories of risk factors. Such data could potentially have strengthened the observed associations in this study and provided additional insights.
5 Conclusions
This study highlights RAS as a potential therapeutic target in certain subsets of HNC patients. The findings underscore the differences in the prevalence rates of HRAS, KRAS, and NRAS according to tumor anatomical site and geographical region. The analysis also demonstrates that RAS mutations are associated with tumor stage and HPV status.
Data Availability Statement
The original contributions presented in the study are included in the article/Supplementary Material. Further inquiries can be directed to the corresponding authors.
Author Contributions
Conceptualization: ON, SJ, ME. data curation: ON, SJ. Formal analysis: ON, SJ, OR, IM. Methodology: ON, SJ, OR, IM. Resources: ME. Visualization: ON, SJ, OR, ME. Writing-original draft: ON, OR. Writing-review and editing: ON, SJ, OR, ME, IM. All listed authors have made substantial, contributions and have approved the submitted version. All authors have read and agreed to the published version of the manuscript.
Funding
This work was funded by the Israel Science Foundation (ISF, 302/21 and 700/16) (to ME), the Israel Cancer Research Foundation (ICRF, 17-1693-RCDA) (to ME), United States-Israel Binational Science Foundation (BSF, 2017323) (to ME).
Conflict of Interest
The authors declare that the research was conducted in the absence of any commercial or financial relationships that could be construed as a potential conflict of interest.
Publisher’s Note
All claims expressed in this article are solely those of the authors and do not necessarily represent those of their affiliated organizations, or those of the publisher, the editors and the reviewers. Any product that may be evaluated in this article, or claim that may be made by its manufacturer, is not guaranteed or endorsed by the publisher.
Acknowledgments
Fellowships: Eileen & Louis Dubrovsky Doctoral Cancer Fellowship Endowment Fund, BGU fellow to ON, and a PBC post-doctoral fellowship from Israeli Council for Higher Education to SJ.
Supplementary Material
The Supplementary Material for this article can be found online at: https://www.frontiersin.org/articles/10.3389/fonc.2022.838911/full#supplementary-material
References
2. Boras VV, Fučić A, Baranović S, Blivajs I, Milenović M, Bišof V, et al. Environmental and Behavioural Head and Neck Cancer Risk Factors. Cent Eur J Public Health (2019) 27:106–9. doi: 10.21101/cejph.a5565
3. Leemans CR, Snijders PJFF, Brakenhoff RH. The Molecular Landscape of Head and Neck Cancer (2018). (Accessed June 6, 2019).
4. Stransky N, Egloff AM, Tward AD, Kostic AD, Cibulskis K, Sivachenko A, et al. The Mutational Landscape of Head and Neck Squamous Cell Carcinoma. Science (80- ) (2011) 333:1157–60. doi: 10.1126/science.1208130
5. Lawrence MS, Sougnez C, Lichtenstein L, Cibulskis K, Lander E, Gabriel SB, et al. Comprehensive Genomic Characterization of Head and Neck Squamous Cell Carcinomas. Nature (2015) 517:576–82. doi: 10.1038/NATURE14129
6. Simanshu DK, Nissley DV, McCormick F. RAS Proteins and Their Regulators in Human Disease. Cell (2017) 170:17. doi: 10.1016/J.CELL.2017.06.009
7. Mo SP, Coulson JM, Prior IA. RAS Variant Signalling. Biochem Soc Trans (2018) 46:1325–32. doi: 10.1042/BST20180173
8. Prior IA, Hood FE, Hartley JL. The Frequency of Ras Mutations in Cancer. Cancer Res (2020) 80:2669–974. doi: 10.1158/0008-5472.CAN-19-3682
9. Chen K, Zhang Y, Qian L, Wang P. Emerging Strategies to Target RAS Signaling in Human Cancer Therapy. J Hematol Oncol (2021) 14:1–23. doi: 10.1186/s13045-021-01127-w
10. Skoulidis F, Li BT, Dy GK, Price TJ, Falchook GS, Wolf J, et al. Sotorasib for Lung Cancers With KRAS P.G12C Mutation. N Engl J Med (2021) 384:2371–81. doi: 10.1056/nejmoa2103695
11. Hong DS, Fakih MG, Strickler JH, Desai J, Durm GA, Shapiro GI, et al. KRAS G12C Inhibition With Sotorasib in Advanced Solid Tumors. N Engl J Med (2020) 383:1207–17. doi: 10.1056/nejmoa1917239
12. Hanna GJ, Guenette JP, Chau NG, Sayehli CM, Wilhelm C, Metcalf R, et al. Tipifarnib in Recurrent, Metastatic HRAS-Mutant Salivary Gland Cancer. Cancer (2020) 126:3972–81. doi: 10.1002/cncr.33036
13. Ho AL, Brana I, Haddad R, Bauman J, Bible K, Oosting S, et al. Tipifarnib in Head and Neck Squamous Cell Carcinoma With HRAS Mutations. J Clin Oncol (2021) 39:1856–64. doi: 10.1200/JCO.20.02903
14. Moher D, Liberati A, Tetzlaff J, Altman DG, Group TP. Preferred Reporting Items for Systematic Reviews and Meta-Analyses: The PRISMA Statement. PloS Med (2009) 6:e1000097. doi: 10.1371/JOURNAL.PMED.1000097
15. Aromataris E, Munn Z eds. JBI Manual for Evidence Synthesis. JBI (2020). Available at: https://synthesismanual.jbi.global. doi: 10.46658/jbimes-20-01
16. Egger M, Smith GD, Schneider M, Minder C. Bias in Meta-Analysis Detected by a Simple, Graphical Test. Br Med J (1997) 315:629–34. doi: 10.1136/bmj.315.7109.629
17. Viechtbauer W. Conducting Meta-Analyses in R With the Metafor. J Stat Softw (2010) 36:1–48. doi: 10.18637/jss.v036.i03
18. Balduzzi S, Rücker G, Schwarzer G. How to Perform a Meta-Analysis With R: A Practical Tutorial. Evid Based Ment Health (2019) 22:153–60. doi: 10.1136/ebmental-2019-300117
19. Wang N. How to Conduct a Meta-Analysis of Proportions in R : A Comprehensive Tutorial Conducting Meta-Analyses of Proportions in R. (2018). doi: 10.13140/RG.2.2.27199.00161.
20. Hashim D, Genden E, Posner M, Hashibe M, Boffetta P. Head and Neck Cancer Prevention: From Primary Prevention to Impact of Clinicians on Reducing Burden. Ann Oncol (2019) 30:744. doi: 10.1093/ANNONC/MDZ084
21. Cerami E, Gao J, Dogrusoz U, Gross BE, Sumer SO, Aksoy BA, et al. The Cbio Cancer Genomics Portal: An Open Platform for Exploring Multidimensional Cancer Genomics Data. Cancer Discov (2012) 2:401–4. doi: 10.1158/2159-8290.CD-12-0095
22. Sayáns MP, Petronacci CMC, Pouso AIL, Iruegas EP, Carrión AB, Peñaranda JMS, et al. Comprehensive Genomic Review of TCGA Head and Neck Squamous Cell Carcinomas (HNSCC). J Clin Med (2019) 8:1896. doi: 10.3390/jcm8111896
23. Simard EP, Torre LA, Jemal A. International Trends in Head and Neck Cancer Incidence Rates: Differences by Country, Sex and Anatomic Site. Oral Oncol (2014) 50:387–403. doi: 10.1016/J.ORALONCOLOGY.2014.01.016
24. Das N, Majumder J, Dasgupta UB. Ras Gene Mutations in Oral Cancer in Eastern India. Oral Oncol (2000) 36:76–80. doi: 10.1016/S1368-8375(99)00058-5
25. Saranath D, Chang SE, Bhoite LT, Panchal RG, Kerr IB, Mehta AR, et al. High Frequency Mutation in Codons 12 and 61 of H-Ras Oncogene in Chewing Tobacco-Related Human Oral Carcinoma in India. Br J Cancer (1991) 63:573–8. doi: 10.1038/bjc.1991.133
26. Paterson IC, Eveson JW, Prime SS. Molecular Changes in Oral Cancer may Reflect Aetiology and Ethnic Origin. Eur J Cancer Part B Oral Oncol (1996) 32:150–3. doi: 10.1016/0964-1955(95)00065-8
27. Chang SE, Bhatia P, Johnson NW, Morgan PR, McCormick F, Young B, et al. Ras Mutations in United Kingdom Examples of Oral Malignancies Are Infrequent. Int J Cancer (1991) 48:409–12. doi: 10.1002/ijc.2910480318
28. Rahman M, Sakamoto J, Fukui T. Bidi Smoking and Oral Cancer: A Meta-Analysis. Int J Cancer (2003) 106:600–4. doi: 10.1002/IJC.11265
29. Jayalekshmi PA, Gangadharan P, Akiba S, Koriyama C, Nair RRK. Oral Cavity Cancer Risk in Relation to Tobacco Chewing and Bidi Smoking Among Men in Karunagappally, Kerala, India: Karunagappally Cohort Study. Cancer Sci (2011) 102:460–7. doi: 10.1111/j.1349-7006.2010.01785.x
30. Garg A, Chaturvedi P, Gupta PC. A Review of the Systemic Adverse Effects of Areca Nut or Betel Nut. Indian J Med Paediatr Oncol (2014) 35:3–9. doi: 10.4103/0971-5851.133702
31. Gupta B, Bray F, Kumar N, Johnson NW. Associations Between Oral Hygiene Habits, Diet, Tobacco and Alcohol and Risk of Oral Cancer: A Case–Control Study From India. Cancer Epidemiol (2017) 51:7–14. doi: 10.1016/J.CANEP.2017.09.003
32. Hsieh L, Wang P, Chen I, Liao C, Wang H, Chen M, et al. Characteristics of Mutations in the P53 Gene in Oral Squamous Cell Carcinoma Associated With Betel Quid Chewing and Cigarette Smoking in Taiwanese G : C to A : T Transitions Were the Predominant Mutations Still Showed an Independent Effect on G : C to A . Carcinogenesis (2001) 22:1497–503. doi: 10.1093/carcin/22.9.1497
33. Chang Y, Tai K, Chou M, Tseng T. Synergistic Effects of Peroxynitrite on Arecoline-Induced Cytotoxicity in Human Buccal Mucosal Fibroblasts. Toxicol Lett (2000) 118:61–8. doi: 10.1016/S0378-4274(00)00262-9
34. Cancer I, Consortium G. Mutational Landscape of Gingivo-Buccal Oral Squamous Cell Carcinoma Reveals New Recurrently-Mutated Genes and Molecular Subgroups. Nat Commun (2013) 4:2873. doi: 10.1038/ncomms3873
35. Wu IC, Lu CY, Kuo FC, Tsai SM, Lee KW, Kuo WR, et al. Interaction Between Cigarette, Alcohol and Betel Nut Use on Esophageal Cancer Risk in Taiwan. Eur J Clin Invest (2006) 36:236–41. doi: 10.1111/J.1365-2362.2006.01621.X
36. Urano M, Nakaguro M, Yamamoto Y, Hirai H, Tanigawa M, Saigusa N, et al. Diagnostic Significance of HRAS Mutations in Epithelial-Myoepithelial Carcinomas Exhibiting a Broad Histopathologic Spectrum. Am J Surg Pathol (2019) 43:984–94. doi: 10.1097/PAS.0000000000001258
37. Zhang Z-CC, Fu S, Wang F, Wang H-YY, Zeng Y-XX, Shao J-YY. Oncogene Mutational Profile in Nasopharyngeal Carcinoma. Onco Targets Ther (2014) 7:457–67. doi: 10.2147/OTT.S58791
38. Seiwert TY, Zuo Z, Keck MK, Khattri A, Pedamallu CS, Stricker T, et al. Integrative and Comparative Genomic Analysis of HPV-Positive and HPV-Negative Head and Neck Squamous Cell Carcinomas. Clin Cancer Res (2015) 21:632. doi: 10.1158/1078-0432.CCR-13-3310
39. Wang HM, Liao CT, Yen TC, Chen SJ, Lee LY, Hsieh CH, et al. Clues Toward Precision Medicine in Oral Squamous Cell Carcinoma: Utility of Next-Generation Sequencing for the Prognostic Stratification of High-Risk Patients Harboring Neck Lymph Node Extracapsular Extension. Oncotarget (2016) 7:63082–92. doi: 10.18632/oncotarget.11762
40. Reder H, Wagner S, Wuerdemann N, Langer C, Sandmann S, Braeuninger A, et al. Mutation Patterns in Recurrent and/or Metastatic Oropharyngeal Squamous Cell Carcinomas in Relation to Human Papillomavirus Status. Cancer Med (2021) 10:1347–56. doi: 10.1002/cam4.3741
41. Sathyan KM, Nalinakumari KR, Kannan S. H-Ras Mutation Modulates the Expression of Major Cell Cycle Regulatory Proteins and Disease Prognosis in Oral Carcinoma. Mod Pathol (2007) 20:1141–8. doi: 10.1038/modpathol.3800948
42. Szablewski V, Solassol J, Poizat F, Larrieux M, Crampette L, Mange A, et al. EGFR Expression and KRAS and BRAF Mutational Status in Intestinal-Type Sinonasal Adenocarcinoma. Int J Mol Sci (2013) 14:5170–81. doi: 10.3390/IJMS14035170
43. Ruíz-Godoy RLM, García-Cuellar CM, Herrera González NE, Suchil BL, Pérez-Cárdenas E, Sánchez-Pérez Y, et al. Mutational Analysis of K-Ras and Ras Protein Expression in Larynx Squamous Cell Carcinoma. J Exp Clin Cancer Res (2006) 25:73–8.
44. Tinhofer I, Budach V, Saki M, Konschak R, Niehr F, Jöhrens K, et al. Targeted Next-Generation Sequencing of Locally Advanced Squamous Cell Carcinomas of the Head and Neck Reveals Druggable Targets for Improving Adjuvant Chemoradiation. Eur J Cancer (2016) 57:78–86. doi: 10.1016/J.EJCA.2016.01.003
45. Hoover AC, Strand GL, Nowicki PN, Anderson ME, Vermeer PD, Klingelhutz AJ, et al. Impaired PTPN13 Phosphatase Activity in Spontaneous or HPV-Induced Squamous Cell Carcinomas Potentiates Oncogene Signaling Through the MAP Kinase Pathway. Oncogene (2009) 28:3960–70. doi: 10.1038/onc.2009.251
46. Mammas IN, Zafiropoulos A, Sifakis S, Sourvinos G, Spandidos DA. Human Papillomavirus (HPV) Typing in Relation to Ras Oncogene mRNA Expression in HPV-Associated Human Squamous Cervical Neoplasia. Int J Biol Markers (2005) 20:257–63. doi: 10.1177/172460080502000409
47. Narisawa-Saito M, Inagawa Y, Yoshimatsu Y, Haga K, Tanaka K, Egawa N, et al. A Critical Role of MYC for Transformation of Human Cells by HPV16 E6E7 and Oncogenic HRAS. Carcinogenesis (2012) 33:910–7. doi: 10.1093/carcin/bgs104
Keywords: HRAS, KRAS, NRAS, head and neck cancer, meta-analysis, clinical characteristics
Citation: Novoplansky O, Jagadeeshan S, Regev O, Menashe I and Elkabets M (2022) Worldwide Prevalence and Clinical Characteristics of RAS Mutations in Head and Neck Cancer: A Systematic Review and Meta-Analysis. Front. Oncol. 12:838911. doi: 10.3389/fonc.2022.838911
Received: 18 December 2021; Accepted: 11 April 2022;
Published: 06 May 2022.
Edited by:
Hua Tan, National Human Genome Research Institute (NIH), United StatesReviewed by:
Moran Amit, University of Texas MD Anderson Cancer Center, United StatesChen Li, Free University of Berlin, Germany
Copyright © 2022 Novoplansky, Jagadeeshan, Regev, Menashe and Elkabets. This is an open-access article distributed under the terms of the Creative Commons Attribution License (CC BY). The use, distribution or reproduction in other forums is permitted, provided the original author(s) and the copyright owner(s) are credited and that the original publication in this journal is cited, in accordance with accepted academic practice. No use, distribution or reproduction is permitted which does not comply with these terms.
*Correspondence: Ofra Novoplansky, novoplan@post.bgu.ac.il; Moshe Elkabets, moshee@bgu.ac.il
†These authors have contributed equally to this work