- 1Department of Life Sciences, University of Siena, Siena, Italy
- 2Institute of Endocrinology and Experimental Oncology “G. Salvatore”, National Research Council, Naples, Italy
- 3Hematology and Clinical Immunology Unit, Department of Medicine, University of Padua, Padua, Italy
- 4Department of Biomedical Sciences, National Research Council, Rome, Italy
An imbalance in the expression of pro- and anti-apoptotic members of the Bcl-2 family of apoptosis-regulating proteins is one of the main biological features of CLL, highlighting these proteins as therapeutic targets for treatment of this malignancy. Indeed, the Bcl-2 inhibitor Venetoclax is currently used for both first-line treatment and treatment of relapsed or refractory CLL. An alternative avenue is the transcriptional modulation of Bcl-2 family members to tilt their balance towards apoptosis. Glycerophosphoinositol (GroPIns) is a biomolecule generated from membrane phosphoinositides by the enzymes phospholipase A2 and lysolipase that pleiotropically affects key cellular functions. Mass-spectrometry analysis of GroPIns interactors recently highlighted the ability of GroPIns to bind to the non-receptor tyrosine phosphatase SHP-1, a known promoter of Bax expression, suggesting that GroPIns might correct the Bax expression defect in CLL cells, thereby promoting their apoptotic demise. To test this hypothesis, we cultured CLL cells in the presence of GroPIns, alone or in combination with drugs commonly used for treatment of CLL. We found that GroPIns alone increases Bax expression and apoptosis in CLL cells and enhances the pro-apoptotic activity of drugs used for CLL treatment in a SHP-1 dependent manner. Interestingly, among GroPIns interactors we found Bax itself. Short-term treatments of CLL cells with GroPIns induce Bax activation and translocation to the mitochondria. Moreover, GroPIns enhances the pro-apoptotic activity of Venetoclax and Fludarabine in CLL cells. These data provide evidence that GroPIns exploits two different pathways converging on Bax to promote apoptosis of leukemic cells and pave the way to new studies aimed at testing GroPIns in combination therapies for the treatment of CLL.
Introduction
Chronic lymphocytic leukemia (CLL), the most common lymphoid malignancy in Western countries, is characterized by the accumulation of monoclonal CD5+ B cells in peripheral blood, bone marrow and secondary lymphoid organs (1). Although the clinical course is highly variable, the most conserved feature of CLL is the extended survival of malignant B cells, which has been associated to defects in the apoptotic machinery (1, 2).
Alterations in the expression of pro-survival and pro-apoptotic members of the B-cell leukemia/lymphoma-2 (Bcl-2) family of apoptosis-regulating proteins is a hallmark of CLL and a key intrinsic factor underlying the longevity of CLL cells (1, 2). Increased expression of pro-survival members such as Bcl-2 and Mcl-1 (3, 4), concomitant with impaired expression of pro-apoptotic members such as Bax and Bak (5), tilts the finely regulated balance towards survival, leading to the accumulation of long-lived neoplastic cells that further acquire stroma-derived survival signals during their transit through secondary lymphoid organs (2, 6). It is therefore not surprising that restoring the Bcl-2 family balance has been pinpointed as strategy for overcoming the apoptosis defects of CLL cells, as witnessed by the recent approval of the Bcl-2 selective inhibitor Venetoclax for CLL treatment (7, 8). This effect is also elicited by chemotherapeutic drugs such as the fluorinated nucleotide analog Fludarabine, which affects the Bcl-2 family balance by indirectly promoting both expression and activation of Bax (9, 10). As opposed to Bcl-2, no drugs that specifically target Bax to enhance its expression or activation have been as yet developed (11).
Glycerophosphoinositols (GPIs) are water-soluble bioactive phospholipid derivatives of increasing interest as intracellular and paracrine mediators of eukaryotic cell functions. Generated from membrane phosphoinositides by the phospholipase cPLA2α, GPIs have diverse effects in a variety of cell types (12, 13). The most representative compound of the family is glycerophosphoinositol (GroPIns), a ubiquitous component of mammalian cells that participates in cell proliferation and survival in response to extracellular stimuli (14). When added exogenously, GroPIns elicits pharmacological effects relevant to both inflammatory responses and tumor spreading. In human blood monocytes GroPIns counteracts the LPS-induced proinflammatory and prothrombotic responses, inhibiting TLR4 signaling and leading to a decrease in the NF-κB-dependent transcription of inflammatory genes (15). GroPIns has also been recently found to reduce the invasive potential of melanoma cells through its ability to interact with and regulate the non-receptor tyrosine phosphatase Src homology region 2 domain-containing phosphatase-1 (SHP-1) (16, 17). GroPIns interaction with SHP-1 facilitates SHP-1 localization to invadopodia where it dephosphorylates cortactin, with subsequent impaired invadopodia function and hampered metastasis of melanoma cells both in vitro and in vivo (17).
Mainly expressed in hematopoietic and epithelial cells, the tyrosine phosphatase SHP-1 is a negative regulator of signaling pathways leading to cell proliferation, differentiation, survival and adhesion (18). Its dephosphorylating activity makes it a key regulator of cancer progression. Both expression and activity of SHP-1 are impaired in a number of cancer cell lines and tissues (19–21). Several pharmacological drugs used for cancer treatment enhance SHP-1 expression, which in turn downregulates aberrantly activated tyrosine kinase-dependent signaling pathways (22). The involvement of SHP-1 in cancer progression is also supported by evidence that SHP-1 promotes cancer cell apoptosis (23, 24) by enhancing the expression of Bax (23, 25). Although its expression levels are unaffected in CLL cells, SHP-1 activity is inhibited as a result of phosphorylation of the inhibitory residue Ser591 (26), making it an interesting molecular target for the treatment of this disease.
Here we asked whether GroPIns affects CLL cell apoptosis. We show that GroPIns exploits its SHP-1 modulating activity to promote CLL cell apoptosis by enhancing Bax expression. Moreover, we show that GroPIns directly interacts with Bax, rapidly promoting its activation and recruitment to the mitochondria. Hence GroPIns promotes CLL cell apoptosis by regulating the expression and activation of Bax through different pathways, highlighting the potential exploitability of this glycerophospholipid to overcome the apoptosis defects of CLL cells.
Materials and Methods
Cells, Antibodies and Reagents
Peripheral blood samples were collected from 40 treatment-naive CLL patients. Diagnosis of CLL was made according to international workshop on CLL (iwCLL) 2008 criteria (27). The immunophenotypic analysis of lymphocytes obtained from peripheral blood of CLL patients was performed by flow cytometry. All patients expressed the typical phenotypic profile according to standard criteria for CLL diagnosis and were positive for CD19, CD5, CD23 and CD200. Flow cytometric plots of a representative CLL patient are shown in Supplementary Figure 1. Mutational IGHV status was assessed as reported (28). The main clinical features of CLL patients used in this study are listed in Supplementary Table 1. B cells from 24 buffy coats were used as healthy population controls. B cells were purified by negative selection using RosetteSep B-cell enrichment Cocktail (StemCell Technologies, Vancouver, Canada) followed by density gradient centrifugation on Lympholite (Cedarlane Laboratories, The Netherlands), as reported (29). Human HS-5 (30) stromal cells were used for co-culture experiments, as reported (31). Cells were maintained in RPMI (Roswell Park Memorial Institute)-1640 (Merck, #R8758) containing 7.5% Bovine Calf Serum (BCS) (HyClone, #SH30072.03). GroPIns was kindly provided by Euticals S.p.a (Lodi, Italy). GroPIns-Bio was obtained from Echelon Biosciences (Salt Lake City, UT, USA). NSC-87887 (Merck, #565851and Fludarabine (Merck, #F9813) were from Merck. Venetoclax was from Selleck Chemicals (#S8048). His-tagged Bax-α lacking 21 amino acids at the C-terminus (His-BaxΔTM) cloned in the pTrcHis vector (Invitrogen Srl) was a kind gift of Ingram Iaccarino. This construct was expressed in E. coli BL21(DE3)/pLysS cells and purified as described (32).
Cell Treatments, Antibodies and Immunoblots
Treatments with 100 μM GroPIns, 35 μM Fludarabine, 3.5 nM Venetoclax or combination treatments were carried out at 37°C in RPMI 7.5% BCS for the indicated times. Control samples were treated with DMSO (Merck Millipore, #102952). Dose-response and time course experiments of CLL B cells treated with GroPIns are shown in Supplementary Figure 2. When required, cells were pretreated at 37°C for 20 min with 50 μM NSC-87887. Cells (5×106 cells/sample) were lysed in 1% (v/v) Triton X-100 in 20 mM Tris-HCl pH 8, 150 mM NaCl, in the presence of a cocktail of protease inhibitors (Calbiochem, #539134) and 0.2 mg/ml Na orthovanadate (Merck, #S6508), resolved by SDS-PAGE and transferred to nitrocellulose (GE Healthcare, #9004-70-0). Immunoblots were carried out using mouse anti-Bax (BD Biosciences, #610982), anti-penta-His (Life Technologies, #P21315) and anti-actin (Millipore, #MAB1501) primary antibodies. Secondary peroxidase-labeled anti-mouse antibodies were from Jackson Immuno-Research (#115-035-146). Labeled antibodies were detected using ECL kit (SuperSignal® West Pico Chemiluminescent Substrate, Thermo Scientific) and scanned immunoblots were quantified using the ImageJ software.
Intracellular Staining, Apoptosis, TMRM Assays and Flow Cytometry
Cells (2×105 cells/sample) were treated for 20 min in complete medium at 37°C as above, washed with PBS and fixed in 100 μl of fixation buffer (eBiosciences, #420801) for 15 minutes at RT. Cells were then washed with PBS added with 1% BSA (AppliChem PanReac, #A6588) and incubated with 10 μl permeabilization buffer (eBiosciences, #421008) containing either mouse anti-Bax (B-9) (Santa Cruz Biotechnology Inc., #sc-7480) or rabbit anti-phospho-SHP-1 Tyr564 (Cell Signaling, #D11G5) antibodies at RT for 1 h, washed twice in PBS 1% BSA and then incubated with 10 μl permeabilization buffer containing Alexa Fluor anti-mouse-488 (Thermo Fisher Scientific, #A11001) or anti-rabbit-488 (Thermo Fisher Scientific, #A11008) secondary antibodies for 45 min. After washing with PBS 1% BSA, cell pellets were resuspended in 200 μl PBS 1% BSA and subjected to flow cytometric analysis. Early apoptotic cells were quantified by flow cytometric analysis of 1×106 cells stained with FITC-labeled Annexin V (e-Bioscience, #88-8005-74) and Propidium iodide (PI, 20 µg/mL, Biotium, #40017). Mitochondrial membrane potential was measured using the fluorescent probe tetramethylrhodamine methyl ester (TMRM, Molecular Probes Europe BV). Cells (106 cells/sample) were suspended in 200 μl RPMI-1640 w/o phenol Red (Invitrogen srl) added with 25 mM Hepes pH 7.4 and 200 nM TMRM and incubated for 20 min at 37°C. Cells were then added with 500 ng/ml of the calcium ionophore A23187 (Sigma-Aldrich #C7522), incubated for 10 min at 37°C and subjected to flow cytometric analysis. Flow cytometry was carried out using a Guava Millipore cytometer as described (29). Data were analyzed using Flowjo (Tree Star, Inc.).
Co-Culture Experiments
Stromal cells were seeded on 96-well plates (1.5 × 105 cells/well) in complete culture medium and cultured to confluence. 2 × 105 cells/well CLL cells were added. Cells were co-cultured for 24 h at 37°C in the presence of either Venetoclax or DMSO. Wells were gently washed with RPMI to recover CLL cells, avoiding HS-5 cell detachment from the wells. Samples were stained with either CD19-FITC antibody (Biolegend, #392503) to identify the CLL cell population or with FITC-labeled Annexin V/Propidium iodide to evaluate early apoptotic cells, and analyzed by flow cytometry.
GroPIns-Bio Pull-Down Assay
GroPIns-Bio pull-down assays were previously described (16). Briefly, Raw 264.7 cells were centrifuged, washed with PBS and re-suspended in lysis buffer supplemented with a protease inhibitor cocktail (Complete Mini EDTA-free, Roche). The cell lysate was kept on a rotating wheel for 30 min at 4°C, centrifuged and the supernatant recovered, brought to a 0.2% (w/v) final concentration of Triton X-100, and dialyzed at 4°C. The cell extract was then precleared on 1 mg of uncoupled streptavidin-conjugated paramagnetic beads (Invitrogen Srl) on a rotating wheel, recovered and incubated with 1 mg of streptavidin-conjugated beads previously incubated with 2.5 nmoles of GroPIns-Bio or biotin in binding buffer (50 mM Tris-HCl, pH 7.6, 50 mM KCl, 10 mM EDTA) supplemented with the protease inhibitor cocktail. Following incubation, the unbound materials were separated and the beads were washed with binding buffer. GroPIns-bound proteins were specifically eluted with 5 mM GroPIns. The elution was performed for 30 min at 4°C on a rotating wheel, eluted proteins were recovered, resuspended in SDS sample buffer and analyzed by SDS-PAGE. Protein bands were analyzed by liquid chromatography coupled to tandem mass spectrometry (LC/MS-MS). For GroPIns-Bio pull-down assays with purified Bax, 100 ng of purified His-Bax were incubated for 2 h at 4°C with 0.5 mg of streptavidin-conjugated paramagnetic beads in the presence of 2.5 nmoles of biotin (Sigma-Aldrich, #B4501) or GroPIns-Bio in binding buffer plus protease inhibitors (Complete Mini EDTA-free, Roche). Following incubation, the unbound material was removed, and beads were washed with binding buffer. The beads with bound protein were boiled in 100 μl of SDS-sample buffer.
Immunofluorescence and Confocal Microscopy
Cells (1×105/sample) were cultured at 37°C in culture medium w/o BCS in the presence of 250 nM Mitotracker Orange (Invitrogen, Molecular Probes, #M7511) in the dark, then washed with PBS and treated for 20 min in culture medium w/o BCS at 37°C in the presence of 100 μM GroPIns, 35 μM Fludarabine or the combination of both. Diagnostic microscope slides were coated with polylysine (Sigma-Aldrich, #1274) and treated cells were allowed to adhere for 10 min. Slides were immediately fixed in methanol (Carlo Erba, #412383) at -20°C for 10 min as described (33). Following fixation, samples were washed 5 min in PBS and incubated with anti-Bax (B-9) primary antibodies o/n at 4°C or 1 h at RT. After washing in PBS, samples were incubated for 1 h at RT with Alexa Fluor 488-labeled secondary antibodies. Confocal microscopy was carried out on a Zeiss LSM700 using a 63× objective, as reported (33). Images were processed with Zen 2009 image software (Carl Zeiss, Jena, Germany) and analyses were performed using ImageJ software (downloaded from http://www.embl-heidelberg.de/eamnet/).
RNA Isolation, Reverse Transcription and Real-Time Quantitative PCR
RNA was extracted and retrotranscribed as described (34). Real-time PCR was performed in triplicate on 96-well optical PCR plates (Sarstedt AG, Nümbrecht, Germany) using SSo FastTM EvaGreenR SuperMix and a CFX96 Real-Time system (Bio-Rad Laboratories, Waltham, MA). Results were processed and analyzed as described (34). Values are expressed as ΔΔCT relative to the housekeeping gene HPRT1. Primers used for real-time quantitative PCR amplification are listed in Supplementary Table 2.
Statistical Analyses
One-way ANOVA with post-hoc Tukey was used for experiments where multiple groups were compared. Mann-Whitney rank-sum tests were performed to determine the significance of the differences between two groups. Statistical analyses were performed using GraphPad Software (La Jolla, CA). P values <0.05 were considered significant.
Combination Index Calculation
The Combination index (Bliss index) was calculated according to the literature (35, 36). Briefly, CLL cells from 2 patients were mixed and plated into 96 well plates in 100 μl culture medium. GroPIns, Fludarabine and/or Venetoclax were added at different concentrations for 24 h, alone or in combination. Cell apoptosis was analyzed as above and the Combination index was calculated as in (35).
Study Approval
Written informed consent was received from CLL patients and healthy donors prior to inclusion in the study according to the Declaration of Helsinki. Experiments were approved by the local Ethics Committee.
Results
GroPIns Has a Pro-Apoptotic Activity on CLL Cells Which Depends on SHP-1
The activity of the tyrosine phosphatase SHP-1, known to promote apoptosis (18, 25), has been shown to be impaired in CLL cells (26). Since GroPIns is a well-known regulator of SHP-1 in melanoma cells (17), we asked whether it promotes apoptosis of CLL cells through a SHP-1-dependent mechanism. B cells purified from peripheral blood of CLL patients were cultured for 24 h in the presence of 100 μM GroPIns and the percentage of early apoptotic Annexin V+/PI- cells was quantified by flow cytometry. B cells from healthy donors were used as control. GroPIns enhanced apoptosis of CLL cells (Figures 1A, B; Supplementary Figure 2). Apoptosis of healthy B cells was also enhanced by GroPIns, although at significantly lower levels compared to CLL cells (Figure 1A). The pro-apoptotic activity of GroPIns was partly reversed by the SHP-1-specific inhibitor NSC-87887 (Figure 1B), demonstrating that the pro-apoptotic activity of GroPIns relies on the tyrosine phosphatase activity of SHP-1.
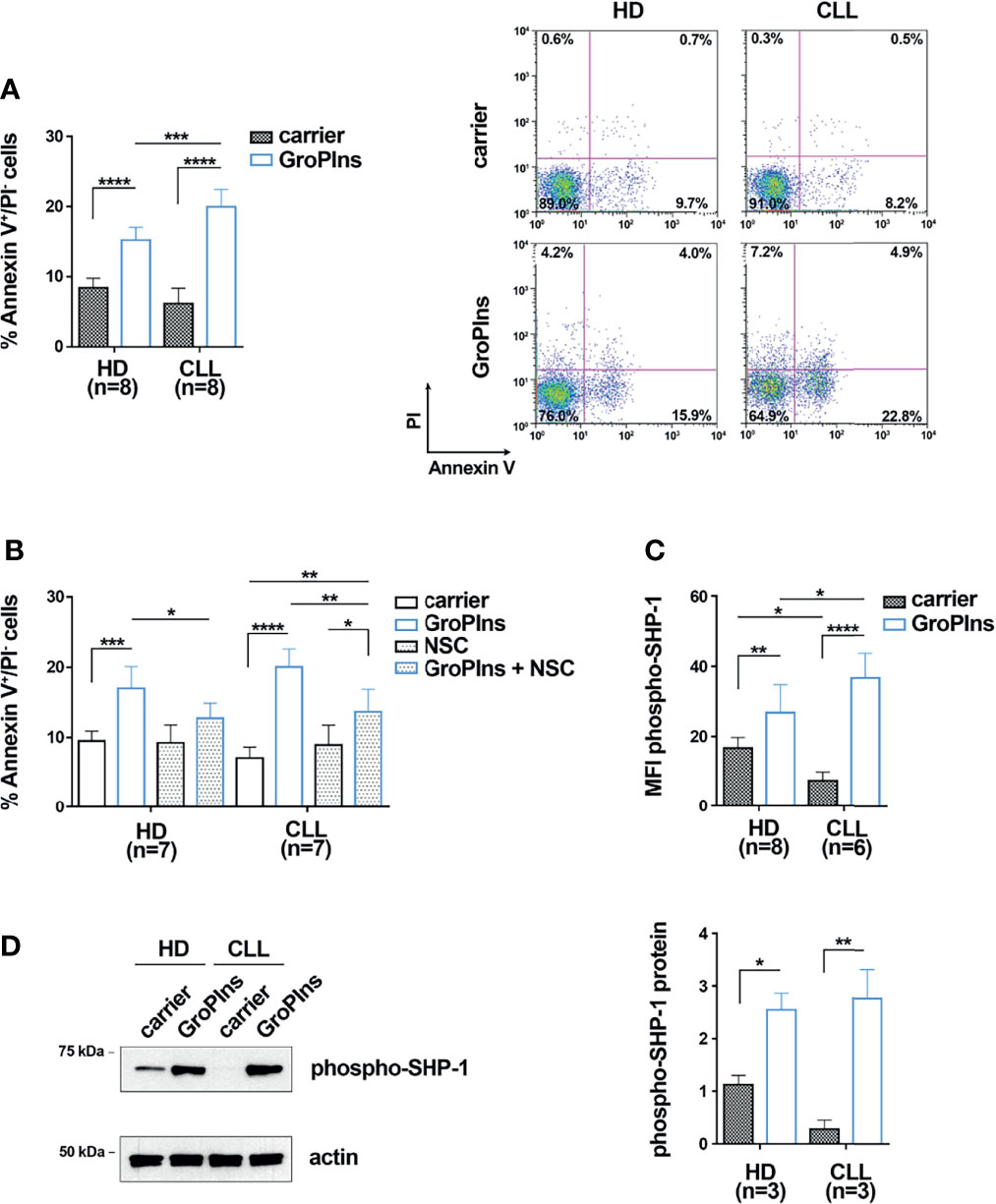
Figure 1 GroPIns promotes CLL cell apoptosis in a SHP-1-dependent manner. (A) Flow cytometric analysis of the percentages of Annexin V+/PI- cells in B lymphocytes purified from peripheral blood of healthy donors (HD; n=8) and CLL patients (CLL; n=8). Samples were treated with either carrier or 100 μM GroPIns for 24 h at 37°C. Representative panels are shown on the right. (B) Flow cytometric analysis of the percentages of Annexin V+/PI- cells in B lymphocytes purified from peripheral blood of healthy donors (HD; n=7) and CLL patients (CLL; n=7). Samples were treated for 24 h at 37°C with either carrier or 100 μM GroPIns in the presence or absence of 50 μM NSC-87887 (NSC). (C) Flow cytometric analysis of phospho-SHP-1 in B lymphocytes purified from peripheral blood of healthy donors (HD; n=8) and CLL patients (CLL; n=6), treated with either carrier or 100 μM GroPIns for 30 min at 37°C. Data are expressed as MFI phospho-SHP-1 in live cells. (D) Immunoblot analysis with anti-phospho-SHP-1 antibodies of postnuclear supernatants of B lymphocytes purified from peripheral blood of healthy donors (HD; n=3) and CLL patients (CLL; n=3). Samples were treated with either carrier or 100 μM GroPIns for 30 min at 37°C. The stripped filters were reprobed with anti-actin antibodies. Molecular weights (kDa) are indicated on the left of the panel. The quantification of three independent experiments is shown on the right. Mean ± SD. Anova two-way test, Multiple Comparison. p ≤ 0.0001, ****; p ≤ 0.001, ***; p ≤ 0.01, **; p ≤ 0.05, *.
The active form of SHP-1 is phosphorylated on tyrosine 564 (37). We hypothesized that, similar to melanoma cells (17), GroPIns interacts with and activates SHP-1 in CLL cells, thereby promoting their apoptosis. To test this hypothesis, B cells purified from peripheral blood of CLL patients and healthy controls were cultured in the presence of GroPIns and the active, phosphorylated form of SHP-1 was quantified by flow cytometry using a phospho-Y564-specific antibody (37). Consistent with previous reports (26), basal SHP-1 phosphorylation levels were significantly lower in CLL cells compared to healthy B cells (Figures 1C, D; Supplementary Figure 3). GroPIns enhanced SHP-1 phosphorylation (Figures 1C, D). These data suggest that GroPIns promotes CLL cell apoptosis by activating SHP-1. However, the fact that the enhancing effects of GroPIns on B cell apoptosis were only partially reversed by the SHP-1 inhibitor suggests that other, SHP-1-independent mechanisms may contribute to this function.
GroPIns Enhances the Expression of Bax in CLL Cells in a SHP-1-Dependent Manner
The apoptosis defects of CLL cells are caused in part by the decreased expression of the pro-apoptotic protein Bax (2). Since the phosphatase activity of SHP-1 has been causally linked to enhanced Bax expression and increased apoptosis in acute promyelocytic leukemia cells (25), we asked whether GroPIns promotes CLL cell apoptosis by upregulating Bax expression in a SHP-1-dependent manner. B cells purified from peripheral blood of CLL patients and healthy donors were cultured for 24 h in the presence of GroPIns. Bax expression was assessed by both immunoblot and qRT-PCR. Consistent with previous reports (2, 5), untreated CLL cells expressed lower Bax levels compared to healthy B cells (Figures 2A–C). GroPIns enhanced Bax expression in both CLL cells and healthy B cells (Figures 2A–C). Although the overall protein and mRNA amount of Bax was similar in healthy and CLL cells treated with GroPIns, the fold Bax expression, calculated as the ratio of Bax expression in treated versus untreated samples, was significantly higher in CLL cells compared to healthy B cells (Figures 2D, E). These results suggest a higher sensitivity of CLL cells to GroPIns compared to healthy B cells. NSC-87887 almost completely abolished the GroPIns-dependent Bax increase, demonstrating that the Bax-elevating activity of GroPIns depends on the phosphatase activity of SHP-1 (Figure 2F). Hence GroPIns promotes CLL cell apoptosis by enhancing Bax expression in a SHP-1-dependent manner. Of note, GroPIns also decreased the mRNA expression of the pro-survival Bcl-2 family members Bcl-2, MCL-1 and B2CL1 in CLL cells (Figure 2G) in a SHP-1-dependent manner (Supplementary Figure 4). These data provide evidence that GroPIns profoundly shifts the Bcl-2 family balance toward apoptosis.
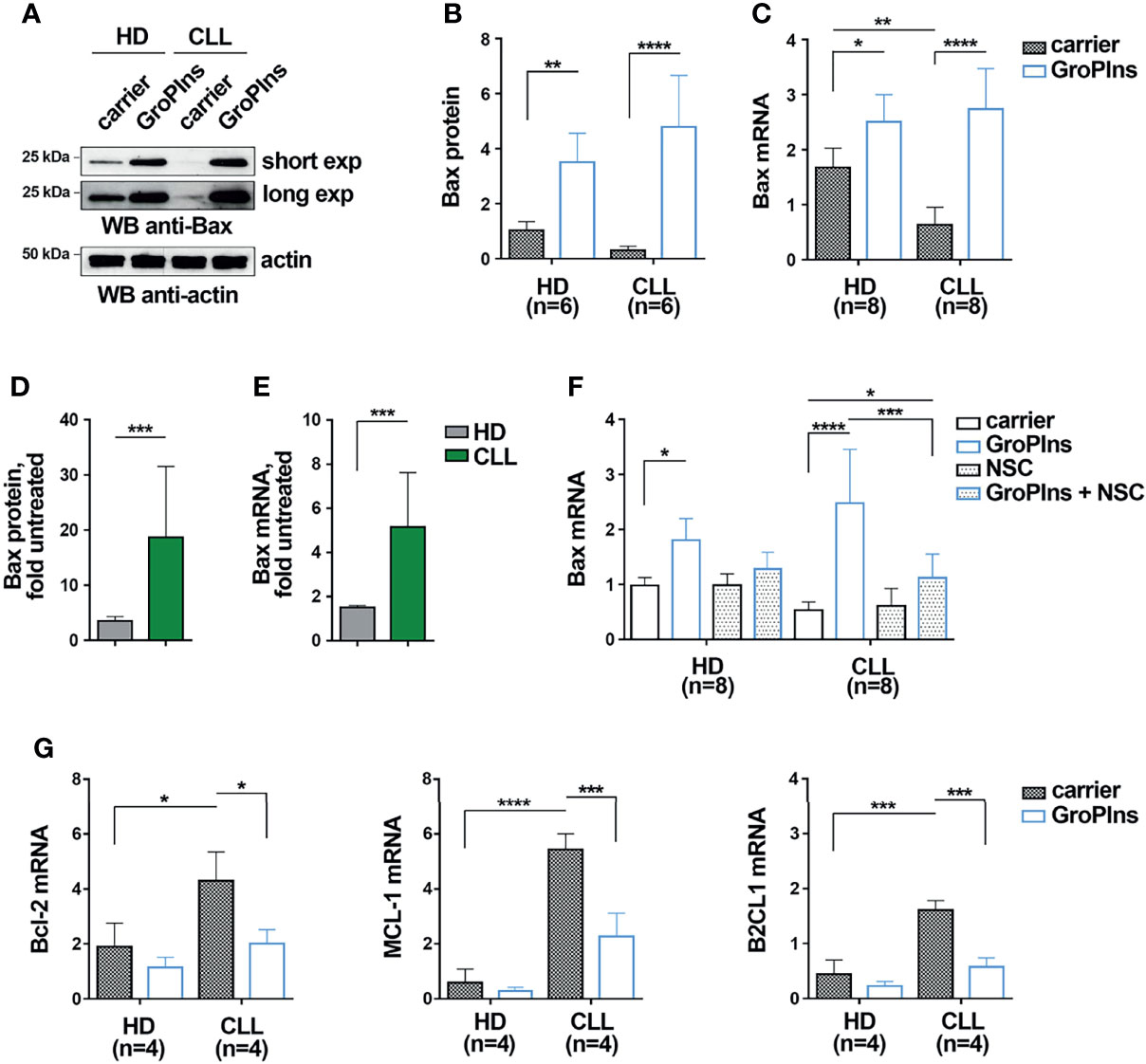
Figure 2 GroPIns promotes Bax expression in CLL cells. (A, B) Immunoblot analysis with anti-Bax antibodies of postnuclear supernatants of B lymphocytes purified from peripheral blood of healthy donors (HD; n=6) and CLL patients (CLL; n=6). Samples were treated with either carrier or 100 μM GroPIns for 24 h at 37°C. The stripped filters were reprobed with anti-actin antibodies. Molecular weights (kDa) are indicated on the left of the panel. The quantification of eight independent experiments is shown in (B, C). Quantitative RT-PCR analysis of Bax mRNA in B lymphocytes purified from peripheral blood of healthy donors (HD; n=8) and CLL patients (CLL; n=8), treated with either carrier or 100 μM GroPIns for 24 h at 37°C. The relative gene transcript abundance was determined on triplicate samples using the ddCt method and normalized to HPRT1. (D, E)). Fold protein (D) and mRNA (E) expression levels of Bax in samples from healthy donors and CLL patients. Data were calculated as fold Bax protein quantification of treated vs untreated samples shown in (B, C). (F) Quantitative RT-PCR analysis of Bax mRNA in B lymphocytes purified from peripheral blood of healthy donors (HD; n=8) and CLL patients (CLL; n=7), treated for 24 h at 37°C with either carrier or 100 μM GroPIns in the presence or absence of 50 μM NSC-87887 (NSC). The relative gene transcript abundance was determined on triplicate samples using the ddCt method and normalized to HPRT1. (G) Quantitative RT-PCR analysis of Bcl-2, MCL-1 and B2CL1 mRNA in B lymphocytes purified from peripheral blood of healthy donors (HD; n=4) and CLL patients (CLL; n=4), treated with either carrier or 100 μM GroPIns for 24 h at 37°C. The relative gene transcript abundance was determined on triplicate samples using the ddCt method and normalized to HPRT1. Mean ± SD. (B, C, F, G): Anova two-way test, Multiple Comparison. (D, E): Mann Whitney Rank Sum Test. p ≤ 0.0001, ****; p ≤ 0.001, ***; p ≤ 0.01, **; p ≤ 0.05, *.
GroPIns Interacts With and Activates Bax in CLL Cells
We previously identified SHP-1 as a direct cellular target of GroPIns by pull-down assay coupled with liquid chromatography-tandem mass-spectrometry analysis (16). Among direct interactors of GroPIns (listed in Table 1) we also found Bax. We validated the direct binding of GroPIns with Bax in in vitro pull-down assays. The immunoblot analysis of Bax showed that purified recombinant Bax was specifically pulled-down by GroPIns-Bio-bound beads but not by control Biotin-bound beads, confirming that GroPIns directly binds Bax (Figure 3A).
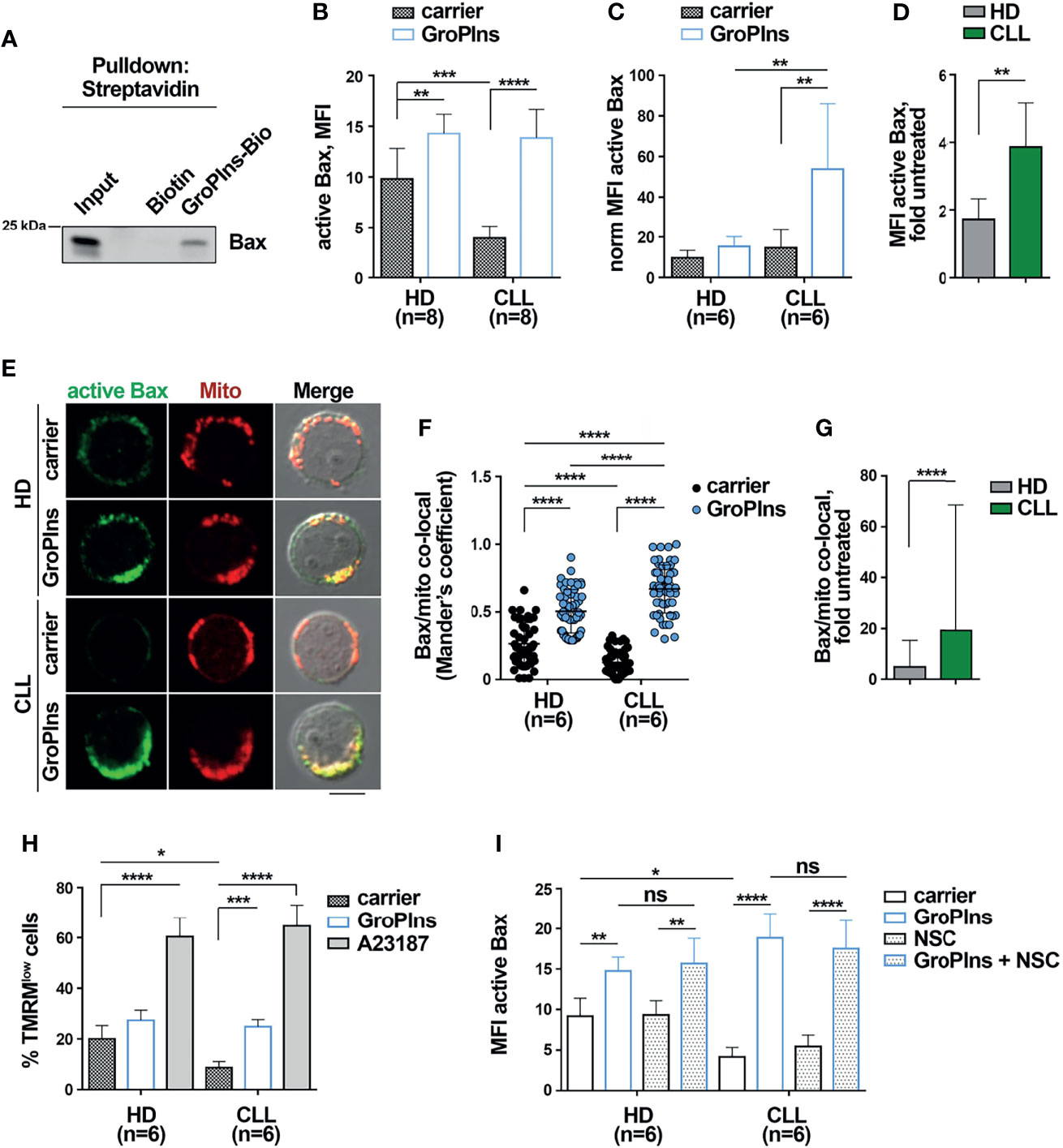
Figure 3 GroPIns interacts with and activates Bax. (A) Representative pull-down of streptavidin-conjugated beads using Biotin or biotinylated GroPIns (GroPIns-Bio) with His-Bax. Eluted proteins were analyzed by immunoblot using anti-His antibodies. Molecular weights (kDa) are indicated on the left of the panel. (B) Flow cytometric analysis of active Bax in B lymphocytes purified from peripheral blood of healthy donors (HD; n=8) and CLL patients (CLL; n=8). Samples were treated for 20 min at 37°C with either carrier or 100 μM GroPIns. (C) The MFI of active Bax shown in panel (B) was normalized to Bax protein levels of untreated cells shown in Figure 2B (n=6). (D) Fold MFI active Bax in samples from healthy donors and CLL patients shown in panel (C). Data were calculated as fold MFI of active Bax of treated vs. untreated samples. (E) Immunofluorescence analysis of active Bax (green) and mitochondria (Mitotracker) (red) in B lymphocytes purified from peripheral blood of healthy donors (HD; n=6) and CLL patients (CLL; n=6) treated for 20 min at 37°C with either carrier or 100 μM GroPIns. Immunofluorescence images were acquired on confocal microscope using 60 × objective. Representative immunofluorescence images are shown. Size bar, 5 μm. The quantification using Mander’s coefficient of the weighted colocalization of active Bax with mitochondria in individual medial confocal sections is shown in (F). (G) Fold active Bax/mitochondria co-localization in cells from healthy donors and CLL patients. Data were calculated as fold active Bax/mitochondria co-localization of treated vs untreated samples. (H) Flow cytometric analysis of the percentage of TMRMlow cells in B lymphocytes purified from peripheral blood of healthy donors (HD; n=6) and CLL patients (CLL; n=6). Samples were treated for 4 h at 37°C with either carrier or 100 μM GroPIns or 500 ng/ml A23187. Stainings were performed in duplicate. (I) Flow cytometric analysis of active Bax in B lymphocytes purified from peripheral blood of healthy donors (HD; n=6) and CLL patients (CLL; n=6). Samples were treated for 20 min at 37°C with either carrier or 100 μM GroPIns in the presence or in the absence of NSC-87887. Mean ± SD. (B, C, F, H, I): Anova two-way test, Multiple Comparison. (D, G): Mann Whitney Rank Sum Test. p ≤ 0.0001, ****; p ≤ 0.001, ***; p ≤ 0.01, **; p ≤ 0.05, *; ns, not significant..
Following pro-apoptotic stimulation, Bax undergoes a conformational change to become an active apoptosis promoter (9, 11). We assessed whether GroPIns promotes Bax activation. Purified healthy and CLL cells were treated with GroPIns for 20 min and Bax activation was assessed by flow cytometric analysis of cells stained with an anti-active Bax antibody that specifically recognizes the N-terminus of Bax which is exposed after the conformational change that accompanies Bax activation (9). The basal levels of Bax activation were significantly lower in CLL cells compared to healthy B cells (Figures 3B, D; Supplementary Figure 3). This was a consequence of the lower overall Bax levels, as assessed by normalizing the MFI of active Bax to the expression levels of Bax protein shown in Figure 2B (Figure 3C). GroPIns elicited Bax activation in CLL cells (Figures 3B, C). The fold Bax activation, calculated as the ratio of the MFI of active Bax in treated versus untreated samples, was significantly higher in CLL cells compared to healthy B cells (Figure 3D), further witnessing to a higher sensitivity of CLL cells to GroPIns compared to healthy B cells.
Active Bax translocates to the mitochondria (11). Immunofluorescence analysis of cells stained with anti-active Bax antibodies and Mitotracker Orange, a fluorescent probe that selectively stains mitochondria, showed that the colocalization of active Bax with mitochondria was significantly enhanced in both healthy and CLL cells treated for 20 min with GroPIns compared to untreated cells (Figures 3E, F). The fold active Bax/mitochondria co-localization was significantly higher in CLL cells compared to healthy B cells (Figure 3G), again demonstrating the higher sensitivity of leukemic cells to GroPIns.
Bax translocation to mitochondria leads to its oligomerization at the outer mitochondrial membrane, which in turn promotes mitochondrial depolarization (11). Purified healthy and CLL cells loaded with the fluorescent probe TMRM were treated for 4 h with GroPIns or with the calcium ionophore A23187, a potent inducer of apoptosis (38), and mitochondria depolarization was assessed by flow cytometric quantification of the percentage of TMRMlow cells (Supplementary Figure 5). Mitochondrial depolarization was significantly enhanced in CLL cells treated with GroPIns when compared to untreated cells (Figure 3H). Of note, GroPIns elicited a slight, yet not significant increase in mitochondrial depolarization in healthy B cells (Figure 3H). These data demonstrate that GroPIns potently acts on CLL cells to restore apoptosis. The SHP-1 inhibitor NSC-87887 did not impair GroPIns-mediated Bax activation (Figure 3I), suggesting that GroPIns-mediated Bax activation does not require SHP-1. Collectively, these results support the existence of two unrelated pathways, of which one is SHP-1-dependent and one independent, converging on Bax and exploited by GroPIns to promote CLL cell apoptosis.
GroPIns Enhances the Pro-Apoptotic Effects of Venetoclax on CLL Cells
The Bcl-2 inhibitor Venetoclax promotes CLL cell apoptosis (39), and induces rapid and pronounced activation and mitochondrial translocation of Bax in cell lines of acute myeloid leukemia (40). We tested whether the combination of GroPIns with Venetoclax further enhances Venetoclax-induced CLL cell apoptosis. As shown in Figure 4A, the combination of GroPIns and Venetoclax enhanced apoptosis of leukemic cells compared to single treatments (Figure 4A), suggesting a synergic pro-apoptotic activity of GroPIns and Venetoclax in these cells. This was confirmed by analyzing Bax expression (Figure 4B) and activation (Figure 4C), which were enhanced in CLL cells subjected to combination treatments compared to single treatments (Figures 4B, C). Of note, while Venetoclax did not affect the expression of MCL-1 and B2CL1 in CLL cells, it led to a decrease in Bcl-2 expression to levels similar to GroPIns, which were further decreased in combination treatments (Figure 4D). The flow cytometric analysis of early apoptotic cells performed in CLL cells treated for 24 h with increasing concentrations of GroPIns alone or in combination with Venetoclax showed a Combination Index (CI) below 1 (CI=0.68; Figure 4E), indicating a synergic cooperation between GroPIns and Venetoclax to promote CLL cell apoptosis.
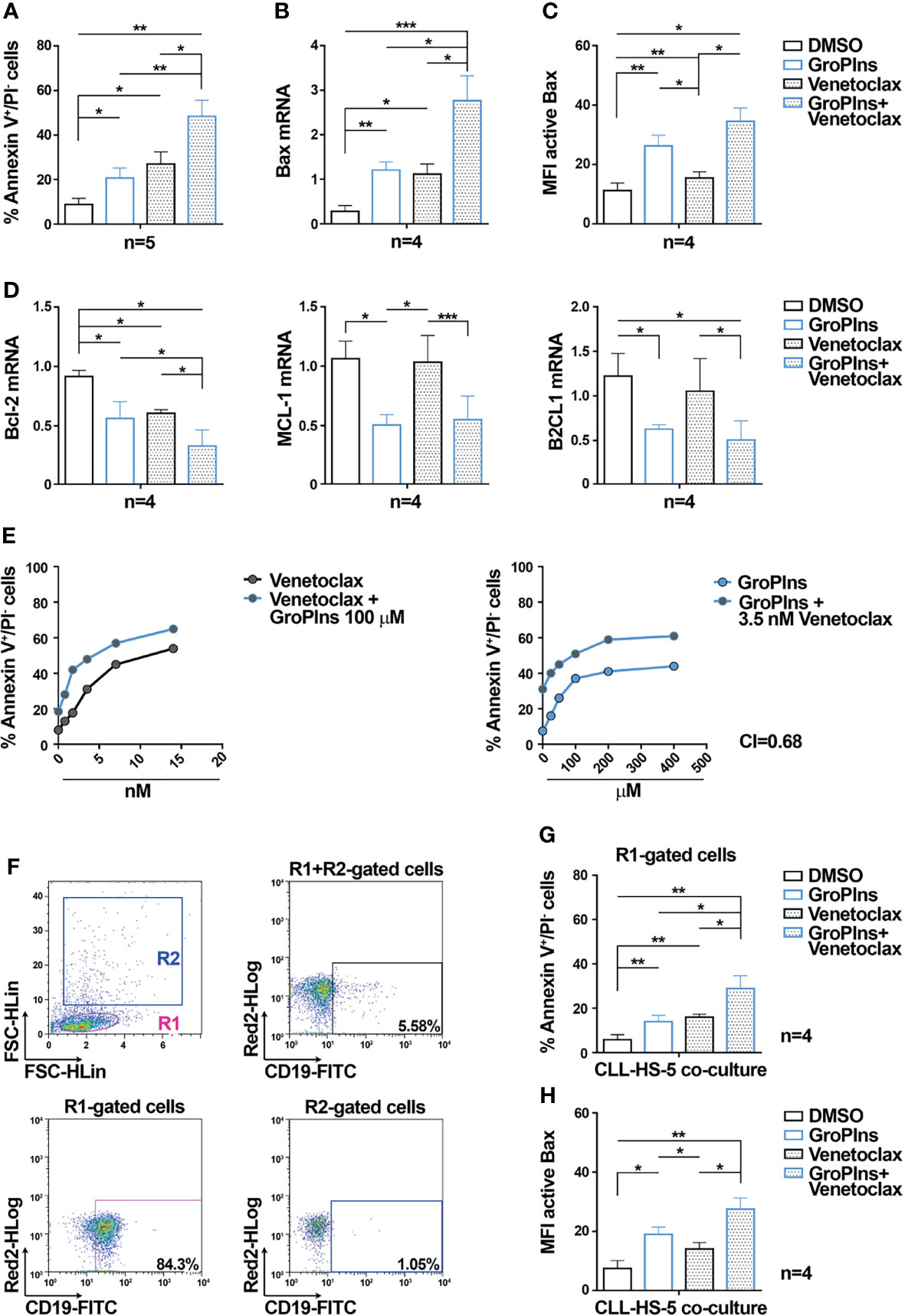
Figure 4 GroPIns enhances the pro-apoptotic activity of Venetoclax in CLL cells. (A) Flow cytometric analysis of the percentages of Annexin V+/PI- cells in B lymphocytes purified from peripheral blood of CLL patients (CLL; n=5) treated with either 100 μM GroPIns or 3.5 nM Venetoclax or the combination of both for 24 h at 37°C. (B) Quantitative RT-PCR analysis of Bax mRNA in B lymphocytes purified from peripheral blood of CLL patients (CLL; n=4) and treated as in (A). The relative gene transcript abundance was determined on triplicate samples using the ddCt method and normalized to HPRT1. (C) Flow cytometric analysis of active Bax in B lymphocytes purified from peripheral blood of CLL patients (CLL; n=4) and treated for 20 min at 37°C with either 100 μM GroPIns or 3.5 nM Venetoclax or the combination of both. (D) Quantitative RT-PCR analysis of Bcl-2, MCL-1 and B2CL1 mRNA in B lymphocytes purified from peripheral blood of healthy donors (HD; n=4) and CLL patients (CLL; n=4), treated as above. The relative gene transcript abundance was determined on triplicate samples using the ddCt method and normalized to HPRT1. (E) Flow cytometric analysis of the percentages of Annexin V+/PI- cells in B lymphocytes purified from peripheral blood of 2 CLL patients treated with either GroPIns or Venetoclax or with the combination of both at the indicated concentrations for 24 h at 37°C. The calculated Cooperation Index (CI) is indicated. (F–H) Flow cytometric analysis of the percentages of Annexin V+/PI- cells (G) and of Bax activation (H) in B lymphocytes purified from peripheral blood of CLL patients (CLL; n=4) co-cultured with HS-5 stromal cells for 24 h at 37°C in the presence of either 100 μM GroPIns or 3.5 nM Venetoclax or the combination of both. Analysis was carried out on R1-gated CD19+ cells. The gating strategy is shown in (F). Mean ± SD. Anova one-way test, Multiple Comparison. p ≤ 0.001, ***; p ≤ 0.01, **; p ≤ 0.05, *.
Fludarabine, a chemotherapeutic drug used in the treatment of a small subset of CLL patients alone or in combination with other chemotherapeutic or immunomodulatory drugs, enhances Bax activation and expression and promotes apoptosis of CLL cells (9, 10). We tested whether, similar to Venetoclax, the combination of GroPIns with Fludarabine further enhances Fludarabine-induced CLL cell apoptosis. GroPIns enhanced Fludarabine-induced CLL cell apoptosis as well as Bax activation, expression and translocation to mitochondria compared to single treatments (Supplementary Figure 6A-F). However, as opposed to Venetoclax, Fludarabine and GroPIns did not act in synergy to enhance CLL cell apoptosis, but rather showed independent effects (Supplementary Figure 6G).
The stromal microenvironment strongly contributes to protect CLL cells from apoptosis (6). We assessed the pro-apoptotic effect of GroPIns and Venetoclax, alone or in combination treatments, in CLL cells co-cultured for 24 h with the human stromal cell line HS-5 (29). As shown in Figures 4F–H, the combination of GroPIns and Venetoclax enhanced both apoptosis and Bax activation in leukemic cells co-cultured with HS-5 cells compared to single treatments (Figure 4A), albeit with less pronounced effects which are likely to be accounted for by the protective role of stromal cells on CLL cells.
These results demonstrate that GroPIns displays a pro-apoptotic activity also in the presence of drugs known to promote CLL cell apoptosis.
Discussion
Apoptosis, which plays important roles in organism development and tissue homeostasis, becomes critical for the elimination of unwanted, damaged or infected cells (41). Insufficient apoptosis has been related to the onset and progression of cancer by extending tumor cell survival and promoting their resistance to treatment (42). A profound imbalance among Bcl-2 family members is a major factor in the apoptosis defects of CLL cells, which play a major role in leukemic cell accumulation in secondary lymphoid organs, where they are protected from chemotherapy (1, 2). The pro-survival protein Bcl-2, whose expression is frequently upregulated in CLL as a result of deletion of mir15-a/mir16-1, located at 13q14 and known to target BCL-2 mRNA (43), had long been viewed as a promising target for CLL therapy. In 2016 the selective Bcl-2 inhibitor Venetoclax, which acts as a BH3-mimetic to facilitate the activation of pro-apoptotic Bcl-2 family members, was approved for relapsed/refractory CLL (7). Since then, new combination therapy regimens have been approved for CLL treatment (8) and usually applied as first-line therapy. The use of chemoimmunotherapeutics such as Fludarabine, cyclophosphamide and rituximab has progressively decreased through the years as a consequence of the higher efficacy and better tolerability of targeted agents like Venetoclax. However, none of the recently introduced therapies appears to cure CLL, and some patients become resistant to Venetoclax due to the acquisition of Bcl-2 mutations.
Pro-apoptotic stimuli activate Bax, a major pro-apoptotic member of the Bcl-2 family, either directly or indirectly, leading to mitochondrial membrane permeabilization, release of the apoptotic factor cytochrome c and cancer cell death (11). The expression of Bax is profoundly impaired in CLL cells (2), which contributes to their apoptosis defects. A number of drugs currently in clinical use for the treatment of several types of cancer are known to indirectly enhance Bax expression and activation, including Fludarabine (9, 10) and Venetoclax (40). Here we demonstrate that GroPIns promotes CLL cell apoptosis by enhancing Bax expression. Moreover, GroPIns enhances the pro-apoptotic effects of both Venetoclax and Fludarabine, leading to higher levels of CLL cell apoptosis compared to single treatments. Interestingly, the activity of GroPIns and Venetoclax converge toward tilting the Bcl-2 family balance toward apoptosis, on the one hand by enhancing the expression and potentiating the activation of Bax, and on the other hand by decreasing the expression and inhibiting the activity of Bcl-2. Our findings highlight a potential new combinatorial strategy aimed at potentiating the pro-apoptotic activity of Venetoclax with a natural and well-tolerated compound, which could overcome potential resistance mechanisms to Venetoclax used as single agent (44).Several classes of small molecules have been identified in the last decade that selectively activate Bax to induce apoptosis, which demonstrated good in vitro but moderate in vivo anti-cancer activity (45, 46). The compound SMBA1 potently activates Bax and acts both in vitro and in vivo against lung cancer (47). New recently synthesized SMBA1 analogs show anti-proliferative activity against breast cancer (48). However, none of these molecules has been tested in CLL to date. In 2020 the small molecule BDA-366, a BH4-domain antagonist that kills both lung cancer and multiple myeloma cells, was tested for its therapeutic potential and mechanism of action in CLL and DLBCL. However, although BDA-366 displayed selective toxicity against both cell types, the underlying mechanism of Bax activation is as yet unknown (11, 49). Here we identified GroPIns as a naturally-occurring molecule provided with the intrinsic ability to bind and activate Bax. This makes of GroPIns an interesting pro-apoptotic molecule to be tested in malignancies characterized by hypoexpression or hypoactivation of Bax.
Along with an aberrant expression of anti-apoptotic molecules, CLL cells show high levels of intracellular phosphorylation mediated by the hyperactivation of several kinases downstream of the B-cell receptor, such as Lyn, Syk, Btk, PI3K, and AKT (50, 51). This condition is further sustained by an impairment in the expression or function of phosphatases. The expression of PTEN (52), CD45 (53), PTPROt (54), PHLPP1 (55, 56), PP2A (57), and SHIP1 (58) are significantly decreased in CLL cells, whereas PTPN22, which acts as a positive regulator of anti-apoptotic signals by hampering the negative regulation of B-cell receptor-dependent signaling pathways, is overexpressed (59). By contrast SHP-1, a tyrosine phosphatase that participates in signaling pathways regulating proliferation, survival and apoptosis of both hematopoietic and non-hematopoietic cells (18), is expressed in CLL cells at levels comparable to normal B cells (60) but is functionally dysregulated by mechanisms that are mediated by the Src family kinase Lyn (26), making this phosphatase an interesting target for activating-drug discovery.
Drugs able to promote phosphatase activity have been demonstrated to be effective in CLL. The novel SHIP-1 activator AQX-435 was demonstrated to be effective in the inhibition of anti-IgM-induced AKT phosphorylation, resulting in CLL cell apoptosis in vitro (61). Conversely, SHP-1 has proven to be an extremely challenging drug target, due both to the highly conserved and positively charged nature of its phosphatase active site, and to the lack of either appropriate selectivity or membrane permeability of the majority of phosphatase inhibitors (62). We previously reported that in melanoma cells GroPIns interacts with SHP-1, promoting its recruitment to invadopodia where it dephosphorylates critical components of the actin polymerization pathways leading to matrix invasion, thereby counteracting metastasis (17). Here we added a tile to the puzzle by demonstrating that in CLL cells GroPIns enhances SHP-1 phosphorylation. Although the molecular mechanism underlying the GroPIns-dependent enhancement in SHP-1 phosphorylation remains unknown, we hypothesize that the interaction of GroPIns with SHP-1 might either stabilize SHP-1 in an active conformation, or alternatively promote its interaction with a specific kinase, thereby favoring SHP-1 phosphorylation. It is noteworthy that SHP-1 not only acts through dephosphorylation (18), but also promotes Bax expression (23, 25) through signaling pathways involving the MAP kinase p38 (25) and the transcription factor STAT3 (23). Our data show that, by promoting SHP-1 phosphorylation, GroPIns enhances Bax expression and CLL cell apoptosis. The existence of two distinct and independent pathways that, by taking advantage of the two GroPIns interactors SHP-1 and Bax, both converge to promote CLL cell apoptosis, contribute to enhance the activity of this compound. In this scenario GroPIns, via direct binding to and modulation of SHP-1 and Bax, could be an interesting tool to restore apoptosis in CLL cells.
Data Availability Statement
The original contributions presented in the study are included in the article/Supplementary Material. Further inquiries can be directed to the corresponding authors.
Ethics Statement
The studies involving human participants were reviewed and approved by Azienda Ospedaliera Universitaria di Padova, Padova Hospital and Azienda Ospedaliera Universitaria di Siena, Siena Hospital. The patients/participants provided their written informed consent to participate in this study.
Author Contributions
GB, ASV, VT, NC, FF, ADV, LT, DC, LP, and CB designed research and analyzed and interpreted data. GB, ASV, VT, NC, FF, ADV, and LP performed research. ASV, FF, ADV, LT, and DC contributed vital reagents. ASV, NC, FF, ADV, LT, DC, LP, and CB drafted the manuscript. All authors contributed to the article and approved the submitted version.
Funding
The research leading to these results has received funding from AIRC under IG 2017 - ID. 20148 – and Regione Toscana, ID. PRECISE-CLL- to Baldari Cosima. This work was also supported by grants from AIRC to LT (IG-25024) and AIRC (IG-18776), PRIN (20177XJCHX) and the SATIN project 2014–2020 to DC.
Conflict of Interest
The authors declare that the research was conducted in the absence of any commercial or financial relationships that could be construed as a potential conflict of interest.
Publisher’s Note
All claims expressed in this article are solely those of the authors and do not necessarily represent those of their affiliated organizations, or those of the publisher, the editors and the reviewers. Any product that may be evaluated in this article, or claim that may be made by its manufacturer, is not guaranteed or endorsed by the publisher.
Acknowledgments
The authors would like to thank Stefania Mariggiò for initial discussion of this project, and Professor Piero Pucci and the Proteomic Facility at CEINGE Biotecnologie Avanzate for support in proteomic and data analysis.
Supplementary Material
The Supplementary Material for this article can be found online at: https://www.frontiersin.org/articles/10.3389/fonc.2022.835290/full#supplementary-material
References
1. Kipps TJ, Stevenson FK, Wu CJ, Croce CM, Packham G, Wierda WG, et al. Chronic Lymphocytic Leukaemia. Nat Rev Dis Prim (2017) 3:16096. doi: 10.1038/nrdp.2016.96
2. Packham G, Stevenson FK. Bodyguards and Assassins: Bcl-2 Family Proteins and Apoptosis Control in Chronic Lymphocytic Leukaemia. Immunology (2005) 114:441–9. doi: 10.1111/j.1365-2567.2005.02117.x
3. Pekarsky Y, Croce CM. Role of miR-15/16 in CLL. Cell Death Differ (2015) 22:6–11. doi: 10.1038/cdd.2014.87
4. Saxena A, Viswanathan S, Moshynska O, Tandon P, Sankaran K, Sheridan DP. Mcl-1 and Bcl-2/Bax Ratio Are Associated With Treatment Response But Not With Rai Stage in B-Cell Chronic Lymphocytic Leukemia. Am J Hematol (2004) 75:22–33. doi: 10.1002/ajh.10453
5. Capitani N, Lucherini OM, Sozzi E, Ferro M, Giommoni N, Finetti F, et al. Impaired Expression of p66Shc, a Novel Regulator of B-Cell Survival, in Chronic Lymphocytic Leukemia. Blood (2010) 115:3726–36. doi: 10.1182/blood-2009-08-239244
6. Ten Hacken E, Burger JA. Microenvironment Interactions and B-Cell Receptor Signaling in Chronic Lymphocytic Leukemia: Implications for Disease Pathogenesis and Treatment. Biochim Biophys Acta (2016) 1863:401–13. doi: 10.1016/j.bbamcr.2015.07.009
7. Roberts AW, Davids MS, Pagel JM, Kahl BS, Puvvada SD, Gerecitano JF, et al. Targeting BCL2 With Venetoclax in Relapsed Chronic LymphocyticLeukemia. N Engl J Med (2016) 374:311. doi: 10.1056/NEJMOA1513257
8. Hallek M. Chronic Lymphocytic Leukemia: 2020 Update on Diagnosis, Risk Stratification and Treatment. Am J Hematol (2019) 94:1266–87. doi: 10.1002/AJH.25595
9. Bellosillo B, Villamor N, López-Guillermo A, Marcé S, Bosch F, Campo E, et al. Spontaneous and Drug-Induced Apoptosis Is Mediated by Conformational Changes of Bax and Bak in B-Cell Chronic Lymphocytic Leukemia. Blood (2002) 100:1810–6. doi: 10.1182/blood-2001-12-0327
10. Zhou Z, Fang Q, Li P, Ma D, Zhe N, Ren M, et al. Entinostat Combined With Fludarabine Synergistically Enhances the Induction of Apoptosis in TP53 Mutated CLL Cells via the HDAC1/HO-1 Pathway. Life Sci (2019) 232:116583. doi: 10.1016/j.lfs.2019.116583
11. Liu Z, Ding Y, Ye N, Wild C, Chen H, Zhou J. Direct Activation of Bax Protein for Cancer Therapy. Med Res Rev (2016) 36:313–41. doi: 10.1002/med.21379
12. Valitutti S, Cucchi P, Colletta G, Di Filippo C, Corda D. Transformation by the K-Ras Oncogene Correlates With Increases in Phospholipase A2 Activity, Glycerophosphoinositol Production and Phosphoinositide Synthesis in Thyroid Cells. Cell Signal (1991) 3:321–32. doi: 10.1016/0898-6568(91)90061-X
13. Corda D, Zizza P, Varone A, Filippi BM, Mariggiò S. The Glycerophosphoinositols: Cellular Metabolism and Biological Functions. Cell Mol Life Sci (2009) 66:3449–67. doi: 10.1007/s00018-009-0113-4
14. Patrussi L, Mariggiò S, Corda D, Baldari CT. The Glycerophosphoinositols: From Lipid Metabolites to Modulators of T-Cell Signaling. Front Immunol (2013) 4:213. doi: 10.3389/fimmu.2013.00213
15. Vessichelli M, Mariggiò S, Varone A, Zizza P, Di Santo A, Amore C, et al. The Natural Phosphoinositide Derivative Glycerophosphoinositol Inhibits the Lipopolysaccharide-Induced Inflammatory and Thrombotic Responses. J Biol Chem (2017) 292:12828–41. doi: 10.1074/jbc.M116.773861
16. Varone A, Mariggiò S, Patheja M, Maione V, Varriale A, Vessichelli M, et al. A Signalling Cascade Involving Receptor-Activated Phospholipase A2, Glycerophosphoinositol 4-Phosphate, Shp1 and Src in the Activation of Cell Motility. Cell Commun Signal (2019) 17:20. doi: 10.1186/s12964-019-0329-3
17. Varone A, Amoruso C, Monti M, Patheja M, Greco A, Auletta L, et al. The Phosphatase Shp1 Interacts With and Dephosphorylates Cortactin to Inhibit Invadopodia Function. Cell Commun Signal (2021) 19:64. doi: 10.1186/s12964-021-00747-6
18. Varone A, Spano D, Corda D. Shp1 in Solid Cancers and Their Therapy. Front Oncol (2020) 10:935. doi: 10.3389/fonc.2020.00935
19. Zhang Q, Wang HY, Marzec M, Raghunath PN, Nagasawa T, Wasik MA. STAT3- and DNA Methyltransferase 1-Mediated Epigenetic Silencing of SHP-1 Tyrosine Phosphatase Tumor Suppressor Gene in Malignant T Lymphocytes. Proc Natl Acad Sci USA (2005) 102:6948–53. doi: 10.1073/pnas.0501959102
20. Wen LZ, Ding K, Wang ZR, Ding CH, Lei SJ, Liu JP, et al. SHP-1 Acts as a Tumor Suppressor in Hepatocarcinogenesis and HCC Progression. Cancer Res (2018) 78:4680–91. doi: 10.1158/0008-5472.CAN-17-3896
21. Koh JS, Joo MK, Park JJ, Yoo HS, Il CB, BJ L, et al. Inhibition of STAT3 in Gastric Cancer: Role of Pantoprazole as SHP-1 Inducer. Cell Biosci (2018) 8:50. doi: 10.1186/s13578-018-0248-9
22. Sharma Y, Ahmad A, Bashir S, Elahi A, Khan F. Implication of Protein Tyrosine Phosphatase SHP-1 in Cancer-Related Signaling Pathways. Futur Oncol (2016) 12:1287–98. doi: 10.2217/fon-2015-0057
23. Chen H, Zhu B, Zhao L, Liu Y, Zhao F, Feng J, et al. Allicin Inhibits Proliferation and Invasion In Vitro and In Vivo via SHP-1-Mediated STAT3 Signaling in Cholangiocarcinoma. Cell Physiol Biochem (2018) 47:641–53. doi: 10.1159/000490019
24. Saraswati S, Alhaider A, Abdelgadir AM, Tanwer P, Korashy HM. Phloretin Attenuates STAT-3 Activity and Overcomes Sorafenib Resistance Targeting SHP-1–Mediated Inhibition of STAT3 and Akt/VEGFR2 Pathway in Hepatocellular Carcinoma. Cell Commun Signal (2019) 17:127. doi: 10.1186/S12964-019-0430-7
25. Gan L, Zhong L, Shan Z, Xiao C, Xu T, Song H, et al. Epigallocatechin-3-Gallate Induces Apoptosis in Acute Promyelocytic Leukemia Cells via a SHP-1-P38α MAPK-Bax Cascade. Oncol Lett (2017) 14:6314–20. doi: 10.3892/ol.2017.6980
26. Tibaldi E, Pagano MA, Frezzato F, Trimarco V, Facco M, Zagotto G, et al. Targeted Activation of the SHP-1/PP2A Signaling Axis Elicits Apoptosis of Chronic Lymphocytic Leukemia Cells. Haematologica (2017) 102:1401–12. doi: 10.3324/haematol.2016.155747
27. Hallek M, Cheson BD, Catovsky D, Caligaris-Cappio F, Dighiero G, Döhner H, et al. Hallek 2008 Blood Glines for Diagnois and Treament of Chrn Lymph Leuk. Blood (2008) 111:5446–56. doi: 10.1182/blood-2007-06-093906
28. Visentin A, Facco M, Gurrieri C, Pagnin E, Martini V, Imbergamo S, et al. Prognostic and Predictive Effect of IGHV Mutational Status and Load in Chronic Lymphocytic Leukemia: Focus on FCR and BR Treatments. Clin Lymphoma Myeloma Leuk (2019) 19:678–685.e4. doi: 10.1016/j.clml.2019.03.002
29. Patrussi L, Manganaro N, Capitani N, Ulivieri C, Tatangelo V, Libonati F, et al. Enhanced IL-9 Secretion by p66Shc-Deficient CLL Cells Modulates the Chemokine Landscape of the Stromal Microenvironment. Blood (2021) 137:2182–95. doi: 10.1182/blood.2020005785
30. Roecklein BA, Torok-Storb B. Functionally Distinct Human Marrow Stromal Cell Lines Immortalized by Transduction With the Human Papilloma Virus E6/E7 Genes. Blood (1995) 85:997–1005. doi: 10.1182/blood.V85.4.997.bloodjournal854997
31. Patrussi L, Capitani N, Cannizzaro E, Finetti F, Lucherini OM, Pelicci PG, et al. Negative Regulation of Chemokine Receptor Signaling and B-Cell Chemotaxis by p66Shc. Cell Death Dis (2014) 5:e1068. doi: 10.1038/cddis.2014.44
32. Marani M, Tenev T, Hancock D, Downward J, Lemoine NR. Identification of Novel Isoforms of the BH3 Domain Protein Bim Which Directly Activate Bax To Trigger Apoptosis. Mol Cell Biol (2002) 22:3577–89. doi: 10.1128/mcb.22.11.3577-3589.2002
33. Finetti F, Patrussi L, Masi G, Onnis A, Galgano D, Lucherini OM, et al. Specific Recycling Receptors are Targeted to the Immune Synapse by the Intraflagellar Transport System. J Cell Sci (2014) 127:1924–37. doi: 10.1242/jcs.139337
34. Patrussi L, Capitani N, Cattaneo F, Manganaro N, Gamberucci A, Frezzato F, et al. p66Shc Deficiency Enhances CXCR4 and CCR7 Recycling in CLL B Cells by Facilitating Their Dephosphorylation-Dependent Release From β-Arrestin at Early Endosomes. Oncogene (2018) 37:1534–50. doi: 10.1038/s41388-017-0066-2
35. Liu Q, Yin X, Languino LR, Altieri DC. Evaluation of Drug Combination Effect Using a Bliss Independence Dose–Response Surface Model. Stat Biopharm Res (2018) 10:112–22. doi: 10.1080/19466315.2018.1437071
36. Chou TC, Talalay P. Quantitative Analysis of Dose-Effect Relationships: The Combined Effects of Multiple Drugs or Enzyme Inhibitors. Adv Enzyme Regul (1984) 22:27–55. doi: 10.1016/0065-2571(84)90007-4
37. Xiao W, Ando T, Wang HY, Kawakami Y, Kawakami T. Lyn-And PLC-β3-Dependent Regulation of SHP-1 Phosphorylation Controls Stat5 Activity and Myelomonocytic Leukemia-Like Disease. Blood (2010) 116:6003–13. doi: 10.1182/blood-2010-05-283937
38. Bortner CD, Cidlowski JA. Caspase Independent/Dependent Regulation of K+, Cell Shrinkage, and Mitochondrial Membrane Potential During Lymphocyte Apoptosis. J Biol Chem (1999) 274:21953–62. doi: 10.1074/jbc.274.31.21953
39. Itchaki G, Brown JR. The Potential of Venetoclax (ABT-199) in Chronic Lymphocytic Leukemia. Ther Adv Hematol (2016) 7:270–87. doi: 10.1177/2040620716655350
40. Rahmani M, Nkwocha J, Hawkins E, Pei X, Parker RE, Kmieciak M, et al. Cotargeting BCL-2 and PI3K Induces BAX-Dependent Mitochondrial Apoptosis in AML Cells. Cancer Res (2018) 78:3075–86. doi: 10.1158/0008-5472.CAN-17-3024
41. Ashkenazi A, Salvesen G. Regulated Cell Death: Signaling and Mechanisms. Annu Rev Cell Dev Biol (2014) 30:337–56. doi: 10.1146/annurev-cellbio-100913-013226
42. Carneiro BA, El-Deiry WS. Targeting Apoptosis in Cancer Therapy. Nat Rev Clin Oncol (2020) 17:395–417. doi: 10.1038/s41571-020-0341-y
43. Cimmino A, Calin GA, Fabbri M, Iorio MV, Ferracin M, Shimizu M, et al. miR-15 and miR-16 Induce Apoptosis by Targeting BCL2. Proc Natl Acad Sci USA (2005) 102:13944–9. doi: 10.1073/pnas.0506654102
44. Fürstenau M, Eichhorst B. Novel Agents in Chronic Lymphocytic Leukemia: New Combination Therapies and Strategies to Overcome Resistance. Cancers (Basel) (2021) 13:1–18. doi: 10.3390/cancers13061336
45. Stornaiuolo M, La Regina G, Passacantilli S, Grassia G, Coluccia A, La Pietra V, et al. Structure-Based Lead Optimization and Biological Evaluation of BAX Direct Activators as Novel Potential Anticancer Agents. J Med Chem (2015) 58:2135–48. doi: 10.1021/jm501123r
46. Zhao G, Zhu Y, Eno CO, Liu Y, DeLeeuw L, Burlison JA, et al. Activation of the Proapoptotic Bcl-2 Protein Bax by a Small Molecule Induces Tumor Cell Apoptosis. Mol Cell Biol (2014) 34:1198–207. doi: 10.1128/mcb.00996-13
47. Xin M, Li R, Xie M, Park D, Owonikoko TK, Sica GL, et al. Small-Molecule Bax Agonists for Cancer Therapy. Nat Commun (2014) 5:4935. doi: 10.1038/ncomms5935
48. Liu G, Yin T, Kim H, Ding C, Yu Z, Wang H, et al. Structure-Activity Relationship Studies on Bax Activator SMBA1 for the Treatment of ER-Positive and Triple-Negative Breast Cancer. Eur J Med Chem (2019) 178:589–605. doi: 10.1016/j.ejmech.2019.06.004
49. Vervloessem T, Sasi BK, Xerxa E, Karamanou S, Kale J, La Rovere RM, et al. BDA-366, a Putative Bcl-2 BH4 Domain Antagonist, Induces Apoptosis Independently of Bcl-2 in a Variety of Cancer Cell Models. Cell Death Dis (2020) 11:769. doi: 10.1038/s41419-020-02944-6
50. Zhang S, Kipps TJ. The Pathogenesis of Chronic Lymphocytic Leukemia. Annu Rev Pathol (2014) 9:103–18. doi: 10.1146/annurev-pathol-020712-163955
51. Stevenson FK, Forconi F, Packham G. The Meaning and Relevance of B-Cell Receptor Structure and Function in Chronic Lymphocytic Leukemia. Semin Hematol (2014) 51:158–67. doi: 10.1053/j.seminhematol.2014.05.003
52. Zou ZJ, Fan L, Wang L, Xu J, Zhang R, Tian T, et al. miR-26a and miR-214 Down-Regulate Expression of the PTEN Gene in Chronic Lymphocytic Leukemia, But Not PTEN Mutation or Promoter Methylation. Oncotarget (2015) 6:1276–85. doi: 10.18632/oncotarget.2626
53. Rizzo D, Lotay A, Gachard N, Marfak I, Faucher JL, Trimoreau F, et al. Very Low Levels of Surface CD45 Reflect CLL Cell Fragility, are Inversely Correlated With Trisomy 12 and Are Associated With Increased Treatment-Free Survival. Am J Hematol (2013) 88:747–53. doi: 10.1002/ajh.23494
54. Motiwala T, Majumder S, Kutay H, Smith DS, Neuberg DS, Lucas DM, et al. Methylation and Silencing of Protein Tyrosine Phosphatase Receptor Type O in Chronic Lymphocytic Leukemia. Clin Cancer Res (2007) 13:3174–81. doi: 10.1158/1078-0432.CCR-06-1720
55. Suljagic M, Longo PG, Bennardo S, Perlas E, Leone G, Laurenti L, et al. The Syk Inhibitor Fostamatinib Disodium (R788) Inhibits Tumor Growth in the E - TCL1 Transgenic Mouse Model of CLL by Blocking Antigen-Dependent B-Cell Receptor Signaling. Blood (2010) 116:4894–905. doi: 10.1182/blood-2010-03-275180
56. O’Hayre M, Niederst M, Fecteau JF, Nguyen VM, Kipps TJ, Messmer D, et al. Mechanisms and Consequences of the Loss of PHLPP1 Phosphatase in Chronic Lymphocytic Leukemia (CLL). Leukemia (2012) 26:1689–92. doi: 10.1038/leu.2012.6
57. Zonta F, Pagano MA, Trentin L, Tibaldi E, Frezzato F, Trimarco V, et al. Lyn Sustains Oncogenic Signaling in Chronic Lymphocytic Leukemia by Strengthening SET-Mediated Inhibition of PP2A. Blood (2015) 125:3747–55. doi: 10.1182/blood-2014-12-619155
58. Cui B, Chen L, Zhang S, Mraz M, Fecteau JF, Yu J, et al. Micro RNA-155 Influences B-Cell Receptor Signaling and Associates With Aggressive Disease in Chronic Lymphocytic Leukemia. Blood (2014) 124:546–54. doi: 10.1182/blood-2014-03-559690
59. Negro R, Gobessi S, Longo PG, He Y, Zhang ZY, Laurenti L, et al. Overexpression of the Autoimmunity-Associated Phosphatase PTPN22 Promotes Survival of Antigen-Stimulated CLL Cells by Selectively Activating AKT. Blood (2012) 119:6278–87. doi: 10.1182/blood-2012-01-403162
60. Tsui FWL, Martin A, Wang J, Tsui HW. Investigations Into the Regulation and Function of the SH2 Domain-Containing Protein-Tyrosine Phosphatase, SHP-1. Immunol Res (2006) 35:127–36. doi: 10.1385/IR:35:1:127
61. Lemm EA, Valle-Argos B, Smith LD, Richter J, Gebreselassie Y, Carter MJ, et al. Preclinical Evaluation of a Novel SHIP1 Phosphatase Activator for Inhibition of PI3K Signaling in Malignant B Cells. Clin Cancer Res (2020) 26:1700–11. doi: 10.1158/1078-0432.CCR-19-2202
Keywords: CLL, apoptosis, Bax, glycerophosphoinositol, SHP-1
Citation: Boncompagni G, Varone A, Tatangelo V, Capitani N, Frezzato F, Visentin A, Trentin L, Corda D, Baldari CT and Patrussi L (2022) Glycerophosphoinositol Promotes Apoptosis of Chronic Lymphocytic Leukemia Cells by Enhancing Bax Expression and Activation. Front. Oncol. 12:835290. doi: 10.3389/fonc.2022.835290
Received: 14 December 2021; Accepted: 28 February 2022;
Published: 22 March 2022.
Edited by:
Valeria Poli, University of Turin, ItalyReviewed by:
Giovanna Carrà, San Luigi Gonzaga University Hospital, ItalyMarta Coscia, University of Turin, Italy
Copyright © 2022 Boncompagni, Varone, Tatangelo, Capitani, Frezzato, Visentin, Trentin, Corda, Baldari and Patrussi. This is an open-access article distributed under the terms of the Creative Commons Attribution License (CC BY). The use, distribution or reproduction in other forums is permitted, provided the original author(s) and the copyright owner(s) are credited and that the original publication in this journal is cited, in accordance with accepted academic practice. No use, distribution or reproduction is permitted which does not comply with these terms.
*Correspondence: Laura Patrussi, patrussi2@unisi.it; Daniela Corda, daniela.corda@cnr.it; Cosima T. Baldari, cosima.baldari@unisi.it
†These authors share first authorship