- 1Cancer Center, The First Affiliated Hospital of Zhengzhou University, Zhengzhou, China
- 2Department of Human Anatomy, I. M. Sechenov First Moscow State Medical University of the Ministry of Health of the Russian Federation (Sechenov University), Moscow, Russia
- 3Center of Excellence in Molecular Biology and Regenerative Medicine (CEMR), Department of Biochemistry, JSS Academy of Higher Education and Research (JSS AHER), JSS Medical College, Mysuru, India
- 4Discipline of Health Sciences, College of Nursing and Health Sciences, Flinders University, Adelaide, SA, Australia
Obesity and associated chronic inflammation were shown to facilitate breast cancer (BC) growth and metastasis. Leptin, adiponectin, estrogen, and several pro-inflammatory cytokines are involved in the development of obesity-driven BC through the activation of multiple oncogenic and pro-inflammatory pathways. The aim of this study was to assess the reported mechanisms of obesity-induced breast carcinogenesis and effectiveness of conventional and complementary BC therapies. We screened published original articles, reviews, and meta-analyses that addressed the involvement of obesity-related signaling mechanisms in BC development, BC treatment/prevention approaches, and posttreatment complications. PubMed, Medline, eMedicine, National Library of Medicine (NLM), and ReleMed databases were used to retrieve relevant studies using a set of keywords, including “obesity,” “oncogenic signaling pathways,” “inflammation,” “surgery,” “radiotherapy,” “conventional therapies,” and “diet.” Multiple studies indicated that effective BC treatment requires the involvement of diet- and exercise-based approaches in obese postmenopausal women. Furthermore, active lifestyle and diet-related interventions improved the patients’ overall quality of life and minimized adverse side effects after traditional BC treatment, including postsurgical lymphedema, post-chemo nausea, vomiting, and fatigue. Further investigation of beneficial effects of diet and physical activity may help improve obesity-linked cancer therapies.
1 Introduction
The current underlying mechanisms by which obesity can induce cancer include (a) substantial amounts of growth factors such as insulin and insulin-like growth factor 1 (IGF-1); (b) enhanced levels of sex steroid hormones, primarily estrogen, and other factors affecting metabolism; (c) changes in adipokines (leptin, adiponectin, and visfatin) that are typically involved in modulating the immune, tumor-regulatory mechanisms; and (d) low-grade inflammation and cytokine factors involved in fostering oxidative stress. Furthermore, recent evidence reported that obesity-driven changes in the intestinal flora microbiome promoted cancer incidence and progression (1–4).
In obese patients, body mass index (BMI) levels are extremely enhanced, while moderately increased BMI can improve overall survival and responses to therapy (4). The protective effect of moderately increased BMI is lost when BMI reaches the morbid obesity level, which is often referred to as “obesity paradox.” The effect of morbid obesity level on the response to cancer treatment remains poorly understood (5). This phenomenon was less examined in BC and some studies applied the inadequate use of BMI for overweight patients, missing the point that muscle mass could also contribute to the BMI (6). The higher adipose tissue mass may indicate energy stores that ensure a longer time in some patients during devastating effects of chemotherapy. Enhanced adipose stores may provide an energy reserve, which enables a longer survival time (7). This study aimed to evaluate controversial cancer and obesity-related concepts, noting that obesity-induced chronic inflammation may promote cancer, whereas a low BMI can also generate an immunodeficient state and confer the immune escape of cancer cells. Furthermore, once the cancer has been diagnosed and treated in obese individuals, the pro-inflammatory state may support the immune response against the tumor (7).
In premenopausal women, ovaries are the major source of estrogen. However, the adipose tissue is the significant source of estrogen in postmenopausal women (8). Previous studies have reported that there is an increasing risk of developing estrogen-dependent BC in obese postmenopausal women. A systemic chronic inflammatory state accompanies altered metabolism in obese individuals, evidenced by the rise in both inflammatory cells and inflammatory biochemical markers (9). Furthermore, an association between obesity and triple-negative breast cancers (TNBCs) has been demonstrated (5–7). The association between obesity, cardiovascular disease, and type 2 diabetes mellitus (10, 11) is well documented in the literature, but evidence linking obesity-triggered inflammation to cancer is scarce. Therefore, it is important to clarify whether obesity-induced inflammation plays a vital role in the pathogenesis of cancer and what are the associated mechanisms of this process.
A higher BMI is also associated with higher morbidity and mortality in BC patients (12). Abnormal adipocyte hypertrophy can lead to hypoxia and initiation of inflammatory responses. Inflammatory mediators such as interleukin 6 (IL-6) and tumor necrosis factor-α (TNF-α) are known activators of the nuclear factor (NF)-κB signaling pathway, the upstream regulator of aromatase expression in adipose stromal cells in the breasts (13). Aromatase is the key enzyme of estrogen synthesis, the growth-stimulating hormone for BC cells. Obesity and its involvement in the regulation of estrogen synthesis and pro-inflammatory conditions have been shown to influence BC recurrence, distant metastasis of TNBCs, and the overall survival of patients (14, 15). Despite significant advances in our understanding and management of patients with BC, the therapeutic focus can shift toward identifying the patients at higher risk of developing resistant BC in patients with high BMI and chronic low-level inflammation. The obesity-targeting therapies may facilitate novel ways to reduce the development and progression of BC.
In this review, we identified and analyzed 86 relevant keyword-linked papers, screened out of 256 research articles that reflect the impact of obesity on BC development, treatment/prevention approaches (16–18), and posttreatment pathologies. Additionally, we summarized the relationship between BC, obesity, inflammation, and estrogen signaling network. Our discussion provides new insight on obesity-linked prophylaxis and therapeutic management of estrogen-dependent BC in obese postmenopausal women. Our analysis is also focused on the role of obesity in modulating BC occurrence and immunotherapy efficiency (16–18) in obesity-driven TNBCs. We accent complementary BC treatment strategies including dietary practices and physical activity.
2 Literature Search and Data Collection
We conducted a systematic review using the indicated keywords and limited the search to the published meta-analyses and review articles that addressed the impact of obesity on BC development, treatment/prevention approaches, and posttreatment pathologies. PubMed, Medline, eMedicine, National Library of Medicine (NLM), and ReleMed were screened to retrieve practical meta-analysis studies using keywords/phrases as follows: “obesity,” “inflammation,” “surgery,” “conventional therapies,” and “diet.” A total of 86 research papers were considered suitable and included in the final review.
2.1 Study Selection (Inclusion/Exclusion Criteria)
The selected articles addressed the obesity paradox and potential roles of obesity and chronic inflammation in BC progression. Furthermore, articles that discussed BC surgery and other conventional therapeutic modalities were also included for the analysis of information related to specific recommendations on patient management. A total of 462 papers were identified using keywords “obesity,” “inflammation,” “multiple oncogenic pathways,” “breast cancer,” “surgery,” “conventional therapies,” and “diet.” During primary screening, the actuality, publication date, access to article text, and the content were considered, resulting in the selection of 74 research papers. After removal of duplications and full-text review, a total of 86 papers were considered as suitable for final review (Flowchart). Analysis of references contributed 23 additional articles.
3 Lifestyle Factors Driving the Incidence of Breast Cancer
According to the World Health Organization (WHO), obesity, electromagnetic pollution, environmental pollution, smoking, alcohol consumption, shift work factors, and usage of excessive antiperspirants and breast implants could be major significant factors conducive to BC development (19). For instance, body weight has been playing a prominent role in modulating inflammation, serum leptin, and estrogen and adiponectin levels subsequently associated with an increased postmenopausal BC risk (20). Furthermore, it has been delineated that obesity at the time of childhood and adolescence has an inverse relation with premenopausal incidence of BC without the influence of adult BMI (21–23). A mitigated BC risk has been observed upon a weight loss of 4.5 kg during the age period of 18–30 years in women in the premenopausal period (24).
Smoking is another lifestyle factor, which has a significant influence on the development of BC, as majority of carcinogens in cigar smoke could induce polymorphism in N-acetyltransferase-2, which in turn enhances the chances of BC development (25). Alcohol consumption can enhance mammary epithelial cell growth and induce enhancement in serum estradiol levels during premenopause conditions, which consequently provoke BC development in women (26). A few reports concluded that the risk of BC development is significantly higher with every additional 10 g of ethanol consumption on a daily basis (27–29). In case of shift-based work, mainly the women who have been working on night shifts are more prone to BC development when compared to day shift workers (30–32). Antiperspirant usage is another lifestyle-driven risk factor in women that can drive the incidence of BC, as majority of deodorants are composed of parabens, which have significant estrogenic properties (33–35). In addition, aesthetic surgeries using breast implantations (for example, silicone breast implants) for a better breast augmentation could be another risk factor that can induce malignancy in breast tissue, which yet requires substantial research studies (36–39).
4 Obesity-Linked Effects on Breast Cancer Incidence, Treatment, and Prognosis
4.1 Obesity Is Associated With Activation of Multiple Oncogenic Pathways in Breast Cancers
The obesity-driven BC pathology was associated with the activation of several oncogenic pathways, including leptin signaling network and oxidative stress mechanisms. BC is marked by increased reactive oxygen species (ROS) production in epithelial mammary cells (40), although the chronically increased leptin could mitigate ROS levels in MCF-7 cells (41). Leptin can regulate metabolic reprogramming during activated cell growth, control autophagy, and inhibit BC cell apoptosis (42, 43). Leptin can modulate both tumor–stromal interactions (44) and activity of M2 type macrophages via generation of IL-18 and IL-8 that foster BC growth and metastasis (45). Leptin was also shown to enhance BC stemness via enhanced expression of stemness/epithelial–mesenchymal transition (EMT)-related genes (46). Activated leptin receptors promote several signaling cascades through the canonical pathways, such as Janus kinase 2 (JAK2)/signal transducer and activator of transcription (STAT) proteins, mitogen-activated protein kinase (MAPK/ERK1/2), phosphoinositide 3-kinase (PI3K)/V-akt murine thymoma viral oncogene homolog (AKT), and noncanonical signaling via protein kinase C (PKC), c-Jun N-terminal kinase (JNK), p38 MAPK, and AMP-activated protein kinase (AMPK) (20–25). BC progression and proliferation are regulated by leptin-mediated JAK2/STAT3 signaling in patients with obesity-like conditions (47). Leptin also regulates the cell cycle and enhances the cyclin D1 expression via the STAT3 network (48). BC proliferation can be triggered by enhanced hTERT activity and expression that are under the control of STAT3 phosphorylation (49). STAT3 signaling influences EMT and foster BC invasion and migration (50). TNBC chemoresistance and cancer stem cell proliferation were shown to be controlled by leptin-induced STAT3 (51, 52). Leptin can modulate BC proliferation via MAPK/ERK1/2 signaling, the pathway that was linked to estrogen receptor alpha (ERα) and aromatase activities (53).
In obese patients, leptin signaling correlated with the increased risk of BC (54, 55) via activation of prosurvival effectors, including PI3K/AKT, and activation of EMT (56, 57) (Figure 2). PI3K/AKT/SREBP2 signaling axis can enhance the expression of acetyl-CoA acetyltransferase 2 (ACAT2) and promote BC proliferation and migration (58). The signaling cascade that is triggered by PI3K/AKT/mammalian target of rapamycin (mTOR) pathway was shown to enhance levels of insulin, which can promote mitogenesis and growth of BCs, which often express type A insulin receptors (59–61). Insulin can enhance the production of IGF-1 and mitigate IGF-1-binding protein activation, consequently promoting tumor growth (62–65) (Figure 2). Higher IGF-1 activity and IGF-1 receptor expression were reported in different types of BCs, including TNBCs (Figure 2) (66). Insulin and estrogen signaling can coordinate therapy responses in many BCs. Natural estrogen 17β-estradiol binds ERα and promotes cancer growth in ER+ BCs (15). Previous reports indicated that gut microflora can affect IGF-1 and estrogen responses, estrogen-regulated metabolism, and estradiol production in obese postmenopausal BC patients (67). For instance, 17H-hydroxysteroid dehydrogenase 1 gene (17HSD1), which is located near the BReast CAncer susceptibility gene 1 (BRCA1) in the human genome, effectively controls the conversion of estrone to estradiol and stimulates BC growth (68, 69). Whether the activation of estrogen signaling axis is facilitated or inhibited by a low-profile inflammation and leptin-activated network in BC patients remains unclear.
4.2 Obesity and Metabolic Transformation as Triggers of Inflammation and Oncogenesis
Epidemiological studies indicated the role of obesity as a driving force of BC. The excess calories stored as triglycerides in adipocytes can lead to endoplasmic reticulum stress, adipose tissue fibrosis, and hypoxia, which result in adipocyte cell death and initiation of inflammatory immune responses (70). Especially in obese individuals, chronic inflammation is observed, marked by the formation of a distinct histological structure (“crown-like structures”) (Figure 1), associated with the infiltration and accumulation of activated M1 macrophages in adipose tissue (72). The M1 macrophages (73) are involved in the promotion of inflammation, generation of ROS, and release of pro-inflammatory cytokines (IL-6 and TNF-α) (74). TNF-α, IL-6, IL-11, leukemia inhibitory factor, and prostaglandin-E2 have been shown to activate NF-κB signaling pathway and increase the expression of aromatase in breast adipose stromal cells (13). The effect led to the suppression of apoptosis and promotion of cell cycle progression (75). The roles of mitochondria and ROS are well defined in obesity-mediated BC progression (76). The excessive energy stores in obese patients can result in mitochondrial dysfunction (77) that is associated with carcinogenic transformation and metastasis (78).
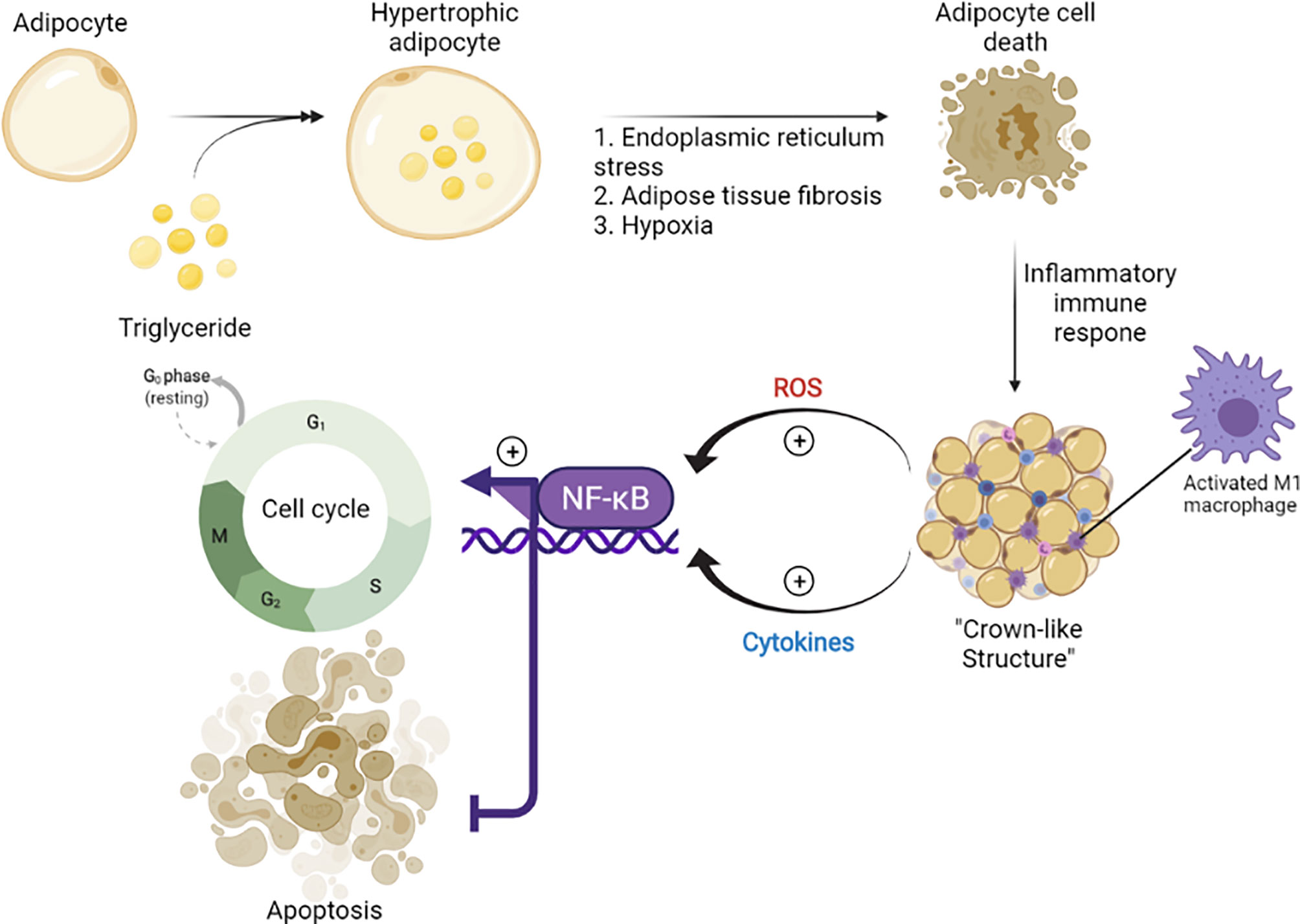
Figure 1 The role of obesity in the stimulation of carcinogenesis (71). Chronic low level of inflammation was reported in obese individuals and associated with the inflammatory immune responses initiated by the death of adipocytes. During this process, M1 macrophages are accumulated and form a crown-like structure, which provokes cell cycle progression in adipose stromal cells of the breast via secretion of inflammatory mediators (TNF-α, IL-6, IL-11) and activation of NF-κB. tumor necrosis factor-alpha, TNF-a; interleukin 6, IL-6; interleukin 11, IL-11; nuclear factor kappa-light-chain-enhancer of activated B cells, NF-kB.
Procarcinogenic effects of estrogen were registered in vivo using large doses of natural and synthetic estrogens in experimental animals (79). The adipocytes synthesize substantial levels of estrogen, which can also cause ER+ BC progression (80). However, estrogen, as an important steroid hormone, is essential for normal functioning of endocrine-responsive tissues. Estrogen is used as an oral contraceptive and prescribed as treatment for osteoporosis, cardiovascular diseases, atrophic vaginitis, hypertension, and stroke in postmenopausal women. Estrogen can increase the levels of apolipoproteins A-I and high-density lipoproteins (HDLs) (“good cholesterol”), while the level of low-density lipoproteins (LDLs) (“bad cholesterol”) can be decreased by this steroid hormone (81).
However, the precise biological mechanism of estrogen-induced cancer progression and spreading remains unclear. One of the cancer-promoting mechanisms is associated with transactivation of growth factor receptors. Epidermal growth factor receptor (EGFR) transactivation by estrogen is an important signaling mechanism in the regulation of cancer cell growth (82). Several hypotheses were also reported that described ER signaling as a trigger for precancer cell transformation into malignant cells and the shift of dormant cells from G0 to G1-S active phase (Figure 1). This process was referred to as “estrogenic recruitment.” During this activation, the synthesis of growth factors could promote tumor growth and pro-oncogenic signaling, increase the formation of free radicals, inhibit apoptosis in transformed cells, and stimulate the synthesis of cathepsin D and plasminogen activator factor (PAF) required for BC progression (79).
It has been observed that 5′-AMPK, liver kinase B1 (LKB1), and the metabolic sensors are crucial modulators of ATP synthesis and energy utilization (83). The LKB1/AMPK axis inhibits nuclear entry of tCREB-coactivator (cAMP response element-binding protein) and CRTC2 (CREB-regulated transcription cofactor 2). These factors ultimately lead to the inhibition of aromatase expression in the isolated adipose stromal cells (84) and may significantly impact BC progression. This mechanism may be explored and utilized for therapeutic interventions in obese BC patients.
4.3 Obesity and ER+ Breast Cancer in Postmenopausal Women
The positive association between obesity and the risk of ER+ BC in postmenopausal woman has been reported (85). Interestingly, a higher BMI in young women (at the age of 18 years) correlated with the lowest BC rate before and after menopause (65). The weight gain after 18 years of age in women was not related to the incidence of BC before menopause, while it was positively linked to the higher risk of BC after menopause (86). The mitigation of body fat levels was positively associated with decreased circulating estrogen and inversely associated with the risk of estrogen-dependent BC in postmenopausal women (87, 88). Several studies demonstrated that weight loss is associated with the decrease in serum C-reactive protein (CRP) (89–91), IL-6 (91), and serum amyloid A (92) in postmenopausal women. Since most (nearly 80%) BCs in postmenopausal women are estrogen-dependent, prospective therapeutic interventions should focus on reducing chronic inflammation, fat-linked estrogen production, and weight loss. Another study by Tchernof et al. (93) suggested calorie restriction and the intake of 1,200 kcal a day for an average of 13.9 months to reduce the level of circulating estrogens. This approach resulted in an average weight loss of 14.5 kg and serum estradiol reduction of 21.7 pg/ml (93).
The high level of BMI significantly impacts BC prognosis. A higher production of peripheral estrogen in adipose tissues correlated with the higher BMI in postmenopausal patients. A minimal level of sex hormone-binding globulin was observed in patients with a high BMI and could be responsible for poor BC prognosis. The substantial increase in aromatase activity and estrogen synthesis was also linked to uncontrolled proliferation of BC cells (94, 95). Therefore, an elevated BMI may negatively impact BC patients receiving aromatase inhibitors (96). However, older BC patients with higher BMI may benefit and better withstand chemotherapy and the associated adverse toxicity (97). It is unclear whether all BC patients with a high BMI acquire higher insulin, IGF, and steroid hormone levels that could provoke a substantial mitogenic activity (98). Notably, cytokines generated in the adipose tissue of obese individuals may enhance BC progression through the upregulation of stem cell signaling, metastasis, and angiogenesis (99, 100). Several studies reported that premenopausal and perimenopausal BC patients with a higher BMI (estimated as obesity or overweight) indicated poorer prognosis regardless of the tumor subtype (101–104). A higher BMI ≥25.8 kg/m2 also resulted in higher mortality in premenopausal patients (71, 83).
4.4 Clinical Reports of Therapies Targeting Oncogenic Pathways in Breast Cancers
BC can be categorized into 4 subtypes according to molecular markers and mammary epithelial biology (105). The most common molecular markers used for disease prognosis and methods of treatment (106) are presented in Table 1. BCs expressing hormone receptors, i.e., ER (ER+) or progesterone receptor (PR+), are classified as luminal A and luminal B BC types (107). Erb-B2 receptor tyrosine kinase 2 (ERBB2+/HER2+) cancers are characterized by human epidermal growth factor receptor 2 (HER2) gene amplification (109). Even though estrogen has been proven as a potent mitogen in ER+ BCs, it has been found that patients with this type of malignancy have a relatively better prognosis (111). Table 2 presents epidemiological BC data [2014–2018 described by the National Institutes of Health (NIH)] and summarizes information about the four BC subtypes.
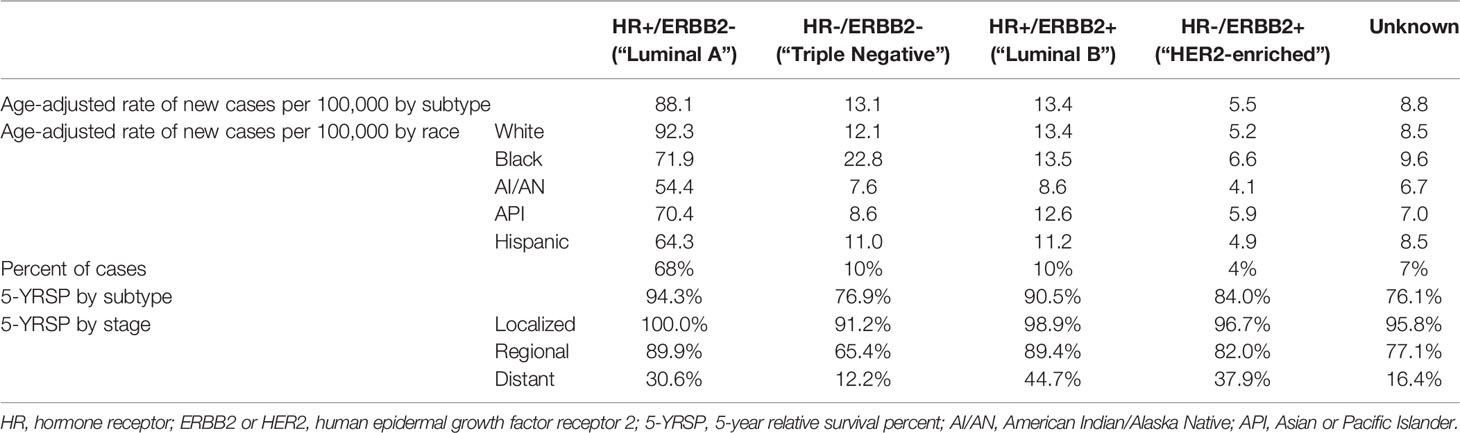
Table 2 Statistics based on 2014–2018 cases according to the National Institutes of Health (112).
Hormone replacement therapy (HRT) produced a favorable clinical outcome against luminal A ER+ tumors (111). HRT is considered as the best option for patients with highly endocrine-responsive tumors, while cell cycle targeting (cytotoxic) chemotherapy is often used with non-endocrine-responsive tumors. A combinatorial regimen of cytotoxic drugs (113) along with endocrine therapy is defined according to the tumor size, grade, presence of peritumoral vascular invasion, and nodal status. Ki67 is one of the crucial parameters that is used during the decision about chemotherapy approach. A combinatorial regimen with HRT is often prescribed for the treatment of obese BC patients with Luminal-A molecular subtypes (114). The administration of trastuzumab can be beneficial in patients with HER2+ BCs with more than 10% invasive tumor cells or showing HER2 gene amplification (115). Additionally, conventional TNBC therapy involves combined HRT (116) and immunotherapy agents (117). However, application of these therapeutic agents was associated with the development of multidrug resistance (MDR) in obese/overweight patients (117). Several other effective chemotherapeutic drugs, including antitubulin agents, platinum agents, EGFR inhibitors, antiangiogenic agents, androgen receptor (AR) antagonists, histone deacetylase (HDAC) inhibitors, PI3K/AKT/mTOR pathway inhibitors, MAPK/MEK modulators, and checkpoint kinase 1 (Chk-1) inhibitors, were shown to target oncogenic signaling pathways (Figure 2) (118–121). Neoadjuvant chemotherapy with these agents (122–124) is a multicomponent therapeutic regimen used in advanced BC (125, 126).
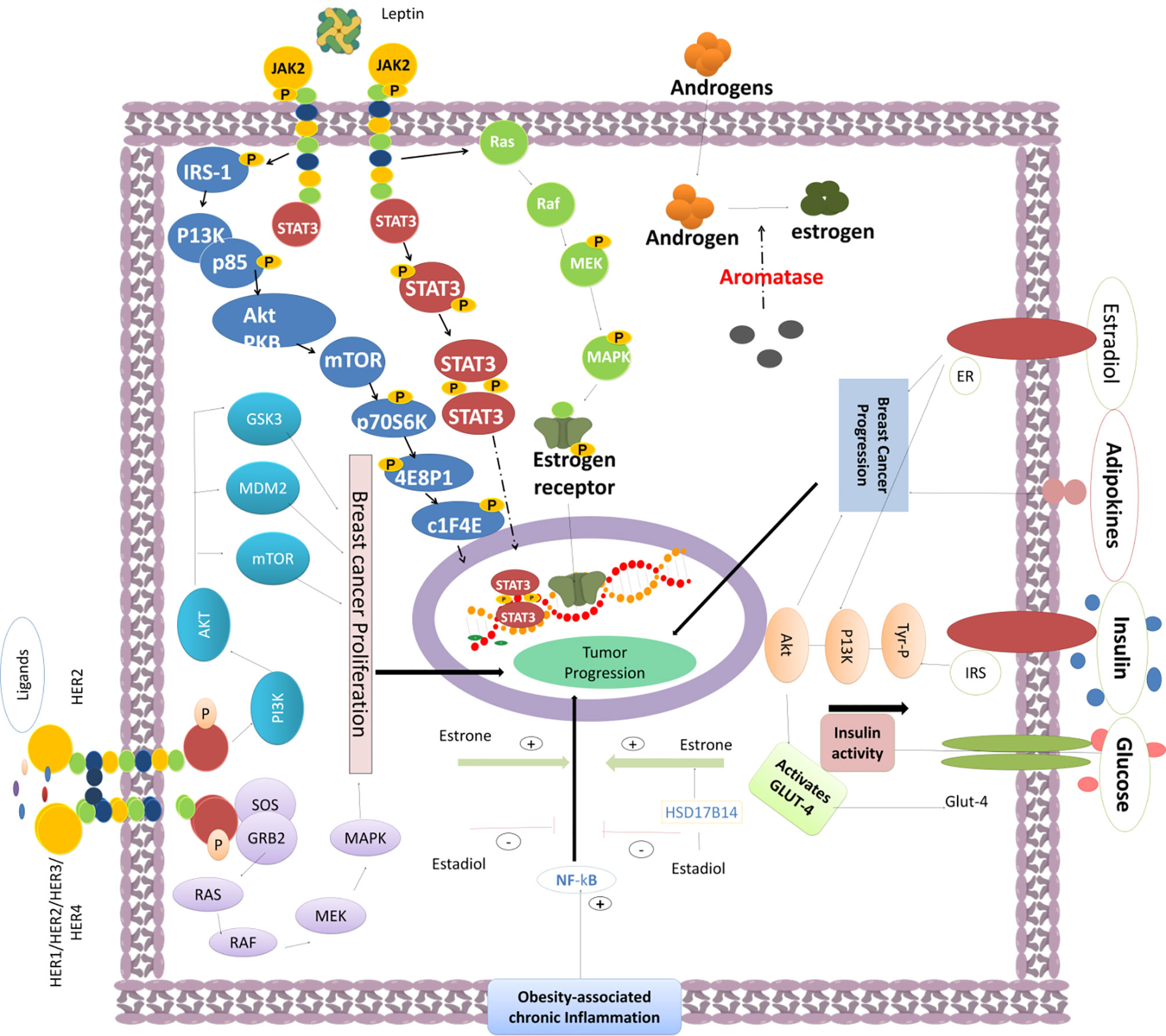
Figure 2 The activity of various oncogenic pathways triggers BC epithelial cell proliferation and tumor progression. Obesity-induced chronic inflammation can promote the NF-κB signaling. Fat cell-produced estrone can enhance the activation of NF-κB and promote BC progression. Leptin can modulate the PI3K/AKT signaling through IRS-1, enhance mTOR activity, trigger 4EBP1 and elF4E phosphorylation, and mediate the STAT3-promoted gene transcription required for cancer cell growth. Furthermore, the JAK-STAT and MAPK pathways are enhanced by the leptin-induced JAK2 signaling. MEK phosphorylation strongly promotes gene expression for efficient tumor progression. Aromatase activity promotes the androgen to estrogen conversion required for tumor progression. Other signaling effectors such as insulin, estradiol, adipokines, and high glucose can also enhance BC progression. Insulin can promote IRS and Tyr-P signaling and induce the activation of PI3K/AKT via stimulation of GLUT-4, which consequently enhances the glucose uptake into BC cells. Several HER1/HER2/HER3/HER4 ligands can enhance the activity of HER2, promote the tyrosine kinase domain phosphorylation, and induce BC progression through the signaling cascade mediated through the PI3K/AKT/mTOR and RAS-RAF-MEK-MAPK pathways to foster cell growth and proliferation.
4.4.1 Obesity in Patients With Triple-Negative Breast Cancers and the Effectiveness of Chemotherapy
The first generation of chemotherapy, for example, cyclophosphamide, methotrexate, 5-fluorouracil (5-FU), can induce 35% reduction in BC mortality when compared to adjuvant chemotherapy (127). However, the adverse effects of chemotherapy (128–133), such as alopecia, fatigue, nausea, vomiting, myelosuppression, acute leukemia, cardiac toxicity, and neuropathy (134), should also be considered. Adjuvant systemic chemotherapy was shown to induce less adverse side effects while delivering sufficiently decreased metastasis and BC mortality rate (135). However, the development of resistance to chemotherapy was observed in nearly 40% of BC patients during the first 5 years of treatment. TNBCs belong to the advanced type of malignancies that demonstrate lower rates of chemotherapy response and higher rates of resistance. TNBC patients are diagnosed with a relatively larger tumor size and higher T stage and tumor grade. TNBCs are often detected in obese patients. Previous reports demonstrated that a substantial portion of obese/overweight patients is diagnosed with TNBC (136). Another retrospective study reported that the incidence of TNBC in obese individuals is significantly higher than that in non-obese TNBC patients (137).
HR-negative (HR-) BCs (including TNBC) exhibit a higher disease recurrence rate compared to HR-positive (HR+) BCs (138). AR antagonists (such as nonsteroidal anti-androgen bicalutamides) demonstrated good clinical efficacy in Phase II single-arm trials in TNBC patients harboring AR-positive tumors (139). However, the clinical efficacy of AR antagonists is still debatable and/or controversial. The modest pharmacological activity of bicalutamides may be associated with the indolent nature of the luminal disease.
The recurrence rate could be decreased by the application of adjuvant chemotherapy prior to surgical intervention with good clinical outcomes if administered before (neoadjuvant) or after (adjuvant) surgery (140, 141). Breast-conserving surgery is commonly followed by the administration of systemic therapeutic regimens to enhance clinical responses (neoadjuvant therapy). However, in obese TNBC patients, the risk of relapse is high (120–123). The clinical trial design should target overweight/obese patients as a separate cohort and test novel therapeutic agents mainly against TNBCs in obese individuals (142). TNBCs often express EGFR, and preclinical research demonstrated that bilateral BCs could be treated using anti-EGF therapeutic agents, including EGFR inhibitors (141, 143). The combined EGFR inhibitor therapy (cetuximab with platinum) was evaluated for its effectiveness in clinical trials (144). However, more studies are warranted to address chemotherapy responses in obese BC cohorts.
4.4.2 Histone Deacetylase Inhibitors
Histone deacetylase (HDAC) inhibitors were used to target epigenetic regulation by HDAC, the enzyme involved in chromatin modeling and gene transcription in TNBCs. HDAC inhibitors modulate the acetylation and deacetylation of core histones, thereby reprogramming the expression of various genes involved in controlling cell proliferation, survival, metastasis, and angiogenesis (145, 146). HDAC inhibitors were tested against TNBC in preclinical models. For instance, the combination of vorinostat with neoadjuvant therapeutic regimens of carboplatin and nab-paclitaxel has not delivered improved pathological complete response (pCR) rates in TNBC patients during randomized Phase II trials. HDAC inhibitors provoked genome-wide effects by enhancing the reexpression of ER or BRCA1/2, which is accompanied by the silencing of other tumor-suppressive genes (147). Hence, the identification of new classes of HDAC inhibitors to control the functions of tumor-promoting genesis in obese TNBC patients is warranted (Figure 3).
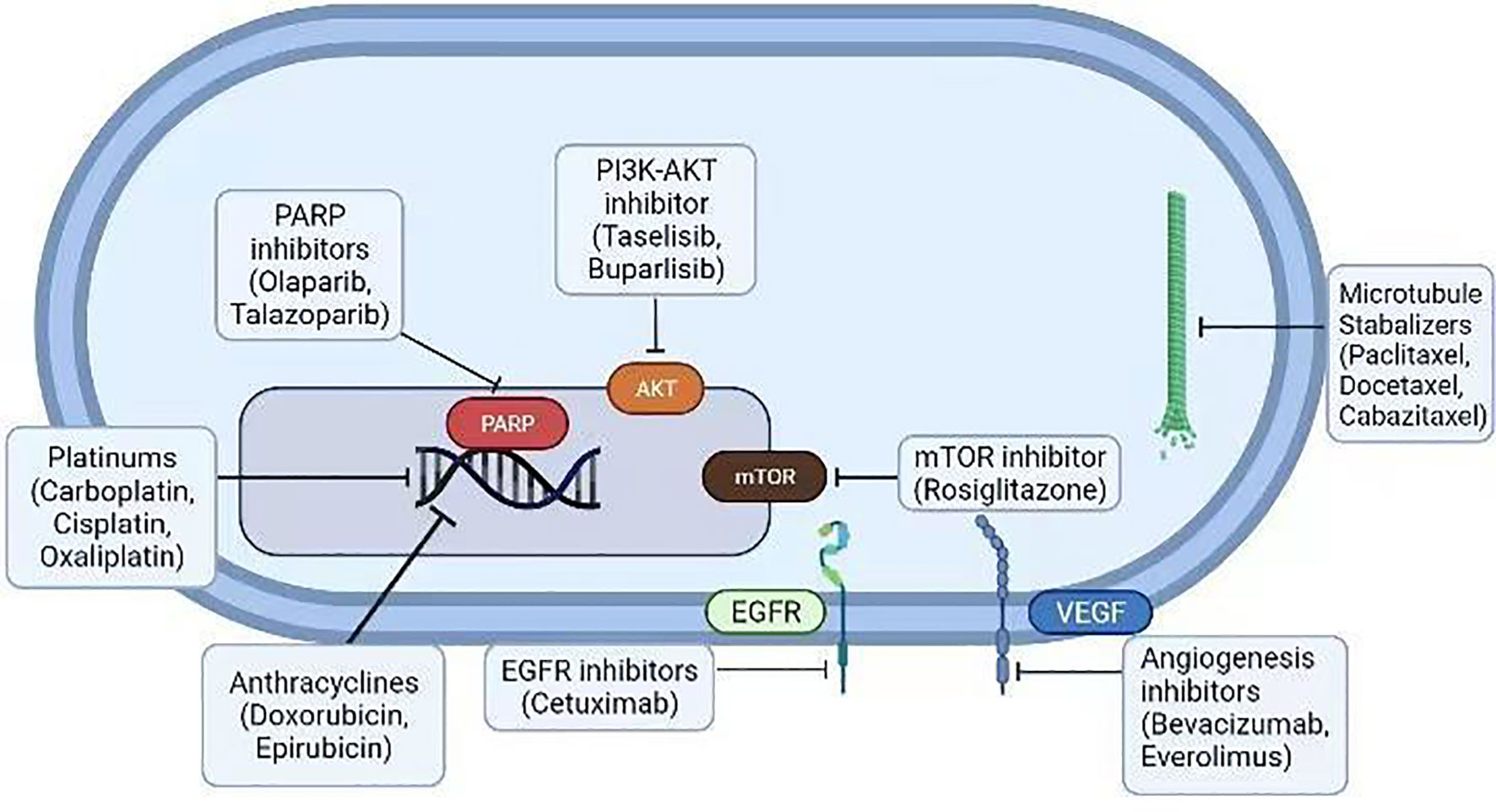
Figure 3 Various conventional therapeutic agents (platinum drugs, anthracyclines, EGFR blockers, mTOR blockers, angiogenesis inhibitors, microtubule stabilizers, and PARP and PI3K-Akt inhibitors) were examined against the BCs. Many of them are FDA-approved drugs for BC therapy.
MAPK kinase/MEK pathway activation in the TNBC is mediated by the upstream receptor tyrosine kinases (RTKs) (148). Impairment of MEK could induce compensatory mechanisms and trigger a variety of RTKs in TNBCs (148). Molecular signaling that involves PI3K/AKT activation was reported in several malignancies including BRCA-associated BCs in obese patients (149, 150). The assessment of clinical efficacy of MEK inhibitors (for example, GSK1120212 or trametinib) and PI3K/AKT inhibitors against TNBC and other devastating malignancies is currently being addressed. Several trials aim to characterize the antitumor effects of these combinatorial regimens against both basal-like BCs and mesenchymal subtypes of TNBC (151, 152).
Immune Chk inhibitors are reported to be highly efficient against TNBC harboring p53 mutations (153). Cancer cells require Chk-1 to modulate the cell cycle. Previous studies used p-53-deficient mouse models of BC and showed the induction of apoptosis upon Chk-1 inhibition (153, 154). Immune modulation with Chk-1 inhibitors is a promising BC treatment strategy in obese individuals with aggressive cancer biology. Immunotherapy agents (blocking or activating specific targets), including PD-1 or CTLA-4 blockers (155), were approved for antitumor evaluation in clinical studies. The immunotherapy strategies that target tumor-infiltrating lymphocytes warrant testing in TNBC patients (155).
4.4.3 Poly ADP-Ribose Polymerase Inhibitors Against Triple-Negative Breast Cancer
The poly ADP-ribose polymerase (PARP) family includes enzymes responsible for DNA repair. DNA damage activates nuclear PARPs that are required for DNA repair in BC cells (156). PARP activation contributes to the recruitment of base excision repair (BER) to the site of DNA damage and fosters chromatic relaxation to induce DNA repair (157–159). Hence, PARP inhibitors could induce synthetic lethality when administered in combination to target TNBCs in obese individuals. Successful TNBC cell survival and proliferation depend on DNA repair pathways and other homologous recombination repair (HRR) mechanisms. Olaparib is a PARP inhibitor primarily examined as a choice of chemotherapy against germline BRCA mutated (gBRCAm) HER2-negative (HER2-) BCs (142). This drug invoked radiological responses in 60% of patients compared to the 29% of the group receiving chemotherapy alone. Furthermore, TNBC progression was delayed from 4.2 to 7.0 months by the PARP inhibitor treatment. The clinical efficacy of PARP inhibitor combinatorial regimen (carboplatin with veliparib to Adriamycin®) was ascertained in comparison to cyclophosphamide (AC)-paclitaxel or AC-paclitaxel with carboplatin (160). The observed effects supported the addition of carboplatin that improved the clinical benefit of veliparib, although further testing is required (161). Synthetic lethality was a more profound clinical effect observed during PARP inhibition. PARP1 enzymatic inhibition could foster the formation of unrepaired single-strand DNA breaks, which consequently induce cell death in cancer cells defective in homologous recombination DNA repair (HRR) mechanisms. Thus, the molecular signaling of PARP could be impaired by targeted inhibition, producing potent cytotoxic effects in BC cells. Accordingly, the administration of olaparib, a strong PARP-trapping agent (162, 163), was evaluated in combination with platinum drugs in OlympiA PARTNER neoadjuvant clinical study [ClinicalTrials.gov] (142). Talazoparib is another PARP inhibitor that exhibits PARP-trapping activity and catalytic efficacy. The agent produced a significant clinical response when used as neoadjuvant monotherapy in gBRCAm patients (164). In another clinical Phase II study, patients who were diagnosed with gBRCAm, TNBC, and HR+/HER2- were administered PARP inhibitor monotherapy for 6 months prior to surgical intervention. The treatment resulted in a 59% rate of residual cancer burden (RCB)-0/I (142). Considering the success of these regimens, the approach should be examined for clinical outcomes in obese/overweight BC patients with different BC molecular subtypes.
4.4.4 Microtubule Stabilizers Against Triple-Negative Breast Cancer
Mammalian‐enabled (MENA) protein is an invasive form of microtubule protein that promotes BC cell proliferation and metastasis. MENA is an Ena/VASP family protein and is the most common elongation factor involved in fostering the cancer cell division including BC (159). To combat BC and improve clinical outcomes, microtubule-targeting therapy in obese patients with TNBC warrants considerations and testing (159). Taxane derivatives, including paclitaxel, docetaxel, and cabazitaxel, are potent tubulin-polymerizing agents that induce microtubule stabilization. They are more effective against TNBCs than ER+/PR+ BCs (165, 166). For instance, obese patients with TNBCs who have been receiving uncapped doses of taxane derivatives as neoadjuvant chemotherapy demonstrated higher pCR followed by good progression-free survival (PFS) (167).
The administration of paclitaxel for 8 weeks, as neoadjuvant after 4 treatment cycles of 5-FU, epirubicin, and cyclophosphamide, was accompanied by substantial enhancement in the clinical outcomes in TNBC patients (165). Ixabepilone is another microtubule stabilizer that is actively used for the treatment of TNBC patients (168, 169). However, the administration of this drug could be bypassed by chemoresistance mechanisms exerted by cancer cells. Therefore, ixabepilone is not as efficient as other anthracyclines and taxanes. Metastatic TNBC, treated with taxane/anthracycline, was shown to develop resistance, although combinatorial regimen with ixabepilone and capecitabine (a prodrug of 5-FU) delivered improved PFS compared to capecitabine alone (169). This combinatorial regimen could be a preferred therapeutic option for BC patients exhibiting cisplatin chemoresistance. Taxane-based chemotherapy is the recommended adjuvant regimen and one of the standard of care for patients with high-risk/high-grade/node-positive BCs, including TNBCs, HER2+, and HR+/HER2- cancers (170). Several studies and meta-analyses reported that the postsurgical anthracycline–taxane treatment was associated with enhanced relapse-free survival and better overall survival compared to the monotherapy of anthracycline alone (171, 172). The GeparTrio clinical study showed that patients who received 8 cycles of neoadjuvant TAC (docetaxel, doxorubicin, cyclophosphamide) combinatorial therapy displayed 37% of pCR rate in a TNBC cohort (173). However, the choice and schedule of soluble taxane derivatives remain to be assessed for clinical efficacy against TNBCs (166, 174). Albumin-bound paclitaxel (nab-paclitaxel) exhibited antitumor efficacy as neoadjuvant treatment in BC patients. The GeparSepto trial reported 38% increases in pCR rates with sequential combinatorial regimen (epirubicin, cyclophosphamide, and nab-paclitaxel) compared to the pCR rate displayed by the soluble paclitaxel alone given every week continuously for 12 weeks (175). Nab-paclitaxel was reported to induce lower anaphylaxis and hypersensitivity rates. ETNA clinical trials have reported better pCR with nab-paclitaxel (22.5%) compared to that with paclitaxel alone (pCR rate was 18.6%). However, the clinical use of taxane is limited due to the hypersensitivity observed in TNBC patients.
4.4.5 Anthracyclines Against Triple-Negative Breast Cancers
Anthracycline derivatives are one of the significant chemotherapies used to treat TNBCs and other BCs by targeting DNA topoisomerase II (176). Doxorubicin and epirubicin are preferred neoadjuvant and adjuvant therapeutic agents in obese individuals with BCs. TNBCs exhibit chemosensitivity to anthracycline derivatives, although their clinical efficacies are controversial (177, 178). A retrospective study reported a 22% pCR rate with an epirubicin-containing regimen in TNBC patients, which was an increased rate compared to that in non-TNBC patients who exhibited an 11% pCR rate with the same regimen (177). TNBC patients demonstrated substantially improved clinical responses to doxorubicin and cyclophosphamide therapy compared to non-TNBC patients (178). Furthermore, a specific clinical benefit was observed with anthracycline and taxane combinations in node-positive TNBCs, although the efficacy of anthracyclines was not confirmed (179).
The WSG AM-01 trial indicated a clinical benefit of adjuvant anthracycline therapeutic modality in TNBC patients. The study compared a group of patients who received dose-dense conventional chemotherapy with another group that received a rapidly cycled high-dose regimen. The results indicated a 5-year event-free survival (EFS) rate equal to 71% in TNBC patients compared to 26% EFS for those who received the conventional dose-dense chemotherapy (180). However, the clinical efficacy of anthracycline-based chemotherapy remains controversial despite high response rates to anthracyclines alone as neoadjuvant treatment (181). The main reason for this controversy may be explained by the heterogeneous nature of TNBCs. Moreover, it is still unclear in terms of anthracycline sensitivity whether BRCA1-associated TNBC is functionally similar to sporadic disease (180, 182).
4.4.6 Platinum Derivatives Against Triple-Negative Breast Cancer
Platinum-bound agents, including carboplatin, cisplatin, and oxaliplatin, can induce DNA double-strand breaks (DSBs). The agents facilitate DNA adduct formation, which consequently blocks DNA replication (183, 184). Furthermore, the platinum drugs are reported to be active against TNBC subtypes in obese individuals. The platinum-based drugs were shown to be highly effective regimens in obese individuals with BCs (185). The platinum-containing regimens induced a modest PFS improvement in metastatic TNBC but little to no effect on PFS or overall survival in non-metastatic BCs (185). Carboplatin was reported to be more effective in unselected metastatic TNBC when compared to docetaxel in first-line treatment of metastatic TNBC cases as reported in TNBC trials (186). Platinum derivatives could be considered as effective chemotherapeutic agents in patients with metastatic BCs. Clinical responses were observed in gBRCAm carriers but not in the epigenetic-driven BRCA carriers with TNBCs. A retrospective study of a large cohort of metastatic TNBC indicated the clinical efficacy of platinum-based drugs as first-line therapy with improved PFS compared to the patients without platinum therapy (187). The GeparSixto trial tested the effect of carboplatin that was added to paclitaxel and liposomal doxorubicin on a weekly basis for a continuous 18 weeks (preoperative period) in HR- BCs (188). In case of TNBC, the pCR rate was higher (57%) and patients exhibited a superior recurrence-free survival with carboplatin addition (189, 190). Thus, the addition of carboplatin to neoadjuvant therapy significantly enhanced pCR rates, but the survival-related data are currently unequivocal.
Cyclophosphamide is another anticancer drug used in TNBC therapy. This therapeutic molecule regulates alkylation and can induce cytotoxic effects by promoting the formation of DSB-mediated DNA interstrand cross-links. The GeparSixto study described that carboplatin and high-dose cyclophosphamide may be replaced by the combinatorial regimen of taxanes and anthracyclines and displayed similar pCR rates of 48.3% in intense dose-dense epirubicin, paclitaxel, and cyclophosphamide (iddEPC) arm and 48% in non-pegylated liposomal doxorubicin + carboplatin in TNBC (PMCb) arm (191). However, the administration of carboplatin has not been referred to as the standard of care (neoadjuvant therapy) against TNBC. The usage of this agent is still constrained by several adverse effects (neutropenia, anemia, and thrombocytopenia). It is essential to carefully define the patient subgroup that may benefit from this treatment approach and have a lower number of adverse complications. Moreover, in patients who were prescribed neoadjuvant therapy with platinum derivatives, the absence of BRCA is reported to be a good predictive biomarker (192).
4.4.7 Antiangiogenesis Agents Against Triple-Negative Breast Cancer
Angiogenesis promotes tumorigenesis and is regulated by cancer cells. A higher tumor microvessel density is always observed in TNBC compared to other BC subtypes (193). Vascular endothelial growth factor (VEGF) is a crucial regulator of angiogenesis in TNBCs (190). For instance, bevacizumab is a monoclonal antibody that induces VEGF blockade. Bevacizumab was initially assessed in metastatic TNBC and displayed good PFS when examined in Phase II studies (194). The rationale for the assessment of antiangiogenic agents against TNBC in neoadjuvant settings includes the fact that cancer invasion and metastasis need neovascularization. Enhanced tumor cell escape from the original tumor is followed by intravasation into the circulation (195, 196). The GeparQuinto clinical Phase III neoadjuvant study reported the improved pCR rates from 33% to 43% with the addition of bevacizumab to anthracycline–taxane combinatorial regimen (197). The UK-led Phase III clinical study (ARTEMIS) also delineated the administration of 4 cycles of bevacizumab to the above combinatorial regimen. The resulting combined pCR rate for both HR+ and HR- types was 22% with bevacizumab and 17% for chemotherapy alone (198). However, bevacizumab did not display substantial clinical efficacy to mitigate distant recurrence, and the agent is not recommended for regular usage in neoadjuvant-treated TNBC. Several other antiangiogenic inhibitors, sunitinib and pazopanib, are currently under clinical investigation as neoadjuvant therapies against TNBCs.
4.4.8 Neoadjuvant Immunotherapies: Successes and Failures
Tumor heterogeneity provides a background for cancer adaptability and failure of several neoadjuvant and adjuvant therapies. Majority of BCs acquire drug resistance through selective enrichment of residual (resistant) tumor cells within the tumor microenvironment (TME) (199, 200). TNBCs are surrounded by divergent TME. Many cytotoxic agents were reported to transform EMT responses, accompanied by the invasion, and metastasis (199). Other agents were shown to reverse EMT and impair metastasis (201, 202).
Neoadjuvant immunotherapies improve the pCR rate with a favorable prognosis, but the prognosis for TNBC patients with residual cancer is variable and less promising in obese individuals (203, 204). The 5-year recurrence rate is predominantly higher for patients with extensive residual disease than that in patients with minimal residual disease after neoadjuvant therapy (205). Additionally, favorable clinical outcomes for continuing systemic therapy in extensive residual TNBC patients were not reported for patients who are at higher recurrence risk despite receiving taxane- and anthracycline-containing neoadjuvant therapy. The tumor-specific ctDNA (circulating DNA) in the plasma of TNBC patients could be used as a marker for the identification of minimal residual disease following neoadjuvant chemotherapy and enables tracking of early therapeutic intervention to clear the ctDNA (142). For instance, the identification of ctDNA in the plasma of these patients would benefit clinicians to choose specific immunotherapy with pembrolizumab. Monitoring of patients with ctDNA for metastasis was recommended (142). Furthermore, the immune-oncology therapeutic strategies are gaining attention as a promising treatment for TNBCs. Application of checkpoint inhibitors with neoadjuvant chemotherapy in the I-SPY2 trials altered the treatment paradigms that promised to attain good clinical outcomes. However, the immune-related adverse toxicities require further testing. There is a need for the development of neoadjuvant therapies against TNBC type 4 subgroups to enhance the maximal clinical response (142).
The failure to attain effective pCR rates with several neoadjuvant therapeutic agents is associated with MDR that is mediated through several cell signaling pathways, including G protein-coupled receptor (GPCR)/RAS/RAF/MAPK, IL6-JAK/STAT3/mTOR, and HER2/PI3K-AKT signaling networks (206). Other signaling pathways that promoted drug-resistant BCs activated the expression of transporter gene ABCG2, survival-linked genes STAT3 and HER2, transcription factors (AP-1 and NF-κB), and epigenetic factors HDACs (207). The raised IL-6 and IL-8 secretion and STAT3 activity are crucial for the development of MDR in BC cells, limiting the pharmacological activity of chemotherapeutic drugs (208–210).
4.4.9 Neoadjuvant Endocrine Immunotherapies and Relevant Clinical Trials
A plethora of clinical reports indicated that neoadjuvant therapy can generate a similar overall and disease-free survival rate observed during adjuvant therapy in ER-BC patients with higher rates of breast-conserving surgery (211–214). ER+ BCs did not demonstrate effective clinical responses after the administration of neoadjuvant therapeutic molecules and hence need the administration of robust alternatives in both normal and obese individuals (213, 214). Among these alternatives, the addition of neoadjuvant endocrine therapy is one of the significant options of chemotherapy to combat TNBCs. Major types of neoadjuvant endocrine therapy include the application of an ER antagonist (tamoxifen), a selective ER modulator (fulvestrant), and aromatase inhibitors (letrozole and anastrozole). All those agents were shown to block estrogen/ER signaling, ER expression, and/or estrogen synthesis (215). These drugs are recommended for patients who are not suitable for surgical interventions. The optimal duration of the therapeutic regimen is hard to define for all patients compared to neoadjuvant chemotherapy alone (215).
Adjuvant endocrine therapy is a combination of endocrine therapy with chemotherapy drugs that have already shown effectiveness in a metastatic situation to overcome BC endocrine resistance (216). This approach provides a good therapeutic perspective for obese patients with TNBCs. After the introduction of everolimus in the treatment of patients with metastatic BC (217), the adjuvant studies were subsequently started (e.g., NCT01674140, NCT01805271 www.clinicaltrial.gov). Everolimus was substantially evaluated as the adjuvant therapy agent in two clinical studies, i.e., SWOG1207 (NCT01674140). The trial is designed to randomly assign everolimus or placebo to high-risk premenopausal and postmenopausal patients who are on their standard adjuvant chemotherapy. Another clinical study (NCT01805271) evaluated the addition of everolimus to adjuvant endocrine therapy in high-risk ER+/HER2- BC patients. The study demonstrated an improved disease-free survival after 1 year of treatment.
Another option is to use a combination of CDK4/6 inhibitors that are less toxic. Dowsett et al. (218) described the effect of palbociclib along with 3 months of neoadjuvant endocrine therapy with letrozole. Results of this study have concluded the substantial palbociclib-mediated antiproliferative effect against BC. The percentage of tumors that underwent a complete cell cycle arrest, indicated in the form of a Ki-67 value <2.7%, during neoadjuvant therapy was reported to be enhanced from 58.5% to 90.4% by the addition of palbociclib (218). Novel effective combination therapies, adjuvant therapy studies at neoadjuvant settings must be conducted for all CDK4/6 inhibitors against TNBCs (219).
Ribociclib is a CDK4/6 inhibitor reported to mitigate recurrence in patients with HR+ early BC when it is preferred in combination with standard adjuvant endocrine therapy (NCT03078751) (220). Ribociclib administration demonstrated an effective clinical efficacy with limited toxicity when combined with ET in patients with HR+, HER2- advanced BC. The purpose of this study was to evaluate the preliminary safety and tolerability of ribociclib to standard adjuvant ET in patients with HR+, HER2- high-risk BCs [https://clinicaltrials.gov]. Application of these molecules is preferred for the treatment of normal-weight and obese BC patients.
4.4.10 Radiation Therapy
Our search identified a total of 36 trials’ clinical data that compared the efficacy of radiotherapy to surgery in BC patients. Majority of the trials indicated that additional radiation therapy can strongly decrease the rate of local recurrence. Radiotherapy after surgery is three times more effective than surgery alone. However, the 10-year survival rate analysis for both methods did not show a significant difference (221). The assessment did not compare the treatment outcomes in obese vs. normal-weight BC patients.
4.4.11 Aromatase Inhibitors
Aromatase is the main enzyme responsible for estrogen biosynthesis in postmenopausal women (222). Aromatase is found in adipose tissue of both normal breast and tumor tissues (223). The microsomal enzyme aromatase is encoded by human CYP19 single-copy gene located on chromosome 15p21.2, which can catalyze the conversion of testosterone and androstenedione to 17-beta-estradiol and estrone (224). Aromatase inhibitors are reported to reduce the circulating estrogen level by blocking the aromatase enzyme activity. However, they are found to be effective only in postmenopausal women. The inhibitors provide time-limited effects, as estrogen levels eventually return to normal once aromatase inhibitors are withdrawn as a BC therapeutic regimen (225). Two types of aromatase inhibitors were designed: steroidal and nonsteroidal agents. Exemestane is a third-generation steroidal aromatase inhibitor and can be administered as an adjuvant therapy in postmenopausal women with early-stage or advanced BCs (226). Everolimus, an immunosuppressant, can be coadministered (combinatorial regimen) with exemestane. This approach greatly enhanced the PFS compared to the exemestane BC monotherapy alone (227).
4.4.12 Application of Estrogen Receptor Antagonists
ERs are activated by estradiol to foster cell differentiation and proliferation in BCs (228). Tamoxifen citrate forces BC to regress, acting as a competitive ER antagonist. The administration of tamoxifen in certain BC patients can reduce the need for oophorectomy, hypophysectomy, or adrenalectomy (229, 230). According to numerous reports, tamoxifen citrate arrests cancer cell growth, induces apoptosis, and modulates the activation of many proliferation- and survival-related signaling proteins (such as calmodulin, protein kinase C, protooncogene c-myc, and transforming growth factor-beta) (231). However, prolonged treatment with tamoxifen results in the development of resistance (232).
4.4.12 Targets for HER2+ Metastatic Breast Cancers
HER2 gene amplification and overexpression were found in approximately 20%–25% of BCs (233). HER2 activates several downstream pathways required for the proliferation of cancer cells (234). Therefore, HER2 inhibitors are used for HER2+ BC treatment. For instance, trastuzumab is produced as a recombinant humanized monoclonal antibody and specifically used against BCs overexpressing HER2 (235). It has been confirmed that trastuzumab improves the clinical benefits when combined with other chemotherapy agents in HER2+ metastatic BCs (236). There are also other novel options including gene therapy, oncogene inactivation, augmentation of tumor suppresser genes, cell-target suicide, chemoprotection approach, virus-mediated oncolysis, and immunomodulation (237), which require intensive clinical testing.
4.5 Surgical Interventions
4.5.1 Breast Surgery
This includes mastectomy and breast-conserving surgery that consists of lumpectomy, wide excision, quadrantectomy, and da Vinci robot-assisted axillary lymph node dissection for BC patients with obesity (238). A prospective study was conducted for 20 years of follow-up of patients with radical mastectomy (Halsted). The study compared the efficacy of breast-conserving surgery to other more radical BC surgeries (radical mastectomy) in normal and obese individuals. It has been concluded that breast-conserving surgery is a better treatment choice for patients with relatively small tumors. However, the data indicated that the long-term survival rate does not differ for the compared types of surgeries (239). Primary and secondary lymphedema are the most significant complications among the BC patients who underwent radical mastectomy. During both types of surgical intervention, the time of the surgical procedures should be considered as the impact factor for the outcome in older patients. Two independent population-based studies revealed that the prolonged time of the surgical intervention could be conducive to the low overall and disease-specific survival (240).
4.5.2 Oophorectomy
For less than 50-year-old patients with early BC, the removal of ovaries (241) substantially increased long-term survival in the absence of follow-up chemotherapy. The Early BC Trialists’ Collaborative Group reported 130 deaths and 153 recurrences among 2,102 patients (<50 years old). In this cohort, the 15-year survival rate was highly increased among the patients who underwent ovarian ablation [52.4% vs. 46.1%, 6.3 (SD 2.3)]. The relatively low number of deaths (per 100 women, log rank 2p = 0.001) also correlated with significantly improved recurrence-free survival (45.0% vs. 39.0%, 2p = 0.0007) (242).
4.6 Obesity and Complementary Therapeutic Implications Against Breast Cancer
The improvement in surgical techniques and medications can effectively mitigate the mortality rate of BC patients. However, a plethora of scientific evidence reported that we need to apply different complementary approaches to improve the overall survival rate and the quality of life (QoL) of BC survivors with obesity. Therefore, it is crucial to mitigate obesity and its related complications pertaining to the BC incidence and enhancement using complementary nutritional interventions as adjuvant therapy in addition to the conventional therapeutic management.
4.6.1 Physical Activity as a Promising Weight-Reducing Approach in Breast Cancer Patients
Regular endurance exercise is believed to be an effective approach to increase QoL in obese patients who may be prone to BCs. The cardiorespiratory function, physical fitness, and fatigue in BC survivors can be modulated using exercise strategies (243). Aerobic exercise therapy was shown to improve social/family wellbeing, mental health (244), and self-esteem (245) in addition to other physical benefits. More than 150 min of moderate or 75 min of intense aerobic exercise per week and more than 2 days of strength training per week are recommended to complement the adjuvant or hormone-based chemotherapies (American Cancer Society Guidelines) (226–230). Exercise is a safe and feasible technique for improving the physical functioning, fatigue, and overall QoL (246). Additionally, several studies showed that moderate physical activity can increase chemotherapy effectiveness (245). The exercise-based complementary strategy was suggested to improve QoL of obese BC patients. A decrease in serum CRP after intensive physical training has been reported (247). Relevant studies were summarized in Table 3 and indicate positive outcomes of physical activity in obese BC patients (253).
4.6.2 Healthy Weight and Breast Cancer Risk
Regular maintenance of healthy weight is a critical process, and a number of studies over the past decades proved that being overweight or obese at the time of diagnosis indicates a poor prognosis and relatively causes a higher chance of developing primary and secondary lymphedema (254). The data strongly suggest that body weight is closely linked to the overall clinical outcomes in individuals with BC (255). Furthermore, the pooled analysis of several major prospective cohort studies suggested the positive interaction between body size including height, weight, and risk of postmenopausal BC (256). Body weight is also found to be the only risk factor between BC incidence and subsequent prognosis among women in a randomized trial of adjuvant ovarian ablation (257). Furthermore, obesity is also characterized with a higher risk of developing postsurgical lymphedema (258) that is one of the most common complications of BC surgery and radiation therapy (259). Modest weight loss (5%–10%) is recommended for BC women who are overweight or obese (260). A 12-week weight loss diet and training trial revealed a reduction in breast ductal fluid estrogens with weight loss in postmenopausal women (261). There are several interventions that can be employed according to the patient’s needs and diagnostic factors (Table 4).
4.6.3 Dietary Choices as Complementary Approach for Breast Cancer Treatment
Healthy and balanced diet is an important component of recovery after and during BC treatment. It helps not only to improve QoL but also to provide better treatment outcomes. A diet rich in vegetables, fruits, and whole grains is the preferred regimen for obese women with BC (266). Moreover, a diet with low saturated fats and limited alcohol consumption are beneficial strategies to mitigate BC pathophysiology and adiposity-induced complications. Several pro-oncogenic pathways are targeted by healthy diet components. Previous research reports suggested a 43% decrease in overall mortality in BC survivors who consumed high-vegetable and -whole grain diets (267). Another study indicated a decrease in serum CRP, matrix metalloproteinase 9 (MMP-9), vascular cell adhesion molecule 1 (VCAM), and P-selectin levels in patients on a high-fiber and low-fat diet (268). A low-fat diet reduced the risk of invasive BC, suggesting that a low fat intake has potential long-term benefits (269). Restricted alcohol intake was also shown to be beneficial in several observational studies (270). Excessive ethanol intake can alter hormonal hemostasis and increase BC susceptibility (271). However, the association between some protein-based diets, including soy and dairy intake, and the increased risk of BC remains controversial. Several reports suggested the protective role of dairy products in BC patients (272–275). The evidence of isoflavone-related benefits in BC warrants further investigation (276).
4.6.4 Dietary Supplement as a Complementary Approach in Breast Cancer Patients
There is insufficient scientific evidence available to support the benefits of dietary supplements, including vitamins, minerals, and traditional medicinal herbs. However, BC patients reported the highest intake of dietary supplements (277). Notably, BC patients exhibited higher rates of vitamin D insufficiency (278), although calcium and vitamin D supplements failed to reduce the incidence of invasive BC in postmenopausal women. A recent study reported a comparative efficacy and safety of natural therapies and herbal medicines against invasive BC (279). However, herbal medicine efficacy and health adverse effects remain underaddressed. The application of herbal remedies warrants further investigation, especially in advanced and metastatic BC patients (280). A remedy with established biochemical or clinical benefits in BC patients with nutritional deficiency may be recommended as a supplemental anticancer intervention (281). However, robust clinical trials are required to confirm the efficacy and absence of adverse effects in BC patients who consume herbal medicinal and/or nutritional agents as dietary supplements for weight reduction.
5 Conclusions and Summary
Obesity is one of the significant health-related complications in the modern affluent society. Approximately 2 billion adults were considered overweight or obese worldwide in 2015, accounting for 38%–40% of the world’s population (282). Obesity is associated with higher morbidity and mortality rates for many chronic diseases, including BC. The incidence of obesity has been increasing in the aging population (283–286). Excessive adipose tissues and adipocyte hypertrophy were associated with higher levels of local estrogen and chronic inflammation, the factors responsible for BC development and progression in postmenopausal women. However, not all questions related to the role of adipose tissue in cancer patients have been clarified. Moderately overweight patients have shown better survival outcomes after extensive chemotherapy treatments, suggesting that the “obesity paradox” should be thoroughly investigated. BMI has significant implications for BC treatment, the development of novel targeted therapies, and better BC prognosis. Current conventional strategies should be complemented by weight management and primary prevention programs. Early diagnosis and treatment markers may also include some adiposity-related targets. The “personalized medicine” approach should include diet and lifestyle approaches to reach better treatment outcomes. However, overweight reduction, the target for novel therapeutic and preventive measures, should be considered individually after consideration of all confounding factors. Physical activity, diet changes, and optimal weight management should be considered as the most valuable complementary methods that can help to reach better BC surgery and chemotherapy and/or radiotherapy outcomes.
Data Availability Statement
The original contributions presented in the study are included in the article/supplementary material. Further inquiries can be directed to the corresponding authors.
Author Contributions
KC, JZ, NB, and OS conceptualized and designed the study. CT, YB, IR, OS, MS, JCZ, XZ, PL, JL, RF, NB, and KC performed the literature analysis and wrote different parts of the original article draft. NB, KC, MS, OS, RF, and PL revised, edited, and extended the final draft. All authors have reviewed and approved the article before submission.
Funding
The authors are thankful to the National Natural Science Foundation of China (No. 81703158) and the Russian Academic Excellence project “5-100” of the Sechenov University, Moscow, Russia, for the financial support.
Conflict of Interest
The authors declare that the research was conducted in the absence of any commercial or financial relationships that could be construed as a potential conflict of interest.
Publisher’s Note
All claims expressed in this article are solely those of the authors and do not necessarily represent those of their affiliated organizations, or those of the publisher, the editors and the reviewers. Any product that may be evaluated in this article, or claim that may be made by its manufacturer, is not guaranteed or endorsed by the publisher.
References
1. Nock NL, Berger NA. Obesity and Cancer: Overview of Mechanisms. In: Berger N. (eds) Cancer and Energy Balance, Epidemiology and Overview. Energy Balance and Cancer, (2010). New York, NY.: Springer 2. 129–79. doi: 10.1007/978-1-4419-5515-9_5
2. Hursting SD, Berger NA. Energy Balance, Host-Related Factors, and Cancer Progression. J Clin Oncol (2010) 28(26):4058. doi: 10.1200/JCO.2010.27.9935
3. Hursting SD. Obesity, Energy Balance, and Cancer: A Mechanistic Perspective. In: Zappia V., Panico S., Russo G., Budillon A., Della Ragione F. (eds) Advances in Nutrition and Cancer. Cancer Treatment and Research, (2014) vol. 159. Springer, Berlin, Heidelberg. doi: 10.1007/978-3-642-38007-5_2
4. Berger NA. Obesity and Cancer Pathogenesis. Ann N Y Acad Sci (2014) 1311:57. doi: 10.1111/nyas.12416
5. Lennon H, Sperrin M, Badrick E, Renehan AG. The Obesity Paradox in Cancer: A Review. Curr Oncol Rep (2016) 18(9):1–8. doi: 10.1007/s11912-016-0539-4
6. Cespedes Feliciano EM, Kroenke CH, Caan BJ. The Obesity Paradox in Cancer: How Important is Muscle? Annu Rev Nutr (2018) 38:357–79. doi: 10.1146/annurev-nutr-082117-051723
7. Sánchez-Jiménez F, Pérez-Pérez A, de la Cruz-Merino L, Sánchez-Margalet V. Obesity and Breast Cancer: Role of Leptin. Front Oncol (2019) 9:596. doi: 10.3389/fonc.2019.00596
8. Brown KA, Simposon ER. Obesity and Breast Cancer: Mechanisms and Therapeutic Implications. Front Biosci (Elite Ed) (2012) 4:2515–24. doi: 10.2741/e562
9. Shoelson SE, Herrero L, Naaz A. Obesity, Inflammation, and Insulin Resistance. Gastroenterology (2007) 132(6):2169–80. doi: 10.1053/j.gastro.2007.03.059
10. Beeraka NM, Tomilova IK, Batrak GA, Zhaburina MV, Nikolenko VN, Sinelnikov MY, et al. Recent Insights Into the Nutritional Antioxidant Therapy in Prevention and Treatment of Diabetic Vascular Complications-A Comprehensive Review. Curr Med Chem (2021) 29(16):1920–1935. doi: 10.2174/0929867328666210810142527
11. Salem SR, Beeraka NM, Roaeid RB, Huria TR. Use of Metformin According to Renal Function in Patients With Type II Diabetes. Clin Pharmacol Toxicol Res (2021) 4:1. Available at: https://www.researchgate.net/profile/Narasimha-Beeraka/publication/349882520_Use_of_metformin_according_to_renal_function_in_patients_with_type_II_diabetes/links/6045e6b392851c077f248f50/Use-of-metformin-according-to-renal-function-in-patients-with-type-II-diabetes.pdf.
12. Protani M, Coory M, Martin JH. Effect of Obesity on Survival of Women With Breast Cancer: Systematic Review and Meta-Analysis. Breast Cancer Res Treat (2010) 123(3):627–35. doi: 10.1007/s10549-010-0990-0
13. Crichton MB, Nichols JE, Zhao Y, Bulun SE, Simpson ER. Expression of Transcripts of Interleukin-6 and Related Cytokines by Human Breast Tumors, Breast Cancer Cells, and Adipose Stromal Cells. Mol Cell Endocrinol (1996) 118(1):215–20. doi: 10.1016/0303-7207(96)03761-6
14. Ougolkov AV, Fernandez-Zapico ME, Bilim VN, Smyrk TC, Chari ST, Billadeau DD. Aberrant Nuclear Accumulation of Glycogen Synthase Kinase-3β in Human Pancreatic Cancer: Association With Kinase Activity and Tumor Dedifferentiation. Clin Cancer Res (2006) 12(17):5074–81. doi: 10.1158/1078-0432.CCR-06-0196
15. Sun H, Zou J, Chen L, Zu X, Wen G, Zhong J. Triple-negative Breast Cancer and its Association With Obesity. Mol Clin Oncol (2017) 7(6):935–42. doi: 10.3892/mco.2017.1429
16. Cortellini A, Bersanelli M, Buti S, Cannita K, Santini D, Perrone F, et al. A Multicenter Study of Body Mass Index in Cancer Patients Treated With Anti-PD-1/PD-L1 Immune Checkpoint Inhibitors: When Overweight Becomes Favorable. J Immunother Cancer (2019) 7(1):1–11. doi: 10.1186/s40425-019-0527-y
17. McQuade JL, Daniel CR, Hess KR, Mak C, Wang DY, Rai RR, et al. Association of Body-Mass Index and Outcomes in Patients With Metastatic Melanoma Treated With Targeted Therapy, Immunotherapy, or Chemotherapy: A Retrospective, Multicohort Analysis. Lancet Oncol (2018) 19(3):310–22. doi: 10.1016/S1470-2045(18)30078-0
18. Fang S, Wang Y, Dang Y, Gagel A, Ross MI, Gershenwald JE, et al. Association Between Body Mass Index, C-Reactive Protein Levels, and Melanoma Patient Outcomes. J Invest Dermatol (2017) 137(8):1792–5. doi: 10.1016/j.jid.2017.04.007
19. Dieterich M, Stubert J, Reimer T, Erickson N, Berling A. Influence of Lifestyle Factors on Breast Cancer Risk. Breast Care (2014) 9(6):407–14. doi: 10.1159/000369571
20. McTiernan A. Obesity and Cancer: The Risks, Science, and Potential Management Strategies. Oncology (Williston Park NY) (2005) 19(7):871–881; discussion 881.
21. Marmot M, Atinmo T, Byers T, Chen J, Hirohata T, Jackson A, et al. Food, Nutrition, Physical Activity, and the Prevention of Cancer: A Global Perspective. (2007).
22. Thomson CA, McCullough ML, Wertheim BC, Chlebowski RT, Martinez ME, Stefanick ML, et al. Nutrition and Physical Activity Cancer Prevention Guidelines, Cancer Risk, and Mortality in the Women's Health Initiative. Cancer Prev Res (2014) 7(1):42–53. doi: 10.1158/1940-6207.CAPR-13-0258
23. Baer HJ, Colditz GA, Rosner B, Michels KB, Rich-Edwards JW, Hunter DJ, et al. Body Fatness During Childhood and Adolescence and Incidence of Breast Cancer in Premenopausal Women: A Prospective Cohort Study. Breast Cancer Res (2005) 7(3):1–12. doi: 10.1186/bcr998
24. Kotsopoulos J, Olopade OI, Ghadirian P, Lubinski J, Lynch HT, Isaacs C, et al. Changes in Body Weight and the Risk of Breast Cancer in BRCA1 and BRCA2mutation Carriers. Breast Cancer Res (2005) 7(5):1–11. doi: 10.1186/bcr1293
25. Krajinovic M, Ghadirian P, Richer C, Sinnett H, Gandini S, Perret C, et al. Genetic Susceptibility to Breast Cancer in French-Canadians: Role of Carcinogen-Metabolizing Enzymes and Gene–Environment Interactions. Int J Cancer (2001) 92(2):220–5. doi: 10.1002/1097-0215(200102)9999:9999<::AID-IJC1184>3.0.CO;2-H
26. Kohlmeier L. Biomarkers of Fatty Acid Exposure and Breast Cancer Risk. Am J Clin Nutr (1997) 66(6):1548S–56S. doi: 10.1093/ajcn/66.6.1548S
27. Smith-Warner SA, Spiegelman D, Yaun S-S, Van Den Brandt PA, Folsom AR, Goldbohm RA, et al. Alcohol and Breast Cancer in Women: A Pooled Analysis of Cohort Studies. Jama (1998) 279(7):535–40. doi: 10.1001/jama.279.7.535
28. Zhang SM, Lee I-M, Manson JE, Cook NR, Willett WC, Buring JE. Alcohol Consumption and Breast Cancer Risk in the Women's Health Study. Am J Epidemiol (2007) 165(6):667–76. doi: 10.1093/aje/kwk054
29. Cancer CGoHFiB. Alcohol, Tobacco and Breast Cancer–Collaborative Reanalysis of Individual Data From 53 Epidemiological Studies, Including 58 515 Women With Breast Cancer and 95 067 Women Without the Disease. Br J Cancer (2002) 87(11):1234. doi: 10.1038/sj.bjc.6600596
30. Fritschi L, Erren T, Glass DC, Girschik J, Thomson A, Saunders C, et al. The Association Between Different Night Shiftwork Factors and Breast Cancer: A Case–Control Study. Br J Cancer (2013) 109(9):2472–80. doi: 10.1038/bjc.2013.544
31. Kamdar BB, Tergas AI, Mateen FJ, Bhayani NH, Oh J. Night-Shift Work and Risk of Breast Cancer: A Systematic Review and Meta-Analysis. Breast Cancer Res Treat (2013) 138(1):291–301. doi: 10.1007/s10549-013-2433-1
32. Erren TC, Pape HG, Reiter RJ, Piekarski C. Chronodisruption and Cancer. Naturwissenschaften (2008) 95(5):367–82. doi: 10.1007/s00114-007-0335-y
33. Fakri S, Al Azzawi A, Al Tawil N. Antiperspirant Use as a Risk Factor for Breast Cancer in Iraq. East Mediterr Health J (2006) 12(3-4):478–82.
34. McGrath K. “An Earlier Age of Breast Cancer Diagnosis Related to More Frequent Use of Antiperspirants/Deodorants and Underarm Shaving.” European Journal of Cancer Prevention (2003) 12(6):479–85. JSTOR, http://www.jstor.org/stable/45074373. Accessed 2 Jun. 2022. doi: 10.1097/00008469-200312000-00006
35. Darbre PD. Recorded Quadrant Incidence of Female Breast Cancer in Great Britain Suggests a Disproportionate Increase in the Upper Outer Quadrant of the Breast. Anticancer Res (2005) 25(3C):2543–50.
36. Spear SL, Murphy DK, Slicton A, Walker PS, Group ISBIUS. Inamed Silicone Breast Implant Core Study Results at 6 Years. Plast Reconstr Surg (2007) 120(7):8S–16S. doi: 10.1097/01.prs.0000286580.93214.df
37. Bengtson BP, Van Natta BW, Murphy DK, Slicton A, Maxwell GP, Group SUCCS. Style 410 Highly Cohesive Silicone Breast Implant Core Study Results at 3 Years. Plast Reconstr Surg (2007) 120(7):40S–8S. doi: 10.1097/01.prs.0000286666.29101.11
38. Cunningham B, McCue J. Safety and Effectiveness of Mentor’s MemoryGel Implants at 6 Years. Aesthetic Plast Surg (2009) 33(3):440–4. doi: 10.1007/s00266-009-9364-6
39. Maxwell GP, Van Natta BW, Murphy DK, Slicton A, Bengtson BP. Natrelle Style 410 Form-Stable Silicone Breast Implants: Core Study Results at 6 Years. Aesthetic Surg J (2012) 32(6):709–17. doi: 10.1177/1090820X12452423
40. Mahbouli S, Der Vartanian A, Ortega S, Rougé S, Vasson M-P, Rossary A. Leptin Induces ROS via NOX5 in Healthy and Neoplastic Mammary Epithelial Cells. Oncol Rep (2017) 38(5):3254–64. doi: 10.3892/or.2017.6009
41. Nadal-Serrano M, Sastre-Serra J, Valle A, Roca P, Oliver J. Chronic-Leptin Attenuates Cisplatin Cytotoxicity in MCF-7 Breast Cancer Cell Line. Cell Physiol Biochem (2015) 36(1):221–32. doi: 10.1159/000374066
42. Jardé T, Perrier S, Vasson M-P, Caldefie-Chézet F. Molecular Mechanisms of Leptin and Adiponectin in Breast Cancer. Eur J Cancer (2011) 47(1):33–43. doi: 10.1016/j.ejca.2010.09.005
43. Nepal S, Kim MJ, Hong JT, Kim SH, Sohn D-H, Lee SH, et al. Correction: Autophagy Induction by Leptin Contributes to Suppression of Apoptosis in Cancer Cells and Xenograft Model: Involvement of P53/FoxO3A Axis. Oncotarget (2020) 11(30):2956. doi: 10.18632/oncotarget.27467
44. Barone I, Catalano S, Gelsomino L, Marsico S, Giordano C, Panza S, et al. Leptin Mediates Tumor–Stromal Interactions That Promote the Invasive Growth of Breast Cancer Cells. Cancer Res (2012) 72(6):1416–27. doi: 10.1158/0008-5472.CAN-11-2558
45. Li K, Wei L, Huang Y, Wu Y, Su M, Pang X, et al. Leptin Promotes Breast Cancer Cell Migration and Invasion via IL-18 Expression and Secretion. Int J Oncol (2016) 48(6):2479–87. doi: 10.3892/ijo.2016.3483
46. Bowers LW, Rossi EL, McDonell SB, Doerstling SS, Khatib SA, Lineberger CG, et al. Leptin Signaling Mediates Obesity-Associated CSC Enrichment and EMT in Preclinical TNBC Models. Mol Cancer Res (2018) 16(5):869–79. doi: 10.1158/1541-7786.MCR-17-0508
47. Mullen M, Gonzalez-Perez RR. Leptin-Induced JAK/STAT Signaling and Cancer Growth. Vaccines (2016) 4(3):26. doi: 10.3390/vaccines4030026
48. Saxena NK, Vertino PM, Anania FA, Sharma D. Leptin-Induced Growth Stimulation of Breast Cancer Cells Involves Recruitment of Histone Acetyltransferases and Mediator Complex to CYCLIN D1 Promoter via Activation of Stat3. J Biol Chem (2007) 282(18):13316–25. doi: 10.1074/jbc.M609798200
49. Taub M, Springate JE, Cutuli F. Targeting of Renal Proximal Tubule Na, K-ATPase by Salt-Inducible Kinase. Biochem Biophys Res Commun (2010) 393(3):339–44. doi: 10.1016/j.bbrc.2010.02.037
50. Park JW, Zhao L, Willingham MC, Cheng S-Y. Inhibition of STAT3 Signaling Blocks Obesity-Induced Mammary Hyperplasia in a Mouse Model. Am J Cancer Res (2017) 7(3):727.
51. Thiagarajan PS, Zheng Q, Bhagrath M, Mulkearns-Hubert EE, Myers MG, Lathia JD, et al. STAT3 Activation by Leptin Receptor is Essential for TNBC Stem Cell Maintenance. Endocr Relat Cancer (2017) 24(8):415–26. doi: 10.1530/ERC-16-0349
52. Wang T, Fahrmann JF, Lee H, Li Y-J, Tripathi SC, Yue C, et al. JAK/STAT3-Regulated Fatty Acid β-Oxidation is Critical for Breast Cancer Stem Cell Self-Renewal and Chemoresistance. Cell Metab (2018) 27(1):136–150.e135. doi: 10.1016/j.cmet.2017.11.001
53. Zahid H, Subbaramaiah K, Iyengar NM, Zhou XK, Chen I-C, Bhardwaj P, et al. Leptin Regulation of the P53-HIF1α/PKM2-Aromatase Axis in Breast Adipose Stromal Cells: A Novel Mechanism for the Obesity–Breast Cancer Link. Int J Obes (2018) 42(4):711–20. doi: 10.1038/ijo.2017.273
54. Barone I, Giordano C, Bonofiglio D, Andò S, Catalano S. Leptin, Obesity and Breast Cancer: Progress to Understanding the Molecular Connections. Curr Opin Pharmacol (2016) 31:83–9. doi: 10.1016/j.coph.2016.10.003
55. Allison MB, Myers MG Jr. Connecting Leptin Signaling to Biological Function. J Endocrinol (2014) 223(1):T25. doi: 10.1530/JOE-14-0404
56. Lianyuan T, Dianrong X, Chunhui Y, Zhaolai M, Bin J. The Predictive Value and Role of Stromal Tumor-Infiltrating Lymphocytes in Pancreatic Ductal Adenocarcinoma (PDAC). Cancer Biol Ther (2018) 19(4):296–305. doi: 10.1080/15384047.2017.1416932
57. Mei Z, Zhang X, Yi J, Huang J, He J, Tao Y. Sirtuins in Metabolism, DNA Repair and Cancer. J Exp Clin Cancer Res (2016) 35(1):1–14. doi: 10.1186/s13046-016-0461-5
58. Huang Y, Jin Q, Su M, Ji F, Wang N, Zhong C, et al. Leptin Promotes the Migration and Invasion of Breast Cancer Cells by Upregulating ACAT2. Cell Oncol (2017) 40(6):537–47. doi: 10.1007/s13402-017-0342-8
59. Frasca F, Pandini G, Scalia P, Sciacca L, Mineo R, Costantino A, et al. Insulin Receptor Isoform A, a Newly Recognized, High-Affinity Insulin-Like Growth Factor II Receptor in Fetal and Cancer Cells. Mol Cell Biol (1999) 19(5):3278–88. doi: 10.1128/MCB.19.5.3278
60. Harrington SC, Weroha SJ, Reynolds C, Suman VJ, Lingle WL, Haluska P. Quantifying Insulin Receptor Isoform Expression in FFPE Breast Tumors. Growth Horm IGF Res (2012) 22(3-4):108–15. doi: 10.1016/j.ghir.2012.04.001
61. Heni M, Hennenlotter J, Scharpf M, Lutz SZ, Schwentner C, Todenhöfer T, et al. Insulin Receptor Isoforms A and B as Well as Insulin Receptor Substrates-1 and-2 are Differentially Expressed in Prostate Cancer. PloS One (2012) 7(12):e50953. doi: 10.1371/journal.pone.0050953
62. Del Giudice ME, Fantus IG, Ezzat S, McKeown-Eyssen G, Page D, Goodwin PJ. Insulin and Related Factors in Premenopausal Breast Cancer Risk. Breast Cancer Res Treat (1998) 47(2):111–20. doi: 10.1023/A:1005831013718
63. Thissen J-P, Ketelslegers J-M, Underwood LE. Nutritional Regulation of the Insulin-Like Growth Factors. Endocr Rev (1994) 15(1):80–101. doi: 10.1210/edrv-15-1-80
64. Taniguchi CM, Kahn CR. Insulin/IGF-1 Signaling Nodes and Their Role in Carcinogenesis. In: Insulin Resistance and Cancer. New York, NY: Springer. (2011). p. pp53–76. doi: 10.1007/978-1-4419-9911-5_2
65. Babichev Y, Khalid S, Fantus IG. Potential Mechanisms Linking Insulin to Cancer. In: Insulin Resistance and Cancer. New York, NY: Springer. (2011). p. 159–80.
66. Belardi V, Gallagher EJ, Novosyadlyy R, LeRoith D. Insulin and IGFs in Obesity-Related Breast Cancer. J Mammary Gland Biol Neoplasia (2013) 18(3-4):277–89. doi: 10.1007/s10911-013-9303-7
67. Plottel CS, Blaser MJ. Microbiome and Malignancy. Cell Host Microbe (2011) 10(4):324–35. doi: 10.1016/j.chom.2011.10.003
68. Black DM, Solomon E. The Search for the Familial Breast/Ovarian Cancer Gene. Trends Genet (1993) 9(1):22–6. doi: 10.1016/0168-9525(93)90068-S
69. Fournier S, Brihmat F, Durand J, Sterkers N, Martin P, Kuttenn F, et al. Estradiol 17β-Hydroxysteroid Dehydrogenase, a Marker of Breast Cancer Hormone Dependency. Cancer Res (1985) 45(6):2895–9.
70. Lee YS, Kim JW, Osborne O, Oh DY, Sasik R, Schenk S, et al. Increased Adipocyte O2 Consumption Triggers HIF-1alpha, Causing Inflammation and Insulin Resistance in Obesity. Cell (2014) 157(6):1339–52. doi: 10.1016/j.cell.2014.05.012
71. Available at: https://app.biorender.com/.
72. Zahid H, Simpson ER, Brown KA. Inflammation, Dysregulated Metabolism and Aromatase in Obesity and Breast Cancer. Curr Opin Pharmacol (2016) 31:90–6. doi: 10.1016/j.coph.2016.11.003
73. Dallavalasa S, Beeraka NM, Basavaraju CG, Tulimilli SV, Sadhu SP, Rajesh K, et al. The Role of Tumor Associated Macrophages (TAMs) in Cancer Progression, Chemoresistance, Angiogenesis and Metastasis-Current Status. Curr Med Chem (2021) 28(34):8203–8236. doi: 10.2174/0929867328666210720143721
74. Lumeng CN, Bodzin JL, Saltiel AR. Obesity Induces a Phenotypic Switch in Adipose Tissue Macrophage Polarization. J Clin Invest (2007) 117(1):175–84. doi: 10.1172/JCI29881
75. Elinav E, Nowarski R, Thaiss CA, Hu B, Jin C, Flavell RA. Inflammation-Induced Cancer: Crosstalk Between Tumours, Immune Cells and Microorganisms. Nat Rev Cancer (2013) 13(11):759–71. doi: 10.1038/nrc3611
76. Lenaz G. Role of Mitochondria in Oxidative Stress and Ageing. Biochim Biophys Acta (BBA)-Bioenerg (1998) 1366(1-2):53–67. doi: 10.1016/S0005-2728(98)00120-0
77. Bournat JC, Brown CW. Mitochondrial Dysfunction in Obesity. Curr Opin Endocrinol Diabetes Obes (2010) 17(5):446–52. doi: 10.1097/MED.0b013e32833c3026
78. Chen K, Lu P, Beeraka NM, Sukocheva OA, Madhunapantula SV, Liu J, et al. Mitochondrial Mutations and Mitoepigenetics: Focus on Regulation of Oxidative Stress-Induced Responses in Breast Cancers. Semin Cancer Biol (2020). In Press. doi: 10.1016/j.semcancer.2020.09.012
79. Lupulescu A. Estrogen Use and Cancer Incidence: A Review. Cancer Invest (1995) 13(3):287–95. doi: 10.3109/07357909509094464
80. Kulkoyluoglu-Cotul E, Arca A, Madak-Erdogan Z. Crosstalk Between Estrogen Signaling and Breast Cancer Metabolism. Trends Endocrinol Metab (2019) 30(1):25–38. doi: 10.1016/j.tem.2018.10.006
81. Martin KA, Freeman MW. Postmenopausal Hormone-Replacement Therapy. N Engl J Med (1993) 328(15):1115–7. doi: 10.1056/nejm199304153281509
82. Sukocheva O, Wadham C, Holmes A, Albanese N, Verrier E, Feng F, et al. Estrogen Transactivates EGFR via the Sphingosine 1-Phosphate Receptor Edg-3: The Role of Sphingosine Kinase-1. J Cell Biol (2006) 173(2):301–10. doi: 10.1083/jcb.200506033
83. Brown KA, Samarajeewa NU, Simpson ER. Endocrine-Related Cancers and the Role of AMPK. Mol Cell Endocrinol (2013) 366(2):170–9. doi: 10.1016/j.mce.2012.06.016
84. Samarajeewa NU, Docanto MM, Simpson ER, Brown KA. CREB-Regulated Transcription Co-Activator Family Stimulates Promoter II-Driven Aromatase Expression in Preadipocytes. Horm Cancer (2013) 4(4):233–41. doi: 10.1007/s12672-013-0142-1
85. White AJ, Nichols HB, Bradshaw PT, Sandler DP. Overall and Central Adiposity and Breast Cancer Risk in the Sister Study. Cancer (2015) 121(20):3700–8. doi: 10.1002/cncr.29552
86. Huang Z, Hankinson SE, Colditz GA, Stampfer MJ, Hunter DJ, Manson JE, et al. Dual Effects of Weight and Weight Gain on Breast Cancer Risk. Jama (1997) 278(17):1407–11. doi: 10.1001/jama.1997.03550170037029
87. Eliassen AH, Colditz GA, Rosner B, Willett WC, Hankinson SE. Adult Weight Change and Risk of Postmenopausal Breast Cancer. JAMA (2006) 296(2):193–201. doi: 10.1001/jama.296.2.193
88. Chen K, Beeraka N M, Zhang J, Reshetov IV, Nikolenko VN, Sinelnikov MY, et al. Efficacy of Da Vinci Robot-Assisted Lymph Node Surgery Than Conventional Axillary Lymph Node Dissection in Breast Cancer–A Comparative Study. Int J Med Robot Comput Assist Surg (2021) 17(6):e2307. doi: 10.1002/rcs.2307
89. Kopp H-P, Kopp C, Festa A, Krzyzanowska K, Kriwanek S, Minar E, et al. Impact of Weight Loss on Inflammatory Proteins and Their Association With the Insulin Resistance Syndrome in Morbidly Obese Patients. Arterioscler Thromb Vasc Biol (2003) 23(6):1042–7. doi: 10.1161/01.ATV.0000073313.16135.21
90. McLaughlin T, Abbasi F, Lamendola C, Liang L, Reaven G, Schaaf P, et al. Differentiation Between Obesity and Insulin Resistance in the Association With C-Reactive Protein. Circulation (2002) 106(23):2908–12. doi: 10.1161/01.CIR.0000041046.32962.86
91. Ryan AS, Nicklas BJ. Reductions in Plasma Cytokine Levels With Weight Loss Improve Insulin Sensitivity in Overweight and Obese Postmenopausal Women. Diabetes Care (2004) 27(7):1699–705. doi: 10.2337/diacare.27.7.1699
92. Yang R-Z, Lee M-J, Hu H, Pollin TI, Ryan AS, Nicklas BJ, et al. Acute-Phase Serum Amyloid A: An Inflammatory Adipokine and Potential Link Between Obesity and its Metabolic Complications. PloS Med (2006) 3(6):e287. doi: 10.1371/journal.pmed.0030287
93. Tchernof A, Nolan A, Sites CK, Ades PA, Poehlman ET. Weight Loss Reduces C-Reactive Protein Levels in Obese Postmenopausal Women. Circulation (2002) 105(5):564–9. doi: 10.1161/hc0502.103331
94. Key T, Appleby P, Reeves G, Roddam A, Dorgan J. Longcope CEndogenous Hormones Breast Cancer Collaborative Group. Body Mass Index, Serum Sex Hormones, and Breast Cancer Risk in Postmenopausal Women. J Natl Cancer Inst (2003) 95:1218–26. doi: 10.1093/jnci/djg022
95. Bulun SE, Chen D, Moy I, Brooks DC, Zhao H. Aromatase, Breast Cancer and Obesity: A Complex Interaction. Trends Endocrinol Metab (2012) 23(2):83–9. doi: 10.1016/j.tem.2011.10.003
96. Folkerd EJ, Dixon JM, Renshaw L, A'Hern RP, Dowsett M. Suppression of Plasma Estrogen Levels by Letrozole and Anastrozole is Related to Body Mass Index in Patients With Breast Cancer. J Clin Oncol (2012) 30(24):2977–80. doi: 10.1200/JCO.2012.42.0273
97. Griggs JJ, Sorbero ME, Lyman GH. Undertreatment of Obese Women Receiving Breast Cancer Chemotherapy. Arch Internal Med (2005) 165(11):1267–73. doi: 10.1001/archinte.165.11.1267
98. Stephenson GD, Rose DP. Breast Cancer and Obesity: An Update. Nutr Cancer (2003) 45(1):1–16. doi: 10.1207/S15327914NC4501_1
99. Lorincz A, Sukumar S. Molecular Links Between Obesity and Breast Cancer. Endocr Relat Cancer (2006) 13(2):279–92. doi: 10.1677/erc.1.00729
100. Picon-Ruiz M, Morata-Tarifa C, Valle-Goffin JJ, Friedman ER, Slingerland JM. Obesity and Adverse Breast Cancer Risk and Outcome: Mechanistic Insights and Strategies for Intervention. CA Cancer J Clin (2017) 67(5):378–97. doi: 10.3322/caac.21405
101. Calle EE, Rodriguez C, Walker-Thurmond K, Thun MJ. Overweight, Obesity, and Mortality From Cancer in a Prospectively Studied Cohort of US Adults. N Engl J Med (2003) 348(17):1625–38. doi: 10.1056/NEJMoa021423
102. Dawood S, Lei X, Litton JK, Buchholz TA, Hortobagyi GN, Gonzalez-Angulo AM. Impact of Body Mass Index on Survival Outcome Among Women With Early Stage Triple-Negative Breast Cancer. Clin Breast Cancer (2012) 12(5):364–72. doi: 10.1016/j.clbc.2012.07.013
103. Kwan ML, Chen WY, Kroenke CH, Weltzien EK, Beasley JM, Nechuta SJ, et al. Pre-Diagnosis Body Mass Index and Survival After Breast Cancer in the After Breast Cancer Pooling Project. Breast Cancer Res Treat (2012) 132(2):729–39. doi: 10.1007/s10549-011-1914-3
104. Warren LE, Ligibel JA, Chen Y-H, Truong L, Catalano PJ, Bellon JR. Body Mass Index and Locoregional Recurrence in Women With Early-Stage Breast Cancer. Ann Surg Oncol (2016) 23(12):3870–9. doi: 10.1245/s10434-016-5437-3
105. Perou CM, Sørlie T, Eisen MB, Van De Rijn M, Jeffrey SS, Rees CA, et al. Molecular Portraits of Human Breast Tumours. Nature (2000) 406(6797):747–52. doi: 10.1038/35021093
106. Tamimi RM, Colditz GA, Hazra A, Baer HJ, Hankinson SE, Rosner B, et al. Traditional Breast Cancer Risk Factors in Relation to Molecular Subtypes of Breast Cancer. Breast Cancer Res Treat (2012) 131(1):159–67. doi: 10.1007/s10549-011-1702-0
107. Brooks MD, Burness ML, Wicha MS. Therapeutic Implications of Cellular Heterogeneity and Plasticity in Breast Cancer. Cell Stem Cell (2015) 17(3):260–71. doi: 10.1016/j.stem.2015.08.014
108. Toft DJ, Cryns VL. Minireview: Basal-Like Breast Cancer: From Molecular Profiles to Targeted Therapies. Mol Endocrinol (Baltimore Md) (2011) 25(2):199–211. doi: 10.1210/me.2010-0164
109. Perou CM, Sørlie T, Eisen MB, van de Rijn M, Jeffrey SS, Rees CA, et al. Molecular Portraits of Human Breast Tumours. Nature (2000) 406(6797):747–52. doi: 10.1038/35021093
110. Prat A, Pascual T, De Angelis C, Gutierrez C, Llombart-Cussac A, Wang T, et al. HER2-Enriched Subtype and ERBB2 Expression in HER2-Positive Breast Cancer Treated With Dual HER2 Blockade. J Natl Cancer Inst (2020) 112(1):46–54. doi: 10.1093/jnci/djz042
111. Sorlie T, Perou CM, Tibshirani R, Aas T, Geisler S, Johnsen H, et al. Gene Expression Patterns of Breast Carcinomas Distinguish Tumor Subclasses With Clinical Implications. Proc Natl Acad Sci U S A (2001) 98(19):10869–74. doi: 10.1073/pnas.191367098
112. Available at: https://seer.cancer.gov/statfacts/html/breast-subtypes.html.
113. Chubarev VN, Beeraka NM, Sinelnikov MY, Bulygin KV, Nikolenko VN, Mihaylenko E, et al. Health Science Community Will Miss This Bright and Uniting Star: In Memory of Professor Gjumrakch Aliev, MD, Ph. D. Multidisciplinary Digital Publishing Institute (2021). Cancers 13(8):1965. doi: 10.3390/cancers13081965
114. Goldhirsch A, Ingle JN, Gelber R, Coates A, Thürlimann B, Senn H-J. Thresholds for Therapies: Highlights of the St Gallen International Expert Consensus on the Primary Therapy of Early Breast Cancer 2009. Ann Oncol (2009) 20(8):1319–29. doi: 10.1093/annonc/mdp322
115. Viale G. The Current State of Breast Cancer Classification. Ann Oncol (2012) 23 Suppl 10:x207–210. doi: 10.1093/annonc/mds326
116. Chen K, Beeraka NM, Gu Y, Li J, Sinelnikov M, Han N, et al. Totally Implantable Venous Access Port Systems: Implant Depth-Based Complications in Breast Cancer Therapy - A Comparative Study. Current Pharmaceutical Design (2021) 27(6):4671–4676. doi: 10.2174/1381612827666210901170522
117. Andreopoulou E, Schweber SJ, Sparano JA, McDaid HM. Therapies for Triple Negative Breast Cancer. Expert Opin Pharmacother (2015) 16(7):983–98. doi: 10.1517/14656566.2015.1032246
118. Parker JS, Mullins M, Cheang MC, Leung S, Voduc D, Vickery T, et al. Supervised Risk Predictor of Breast Cancer Based on Intrinsic Subtypes. J Clin Oncol (2009) 27(8):1160. doi: 10.1200/JCO.2008.18.1370
119. Fong PC, Boss DS, Yap TA, Tutt A, Wu P, Mergui-Roelvink M, et al. Inhibition of Poly (ADP-Ribose) Polymerase in Tumors From BRCA Mutation Carriers. N Engl J Med (2009) 361(2):123–34. doi: 10.1056/NEJMoa0900212
120. Kriege M, Seynaeve C, Meijers-Heijboer H, Collee JM, Menke-Pluymers M, Bartels C, et al. Sensitivity to First-Line Chemotherapy for Metastatic Breast Cancer in BRCA1 and BRCA2 Mutation Carriers. J Clin Oncol (2009) 27(23):3764–71. doi: 10.1200/JCO.2008.19.9067
121. Kriege M, Jager A, Hooning MJ, Huijskens E, Blom J, van Deurzen CH, et al. The Efficacy of Taxane Chemotherapy for Metastatic Breast Cancer in BRCA1 and BRCA2 Mutation Carriers. Cancer (2012) 118(4):899–907. doi: 10.1002/cncr.26351
122. Beeraka NM, Bovilla VR, Doreswamy SH, Puttalingaiah S, Srinivasan A, Madhunapantula SV. The Taming of Nuclear Factor Erythroid-2-Related Factor-2 (Nrf2) Deglycation by Fructosamine-3-Kinase (FN3K)-Inhibitors-A Novel Strategy to Combat Cancers. Cancers (2021) 13(2):281. doi: 10.3390/cancers13020281
123. Beeraka NM, Doreswamy SH, Sadhu SP, Srinivasan A, Pragada RR, Madhunapantula SV, et al. The Role of Exosomes in Stemness and Neurodegenerative Diseases—Chemoresistant-Cancer Therapeutics and Phytochemicals. Int J Mol Sci (2020) 21(18):6818. doi: 10.3390/ijms21186818
124. Chen K, Lu P, Beeraka NM, Sukocheva OA, Madhunapantula SV, Liu J, et al. Mikhaleva LM Mitochondrial Mutations and Mitoepigenetics: Focus on Regulation of Oxidative Stress-Induced Responses in Breast Cancers. In: Seminars in Cancer Biology. Elsevier Academic Press (2020). doi: 10.1016/j.semcancer.2020.09.012
125. Gradishar WJ, Anderson BO, Balassanian R, Blair SL, Burstein HJ, Cyr A, et al. NCCN Guidelines Insights: Breast Cancer, Version 1.2017. J Natl Compr Cancer Netw (2017) 15(4):433–51. doi: 10.6004/jnccn.2017.0044
126. Pernaut C, Lopez F, Ciruelos E. Standard Neoadjuvant Treatment in Early/Locally Advanced Breast Cancer. Breast Care (2018) 13(4):244–9. doi: 10.1159/000491759
127. Peto R, Davies C, Godwin J, Gray R, Pan H, Clarke M, et al. Early Breast Cancer Trialists’ Collaborative G. Comparisons Between Different Polychemotherapy Regimens for Early Breast Cancer: Meta-Analyses of Long-Term Outcome Among 100,000 Women in 123 Randomised Trials. Lancet (2012) 379(9814):432–44. doi: 10.1016/S0140-6736(11)61625-5
128. Gilyazova IR, Beeraka NM, Klimentova EA, Bulygin KV, Nikolenko VN, Izmailov AA, et al. Novel MicroRNA Binding Site SNPs and the Risk of Clear Cell Renal Cell Carcinoma (ccRCC): A Case-Control Study. Curr Cancer Drug Targets (2021) 21(3):203–12. doi: 10.2174/1568009620666201120151226
129. Manogaran P, Beeraka NM, Huang C-Y, Padma VV. Neferine and Isoliensinine From Nelumbo Nucifera Induced Reactive Oxygen Species (ROS)-Mediated Apoptosis in Colorectal Cancer HCT-15 Cells. Afr J Pharm Pharmacol (2019) 13(8):90–9. doi: 10.5897/AJPP2019.5036
130. Manogaran P, Beeraka NM, Huang C-Y, Padma VV. Neferine and Isoliensinine Enhance ‘Intracellular Uptake of Cisplatin’and Induce ‘ROS-Mediated Apoptosis’ in Colorectal Cancer Cells–A Comparative Study. Food Chem Toxicol (2019) 132:110652. doi: 10.1016/j.fct.2019.110652
131. Manogaran P, Beeraka NM, Padma VV. The Cytoprotective and Anti-Cancer Potential of Bisbenzylisoquinoline Alkaloids From Nelumbo Nucifera. Curr Top Med Chem (2019) 19(32):2940–57. doi: 10.2174/1568026619666191116160908
132. Reddy BD, Beeraka NM, Chitturi C, Madhunapantula SV. An Overview of Targeting Legumain for Inhibiting Cancers. Curr Pharm Des (2021) 27(12):3337–3348. doi: 10.2174/1381612826666201125111625
133. Chen K, Beeraka NM, Li J, Lu P. Anterior Abdominal Wall Defect Closed by the Anterior Sheath of the Upper Rectus Abdominis Muscle in a Patient With Prior “TRAM Flap Breast Reconstruction”. Indian J Surg (2021) 1–3. doi: 10.1007/s12262-021-02908-w
134. Mayer EL. Early and Late Long-Term Effects of Adjuvant Chemotherapy. Am Soc Clin Oncol Educ Book (2013) 33):9–14. doi: 10.14694/EdBook_AM.2013.33.9
135. Anampa J, Makower D, Sparano JA. Progress in Adjuvant Chemotherapy for Breast Cancer: An Overview. BMC Med (2015) 13(1):195. doi: 10.1186/s12916-015-0439-8
136. Trivers KF, Lund MJ, Porter PL, Liff JM, Flagg EW, Coates RJ, et al. The Epidemiology of Triple-Negative Breast Cancer, Including Race. Cancer Causes Control (2009) 20(7):1071–82. doi: 10.1007/s10552-009-9331-1
137. Mowad R, Chu QD, Li BD, Burton GV, Ampil FL, Kim RH. Does Obesity Have an Effect on Outcomes in Triple-Negative Breast Cancer? J Surg Res (2013) 184(1):253–9. doi: 10.1016/j.jss.2013.05.037
138. Matutino A, Joy A, Brezden-Masley C, Chia S, Verma S. Hormone Receptor–Positive, HER2-Negative Metastatic Breast Cancer: Redrawing the Lines. Curr Oncol (2018) 25(Suppl 1):S131. doi: 10.3747/co.25.4000
139. Gucalp A, Tolaney S, Isakoff SJ, Ingle JN, Liu MC, Carey LA, et al. Phase II Trial of Bicalutamide in Patients With Androgen Receptor–Positive, Estrogen Receptor–Negative Metastatic Breast Cancer. Clin Cancer Res (2013) 19(19):5505–12. doi: 10.1158/1078-0432.CCR-12-3327
140. Mansour EG, Gray R, Shatila AH, Osborne C, Tormey DC, Gilchrist KW, et al. Efficacy of Adjuvant Chemotherapy in High-Risk Node-Negative Breast Cancer. N Engl J Med (1989) 320(8):485–90. doi: 10.1056/NEJM198902233200803
141. Mauri D, Pavlidis N, Ioannidis JP. Neoadjuvant Versus Adjuvant Systemic Treatment in Breast Cancer: A Meta-Analysis. J Natl Cancer Inst (2005) 97(3):188–94. doi: 10.1093/jnci/dji021
142. Fitzpatrick A, Tutt A. Controversial Issues in the Neoadjuvant Treatment of Triple-Negative Breast Cancer. Ther Adv Med Oncol (2019) 11:1758835919882581. doi: 10.1177/1758835919882581
143. Livasy CA, Karaca G, Nanda R, Tretiakova MS, Olopade OI, Moore DT, et al. Phenotypic Evaluation of the Basal-Like Subtype of Invasive Breast Carcinoma. Mod Pathol (2006) 19(2):264–71. doi: 10.1038/modpathol.3800528
144. Carey LA, Rugo HS, Marcom PK, Mayer EL, Esteva FJ, Ma CX, et al. TBCRC 001: Randomized Phase II Study of Cetuximab in Combination With Carboplatin in Stage IV Triple-Negative Breast Cancer. J Clin Oncol (2012) 30(21):2615. doi: 10.1200/JCO.2010.34.5579
145. Keen JC, Yan L, Mack KM, Pettit C, Smith D, Sharma D, et al. A Novel Histone Deacetylase Inhibitor, Scriptaid, Enhances Expression of Functional Estrogen Receptor α (ER) in ER Negative Human Breast Cancer Cells in Combination With 5-Aza 2′-Deoxycytidine. Breast Cancer Res Treat (2003) 81(3):177–86. doi: 10.1023/A:1026146524737
146. Tate CR, Rhodes LV, Segar HC, Driver JL, Pounder FN, Burow ME, et al. Targeting Triple-Negative Breast Cancer Cells With the Histone Deacetylase Inhibitor Panobinostat. Breast Cancer Res (2012) 14(3):R79. doi: 10.1186/bcr3192
147. Connolly R, Jeter S, Zorzi J, Zhang Z, Armstrong D, Fetting J, et al. A Multi-Institutional Double-Blind Phase II Study Evaluating Response and Surrogate Biomarkers to Carboplatin and Nab-Paclitaxel (CP) With or Without Vorinostat as Preoperative Systemic Therapy (PST) in HER2-Negative Primary Operable Breast Cancer (TBCRC008). J Clin Oncol (2010) 28(15_suppl):TPS111–1. doi: 10.1200/jco.2010.28.15_suppl.tps111
148. Hoeflich KP, O'Brien C, Boyd Z, Cavet G, Guerrero S, Jung K, et al. In Vivo Antitumor Activity of MEK and Phosphatidylinositol 3-Kinase Inhibitors in Basal-Like Breast Cancer Models. Clin Cancer Res (2009) 15(14):4649–64. doi: 10.1158/1078-0432.CCR-09-0317
149. Johnson GL, Amos KD, Duncan JS, Whittle M, Zawistowki J, Goulet D, et al. Kinome Reprogramming Response to MEK Inhibition: A Window-of-Opportunity Trial in Triple-Negative Breast Cancer (TNBC). Am Soc Clin Oncol (2013) 512–512. doi: 10.1200/jco.2013.31.15_suppl.512
150. Mirzoeva OK, Das D, Heiser LM, Bhattacharya S, Siwak D, Gendelman R, et al. Basal Subtype and MAPK/ERK Kinase (MEK)-Phosphoinositide 3-Kinase Feedback Signaling Determine Susceptibility of Breast Cancer Cells to MEK Inhibition. Cancer Res (2009) 69(2):565–72. doi: 10.1158/0008-5472.CAN-08-3389
151. Roberts PJ, Usary JE, Darr DB, Dillon PM, Pfefferle AD, Whittle MC, et al. Combined PI3K/mTOR and MEK Inhibition Provides Broad Antitumor Activity in Faithful Murine Cancer Models. Clin Cancer Res (2012) 18(19):5290–303. doi: 10.1158/1078-0432.CCR-12-0563
152. Schweber SJ, Rodriguez-LaRocca AG, Calvert V, Petricoin E, Horwitz SB, Andreopoulou E, et al. Protein Pathway Activation Mapping Guided Biomarker Development to Identify Optimal Combinations of MEK Inhibitor With PI3K/mTOR Pathway Inhibitors for the Treatment of Triple-Negative Breast Cancer. Am Soc Clin Oncol (2013) 2612–2612. doi: 10.1200/jco.2013.31.15_suppl.2612
153. Ma CX, Cai S, Li S, Ryan CE, Guo Z, Schaiff WT, et al. Targeting Chk1 in P53-Deficient Triple-Negative Breast Cancer is Therapeutically Beneficial in Human-in-Mouse Tumor Models. J Clin Invest (2012) 122(4):1541–52. doi: 10.1172/JCI58765
154. Chen M-S, Ryan CE, Piwnica-Worms H. Chk1 Kinase Negatively Regulates Mitotic Function of Cdc25A Phosphatase Through 14-3-3 Binding. Mol Cell Biol (2003) 23(21):7488–97. doi: 10.1128/MCB.23.21.7488-7497.2003
155. Stagg J, Allard B. Immunotherapeutic Approaches in Triple-Negative Breast Cancer: Latest Research and Clinical Prospects. Ther Adv Med Oncol (2013) 5(3):169–81. doi: 10.1177/1758834012475152
156. Curtin NJ. PARP Inhibitors and Cancer Therapy. In: Poly (ADP-Ribosyl) Ation. Boston, MA: Springer. (2006). p. 218–33. doi: 10.1007/0-387-36005-0_18
157. Alli E, Sharma VB, Sunderesakumar P, Ford JM. Defective Repair of Oxidative Dna Damage in Triple-Negative Breast Cancer Confers Sensitivity to Inhibition of Poly (ADP-Ribose) Polymerase. Cancer Res (2009) 69(8):3589–96. doi: 10.1158/0008-5472.CAN-08-4016
158. Narod SA. Modifiers of Risk of Hereditary Breast and Ovarian Cancer. Nat Rev Cancer (2002) 2(2):113–23. doi: 10.1038/nrc726
159. Vinayak S, Ford JM. PARP Inhibitors for the Treatment and Prevention of Breast Cancer. Curr Breast Cancer Rep (2010) 2(4):190–7. doi: 10.1007/s12609-010-0026-0
160. Loibl S, O'Shaughnessy J, Untch M, Sikov WM, Rugo HS, McKee MD, et al. Addition of the PARP Inhibitor Veliparib Plus Carboplatin or Carboplatin Alone to Standard Neoadjuvant Chemotherapy in Triple-Negative Breast Cancer (BrighTNess): A Randomised, Phase 3 Trial. Lancet Oncol (2018) 19(4):497–509. doi: 10.1016/S1470-2045(18)30111-6
161. Sikov WM, Berry DA, Perou CM, Singh B, Cirrincione CT, Tolaney SM, et al. Impact of the Addition of Carboplatin and/or Bevacizumab to Neoadjuvant Once-Per-Week Paclitaxel Followed by Dose-Dense Doxorubicin and Cyclophosphamide on Pathologic Complete Response Rates in Stage II to III Triple-Negative Breast Cancer: CALGB 40603 (Alliance). J Clin Oncol (2015) 33(1):13. doi: 10.1200/JCO.2014.57.0572
162. Lord CJ, Ashworth A. PARP Inhibitors: Synthetic Lethality in the Clinic. Science (2017) 355(6330):1152–8. doi: 10.1126/science.aam7344
163. Tutt A, Kaufman B, Garber J, Gelber R, McFadden E, Goessl C, et al. 216tipolympia: A Randomized Phase III Trial of Olaparib as Adjuvant Therapy in Patients With High-Risk HER2-Negative Breast Cancer (BC) and a Germline BRCA1/2 Mutation (gBRCAm). Ann Oncol (2017) 28(suppl_5):p.v67. doi: 10.1093/annonc/mdx362.065
164. Litton J, Scoggins M, Ramirez D, Murthy R, Whitman G, Hess K, et al. A Feasibility Study of Neoadjuvant Talazoparib for Operable Breast Cancer Patients With a Germline BRCA Mutation Demonstrates Marked Activity. NPJ Breast Cancer (2017) 3(1):1–6. doi: 10.1038/s41523-017-0052-4
165. Martín M, Rodríguez-Lescure Á, Ruiz A, Alba E, Calvo L, Ruiz-Borrego M, et al. Molecular Predictors of Efficacy of Adjuvant Weekly Paclitaxel in Early Breast Cancer. Breast Cancer Res Treat (2010) 123(1):149–57. doi: 10.1007/s10549-009-0663-z
166. Sparano JA, Wang M, Martino S, Jones V, Perez EA, Saphner T, et al. Weekly Paclitaxel in the Adjuvant Treatment of Breast Cancer. N Engl J Med (2008) 358(16):1663–71. doi: 10.1056/NEJMoa0707056
167. Farr A, Stolz M, Baumann L, Bago-Horvath Z, Oppolzer E, Pfeiler G, et al. The Effect of Obesity on Pathological Complete Response and Survival in Breast Cancer Patients Receiving Uncapped Doses of Neoadjuvant Anthracycline-Taxane-Based Chemotherapy. Breast (2017) 33:153–8. doi: 10.1016/j.breast.2017.04.001
168. Baselga J, Zambetti M, Llombart-Cussac A, Manikhas G, Kubista E, Steger GG, et al. Phase II Genomics Study of Ixabepilone as Neoadjuvant Treatment for Breast Cancer. J Clin Oncol (2009) 27(4):526–34. doi: 10.1200/JCO.2007.14.2646
169. Pivot XB, Li RK, Thomas ES, Chung H-C, Fein LE, Chan VF, et al. Activity of Ixabepilone in Oestrogen Receptor-Negative and Oestrogen Receptor-Progesterone Receptor-Human Epidermal Growth Factor Receptor 2-Negative Metastatic Breast Cancer. Eur J Cancer (2009) 45(17):2940–6. doi: 10.1016/j.ejca.2009.07.015
170. Onitilo AA, Engel JM, Greenlee RT, Mukesh BN. Breast Cancer Subtypes Based on ER/PR and Her2 Expression: Comparison of Clinicopathologic Features and Survival. Clin Med Res (2009) 7(1-2):4–13. doi: 10.3121/cmr.2008.825
171. Nowak A, Wilcken N, Stockler M, Hamilton A, Ghersi D. Systematic Review of Taxane-Containing Versus non-Taxane-Containing Regimens for Adjuvant and Neoadjuvant Treatment of Early Breast Cancer. Lancet Oncol (2004) 5(6):372–80. doi: 10.1016/S1470-2045(04)01494-9
172. De Laurentiis M, Cancello G, D'Agostino D, Giuliano M, Giordano A, Montagna E, et al. “Taxane-Based Combinations as Adjuvant Chemotherapy of Early Breast Cancer: A Meta-Analysis of Randomized Trials.” In: Database of Abstracts of Reviews of Effects (DARE): Quality-Assessed Reviews. Centre for Reviews and Dissemination (UK) (2008).
173. von Minckwitz G, Blohmer JU, Costa SD, Denkert C, Eidtmann H, Eiermann W, et al. Response-Guided Neoadjuvant Chemotherapy for Breast Cancer. J Clin Oncol (2013) 31(29):3623–30. doi: 10.1200/JCO.2012.45.0940
174. Sparano JA, Zhao F, Martino S, Ligibel JA, Perez EA, Saphner T, et al. Long-Term Follow-Up of the E1199 Phase III Trial Evaluating the Role of Taxane and Schedule in Operable Breast Cancer. J Clin Oncol (2015) 33(21):2353. doi: 10.1200/JCO.2015.60.9271
175. Untch M, Jackisch C, Schneeweiss A, Conrad B, Aktas B, Denkert C, et al. Nab-Paclitaxel Versus Solvent-Based Paclitaxel in Neoadjuvant Chemotherapy for Early Breast Cancer (GeparSepto—GBG 69): A Randomised, Phase 3 Trial. Lancet Oncol (2016) 17(3):345–56. doi: 10.1016/S1470-2045(15)00542-2
176. Weigelt B, Horlings H, Kreike B, Hayes M, Hauptmann M, Wessels L, et al. Refinement of Breast Cancer Classification by Molecular Characterization of Histological Special Types. J Pathol (2008) 216(2):141–50. doi: 10.1002/path.2407
177. Liedtke C, Mazouni C, Hess KR, André F, Tordai A, Mejia JA, et al. Response to Neoadjuvant Therapy and Long-Term Survival in Patients With Triple-Negative Breast Cancer. J Clin Oncol (2008) 26(8):1275–81. doi: 10.1200/JCO.2007.14.4147
178. Carey LA, Dees EC, Sawyer L, Gatti L, Moore DT, Collichio F, et al. The Triple Negative Paradox: Primary Tumor Chemosensitivity of Breast Cancer Subtypes. Clin Cancer Res (2007) 13(8):2329–34. doi: 10.1158/1078-0432.CCR-06-1109
179. Esserman LJ, Berry DA, DeMichele A, Carey L, Davis SE, Buxton M, et al. Pathologic Complete Response Predicts Recurrence-Free Survival More Effectively by Cancer Subset: Results From the I-SPY 1 TRIAL—CALGB 150007/150012, ACRIN 6657. J Clin Oncol (2012) 30(26):3242. doi: 10.1200/JCO.2011.39.2779
180. Gluz O, Liedtke C, Gottschalk N, Pusztai L, Nitz U, Harbeck N. Triple-Negative Breast Cancer—Current Status and Future Directions. Ann Oncol (2009) 20(12):1913–27. doi: 10.1093/annonc/mdp492
181. Slamon D, Mackey J, Robert N, Crown J, Martin M, Eiremann W, et al. Role of Anthracycline-Based Therapy in the Adjuvant Treatment of Breast Cancer: Efficacy Analyses Determined by Molecular Subtypes of the Disease. In: Breast Cancer Research and Treatment NEW YORK, NY USA: SPRINGER (2007). 106.
182. Gennari A, Sormani MP, Pronzato P, Puntoni M, Colozza M, Pfeffer U, et al. HER2 Status and Efficacy of Adjuvant Anthracyclines in Early Breast Cancer: A Pooled Analysis of Randomized Trials. J Natl Cancer Inst (2008) 100(1):14–20. doi: 10.1093/jnci/djm252
183. Tassone P, Tagliaferri P, Perricelli A, Blotta S, Quaresima B, Martelli M, et al. BRCA1 Expression Modulates Chemosensitivity of BRCA1-Defective HCC1937 Human Breast Cancer Cells. Br J Cancer (2003) 88(8):1285–91. doi: 10.1038/sj.bjc.6600859
184. Turner NC, Tutt AN. Platinum Chemotherapy for BRCA1-Related Breast Cancer: Do We Need More Evidence? BioMed Cent (2012) 14.6:1–3. doi: 10.1186/bcr3332
185. Yardley D, Coleman R, Conte P, Cortes J, Brufsky A, Shtivelband M, et al. Nab-Paclitaxel Plus Carboplatin or Gemcitabine Versus Gemcitabine Plus Carboplatin as First-Line Treatment of Patients With Triple-Negative Metastatic Breast Cancer: Results From the Tnacity Trial. Ann Oncol (2018) 29(8):1763–70. doi: 10.1093/annonc/mdy201
186. Tutt A, Tovey H, Cheang MCU, Kernaghan S, Kilburn L, Gazinska P, et al. Carboplatin in BRCA1/2-Mutated and Triple-Negative Breast Cancer BRCAness Subgroups: The TNT Trial. Nat Med (2018) 24(5):628–37. doi: 10.1038/s41591-018-0009-7
187. Zhang J, Fan M, Xie J, Wang Z, Wang B, Zhang S, et al. Chemotherapy of Metastatic Triple Negative Breast Cancer: Experience of Using Platinum-Based Chemotherapy. Oncotarget (2015) 6(40):43135. doi: 10.18632/oncotarget.5654
188. Gelmon KA, Tischkowitz M, Mackay H, Swenerton K, Robidoux A, Tonkin K, et al. Olaparib in Patients With Recurrent High-Grade Serous or Poorly Differentiated Ovarian Carcinoma or Triple-Negative Breast Cancer: A Phase 2, Multicentre, Open-Label, non-Randomised Study. Lancet Oncol (2011) 12(9):852–61. doi: 10.1016/S1470-2045(11)70214-5
189. Bouwman P, Jonkers J. Molecular Pathways: How can BRCA-Mutated Tumors Become Resistant to PARP Inhibitors? Clin Cancer Res (2014) 20(3):540–7. doi: 10.1158/1078-0432.CCR-13-0225
190. Linderholm B, Hellborg H, Johansson U, Elmberger G, Skoog L, Lehtiö J, et al. Significantly Higher Levels of Vascular Endothelial Growth Factor (VEGF) and Shorter Survival Times for Patients With Primary Operable Triple-Negative Breast Cancer. Ann Oncol (2009) 20(10):1639–46. doi: 10.1093/annonc/mdp062
191. Schneeweiss A, Möbus V, Tesch H, Hanusch C, Denkert C, Lübbe K, et al. Intense Dose-Dense Epirubicin, Paclitaxel, Cyclophosphamide Versus Weekly Paclitaxel, Liposomal Doxorubicin (Plus Carboplatin in Triple-Negative Breast Cancer) for Neoadjuvant Treatment of High-Risk Early Breast Cancer (GeparOcto—GBG 84): A Randomised Phase III Trial. Eur J Cancer (2019) 106:181–92. doi: 10.1016/j.ejca.2018.10.015
192. Pohl-Rescigno E, Hauke J, Rhiem K, Möbus V, Furlanetto J, Denkert C, et al. Germline Mutation Status and Therapy Response in High-Risk Early Breast Cancer: Results of the GeparOcto Study (NCT02125344). Am Soc Clin Oncol (2019) 573–573. doi: 10.1200/JCO.2019.37.15_suppl.573
193. Mohammed RA, Ellis IO, Mahmmod AM, Hawkes EC, Green AR, Rakha EA, et al. Lymphatic and Blood Vessels in Basal and Triple-Negative Breast Cancers: Characteristics and Prognostic Significance. Mod Pathol (2011) 24(6):774–85. doi: 10.1038/modpathol.2011.4
194. Thomssen C, Pierga J-Y, Pritchard KI, Biganzoli L, Cortes-Funes H, Petráková K, et al. First-Line Bevacizumab-Containing Therapy for Triple-Negative Breast Cancer: Analysis of 585 Patients Treated in the ATHENA Study. Oncology (2012) 82(4):218–27. doi: 10.1159/000336892
195. Dua R, Gui G, Isacke C. Endothelial Adhesion Molecules in Breast Cancer Invasion Into the Vascular and Lymphatic Systems. Eur J Surg Oncol (2005) 31(8):824–32. doi: 10.1016/j.ejso.2005.05.015
196. Bockhorn M, Jain RK, Munn LL. Active Versus Passive Mechanisms in Metastasis: Do Cancer Cells Crawl Into Vessels, or are They Pushed? Lancet Oncol (2007) 8(5):444–8. doi: 10.1016/S1470-2045(07)70140-7
197. Gerber B, Loibl S, Eidtmann H, Rezai M, Fasching PA, Tesch H, et al. Neoadjuvant Bevacizumab and Anthracycline–Taxane-Based Chemotherapy in 678 Triple-Negative Primary Breast Cancers; Results From the Geparquinto Study (GBG 44). Ann Oncol (2013) 24(12):2978–84. doi: 10.1093/annonc/mdt361
198. Earl HM, Hiller L, Dunn JA, Blenkinsop C, Grybowicz L, Vallier A-L, et al. Efficacy of Neoadjuvant Bevacizumab Added to Docetaxel Followed by Fluorouracil, Epirubicin, and Cyclophosphamide, for Women With HER2-Negative Early Breast Cancer (ARTemis): An Open-Label, Randomised, Phase 3 Trial. Lancet Oncol (2015) 16(6):656–66. doi: 10.1016/S1470-2045(15)70137-3
199. Creighton CJ, Li X, Landis M, Dixon JM, Neumeister VM, Sjolund A, et al. Residual Breast Cancers After Conventional Therapy Display Mesenchymal as Well as Tumor-Initiating Features. Proc Natl Acad Sci (2009) 106(33):13820–5. doi: 10.1073/pnas.0905718106
200. Balko JM, Cook RS, Vaught DB, Kuba MG, Miller TW, Bhola NE, et al. Profiling of Residual Breast Cancers After Neoadjuvant Chemotherapy Identifies DUSP4 Deficiency as a Mechanism of Drug Resistance. Nat Med (2012) 18(7):1052–9. doi: 10.1038/nm.2795
201. Yoshida T, Ozawa Y, Kimura T, Sato Y, Kuznetsov G, Xu S, et al. Eribulin Mesilate Suppresses Experimental Metastasis of Breast Cancer Cells by Reversing Phenotype From Epithelial–Mesenchymal Transition (EMT) to Mesenchymal–Epithelial Transition (MET) States. Br J Cancer (2014) 110(6):1497–505. doi: 10.1038/bjc.2014.80
202. Hu J, Horwitz SB, McDaid HM. Abstract B165: The Antitumor Efficacy of Eribulin Is Mediated via Suppression of Invasion and Profound Induction of Cell Death in Triple Negative Breast Cancer. Boston, MA. Philadelphia (PA): AACR (2013) 12(11 Suppl):Abstract nr B165. doi: 10.1158/1535-7163.TARG-13-B165
203. Von Minckwitz G, Untch M, Blohmer J-U, Costa SD, Eidtmann H, Fasching PA, et al. Definition and Impact of Pathologic Complete Response on Prognosis After Neoadjuvant Chemotherapy in Various Intrinsic Breast Cancer Subtypes. J Clin Oncol (2012) 30(15):1796–804. doi: 10.1200/JCO.2011.38.8595
204. Buchholz TA, Hill BS, Tucker SL, Frye DK, Kuerer HM, Buzdar AU, et al. Factors Predictive of Outcome in Patients With Breast Cancer Refractory to Neoadjuvant Chemotherapy. Cancer J (2001) 7(5):413–20.
205. Symmans WF, Peintinger F, Hatzis C, Rajan R, Kuerer H, Valero V, et al. Measurement of Residual Breast Cancer Burden to Predict Survival After Neoadjuvant Chemotherapy. J Clin Oncol (2007) 25(28):4414–22. doi: 10.1200/JCO.2007.10.6823
206. Han J, Lim W, You D, Jeong Y, Kim S, Lee JE, et al. Chemoresistance in the Human Triple-Negative Breast Cancer Cell Line MDA-MB-231 Induced by Doxorubicin Gradient is Associated With Epigenetic Alterations in Histone Deacetylase. J Oncol (2019) 2019:12. doi: 10.1155/2019/1345026
207. Chen KG, Sikic BI. Molecular Pathways: Regulation and Therapeutic Implications of Multidrug Resistance. Clin Cancer Res (2012) 18(7):1863–9. doi: 10.1158/1078-0432.CCR-11-1590
208. VanKlompenberg MK, Leyden E, Arnason AH, Zhang J-T, Stefanski CD, Prosperi JR. APC Loss in Breast Cancer Leads to Doxorubicin Resistance via STAT3 Activation. Oncotarget (2017) 8(61):102868. doi: 10.18632/oncotarget.22263
209. Liu Y, Li P-K, Li C, Lin J. Inhibition of STAT3 Signaling Blocks the Anti-Apoptotic Activity of IL-6 in Human Liver Cancer Cells. J Biol Chem (2010) 285(35):27429–39. doi: 10.1074/jbc.M110.142752
210. Cheng M, Cai W, Huang W, Chen Y, Wu Z, Luo P, et al. Histone Deacetylase 6 Regulated Expression of IL-8 Is Involved in the Doxorubicin (Dox) Resistance of Osteosarcoma Cells via Modulating ABCB1 Transcription. Eur J Pharmacol (2018) 840:1–8. doi: 10.1016/j.ejphar.2018.09.032
211. Mauriac L, Durand M, Avril A, Dilhuydy J-M. Effects of Primary Chemotherapy in Conservative Treatment of Breast Cancer Patients With Operable Tumors Larger Than 3 Cm: Results of a Randomized Trial in a Single Centre. Ann Oncol (1991) 2(5):347–54. doi: 10.1093/oxfordjournals.annonc.a057953
212. Fisher B, Bryant J, Wolmark N, Mamounas E, Brown A, Fisher ER, et al. Effect of Preoperative Chemotherapy on the Outcome of Women With Operable Breast Cancer. J Clin Oncol (1998) 16(8):2672–85. doi: 10.1200/JCO.1998.16.8.2672
213. Mauriac L, MacGrogan G, Avril A, Durand M, Floquet A, Debled M, et al. Neoadjuvant Chemotherapy for Operable Breast Carcinoma Larger Than 3 Cm: A Unicentre Randomized Trial With a 124-Month Median Follow-Up. Ann Oncol (1999) 10(1):47–52. doi: 10.1023/A:1008337009350
214. Wolmark N, Wang J, Mamounas E, Bryant J, Fisher B. Preoperative Chemotherapy in Patients With Operable Breast Cancer: Nine-Year Results From National Surgical Adjuvant Breast and Bowel Project B-18. JNCI Monogr (2001) 30):96–102. doi: 10.1093/oxfordjournals.jncimonographs.a003469
215. Suman VJ, Ellis MJ, Ma CX. The ALTERNATE Trial: Assessing a Biomarker Driven Strategy for the Treatment of Post-Menopausal Women With ER+/Her2– Invasive Breast Cancer. Chin Clin Oncol (2015) 4(3):34. doi: 10.3978/j.issn.2304-3865.2015.09.01
217. Royce ME, Osman D. Everolimus in the Treatment of Metastatic Breast Cancer. Breast Cancer: Basic Clin Res (2015) 9:S29268. doi: 10.4137/BCBCR.S29268
218. Dowsett M, Jacobs S, Johnston S, Bliss J, Wheatley D, Holcombe C, et al. PALLET: A Neoadjuvant Study to Compare the Clinical and Antiproliferative Effects of Letrozole With and Without Palbociclib. In: Cancer Research, vol. vol 4. Amer Assoc Can Res. Philadelphia, PA (2019). doi: 10.1158/1538-7445.SABCS18-GS3-02
219. Hartkopf AD, Müller V, Wöckel A, Lux MP, Janni W, Nabieva N, et al. Update Mammakarzinom 2019 Teil 1–Implementierung Der Ergebnisse Neuer Studienkonzepte Beim Frühen Mammakarzinom in Die Klinische Praxis. Senologie-Zeitschrift für Mammadiagnostik und-therapie (2019) 16(01):33–44. doi: 10.1055/a-0982-9861
220. Pernas S, Tolaney SM, Winer EP, Goel S. CDK4/6 Inhibition in Breast Cancer: Current Practice and Future Directions. Ther Adv Med Oncol (2018) 10:1758835918786451. doi: 10.1177/1758835918786451
221. Group EBCTC. Effects of Radiotherapy and Surgery in Early Breast Cancer—an Overview of the Randomized Trials. N Engl J Med (1995) 333(22):1444–56. doi: 10.1056/NEJM199511303332202
222. Simpson ER, Ackerman GE, Smith ME, Mendelson CR. Estrogen Formation in Stromal Cells of Adipose Tissue of Women: Induction by Glucocorticosteroids. Proc Natl Acad Sci (1981) 78(9):5690–4. doi: 10.1073/pnas.78.9.5690
223. Miller W. Aromatase and the Breast: Regulation and Clinical Aspects. Maturitas (2006) 54(4):335–41. doi: 10.1016/j.maturitas.2006.04.020
224. Ma CX, Adjei AA, Salavaggione OE, Coronel J, Pelleymounter L, Wang L, et al. Human Aromatase: Gene Resequencing and Functional Genomics. Cancer Res (2005) 65(23):11071–82. doi: 10.1158/0008-5472.CAN-05-1218
225. Miller WR. Biological Rationale for Endocrine Therapy in Breast Cancer. Best Pract Res Clin Endocrinol Metab (2004) 18(1):1–32. doi: 10.1016/s1521-690x(03)00044-7
226. Deeks ED, Scott LJ. Exemestane: A Review of its Use in Postmenopausal Women With Breast Cancer. Drugs (2009) 69(7):889–918. doi: 10.2165/00003495-200969070-00007
227. Pritchard KI, Burris HA, Ito Y, Rugo HS, Dakhil S, Hortobagyi GN, et al. Safety and Efficacy of Everolimus With Exemestane vs. Exemestane Alone in Elderly Patients With HER2-Negative, Hormone Receptor-Positive Breast Cancer in BOLERO-2. Clin Breast Cancer (2013) 13(6):421–432 e428. doi: 10.1016/j.clbc.2013.08.011
228. Greene GL, Sobel NB, King W, Jensen EV. Immunochemical Studies of Estrogen Receptors. J Steroid Biochem (1984) 20(1):51–6. doi: 10.1016/0022-4731(84)90188-2
229. Osborne CK. Tamoxifen in the Treatment of Breast Cancer. N Engl J Med (1998) 339(22):1609–18. doi: 10.1056/nejm199811263392207
230. Jordan VC. Tamoxifen: A Most Unlikely Pioneering Medicine. Nat Rev Drug Discov (2003) 2(3):205–13. doi: 10.1038/nrd1031
231. Mandlekar S, Kong ANT. Mechanisms of Tamoxifen-Induced Apoptosis. Apoptosis (2001) 6(6):469–77. doi: 10.1023/A:1012437607881
232. Sukocheva O, Wang L, Verrier E, Vadas MA, Xia P. Restoring Endocrine Response in Breast Cancer Cells by Inhibition of the Sphingosine Kinase-1 Signaling Pathway. Endocrinology (2009) 150(10):4484–92. doi: 10.1210/en.2009-0391
233. Slamon DJ, Clark GM, Wong SG, Levin WJ, Ullrich A, McGuire WL. Human Breast Cancer: Correlation of Relapse and Survival With Amplification of the HER-2/Neu Oncogene. Science (1987) 235(4785):177–82. doi: 10.1126/science.3798106
234. Browne BC, O'Brien N, Duffy MJ, Crown J, O'Donovan N. HER-2 Signaling and Inhibition in Breast Cancer. Curr Cancer Drug Targets (2009) 9(3):419–38. doi: 10.2174/156800909788166484
235. Carter P, Presta L, Gorman CM, Ridgway JB, Henner D, Wong WL, et al. Humanization of an Anti-P185her2 Antibody for Human Cancer Therapy. Proc Natl Acad Sci U S A (1992) 89(10):4285–9. doi: 10.1073/pnas.89.10.4285
236. Slamon DJ, Leyland-Jones B, Shak S, Fuchs H, Paton V, Bajamonde A, et al. Use of Chemotherapy Plus a Monoclonal Antibody Against HER2 for Metastatic Breast Cancer That Overexpresses Her2. N Engl J Med (2001) 344(11):783–92. doi: 10.1056/nejm200103153441101
237. Sharma GN, Dave R, Sanadya J, Sharma P, Sharma KK. Various Types and Management of Breast Cancer: An Overview. J Adv Pharm Technol Res (2010) 1(2):109–26.
238. Lu P, Beeraka NM, Zhang J, Gu Y, Fan R, Liu J, et al. Efficacy of Da Vinci Robot-Assisted Lymph Node Surgery Than Conventional Axillary Lymph Node Dissection in Breast Cancer–A Comparative Study. Int J Med Robot Comput Assist Surg (2021) 17(6):e2307. doi: 10.1002/rcs.2307
239. Veronesi U, Cascinelli N, Mariani L, Greco M, Saccozzi R, Luini A, et al. Twenty-Year Follow-Up of a Randomized Study Comparing Breast-Conserving Surgery With Radical Mastectomy for Early Breast Cancer. N Engl J Med (2002) 347(16):1227–32. doi: 10.1056/NEJMoa020989
240. Bleicher RJ, Ruth K, Sigurdson ER, Beck JR, Ross E, Wong YN, et al. Time to Surgery and Breast Cancer Survival in the United States. JAMA Oncol (2016) 2(3):330–9. doi: 10.1001/jamaoncol.2015.4508
241. Mikhaleva LM, Solomatina AA, Milovanov AP, Beeraka NM, Khovanskaya TN, Chabieva LB, et al. Histomorphological and Functional Features of the Eutopic Endometrium in Patients With Ovarian Endometriosis After Surgery—a Clinical Study. Reprod Sci (2021) 28(8):2350–58. doi: 10.1007/s43032-021-00508-3
242. Early Breast Cancer Trialists’ Collaborative Group. Ovarian Ablation for Early Breast Cancer (2000). Cochrane Database Syst Rev. 1998(2):Cd000485. doi: 10.1002/14651858.Cd000485
243. McNeely ML, Campbell KL, Rowe BH, Klassen TP, Mackey JR, Courneya KS. Effects of Exercise on Breast Cancer Patients and Survivors: A Systematic Review and Meta-Analysis. CMAJ (2006) 175(1):34–41. doi: 10.1503/cmaj.051073
244. Daley AJ, Crank H, Saxton JM, Mutrie N, Coleman R, Roalfe A. Randomized Trial of Exercise Therapy in Women Treated for Breast Cancer. J Clin Oncol (2007) 25(13):1713–21. doi: 10.1200/JCO.2006.09.5083
245. Courneya KS, Segal RJ, Mackey JR, Gelmon K, Reid RD, Friedenreich CM, et al. Effects of Aerobic and Resistance Exercise in Breast Cancer Patients Receiving Adjuvant Chemotherapy: A Multicenter Randomized Controlled Trial. J Clin Oncol (2007) 25(28):4396–404. doi: 10.1200/JCO.2006.08.2024
246. Schmitz KH, Courneya KS, Matthews C, Demark-Wahnefried W, Galvão DA, Pinto BM, et al. American College of Sports Medicine Roundtable on Exercise Guidelines for Cancer Survivors. Med Sci Sports Exerc (2010) 42(7):1409–26. doi: 10.1249/MSS.0b013e3181e0c112
247. Oberbach A, Tönjes A, Klöting N, Fasshauer M, Kratzsch, Busse MW, et al. Effect of a 4 Week Physical Training Program on Plasma Concentrations of Inflammatory Markers in Patients With Abnormal Glucose Tolerance. Eur J Endocrinol (2006) 154(4):577–85. doi: 10.1530/eje.1.02127
248. Schwartz AL. Daily Fatigue Patterns and Effect of Exercise in Women With Breast Cancer. Cancer Pract (2000) 8(1):16–24. doi: 10.1046/j.1523-5394.2000.81003.x
249. Young-McCaughan S, Sexton DL. A Retrospective Investigation of the Relationship Between Aerobic Exercise and Quality of Life in Women With Breast Cancer. Oncol Nurs Forum (1991) 18(4):751–7.
250. McNeely ML, Campbell K, Ospina M, Rowe BH, Dabbs K, Klassen TP, et al. Exercise Interventions for Upper-Limb Dysfunction Due to Breast Cancer Treatment. Cochrane Database Syst Rev (2010) 6(6):CD005211. doi: 10.1002/14651858.CD005211.pub2
251. Brdareski Z, Đurović A, Šušnjar S, Životić-Vanović M, Ristić A, Konstantinović L, et al. Effects of a Short-Term Differently Dosed Aerobic Exercise on Maximum Aerobic Capacity in Breast Cancer Survivors: A Pilot Study. Vojnosanit Pregl (2012) 69(3):237–42. doi: 10.2298/VSP101117004B
252. Milne HM, Wallman KE, Gordon S, Courneya KS. Effects of a Combined Aerobic and Resistance Exercise Program in Breast Cancer Survivors: A Randomized Controlled Trial. Breast Cancer Res Treat (2008) 108(2):279–88. doi: 10.1007/s10549-007-9602-z
253. Markes M, Brockow T, Resch KL. Exercise for Women Receiving Adjuvant Therapy for Breast Cancer. Cochrane Database Syst Rev (2006) 4. doi: 10.1002/14651858.CD005001.pub2
254. Patterson RE, Cadmus LA, Emond JA, Pierce JP. Physical Activity, Diet, Adiposity and Female Breast Cancer Prognosis: A Review of the Epidemiologic Literature. Maturitas (2010) 66(1):5–15. doi: 10.1016/j.maturitas.2010.01.004
255. Chlebowski RT, Aiello E, McTiernan A. Weight Loss in Breast Cancer Patient Management. J Clin Oncol (2002) 20(4):1128–43. doi: 10.1200/JCO.2002.20.4.1128
256. Van den Brandt PA, Spiegelman D, Yaun S-S, Adami H-O, Beeson L, Folsom AR, et al. Pooled Analysis of Prospective Cohort Studies on Height, Weight, and Breast Cancer Risk. Am J Epidemiol (2000) 152(6):514–27. doi: 10.1093/aje/152.6.514
257. Boyd NF, Campbell JE, Germanson T, Thomson DB, Sutherland DJ, Meakin JW. Body Weight and Prognosis in Breast Cancer. J Natl Cancer Inst (1981) 67(4):785–9. doi: 10.1093/jnci/67.4.785
258. Helyer LK, Varnic M, Le LW, Leong W, McCready D. Obesity Is a Risk Factor for Developing Postoperative Lymphedema in Breast Cancer Patients. Breast J (2010) 16(1):48–54. doi: 10.1111/j.1524-4741.2009.00855.x
259. Chen K, Sinelnikov MY, Reshetov IV, Timashev P, Gu Y, Mu L, et al. Therapeutic Potential of Mesenchymal Stem Cells for Postmastectomy Lymphedema: A Literature Review. Clin Trans Sci (2021) 14(1):54–61. doi: 10.1111/cts.12864
260. Harvie M, Howell A, Evans DG. Can Diet and Lifestyle Prevent Breast Cancer: What is the Evidence? Am Soc Clin Oncol Educ Book (2015) 35(1):e66–73. doi: 10.14694/EdBook_AM.2015.35.e66
261. Carpenter CL, Duvall K, Jardack P, Li L, Henning SM, Li Z, et al. Weight Loss Reduces Breast Ductal Fluid Estrogens in Obese Postmenopausal Women: A Single Arm Intervention Pilot Study. Nutr J (2012) 11(1):102. doi: 10.1186/1475-2891-11-102
262. Travier N, Fonseca-Nunes A, Javierre C, Guillamo E, Arribas L, Peiro I, et al. Effect of a Diet and Physical Activity Intervention on Body Weight and Nutritional Patterns in Overweight and Obese Breast Cancer Survivors. Med Oncol (2014) 31(1):783. doi: 10.1007/s12032-013-0783-5
263. Dieli-Conwright CM, Courneya KS, Demark-Wahnefried W, Sami N, Lee K, Sweeney FC, et al. Aerobic and Resistance Exercise Improves Physical Fitness, Bone Health, and Quality of Life in Overweight and Obese Breast Cancer Survivors: A Randomized Controlled Trial. Breast Cancer Res (2018) 20(1):124. doi: 10.1186/s13058-018-1051-6
264. Yanovski SZ, Yanovski JA. Long-Term Drug Treatment for Obesity: A Systematic and Clinical Review. Jama (2014) 311(1):74–86. doi: 10.1001/jama.2013.281361
265. O'Brien PE. Bariatric Surgery: Mechanisms, Indications and Outcomes. J Gastroenterol Hepatol (2010) 25(8):1358–65. doi: 10.1111/j.1440-1746.2010.06391.x
266. Aune D, Chan D, Greenwood D, Vieira A, Rosenblatt DN, Vieira R, et al. Dietary Fiber and Breast Cancer Risk: A Systematic Review and Meta-Analysis of Prospective Studies. Ann Oncol (2012) 23(6):1394–402. doi: 10.1093/annonc/mdr589
267. Kwan ML, Weltzien E, Kushi LH, Castillo A, Slattery ML, Caan BJ. Dietary Patterns and Breast Cancer Recurrence and Survival Among Women With Early-Stage Breast Cancer. J Clin Oncol (2009) 27(6):919. doi: 10.1200/JCO.2008.19.4035
268. Roberts CK, Won D, Pruthi S, Kurtovic S, Sindhu RK, Vaziri ND, et al. Effect of a Short-Term Diet and Exercise Intervention on Oxidative Stress, Inflammation, MMP-9, and Monocyte Chemotactic Activity in Men With Metabolic Syndrome Factors. J Appl Physiol (2006) 100(5):1657–65. doi: 10.1152/japplphysiol.01292.2005
269. Prentice RL, Caan B, Chlebowski RT, Patterson R, Kuller LH, Ockene JK, et al. Low-Fat Dietary Pattern and Risk of Invasive Breast Cancer: The Women's Health Initiative Randomized Controlled Dietary Modification Trial. Jama (2006) 295(6):629–42. doi: 10.1001/jama.295.6.629
270. Suzuki R, Orsini N, Mignone L, Saji S, Wolk A. Alcohol Intake and Risk of Breast Cancer Defined by Estrogen and Progesterone Receptor Status—a Meta-Analysis of Epidemiological Studies. Int J Cancer (2008) 122(8):1832–41. doi: 10.1002/ijc.23184
271. Scoccianti C, Lauby-Secretan B, Bello P-Y, Chajes V, Romieu I. Female Breast Cancer and Alcohol Consumption: A Review of the Literature. Am J Prev Med (2014) 46(3, Supplement 1):S16–25. doi: 10.1016/j.amepre.2013.10.031
272. Knekt P, Järvinen R, Seppänen R, Pukkala E, Aromaa A. Intake of Dairy Products and the Risk of Breast Cancer. Br J Cancer (1996) 73(5):687–91. doi: 10.1038/bjc.1996.119
273. Dong J-Y, Zhang L, He K, Qin L-Q. Dairy Consumption and Risk of Breast Cancer: A Meta-Analysis of Prospective Cohort Studies. Breast Cancer Res Treat (2011) 127(1):23–31. doi: 10.1007/s10549-011-1467-5
274. Shin M-H, Holmes MD, Hankinson SE, Wu K, Colditz GA, Willett WC. Intake of Dairy Products, Calcium, and Vitamin D and Risk of Breast Cancer. J Natl Cancer Inst (2002) 94(17):1301–10. doi: 10.1093/jnci/94.17.1301
275. McCullough ML, Rodriguez C, Diver WR, Feigelson HS, Stevens VL, Thun MJ, et al. Dairy, Calcium, and Vitamin D Intake and Postmenopausal Breast Cancer Risk in the Cancer Prevention Study II Nutrition Cohort. Cancer Epidemiol Prev Biomarkers (2005) 14(12):2898–904. doi: 10.1158/1055-9965.EPI-05-0611
276. Finkeldey L, Schmitz E, Ellinger S. Effect of the Intake of Isoflavones on Risk Factors of Breast Cancer—A Systematic Review of Randomized Controlled Intervention Studies. Nutrients (2021) 13(7):2309. doi: 10.3390/nu13072309
277. Velicer CM, Ulrich CM. Vitamin and Mineral Supplement Use Among US Adults After Cancer Diagnosis: A Systematic Review. J Clin Oncol (2008) 26(4):665–73. doi: 10.1200/JCO.2007.13.5905
278. Neuhouser ML, Sorensen B, Hollis BW, Ambs A, Ulrich CM, McTiernan A, et al. Vitamin D Insufficiency in a Multiethnic Cohort of Breast Cancer Survivors. Am J Clin Nutr (2008) 88(1):133–9. doi: 10.1093/ajcn/88.1.133
279. Chlebowski RT, Johnson KC, Kooperberg C, Pettinger M, Wactawski-Wende J, Rohan T, et al. Calcium Plus Vitamin D Supplementation and the Risk of Breast Cancer. J Natl Cancer Inst (2008) 100(22):1581–91. doi: 10.1093/jnci/djn360
280. Cassidy A. Are Herbal Remedies and Dietary Supplements Safe and Effective for Breast Cancer Patients? Breast Cancer Res (2003) 5(6):300–2. doi: 10.1186/bcr724
281. Rock CL, Doyle C, Demark-Wahnefried W, Meyerhardt J, Courneya KS, Schwartz AL, et al. Nutrition and Physical Activity Guidelines for Cancer Survivors. CA Cancer J Clin (2012) 62(4):243–74. doi: 10.3322/caac.21142
282. Study GBoD. Global Burden of Disease Study 2015 (GBD 2015) Obesity and Overweight Prevalence 1980–2015. In: Institute for Health Metrics and Evaluation (IHME) Seattle. United States (2017).
283. Chooi YC, Ding C, Magkos F. The Epidemiology of Obesity. Metabolism (2019) 92:6–10. doi: 10.1016/j.metabol.2018.09.005
284. Ge S, Wang Z, Wang B, Ma X.. Common Multiple Primary Cancers Associated With Breast and Gynecologic Cancers and Their Risk Factorss, Pathogenesis Treatments and Prognosis: A Review. doi: 10.3389/fonc.2022.840431
285. Fang X, Zhang J, Roman RJ, Fan F.. Fang X, Zhang J, Roman RJ, Fan F. From 1901 to 2022, How Far Are We From Truly Understanding teh Pathogensis of Age-Related Dementia? GeroScience (2022). doi: 10.1007/s11357-022-00591-7
Keywords: breast cancer, obesity, oncogenic signaling, inflammation, estrogen, neoadjuvant therapy, preventive measures
Citation: Chen K, Zhang J, Beeraka NM, Tang C, Babayeva YV, Sinelnikov MY, Zhang X, Zhang J, Liu J, Reshetov IV, Sukocheva OA, Lu P and Fan R (2022) Advances in the Prevention and Treatment of Obesity-Driven Effects in Breast Cancers. Front. Oncol. 12:820968. doi: 10.3389/fonc.2022.820968
Received: 20 January 2022; Accepted: 16 May 2022;
Published: 22 June 2022.
Edited by:
Maria Ida Amabile, Sapienza University of Rome, ItalyReviewed by:
Patrizia Pasanisi, National Cancer Institute Foundation (IRCCS), ItalyTakahiro Kogawa, Cancer Institute Hospital of Japanese Foundation for Cancer Research, Japan
Copyright © 2022 Chen, Zhang, Beeraka, Tang, Babayeva, Sinelnikov, Zhang, Zhang, Liu, Reshetov, Sukocheva, Lu and Fan. This is an open-access article distributed under the terms of the Creative Commons Attribution License (CC BY). The use, distribution or reproduction in other forums is permitted, provided the original author(s) and the copyright owner(s) are credited and that the original publication in this journal is cited, in accordance with accepted academic practice. No use, distribution or reproduction is permitted which does not comply with these terms.
*Correspondence: Ruitai Fan, ZmNjZmFucnRAenp1LmVkdS5jbg==; UnVpdGFpZmFuMjAwOEBnbWFpbC5jb20=; Pengwei Lu, bHZwZW5nd2VpMTk4M0Bob3RtYWlsLmNvbQ==
†ORCID: Kuo Chen, orcid.org/0000-0003-0095-8545
Jin Zhang, orcid.org/0000-0002-2566-7501
Chengyun Tang, orcid.org/0000-0001-7536-9740
Mikhail Y. Sinelnikov, orcid.org/0000-0002-0862-6011
Jiacheng Zhang, orcid.org/0000-0001-7024-2126
Xinliang Zhang, orcid.org/0000-0003-0625-4346
‡These authors have contributed equally to this work