- 1Institute of Modern Physics, Chinese Academy of Sciences, Lanzhou, China
- 2Department of Radiation Medicine, School of Basic Medical Sciences, Peking University Health Science Center, Beijing, China
- 3Huizhou Research Center of Ion Science, Chinese Academy of Sciences, Huizhou, China
- 4School of Nuclear Science and Technology, University of Chinese Academy of Sciences, Beijing, China
Proton and heavy ion therapy offer superior relative biological effectiveness (RBE) in the treatment of deep-seated tumors compared with conventional photon radiotherapy due to its Bragg-peak feature of energy deposition in organs. Many proton and carbon ion therapy centers are active all over the world. At present, five particle radiotherapy institutes have been built and are receiving patient in China, mainly including Wanjie Proton Therapy Center (WPTC), Shanghai Proton Heavy Ion Center (SPHIC), Heavy Ion Cancer Treatment Center (HIMM), Chang Gung Memorial Hospital (CGMH), and Ruijin Hospital affiliated with Jiao Tong University. Many cancer patients have benefited from ion therapy, showing unique advantages over surgery and chemotherapy. By the end of 2020, nearly 8,000 patients had been treated with proton, carbon ion or carbon ion combined with proton therapy. So far, there is no systemic review for proton and carbon ion therapy facility and clinical outcome in China. We reviewed the development of proton and heavy ion therapy, as well as providing the representative clinical data and future directions for particle therapy in China. It has important guiding significance for the design and construction of new particle therapy center and patients’ choice of treatment equipment.
Introduction
According to the latest Statistics on Cancer, there were an estimated 19.29 million new cases and 9.96 million cancer deaths worldwide in 2020 (1). Among them, 4.57 million new cancer cases and 3 million deaths were reported in China. Surgery, radiotherapy (RT) and chemotherapy are the standard methods of cancer treatment (2). RT is one of the most effective manners for the treatment of primary and metastatic solid tumors, microscopic tumor extensions, as well as regional lymph nodes. Conventional radiotherapy (photon or electron) has some disadvantages because of the limitation of tumor location, beam arrangement, technique and modality (3, 4). It is difficult to avoid radiation exposure to important organs and tissues around the tumor, resulting in certain short-term and long-term complications and sequelae. These radiation complications seriously affect the quality of life of patients. In order to ensure that the radiation dose of normal organs and tissues around the tumor does not exceed the tolerance dose, the radiation dose to target has to be reduced, resulting in the reduction of local control rate (5).
In recent decades, particle radiotherapy has been developed in clinical practice in United States, Japan, Germany and other countries, including proton beam and heavy ion (mainly using carbon ions). According to data released by Particle Therapy Co-Operative Group (PTCOG), more than 290,000 patients have been treated worldwide with particle therapy by the end of 2020, including almost 250,000 with protons and 40,000 with carbon-ions (6). Consensus Guidelines for pencil-beam scanning proton beam therapy (PBT), particularly intensity modulated PBT for thoracic malignancies and for Prostate Cancer mark the growing acceptance of proton therapy as a standard treatment for these tumor (7, 8). Fascinated by those results, China began to explore the development of proton radiotherapy since 1996. In the past more than 20 years, several proton projects have been put on the agenda, but some of them have been stopped due to capital chain rupture or high maintenance cost. Even so, several particle radiotherapy institutes have been built and are receiving patient in China, mainly including WPTC (Wanjie, Zi-Bo), Shanghai Proton Heavy Ion Center (SPHIC, Shanghai), Heavy Ion Cancer Treatment Center (HIMM, Wuwei, Gansu), and Chang Gung Memorial Hospital (CGMH, Linkou and Kaohsiung). Ruijin Hospital also established a proton center at the end of 2021. China was the fourth country, of the now five countries, using carbon ion therapy. Recently, ion therapy guideline (Version 2020) based on the latest research data has been formulated, which guides the clinical practice of ion therapy in China and promote the popularization and application of ion therapy (9). However, there is currently no systematic review of particle therapy equipment and treatment outcomes in China. In this review, we will briefly summarize the history and current situation of the development of PBT and carbon ion radiotherapy (CIRT) in China, analyze the clinical cases, and discuss the future development and challenges of proton and heavy ion equipment in China based on national circumstances and the current situation of ion radiotherapy.
Physical and Radiobiological Basis of Proton ad Carbon Ion Radiotherapy
Physical Characteristics
The advantages of the proton and heavy ion medical accelerator are mainly reflected in the Bragg Peak distribution of the linear energy transfer (LET) of high-energy particle beams in human tissues. Thanks to this characteristic in human tissues, we can use this property to selectively deposit energy mainly in the tumor sites, while less depositing in normal tissues, so as to eliminate cancer without damaging or less damaging normal tissues (10). Several treatment planning studies between photon, proton, and carbon ion radiotherapy were carried out in China. Those three modalities achieved similar levels of target conformation. However, PBT and CIRT significantly reduced the OARs dose in the treatment of locally recurrent nasopharyngeal carcinoma (LR-NPC) (11, 12).
Although the physical properties of carbon ions and protons are very similar, carbon ion radiotherapy has its own characteristics compared with protons. (i) transverse scattering and range straggling are relatively small, which means smaller beam halo (13); (ii) Compared with proton beams, heavy ion beams require higher energy to reach deep tumors. Therefore, manufacturing carbon ion equipment requires larger accelerators and beam delivery systems (14).
Radiobiological Characteristics
Relative biological effectiveness (RBE) is the ratio of absorbed doses of two radiations required to produce the same biologic effect (15). RBE is affected by the ion species, dose, LET, cell and tissue type, biological endpoint and other factors (16). Within a certain range, RBE increases with the increase of LET (17). Compared with photon or PBT, CIRT has a higher LET, and the estimated RBE of CIRT has a 2 to 5 larger times RBE than photon RT depending on the irradiated tissues and cells (18, 19). The Bragg peak region of the carbon ion beam appears at the same position as the RBE peak region, which allows the carbon ion beam energy to act on the target region to the maximum, while the normal tissues that the beam passes through are less affected (20, 21). Carbon ions use their biological properties to cause severe clustered DNA double-strand breaks to kill tumor cells (22) (Figure 1). At the front or tail of the Bragg peak, most normal cells are repaired through single-strand or double-strand break repair mechanisms. The detailed mechanism of ionizing radiation induced DNA damage repair has been discussed in previous publication (23). In addition, there are some reports that these radiations, especially secondary neutron, may induce secondary tumors (24).
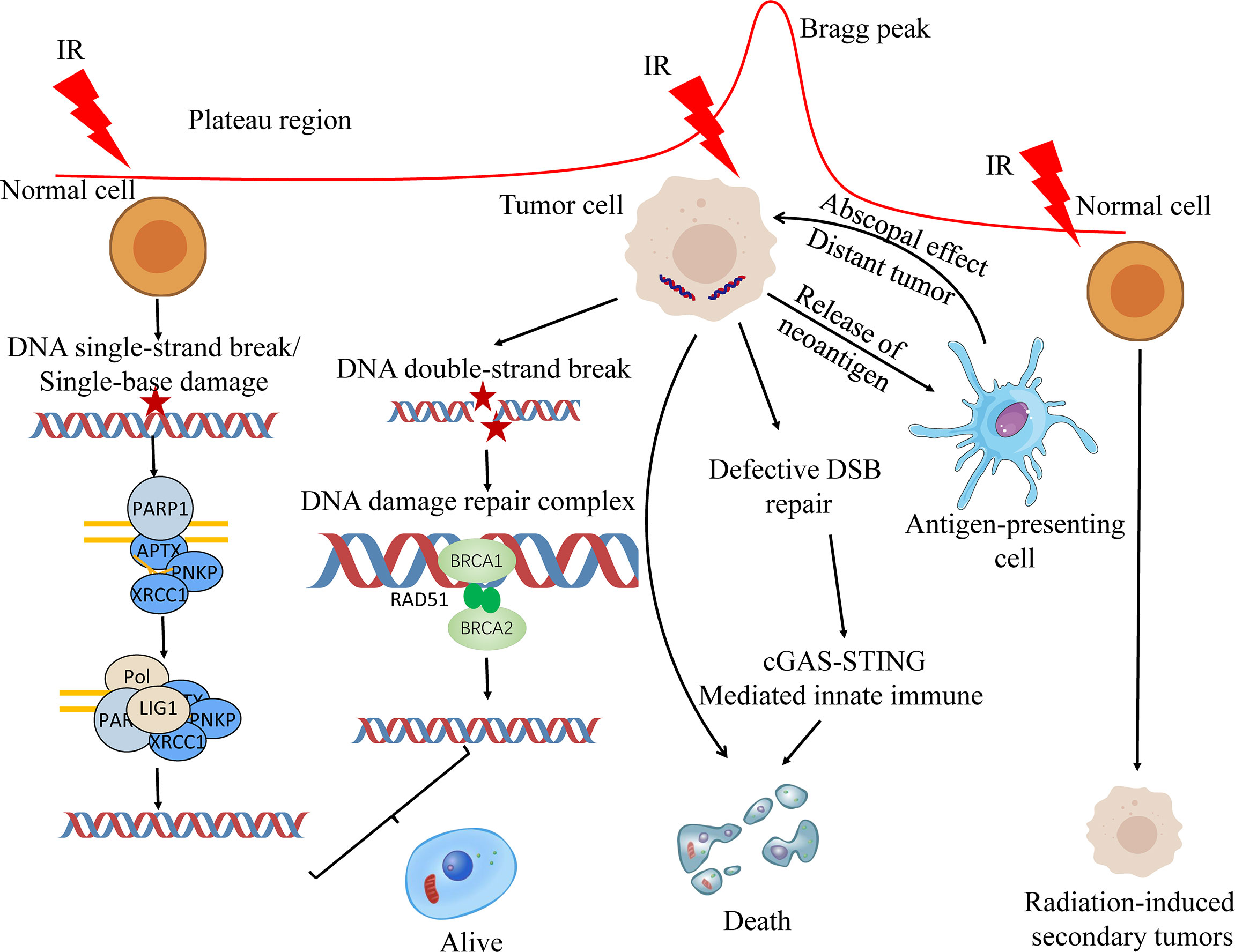
Figure 1 Biological principles of proton and carbon ion radiotherapy. Ionizing radiation primarily causes single-base damage, single strand break damage, and double strand break damage. Proton and carbon ion beams can cause serious damage to tumor cells by their unique Bragg peak, and most of the cells can’t be repaired in time, resulting in apoptosis. The first two types of damage are mainly repaired by the base excision repair (BER) pathway. homologous recombination (HR) and non-homologous end joining (NHEJ) are both involved in the repair of DNA double-strand breaks (DSBs). On the one hand, radiotherapy can kill the DSB repair-deficient cells; on the other hand, the neoantigens produced by radiotherapy can be processed by lymphocytes to produce lethal effects. At the same time, radiotherapy can promote the release of nuclear and mitochondrial DNA, and cells can increase the play of innate immune function through cGAS-STING mediated innate immune, and jointly promote the death of tumor cells.
In addition to high RBE, carbon ions also have a lower Oxygen Enhancement Ratio (OER). OER refers to the ratio of the dose required for hypoxic cells and normoxic cells to produce the same biological effect. Due to the rapid growth of tumor cells, the surrounding necrotic tissue hinders the supply of oxygen, so the tumor cells are in a state of hypoxia. Photons, X-rays, and protons have higher OERs and lower lethality to hypoxic tumor cells than carbon ions (25). At present, there have many pre-clinical radiobiology facilities to do in-vitro and in-vivo studies in China (Table 1).
Proton and Carbon Ion Therapy Facilities
Up to December 2021, there are 107 proton and heavy ion therapy centers in operation all over the worldwide based on PTCOG data (26). However, there are only twelve operational carbon ion therapy centers worldwide. The availability of heavy ion treatment is quite limited worldwide due to the high requirement of related technological research and the costly construction of medically dedicated accelerator and support systems (27). The heavy ion equipment is large in size, high in installation and operating costs, and the rotating gantry treatment technology is easier to implement in the proton equipment. At present, the Heidelberg Ion Treatment Center (HIT) in Germany and QST in Japan are the only carbon ion treatment centers in the world with rotating gantry treatment technology (28, 29).
Although there are many proton and heavy ion centers in preparation, only five proton and heavy ion treatment centers are in operation in China (Table 2). The good news is that, eleven and ten proton and heavy ion sites are under construction (Table S1) or in planning stage (Table S2) based on PTCOG data, respectively. In addition to the different particles used in the five particle therapy centers, the main differences are the beam delivery system, rotating gantry, and treatment planning system. Among them, only HIMM is a self-developed medical device in China. In addition, the first proton therapy device (Shanghai Advanced Proton Therapy Device, APTRON) has been independently developed by Shanghai Ruijin Hospital, China (30). Two patients (one for recurrent pituitary adenoma and another for recurrent cranial base chordoma) were treated at Ruijin hospital up to now. As of the date of writing this manuscript, about 8,000 patients in China have received particle therapy.
Proton and heavy ion therapy have gradually developed in the past two decades in China. In 1989, the Institute of Modern Physics (IMP) established the Heavy Ion Research Facility in Lanzhou (HIRFL), and began basic research work on heavy ion therapy for tumors in 1995. To realize the transformation from bench to bedside, a total of 213 patients were treated with CIRT at the HIRFL of IMP from 2006 to 2013, satisfying efficacy and acceptable toxicities has been obtained in several types of tumors (31). Encouraged by the results from the HIRFL of IMP, a heavy ion therapy demonstration device assigned for clinical use, i.e., the heavy‐ion medical machine (HIMM), has been designed and built independently by the IMP in Wuwei and Lanzhou, Gansu Province, China. In 2019, HIMM completed clinical trials of 47 patients. Since then, proton and heavy ion radiotherapy in China has begun to develop rapidly (Figure 2). The WPTC is the first hospital to carry out proton therapy in China in 2004. Due to the expensive maintenance costs of the equipment, the center ceased operations for a few years and reopened in 2015. Shanghai Proton Heavy Ion center (SPHIC) installed the IONTRIS device (IONTRIS device) manufactured by Siemens in Germany. At the request of the China Food and Drug Administration (CFDA), SPHIC conducted a clinical registration trial for IONTRIS to further verify the safety and efficacy of IONTRIS in the treatment of cancer patients. CGMH has built two particle therapy centers in Taiwan, and received patient in 2015 and 2018, respectively. Those significant peer-reviewed publications in clinical, physics, and biology of particle radiotherapy from these centers have been listed in Table 3.
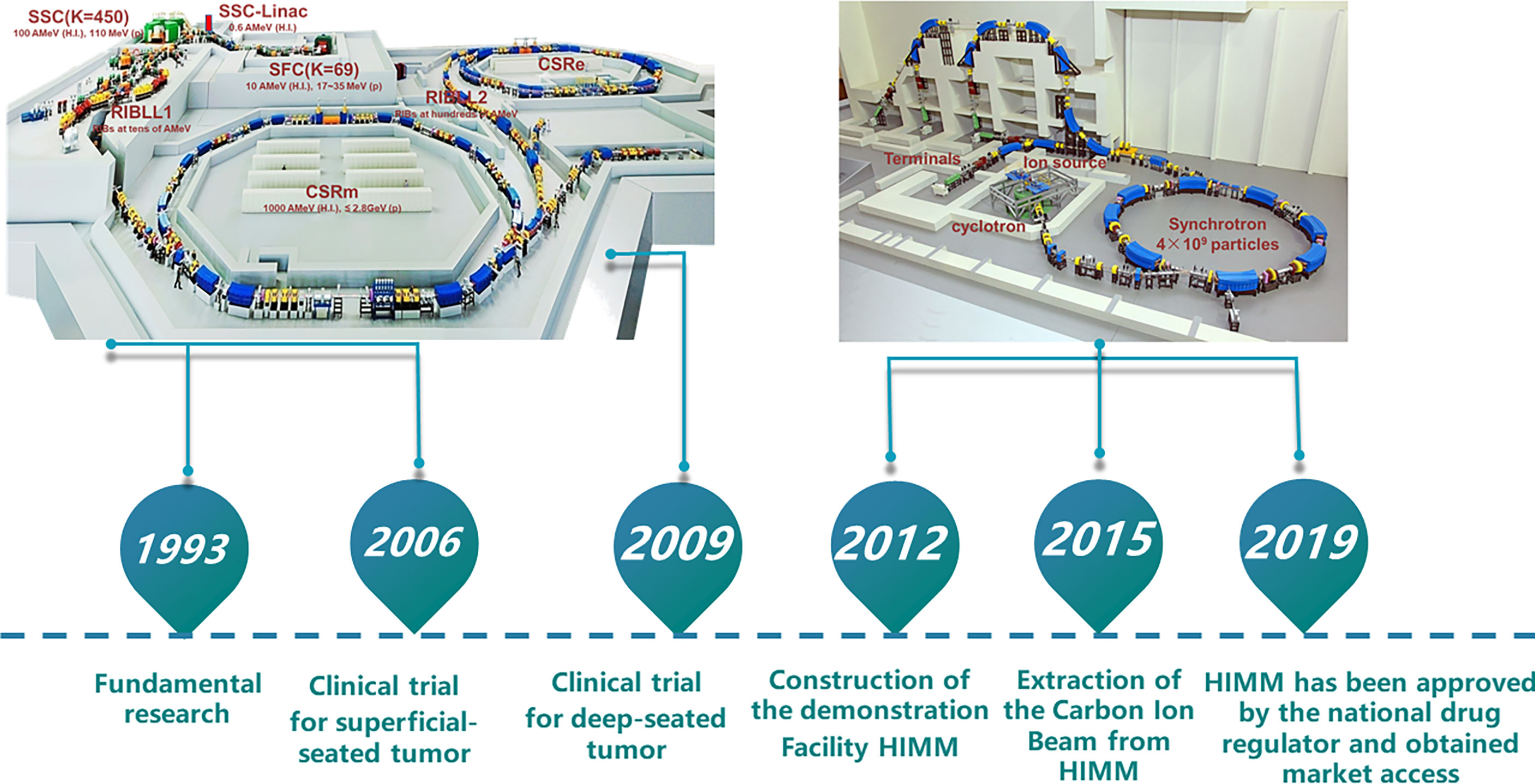
Figure 2 Research roadmap of heavy ion cancer treatment at IMP. The Institute of Modern Physics (IMP) built two cancer treatment terminals on Heavy Ion Research Facility in Lanzhou (HIRFL). Based on continuous research and development of advanced accelerator technology and nuclear detection technology, biological basic research and preliminary clinical trial of CIRT, the IMP has successfully developed HIMM. CSR, Cooler-Storage-Ring; CSRm, CSR main ring; CSRe, CSR experimental ring; RIB, Radioactive Ion Beams; SSC, Separated Sector Cyclotron; SFC, Sector-Focusing Cyclotron; RIBLL1, Radioactive Ion Beam Line 1; RIBLL2, Radioactive Ion Beam Line 2; H.I., Heavy Ions.

Table 3 Publication lists in clinical, physics, and biology of particle radiotherapy from these centers (update Jan 2022).
Treatment planning system (TPS) is the nerve center or command center in radiotherapy process. TPS refers to software designed to help doctors or physicists design and optimize treatment planning. Currently commercially available treatment planning systems are developed by business companies, such as RayStation (RaySearch Medical Laboratories, Stockholm, Sweden), XiO (Elekta., Stockholm, Sweden), Eclipse (Varian Medical Systems, Palo Alto, CA, USA), and Pinnacle (Philips Healthcare, Andover, MA, USA) (93). Conventional radiotherapy started relatively early in China, and it has gradually matured (94). Proton radiotherapy equipment and radiotherapy planning system started late in China. At present, most of the proton therapy centers in operation in China use the commercial TPS. The Syngo treatment planning software system (versions VC11 and VC13; Siemens Healthcare, Erlangen, Germany) was adopted at the SPHIC, which considers local values of the RBE based on the local effect model (LEM) (18). A self-developed heavy ion radiotherapy treatment planning system was adopted at the HIMM (66, 95).
Initial Clinical Outcomes of PBT and CIRT in China
Several clinical practices have confirmed potential advantages of PBT and CIRT (96). Although there are only preliminary clinical data, China has also gradually gained experience on the basis of clinical trials. Up to date, fifteen observational or interventional clinical trials are registered in Chinese Clinical Trial Registry (ChiCTR, https://www.chictr.org.cn/) and eighteen clinical trials are registered in ClinicalTrials.gov (https://clinicaltrials.gov/) at SPHIC (Table 4). At CGMH, three clinical trials were registered in ClinicalTrials.gov. In HIMM, six clinical trials are recruiting patients, including pancreatic cancer, lung cancer, three for esophageal cancer and muscular-infiltrating bladder cancer in ChiCTR in 2021. It can be seen that large parts of the clinical trials are recruiting patients, and several trials were retracted because of shortage of patients. One possible reason is that the acceptance of particle beam therapy is not enough among cancer patients in China. To assure the reliability, we mainly summarize the clinical results that have been published or reported in this section.
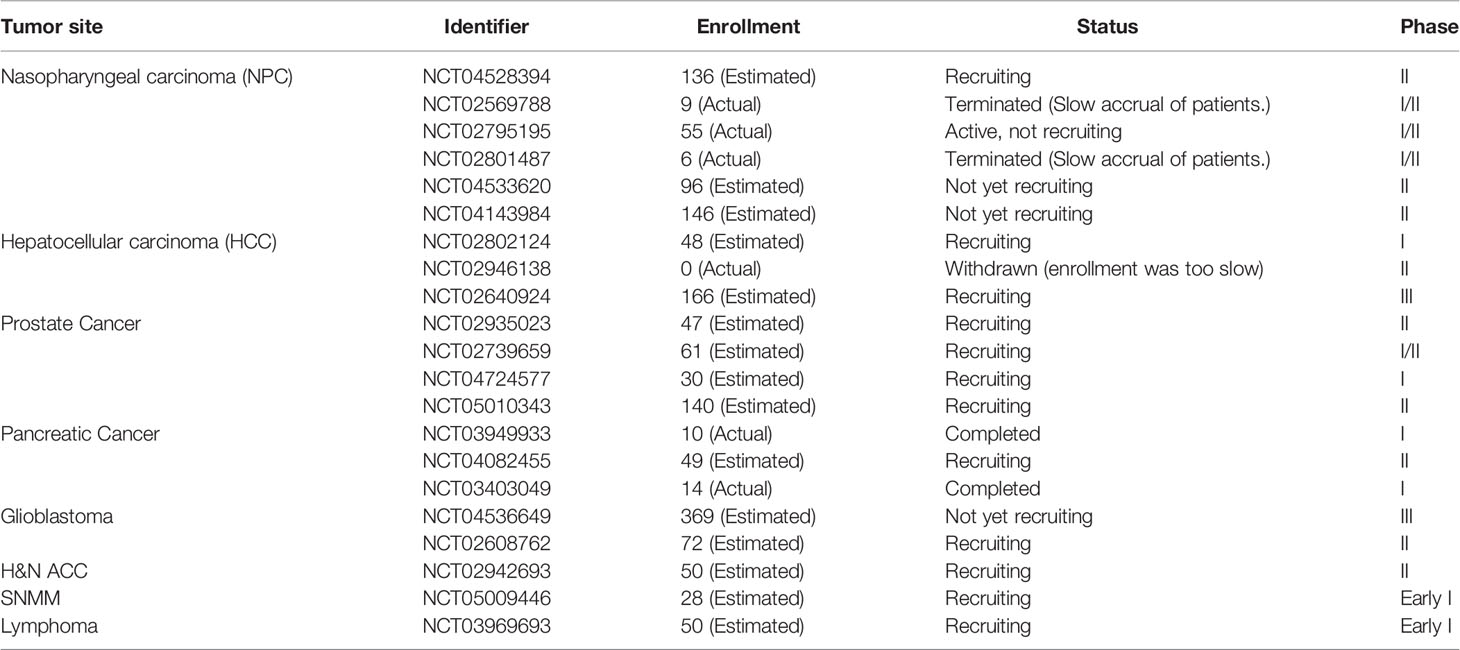
Table 4 Clinical trials registered in ClinicalTrials.gov for radiotherapy in China (up to January 2022, data collected from https://clinicaltrials.gov/).
To register as a medical institution, safety and efficacy have been verified at SPHIC and HIMM. From June 2014 to December 2014, SPHIC completed a phase II clinical trial (IONTRIAL) designed to verify the safety and efficacy of Siemens IONTRIS ion therapy system (97). All 35 patients enrolled in the IONTRIAL trial, and there was no case of grade 2 or above adverse reactions during the treatment and follow-up. No patient death or treatment-related moderate or severe adverse events have occurred in all patients during follow-up (98).
From 2006 to 2008, 103 cases of shallow-seated tumor patients, including skin squamous cell carcinoma and malignant lymphoma, treated at IMP (31). In the next five years, 110 deep-seated tumor patients have been treated with high-energy carbon ions at IMP. The tumor volume was reduced to varying degrees. There was no obvious side-effect in the irradiated site and the whole body after treatment (99).
In order to build a more compact medical accelerator, HIMM was built based on the clinical experience gained in the previous stage of IMP. The clinical trial of HIMM began on November 6, 2018, and was completed on February 25, 2019 in all 46 subjects. At the end of treatment, all 46 patients were evaluated according to the Response Evaluation Criteria in Solid Tumors RECIST Version 1.1. Ninety days after the end of treatment, the local control rate was 100%, and the objective response rate was 28.26%. No adverse events occurred according to the Common Terminology Criteria for Adverse Events (CTC.AE) version 4.03 (100).
Clinical Practices
Major cancer types treated with particle therapy in China have been summarized in Table 5, including treatment modality, number of patients, gender of patients, age of patients, overall survival, local control, late toxicity and treatment center. The detailed description for different cancers has been discussed.
Prostate Cancer
Prostate cancer accounts for 26% of all incident cases and is one of the greatest number of deaths in men in 2021 (101). Prostate cancer is also very common and fast-growing in Chinese population. Recently, the Genitourinary Subcommittee of PTCOG prepared a consensus Statement on Proton therapy for prostate Cancer, which will guide the clinical practice and research direction of proton therapy for prostate cancer (8). CIRT has been used to treat clinically localized prostate cancer. According to published clinical trials and practice, CIRT showed excellent disease control and acceptable toxicity for prostate cancer (45, 102, 103).
From June 2014 to October 2019, 154 prostate cancer patients were treated in the SPHIC (104). The percentage of intermediate-, and high-risk patients was 94%. Overall survival rate and biochemical control rate is 100% and 93% in three years, respectively. Sixty-four patients with localized prostate cancer underwent CIRT to assess toxicity and quality-of-life at the SPHIC using the Expanded Prostate Cancer Index Composite-26 (EPIC-26) (45). Forty-six (71.9%) men were treated with 59.2–60.8 GyE/16 fractions, whereas 18 men (28.1%) received a prescription dose of 66 GyE/24 fractions. The incidence of acute grade 1 and 2 and late Grade 1 and 2 genitourinary toxicity was 20.3 and 10.9%, 3.1 and 1.6%, respectively. No acute or late gastrointestinal toxicity occurred. Those results are similar to the results reported in Japan (105). Because the follow-up time period is too short in SPHIC, mid-term and long-term follow-up data of efficacy and toxicity should be regularly follow-up and the results will be reported in future publications, so as to compare the toxicity and patients benefit with conventional treatment modalities.
Head and Neck
Chordoma or Chondrosarcoma
Sarcomas of the base of the skull (SBS) accounts for a small proportion of head and neck malignancies. Surgery is the common treatment manner for SBS. Whereas, due to the anatomical complexity and radioresistant of SBS, the efficacy of conventional radiotherapy was weakened. Chen et al. (32) reported 31 patients underwent PBT of their chordoma at the WPTC. The prescribed dose was 50.4-80 GyE/28-40 fractions. With a median follow-up of 38.2 (range 6–76) months, the 3-year and 5-year OS and PFS were 90.3 and 80.4%, 87.1 and 60.5%, respectively (32).
A total of 62 patients with SBS received PBT and CIRT at the SPHIC between July 2014 and May 2019 (46). With a median follow-up of 20.4 (range 2.73–91.67) months, the 2-year OS and LRFS were 80.2% and 80.2%, respectively (39). There have two patients with Grade ≥ 3 toxicities occurred. It should be noted that these results are not the result of PBT and CIRT alone, and there is also the effect of chemotherapy.
Orbital Malignancies
Although orbital tumors are relatively rare, treatment is complicated because of their proximity to the critical OARs. With the rapid development of CIRT or PBT in the management of head and neck malignancies, many radiation oncologists have turned their attention to treat head and neck malignancies with CIRT or PBT (106). 22 patients with orbital malignancies received eye-sparing surgery followed by CIRT (n = 18), PBT (n = 1), or PBT plus CIRT boost (n = 3) at the SPHIC. With a median follow-up of 20.25 (range 3.8–38.8) months, the 2-year OS, PFS, and local progression-free survival (LPFS) were 100, 57.9, 92.9%, respectively. No acute severe (i.e., ≥grade 3) toxicity was observed, except for two patients with severe visual impairment (47).
Locoregionally Recurrent Head and Neck Malignancies
Sarcomas of the head and neck is considered a rare clinicopathological entity. It is difficult to en bloc surgical removal, so radiotherapy is a good choice. Salvage radiotherapy is usually limited by the adverse-effects. Although there have several publications reported the clinical outcomes in CIRT sarcoma treatment, but re-irradiation for HNS sarcoma is limited. PBT and CIRT can give full play to its advantages to extend the survival rate of patients. 141 patients with LR-HNS treated with IMCT at SPHIC (18, 107). The median dose was 60 GyE (range 50–69 GyE, 2.0–3.5 GyE/daily fraction). The 1-year overall survival rate was 95.9%, which is better than reported one-year OS rates (30–40%) (18). 7.1% of the patients reached grade ≥3 acute or late toxicities. Ten patients experienced Grade 4 hemorrhage. These results are highly analogue to patients treated at the Heidelberg Ion Therapy center (HIT) (108). It can be seen that salvage radiotherapy for HNS sarcoma may be associated with severe adverse effects and only provide palliative effects.
Sinonasal Malignancies
Sinonasal malignancies (SNM) accounts for 3%~5% of head and neck malignancies. The efficacy of IMRT over conventional RT for SNM remains to be controversial. The advent of PBT and CIRT brings hope for the treatment of SNM depending on their advantages in physical and radiobiological aspects (109). One-hundred-and-eleven patients received particle-beam radiation therapy (PBRT) at the SPHIC, including CIRT alone (n = 70), PBT plus CIRT boost (n = 37), and PBT alone (n = 4). The median follow-up was 20.2 months. The 2-year OS rates, PFS, and LPFS were 82, 66, and 83%, respectively. Grades 3-4 late toxicity occurred only 4 (3.6%) patients (48). A clinical experience and short-term efficacy in the management of olfactory neuroblastoma (ONB) also shown that PBRT is well tolerated and safe and effective for the treatment of ONB at the SPHIC (110).
Head and Neck Adenoid Cystic Carcinoma
Adenoid cystic carcinoma is a rare malignant tumor of the head and neck, commonly found in the salivary glands. Slow growth, local recurrence and distant metastasis have always been considered as typical clinical features of the disease. Eight patients with H&N ACC were treated using PBT or CIRT to study the early response and toxicities at the SPHIC (111). Seven patients received IMPT followed by IMCT boost. One of them received IMPT alone. With a 3-months follow-up, 50% patients achieved PR or CR. Except for 2 patients who experienced grade 3 mucositis, no patients experienced serious toxicities.
Nasopharyngeal Carcinoma
Nasopharyngeal carcinoma is one of the common malignant tumors in China, especially in the south. Local recurrence remains one of the most important modes of treatment failure in the management of patients with NPC. Radiotherapy is currently the preferred treatment for nasopharyngeal carcinoma. The benefits of CIRT in the salvage treatment of patients with locally recurrent head and neck malignancies have been reported (96). SPHIC and CGHM have achieved some exciting results in NPC radiotherapy (Table 5).
Between 2016 and 2018, 80 patients with NPC who received intensity-modulated proton therapy (IMPT) at the CGMH. The prescribed dose was 69.96 GyE for IMPT given in 2.12 GyE fractions. Median follow-up time was 24.1 months (18.2–34.3) for patients treated using IMPT. No patient died in the IMPT group. The 2-year overall survival (OS) rates were 100% for the IMPT. Four events (recurrence or death) were observed in the IMPT group, with a two-year progression-free survival (PFS) rate of 94.4%. Four patients (5%) treated with IMPT required NG tube placement. The mean percentage of body weight loss (BWL) during RT was 4.87% in the IMPT group (49). Propensity score matching analysis of patients treated with IMPT and Volumetric Modulated Arc Therapy (VMAT) has shown IMPT is safe and beneficial for NPC patients.
Satisfactory efficacy and acceptable toxicity of IMRT with CIRT boost has been achieved in a small group of patients (112). A large sample size of 69 NPC patients treated with mixed-beam radiotherapy using IMRT and CIRT boost at the SPHIC (42). With a median follow-up of 31.9 months, the 3-year OS, PFS, local control rates were 94.9, 85.2, 96.9%, respectively. No severe radiation-induced late toxicity was observed, except for two patients with dermatitis. Therefore, mixed photon and carbon-ion beam radiotherapy present an excellent disease control and acceptable toxicity for NPC patients.
Intensity-modulated carbon-ion therapy (IMCT) treatment of NPC has obvious advantages over IMRT in terms of serious adverse reactions (108). Kong et al. reported 206 cases of locally recurrent nasopharyngeal carcinoma treated with CIRT. The dose of salvage radiotherapy is 50-69 GyE (2.0-3.0 GyE/daily fraction) (38, 113). With a median follow-up of 22.8 months, the 2-year OS, local control, regional control, and distant control rates were 83.7%, 58.0%, 87.3%, and 94.7%, respectively. There was no case of mucosal or other radiotherapy-related adverse reactions of grade 2 or higher during follow-up. Whereas, long-term follow-up is necessary to determine the optimal dose and long-term outcome and late toxicities.
Intracranial Tumors
ICT refers to tumors that are primary or secondary to the cranial cavity. ICT has gradually become one of the important tumor diseases that endanger the health of the Chinese population and cause death. At present, the main treatment methods for ICT include surgery, radiotherapy, chemotherapy, interventional therapy, and targeted therapy. Radiotherapy is the standard adjuvant therapy to intracranial tumor after surgery (114). Due to the complicated histological subtypes of brain tumor, the clinical outcomes will be presented in two parts.
Gliomas
Glioma ranks first among adult central nervous system malignant tumors. The tumor progresses rapidly, the recurrence rate after treatment is high, and the cure rate is low, making it a major medical challenge. Surgical resection is the main treatment method. Due to its biological characteristics of invasive growth, surgery is difficult to completely remove, and it is prone to recurrence after surgery, so postoperative radiotherapy is often used as adjuvant treatment. From December 2004 to October 2006, 46 cases of gliomas were treated at the WPTC (33). The total dose ranged from 45 to 72 GyE and each fraction dose from 1.8 to 5 GyE in malignant tumor. The follow-up period for glioma patients range from 1 to 20 months (averaged 9.8 months). Thirteen patients showed disappearance of the tumor (27.1%), 29 cases with PR (60.4%).
Between May 2015 and October 2018, 50 patients with histology confirmed high-grade gliomas (HGG) received either PBT or PBT with a CIRT boost at the SPHIC (50). All patients received temozolomide. Twenty-four patients received PBT at a dose of 60 GyE/30 fractions, and 26 patients received PBT plus a CIRT boost in various protocols. At a median follow-up of 14.3 months (range, 4.8-39.6 months), the 12-month and 18-month OS rates were 87.8% and 72.8%, respectively, and the 12-month and 18-month PFS rates were 74.2% and 59.8%, respectively. Twenty-nine patients experienced grade 1 treatment-related acute adverse effects, and 11 developed grade 1 (n = 6) or grade 2 (n = 5) late adverse effect of radiation-induced brain necrosis. No grade 3, 4, or 5 toxicities were observed. To assess the effect of CTRT boost after PBT, a randomized trial is recruiting patients (37).
Meningioma
Meningioma is one of the most common intracranial tumors, accounting for about 33% of central nervous system tumors (115). PBT has a better dose distribution than conventional photon therapy, so it can improve tumor control and better protect normal brain tissue (116). Twenty-six patients with meningioma treated with PBRT from May 2015 to October 2018 at the SPHIC. The median dose was 54 GyE (range 50.4-60 GyE, 1.8-2 GyE/daily fraction). With a median follow-up of 22.2 (range 1.6-36.4) months, the 2-year overall survival and progression-free survival rates were both 100%. Grade I skin erythema and alopecia were observed in 22 patients and Grade I mucositis was observed in 2 patients. No acute of late toxicities of Grade 2 or above was observed (117). The safety and favorable toxicity profile of CIRT for meningioma also were confirmed at many institutes (118).
Thoracic Cancers
Thoracic tumors are the most morbidity sites in China, and radiotherapy is one of the main treatments for chest tumors, including Lung cancer, breast cancer, esophageal cancer, etc. While thoracic radiotherapy kills tumors, it often causes radiation-induced lung damage, which limits the further increase in the dose of radiotherapy to eliminate tumors and also seriously affects the quality of life of patients (119).
Lung Cancer
According to global cancer statistics 2021, lung cancer is the leading cause of death among males (101). The highest incidence rates among men are observed in China (rates are above 40 per 100,000). Currently, as for those patients who are not suitable for surgical resection, radiotherapy is still an important and potentially treatment for local and recurrent non-small cell lung cancer (NSCLC) (120).
From August 2014 to December 2015, 10 patients with stage I non-small cell lung cancer (NSCLC) who were inoperable or refused surgery were treated by proton alone or proton combination with carbon-ion RT to evaluate the safety and efficacy of PBT and CIRT for stage I NSCLC with pencil beam scanning technique at the SPHIC (121). The prescribed dose was 50-72 GyE with 10-24 fractions. With the median follow-up of 18.1 (11.9-28.1) months, local control was found in all patients with 6 complete response (CR), 3 partial response (PR), and 1 stable disease (SD). No Grade ≥ 3 toxicity occurred in all patients. Two patients occurred grade 2 toxicities with acute skin reaction and leucopenia.
Tracheal Adenoid Cystic Carcinoma
TACC is a kind of tumor with low incidence and not easy to be found. Although it has been reported that radiotherapy can be used for the treatment of TACC, its specific role is unknown (122). Eighteen patients with TACC were treated using CIRT at the SPHIC. The patient received 66–72.6 GyE/22–23 fractions. During a median follow-up period of 20.7 (range 5.8–44.1) months, 3 patients developed lung metastasis, and one of them experienced local recurrence. The lung metastasis rate is consistent with previous reports (123).The rates of 2-year OS, LC, and PFS were 100, 100, and 61.4%, respectively. Only one patient experienced grade 4 tracheal stenosis, no other grade ≥ 3 adverse effects were observed (40).
Hepatocellular Carcinomas
Hepatocellular carcinoma (HCC) is a type of malignant tumor with high morbidity and mortality. In China, the total number of HCC patients accounts for more than half of the world’s cases every year (124). Most patients are associated with chronic viral hepatitis B infection and cirrhosis (125). Although surgery or radiofrequency ablation can cure hepatocellular carcinoma, few patients are operable due to advanced disease or medical comorbidities. Due to the high radiosensitivity of normal liver tissue and respiration motion of patient, RT was very limited for liver cancers. To reduce the unwanted effect in HCC RT, some methods have been developed, especially respiratory control and image guidance technology, which can achieve encouraging responses and only minimal toxicities (126–128).
Between 2005 and 2007, a total of 32 patients with primary hepatocellular carcinoma have been treated with PBT at the WPTC in ZIBO, China (34). PBT was delivered to a total dose of 60 -70 GyE in 7 fractions. In this study, 9 cases achieved clinical CR, 37 cases achieved PR and 3 cases suffered SD. The objective therapeutic effect is approximately 90.6%. According to Karnofsky Performance Status (KPS) score, 10 cases improved the quality of life (31.25%). The improvement rate of liver function reached 53.13%. Adverse reactions were classified according to Radiation Therapy Oncology Group (RTOG) criteria for acute radiation injury, including 0 Grade (16 cases), 1 Grade (12 cases), 2 Grade (3 cases), 3 Grade (1 cases), 4 Grade (0 cases). Proton therapy can not only improve the quality of life of patients, which may indicate reduced toxicity with PBT.
From January 2007 to January 2018, a total of 55 patients treated with PBT were enrolled for primary hepatocellular carcinoma at CGMH. According to the tumor location, 72.6 GyE in 22 fractions or 66 GyE in 10 fractions were prescribed. Through a propensity-matched analysis, compared with photon radiotherapy, significant survival benefit in the proton group and lower risk of RILD (11.8% vs. 36%, p =0.004) was achieved (53). Between November 2015 and December 2017, 30 patients with unresectable cholangiocarcinoma (CC) had undergone PBT. The median radiation dose was 72.6 GyE. The median OS and PFS were 19.3 and 10.4 months, respectively. Seven percentage of patients suffered acute skin reactions. Three patients had grade ≥ III toxicities and two patients had radiation-induced liver disease (129).
Skin Malignant Neoplasm
The incidence of skin cancers has continuously risen. Although surgery is the preferred strategy, radiation therapy is recommended for tumors that are difficult to remove, for example, primary or lymph node-invaded melanoma. Most of CIRT are focused on deep-seated tumors, but treatment of skin carcinomas is limited. Between November 2006 and March 2009, forty-five patients with superficial carcinoma were treated with CIRT at the IMP (55). The range of total dose was 60 to70 GyE for squamous cell carcinoma (SCC) and basal cell carcinoma (BCC), 61–75 GyE for malignant melanoma (MM), 60 GyE for Bowen disease and 42.5 GyE for Paget disease, administered in 6-11 fractions within 6-11 days. The single dose was 7-10 GyE. The median follow-up period was 24 months (range,12-36 months) and the follow-up rate was 100%. The 3-years actuarial local control rates were 65.5% for SCC, 80.2% for BCC, 42.9% for MM, 90% for Bowen and Paget diseases, respectively. The actuarial 1- and 3-year overall survival rates reached 86%. No severe side-effects greater than grade 3 have been observed.
Keloids
Keloid is a benign tumor of the skin, but it has the characteristics of malignant tumor infiltrating into the surrounding normal tissues (130). Keloid is accompanied by chronic inflammation, which is prone to infection and ulceration. At present, the main treatment for keloids is surgical resection, but there is a high recurrence rate after surgical resection alone, so it is often necessary to combine other adjuvant treatments after surgical resection (131). Surgical resection combined with radiotherapy is a more conventional treatment for keloids. At present, the principle of radiotherapy for keloids is still unclear. Recently, 16 patients with keloids received postoperative CIRT at the IMP. The prescribed dose was 16GyE/8 fractions (56). With a medium follow-up of 29.7 months (range 24.335.3 months), 95% success rates were achieved. No grade 3 or higher toxicity and complication occurred. As far as we know, this should be the first report of CIRT for keloids. This result indicates that surgery combined with CIRT can be used in the treatment of keloids in the future (56).
Rectal Cancer
Rectal cancer is one of the common gastrointestinal malignancies. Due to the lack of specific symptoms of rectal cancer, many patients have undergone local infiltration or distant metastasis. Total mesorectal excision (TME) after preoperative neoadjuvant chemoradiotherapy has become the main treatment manner for patients with locally advanced rectal cancer. Many clinical studies have demonstrated that preoperative radiotherapy can improve local control rate and reduce the risk of recurrence. Twenty-five patients with unresectable locally recurrent rectal cancer (LRRC) were treated by CIRT from July 2015 to April 2019 at SPHIC (51). The LC rates at 1 and 2 years were 90.4 and 71.8%. The OS rates at 1 and 2 years were 82.9 and 65.1%. No Grade 4 or higher toxicity was observed. In contrast to National Institutional of Radiological Sciences Hospital (NIRS) (132), there was a good correlation between prescribed dose and local control rate.
Pancreatic Cancer
Pancreatic cancer has a high mortality rate, and 80% to 85% of patients present with locally advanced or distant metastases. Radiotherapy plays an important role in the treatment of pancreatic cancer and has achieved encouraging therapeutic effects. A phase I dose escalation study of PBT and CIRT has been carried out for the patients with locally advanced pancreatic cancer (LAPC) at SPHIC (52). The proton dose of 50.4 GyE and carbon ion as a boost dose varying from 62.4 GyE/32 fractions to 68.4 GyE/34 fractions were delivered. With a median follow-up of 17.4 months, no patient developed dose limiting toxicity, and 40% of patients suffered from acute gastrointestinal (GI) and hepatic toxicity. The OS rates at 1 and 2 years were 80.0% and 13.3%. It is worth noting that only ten patients were enrolled in this study. Therefore, more patients and long-term follow-up are needed to determine the outcome of treatment. According to NIRS reports, the OS rates at 1 and 2 years were 73% and 35% (133). The difference may be due to different prescribed dose and combination modality of radiotherapy.
Future Challenges
Over the past decade there has been considerable interest and progress in the use of protons and carbon in an attempt to improve the effectiveness of radiotherapy in China. Considering the actual situation in China, it is necessary to strengthen the talent training of medical physicists and develop advanced radiotherapy technology and supporting equipment such as rotating gantry. These centers should coordinate and integrate all social resources to promote market application as soon as possible (134). Small sample size and high heterogeneity are the common features for particle radiotherapy in China. Owing to the length of time needed to accumulate epidemiologic data, a concerted multicenter, international effort should be established for long-term follow-up of charged particle RT patients in China. Although China has been in the forefront of proton and heavy ion therapy in the world, and has achieved some encouraging results in the treatment of cancer, the five proton or heavy ion treatment centers in China are only expert in a few types of cancer. Other types of cancer treatment need long-term exploration and optimization to achieve the best therapeutic effect. The incidence of cancer in China is very different from that in Europe, America and Japan (1), for instance, the incidence of breast cancer ranks first in the world, but ranks fourth in China after lung cancer, colorectal cancer and stomach cancer. Therefore, proton and heavy ion medical facilities in China should pay more attention to the more common types of cancer in China and actively explore new treatment technologies. The early data suggest that carbon ion radiotherapy provides favorable local tumor control and overall survival with acceptable rates of late complications. In addition, some challenges are the same as other particle therapy centers around the world, for instance, long-term follow-up of patients after treatment is necessary in order to compare with other conventional treatments and to highlight their advantages.
Discussion and Conclusion
China has the largest population and ranks first in the number of new cancer cases and deaths in the world (135), but the number of PBT and CIRT centers in operation in China is very small. Early PBT and CIRT results are satisfactory in China. However, we need to interpret the data with caution. Domestically, there is still no comparative analysis of the therapeutic effects of the three radiotherapy methods among IMRT, PBT and CIRT. Therefore, there is still a long road for researchers and physicians to explore the advantages of PBT and CIRT.
Tumor cells treated with carbon ions sensitized to several chemotherapeutic drugs (carboplatin, paclitaxel, and etoposide) (136). Until now, several clinical trials are available on the combination of chemotherapy and PBT or CIRT (137). Only one clinical trial to evaluate the efficacy of CIRT with concurrent cisplatin for LR-NPC at the SPHIC was registered (NCT02801487). In addition, radiotherapy fractionation for different tumor sites and sequential treatment of chemotherapy and radiotherapy is also important for the treatment outcome (138, 139). The same is true in China, where more clinical trials are needed to determine the optimal fractional dose and treatment sequence. RT is a particularly promising candidate for combination with immune checkpoint blocker (ICB) (140). A search for “radiotherapy plus immunotherapy” on ClinicalTrials.gov yielded 217 results (141). Indeed, radiotherapy not only has a direct killing effect on tumor cells, but also reprograms the tumor microenvironment to exert an effective anti-tumor immune response (142). The new treatment strategy of radiotherapy combined with immunotherapy has achieved remarkable curative effect on a variety of tumor models. Preliminary clinical trials have shown that this new therapy has achieved good results for patients with metastatic solid tumors, especially breast cancer, prostate cancer and NSCLC (143). Thus, combining radiotherapy and immunotherapy is a crucial strategy to improve patient survival (144). More detailed discussion of the mechanisms of CIRT and combination immunotherapy can be found in several review articles (145, 146). We have reason to believe that clinical trials combining radiotherapy and immunotherapy will be carried out in China in the next few years.
Although particle radiotherapy is a promising treatment method, the cost-efficiency ratio is an urgent problem to be solved (134, 147). Although heavier ions have the potential to significantly improve clinical results for radio-resistant indications and especially hypoxic tumors, carbon ion facilities cost about 2–3 times more than proton facilities. Building CIRT centers is also complicated. Design of these facilities should take into consideration the treatment delivery method, number of rooms needed, choice of gantry (or not), and the expected number of fractions or patients to be treated annually. To maximize the advantage of PBT and CIRT and minimum the costs, physicists and physicians should strengthen collaboration. More attention should be paid for physical technical, beam-delivery systems and treatment technology. Many improvements are already in progress in China. More recently, a variable cycle-based respiratory guidance method for moving target treatment was developed at the IMP, which will improve the CIRT treatment efficiency and precision for organs that move with breathing (127). In addition, a fast 3D rescanning method allows for 3D scanning that is about 100 times faster than conventional systems (148). Image-guided radiation therapy (IGRT) directs irradiation utilizing the imaging coordinates of the actual treatment plan, has further improved the accuracy and safety (149). Therefore, these two advanced technologies are the focus and difficulty of future research and development in China. In order to more conveniently carry out all-round RT for patients, a superconducting rotating gantry is also being developed at the IMP to reduce the footprint occupied by the equipment as much as possible.
There is an unmet need for comparative treatment planning study among different radiotherapy manners for patients to assess the potential benefits and limitations of different treatment modalities (11). In addition, there have been a number of preclinical studies based on cells and animals showing that ultra-high dose rate (FLASH) irradiation reduces damage to normal tissues while preserving the ability to treat tumors (150). As traditional therapeutic devices cannot meet the technical and safety requirements of FLASH radiotherapy, there are no publicly reported domestic studies on FLASH radiotherapy. However, with the successful application of FLASH radiotherapy in the first tumor patient (151), we believe that China will also pay attention to the development of new radiotherapy technologies in the future.
With the development of whole genome sequencing technology, the era of personalized therapy has arrived, which can extend to radiotherapy (152). There is increasing evidence that genetic mutations are closely related to radiosensitivity of tumors (153). PBT and CIRT centers are especially in short supply in China. In order to maximize the treatment efficiency of patients and save resources, we should consider detecting genetic mutations in patients in the future (154).
The Chinese health care system and specifically, radiation oncology, has clearly improved during the past 30 years in equipment and its use, although the shortage of facilities and workforce remain to be improved. In addition to the rapid increase in the number and quality of the facilities in China, the training of radiation oncologists and medical physicists (radiation oncology residency programs) also improved (134). Unresectable sarcoma became the only tumor type where CIRT is covered under the national healthcare insurance in Japan since 2016 (155). In China, the cost of particle therapy is borne by the patient themselves. The Chinese healthcare reimbursement is expected in the near future with cancer sites expected to be covered by the national insurance (134). At present, the SPHIC adopts a method of cooperation with commercial insurance.
There are too few facilities to conduct proton vs carbon prospective and randomized clinical trials required to compare the two modalities (156). Meanwhile, multi-institutional analysis studies of CIRT for different cancer sites is rare due to the small number of CIRT institutions in the world (157). Multi-institutional analysis can demonstrate standard and average treatment outcomes that can be utilized as reference in China. At present, there are several multi-institutional retrospective studies to evaluate the efficacy and safety of proton or carbon-ion radiotherapy (158–163). A randomized controlled trial comparing IMRT, PBT and CIRT would require a very large patient cohort to show significant differences in local control and/or toxicity. Hence, the multicenter international Radiation Oncology Collaborative Comparison (ROCOCO) was initiated in 2007 conducting several comparatives in silico trials in multiple primary tumor sites (164–166). In addition, physical therapist for tumor radiotherapy should adopt the best modality to specific tumor individuals according to multi-institutional analysis.
In short, although particle therapy in China is in the stage of rapid development, it is believed that particle therapy in China will be bright with the cultivation of domestic talents and the maturity of technology.
Author Contributions
YL selected the references, wrote the text, and approved the final version of this manuscript. JY, XL, MT, YG, SW, and JX contributed to discussing the content, review and editing of manuscript before submission. All authors contributed to the article and approved the submitted version.
Funding
This work was supported by grants from Guangdong Innovative and Entrepreneurial Research Team Program (No. 2016ZT06G373).
Conflict of Interest
The authors declare that the research was conducted in the absence of any commercial or financial relationships that could be construed as a potential conflict of interest.
Publisher’s Note
All claims expressed in this article are solely those of the authors and do not necessarily represent those of their affiliated organizations, or those of the publisher, the editors and the reviewers. Any product that may be evaluated in this article, or claim that may be made by its manufacturer, is not guaranteed or endorsed by the publisher.
Acknowledgments
We thanks to Jian Shi providing the information about facilities at the HIMM.
Supplementary Material
The Supplementary Material for this article can be found online at: https://www.frontiersin.org/articles/10.3389/fonc.2022.819905/full#supplementary-material
Abbreviations
RBE, relative biological effectiveness; WPTC, Wanjie Proton Therapy Center; SPHIC, Shanghai Proton Heavy Ion Center; HIMM, Heavy Ion Cancer Treatment Center; CGMH, Chang Gung Memorial Hospital; RT, radiotherapy; PTCOG, Particle Therapy Co-Operative Group; PBT, proton beam therapy; CIRT, carbon ion radiotherapy; LET, linear energy transfer; LR-NPC, locally recurrent nasopharyngeal carcinoma; OER, Oxygen Enhancement Ratio; IMP, Institute of Modern Physics; HCC, Hepatocellular carcinoma; OS, overall survival; PFS, progression-free survival.
References
1. Sung H, Ferlay J, Siegel RL, Laversanne M, Soerjomataram I, Jemal A, et al. Global Cancer Statistics 2020: GLOBOCAN Estimates of Incidence and Mortality Worldwide for 36 Cancers in 185 Countries. CA Cancer J Clin (2021) 71(3):209–49. doi: 10.3322/caac.21660
2. Schnipper LE, Davidson NE, Wollins DS, Tyne C, Blayney DW, Blum D, et al. American Society of Clinical Oncology Statement: A Conceptual Framework to Assess the Value of Cancer Treatment Options. J Clin Oncol (2015) 33(23):2563–77. doi: 10.1200/JCO.2015.61.6706
3. Kamada T, Tsujii H, Blakely EA, Debus J, De Neve W, Durante M, et al. Carbon Ion Radiotherapy in Japan: An Assessment of 20 Years of Clinical Experience. Lancet Oncol (2015) 16(2):e93–e100. doi: 10.1016/S1470-2045(14)70412-7
4. Gupta T, Sinha S, Ghosh-Laskar S, Budrukkar A, Mummudi N, Swain M, et al. Intensity-Modulated Radiation Therapy Versus Three-Dimensional Conformal Radiotherapy in Head and Neck Squamous Cell Carcinoma: Long-Term and Mature Outcomes of a Prospective Randomized Trial. Radiat Oncol (2020) 15(1):218. doi: 10.1186/s13014-020-01666-5
5. Halperin EC. Particle Therapy and Treatment of Cancer. Lancet Oncol (2006) 7(8):676–85. doi: 10.1016/S1470-2045(06)70795-1
6. Patient statistics per end of 2020. (2020). Available at: https://www.ptcog.ch/images/patientstatistics/Patientstatistics-updateDec2020_11:2021.pdf (Accessed January 10, 2022).
7. Chang JY, Zhang X, Knopf A, Li H, Mori S, Dong L, et al. Consensus Guidelines for Implementing Pencil-Beam Scanning Proton Therapy for Thoracic Malignancies on Behalf of the PTCOG Thoracic and Lymphoma Subcommittee. Int J Radiat Oncol Biol Phys (2017) 99(1):41–50. doi: 10.1016/j.ijrobp.2017.05.014
8. Bryant CM, Henderson RH, Nichols RC, Mendenhall WM, Hoppe BS, Vargas CE, et al. Consensus Statement on Proton Therapy for Prostate Cancer. Int J Part Ther (2021) 8(2):1–16. doi: 10.14338/ijpt-20-00031.1
9. Zhang Q, Kong L, Liu R, Wang X. Ion Therapy Guideline (Version 2020). Precis Radiat Oncol (2021) 5(2):73–83. doi: 10.1002/pro6.1120
10. Kanai T, Furusawa Y, Fukutsu K, Itsukaichi H, Eguchi-Kasai K, Ohara H. Irradiation of Mixed Beam and Design of Spread-Out Bragg Peak for Heavy-Ion Radiotherapy. Radiat Res (1997) 147(1):78–85. doi: 10.2307/3579446
11. Liu SW, Li JM, Chang JY, Yu JM, Chen Q, Jiang QA, et al. A Treatment Planning Comparison Between Proton Beam Therapy and Intensity-Modulated X-Ray Therapy for Recurrent Nasopharyngeal Carcinoma. J Xray Sci Technol (2010) 18(4):443–50. doi: 10.3233/XST-2010-0265
12. Wang L, Hu J, Liu X, Wang W, Kong L, Lu JJ. Intensity-Modulated Carbon-Ion Radiation Therapy Versus Intensity-Modulated Photon-Based Radiation Therapy in Locally Recurrent Nasopharyngeal Carcinoma: A Dosimetric Comparison. Cancer Manag Res (2019) 11:7767–77. doi: 10.2147/CMAR.S205421
13. Kim J-I, Park JM, Wu H-G. Carbon Ion Therapy: A Review of an Advanced Technology. Prog Med Phys (2020) 31(3):71–80. doi: 10.14316/pmp.2020.31.3.71
14. Phillips MH, Frankel KA, Lyman JT, Fabrikant JI, Levy RP. Comparison of Different Radiation Types and Irradiation Geometries in Stereotactic Radiosurgery. Int J Radiat Oncol Biol Phys (1990) 18(1):211–20. doi: 10.1016/0360-3016(90)90286-s
15. Karger CP, Glowa C, Peschke P, Kraft-Weyrather W. The RBE in Ion Beam Radiotherapy: In Vivo Studies and Clinical Application. Z Med Phys (2021) 31(2):105–21. doi: 10.1016/j.zemedi.2020.12.001
16. Tinganelli W, Durante M. Carbon Ion Radiobiology. Cancers (Basel) (2020) 12(10):3022. doi: 10.3390/cancers12103022
17. Barendsen GW. RBE-LET Relationships for Lethal, Potentially Lethal and Sublethal Damage in Mammalian Cells: Implications for Fast Neutron Radiotherapy. Bull Cancer Radiother (1996) 83:15s–8s. doi: 10.1016/0924-4212(96)84878-x
18. Gao J, Hu J, Guan X, Yang J, Hu W, Kong L, et al. Salvage Carbon-Ion Radiation Therapy For Locoregionally Recurrent Head and Neck Malignancies. Sci Rep (2019) 9(1):4259. doi: 10.1038/s41598-019-39241-y
19. Karger CP, Peschke P. RBE and Related Modeling in Carbon-Ion Therapy. Phys Med Biol (2017) 63(1):01TR02. doi: 10.1088/1361-6560/aa9102
20. Weber U, Kraft G. Comparison of Carbon Ions Versus Protons. Cancer J (2009) 15(4):325–32. doi: 10.1097/PPO.0b013e3181b01935
21. Ando K, Kase Y. Biological Characteristics of Carbon-Ion Therapy. Int J Radiat Biol (2009) 85(9):715–28. doi: 10.1080/09553000903072470
22. Asaithamby A, Chen DJ. Mechanism of Cluster DNA Damage Repair in Response to High-Atomic Number and Energy Particles Radiation. Mutat Res (2011) 711(1-2):87–99. doi: 10.1016/j.mrfmmm.2010.11.002
23. Hagiwara Y, Oike T, Niimi A, Yamauchi M, Sato H, Limsirichaikul S, et al. Clustered DNA Double-Strand Break Formation and the Repair Pathway Following Heavy-Ion Irradiation. J Radiat Res (2019) 60(1):69–79. doi: 10.1093/jrr/rry096
24. DeSisto J, Lucas JT Jr., Xu K, Donson A, Lin T, Sanford B, et al. Comprehensive Molecular Characterization of Pediatric Radiation-Induced High-Grade Glioma. Nat Commun (2021) 12(1):5531. doi: 10.1038/s41467-021-25709-x
25. Valable S, Gerault AN, Lambert G, Leblond MM, Anfray C, Toutain J, et al. Impact of Hypoxia on Carbon Ion Therapy in Glioblastoma Cells: Modulation by LET and Hypoxia-Dependent Genes. Cancers (Basel) (2020) 12(8):2019. doi: 10.3390/cancers12082019
26. Facilities-in-operation. (2022). Available at: https://www.ptcog.ch/index.php/facilities-in-operation (Accessed January 10, 2022).
27. Durante M, Debus J, Loeffler JS. Physics and Biomedical Challenges of Cancer Therapy With Accelerated Heavy Ions. Nat Rev Phys (2021) 3(12):777–90. doi: 10.1038/s42254-021-00368-5
28. Combs SE, Jakel O, Haberer T, Debus J. Particle Therapy at the Heidelberg Ion Therapy Center (HIT) - Integrated Research-Driven University-Hospital-Based Radiation Oncology Service in Heidelberg, Germany. Radiother Oncol (2010) 95(1):41–4. doi: 10.1016/j.radonc.2010.02.016
29. Kanematsu N, Furukawa T, Hara Y, Inaniwa T, Iwata Y, Mizushima K, et al. New Technologies for Carbon-Ion Radiotherapy - Developments at the National Institute of Radiological Sciences, QST, Japan. Radiat Phys Chem (2019) 162:90–5. doi: 10.1016/j.radphyschem.2019.04.038
30. Zhang Y, Fang WC, Huang XX, Tan JH, Zhong SP, Xiao CC, et al. Radio Frequency Conditioning of an S-Band Accelerating Structure Prototype for Compact Proton Therapy Facility. Nucl Sci Tech (2021) 32(6):64. doi: 10.1007/s41365-021-00891-1
31. Li QA, Dai ZY, Yan X, Jin XD, Liu XG, Xiao GQ. Heavy-Ion Conformal Irradiation in the Shallow-Seated Tumor Therapy Terminal at HIRFL. Med Biol Eng Comput (2007) 45(11):1037–43. doi: 10.1007/s11517-007-0245-3
32. Ji-suo C, Yu-xia Y, Xiang-hua H, Qing C, Lei Y. Preliminary Analysis of Proton Radiation Therapy for Chordomas in the Skull Base. Chin J Neuromed (2012) 11(9):895–8. doi: 10.3760/cma.j.issn.1671-8925.2012.09.008
33. Fan YF, Chen JS, Yu JW, Tao F, Yu XL, Li X, et al. Analysis of Short-Term Curative Effect of Proton Therapy in 66 Cases of Intracranial Tumors. Chin J Neuro-Oncol (2006) 4(4):251–5.
34. Li J, Liu S-W, Huang S, Zhang J-G, Chen Q, Mu X, et al. Clinical Value and Prognostic Factors of Proton Beam Therapy for Hepatocellular Carcinoma. Chin J Radiat Oncol (2012) 21(1):27–8. doi: 10.3760/cma.j.issn.1004-4221.2012.01.009
35. Ma NY, Chen J, Ming X, Jiang GL, Lu JJ, Wu KL, et al. Preliminary Safety and Efficacy of Proton Plus Carbon-Ion Radiotherapy With Concurrent Chemotherapy in Limited-Stage Small Cell Lung Cancer. Front Oncol (2021) 11:766822:766822. doi: 10.3389/fonc.2021.766822
36. Kong L, Hu J, Guan X, Gao J, Lu R, Lu JJ. Phase I/II Trial Evaluating Carbon Ion Radiotherapy for Salvaging Treatment of Locally Recurrent Nasopharyngeal Carcinoma. J Cancer (2016) 7(7):774–83. doi: 10.7150/jca.14399
37. Kong L, Gao J, Hu J, Lu R, Yang J, Qiu X, et al. Carbon Ion Radiotherapy Boost in the Treatment of Glioblastoma: A Randomized Phase I/III Clinical Trial. Cancer Commun (Lond) (2019) 39(1):5. doi: 10.1186/s40880-019-0351-2
38. Hu J, Huang Q, Gao J, Guan X, Hu W, Yang J, et al. Clinical Outcomes of Carbon-Ion Radiotherapy for Patients With Locoregionally Recurrent Nasopharyngeal Carcinoma. Cancer (2020) 126(23):5173–83. doi: 10.1002/cncr.33197
39. Wu S, Li P, Cai X, Hong Z, Yu Z, Zhang Q, et al. Carbon Ion Radiotherapy for Patients With Extracranial Chordoma or Chondrosarcoma - Initial Experience From Shanghai Proton and Heavy Ion Center. J Cancer (2019) 10(15):3315–22. doi: 10.7150/jca.29667
40. Chen J, Mao J, Ma N, Wu KL, Lu J, Jiang GL. Definitive Carbon Ion Radiotherapy for Tracheobronchial Adenoid Cystic Carcinoma: A Preliminary Report. BMC Cancer (2021) 21(1):734. doi: 10.1186/s12885-021-08493-1
41. Yang J, Gao J, Qiu X, Hu J, Hu W, Wu X, et al. Intensity-Modulated Proton and Carbon-Ion Radiation Therapy in the Management of Head and Neck Sarcomas. Cancer Med (2019) 8(10):4574–86. doi: 10.1002/cam4.2319
42. Hu J, Huang Q, Gao J, Hu W, Yang J, Guan X, et al. Mixed Photon and Carbon-Ion Beam Radiotherapy in the Management of Non-Metastatic Nasopharyngeal Carcinoma. Front Oncol (2021) 11:653050:653050. doi: 10.3389/fonc.2021.653050
43. Zhang W, Hu W, Hu J, Gao J, Yang J, Kong L, et al. Carbon Ion Radiation Therapy for Sinonasal Malignancies: Promising Results From 2282 Cases From the Real World. Cancer Sci (2020) 111(12):4465–79. doi: 10.1111/cas.14650
44. Guan X, Gao J, Hu J, Hu W, Yang J, Qiu X, et al. The Preliminary Results of Proton and Carbon Ion Therapy for Chordoma and Chondrosarcoma of the Skull Base and Cervical Spine. Radiat Oncol (2019) 14(1):206. doi: 10.1186/s13014-019-1407-9
45. Zhang Y, Li P, Yu Q, Wu S, Chen X, Zhang Q, et al. Preliminary Exploration of Clinical Factors Affecting Acute Toxicity and Quality of Life After Carbon Ion Therapy for Prostate Cancer. Radiat Oncol (2019) 14(1):94. doi: 10.1186/s13014-019-1303-3
46. Yang J, Hu W, Guan X, Hu J, Gao J, Qiu X, et al. Particle Beam Radiation Therapy for Skull Base Sarcomas. Front Oncol (2020) 10:1368:1368. doi: 10.3389/fonc.2020.01368
47. Hu W, Hu J, Gao J, Yang J, Qiu X, Kong L, et al. Outcomes of Orbital Malignancies Treated With Eye-Sparing Surgery and Adjuvant Particle Radiotherapy: A Retrospective Study. BMC Cancer (2019) 19(1):776. doi: 10.1186/s12885-019-5964-y
48. Hu W, Hu J, Huang Q, Gao J, Yang J, Qiu X, et al. Particle Beam Radiation Therapy for Sinonasal Malignancies: Single Institutional Experience at the Shanghai Proton and Heavy Ion Center. Cancer Med (2020) 9(21):7914–24. doi: 10.1002/cam4.3393
49. Chou YC, Fan KH, Lin CY, Hung TM, Huang BS, Chang KP, et al. Intensity Modulated Proton Beam Therapy Versus Volumetric Modulated Arc Therapy for Patients With Nasopharyngeal Cancer: A Propensity Score-Matched Study. Cancers (Basel) (2021) 13(14):3555. doi: 10.3390/cancers13143555
50. Kong L, Wu J, Gao J, Qiu X, Yang J, Hu J, et al. Particle Radiation Therapy in the Management of Malignant Glioma: Early Experience at the Shanghai Proton and Heavy Ion Center. Cancer (2020) 126(12):2802–10. doi: 10.1002/cncr.32828
51. Cai X, Du YY, Wang Z, Li P, Yu Z, Zhang Q, et al. The Role of Carbon Ion Radiotherapy for Unresectable Locally Recurrent Rectal Cancer: A Single Institutional Experience. Radiat Oncol (2020) 15(1):ARTN 209. doi: 10.1186/s13014-020-01653-w
52. Yu Z, Hong Z, Zhang Q, Lin LC, Shahnazi K, Wu X, et al. Proton and Carbon Ion Radiation Therapy for Locally Advanced Pancreatic Cancer: A Phase I Dose Escalation Study. Pancreatology (2020) 20(3):470–6. doi: 10.1016/j.pan.2020.01.010
53. Cheng JY, Liu CM, Wang YM, Hsu HC, Huang EY, Huang TT, et al. Proton Versus Photon Radiotherapy for Primary Hepatocellular Carcinoma: A Propensity-Matched Analysis. Radiat Oncol (2020) 15(1):159. doi: 10.1186/s13014-020-01605-4
54. Hsieh CE, Venkatesulu BP, Lee CH, Hung SP, Wong PF, Aithala SP, et al. Predictors of Radiation-Induced Liver Disease in Eastern and Western Patients With Hepatocellular Carcinoma Undergoing Proton Beam Therapy. Int J Radiat Oncol Biol Phys (2019) 105(1):73–86. doi: 10.1016/j.ijrobp.2019.02.032
55. Zhang H, Li S, Wang XH, Li Q, Wei SH, Gao LY, et al. Results of Carbon Ion Radiotherapy for Skin Carcinomas in 45 Patients. Br J Dermatol (2012) 166(5):1100–6. doi: 10.1111/j.1365-2133.2011.10764.x
56. Chen Y, Dong F, Wang X, Xue J, Zhang H, Gao L, et al. Postoperative Carbon Ion Radiotherapy for Keloids: A Preliminary Report of 16 Cases and Review of the Literature. Wounds (2014) 26(9):264–72.
57. Yang SY, Zhao JF, Zhuo WH, Shen H, Chen B. Measurement of Therapeutic C-12 Beam in a Water Phantom Using CR-39. J Radiol Prot (2021) 41(2):279–90. doi: 10.1088/1361-6498/abd88c
58. Wang X, Du N, Wang L. Analysis of Key Points of Radiation Sources in Proton and Carbon Ion Radiotherapy Facilities in Shanghai. Zhongguo Yi Liao Qi Xie Za Zhi (2020) 44(6):476–80. doi: 10.3969/j.issn.1671-7104.2020.06.002
59. Wu S, Jiao Y, Zhang Y, Ren X, Li P, Yu Q, et al. Imaging-Based Individualized Response Prediction Of Carbon Ion Radiotherapy For Prostate Cancer Patients. Cancer Manag Res (2019) 11:9121–31. doi: 10.2147/CMAR.S214020
60. Zhang L, Wang W, Hu J, Lu J, Kong L. RBE-Weighted Dose Conversions for Patients With Recurrent Nasopharyngeal Carcinoma Receiving Carbon-Ion Radiotherapy From the Local Effect Model to the Microdosimetric Kinetic Model. Radiat Oncol (2020) 15(1):277. doi: 10.1186/s13014-020-01723-z
61. D'Ascenzo N, Gao M, Antonecchia E, Gnudi P, Chen HH, Chen FH, et al. New Digital Plug and Imaging Sensor for a Proton Therapy Monitoring System Based on Positron Emission Tomography. Sens (Basel) (2018) 18(9):3006. doi: 10.3390/s18093006
62. Wang CC, McNamara AL, Shin J, Schuemann J, Grassberger C, Taghian AG, et al. End-Of-Range Radiobiological Effect on Rib Fractures in Patients Receiving Proton Therapy for Breast Cancer. Int J Radiat Oncol Biol Phys (2020) 107(3):449–54. doi: 10.1016/j.ijrobp.2020.03.012
63. Li QA, Sihver L. Therapeutic Techniques Applied in the Heavy-Ion Therapy at IMP. Nucl Instrum Methods Phys Res Sect B-Beam Interact Mater Atoms (2011) 269(7):664–70. doi: 10.1016/j.nimb.2011.01.125
64. Dai ZY, Li Q, Liu XG, Jin XD, Huang QY, Xiao GQ. Active Spot-Scanning Test With Heavy Ions at HIRFL-CSR. Chin Phys C (2012) 36(8):784–91. doi: 10.1088/1674-1137/36/8/018
65. Li Y, Gao YZ, Liu XG, Shi J, Xia JW, Yang JC, et al. The Influence of Beam Delivery Uncertainty on Dose Uniformity and Penumbra for Pencil Beam Scanning in Carbon-Ion Radiotherapy. PloS One (2021) 16(4):ARTN e0249452. doi: 10.1371/journal.pone.0249452
66. Wei K, Xu Z, Mao R, Zhao Z, Zhao T, She Q, et al. Performances of the Beam Monitoring System and Quality Assurance Equipment for the HIMM of Carbon-Ion Therapy. J Appl Clin Med Phys (2020) 21(8):289–98. doi: 10.1002/acm2.12916
67. Li JQ, Cao Y, Sun LT, Zhang XZ, Guo JW, Fang X, et al. Intense Carbon Beams Production With an All Permanent Magnet Electron Cyclotron Resonance Ion Source for Heavy Ion Medical Machine. Rev Sci Instrum (2020) 91(1):Artn 013307. doi: 10.1063/1.5128488
68. Cao Y, Li JQ, Sun LT, Zhang XZ, Feng YC, Wang H, et al. An All Permanent Magnet Electron Cyclotron Resonance Ion Source for Heavy Ion Therapy. Rev Sci Instrum (2014) 85(2):Artn 02a960. doi: 10.1063/1.4852335
69. Zhang H, Dai ZY, Liu XG, Chen WQ, Ma YY, He PB, et al. A Novel Pencil Beam Model for Carbon-Ion Dose Calculation Derived From Monte Carlo Simulations. Phys Med-Eur J Med Phys (2018) 55:15–24. doi: 10.1016/j.ejmp.2018.10.014
70. Dai T, Li Q, Liu X, Dai Z, He P, Ma Y, et al. Nanodosimetric Quantities and RBE of a Clinically Relevant Carbon-Ion Beam. Med Phys (2020) 47(2):772–80. doi: 10.1002/mp.13914
71. He P, Mori S. Perturbation Analysis of 4D Dose Distribution for Scanned Carbon-Ion Beam Radiotherapy. Phys Med (2020) 74:74–82. doi: 10.1016/j.ejmp.2020.05.003
72. Chen M, Yepes P, Hojo Y, Poenisch F, Li Y, Chen J, et al. Transitioning From Measurement-Based to Combined Patient-Specific Quality Assurance for Intensity-Modulated Proton Therapy. Br J Radiol (2020) 93(1107):20190669. doi: 10.1259/bjr.20190669
73. Shu H, Yin C, Zhang H, Liu M, Zhang M, Zhao L, et al. Scanned Proton Beam Performance and Calibration of the Shanghai Advanced Proton Therapy Facility. MethodsX (2019) 6:1933–43. doi: 10.1016/j.mex.2019.08.001
74. Shang H, Pu Y, Chen Z, Wang X, Yuan C, Jin X, et al. Impact of Multiple Beams on Plan Quality, Linear Energy Transfer Distribution, and Plan Robustness of Intensity Modulated Proton Therapy for Lung Cancer. ACS Sens (2021) 6(2):408–17. doi: 10.1021/acssensors.0c01879
75. Yang YH, Zhang MZ, Li DM. Simulation Study of Slow Extraction for the Shanghai Advanced Proton Therapy Facility. Nucl Sci Tech (2017) 28(9):120:ARTN 120. doi: 10.1007/s41365-017-0273-0
76. Zhao BQ, Zhao MH, Liu M, Yin CX, Shu H. The Front-End Electronics Design of Dose Monitors for Beam Delivery System of Shanghai Advanced Proton Therapy Facility. Nucl Sci Tech (2017) 28(6):1–7:ARTN 83. doi: 10.1007/s41365-017-0230-y
77. Wu J, Du HW, Xue S, Pan JZ, Du YF, Long YW. Gantry Optimization of the Shanghai Advanced Proton Therapy Facility. Nucl Sci Tech (2015) 26(4):5. doi: 10.13538/j.1001-8042/nst.26.040201
78. Ning R, Pei Y, Li P, Hu W, Deng Y, Hong Z, et al. Carbon Ion Radiotherapy Evokes a Metabolic Reprogramming and Individualized Response in Prostate Cancer. Front Public Health (2021) 9:777160. doi: 10.3389/fpubh.2021.777160
79. Huang Q, Sun Y, Wang W, Lin LC, Huang Y, Yang J, et al. Biological Guided Carbon-Ion Microporous Radiation to Tumor Hypoxia Area Triggers Robust Abscopal Effects as Open Field Radiation. Front Oncol (2020) 10:597702. doi: 10.3389/fonc.2020.597702
80. Huang YL, Dong YL, Zhao JF, Zhang LJ, Kong L, Lu JDJ. Comparison of the Effects of Photon, Proton and Carbon-Ion Radiation on the Ecto-Calreticulin Exposure in Various Tumor Cell Lines. Ann Trans Med (2019) 7(20):542. doi: 10.21037/atm.2019.09.128. ARTN 542.
81. Chi HC, Tsai CY, Tsai MM, Lin KH. Impact of DNA and RNA Methylation on Radiobiology and Cancer Progression. Int J Mol Sci (2018) 19(2):555. doi: 10.3390/ijms19020555
82. Wen-jian L, Guang-ming Z, Zeng-quan W, Ju-fang W, Qiang L, Bing-rong D, et al. A Possible Molecular Mechanism of Radiosensitivity in Mammalian Cells Exposed to Heavy Ions. Nucl Phys Rev (2003) 20(1):42. doi: 10.11804/NuclPhysRev.20.01.042
83. Li Q. Biomedical Research With Heavy Ions at the IMP Accelerators. Adv Space Res (2007) 40(4):455–60. doi: 10.1016/j.asr.2007.03.096
84. Zhou G, Li W, Wang J, He J, Li Q, Dang B, et al. DNA Double-Strand Break Induced by Ionizing Radiation. Shengwu Wuli Xuebao (2000) 16(1):139–44. doi: 10.3321/j.issn:1000-6737.2000.01.020
85. Jin X-D, Li Q, Li W-J, Wang J-F, Guo C-L, Hao J-F. The Hyper-Radiosensitivity Effect of Human Hepatoma SMMC-7721 Cells Exposed to Low Dose γ-Rays and 12C Ions. Nucl Instrum Methods Phys Res Sect B: Beam Interact Mater Atoms (2006) 245(1):310–3. doi: 10.1016/j.nimb.2005.11.120
86. Gong L, Jin X, Li Q, Liu J, An L. Heavy Ion Beams Induce Survivin Expression in Human Hepatoma SMMC-7721 Cells More Effectively Than X-Rays. Acta Biochim Et Biophys Sin (2007) 39(8):575–82. doi: 10.1111/j.1745-7270.2007.00314.x
87. Zhang Q, Kong Y, Yang Z, Liu Y, Liu R, Geng Y, et al. Preliminary Study on Radiosensitivity to Carbon Ions in Human Breast Cancer. J Radiat Res (2020) 61(3):399–409. doi: 10.1093/jrr/rraa017
88. Ran JT, Wang JT, Dai ZY, Miao YD, Gan J, Zhao CP, et al. Irradiation-Induced Changes in the Immunogenicity of Lung Cancer Cell Lines: Based on Comparison of X-Rays and Carbon Ions. Front Public Health (2021) 9:666282. doi: 10.3389/fpubh.2021.666282. ARTN 666282.
89. Jin X, Zheng X, Li F, Liu B, Li H, Hirayama R, et al. Fragmentation Level Determines Mitochondrial Damage Response and Subsequently the Fate of Cancer Cells Exposed to Carbon Ions. Radiother Oncol (2018) 129(1):75–83. doi: 10.1016/j.radonc.2017.11.019
90. Liu XX, Li P, Hirayama R, Niu YZ, Liu XG, Chen WQ, et al. Genistein Sensitizes Glioblastoma Cells to Carbon Ions via Inhibiting DNA-PKcs Phosphorylation and Subsequently Repressing NHEJ and Delaying HR Repair Pathways. Radiother Oncol (2018) 129(1):84–94. doi: 10.1016/j.radonc.2018.04.005
91. Mao A, Guo H, Liu Y, Wang F, Tang J, Liao S, et al. Exogenous Melatonin Modulates Carbon Ion Radiation-Induced Immune Dysfunction in Mice. Toxicology (2019) 417:35–41. doi: 10.1016/j.tox.2019.01.019
92. Zhou H, Yang P, Li H, Zhang L, Li J, Zhang T, et al. Carbon Ion Radiotherapy Boosts Anti-Tumour Immune Responses by Inhibiting Myeloid-Derived Suppressor Cells in Melanoma-Bearing Mice. Cell Death Discovery (2021) 7(1):332. doi: 10.1038/s41420-021-00731-6
93. Chen J, Dai J, Nobah A, Bai S, Bi N, Lai Y, et al. A Special Report on 2019 International Planning Competition and a Comprehensive Analysis of Its Results. Front Oncol (2020) 10:571644:571644. doi: 10.3389/fonc.2020.571644
94. Yan H, Hu Z, Huang P, Men K, Zhang Y, Wang LH, et al. The Status of Medical Physics in Radiotherapy in China. Phys Med (2021) 85:147–57. doi: 10.1016/j.ejmp.2021.05.007
95. Dang JW, Wang YP, Li S, Zhu ZP. Heavy-Ion Radiotherapy Treatment Planning System and Medical Image Processing Algorithm Used in it. In: 2008 Ieee International Conference on Automation Science and Engineering, Vols 1 and 2. Arlington, VA, USA: IEEE (2008). p. 726–+. doi: 10.1109/Coase.2008.4626514
96. Mizoe JE, Tsujii H, Kamada T, Matsuoka Y, Tsuji H, Osaka Y, et al. Dose Escalation Study of Carbon Ion Radiotherapy for Locally Advanced Head-And-Neck Cancer. Int J Radiat Oncol Biol Phys (2004) 60(2):358–64. doi: 10.1016/j.ijrobp.2004.02.067
97. Lu JD, Ye M, Guo Q, Fu JF, Moyers XM, Zhang ST, et al. The Preliminary Report of a Registration Clinical Trial of Proton and Heavy Ion Irradiation. Zhonghua Zhong Liu Za Zhi (2018) 40(1):52–6. doi: 10.3760/cma.j.issn.0253-3766.2018.01.009
98. Lu JJ, Kong L, Gao J, Jiang GL. An Analysis of the on-Going Clinical Trials on Proton and Heavy-Ion Radiation Therapy and Their Impact on the Clinical Application and Future Development. Chin J Radiol Med Prot (2016) 36(8):611–5. doi: 10.3760/cma.j.issn.0254-5098.2016.08.011
99. IMP, B. O. D. a. Planning. Significant Progress has Been Made in the Treatment of Cancer by Heavy Ions in China. Bull Chin Acad Sci (2012) 27(4):502–3. doi: 10.3969/j.issn.1000-3045.2012.04.014
100. Yancheng Y, Ying Q, Fangfang Z, Wanbin M, Xiaoyun M, Jian W. Progress in Clinical Trials of Wuwei Heavy Ion Treatment Demonstration Device. Sci Technol Dev (2020) 16(1):34–8. doi: 10.11842/chips.20190813001
101. Siegel RL, Miller KD, Fuchs HE, Jemal A. Cancer Statistics, 2021. CA Cancer J Clin (2021) 71(1):7–33. doi: 10.3322/caac.21654
102. Habl G, Uhl M, Katayama S, Kessel KA, Hatiboglu G, Hadaschik B, et al. Acute Toxicity and Quality of Life in Patients With Prostate Cancer Treated With Protons or Carbon Ions in a Prospective Randomized Phase II Study–The IPI Trial. Int J Radiat Oncol Biol Phys (2016) 95(1):435–43. doi: 10.1016/j.ijrobp.2016.02.025
103. Qi WX, Zhang Q, Li P, Zhang XM, Zhang GY, Wu B, et al. The Predictive Role of ADC Values in Prostate Cancer Patients Treated With Carbon-Ion Radiotherapy: Initial Clinical Experience at Shanghai Proton and Heavy Ion Center (SPHIC). J Cancer Res Clin Oncol (2016) 142(6):1361–7. doi: 10.1007/s00432-016-2142-3
104. Li Y, Li P, Hsi W, Hong Z, Fu S, Zhang Q. Normal Tissue Complication Probability (NTCP) Models of Acute Urinary Toxicity (AUT) Following Carbon Ion Radiotherapy (CIRT) for Prostate Cancer. Radiother Oncol (2021) 156:69–79. doi: 10.1016/j.radonc.2020.12.009
105. Nomiya T, Tsuji H, Maruyama K, Toyama S, Suzuki H, Akakura K, et al. Phase I/II Trial of Definitive Carbon Ion Radiotherapy for Prostate Cancer: Evaluation of Shortening of Treatment Period to 3 Weeks. Br J Cancer (2014) 110(10):2389–95. doi: 10.1038/bjc.2014.191
106. Holliday EB, Esmaeli B, Pinckard J, Garden AS, Rosenthal DI, Morrison WH, et al. A Multidisciplinary Orbit-Sparing Treatment Approach That Includes Proton Therapy for Epithelial Tumors of the Orbit and Ocular Adnexa. Int J Radiat Oncol Biol Phys (2016) 95(1):344–52. doi: 10.1016/j.ijrobp.2015.08.008
107. Yang J, Gao J, Wu X, Hu J, Hu W, Kong L, et al. Salvage Carbon Ion Radiation Therapy for Locally Recurrent or Radiation-Induced Second Primary Sarcoma of the Head and Neck. J Cancer (2018) 9(12):2215–23. doi: 10.7150/jca.24313
108. Combs SE, Kalbe A, Nikoghosyan A, Ackermann B, Jakel O, Haberer T, et al. Carbon Ion Radiotherapy Performed as Re-Irradiation Using Active Beam Delivery in Patients With Tumors of the Brain, Skull Base and Sacral Region. Radiother Oncol (2011) 98(1):63–7. doi: 10.1016/j.radonc.2010.10.010
109. Jensen AD, Nikoghosyan AV, Ecker S, Ellerbrock M, Debus J, Munter MW. Carbon Ion Therapy for Advanced Sinonasal Malignancies: Feasibility and Acute Toxicity. Radiat Oncol (2011) 6:30. doi: 10.1186/1748-717X-6-30
110. Hu W, Hu J, Gao J, Yang J, Qiu X, Kong L, et al. Intensity-Modulated Particle Beam Radiation Therapy in the Management of Olfactory Neuroblastoma. Ann Transl Med (2020) 8(15):926. doi: 10.21037/atm-19-4790
111. Gao J, Kong L, Guan X, Hu J, Zhang X, Zhu Y, et al. Early Response and Acute Adverse Effect After Particle Radiation Therapy for Adenoid Cystic Carcinoma of the Head and Neck. Chin J Radiol Med Prot (2016) 36(8):607–10. doi: 10.3760/cma.j.issn.0254-5098.2016.08.010
112. Akbaba S, Held T, Lang K, Forster T, Federspil P, Herfarth K, et al. Bimodal Radiotherapy With Active Raster-Scanning Carbon Ion Radiotherapy and Intensity-Modulated Radiotherapy in High-Risk Nasopharyngeal Carcinoma Results in Excellent Local Control. Cancers (Basel) (2019) 11(3):379. doi: 10.3390/cancers11030379
113. Hu J, Bao C, Gao J, Guan X, Hu W, Yang J, et al. Salvage Treatment Using Carbon Ion Radiation in Patients With Locoregionally Recurrent Nasopharyngeal Carcinoma: Initial Results. Cancer (2018) 124(11):2427–37. doi: 10.1002/cncr.31318
114. Buszek SM, Al Feghali KA, Elhalawani H, Chevli N, Allen PK, Chung C. Optimal Timing of Radiotherapy Following Gross Total or Subtotal Resection of Glioblastoma: A Real-World Assessment Using the National Cancer Database. Sci Rep (2020) 10(1):4926. doi: 10.1038/s41598-020-61701-z
115. Ostrom QT, Gittleman H, Truitt G, Boscia A, Kruchko C, Barnholtz-Sloan JS. CBTRUS Statistical Report: Primary Brain and Other Central Nervous System Tumors Diagnosed in the United States in 2011-2015. Neuro Oncol (2018) 20(suppl_4):iv1–86. doi: 10.1093/neuonc/noy131
116. Dutz A, Agolli L, Butof R, Valentini C, Baumann M, Luhr A, et al. Neurocognitive Function and Quality of Life After Proton Beam Therapy for Brain Tumour Patients. Radiother Oncol (2020) 143:108–16. doi: 10.1016/j.radonc.2019.12.024
117. Jing G, Jiyi H, Jing Y, Xianxin Q, Weixu H, Jiade L, et al. Primary Results of Proton Radiotherapy for 26 Meningioma Patients. Chin J Radiol Med Prot (2020) 40(4):302–7. doi: 10.3760/cma.j.issn.0254-5098.2020.04.010
118. Adeberg S, Harrabi SB, Verma V, Bernhardt D, Grau N, Debus J, et al. Treatment of Meningioma and Glioma With Protons and Carbon Ions. Radiat Oncol (2017) 12(1):193. doi: 10.1186/s13014-017-0924-7
119. Wang K, Tepper JE. Radiation Therapy-Associated Toxicity: Etiology, Management, and Prevention. CA Cancer J Clin (2021) 71(5):437–54. doi: 10.3322/caac.21689
120. Moore S, Leung B, Wu J, Ho C. Population-Based Analysis of Curative Therapies in Stage II non-Small Cell Lung Cancer: The Role of Radiotherapy in Medically Inoperable Patients. Radiat Oncol (2020) 15(1):23. doi: 10.1186/s13014-020-1466-y
121. Ma N, Mao J, Chen J, Jiang G, Cai X, Lu J. Preliminary Clinical Results After Pencil Beam Scanning Particle Radiotherapy for Stage I Non-Small Cell Lung Cancer. Chin J Radiol Med Prot (2017) 37(5):321–6. doi: 10.3760/cma.j.issn.0254-5098.2017.05.001
122. Levy A, Omeiri A, Fadel E, Le Pechoux C. Radiotherapy for Tracheal-Bronchial Cystic Adenoid Carcinomas. Clin Oncol (R Coll Radiol) (2018) 30(1):39–46. doi: 10.1016/j.clon.2017.10.012
123. Hogerle BA, Lasitschka F, Muley T, Bougatf N, Herfarth K, Adeberg S, et al. Primary Adenoid Cystic Carcinoma of the Trachea: Clinical Outcome of 38 Patients After Interdisciplinary Treatment in a Single Institution. Radiat Oncol (2019) 14(1):117. doi: 10.1186/s13014-019-1323-z
124. Chen M, Therneau T, Orsini LS, Qiao YL. Design and Rationale of the HCC BRIDGE Study in China: A Longitudinal, Multicenter Cohort Trial in Hepatocellular Carcinoma. BMC Gastroenterol (2011) 11(1):53. doi: 10.1186/1471-230X-11-53
125. Younossi ZM, Stepanova M, Younossi I, Papatheodoridis G, Janssen HLA, Agarwal K, et al. Patient-Reported Outcomes in Patients Chronic Viral Hepatitis Without Cirrhosis: The Impact of Hepatitis B and C Viral Replication. Liver Int (2019) 39(10):1837–44. doi: 10.1111/liv.14171
126. Law AL, Ng WT, Lee MC, Chan AT, Fung KH, Li F, et al. Treatment of Primary Liver Cancer Using Highly-Conformal Radiotherapy With kV-Image Guidance and Respiratory Control. Radiother Oncol (2012) 102(1):56–61. doi: 10.1016/j.radonc.2011.05.022
127. He PB, Li Q. Motion Management With Variable Cycle-Based Respiratory Guidance Method for Carbon-Ion Pencil Beam Scanning Treatment. Phys Med-Eur J Med Phys (2021) 87:99–105. doi: 10.1016/j.ejmp.2021.06.005
128. He P, Li Q, Liu X, Dai Z, Zhao T, Fu T, et al. Respiratory Motion Management Using Audio-Visual Biofeedback for Respiratory-Gated Radiotherapy of Synchrotron-Based Pulsed Heavy-Ion Beam Delivery. Med Phys (2014) 41(11):111708. doi: 10.1118/1.4897391
129. Hung SP, Huang BS, Hsieh CE, Lee CH, Tsang NM, Chang JT, et al. Clinical Outcomes of Patients With Unresectable Cholangiocarcinoma Treated With Proton Beam Therapy. Am J Clin Oncol (2020) 43(3):180–6. doi: 10.1097/COC.0000000000000646
130. Trace AP, Enos CW, Mantel A, Harvey VM. Keloids and Hypertrophic Scars: A Spectrum of Clinical Challenges. Am J Clin Dermatol (2016) 17(3):201–23. doi: 10.1007/s40257-016-0175-7
131. Gold MH, Nestor MS, Berman B, Goldberg D. Assessing Keloid Recurrence Following Surgical Excision and Radiation. Burns Trauma (2020) 8:tkaa031. doi: 10.1093/burnst/tkaa031
132. Shinoto M, Yamada S, Okamoto M, Shioyama Y, Ohno T, Nakano T, et al. Carbon-Ion Radiotherapy for Locally Recurrent Rectal Cancer: Japan Carbon-Ion Radiation Oncology Study Group (J-CROS) Study 1404 Rectum. Radiother Oncol (2019) 132:236–40. doi: 10.1016/j.radonc.2018.10.007
133. Shinoto M, Yamada S, Terashima K, Yasuda S, Shioyama Y, Honda H, et al. Carbon Ion Radiation Therapy With Concurrent Gemcitabine for Patients With Locally Advanced Pancreatic Cancer. Int J Radiat Oncol Biol Phys (2016) 95(1):498–504. doi: 10.1016/j.ijrobp.2015.12.362
134. Dai Z, Ma Y, Li Q. China's Particle Therapy Equipment Market: Opportunities Outweigh Challenges. Front Public Health (2020) 8:602776:602776. doi: 10.3389/fpubh.2020.602776
135. Chen W, Xia C, Zheng R, Zhou M, Lin C, Zeng H, et al. Disparities by Province, Age, and Sex in Site-Specific Cancer Burden Attributable to 23 Potentially Modifiable Risk Factors in China: A Comparative Risk Assessment. Lancet Glob Health (2019) 7(2):e257–69. doi: 10.1016/S2214-109X(18)30488-1
136. Oike T, Sato H, Noda SE, Nakano T. Translational Research to Improve the Efficacy of Carbon Ion Radiotherapy: Experience of Gunma University. Front Oncol (2016) 6:139. doi: 10.3389/fonc.2016.00139
137. Vitolo V, Cobianchi L, Brugnatelli S, Barcellini A, Peloso A, Facoetti A, et al. Preoperative Chemotherapy and Carbon Ions Therapy for Treatment of Resectable and Borderline Resectable Pancreatic Adenocarcinoma: A Prospective, Phase II, Multicentre, Single-Arm Study. BMC Cancer (2019) 19(1):922. doi: 10.1186/s12885-019-6108-0
138. Fernando IN, Bowden SJ, Herring K, Brookes CL, Ahmed I, Marshall A, et al. Synchronous Versus Sequential Chemo-Radiotherapy in Patients With Early Stage Breast Cancer (SECRAB): A Randomised, Phase III, Trial. Radiother Oncol (2020) 142:52–61. doi: 10.1016/j.radonc.2019.10.014
139. Chen SY, Tang Y, Wang SL, Song YW, Fang H, Wang JY, et al. Timing of Chemotherapy and Radiotherapy Following Breast-Conserving Surgery for Early-Stage Breast Cancer: A Retrospective Analysis. Front Oncol (2020) 10:571390(2040):571390. doi: 10.3389/fonc.2020.571390
140. Ko EC, Formenti SC. Radiotherapy and Checkpoint Inhibitors: A Winning New Combination? Ther Adv Med Oncol (2018) 10:1758835918768240. doi: 10.1177/1758835918768240
141. National Library of Medicine. (2022). Available at: http://ClinicalTrials.gov (Accessed January 10, 2022).
142. Lhuillier C, Vanpouille-Box C, Galluzzi L, Formenti SC, Demaria S. Emerging Biomarkers for the Combination of Radiotherapy and Immune Checkpoint Blockers. In: Semin Cancer Biol. (2018) 52(Pt2):125–34. doi: 10.1016/j.semcancer.2017.12.007
143. Wilkins A, McDonald F, Harrington K, Melcher A. Radiotherapy Enhances Responses of Lung Cancer to CTLA-4 Blockade. J Immunother Cancer (2019) 7(1):64. doi: 10.1186/s40425-019-0542-z
144. Weichselbaum RR, Liang H, Deng L, Fu YX. Radiotherapy and Immunotherapy: A Beneficial Liaison? Nat Rev Clin Oncol (2017) 14(6):365–79. doi: 10.1038/nrclinonc.2016.211
145. Durante M, Formenti S. Harnessing Radiation to Improve Immunotherapy: Better With Particles? Br J Radiol (2020) 93(1107):20190224. doi: 10.1259/bjr.20190224
146. Helm A, Ebner DK, Tinganelli W, Simoniello P, Bisio A, Marchesano V, et al. Combining Heavy-Ion Therapy With Immunotherapy: An Update on Recent Developments. Int J Part Ther (2018) 5(1):84–93. doi: 10.14338/IJPT-18-00024.1
147. Li G, Qiu B, Huang YX, Doyen J, Bondiau PY, Benezery K, et al. Cost-Effectiveness Analysis of Proton Beam Therapy for Treatment Decision Making in Paranasal Sinus and Nasal Cavity Cancers in China. BMC Cancer (2020) 20(1):599. doi: 10.1186/s12885-020-07083-x
148. Noda K. Beam Delivery Method for Carbon-Ion Radiotherapy With the Heavy-Ion Medical Accelerator in Chiba. Int J Part Ther (2016) 2(4):481–9. doi: 10.14338/IJPT-15-00041.1
149. Habermehl D, Henkner K, Ecker S, Jakel O, Debus J, Combs SE. Evaluation of Different Fiducial Markers for Image-Guided Radiotherapy and Particle Therapy. J Radiat Res (2013) 54:i61–8. doi: 10.1093/jrr/rrt071
150. Lin B, Gao F, Yang Y, Wu D, Zhang Y, Feng G, et al. FLASH Radiotherapy: History and Future. Front Oncol (2021) 11:644400. doi: 10.3389/fonc.2021.644400
151. Bourhis J, Sozzi WJ, Jorge PG, Gaide O, Bailat C, Duclos F, et al. Treatment of a First Patient With FLASH-Radiotherapy. Radiother Oncol (2019) 139:18–22. doi: 10.1016/j.radonc.2019.06.019
152. Baumann M, Krause M, Overgaard J, Debus J, Bentzen SM, Daartz J, et al. Radiation Oncology in the Era of Precision Medicine. Nat Rev Cancer (2016) 16(4):234–49. doi: 10.1038/nrc.2016.18
153. Amornwichet N, Oike T, Shibata A, Nirodi CS, Ogiwara H, Makino H, et al. The EGFR Mutation Status Affects the Relative Biological Effectiveness of Carbon-Ion Beams in non-Small Cell Lung Carcinoma Cells. Sci Rep (2015) 5(1):11305. doi: 10.1038/srep11305
154. Darwis NDM, Nachankar A, Sasaki Y, Matsui T, Noda SE, Murata K, et al. FGFR Signaling as a Candidate Therapeutic Target for Cancers Resistant to Carbon Ion Radiotherapy. Int J Mol Sci (2019) 20(18):4563. doi: 10.3390/ijms20184563
155. Mohamad O, Makishima H, Kamada T. Evolution of Carbon Ion Radiotherapy at the National Institute of Radiological Sciences in Japan. Cancers (Basel) (2018) 10(3):66. doi: 10.3390/cancers10030066
156. Goetz G, Mitic M, Mittermayr T, Wild C. Health Technology Assessment of Carbon-Ion Beam Radiotherapy: A Systematic Review of Clinical Effectiveness and Safety for 54 Oncological Indications in 12 Tumour Regions. Anticancer Res (2019) 39(4):1635–50. doi: 10.21873/anticanres.13269
157. Nomiya T, Tsuji H, Kawamura H, Ohno T, Toyama S, Shioyama Y, et al. A Multi-Institutional Analysis of Prospective Studies of Carbon Ion Radiotherapy for Prostate Cancer: A Report From the Japan Carbon Ion Radiation Oncology Study Group (J-CROS). Radiother Oncol (2016) 121(2):288–93. doi: 10.1016/j.radonc.2016.10.009
158. Shibuya K, Ohno T, Terashima K, Toyama S, Yasuda S, Tsuji H, et al. Short-Course Carbon-Ion Radiotherapy for Hepatocellular Carcinoma: A Multi-Institutional Retrospective Study. Liver Int (2018) 38(12):2239–47. doi: 10.1111/liv.13969
159. Mizumoto M, Murayama S, Akimoto T, Demizu Y, Fukushima T, Ishida Y, et al. Preliminary Results of Proton Radiotherapy for Pediatric Rhabdomyosarcoma: A Multi-Institutional Study in Japan. Cancer Med (2018) 7(5):1870–4. doi: 10.1002/cam4.1464
160. Kawashiro S, Yamada S, Okamoto M, Ohno T, Nakano T, Shinoto M, et al. Multi-Institutional Study of Carbon-Ion Radiotherapy for Locally Advanced Pancreatic Cancer: Japan Carbon-Ion Radiation Oncology Study Group (J-CROS) Study 1403 Pancreas. Int J Radiat Oncol Biol Phys (2018) 101(5):1212–21. doi: 10.1016/j.ijrobp.2018.04.057
161. Ariga T, Toita T, Kato S, Kazumoto T, Kubozono M, Tokumaru S, et al. Treatment Outcomes of Patients With FIGO Stage I/II Uterine Cervical Cancer Treated With Definitive Radiotherapy: A Multi-Institutional Retrospective Research Study. J Radiat Res (2015) 56(5):841–8. doi: 10.1093/jrr/rrv036
162. Shirai K, Koto M, Demizu Y, Suefuji H, Ohno T, Tsuji H, et al. Multi-Institutional Retrospective Study of Mucoepidermoid Carcinoma Treated With Carbon-Ion Radiotherapy. Cancer Sci (2017) 108(7):1447–51. doi: 10.1111/cas.13270
163. Ikushima H, Wakatsuki M, Ariga T, Kaneyasu Y, Tokumaru S, Isohashi F, et al. Radiotherapy for Vaginal Cancer: A Multi-Institutional Survey Study of the Japanese Radiation Oncology Study Group. Int J Clin Oncol (2018) 23(2):314–20. doi: 10.1007/s10147-017-1205-z
164. Roelofs E, Engelsman M, Rasch C, Persoon L, Qamhiyeh S, de Ruysscher D, et al. Results of a Multicentric in Silico Clinical Trial (ROCOCO): Comparing Radiotherapy With Photons and Protons for non-Small Cell Lung Cancer. J Thorac Oncol (2012) 7(1):165–76. doi: 10.1097/JTO.0b013e31823529fc
165. Eekers DBP, Roelofs E, Jelen U, Kirk M, Granzier M, Ammazzalorso F, et al. Benefit of Particle Therapy in Re-Irradiation of Head and Neck Patients. Results of a Multicentric in Silico ROCOCO Trial. Radiother Oncol (2016) 121(3):387–94. doi: 10.1016/j.radonc.2016.08.020
Keywords: radiotherapy, proton, carbon ion, clinical trial, radiation oncology, proton, tumor
Citation: Li Y, Li X, Yang J, Wang S, Tang M, Xia J and Gao Y (2022) Flourish of Proton and Carbon Ion Radiotherapy in China. Front. Oncol. 12:819905. doi: 10.3389/fonc.2022.819905
Received: 22 November 2021; Accepted: 24 January 2022;
Published: 14 February 2022.
Edited by:
Marco Durante, Helmholtz Association of German Research Centres (HZ), GermanyReviewed by:
Arnold Pompos, University of Texas Southwestern Medical Center, United StatesMansoor Ahmed, National Cancer Institute (NCI), United States
Lembit Sihver, Vienna University of Technology, Austria
Copyright © 2022 Li, Li, Yang, Wang, Tang, Xia and Gao. This is an open-access article distributed under the terms of the Creative Commons Attribution License (CC BY). The use, distribution or reproduction in other forums is permitted, provided the original author(s) and the copyright owner(s) are credited and that the original publication in this journal is cited, in accordance with accepted academic practice. No use, distribution or reproduction is permitted which does not comply with these terms.
*Correspondence: Yue Li, liyue@impcas.ac.cn